- 1Instituto de Histología y Embriología (IHEM) “Dr. Mario H. Burgos” CONICET, Universidad Nacional de Cuyo Mendoza, Mendoza, Argentina
- 2Departamento Bases Científicas en Salud-Facultad de Ciencias Médicas, Facultad de Medicina, Biología Celular y Molecular, Universidad Nacional de Cuyo, Mendoza, Argentina
- 3Departamento de Biología, Facultad de Ciencias Exactas y Naturales, Universidad Nacional de Cuyo, Mendoza, Argentina
- 4Facultad de Odontología, Microbiología, Parasitología e Inmunología, Universidad Nacional de Cuyo, Mendoza, Argentina
Senescent cells accumulate in multicellular animals with aging, resulting in organ or tissue dysfunction. These alterations increase the incidence of a variety of illnesses, including infectious diseases, and, in certain instances, its severity. In search of a rationale for this phenomenon, we focused on the endophagocytic pathway in senescent cells. We first described the endocytic vesicle populations at different stages of maturation using confocal microscopy. There was an increase in the number of vacuoles per cell, which was partially explained by an increase in cell size. No changes in vesicle maturation or degradation capacities were determined by microscopy or Western blot assays. Also, we studied the internalization of various endophagocytic cargoes in senescent cells and observed only a decrease in the intracellular recovery of bacteria such as Staphylococcus aureus. Afterwards, we studied the intracellular traffic of S. aureus, and observed no differences in the infection between control and senescent cells. In addition we quantified the recovery of bacteria from control and senescent cells infected in the presence of several inhibitors of endophagosomal maturation, and no changes were observed. These results suggest that bacterial internalization is affected in senescent cells. Indeed, we confirmed this hypothesis by determining minor bacterial adherence and internalization by confocal microscopy. Furthermore, it is important to highlight that we found very similar results with cells from aged animals, specifically BMDMs. This alteration in senescent cells enlightens the diminished bacterial clearance and may be a factor that increases the propensity to suffer severe infectious conditions in the elderly.
1 Introduction
Multiple defense mechanisms exist in multicellular organisms to prevent the spread of single-cell DNA damage. Among them, cell death by apoptosis or necrosis, blockage of cell replication by activating senescence, and remodeling of the cytoplasm by autophagy may take place. Phagocytosis is the process that eventually eliminates senescent, apoptotic, and necrotic cells. The initial reports about senescence date back to 1961, when Hayflick and Moorehead described alterations of in vitro cultured cells after several passages (Hayflick and Moorhead, 1961). DNA damage is the primary stimulus that induces senescence, but oxidative stress, mitochondrial damage, hypoxia, and oncogene activation could also activate this pathway (Chen et al., 1995; Muñoz-Espín and Serrano, 2014; Roy et al., 2020). Activating senescence results in morphological and functional alterations in cells (Campisi and D’Adda Di Fagagna, 2007). The list of cell changes includes resistance to apoptotic signals, arrest of which proliferation, and changes in gene expression (Campisi and D’Adda Di Fagagna, 2007).
Senescent activation is not defined by a particular characteristic. Nevertheless, the accumulation of senescence-associated β-galactosidase (SA-β-gal) and the increase in lysosomal SA-β-gal activity are senescence markers widely used (Dimri et al., 1995; Lee et al., 2006; Debacq-Chainiaux et al., 2009). In vitro, the senescent phenotype is characterized by altered nuclear morphology and chromatin structure as well as increased cell size (Narita et al., 2003; Rai and Adams, 2012). Another characteristic of senescence activation is a process called senescence-associated secretory phenotype (SASP) (Coppé et al., 2008; Byun et al., 2015), where cells secrete several factors affecting tissue remodeling, inflammation, and cell growth (Kuilman and Peeper, 2009; Freund et al., 2010; Basisty et al., 2020). One of the functions of SASP is to modulate the immune system in order to promote the elimination of senescent cells (Weisheit et al., 2015; Casiraghi et al., 2016). But if the signal persists, non-damaged cells may undergo senescence (Acosta et al., 2013).
The deterioration of the immune system with aging causes a disfunction in immunological surveillance (Schneider, 1983) increasing the prevalence and severity of infectious diseases (Aw et al., 2007; Pawelec, 2012; Martín et al., 2017). This phenomenon, known as immunosenescence, may increase the risk of Staphylococcus aureus infections among the elderly (McClelland et al., 1999; Kang et al., 2011; Thorlacius-Ussing et al., 2019). In addition, the clinical manifestations are more severe in older adults compared to younger individuals (McClelland et al., 1999; Tacconelli et al., 2006; Bassetti et al., 2018).
The endophagocytic pathway refers to the process by which cells ingest extracellular molecules or particles and degrade them within lysosomes (Huotari and Helenius, 2011). After the material has been taken in by the cell, a vesicle called an “endosome” is formed. The endosome fuses with lysosomal vesicles containing digestive enzymes that degrade the vesicle’s contents (Huotari and Helenius, 2011; Scott et al., 2014). Phagocytosis, pinocytosis, and clathrin-mediated endocytosis are three distinct types of endocytosis, each of which involves the ingestion of foreign components through slightly different molecular mechanisms (Huotari and Helenius, 2011). Endocytosis is essential for a wide range of physiological processes, including nutrient uptake, waste elimination, and the internalization of signaling molecules. Phagocytosis is characterized by the uptake of large extracellular particles, such as microorganisms or dead cells (Gordon, 2016; Lancaster et al., 2019; Uribe-Querol and Rosales, 2020), and this mechanism may change when immunosenescence occurs. Macrophages from older patients have a reduced phagocytic function (De La Fuente, 1985; Khare et al., 1996; De La Fuente et al., 2000), Interestingly, a similar change has been observed in aged Drosophila melanogaster hemocytes (Horn et al., 2014).
Autophagy is an important pathway connected to the endolysosomal pathway that maintains cellular homeostasis by eliminating damaged proteins and organelles (Mizushima et al., 2008). This process begins when a double membrane structure closes and sequesters cytoplasmic material such as protein aggregates, ribosomes, and even whole organelles like mitochondria (Eskelinen, 2005). These vesicles fuse sequentially with late endosomes and lysosomes, forming an autolysosome where the sequestered material is degraded (Eskelinen, 2005).
Numerous microorganisms are capable of surviving and replicating within phagocytic cells. They have developed a variety of protection strategies, like preventing the maturation of phagosomes or damaging this compartment letting the bacteria escape into the cytoplasm (Flannagan et al., 2009). In infections caused by S. aureus, a large number of bacteria are degraded following phagocytosis and those that survive. After that, the bacteria lysate the vacuole and then the cell, causing the bacterial spreading (Kubica et al., 2008).
Multiple studies have demonstrated that the autophagic pathway is essential for the survival and multiplication of S. aureus within cells. These studies demonstrated that the vacuoles harboring the bacteria are modified autophagosomes that do not become degradative compartments (Schnaith et al., 2007; Mestre and Colombo, 2012).
In the current report, we focused on the endophagocytic pathway in senescent epithelial and phagocytic cells. Surprisingly, after examining vesicle populations with confocal microscopy, we observed an increase in vacuole counts in all cases when senescence is activated. However, there were no alterations in vesicle maturation or degradation capacity. Next, we examined the internalization of various endophagocytic cargoes, observing a decrease in the intracellular recovery of the bacteria S. aureus. Then, we noticed that a decrease in bacterial internalization rather than an increase in bacterial degradation inside the cell was the cause of the observed effect. The alteration of S. aureus phagocytosis was also observed in Raw monocytes, which are professional phagocytes. Finally, using primary cultures of macrophages from aged animals, we found the same phagocytosis defects, confirming our hypothesis. Our results suggest that due to senescence activation-related defects in the early phagocytic process, bacterial clearance could be diminished in an organism. This failure may contribute to the observed susceptibility of the elderly to severe infectious diseases such as sepsis.
2 Methods
2.1 Reagents and antibodies
For specific assays, cells were treated with Bafilomycin A1 (Merck Milllipore, # 11707), NH4Cl (Merck Milllipore, # A9434-500G), and tert-butyl hydroperoxide (Merck Milllipore, # 458139). For specific transfections, we used EGFP-tagged human Rab5, which was kindly provided by Philip D. Stahl (Washington University, St. Louis), and EGFP-RAB7A, which was given by Bo van Deurs (University of Copenhagen, Denmark). In the current work, we used the following antibodies: rabbit antiserum against MAP1LC3B (Sigma-Aldrich # L7543, RRID:AB_796155), rabbit anti-cathepsinD (Dako, #A0561), mouse anti-LAMP2 (Santa Cruz Biotechnology, # sc-18822, RRID:AB_626858), mouse anti-tubulin (Abcam # ab7750, RRID:AB_306044), and mouse monoclonal anti-actin (Sigma-Aldrich, # A5441, RRID:AB_476744). The secondary antibodies used in the work were: HRP-conjugated goat anti-rabbit antiserum (Jackson ImmunoResearch Labs, # 111-035-003, RRID:AB_2313567), HRP-conjugated goat anti-mouse antiserum (Jackson ImmunoResearch Labs, # 115-035-003, RRID: AB_10015289), and Alexa Fluor 573-conjugated goat anti-mouse antiserum (Molecular Probes, # A-21424, RRID:AB_141780).
