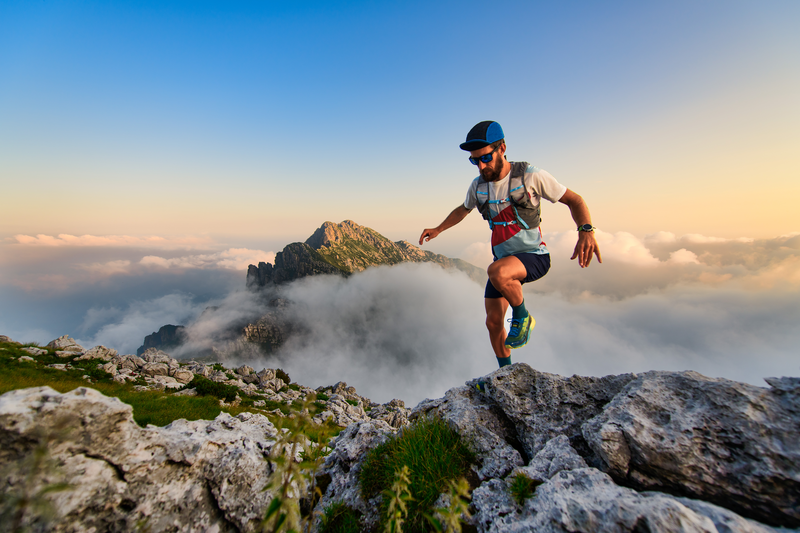
94% of researchers rate our articles as excellent or good
Learn more about the work of our research integrity team to safeguard the quality of each article we publish.
Find out more
ORIGINAL RESEARCH article
Front. Aging , 27 April 2022
Sec. Aging and the Immune System
Volume 3 - 2022 | https://doi.org/10.3389/fragi.2022.848925
This article is part of the Research Topic Insights in Aging and the Immune System: 2021 View all 7 articles
Increased cancer incidence occurs with the emergence of immunosenescence, highlighting the indispensability of the immune system in preventing cancer and its dysregulation with aging. Tumor-associated macrophages (TAMs) are often present in high numbers and are associated with poor clinical outcomes in solid cancers, including mesothelioma. Monocytes and macrophages from the bone marrow and spleen can respond to tumor-derived factors, such as CSF-1, and initiation of the CSF-1R signaling cascade results in their proliferation, differentiation, and migration to the tumor. Age-related changes occur in monocytes and macrophages in terms of numbers and function, which in turn can impact tumor initiation and progression. Whether this is due to changes in CSF-1R expression with aging is currently unknown and was investigated in this study. We examined monocytes and macrophages in the bone marrow and spleen during healthy aging in young (3–4 months) and elderly (20–24 months) female C57BL/6J mice. Additionally, changes to these tissues and in TAMs were examined during AE17 mesothelioma tumor growth. Healthy aging resulted in an expansion of Ly6Chigh monocytes and macrophages in the bone marrow and spleen. CSF-1R expression levels were reduced in elderly splenic macrophages only, suggesting differences in CSF-1R signaling between both cell type and tissue site. In tumor-bearing mice, Ly6Chigh monocytes increased with tumor growth in the spleen in the elderly and increased intracellular CSF-1R expression occurred in bone marrow Ly6Chigh monocytes in elderly mice bearing large tumors. Age-related changes to bone marrow and splenic Ly6Chigh monocytes were reflected in the tumor, where we observed increased Ly6Chigh TAMs earlier and expansion of Ly6Clow TAMs later during AE17 tumor growth in the elderly compared to young mice. F4/80high TAMs increased with tumor growth in both young and elderly mice and were the largest subset of TAMs in the tumor. Together, this suggests there may be a faster transition of Ly6Chigh towards F4/80high TAMs with aging. Amongst TAM subsets, expression of CSF-1R was lowest in F4/80high TAMs, however Ly6Clow TAMs had higher intracellular CSF-1R expression. This suggests downstream CSF-1R signaling may vary between macrophage subsets, which can have implications towards CSF-1R blockade therapies targeting macrophages in cancer.
