- 1Pharmacology and Toxicology Department, Faculty of Pharmacy, Badr University in Cairo (BUC), Cairo, Egypt
- 2Faculty of Pharmacy, Badr University in Cairo (BUC), Cairo, Egypt
- 3Nanotechnology Research Center (NTRC), The British University in Egypt (BUE), El-Sherouk City, Egypt
- 4Pharmacology and Toxicology Department, Faculty of Pharmacy, Misr International University, Cairo, Egypt
The pathophysiology of different neurodegenerative illnesses is significantly influenced by the polarization regulation of microglia and macrophages. Traditional classifications of macrophage phenotypes include the pro-inflammatory M1 and the anti-inflammatory M2 phenotypes. Numerous studies demonstrated dynamic non-coding RNA modifications, which are catalyzed by microglia-induced neuroinflammation. Different nutraceuticals focus on the polarization of M1/M2 phenotypes of microglia and macrophages, offering a potent defense against neurodegeneration. Caeminaxin A, curcumin, aromatic-turmerone, myricetin, aurantiamide, 3,6′-disinapoylsucrose, and resveratrol reduced M1 microglial inflammatory markers while increased M2 indicators in Alzheimer’s disease. Amyloid beta-induced microglial M1 activation was suppressed by andrographolide, sulforaphane, triptolide, xanthoceraside, piperlongumine, and novel plant extracts which also prevented microglia-mediated necroptosis and apoptosis. Asarone, galangin, baicalein, and a-mangostin reduced oxidative stress and pro-inflammatory cytokines, such as interleukin (IL)-1, IL-6, and tumor necrosis factor-alpha in M1-activated microglia in Parkinson’s disease. Additionally, myrcene, icariin, and tenuigenin prevented the nod-like receptor family pyrin domain-containing 3 inflammasome and microglial neurotoxicity, while a-cyperone, citronellol, nobiletin, and taurine prevented NADPH oxidase 2 and nuclear factor kappa B activation. Furthermore, other nutraceuticals like plantamajoside, swertiamarin, urolithin A, kurarinone, Daphne genkwa flower, and Boswellia serrata extracts showed promising neuroprotection in treating Parkinson’s disease. In Huntington’s disease, elderberry, curcumin, iresine celosia, Schisandra chinensis, gintonin, and pomiferin showed promising results against microglial activation and improved patient symptoms. Meanwhile, linolenic acid, resveratrol, Huperzia serrata, icariin, and baicalein protected against activated macrophages and microglia in experimental autoimmune encephalomyelitis and multiple sclerosis. Additionally, emodin, esters of gallic and rosmarinic acids, Agathisflavone, and sinomenine offered promising multiple sclerosis treatments. This review highlights the therapeutic potential of using nutraceuticals to treat neurodegenerative diseases involving microglial-related pathways.
1 Introduction
1.1 Microglia pathway
Microglia are specialized macrophages, that constitute the primary central nervous system (CNS) innate immune cells. They are the first glial cells that enter the CNS during prenatal development. They represent approximately 10%–15% of all CNS cells. Microglia control CNS homeostasis at rest by eliminating pathogens and cell residue through phagocytic activity. Resting microglia become activated and generate inflammatory mediators, thus providing neurons protection and defense against infections. In addition to supporting the CNS, they are linked to the development of many inflammatory and neurodegenerative disorders (Colonna and Butovsky, 2017; Saitgareeva et al., 2020). Depending on the activation, microglia are separated into two categories: M1 microglia, which stimulates inflammation and neurotoxicity, and M2 microglia, which stimulates anti-inflammatory and neuroprotective effects (Qin et al., 2023).
M1 cells act as the innate immune system’s first line of defense, frequently within the first few hours or days. They use a wide range of immunological receptors to detect harmful stimuli; for example, nucleotide-binding oligomerization domains (NODs), NOD-like receptors, toll-like receptors (TLRs), and multiple scavenger receptors. They are activated by interferon-gamma (IFN-γ) and lipopolysaccharide (LPS). After activation, microglial cells are motivated to release pro-inflammatory factors with neurotoxic effects. Furthermore, IFN-γ stimulates the transcription factor signal transducer and activator of transcription 1 (STAT1) via Janus kinase (JAK)1/JAK2 signaling and stimulates the production of reactive oxygen species (ROS) and nitric oxide (NO) in addition to pro-inflammatory chemotactic factors and cytokines, like tumor necrosis factor-alpha (TNF-α), interleukin-23 (IL-23), IL-2, IL-1β, C-X-C motif chemokine ligand-9 (CXCL9), and CXCL10 (Orihuela et al., 2016). Activation of M1 can also be induced by another pathway through the activation of TLR4 by LPS or damage-associated molecular pattern (DAMP). After that, an “activation complex” is formed, comprising P65, P38, myeloid differentiation factor 88 (Myd88), interferon regulatory factor 3 (IRF3), and nuclear factor kappa B (NFκB), which is a highly conserved transcription factor that controls a variety of crucial physiological processes, including inflammatory reactions, cellular proliferation, and apoptosis. In turn, the complex formed controls the expression of inflammatory mediators from the polarized cell, such as inducible nitric oxide synthase (iNOS), CD16, and CD32, and the major histocompatibility complex-II, CD86, and other cell surface markers (Zhao et al., 2017). On the other hand, M2 microglia are activated by IL-4, IL-10, or IL-13, which motivate microglial cells to release abrineurin, found in inflammatory zone 1, Ym1, and anti-inflammatory cytokines, such as transforming growth factor ß (TGF-β), IL-1, and IL-4, which suppress inflammatory responses, encourage repair and regeneration and have neuroprotective effects (Yao and Zu, 2020; Li et al., 2021; Guo et al., 2022). The impact of microglia polarization to M1 or M2 on neurodegeneration and the involved mediators is illustrated in Figure 1.
2 Role of non-coding RNAs in microglia (M1/M2) pathway
Non-coding RNAs (ncRNAs) are a diverse class of ncRNA transcripts that do not play any role in protein coding. Nevertheless, it has been proven that they are key factors in many biological processes, including disease progression (Sun and Chen, 2020). Regulatory ncRNAs can be further divided into small non-coding RNAs (sncRNAs), which contain transcripts with fewer than 200 nucleotides (nt), and long non-coding RNAs (lncRNAs), which contain transcripts with more than 200 nt. The three primary types of small ncRNAs are PIWI-interacting RNAs (piRNAs), small-interfering RNA (siRNA), and microRNAs (miRNAs). Certain ncRNAs have different lengths, such as circular RNAs (circRNAs), enhancer RNAs (eRNAs), and promoter-associated transcripts (PATs) may belong to two classes at the same time (Zhang et al., 2019).
ncRNAs play key roles at the post-transcriptional level in different pathways and diseases, including neurodegenerative disorders (Nhung Nguyen et al., 2022; Salama et al., 2022; Elazazy et al., 2023) Table 1. Numerous studies have been conducted on the dynamic ncRNA alterations caused by microglia-induced neuroinflammation (J. Huang et al., 2023; Li et al., 2020; 2022). In the context of Alzheimer’s disease (AD), miRNA-155 regulates synaptic homeostasis of microglia Aβ internalization and synaptic pruning. Deletion of the microglia-specific miRNA-155 resulted in early onset hyper-excitability, frequent spontaneous seizures, seizure-related mortality, and decreased amyloid-beta (Aβ) pathology. As miRNA-155 deletion changed how the microglia internalized synaptic material, this impacted how the microglia mediated the synaptic pruning (Aloi et al., 2023).
Metastatic-associated lung adenocarcinoma transcript 1 (MALAT1), also known as the nuclear-enriched abundant transcript 2, is a lncRNA that is a crucial factor in the pathogenesis of Parkinson’s disease (PD) (Abrishamdar et al., 2022). PD onset and prognosis were correlated with MALAT1-relevant single nucleotide polymorphisms (SNPs), and MALAT1 contributed to increasing the neuronal inflammation of the pathogenesis of PD (Yang, 2021). MALAT1 is overexpressed due to participation in activating inflammatory vesicles in microglia (Geng et al., 2023). In PD models, MALAT1 induces apoptosis of dopamine neurons via sponging miRNA-124 (Liu et al., 2017) and acts as a miR-23b-3p sponge that inhibits microglial autophagy and inflammatory responses to promote dopaminergic neuronal apoptosis. The potential mechanism is that the miR-23b-3p/α-synuclein molecular axis is regulated to promote dopaminergic neuronal cell apoptosis by affecting the endocytosis and intercellular communication of the a-synuclein (α-syn) nucleoprotein. As a result, microglia may exhibit impaired autophagy and inflammatory reactions. Consequently, this provides a new treatment target for PD (Geng et al., 2023). Due to its effects on dopaminergic neuron apoptosis, lncRNA MALAT1 may be a new treatment target in PD.
In the CNS, miRNA-124 is highly expressed and perfectly conserved (Wohl and Reh, 2016; Chen et al., 2023). It plays an important role in a variety of neurodegenerative diseases, as well as memory development. Huang et al. found that miRNA-124 polarization of microglia from M1 to M2 significantly reduced the neuroinflammation generated by d-galactose. This was also shown by the presence of iNOS, arginase-1 (Arg-1), and ionized calcium-binding adapter molecule 1 (Iba-1), which also upregulated the anti-inflammatory mediators IL-4 and IL-10 and downregulated the inflammatory mediators TNF-α and IL-1β (J. Huang et al., 2023). Another study focused on miRNA-124 discovered that human serum and cerebrospinal fluid (CSF) both showed significantly higher levels of circHIPK3 expression in PD compared to controls, but miRNA-124 expression was markedly decreased. In BV2 cells (a type of microglial cells), the overexpression of circHIPK3 enhanced the release of IL-6, IL-1β, and TNF-α. Following the expression of circHIPK3, the microglia markers CD11b and Iba-1 protein expressions and pyroptosis-related factors, nucleotide-binding domain, leucine-rich–containing family, pyrin domain–containing-3 (NLRP3), caspase-1, and apoptosis-associated speck-like protein containing a caspase recruitment domain (ASC) were elevated. When miRNA-124 was added, all these effects were reversed. This is due to circHIPK3 increasing neuroinflammation via sponging miRNA-124 and controlling the miRNA-124-mediated STAT3/NACHT, LRR, and PYD domains-containing protein 3 pathway (Zhang et al., 2022). Nutraceuticals may play an important role in the treatment of different diseases by targeting ncRNAs (El-Shehawy et al., 2023; Jiang et al., 2023; Zhang et al., 2023). However, no studies were found that demonstrate an effect of nutraceuticals on the microglia pathway acting via the ncRNAs pathways.
3 Neurodegenerative diseases involving microglia (M1/M2) pathway
Neurodegenerative disorders such as AD, PD, Huntington’s disease (HD), amyotrophic lateral sclerosis, and multiple sclerosis (MS) are distinguished by neurodegeneration in particular parts of the CNS and share very similar pathophysiological processes (Song and Suk, 2017). Microglia-induced neuroinflammation has become widely recognized as a dualistic phenomenon in the field of neurodegenerative disorders, comprising both negative and positive effects on neuronal functioning and the surrounding environment (Tang and Le, 2016).
In various neurodegenerative disorders, neuroinflammation caused by microglia, macrophages that are found in the brain, is a prevalent hallmark, and various inflammatory mediators generated by M1 microglia have a role in the development of neurodegeneration and myelin damage in these diseases. Nevertheless, M2 microglia activation is required for tissue maintenance and repair (Jha et al., 2016; Song and Suk, 2017). Multiple studies have confirmed that natural products can both prevent and treat neurodegenerative diseases by influencing the polarization of microglia towards M1/M2 phenotypes. These natural compounds can potentially inhibit or reduce the inflammatory toxicity of M1 microglia. Furthermore, they can help repair and regenerate damaged neurons, axons, or myelin by improving the release of neurotrophic factors or cytokines from M2 microglia (Jin et al., 2019).
3.1 Alzheimer’s disease
3.1.1 Role of microglia in Alzheimer’s disease
Alzheimer’s disease is the prevailing type of dementia, associated with damaged locomotor ability, thinking, judgment ability, increasing memory loss, and cognitive decline (Fu et al., 2016). It is distinguished by the abnormal presence of Aβ-containing plaques extracellularly and the creation of neurofibrillary tangles inside the cell composed of hyperphosphorylated tau protein (Prince et al., 2016; Baufeld et al., 2017).
Postmortem analysis revealed an overwhelming number of “plaques” and “tangles” as distinguishing signs of AD. These senile plaques are accumulations of fibrils and aggregations of Aβ located outside of cells caused by abnormal proteolytic degradation of the amyloid precursor protein (APP), which is enhanced by presenilin-1 (PS1) (Citron et al., 1992; Mattson, 2004). In vivo, amyloid plaques can attract and stimulate microglia cells (Meyer-Luehmann et al., 2008), while in vitro studies have demonstrated that Aβ peptides can stimulate primary microglia activation and promote NO generation. However, this microglia activation can result in the adoption of many phenotypes, which is further increased by the presence of amyloid fibrils and extracellular Aβ peptides (Walker et al., 2006; Maezawa et al., 2011).
Furthermore, neuroinflammation has an important role in the etiology of AD (Baufeld et al., 2017). In the CSF of mild memory impairment patients who developed AD, researchers discovered higher TNF-α (a pro-inflammatory cytokine) and lower TNF-β (anti-inflammatory cytokine) levels compared to controls who had not experienced AD (Tarkowski et al., 2003). The neuroinflammatory response is demonstrated by modifications to the structure of microglia and astrocytes in proximity to senile plaques (Glass et al., 2010). Both astrocytes and microglia interact with Aβ, and interruptions in their metabolism and functioning can result in Aβ depositions (Yan et al., 2013; Hickman et al., 2018). As a result, Aβ uses TLRs to stimulate astrocytes and microglia, promoting neurodegeneration by causing the production of neuroinflammatory mediators (Glass et al., 2010).
Microglia also can respond to potentially damaging stimuli such as misfolded Aβ proteins (Sarlus and Heneka, 2017). M2 microglia, which are mainly accountable for up-taking and eliminating insoluble fibrillar Aβ deposits, perform a protective function in the brain. Microglia can break down Aβ by producing enzymes such as insulin-degrading enzymes (Heneka, 2017), hence minimizing AD incidence (Hansen et al., 2018).
At the outset of Aβ pathology, the microglia that encircle the Aβ plaques are typical of the neuroprotective phenotype, identified as Ym1. Thus, Microglia perform an important role in reducing the accumulation of potentially neurotoxic Aβ aggregates while also protecting neurons from localized toxicity (Condello et al., 2015). However, an age-dependent rise of both the size and amount of Aβ plaques in AD may represent a reduction in microglial phagocytic abilities (Mawuenyega et al., 2010), and this neuroprotective phenotype eventually changes to the neurologically harmful pro-inflammatory one at the final stages of the disease (Tang and Le, 2016).
Pro-inflammatory cytokines reduce microglia phagocytic activity and additionally are likely to shift microglia into pro-inflammatory phenotypes. Consequently, pro-inflammatory microglia promote tau phosphorylation (Lee et al., 2010). Furthermore, Microglia release neurotoxic cytokines that directly harm neurons or activate neurotoxic astrocytes (Hansen et al., 2018). It was also revealed that synaptic loss and aberrant tau phosphorylation in AD are caused by the dysregulation of Wnt pathways and that Wnt signaling regulates microglial inflammation (Yang and Zhang, 2020).
The dynamic nature of microglial activation involves constant transitions between different phenotypes. In a study conducted by Fan et al., it was suggested that microglial activation in AD may exhibit two distinct peaks (Fan et al., 2017). The first peak, occurring in the preclinical stage, is characterized by an anti-inflammatory response. The second peak, observed in the clinical stage as the disease advances and Aβ clearance mechanisms fail, demonstrates a pro-inflammatory reaction. These findings align with microglia’s dual role in AD pathogenesis.
3.1.2 Nutraceuticals that influence microglial activation in Alzheimer’s disease
The impact of different natural products and nutraceuticals on AD through regulation of M1/M2 microglia polarization is presented in Table 2 and Figures 2, 3.
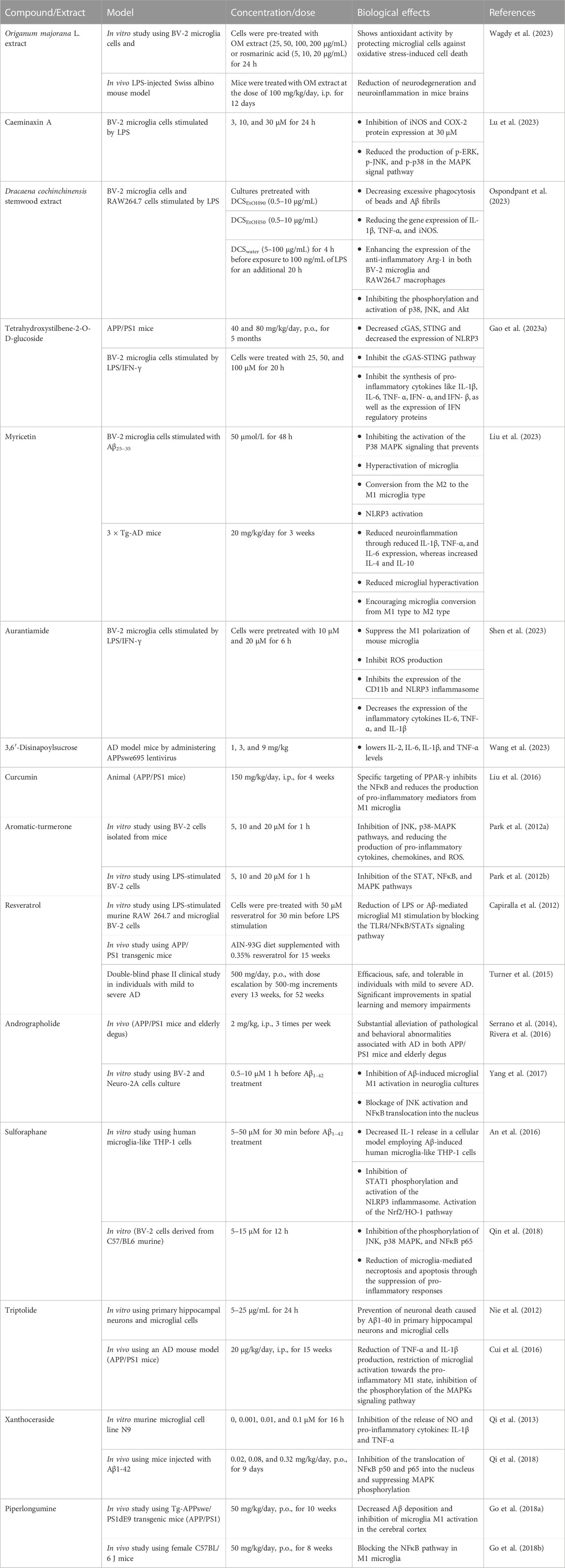
TABLE 2. The effects of different nutraceuticals on microglia in Alzheimer’s disease related models.
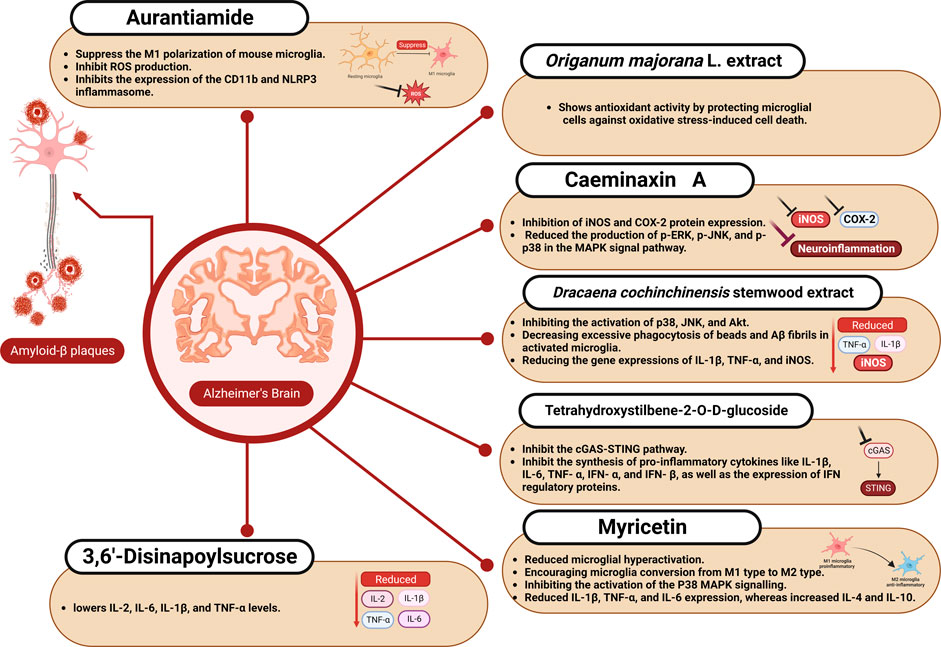
FIGURE 2. The effect of aurantiamide, myricetin, and other nutraceuticals on microglial activation in Alzheimer’s disease.