2.2 Cell culture, drug treatments and transfection
HeLa cells were cultured in Gibco Dulbecco’s Modified Eagle Medium (ThermoFisher Scientific # 12800-058), while CHO GFP-LC3 and Raw cells were grown in alpha MEM (ThermoFisher Scientific # 10800-058) medium supplemented with 10% heat-inactivated fetal bovine serum (FBS, Natocor), 2.2 g/L sodium bicarbonate, 2 mM glutamine, and 0.1% penicillin-streptomycin (ThermoFisher Scientific # 15140148). Cells were maintained at 37°C in a 5% CO2 atmosphere. Cells were subcultured after trypsinization (0.025% trypsine-EDTA, ThermoFisher Scientific, #15400054). For senescence activation, HeLa cells were grown to reach 80% confluence and then treated with tert-butyl hydroperoxide at 250 μM for 2 h. Subsequently, cells were washed and incubated in D-MEM + 10% FBS for 5 days. To perform autophagy experiments, control or infected cells were incubated at 37°C for 2 h in the presence or absence of 100 nM bafilomycin A1. Afterwards, cells were lysed and processed to be analyzed by Western blot. For transient expressions, Lipofectamine 3000 (ThermoFisher Scientific, # L3000008) was used according to the manufacturer’s instructions.
2.3 Cell primary culture
Vascular smooth muscle cells (VSMC) derived from mesenteric arteries were isolated using a method previously described (Touyz et al., 1994; Gil Lorenzo et al., 2014). Cells were obtained from Wistar-Kyotto (WKY) male rats (8–10 weeks old). VSMCs were identified by positive antibody staining against α-actin between the second and ninth passages. The procedures were carried out on subconfluent (50%–90%) cultures.
Following a previously described protocol (Weischenfeldt and Porse, 2008; Zhang et al., 2008; Vanrell et al., 2022), bone marrow cells were obtained from the femur bones of C57BL/6J WT mice and suspended in cold alpha MEM containing 40 g/mL of gentamicin. These bone marrow progenitor cells were isolated in 100-mm plates containing 10% FBS, 40 g/mL gentamicin, 2 mM L-glutamine, and conditioned medium derived from a 4-day culture of 30% L929 cells. The cells were then washed, and the same medium was added for an additional 6 days, leading to macrophage differentiation. Finally, macrophages were identified using CD11B and F480 as markers.
2.4 Cell staining, visualization, and image processing
For immunofluorescence, HeLa cells were grown on coverslips overnight to reach 50%–80% confluence. After incubation under different experimental conditions, cells were fixed with 3% paraformaldehyde (Merck Millipore, 158127) in PBS for 10 min at 37°C, washed with PBS, and reacted groups blocked with 50 mM NH4Cl (Merck Millipore, #213330) in PBS. Subsequently, they were permeabilized with 0.05% saponin (Sigma-Aldrich, #S4521) in PBS containing 0.5% BSA (Sigma-Aldrich, # A2153) and mounted in MOWIOL (Merck Milllipore, # 475904-M). Fixed cells were analyzed in an Olympus Fluoview 1000 confocal microscope using a 60x PlanApo oil NA 1.42 objective. All images were processed with the Fiji ImageJ software (Wayne Rasband, National Institutes of Health, RRID:SCR_002285). For the colocalization analysis, a single plane image from each channel was acquired, processed as described before, and then the Manders coefficient was calculated with Fiji ImageJ.
2.5 SA-β-galactosidase staining
HeLa cells were stained for SA-β-glactosidase according to the manufacturer’s instructions (Cell Signaling Technologies, catalog number 9860). Briefly, cells were washed three times with PBS and then fixed with 3% paraformaldehyde (Merck Millipore, 158127) in PBS for 15 min at room temperature. After two PBS washes, a β-galactosidase staining solution containing 1 mg/mL X-gal at a pH of 6.0 was added to the cells, and the culture plate was sealed with parafilm and kept at 37°C in a dry incubator overnight. The β-galactosidase staining solution was washed away and substituted by Mowiol. Pictures were captured at ×20 magnification using a Nikon DS-Ri2 camera and a Nikon Ti wide-field light microscope from Tokyo, Japan.
2.6 Western blots
Cells were lysed for 30 min at 4°C with RIPA buffer supplemented with the following protease activity inhibitors: 10 μg/mL aprotinin, 10 μg/mL leupeptin, 5 μg/mL pepstatin A, 1 mM sodium orthovanadate, and 1 mM sodium fluoride. Lysates were treated with Laemmli’s buffer and separated by SDS-PAGE. After electrophoresis, proteins were transferred onto nitrocellulose at 200 mA for 1 h at room temperature. Protein bands were detected by incubating membranes with the specific primary antibody for 1 h at room temperature, followed by a washing step with PBS containing 0.05% Tween 20, and then incubation with an HRP-conjugated secondary antibody for 1 h. Membranes were washed with PBS-Tween20 and then incubated with the Enhanced Chemiluminescence (ECL) reagent (General Electric, # RPN2232) and analyzed with Fujifilm LAS-4000 equipment. The densitometric analysis of the bands was performed with the ImageJ software.
2.7 Statistical analysis
Results are presented as the mean ± SEM from three independent experiments. T-test or ANOVA in conjunction with Tuckey and Dunnett tests were used to perform sample comparisons. Significant differences: *p < 0.01; **p < 0.005; ***p < 0.001.
3 Results
3.1 Senescence increases the number of endocytic vesicles
In the context of senescence activation, the endocytic pathway has received little attention. Previous studies have mainly concentrated on endocytic changes that lead to senescence (Shin et al., 2021). In this study, we were interested in investigating whether senescence impacts the endophagosomal pathway. Senescence is divided into replicative and stress-induced premature senescence (SIPS) categories (Toussaint et al., 2000). The latter refers to the activation of senescence by a stimulus like UV radiation or oxidative stress. In vitro, H2O2 or analogs such as tert-butylhydroperoxide (tBHP) treatment generally induce SIPS (Toussaint et al., 1992; Duan et al., 2005). Thus, we used tBHP to induce senescence in HeLa cells. The activation of the pathway was then assessed by staining the senescence-associated β-galactosidase which is known to mark predominantly senescent cells but not quiescent cells (Figure 1A). In order to corroborate the activation, we also evaluated some phenotypical characters such as cell and nucleus dimensions (Figures 1B, C), observing a marked size increase in both cells and nuclei, in agreement with previous publications (Gottwald et al., 2020).
