- 1Department of Neurology, University of Michigan, Ann Arbor, MI, United States
- 2Michigan Institute for Neurological Disorders, Farmington Hills, MI, United States
- 3Autoimmune Center of Excellence, University of Michigan, Ann Arbor, MI, United States
- 4Graduate Program in Immunology, Program in Biomedical Sciences, University of Michigan, Ann Arbor, MI, United States
Aging is associated with a progressive decline of innate and adaptive immune responses, called immunosenescence. This phenomenon links to different multiple sclerosis (MS) disease courses among different age groups. While clinical relapse and active demyelination are mainly related to the altered adaptive immunity, including invasion of T- and B-lymphocytes, impairment of innate immune cell (e.g., microglia, astrocyte) function is the main contributor to disability progression and neurodegeneration. Most patients with MS manifest the relapsing-remitting phenotype at a younger age, while progressive phenotypes are mainly seen in older patients. Current disease-modifying therapies (DMTs) primarily targeting adaptive immunity are less efficacious in older patients, suggesting that immunosenescence plays a role in treatment response. This review summarizes the recent immune mechanistic studies regarding immunosenescence in patients with MS and discusses the clinical implications of these findings.
1 Introduction
Traditionally, multiple sclerosis (MS) is considered an immune-mediated inflammatory demyelinating disease of the central nervous system (CNS). However, it is well known that neurodegenerative processes are also involved and play a key role in cumulative disability. While current disease-modifying therapies (DMTs) effectively control inflammation, they do not fully stop disability progression, possibly due to inadequate targeting of neurodegeneration. The lack of clarity regarding the etiology of progression hinders the development of effective therapies for progressive disease. Immunosenescence has emerged as a possible mechanism (Dema et al., 2021).
Immunosenescence is an age-related weakening of adaptative and innate immune responses with altered activation (Nikolich-Žugich, 2018). As a result, older individuals may clear pathogens less effectively, resulting in chronic low-grade inflammation, known as inflammaging, which contributes to tissue damage and neurodegenerative diseases. A multifaceted etiology of inflammaging has been suggested including reduced diversity of gut microbiota, genetic polymorphisms, and obesity, in addition to immunosenescence (Ferrucci and Fabbri, 2018).
Some studies have demonstrated premature immunosenescence in MS (Haegert et al., 2011) and experimental autoimmune encephalitis (EAE) in mice (Zeydan and Kantarci, 2020). Immune system changes have a significant impact on the MS disease course, risk of infection, vaccine efficacy, and response to treatment (Grebenciucova and Berger, 2017). Due to longevity and DMT availability, the number of older patients with MS is increasing rapidly. Though 20 to 40 is the average age of MS onset, 10% develop the disease after age 50, known as late-onset MS (LOMS). Patients with LOMS have a higher proportion of primary progressive MS (PPMS) and a faster rate of progressive disability when compared to those who develop MS before age 50. However, it is interesting to note that the same study found that patients who developed disease prior to the age of 50 had a higher risk of reaching Expanded Disability Status Scale (EDSS) 6 (Andersen et al., 2021). This yields consideration that age-related immune changes may play a role in disease progression. Consequently, understanding these changes in a setting of immunosenescence is crucial to the care of patients with MS in an aging world.
In this review, we summarize the current knowledge of immunosenescence, focusing on humans (Figure 1). We then discuss age-related changes in the adaptive, innate, and CNS immune systems. Finally, we discuss immunosenescence in patients with MS and the clinical implications of this emerging knowledge.
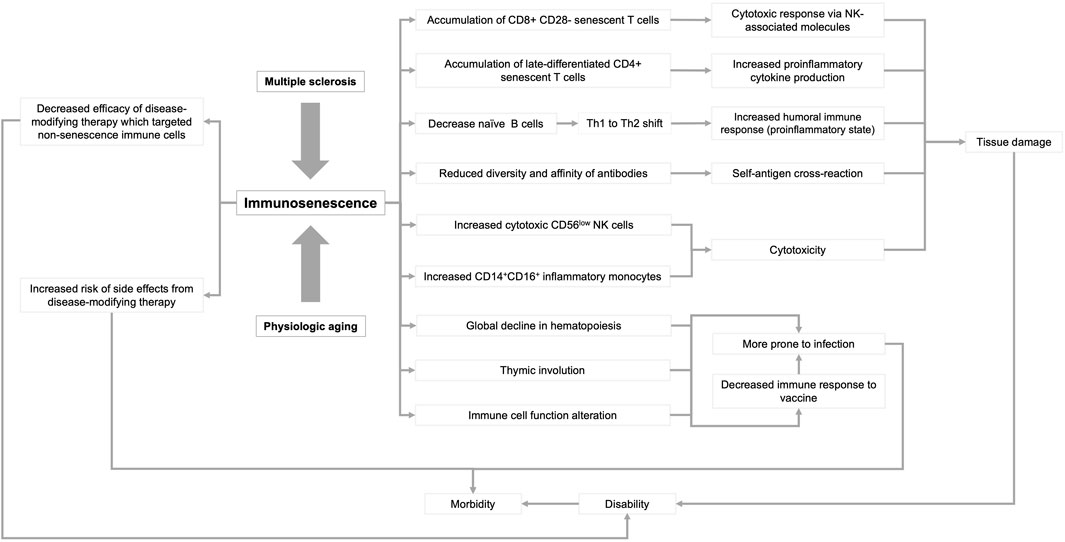
FIGURE 1. Influence of multiple sclerosis and physiological aging on immunosenescence. Immune dysfunction that occurs as a result of aging and multiple sclerosis can lead to a variety of physiological responses that can ultimately lead to disability and morbidity. Arrowheads indicate the directionality of the sequence of events.
2 Aging and immune system changes
Immune cells are generated from hematopoietic stem cells (HSCs) in the bone marrow. They stepwise differentiate and undergo selection and proliferation upon antigenic exposure; thus, becoming prone to the senescence process. Telomere shortening and cell cycle arrest in aging HSCs result in fewer immune cells (Rossi et al., 2005; Vaiserman and Krasnienkov, 2020). Senescent cells can undergo changes to their secretory profile, increasing soluble proteases or insoluble extracellular proteins, which contribute to a pro-inflammatory milieu known as the senescence-associated secretory phenotype (SASP). (Adler et al., 2007; Coppé et al., 2010; Muñoz-Espín and Serrano, 2014). A combination of accumulation of cell debris, self-antigens, and the SASP leads to inflammaging. Immunosenescence impairs the immune response to infection and vaccines due to reduced antigenic response by T- and B-cells (Castle, , 2000; Frasca et al., 2016), contributing to cancer risk (Lian et al., 2020) and immune-mediated disorders in the elderly (Manoussakis et al., 1987; Ramos-Casals et al., 2003).
2.1 Adaptive immune system
Progressive thymus gland involution reduces the naïve T-cell pool and diversity of the T-cell receptor (TCR) repertoire. Reactive dendritic and B-cell homeostatic proliferation then leads to clonal expansion of memory T-cells, further depleting the TCR repertoire (Naylor et al., 2005; Pfister et al., 2006; Qi et al., 2014).
T-cell senescence results in a decrease in CD4+ T-cells and increase in CD8+ T-cells, causing an inversion of the CD4+/CD8+ ratio (Olsso et al., 2000). Late-differentiated memory CD8+ T-cells become senescent, characterized by CD28 loss, telomere shortening, and resistance to apoptosis (Derhovanessian et al., 2011). These senescent T-cells express CD57, killer-cell lectin-like receptor G1 (KLRG1), and natural killer (NK)-associated receptors NKG2D (Vallejo, 2005) and acquire a cytotoxic response via NK-associated molecules while losing response to TCR-mediated signals (Pereira et al., 2020). CD8+ T cells display SASP regulated by p38 MAPK signaling (Callender et al., 2018). Late-differentiated CD4+T-cells feature decreased CD28, increased NKG2D expression, elevated interferon-gamma (IFN-γ) production (Weyand et al., 1998; Alonso-Arias et al., 2011; Dema et al., 2021), and a lack of CD40 ligand expression. A shift from Th1 to Th2 cells and reduced IL-2 production were observed with aging (Shearer, 1997; Ginaldi et al., 1999a; Ginaldi et al., 1999b).
The percentage of total B-cells, including B-cell activating factor (BAFF) and A-proliferation-inducing ligand (APRIL), abruptly decreases after age 75 due to hematopoiesis. (Jin et al., 2008; Bulati et al., 2011). Progenitor B-cells, B-cell lymphopoiesis, and absolute count of relative peripheral CD19+ B-cells decrease with age, while proportions of B-cell subsets remain independent of age (Perdaens and van Pesch, 2021). The memory B-cell population expands with age (Frasc et al., 2017; Frasca et al., 2017). Naïve mature (IgD+CD27−) B-cells decrease while exhausted double-negative (IgD−CD27−) B-cells increase (Colonna-Romano et al., 2009) and produce higher levels of pro-inflammatory cytokines, including tumor necrosis factor-alpha (TNF-α), IL-6, and IL-8 in the elderly compared to younger individuals (Frasc et al., 2017). Immature transitional immunoregulatory CD24highCD38high B-cells reduce with age, causing less IL-10 production (Duggal et al., 2013).
Serum IgM and IgD decrease with age, while concentrations of IgG and IgA increase due to a reduction in antibody class switching and affinity maturation in B-cells during clonal expansion (Listì et al., 2006; Frasca et al., 2008). Although B-cell antigen receptor repertoire diversity and response in the peripheral blood and lymph nodes are reduced in the elderly, somatic hypermutation does not change with age (Tabibian-Keissar et al., 2016).
Age-associated B-cells (ABCs), including IgD−CD27− (aka. double negative B-cell) and CD21−CD11c+ (aka. CD21low B-cell) (Hao et al., 2011; Rubtsov et al., 2011) are a subset of B-cells that increase with age, are pro-inflammatory, and prematurely increase with immune-mediated diseases (Ca and ncro, 2020). However, a recent study demonstrated a decline in B-cell population and inactivation of B-cell specific loci in men over 65 (Márquez et al., 2020).