Advancing age is associated with changes to the immune system, described as immunosenescence (Pawelec and Solana, 1997; Pawelec, 2012). A chronic, low-grade increase in circulating inflammatory factors is also associated with aging, known as inflammaging (Franceschi et al., 2000; Franceschi et al., 2007) and is a likely contributing factor to immunosenescence (Pawelec, 2012; G.; Pawelec and Solana, 1997). Moreover, increased cancer incidence occurs with the emergence of immunosenescence, highlighting the indispensability of the immune system in preventing cancer and its degradation with aging.
Tumor-associated macrophages (TAMs) are often present in high numbers and are associated with poor clinical outcomes in solid cancers (Zhang et al., 2013; Yang et al., 2018; Ding et al., 2019; Yagi et al., 2019). Monocytes are recruited to the tumor from the bone marrow in response to chemotactic signals (Sanford et al., 2013; Shand et al., 2014). In chronic inflammation, monocytes can also be recruited to the tumor from the spleen (Cortez-Retamozo et al., 2012; Cortez-Retamozo et al., 2013; Shand et al., 2014). Tumour infiltrating monocytes are predominantly Ly6Chigh and can be recruited via CSF-1/CSF-1R signaling (Ryder et al., 2013; Ries et al., 2014; Wang et al., 2016; Gyori et al., 2018). As well as stimulating chemotaxis, CSF-1 is a primary growth factor for macrophages and subsequently drives differentiation of myeloid progenitor cells into mature macrophages (Pixley and Stanley, 2004). Following CSF activation, the CSF-1R is internalized and eventually degraded (Guilbert et al., 1986; Kanagasundaram et al., 1998; Lou et al., 2014). Monocytes maturing into TAMs can be distinguished through differential expression of Ly6C and F4/80, with downregulation of Ly6C and upregulation of F4/80 (Shi and Pamer, 2011; Ramachandran et al., 2012; Crane et al., 2014; Franklin et al., 2014).
Age-related changes to monocytes/macrophages could impact tumor initiation and progression. For example, studies have shown a shift towards myelopoiesis with healthy aging (Rossi et al., 2005; Beerman et al., 2010; Pioli et al., 2019), which may lead to a larger pool of monocytes available for recruitment during tumor development. This is supported by our previous study, which showed higher numbers of macrophages in mesotheliomas from elderly compared to young mice (Duong et al., 2018). Function may also be impacted by aging as murine bone marrow-derived macrophages and human peripheral blood mononuclear cell-derived macrophages from aged cohorts stimulated ex vivo with LPS increased levels of pro-inflammatory cytokines such as TNF and IL-6 (Bouchlaka et al., 2013; Barrett et al., 2015; Thevaranjan et al., 2017). In contrast, splenic macrophages from elderly mice stimulated with LPS had reduced TNF, IL-6 and IL-1β production compared to young mice (Gomez et al., 2010; Mahbub et al., 2012). Splenic and peritoneal macrophages from elderly mice also exhibit reduced phagocytosis (Linehan et al., 2014; Tomay et al., 2018). Whether this is due to changes in CSF-1R signaling with aging is currently unknown. Interestingly, two cytokines associated with inflammaging, TNF and IFN-γ, have been shown to downregulate CSF-1R expression (Rovida et al., 2001; Delneste et al., 2003) and circulating CSF-1 is also increased during aging (Suehiro et al., 1999; Larsson et al., 2015; Lira-Junior et al., 2017). Therefore, inflammaging could impact monocyte/macrophage differentiation and CSF-1R expression during aging and cancer.
Given that monocyte/macrophage numbers increase in elderly healthy mice, as do TAMs in tumors from elderly mice (Jackaman et al., 2013), their infiltration into tumors may be mediated by increased CSF-1/CSF-1R signaling. Moreover, altered macrophage responses to pro- and anti-inflammatory stimuli combined with the inflammaging microenvironment may further alter their response. Therefore, this study first investigated changes to monocyte/macrophage proportions and CSF-1/CSF-1R signaling in bone marrow and spleen during healthy aging. We then assessed whether the balance was altered during tumor growth in a murine mesothelioma model, a CSF-1 secreting cancer predominantly found in the elderly (Bianchi and Bianchi, 2007; Magkouta et al., 2021).