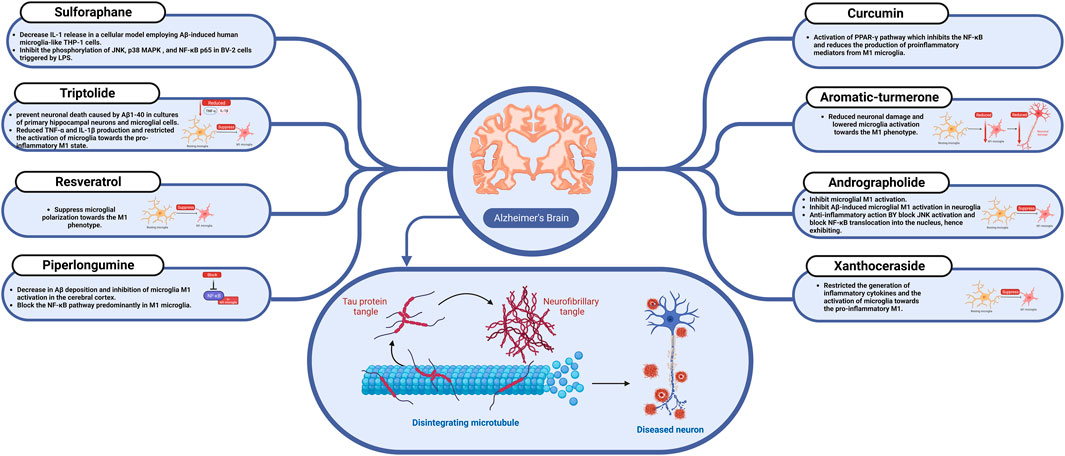
FIGURE 3. The effect of sulforaphane, resveratrol, curcumin, and other nutraceuticals on microglial activation in Alzheimer’s disease.
3.1.2.1 Origanum majorana L.
Origanum majorana L. is an aromatic plant used to treat various diseases in folk medicine, including intestinal antispasmodic, intestinal hypertension, allergies, respiratory infections, diabetes, and stomach pain (Bouyahya et al., 2021). Origanum majorana is a plant rich in phenolic compounds like rosmarinic acid and its derivatives. Origanum majorana extract and rosmarinic acid strongly protected microglial cells from oxidative stress-induced cell death by the antioxidant activity of rosmarinic acid. Origanum majorana additionally protected mice from the alterations in recognition and spatial memory induced by LPS and reduced expression of glial fibrillary acidic protein and cyclooxygenase-2 (COX-2) in mouse brain tissue (Wagdy et al., 2023).
3.1.2.2 Caesalpinia dinax
Caesalpinia minax Hance is a species of plant that belongs to the Fabaceae family and spreads throughout Southeast Asia’s tropical and subtropical regions. This plant’s seeds, known in China as “Ku-shi-lian,” have long been used in traditional medicine to treat fever, diarrhea, and the common cold (Jing et al., 2019; Ruan et al., 2019). Twenty cassane diterpenoids were identified in the leaves of Caesalpinia minax, including two unique ones (caeminaxins A and B). To evaluate the anti-neuritis activity of these 20 compounds, Lu et al. measured the amount of NO production of mouse microglia BV-2 cells stimulated by LPS as neuro-inflammatory diseases are characterized by an increase in NO production. The most potent inhibitory effect was produced by caeminaxin A. Different concentrations of this compound (3, 10, and 30 μM) were used to treat BV-2 cells stimulated by LPS. Caeminaxin A at 30 μM significantly inhibited iNOS and COX-2 protein expression. Additionally, Caeminaxin A reduced the production of p-ERK, p-JNK, and p-p38 in the mitogen-activated protein kinase (MAPK) signal pathway, which suppressed neuroinflammation (Lu et al., 2023).
3.1.2.3 Dracaena cochinchinensis
Dracaena cochinchinensis is a tropical forest plant belonging to the family Asparagaceae (Wu et al., 2019). Dracaena cochinchinensis stemwood is used in traditional Chinese medicine to treat blood stasis, severe injuries, and pain (Fan et al., 2014; Ospondpant et al., 2022). In LPS-activated microglia, D. cochinchinensis stemwood extract reduced the neuroinflammatory response by reducing pro-inflammatory factors and inhibiting the activation of p38, JNK, and Akt and by reducing the gene expressions of IL-1β, TNF-α, and iNOS. Additionally, D. cochinchinensis stemwood extract could decrease excessive phagocytosis of beads and Aβ fibrils in activated microglia (Ospondpant et al., 2023). Dracaena cochinchinensis stemwood extract can also prevent the formation of Aβ fibrils, protecting against Aβ-mediated cell damage and stimulating neural differentiation (Ospondpant et al., 2022).
3.1.2.4 Polygonum multiflorum
Polygonum multiflorum is traditional Chinese herbal medicine used for centuries as a treatment for a wide range of conditions, including dizziness, liver disease, graying of the hair, and constipation (Xue et al., 2020; Guo et al., 2023). Chinese knotweed, Fo-Ti, Shou Wu Pian, and He Shou Pian are other names for P. multiflorum (Gumber and Barmota, 2023). The main active compound from Polygonum multiflorum is tetrahydroxystilbene-2-O-D-glucoside (TSG, C20H22O9) (Wang et al., 2022). TSG caused a decrease in cyclic GMP-AMP synthase (cGAS), a decrease in the immune response that was induced by the stimulator of interferon genes (STING), and decreased the expression of NLRP3 inflammasome by inhibition of the activation of the cGAS-STING pathway in APP/PS1 mice. Additionally, cell culture using LPS and IFN-γ to activate microglia indicated that TSG reversed the polarization status of M1-type microglia to restore quiescence and inhibit cGAS-STING pathway as active microglia showed higher cGAS-STING levels. TSG also reduced the inflammatory response induced by LPS/IFN-γ in BV2 cells by inhibiting the synthesis of pro-inflammatory cytokines such as IL-1β, IL-6, TNF- α, IFN- α, and IFN- β, as well as the expression of IFN regulatory proteins like IFIT1 and IRF7 (Gao et al., 2023a).
3.1.2.5 Myricetin
Myricetin is a flavonoid compound in various natural plants (Song et al., 2021). It can be found in foods, including fruits, vegetables, tea, and wine. The richest sources of myricetin are the families Myricaceae, Polygonaceae, Primulaceae, Pinaceae, and Anacardiaceae. Previous studies have demonstrated that myricetin has various pharmacological effects, including its anticancer, anti-diabetic, anti-obesity, cardiovascular, anti-osteoporosis, anti-inflammatory, and hepatoprotective (Imran et al., 2021). Myricetin has neuroprotective action, which has been demonstrated in preclinical studies on amyotrophic lateral sclerosis, PD, AD, and HD (Taheri et al., 2020). According to the findings of in vitro AD model (BV-2 microglia cells stimulated with Aβ25–35), myricetin prevented the hyperactivation of microglia and the conversion from the M2 to the M1 type and inhibited NLRP3 activation by inhibiting the activation of the P38 MAPK signaling pathway. In 3 × Tg-AD mice, myricetin reduced microglia hyperactivation, encouraged microglia conversion from M1 type to M2 type, and effectively reduced neuroinflammation through reduced IL-1β, TNF-α, and IL-6 expression, whereas increased IL-4 and IL-10. In addition, it could significantly enhance memory loss, spatial learning capacity, Aβ plaque formation, and neuronal and synaptic damage (Liu et al., 2023).
3.1.2.6 Aurantiamide
Aurantiamide is a natural product found in various plants, such as Portulaca oleracea L. and Zanthoxylum dissitum. It shows a variety of biological activities like anti-HIV, anti-inflammatory, antibacterial, and antioxidant effects (Suhas and Channe Gowda, 2012; Chen et al., 2016). Aurantiamide was found to decrease central neuroinflammation, inhibit ROS production, suppress the M1 polarization of mouse microglia, and enhance cognitive performance in mice. Additionally, Aurantiamide inhibits the expression of the CD11b and NLRP3 inflammasome and also decreases the expression of the inflammatory cytokines IL-6, TNF-α, and IL-1β (Shen et al., 2023).
3.1.2.7 3,6′-Disinapoylsucrose
3,6′-Disinapoylsucrose is an oligosaccharide ester bioactive compound obtained from Polygalae Radix that significantly reduces depression (Zhang et al., 2022). In AD model mice, 3,6′-Disinapoylsucrose can greatly reduce neuroinflammation, problems with spatial learning, and memory issues. 3,6′-Disinapoylsucrose enhances cognitive function, lowers IL-2, IL-6, IL-1β, and TNF-α levels, reduces NFκB p65 expression, and reduces Aβ deposition and nerve cell injury. 3,6′-Disinapoylsucrose can control the hippocampus’s tyrosine kinase B/brain-derived neurotrophic factor signaling (Wang et al., 2023).
3.1.2.8 Curcumin
Curcumin is an important polyphenolic component found in the rhizome of Curcuma longa (Zingiberaceae). It has a rich history of traditional use as a food and herbal medicine in Asia and has emerged as a highly promising phytochemical candidate for treating AD owing to its notable anti-inflammatory and immunomodulatory properties (Ruby et al., 1995). Administering curcumin to APP/PS1 mice at a dose of 150 mg/kg through intraperitoneal injection for 4 weeks significantly improved spatial learning and memory impairments. Its effects were notable for efficiently inhibiting the NFκB signaling pathway and lowering the production of pro-inflammatory mediators from M1 microglia. It specifically targeted peroxisome proliferator-activated receptor gamma (PPAR-γ) to obtain these positive results (Liu et al., 2016).
Several challenges arise from the pharmacokinetic properties of curcumin, including rapid degradation, low bioavailability, and limited solubility in body fluids. Various innovative drug delivery systems for curcumin have been developed to address these limitations. One such system is the solid-lipid curcumin particle, which has demonstrated remarkable enhancements in anti-amyloid, anti-inflammatory, and neuroprotective effects compared to natural curcumin (Maiti et al., 2018).
3.1.2.9 Aromatic-turmerone
Aromatic-turmerone, a prominent phytoconstituent found in Curcuma longa essential oil, has a comparable chemical structure and potential bioactivities to curcumin and 6-shogaol derived from ginger. In an in vitro study, the aromatic-turmerone was shown to decrease the synthesis of pro-inflammatory chemicals in BV-2 cells, derived from C57/BL6 murine, which is a well-characterized and extensively employed model system for microglia, triggered by LPS via inhibiting the STATs and mitogen-activated protein kinase pathways (Park et al., 2012b). Another in vitro study found that administrating aromatic-turmerone reduced neuronal damage and lowered microglia activation towards the M1 phenotype. These data support the anti-inflammatory and neuroprotective properties of aromatic-turmerone. These positive benefits were connected to avoiding neuronal injury by limiting microglial M1 activation and decreasing inflammatory cytokine production (Park et al., 2012a).
3.1.2.10 Resveratrol
Many different plants produce resveratrol, a natural phenol, and stilbenoid, as a defense mechanism against damage or pathogen attacks. It is plentiful in grape skin and blueberries and has significant anti-inflammatory benefits. Numerous studies have shown that it can suppress microglial polarization towards the M1 phenotype and improve cognitive deficits in cellular and animal models of dementia. In addition, Resveratrol reduced LPS or Aβ-mediated microglial M1 stimulation by blocking the TLR4/NFκB/STATs signaling pathway (Capiralla et al., 2012). Furthermore, resveratrol was shown to be efficacious, safe, and tolerable in individuals with mild to severe AD in a double-blind phase II clinical study (Turner et al., 2015).
3.1.2.11 Andrographolide
Andrographolide is a labdane diterpenoid present throughout the whole Andrographis paniculata plant. It has attracted interest due to its powerful anti-inflammatory characteristics and potential for treating AD. Andrographolide, taken intraperitoneally at a dose of 2.0 mg/kg, substantially alleviated pathological and behavioral abnormalities, which implies AD in both APP/PS1 mice and elderly degus (56-month-old) as demonstrated in the in vivo studies (Serrano et al., 2014; Rivera et al., 2016). Furthermore, in vitro investigations have demonstrated that andrographolide can inhibit microglial M1 activation. Andrographolide inhibited Aβ-induced microglial M1 activation in neuroglia cultures when administered at a dose of 5 μM. Andrographolide has been shown in mechanistic studies to block JNK activation and block NFκB translocation into the nucleus, hence exhibiting anti-inflammatory actions (Yang et al., 2017).
3.1.2.12 Sulforaphane
Sulforaphane, an organosulfur isothiocyanate substance, is mainly found in cruciferous vegetables such as broccoli, mustard radish, and cabbage. It is a powerful activator of the nuclear factor erythroid 2–related factor 2/heme oxygenase 1 (Nrf2/HO1) pathway with a wide range of biological and pharmacological actions, including anti-inflammatory and antioxidant properties (Zhang et al., 2017). An in vitro study found that sulforaphane at a dose of 5 μM decreased IL-1 release in a cellular model employing Aβ-induced human microglia-like THP-1 cells. This was accomplished via inhibiting STAT1 phosphorylation and activation of the NLRP3 inflammasome. The activation of the Nrf2/HO-1 pathway relates to sulforaphane’s latent mechanism (An et al., 2016). Furthermore, previous in vitro research found that sulforaphane at doses ranging from 5 to 15 μM inhibited the phosphorylation of JNK, p38 MAPK, and NFκB p65 in mentioned BV-2 cells, derived from C57/BL6 murine, triggered by LPS. Microglia-mediated necroptosis and apoptosis were reduced due to this indirect suppression of pro-inflammatory responses (Qin et al., 2018).
3.1.2.13 Triptolide
Triptolide, a diterpenoid molecule produced from the plant Tripterygium wilfordii, is well-known for its medicinal promise in the treatment of inflammatory and autoimmune conditions such as systemic lupus erythematosus, rheumatoid arthritis, and nephrotic syndrome. Additionally, multiple in vitro studies have shown that triptolide at 5 and 25 μg/mL doses successfully prevented neuronal death caused by Aβ1-40 in cultures of primary hippocampal neurons and microglial cells (Nie et al., 2012). Another in vivo study using an AD mouse model (APP/PS1 mice) discovered that triptolide (20 g/kg intraperitoneally for 15 weeks) effectively reduced TNF-α and IL-1β production and restricted the activation of microglia towards the pro-inflammatory M1 state. These results were obtained by inhibiting the phosphorylation of the MAPK signaling pathway (Cui et al., 2016).
3.1.2.14 Xanthoceraside
Xanthoceraside is a novel triterpenoid saponin isolated from the husk of Xanthoceras sorbifolia that has traditionally been used as an anti-rheumatic treatment (Zhou et al., 2022). Xanthoceraside has a remarkable promise in treating AD caused by inflammation via targeting the NFκB pathway. In a recent in vivo investigation, oral administration of xanthoceraside (0.08 or 0.32 mg/kg for 9 days) significantly restricted the generation of inflammatory cytokines and the activation of microglia towards the pro-inflammatory M1 state in the hippocampus of mice injected with Aβ1-42. Blocking the translocation of NFκB p50 and p65 into the nucleus and suppressing the phosphorylation of MAPK signaling pathways is considered the main xanthoceraside inhibitory mechanism (Qi et al., 2018; 2013).
3.1.2.15 Piperlongumine
Piperlongumine, an amide alkaloid produced from the fruit of the long pepper plant (Piper longum), which is native to southern India and Southeast Asia, has piqued the interest of pharmacologists due to its possible medicinal qualities (Kumar et al., 2011). Numerous studies have been undertaken employing various animal models to evaluate the effects of piperlongumine on AD-like pathology. Piperlongumine (50 mg/kg/day, i. g, for 2.5 months) was given to APP/PS1 mice in an in vivo study, resulting in significant restoration of cognitive function (Go et al., 2018b). This improvement was attributable to a decrease in Aβ deposition and inhibition of microglia M1 activation in the cerebral cortex (Go et al., 2018a). Furthermore, previous studies have proven that piperlongumine has neuroprotective benefits in AD rat models via blocking the NFκB pathway predominantly in M1 microglia (Gu et al., 2018).
3.2 Parkinson’s disease
3.2.1 Role of M1/M2 in Parkinson’s disease
Just behind AD, PD ranks as the second most prevalent neurodegenerative disorder. It is distinguished by the slow degeneration of dopamine neurons in the midbrain’s substantia nigra pars compacta (SNpc) and A1 neurotoxic astrocyte activation. Lewy bodies (LBs), which are primarily composed of filamentous a-syn, are a distinguishing hallmark of PD. These protein aggregates are a fundamental illness feature and are important in its pathogenesis. Additionally, there is an excessive proliferation of reactive microglia, a type of immune cell in the brain (Eriksen et al., 2005; Tang et al., 2013; Song and Suk, 2017). Through functional alterations, astrocytes play key roles in PD pathogenesis (González-Reyes et al., 2017). Dopamine regulates Ca2+ signals in astrocytes (Vaarmann et al., 2010). Dopamine neuron loss may alter astrocyte Ca2+ homeostasis, and Ca2+ imbalance may result in the generation of toxic compounds and cell death in PD (Zaichick et al., 2017). Furthermore, emerging data from multiple studies suggest that glia maturation factor (GMF) produced from astrocytes stimulates the NFκB signaling pathway and consequent granulocyte macrophage-colony stimulating factor (GM-CSF) release. Increased levels of GM-CSF have been implicated in microglia activation and subsequent generation of inflammatory molecules such as IL-1β, TNF-α, and macrophage inflammatory proteins-1 beta (MIP-1β) (Zaheer et al., 2007; Fan et al., 2018).
The presence of a-syn in dopaminergic cells has been associated with increased amounts of ROS. This implies that a-syn may contribute to oxidative damage by influencing mitochondrial activity. Overexpression of mutant a-syn has been demonstrated to make dopaminergic neurons more susceptible to mitochondrial toxins such as 6-hydroxydopamine (6-OHDA) and mitochondrial processing peptidases 1-methyl-4-phenylpyridinium ion (MPP+), leading to elevated protein carbonylation and lipid peroxidation. These results demonstrate that a-syn may regulate oxidative stress and mitochondrial dysfunction in dopaminergic cells (Chinta and Andersen, 2008).
Microglia-mediated neuroinflammation has a complicated function in PD since it can have both neuroprotective and neurotoxic effects. Microglia are triggered in the early stages of PD by factors such as a-syn, infections, or environmental pollutants. At this stage, microglia are generally static and have little relationship with the severity of clinical symptoms (Tang and Le, 2016). During this initial stage, microglia secrete anti-inflammatory cytokines to decrease the inflammatory response and promote tissue healing and repair (Du et al., 2018). This activation is critical for immunological defense and survival of neurons (Tang et al., 2013). However, when PD advances, persistent microglial activation becomes harmful. Prolonged stimulation can exacerbate motor impairments and cause extensive neuronal damage in neighboring areas (Song and Suk, 2017). Moreover, aggregated a-syn can directly motivate microglia to adopt a pro-inflammatory M1 phenotype. This aggravates motor impairments and severely damages adjacent neurons. Understanding the dynamic nature of microglial activation and its role in the etiology of PD is critical for developing treatment techniques to control neuroinflammation and offer neuroprotection (Tang and Le, 2016; Song and Suk, 2017; Du et al., 2018).
3.2.2 Nutraceuticals that influence microglial activation in Parkinson’s disease
The impact of different natural products and nutraceuticals on PD through regulation of M1/M2 microglia polarization is presented in Table 3 and Figure 4.