2.2 Innate immune system
Aging affects the innate immune response through impaired pathogen associated molecular patterns (PAMPs)-mediated response, decreased type I interferon production, and fewer plasmacytoid dendritic cells (Molony et al., 2017; Feng et al., 2021). Monocyte number remains stable in the elderly (Seidler et al., 2010; Ratts and Weng, 2012), but there is an increase in CD14+CD16+ inflammatory monocytes (Nyugen et al., 2010; Seidler et al., 2010; Hearps et al., 2012). These aging monocytes produce less IFN-α, IFN-γ, IL-1β, CCL8, and CCL20 and express CX3C chemokine receptor-1 (CX3CR1), which plays a crucial role in monocyte survival (Metcalf et al., 2017). Moreover, they also overexpressed Tyro 3, Axl, and Mer (TAM) receptors, resulting in impaired clearance of apoptotic cells contributing to inflammation (Wang et al., 2018) and increased TLR4-and TLR8-dependent cytokine production.
Immunoregulatory CD56high NK cells, which augment cytokine and chemokine production, decrease with aging while cytotoxic CD56low NK cells increase (Caligiuri, 2008). NK cells also have defective granulation capacity (Borrego et al., 1999; Le Garff-Tavernier et al., 2010). Neutrophils function in the elderly has diminished suppressor of cytokine signaling 1 (SOCS1) and SOCS3 expression, dysregulation of the JAK-STAT pathway (Fo et al., 2007), and a defective response to triggering receptor expressed on myeloid cells 1 (TREM1), which is responsible for regulating cytokines, chemokines, and reactive oxygen species (ROS) production (Fortin et al., 2006). This can lead to apoptosis dysfunction, limited ROS generation, respiratory burst impairment, and altered cell surface molecule expression, and increased susceptibility to microbial infections (Butcher et al., 2001; Hazeldine et al., 2014; Lopes et al., 2018).
2.3 CNS immunity
Microglia and astrocytes are regulators of the innate and adaptive immune responses in the CNS. Microglia in the aging brain progressively lose their homeostatic molecular signature by developing increased production of pro-inflammatory cytokines, ROS, and dysfunctional lysosomal deposits, which are all linked to the pathogenesis of a growing number of neurodegenerative diseases (Angelova and Brown, 2019). These aged microglia contribute to recruitment of T cells into the CNS via increased TNF-α, which upregulates the adhesion molecules VCAM-1 and ICAM-1 (Zhang et al., 2022). Additionally, a subset of aged human microglia expresses high amounts of ferritin, which may be associated with dystrophic change and senescence, due to iron accumulation and dyshomeostasis, (Lopes et al., 2008; Ward et al., 2014) and may be a consequence of iron-containing oligodendrocyte destruction.
Senescent astrocytes lose homeostatic glutamate uptake, exhibit a propensity for pro-inflammatory responses, and increased expression of glial fibrillary acidic protein (GFAP) and vimentin (Sloane et al., 2000; Porchet et al., 2003) via transforming growth factor-beta 1 (TGFβ1) signaling. TGFβ1 inhibits astrocyte proliferation and induces SASP by increasing pro-inflammatory molecule expression (Coppé et al., 2010). Clearance of senescent astrocytes may also be impaired as age-related thickening of meningeal-lymphatic vessels has been observed in humans (Albayram et al., 2022).
Choroid plexus epithelium (CPE) also undergoes several senescent changes, which may contribute to inflammaging. Alterations in the CPE cytokine and chemokine profile, such as an increased IL-4:IFN-γ ratio, may contribute to a pro-inflammatory state by upregulation of CCL11, which has been implicated in age-related cognitive decline in murine models (Baruch et al., 2013). Likewise, reduced expression of the transmembrane protein Klotho by the CPE leads to increased MHC II expression, microglial activation, and infiltration by peripheral macrophages (Zhu et al., 2018).
3 Immunosenescence in multiple sclerosis
Several immunosenescent phenomena have been observed prematurely in patients with MS, including thymic involution (Duszczyszyn et al., 2010), increased late-differentiated CD8+ memory T cells (Haegele et al., 2007), expansion of ABCs (Claes et al., 2016), and somatic telomere length shortening (Guan et al., 2015). Premature immunosenescence in MS may be caused by repeated antigen exposure leading to chronic immune activation, senescent immune cell endurance, and genotypes that enhance primary differentiation (Bolton and Smith, 2018). Evidence of inflammatory synaptopathy, impairment of synaptic plasticity, and altered microglial response to demyelination has also been shown in the brains of older individuals with MS (Musella et al., 2018) and aged mice (Klein et al., 2018), which may contribute to neurodegeneration (Klein et al., 2018; Musella et al., 2018).
Impaired remyelination with advancing age also contributes to inflammaging. This is, in part, due to a decline in maturation of oligodendrocyte precursor cells (OPCs) (Rist and Franklin, 2008). Additionally, aging macrophages have been shown to exhibit reduced efficiency in the clearance of myelin debris in murine models of demyelination (Natra et al., 2015).
Some changes with DMTs may induce changes to the immune system repertoire that are similar to immunosenescence (Mills and Mao-Draayer, 2018). Most other changes with DMTs have anti-inflammatory effects. Fingolimod and glatiramer were shown to have protective effects on rodent microglia (Pul et al., 2011; Noda et al., 2013). In a recent human study, dimethyl fumarate (DMF) decreased the activation and iron content of microglia in vitro and reduced susceptibility in rim lesions on magnetic resonance imaging (Zinger et al., 2022).
4 Clinical significances of aging in multiple sclerosis
Evidence suggests that the pathogenesis of MS is typified by two concurrent yet distinct inflammatory processes. The first is the invasion of CD8+ T cells and CD20+ B-cells into the CNS, which is associated with blood-brain barrier breakdown, acute demyelination, and clinical relapse. The second is a slow accumulation of late-differentiated T- and B-cells affecting meninges and large periventricular spaces. This latter process is the predominant driver of inflammaging. However, instead of a direct T- or B-cell immune response, it is the downstream effect of microglia and macrophage activation, oxidative injury, and mitochondrial damage, which contribute to neuroinflammatory tissue injury, neurodegeneration, and disability progression and thus could be relevant therapeutic targets for progressive MS (Lassmann, 2018).
The age-dependent nature of relapse rates affected by inflammation and disability progression in MS supports the hypothesis that mechanisms of immune system aging contribute to disease progression (Tremlett et al., 2008; von Wyl et al., 2020). A cohort study from 2008 suggests that annualized MS relapse rate declines after the third decade of life, even when accounting for DMT use (Tremlett et al., 2008). A subsequent study of 9,705 patients with MS, including 236 pediatric-onset patients with MS, further demonstrates that relapse risk was highest in childhood and decreases continuously to about 35 years of age, stabilizes for about a decade, then subsequently decreases once again. In contrast, disability catalysts remained stable from childhood to age 32 and then increased rapidly around age 45 (von Wyl et al., 2020). This observed decline in relapse rate with advancing age does appear to temporally coincide with the age in which immunosenescent changes naturally occur (Rist and Franklin, 2008). The onset of clinical progression depends more on age rather than disease duration, with progression onset at age 45, controlling for SPMS, single attack progressive MS, and PPMS (Tutuncu et al., 2013). Immunosenescent processes, most predominant with increasing age and an altered inflammatory landscape, may contribute to the annual decline of relapse, which requires a modified therapeutic approach.
Epigenetic changes to immune cell subsets occur gradually, but discrete periods of accelerated change have been identified. The first period occurs between 30 and 40 years, while the second typically occurs in the 60s for men and in the 70s for women (Márquez et al., 2020). The first period may influence relapse risk, while the second drives progression. There is evidence that patients with MS experience altered and potentially accelerated immune cell aging (Theodoropoulou et al., 2019; Hecker et al., 2021). Regarding sex, men display accelerated immune aging, possibly accounting for characteristic aggressive disease progression (Moretto et al., 2008; Jergović et al., 2018). In terms of immune cell subsets, women tend to maintain CD4+ T-cell function longer than men, whereas men show a higher degree of epigenetic changes in B cells indicative of a decline or change in function (Márquez et al., 2020). Studies using Siponimod in progressive MS populations suggest that B-cells may be involved in progression (Wu et al., 2020) via epigenetically driven immunological aging (Márquez et al., 2020) as the clinical efficacy of Siponimod may be explained by the shift toward an anti-inflammatory and suppressive homeostatic state (Wu et al., 2020).
5 Disease-modifying therapies in older patients with multiple sclerosis
In progressive MS trials, younger age was associated with positive outcomes of therapeutics targeting the adaptive immune system (Mills et al., 2018). The impact of DMTs on aging, compensatory, inflammatory, and neurodegenerative processes is unclear. Some DMTs may modify microglial cell efficacy (Wesselingh et al., 2019), but their effect on innate immune system inflammaging and disease progression is uncertain. Clinical trials commonly exclude older patients, leading to a lack of understanding of the impact of DMTs on age-related immune changes.
To maximize the therapeutic effect and minimize adverse effects, both animal and human trials sample from a specific age range, usually less than 55 years in humans (Mills et al., 2018; Schweitzer et al., 2019; Zeydan and Kantarci, 2020), which can magnify the effect of suppression on active inflammatory demyelination. In progressive MS trials, the primary benefit demonstrated is the reduction of inflammatory relapse, with only a few trials showing a benefit in disability progression (Mills et al., 2018). With the number of elderly patients with MS increasing (Marrie et al., 2010; Daltrozzo et al., 2018), applying study results to the older population is both necessary and challenging. A meta-analysis of clinical trials showed an age-dependent decline in the effectiveness of DMTs and a higher incidence of adverse effects in the older population (Weideman et al., 2017; Vollmer et al., 2021), and it is known that relapse rates also follow an age-dependent decline, suggesting that, in spite of inflammaging, inflammatory demyelination may be less active in the elderly due to immunosenescent changes.