Female C57BL/6J mice were obtained from the Animal Research Centre (ARC, Murdoch, WA, Australia) and maintained at Curtin University animal facilities under specific-pathogen free conditions. Young mice were aged 3–4 months (equivalent to 18 year-old humans) and elderly mice were 20–24 months (60–70 year-old humans), as defined by the Jackson Laboratory (Yuan et al., 2009). Mice were excluded from the study if they had enlarged organs, a palpable mass or experienced excessive body weight loss (>15% from age 12 months) prior to tumor inoculation. Animals were housed in a standard light/dark cycle and fed a standard chow diet ab libitum. Experiments were performed as per the Curtin University Animal Ethics Committee (AEC) in accordance to the Australian Code of Practice for the use and care of animals for scientific purposes (AEC approval numbers: AEC_2016_04 and AEC_2020_03).
AE17 is a murine malignant mesothelioma cell line generated by inoculation of asbestos fibers and was derived from orthotopic tumor deposits that emerged in elderly C57BL/6J mice and is histologically representative of human mesothelioma (Jackaman et al., 2003). Mesothelioma cell lines, including AE17, have also been reported to express CSF-1 (Fox et al., 2012; Cioce et al., 2014). Cells were maintained in complete medium, containing RPMI 1640 (Invitrogen) supplemented with 10% HyClone™ fetal bovine serum (FBS, Cytiva, Utah, America), 2 mM L-glutamax (Life Technologies, Victoria, Australia), 100 units/ml of penicillin, 100 μg/ml streptomycin (Life Technologies) and 0.05 mM 2-mercaptoethanol (Sigma-Aldrich) at 37°C with 5% CO2. Cells were collected for tumor inoculation when ≥80% confluent. Mice were inoculated subcutaneously (s.c.) with 5 × 105 cells in 100 μl PBS and body weight, body condition score and tumors monitored daily. Tumor sizes were taken daily using calipers and determined by measurement of tumor width (mm) and length (mm), and calculated as width × length in mm2. Each mouse was tracked individually for tumor growth. Mice were monitored until their individual maximum tumor size reached 140 mm2, weight loss exceeded >20% or reached experimental endpoint. Based on our previous studies, age impacted tumor growth in that tumors grew faster in elderly compared to young mice (Duong et al., 2018). In order to control for variation between young and elderly tumor growth, samples were collected based on tumor size. Tumors were collected at either early (7–10 days post s.c. injection) or late timepoints (18–23 days post s.c. injection); small tumors measured <30 mm2 and large tumors were between 65 and 140 mm2 (Jackaman et al., 2003; Jackaman et al., 2011; Jackaman et al., 2016).
To ensure temperature did not impact CSF-1R expression, all reagents were pre-cooled on ice and samples kept on ice throughout staining. Sample collection was also timed so that young and elderly samples spent the same amount of time in processing ex vivo once tissues were out of the animal. Similar numbers of young and elderly samples were also included in each flow cytometry experiment to control for any inter-assay staining variation. Bone marrow, spleen and tumors were collected into collection/staining buffers: ice-cold phosphate buffered solution (PBS) containing 2% fetal bovine serum and 2 mM EDTA (Sigma-Aldrich). Tibiae and femurs were flushed with a 29 g needle to isolate and create single cell suspension of bone marrow cells. Spleens and tumors were disaggregated gently into single cell suspension between two frosted slides in staining buffer. For flow cytometry surface staining, single cell suspensions were blocked with anti-mouse CD16/32 (clone 93, Biolegend) for 15 min on ice, all subsequent steps were performed on ice in the dark unless otherwise stated. A combination of anti-mouse antibodies diluted in staining buffer were incubated for 30 min followed by PBS and incubated for 15 min with Zombie-NIR™ (Biolegend). Intracellular staining was performed after fixing and permeabilization with True-Nuclear™ Transcription Factor Buffer Set (Biolegend) as per manufacturer’s instructions. Surface and intracellular antibodies were diluted in 1x Transcription Factor permeabilization buffer for 1 h in the dark at room temperature. A combination of the following anti-mouse antibodies were used: anti-CD11b BUV395 (BD) or anti-CD11b Alexafluor® 488 (clone M1/70, Biolegend), Ly6C BV510 (clone HK1.4, Biolegend), anti-Ly6G Brilliant Violet 785™ (clone 1A8, Biolegend), anti-F4/80 PE-Dazzle-594 (clone BM8, Biolegend) or anti-F4/80 Alexafluor® 647 (clone BM8, Biolegend), anti-CD115 (CSF-1R) Brilliant Violet 421™ (clone AFS98, Biolegend) and anti-CD206-PE-Cy7 (clone C068C2, Biolegend). After staining, cells were washed twice and resuspending in staining buffer for acquisition on BD LSRFortessa™ flow cytometer using FACSDiva (BD Biosciences). Unstained, single stains and fluorescent minus one controls were used for instrument setup, compensation and analysis. Data was analyzed using FlowJo version 10.7 (BD Bioscience).
