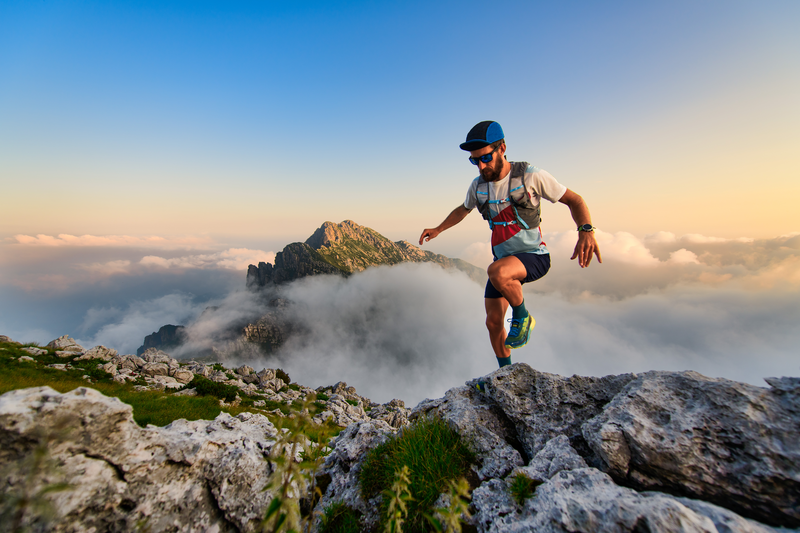
95% of researchers rate our articles as excellent or good
Learn more about the work of our research integrity team to safeguard the quality of each article we publish.
Find out more
ORIGINAL RESEARCH article
Front. Agron. , 17 July 2020
Sec. Disease Management
Volume 2 - 2020 | https://doi.org/10.3389/fagro.2020.00006
This article is part of the Research Topic Fungal Wheat Diseases: Etiology, Breeding, and Integrated Management View all 27 articles
Management of wheat stem rust in Western Siberia has gained importance since the first outbreaks in 2007–2010 and 2016. The race composition and virulence patterns were investigated for the enlarged Puccinia graminis f. sp. tritici (Pgt) samples collected in three neighboring regions Omsk, Novosibirsk, and Altai during 2017–2018. Most of Pgt isolates were identified as virulent to wheat lines with genes Sr5, Sr9a, Sr10, Sr38, SrMcN, and avirulent to Sr24, Sr31. Differentiation ability of genes Sr6, Sr7b, Sr8a, Sr9b, Sr9d, Sr9g, Sr9e, Sr11, Sr17, Sr21, Sr30, Sr36, and SrTmp to distinguish between the regional populations was established. A total of 33 virulence phenotypes or races were detected among 115 Pgt isolates tested. Based on virulence phenotypes, two different Pgt subpopulations were identified in the Altai and Omsk regions likely originating from asexual and sexual cycles, respectively. The Novosibirsk pathogen population seems to be a mixture of isolates originated from both neighboring regions with virulence phenotypes that developed in the west, Omsk (TKRPF, QHHSF, and MLLTF), and in the south, Altai (NFMSF, LKCSF, LKMSF, and PKCSF), of Western Siberia.