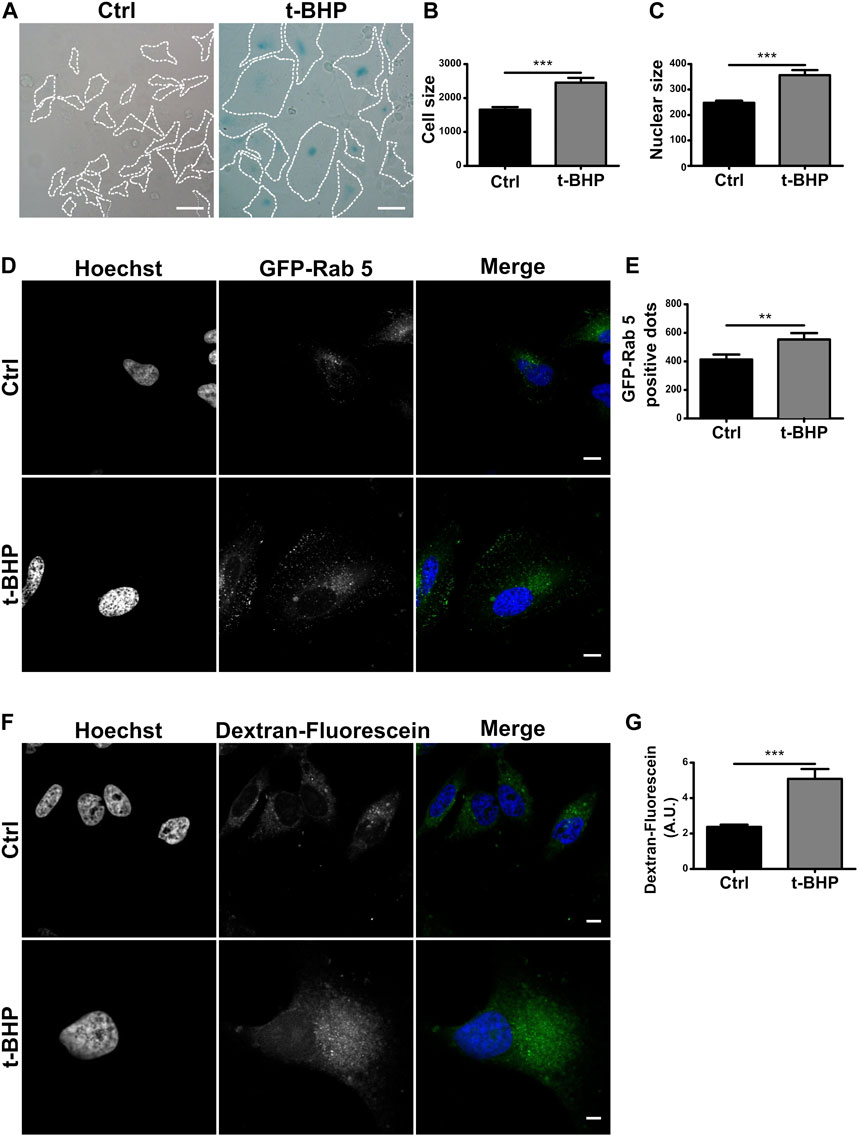
FIGURE 1. Senescence increases the number of endosomes per cell. (A) HeLa cells were treated with t-BHP for 2 h, then washed and cultured for 5 days to induce senescence. Next, both control and senescent cells were fixed and stained with X-gal (Scale bar, 50 um). (B) Measurement of cellular size. [n = 3 (in each experiment 60 cells were examined), mean ± SEM ***p < 0.0001] (C) Hoechst was used to stain control and senescent cells to visualize DNA. Nuclear size in untreated and t-BHP-treated cells was measured [n = 3 (60 cells were analyzed in each triplicate), mean ± SEM ***p < 0.0001]. (D) Cells treated and untreated with t-BHP were transfected with GFP-Rab5 and analyzed by confocal microscopy 24 h after transfection. (E) The number of Rab5 positive dots was quantified [n = 3 (35 cells in each triplicate), mean ± SEM *p = 0.0148.] (Scale bar, 10 μm). t-BHP-treated and untreated cells were incubated with dextran-fluorescein for 2 h, then fixed and analyzed by confocal microscopy. Subsequently, the dextran-fluorescein fluorescence intensity per cell was quantified [n = 3 (32 cells were analyzed for each experimental triplicate), mean ± SEM ***p < 0.0001] (Scale bar, 10 μm).
To examine endosome populations in senescent cells, we initially use the early endosome marker Rab5, a small GTPase that binds to the membrane of early endosomes and is one of the most frequently used endocytic markers (Numrich and Ungermann, 2014; Wandinger-Ness and Zerial, 2014) of HeLa cells overexpressing GFP-Rab5 (Figure 1D), the number vacuoles increased in senescent cells compared to control cells (Figure 1E). In addition, we investigated the cells ability to internalize dextran particles marked with fluorescein. This particle is not degraded, so it accumulates in endosomes at different stages of maturation, allowing for monitoring the entire pathway, including endolysosomes. Similar to the above results, senescence increased the number of vesicles containing fluorescent dextran (Figures 1F, G).
3.2 Endosome maturation is not affected in senescent cells
When the maturation of the endocytic pathway is blocked, early endosomes accumulate. To determine whether the increased quantity of vesicles is due to an endosome maturation defect, we monitored mature endosomes overexpressing GFP-Rab7 (Figure 2A) or detecting endogenous LAMP2 (Supplementary Figure S1A), two markers of late endosomes (Numrich and Ungermann, 2014; Wandinger-Ness and Zerial, 2014). When the number of labeled vesicles was determined, senescent cells also contained a greater number of Rab7 (Figure 2B) or LAMP2 (Supplementary Figure S1B) vesicles than normal cells, suggesting that the transition from early to late endosomes does not seem to be affected. Thus, we hypothesized that changes in cell size might explain the observed increase. Comparing the ratio obtained by dividing the number of LAMP2-positive (Supplementary Figure S1C) or Rab7-positive vesicles (Supplementary Figure S1D) per cell by the size of the cell, no significant difference between normal and senescent cells was observed. These findings suggest that the increased number of endosomes per cell observed in senescent cells is due to their larger size. In addition, we evaluated the colocalization of GFP-Rab5 and eLAMP2 (Figure 2C) or GFP-Rab7 and LAMP2 (Supplementary Figure S1E), and consistent with the previous observation, we did not observe differences between senescent and normal cells in either case (Figure 2D; Supplementary Figure S1F).
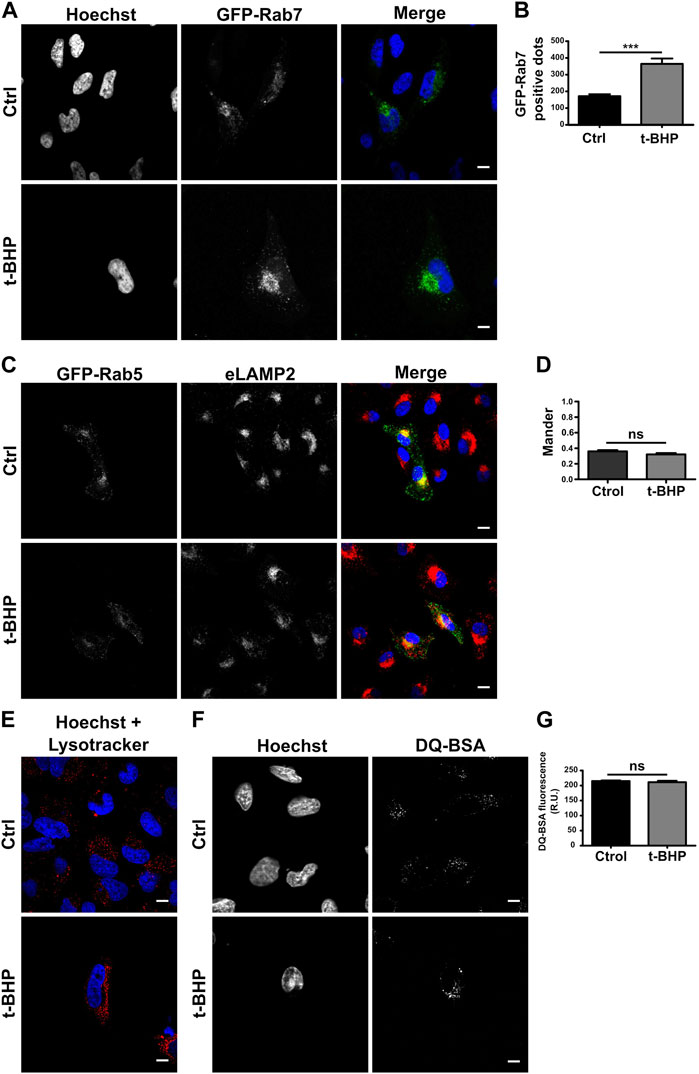
FIGURE 2. Senescence has no effect on endosome maturation. (A) HeLa cells treated or untreated with t-BHP were transfected with GFP-Rab7 and examined 24 h after transfection using confocal microscopy. (B) The number of Rab7-positive dots was quantified [n = 3 (each n tested = 33 cells), mean SEM ***p 0.0001]. (C) Both t-BHP-treated or untreated cells were transfected with GFP-Rab5, and 24 h after transfection, cells were fixed and immunostained with an anti-LAMP2 antibody and a specific secondary antibody. (D) Samples were analyzed by confocal microscopy, and colocalization of GFP-Rab5 with LAMP2 was calculated [n = 3 (each n tested = 45 cells), mean ± SEM ns p = 0.1938]. (E) Control and senescent cells were stained with LysoTracker red and then analyzed by confocal microscopy. (F) Evaluation of control and senescent cells incubated with DQ-BSA. (G) Quantification of DQ BSA fluorescence intensity from confocal microscopy images [n = 3 (each n tested 42 cells), mean ± SEM ns p = 0.4049] (Scale bar, 10 μm).
To assess whether the endosomes mature properly in senescent cells, LysoTracker red, a molecule that accumulates in acidic compartments, was used to determine the pH of the vesicles. Similar to normal cells, senescent cells feature many LysoTracker-red staining vesicles, indicating that senescence activation has no influence on the acidification process (Figure 2E). Then, we analyzed its functioning, such as its capacity to degrade bovine serum albumin (BSA). We utilized a BSA derivative that was so densely labeled with the green fluorescent BODIPY® FL dye that the fluorescence emission is self-quenched. When DQ-BSA reaches a degradative compartment, it is cleaved, releasing fluorescent fragments. As shown in Figures 2F, G, the activation of senescence does not prevent the accumulation of this fluorescent probe in cells. In addition, the conversion of the lysosomal enzyme pro-cathepsin D to cathepsin D was analyzed. Cathepsins and other lysosomal proteases are transported by vesicles that originate from the trans-Golgi apparatus and become active upon autoprocessing within late endosomes or lysosomes. Cathepsins need to reach an acidic vesicle in order to suffer this cleavage (Supplementary Figure S2A). We examined pro-cathepsin D cleavage by Western blot (Supplementary Figure S2B) and found no variation in the protein fragments generated by normal or senescent cells (Supplementary Figure S2C).