Whole blood was collected directly into EDTA (Sigma-Aldrich) and plasma stored at −20°C until analysis. Samples were collected from young healthy mice, elderly healthy mice, large tumor-bearing young mice and large tumor-bearing elderly mice. The concentration of CSF-1 in plasma samples was measured using a LEGENDplex™ Mouse Hematopoietic Stem Cell Panel (Biolegend) as per manufacturer’s instructions. Data was collected on a LSRFortessa™ flow cytometer using FACSDiva (BD Biosciences) and analyzed using FlowJo version 10.7 (BD Bioscience).
GraphPad Prism version 9 (California, CA, United States) was used to analyze data. Data presented as means ± SEM. Mann-Whitney U-test was used to determine differences between two populations. A relationship between two variables was determined by Pearson’s correlation coefficient test. p-values of <0.05 were considered statistically significant.
Ex vivo flow cytometry analysis of bone marrow from young and elderly healthy mice (gating strategy shown in Figure 1A) revealed an age-specific expansion of CD11b+Ly6GnegF4/80neg monocytes (Figure 1B), a Ly6Chigh subpopulation (Figure 1C) and CD11b+F4/80+Ly6Gneg macrophages (Figure 1D). We next investigated the spleen as it can serve as a site for extramedullary hematopoiesis and contains a reservoir of monocytes that may be deployed to the tumor (Cortez-Retamozo et al., 2012; Cortez-Retamozo et al., 2013). Similar to bone marrow, healthy aging leads to the expansion of total monocytes (Figure 1E), the Ly6Chigh subpopulation (Figure 1F) and CD11b+F4/80+Ly6Gneg red pulp macrophages (Figure 1G) in the spleen.
FIGURE 1. Bone marrow and splenic monocytes and macrophages increased with healthy aging. Bone marrow and spleen from healthy, non-cancer-bearing mice were dissociated into single cell suspension and stained for CD11b, F4/80, Ly6G and Ly6C and analyzed via flow cytometry. Macrophages were recognized as CD11b+F4/80+Ly6Gneg and total monocytes as CD11b+F4/80negLy6Gneg with Ly6Chigh monocytes further identified [a representative gating strategy shown in (A). Total monocytes, Ly6Chigh monocytes and macrophages shown as percentage of total cells in the bone marrow (B−D), respectively] and in the spleen [(E−G), respectively]. HY, healthy young; HE, healthy elderly. Data shown as mean ± SEM; n = 7 mice/group; *p < 0.05, **p < 0.01, ***p < 0.005.
As CSF-1R signaling is crucial in monocyte to macrophage differentiation, expansion, and migration (reviewed in Pixley and Stanley, 2004), we next measured expression of this molecule to determine whether altered expression levels may account for the increased proportion of these cells in older tissues. While CSF-1R surface and intracellular expression was unchanged between young and elderly healthy macrophage and monocyte populations in the bone marrow (Figure 2A and Supplementary Figure S1) and splenic monocytes (Supplementary Figure S2), a significant age-specific decrease in surface and intracellular CSF-1R was observed in splenic macrophages (Figures 2B,C, respectively). These data show that CSF-1R expression is reduced with healthy aging in splenic macrophages, highlighting age- and tissue-related differential regulation of CSF-1R expression.
FIGURE 2. CSF-1R expression decreased in splenic macrophages with healthy aging. CD11b+F4/80+Ly6Gneg macrophage CSF-1R surface expression in the bone marrow (A) and spleen (B) and intracellular CSF-1R expression in the spleen (C) shown as median fluorescence intensity (MFI). HY—healthy young, HE, healthy elderly. Data shown as mean ± SEM; n = 7–8 mice/group; *p < 0.05, **p < 0.01.