Western Siberia is an extremely important wheat production area in Russia with ~7 million ha of spring wheat, along with increasing areas of winter wheat and some durum wheat. The genetic basis of resistance to stem rust in Siberian germplasm is narrow and limited to several Sr genes, including Sr25, Sr31, Sr36, Sr6Ai, Sr6Ai#2, and some unknown major genes (Shamanin et al., 2016). The devastating disease caused by Puccinia graminis f. sp. tritici (Pgt) was not considered economically important in Western Siberia until the first reports of stem rust outbreak in 2007–2010 (Shamanin et al., 2015; Sochalova and Lihenko, 2015). In the Omsk region, southwest of Western Siberia, wheat production was affected by heavy epidemic in 2015; yield loss was estimated at more than 2 million tons. Understanding population structure, virulence diversity, and distribution of Pgt in the Omsk and neighboring regions has been essential for effective disease management based on resistance genes. Integrated analysis of pathogen virulence in Western Siberia has just been launched. In 2017, field trip for assessing the stem rust situation was conducted, and the samples of stem rust were collected for determining virulence phenotypes at the Global Rust Reference Center (GRRC) (GRRC Report: Samples of Stem Rust Infected Wheat From Russia, 2017). Significant race diversity coupled with high virulence of local pathogen was revealed in the samples from Omsk and Altai (Shamanin et al., 2020). Further study of virulence dynamics in the vast territory of Western Siberia was highlighted as the main result of international screening (Hodson et al., 2017). Moreover, the global tracking of aggressive races Ug99 and its variants spreading across the continents makes the race surveys highly actual at every location of wheat crops (Pretorius et al., 2000; Olivera et al., 2015; Bhattacharya, 2017). The objective of our study was to investigate the race composition and virulence patterns of the enlarged Pgt samples collected in three neighboring regions Omsk, Novosibirsk, and Altai during 2017–2018.
In 2017–2018, 77 infected stems of susceptible cultivars of bread wheat (cvs. Chernyava 13, Khakasskaya, Duet) with urediniospores of Pgt were sampled in the neighboring regions Omsk, Novosibirsk, and Altai. Additionally, 30 samples of infected leaves with aeciospores of Pgt, were collected from barberry, the alternative host of stem rust, in Omsk 2018 (Figure 1). Bulk samples were stored in the automatic desiccator at dry cold conditions. After recovering on susceptible wheat cultivars Khakasskaya and Morocco, a total of 115 single pustule Pgt isolates were obtained from 107 bulk field samples. In 2017, 51 single pustule isolates were obtained from 39 samples and tested at the GRRC in Denmark. The rest 64 single pustule isolates were obtained from 68 samples and analyzed at the Institute of Cytology and Genetics.
Figure 1. Regions for the sampling of Puccinia graminis f. sp. tritici in Western Siberia, 2017–2018.
Single pustule isolates were used for determining virulence phenotypes based on a set of 20 North American wheat differential lines, containing Sr genes: Sr5 (ISr5-Ra), Sr21 (CnS_Triticum monoc. Deriv.), Sr9e (Vernstein), Sr7b (ISr7b-Ra), Sr11 (ISr11-Ra), Sr6 (ISr6a-Ra), Sr8a (ISr8a-Ra), Sr9g (CnSr9g), Sr36 (W2691SrTt-1), Sr9b (W2691Sr9b), Sr30 (BtSr30Wst), Sr17+13 (Combination VII), Sr9a (ISr9a-Ra), Sr9d (ISr9d-Ra), Sr10 (W2691Sr10), SrTmp (CnsSrTmp), Sr24 (LcSr24Ag), Sr31 (Benno Sr31/6*LMPG), Sr38 (VPM-1), and SrMcN (McNair 701). According to the international protocols of virulence analysis, seedling plants were used (Roelfs et al., 1992). Each of 115 single pustule isolates was tested at least twice on the set of differentials with the susceptible checks (cultivars Khakasskaya and Morocco). The spores were dissolved in Novec 7100 oil and applied with an airbrush on seedlings planted 5 to 10 per pot. Inoculated plants were placed in a dark dew chamber at 18°C ± 2°C for 24 h and then transferred to a growing room at 20°C ± 2°C with a photoperiod of 16 h. The entire set of tested plants was covered with a cellophane box to avoid contamination (Figure 2). Infection types as described by Stakman et al. (1962) were assessed 14 to 16 days after inoculation; infection types 0 to 2 and 3 to 4 were interpreted as low (avirulent) and high (virulent), respectively. Races were designated following the North American (hexadecimal) Pgt coding system (Roelfs and Martens, 1988; Jin et al., 2008). A total of 33 virulence phenotypes or races were detected among 115 Pgt isolates tested.
Figure 2. Procedures for laboratory testing of spore material, facility of the Institute of Cytology and Genetics (IC&G). (A) Spore recovering; (B) spore multiplication on susceptible cultivar; (C,D) spore collection with the vacuum pump; (E) spore inoculation with the airbrush; (F) infected wheat seedlings of the differential set covered with cellophane isolating box.