Taken together, our results indicate that the maturation process and the functionality of the endocytic pathway do not seem to be altered in senescent cells.
3.3 Senescence does not modify S. aureus intracellular trafficking
Defense is an important function of the endo/phagocytic pathway, especially in professional phagocytes like macrophages and neutrophils but also in non-professional phagocytes like epithelial cells (Rabinovitch, 1995; Uribe-Querol and Rosales, 2020). The function of non-professional phagocytes like epithelial cells in vivo is poorly understood. However, epithelial cells have been extensively utilized in the study of bacterial internalization and intracellular events involving a vast array of bacteria. To examine whether senescence influences bacterial phagocytosis in epithelial cells, HeLa cells were infected with gram-positive S. aureus or gram-negative Yersinia enterocolitica bacteria. After 2 h, cells were lysed, and the recovered bacteria were quantified by counting the colony-forming units (CFU) on the appropriate agar plates (Figure 3A). As indicated in Figure 3B, S. aureus recovery was decreased in senescent infected cells. Similarly, the recovery of Y. enterocolitica from senescent cells was diminished compared to normal cells (Figure 3C).
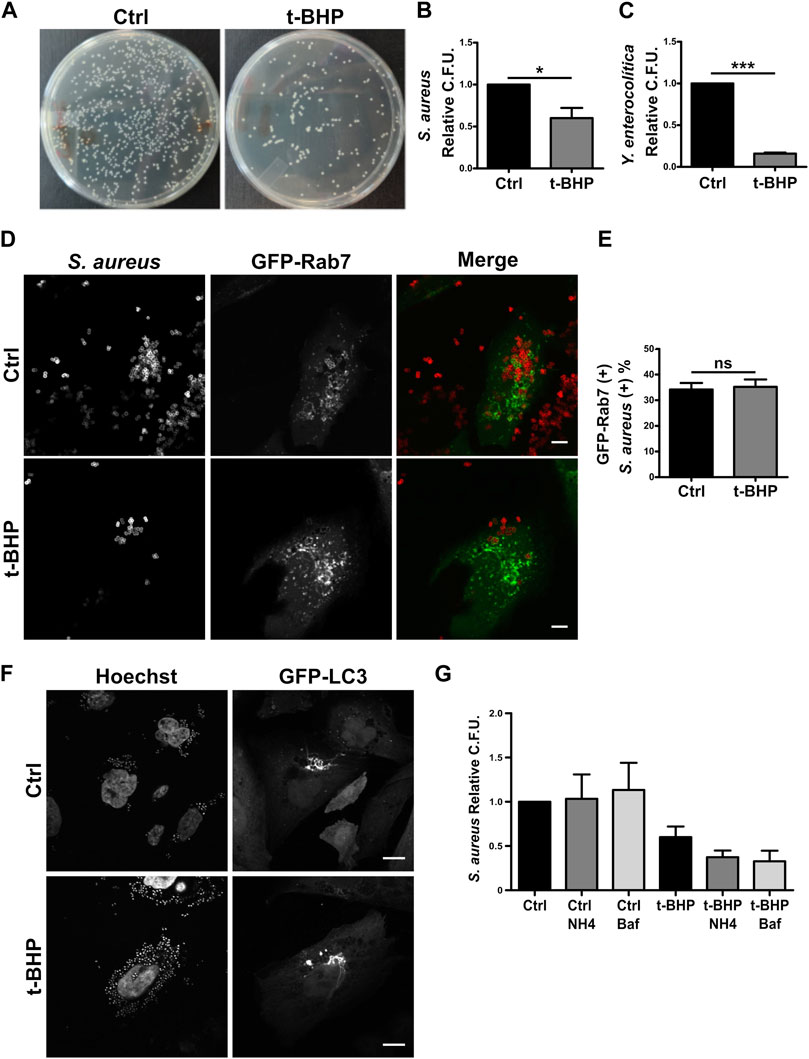
FIGURE 3. The recovery of bacteria was lower in infected senescent cells than in infected control cells. (A) Senescent cells where obtained in accordance with the previously described methodology. Then, both control and senescent cells were infected with S. aureus or Y. enterocolitica for 1 h. Afterwards, cells were lysed, placed on LB agar, and incubated for 24 h (B) S. aureus CFU quantification (n = 3, mean ± SEM *p = 0.0458) (C) Y. enterocolítica CFU quantification. (n = 3, mean ± SEM *p = 0.0002). (D) Confocal microscopy of control and senescent cells, transfected with Rab7, infected with S. aureus (M.O.I. = 10), and fixed at 1 h post infection (1hpi) (scale bar = 10 um). (E) Quantification of the proportion of bacteria present on GFP-Rab7 positive vesicles in control and senescent cells. [n = 3 (each n tested = 30 cells), (Mean ± SEM ns p = 0.7878)] (F) Confocal microscopy of CHO cells stable transfected with GFP-LC3, treated or untreated with t-BHP, infected with S. aureus (M.O.I = 10), and fixed 4 h after infection. (scale bar = 10 μm). (G) Control and senescent HeLa cells were pre-treated with inhibitors of endosome maturation, washed, and then infected. Following 10 min of internalization, ClNH4 or BafilomycinA1 was added to the media to block autophagic flux. At 1 hpi, cells were lysed and CFU were measured on LB agar plates. Mean ± SEM. n = 3 from three independent experiments.
Subsequently, due to the bacteria’s clinical relevance, we centered our interest on a more in-depth study of S. aureus. Reduced bacterial recovery from senescent cells may be the result of either lower bacterial internalization or higher bacterial degradation within the cell. We first examined S. aureus intracellular traffic using confocal fluorescence microscopy. We found no differences between control and senescent cells in the colocalization of bacteria with Rab5 (Supplementary Figures 3A, 3B) or Rab7 (Figures 3D, E).
A hallmark of the intracellular cycle of S. aureus is the interaction with the autophagic pathway. Late in the process, the vesicles containing the bacteria fuse to form honeycomb-like structures decorated with the protein LC3 falta nuestra cita. As shown in Figure 3F, senescence does not prevent the formation of such arrangements. The majority of MAP1LC3 is located in the cytosol; however, when autophagy is induced, this protein is cleaved and lipidated, allowing its association with the autophagosome membrane. Due to the differing electrophoretic mobilities of the two forms of MAP1LC3 (MAP1LC3-I, which is non-lipidated, and MAP1LC3-II, which is lipidated), this post-translational processing can be studied by Western blot. It has been shown that following autophagy induction, the level of MAP1LC3B-II increases significantly (Kabeya et al., 2000). This accumulation is more pronounced when autophagosome maturation (i.e., fusion with lysosomes) is inhibited because the autophagic flux blockage prevents MAPLC3 degradation (e.g., by chloroquine). Similar to the findings shown in previous studies (Luo et al., 2013; Tang et al., 2017; Wang et al., 2018; Kang et al., 2019; Zheng et al., 2019; Chen et al., 2020; Lu et al., 2021), in HeLa senescent cells we observed a reduction in the autophagic flux under basal conditions (ratio between Baf1 treated and Baf1 untreated conditions, Supplementary Figure S3C, columns 2 and 1). Then, on S. aureus-infected cells, the autophagic flux observed in normal and senescent cells did not differ (ratio between Baf1-treated and Baf1-untreated conditions, Supplementary Figure S3C, columns 4 and 3 lines) and seemed to be blocked. These findings suggest that stimulation of senescence does not modify the autophagic pathway related to the infection.
Next, with the purpose of determining whether bacterial degradation within the cell might be altered in senescent cells, we determined the recovered bacteria by counting the colony-forming units (CFU) using various degradation inhibitors. To this end, we infected control and senescent cells in the presence and absence of several phagosome maturation inhibitors. The cells were then lysed, and CFU were used to count the bacteria in relation to the number of cells in each condition and relativized to the number of untreated normal cells (which have the value 1). As shown in Figure 3G, bars 1–3, in the presence of inhibitors, the bacterial recovery of normal cells does not differ significantly from that of untreated cells. Similarly, senescent cells treated with the inhibitors do not exhibit an improvement in bacterial recovery (Figure 3G, bars 4–6). Our findings indicate that senescence does not appear to affect S. aureus intracellular trafficking or replication.