Previously we showed that solid tumors impact the bone marrow macrophage compartment, leading to increased bone marrow macrophage proliferation in elderly-tumor bearing mice compared to young mice (Duong et al., 2018). In this study, we expanded this work to include monocytes with a particular focus on the Ly6Chigh subset, which infiltrates tumors and differentiates into TAMs (Movahedi et al., 2010; Van Overmeire et al., 2015). As various stages of tumor progression may impact these cells differently, we investigated the bone marrow at small (early stage) and large (late stage) AE17 mesothelioma tumors. Total bone marrow monocytes increased due to aging rather than tumor growth (Figure 3A). Similarly, the proportion of Ly6Chigh monocytes increased with healthy aging in the bone marrow and was not further affected by tumor growth (Figure 3B). Macrophage proportions remained similar between young and elderly mice bearing tumors (Figure 3C). However, when compared to healthy elderly controls, macrophage proportions were reduced in the bone marrow of elderly mice with cancer (Figure 3C), despite displaying increased proliferation (Duong et al., 2018). It is possible this is due to reduced survival or migration of the cells from the bone marrow to support tumor growth. Next, the splenic monocyte and macrophage compartments were examined during AE17 tumor development. The profile of total splenic monocytes was similar to that seen in the bone marrow with an age-related increase in numbers in small and large AE17 tumors (Figure 3D). However, splenic Ly6Chigh monocytes increased markedly in elderly mice during tumor progression while their proportions remained steady in young spleens (Figure 3E). This resulted in an almost 3-fold increase in Ly6Chigh proportions in the elderly compared to young mice with large tumors. Similarly, splenic macrophage proportions were significantly higher in elderly vs. young AE17-bearing hosts and was further augmented with tumour growth (Figure 3F).
FIGURE 3. Splenic monocyte and macrophage pools increase with age and is further exacerbated with tumor growth. Young and elderly C57BL/6J mice were inoculated with AE17 mesothelioma tumor cells and sacrificed when tumors were small (early time point, tumor size <30 mm2) or large (late time point, tumor size 65–140 mm2). Total monocytes (CD11b+F4/80negLy6Gneg), Ly6Chigh monocytes and macrophages (CD11b+F4/80+Ly6Gneg) shown as percentage of total cells in the bone marrow [(A−C), respectively) and the spleen [(D–F), respectively). Data on healthy mice (Figure 1) have been included here as controls. HY, healthy young; HE, healthy elderly; STY, small tumor young; STE, small tumor elderly; LTY, large tumor young; LTE, large tumor elderly. Data shown as mean ± SEM; n = 6–7 mice/group; *p < 0.05, **p < 0.01.
We also examined levels of CSF-1R expression in monocytes and macrophages from the bone marrow and splenic compartments in tumor-bearing mice. Interestingly, intracellular CSF-1R expression in Ly6Chigh bone marrow monocytes increased in the elderly compared to young with large tumors (Figure 4A), which may reflect increased internalization of activated CSF-1R (Yeung and Stanley, 2003). Furthermore, intracellular CSF-1R expression increased in macrophages of elderly mice during early tumor growth but matched the levels of younger counterparts when tumors were larger (Figure 4B). Surface expression of CSF-1R in bone marrow macrophages was slightly but not significantly reduced in elderly vs. young mice with large tumors (Figure 4C). In the spleen, intracellular CSF-1R expression in total monocytes remained similar through all cohorts and tumor growth (Supplementary Figure S3). However, splenic macrophages in young tumor-bearing mice demonstrated increased CSF-1R expression that was restricted to the intracellular compartment (Figure 4D) and not seen on the cell surface (Figure 4E) when compared to the healthy setting. Intracellular CSF-1R expression in these cells also increased with tumor growth (Figure 4D). These data suggest CSF-1R internalization in young mice during tumor growth. However, there were no changes to CSF-1R expression during tumor growth in elderly mice (and compared to healthy) suggesting splenic macrophages from tumor-bearing mice could be refractory to CSF-1R signaling (Figure 4D,E). These data suggest that CSF-1R expression may be impacted with aging and tumor progression but varies depending on cell type. Previous studies have reported increased circulating CSF-1 during aging (Suehiro et al., 1999; Larsson et al., 2015; Lira-Junior et al., 2017) which could impact the spleen as a blood filter. Furthermore, the AE17 cell line used in this study expresses CSF-1 (Fox et al., 2012). However, we observed no difference in CSF-1 circulating protein levels during healthy aging (Figure 4F) and circulating CSF-1 levels were decreased in the plasma of elderly large tumor-bearing mice compared to elderly healthy controls (Figure 4F). Combined with the overall changes to CSF-1R expression it is possible there is increased utilization of CSF-1 in elderly tumor-bearing mice.