Discontinuation of DMTs in older patients is challenging, and there is no consensus on timing. Early studies, which included younger patients, demonstrated a high relapse rate after DMT discontinuation (Kister et al., 2016; Bsteh et al., 2017). A small study cohort demonstrated that almost 90% of older patients with MS who had no evidence of active inflammation for two years or more had no clinical relapse after one year of DMT discontinuation. In contrast, a high relapse rate was seen in younger patients who had active CNS inflammation within two years prior to discontinuation (Birnbaum, 2017). In another cohort study investigating 600 patients with MS over the age of 60, the vast majority of 178 patients who discontinued DMT remained off treatment, with only one relapse (0.6%), three radiologic progressions (1.2%) (Hua et al., 2019a), and minimal effect on patient-reported outcomes (Hua et al., 2019b). However, most patients included in these studies used either interferons or glatiramer acetate prior to discontinuation. Therefore, this data should be interpreted with caution in patients using highly effective DMTs. Another important consideration in clinical trials using DMTs with immunosuppressive effects is the increased risk of infections seen in the elderly and with accumulated disability (Vollmer et al., 2021; Brand et al., 2022).
A recent clinical trial evaluated the effects of DMT discontinuation in patients aged 55 or older (ClinicalTrials.gov Identifier: NCT03073603). Although it could not be definitively concluded that discontinuing treatment was non-inferior to continuing, a salient takeaway is that new clinical relapses and new radiographic lesions were rare in both study groups (Corboy et al., 2023). There is now an ongoing extension of the DISCOMS study which will provide further information about the safety of DMT discontinuation in older populations.
6 Aging and infections in multiple sclerosis
Progressive multifocal leukoencephalopathy (PML) is a severe adverse effect of DMTs, particularly natalizumab, for MS (Warnke et al., 2015). Older age may predict a higher risk of PML development and severe outcomes (Jordan et al., 2020). Although uncommon, PML can occur in older adults without immunosuppression or other comorbidities, causing an immunocompromised state (Chang et al., 2007; Zucker and Stacpoole, 2018) and worse outcomes (Havla et al., 2016; Förster et al., 2019). In an Italian cohort, PML occurred after minimal infusions involving older patients (Prosperini et al., 2016). The risks of HSV1 and VZV reactivation, as well as malignancy, potentially increased with age and DMT use (Schweitzer et al., 2019), likely due to immunosenescence. Several cohort studies investigating the outcomes of COVID-19 in patients with MS showed that older age was associated with greater severity and inferior outcomes (Chaudhry et al., 2020; Louapre et al., 2020; Salter et al., 2021; Simpson-Yap et al., 2021). Specific B-cell depleting therapies (i.e., rituximab and ocrelizumab) were also independently associated with worse outcomes when compared to other DMTs. Therefore, B-cell depleting therapies should be used with caution in older patients with MS during COVID-19 pandemic.
7 Aging and vaccine efficacy in multiple sclerosis
Older age is associated with an impaired immune response to primary and booster vaccinations. T-cells favor the generation of short-lived effectors over memory cells (Gustafson et al., 2020), and B-cell response to new antigens is impaired in the elderly, as discussed in Section 2.1. Current evidence suggests that B-cell depleting therapies (Bar-Or et al., 2020) and sphingosine-1-phosphate modulators (Kappos et al., 2015; Ufer et al., 2017; Olberg et al., 2018) significantly blunt humoral immune responses to several vaccines (Ciotti et al., 2020), including influenza, tetanus toxoid (TT), and pneumococcal vaccine. Alemtuzumab may blunt the immune response to vaccines in the first six months after infusion, while the response to prior vaccinations is maintained following alemtuzumab therapy (McCarthy et al., 2013). The above studies did not differentiate between impaired vaccine responses related to disease-specific factors, DMT treatment or aging effect. A few studies demonstrated that natalizumab compromises response to vaccinations, although natalizumab is not known to suppress the systemic immune response (Olberg et al., 2014; Olberg et al., 2018; Metze et al., 2019). In an observational study (Olberg et al., 2014), patients with MS on glatiramer acetate had a lower rate of seroprotection after the 2009 H1N1 “swine flu” vaccination compared to healthy controls. This finding was not reproduced in subsequent studies that used seasonal influenza vaccines (Olberg et al., 2018; Metze et al., 2019). Age, duration of disease, current and prior DMT use may all influence vaccine response (Metze et al., 2019). A randomized control study that compared antibody response to rabies vaccine in healthy individuals who took teriflunomide versus placebo demonstrated that the rabies antibody response was lower in the teriflunomide group. However, all subjects with teriflunomide achieved seroprotective antibody levels (Bar-Or et al., 2015). Several studies have shown that DMF (von Hehn et al., 2018) and beta-interferons do not reduce the immune response to vaccinations (Olberg et al., 2014; Olberg et al., 2018; Metze et al., 2019). In a cohort study investigating the immune response to the hepatitis B virus (HBV), vaccination in older patients with MS was associated with lower antibodies against the HBV surface antigen level following HBV immunization (Faustino et al., 2021).
Regarding COVID-19 vaccines, mRNA vaccines (BNT162b2 and mRNA-1273) have demonstrated high efficacy and immunogenicity. The Ad26.COV2.S vector vaccine was designed as a single-dose vaccine to improve compliance, particularly for older people. This single dose of vaccine resulted in a strong humoral immune response independent of age. Another vector vaccine (ChAdOx1 nCoV-19) needs further investigation to determine the effect of age on immune responses (Teo, 2021). In patients with MS on DMTs, the humoral immune response to the COVID-19 vaccine was reported to be age-independent (Ali et al., 2021). The study also showed that older age, male sex and active smoking were significantly associated with lower antibody titers against SARS-CoV-2 (Pitzalis et al., 2021).Research in this evolving field will help ascertain the aging effects of the COVID-19 and other vaccines.
8 Aging and cancer risk in multiple sclerosis
Older age is one of the most important risk factors for the development of cancer, and there is evidence that immunosenescent changes are necessary to promote tumorigenesis. Several changes in the innate and adaptive immune response described earlier in this paper, which occur in the natural aging process, are also induced by the tumor microenvironment. These include a loss of T cell CD28 expression, increased expression of program death ligand 1 by dendritic cells, and a reduction in pro-inflammatory cytokines, all of which contribute to an immunosuppressive state (Ye et al., 2013). Dysfunction of NK-cell-mediated cytotoxicity and a reduction in naive NK cells can also occur in the elderly and has been suggested to play a role in the development of malignancy (Waldhauer and Steinle, 2008).
Autoimmune conditions and cancer are both a result of immune dysregulation. However, there remains conflicting research regarding whether patients with multiple sclerosis have protective effects against cancer due to increased immunosurveillance or whether they are at increased risk due to chronic inflammation. A higher total incidence of malignancy, as well as an increase in malignancy subtypes (e.g., colorectal, prostate, breast), among MS patients compared to the general population was suggested in one French cohort (Bosco-Levy et al., 2022). Regarding the associated risk among elderly MS patients, an Italian cohort found an increased risk of malignancy in the subpopulation of women with MS over the age of 50, although there was no significant difference between malignancy incidence overall compared to the general population (D'Amico et al., 2019). Likewise, an increase in breast cancer among postmenopausal women with MS compared to age-matched controls was observed in Sweden, whereas there was no increased incidence among premenopausal women (Hajiebrahimi et al., 2016). In contrast, reduced malignancy incidence was observed in cohorts in British Columbia (Kingwell et al., 2012) and New York (Gaindh et al., 2016).
Additionally, the initiation of DMTs for treatment may lead to further immunomodulation or immunosuppression, increasing cancer risk (Bahmanyar et al., 2009). In prior clinical trials, interferons and glatiramer acetate have not been shown to increase the incidence of cancer, unlike natalizumab (Polman et al., 2006), fingolimod (Cohen et al., 2010; Comi et al., 2010; Cala et al., 2014; Lublin et al., 2016), siponimod (Kappos et al., 2018), teriflunomide (O'Connor et al., 2011; Vermersch et al., 2014), cladribine (G et al., 2010), alemtuzumab (Coles et al., 2017; Havrdova et al., 2017), rituximab (Salzer et al., 2016), ocrelizumab (Hauser et al., 2017; Montalban et al., 2017), and DMF (Fox et al., 2012; Gold et al., 2012). Additionally, some DMTs, such as rituximab and cladribine, have been used as cancer treatment prior to their utility in MS. Therefore, there remains a complex interplay between cancer risk and multiple sclerosis and treatment. Prior studies have shown that increased cancer risk in patients on azathioprine, methotrexate, cyclophosphamide, and mitoxantrone is partially attributed to the family history of cancer and cumulative dosage and duration of their treatment (Lebrun et al., 2011; Ragonese et al., 2017).
9 Knowledge gap
Aging is associated with immunosenescence, but the specific changes relevant to disease progression in MS are not well established. The timing of a future progression-targeted therapeutic intervention is crucial and may require non-invasive biomarkers for detection. Biological age measured by leukocyte telomere length (Krysko et al., 2019) and ovarian age measured by the anti-Mullerian hormone is associated with disability progression in MS (Graves et al., 2018). However, the impact of ovarian aging and sex hormones on immunosenescence is not well understood.
Although some DMTs, such as fingolimod (Noda et al., 2013) and glatiramer acetate (Pul et al., 2011), show effects on microglia in animal models, their clinical benefit in human progressive MS has not been proven. Further studies are needed to understand why these effects have not translated to clinical benefit. DMF has shown promising results in reducing microglia activation and iron content on susceptibility MRI (Zinger et al., 2022), but more validation is needed for its use as an imaging marker. It is important to investigate DMTs targeting senescent and chronically activated innate immune cells and their effects on nonactive progressive MS (Mills and Mao-Draayer, 2018). Rejuvenation strategies targeting various immune system components have been proposed, including hematopoietic stem cells, oligodendrocyte precursor cells, microglia, monocytes, thymus, and senescent lymphocytes (Dema et al., 2021).
10 Conclusion
Immunosenescence is an age-related loss of innate and adaptive immune system proficiencies, which influences the course of and accelerates MS. Thus, senescent immune cells may play an essential role in progressive MS. Some DMTs may induce similar immune system changes. Age-related changes in the immune system may contribute to the risk of adverse events, particularly infection, in older patients with MS. However, none of the available DMTs effectively target the immunosenescence process. Therefore, the development of treatment strategies to rejuvenate the immune system may be of hope for patients with progressive MS.