Virulence frequencies were calculated for all regional populations for each separate year and for the pool of 2017 and 2018. The relationships of the P. graminis f. sp. tritici populations were analyzed using the assignment-based approaches. The corresponding Kosman dispersion (KW) within and distance (KB) between populations with regard to the simple mismatch dissimilarity between virulence patterns were calculated (Kosman, 1996, 2014; Kosman and Leonard, 2007). Differentiation among populations was estimated with the permutation test (1,000 random partitions) for differentiation statistic difKW (Equation 1 in Gultyaeva et al., 2020) similar to those of Jost (2008; Equations 9, 11), and in accordance with explanations in Kosman (2014, p. 565). The corresponding calculations were performed with Virulence Analysis Tool (VAT) software (Kosman et al., 2008; Schachtel et al., 2012) and its extension. The structural diversity of a population (effective number of equally distant isolates) was measured by the Hill number of order 1, 1D(T), based on the matrix of simple mismatch dissimilarities between isolates (Equation 4 in Scheiner et al., 2017). Then the structural variation within a given population (effective number of different isolates) is a function of both the dispersion KW and structural diversity 1D(T), where k is the number of isolates in the population:
This estimate is similar to qD(TM) from Equation 5 for q = 1 and dispersion M in Scheiner et al. (2017). The structural variation 1D(T, KW) has a range [1, k], whereas its normalized version (corrected Equation 5 in Kosman et al., 2019) ranges between 0 and 1 and thus is more suitable for comparison of populations with different numbers of isolates:
This indicator expresses an extent of variability within the pathogen populations.
NMDS plots with regard to the Kosman distance (KB) between the regional collections of P. graminis f. sp. tritici in 2017 and 2018 were derived using the Mdscale program of the NTSYSpc package, version 2.2 (Exeter Software, Setauket, NY, USA). A UPGMA dendrogram based on the simple mismatch dissimilarity between virulence phenotypes detected in each separate population was derived using the SAHN program of the NTSYSPC package, v. 2.1 (Exeter Software).
Wheat crop occupies the territory of more than 1.4 million ha in the Omsk region, more than 1 million ha in Novosibirsk region, and ~2.1 million ha in the Altai. Stem rust was observed as a late infection. When sampled, wheat crop was close to maturity or mature. Both years, 2017 and 2018, were non-epidemic for stem rust in Western Siberia. Among the inspected field plots and commercial fields, the disease incidence ranged between 0 and 45% with severity up to 60S. The Novosibirsk region is the central part of Western Siberia, bordered by the Omsk region in the west and the Altai region in the south.
Out of 115 Pgt isolates tested, 46 isolates originated from Omsk samples, 35 from Novosibirsk samples, and 34 from Altai. The virulence phenotypes of Pgt isolates are listed in Table 1. During the survey of 2017–2018, no avirulent isolates to Sr5, Sr9a, Sr10, Sr38, SrMcN resistant genes were revealed in West Siberian population of stem rust. All tested Pgt isolates were avirulent to Sr31, which was consistent with the field screening of wheat lines Sr31(Benno)/6*LMPG-6 DK42, Seri 82, PBW343, Cham 10, Bacanora carrying the rye translocation (Shamanin et al., 2010; Skolotneva et al., 2018). An alarming occurrence of virulence to Sr24 was recorded among Omsk samples in 2017 (four Pgt isolates, Table 1) because other samples consisted of 100% avirulent to Sr24 pathotypes.
Table 1. Virulent phenotypes of Puccinia graminis f. sp. tritici isolates identified in Western Siberia 2017–2018.
The virulence of Pgt isolates to Sr6, Sr8a, Sr9b, Sr9d, Sr9g, Sr17, and Sr36 were high in each geographical sample, with frequencies of 52.0 to 95.7%. Virulence frequency of 100% was detected toward Sr9d, Sr9g, Sr17 among the Altai samples (Table 2). The virulence of Pgt isolates to Sr9e, Sr11, Sr30 were low, with maximum frequencies of 44.1, 34.2, and 14.7% in Omsk, respectively. For Sr30, no virulent isolates were identified in the Altai samples. Geographic samples varied greatly in virulence for Sr7b, Sr21, and SrTmp (Figure 3). While the Omsk samples were the most virulent with frequencies of 91.1, 67.7, and 85.3%, respectively, the Altai samples were low virulent (23.5% of Pgt isolates infected differential line Sr7b) or even avirulent (toward differential lines Sr21, SrTmp).