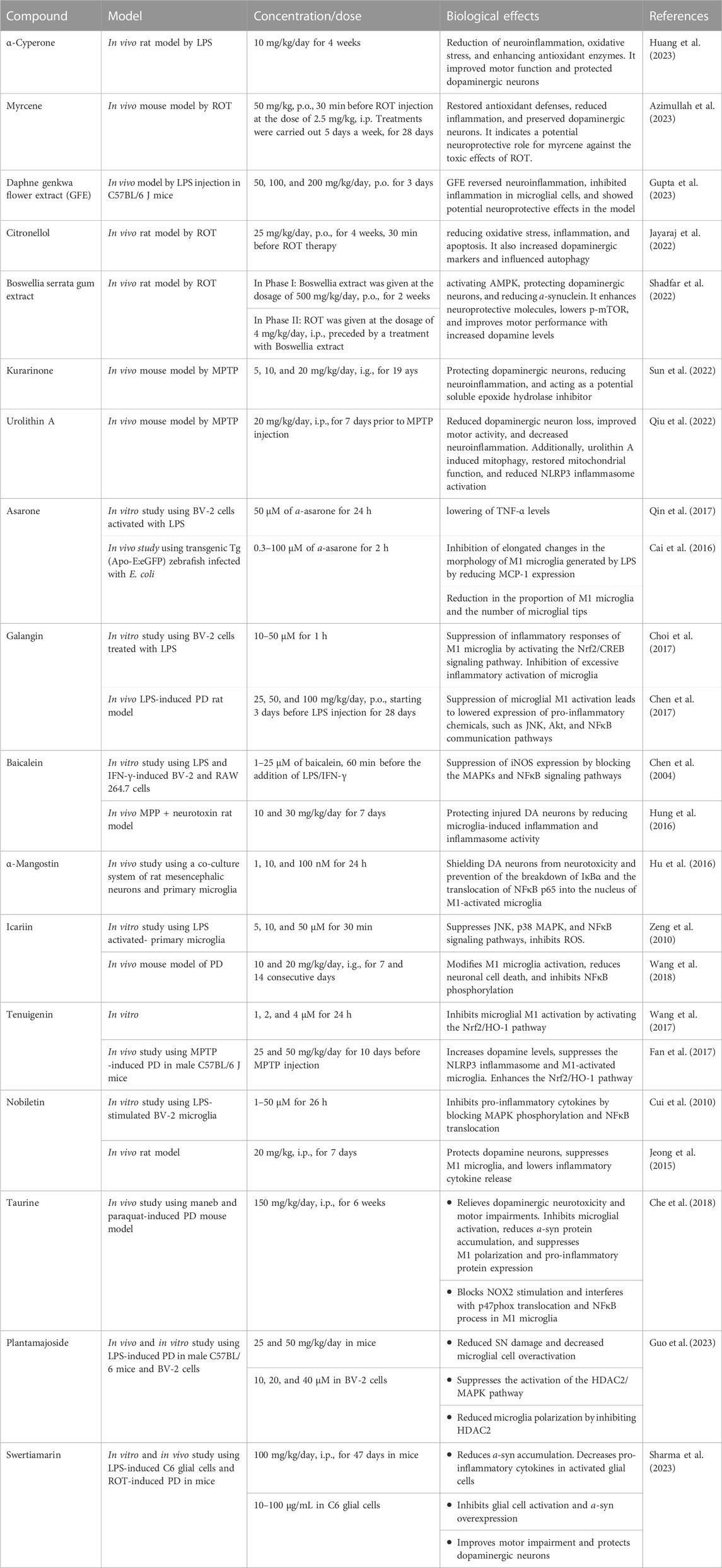
TABLE 3. The effects of different nutraceuticals on microglia in Parkinson’s disease related models.
3.2.2.1 a-Cyperone
α-Cyperone, a major active compound of Cyperus rotundus L., which is often known as purple nutsedge or nut grass, is a common and widespread weed in tropical, subtropical, and temperate climates (Azimi et al., 2016). This plant has received a lot of attention for its medicinal potential, thanks to centuries of traditional medicine (Seo et al., 2001). It has been praised for its anti-arthritic, antidiarrheal, and antiplatelet characteristics, as well as its potential to treat numerous CNS illnesses such as epilepsy, depression, and inflammatory conditions (Jebasingh et al., 2012; Azimi et al., 2016). In a conducted investigation, researchers discovered that a-Cyperone at a dose of 10 mg/kg per day significantly improved motor function impairment, protected dopaminergic neurons, and counteracted the decrease of dopamine and its metabolites in a rat model of PD caused by lipopolysaccharide (LPS). Furthermore, a-Cyperone substantially reduced microglia activation and the production of many neuroinflammatory factors, including IL-6, IL-1β, TNF-α, iNOS, ROS, and COX-2 (B. Huang et al., 2023). The protective effects of a-Cyperone on microglia were explained by its ability to suppress neuroinflammation and oxidative stress, which was accomplished by activating the Nrf2/HO-1 pathway while simultaneously blocking the NFκB signaling pathway, according to meticulous molecular mechanism studies (B. Huang et al., 2023; Li et al., 2021). Furthermore, a-Cyperone aided in the overexpression of antioxidant enzymes such as glutamate-cysteine ligase catalysis, glutamate-cysteine ligase modifier, and nicotinamide quinone oxidoreductase 1 in microglia, adding to its neuroprotective characteristics (B. Huang et al., 2023; Jayaram and Krishnamurthy, 2021).
3.2.2.2 Myrcene
Myrcene, sometimes known as ß-myrcene, is an abundant monoterpene found in a variety of plant species, including hops and cannabis (Surendran et al., 2021). Notably, myrcene is widely used in the food and beverage industries as a flavor and aroma enhancer, and it is also used as a food additive throughout the production process (Tyler, 1996; Surendran et al., 2021). Myrcene at a dose of 50 mg/kg, provided 30 min before rotenone (ROT) injections, demonstrated neuroprotective benefits in a mouse model of Parkinson’s disease (PD). ROT exposure resulted in the death of dopaminergic neurons, a decrease in antioxidant defenses, a rise in lipid peroxidation, and the activation of microglia and astrocytes. Pro-inflammatory cytokine levels were also raised, and the autophagy lysosomal pathway was disrupted, both of which contributed to dopaminergic neurodegeneration. Myrcene therapy, on the other hand, significantly restored antioxidant defenses, reduced lipid peroxidation, and pro-inflammatory cytokines, and reduced microglial and astrocyte activation. It also increased mTOR phosphorylation, which helped to restore neuronal homeostasis and autophagy-lysosomal activity. Surprisingly, myrcene treatment reduced a-synuclein expression, resulting in dopaminergic neuron preservation and rescue (Azimullah et al., 2023).
3.2.2.3 Daphne genkwa flower
In East Asia, the flower buds of Daphne genkwa have been used as a traditional herbal medicine. Flavonoids derived from D. genkwa flower extract (GFE) have previously been investigated for their anti-inflammatory and antioxidant properties (Guo et al., 2020). Daphne GFE has also been shown to have anti-inflammatory properties as well as the ability to slow disease development in a PD animal model (Jiang et al., 2014; Guo et al., 2020). Researchers used neuroinflammation in vivo animal models produced by LPS to investigate the impact of Daphne GFE. C57BL/6 J mice aged eight to 9 weeks were randomly assigned to one of three groups: vehicle, LPS, or LPS + GFE. GFE was given at three different dosages (50, 100, and 200 mg/kg) orally over 3 days. LPS (4 mg/kg) intraperitoneal injection induced neuroinflammation 1 hour after the initial oral therapy. GFE inhibited the production of inflammatory factors (NO, iNOS, and TNF-α) in microglial and primary glial cells, resulting in significant anti-inflammatory and neuroprotective effects. It also stimulated phagocytosis in microglia and boosted the expression of neuroprotective markers (Arg-1 and brain-derived neurotrophic factor mRNA) in primary glial cells, emphasizing its potential as a PD treatment (Gupta et al., 2023).
3.2.2.4 Citronellol
Citronellol (CT) is a monoterpene alcohol found in the essential oils of various plants that are used in cooking and traditional medicine (Santos et al., 2019). ROT was given once daily at a dose of 2.5 mg/kg for 4 weeks in a PD rat model. Concurrently, CT was administered orally once daily for 4 weeks at a dose of 25 mg/kg, 30 min before ROT therapy. CT had neuroprotective properties, suppressing ROS and lipid peroxidation while boosting antioxidant enzymes and decreasing brain inflammation. It also inhibited microglia and astrocyte activation, resulting in lower COX-2 and iNOS-2 expression. Furthermore, CT preserved and increased the levels of tyrosine hydroxylase in the substantia nigra pars compacta and striatum. Furthermore, it reduced apoptosis by decreasing Bax and a-synuclein levels while raising Bcl-2 and mTOR (Jayaraj et al., 2022).
3.2.2.5 Methanolic extract of Boswellia serrata gum
For centuries, the resin of Boswellia species, namely, Boswellia serrata (Salai/Salai guggul), has been employed in religious, cultural, and medical traditions (Salama et al., 2023). The oleo gum resin is extracted from the tree’s trunk and comprises resin, essential oils, and polysaccharides. It is derived from many states in India and has historically been used in folk medicine to treat chronic inflammatory illnesses (Siddiqui, 2011). The researchers examined the neuroprotective properties of Boswellia serrata gum extract in PD in previous research. The extract activated AMPK and downstream neuroprotection pathways. In vivo, it protected nigrostriatal dopaminergic neurons while decreasing a-synuclein accumulation (Ameen et al., 2017).
During Phase I, to investigate whether Boswellia extract increases neuroprotective molecules, oral Boswellia extract (500 mg/kg/day) was given for 2 weeks, and Western blotting was used to identify neuroprotective compounds. In Phase II, to explore the neuroprotective effects of Boswellia extract on ROT neurotoxicity, four groups were studied: one as a control, one receiving oral Boswellia extract group, one receiving ROT (4 mg/kg/day, i. p.), and one receiving both Boswellia extract and ROT. Boswellia enhanced AMPK phosphorylation, decreased p-mTOR and p-α-synuclein in the striatum, and raised the expression of Beclin1 and brain-derived neurotrophic factor. Boswellia reduced dopaminergic neuron loss, microglial activation, and a-synuclein accumulation in ROT-injected rats, increasing striatal dopamine levels and motor performance (Shadfar et al., 2022).
3.2.2.6 Kurarinone
Kurarinone is a naturally occurring prenylated flavanone isolated from Sophora flavescens (Huang et al., 2020). Kurarinone demonstrated promising therapeutic results in a PD mice model. It alleviated behavioral impairments and rescued dopaminergic neurons from 1-methyl-4-phenyl-1,2,3,6-tetrahydropyridine (MPTP)-induced neurotoxicity at dosages of 5, 10, and 20 mg/kg. In the substantia nigra and striatum, kurarinone retained neurotransmitters and tyrosine hydroxylase-positive cells. It also lowered neuroinflammation by blocking microglial activation via the NFκB signaling pathway. Kurarinone has been found to target soluble epoxide hydrolase, which is associated with neuroinflammation in PD (Sun et al., 2022).
3.2.2.7 Urolithin A
Urolithin A is synthesized by gut bacteria from ellagitannin-rich food (Singh et al., 2021). Urolithin A therapy showed neuroprotective effects in a mouse model of PD. In mice, Urolithin A effectively reduced dopaminergic neuron loss, corrected behavioral impairments, and reduced neuroinflammation caused by MPTP (Ren et al., 2018). Further research indicated that Urolithin A induced mitophagy in BV2 cells (a type of microglial cell derived from C57/BL6 murine) that were exposed to LPS, restored mitochondrial function, and decreased the pro-inflammatory response. Furthermore, urolithin A significantly reduced NLRP3 inflammasome activation. To create the MPTP mouse model, mice were given 15 mg/kg MPTP intraperitoneally four times a day within a 2-h interval. Mice were given 20 mg/kg urolithin A intraperitoneally for 7 days before MPTP injection for urolithin A therapy. The rotarod, pole, and suspension tests all revealed significant impairment in motor activity after MPTP treatment. However, Urolithin A treatment dramatically corrected these motor abnormalities, suggesting the potential therapeutic advantages of Urolithin A in reducing motor impairments associated with PD (Qiu et al., 2022).
3.2.2.8 Asarone
Asarone represents a phenylpropanoid found in plants such as Acorus and Asarum. It comes in two isomeric forms, a and ß. Because of its capacity to pass the BBB, a-asarone has significant neuroprotective effects. A dosage of 50 μM of a-asarone dramatically lowered TNF-α levels in tests using BV-2 cells activated with LPS (Qin et al., 2017). Interestingly, a lower quantity of a-asarone (3 μM) did not affect the generation of pro-inflammatory cytokines caused by LPS. However, it inhibited the elongated changes in the morphology of M1 microglia generated by LPS by lowering monocyte chemotactic protein-1 (MCP-1) expression. An in vivo study using transgenic Tg (Apo-E:eGFP) zebrafish infected with E. coli found that at the same dosage, a-asarone reduced the proportion of M1 microglia and the number of microglial tips. These findings emphasize a-asarone’s neuroprotective potential, specifically its potential to modulate inflammation and morphological alterations in microglia (Cai et al., 2016).
3.2.2.9 Galangin
Galangin, a natural flavonol, predominantly exists in the rhizome of the therapeutic plant Alpinia officinarum (Zingiberaceae). This molecule was widely investigated and has been shown to stimulate PPAR-γ, a receptor involved in cellular process regulation. Galangin suppressed inflammatory responses of M1 microglia in tests employing BV-2 cells treated with LPS. This was accomplished by activating the Nrf2/cAMP response element-binding protein (CREB) signaling pathway at doses ranging from 10 to 50 μM (Choi et al., 2017). Furthermore, galangin showed encouraging results in an in vivo experimental rat model mimicking PD by inhibiting excessive inflammatory activation of microglia. Galangin also dramatically suppressed microglial M1 activation produced by LPS, lowering pro-inflammatory chemical expression. These effects were linked to changes in the JNK, Akt, and NFκB communication pathways. These findings emphasize galangin’s possible therapeutic role in modulating microglial inflammation, implying its potential as a therapy method for neurodegenerative disorders such as PD (Chen et al., 2017).
3.2.2.10 Baicalein
Baicalein, which is derived from the root of Scutellaria baicalensis (Labiatae), has the potential to be used as a treatment for inflammatory conditions. Baicalein significantly suppressed the expression of iNOS in experiments involving BV-2 cells and primary microglia activated with LPS and IFN-γ. The effect mentioned was obtained by blocking the MAPKs and NFκB signaling pathways (Chen et al., 2004). Furthermore, baicalein displayed neuroprotective properties in a PD in vivo experimental model using MPP + neurotoxin. Baicalein protected injured DA neurons by reducing the activation of microglia-induced inflammation by reducing inflammasome activity. These findings emphasize baicalein’s medicinal potential in reducing inflammatory responses and protecting against neurotoxicity (Hung et al., 2016).
3.2.2.11 a-Mangostin
α-Mangostin is a plant polyphenol produced from various parts of the Garcinia mangostana, a plant that has a xanthone core structure. The results of a recent in vivo study showed that a-Mangostin demonstrated a potent neuroprotective quality dependence on concentration. a-Mangostin at doses of 1, 10, and 100 nM effectively shielded DA neurons from neurotoxicity caused by microglial activation caused by a-syn in a co-culture system of rat mesencephalic neurons and primary microglia. The potential of a-Mangostin to prevent the breakdown of IκBα and the translocation of NFκB p65 into the nucleus of M1-activated microglia caused by a-syn was linked to its neuroprotective action. These data imply that a-Mangostin has potential as a neuroprotective drug against a-syn-mediated microglial neurotoxicity (Hu et al., 2016).
3.2.2.12 Icariin
Icariin is a flavanol glycoside that has been prenylated and produced from several Epimedium species. It is the 8-prenyl derivative of kaempferol 3,7-O-diglucoside. In previous in vitro investigations, different concentrations of icariin (5, 10, and 50 μM) showed neuroprotective properties against the neurotoxicity induced by primary microglia activated with LPS. Additional research into the underlying mechanisms showed that icariin, in a manner dependent on the dosage used, suppressed the JNK, p38 MAPK, and NFκB signaling pathways by inhibiting the production of ROS and pro-inflammatory cytokines, including IL-6, TNF-α, and IL-1β in M1-activated microglia (Zeng et al., 2010). The neuroprotective characteristics of icariin were further investigated in a PD in vivo mouse model. Icarin, at doses of 0.01 and 0.1 μM, has been shown to modify M1 microglia activation produced by LPS/6-OHDA. This was accomplished by inhibiting NFκB p65 phosphorylation, which results in an indirect reduction in neuronal cell death (apoptosis) in primary rat midbrain neuroglia co-cultures (Wang et al., 2018).
3.2.2.13 Tenuigenin
Tenuigenin is a bioactive terpenoid found naturally in the roots of Polygala tenuifolia (Polygalaceae). It has neuroprotective benefits such as antioxidant, anti-aging, and anti-inflammatory capabilities. An in vitro study using different doses of tenuigenin (1, 2, and 4 μM) revealed a dose-dependent decrease in LPS-induced microglial M1 activation. The activation of the Nrf2/HO-1 pathway was directly linked to this neuroprotective significance (Wang et al., 2017). Furthermore, by blocking DA breakdown, a higher dosage of tenuigenin (50 mg/kg) increased DA levels in mice’s striatum. Tenuigenin dramatically decreased DA neurodegeneration by suppressing the NLRP3 inflammasome and lowering the stimulation of M1-activated microglia. With its capacity to minimize microglial activation, inhibit the NLRP3 inflammasome, and enhance Nrf2/HO-1 pathway activation, tenuigenin has the potential to be a promising neuroprotective agent (Fan et al., 2017).
3.2.2.14 Nobiletin
Nobiletin is a flavonoid compound in Tangerine peel (Citri reticulatae pericarpium). Numerous studies have demonstrated its antioxidant, anti-atherogenic, and anti-inflammatory qualities. Nobiletin administration at various doses (range from 1 to 50 μM) significantly suppressed the generation of pro-inflammatory cytokines, including IL-1β and TNF-α in a dose-dependent manner in a previous in vitro study using LPS-stimulated BV-2 microglia. According to detailed analysis, the underlying mechanism includes suppressing of phosphorylation of MAPKs and the translocation of NFκB into the nucleus (Cui et al., 2010). One notable feature of nobiletin is that it can penetrate the BBB and concentrate more in the brain than in peripheral organs. This distinct characteristic enables nobiletin to alleviate neuroinflammation and mitigate neuronal damage caused by M1-activated microglia (Singh et al., 2011). Furthermore, in a PD in vivo animal model, in which rats were injected with MPP+ in the middle forebrain bundle, using nobiletin (20 mg/kg, i. p., for 7 days) gave significant protection to DA neurons located in the SNpc. Nobiletin has also been shown to decrease M1 microglia activation, lowering inflammatory cytokines release (Jeong et al., 2015).
3.2.2.15 Taurine
Taurine, a non-protein amino acid containing sulfur, is found in bile and several mammalian organs. The neuroprotective effect of taurine was explored in previous in vivo research employing a maneb and paraquat-induced model of PD. The researchers discovered that mice who received maneb and paraquat suffered from severe dopaminergic neurotoxicity and motor impairments, which were considerably relieved by taurine administration. Notably, taurine reduced the accumulation of a-syn, a characteristic protein linked with PD, in maneb and paraquat-intoxicated rats (Che et al., 2018). Additional studies into the mechanisms behind taurine’s neuroprotective properties found that Taurine can inhibit microglial activation caused by maneb and paraquat. Interestingly, reducing microglia activation abolished taurine’s neurological protective effects, demonstrating the importance of microglial activation in taurine-mediated neural protection. Taurine was also observed to decrease the M1 polarization of microglia and lower the expression levels of pro-inflammatory proteins induced by maneb and paraquat (Wang et al., 2021). Moreover, taurine inhibited the stimulation of NOX2, a critical enzyme implicated in oxidative stress. This was accomplished by interfering with the translocation of the cytosolic component p47phox and the NFκB process. NOX2 activation and NFκB signaling are essential in beginning and maintaining the inflammatory response of M1 microglia (Che et al., 2018).
3.2.2.16 Plantamajoside
Plantamajoside, a natural product that comes from plantain seeds, has a wide range of biological activities, including anticancer, anti-inflammatory, and antioxidative features (Ravn et al., 2015). In an earlier in vivo research study, researchers used male C57BL/6 mice to induce a PD animal model by injecting LPS into the substantia nigra (SN) located in the midbrain region on the right side. The researchers discovered that Plantamajoside substantially alleviated the behavioral impairment caused by LPS in Parkinson’s disease rats. In addition, Plantamajoside has been shown to reduce SN damage produced by LPS and to decrease microglial cell over-activation in PD rats. Further investigation revealed that Plantamajoside exerted its effects in both PD mice and BV-2 cells by suppressing the activation of the histone deacetylase-2 (HDAC2)/MAPK pathway. Notably, Plantamajoside demonstrated its ability to reduce microglia polarization by inhibiting HDAC2 (Guo et al., 2023).
3.2.2.17 Swertiamarin
Swertiamarin, a widely researched natural compound, has anti-inflammatory effects. It is obtained from Enicostemma littorale Blume and is classified as a secoiridoid glycoside (Fadzil et al., 2021). Swertiamarin has been demonstrated in the setting of PD to inhibit the accumulation of a-syn in a Caenorhabditis elegans model produced by 6-OHDA. A prior in vivo study aimed to assess swertiamarin’s anti-inflammatory action against LPS-induced activation of C6 glial cells and its neuroprotective benefits in a rat model of PD caused by intra-striatal ROT. Surprisingly, swertiamarin treatment reduced the levels of IL-1β, TNF-α, and IL-6 in LPS-induced C6 glial cell activation. In a mouse model of ROT-induced PD, swertiamarin (at a dosage of 100 mg/kg, i. p.) substantially inhibited the activation of microglial and astroglial cells in the SN. Furthermore, swertiamarin reduced ROT-induced a-syn overexpression in the striatum and the SN. Notably, swertiamarin improved motor impairment caused by ROT-induced neurotoxicity and reduced the loss of dopaminergic neurons in the nigrostriatal pathway. These data suggest that swertiamarin has tremendous promise as an additional treatment for PD (Sharma et al., 2023).
