- Department of Health Sciences and Technology, ETH Zurich, Zurich, Switzerland
With an increasing aging population, the burden of age-related diseases magnifies. To alleviate this burden, geroprotection has been an area of intense research focus with the development of pharmacological interventions that target lifespan and/or healthspan. However, there are often sex differences, with compounds mostly tested in male animals. Given the importance of considering both sexes in preclinical research, this neglects potential benefits for the female population, as interventions tested in both sexes often show clear sexual dimorphisms in their biological responses. To further understand the prevalence of sex differences in pharmacological geroprotective intervention studies, we performed a systematic review of the literature according to the PRISMA guidelines. Seventy-two studies met our inclusion criteria and were classified into one of five subclasses: FDA-repurposed drugs, novel small molecules, probiotics, traditional Chinese medicine, and antioxidants, vitamins, or other dietary supplements. Interventions were analyzed for their effects on median and maximal lifespan and healthspan markers, including frailty, muscle function and coordination, cognitive function and learning, metabolism, and cancer. With our systematic review, we found that twenty-two out of sixty-four compounds tested were able to prolong both lifespan and healthspan measures. Focusing on the use of female and male mice, and on comparing their outcomes, we found that 40% of studies only used male mice or did not clarify the sex. Notably, of the 36% of pharmacologic interventions that did use both male and female mice, 73% of these studies showed sex-specific outcomes on healthspan and/or lifespan. These data highlight the importance of studying both sexes in the search for geroprotectors, as the biology of aging is not the same in male and female mice.
Systematic Review Registration: [website], identifier [registration number].
Introduction
The world population is aging. Life expectancy has increased by 30 years over the last century (Olshansky, 2018) and in 2018, people over 65 years of age outnumbered children below 5 years for the first time (Shetty, 2012; United Nations, 2019). This demographic shift is predicted to continue, as the number of people over 65 years old is expected to triple by 2050 (Shetty, 2012). The extension of human lifespan does not always guarantee an extension of healthspan (defined as the period free from disease), as the two are not necessarily linked (Prince et al., 2015; Fischer et al., 2016; Hansen and Kennedy, 2016; Mitchell et al., 2016; Atella et al., 2019; GBD Ageing Collaborators, 2022; GBD Ageing Collborators, 2022; Statzer et al., 2022). This is demonstrated by a global increase in disease burden related to old age which goes hand in hand with the rise of the aging population (Prince et al., 2015; Atella et al., 2019; GBD Ageing Collborators, 2022; GBD Ageing Collborators, 2022). Research has focused on understanding the biological mechanisms of aging in hope of finding ways to extend lifespan and healthspan (Sinclair, 2005; Sierra, 2016; Weir et al., 2017; Olshansky, 2018). For many, extending the years lived in good health with a reduced burden of chronic diseases is a more actionable and perhaps more attractive goal than an extended lifespan (Sierra, 2016; Olshansky, 2018; Mitchell et al., 2019; Aon et al., 2020).
While lifespan was classically considered the gold standard for determining the success of geroprotectors, over the last 10 years, researchers have started to include differential measures of healthspan in their studies. The concept of frailty as a state of overall decline is increasingly utilized to assess the risk of disease and mortality in old age (Kane and Howlett, 2017; Rockwood et al., 2017; Palliyaguru et al., 2019). Tools for assessing frailty in mice (Liu et al., 2014; Whitehead et al., 2014; Hession et al., 2022), which have been reverse-translated from human scales, have become more widely utilized in recent years as markers of healthspan (Sukoff Rizzo et al., 2018; Bellantuono et al., 2020; Palliyaguru et al., 2021a) and have been shown to be modifiable by dietary and pharmacological interventions that increase lifespan (Kane et al., 2016; Palliyaguru et al., 2019). Beyond the mouse frailty index, other important assays to assess health broadly span the domains of muscle function and coordination, cognitive function and memory, metabolic function, and tumor incidence (Ackert-Bicknell et al., 2015; Bellantuono et al., 2020). While there is no established stringent set of measures agreed upon by the entire community to fully define healthspan in mice, a number of important publications have established at least a panel of markers with demonstrated utility in the assessment of healthspan (Richardson et al., 2016; Bellantuono et al., 2020).
Interventions to increase lifespan and healthspan comprise behavioral, dietary, and pharmacological approaches (Longo et al., 2015), and are commonly referred to as geroprotectors (Moskalev et al., 2016). Potential geroprotectors are defined as interventions which may extend lifespan and/or healthspan by targeting one or more of the hallmarks of aging (Moskalev et al., 2016; Janssens and Houtkooper, 2020; López-Otín et al., 2023). Examples of successful geroprotectors include rapamycin and metformin (Martin-Montalvo et al., 2013; Bitto et al., 2016; Glossmann and Lutz, 2019; Selvarani et al., 2020; Moskalev et al., 2022). The development of geroprotectors is based on the “Geroscience hypothesis” (Sierra and Kohanski, 2017), in which aging plays a central role in many, if not all, chronic diseases. Interventions that retard aging should simultaneously delay the onset of many diseases according to this hypothesis. This foundational framework has proposed a roadmap for how geroprotectors should impact aging. A number of recent reviews have eloquently described the role of dietary interventions as potential geroprotectors (Brandhorst and Longo, 2019; Green et al., 2022; Longo and Anderson, 2022; Mitchell and Mitchell, 2022), so they will not be included here.
It is well-known that there are sexual dimorphisms in the aging process, including in healthspan, muscle mass maintenance and physical performance, sex-hormones, age-related diseases and lifespan (Austad and Fischer, 2016; Le Couteur et al., 2018; Sampathkumar et al., 2019; Decaroli et al., 2021; Bronikowski et al., 2022; Viecelli and Ewald, 2022; Della Peruta et al., 2023). It is noteworthy that men and women have different susceptibility to various age-related diseases, such as women being more likely to develop osteoporosis, and men being more prone to cardiovascular diseases (Crimmins et al., 2019). This is partly influenced by sex-specific alterations in sex hormones with age, including a decrease in estrogen levels during menopause for women and a decline in testosterone with age for men (Guarner-Lans et al., 2011; Horstman et al., 2012; Decaroli et al., 2021). Further, women and females of other species tend to have significantly longer lifespans, but experience higher levels of frailty at a given age when assessed clinically (Le Couteur et al., 2018; Gordon and Hubbard, 2019; Kane and Howlett, 2021). In mice, sex-related differences can be seen in physical performance, which was shown to be lower in aging males (Tran et al., 2021), while anxiety-like behaviors were increased in aging males (Kobayashi et al., 2021). Even on the tissue and molecular level, there are vast sex-specific differences in mice’s gene expression signatures associated with longevity (Vitiello et al., 2021). Further, sexual dimorphisms can be observed in geroprotective interventions aiming to increase lifespan or healthspan (Sampathkumar et al., 2019). Both dietary and pharmacological interventions, such as rapamycin and calorie restriction, have been shown to have sexually dimorphic effects when tested in mice (Anisimov et al., 2010; Harrison et al., 2014; Miller et al., 2014; Mitchell et al., 2016; Bielas et al., 2018; Cabo and Mattson, 2019; Sampathkumar et al., 2019; Berry et al., 2020; Henderson et al., 2021). These findings suggest underlying biological differences in the mechanisms of aging between the sexes and highlight the importance of considering sex as a biological variable. Despite a 2016 NIH mandate requiring both sexes to be used in preclinical research (NOT-OD-15-102, 2015: Consideration of Sex as a Biological Variable in NIH-funded Research,” 2015), many fields, including the aging field, still face challenges to the inclusion of both sexes in their studies (Plevkova et al., 2020; Shansky and Murphy, 2021; Carmody et al., 2022; Merone et al., 2022). To comprehensively compile the current literature and provide a summary of findings, we performed a systematic review of original research publications from 1970 to 2022 and reviewed what is known about sexual dimorphisms in the lifespan and healthspan outcomes of mice undergoing some form of pharmacological intervention. Our findings are presented herein.
Methods
A systematic review of the literature was conducted according to the PRISMA guidelines (Page et al., 2021) to identify publications reporting on pharmacological interventions in mice and their effects on lifespan and/or healthspan, in a sexually dimorphic manner. PubMed (RRID:SCR_004846) was utilized as the search tool and database to screen the title, abstract, and keywords of all articles (excluding reviews) using the search terms with Boolean operators as outlined in Table 1. The search period was limited to all published within the period of 1970 to 1. January 2022. All identified records were exported to excel, where the authors (M.K. and S.J.M) screened them for the eligibility criteria and removed duplicate records, irrelevant titles/abstracts, as well as non-original research (re-analysis of previously published data, commentaries, etc.). To ensure all relevant research was included, an additional manual review of the literature was performed via PubMed, which produced four further studies. Of the remaining potential records, the full-text articles were screened against the exclusion criteria (Table 1) and the resulting 72 eligible articles were used as the basis for this systematic review. Figure 1 shows the workflow for the literature search and study selection, including the identification of the final 150 full-text articles, of which 72 were included in the final analysis. From the final articles, we extracted all relevant information for this review. This included details of the study design as well as the study outcomes for lifespan and healthspan parameters. For the study design, the compound used in the intervention, its dose and method of administration, the age at onset of the intervention and its duration, and the mouse strain used were recorded. The lifespan measures were separated into measures of median lifespan and maximal lifespan due to inherent differences in how authors report these findings. Due to the diversity of the healthspan measurements in mice, we defined healthspan parameters according to published recommendations (Richardson et al., 2016; Bellantuono et al., 2020) to include frailty, muscle function and coordination, cognitive function and memory, metabolic function, and cancer incidence. The limitation of these assays is the missing consensus of what measure(s) reliably demonstrate improvements in healthspan. For all outcomes it was reported whether there was a significant improvement (↑) or worsening (↓) of the parameter during the study in the intervention group relative to the control group stratified by sex. We also reported whether there was a sexual dimorphism (defined as opposing directionalities of the effect, i.e., improved in males, worsened in females) in the measured outcome. If the outcome was not reported in the respective study this was denoted with a “n.m.” (not measured). For median and maximal lifespan, p-values were added if reported by the authors. All the information was then structured according to the drug class of the compound used, covering repurposed FDA drugs, novel small molecules, probiotics, traditional Chinese medicine, and supplements, including vitamins and antioxidants.
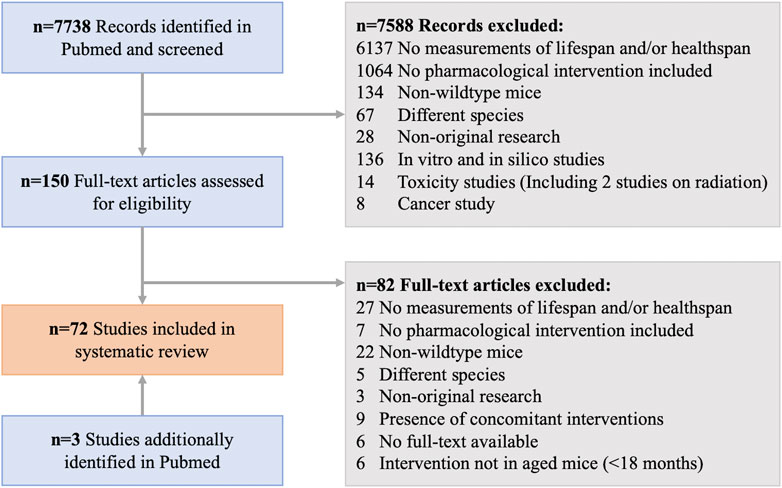
FIGURE 1. Workflow for the systematic review from identified records to all included studies in this manuscript.