Table 2. Virulence frequency of Puccinia graminis f. sp. tritici on a selected set of NA differentials with polymorphic reaction type in the regions of Western Siberia in 2017–2018, %.
Figure 3. Selected set of North American wheat differentials for stem rust with polymorphic infection types of Puccinia graminis f. sp. tritici isolates from Western Siberia in 2017–2018.
A total of 33 virulence phenotypes or races were detected among 115 Pgt isolates tested. No races were identified as common for all three geographical samples. But Novosibirsk samples shared races TKRPF, QHHSF, and MLLTF with Omsk samples and races NFMSF, LKCSF, LKMSF, and PKCSF with the Altai. Among the Omsk and Novosibirsk samples, the most frequently identified race was TKRPF (virulent to Sr5, Sr21, Sr9e, Sr7b, Sr6, Sr8a, Sr9g, Sr36, Sr9b, Sr17, Sr9a, Sr10, SrTmp, Sr38, and SrMcN), which comprised up to 36% of Pgt collection obtained during 2017–2018 (Table 1). Among the Altai and Novosibirsk samples, races LKCSF and PKCSF were predominant with frequencies of 16 and 11%. Rare phenotypes were more frequent in collections from Omsk region (RRGTF, RKRSP, RFRSF, RFRTF, RCRTP, QFRCF, QHHSF, and SHHSF). In general, virulence to Sr21 differentiated the races on geographical origin: Q, R, ant T races shared by the Omsk and Novosibirk samples, but L and P races shared by the Altai and Novosibirsk samples. The interesting group of M races (MPLTF, MTNTF, MTLTF, MLNTF, MQNTF, MLLTF, and MQLTF) was identified in the bulk sample from Omsk barberry, which was included in the survey in 2018. They differ in virulence of up to 3 resistance genes (Sr6, Sr8a, Sr9g, Sr21, Sr30) and look like a family of virulence phenotypes resulting from the sexual process. Race MLLTF was also identified among samples from Novosibirsk wheat in 2018.
The relationships between virulence phenotypes are shown on the UPGMA dendrogram (Figure 4). Virulence phenotypes were separated into four clusters. Two clusters contain phenotypes shared by samples from Novosibirsk and Omsk only in 2017 (group A) and in both years surveyed (group C). All the Altai phenotypes along with the races NFMSF, LKCSF, LKMSF, and PKCSF from Novosibirsk belong to cluster B. Closely related M-phenotypes detected mainly in Omsk in 2018 are grouped within the distinct cluster D.
Figure 4. UPGMA dendrogram of relationships between virulence phenotypes of Puccinia graminis f. sp. tritici in the regions of Western Siberia in 2017–2018. Code indicates the location of sampling (No = Novosibirsk region, Om = Omsk region, Al = Altai region), year of survey (7 = 2017, 8 = 2018), and name of race. Letters A, B, C, D indicate the group of closely related Pgt races.
Variability within the geographical samples of Pgt changed differently from 2017 to 2018 according to the KW dispersion and the normalized number of effectively different isolates 1nD(T, KW) (Table 3). According to both the estimates, in 2018 variability was approximately 40% larger within the Omsk samples, 40% smaller within the Altai samples, and nearly the same in Novosibirsk. Differences in variability were remarkable between the regions with highest values of KW dispersion in Novosibirsk (0.316 in 2017 and 0.356 in 2018). In the Altai samples, variability was almost two times smaller than in other regions (0.155 in 2017 and 0.107 in 2018). Similar results were established with the normalized effective number of different isolates 1nD(T, KW).
Table 3. Variation within samples of Puccinia graminis f. sp. tritici in the regions of Western Siberia in 2017–2018.