3.3 Huntington’s disease
3.3.1 Role of microglia M1/M2 in Huntington’s disease
Huntington’s disease is a neurodegenerative condition with hereditary autosomal dominant traits. The main cause is a gene identified as huntingtin, which is found on the short arm p) of chromosome 4 and mutated to cause the disease (Gómez-Jaramillo et al., 2022). The CAG trinucleotide repeat expansion on chromosome 4 is the root cause of HD. Atypical involuntary motions, cognitive deterioration, and behavioral alterations are features of HD, in which the most apparent symptom is chorea (Gibson and Claassen, 2021). In contrast to normal control brains, considerable astrogliosis and microgliosis were found in the post-mortem brains of HD patients. According to a previous study, the density of microglia in the brains of HD patients fluctuated relative to the severity of neuronal loss (H. M. Yang et al., 2017). Furthermore, the extensive detection of M1 microglia significant biomarkers in HD brain suggests that M1 microglia may be important in the pathophysiology of HD. An alternatively activated M2 phenotype, however, has the potential to be neuroprotective, which contributes to HD recovery (Ji et al., 2018). Consequently, Pena-Altamira et al. concentrated on nutritional methods through the consumption of food-bioactive substances like carotenoids, phytosterols, and other substances that may affect microglial polarization, aid in neuron survival, and consequently lessen cognitive impairment associated with aging (Tang, 2018).
3.3.2 Nutraceuticals that influence microglial activation in Huntington’s disease
The impact of different natural products and nutraceuticals on HD through regulation of M1/M2 microglia polarization is presented in Table 4 and Figure 5.
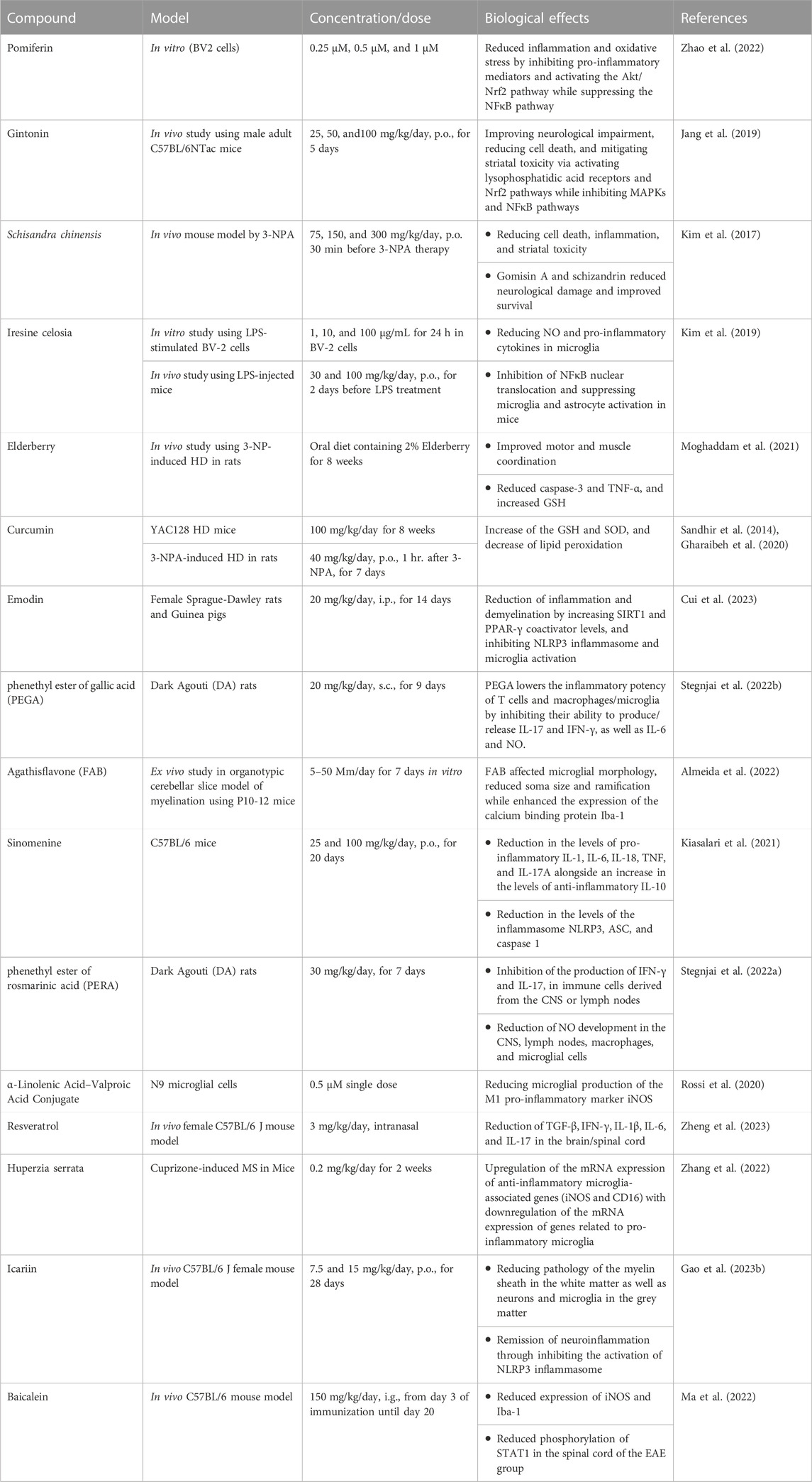
TABLE 4. The effects of different nutraceuticals on microglia in Huntington’s disease and Multiple sclerosis related models.
3.3.2.1 Pomiferin
The main components of Maclura pomifera fruits are prenylated isoflavones, osajin, and pomiferin (Son et al., 2007). In a recent study exploring neurodegenerative conditions such as HD, researchers used many methods such as cck-8, LDH, quantitative PCR, and ELISA to explore the effects of pomiferin on BV2 cell inflammation. The results showed that pomiferin, at different concentrations (0.25 μM, 0.5 μM, and 1 μM), successfully decreased the production of NO, ROS, and pro-inflammatory mediators such as IL-6, TNF-α, iNOS, and COX-2 in BV2 cells (Zhao et al., 2022). Further research into pomiferin’s mechanism of action revealed that it activated the Akt/Nrf2 pathway while suppressing the NFκB pathway. These data show that pomiferin may have therapeutic potential for reducing neuroinflammation in neurodegenerative conditions via microglial pathways (Kobayashi and Yamamoto, 2005; Nguyen et al., 2009; Zhao et al., 2022).
3.3.2.2 Gintonin
Gintonin is a glycolipid protein conjugated with lysophosphatidic acid that was originally extracted from Korean ginseng (Lim et al., 2022). Gintonin, administered orally at doses of 25, 50, or 100 mg/kg/day, showed promising results in reducing neurological impairment and striatal toxicity in an HD adult C57BL/6NTac adult mice model. After exposure to 3-nitropropionic acid (3-NPA), the striatum’s production of inflammatory mediators, microglial activation, and mitochondrial dysfunction was all decreased by pre-treatment with gintonin. Furthermore, gintonin suppressed MAPKs and NFκB signaling while activating lysophosphatidic acid receptors and Nrf2 signaling pathways (Jang et al., 2019). In mice with N171-82Q-mutant HTT overexpression, gintonin had neuroprotective effects by lowering cell death and mutant huntingtin protein aggregates in STHdh cells and improving neurological impairment. The beneficial effects of gintonin were lessened by pre-inhibiting lysophosphatidic acid receptors with Ki16425 (Wild and Tabrizi, 2014; Jang et al., 2019).
3.3.2.3 Schisandra chinensis
A versatile traditional Chinese medicine; schisandra (Schisandra chinensis) is used for a variety of health problems, including respiratory, hepatic, gastrointestinal, and stress-related diseases. Additionally, it may help with menopausal symptoms, diabetes, polycystic ovarian syndrome, obesity, hepatitis, cognitive function, physical stamina, and as a preventative step for estrogen-dependent malignancies (Bokelmann, 2022). A mouse model of HD produced by 3-NPA was used to investigate Schisandra chinensis extract. The mice were given Schisandra chinensis extract at various doses (75, 150, or 300 mg/kg/day, orally) before or after 3-NPA therapy, Neuroprotective effects were investigated both before and after therapy, with pre-treatment being more beneficial. Schisandra chinensis extract decreased inflammatory markers, lesion area, cell death, and microglial activation. It inhibited the MAPK and NFκB signaling pathways while promoting the Nrf-2 pathway. Schizandrin and gomisin A, two Schisandra chinensis extract ingredients, reduced 3-NPA-induced brain damage and mortality (Kim et al., 2017).
3.3.2.4 Iresine celosia
Iresine celosia is a cytochrome-flavoprotein with potent antioxidant properties (Porru et al., 2017). Neurological diseases, such as HD, are influenced by aberrant inflammatory responses in the central nervous system (Nayak et al., 2011). A recent research investigated managing neuroinflammation using natural therapies like Iresine celosia to address this. The researchers looked at the effects of Iresine celosia extract on LPS-stimulated BV2 cells in mouse models. NO and pro-inflammatory cytokines in microglia cells were considerably reduced by Iresine celosia extract at various doses (1–100 μg/mL) without causing any harm. In mice with neuroinflammation, it also prevented NFκB translocation and improved behavioral impairments (Kim et al., 2019).
3.3.2.5 Elderberry
Sambucus spp. elderberries are cultivated in Europe, Asia, North Africa, and North America. Recent research indicates that elderberry assists in reducing some viral infections’ symptoms (Zakay-Rones et al., 2007). It has been established that anthocyanins, such as cyanidin-3-O-sambubioside and cyanidin-3-O-glucoside, and flavonoids, such as quercetin and rutin, are the antioxidative and anti-inflammatory substances present in the highest concentration in berries and flowers (Mikulic-Petkovsek et al., 2012). According to several additional studies, elderberry possesses various neuroprotective qualities (by reducing microglial activation), which can lower the loss of neuronal cells (Jiang et al., 2015; Zielińska-Wasielica et al., 2019).
To demonstrate the extreme nature of neuro-inflammatory processes, immunohistochemistry staining for the microglia marker (Iba-1) was performed in recent investigations. The results showed that rats given elderberry had significantly decreased microgliosis. The study concluded that elderberry’s antioxidant action increased GSH concentration and reduced ROS production in the tissue that had been damaged. Additionally, by reducing microglia’s production of TNF-α, this study sheds new illumination on using elderberry as neuroprotective medicine to treat HD by improving neuron survival due to microglial inactivation (Moghaddam et al., 2021).
3.3.2.6 Curcumin
According to a previous in vivo study in YAC128 HD mice, solid lipid nanoparticles of curcumin reduced HD-like neurodegeneration. By boosting glutathione levels and reducing superoxide dismutase activity, solid lipid nanoparticles of curcumin greatly reduced protein carbonyl formation, lipid peroxidation, ROS levels, and mitochondrial swelling (Gharaibeh et al., 2020). Additionally, in a 3-NPA-induced HD model in rats, curcumin therapy is believed to improve cognitive and motor abilities, regain succinate dehydrogenase activity, and lessen oxidative stress (Sandhir et al., 2014). Curcumin can cross across the BBB because it is lipid soluble. It then blocks the activation of microglia by decreasing the expression of iNOS. Curcumin suppresses cytokines release and oxidative stress and lowers NO generation, and the related signaling pathways, which has an anti-inflammatory effect on microglia. In addition, curcumin inhibits apoptosis, PI3k/Akt and iNOS, lipoxygenase, and COX-2, and induces activation of HO-1, Nrf-2, and the antioxidant response element mechanism in neuronal cells as well as microglia (Ghasemi et al., 2019). Furthermore, curcumin also rescues downregulated molecular chaperones in HD, including Hsp40 and Hsp70, which have superior roles in the disease progression (Suzuki et al., 2023).
3.4 Multiple sclerosis
3.4.1 Role of M1/M2 in multiple sclerosis
The most prevalent autoimmune disease that results in non-traumatic neurological damage in young adults is MS. Inflammation with demyelination and astroglial growth (gliosis) and neurodegeneration are the two pathological hallmarks of MS (Hauser and Cree, 2020). In the early stages of MS, axonal damage is detected. Acute, inflammatory lesions consisted of more transected or swollen axons than chronic plaques. It has been hypothesized that inflammation plays an essential role in the ongoing neurodegeneration in MS because of the beneficial association between axonal inflammation and structural modifications (Correale, 2014). It has been observed that peripheral inflammatory cells infiltrate into the CNS tissues, presumably because of BBB breaking down in the sick condition. The majority of the peripheral inflammatory cells that are infiltrating are macrophages and microglia, followed by CD4+ T cells, whereas the CNS consists of just a few CD8+ T cells, B cells, and plasma cells (Chu et al., 2018). Microglial activation in early lesions with a proliferation of macrophages in seriously injured tissue has also been associated with active demyelination and neurodegeneration (Correale, 2014). Using a panel of markers that distinguish M1-or M2-type macrophages/microglia, it was discovered that M1-type differentiated cells accumulated preferentially around the lesion edge, suggesting a vital purpose for these cells in the development of MS lesions (Ja et al., 2020).
The presence of myelin and axonal remains, the elevated levels of major histocompatibility complex-II and co-stimulatory molecules, and the release of several inflammatory and neurotoxic mediators through activated macrophages and microglia in MS and experimental autoimmune encephalomyelitis (EAE) lesions are all consistent findings. Additionally, studies using bone marrow chimeras demonstrated that the activation and proliferation of microglia preceded the beginning of EAE and that suppressing their activation prevents the growth and maintenance of inflammatory lesions in the CNS. It is important to observe that MS patients have active microglia clusters dispersed throughout their generally normal-looking white matter (Bogie et al., 2014).
3.4.2 Nutraceuticals that influence microglial activation in multiple sclerosis
The impact of different natural products and nutraceuticals on MS through regulation of M1/M2 microglia polarization is presented in Table 4 and Figure 6.
3.4.2.1 Emodin
Emodin (1, 3, 8-trihydroxy-6-methylanthraquinone), a chemical derived from herbs such as rhubarb, has a neuroprotective effect (Zhu et al., 2019). Emodin has been shown in studies to have a variety of pharmacological actions, including antioxidant, immunomodulatory, and anticancer activities involving autophagy and apoptosis (Mitra et al., 2022). Emodin suppresses the action of the casein kinase 2 protein kinase. In EAE, which is an inflammatory, autoimmune demyelinating condition that affects rodents’ CNS and is clinically and pathologically similar to MS in humans, systemic casein kinase 2 inhibition or its reduction in CD4+ T cells has neuroprotective effects (Gibson and Benveniste, 2018). In vitro investigations with female Sprague-Dawley rats and guinea pigs indicated that emodin reduced the severity of EAE; also, the expression levels of NLRP3 signaling pathway components, COX-2, TNF-α, and IL-6 were lowered in the EAE group treated with emodin (Cui et al., 2023). COX-2 is secreted by immune cells, and its greater expression could indicate immune cell activity and increased inflammation (Nitric et al., 2005). Treatment with emodin restored iNOS and NO production in the research, which is consistent with the benefits of Emodin treatment found in other animal models of disease, suggesting the therapeutic effects of emodin in EAE rats and the probable involvement of the NLRP3 signaling system (Hu et al., 2021). Furthermore, produced inflammatory cytokines can encourage the aggregation and activation of resting microglia, which in response produce inflammatory cytokines, creating a vicious cycle (Wu et al., 2021). However, they discovered that emodin therapy decreased microglial aggregation and activation in EAE mice and lowered inflammatory levels in BV2 cells, resulting in neuroprotective advantages. Nonetheless, they discovered that EAE rats had lower levels of sirtuin 1 and Peroxisome proliferator-activated receptor-gamma coactivator expression and higher levels of NLRP3 inflammasome component expression than EAE + emodin rats. In EAE rats, emodin therapy resulted in elevated sirtuin 1 and Peroxisome proliferator-activated receptor-gamma coactivator levels as well as symptom relief (Cui et al., 2023).
3.4.2.2 Gallic acid
Gallic acid (GA), a secondary metabolite abundant in many plants, nuts, and fruits, has been associated with anti-inflammatory activities. GA’s anti-inflammatory activities have been attributed to its capacity to interfere with MAPK and NFκB signaling, restricting immune cell activation and effector qualities (Bai et al., 2021). The phenethyl ester of gallic acid (PEGA) was developed to enhance gallic acid bioavailability and consequently its therapeutic potential. PEGA has been demonstrated to affect the inflammatory activities of T-cells and macrophages/microglia in an in vivo study of its effects on encephalitogenic cells (Stegnjai et al., 2022b) PEGA lowered the inflammatory potency of T-cells and microglia by inhibiting their ability to produce or release IL-17 and IFN-γ, as well as IL-6 and NO. Additionally, it significantly limits ongoing inflammatory responses against the CNS while also alleviating EAE. The reported effect of PEGA on T cells in the lymph nodes and the spinal cord’s ability to produce IFN-γ and IL-17 is particularly relevant for its advantageous effect on EAE.
Furthermore, limiting NO production by immune cells in the CNS is critical for PEGA’s beneficial impact on EAE. Much of the CNS tissue death during neuroinflammation is caused by the harmful effects of NO and its metabolite peroxy-nitrite (Spasojevic and Miljkovic, 2013). Furthermore, macrophage/microglia production of inflammatory cytokines TNF-α and IL-6 have been related to the etiology of CNS autoimmunity (Shemer and Jung, 2015; O’Loughlin et al., 2018). As a result, the suppressive impact of PEGA on the release of IL-6 in macrophages/microglia and TNF-α in microglia may contribute to the agent’s amelioration of EAE. PEGA’s inhibitory effects on NO, TNF-α, and IL-6 are consistent with the previously reported effects of GA and GA-like substances on immune cells in both in vivo and in vitro studies (Stegnjai et al., 2022b).
3.4.2.3 Flavonoids
Flavonoids are plant-derived natural chemicals that have powerful anti-oxidant, anti-inflammation, and anti-neurodegeneration properties (Lima et al., 2016). Agathisflavone (FAB) is a bioflavonoid isolated from Poincianella pyramidalis that has minimal toxicity and a variety of biological activities, including anti-inflammatory, neuroprotective, and neurogenic properties, as well as the ability to reduce astrogliosis and microgliosis after lysolecithin-induced demyelination (Almeida et al., 2020; dos Santos Souza et al., 2018). Proteolipid protein, a cholesterol-associated protein with important roles in myelin membrane intracellular trafficking; myelin basic protein, is a key protein involved in myelin compaction. Meanwhile, cyclic nucleotide phosphodiesterase is an important protein involved in the maintenance of a normal inner tongue during the myelination of small-diameter axons. The absence of any of these proteins results in significant myelin sheath alterations and/or demyelination (Snaidero et al., 2017). In the previous investigations at doses above 5 μg, FAB enhances the amount of myelin basic protein immunolabelling of NF+ axons in postnatal cerebellar slice cultures. At these doses, however, the numbers of oligodendrocyte progenitor cells and oligodendrocytes were unaltered, showing that FAB enhanced the degree of myelination per oligodendrocyte rather than increasing their overall number (Almeida et al., 2022). Although, they demonstrate how FAB affected microglial morphology, reducing soma size and ramification while enhancing the expression of the calcium-binding protein Iba1. On the other hand, FAB did not influence microglial connections with oligodendrocytes, which may be essential to the release of trophic substances onto oligodendrocytes (Ioannidou et al., 2014; Domingues et al., 2016). FAB modulates microglia, which is consistent with in vitro data that FAB is anti-inflammatory and may shift microglia to a less active state (Almeida et al., 2022).