3.4 Senescent cells fail in the S. aureus internalization step
Given that our findings revealed that bacterial degradation was not enhanced in senescent cells, we thought that when senescence is induced, the bacterial internalization process may be altered. Using confocal microscopy, this hypothesis was verified with a methodology that eliminates extracellular bacteria with a mild trypsin treatment followed by repeated washes. Thus, only S. aureus that has been phagocytosed by the cells is visualized. As shown in Figures 4A, B, senescence dramatically reduced the number of bacteria that were internalized. The first step in the phagocytic process is the particle’s adhesion to the cell membrane. Then, we determine if the decrease in intracellular S. aureus is attributable to a failure of bacterial adhesion to the cell. To achieve this, S. aureus was incubated at 4°C for 30 min, a temperature that enables binding but prevents internalization. The cells were subsequently fixed, and confocal microscopy was used to evaluate the samples. In senescent cells, we observed significant decreases in the amount of bacteria per cell (Figures 4C, D). These findings demonstrated that inefficient adhesion is likely the cause of the decreased internalization of S. aureus by epithelial cells.
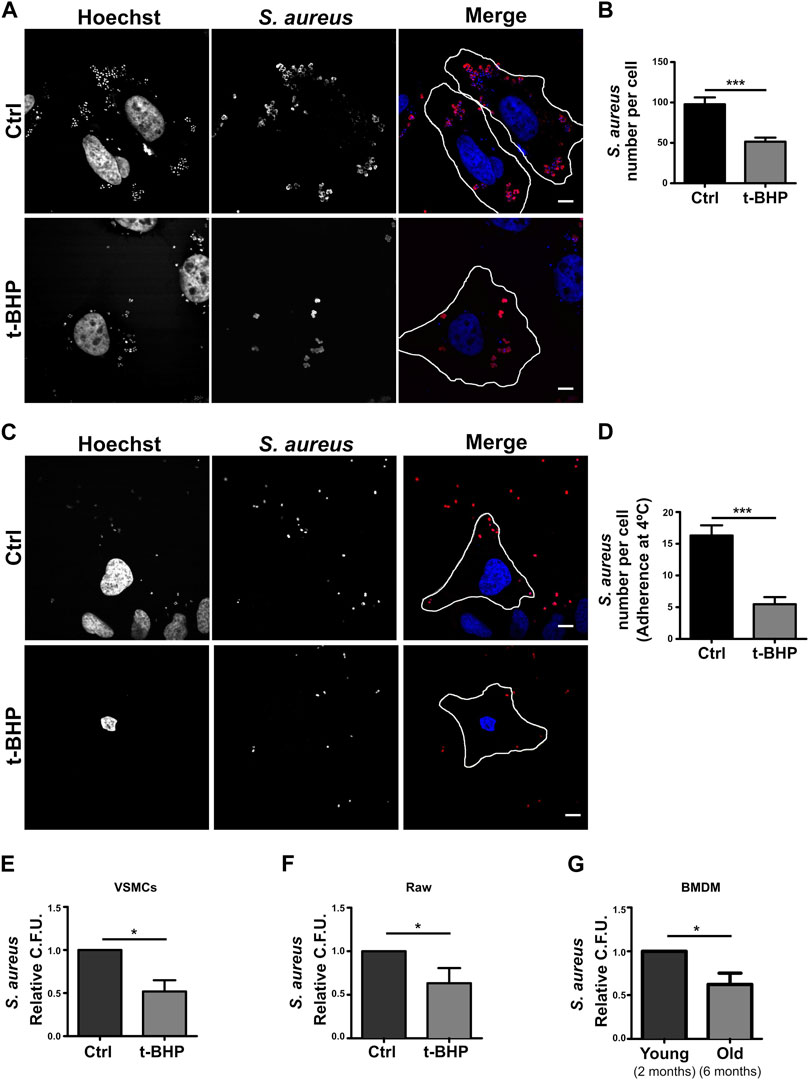
FIGURE 4. S. aureus adhesion and internalization is altered in senescent cells. (A) Control and senescent cells were incubated with S. aureus for 10 min. Subsequently, extracellular bacteria were removed by a mild trypsin treatment followed by multiple washings. The cells were then fixed 1 h post-infection. M.O.I. = 10 (scale bar = 10 μm). (B) Quantification of bacteria per cell. [n = 3 (each n tested = 28 cells) Mean + SEM ***p < 0.0001]. (C) Control and senescent cells were incubated with S. aureus at 4°C for 30 min and then fixed. M.O.I. = 10 (scale bar = 10 um). (D) Quantification of the number of bacteria adhered to the surface of the cell. [n = 3 (each n tested 25 cells) Mean + SEM ***p < 0.0001]. (E) Senescence induction was performed in VSMCs cell in accordance with the previously described methodology (see Methods). Then, both control and senescent cells were infected with S. aureus (MOI = 10) for 1 h. The cells were then lysed, samples placed on LB agar, and incubated for 24 h. S. aureus CFU quantification (n = 3, mean ± SEM *p = 0.0458). (F) Senescence activation was performed in RAW cells as described in methods. Afterwards, both control and senescent cells were infected with S. aureus for 1 h. Cells were then lysed, placed on LB agar, and incubated for 24 h. S. aureus CFU quantification (n = 3, mean ± SEM *p = 0.0458). (G) BMDMs from mice of two or 6 months old were infected with S. aureus for 1 h. The cells were then lysed, placed on LB agar, and incubated for 24 h. S. aureus CFU quantification (n = 3, mean ± SEM *p = 0.0458).
All previous experiments in this study were conducted with a cancer-derived cell line. Thus, to have a more physiological model, we used a primary culture of mesenteric artery vascular smooth muscle cells (VSMC). These cells were treated with tBHP as previously described. Subsequently, the cells were infected with S. aureus for 2 h, washed, and lysed, and the recovered bacteria from the lysate were quantified using CFUs relative to cell number. Figure 4E demonstrates that, comparable to HeLa cells, in senescent VSMC, a diminished recovery of S. aureus was also found.
Professional phagocytes are responsible for eliminating microorganisms within an organism. To determine if senescence has a similar effect on immune cells, we replicated the above assays with Raw 264.7 cells, a monocyte cell line, using the same protocol as with HeLa and VSMC. We determined that senescence also impacts the internalization of bacteria by Raw cells (Figure 4F). Lastly, we wondered whether this process occurs in an organism that gets old. To answer this question, we compared the phagocytosis of macrophages derived from the bone marrow (BMDMs) of 2- and 6-month-old animals. When we infected these BMDMs for 2 h and then counted the recovered bacteria by CFUs after cell lysis, we observed a result that was very similar to that observed with the stress-induced premature senescence model (Figure 4G).
In summary, all these results performed in different cell lines (professional or non-professional phagocytes) support the hypothesis that senescence affects bacterial adhesion to the cells, altering the amount of internalized bacteria. Besides, results primary obtained with culture cells derived from aged organisms show that the phagocytic dysfunction is also observed in aging.
4 Discussion
Several studies demonstrate that senescent cells are detectable in tissues, indicating that they persist and accumulate with age (Dimri et al., 1995; Biran et al., 2017; Evangelou et al., 2017). This indicates that senescent cells may still retain some cellular capabilities. In the current study, we focused on endo/phagocytic activity. First, we observed that senescent cells exhibit an increase in endosomes at each stage of their maturation process. The change in the number of vesicles per cell was partially attributable to an increase in cell size. Nevertheless, neither the maturation nor the degradation capabilities of vesicles were affected. Our findings support the conclusion of Gurley and Dice that there are no differences in the intralysosomal degradation rates of several endocytosed proteins between young and senescent fibroblast cells (Gurley and Dice, 1988). On the other hand, there are reports that senescent cells undergo receptor-mediated endocytosis changes (Park et al., 2001b; Park et al., 2002). Noteworthy, these works studied how alterations in the endocytic pathway induce senescence but did not examine how senescence can affect the endocytic pathway (Park, 2002; Shin et al., 2021). In addition, some studies indicate that senescence influences bacterial phagocytosis but not other intracellular processes, such as superoxide generation (Butcher et al., 2001). Taken together, the existing information suggests that senescence modifies the internalization of particular cargoes.
According to our study, the recovery of gram-positive and gram-negative bacteria following infection of senescent cells is lower than in normal cells. Interestingly, senescence had little impact on intracellular S. aureus trafficking (i.e., colocalization with endocytic markers) or their interactions with autophagy. Interestingly, we demonstrated that senescence affects the internalization stage, particularly bacterial adhesion, in both professional and non-professional phagocytes. Our findings may contribute to explaining why the clinical manifestations of S. aureus infection vary between young and elderly people. This notion was strengthened by the results obtained in the BMDM from aged animals, where a defect in the internalization of S. aureus was observed in the absence of tBHP treatment.
In young patients, infectious metastases are the most frequent consequences. The proposed process involves bacterial internalization by cells with subsequent intracellular organism dissemination (Mosser and Edwards, 2008). Although the bacteria are capable of surviving and replicating within cells, this procedure would prevent them from replicating in the bloodstream, where they could cause more severe clinical conditions. A defect in internalization cell capacity may result in an increase in extracellular bacterial replication, thereby promoting the occurrence of a more severe infection. In the present study, we established that senescence influences the internalization of S. aureus. As mentioned before, many studies have shown the accumulation of senescent cells in older individuals. This accumulation, in conjunction with the data presented in this report, may explain the high prevalence of S. aureus bacteremias and septic shock among the elderly (Gavazzi et al., 2002; Vardi et al., 2013).