Author contributions
ST conceptualized, outlined, and drafted the manuscript. EM, JY, and TC participated in drafting the manuscript. JD, MB, and CS reviewed and edited the manuscript. YM-D conceptualized, reviewed, and edited the manuscript, as well as supervised the reviewing process. All authors contributed to the article and approved the submitted version.
Funding
YM-D was supported by grants from NIH NIAID Autoimmune Center of Excellence: UM1-AI110557-05, UM1 AI144298-01, PCORI, Novartis, Genentech-Roche, Sanofi-Genzyme, and Chugai.
Conflict of interest
MB has served as a consultant and/or speaker for Biogen, Sanofi, Genentech, Alexion, Horizon, EMD Serono, Bristol Myers Squibb, and TG Therapeutics. YM-D has served as a consultant and/or received grant support from Acorda, Bayer Pharmaceutical, Biogen Idec, Celgene/Bristol Myers Squibb, EMD Serono, Sanofi-Genzyme, Genentech-Roche, Horizon, Novartis, Questor, Janssen, TG Therapeutics, and Teva Neuroscience.
The remaining authors declare that the research was conducted in the absence of any commercial or financial relationships that could be construed as a potential conflict of interest.
Publisher’s note
All claims expressed in this article are solely those of the authors and do not necessarily represent those of their affiliated organizations, or those of the publisher, the editors and the reviewers. Any product that may be evaluated in this article, or claim that may be made by its manufacturer, is not guaranteed or endorsed by the publisher.
References
Adler, A. S., Sinha, S., Kawahara, T. L., Zhang, J. Y., Segal, E., and Chang, H. Y. (2007). Motif module map reveals enforcement of aging by continual NF-kappaB activity. Genes & Dev. 21 (24), 3244–3257. doi:10.1101/gad.1588507
Albayram, M. S., Smith, G., Tufan, F., Tuna, I. S., Bostanciklioglu, M., Zile, M., et al. (2022). Non-invasive MR imaging of human brain lymphatic networks with connections to cervical lymph nodes. Nat. Commun. 13 (1), 203. doi:10.1038/s41467-021-27887-0
Ali, A., Dwyer, D., Wu, Q., Wang, Q., Dowling, C. A., Fox, D. A., et al. (2021). Characterization of humoral response to COVID mRNA vaccines in multiple sclerosis patients on disease modifying therapies. Vaccine 39 (41), 6111–6116. doi:10.1016/j.vaccine.2021.08.078
Alonso-Arias, R., Moro-García, M. A., López-Vázquez, A., Rodrigo, L., Baltar, J., García, F. M. S., et al. (2011). NKG2D expression in CD4+ T lymphocytes as a marker of senescence in the aged immune system. Age (Dordr) 33 (4), 591–605. doi:10.1007/s11357-010-9200-6
Andersen, M. A., Buron, M. D., and Magyari, M. (2021). Late-onset MS is associated with an increased rate of reaching disability milestones. J. Neurol. 268 (9), 3352–3360. doi:10.1007/s00415-021-10490-0
Angelova, D. M., and Brown, D. R. (2019). Microglia and the aging brain: are senescent microglia the key to neurodegeneration? J. Neurochem. 151 (6), 676–688. doi:10.1111/jnc.14860
Bahmanyar, S., Montgomery, S. M., Hillert, J., Ekbom, A., and Olsson, T. (2009). Cancer risk among patients with multiple sclerosis and their parents. Neurology 72 (13), 1170–1177. doi:10.1212/01.wnl.0000345366.10455.62
Bar-Or, A., Calkwood, J. C., Chognot, C., Evershed, J., Fox, E. J., Herman, A., et al. (2020). Effect of ocrelizumab on vaccine responses in patients with multiple sclerosis: the VELOCE study. Neurology 95 (14), e1999–e2008. doi:10.1212/WNL.0000000000010380
Bar-Or, A., Wiendl, H., Miller, B., Benamor, M., Truffinet, P., Church, M., et al. (2015). Randomized study of teriflunomide effects on immune responses to neoantigen and recall antigens. Neurol. Neuroimmunol. Neuroinflamm 2 (2), e70. doi:10.1212/NXI.0000000000000070
Baruch, K., Ron-Harel, N., Gal, H., Deczkowska, A., Shifrut, E., Ndifon, W., et al. (2013). CNS-specific immunity at the choroid plexus shifts toward destructive Th2 inflammation in brain aging. Proc. Natl. Acad. Sci. U. S. A. 110 (6), 2264–2269. doi:10.1073/pnas.1211270110
Birnbaum, G. (2017). Stopping Disease-Modifying Therapy in Nonrelapsing Multiple Sclerosis: experience from a Clinical Practice. Int. J. MS care 19 (1), 11–14. doi:10.7224/1537-2073.2015-032
Bolton, C., and Smith, P. A. (2018). The influence and impact of ageing and immunosenescence (ISC) on adaptive immunity during multiple sclerosis (MS) and the animal counterpart experimental autoimmune encephalomyelitis (EAE). Ageing Res. Rev. 41, 64–81. doi:10.1016/j.arr.2017.10.005
Borrego, F., Alonso, M. C., Galiani, M. D., Carracedo, J., Ramirez, R., Ostos, B., et al. (1999). NK phenotypic markers and IL2 response in NK cells from elderly people. Exp. Gerontol. 34 (2), 253–265. doi:10.1016/s0531-5565(98)00076-x
Bosco-Levy, P., Foch, C., Grelaud, A., Sabido, M., Lacueille, C., Jove, J., et al. (2022). Incidence and risk of cancer among multiple sclerosis patients: A matched population-based cohort study. Eur. J. Neurol. 29 (4), 1091–1099. doi:10.1111/ene.15226
Brand, J. S., Smith, K. A., Piehl, F., Olsson, T., and Montgomery, S. (2022). Risk of serious infections in multiple sclerosis patients by disease course and disability status: results from a Swedish register-based study. Brain Behav. Immun. Health 22, 100470. doi:10.1016/j.bbih.2022.100470
Bsteh, G., Feige, J., Ehling, R., Auer, M., Hegen, H., Di Pauli, F., et al. (2017). Discontinuation of disease-modifying therapies in multiple sclerosis - Clinical outcome and prognostic factors. Mult. Scler. 23 (9), 1241–1248. doi:10.1177/1352458516675751
Bulati, M., Buffa, S., Candore, G., Caruso, C., Dunn-Walters, D. K., Pellicanò, M., et al. (2011). B cells and immunosenescence: a focus on IgG+IgD-CD27- (DN) B cells in aged humans. Ageing Res. Rev. 10 (2), 274–284. doi:10.1016/j.arr.2010.12.002
Butcher, S. K., Chahal, H., Nayak, L., Sinclair, A., Henriquez, N. V., Sapey, E., et al. (2001). Senescence in innate immune responses: reduced neutrophil phagocytic capacity and CD16 expression in elderly humans. J. Leukoc. Biol. 70 (6), 881–886. doi:10.1189/jlb.70.6.881
Cancro, M. P. (2020). Age-Associated B Cells. Annu. Rev. Immunol. 38, 315–340. doi:10.1146/annurev-immunol-092419-031130
Calabresi, P. A., Radue, E. W., Goodin, D., Jeffery, D., Rammohan, K. W., Reder, A. T., et al. (2014). Safety and efficacy of fingolimod in patients with relapsing-remitting multiple sclerosis (FREEDOMS II): a double-blind, randomised, placebo-controlled, phase 3 trial. Lancet Neurol. 13 (6), 545–556. doi:10.1016/S1474-4422(14)70049-3
Caligiuri, M. A. (2008). Human natural killer cells. Blood 112 (3), 461–469. doi:10.1182/blood-2007-09-077438
Callender, L. A., Carroll, E. C., Beal, R. W. J., Chambers, E. S., Nourshargh, S., Akbar, A. N., et al. (2018). Human CD8(+) EMRA T cells display a senescence-associated secretory phenotype regulated by p38 MAPK. Aging Cell 17 (1), e12675. doi:10.1111/acel.12675
Castle, S. C. (2000). Clinical relevance of age-related immune dysfunction. Clin. Infect. Dis. official Publ. Infect. Dis. Soc. Am. 31 (2), 578–585. doi:10.1086/313947
Chang, Y. Y., Lan, M. Y., Peng, C. H., Wu, H. S., Chang, D., and Liu, J. S. (2007). Progressive multifocal leukoencephalopathy in an immunocompetent Taiwanese patient. J. Formos. Med. Assoc. = Taiwan yi zhi 106 (2), S60–S64. doi:10.1016/s0929-6646(09)60355-7
Chaudhry, F., Bulka, H., Rathnam, A. S., Said, O. M., Lin, J., Lorigan, H., et al. (2020). COVID-19 in multiple sclerosis patients and risk factors for severe infection. J. Neurol. Sci. 418, 117147. doi:10.1016/j.jns.2020.117147
Ciotti, J. R., Valtcheva, M. V., and Cross, A. H. (2020). Effects of MS disease-modifying therapies on responses to vaccinations: A review. Mult. Scler. Relat. Disord. 45, 102439. doi:10.1016/j.msard.2020.102439
Claes, N., Fraussen, J., Vanheusden, M., Hellings, N., Stinissen, P., Van Wijmeersch, B., et al. (2016). Age-associated B Cells with proinflammatory characteristics are expanded in a proportion of multiple sclerosis patients. J. Immunol. 197 (12), 4576–4583. doi:10.4049/jimmunol.1502448