3.4.2.4 Sinomenine
An alkaloid called Sinomenine exists in the roots of Sinomenium Acutum and has previously been used to treat arthritis. Sinomenine also has anti-inflammatory and immunosuppressive properties, as well as potential benefits for treating neurological diseases (Hong et al., 2022). Recently, the natural alkaloid sinomenine can significantly reduce neuroinflammation and demyelination in the spinal cord of a myelin oligodendrocyte glycoprotein 35–55 immune-mediated EAE model of MS. This was shown by partial suppression of microglial and astrocytic activation and pro-inflammatory cytokines, as well as by restoration of anti-inflammatory cytokines and improvement of motor functions. Inflammasome NLRP3 and the associated caspase-1 pyroptotic pathway were additionally suppressed by sinomenine (Kiasalari et al., 2021). Furthermore, abnormal alterations in pro-inflammatory and anti-inflammatory cytokines play critical roles in the pathophysiology of autoimmune illnesses such as MS and EAE (Fontes et al., 2017; Ma et al., 2017). According to the findings, sinomenine can reduce the tissue levels of TNF-α, IL-6, IL-18, IL-1, and IL-17, while suitably elevating anti-inflammatory IL-10 in the myelin oligodendrocyte glycoprotein-immunized EAE group. Consistent with these findings, sinomenine has been shown to reduce pro-inflammatory mediators and downregulate oxidative stress in a mouse model of ankylosing spondylitis (Dong et al., 2019), and sinomenine has been shown to protect against inflammation-related pain by reducing inflammatory mediators via p38-MAPK/NF-B signaling (Yuan et al., 2018).
3.4.2.5 Rosmarinic acid
Rosmarinic acid is a polyphenolic chemical found in abundance in Lamiaceae herbs. It has been shown to have powerful anti-inflammatory effects both in vitro and in vivo studies (Luo et al., 2020). Rosmarinic acid has been demonstrated to block the T helper-17 axis in psoriasis-like illness in mice, as well as dendritic cell antigen-presenting potency in vitro (Zhang et al., 2021). These rosmarinic acid actions are crucial for MS therapy because T-helper-17 cells are among the most significant pathogenic cells in this disease (Murúa et al., 2022). Rosmarinic acid’s in vivo effects are limited because its polar carboxylic acid group inhibits the compound’s ability to permeate cellular membranes. To increase its bioavailability, lipophilic ester- and amide-derivatives of rosmarinic acid (Gerogianni et al., 2018), such as phenethyl ester of rosmarinic acid (PERA), were synthesized (Stegnjai et al., 2022a). A recent study showed that PERA significantly lowers the clinical course of EAE. This effect corresponds to a decrease in NO in both the CNS and the peripheral immune compartment. In addition, PERA inhibits IFN-γ and IL-17 production by popliteal lymph node cells and spinal cord immune cells in an in vitro study (Stegnjai et al., 2022a). NO, and its product peroxynitrite is a significant participant in the destruction of CNS tissue during neuroinflammation (Cross et al., 1997). Indeed, significant amounts of NO released locally within the CNS were found to be harmful in DA rats with EAE (Miljković et al., 2011; Petković et al., 2013). Thus, the reported NO-inhibiting actions of PERA on macrophages and microglia are extremely relevant to the PERA model’s positive benefits. Furthermore, PERA’s inhibitory effect on microglia release of the inflammatory cytokines; TNF-α and IL-6, is likely to contribute to the compound’s demonstrated positive effects in EAE, as microglia production of these cytokines has been linked to the etiology of CNS autoimmunity (Shemer and Jung, 2015; O’Loughlin et al., 2018). These PERA effects are consistent with the previously documented effects of rosmarinic acid and its related compounds on NO, TNF-α, and IL-6 in vivo and in vitro (Stegnjai et al., 2022a).
3.4.2.6 a-Linolenic acid–valproic acid conjugate
Rossi et al. developed medications that incorporated valproic acid and a-linolenic acid, either linked or conjugated. By using N9 microglial cells that have been treated with 100 ng/mL LPS, they found that diamide conjugate and ethanolamide conjugate significantly reduced microglia production of the M1 pro-inflammatory marker iNOS at low concentrations (0.5 μM) (Rossi et al., 2020).
3.4.2.7 Resveratrol
Resveratrol regulates TLR4 signaling, and immunological activators such as LPS, along with additional immune stimulants (Malaguarnera, 2019). The NF-kB pathway, COX-2 pathway, TLR expression, and activation of the NLRP3-inflammasome are all inhibited by resveratrol, which mediates these effects and promotes the production of anti-inflammatory M2 macrophages (Moudgil and Venkatesha, 2023). As a result, an approach being developed to treat MS involves intranasal delivery of RAW-Exo formulation with added resveratrol had led to improving the clinical progression of MS in an in vivo study, and greatly reduced inflammatory responses in the CNS and peripheral system, by affecting microglia (Zheng et al., 2023).
3.4.2.8 Huperzia serrata
Huperzia serrata has been demonstrated to play a role in several animal models of neurological illnesses, as well as to alleviate cognitive impairment, diminish neuroinflammation, improve neuroprotection by boosting cortical inhibition, and enhance neuroprotection at low dosages. Multiple in vivo and in vitro research investigations have demonstrated that H. serrata may directly influence microglia to decrease CNS inflammation. It was discovered that the hippocampus and corpus callosum both had considerably fewer microglia following H. serrata therapy. In the H. serrata-treated mice, there were significantly fewer microglia branch endings and shorter overall branch lengths, indicating that H. serrata could effectively control microglia activation (H. Zhang et al., 2022). It has been proven that the pathophysiology of MS corresponds to the microglia-associated mRNA of pro-inflammatory and anti-inflammatory genes. Quantitative RT-PCR was applied to assess the mRNA expression of several pro-inflammatory and anti-inflammatory genes in the microglia to examine the potential role of H. serrata in cuprizone-induced inflammation. According to the study’s results, H. serrata significantly upregulated the mRNA expression of anti-inflammatory microglia-associated genes (iNOS and CD16) while significantly downregulating the mRNA expression of genes related to pro-inflammatory microglia (Zhang et al., 2022).
3.4.2.9 Icariin
Icariin is a naturally present flavonoid glucoside that has been identified in the family of Epimedium that is frequently utilized in traditional Chinese medicine (Gao et al., 2023b). Degeneration of the white matter is commonly associated with MS. Comprehensive examination of the neuropathology also indicates severe pathology in the cortical and deep grey matter. Examination for NeuN expression in, the neuronal marker protein that is used to estimate neuronal density in the brain, determined the effect of Icariin on the grey matter. In the hippocampus and cortex of EAE mice modeled in vitro, there were considerably fewer NeuN + neurons than in the control group (Gao et al., 2023b). Glial cell activation is a hallmark of neuroinflammation. In the hippocampus and cortex of EAE animals, there was an apparent increase in the expression of Iba1+, markers of microglia activation, and the microglia’s structure was investigated to be longitudinally branching. Importantly, these defects almost completely resolved in mice administered high doses of Icariin (Gao et al., 2023b).
3.4.2.10 Baicalein
The primary flavonoid found in S. baicalensis Georgi, a traditional Chinese medicine, is called baicalein. It exhibits antioxidant, anti-inflammatory, anticancer, antiviral, and neuroprotective pharmacological activities. (Ma et al., 2022). Iba-1 is an indication of activated macrophages and microglia. Arg1 is a polarized marker for M2 microglia/macrophages, while iNOS is a marker for M1 microglia/macrophages. In recent in vivo and in vitro studies, the number of microglia and macrophages that were both iNOS- and Iba-1-positive decreased considerably after baicalein therapy. In addition, resulting from baicalein treatment, Iba-1, and iNOS expression significantly lowered in comparison to the EAE model group. Nonetheless, qRT-PCR was applied to identify the expression of M1 inflammatory markers in each group’s mouse spinal cord. The outcomes demonstrated that animals receiving baicalein or dexamethasone had significantly lower mRNA levels of iNOS, CD86, IL-12p35, IL-12p40, and IL-18 in their spinal cords than the EAE model group (Ma et al., 2022).
4 Clinical trials on nutraceuticals in neurodegenerative diseases
Concerning human data, a few randomized clinical trials have been conducted for neurodegenerative disorders after natural product administration. Only a small number of clinical research has looked at the impact of curcumin on human cognitive performance in AD, compared with animal studies. The findings of these investigations are contradictory. Some studies show no cognitive enhancing benefits of curcumin (Baum et al., 2008; Ringman et al., 2012), whilst other studies suggested a positive effect of curcumin on cognition (Cox et al., 2015; Rainey-Smith et al., 2016; Small et al., 2018). Several investigations reveal protective mechanisms of curcumin against cognitive impairment, similar to animal research (Baum et al., 2008; Small et al., 2018). However, neuroimaging suggests that curcumin reduces Aβ deposits in the brain (Small et al., 2018). Results regarding Aβ reduction are ambiguous because most peripheral measurements, such as plasma, serum, and CSF levels, have not detected significant changes in Aβ or tau levels between curcumin and placebo (Baum et al., 2008; Ringman et al., 2012). Unfortunately, only one study by Baum et al. published measures of oxidative stress biomarkers, while no other study reported measurements of inflammatory biomarkers, even though these are the principal targets of curcumin and have demonstrated significant improvement in animal research (Baum et al., 2008). Meanwhile, data from human studies of curcumin in Huntington’s disease is lacking, and clinical trials should be strongly promoted in this area.
Regarding resveratrol, a phase II clinical trial evidenced the safety and well-tolerated effects of 500 mg orally once daily resveratrol on mild to moderate AD patients for 52 weeks, with no observed effects on AD biomarkers (Turner et al., 2015). However, decreased MMP 9 in CSF, controlled neuro-inflammation, adaptive immunity generation, and attenuated cognitive decline were confirmed in further investigation (Moussa et al., 2017). Meanwhile, another randomized placebo-controlled study on a small number of AD patients that received resveratrol (5 mg/day), showed less cognitive deterioration. In contrast, a dose of 200 mg/day of RV for 26 weeks did not experience any appreciable improvements in verbal memory function in 60 elderly individuals (Huhn et al., 2018).
Furtherly, in patients with AD, H. serrata appears to positively impact the restoration of cognitive function, performing everyday tasks, and overall clinical assessment (Yang et al., 2013). In addition, according to a clinical investigation, taurine has a positive effect on PD patients’ motor symptoms, and its levels in their plasma were decreased (Zhang et al., 2016). A prospective cohort study showed that a-Linolenic acid is associated with improved magnetic resonance imaging lesions activity in 87 multiple sclerosis patients (Bjornevik et al., 2019).
5 Conclusion
In our review, we emphasized the role and polarization control of microglia in the pathophysiology of neurodegenerative disorders and briefly introduced the function and phenotype of microglia to explore prospective therapeutic approaches. Transcription factors, receptors, and cytokines are merely a few examples of the numerous categories that modulate microglia polarization from M1 to M2. TNF-α, ILs, CXCLs, ROS, and NO, which have pro-inflammatory and neurotoxic effects, are released when the M1 phenotype is activated by IFN- γ, LPS, and TLR pathways. Meanwhile, M2 microglia are activated by different types of ILs to release abrineurin and anti-inflammatory cytokines, such as TGF-β, which suppress inflammatory responses and have neuroprotective effects. NcRNAs, such as miRNA-155, miR-23b-3p, and circHIPK3, seem to represent key factors in shifting microglia from M1 to M2. Consequently, inhibiting M1 microglia and promoting M2 microglial activation is required for neurodegenerative cure. In AD, curcumin, aromatic-turmerone, caeminaxin A, myricetin, aurantiamide, 3,6′-disinapoylsucrose, and resveratrol restored the normal balance of M1/M2 markers in microglia, which was also achieved by plant extracts of D. cochinchinensis stemwood and O. majorana L. Microglia-mediated apoptosis was prohibited because of andrographolide, sulforaphane, triptolide, xanthoceraside, and piperlongumine inhibition of Aβ-induced microglial M1 activation. In PD, urolithin A, kurarinone, asarone, galangin, baicalein, and mangostin hindered ROS and pro-inflammatory cytokines in M1-activated microglia. Further, icariin and tenuigenin suppressed microglial neurotoxicity via inhibiting NLRP3 inflammasome. Likewise, citronellol, myrcene, a-cyperone, nobiletin, taurine, plantamajoside, and swertiamarin showed neuroprotective effects in Parkinson’s. Similarly, elderberry, curcumin, iresine celosia, Schisandra chinensis, gintonin, and pomiferin showed promising results in improved symptoms in HD patients. In MS, linolenic acid, resveratrol, H. serrata, icariin, and baicalein are potent inhibitors of microglial activation, in addition to emodin, PEGA, FAB, sinomenine, and PERA, which all showed improved disease outcomes. Therefore, additional studies are required to resolve the significant enigma surrounding microglia polarization. It is important to note that these studies in the particular context of neurodegenerative illnesses clearly demand additional research. Moreover, several regulators of the microglia phenotype, which connects cytokine and signal pathways, may be linked to one another. It is still unknown how other risk genes affect microglia polarization in neurodegenerative disorders. Finally, the available findings encourage incorporating the natural product in treatment guidelines for neurodegenerative diseases, but more proof from randomized controlled studies on their effectiveness is highly warranted.
Author contributions
Conceptualization: SD and RS; investigation, and writing—original draft: AbE, AmE, and GS; visualization: AbE; writing—review and editing: SD, AbE, AmE, GS, and RS. All authors contributed to the article and approved the submitted version.
Conflict of interest
The authors declare that the research was conducted in the absence of any commercial or financial relationships that could be construed as a potential conflict of interest.
Publisher’s note
All claims expressed in this article are solely those of the authors and do not necessarily represent those of their affiliated organizations, or those of the publisher, the editors and the reviewers. Any product that may be evaluated in this article, or claim that may be made by its manufacturer, is not guaranteed or endorsed by the publisher.
References
Abrishamdar, M., Jalali, M. S., and Rashno, M. (2022). MALAT1 lncRNA and Parkinson’s disease: the role in the pathophysiology and significance for diagnostic and therapeutic approaches. Mol. Neurobiol. 59, 5253–5262. doi:10.1007/s12035-022-02899-z
Almeida, M. M. A. de, Pieropan, F., Footz, T., David, J. M., David, J. P., da Silva, V. D. A., et al. (2022). Agathisflavone modifies microglial activation state and myelination in organotypic cerebellar slices culture. J. Neuroimmune Pharmacol. 17, 206–217. doi:10.1007/s11481-021-09991-6
Almeida, M. M. A. de, Souza, C., dos, S., Dourado, N. S., da Silva, A. B., Ferreira, R. S., et al. (2020). Phytoestrogen Agathisflavone ameliorates neuroinflammation-induced by LPS and IL-1β and protects neurons in cocultures of glia/neurons. Biomolecules 10, 562. doi:10.3390/biom10040562
Aloi, M. S., Prater, K. E., Sánchez, R. E. A., Beck, A., Pathan, J. L., Davidson, S., et al. (2023). Microglia specific deletion of miR-155 in Alzheimer’s disease mouse models reduces amyloid-β pathology but causes hyperexcitability and seizures. J. Neuroinflammation 20, 60. doi:10.1186/s12974-023-02745-6
Ameen, A. M., Elkazaz, A. Y., Mohammad, H. M. F., and Barakat, B. M., 2017. Anti-inflammatory and neuroprotective activity of boswellic acids in rotenone parkinsonian rats. 819–829. doi:10.1139/CJPP-2016-0158
An, Y. W., Jhang, K. A., Woo, S. Y., Kang, J. L., and Chong, Y. H. (2016). Sulforaphane exerts its anti-inflammatory effect against amyloid-β peptide via STAT-1 dephosphorylation and activation of Nrf2/HO-1 cascade in human THP-1 macrophages. Neurobiol. Aging 38, 1–10. doi:10.1016/J.NEUROBIOLAGING.2015.10.016
Azimi, A., Ghaffari, S. M., Riazi, G. H., Arab, S. S., Tavakol, M. M., and Pooyan, S. (2016). α-Cyperone of Cyperus rotundus is an effective candidate for reduction of inflammation by destabilization of microtubule fibers in brain. J. Ethnopharmacol. 194, 219–227. doi:10.1016/J.JEP.2016.06.058
Azimullah, S., Jayaraj, R. L., Meeran, M. F. N., Jalal, F. Y., Adem, A., Ojha, S., et al. (2023). Myrcene salvages rotenone-induced loss of dopaminergic neurons by inhibiting oxidative stress, inflammation, apoptosis, and autophagy. Mol. 2023 28, 685. doi:10.3390/MOLECULES28020685
Bai, J., Zhang, Y., Tang, C., Hou, Y., Ai, X., Chen, X., et al. (2021). Gallic acid: pharmacological activities and molecular mechanisms involved in inflammation-related diseases. Biomed. Pharmacother. 133, 110985. doi:10.1016/j.biopha.2020.110985
Baufeld, C., O’Loughlin, E., Calcagno, N., Madore, C., and Butovsky, O. (2017). Differential contribution of microglia and monocytes in neurodegenerative diseases. J. Neural Transm. 125, 809–826. doi:10.1007/S00702-017-1795-7
Baum, L., Lam, C. W. K., Cheung, S. K. K., Kwok, T., Lui, V., Tsoh, J., et al. (2008). Six-month randomized, placebo-controlled, double-blind, pilot clinical trial of curcumin in patients with Alzheimer disease. J. Clin. Psychopharmacol. 28, 110–113. doi:10.1097/JCP.0B013E318160862C
Bjornevik, K., Myhr, K. M., Beiske, A., Bjerve, K. S., Holmøy, T., Hovdal, H., et al. (2019). α-Linolenic acid is associated with MRI activity in a prospective cohort of multiple sclerosis patients. Multiple Scler. J. 25, 987–993. doi:10.1177/1352458518779925
Bogie, J. F. J., Stinissen, P., and Hendriks, J. J. A., 2014. Macrophage subsets and microglia in multiple sclerosis 191–213. doi:10.1007/s00401-014-1310-2
Bokelmann, J. M. (2022). Schisandra/schizandra (schisandra chinensis). Med. Herbs Prim. Care, 587–592. doi:10.1016/B978-0-323-84676-9.00069-6
Bouyahya, A., Chamkhi, I., Benali, T., Guaouguaou, F.-E., Balahbib, A., El Omari, N., et al. (2021). Traditional use, phytochemistry, toxicology, and pharmacology of Origanum majorana L. J. Ethnopharmacol. 265, 113318. doi:10.1016/j.jep.2020.113318
Cai, Q., Li, Y., Mao, J., and Pei, G. (2016). Neurogenesis-promoting natural product α-asarone modulates morphological dynamics of activated microglia. Front. Cell Neurosci. 10, 280. doi:10.3389/fncel.2016.00280
Capiralla, H., Vingtdeux, V., Zhao, H., Sankowski, R., Al-Abed, Y., Davies, P., et al. (2012). Resveratrol mitigates lipopolysaccharide- and Aβ-mediated microglial inflammation by inhibiting the TLR4/NF-κB/STAT signaling cascade. J. Neurochem. 120, 461–472. doi:10.1111/J.1471-4159.2011.07594.X
Che, Y., Hou, L., Sun, F., Zhang, C., Liu, X., Piao, F., et al. (2018). Taurine protects dopaminergic neurons in a mouse Parkinson’s disease model through inhibition of microglial M1 polarization. Cell Death Dis. 9, 435. doi:10.1038/s41419-018-0468-2
Chen, C. J., Raung, S. L., Liao, S. L., and Chen, S. Y. (2004). Inhibition of inducible nitric oxide synthase expression by baicalein in endotoxin/cytokine-stimulated microglia. Biochem. Pharmacol. 67, 957–965. doi:10.1016/J.BCP.2003.10.010
Chen, G., Liu, J., Jiang, L., Ran, X., He, D., Li, Y., et al. (2017). Galangin reduces the loss of dopaminergic neurons in an LPS-evoked model of Parkinson’s disease in rats. Int. J. Mol. Sci. 19 (12), 12. doi:10.3390/IJMS19010012
Chen, L., Liu, Y., Jia, D., Yang, J., Zhao, J., Chen, C., et al. (2016). Pharmacokinetics and biodistribution of aurantiamide and aurantiamide acetate in rats after oral administration of portulaca oleracea L. extracts. J. Agric. Food Chem. 64, 3445–3455. doi:10.1021/acs.jafc.6b00470
Chen, Y., Schlotterer, A., Kurowski, L., Li, L., Dannehl, M., Hammes, H. P., et al. (2023). miRNA-124 prevents rat diabetic retinopathy by inhibiting the microglial inflammatory response. Int. J. Mol. Sci. 24, 2291. doi:10.3390/ijms24032291
Chinta, S. J., and Andersen, J. K. (2008). Redox imbalance in Parkinson’s disease. Biochimica Biophysica Acta (BBA) - General Subj. 1780, 1362–1367. doi:10.1016/J.BBAGEN.2008.02.005
Choi, M. J., Lee, E. J., Park, J. S., Kim, S. N., Park, E. M., and Kim, H. S. (2017). Anti-inflammatory mechanism of galangin in lipopolysaccharide-stimulated microglia: critical role of PPAR-γ signaling pathway. Biochem. Pharmacol. 144, 120–131. doi:10.1016/J.BCP.2017.07.021
Chu, F., Shi, M., Zheng, C., Shen, D., Zhu, J., Zheng, X., et al. (2018). The roles of macrophages and microglia in multiple sclerosis and experimental autoimmune encephalomyelitis. J. Neuroimmunol. 318, 1–7. doi:10.1016/j.jneuroim.2018.02.015
Citron, M., Oltersdorf, T., Haass, C., McConlogue, L., Hung, A. Y., Seubert, P., et al. (1992). Mutation of the β-amyloid precursor protein in familial Alzheimer’s disease increases β-protein production. Nature 360, 672–674. doi:10.1038/360672a0
Colonna, M., and Butovsky, O. (2017). Microglia function in the central nervous system during health and neurodegeneration. Annu. Rev. Immunol. 35, 441–468. doi:10.1146/ANNUREV-IMMUNOL-051116-052358
Condello, C., Yuan, P., Schain, A., and Grutzendler, J. (2015). Microglia constitute a barrier that prevents neurotoxic protofibrillar Aβ42 hotspots around plaques. Nat. Commun. 6, 6176. doi:10.1038/ncomms7176
Correale, J. (2014). The role of microglial activation in disease progression, 1288–1295. doi:10.1177/1352458514533230
Cox, K. H. M., Pipingas, A., and Scholey, A. B. (2015). Investigation of the effects of solid lipid curcumin on cognition and mood in a healthy older population. J. Psychopharmacol. 29, 642–651. doi:10.1177/0269881114552744
Cross, A. H., Manning, P. T., Stern, M. K., and Misko, T. P. (1997). Evidence for the production of peroxynitrite in inflammatory CNS demyelination.