Results
The final 72 eligible studies (Figure 1) that were included in this review and published between 1970 and 2022 reveal that 36% (26/72) included both female and male mice in their research, while 33% (24/72) used only male mice and 20% (14/72) used only female mice (Figure 2). Out of the 26 studies including both sexes, a large part showed sex-specific results (19/26). Next to measurements of lifespan, a wide variety of healthspan metrics started to be included in studies from the year 2000 onwards, with a continuous increase in their implementation over time. All but one study conducted since 2020 included some sort of healthspan parameter. Of all compounds tested, 22 out of 64 were able to show positive effects on both lifespan and healthspan measures.
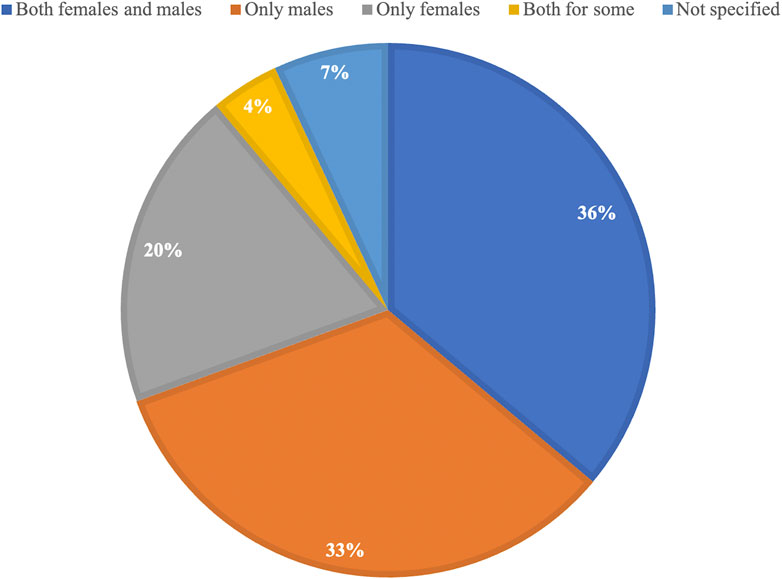
FIGURE 2. Proportional representation of the use of female and male mice in all studies included in this manuscript.
Repurposed FDA drugs
Repurposing FDA drugs for use in age-related diseases has been a popular strategy for identifying new geroprotectors (Table 2). One of the attractive benefits of this strategy is the wealth of pharmacology, safety, and efficacy data already available for these compounds. This means investigators can direct resources to validating the compound in the appropriate model, rather than re-hashing safety data that already exists. Many proposed geroprotectors have been tested in the NIA Interventions Testing Program (ITP) in the United States (Miller et al., 2007). This program solicits investigator-proposed compounds and tests their lifespan potential in genetically heterogeneous UM-HET3 mice. Started in the early 2000s, this program to date has tested more than 60 different compounds.
One of the most promising compounds that came out of the ITP is rapamycin. Rapamycin has potent antitumor and immunosuppressive activity and was originally discovered in soil samples from the Easter Island. In mice, rapamycin has been tested at various doses, as well as at different ages of onset. Most data are consistent with the notion that rapamycin extends lifespan in both males and females, with stronger effects shown in females (Harrison et al., 2009; Miller et al., 2011; Miller et al., 2014; Zhang et al., 2014; Strong et al., 2020). Healthspan data supports the concept that age-related deficits are mitigated with rapamycin treatment (Flynn et al., 2013; Neff et al., 2013; Zhang et al., 2014), although specific tests may or may not show sexually dimorphic results (Table 2). Recent work has demonstrated that rapamycin treatment in the first 45 days of life is sufficient to improve healthspan, reduce frailty and extend median lifespan, at least in males (Shindyapina et al., 2022). Moreover, rapamycin treatment for 3 months during middle age (20–21 months) increased median lifespan by 14% for males and 9% for females (Bitto et al., 2016). This data highlights the importance of considering the age of onset of these therapeutics and that lifelong treatment may not be necessary. Other drugs tested in the ITP include aspirin, canagliflozin, candesartan, metformin, sibutramine, simvastatin, and acarbose (Strong et al., 2008; Miller et al., 2011; Smith et al., 2011; Harrison et al., 2014; Smith et al., 2019; Miller et al., 2020; Harrison et al., 2021). Canagliflozin, a diabetes drug, showed sexually dimorphic effects on lifespan, with an increase in both median and maximal lifespan of 14% and 9%, respectively, only in males (Miller et al., 2020). In a parallel study, canagliflozin was found to retard age-related lesions in males only, suggesting that the lifespan extension in the treated males is likely a reflection of delay in lethal neoplasms (Snyder et al., 2022). Interestingly, another diabetes drug, metformin, did not show lifespan extension in genetically heterogeneous males but did have small but significant effects on median lifespan in C57BL/6 J males, and a trend towards an effect in B6C3F1 male mice (Martin-Montalvo et al., 2013). Metformin at 0.1% improved markers of health in these mice, however, it must be noted that 1% metformin caused significant kidney damage and significantly reduced lifespan by 14% (Martin-Montalvo et al., 2013). Others have also tested metformin and shown that 1% metformin improved median and maximal lifespan in female SHR mice by 91.9% and 10.3%, respectively (Anisimov et al., 2008). When tested in 129/sv mice, the same concentration improved median lifespan in females by 7.8% but reduced it by 13.4% in males. In a recent study in female mice of an unknown strain, 1% metformin reduced their median lifespan (Zhu et al., 2020). Taken together, there are clear sexual dimorphic effects of metformin in different mouse strains on lifespan, with a lack of a clear directionality effect across strains. Several studies were able to show positive healthspan effects of metformin doses ranging from 1% to 5% in C57BL/6 J mice (Martin-Montalvo et al., 2013; Palliyaguru et al., 2020), illustrating the uncoupling of lifespan and healthspan outcomes. A third diabetes drug, acarbose, showed promising effects on lifespan in both female and male genetically heterogenous mice, with larger effects in males (Harrison et al., 2014; Smith et al., 2019). Healthspan was not tested in these studies. Aspirin, a classic anti-inflammatory drug, extended median lifespan in male, but not in female UM-HET3 mice (Strong et al., 2008). No effects on lifespan or healthspan were shown by the drugs Candesartan (Harrison et al., 2021), an antihypertensive drug, Sibutramine (Smith et al., 2011), an appetite suppressant, or Simvastatin (Miller et al., 2011), a statin reducing cholesterol. As these compounds were tested as part of the ITP, healthspan measures were not included in these studies.
Drugs that were tested outside of the ITP include tranexamic acid, Deltaran, Etanercept, L-Dopa, and Prednisolone. Deltaran, Etanercept, and Prednisolone are all anti-inflammatory drugs that were tested in female mice, from which the first two had positive effects on lifespan and healthspan (Popovich et al., 2003; Sciorati et al., 2020). Prednisolone showed no effects on lifespan (Forbes, 1975). L-Dopa, a precursor to the neurotransmitters dopamine, noradrenaline, and adrenaline, showed positive effects on male lifespan but had no impact on healthspan (Cotzias et al., 1974). Tranexamic acid, an antifibrinolytic, positively impacted male lifespan and healthspan parameters (Hiramoto et al., 2020; 2019).
Overall, using repurposed FDA drugs as geroprotectors is a promising strategy. Still, more research is needed to determine the optimal doses, ages of onset, and specific indications for these drugs, as well as the effectiveness in both sexes.
Novel small molecules
Beyond repurposing already approved drugs, a common approach in drug development is developing novel small molecules, which allows for a more target-specific approach. Examples of pathways that novel small molecule may target in the aging field, include oxidative stress, inflammation, AMPK, or senescence (Table 3). These are some of the processes implicated as hallmarks of aging (López-Otín et al., 2023).
Promising results have been shown with a carboxy-fullerene superoxide dismutase (SOD) mimetic and 17-α-estradiol. The SOD mimetic with its antioxidant properties was able to extend female and male lifespans by 11% and improved the mice’s cognition and learning (Quick et al., 2008). In the ITP, 17-α-estradiol, a synthetic form of the hormone estradiol with proposed neuroprotective properties, has been found to extend lifespan in male mice in repeated studies but not in females (Harrison et al., 2014; Harrison et al., 2021). Lifespan effects have ranged from a median lifespan increase in male mice of 12% (Harrison et al., 2014) up to 19% (Harrison et al., 2021). While healthspan was not measured in these two studies, independent studies have shown that healthspan benefits are seen in both male rats and mice with 17-a-estradiol (Mann et al., 2020), highlighting the importance of cross-species validation of potential geroprotectors.
Two small molecules, SRT1720 and SRT2104, which were developed as specific sirtuin 1 (SIRT1) activators, have shown benefits in both healthspan and lifespan measures in both a high-fat diet (HFD) background, as well as a standard diet background. Mitchell et al. found that SRT1720 improved several measures of healthspan in male mice as well as mean lifespan, but only a trend towards increasing median lifespan (Mitchell et al., 2014). More striking effects were seen when HFD-fed mice were treated with SRT1720 (Minor et al., 2011). Although in a second study of SRT1720 on an HFD, bodyweight and rotarod performance were not significantly different from the control group (Palliyaguru et al., 2020). A significant limitation of this work was that these compounds were not tested in female mice, thereby limiting the generalizability of these results.
Age-related changes in the immune system, often referred to as “inflamm-aging” (Franceschi et al., 2018), contribute to the pathogenesis of many age-related diseases. Alpha-fetoprotein, an immunoregulator, improved muscle function and coordination in female mice (Krut’ko et al., 2016). The anti-inflammatory molecules MIF098 (Harrison et al., 2021), dimethylaminomicheliolide (Sun et al., 2019), and nitroflurbiprofen (Strong et al., 2008), as well as the antioxidant 4-OH-PBN (Strong et al., 2008) and the insulin sensitivity promoting geranylgeranyl-acetone (Harrison et al., 2021) showed no effect on lifespan when tested in male and female UM-HET3 mice. Beta-guadinidinopropionic acid, an AMPK activator (Dorigatti et al., 2021), showed improvements in muscle function and coordination independent of sex when measured with gait and rotarod performance, but not with grip strength or exercise tolerance tests, while lifespan was not measured.
Probiotics
We only identified one probiotic, Akkermansia muciniphila, that has been tested as a potential geroprotector and fulfilled the criteria to be included in this review. Two studies, displayed in Table 4, found that Akkermansia muciniphila had no effect on lifespan, but did show minor improvements in healthspan measures such as frailty, muscle function, and cognitive function in female mice (Cerro et al., 2021; Shin et al., 2021). Further research is needed to fully understand the potential of A. muciniphila and other probiotics as potential geroprotectors including determining the optimal dosage and administration for use in humans.