This leads to the idea that senescent cells may play a significant role in aging and disorders associated with aging. Their removal by the immune system may be essential to preserving health (Lujambio, 2016; Kale et al., 2020). The failure to eliminate senescent cells causes the organism to malfunction (Campisi and D’Adda Di Fagagna, 2007; Kale et al., 2020). In addition, our findings open the door to novel therapeutic options, such as the use of senolytics to prevent senescent cell accumulation or medications that can affect the surface pattern of senescent cells in order to reverse internalization defects. For this objective, an in-depth understanding of membrane modifications that limit bacterial adhesion is required. Currently, we are focusing on the investigation of several proteins that function as bacterial receptors, such as integrins and multiple TLRs. Also, we are investigating lipid rafts, which have been linked to alterations in receptor-mediated endocytosis (Park et al., 2001a; Park et al., 2001b; Park et al., 2002). We believe that the findings of our current and future investigations will increase the general understanding of infectious diseases.
Data availability statement
The original contributions presented in the study are included in the article/Supplementary Material, further inquiries can be directed to the corresponding authors.
Author contributions
MA and ER drafted the manuscript with the input and evaluation of IAV and MIC. ER and PB conducted the experiments and analysis used to prepare the figures and graphics. MA provided the study’s primary resources and finance, as well as supervision. MC contributed project funds and guidance. All authors contributed to the article and approved the submitted version.
Funding
This work was supported by the PUE-0025 from CONICET to MA and MC, PICT 2019-0038 to MA and PICT 2018-04427 to MC from Agencia Nacional de Promoción de la Investigación, el Desarrollo Tecnológico y la Innovación (ANPCYT), and SIIP2019 of the Universidad Nacional de Cuyo to MA. ER and PB were supported by CONICET fellowships.
Acknowledgments
We appreciate Bo van Deurs and Philip D. Stahl for providing some materials. We appreciate the technical support of Luz Estefanía Farías Altamirano, Elisa Bocanegra, and Clara García Samartino with tissue culture and microscopy assistance. We also thank to Andrea Fernanda Gil Lorenzo and Patricia G. Vallés (IMBECU; CONICET) for providing the VSMCs and to Julieta Scelta and Mónica Chamorro for technical support with animals manipulation. Dr. Laura Ruth Delgui, Dr. Walter Berón and Dr. Oscar Daniel Bello are also acknowledged for their meticulous proofreading of the manuscript.
Conflict of interest
The authors declare that the research was conducted in the absence of any commercial or financial relationships that could be construed as a potential conflict of interest.
Publisher’s note
All claims expressed in this article are solely those of the authors and do not necessarily represent those of their affiliated organizations, or those of the publisher, the editors and the reviewers. Any product that may be evaluated in this article, or claim that may be made by its manufacturer, is not guaranteed or endorsed by the publisher.
Supplementary material
The Supplementary Material for this article can be found online at: https://www.frontiersin.org/articles/10.3389/fragi.2023.1198241/full#supplementary-material
SUPPLEMENTARY FIGURE S1 | Senescence has no effect on endosome maturation. (A) HeLa cells treated or untreated with t-BHP, fixed and immunostained with an anti-LAMP2 antibody and a specific secondary antibody. (Scale bar, 10 μm). (B) The number of LAMP2 positive dots was quantified [n = 3 (each n tested = 30 cells), mean SEM ***p 0.0001]. (C) Quantification of LAMP2 positive vesicles in control and senescent cells per cell relative to the cell size (Mean ± SEM ns p = 0.8752). (D) Quantification of GFP-Rab 7 positive vesicles in control and senescent cells per cell relative to the cell size (Mean ± SEM ns p = 0.1073). (E) Both t-BHP-treated and untreated cells were transfected with GFP-Rab7, and 24 h after transfection, cells were fixed and immunostained with an anti-LAMP2 antibody and a specific secondary antibody. (Scale bar, 10 μm). (F) Samples were analyzed by confocal microscopy, and colocalization of GFP-Rab7 with LAMP2 was calculated [n = 3 (each n tested = 40 cells), mean ± SEM ns p = 0.2075].
SUPPLEMENTARY FIGURE S2 | Senescence does not alter cathepsinD processing. (A) Process of conversion of pro-cathepsin D to cathepsin D in endo/lysosomal compartments. (B) HeLa cells treated or untreated with t-BHP were processed for Western blot with an antibody against cathepsinD. (C) Quantification of cathepsinD intensity over pro-cathepsinD intensity (n = 3, mean ± SEM ns p = 0.2558).
SUPPLEMENTARY FIGURE S3 | Senescence modify S. aureus internalization but not intracellular trafficking. (A) Confocal microscopy of control and senescent cells, transfected with Rab5, infected with S. aureus, and fixed at 1 h.p.i. M.O.I. = 10 (scale bar= 10 um). (B) Quantification of the proportion of bacteria present on GFP-Rab5 positive vesicles in control and senescent cells. (Mean ± SEM ns p = 0.9881). (C) Cells treated with t-BHP or untreated were pre-incubated for 1 h with Bafilmycin A1 and infected with S. aureus M.O.I. = 10. Subsequently, they were processed by Western blot to detect LC3 or actin. One image representative from three experiments.
References
Acosta, J. C., Banito, A., Wuestefeld, T., Georgilis, A., Janich, P., Morton, J. P., et al. (2013). A complex secretory program orchestrated by the inflammasome controls paracrine senescence. Nat. Cell Biol. 15, 978–990. doi:10.1038/ncb2784
Aw, D., Silva, A. B., and Palmer, D. B. (2007). Immunosenescence: Emerging challenges for an ageing population. Immunology 120, 435–446. doi:10.1111/j.1365-2567.2007.02555.x
Basisty, N., Kale, A., Jeon, O. H., Kuehnemann, C., Payne, T., Rao, C., et al. (2020). A proteomic atlas of senescence-associated secretomes for aging biomarker development. PLoS Biol. 18, e3000599. doi:10.1371/journal.pbio.3000599
Bassetti, M., Righi, E., Del Giacomo, P., Sartor, A., Ansaldi, F., Trucchi, C., et al. (2018). Predictors of mortality with Staphylococcus aureus bacteremia in elderly adults. J. Am. Geriatr. Soc. 66, 1284–1289. doi:10.1111/jgs.15391
Biran, A., Zada, L., Abou Karam, P., Vadai, E., Roitman, L., Ovadya, Y., et al. (2017). Quantitative identification of senescent cells in aging and disease. Aging Cell 16, 661–671. doi:10.1111/acel.12592
Butcher, S. K., Chahal, H., Nayak, L., Sinclair, A., Henriquez, N. V., Sapey, E., et al. (2001). Senescence in innate immune responses: Reduced neutrophil phagocytic capacity and CD16 expression in elderly humans. J. Leukoc. Biol. 70, 881–886. doi:10.1189/jlb.70.6.881
Byun, H. O., Lee, Y. K., Kim, J. M., and Yoon, G. (2015). From cell senescence to age-related diseases: Differential mechanisms of action of senescence-associated secretory phenotypes. BMB Rep. 48, 549–558. doi:10.5483/BMBRep.2015.48.10.122
Campisi, J., and D’Adda Di Fagagna, F. (2007). Cellular senescence: When bad things happen to good cells. Nat. Rev. Mol. Cell Biol. 8, 729–740. doi:10.1038/nrm2233
Casiraghi, F., Perico, N., Cortinovis, M., and Remuzzi, G. (2016). Mesenchymal stromal cells in renal transplantation: Opportunities and challenges. Nat. Rev. Nephrol. 12, 241–253. doi:10.1038/nrneph.2016.7