Cui, Y.-Q., Wang, Q., Zhang, D.-M., Wang, J.-Y., Xiao, B., Zheng, Y., et al. (2016). Triptolide rescues spatial memory deficits and amyloid-? Aggregation accompanied by inhibition of inflammatory responses and MAPKs activity in APP/PS1 transgenic mice. Curr. Alzheimer Res. 13, 288–296. doi:10.2174/156720501303160217122803
Cui, Y., Bu, Z., Yu, H., Yan, L., and Feng, J. (2023). Emodin attenuates inflammation and demyelination in experimental autoimmune encephalomyelitis. Neural Regen. Res. 18, 1535–1541. doi:10.4103/1673-5374.358612
Cui, Y., Wu, J., Jung, S. C., Park, D. B., Maeng, Y. H., Hong, J. Y., et al. (2010). Anti-neuroinflammatory activity of nobiletin on suppression of microglial activation. Biol. Pharm. Bull. 33, 1814–1821. doi:10.1248/BPB.33.1814
Domingues, H. S., Portugal, C. C., Socodato, R., and Relvas, J. B. (2016). Corrigendum: oligodendrocyte, astrocyte and microglia crosstalk in myelin development, damage, and repair. Front. Cell Dev. Biol. 4, 79. doi:10.3389/fcell.2016.00079
Dong, H., Li, Pei-nan, Chen, C., Xu, X., Zhang, H., Liu, G., et al. (2019). Sinomenine attenuates cartilage degeneration by regulating miR-223-3p/NLRP3 inflammasome signaling. NLRP3 Inflammasome Signal. 42, 1265–1275. doi:10.1007/s10753-019-00986-3
dos Santos Souza, C., Grangeiro, M. S., Lima Pereira, E. P., dos Santos, C. C., da Silva, A. B., Sampaio, G. P., et al. (2018). Agathisflavone, a flavonoid derived from Poincianella pyramidalis (Tul.), enhances neuronal population and protects against glutamate excitotoxicity. Neurotoxicology 65, 85–97. doi:10.1016/J.NEURO.2018.02.001
Du, R. H., Sun, H. Bin, Hu, Z. L., Lu, M., Ding, J. H., and Hu, G. (2018). Kir6.1/K-ATP channel modulates microglia phenotypes: implication in Parkinson’s disease. Cell Death Dis. 9, 404–413. doi:10.1038/s41419-018-0437-9
El-Shehawy, A. A., Elmetwalli, A., El-Far, A. H., Mosallam, S. A. E.-R., Salama, A. F., Babalghith, A. O., et al. (2023). Thymoquinone, piperine, and sorafenib combinations attenuate liver and breast cancers progression: epigenetic and molecular docking approaches. BMC Complement. Med. Ther. 23, 69. doi:10.1186/s12906-023-03872-6
Elazazy, O., Midan, H. M., Shahin, R. K., Elesawy, A. E., Elballal, M. S., Sallam, A.-A. M., et al. (2023). Long non-coding RNAs and rheumatoid arthritis: pathogenesis and clinical implications. Pathol. Res. Pract. 246, 154512. doi:10.1016/j.prp.2023.154512
Eriksen, J. L., Wszolek, Z., and Petrucelli, L. (2005). Molecular pathogenesis of Parkinson disease. Arch. Neurol. 62, 353–357. doi:10.1001/ARCHNEUR.62.3.353
Fadzil, N. S. M., Sekar, M., Gan, S. H., Bonam, S. R., Wu, Y. S., Vaijanathappa, J., et al. (2021). Chemistry, pharmacology and therapeutic potential of swertiamarin – a promising natural lead for new drug discovery and development. Drug Des. Devel Ther. 15, 2721–2746. doi:10.2147/DDDT.S299753
Fan, J.-Y., Yi, T., Sze-To, C.-M., Zhu, L., Peng, W.-L., Zhang, Y.-Z., et al. (2014). A systematic review of the botanical, phytochemical and pharmacological profile of Dracaena cochinchinensis, a plant source of the ethnomedicine “Dragon”s blood. Molecules 19, 10650–10669. doi:10.3390/molecules190710650
Fan, J., Fong, T., Chen, X., Chen, C., Luo, P., and Xie, H. (2018). Glia maturation factor-β: a potential therapeutic target in neurodegeneration and neuroinflammation. Neuropsychiatr. Dis. Treat. 14, 495–504. doi:10.2147/NDT.S157099
Fan, Z., Brooks, D. J., Okello, A., and Edison, P. (2017). An early and late peak in microglial activation in Alzheimer’s disease trajectory. Brain 140, 792–803. doi:10.1093/BRAIN/AWW349
Fan, Z., Liang, Z., Yang, H., Pan, Y., Zheng, Y., and Wang, X. (2017). Tenuigenin protects dopaminergic neurons from inflammation via suppressing NLRP3 inflammasome activation in microglia. J. Neuroinflammation 14, 256. doi:10.1186/S12974-017-1036-X
Fontes, L. B. A., Dias, D., dos, S., Aarestrup, B. J. V., Aarestrup, F. M., Da Silva Filho, A. A., et al. (2017). β-Caryophyllene ameliorates the development of experimental autoimmune encephalomyelitis in C57BL/6 mice. Biomed. Pharmacother. 91, 257–264. doi:10.1016/J.BIOPHA.2017.04.092
Fu, A. K. Y., Hung, K. W., Yuen, M. Y. F., Zhou, X., Mak, D. S. Y., Chan, I. C. W., et al. (2016). IL-33 ameliorates Alzheimer’s disease-like pathology and cognitive decline. Proc. Natl. Acad. Sci. U. S. A. 113, E2705–E2713. doi:10.1073/pnas.1604032113
Gao, D., Hao, J., Li, B., Zheng, C., Miao, B., Zhang, Li, et al. (2023a). Tetrahydroxy stilbene glycoside ameliorates neuroinflammation for Alzheimer’s disease via cGAS-STING. Eur. J. Pharmacol. 953, 175809. doi:10.1016/j.ejphar.2023.175809
Gao, D., Zheng, C., Hao, J., Yang, C., and Hu, C. (2023b). Icariin ameliorates behavioral deficits and neuropathology in a mouse model of multiple sclerosis. Brain Res. 1804, 148267. doi:10.1016/j.brainres.2023.148267
Geng, X., Zou, Y., Li, S., Qi, R., Yu, H., and Li, J. (2023). MALAT1 mediates α-synuclein expression through miR-23b-3p to induce autophagic impairment and the inflammatory response in microglia to promote apoptosis in dopaminergic neuronal cells. Mediat. Inflamm. 2023, 4477492. doi:10.1155/2023/4477492
Gerogianni, P. S., Chatziathanasiadou, M. V., Diamantis, D. A., Tzakos, A. G., and Galaris, D. (2018). Lipophilic ester and amide derivatives of rosmarinic acid protect cells against H2O2-induced DNA damage and apoptosis: the potential role of intracellular accumulation and labile iron chelation. Redox Biol. 15, 548–556. doi:10.1016/j.redox.2018.01.014
Gharaibeh, A., Maiti, P., Culver, R., Heileman, S., Srinageshwar, B., Story, D., et al. (2020). Solid lipid curcumin particles protect medium spiny neuronal morphology, and reduce learning and memory deficits in the YAC128 mouse model of Huntington’s disease. Int. J. Mol. Sci. 21, 9542. doi:10.3390/IJMS21249542
Ghasemi, F., Bagheri, H., Barreto, G. E., Read, M. I., and Sahebkar, A. (2019). Effects of curcumin on microglial cells. Neurotox. Res. 36, 12–26. doi:10.1007/s12640-019-00030-0
Gibson, J. S., and Claassen, D. O. (2021). State-of-the-art pharmacological approaches to reduce chorea in Huntington’s disease. Expert Opin. Pharmacother. 22, 1015–1024. doi:10.1080/14656566.2021.1876666
Gibson, S. A., and Benveniste, E. N. (2018). Protein kinase CK2: an emerging regulator of immunity. Trends Immunol. 39, 82–85. doi:10.1016/j.it.2017.12.002
Glass, C. K., Saijo, K., Winner, B., Marchetto, M. C., and Gage, F. H. (2010). Mechanisms underlying inflammation in neurodegeneration. Cell 140, 918–934. doi:10.1016/J.CELL.2010.02.016
Go, J., Ha, T. K. Q., Seo, J. Y., Park, T. S., Ryu, Y. K., Park, H. Y., et al. (2018a). Piperlongumine activates Sirtuin1 and improves cognitive function in a murine model of Alzheimer’s disease. J. Funct. Foods 43, 103–111. doi:10.1016/J.JFF.2018.02.002
Go, J., Park, T. S., Han, G. H., Park, H. Y., Ryu, Y. K., Kim, Y. H., et al. (2018b). Piperlongumine decreases cognitive impairment and improves hippocampal function in aged mice. Int. J. Mol. Med. 42, 1875–1884. doi:10.3892/IJMM.2018.3782
Gómez-Jaramillo, L., Cano-Cano, F., González-Montelongo, M. D. C., Campos-Caro, A., Aguilar-Diosdado, M., and Arroba, A. I. (2022). A new perspective on Huntington’s disease: how a neurological disorder influences the peripheral tissues. Int. J. Mol. Sci. 23, 6089. doi:10.3390/ijms23116089
González-Reyes, R. E., Nava-Mesa, M. O., Vargas-Sánchez, K., Ariza-Salamanca, D., and Mora-Muñoz, L. (2017). Involvement of astrocytes in Alzheimer’s disease from a neuroinflammatory and oxidative stress perspective. Front. Mol. Neurosci. 10, 427. doi:10.3389/fnmol.2017.00427
Gu, S. M., Lee, H. P., Ham, Y. W., Son, D. J., Kim, H. Y., Oh, K. W., et al. (2018). Piperlongumine improves lipopolysaccharide-induced amyloidogenesis by suppressing NF-KappaB pathway. Neuromolecular Med. 20, 312–327. doi:10.1007/s12017-018-8495-9
Gumber, K., and Barmota, H. (2023). Species of the genus polygonatum found in the western himalayas. Immun. Boost. Med. Plants West. Himalayas, 371–392. doi:10.1007/978-981-19-9501-9_16
Guo, R., Ren, Q., Tang, Y. X., Zhao, F., Lin, B., Huang, X. X., et al. (2020). Sesquiterpenoids from the roots of Daphne genkwa Siebold et Zucc. With potential anti-inflammatory activity. Phytochemistry 174, 112348. doi:10.1016/J.PHYTOCHEM.2020.112348
Guo, S., Wang, H., and Yin, Y. (2022). Microglia polarization from M1 to M2 in neurodegenerative diseases. Front. Aging Neurosci. 14, 815347. doi:10.3389/fnagi.2022.815347
Guo, Xiaoyuan, Chen, L., and Li, J. (2023). Plantamajoside alleviates substantia nigra damage in Parkinson’s disease mice by inhibiting HDAC2/MAPK signaling and reducing microglia polarization. ACS Chem. Neurosci. 14, 1119–1125. doi:10.1021/acschemneuro.2c00668
Guo, Xin, Li, R., Huang, N., Zhang, T., Li, J., Gong, L., et al. (2023). Efficacy and safety of shouhui tongbian capsules in the treatment of constipation: a systematic review and meta-analysis. Phytomedicine 108, 154541. doi:10.1016/J.PHYMED.2022.154541
Gupta, D. P., Park, S. H., Lee, Y. S., Lee, S., Lim, S., Byun, J., et al. (2023). Daphne genkwa flower extract promotes the neuroprotective effects of microglia. Phytomedicine 108, 154486. doi:10.1016/J.PHYMED.2022.154486
Hansen, D. V., Hanson, J. E., and Sheng, M. (2018). Microglia in Alzheimer’s disease. J. Cell Biol. 217, 459–472. doi:10.1083/JCB.201709069
Hauser, S. L., and Cree, B. A. C. (2020). Treatment of multiple sclerosis: a review. Am. J. Med. 133, 1380–1390. doi:10.1016/J.AMJMED.2020.05.049
Heneka, M. T. (2017). Inflammasome activation and innate immunity in Alzheimer’s disease. Brain Pathol. 27, 220–222. doi:10.1111/BPA.12483
Hickman, S., Izzy, S., Sen, P., Morsett, L., and El Khoury, J. (2018). Microglia in neurodegeneration. Nat. Neurosci. 21 (10 21), 1359–1369. doi:10.1038/s41593-018-0242-x
Hong, H., Lu, X., Lu, Q., Huang, C., and Cui, Z. (2022). Potential therapeutic effects and pharmacological evidence of sinomenine in central nervous system disorders. Front. Pharmacol. 13. doi:10.3389/fphar.2022.1015035
Hu, H., Song, X., Li, Y., Ma, T., Bai, H., Zhao, M., et al. (2021). Emodin protects knee joint cartilage in rats through anti-matrix degradation pathway: an in vitro and in vivo study. Life Sci. 269, 119001. doi:10.1016/j.lfs.2020.119001
Hu, Z., Wang, W., Ling, J., and Jiang, C. (2016). α-Mangostin inhibits α-synuclein-induced microglial neuroinflammation and neurotoxicity. Cell Mol. Neurobiol. 36, 811–820. doi:10.1007/s10571-015-0264-9
Huang, B., Hu, G., Zong, X., Yang, S., He, D., Gao, X., et al. (2023). α-Cyperone protects dopaminergic neurons and inhibits neuroinflammation in LPS-induced Parkinson’s disease rat model via activating Nrf2/HO-1 and suppressing NF-κB signaling pathway. Int. Immunopharmacol. 115, 109698. doi:10.1016/J.INTIMP.2023.109698
Huang, J., Chen, D., Lin, Xinyi, Yang, C., and Lin, Xianzhong (2023). miRNA-124 alleviated memory impairment induced by d-galactose rapidly in male rats via microglia polarization. Hippocampus 33, 96–111. doi:10.1002/hipo.23491
Huang, Y., Lin, H., Chen, Y., and Huang, X. (2020). Pharmacokinetic and bioavailability study of kurarinone in dog plasma by UHPLC–MS/MS. Biomed. Chromatogr. 34, e4945. doi:10.1002/BMC.4945
Huhn, S., Beyer, F., Zhang, R., Lampe, L., Grothe, J., Kratzsch, J., et al. (2018). Effects of resveratrol on memory performance, hippocampus connectivity and microstructure in older adults – a randomized controlled trial. Neuroimage 174, 177–190. doi:10.1016/J.NEUROIMAGE.2018.03.023
Hung, K. C., Huang, H. J., Wang, Y. T., and Lin, A. M. Y. (2016). Baicalein attenuates α-synuclein aggregation, inflammasome activation and autophagy in the MPP+-treated nigrostriatal dopaminergic system in vivo. J. Ethnopharmacol. 194, 522–529. doi:10.1016/J.JEP.2016.10.040
Imran, M., Saeed, F., Hussain, G., Imran, A., Mehmood, Z., Gondal, T. A., et al. (2021). Myricetin: a comprehensive review on its biological potentials. Food Sci. Nutr. 9, 5854–5868. doi:10.1002/FSN3.2513
Ioannidou, K., Anderson, K. I., Strachan, D., Edgar, J. M., and Barnett, S. C. (2014). Astroglial-axonal interactions during early stages of myelination in mixed cultures using in vitro and ex vivo imaging techniques. BMC Neurosci. 15, 59. doi:10.1186/1471-2202-15-59
Ja, K., Zeis, T., Schaeren-wiemers, N., Junker, A., Meer, F., Kramann, N., et al. (2020). Molecular signature of slowly expanding lesions in progressive multiple sclerosis. 2073–2088. doi:10.1093/brain/awaa158
Jang, M., Choi, J. H., Chang, Y., Lee, S. J., Nah, S. Y., and Cho, I. H. (2019). Gintonin, a ginseng-derived ingredient, as a novel therapeutic strategy for Huntington’s disease: activation of the Nrf2 pathway through lysophosphatidic acid receptors. Brain Behav. Immun. 80, 146–162. doi:10.1016/J.BBI.2019.03.001
Jayaraj, R. L., Azimullah, S., Parekh, K. A., Ojha, S. K., and Beiram, R. (2022). Effect of citronellol on oxidative stress, neuroinflammation and autophagy pathways in an in vivo model of Parkinson’s disease. Heliyon 8, e11434. doi:10.1016/J.HELIYON.2022.E11434
Jayaram, S., and Krishnamurthy, P. T. (2021). Role of microgliosis, oxidative stress and associated neuroinflammation in the pathogenesis of Parkinson’s disease: the therapeutic role of Nrf2 activators. Neurochem. Int. 145, 105014. doi:10.1016/J.NEUINT.2021.105014
Jebasingh, D., Venkataraman, S., Jackson, D., and Emerald, B. (2012). Physiochemical and toxicological studies of the medicinal plant Cyperus rotundus L (Cyperaceae). Int. J. Appl. Res. Nat. Prod.
Jeong, K. H., Jeon, M. T., Kim, H. D., Jung, U. J., Jang, M. C., Chu, J. W., et al. (2015). Nobiletin protects dopaminergic neurons in the 1-methyl-4-phenylpyridinium-treated rat model of Parkinson’s disease. 409–414. doi:10.1089/JMF.2014.3241
Jha, M. K., Lee, W. H., and Suk, K. (2016). Functional polarization of neuroglia: implications in neuroinflammation and neurological disorders. Biochem. Pharmacol. 103, 1–16. doi:10.1016/J.BCP.2015.11.003
Ji, J., Xue, T.-F., Guo, X.-D., Yang, J., Guo, R.-B., Wang, J., et al. (2018). Antagonizing peroxisome proliferator-activated receptor γ facilitates M1-to-M2 shift of microglia by enhancing autophagy via the LKB1–AMPK signaling pathway. Aging Cell 17, e12774. doi:10.1111/acel.12774
Jiang, C. P., He, X., Yang, X. L., Zhang, S. L., Li, H., Song, Z. J., et al. (2014). Anti-rheumatoid arthritic activity of flavonoids from Daphne genkwa. Phytomedicine 21, 830–837. doi:10.1016/J.PHYMED.2014.01.009
Jiang, J. M., Zong, Y., Chuang, D. Y., Lei, W., Lu, C. H., Gu, Z., et al. (2015). Effects of elderberry juice from different genotypes on oxidative and inflammatory responses in microglial cells. Acta Hortic. 1061, 281–288. doi:10.17660/ACTAHORTIC.2015.1061.31
Jiang, M.-J., Li, J., Luo, C.-H., Zhu, C., Chen, Z.-J., Bai, W., et al. (2023). Rhynchophylline inhibits methamphetamine dependence via modulating the miR-181a-5p/GABRA1 axis. J. Ethnopharmacol. 314, 116635. doi:10.1016/j.jep.2023.116635
Jin, X., Liu, M. Y., Zhang, D. F., Zhong, X., Du, K., Qian, P., et al. (2019). Natural products as a potential modulator of microglial polarization in neurodegenerative diseases. Pharmacol. Res. 145, 104253. doi:10.1016/J.PHRS.2019.104253
Jing, W., Zhang, X., Zhou, H., Wang, Y., Yang, M., Long, L., et al. (2019). Naturally occurring cassane diterpenoids (CAs) of Caesalpinia: a systematic review of its biosynthesis, chemistry and pharmacology. Fitoterapia 134, 226–249. doi:10.1016/j.fitote.2019.02.023
Kiasalari, Z., Afshin-majd, S., Baluchnejadmojarad, T., and Azadi-ahmadabadi, E. (2021). Sinomenine alleviates murine experimental autoimmune encephalomyelitis model of multiple sclerosis through inhibiting NLRP3 inflammasome. 215–224.