Traditional Chinese medicine
Traditional Chinese medicine (TCM) is another field where researchers have tested compounds for their effects on longevity and healthspan (Zhao and Luo, 2017) (Table 5). TCM has a long history, and many herbs and their components are being studied now in a variety of diseases where they show beneficial effects, including aging (Chen et al., 2019; Bi et al., 2022; Xue et al., 2022). The flavanol Icariin, an ingredient of the herb Epimedium, improved the median lifespan in male mice by 8%, accompanied by improved muscle function and coordination. These beneficial effects could be attributed to its purported anti-inflammatory and anti-oxidant properties (Zhang et al., 2015; Bi et al., 2022). A late-onset treatment (22–23 months of age) with Liuwei Dihuang, an anti-oxidant TCM formula comprised of six different herbs, increased maximal lifespan significantly with a dose of 4 ppm, but showed no significant effect at a higher dose of 7 ppm (Chen et al., 2019). Healthspan parameters, including frailty and cognitive function, were improved by extracts of the medicinal mushroom Hericium erinaceus, when given for 2 months to 21–23 months old male mice (Roda et al., 2021). Their healthspan measure was an alternative version of a frailty index, the locomotor frailty index which is determined via average speed and resting time. Their study found a 10% reduction in frailty in the treatment group and no sex-specific effects were observed. In a similar study design, the anti-oxidant acer truncatum seed oil was shown to improve cognitive function in male mice as measured with the Morris Water Maze test (Li et al., 2021). These results are promising and show the potential of medicinal herbs and their bioactive components as geroprotectors. However, further research is needed to understand what exactly is mediating the beneficial effects, especially in formulas such as Liuwei Dihuang, which mixes six different herbs.
Vitamins, supplements, antioxidants, and other compounds
Many vitamins, supplements, and antioxidants have been studied for their ability to improve healthspan and/or lifespan, as shown in Table 6. Interestingly, the strains of mice used in these studies vary beyond just the standard C57BL/6 mice commonly used in biomedical and aging studies (Palliyaguru et al., 2021b). In the case of SkQ1, for example, the authors used three different strains of mice (Anisimov et al., 2011) which ensures any effects observed are tested across different genetic backgrounds. A number of these compounds, which were tested in UM-HET3 mice as part of the Interventions Testing Program, did not have any significant effects on lifespan outcomes in either sex (Miller and Chrisp, 1999; Strong et al., 2013), with the exceptions of methylene blue and nordihydroguaiaretic acid (NHGA) showing sexually dimorphic lifespan effects (Strong et al., 2008; Harrison et al., 2014). Methylene blue improved lifespan only in female mice (Harrison et al., 2014), while NHGA improved only male lifespan (Strong et al., 2008; Harrison et al., 2014). Healthspan metrics were not measured in these animals. Additionally, glycine, which was also tested as part of the Interventions Testing Program, increased median lifespan by 4% in females and by 6% in males and significantly reduced the risk for lung adenocarcinomas (Miller et al., 2019).
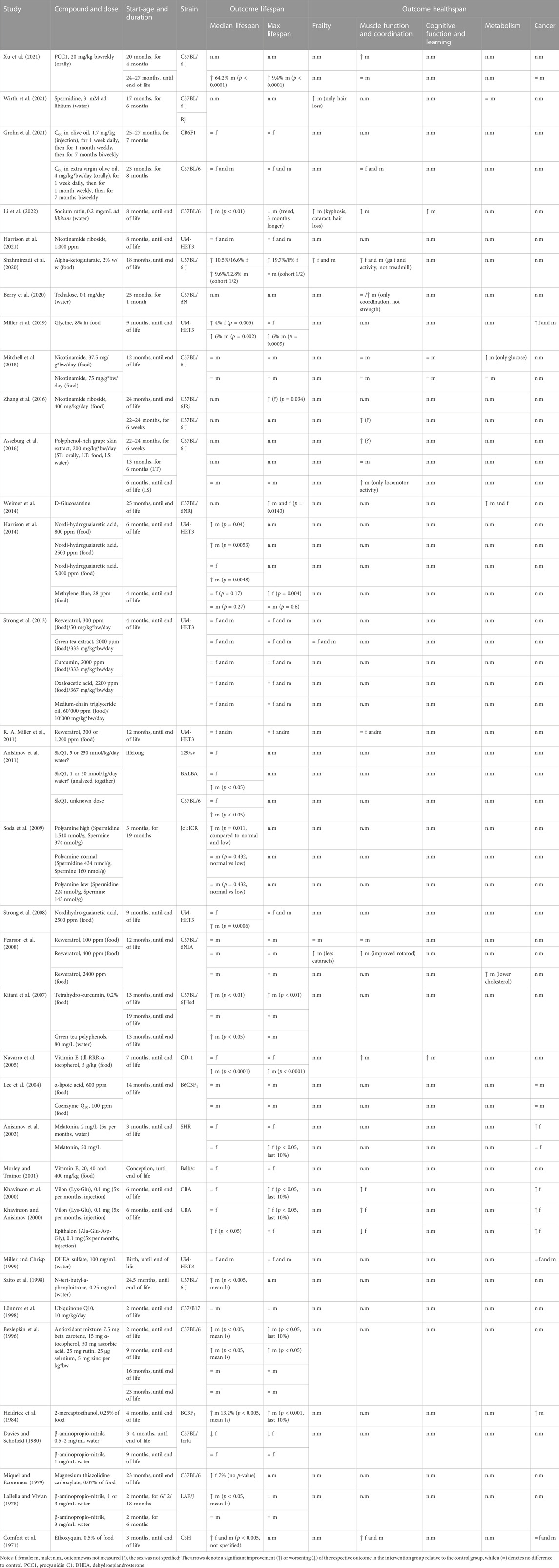
TABLE 6. Study details and results for interventions with vitamins, supplements, antioxidants, and other compounds.
Of the studies that used standard C57BL/6 mice, several found improvements in lifespan as well as healthspan metrics. Alpha-ketoglutarate was tested in both female and male mice and showed positive effects on lifespan and healthspan in a sex-independent manner, although stronger lifespan effects were observed in female mice (Shahmirzadi et al., 2020). Healthspan showed similar effects in both sexes, with particularly good improvements in female fur color. A sex-independent increase in median and maximal lifespan was also achieved with D-glucosamine, with a treatment onset at 25 months, and additional improvements in glucose metabolism were observed (Weimer et al., 2014). Treatment with procyanidin C1 (PCC1) from grape seeds (Xu et al., 2021), as well as treatment with sodium rutin, a flavonoid (Li et al., 2022), in male mice increased lifespan and several measures of healthspan, such as frailty, muscle function, and cognitive function. Multiple other studies measuring solely lifespan in C57BL/6 mice showed improvements with compounds including antioxidants and polyphenol mixtures, however, most were tested in male mice only (Bezlepkin et al., 1996; Saito et al., 1998; Kitani et al., 2007). Future studies with these compounds should involve healthspan outcomes as well as validating findings in female mice.
Declining NAD levels with age are thought to be one contributor to age-related degeneration (Gomes et al., 2013; McReynolds et al., 2020), with supplementation of NAD precursors evaluated as therapeutic avenues. In C57BL/6 mice, 400 ppm of nicotinamide riboside increased the maximal lifespan as well as muscle function and coordination in mice when given late in life (22–24 months) (Zhang et al., 2016), however, sex of the mice in this study was not specified. A higher dose of 1,000 ppm of nicotinamide riboside started early in life (8 months) did not improve lifespan parameters in female and male UM-HET3 mice (Harrison et al., 2021). Supplementation with nicotinamide started at 12 months improved glucose metabolism but was also not able to improve lifespan parameters in male C57BL/6 mice (Mitchell et al., 2018).
The already mentioned SkQ1, which was tested in different genetic backgrounds, showed sexually dimorphic effects in C57BL/6 mice as well as BALB/c mice, where it was only able to extend male, but not female lifespan (Anisimov et al., 2011). Of the compounds in this category, magnesium thiazolidine carboxylate was the only compound that extended female median lifespan, even though p-values are missing in this study (Miquel and Economos, 1979). Studies testing spermidine, trehalose, polyphenol-rich grape skin extract, and resveratrol found improvements in several healthspan metrics in male C57BL/6 mice (Pearson et al., 2008; Asseburg et al., 2016; Berry et al., 2020; Wirth et al., 2021), however, they either observed no effects on lifespan (Pearson et al., 2008; Asseburg et al., 2016; Wirth et al., 2021), or lifespan was not measured (Berry et al., 2020). Of note, a number of these studies included males only. No effects on lifespan or healthspan could be shown with C60 in olive oil in either female or male C57BL/6 mice (Grohn et al., 2021). Interestingly, b-aminopropionitrile was shown to reduce female lifespan (Davies and Schofield, 1980), despite an earlier study reporting it increased lifespan in male LAF/J mice (LaBella and Vivian, 1978). This highlights the importance of using both sexes and a variety of mouse strains. Studies on vitamin E discovered an interesting sexual dimorphism, as it only had an effect on male, but not female lifespan (Morley and Trainor, 2001; Navarro et al., 2005). Vilon and Epithalon, both synthetic peptides, were shown to significantly improve female lifespan as well as multiple metrics of healthspan (Khavinson and Anisimov, 2000; Khavinson et al., 2000). Anisimov et al. also observed an interesting effect of melatonin on uncoupling lifespan and healthspan. At a low dose (2 mg/L), tumor incidence was reduced, but lifespan was unaffected; meanwhile at a higher dose (20 mg/L), lifespan was increased, but tumor incidence was unaffected (Anisimov et al., 2003). Dose-dependent effects were also observed by Soda and colleagues (Soda et al., 2009) when testing a combination of polyamines (spermidine and spermine), with the highest dose improving lifespan when compared to lower doses, which were solely tested in male mice. Further, 2-mercapto-ethanol was shown to improve lifespan and healthspan, but it was only tested in male mice (Heidrick et al., 1984). Ethoxyquin, a quinoline-based antioxidant, was shown to improve lifespan and healthspan in both males and females (Comfort et al., 1971). However, interventions with ubiquinone (Lönnrot et al., 1998; Lee et al., 2004) and alpha-lipoic acid in male mice did not show any effects on lifespan (Lee et al., 2004). While some of the discussed compounds have shown promising results in extending lifespan and improving healthspan in mice, most studies have only been conducted on one sex, leaving questions about their potential benefit on the opposite sex.
Adherence to the SABV mandate for preclinical studies
Since 2020, the NIH mandate has been in place, which requires authors to include both males and females in NIH-funded preclinical research studies. Of the 17 studies published since 2020 included in this systematic review, only six used both female and male mice and compared their outcomes (35%) (Figure 3). This is the same number of studies that used only male mice for their research (35%). When looking at the proportion of studies that used both sexes in all included studies, which is 36% (26/72) (Figure 2), nothing has changed despite the mandate being in place. This highlights the need for journals to further encourage or require compliance with the mandate and to promote the integration of sex as a biological variable in preclinical research studies. This could lead to a better understanding of the potential sex-specific differences in the outcomes of these studies and lead to improved treatments for all patients, regardless of their biological sex. It is also important for researchers to be aware of the potential impact of sex on the outcomes of their studies and to design studies that accurately represent the populations they aim to serve.