FIGURE 4. Bone marrow and splenic monocyte and macrophage CSF-1R expression is differentially impacted by aging and cancer. Young and elderly C57BL/6J mice were inoculated with AE17 mesothelioma cells and sacrificed when tumors were at small (early time point, tumor size <30 mm2) or large (late time point, tumor size 65–140 mm2). Bone marrow Ly6Chigh monocyte (CD11b+F4/80negLy6GnegLy6Chigh) (A) and macrophage (CD11b+F4/80+Ly6Gneg) intracellular (B) and surface (C) CSF-1R expression shown as median fluorescence intensity (MFI). Splenic macrophage intracellular (D) and cell surface (E) CSF-1R expression shown as MFI. Data on healthy mice (Figure 1) have been included here as controls. Expression shown as median fluorescence intensity (MFI). Circulating CSF-1 levels in plasma samples shown in (F). HY, healthy young; HE, healthy elderly; STY, small tumor young; STE, small tumor elderly; LTY, large tumor young; LTE, large tumor elderly. Data shown as mean ± SEM; n = 6–8 mice/group for (A–E) and 17–19 mice/group for (F); *p < 0.05, **p < 0.01, ***p < 0.005.
The data presented thus far indicate an increased supply of monocytes and macrophages during aging, which may be further expanded with tumor growth. Ly6Chigh monocytes may be particularly important for the supply of TAMs to tumors. Increased availability of these cells could result in faster tumor growth, as reported in our earlier study (Duong et al., 2018). Furthermore, alterations to CSF-1R expression may impact the supply potential of these cells in terms of numbers and migration. This prompted us to investigate infiltrating monocyte/macrophages and their maturation in the tumor via differential expression of Ly6C and F4/80 (Ramachandran et al., 2012; Crane et al., 2014): with F4/80intLy6Chigh (Ly6Chigh) TAMs, representing early monocyte-derived TAMs. The loss of Ly6C expression is associated with maturation and therefore we identified F4/80intLy6Clow (Ly6Clow) TAMs as an intermediate subset, and F4/80highLy6Clow/high (F4/80high) TAMs as a mature population (gating strategy shown in Figure 5A). We found that Ly6Chigh TAMs increased significantly in the elderly early during tumor development (Figure 5B), whereas Ly6Clow proportions increased with aging, which was further exacerbated with tumor growth in the elderly (Figure 5C). However, F4/80high TAMs expanded with tumor growth in both young and elderly and were the largest pool of TAM subsets in the tumor, particularly at later stages of tumor growth (Figure 5D). There was no difference in expression of the pro-tumoral marker CD206 in Ly6Chigh or Ly6Clow TAMs during young and elderly tumor growth (Figures 5E,F respectively). In contrast F4/80high TAMs from elderly tumor-bearing mice displayed increased CD206 expression with increasing tumor growth and compared to young mice (Figure 5G). Together, this may suggest a faster transition of Ly6Chigh monocyte-derived TAMs towards Ly6Clow and pro-tumoral F4/80high TAMs with aging. CSF-1R expression was similar between TAMs in young and elderly, however F4/80high cells displayed the lowest expression of CSF-1R and intracellular CSF-1R expression was highest in Ly6Clow TAMs (Supplementary Figure S4A,B). This suggests CSF-1R signaling may vary across TAM subsets.
FIGURE 5. Ly6Chigh tumor-associated macrophages (TAMs) increased earlier and Ly6Clow TAMs later during AE17 tumor development. Young and elderly C57BL/6J mice were inoculated with AE17 mesothelioma cells and sacrificed when tumors were at small (early time point, tumor size <30 mm2) or large (late time point, tumor size 65–140 mm2). Tumors were dissociated into single cell suspension and stained for CD11b, F4/80, Ly6G and Ly6C and analyzed via flow cytometry [a representative gating strategy shown in (A)]. The percentage of total cells and intracellular CD206 expression shown for each TAM subset: Ly6Chigh (CD11b+Ly6GnegF4/80intLy6Chigh) (B and E), Ly6Clow (CD11b+Ly6GnegF4/80intLy6Clow) (C and F) and F4/80high (CD11b+Ly6GnegF4/80highLy6Chigh/low) (D and G). STY—small tumor young, STE, small tumor elderly; LTY, large tumor young; LTE, large tumor elderly. Data shown as mean ± SEM; n = 9 mice/group; *p < 0.05, ****p < 0.001.