Cohen, J. A., Barkhof, F., Comi, G., Hartung, H. P., Khatri, B. O., Montalban, X., et al. (2010). Oral fingolimod or intramuscular interferon for relapsing multiple sclerosis. N. Engl. J. Med. 362 (5), 402–415. doi:10.1056/NEJMoa0907839
Coles, A. J., Cohen, J. A., Fox, E. J., Giovannoni, G., Hartung, H. P., Havrdova, E., et al. (2017). Alemtuzumab CARE-MS II 5-year follow-up: efficacy and safety findings. Neurology 89 (11), 1117–1126. doi:10.1212/WNL.0000000000004354
Colonna-Romano, G., Bulati, M., Aquino, A., Pellicanò, M., Vitello, S., Lio, D., et al. (2009). A double-negative (IgD-CD27-) B cell population is increased in the peripheral blood of elderly people. Mech. ageing Dev. 130 (10), 681–690. doi:10.1016/j.mad.2009.08.003
Comi, G., O'Connor, P., Montalban, X., Antel, J., Radue, E. W., Karlsson, G., et al. (2010). Phase II study of oral fingolimod (FTY720) in multiple sclerosis: 3-year results. Mult. Scler. 16 (2), 197–207. doi:10.1177/1352458509357065
Coppé, J. P., Desprez, P. Y., Krtolica, A., and Campisi, J. (2010). The senescence-associated secretory phenotype: the dark side of tumor suppression. Annu. Rev. pathology 5, 99–118. doi:10.1146/annurev-pathol-121808-102144
Corboy, J. R., Fox, R. J., Kister, I., Cutter, G. R., Morgan, C. J., Seale, R., et al. (2023). Risk of new disease activity in patients with multiple sclerosis who continue or discontinue disease-modifying therapies (DISCOMS): a multicentre, randomised, single-blind, phase 4, non-inferiority trial. Lancet Neurol. 22 (7), 568–577. doi:10.1016/S1474-4422(23)00154-0
D'Amico, E., Chisari, C. G., Arena, S., Zanghi, A., Toscano, S., Lo Fermo, S., et al. (2019). Cancer Risk and Multiple Sclerosis: evidence From a Large Italian Cohort. Front. Neurol. 10, 337. doi:10.3389/fneur.2019.00337
Daltrozzo, T., Hapfelmeier, A., Donnachie, E., Schneider, A., and Hemmer, B. (2018). A systematic assessment of prevalence, incidence and regional distribution of multiple sclerosis in Bavaria from 2006 to 2015. Front. Neurol. 9, 871. doi:10.3389/fneur.2018.00871
Dema, M., Eixarch, H., Villar, L. M., Montalban, X., and Espejo, C. (2021). Immunosenescence in multiple sclerosis: the identification of new therapeutic targets. Autoimmun. Rev. 20 (9), 102893. doi:10.1016/j.autrev.2021.102893
Derhovanessian, E., Maier, A. B., Hähnel, K., Beck, R., de Craen, A. J. M., Slagboom, E. P., et al. (2011). Infection with cytomegalovirus but not herpes simplex virus induces the accumulation of late-differentiated CD4+ and CD8+ T-cells in humans. J. general virology 92 (12), 2746–2756. doi:10.1099/vir.0.036004-0
Duggal, N. A., Upton, J., Phillips, A. C., Sapey, E., and Lord, J. M. (2013). An age-related numerical and functional deficit in CD19(+) CD24(hi) CD38(hi) B cells is associated with an increase in systemic autoimmunity. Aging Cell 12 (5), 873–881. doi:10.1111/acel.12114
Duszczyszyn, D. A., Williams, J. L., Mason, H., Lapierre, Y., Antel, J., and Haegert, D. G. (2010). Thymic involution and proliferative T-cell responses in multiple sclerosis. J. Neuroimmunol. 221 (1-2), 73–80. doi:10.1016/j.jneuroim.2010.02.005
Faustino, P., Coutinho, M., Leitão, L., Capela, C., Brum, M., Parra, J., et al. (2021). Seroconversion rate following HBV vaccination in clinical practice: the role of age and DMT treatment. Mult. Scler. Relat. Disord. 50, 102859. doi:10.1016/j.msard.2021.102859
Feng, E., Balint, E., Poznanski, S. M., Ashkar, A. A., and Loeb, M. (2021). Aging and Interferons: impacts on Inflammation and Viral Disease Outcomes. Cells 10 (3), 708. doi:10.3390/cells10030708
Ferrucci, L., and Fabbri, E. (2018). Inflammageing: chronic inflammation in ageing, cardiovascular disease, and frailty. Nat. Rev. Cardiol. 15 (9), 505–522. doi:10.1038/s41569-018-0064-2
Fortin, C. F., Lesur, O., and Fulop, T. (2007). Effects of aging on triggering receptor expressed on myeloid cells (TREM)-1-induced PMN functions. FEBS Lett. 581 (6), 1173–1178. doi:10.1016/j.febslet.2007.02.029
Förster, M., Küry, P., Aktas, O., Warnke, C., Havla, J., Hohlfeld, R., et al. (2019). Managing risks with immune therapies in multiple sclerosis. Drug Saf. 42 (5), 633–647. doi:10.1007/s40264-018-0782-8
Fortin, C. F., Larbi, A., Lesur, O., Douziech, N., and Fulop, T. (2006). Impairment of SHP-1 down-regulation in the lipid rafts of human neutrophils under GM-CSF stimulation contributes to their age-related, altered functions. J. Leukoc. Biol. 79 (5), 1061–1072. doi:10.1189/jlb.0805481
Fox, R. J., Miller, D. H., Phillips, J. T., Hutchinson, M., Havrdova, E., Kita, M., et al. (2012). Placebo-controlled phase 3 study of oral BG-12 or glatiramer in multiple sclerosis. N. Engl. J. Med. 367 (12), 1087–1097. doi:10.1056/NEJMoa1206328
Frasca, D., Diaz, A., Romero, M., and Blomberg, B. B. (2017). Human peripheral late/exhausted memory B cells express a senescent-associated secretory phenotype and preferentially utilize metabolic signaling pathways. Exp. Gerontol. 87, 113–120. doi:10.1016/j.exger.2016.12.001
Frasca, D., Diaz, A., Romero, M., and Blomberg, B. B. (2016). The generation of memory B cells is maintained, but the antibody response is not, in the elderly after repeated influenza immunizations. Vaccine 34 (25), 2834–2840. doi:10.1016/j.vaccine.2016.04.023
Frasca, D., Diaz, A., Romero, M., D'Eramo, F., and Blomberg, B. B. (2017). Aging effects on T-bet expression in human B cell subsets. Cell Immunol. 321, 68–73. doi:10.1016/j.cellimm.2017.04.007
Frasca, D., Landin, A. M., Lechner, S. C., Ryan, J. G., Schwartz, R., Riley, R. L., et al. (2008). Aging down-regulates the transcription factor E2A, activation-induced cytidine deaminase, and Ig class switch in human B cells. J. Immunol. 180 (8), 5283–5290. doi:10.4049/jimmunol.180.8.5283
Giovannoni, G., Comi, G., Cook, S., Rammohan, K., Rieckmann, P., Soelberg Sorensen, P., et al. (2010). A placebo-controlled trial of oral cladribine for relapsing multiple sclerosis. N. Engl. J. Med. 362 (5), 416–426. doi:10.1056/NEJMoa0902533
Gaindh, D., Kavak, K. S., Teter, B., Vaughn, C. B., Cookfair, D., Hahn, T., et al. (2016). Decreased risk of cancer in multiple sclerosis patients and analysis of the effect of disease modifying therapies on cancer risk. J. Neurol. Sci. 370, 13–17. doi:10.1016/j.jns.2016.09.005
Ginaldi, L., De Martinis, M., D'Ostilio, A., Marini, L., Loreto, M. F., Corsi, M. P., et al. (1999a). The immune system in the elderly: I. Specific humoral immunity. Immunol. Res. 20 (2), 101–108. doi:10.1007/BF02786466
Ginaldi, L., De Martinis, M., D'Ostilio, A., Marini, L., Loreto, M. F., Martorelli, V., et al. (1999b). The immune system in the elderly: II. Specific cellular immunity. Immunol. Res. 20 (2), 109–115. doi:10.1007/BF02786467
Gold, R., Kappos, L., Arnold, D. L., Bar-Or, A., Giovannoni, G., Selmaj, K., et al. (2012). Placebo-controlled phase 3 study of oral BG-12 for relapsing multiple sclerosis. N. Engl. J. Med. 367 (12), 1098–1107. doi:10.1056/NEJMoa1114287
Graves, J. S., Henry, R. G., Cree, B. A. C., Lambert-Messerlian, G., Greenblatt, R. M., Waubant, E., et al. (2018). Ovarian aging is associated with gray matter volume and disability in women with MS. Neurology 90 (3), e254–e260. doi:10.1212/WNL.0000000000004843
Grebenciucova, E., and Berger, J. R. (2017). Immunosenescence: the role of aging in the predisposition to neuro-infectious complications arising from the treatment of multiple sclerosis. Curr. neurology Neurosci. Rep. 17 (8), 61. doi:10.1007/s11910-017-0771-9
Guan, J. Z., Guan, W. P., Maeda, T., Guoqing, X., GuangZhi, W., and Makino, N. (2015). Patients with multiple sclerosis show increased oxidative stress markers and somatic telomere length shortening. Mol. Cell. Biochem. 400 (1-2), 183–187. doi:10.1007/s11010-014-2274-1