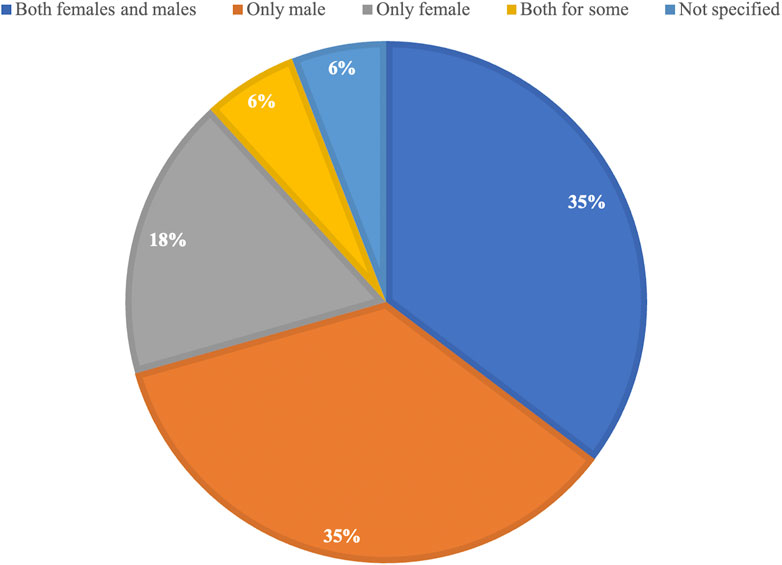
FIGURE 3. Proportional representation of the use of female and male mice in all studies included in this manuscript since 2020.
Discussion
The goal of this manuscript was to systematically review the available literature on sexual dimorphism in the use of pharmacological compounds as potential geroprotectors. We focused on lifespan and healthspan outcomes using mice as a model organism. Of the more than 7000 potentially eligible studies identified through our search, only 72 original research publications met the stringent inclusion and exclusion criteria.
Our results showed that of the 72 included studies, 40% (29/72) of studies only used male mice or did not clarify the sex, 20% (14/72) of studies used only female mice, and only 36% (26/72) of studies used both sexes for all their measurements (Figure 2). Additionally, of all studies using both sexes, 73% (19/26) showed sex-specific outcomes. These data highlight the importance of considering sex as a biological variable (SABV) when testing novel geroprotector interventions. The failure to do so prevents a clear understanding of the sex-specific effects of the tested compounds, particularly as our systematic review found that 73% of studies showed sex differences in the effects of the tested compound on the health or lifespan outcome. It is tempting to speculate how many geroprotectors that have “failed” preclinical testing may have been successful if they were tested in females.
In 2016, the National Institutes of Health mandate came into force, requiring the use of both males and females in NIH-funded research, unless there was a strong scientific justification. This mandate resulted from the workshop on sex as a biological variable. Since then, a number of authors (Garcia-Sifuentes and Maney, 2021; Shansky and Murphy, 2021; Carmody et al., 2022) have looked at adherence to these policies across different scientific disciplines, with a general consensus that adherence should be improved. In addition to inclusion, it is important that authors also provide statistical evidence supporting the difference. A recent report examining sex differences across nine biological disciplines (in 147 articles) found incorrect use of statistics by authors to support their claims, which they suggest may lead to over-reporting or masking of sex-specific differences (Garcia-Sifuentes and Maney, 2021). These examples argue for continuing discussion on the importance of SABV and ongoing efforts to train biomedical researchers in how to test for and report sex differences correctly in their studies. It may be of importance for leading SABV journals to put together a white paper detailing the best practice for incorporating SABV in biomedical research, including how to appropriately use statistical tests to report effects, much like the PRISMA guidelines for systematic reviews. It is, however, encouraging to see journals such as the American Journal of Physiology-Heart and Circulatory Physiology requiring the inclusion of sex as a biological variable in the reporting of published articles (Denfeld et al., 2022) in their journal. Other journals, such as the Journals of Gerontology and Arteriosclerosis, Thrombosis, and Vascular Biology, have published statements recommending this to their authors (Le Couteur et al., 2018; Robinet et al., 2018). In the studies included here and published since 2020, there is no change observable regarding the use of both sexes when compared to all studies that were included.
Two more recent studies and therefore not yet included in this review have implemented the use of both sexes and found improvements in lifespan as well as healthspan. In the first study, the NADase CD38 inhibitor 78c increased median lifespan by 17% in males, but not in females, and improved exercise performance, endurance, and metabolic function in males (Peclat et al., 2022). In the second study, the PI3K p110α inhibitor, which targets the insulin receptor/insulin-like growth factor receptor pathway, extended median and maximal lifespan of both male and female mice and improved muscle function, with more significant effects in females (Hedges et al., 2023). These results further emphasize the importance of considering biological sex in preclinical research.
While including both sexes in preclinical research is critical, it is equally important to consider the genetic diversity of the mouse strains used in these studies. Testing interventions in heterogenous mouse strains provides a more accurate representation of how treatments may perform in a diverse human population, improving our ability to develop safe and effective treatments. Studies comparing genetically diverse inbred mouse strains have found significant differences in lifespan parameters (Yuan et al., 2009; Yuan et al., 2020), highlighting the importance of using multiple mouse strains when researching a potential geroprotector. While the studies included in this review exhibit some level of genetic diversity, there is room for improvement in terms of testing a specific compound on several genetic backgrounds to ensure greater generalizability.
In addition to the healthspan parameters focused on in this review, there are further health assessments that can be useful in intervention studies in aging mice. These include blood chemistry analysis, which provides information on glucose homeostasis, lipid metabolism, liver and kidney function, and inflammatory markers (O’Connell et al., 2015; Palliyaguru et al., 2021a; Zhang et al., 2022). Live animal imaging techniques, such as magnetic resonance imaging (MRI) (Chen et al., 2011) and positron emission tomography (PET) (Borrás et al., 2011; Hulsmans et al., 2018), can allow for the non-invasive visualization of organs and tissue and can therefore provide insights into structural and functional changes occurring with an intervention. Analysis of metabolomics (Adav and Wang, 2021; Tian et al., 2022), proteomics and transcriptomics (Takemon et al., 2021) can be used to identify changes in metabolic pathways, protein expression and gene expression in response to an intervention. Finally, tissue histology can assess changes on a tissue and cellular level (Pettan-Brewer and Treuting, 2011). Generally, it is important to use a wide variety of health assessment tools to get a more comprehensive understanding of the efficacy of geroprotective interventions.
Limitations of the systematic review
There are a number of limitations to consider when interpreting the findings of this systematic review. One limitation is that only one database (Pubmed) was used, which means that there may be a selection bias, as the studies included in the review may not be representative of the overall population of geroprotector studies. Additionally, the studies included in the review used a variety of outcomes and statistical methods, making it harder to compare the results across studies. Some studies also had missing information, which can impact the ability to accurately interpret the results. Furthermore, the quality of the studies included in the review may vary, with some studies having more robust designs, higher statistical power, and more reliable results compared to others. Overall, these limitations should be considered when interpreting the results of the review and planning future research on geroprotectors and their effects on healthspan and lifespan.
Conclusion
Pharmacological interventions represent an attractive therapeutic avenue for modulating age-related diseases and frailty, especially in those individuals for whom dietary interventions are not feasible. The results from our systematic review show that most studies have only been performed in males, meaning the generalizability of these findings to females is unknown. Given that females represent roughly 50% of the population, the knowledge gap surrounding the translational value of these interventions is large, as for half the population we do not know how these may impact healthspan or lifespan. Thus, we reiterate the point that only by studying both males and females can we leverage sex-specific differences to provide novel insights into the pathophysiology of aging and improve healthy aging for all.
Data availability statement
The original contributions presented in the study are included in the article/Supplementary Material, further inquiries can be directed to the corresponding authors.
Author contributions
MK and SM conceptualized and designed the study. MK collected articles. MK and SM reviewed all articles and formulated the results. MK wrote the first draft of the manuscript. SM, MM, and CE edited the manuscript. All authors that contributed to the manuscript revision, read and approved the submitted version.
Funding
This study was supported by the National Institute on Aging, P01AG055369 to SM. Funding from the Swiss National Science Foundation Funding from the SNF P3 Project 190072 to CYE.
Acknowledgments
We apologize to the authors whose work is not included due to space limits.
Conflict of interest
The authors declare that the research was conducted in the absence of any commercial or financial relationships that could be construed as a potential conflict of interest.
Publisher’s note
All claims expressed in this article are solely those of the authors and do not necessarily represent those of their affiliated organizations, or those of the publisher, the editors and the reviewers. Any product that may be evaluated in this article, or claim that may be made by its manufacturer, is not guaranteed or endorsed by the publisher.