Monocyte and macrophage infiltration into the tumor microenvironment may be facilitated by CSF-1/CSF-1R signaling and our previous studies indicate an expansion of TAMs in elderly vs. young mice (Duong et al., 2018). Therefore, in this study we investigated the impact of aging and CSF-1R signaling on monocyte and macrophages in the bone marrow, spleen and in TAMs during tumor growth. We showed an expanded reservoir of macrophages and monocytes, specifically the Ly6Chigh subpopulation, with aging and cancer progression. This corresponds with increased proportions of Ly6Chigh TAMs early and Ly6Clow TAMs later during tumor development in the elderly. Faster maturation of these cells to F4/80high TAMs may occur with aging, supported by increased CD206 expression. Furthermore, we found CSF-1R expression levels differed depending on tissue site, cell type/subset, and that this may be further impacted by age.
It is now well-established that aging leads to an increase in myelopoiesis (Rossi et al., 2005; Cho et al., 2008; Dykstra et al., 2011; Ho et al., 2017), with expansion of monocyte and macrophage numbers (Strohacker et al., 2012; Jackaman et al., 2013; Puchta et al., 2016; Duong et al., 2018). This was corroborated in the present study where we saw increased proportions of these cells in the bone marrow and spleen of healthy elderly mice. The increase in Ly6Chigh monocytes suggests that an augmented pool of these cells can be mobilized when the need arises. Although the main site for this is thought to be the bone marrow, the spleen can provide an emergency supply of these cells if required (Cortez-Retamozo et al., 2012; Cortez-Retamozo et al., 2013).
CSF-1R expression is important for the proliferation, differentiation, maintenance, and migration of monocytes/macrophages (Reviewed in Pixley and Stanley, 2004; Yu et al., 2008; Pixley, 2012; Stanley and Chitu, 2014; Sinha et al., 2021). We did not observe any differences in expression of CSF-1R on monocytes in the bone marrow and spleen. However, in a study by Hearps et al. (2012), aging was found to be associated with decreased CSF-1R on monocytes in humans. Interestingly, we observed decreased CSF-1R in splenic macrophages. This reduction was less apparent in bone marrow macrophages, which suggests there are cell lineage- and site-specific differences. The spleen is one of the main filters for circulating blood and therefore is more likely to be impacted by the aging microenvironment compared to the bone marrow. Surface CSF-1R can be downregulated in several instances, including endocytosis due to CSF-1 ligand binding (Lou et al., 2014) and in the presence of IFN-γ (Delneste et al., 2003). Interestingly, IFN-γ can stimulate monocytes to produce CSF-1 and drive their differentiation towards macrophages (Delneste et al., 2003). Moreover, IFN-γ production was found to be upregulated with age (Bandrés et al., 2000; Singh et al., 2011). Studies have also shown that circulating levels of CSF-1 increase during aging (Suehiro et al., 1999; Larsson et al., 2015; Lira-Junior et al., 2017). In contrast, we observed no difference in CSF-1 levels during healthy aging and there was a decrease in circulating CSF-1 in elderly-tumor bearing mice. These differences between studies could potentially be due to mice strains and/or sex differences (reviewed in Duong et al., 2021) as CSF-1 is reported to be higher in males (Ramsey et al., 2012), with conflicting studies reported in females (discussed in Lira-Junior et al., 2017). Our study examined female mice and analysis of sex-specific differences in CSF-1R expression during aging requires further investigation. Overall, it is possible that aging leads to an increased potential for monocyte to macrophage differentiation, through CSF-1R signaling/increased CSF-1 utilization, that is mediated by both the aging and tumor microenvironment. This may explain the increased macrophage proportions associated with changes to CSF-1R expression during aging.