Kim, E. J., Jang, M., Lee, M. J., Choi, J. H., Lee, S. J., Kim, S. K., et al. (2017). Schisandra chinensis stem ameliorates 3-nitropropionic acid-induced striatal toxicity via activation of the Nrf2 pathway and inhibition of the MAPKs and NF-κB pathways. Front. Pharmacol. 8, 673. doi:10.3389/FPHAR.2017.00673
Kim, N., Martínez, C. C., Jang, D. S., Lee, J. K., and Oh, M. S. (2019). Anti-neuroinflammatory effect of Iresine celosia on lipopolysaccharide-stimulated microglial cells and mouse. Biomed. Pharmacother. 111, 1359–1366. doi:10.1016/J.BIOPHA.2019.01.017
Kobayashi, M., and Yamamoto, M. (2005). Molecular mechanisms activating the Nrf2-Keap1 pathway of antioxidant gene regulation. Antioxid. Redox Signal 7, 385–394. doi:10.1089/ARS.2005.7.385
Kumar, S., Kamboj, J., and SumanSharma, S. (2011). Overview for various aspects of the health benefits of piper longum linn. Fruit. J. Acupunct. Meridian Stud. 4, 134–140. doi:10.1016/S2005-2901(11)60020-4
Lee, D. C., Rizer, J., Selenica, M. L. B., Reid, P., Kraft, C., Johnson, A., et al. (2010). LPS- induced inflammation exacerbates phospho-tau pathology in rTg4510 mice. J. Neuroinflammation 7, 56–16. doi:10.1186/1742-2094-7-56
Li, B., Dasgupta, C., Huang, L., Meng, X., and Zhang, L. (2020). MiRNA-210 induces microglial activation and regulates microglia-mediated neuroinflammation in neonatal hypoxic-ischemic encephalopathy. Cell Mol. Immunol. 17, 976–991. doi:10.1038/s41423-019-0257-6
Li, C., Ren, J., Zhang, M., Wang, H., Yi, F., Wu, J., et al. (2022). The heterogeneity of microglial activation and its epigenetic and non-coding RNA regulations in the immunopathogenesis of neurodegenerative diseases. Cell. Mol. Life Sci. 79, 511. doi:10.1007/s00018-022-04536-3
Li, J., Shui, X., Sun, R., Wan, L., Zhang, B., Xiao, B., et al. (2021). Microglial phenotypic transition: signaling pathways and influencing modulators involved in regulation in central nervous system diseases. Front. Cell Neurosci. 15, 736310. doi:10.3389/fncel.2021.736310
Li, Y., Xia, Y., Yin, S., Wan, F., Hu, J., Kou, L., et al. (2021). Targeting microglial α-synuclein/TLRs/NF-kappaB/NLRP3 inflammasome Axis in Parkinson’s disease. Front. Immunol. 12, 719807. doi:10.3389/fimmu.2021.719807
Lim, H. K., Kim, K., Son, Y. K., Nah, S. Y., Ahn, S. M., and Song, M. (2022). Gintonin stimulates dendritic growth in striatal neurons by activating Akt and CREB. Front. Mol. Neurosci. 15, 1014497. doi:10.3389/fnmol.2022.1014497
Lima, S., Victor, C., Amaral, D., and Cleide, S., 2016. Impact of plant-derived flavonoids on neurodegenerative diseases 41–52. doi:10.1007/s12640-016-9600-1
Liu, P., Zhou, Y., Shi, J., Wang, F., Yang, X., Zheng, X., et al. (2023). Myricetin improves pathological changes in 3×Tg-AD mice by regulating the mitochondria-NLRP3 inflammasome-microglia channel by targeting P38 MAPK signaling pathway. Phytomedicine 115, 154801. doi:10.1016/J.PHYMED.2023.154801
Liu, W., Zhang, Q., Zhang, J., Pan, W., Zhao, J., and Xu, Y. (2017). Long non-coding RNA MALAT1 contributes to cell apoptosis by sponging miR-124 in Parkinson disease. Cell Biosci. 7, 19. doi:10.1186/s13578-017-0147-5
Liu, Z. J., Li, Z. H., Liu, L., Tang, W. X., Wang, Y., Dong, M. R., et al. (2016). Curcumin attenuates beta-amyloid-induced neuroinflammation via activation of peroxisome proliferator-activated receptor-gamma function in a rat model of Alzheimer’s disease. Front. Pharmacol. 7, 261. doi:10.3389/fphar.2016.00261
Lu, W., Chen, J.-T., Shi, Y.-F., Chen, M.-S., Wang, P.-P., Zhang, X.-J., et al. (2023). Diversified cassane family diterpenoids from the leaves of Caesalpinia minax exerting anti-neuroinflammatory activity through suppressing MAPK and NF-κB pathways in BV-2 microglia. J. Ethnopharmacol. 315, 116653. doi:10.1016/j.jep.2023.116653
Luo, C., Zou, L., Sun, H., Peng, J., Gao, C., Bao, L., et al. (2020). A Review of the Anti-In fl ammatory Effects of Rosmarinic Acid on In fl ammatory Diseases, 11, 1–11. doi:10.3389/fphar.2020.00153
Ma, C., Lin, W., Liu, Z., Tang, W., Gautam, R., Li, H., et al. (2017). NDR1 protein kinase promotes IL-17- and TNF-α-mediated inflammation by competitively binding TRAF3. EMBO Rep. 18, 586–602. doi:10.15252/EMBR.201642140
Ma, X., Wang, S., Li, C., Jia, X., Wang, T., Leng, Z., et al. (2022). Baicalein inhibits the polarization of microglia/macrophages to the M1 phenotype by targeting STAT1 in EAE mice. Int. Immunopharmacol. 113, 109373. doi:10.1016/j.intimp.2022.109373
Maezawa, I., Zimin, P. I., Wulff, H., and Jin, L. W. (2011). Amyloid-β protein oligomer at low nanomolar concentrations activates microglia and induces microglial neurotoxicity. J. Biol. Chem. 286, 3693–3706. doi:10.1074/jbc.M110.135244
Maiti, P., Paladugu, L., and Dunbar, G. L. (2018). Solid lipid curcumin particles provide greater anti-amyloid, anti-inflammatory and neuroprotective effects than curcumin in the 5xFAD mouse model of Alzheimer’s disease. BMC Neurosci. 19, 7. doi:10.1186/S12868-018-0406-3
Malaguarnera, L. (2019). Influence of resveratrol on the immune response. Nutrients 11 (5), 946. doi:10.3390/nu11050946
Mattson, M. P. (2004). Pathways towards and away from Alzheimer’s disease. Nature 430, 631–639. doi:10.1038/nature02621
Mawuenyega, K. G., Sigurdson, W., Ovod, V., Munsell, L., Kasten, T., Morris, J. C., et al. (2010). Decreased clearance of CNS β-amyloid in Alzheimer’s disease. Science 330, 1774. doi:10.1126/science.1197623
Meyer-Luehmann, M., Spires-Jones, T. L., Prada, C., Garcia-Alloza, M., De Calignon, A., Rozkalne, A., et al. (2008). Rapid appearance and local toxicity of amyloid-β plaques in a mouse model of Alzheimer’s disease. Nature 451, 720–724. doi:10.1038/nature06616
Mikulic-Petkovsek, M., Schmitzer, V., Slatnar, A., Stampar, F., and Veberic, R. (2012). Composition of sugars, organic acids, and total phenolics in 25 wild or cultivated berry species. J. Food Sci. 77, C1064–C1070. doi:10.1111/J.1750-3841.2012.02896.X
Miljković, D., Momčilović, M., Stanojević, Ž., Rašić, D., and Mostarica-Stojković, M. (2011). It is still not for the old iron: adjuvant effects of carbonyl iron in experimental autoimmune encephalomyelitis induction. J. Neurochem. 118, 205–214. doi:10.1111/J.1471-4159.2011.07303.X
Mitra, S., Anjum, J., Muni, M., Das, R., Rauf, A., Islam, F., et al. (2022). Exploring the journey of emodin as a potential neuroprotective agent: novel therapeutic insights with molecular mechanism of action. Biomed. Pharmacother. 149, 112877. doi:10.1016/j.biopha.2022.112877
Moghaddam, M. H., Bayat, A. H., Eskandari, N., Abdollahifar, M. amin, Fotouhi, F., Forouzannia, A., et al. (2021). Elderberry diet ameliorates motor function and prevents oxidative stress-induced cell death in rat models of Huntington disease. Brain Res. 1762, 147444. doi:10.1016/J.BRAINRES.2021.147444
Moudgil, K. D., and Venkatesha, S. H. (2023). The anti-inflammatory and immunomodulatory activities of natural products to control autoimmune inflammation. Int. J. Mol. Sci. 24, 95. doi:10.3390/ijms24010095
Moussa, C., Hebron, M., Huang, X., Ahn, J., Rissman, R. A., Aisen, P. S., et al. (2017). Resveratrol regulates neuro-inflammation and induces adaptive immunity in Alzheimer’s disease. J. Neuroinflammation 14, 1–10. doi:10.1186/s12974-016-0779-0
Nayak, A., Ansar, R., Verma, S. K., Bonifati, D. M., and Kishore, U. (2011). Huntington’s disease: an immune perspective. Neurol. Res. Int. 2011, 563784. doi:10.1155/2011/563784
Nguyen, T., Nioi, P., and Pickett, C. B. (2009). The Nrf2-antioxidant response element signaling pathway and its activation by oxidative stress. J. Biol. Chem. 284, 13291–13295. doi:10.1074/JBC.R900010200
Nhung Nguyen, T. P., Kumar, M., Fedele, E., Bonanno, G., and Bonifacino, T. (2022). MicroRNA alteration, application as biomarkers, and therapeutic approaches in neurodegenerative diseases. Int. J. Mol. Sci. 23, 4718. doi:10.3390/ijms23094718
Nie, J., Zhou, M., Lü, C., Hu, X., Wan, B., Yang, B., et al. (2012). Effects of triptolide on the synaptophysin expression of hippocampal neurons in the AD cellular model. Int. Immunopharmacol. 13, 175–180. doi:10.1016/J.INTIMP.2012.03.021
Nitric, I., Synthase, O., Kim, S. F., Huri, D. A., and Snyder, S. H. (2005). Supporting online material for 1966. doi:10.1126/science.1119407
O’Loughlin, E., Madore, C., Lassmann, H., and Butovsky, O. (2018). Microglial phenotypes and functions in multiple sclerosis. Cold Spring Harb. Perspect. Med. 8, a028993. doi:10.1101/CSHPERSPECT.A028993
Orihuela, R., McPherson, C. A., and Harry, G. J. (2016). Microglial M1/M2 polarization and metabolic states. Br. J. Pharmacol. 173, 649–665. doi:10.1111/bph.13139
Ospondpant, D., Gao, X., Dong, T. T., and Tsim, K. W. K. (2022). Dracaena cochinchinensis stemwood extracts inhibit amyloid-β fibril formation and promote neuronal cell differentiation. Front. Pharmacol. 13, 943638. doi:10.3389/fphar.2022.943638
Ospondpant, D., Xia, Y., Lai, Q. W. S., Yuen, G. K.-W., Yang, M., Chanthanam, K., et al. (2023). The extracts of Dracaena cochinchinensis stemwood suppress inflammatory response and phagocytosis in lipopolysaccharide-activated microglial cells. Phytomedicine 118, 154936. doi:10.1016/j.phymed.2023.154936
Park, S. Y., Jin, M. L., Kim, Y. H., Kim, Y., and Lee, S. J. (2012a). Anti-inflammatory effects of aromatic-turmerone through blocking of NF-κB, JNK, and p38 MAPK signaling pathways in amyloid β-stimulated microglia. Int. Immunopharmacol. 14, 13–20. doi:10.1016/J.INTIMP.2012.06.003
Park, S. Y., Kim, Y. H., Kim, Y., and Lee, S. J. (2012b). Aromatic-turmerone’s anti-inflammatory effects in microglial cells are mediated by protein kinase A and heme oxygenase-1 signaling. Neurochem. Int. 61, 767–777. doi:10.1016/J.NEUINT.2012.06.020
Petković, F., Blaževski, J., Momčilović, M., Mostarica Stojkovic, M., and Miljković, D. (2013). Nitric oxide inhibits CXCL12 expression in neuroinflammation. Immunol. Cell Biol. 91, 427–434. doi:10.1038/ICB.2013.23
Porru, D., Franco, C. Di, Bobbi, V., Tinelli, C., and Rovereto, B. (2017). A pilot study of clinical results of Odyliresin (Iresine celosia) in symptomatic benign prostatic hyperplasia. J. Clin. Urol. 10, 464–470. doi:10.1177/2051415817698289
Prince, M., Ali, G. C., Guerchet, M., Prina, A. M., Albanese, E., and Wu, Y. T. (2016). Recent global trends in the prevalence and incidence of dementia, and survival with dementia. Alzheimers Res. Ther. 8, 23–13. doi:10.1186/s13195-016-0188-8
Qi, Y., Ji, X. F., Chi, T. Y., Liu, P., Jin, G., Xu, Q., et al. (2018). Xanthoceraside attenuates amyloid β peptide1-42-induced memory impairments by reducing neuroinflammatory responses in mice. Eur. J. Pharmacol. 820, 18–30. doi:10.1016/J.EJPHAR.2017.11.045
Qi, Y., Zou, L. B., Wang, L. H., Jin, G., Pan, J. J., Chi, T. Y., et al. (2013). Xanthoceraside inhibits pro-inflammatory cytokine expression in aβ25–35/IFN-γ–Stimulated microglia through the TLR2 receptor, MyD88, nuclear factor-κb, and mitogen-activated protein kinase signaling pathways. J. Pharmacol. Sci. 122, 305–317. doi:10.1254/JPHS.13031FP
Qin, D. P., Feng, X. L., Zhang, W. Y., Gao, H., Cheng, X. R., Zhou, W. X., et al. (2017). Anti-neuroinflammatory asarone derivatives from the rhizomes of Acorus tatarinowii. RSC Adv. 7, 8512–8520. doi:10.1039/C6RA27786A
Qin, J., Ma, Z., Chen, X., and Shu, S. (2023). Microglia activation in central nervous system disorders: a review of recent mechanistic investigations and development efforts. Front. Neurol. 14, 348. doi:10.3389/fneur.2023.1103416
Qin, S., Yang, C., Huang, W., Du, S., Mai, H., Xiao, J., et al. (2018). Sulforaphane attenuates microglia-mediated neuronal necroptosis through down-regulation of MAPK/NF-κB signaling pathways in LPS-activated BV-2 microglia. Pharmacol. Res. 133, 218–235. doi:10.1016/J.PHRS.2018.01.014
Qiu, J., Chen, Y., Zhuo, J., Zhang, L., Liu, J., Wang, B., et al. (2022). Urolithin A promotes mitophagy and suppresses NLRP3 inflammasome activation in lipopolysaccharide-induced BV2 microglial cells and MPTP-induced Parkinson’s disease model. Neuropharmacology 207, 108963. doi:10.1016/J.NEUROPHARM.2022.108963
Rainey-Smith, S. R., Brown, B. M., Sohrabi, H. R., Shah, T., Goozee, K. G., Gupta, V. B., et al. (2016). Curcumin and cognition: a randomised, placebo-controlled, double-blind study of community-dwelling older adults. Br. J. Nutr. 115, 2106–2113. doi:10.1017/S0007114516001203
Ravn, H. W., Mondolot, L., Kelly, M. T., and Lykke, A. M. (2015). Plantamajoside — a current review. Phytochem. Lett. 12, 42–53. doi:10.1016/J.PHYTOL.2015.02.002
Ren, M., Guo, Y., Wei, X., Yan, S., Qin, Y., Zhang, X., et al. (2018). TREM2 overexpression attenuates neuroinflammation and protects dopaminergic neurons in experimental models of Parkinson’s disease. Exp. Neurol. 302, 205–213. doi:10.1016/J.EXPNEUROL.2018.01.016
Ringman, J. M., Frautschy, S. A., Teng, E., Begum, A. N., Bardens, J., Beigi, M., et al. (2012). Oral curcumin for Alzheimer’s disease: tolerability and efficacy in a 24-week randomized, double blind, placebo-controlled study. Alzheimers Res. Ther. 4, 43. doi:10.1186/ALZRT146
Rivera, D. S., Lindsay, C., Codocedo, J. F., Morel, I., Pinto, C., Cisternas, P., et al. (2016). Andrographolide recovers cognitive impairment in a natural model of Alzheimer’s disease (Octodon degus). Neurobiol. Aging 46, 204–220. doi:10.1016/J.NEUROBIOLAGING.2016.06.021
Rossi, M., Petralla, S., Protti, M., Baiula, M., Kobrlova, T., Soukup, O., et al. (2020). α-Linolenic acid-valproic acid conjugates: toward single-molecule polypharmacology for multiple sclerosis. ACS Med. Chem. Lett. 11, 2406–2413. doi:10.1021/acsmedchemlett.0c00375
Ruan, Q.-F., Zhou, X.-H., Jiang, S.-Q., Yang, B., Jin, J., Cui, H., et al. (2019). Caesalminaxins O-T, cassane diterpenoids from the seeds of Caesalpinia minax and their anti-inflammation. Fitoterapia 134, 50–57. doi:10.1016/j.fitote.2019.02.004
Ruby, A. J., Kuttan, G., Dinesh Babu, K., Rajasekharan, K. N., and Kuttan, R. (1995). Anti-tumour and antioxidant activity of natural curcuminoids. Cancer Lett. 94, 79–83. doi:10.1016/0304-3835(95)03827-J
Saitgareeva, A. R., Bulygin, K. V., Gareev, I. F., Beylerli, O. A., and Akhmadeeva, L. R. (2020). The role of microglia in the development of neurodegeneration. Neurol. Sci. 41, 3609–3615. doi:10.1007/s10072-020-04468-5
Salama, R. M., Abbas, S. S., Darwish, S. F., Sallam, A. A., Elmongy, N. F., and El Wakeel, S. A. (2023). Regulation of NOX/p38 MAPK/PPARα pathways and miR-155 expression by boswellic acids reduces hepatic injury in experimentally-induced alcoholic liver disease mouse model: novel mechanistic insight. Arch. Pharm. Res. 46, 323–338. doi:10.1007/S12272-023-01441-6
Salama, R. M., Darwish, S. F., El Shaffei, I., Elmongy, N. F., Fahmy, N. M., Afifi, M. S., et al. (2022). Morus macroura miq. Fruit extract protects against acetic acid-induced ulcerative colitis in rats: novel mechanistic insights on its impact on miRNA-223 and on the tnfα/nf?b/NLRP3 inflammatory axis. Food Chem. Toxicol. 165, 113146. doi:10.1016/j.fct.2022.113146
Sandhir, R., Yadav, A., Mehrotra, A., Sunkaria, A., Singh, A., and Sharma, S. (2014). Curcumin nanoparticles attenuate neurochemical and neurobehavioral deficits in experimental model of Huntington’s disease. Neuromolecular Med. 16, 106–118. doi:10.1007/s12017-013-8261-y
Santos, P. L., Matos, J. P. S. C. F., Picot, L., Almeida, J. R. G. S., Quintans, J. S. S., and Quintans-Júnior, L. J. (2019). Citronellol, a monoterpene alcohol with promising pharmacological activities - a systematic review. Food Chem. Toxicol. 123, 459–469. doi:10.1016/J.FCT.2018.11.030
Sarlus, H., and Heneka, M. T. (2017). Microglia in Alzheimer’s disease. J. Clin. Invest. 127, 3240–3249. doi:10.1172/JCI90606
Seo, W. G., Pae, H. O., Oh, G. S., Chai, K. Y., Kwon, T. O., Yun, Y. G., et al. (2001). Inhibitory effects of methanol extract of Cyperus rotundus rhizomes on nitric oxide and superoxide productions by murine macrophage cell line, RAW 264.7 cells. J. Ethnopharmacol. 76, 59–64. doi:10.1016/S0378-8741(01)00221-5
Serrano, F. G., Tapia-Rojas, C., Carvajal, F. J., Hancke, J., Cerpa, W., and Inestrosa, N. C. (2014). Andrographolide reduces cognitive impairment in young and mature AβPPswe/PS-1 mice. Mol. Neurodegener. 9, 61. doi:10.1186/1750-1326-9-61
Shadfar, S., Khanal, S., Bohara, G., Kim, G., Sadigh-Eteghad, S., Ghavami, S., et al. (2022). Methanolic extract of Boswellia serrata gum protects the nigral dopaminergic neurons from rotenone-induced neurotoxicity. Mol. Neurobiol. 59, 5874–5890. doi:10.1007/S12035-022-02943-Y
Sharma, M., Malim, F. M., Goswami, A., Sharma, N., Juvvalapalli, S. S., Chatterjee, S., et al. (2023). Neuroprotective effect of swertiamarin in a rotenone model of Parkinson’s disease: role of neuroinflammation and alpha-synuclein accumulation. ACS Pharmacol. Transl. Sci. 6, 40–51. doi:10.1021/acsptsci.2c00120
Shemer, A., and Jung, S. (2015). Differential roles of resident microglia and infiltrating monocytes in murine CNS autoimmunity. Seminars Immunopathol. 37, 613–623. doi:10.1007/S00281-015-0519-Z
Shen, H., Pei, H., Zhai, L., Guan, Q., and Wang, G. (2023). Aurantiamide suppresses the activation of NLRP3 inflammasome to improve the cognitive function and central inflammation in mice with Alzheimer’s disease. CNS Neurosci. Ther. 29, 1075–1085. doi:10.1111/CNS.14082
Siddiqui, M. Z. (2011). Boswellia serrata, A potential antiinflammatory agent: an overview. Indian J. Pharm. Sci. 73, 255–261. doi:10.4103/0250-474X.93507
Singh, A., D’Amico, D., Andreux, P. A., Dunngalvin, G., Kern, T., Blanco-Bose, W., et al. (2021). Direct supplementation with Urolithin A overcomes limitations of dietary exposure and gut microbiome variability in healthy adults to achieve consistent levels across the population. Eur. J. Clin. Nutr. 76 (2), 297–308. doi:10.1038/s41430-021-00950-1
Singh, S. P., WahajuddinTewari, D., Patel, K., and Jain, G. K. (2011). Permeability determination and pharmacokinetic study of nobiletin in rat plasma and brain by validated high-performance liquid chromatography method. Fitoterapia 82, 1206–1214. doi:10.1016/J.FITOTE.2011.08.010
Small, G. W., Siddarth, P., Li, Z., Miller, K. J., Ercoli, L., Emerson, N. D., et al. (2018). Memory and brain amyloid and tau effects of a bioavailable form of curcumin in non-demented adults: a double-blind, placebo-controlled 18-month trial. Am. J. Geriatric Psychiatry 26, 266–277. doi:10.1016/j.jagp.2017.10.010
Snaidero, N., Velte, C., Kursula, P., Simons, M., Snaidero, N., Velte, C., et al. (2017). Antagonistic functions of MBP and CNP establish cytosolic channels in CNS myelin report antagonistic functions of MBP and CNP establish cytosolic channels in CNS myelin. 314–323. doi:10.1016/j.celrep.2016.12.053
Son, I. H., Chung, I. M., Lee, S. I., Yang, H. D., and Moon, H. I. (2007). Pomiferin, histone deacetylase inhibitor isolated from the fruits of Maclura pomifera. Bioorg Med. Chem. Lett. 17, 4753–4755. doi:10.1016/J.BMCL.2007.06.060
Song, G. J., and Suk, K. (2017). Pharmacological modulation of functional phenotypes of microglia in neurodegenerative diseases. Front. Aging Neurosci. 9, 139. doi:10.3389/fnagi.2017.00139
Song, X., Tan, L., Wang, M., Ren, C., Guo, C., Yang, B., et al. (2021). Myricetin: a review of the most recent research. Biomed. Pharmacother. 134, 111017. doi:10.1016/j.biopha.2020.111017
Spasojevic, I., and Miljkovic, D. (2013). Multiple sclerosis: molecular mechanisms and therapeutic opportunities. Antioxid. Redox Signal. 19, 2286–2334. doi:10.1089/ars.2012.5068
Stegnjai, G., Lazarevi, M., Jevti, B., Stanisavljevi, S., Dimitrijevi, M., and Tzakos, A. G. (2022a). Phenethyl ester rosmarinic acid ameliorates Exp. autoimmune Enceph. 252, 9–19. doi:10.1016/j.imlet.2022.09.006
Stegnjai, G., Tsiailanis, A. D., and Lazarevi, M. (2022b). Phenethyl ester of gallic acid ameliorates experimental autoimmune encephalomyelitis.