References
Ackert-Bicknell, C. L., Anderson, L. C., Sheehan, S., Hill, W. G., Chang, B., Churchill, G. A., et al. (2015). Aging research using mouse models. Curr. Protoc. Mouse Biol. 5, 95–133. doi:10.1002/9780470942390.mo140195
Adav, S. S., and Wang, Y. (2021). Metabolomics signatures of aging: Recent advances. Aging Dis. 12, 646–661. doi:10.14336/AD.2020.0909
Anisimov, V. N., Alimova, I. N., Baturin, D. A., Popovich, I. G., Zabezhinski, M. A., Rosenfeld, S. V., et al. (2003). Dose-dependent effect of melatonin on life span and spontaneous tumor incidence in female SHR mice. Exp. Gerontol. 38, 449–461. doi:10.1016/S0531-5565(02)00240-1
Anisimov, V. N., Berstein, L. M., Egormin, P. A., Piskunova, T. S., Popovich, I. G., Zabezhinski, M. A., et al. (2008). Metformin slows down aging and extends life span of female SHR mice. Cell Cycle georget. Tex 7, 2769–2773. doi:10.4161/cc.7.17.6625
Anisimov, V. N., Egorov, M. V., Krasilshchikova, M. S., Lyamzaev, K. G., Manskikh, V. N., Moshkin, M. P., et al. (2011). Effects of the mitochondria-targeted antioxidant SkQ1 on lifespan of rodents. Aging 3, 1110–1119. doi:10.18632/aging.100404
Anisimov, V. N., Piskunova, T. S., Popovich, I. G., Zabezhinski, M. A., Tyndyk, M. L., Egormin, P. A., et al. (2010). Gender differences in metformin effect on aging, life span and spontaneous tumorigenesis in 129/Sv mice. Aging 2, 945–958. doi:10.18632/aging.100245
Aon, M. A., Bernier, M., Mitchell, S. J., Di Germanio, C., Mattison, J. A., Ehrlich, M. R., et al. (2020). Untangling determinants of enhanced health and lifespan through a multi-omics approach in mice. Cell Metab. 32, 100–116. doi:10.1016/j.cmet.2020.04.018
Asseburg, H., Schäfer, C., Müller, M., Hagl, S., Pohland, M., Berressem, D., et al. (2016). Effects of grape skin extract on age-related mitochondrial dysfunction, memory and life span in C57bl/6J mice. NeuroMolecular Med. 18, 378–395. doi:10.1007/s12017-016-8428-4
Atella, V., Piano Mortari, A., Kopinska, J., Belotti, F., Lapi, F., Cricelli, C., et al. (2019). Trends in age-related disease burden and healthcare utilization. Aging Cell 18, e12861. doi:10.1111/acel.12861
Austad, S. N., and Fischer, K. E. (2016). Sex differences in lifespan. Cell Metab. 23, 1022–1033. doi:10.1016/j.cmet.2016.05.019
Austad, S. N. (2016). “The geroscience hypothesis: Is it possible to change the rate of aging?,” in Advances in geroscience. Editors F. Sierra, and R. Kohanski (Cham: Springer International Publishing), 1–36. doi:10.1007/978-3-319-23246-1_1
Bellantuono, I., de Cabo, R., Ehninger, D., Di Germanio, C., Lawrie, A., Miller, J., et al. (2020). A toolbox for the longitudinal assessment of healthspan in aging mice. Nat. Protoc. 15, 540–574. doi:10.1038/s41596-019-0256-1
Berry, A., Marconi, M., Musillo, C., Chiarotti, F., Bellisario, V., Matarrese, P., et al. (2020). Trehalose administration in C57bl/6N old mice affects healthspan improving motor learning and brain anti-oxidant defences in a sex-dependent fashion: A pilot study. Exp. Gerontol. 129, 110755. doi:10.1016/j.exger.2019.110755
Bezlepkin, V. G., Sirota, N. P., and Gaziev, A. I. (1996). The prolongation of survival in mice by dietary antioxidants depends on their age by the start of feeding this diet. Mech. Ageing Dev. 92, 227–234. doi:10.1016/S0047-6374(96)01840-4
Bi, Z., Zhang, W., and Yan, X. (2022). Anti-inflammatory and immunoregulatory effects of icariin and icaritin. Biomed. Pharmacother. 151, 113180. doi:10.1016/j.biopha.2022.113180
Bielas, J., Herbst, A., Widjaja, K., Hui, J., Aiken, J. M., McKenzie, D., et al. (2018). Long term rapamycin treatment improves mitochondrial DNA quality in aging mice. Exp. Gerontol. 106, 125–131. doi:10.1016/j.exger.2018.02.021
Bitto, A., Ito, T. K., Pineda, V. V., LeTexier, N. J., Huang, H. Z., Sutlief, E., et al. (2016). Transient rapamycin treatment can increase lifespan and healthspan in middle-aged mice. eLife 5, e16351. doi:10.7554/eLife.16351
Borrás, C., Monleón, D., López-Grueso, R., Gambini, J., Orlando, L., Pallardó, F. V., et al. (2011). RasGrf1 deficiency delays aging in mice. Aging 3, 262–276. doi:10.18632/aging.100279
Brandhorst, S., and Longo, V. D. (2019). Protein quantity and source, fasting-mimicking diets, and longevity. Adv. Nutr. Bethesda Md 10, S340-S350–S350. doi:10.1093/advances/nmz079
Bronikowski, A. M., Meisel, R. P., Biga, P. R., Walters, J. R., Mank, J. E., Larschan, E., et al. (2022). Sex-specific aging in animals: Perspective and future directions. Aging Cell 21, e13542. doi:10.1111/acel.13542
Cabo, R. de, and Mattson, M. P. (2019). Effects of intermittent fasting on health, aging, and disease. N. Engl. J. Med. 381, 2541–2551. doi:10.1056/NEJMra1905136
Carmody, C., Duesing, C. G., Kane, A. E., and Mitchell, S. J. (2022). Perspective: Is sex as a biological variable still being ignored in pre-clinical aging research? J. Gerontol. Ser. A glac042 77, 2177–2180. doi:10.1093/gerona/glac042
Cerro, E. D.-D., Lambea, M., Félix, J., Salazar, N., Gueimonde, M., and De la Fuente, M. (2021). Daily ingestion of Akkermansia mucciniphila for one month promotes healthy aging and increases lifespan in old female mice. Biogerontology 23, 35–52. doi:10.1007/s10522-021-09943-w
Chen, C.-C. V., Tung, Y.-Y., and Chang, C. (2011). A lifespan MRI evaluation of ventricular enlargement in normal aging mice. Neurobiol. Aging 32, 2299–2307. doi:10.1016/j.neurobiolaging.2010.01.013
Chen, W., Wang, J., Shi, J., Yang, X., Yang, P., Wang, N., et al. (2019). Longevity effect of Liuwei Dihuang in both Caenorhabditis elegans and aged mice. Aging Dis. 10, 578–591. doi:10.14336/AD.2018.0604
Comfort, A., Youhotsky-Gore, I., and Pathmanathan, K. (1971). Effect of ethoxyquin on the longevity of C3H mice. Nature 229, 254–255. doi:10.1038/229254a0
Cotzias, G. C., Miller, S. T., Nicholson, A. R., Maston, W. H., and Tang, L. C. (1974). Prolongation of the life-span in mice adapted to large amounts of L-dopa. Proc. Natl. Acad. Sci. U. S. A. 71, 2466–2469. doi:10.1073/pnas.71.6.2466
Crimmins, E. M., Shim, H., Zhang, Y. S., and Kim, J. K. (2019). Differences between men and women in mortality and the health dimensions of the morbidity process. Clin. Chem. 65, 135–145. doi:10.1373/clinchem.2018.288332
Davies, I., and Schofield, J. D. (1980). Connective tissue ageing: The influence of a lathyrogen (β-aminopropionitrile) on the life span of female C57BL/Icrfat mice. Exp. Gerontol. 15, 487–494. doi:10.1016/0531-5565(80)90057-1
Decaroli, M. C., De Vincentis, S., and Rochira, V. (2021). Aging and sex hormones in males. Vitam. Horm. 115, 333–366. doi:10.1016/bs.vh.2020.12.014
Della Peruta, C., Lozanoska-Ochser, B., Renzini, A., Moresi, V., Sanchez Riera, C., Bouché, M., et al. (2023). Sex differences in inflammation and muscle wasting in aging and disease. Int. J. Mol. Sci. 24, 4651. doi:10.3390/ijms24054651
Denfeld, Q. E., Lee, C. S., and Habecker, B. A. (2022). A primer on incorporating sex as a biological variable into the conduct and reporting of basic and clinical research studies. Am. J. Physiol.-Heart Circ. Physiol. 322, H350–H354. doi:10.1152/ajpheart.00605.2021
Dorigatti, J. D., Thyne, K. M., Ginsburg, B. C., and Salmon, A. B. (2021). Beta-guanidinopropionic acid has age-specific effects on markers of health and function in mice. GeroScience 43, 1497–1511. doi:10.1007/s11357-021-00372-8
Fischer, K. E., Gelfond, J. A. L., Soto, V. Y., Han, C., Someya, S., Richardson, A., et al. (2015). Health effects of long-term rapamycin treatment: The impact on mouse health of enteric rapamycin treatment from four months of age throughout life. PloS One 10, e0126644. doi:10.1371/journal.pone.0126644
Fischer, K. E., Hoffman, J. M., Sloane, L. B., Gelfond, J. A. L., Soto, V. Y., Richardson, A. G., et al. (2016). A cross-sectional study of male and female C57BL/6Nia mice suggests lifespan and healthspan are not necessarily correlated. Aging 8, 2370–2391. doi:10.18632/aging.101059
Flynn, J. M., O’Leary, M. N., Zambataro, C. A., Academia, E. C., Presley, M. P., Garrett, B. J., et al. (2013). Late-life rapamycin treatment reverses age-related heart dysfunction. Aging Cell 12, 851–862. doi:10.1111/acel.12109
Forbes, W. F. (1975). The effect of prednisolone phosphate on the life-span of DBA/2J mice. Exp. Gerontol. 10, 27–29. doi:10.1016/0531-5565(75)90012-1
Franceschi, C., Garagnani, P., Parini, P., Giuliani, C., and Santoro, A. (2018). Inflammaging: A new immune–metabolic viewpoint for age-related diseases. Nat. Rev. Endocrinol. 14, 576–590. doi:10.1038/s41574-018-0059-4
Garcia-Sifuentes, Y., and Maney, D. L. (2021). Reporting and misreporting of sex differences in the biological sciences. eLife 10, e70817. doi:10.7554/eLife.70817
GBD Ageing Collaborators (2022). Global, regional, and national burden of diseases and injuries for adults 70 years and older: Systematic analysis for the global burden of disease 2019 study. BMJ 376, e068208. doi:10.1136/bmj-2021-068208,
Glossmann, H. H., and Lutz, O. M. D. (2019). Metformin and aging: A review. Gerontology 65, 581–590. doi:10.1159/000502257
Gomes, A. P., Price, N. L., Ling, A. J. Y., Moslehi, J. J., Montgomery, M. K., Rajman, L., et al. (2013). Declining NAD+ induces a pseudohypoxic state disrupting nuclear-mitochondrial communication during aging. Cell 155, 1624–1638. doi:10.1016/j.cell.2013.11.037
Gordon, E. H., and Hubbard, R. E. (2019). Do sex differences in chronic disease underpin the sex-frailty paradox? Mech. Ageing Dev. 179, 44–50. doi:10.1016/j.mad.2019.02.004
Green, C. L., Lamming, D. W., and Fontana, L. (2022). Molecular mechanisms of dietary restriction promoting health and longevity. Nat. Rev. Mol. Cell Biol. 23, 56–73. doi:10.1038/s41580-021-00411-4
Grohn, K. J., Moyer, B. S., Wortel, D. C., Fisher, C. M., Lumen, E., Bianchi, A. H., et al. (2021). C60 in olive oil causes light-dependent toxicity and does not extend lifespan in mice. GeroScience 43, 579–591. doi:10.1007/s11357-020-00292-z
Guarner-Lans, V., Rubio-Ruiz, M. E., Pérez-Torres, I., and Baños de MacCarthy, G. (2011). Relation of aging and sex hormones to metabolic syndrome and cardiovascular disease. Exp. Gerontol. 46, 517–523. doi:10.1016/j.exger.2011.02.007
Hansen, M., and Kennedy, B. K. (2016). Does longer lifespan mean longer healthspan? Trends Cell Biol. 26, 565–568. doi:10.1016/j.tcb.2016.05.002
Harrison, D. E., Strong, R., Allison, D. B., Ames, B. N., Astle, C. M., Atamna, H., et al. (2014). Acarbose, 17-α-estradiol, and nordihydroguaiaretic acid extend mouse lifespan preferentially in males. Aging Cell 13, 273–282. doi:10.1111/acel.12170
Harrison, D. E., Strong, R., Reifsnyder, P., Kumar, N., Fernandez, E., Flurkey, K., et al. (2021). 17-a-estradiol late in life extends lifespan in aging UM-HET3 male mice; nicotinamide riboside and three other drugs do not affect lifespan in either sex. Aging Cell 20, e13328. doi:10.1111/acel.13328
Harrison, D. E., Strong, R., Sharp, Z. D., Nelson, J. F., Astle, C. M., Flurkey, K., et al. (2009). Rapamycin fed late in life extends lifespan in genetically heterogeneous mice. Nature 460, 392–395. doi:10.1038/nature08221
Hedges, C. P., Shetty, B., Broome, S. C., MacRae, C., Koutsifeli, P., Buckels, E. J., et al. (2023). Dietary supplementation of clinically utilized PI3K p110α inhibitor extends the lifespan of male and female mice. Nat. Aging 3, 162–172. doi:10.1038/s43587-022-00349-y