Chen, Q., Fischer, A., Reagan, J. D., Yan, L. J., and Ames, B. N. (1995). Oxidative DNA damage and senescence of human diploid fibroblast cells. Proc. Natl. Acad. Sci. U. S. A. 92, 4337–4341. doi:10.1073/pnas.92.10.4337
Chen, Y., Lin, J., Chen, J., Huang, C., Zhang, Z., Wang, J., et al. (2020). Mfn2 is involved in intervertebral disc degeneration through autophagy modulation. Osteoarthr. Cartil. 28, 363–374. doi:10.1016/J.JOCA.2019.12.009
Coppé, J.-P., Patil, C. K., Rodier, F., Sun, Y., Muñoz, D. P., Goldstein, J., et al. (2008). Senescence-associated secretory phenotypes reveal cell-nonautonomous functions of oncogenic RAS and the p53 tumor suppressor. PLoS Biol. 6, 2853–2868. doi:10.1371/journal.pbio.0060301
De La Fuente, M. (1985). Changes in the macrophage function with aging. Comp. Biochem. Physiol. A. Comp. Physiol. 81, 935–938. doi:10.1016/0300-9629(85)90933-8
De La Fuente, M., Medina, S., Del Rio, M., Ferrández, M. D., and Hernanz, A. (2000). Effect of aging on the modulation of macrophage functions by neuropeptides. Life Sci. 67, 2125–2135. doi:10.1016/S0024-3205(00)00799-2
Debacq-Chainiaux, F., Erusalimsky, J. D., Campisi, J., and Toussaint, O. (2009). Protocols to detect senescence-associated beta-galactosidase (SA-betagal) activity, a biomarker of senescent cells in culture and in vivo. Nat. Protoc. 4, 1798–1806. doi:10.1038/nprot.2009.191
Dimri, G. P., Lee, X., Basile, G., Acosta, M., Scott, G., Roskelley, C., et al. (1995). A biomarker that identifies senescent human cells in culture and in aging skin in vivo. Proc. Natl. Acad. Sci. 92, 9363–9367. doi:10.1073/pnas.92.20.9363
Duan, J., Duan, J., Zhang, Z., and Tong, T. (2005). Irreversible cellular senescence induced by prolonged exposure to H 2O2 involves DNA-damage-and-repair genes and telomere shortening. Int. J. Biochem. Cell Biol. 37, 1407–1420. doi:10.1016/j.biocel.2005.01.010
Eskelinen, E. (2005). Maturation of autophagic vacuoles in Mammalian cells. Autophagy 1, 1–10. doi:10.4161/auto.1.1.1270
Evangelou, K., Lougiakis, N., Rizou, S. V., Kotsinas, A., Kletsas, D., Muñoz-Espín, D., et al. (2017). Robust, universal biomarker assay to detect senescent cells in biological specimens. Aging Cell 16, 192–197. doi:10.1111/acel.12545
Flannagan, R. S., Cosío, G., and Grinstein, S. (2009). Antimicrobial mechanisms of phagocytes and bacterial evasion strategies. Nat. Rev. Microbiol. 7, 355–366. doi:10.1038/nrmicro2128
Freund, A., Orjalo, A. V., Desprez, P. Y., and Campisi, J. (2010). Inflammatory networks during cellular senescence: Causes and consequences. Trends Mol. Med. 16, 238–246. doi:10.1016/j.molmed.2010.03.003
Gavazzi, G., Mallaret, M. R., Couturier, P., Iffenecker, A., and Franco, A. (2002). Bloodstream infection: Differences between young-old, old, and old-old patients. J. Am. Geriatr. Soc. 50, 1667–1673. doi:10.1046/j.1532-5415.2002.50458.x
Gil Lorenzo, A. F., Bocanegra, V., Benardon, M. E., Cacciamani, V., and Vallés, P. G. (2014). Hsp70 regulation on Nox4/p22phox and cytoskeletal integrity as an effect of losartan in vascular smooth muscle cells. Cell Stress Chaperones 19, 115–134. doi:10.1007/s12192-013-0439-6
Gordon, S. (2016). Phagocytosis: An immunobiologic process. Immunity 44, 463–475. doi:10.1016/j.immuni.2016.02.026
Gottwald, D., Putz, F., Hohmann, N., Büttner-Herold, M., Hecht, M., Fietkau, R., et al. (2020). Role of tumor cell senescence in non-professional phagocytosis and cell-in-cell structure formation. BMC Mol. Cell Biol. 21, 79–15. doi:10.1186/s12860-020-00326-6
Gurley, R., and Dice, J. F. (1988). Degradation of endocytosed proteins is unaltered in senescent human fibroblasts. Cell Biol. Int. Rep. 12, 885–894. doi:10.1016/0309-1651(88)90052-5
Hayflick, L., and Moorhead, P. S. (1961). The serial cultivation of human diploid cell strains. Exp. Cell Res. 25, 585–621. doi:10.1016/0014-4827(61)90192-6
Horn, L., Leips, J., and Starz-Gaiano, M. (2014). Phagocytic ability declines with age in adult Drosophila hemocytes. Aging Cell 13, 719–728. doi:10.1111/acel.12227
Huotari, J., and Helenius, A. (2011). Endosome maturation. EMBO J. 30, 3481–3500. doi:10.1038/emboj.2011.286
Kabeya, Y., Mizushima, N., Ueno, T., Yamamoto, A., Kirisako, T., Noda, T., et al. (2000). LC3, a mammalian homologue of yeast Apg8p, is localized in autophagosome membranes after processing. EMBO J. 19, 5720–5728. doi:10.1093/emboj/19.21.5720
Kale, A., Sharma, A., Stolzing, A., Stolzing, A., Desprez, P. Y., Desprez, P. Y., et al. (2020). Role of immune cells in the removal of deleterious senescent cells. Immun. Ageing 17, 16. doi:10.1186/s12979-020-00187-9
Kang, C. I., Song, J. H., Ko, K. S., Chung, D. R., and Peck, K. R. (2011). Clinical features and outcome of Staphylococcus aureus infection in elderly versus younger adult patients. Int. J. Infect. Dis. 15, e58–e62. doi:10.1016/j.ijid.2010.09.012
Kang, L., Xiang, Q., Zhan, S., Song, Y., Wang, K., Zhao, K., et al. (2019). Restoration of autophagic flux rescues oxidative damage and mitochondrial dysfunction to protect against intervertebral disc degeneration. Oxid. Med. Cell. Longev. 2019, 7810320. doi:10.1155/2019/7810320
Khare, V., Sodhi, A., and Singh, S. M. (1996). Effect of aging on the tumoricidal functions of murine peritoneal macrophages. Nat. Immun. 15, 285–294.
Kubica, M., Guzik, K., Koziel, J., Zarebski, M., Richter, W., Gajkowska, B., et al. (2008). A potential new pathway for Staphylococcus aureus dissemination: The silent survival of S. aureus phagocytosed by human monocyte-derived macrophages. PLoS One 3, e1409. doi:10.1371/journal.pone.0001409
Kuilman, T., and Peeper, D. S. (2009). Senescence-messaging secretome: SMS-ing cellular stress. Nat. Rev. Cancer 9, 81–94. doi:10.1038/nrc2560
Lancaster, C. E., Ho, C. Y., Hipolito, V. E. B., Botelho, R. J., and Terebiznik, M. R. (2019). Phagocytosis: What’s on the menu? Biochem. Cell Biol. 97, 21–29. doi:10.1139/bcb-2018-0008
Lee, B. Y., Han, J. A., Im, J. S., Morrone, A., Johung, K., Goodwin, E. C., et al. (2006). Senescence-associated β-galactosidase is lysosomal β-galactosidase. Aging Cell 5, 187–195. doi:10.1111/j.1474-9726.2006.00199.x
Lu, H., Jia, C., Wu, D., Jin, H., Lin, Z., Pan, J., et al. (2021). Fibroblast growth factor 21 (FGF21) alleviates senescence, apoptosis, and extracellular matrix degradation in osteoarthritis via the SIRT1-mTOR signaling pathway. Cell Death Dis. 12, 865. doi:10.1038/s41419-021-04157-x
Lujambio, A. (2016). To clear, or not to clear (senescent cells)? That is the question. BioEssays 38, S56–S64. doi:10.1002/bies.201670910
Luo, C., Li, Y., Wang, H., Feng, Z., Li, Y., Long, J., et al. (2013). Mitochondrial accumulation under oxidative stress is due to defects in autophagy. J. Cell. Biochem. 114, 212–219. doi:10.1002/JCB.24356
Martín, S., Pérez, A., and Aldecoa, C. (2017). Sepsis and immunosenescence in the elderly patient: A review. Front. Med. 4, 20. doi:10.3389/fmed.2017.00020
McClelland, R. S., Fowler, V. G., Sanders, L. L., Gottlieb, G., Kong, L. K., Sexton, D. J., et al. (1999). Staphylococcus aureus bacteremia among elderly vs younger adult patients: Comparison of clinical features and mortality. Arch. Intern. Med. 159, 1244–1247. doi:10.1001/archinte.159.11.1244
Mestre, M. B., and Colombo, M. I. (2012). CAMP and EPAC are key players in the regulation of the signal transduction pathway involved in the α-hemolysin autophagic response. PLoS Pathog. 8, e1002664. doi:10.1371/journal.ppat.1002664
Mizushima, N., Levine, B., Cuervo, A. M., and Klionsky, D. J. (2008). Autophagy fights disease through cellular self-digestion. Nature 451, 1069–1075. doi:10.1038/nature06639