Suhas, R., and Channe Gowda, D. (2012). Structure-based rationale design and synthesis of aurantiamide acetate analogues – towards a new class of potent analgesic and anti-inflammatory agents. Chem. Biol. Drug Des. 79, 850–862. doi:10.1111/J.1747-0285.2012.01331.X
Sun, C. P., Zhou, J. J., Yu, Z. L., Huo, X. K., Zhang, J., Morisseau, C., et al. (2022). Kurarinone alleviated Parkinson’s disease via stabilization of epoxyeicosatrienoic acids in animal model. Proc. Natl. Acad. Sci. U. S. A. 119, e2118818119. doi:10.1073/pnas.2118818119
Sun, Y.-M., and Chen, Y.-Q. (2020). Principles and innovative technologies for decrypting noncoding RNAs: from discovery and functional prediction to clinical application. J. Hematol. Oncol. 13, 109. doi:10.1186/s13045-020-00945-8
Surendran, S., Qassadi, F., Surendran, G., Lilley, D., and Heinrich, M. (2021). Myrcene—what are the potential health benefits of this flavouring and aroma agent? Front. Nutr. 8, 699666. doi:10.3389/fnut.2021.699666
Suzuki, M., Yamamoto, Y., Nishijima-Matsunobu, A., Kawasaki, Y., Shibata, H., and Omori, Y. (2023). A curcumin analogue GO-Y030 depletes cancer stem cells by inhibiting the interaction between the HSP70/HSP40 complex and its substrates. FEBS Open Bio 13, 434–446. doi:10.1002/2211-5463.13550
Taheri, Y., Suleria, H. A. R., Martins, N., Sytar, O., Beyatli, A., Yeskaliyeva, B., et al. (2020). Myricetin bioactive effects: moving from preclinical evidence to potential clinical applications. BMC Complement. Med. Ther. 20, 241. doi:10.1186/s12906-020-03033-z
Tang, Y. (2018). Editorial: microglial polarization in the pathogenesis and therapeutics of neurodegenerative diseases. Front. Aging Neurosci. 10, 154. doi:10.3389/fnagi.2018.00154
Tang, Y., and Le, W. (2016). Differential roles of M1 and M2 microglia in neurodegenerative diseases. Mol. Neurobiol. 53, 1181–1194. doi:10.1007/s12035-014-9070-5
Tang, Y., Li, T., Li, J., Yang, J., Liu, H., Zhang, X. J., et al. (2013). Jmjd3 is essential for the epigenetic modulation of microglia phenotypes in the immune pathogenesis of Parkinson’s disease. Cell Death Differ. 21 (3), 369–380. doi:10.1038/cdd.2013.159
Tarkowski, E., Andreasen, N., Tarkowski, A., and Blennow, K. (2003). Intrathecal inflammation precedes development of Alzheimer’s disease. J. Neurol. Neurosurg. Psychiatry 74, 1200–1205. doi:10.1136/JNNP.74.9.1200
Turner, R. S., Thomas, R. G., Craft, S., Van Dyck, C. H., Mintzer, J., Reynolds, B. A., et al. (2015). A randomized, double-blind, placebo-controlled trial of resveratrol for Alzheimer disease. Neurology 85, 1383–1391. doi:10.1212/WNL.0000000000002035
Tyler, V. E. (1996). Encyclopedia of Common Natural Ingredients Used in Food, Drugs, and Cosmetics, Second Edition By Albert Y. Leung (Consultant, Natural Products) and Steven Foster (Consultant, Medicinal and Aromatic Plants). John Wiley and Sons, New York, NY. 1996. xxxviii + 649 pp. 17.5 × 25 cm. $150.00. ISBN 0-532-42154-5. J. Nat. Prod. 59, 1212–1213. doi:10.1021/NP960461R
Vaarmann, A., Gandhi, S., and Abramov, A. Y. (2010). Dopamine induces Ca2+ signaling in astrocytes through reactive oxygen species generated by monoamine oxidase. J. Biol. Chem. 285, 25018–25023. doi:10.1074/jbc.M110.111450
Wagdy, R., Abdel-Kader, R. M., El-Khatib, A. H., Linscheid, M. W., Handoussa, H., and Hamdi, N. (2023). Origanum majorana L. protects against neuroinflammation-mediated cognitive impairment: a phyto-pharmacological study. BMC Complement. Med. Ther. 23, 165. doi:10.1186/s12906-023-03994-x
Walker, D. G., Link, J., Lue, L.-F., Dalsing-Hernandez, J. E., and Boyes, B. E. (2006). Gene expression changes by amyloid β peptide-stimulated human postmortem brain microglia identify activation of multiple inflammatory processes. J. Leukoc. Biol. 79, 596–610. doi:10.1189/JLB.0705377
Wang, C., Dai, S., Gong, L., Fu, K., Ma, C., Liu, Y., et al. (2022). A Review of Pharmacology, Toxicity and Pharmacokinetics of 2,3,5,4′-Tetrahydroxystilbene-2-O-β-D-Glucoside. Front. Pharmacol. 12, 791214. doi:10.3389/fphar.2021.791214
Wang, G. Q., Li, D. Di, Huang, C., Lu, D. S., Zhang, C., Zhou, S. Y., et al. (2018). Icariin reduces dopaminergic neuronal loss and microglia-mediated inflammation in vivo and in vitro. Front. Mol. Neurosci. 10, 441. doi:10.3389/fnmol.2017.00441
Wang, K., Shi, Y., Liu, W., Liu, S., and Sun, M. Z. (2021). Taurine improves neuron injuries and cognitive impairment in a mouse Parkinson’s disease model through inhibition of microglial activation. Neurotoxicology 83, 129–136. doi:10.1016/J.NEURO.2021.01.002
Wang, X., Li, M., Cao, Y., Wang, J., Zhang, H., Zhou, X., et al. (2017). Tenuigenin inhibits LPS-induced inflammatory responses in microglia via activating the Nrf2-mediated HO-1 signaling pathway. Eur. J. Pharmacol. 809, 196–202. doi:10.1016/J.EJPHAR.2017.05.004
Wang, Y. T., Wang, H., Ren, W. J., Dai, X. L., Huo, Q., Wang, S., et al. (2023). 3,6’-Disinapoylsucrose alleviates the amyloid precursor protein and lipopolysaccharide induced cognitive dysfunction through upregulation of the TrkB/BDNF pathway. J. Asian Nat. Prod. Res. 25, 387–402. doi:10.1080/10286020.2022.2069565
Wild, E. J., and Tabrizi, S. J. (2014). Targets for future clinical trials in Huntington’s disease: what’s in the pipeline? Mov. Disord. 29, 1434–1445. doi:10.1002/MDS.26007
Wohl, S. G., and Reh, T. A. (2016). The microRNA expression profile of mouse Müller glia in vivo and in vitro. Sci. Rep. 6, 35423. doi:10.1038/srep35423
Wu, A. G., Zhou, X. G., Qiao, G., Yu, L., Tang, Y., Yan, L., et al. (2021). Targeting microglial autophagic degradation in NLRP3 inflammasome-mediated neurodegenerative diseases. Ageing Res. Rev. 65, 101202. doi:10.1016/J.ARR.2020.101202
Wu, C., Cai, X. Q., Chang, Y., Chen, C. H., Ho, T. J., Lai, S. C., et al. (2019). Rapid identification of dragon blood samples from Daemonorops draco, Dracaena cinnabari and Dracaena cochinchinensis by MALDI-TOF mass spectrometry. Phytochem. Anal. 30, 720–726. doi:10.1002/PCA.2852
Xue, X., Quan, Y., Gong, L., Gong, X., and Li, Y. (2020). A review of the processed Polygonum multiflorum (Thunb.) for hepatoprotection: clinical use, pharmacology and toxicology. J. Ethnopharmacol. 261, 113121. doi:10.1016/j.jep.2020.113121
Yan, L.-J., Xiao, M., Chen, R., and Cai, Z. (2013). Metabolic Dysfunction of Astrocyte: an Initiating Factor in Beta-amyloid Pathology? Aging Neurodegener. 1, 7–14.
Yang, G., Wang, Y., Tian, J., and Liu, J. P. (2013). Huperzine A for Alzheimer’s Disease: a Systematic Review and Meta-Analysis of Randomized Clinical Trials. PLoS One 8, e74916. doi:10.1371/JOURNAL.PONE.0074916
Yang, H. (2021). LncRNA MALAT1 potentiates inflammation disorder in Parkinson’s disease. Int. J. Immunogenet 48, 419–428. doi:10.1111/iji.12549
Yang, H. M., Yang, S., Huang, S. S., Tang, B. S., and Guo, J. F. (2017). Microglial activation in the pathogenesis of Huntington’s Disease. Front. Aging Neurosci. 9, 193–199. doi:10.3389/fnagi.2017.00193
Yang, R., Liu, S., Zhou, J., Bu, S., and Zhang, J. (2017). Andrographolide attenuates microglia-mediated Aβ neurotoxicity partially through inhibiting NF-κB and JNK MAPK signaling pathway. 276–284. doi:10.1080/08923973.2017.1344989
Yang, Y., and Zhang, Z. (2020). Microglia and Wnt Pathways: prospects for Inflammation in Alzheimer’s Disease. Front. Aging Neurosci. 12, 110. doi:10.3389/FNAGI.2020.00110
Yao, K., and Zu, H. (2020). Microglial polarization: novel therapeutic mechanism against Alzheimer’s disease. Inflammopharmacology 28, 95–110. doi:10.1007/s10787-019-00613-5
Yuan, Y., Zhang, Y., He, X., and Fan, S. (2018). Protective Effects of Sinomenine on CFA-Induced Inflammatory Pain in Rats. Med. Sci. Monit. 24, 2018–2024. doi:10.12659/MSM.906726
Zaheer, A., Zaheer, S., Sahu, S. K., Yang, B., and Lim, R. (2007). Reduced severity of experimental autoimmune encephalomyelitis in GMF-deficient mice. Neurochem. Res. 32, 39–47. doi:10.1007/s11064-006-9220-x
Zaichick, S. V., McGrath, K. M., and Caraveo, G. (2017). The role of Ca2+ signaling in Parkinson’s disease. Dis. Model. Mech. 10, 519–535. doi:10.1242/DMM.028738
Zakay-Rones, Z., Varsano, N., Zlotnik, M., Manor, O., Regev, L., Schlesinger, M., et al. (2007). Inhibition of Several Strains of Influenza Virus in vitro and Reduction of Symptoms by an Elderberry Extract (Sambucus nigra L.) during an Outbreak of Influenza B Panama. 361–369. doi:10.1089/ACM.1995.1.361
Zeng, K. W., Fu, H., Liu, G. X., and Wang, X. M. (2010). Icariin attenuates lipopolysaccharide-induced microglial activation and resultant death of neurons by inhibiting TAK1/IKK/NF-kappaB and JNK/p38 MAPK pathways. Int. Immunopharmacol. 10, 668–678. doi:10.1016/J.INTIMP.2010.03.010
Zhang, Di, Lu, R., Wang, M., Ji, J., Zhang, S., Wang, S., et al. (2023). Effects of Banxia Xiexin Decoction on apoptosis of interstitial cells of Cajal via regulation of MiR-451-5p: an in vivo and in vitro study. J. Ethnopharmacol. 314, 116606. doi:10.1016/J.JEP.2023.116606
Zhang, H., Wang, D., Sun, J., Wang, Y., Wu, S., and Wang, J. (2022). Huperzine — A improved animal behavior in cuprizone-induced mouse model by alleviating demyelination and neuroinflammation.
Zhang, J., Xie, J., Liang, Y., Li, Y., Zhang, Y., Wang, C., et al. (2022). Anxiolytic effects, metabolism and plasma pharmacokinetics of 3, 6′ -disinapoylsucrose. Biomed. Pharmacother. 149, 112913. doi:10.1016/J.BIOPHA.2022.112913
Zhang, J., Zhang, R., Zhan, Z., Li, X., Zhou, F., Xing, A., et al. (2017). Beneficial Effects of Sulforaphane Treatment in Alzheimer’s Disease May Be Mediated through Reduced HDAC1/3 and Increased P75NTR Expression. Front. Aging Neurosci. 9, 121. doi:10.3389/FNAGI.2017.00121
Zhang, Li, Yuan, Y., Tong, Q., Jiang, S., Xu, Q., Ding, J., et al. (2016). Reduced plasma taurine level in Parkinson’s disease: association with motor severity and levodopa treatment. Int. J. Neurosci. 126, 630–636. doi:10.3109/00207454.2015.1051046
Zhang, M., Li, N., Cai, R., Gu, J., Xie, F., Wei, H., et al. (2021). Rosmarinic acid protects mice from imiquimod induced psoriasis-like skin lesions by inhibiting the IL-23/Th17 axis via regulating Jak2/Stat3 signaling pathway. Phytotherapy Res. 35, 4526–4537. doi:10.1002/PTR.7155
Zhang, P., Wu, W., Chen, Q., and Chen, M. (2019). Non-Coding RNAs and their Integrated Networks. J. Integr. Bioinform 16, 20190027. doi:10.1515/jib-2019-0027
Zhang, Y.-J., Zhu, W.-K., Qi, F.-Y., and Che, F.-Y. (2022). CircHIPK3 promotes neuroinflammation through regulation of the miR-124-3p/STAT3/NLRP3 signaling pathway in Parkinson’s disease. Adv. Clin. Exp. Med. 32 (0–0), 315–329. doi:10.17219/acem/154658
Zhao, S., Ma, L., Chu, Z., Xu, H., Wu, W., and Liu, F. (2017). Regulation of microglial activation in stroke. Acta Pharmacol. Sin. 38, 445–458. doi:10.1038/aps.2016.162
Zhao, Y., Sang, Y., Sun, Y., and Wu, J. (2022). Pomiferin Exerts Antineuroinflammatory Effects through Activating Akt/Nrf2 Pathway and Inhibiting NF- κ B Pathway. Mediat. Inflamm. 2022, 5824657. doi:10.1155/2022/5824657
Zheng, X., Sun, K., Liu, Y., Yin, X., Zhu, H., Yu, F., et al. (2023). Resveratrol-loaded macrophage exosomes alleviate multiple sclerosis through targeting microglia. J. Control. Release 353, 675–684. doi:10.1016/J.JCONREL.2022.12.026
Zhou, H., Zhao, J., Liu, C., Zhang, Z., Zhang, Y., and Meng, D. (2022). Xanthoceraside exerts anti-Alzheimer’s disease effect by remodeling gut microbiota and modulating microbial-derived metabolites level in rats. Phytomedicine 98, 153937. doi:10.1016/J.PHYMED.2022.153937
Zhu, M., Yuan, K., Lu, Q., Zhu, Q., Zhang, S., Li, X., et al. (2019). Emodin ameliorates rheumatoid arthritis by promoting neutrophil apoptosis and inhibiting neutrophil extracellular trap formation. Mol. Immunol. 112, 188–197. doi:10.1016/j.molimm.2019.05.010
Zielińska-Wasielica, J., Olejnik, A., Kowalska, K., Olkowicz, M., and Dembczyński, R. (2019). Elderberry (Sambucus nigra L.) Fruit Extract Alleviates Oxidative Stress, Insulin Resistance, and Inflammation in Hypertrophied 3T3-L1 Adipocytes and Activated RAW 264.7 Macrophages. Foods 8, 326. doi:10.3390/FOODS8080326
Glossary
Keywords: microglia, M1/M2 pathway, neurodegeneration, nutraceuticals, aging diseases
Citation: Darwish SF, Elbadry AMM, Elbokhomy AS, Salama GA and Salama RM (2023) The dual face of microglia (M1/M2) as a potential target in the protective effect of nutraceuticals against neurodegenerative diseases. Front. Aging 4:1231706. doi: 10.3389/fragi.2023.1231706
Received: 30 May 2023; Accepted: 23 August 2023;
Published: 06 September 2023.
Edited by:
Lukasz Ciesla, University of Alabama, United StatesReviewed by:
Marcella Reale, University of Studies G. d'Annunzio Chieti and Pescara, ItalyAmala Soumyanath, Oregon Health and Science University, United States
Copyright © 2023 Darwish, Elbadry, Elbokhomy, Salama and Salama. This is an open-access article distributed under the terms of the Creative Commons Attribution License (CC BY). The use, distribution or reproduction in other forums is permitted, provided the original author(s) and the copyright owner(s) are credited and that the original publication in this journal is cited, in accordance with accepted academic practice. No use, distribution or reproduction is permitted which does not comply with these terms.
*Correspondence: Rania M. Salama, cmFuaWEuc2FsYW1hQG1pdWVneXB0LmVkdS5lZw==