Relationships between the regional samples were estimated with the KB distances for the virulence profiles of Pgt isolates and only for phenotypes without their abundances; it is represented by the corresponding NMDS plots (Figure 5). Clear separation between the Altai and Omsk samples was established, whereas the Novosibirsk samples were in between, but more similar to the Altai ones. These findings were also confirmed by the permutation test for differentiation statistics difKW. Significant differentiation was ascertained between the Omsk and Novosibirsk samples (at p ≥ 0.95) and the Omsk and Altai samples (at p ≥ 0.99) in both years. Hypothesis of differentiation between the Novosibirsk and Altai samples was rejected.
Figure 5. Non-metric multidimensional scaling (NMDS) plot of relationships among the regional collections of isolates of Puccinia graminis f. sp. tritici in Western Siberia in 2017–2018. The collections are encoded according to the sampling location (No = Novosibirsk region, Om = Omsk region, Al = Altai region) and year of survey. The plot was generated based on KB distance between collections with regard to the simple mismatch dissimilarity between isolates (STRESS1 = 0.001).
Management of wheat stem rust can be more effective if an origin of initial infection is known. To ascertain whether inoculum of Pgt in a specific region is endemic or wind disseminated from neighboring areas, monitoring of the pathogen in Western Siberia was performed in 2017–2018. Based on virulence phenotypes, two absolutely different Pgt subpopulations were discovered in the Altai and Omsk regions. The Novosibirsk pathogen population seems to be a mixture of isolates originated from both the neighboring regions virulence phenotypes that arose in the west (TKRPF, QHHSF, and MLLTF) or in the south (NFMSF, LKCSF, LKMSF, and PKCSF) of Western Siberia.
It appears that sexual reproduction to large extent shapes the structure of the Pgt population in Omsk region. Indeed, barberry plants are common in this area, and they are susceptible to stem rust (Shamanin et al., 2015). High variability is indirect evidence of existing sexual stage in the pathogen development. Race composition was characterized by single predominant TKRPF race along with significant number of rare races not detected in recent years elsewhere in Asia and Africa. The group of isolates with similar M- races was sampled from barberry and wheat in Omsk in 2018. Because new races may appear after sexual recombination on alternate host (Jin, 2011), the virulence composition and phenotype structure of Pgt population are highly dynamic. In North America, stem rust races 56, 15B, and QCC originated from barberry were responsible for the severe epidemics in the mid-1930s, mid-1950s, and between 1989 and 1993 (Stakman and Rodenhiser, 1958; Martens et al., 1989). Races of Pgt with a rich virulence spectrum have been isolated from barberry plants in the central region of Russia from 2000 to 2009 (Skolotneva et al., 2013).
Susceptible barberry plants have not been found in the Altai region yet. Variability within the Altai Pgt samples was much lower than in the Omsk region (e.g., 0.107–0.155 vs. 0.232–0.344 for the KW dispersion, Table 3) and similar to P. triticina asexual population with KW values ranging between 0.09 and 0.11 (Gultyaeva et al., 2020). The Altai samples consisted of several closely related rare virulence phenotypes. Therefore, the clonal structure of Pgt population in the Altai region can be assumed with a high confidence. Because of the mild short winter, the urediniospores are able to survive on winter wheat, whose area has increased over the past decade in the region. The new lineages in asexual fungal populations could be rarely generated by somatic hybridization, which has been widely discussed as a source of low genetic diversity (Johnson and Newton, 1946; Watson and Luig, 1958; Cotter and Roberts, 1963; Luig and Watson, 1972). Emergence through somatic hybridization was proven for sadly famous Ug99 lineage of races (Li et al., 2019). For the Altai Pgt subpopulation, low virulence diversity might be provided by somatic hybridization.