Heidrick, M. L., Hendricks, L. C., and Cook, D. E. (1984). Effect of dietary 2-mercaptoethanol on the life span, immune system, tumor incidence and lipid peroxidation damage in spleen lymphocytes of aging BC3F1 mice. Mech. Ageing Dev. 27, 341–358. doi:10.1016/0047-6374(84)90057-5
Henderson, Y. O., Bithi, N., Link, C., Yang, J., Schugar, R., Llarena, N., et al. (2021). Late-life intermittent fasting decreases aging-related frailty and increases renal hydrogen sulfide production in a sexually dimorphic manner. GeroScience 43, 1527–1554. doi:10.1007/s11357-021-00330-4
Hession, L. E., Sabnis, G. S., Churchill, G. A., and Kumar, V. (2022). A machine-vision-based frailty index for mice. Nat. Aging 2, 756–766. doi:10.1038/s43587-022-00266-0
Hiramoto, K., Yamate, Y., Matsuda, K., Sugiyama, D., and Iizuka, Y. (2020). Tranexamic acid improves memory and learning abilities in aging mice. J. Exp. Pharmacol. 12, 653–663. doi:10.2147/JEP.S284532
Hiramoto, K., Yamate, Y., Sugiyama, D., Matsuda, K., Iizuka, Y., and Yamaguchi, T. (2019). Effect of tranexamic acid in improving the lifespan of naturally aging mice. Inflammopharmacology 27, 1319–1323. doi:10.1007/s10787-019-00616-2
Horstman, A. M., Dillon, E. L., Urban, R. J., and Sheffield-Moore, M. (2012). The role of androgens and estrogens on healthy aging and longevity. J. Gerontol. A. Biol. Sci. Med. Sci. 67, 1140–1152. doi:10.1093/gerona/gls068
Hulsmans, M., Sager, H. B., Roh, J. D., Valero-Muñoz, M., Houstis, N. E., Iwamoto, Y., et al. (2018). Cardiac macrophages promote diastolic dysfunction. J. Exp. Med. 215, 423–440. doi:10.1084/jem.20171274
Janssens, G. E., and Houtkooper, R. H. (2020). Identification of longevity compounds with minimized probabilities of side effects. Biogerontology 21, 709–719. doi:10.1007/s10522-020-09887-7
Kane, A. E., Hilmer, S. N., Boyer, D., Gavin, K., Nines, D., Howlett, S. E., et al. (2016). Impact of longevity interventions on a validated mouse clinical frailty index. J. Gerontol. A. Biol. Sci. Med. Sci. 71, 333–339. doi:10.1093/gerona/glu315
Kane, A. E., and Howlett, S. E. (2017). Advances in preclinical models of frailty. J. Gerontol. A. Biol. Sci. Med. Sci. 72, 867–869. doi:10.1093/gerona/glx072
Kane, A., and Howlett, S. (2021). Sex differences in frailty: Comparisons between humans and preclinical models. Mech. Ageing Dev. 198, 111546. doi:10.1016/j.mad.2021.111546
Khavinson, V. K., and Anisimov, V. N. (2000). A synthetic dipeptide vilon (L-Lys-L-Glu) inhibits growth of spontaneous tumors and increases life span of mice. Dokl. Biol. Sci. Proc. Acad. Sci. USSR Biol. Sci. Sect. 372, 261–263. doi:10.1007/BF02682106
Khavinson, V. K., Anisimov, V. N., Zavarzina, N. Y., Zabezhinskii, M. A., Zimina, O. A., Popovich, I. G., et al. (2000). Effect of vilon on biological age and lifespan in mice. Bull. Exp. Biol. Med. 130, 687–690. doi:10.1007/BF02682106
Kitani, K., Osawa, T., and Yokozawa, T. (2007). The effects of tetrahydrocurcumin and green tea polyphenol on the survival of male C57BL/6 mice. Biogerontology 8, 567–573. doi:10.1007/s10522-007-9100-z
Kobayashi, H., Martínez de Toda, I., Sanz-San Miguel, L., and De la Fuente, M. (2021). Sex-related differences in behavioural markers in adult mice for the prediction of lifespan. Biogerontology 22, 49–62. doi:10.1007/s10522-020-09902-x
Krut’ko, V., Dontsov, V. I., and Khalyavkin, A. (2016). Effect of alpha-fetoprotein on lifespan of old mice. Biochem. Mosc. 81, 1477–1479. doi:10.1134/S0006297916120087
LaBella, F., and Vivian, S. (1978). Beta-aminopropiontrile promotes longevity in mice. Exp. Gerontol. 13, 251–254. doi:10.1016/0531-5565(78)90019-0
Le Couteur, D. G., Anderson, R. M., and de Cabo, R. (2018). Sex and aging. J. Gerontol. A. Biol. Sci. Med. Sci. 73, 139–140. doi:10.1093/gerona/glx221
Lee, C.-K., Pugh, T. D., Klopp, R. G., Edwards, J., Allison, D. B., Weindruch, R., et al. (2004). The impact of alpha-lipoic acid, coenzyme Q10 and caloric restriction on life span and gene expression patterns in mice. Free Radic. Biol. Med. 36, 1043–1057. doi:10.1016/j.freeradbiomed.2004.01.015
Li, S., Li, J., Pan, R., Cheng, J., Cui, Q., Chen, J., et al. (2022). Sodium rutin extends lifespan and health span in mice including positive impacts on liver health. Br. J. Pharmacol. 179, 1825–1838. doi:10.1111/bph.15410
Li, X., Li, T., Hong, X. Y., Liu, J. J., Yang, X. F., and Liu, G. P. (2021). Acer truncatum seed oil alleviates learning and memory impairments of aging mice. Front. Cell Dev. Biol. 9, 680386. doi:10.3389/fcell.2021.680386
Liu, H., Graber, T. G., Ferguson-Stegall, L., and Thompson, L. V. (2014). Clinically relevant frailty index for mice. J. Gerontol. A. Biol. Sci. Med. Sci. 69, 1485–1491. doi:10.1093/gerona/glt188
Longo, V. D., and Anderson, R. M. (2022). Nutrition, longevity and disease: From molecular mechanisms to interventions. Cell 185, 1455–1470. doi:10.1016/j.cell.2022.04.002
Longo, V. D., Antebi, A., Bartke, A., Barzilai, N., Brown-Borg, H. M., Caruso, C., et al. (2015). Interventions to slow aging in humans: Are we ready? Aging Cell 14, 497–510. doi:10.1111/acel.12338
Lönnrot, K., Holm, P., Lagerstedt, A., Huhtala, H., and Alho, H. (1998). The effects of lifelong ubiquinone Q10 supplementation on the Q9 and Q10 tissue concentrations and life span of male rats and mice. Biochem. Mol. Biol. Int. 44, 727–737. doi:10.1080/15216549800201772
López-Otín, C., Blasco, M. A., Partridge, L., Serrano, M., and Kroemer, G. (2023). Hallmarks of aging: An expanding universe. Cell 186, 243–278. doi:10.1016/j.cell.2022.11.001
Majumder, S., Caccamo, A., Medina, D. X., Benavides, A. D., Javors, M. A., Kraig, E., et al. (2012). Lifelong rapamycin administration ameliorates age-dependent cognitive deficits by reducing IL-1β and enhancing NMDA signaling. Aging Cell 11, 326–335. doi:10.1111/j.1474-9726.2011.00791.x
Mann, S. N., Hadad, N., Nelson Holte, M., Rothman, A. R., Sathiaseelan, R., Ali Mondal, S., et al. (2020). Health benefits attributed to 17α-estradiol, a lifespan-extending compound, are mediated through estrogen receptor α. eLife 9, e59616. doi:10.7554/eLife.59616
Martin-Montalvo, A., Mercken, E. M., Mitchell, S. J., Palacios, H. H., Mote, P. L., Scheibye-Knudsen, M., et al. (2013). Metformin improves healthspan and lifespan in mice. Nat. Commun. 4, 2192. doi:10.1038/ncomms3192
McReynolds, M. R., Chellappa, K., and Baur, J. A. (2020). Age-related NAD+ decline. Exp. Gerontol. 134, 110888. doi:10.1016/j.exger.2020.110888
Merone, L., Tsey, K., Russell, D., and Nagle, C. (2022). Sex inequalities in medical research: A systematic scoping review of the literature. Womens Health Rep. 3, 49–59. doi:10.1089/whr.2021.0083
Miller, L. R., Marks, C., Becker, J. B., Hurn, P. D., Chen, W.-J., Woodruff, T., et al. (2017). Considering sex as a biological variable in preclinical research. FASEB J. Off. Publ. Fed. Am. Soc. Exp. Biol. 31, 29–34. doi:10.1096/fj.201600781R
Miller, R. A., and Chrisp, C. (1999). Lifelong treatment with oral DHEA sulfate does not preserve immune function, prevent disease, or improve survival in genetically heterogeneous mice. J. Am. Geriatr. Soc. 47, 960–966. doi:10.1111/j.1532-5415.1999.tb01291.x
Miller, R. A., Harrison, D. E., Allison, D. B., Bogue, M., Debarba, L., Diaz, V., et al. (2020). Canagliflozin extends life span in genetically heterogeneous male but not female mice. JCI Insight 5, 140019. doi:10.1172/jci.insight.140019
Miller, R. A., Harrison, D. E., Astle, C. M., Baur, J. A., Boyd, A. R., de Cabo, R., et al. (2011). Rapamycin, but not resveratrol or simvastatin, extends life span of genetically heterogeneous mice. J. Gerontol. A. Biol. Sci. Med. Sci. 66, 191–201. doi:10.1093/gerona/glq178
Miller, R. A., Harrison, D. E., Astle, C. M., Bogue, M. A., Brind, J., Fernandez, E., et al. (2019). Glycine supplementation extends lifespan of male and female mice. Aging Cell 18, e12953. doi:10.1111/acel.12953
Miller, R. A., Harrison, D. E., Astle, C. M., Fernandez, E., Flurkey, K., Han, M., et al. (2014). Rapamycin-mediated lifespan increase in mice is dose and sex dependent and metabolically distinct from dietary restriction. Aging Cell 13, 468–477. doi:10.1111/acel.12194
Miller, R. A., Harrison, D. E., Astle, C. M., Floyd, R. A., Flurkey, K., Hensley, K. L., et al. (2007). An aging interventions testing program: Study design and interim report. Aging Cell 6, 565–575. doi:10.1111/j.1474-9726.2007.00311.x
Minor, R. K., Baur, J. A., Gomes, A. P., Ward, T. M., Csiszar, A., Mercken, E. M., et al. (2011). SRT1720 improves survival and healthspan of obese mice. Sci. Rep. 1, 70. doi:10.1038/srep00070
Miquel, J., and Economos, A. C. (1979). Favorable effects of the antioxidants sodium and magnesium thiazolidine carboxylate on the vitality and life span of Drosophila and mice. Exp. Gerontol. 14, 279–285. doi:10.1016/0531-5565(79)90039-1
Mitchell, S. J., Bernier, M., Aon, M. A., Cortassa, S., Kim, E. Y., Fang, E. F., et al. (2018). Nicotinamide improves aspects of healthspan, but not lifespan, in mice. Cell Metab. 27, 667–676. doi:10.1016/j.cmet.2018.02.001
Mitchell, S. J., Bernier, M., Mattison, J. A., Aon, M. A., Kaiser, T. A., Anson, R. M., et al. (2019). Daily fasting improves health and survival in male mice independent of diet composition and calories. Cell Metab. 29, 221–228. doi:10.1016/j.cmet.2018.08.011
Mitchell, S. J., Madrigal-Matute, J., Scheibye-Knudsen, M., Fang, E., Aon, M., González-Reyes, J. A., et al. (2016). Effects of sex, strain, and energy intake on hallmarks of aging in mice. Cell Metab. 23, 1093–1112. doi:10.1016/j.cmet.2016.05.027
Mitchell, S. J., Martin-Montalvo, A., Mercken, E. M., Palacios, H. H., Ward, T. M., Abulwerdi, G., et al. (2014). The SIRT1 activator SRT1720 extends lifespan and improves health of mice fed a standard diet. Cell Rep. 6, 836–843. doi:10.1016/j.celrep.2014.01.031
Mitchell, S. J., and Mitchell, J. R. (2022). Sexual dimorphism in the response to dietary restriction in mice: A systematic review of the literature. Nutr. Healthy Aging 7, 87–120. doi:10.3233/NHA-220162
Morley, A. A., and Trainor, K. J. (2001). Lack of an effect of vitamin E on lifespan of mice. Biogerontology 2, 109–112. doi:10.1023/a:1011589218219
Moskalev, A., Chernyagina, E., Tsvetkov, V., Fedintsev, A., Shaposhnikov, M., Krut’ko, V., et al. (2016). Developing criteria for evaluation of geroprotectors as a key stage toward translation to the clinic. Aging Cell 15, 407–415. doi:10.1111/acel.12463
Moskalev, A., Guvatova, Z., Lopes, I. D. A., Beckett, C. W., Kennedy, B. K., Magalhaes, J. P. D., et al. (2022). Targeting aging mechanisms: Pharmacological perspectives. Trends Endocrinol. Metab. 33, 266–280. doi:10.1016/j.tem.2022.01.007
Navarro, A., Gómez, C., Sánchez-Pino, M.-J., González, H., Bández, M. J., Boveris, A. D., et al. (2005). Vitamin E at high doses improves survival, neurological performance, and brain mitochondrial function in aging male mice. Am. J. Physiol. Regul. Integr. Comp. Physiol. 289, R1392–R1399. doi:10.1152/ajpregu.00834.2004
Neff, F., Flores-Dominguez, D., Ryan, D. P., Horsch, M., Schröder, S., Adler, T., et al. (2013). Rapamycin extends murine lifespan but has limited effects on aging. J. Clin. Invest. 123, 3272–3291. doi:10.1172/JCI67674
NOT-OD-15-102: Consideration of sex as a biological variable in NIH-funded research [WWW Document], 2015. Available at: https://grants.nih.gov/grants/guide/notice-files/not-od-15-102.html?itid=lk_inline_enhanced-template (accessed 2.6.23).