Gustafson, C. E., Kim, C., Weyand, C. M., and Goronzy, J. J. (2020). Influence of immune aging on vaccine responses. J. Allergy Clin. Immunol. 145 (5), 1309–1321. doi:10.1016/j.jaci.2020.03.017
Haegele, K. F., Stueckle, C. A., Malin, J. P., and Sindern, E. (2007). Increase of CD8+ T-effector memory cells in peripheral blood of patients with relapsing-remitting multiple sclerosis compared to healthy controls. J. Neuroimmunol. 183 (1-2), 168–174. doi:10.1016/j.jneuroim.2006.09.008
Haegert, D. G., Hackenbroch, J. D., Duszczyszyn, D., Fitz-Gerald, L., Zastepa, E., Mason, H., et al. (2011). Reduced thymic output and peripheral naive CD4 T-cell alterations in primary progressive multiple sclerosis (PPMS). J. Neuroimmunol. 233 (1-2), 233–239. doi:10.1016/j.jneuroim.2010.12.007
Hajiebrahimi, M., Montgomery, S., Burkill, S., and Bahmanyar, S. (2016). Risk of Premenopausal and Postmenopausal Breast Cancer among Multiple Sclerosis Patients. PLoS One 11 (10), e0165027. doi:10.1371/journal.pone.0165027
Hao, Y., O'Neill, P., Naradikian, M. S., Scholz, J. L., and Cancro, M. P. (2011). A B-cell subset uniquely responsive to innate stimuli accumulates in aged mice. Blood 118 (5), 1294–1304. doi:10.1182/blood-2011-01-330530
Hauser, S. L., Bar-Or, A., Comi, G., Giovannoni, G., Hartung, H. P., Hemmer, B., et al. (2017). Ocrelizumab versus Interferon Beta-1a in Relapsing Multiple Sclerosis. N. Engl. J. Med. 376 (3), 221–234. doi:10.1056/NEJMoa1601277
Havla, J., Warnke, C., Derfuss, T., Kappos, L., Hartung, H. P., and Hohlfeld, R. (2016). Interdisciplinary risk management in the treatment of multiple sclerosis. Dtsch. Arztebl Int. 113 (51-52), 879–886. doi:10.3238/arztebl.2016.0879
Havrdova, E., Arnold, D. L., Cohen, J. A., Hartung, H. P., Fox, E. J., Giovannoni, G., et al. (2017). Alemtuzumab CARE-MS I 5-year follow-up: durable efficacy in the absence of continuous MS therapy. Neurology 89 (11), 1107–1116. doi:10.1212/WNL.0000000000004313
Hazeldine, J., Harris, P., Chapple, I. L., Grant, M., Greenwood, H., Livesey, A., et al. (2014). Impaired neutrophil extracellular trap formation: a novel defect in the innate immune system of aged individuals. Aging Cell 13 (4), 690–698. doi:10.1111/acel.12222
Hearps, A. C., Martin, G. E., Angelovich, T. A., Cheng, W. J., Maisa, A., Landay, A. L., et al. (2012). Aging is associated with chronic innate immune activation and dysregulation of monocyte phenotype and function. Aging Cell 11 (5), 867–875. doi:10.1111/j.1474-9726.2012.00851.x
Hecker, M., Fitzner, B., Jager, K., Buhring, J., Schwartz, M., Hartmann, A., et al. (2021). Leukocyte Telomere Length in Patients with Multiple Sclerosis and Its Association with Clinical Phenotypes. Mol. Neurobiol. 58 (6), 2886–2896. doi:10.1007/s12035-021-02315-y
Hua, L. H., Fan, T. H., Conway, D., Thompson, N., and Kinzy, T. G. (2019a). Discontinuation of disease-modifying therapy in patients with multiple sclerosis over age 60. Mult. Scler. 25 (5), 699–708. doi:10.1177/1352458518765656
Hua, L. H., Harris, H., Conway, D., and Thompson, N. R. (2019b). Changes in patient-reported outcomes between continuers and discontinuers of disease modifying therapy in patients with multiple sclerosis over age 60. Mult. Scler. Relat. Disord. 30, 252–256. doi:10.1016/j.msard.2019.02.028
Jergović, M., Smithey, M. J., and Nikolich-Žugich, J. (2018). Intrinsic and extrinsic contributors to defective CD8+ T cell responses with aging. Exp. Gerontol. 105, 140–145. doi:10.1016/j.exger.2018.01.011
Jin, R., Kaneko, H., Suzuki, H., Arai, T., Teramoto, T., Fukao, T., et al. (2008). Age-related changes in BAFF and APRIL profiles and upregulation of BAFF and APRIL expression in patients with primary antibody deficiency. Int. J. Mol. Med. 21 (2), 233–238. doi:10.3892/ijmm.21.2.233
Jordan, A. L., Yang, J., Fisher, C. J., Racke, M. K., and Mao-Draayer, Y. (2020). Progressive multifocal leukoencephalopathy in dimethyl fumarate-treated multiple sclerosis patients. Mult. Scler. 28, 7–15. doi:10.1177/1352458520949158
Kappos, L., Bar-Or, A., Cree, B. A. C., Fox, R. J., Giovannoni, G., Gold, R., et al. (2018). Siponimod versus placebo in secondary progressive multiple sclerosis (EXPAND): a double-blind, randomised, phase 3 study. Lancet 391 (10127), 1263–1273. doi:10.1016/S0140-6736(18)30475-6
Kappos, L., Mehling, M., Arroyo, R., Izquierdo, G., Selmaj, K., Curovic-Perisic, V., et al. (2015). Randomized trial of vaccination in fingolimod-treated patients with multiple sclerosis. Neurology 84 (9), 872–879. doi:10.1212/WNL.0000000000001302
Kingwell, E., Bajdik, C., Phillips, N., Zhu, F., Oger, J., Hashimoto, S., et al. (2012). Cancer risk in multiple sclerosis: findings from British Columbia, Canada. Brain 135 (10), 2973–2979. doi:10.1093/brain/aws148
Kister, I., Spelman, T., Alroughani, R., Lechner-Scott, J., Duquette, P., Grand'Maison, F., et al. (2016). Discontinuing disease-modifying therapy in MS after a prolonged relapse-free period: a propensity score-matched study. J. Neurol. Neurosurg. Psychiatry 87 (10), 1133–1137. doi:10.1136/jnnp-2016-313760
Klein, B., Mrowetz, H., Barker, C. M., Lange, S., Rivera, F. J., and Aigner, L. (2018). Age influences microglial activation after cuprizone-induced demyelination. Front. aging Neurosci. 10, 278. doi:10.3389/fnagi.2018.00278
Krysko, K. M., Henry, R. G., Cree, B. A. C., Lin, J., Caillier, S., Santaniello, A., et al. (2019). Telomere length Is associated with disability progression in multiple sclerosis. Ann. Neurol. 86 (5), 671–682. doi:10.1002/ana.25592
Lassmann, H. (2018). Pathogenic mechanisms associated with different clinical courses of multiple sclerosis. Front. Immunol. 9, 3116. doi:10.3389/fimmu.2018.03116
Le Garff-Tavernier, M., Béziat, V., Decocq, J., Siguret, V., Gandjbakhch, F., Pautas, E., et al. (2010). Human NK cells display major phenotypic and functional changes over the life span. Aging Cell 9 (4), 527–535. doi:10.1111/j.1474-9726.2010.00584.x
Lebrun, C., Vermersch, P., Brassat, D., Defer, G., Rumbach, L., Clavelou, P., et al. (2011). Cancer and multiple sclerosis in the era of disease-modifying treatments. J. Neurol. 258 (7), 1304–1311. doi:10.1007/s00415-011-5929-9
Lian, J., Yue, Y., Yu, W., and Zhang, Y. (2020). Immunosenescence: a key player in cancer development. J. Hematol. Oncol. 13 (1), 151. doi:10.1186/s13045-020-00986-z
Listì, F., Candore, G., Modica, M. A., Russo, M., Di Lorenzo, G., Esposito-Pellitteri, M., et al. (2006). A study of serum immunoglobulin levels in elderly persons that provides new insights into B cell immunosenescence. Ann. N. Y. Acad. Sci. 1089, 487–495. doi:10.1196/annals.1386.013
Lopes, A. B., Lopes, L. B., da Silveira Antunes, R. N., Fukasawa, J. T., de, A. C. D., and Calamita, Z. (2018). Effects of immunosenescence on the lower expression of surface molecules in neutrophils and lymphocytes. Curr. aging Sci. 11 (2), 118–125. doi:10.2174/1874609811666180605092234
Lopes, K. O., Sparks, D. L., and Streit, W. J. (2008). Microglial dystrophy in the aged and Alzheimer's disease brain is associated with ferritin immunoreactivity. Glia 56 (10), 1048–1060. doi:10.1002/glia.20678
Louapre, C., Collongues, N., Stankoff, B., Giannesini, C., Papeix, C., Bensa, C., et al. (2020). Clinical Characteristics and Outcomes in Patients With Coronavirus Disease 2019 and Multiple Sclerosis. JAMA Neurol. 77 (9), 1079–1088. doi:10.1001/jamaneurol.2020.2581
Lublin, F., Miller, D. H., Freedman, M. S., Cree, B. A. C., Wolinsky, J. S., Weiner, H., et al. (2016). Oral fingolimod in primary progressive multiple sclerosis (INFORMS): a phase 3, randomised, double-blind, placebo-controlled trial. Lancet 387 (10023), 1075–1084. doi:10.1016/S0140-6736(15)01314-8
Manoussakis, M. N., Tzioufas, A. G., Silis, M. P., Pange, P. J., Goudevenos, J., and Moutsopoulos, H. M. (1987). High prevalence of anti-cardiolipin and other autoantibodies in a healthy elderly population. Clin. Exp. Immunol. 69 (3), 557–565.