Aecia observed on barberry in the Novosibirsk region explained that the rye special form, P. graminis f. sp. secalis, segregated predominantly among the sexual offspring (Peresypkin, 1979; Skolotneva and Salina, 2016). Field and laboratory virulence tests have shown that barberry is not a source of infection for wheat crops in the Novosibirsk region (Kelbin et al., 2019). Thus, wheat stem rust in the Novosibirsk region is not endemic but carried by wind from both neighboring regions. This makes the virulence pattern of local samples most variable (e.g., 0.316–0.356 for KW, Table 3) and dependent on breeding events in the neighboring regions. Although Novosibirsk Pgt population seems to be a mixture of the Omsk and Altai subpopulations, it was much more similar to the Altai one, which primarily reproduced asexually in the period of our survey.
Our results clearly demonstrate significant differentiation among the regional Pgt populations in Western Siberia. The main driving force of the population diversity and geographic differentiation seems to be sexual reproduction of the pathogen fungi in the Omsk region (northwest of the area), whereas only clonal propagation with no evidence of sex is assumed in the Altai region (southeast of the area). These two well-distinguished poles, both geographically and by reproductive ability of Pgt, predetermined extreme boundaries of composition of the pathogen populations with a gradual mixing of Pgt races along the gradient from southeast to northwest of Western Siberia.
The data will be made available by request to the corresponding author.
ESS conceived the research idea and was responsible for sampling the Pgt collections in Novosibirsk and Altai, and acquisition and interpretation of virulence data. EK performed data analysis and interpretation of results. MP performed the virulence analysis of Siberian samples in GRRC. VK contributed to acquisition of virulence data. AM was responsible for reviewing the current state of stem rust worldwide and organized the international collaboration between IC&G and GRRC. VS sampled the Pgt collections in Omsk. EAS was responsible for general design of the work. ESS and EK prepared the first draft, while all authors contributed to shaping the final version of the manuscript.
The work was supported by the IC&G Budgetary Project No̱ 0259-2019-0001-C-01. Stem rust collection in Novosibirsk and Altai in 2017 and 2018 was supported by the Russian Foundation for Basic Research, project No̱ 17-29-08018. Stem rust collection in Omsk in 2017 and 2018 was supported by the Russian Science Foundation Project 16-16-10005.
The authors declare that the research was conducted in the absence of any commercial or financial relationships that could be construed as a potential conflict of interest.
Sincere thanks are given to Prof. Mogens Hovmøller (GRRC, Aarhus University) for the kind invitation to work in GRRC with Siberian samples and attentive supervision.
GRRC Report: Samples of Stem Rust Infected Wheat From Russia (2017). Available online at: https://wheatrust.org/fileadmin/www.grcc.au.dk/International_Services/Pathotype_SR_Results/Country_report_Russia_-_August2017.pdf
Bhattacharya, S. (2017). Deadly new wheat disease threatens Europe's crops. Nature 542, 145–146. doi: 10.1038/nature.2017.21424
Cotter, R. V., and Roberts, B. J. (1963). Synthetic hybrids of two varieties of Puccinia graminis. Phytopathology 53, 344–346.
Gultyaeva, E. I., Shaidayuk, E. L., and Kosman, E. (2020). Regional and temporal differentiation of virulence phenotypes of Puccinia triticina Eriks. from common wheat in Russia during the period 2001-2018. Plant Pathol. 69, 860–871. doi: 10.1111/ppa.13174
Hodson, D., Hovmøller, M., Morgunov, A., Salina, E., and Shamanin, V. (2017). Assessing the Stem Rust Situation in Western Siberia – Aug 2017. Available online at: https://rusttracker.cimmyt.org/?p=7112 (accessed March 6, 2019).
Jin, Y. (2011). Role of Berberis spp. as alternate hosts in generating new races of Puccinia graminis and P. striiformis. Euphytica 179, 105–108. doi: 10.1007/s10681-010-0328-3
Jin, Y., Szabo, L. J., Pretorius, Z. A., Singh, R. P., Ward, R., and Fetch, T. J. (2008). Detection of virulence to resistance gene Sr24 within race TTKS of Puccinia graminis f. sp. tritici. Plant Dis. 92, 923–926. doi: 10.1094/PDIS-92-6-0923
Johnson, T., and Newton, M. (1946). Specialization, hybridization and mutation in the cereal rusts. Bot. Rev. 12, 337–392. doi: 10.1007/BF02861524
Jost, L. (2008). GST and its relatives do not measure differentiation. Mol. Ecol. 17, 4015–4026. doi: 10.1111/j.1365-294X.2008.03887.x
Kelbin, V. N., Skolotneva, E. S., Vidich, S., and Salina, E. A. (2019). Stem rust infection in Novosibirsk region: life cycle and origin. Polish Mycol. Soc. 128.