O’Connell, K. E., Mikkola, A. M., Stepanek, A. M., Vernet, A., Hall, C. D., Sun, C. C., et al. (2015). Practical murine hematopathology: A comparative review and implications for research. Comp. Med. 65, 96–113.
Olshansky, S. J. (2018). From lifespan to healthspan. JAMA 320, 1323–1324. doi:10.1001/jama.2018.12621
Page, M. J., McKenzie, J. E., Bossuyt, P. M., Boutron, I., Hoffmann, T. C., Mulrow, C. D., et al. (2021). The PRISMA 2020 statement: An updated guideline for reporting systematic reviews. BMJ n71, n71. doi:10.1136/bmj.n71
Palliyaguru, D. L., Minor, R. K., Mitchell, S. J., Palacios, H. H., Licata, J. J., Ward, T. M., et al. (2020). Combining a high dose of metformin with the SIRT1 activator, SRT1720, reduces life span in aged mice fed a high-fat diet. J. Gerontol. A. Biol. Sci. Med. Sci. 75, 2037–2041. doi:10.1093/gerona/glaa148
Palliyaguru, D. L., Moats, J. M., Di Germanio, C., Bernier, M., and de Cabo, R. (2019). Frailty index as a biomarker of lifespan and healthspan: Focus on pharmacological interventions. Mech. Ageing Dev. 180, 42–48. doi:10.1016/j.mad.2019.03.005
Palliyaguru, D. L., Vieira Ligo Teixeira, C., Duregon, E., di Germanio, C., Alfaras, I., Mitchell, S. J., et al. (2021b). Study of longitudinal aging in mice: Presentation of experimental techniques. J. Gerontol. Ser. A 76, 552–560. doi:10.1093/gerona/glaa285
Palliyaguru, D. L., Shiroma, E. J., Nam, J. K., Duregon, E., Vieira Ligo Teixeira, C., Price, N. L., et al. (2021a). Fasting blood glucose as a predictor of mortality: Lost in translation. Cell Metab. 33, 2189–2200.e3. doi:10.1016/j.cmet.2021.08.013
Pearson, K. J., Baur, J. A., Lewis, K. N., Peshkin, L., Price, N. L., Labinskyy, N., et al. (2008). Resveratrol delays age-related deterioration and mimics transcriptional aspects of dietary restriction without extending life span. Cell Metab. 8, 157–168. doi:10.1016/j.cmet.2008.06.011
Peclat, T. R., Thompson, K. L., Warner, G. M., Chini, C. C. S., Tarragó, M. G., Mazdeh, D. Z., et al. (2022). CD38 inhibitor 78c increases mice lifespan and healthspan in a model of chronological aging. Aging Cell 21, e13589. doi:10.1111/acel.13589
Pettan-Brewer, C., and Treuting, P. M. (2011). Practical pathology of aging mice. Pathobiol. Aging Age Relat. Dis. 1, 7202. doi:10.3402/pba.v1i0.7202
Plevkova, J., Brozmanova, M., Harsanyiova, J., Sterusky, M., Honetschlager, J., and Buday, T. (2020). Various aspects of sex and gender bias in biomedical research. Physiol. Res. 69, S367–S378. doi:10.33549/physiolres.934593
Popovich, I. G., Voitenkov, B. O., Anisimov, V. N., Ivanov, V. T., Mikhaleva, I. I., Zabezhinski, M. A., et al. (2003). Effect of delta-sleep inducing peptide-containing preparation Deltaran on biomarkers of aging, life span and spontaneous tumor incidence in female SHR mice. Mech. Ageing Dev. 124, 721–731. doi:10.1016/S0047-6374(03)00082-4
Prince, M. J., Wu, F., Guo, Y., Robledo, L. M. G., O’Donnell, M., Sullivan, R., et al. (2015). The burden of disease in older people and implications for health policy and practice. Lancet 385, 549–562. doi:10.1016/S0140-6736(14)61347-7
Quick, K. L., Ali, S. S., Arch, R., Xiong, C., Wozniak, D., and Dugan, L. L. (2008). A carboxyfullerene SOD mimetic improves cognition and extends the lifespan of mice. Neurobiol. Aging 29, 117–128. doi:10.1016/j.neurobiolaging.2006.09.014
Richardson, A., Fischer, K. E., Speakman, J. R., de Cabo, R., Mitchell, S. J., Peterson, C. A., et al. (2016). Measures of healthspan as indices of aging in mice-A recommendation. J. Gerontol. A. Biol. Sci. Med. Sci. 71, 427–430. doi:10.1093/gerona/glv080
Robinet, P., Milewicz, D. M., Cassis, L. A., Leeper, N. J., Lu, H. S., and Smith, J. D. (2018). Consideration of sex differences in design and reporting of experimental arterial pathology studies: A statement from the atvb council. Arterioscler. Thromb. Vasc. Biol. 38, 292–303. doi:10.1161/ATVBAHA.117.309524
Rockwood, K., Blodgett, J. M., Theou, O., Sun, M. H., Feridooni, H. A., Mitnitski, A., et al. (2017). A frailty index based on deficit accumulation quantifies mortality risk in humans and in mice. Sci. Rep. 7, 43068. doi:10.1038/srep43068
Roda, E., Priori, E. C., Ratto, D., De Luca, F., Di Iorio, C., Angelone, P., et al. (2021). Neuroprotective metabolites of Hericium erinaceus promote neuro-healthy aging. Int. J. Mol. Sci. 22, 6379. doi:10.3390/ijms22126379
Saito, K., Yoshioka, H., and Cutler, R. G. (1998). A spin trap, N-tert-butyl-alpha-phenylnitrone extends the life span of mice. Biosci. Biotechnol. Biochem. 62, 792–794. doi:10.1271/bbb.62.792
Sampathkumar, N., Bravo, J. I., Chen, Y., Danthi, P. S., Donahue, E. K., Lai, R., et al. (2019). Widespread sex dimorphism in aging and age-related diseases. Hum. Genet. 139, 333–356. doi:10.1007/s00439-019-02082-w
Schork, N. J., Beaulieu-Jones, B., Liang, W., Smalley, S., and Goetz, L. H. (2022). Does modulation of an epigenetic clock define a geroprotector? Adv. Geriatr. Med. Res. 4, e220002. doi:10.20900/agmr20220002
Sciorati, C., Gamberale, R., Monno, A., Citterio, L., Lanzani, C., De Lorenzo, R., et al. (2020). Pharmacological blockade of TNFα prevents sarcopenia and prolongs survival in aging mice. Aging 12, 23497–23508. doi:10.18632/aging.202200
Selvarani, R., Mohammed, S., and Richardson, A. (2020). Effect of rapamycin on aging and age-related diseases—Past and future. GeroScience 43, 1135–1158. doi:10.1007/s11357-020-00274-1
Shahmirzadi, A., Azar, P., Edgar, D., Liao, C.-Y., Hsu, Y.-M., Lucanic, M., et al. (2020). Alpha-ketoglutarate, an endogenous metabolite, extends lifespan and compresses morbidity in aging mice. Cell Metab. 32, 447–456. doi:10.1016/j.cmet.2020.08.004
Shansky, R. M., and Murphy, A. Z. (2021). Considering sex as a biological variable will require a global shift in science culture. Nat. Neurosci. 24, 457–464. doi:10.1038/s41593-021-00806-8
Shetty, P. (2012). Grey matter: Ageing in developing countries. Lancet 379, 1285–1287. doi:10.1016/S0140-6736(12)60541-8
Shin, J., Jung-Ran, N., Choe, D., Lee, N., Song, Y., and Cho, S. (2021). Ageing and rejuvenation models reveal changes in key microbial communities associated with healthy ageing. Microbiome 9, 240. doi:10.1186/s40168-021-01189-5
Shindyapina, A. V., Cho, Y., Kaya, A., Tyshkovskiy, A., Castro, J. P., Gordevicius, J., et al. (2022). Rapamycin treatment during development extends lifespan and healthspan. Sci. Adv. 8, 5482. doi:10.1101/2022.02.18.481092
Sierra, F., and Kohanski, R. (2017). Geroscience and the trans-NIH geroscience interest group, GSIG. GSIG. GeroScience 39, 1–5. doi:10.1007/s11357-016-9954-6
Sierra, F. (2016). The emergence of geroscience as an interdisciplinary approach to the enhancement of health span and life span. Cold Spring Harb. Perspect. Med. 6, a025163. doi:10.1101/cshperspect.a025163
Sinclair, D. A. (2005). Toward a unified theory of caloric restriction and longevity regulation. Mech. Ageing Dev. 126, 987–1002. doi:10.1016/j.mad.2005.03.019
Smith, B. J., Miller, R. A., Ericsson, A. C., Harrison, D. C., Strong, R., and Schmidt, T. M. (2019). Changes in the gut microbiome and fermentation products concurrent with enhanced longevity in acarbose-treated mice. BMC Microbiol. 19, 130. doi:10.1186/s12866-019-1494-7
Smith, D. L., Robertson, H. T., Desmond, R. A., Nagy, T. R., and Allison, D. B., 2011. No compelling evidence that sibutramine prolongs life in rodents despite providing a dose-dependent reduction in body weight. Int. J. Obes. 35, 652–657. doi:10.1038/ijo.2010.247
Snyder, J. M., Casey, K. M., Galecki, A., Harrison, D. E., Jayarathne, H., Kumar, N., et al. (2022). Canagliflozin retards age-related lesions in heart, kidney, liver, and adrenal gland in genetically heterogenous male mice. GeroScience 45, 385–397. doi:10.1007/s11357-022-00641-0
Soda, K., Dobashi, Y., Kano, Y., Tsujinaka, S., and Konishi, F. (2009). Polyamine-rich food decreases age-associated pathology and mortality in aged mice. Exp. Gerontol. 44, 727–732. doi:10.1016/j.exger.2009.08.013