Márquez, E. J., Chung, C.-H., Marches, R., Rossi, R. J., Nehar-Belaid, D., Eroglu, A., et al. (2020). Sexual-dimorphism in human immune system aging. Nat. Commun. 11 (1), 751. doi:10.1038/s41467-020-14396-9
Marrie, R. A., Yu, N., Blanchard, J., Leung, S., and Elliott, L. (2010). The rising prevalence and changing age distribution of multiple sclerosis in Manitoba. Neurology 74 (6), 465–471. doi:10.1212/WNL.0b013e3181cf6ec0
McCarthy, C. L., Tuohy, O., Compston, D. A., Kumararatne, D. S., Coles, A. J., and Jones, J. L. (2013). Immune competence after alemtuzumab treatment of multiple sclerosis. Neurology 81 (10), 872–876. doi:10.1212/WNL.0b013e3182a35215
Metcalf, T. U., Wilkinson, P. A., Cameron, M. J., Ghneim, K., Chiang, C., Wertheimer, A. M., et al. (2017). Human monocyte subsets are transcriptionally and functionally altered in aging in response to pattern recognition receptor agonists. J. Immunol. 199 (4), 1405–1417. doi:10.4049/jimmunol.1700148
Metze, C., Winkelmann, A., Loebermann, M., Hecker, M., Schweiger, B., Reisinger, E. C., et al. (2019). Immunogenicity and predictors of response to a single dose trivalent seasonal influenza vaccine in multiple sclerosis patients receiving disease-modifying therapies. CNS Neurosci. Ther. 25 (2), 245–254. doi:10.1111/cns.13034
Mills, E. A., Begay, J. A., Fisher, C., and Mao-Draayer, Y. (2018). Impact of trial design and patient heterogeneity on the identification of clinically effective therapies for progressive MS. Mult. Scler. 24 (14), 1795–1807. doi:10.1177/1352458518800800
Mills, E. A., and Mao-Draayer, Y. (2018). Aging and lymphocyte changes by immunomodulatory therapies impact PML risk in multiple sclerosis patients. Mult. Scler. 24 (8), 1014–1022. doi:10.1177/1352458518775550
Molony, R. D., Nguyen, J. T., Kong, Y., Montgomery, R. R., Shaw, A. C., and Iwasaki, A. (2017). Aging impairs both primary and secondary RIG-I signaling for interferon induction in human monocytes. Sci. Signal 10 (509), eaan2392. doi:10.1126/scisignal.aan2392
Montalban, X., Hauser, S. L., Kappos, L., Arnold, D. L., Bar-Or, A., Comi, G., et al. (2017). Ocrelizumab versus Placebo in Primary Progressive Multiple Sclerosis. N. Engl. J. Med. 376 (3), 209–220. doi:10.1056/NEJMoa1606468
Moretto, M. M., Lawlor, E. M., and Khan, I. A. (2008). Aging mice exhibit a functional defect in mucosal dendritic cell response against an intracellular pathogen. J. Immunol. 181 (11), 7977–7984. doi:10.4049/jimmunol.181.11.7977
Muñoz-Espín, D., and Serrano, M. (2014). Cellular senescence: from physiology to pathology. Nat. Rev. Mol. Cell Biol. 15 (7), 482–496. doi:10.1038/nrm3823
Musella, A., Gentile, A., Rizzo, F. R., De Vito, F., Fresegna, D., Bullitta, S., et al. (2018). Interplay between age and neuroinflammation in multiple sclerosis: effects on motor and cognitive functions. Front. Aging Neurosci. 10, 238. doi:10.3389/fnagi.2018.00238
Natrajan, M. S., de la Fuente, A. G., Crawford, A. H., Linehan, E., Nunez, V., Johnson, K. R., et al. (2015). Retinoid X receptor activation reverses age-related deficiencies in myelin debris phagocytosis and remyelination. Brain 138 (12), 3581–3597. doi:10.1093/brain/awv289
Naylor, K., Li, G., Vallejo, A. N., Lee, W. W., Koetz, K., Bryl, E., et al. (2005). The influence of age on T cell generation and TCR diversity. J. Immunol. 174 (11), 7446–7452. doi:10.4049/jimmunol.174.11.7446
Nikolich-Žugich, J. (2018). The twilight of immunity: emerging concepts in aging of the immune system. Nat. Immunol. 19 (1), 10–19. doi:10.1038/s41590-017-0006-x
Noda, H., Takeuchi, H., Mizuno, T., and Suzumura, A. (2013). Fingolimod phosphate promotes the neuroprotective effects of microglia. J. Neuroimmunol. 256 (1-2), 13–18. doi:10.1016/j.jneuroim.2012.12.005
Nyugen, J., Agrawal, S., Gollapudi, S., and Gupta, S. (2010). Impaired functions of peripheral blood monocyte subpopulations in aged humans. J. Clin. Immunol. 30 (6), 806–813. doi:10.1007/s10875-010-9448-8
O'Connor, P., Wolinsky, J. S., Confavreux, C., Comi, G., Kappos, L., Olsson, T. P., et al. (2011). Randomized trial of oral teriflunomide for relapsing multiple sclerosis. N. Engl. J. Med. 365 (14), 1293–1303. doi:10.1056/NEJMoa1014656
Olberg, H. K., Cox, R. J., Nostbakken, J. K., Aarseth, J. H., Vedeler, C. A., and Myhr, K. M. (2014). Immunotherapies influence the influenza vaccination response in multiple sclerosis patients: an explorative study. Mult. Scler. 20 (8), 1074–1080. doi:10.1177/1352458513513970
Olberg, H. K., Eide, G. E., Cox, R. J., Jul-Larsen, Å., Lartey, S. L., Vedeler, C. A., et al. (2018). Antibody response to seasonal influenza vaccination in patients with multiple sclerosis receiving immunomodulatory therapy. Eur. J. neurology 25 (3), 527–534. doi:10.1111/ene.13537
Olsson, J., Wikby, A., Johansson, B., Löfgren, S., Nilsson, B. O., and Ferguson, F. G. (2000). Age-related change in peripheral blood T-lymphocyte subpopulations and cytomegalovirus infection in the very old: the Swedish longitudinal OCTO immune study. Mech. ageing Dev. 121 (1-3), 187–201. doi:10.1016/s0047-6374(00)00210-4
Perdaens, O., and van Pesch, V. (2021). Molecular Mechanisms of Immunosenescene and Inflammaging: relevance to the Immunopathogenesis and Treatment of Multiple Sclerosis. Front. Neurol. 12, 811518. doi:10.3389/fneur.2021.811518
Pereira, B. I., De Maeyer, R. P. H., Covre, L. P., Nehar-Belaid, D., Lanna, A., Ward, S., et al. (2020). Sestrins induce natural killer function in senescent-like CD8(+) T cells. Nat. Immunol. 21 (6), 684–694. doi:10.1038/s41590-020-0643-3
Pfister, G., Weiskopf, D., Lazuardi, L., Kovaiou, R. D., Cioca, D. P., Keller, M., et al. (2006). Naive T cells in the elderly: are they still there? Ann. N. Y. Acad. Sci. 1067, 152–157. doi:10.1196/annals.1354.018
Pitzalis, M., Idda, M. L., Lodde, V., Loizedda, A., Lobina, M., Zoledziewska, M., et al. (2021). Effect of Different Disease-Modifying Therapies on Humoral Response to BNT162b2 Vaccine in Sardinian Multiple Sclerosis Patients. Front. Immunol. 12, 781843. doi:10.3389/fimmu.2021.781843
Polman, C. H., O'Connor, P. W., Havrdova, E., Hutchinson, M., Kappos, L., Miller, D. H., et al. (2006). A randomized, placebo-controlled trial of natalizumab for relapsing multiple sclerosis. N. Engl. J. Med. 354 (9), 899–910. doi:10.1056/NEJMoa044397
Porchet, R., Probst, A., Bouras, C., Draberova, E., Draber, P., and Riederer, B. M. (2003). Analysis of glial acidic fibrillary protein in the human entorhinal cortex during aging and in Alzheimer's disease. Proteomics 3 (8), 1476–1485. doi:10.1002/pmic.200300456
Prosperini, L., de Rossi, N., Scarpazza, C., Moiola, L., Cosottini, M., Gerevini, S., et al. (2016). Natalizumab-related progressive multifocal leukoencephalopathy in multiple sclerosis: findings from an italian independent registry. PLoS One 11 (12), e0168376. doi:10.1371/journal.pone.0168376
Pul, R., Moharregh-Khiabani, D., Škuljec, J., Skripuletz, T., Garde, N., Voss, E. V., et al. (2011). Glatiramer acetate modulates TNF-α and IL-10 secretion in microglia and promotes their phagocytic activity. J. neuroimmune Pharmacol. official J. Soc. NeuroImmune Pharmacol. 6 (3), 381–388. doi:10.1007/s11481-010-9248-1
Qi, Q., Liu, Y., Cheng, Y., Glanville, J., Zhang, D., Lee, J. Y., et al. (2014). Diversity and clonal selection in the human T-cell repertoire. Proc. Natl. Acad. Sci. U. S. A. 111 (36), 13139–13144. doi:10.1073/pnas.1409155111
Ragonese, P., Aridon, P., Vazzoler, G., Mazzola, M. A., Lo Re, V., Lo Re, M., et al. (2017). Association between multiple sclerosis, cancer risk, and immunosuppressant treatment: a cohort study. BMC Neurol. 17 (1), 155. doi:10.1186/s12883-017-0932-0
Ramos-Casals, M., García-Carrasco, M., Brito, M. P., López-Soto, A., and Font, J. (2003). Autoimmunity and geriatrics: clinical significance of autoimmune manifestations in the elderly. Lupus 12 (5), 341–355. doi:10.1191/0961203303lu383ed
Ratts, R. B., and Weng, N. P. (2012). Homeostasis of lymphocytes and monocytes in frequent blood donors. Front. Immunol. 3, 271. doi:10.3389/fimmu.2012.00271
Rist, J. M., and Franklin, R. J. (2008). Taking ageing into account in remyelination-based therapies for multiple sclerosis. J. Neurol. Sci. 274 (1-2), 64–67. doi:10.1016/j.jns.2008.04.027
Rossi, D. J., Bryder, D., Zahn, J. M., Ahlenius, H., Sonu, R., Wagers, A. J., et al. (2005). Cell intrinsic alterations underlie hematopoietic stem cell aging. Proc. Natl. Acad. Sci. U. S. A. 102 (26), 9194–9199. doi:10.1073/pnas.0503280102
Rubtsov, A. V., Rubtsova, K., Fischer, A., Meehan, R. T., Gillis, J. Z., Kappler, J. W., et al. (2011). Toll-like receptor 7 (TLR7)-driven accumulation of a novel CD11c⁺ B-cell population is important for the development of autoimmunity. Blood 118 (5), 1305–1315. doi:10.1182/blood-2011-01-331462