Kosman, E. (1996). Difference and diversity of plant pathogen populations: a new approach for measuring. Phytopathology 86, 1152–1155.
Kosman, E. (2014). Measuring diversity: from individuals to populations. Eur. J. Plant Pathol. 138, 467–486. doi: 10.1007/s10658-013-0323-3
Kosman, E., Ben-Yehuda, P., Manisterski, J., and Sela, H. (2019). Diversity of virulence phenotypes among annual populations of Puccinia triticina originating from common wheat in Israel during the period 2000-15. Plant Pathol. 68, 1741–1748. doi: 10.1111/ppa.13078
Kosman, E., Dinoor, A., Herrmann, A., and Schachtel, G. A. (2008). Virulence Analysis Tool (VAT). User Manual. Available online at: http://www.tau.ac.il/lifesci/departments/plant_s/members/kosman/VAT.html (accessed 21 May 2019).
Kosman, E., and Leonard, K. J. (2007). Conceptual analysis of methods applied to assessment of diversity within and distance between populations with asexual or mixed mode of reproduction. New Phytol. 174, 683–696. doi: 10.1111/j.1469-8137.2007.02031.x
Li, F., Upadhyaya, N. M., Sperschneider, J., Matny, O., Nguyen-Phuc, H., Mago, R., et al. (2019). Emergence of the Ug99 lineage of the wheat stem rust pathogen through somatic hybridisation. Nat. Commun. 10:5068. doi: 10.1038/s41467-019-12927-7
Luig, N. H., and Watson, I. A. (1972). The role of wild and cultivated grasses in the hybridization of formae speciales of Puccinia graminis. Aust. J. Biol. Sci. 25, 335–342. doi: 10.1071/BI9720335
Martens, J. W., Dunsmore, K. M., and Harder, D. E. (1989). Incidence and virulence of Puccinia graminis in Canada on wheat and barley in 1988. Can. J. Plant. Pathol. 11, 424–430. doi: 10.1080/07060668909501091
Olivera, P., Newcomb, M., Szabo, L. J., Rouse, M., Johnson, J., Gale, S., et al. (2015). Phenotypic and genotypic characterization of race TKTTF of Puccinia graminis f. sp. tritici that caused a wheat stem rust Epidemic in Southern Ethiopiain 2013–14. Phytopathology 105, 917–928. doi: 10.1094/PHYTO-11-14-0302-FI
Pretorius, Z. A., Singh, R. P., Wagoire, W. W., and Payne, T. S. (2000). Detection of virulence to wheat stem rust resistance gene Sr31 in Puccinia graminis f. sp. tritici in Uganda. Plant Dis. 84:203. doi: 10.1094/PDIS.2000.84.2.203B
Roelfs, A. P., and Martens, J. W. (1988). An international system of nomenclature for Puccinia graminis f. sp. tritici. Phytopathology 78, 526–533. doi: 10.1094/Phyto-78-526
Roelfs, A. P., Singh, R. P., and Saari, E. E. (1992). Rust Diseases of Wheat: Concepts and Methods of Disease Management. Mexico: CIMMYT.
Schachtel, G. A., Dinoor, A., Herrmann, A., and Kosman, E. (2012). Comprehensive evaluation of virulence and resistance data: a new analysis tool. Plant Dis. 96, 1060–1063. doi: 10.1094/PDIS-02-12-0114-SR
Scheiner, S. M., Kosman, E., Presley, S. J., and Willig, M. R. (2017). Decomposing functional diversity. Methods Ecol. Evol. 97, 809–820. doi: 10.1111/2041-210X.12696
Shamanin, V., Salina, E., Wanyera, R., Zelenskiy, Y., Olivera, P., and Morgounov, A. (2016). Genetic diversity of spring wheat from Kazakhstan and Russia for resistance to stem rust Ug99. Euphytica 212, 287–296. doi: 10.1007/s10681-016-1769-0
Shamanin, V. P., Gultyaeva, E. I., Shaydyuk, E. L., Petukhovsky, S. L., Pototskaya, I. V., and Levina, S. I. (2015). Monitoring the virulence of the P. triticina fungus populations on the OMGAU experimental field. Bullet. Alt. Gosud. Agr. Univ. 5, 70–75.