Statzer, C., Reichert, P., Dual, J., and Ewald, C. Y. (2022). Longevity interventions temporally scale healthspan in Caenorhabditis elegans. iScience 25, 103983. doi:10.1016/j.isci.2022.103983
Strong, R., Miller, R. A., Astle, C. M., Baur, J. A., de Cabo, R., Fernandez, E., et al. (2013). Evaluation of resveratrol, green tea extract, curcumin, oxaloacetic acid, and medium-chain triglyceride oil on life span of genetically heterogeneous mice. J. Gerontol. A. Biol. Sci. Med. Sci. 68, 6–16. doi:10.1093/gerona/gls070
Strong, R., Miller, R. A., Astle, C. M., Floyd, R. A., Flurkey, K., Hensley, K. L., et al. (2008). Nordihydroguaiaretic acid and aspirin increase lifespan of genetically heterogeneous male mice. Aging Cell 7, 641–650. doi:10.1111/j.1474-9726.2008.00414.x
Strong, R., Miller, R. A., Bogue, M., Fernandez, E., Javors, M. A., Libert, S., et al. (2020). Rapamycin-mediated mouse lifespan extension: Late-life dosage regimes with sex-specific effects. Aging Cell 19, e13269. doi:10.1111/acel.13269
Sukoff Rizzo, S. J., Anderson, L. C., Green, T. L., McGarr, T., Wells, G., and Winter, S. S. (2018). Assessing healthspan and lifespan measures in aging mice: Optimization of testing protocols, replicability, and rater reliability. Curr. Protoc. Mouse Biol. 8, e45. doi:10.1002/cpmo.45
Sun, Z., Zhao, L., Su, L., Fang, Q., Xu, C., Su, Y., et al. (2019). Long-term every-other-day administration of DMAMCL has little effect on aging and age-associated physiological decline in mice. Aging 11, 2583–2609. doi:10.18632/aging.101932
Takemon, Y., Chick, J. M., Gerdes Gyuricza, I., Skelly, D. A., Devuyst, O., Gygi, S. P., et al. (2021). Proteomic and transcriptomic profiling reveal different aspects of aging in the kidney. eLife 10, e62585. doi:10.7554/eLife.62585
Thangthaeng, N., Rutledge, M., Wong, J. M., Vann, P. H., Forster, M. J., and Sumien, N. (2017). Metformin impairs spatial memory and visual acuity in old male mice. Aging Dis. 8, 17–30. doi:10.14336/AD.2016.1010
Tian, H., Ni, Z., Lam, S. M., Jiang, W., Li, F., Du, J., et al. (2022). Precise metabolomics reveals a diversity of aging-associated metabolic features. Small Methods 6, e2200130. doi:10.1002/smtd.202200130
Tran, T., Mach, J., Gemikonakli, G., Wu, H., Allore, H., Howlett, S. E., et al. (2021). Male–female differences in the effects of age on performance measures recorded for 23 hours in mice. J. Gerontol. Ser. A 76, 2141–2146. doi:10.1093/gerona/glab182
United Nations (2019). UN 10 key findings on world population prospects. United Nations, Department of Economic and Social Affairs, Population Division. Available at: https://population.un.org/wpp.
Viecelli, C., and Ewald, C. Y. (2022). The non-modifiable factors age, gender, and genetics influence resistance exercise. Front. Aging 3, 1005848. doi:10.3389/fragi.2022.1005848
Vitiello, D., Dakhovnik, A., Statzer, C., and Ewald, C. Y. (2021). Lifespan-associated gene expression signatures of recombinant BXD mice implicates Coro7 and set in longevity. Front. Genet. 12, 694033. doi:10.3389/fgene.2021.694033
Weimer, S., Priebs, J., Kuhlow, D., Groth, M., Priebe, S., Mansfeld, J., et al. (2014). D-Glucosamine supplementation extends life span of nematodes and of ageing mice. Nat. Commun. 5, 3563. doi:10.1038/ncomms4563
Weir, H. J., Yao, P., Huynh, F. K., Escoubas, C. C., Goncalves, R. L., Burkewitz, K., et al. (2017). Dietary restriction and AMPK increase lifespan via mitochondrial network and peroxisome remodeling. Cell Metab. 26, 884–896. doi:10.1016/j.cmet.2017.09.024
Whitehead, J. C., Hildebrand, B. A., Sun, M., Rockwood, M. R., Rose, R. A., Rockwood, K., et al. (2014). A clinical frailty index in aging mice: Comparisons with frailty index data in humans. J. Gerontol. A. Biol. Sci. Med. Sci. 69, 621–632. doi:10.1093/gerona/glt136
Wilkinson, J. E., Burmeister, L., Brooks, S. V., Chan, C.-C., Friedline, S., Harrison, D. E., et al. (2012). Rapamycin slows aging in mice. Aging Cell 11, 675–682. doi:10.1111/j.1474-9726.2012.00832.x
Wirth, A., Wolf, B., Huang, C.-K., Glage, S., Hofer, S. J., Bankstahl, M., et al. (2021). Novel aspects of age-protection by spermidine supplementation are associated with preserved telomere length. GeroScience 43, 673–690. doi:10.1007/s11357-020-00310-0
Xu, Q., Fu, Q., Li, Z., Liu, H., Wang, Y., Lin, X., et al. (2021). The flavonoid procyanidin C1 has senotherapeutic activity and increases lifespan in mice. Nat. Metab. 3, 1706–1726. doi:10.1038/s42255-021-00491-8
Xue, H., Li, P., Bian, J., Gao, Y., Sang, Y., and Tan, J. (2022). Extraction, purification, structure, modification, and biological activity of traditional Chinese medicine polysaccharides: A review. Front. Nutr. 9, 1005181. doi:10.3389/fnut.2022.1005181
Yuan, R., Musters, C. J. M., Zhu, Y., Evans, T. R., Sun, Y., Chesler, E. J., et al. (2020). Genetic differences and longevity-related phenotypes influence lifespan and lifespan variation in a sex-specific manner in mice. Aging Cell 19, e13263. doi:10.1111/acel.13263
Yuan, R., Tsaih, S.-W., Petkova, S. B., Marin de Evsikova, C., Xing, S., Marion, M. A., et al. (2009). Aging in inbred strains of mice: Study design and interim report on median lifespans and circulating IGF1 levels. Aging Cell 8, 277–287. doi:10.1111/j.1474-9726.2009.00478.x
Zhang, H., Hao, M., Hu, Z., Li, Y., Jiang, X., Wang, J., et al. (2022). Association of immunity markers with the risk of incident frailty: The rugao longitudinal aging study. Immun. Ageing A 19, 1. doi:10.1186/s12979-021-00257-6
Zhang, H., Ryu, D., Wu, Y., Gariani, K., Wang, X., Luan, P., et al. (2016). NAD+ repletion improves mitochondrial and stem cell function and enhances life span in mice. Science 352, 1436–1443. doi:10.1126/science.aaf2693
Zhang, S.-Q., Cai, W.-J., Huang, J.-H., Wu, B., Xia, S.-J., Chen, X.-L., et al. (2015). Icariin, a natural flavonol glycoside, extends healthspan in mice. Exp. Gerontol. 69, 226–235. doi:10.1016/j.exger.2015.06.020
Zhang, Y., Bokov, A., Gelfond, J., Soto, V., Ikeno, Y., Hubbard, G., et al. (2014). Rapamycin extends life and health in C57BL/6 mice. J. Gerontol. A. Biol. Sci. Med. Sci. 69, 119–130. doi:10.1093/gerona/glt056
Zhao, H., and Luo, Y. (2017). Traditional Chinese medicine and aging intervention. Aging Dis. 8, 688–690. doi:10.14336/AD.2017.1002
Keywords: sex differences, lifespan, healthspan, systematic review, pharmacological interventions, aging, mice
Citation: Knufinke M, MacArthur MR, Ewald CY and Mitchell SJ (2023) Sex differences in pharmacological interventions and their effects on lifespan and healthspan outcomes: a systematic review. Front. Aging 4:1172789. doi: 10.3389/fragi.2023.1172789
Received: 23 February 2023; Accepted: 10 May 2023;
Published: 22 May 2023.
Edited by:
Nicola Luigi Bragazzi, University of Parma, ItalyReviewed by:
Yuji Ikeno, The University of Texas Health Science Center at San Antonio, United StatesDevin Wahl, Colorado State University, United States
Copyright © 2023 Knufinke, MacArthur, Ewald and Mitchell. This is an open-access article distributed under the terms of the Creative Commons Attribution License (CC BY). The use, distribution or reproduction in other forums is permitted, provided the original author(s) and the copyright owner(s) are credited and that the original publication in this journal is cited, in accordance with accepted academic practice. No use, distribution or reproduction is permitted which does not comply with these terms.
*Correspondence: Sarah J. Mitchell, c21pdGNoZWxsQGV0aHouY2g=; Collin Y. Ewald, Y29sbGluLWV3YWxkQGV0aHouY2g=
†Present Address: Sarah J. Mitchell, Ludwig Princeton Branch Princeton, University Carl Icahn Laboratory, Princeton, NJ, United States
Michael R. MacArthur, Lewis-Sigler Institute for Integrative Genomics Princeton University, Carl Icahn Laboratory, Princeton, NJ, United States