Mosser, D. M., and Edwards, J. P. (2008). Exploring the full spectrum of macrophage activation. Nat. Rev. Immunol. 8, 958–969. doi:10.1038/nri2448
Muñoz-Espín, D., and Serrano, M. (2014). Cellular senescence: From physiology to pathology. Nat. Rev. Mol. Cell Biol. 15, 482–496. doi:10.1038/nrm3823
Narita, M., Nũnez, S., Heard, E., Narita, M., Lin, A. W., Hearn, S. A., et al. (2003). Rb-mediated heterochromatin formation and silencing of E2F target genes during cellular senescence. Cell 113, 703–716. doi:10.1016/S0092-8674(03)00401-X
Numrich, J., and Ungermann, C. (2014). Endocytic Rabs in membrane trafficking and signaling. Biol. Chem. 395, 327–333. doi:10.1515/hsz-2013-0258
Park, S. C. (2002). Functional recovery of senescent cells through restoration of receptor-mediated endocytosis. Mech. Ageing Dev. 123, 917–926. doi:10.1016/S0047-6374(02)00029-5
Park, S. C., Park, J. S., Park, W. Y., Cho, K. A., Ahn, J. S., and Jang, I. S. (2002). Down-regulation of receptor-mediated endocytosis is responsible for senescence-associated hyporesponsiveness. Ann. N. Y. Acad. Sci. 959, 45–49. doi:10.1111/j.1749-6632.2002.tb02081.x
Park, W. Y., Cho, K. A., Park, J. S., Kim, D. I., and Park, S. C. (2001b). Attenuation of EGF signaling in senescent cells by caveolin. Ann. N. Y. Acad. Sci. 928, 79–84. doi:10.1111/j.1749-6632.2001.tb05638.x
Park, W. Y., Park, W.-Y., Cho, K.-A., Kim, D.-I., Jhun, B.-H., Kim, S.-R., et al. (2001a). Down-regulation of amphiphysin-1 is responsible for reduced receptor-mediated endocytosis in the senescent cells. FASEB J. 15, 1625–1627. doi:10.1096/fj.00-0723fje
Pawelec, G. (2012). Hallmarks of human “immunosenescence”: Adaptation or dysregulation? Immun. Ageing 9, 15. doi:10.1186/1742-4933-9-15
Rabinovitch, M. (1995). Professional and non-professional phagocytes: An introduction. Trends Cell Biol. 5, 85–87. doi:10.1016/S0962-8924(00)88955-2
Rai, T. S., and Adams, P. D. (2012). Lessons from senescence: Chromatin maintenance in non-proliferating cells. Biochim. Biophys. Acta - Gene Regul. Mech. 1819, 322–331. doi:10.1016/j.bbagrm.2011.07.014
Roy, A. L., Sierra, F., Howcroft, K., Singer, D. S., Sharpless, N., Hodes, R. J., et al. (2020). A blueprint for characterizing senescence. Cell 183, 1143–1146. doi:10.1016/j.cell.2020.10.032
Schnaith, A., Kashkar, H., Leggio, S. A., Addicks, K., Krönke, M., and Krut, O. (2007). Staphylococcus aureus subvert autophagy for induction of caspase-independent host cell death. J. Biol. Chem. 282, 2695–2706. doi:10.1074/jbc.M609784200
Schneider, E. L. (1983). Infectious diseases in the elderly. Ann. Intern. Med. 98, 395–400. doi:10.7326/0003-4819-98-3-395
Scott, C. C., Vacca, F., and Gruenberg, J. (2014). Endosome maturation, transport and functions. Semin. Cell Dev. Biol. 31, 2–10. doi:10.1016/j.semcdb.2014.03.034
Shin, E. Y., Soung, N. K., Schwartz, M. A., and Kim, E. G. (2021). Altered endocytosis in cellular senescence. Ageing Res. Rev. 68, 101332. doi:10.1016/j.arr.2021.101332
Tacconelli, E., Pop-Vicas, A. E., and D’Agata, E. M. C. (2006). Increased mortality among elderly patients with meticillin-resistant Staphylococcus aureus bacteraemia. J. Hosp. Infect. 64, 251–256. doi:10.1016/J.JHIN.2006.07.001
Tang, Q., Zheng, G., Feng, Z., Chen, Y., Lou, Y., Wang, C., et al. (2017). Trehalose ameliorates oxidative stress-mediated mitochondrial dysfunction and ER stress via selective autophagy stimulation and autophagic flux restoration in osteoarthritis development. Cell Death Dis. 8, e3081. doi:10.1038/CDDIS.2017.453
Thorlacius-Ussing, L., Sandholdt, H., Larsen, A. R., Petersen, A., and Benfield, T. (2019). Age-dependent increase in incidence of staphylococcus aureus bacteremia, Denmark, 2008–2015. Emerg. Infect. Dis. 25, 875–882. doi:10.3201/eid2505.181733
Toussaint, O., Houbion, A., and Remacle, J. (1992). Aging as a multi-step process characterized by a lowering of entropy production leading the cell to a sequence of defined stages. II. Testing some predictions on aging human fibroblasts in culture. Mech. Ageing Dev. 65, 65–83. doi:10.1016/0047-6374(92)90126-X
Toussaint, O., Medrano, E. E., and Von Zglinicki, T. (2000). Cellular and molecular mechanisms of stress-induced premature senescence (SIPS) of human diploid fibroblasts and melanocytes. Exp. Gerontol. 35, 927–945. doi:10.1016/S0531-5565(00)00180-7
Touyz, R. M., Tolloczko, B., and Schiffrin, E. L. (1994). Mesenteric vascular smooth muscle cells from spontaneously hypertensive rats display increased calcium responses to angiotensin II but not to endothelin-1. J. Hypertens. 12, 663–674.
Uribe-Querol, E., and Rosales, C. (2020). Phagocytosis: Our current understanding of a universal biological process. Front. Immunol. 11, 1066. doi:10.3389/fimmu.2020.01066
Vanrell, M. C., Martinez, S. J., Muñoz, L. I., Salassa, B. N., Gambarte Tudela, J., and Romano, P. S. (2022). Induction of autophagy by ursolic acid promotes the elimination of trypanosoma cruzi amastigotes from macrophages and cardiac cells. Front. Cell. Infect. Microbiol. 12, 919096. doi:10.3389/FCIMB.2022.919096
Vardi, M., Ghanem-Zoubi, N. O., Bitterman, H., Abo-Helo, N., Yurin, V., Weber, G., et al. (2013). Sepsis in nonagenarians admitted to internal medicine departments: A comparative study of outcomes. QJM 106, 261–266. doi:10.1093/qjmed/hcs221
Wandinger-Ness, A., and Zerial, M. (2014). Rab proteins and the compartmentalization of the endosomal system. Cold Spring Harb. Perspect. Biol. 6, a022616. doi:10.1101/cshperspect.a022616
Wang, C., Mao, C., Lou, Y., Xu, J., Wang, Q., Zhang, Z., et al. (2018). Monotropein promotes angiogenesis and inhibits oxidative stress-induced autophagy in endothelial progenitor cells to accelerate wound healing. J. Cell. Mol. Med. 22, 1583–1600. doi:10.1111/jcmm.13434
Weischenfeldt, J., and Porse, B. (2008). Bone marrow-derived macrophages (BMM): Isolation and applications. Cold Spring Harb. Protoc. 3, pdb.prot5080. doi:10.1101/pdb.prot5080
Weisheit, C. K., Engel, D. R., and Kurts, C. (2015). Dendritic cells and macrophages: Sentinels in the kidney. Clin. J. Am. Soc. Nephrol. 10, 1841–1851. doi:10.2215/CJN.07100714
Zhang, X., Goncalves, R., and Mosser, D. M. (2008). The isolation and characterization of murine macrophages. Curr. Protoc. Immunol. 14, 14.1.1–14.1.14. doi:10.1002/0471142735.im1401s83
Keywords: aging, senescence, endophagocytic pathway, Staphylococcus aureus, phagocytosis
Citation: Robledo E, Benito Rodriguez PG, Vega IA, Colombo MI and Aguilera MO (2023) Staphylococcus aureus phagocytosis is affected by senescence. Front. Aging 4:1198241. doi: 10.3389/fragi.2023.1198241
Received: 31 March 2023; Accepted: 03 July 2023;
Published: 31 July 2023.
Edited by:
David Otaegui, Biodonostia Health Research Institute (IIS Biodonostia), SpainReviewed by:
Clara Correia-Melo, Charité University Medicine Berlin, GermanyEdward Fielder, Newcastle University, United Kingdom
Copyright © 2023 Robledo, Benito Rodriguez, Vega, Colombo and Aguilera. This is an open-access article distributed under the terms of the Creative Commons Attribution License (CC BY). The use, distribution or reproduction in other forums is permitted, provided the original author(s) and the copyright owner(s) are credited and that the original publication in this journal is cited, in accordance with accepted academic practice. No use, distribution or reproduction is permitted which does not comply with these terms.
*Correspondence: Milton Osmar Aguilera, maguilera@mendoza-conicet.gob.ar; María Isabel Colombo, mcolombo@fcm.uncu.edu.ar