Shamanin, V. P., Morgounov, A. I., Manes, J., Zelenskiy, Y., Chursin, A. S., and Levshunov, M. A. (2010). Breeding of spring common wheat for resistance to local populations and virulent race Ug99 of stem rust in West Siberia. Vavilov J. Genet. Breed. 14, 223–231.
Shamanin, V. P., Pototskaya, I. V., Shepelev, S. S., Pozherukova, V. E., Salina, E.A., et al. (2020). Stem rust in Western Siberia - population composition and effective resistance genes. Vavilov J. Genet. Breed. 24, 131–138. doi: 10.18699/VJ20.608
Skolotneva, E. S., Lekomtseva, S. N., and Kosman, E. (2013). The wheat stem rust pathogen in the central region of the Russian Federation. Plant Pathol. 62, 1003–1010. doi: 10.1111/ppa.12019
Skolotneva, E. S., Piskarev, V. V., Leonova, I. N., Bukatich, E., and Salina, E. A. (2018). Incidence and Severity of Rust Diseases in Novosibirsk Region, Western Siberia, Russia. Available online at: https://www.globalrust.org/all-bgri-abstracts?field_bgri_year_value%5B0%5D=2018andcombine=andfield_abstract_tags_tid=Allandpage=9
Skolotneva, E. S., and Salina, E. A. (2016). “Features of the causative agent of wheat stem rust in the conditions of Western Siberia,” in Protection of Grain Crops From Diseases, Pests, Weeds: Achievements and Problems (Russia: Bolshiye Vyazemy), 67–71.
Sochalova, L. P., and Lihenko, I. E. (2015). Genetic Diversity of Spring Wheat for Resistance to Migratory Diseases. Novosibirsk: Siberian Research Institute of Plant Cultivation and Breeding.
Stakman, E. C., Levine, M. N., and Loegering, W. Q. (1962). Identification of Physiologic Races of Puccinia graminis var. tritici. Washington, DC: USDA.
Keywords: black rust, race typing, Puccinia graminis f. sp. tritici, Sr genes, Western Siberia
Citation: Skolotneva ES, Kosman E, Patpour M, Kelbin VN, Morgounov AI, Shamanin VP and Salina EA (2020) Virulence Phenotypes of Siberian Wheat Stem Rust Population in 2017–2018. Front. Agron. 2:6. doi: 10.3389/fagro.2020.00006
Received: 07 March 2020; Accepted: 22 May 2020;
Published: 17 July 2020.
Edited by:
Andreas Börner, Leibniz Institute of Plant Genetics and Crop Plant Research (IPK), GermanyReviewed by:
Cátia Pinto, Biocant Park, SA, PortugalCopyright © 2020 Skolotneva, Kosman, Patpour, Kelbin, Morgounov, Shamanin and Salina. This is an open-access article distributed under the terms of the Creative Commons Attribution License (CC BY). The use, distribution or reproduction in other forums is permitted, provided the original author(s) and the copyright owner(s) are credited and that the original publication in this journal is cited, in accordance with accepted academic practice. No use, distribution or reproduction is permitted which does not comply with these terms.
*Correspondence: Ekaterina S. Skolotneva, c2stc2thQHlhbmRleC5ydQ==
Disclaimer: All claims expressed in this article are solely those of the authors and do not necessarily represent those of their affiliated organizations, or those of the publisher, the editors and the reviewers. Any product that may be evaluated in this article or claim that may be made by its manufacturer is not guaranteed or endorsed by the publisher.
Research integrity at Frontiers
Learn more about the work of our research integrity team to safeguard the quality of each article we publish.