Salter, A., Fox, R. J., Newsome, S. D., Halper, J., Li, D. K. B., Kanellis, P., et al. (2021). Outcomes and Risk Factors Associated With SARS-CoV-2 Infection in a North American Registry of Patients With Multiple Sclerosis. JAMA Neurol. 78 (6), 699–708. doi:10.1001/jamaneurol.2021.0688
Salzer, J., Svenningsson, R., Alping, P., Novakova, L., Bjorck, A., Fink, K., et al. (2016). Rituximab in multiple sclerosis: A retrospective observational study on safety and efficacy. Neurology 87 (20), 2074–2081. doi:10.1212/WNL.0000000000003331
Schweitzer, F., Laurent, S., Fink, G. R., Barnett, M. H., Reddel, S., Hartung, H. P., et al. (2019). Age and the risks of high-efficacy disease modifying drugs in multiple sclerosis. Curr. Opin. neurology 32 (3), 305–312. doi:10.1097/WCO.0000000000000701
Seidler, S., Zimmermann, H. W., Bartneck, M., Trautwein, C., and Tacke, F. (2010). Age-dependent alterations of monocyte subsets and monocyte-related chemokine pathways in healthy adults. BMC Immunol. 11, 30. doi:10.1186/1471-2172-11-30
Shearer, G. M. (1997). Th1/Th2 changes in aging. Mech. ageing Dev. 94 (1-3), 1–5. doi:10.1016/s0047-6374(96)01849-0
Simpson-Yap, S., De Brouwer, E., Kalincik, T., Rijke, N., Hillert, J. A., Walton, C., et al. (2021). Associations of Disease-Modifying Therapies With COVID-19 Severity in Multiple Sclerosis. Neurology 97 (19), e1870–e1885. doi:10.1212/WNL.0000000000012753
Sloane, J. A., Hollander, W., Rosene, D. L., Moss, M. B., Kemper, T., and Abraham, C. R. (2000). Astrocytic hypertrophy and altered GFAP degradation with age in subcortical white matter of the rhesus monkey. Brain Res. 862 (1-2), 1–10. doi:10.1016/s0006-8993(00)02059-x
Tabibian-Keissar, H., Hazanov, L., Schiby, G., Rosenthal, N., Rakovsky, A., Michaeli, M., et al. (2016). Aging affects B-cell antigen receptor repertoire diversity in primary and secondary lymphoid tissues. Eur. J. Immunol. 46 (2), 480–492. doi:10.1002/eji.201545586
Teo, S. P. (2021). Review of COVID-19 Vaccines and Their Evidence in Older Adults. Ann. Geriatr. Med. Res. 25 (1), 4–9. doi:10.4235/agmr.21.0011
Theodoropoulou, E., Alfredsson, L., Piehl, F., Marabita, F., and Jagodic, M. (2019). Different epigenetic clocks reflect distinct pathophysiological features of multiple sclerosis. Epigenomics 11 (12), 1429–1439. doi:10.2217/epi-2019-0102
Tremlett, H., Zhao, Y., Joseph, J., and Devonshire, V.UBCMS Clinic Neurologists (2008). Relapses in multiple sclerosis are age- and time-dependent. J. Neurol. Neurosurg. Psychiatry 79 (12), 1368–1374. doi:10.1136/jnnp.2008.145805
Tutuncu, M., Tang, J., Zeid, N. A., Kale, N., Crusan, D. J., Atkinson, E. J., et al. (2013). Onset of progressive phase is an age-dependent clinical milestone in multiple sclerosis. Mult. Scler. 19 (2), 188–198. doi:10.1177/1352458512451510
Ufer, M., Shakeri-Nejad, K., Gardin, A., Su, Z., Paule, I., Marbury, T. C., et al. (2017). Impact of siponimod on vaccination response in a randomized, placebo-controlled study. Neurology(R) Neuroimmunol. neuroinflammation 4 (6), e398–e. doi:10.1212/NXI.0000000000000398
Vaiserman, A., and Krasnienkov, D. (2020). Telomere Length as a Marker of Biological Age: state-of-the-Art, Open Issues, and Future Perspectives. Front. Genet. 11, 630186. doi:10.3389/fgene.2020.630186
Vallejo, A. N. (2005). CD28 extinction in human T cells: altered functions and the program of T-cell senescence. Immunol. Rev. 205, 158–169. doi:10.1111/j.0105-2896.2005.00256.x
Vermersch, P., Czlonkowska, A., Grimaldi, L. M., Confavreux, C., Comi, G., Kappos, L., et al. (2014). Teriflunomide versus subcutaneous interferon beta-1a in patients with relapsing multiple sclerosis: a randomised, controlled phase 3 trial. Mult. Scler. 20 (6), 705–716. doi:10.1177/1352458513507821
Vollmer, B. L., Wolf, A. B., Sillau, S., Corboy, J. R., and Alvarez, E. (2021). Evolution of Disease Modifying Therapy Benefits and Risks: an Argument for De-escalation as a Treatment Paradigm for Patients With Multiple Sclerosis. Front. Neurol. 12, 799138. doi:10.3389/fneur.2021.799138
von Hehn, C., Howard, J., Liu, S., Meka, V., Pultz, J., Mehta, D., et al. (2018). Immune response to vaccines is maintained in patients treated with dimethyl fumarate. Neurol. Neuroimmunol. Neuroinflamm 5 (1), e409. doi:10.1212/NXI.0000000000000409
von Wyl, V., Décard, B. F., Benkert, P., Lorscheider, J., Hänni, P., Lienert, C., et al. (2020). Influence of age at disease onset on future relapses and disability progression in patients with multiple sclerosis on immunomodulatory treatment. Eur. J. Neurol. 27 (6), 1066–1075. doi:10.1111/ene.14191
Waldhauer, I., and Steinle, A. (2008). NK cells and cancer immunosurveillance. Oncogene 27 (45), 5932–5943. doi:10.1038/onc.2008.267
Wang, X., Malawista, A., Qian, F., Ramsey, C., Allore, H. G., and Montgomery, R. R. (2018). Age-related changes in expression and signaling of TAM receptor inflammatory regulators in monocytes. Oncotarget 9 (11), 9572–9580. doi:10.18632/oncotarget.23851
Ward, R. J., Zucca, F. A., Duyn, J. H., Crichton, R. R., and Zecca, L. (2014). The role of iron in brain ageing and neurodegenerative disorders. Lancet Neurology 13 (10), 1045–1060. doi:10.1016/S1474-4422(14)70117-6
Warnke, C., Olsson, T., and Hartung, H. P. (2015). PML: the dark side of immunotherapy in multiple sclerosis. Trends Pharmacol. Sci. 36 (12), 799–801. doi:10.1016/j.tips.2015.09.006
Weideman, A. M., Tapia-Maltos, M. A., Johnson, K., Greenwood, M., and Bielekova, B. (2017). Meta-analysis of the age-dependent efficacy of multiple sclerosis treatments. Front. Neurol. 8, 577. doi:10.3389/fneur.2017.00577
Wesselingh, R., Butzkueven, H., Buzzard, K., Tarlinton, D., O'Brien, T. J., and Monif, M. (2019). Innate Immunity in the Central Nervous System: A Missing Piece of the Autoimmune Encephalitis Puzzle? Front. Immunol. 10, 2066. doi:10.3389/fimmu.2019.02066
Weyand, C. M., Brandes, J. C., Schmidt, D., Fulbright, J. W., and Goronzy, J. J. (1998). Functional properties of CD4+ CD28- T cells in the aging immune system. Mech. ageing Dev. 102 (2-3), 131–147. doi:10.1016/s0047-6374(97)00161-9
Wu, Q., Mills, E. A., Wang, Q., Dowling, C. A., Fisher, C., Kirch, B., et al. (2020). Siponimod enriches regulatory T and B lymphocytes in secondary progressive multiple sclerosis. JCI Insight 5 (3), e134251. doi:10.1172/jci.insight.134251
Ye, J., Ma, C., Hsueh, E. C., Eickhoff, C. S., Zhang, Y., Varvares, M. A., et al. (2013). Tumor-derived γδ regulatory T cells suppress innate and adaptive immunity through the induction of immunosenescence. J. Immunol. 190 (5), 2403–2414. doi:10.4049/jimmunol.1202369
Zeydan, B., and Kantarci, O. H. (2020). Impact of age on multiple sclerosis disease activity and progression. Curr. neurology Neurosci. Rep. 20 (7), 24. doi:10.1007/s11910-020-01046-2
Zhang, X., Wang, R., Chen, H., Jin, C., Jin, Z., Lu, J., et al. (2022). Aged microglia promote peripheral T cell infiltration by reprogramming the microenvironment of neurogenic niches. Immun. Ageing 19 (1), 34. doi:10.1186/s12979-022-00289-6
Zhu, L., Stein, L. R., Kim, D., Ho, K., Yu, G. Q., Zhan, L., et al. (2018). Klotho controls the brain-immune system interface in the choroid plexus. Proc. Natl. Acad. Sci. U. S. A. 115 (48), E11388–E11396. doi:10.1073/pnas.1808609115
Zinger, N., Ponath, G., Sweeney, E., Nguyen, T. D., Lo, C. H., Diaz, I., et al. (2022). Dimethyl Fumarate Reduces Inflammation in Chronic Active Multiple Sclerosis Lesions. Neurol. Neuroimmunol. Neuroinflamm 9 (2), e1138. doi:10.1212/NXI.0000000000001138
Keywords: multiple sclerosis, immunosenescence, inflammaging, neuroinflammation, neurodegeneration
Citation: Thakolwiboon S, Mills EA, Yang J, Doty J, Belkin MI, Cho T, Schultz C and Mao-Draayer Y (2023) Immunosenescence and multiple sclerosis: inflammaging for prognosis and therapeutic consideration. Front. Aging 4:1234572. doi: 10.3389/fragi.2023.1234572
Received: 04 June 2023; Accepted: 29 September 2023;
Published: 13 October 2023.
Edited by:
Gordon D. Waiter, University of Aberdeen, United KingdomReviewed by:
Moisés Evandro Bauer, Pontifical Catholic University of Rio Grande do Sul, BrazilCopyright © 2023 Thakolwiboon, Mills, Yang, Doty, Belkin, Cho, Schultz and Mao-Draayer. This is an open-access article distributed under the terms of the Creative Commons Attribution License (CC BY). The use, distribution or reproduction in other forums is permitted, provided the original author(s) and the copyright owner(s) are credited and that the original publication in this journal is cited, in accordance with accepted academic practice. No use, distribution or reproduction is permitted which does not comply with these terms.
*Correspondence: Yang Mao-Draayer, eWFuZ21hb2RyYWF5ZXJAZ21haWwuY29t