The age-related expansion of bone marrow monocyte and macrophage pools can potentially supply more infiltrating cells to a solid tumor. This may be mediated through CSF-1R signaling. Surface expression of CSF-1R was downregulated in total monocytes suggesting that aging downregulates this molecule. Interestingly, at later stages of tumor development, bone marrow Ly6Chigh monocytes in the elderly had significantly increased intracellular CSF-1R expression compared to their younger counterparts. This was similar in bone marrow macrophages but occurred early during tumor growth. These data suggest increased CSF-1R signaling, which can contribute to the motility and egress of these cells from the bone marrow in response to tumor-derived factors.
Our study showed increased proportions of splenic monocytes in the elderly compared to young, in healthy and tumor-bearing mice. This was likewise observed in splenic macrophages; however, the impact of the tumor was greater in the elderly. The pool of macrophages in the spleen was further amplified in the elderly with tumor induction (healthy vs. early-stage tumors) and progression, whereas macrophage proportions in the young remained similar throughout. Therefore, aging drives an increased pool of monocytes and macrophages in the spleen, which is further amplified with tumor growth. Splenic Ly6Chigh monocytes in the elderly also follow this expansion process during tumor growth, resulting in almost a tripling of proportions compared to young at late tumor growth. The increase in the availability of these cells in the elderly may be critical to cancer growth and outcomes, as splenic Ly6Chigh monocytes are important tumor infiltrating cells (Cortez-Retamozo et al., 2013; Shand et al., 2014; de-Brito et al., 2019). However, the contribution of the spleen to the tumor during aging should be investigated further, as these studies were conducted using young mice.
The increased reservoir of monocytes and macrophages with aging and its further exacerbation with cancer in the elderly may translate to changes to TAM proportions. Indeed, we observed increased monocyte-derived Ly6Chigh TAMs at early stages of tumor development, followed by intermediate Ly6Clow TAMs expanding at later stages of tumor development in the elderly compared to young. This suggests that more TAM progenitors infiltrate the tumor and mature more quickly into F4/80high TAMs in the elderly. This was also supported by increased expression of the pro-tumoral marker CD206 in F4/80high TAMs during aging. The highest proportion of TAM subsets in the tumor at any given stage are the F4/80high cells (Figure 5), which further increase with tumor growth. The implication of this may be drawn from our previous study, showing F4/80high TAMs are responsible for poorer anti-tumor immunity, as depletion of this population significantly slowed tumor growth and improved immunotherapy in the elderly (Duong et al., 2018).
CSF-1R expression may be differentially regulated in distinct TAM populations, with intracellular levels highest amongst the Ly6Clow subset. In contrast, F4/80high TAMs had relatively lower CSF-1R expression both surface and intracellularly, which may impact their response to CSF-1R blockade therapies in cancer. In previous studies, CSF-1R inhibition was shown to target pro-tumorigenic TAMs in glioblastoma (Pyonteck et al., 2013) and pancreatic tumor models in mice (Zhu et al., 2014). Counterintuitively to our data, Zhang et al. (2020) showed in Renca tumours that F4/80high expressing TAMs were preferentially depleted and F4/80low TAMs increased proportionally with treatment, likely owing to differential sensitivity to CSF-1R blockade. However, another possibility is that CSF-1R inhibition targeted “earlier” TAM populations, minimizing the transition of these cells to mature F4/80high TAMs and therefore reducing their proportion in the tumor. Interestingly, timing of CSF-1R inhibition can impact anti-tumor response with earlier treatment being more effective (O’Brien et al., 2021), likely due to the distribution of TAM subsets at different stages of tumor development. This may therefore have severe implications in the aging environment where differences were observed in TAM subsets and to progenitor cells in the bone marrow and spleen. Thus, future work should delineate the effects of CSF-1R inhibition on these cell populations.
In our study we described the changes to CSF-1R expression with aging and cancer in monocytes and macrophages, however the presence of CSF-1 affects surface and intracellular expression of CSF-1R. This includes receptor dimerization and autophosphorylation, initiation of downstream signaling, internalization of the receptor and its degradation via the lysosome pathway (Yeung and Stanley, 2003). Thus, future work could investigate CSF-1R pathway activation, receptor internalization and degradation during aging. In summary, we have shown that monocyte and macrophage populations in the bone marrow and spleen increased during healthy aging and was further impacted with cancer. CSF-1R expression in these cells were altered with age, which may impact the migration, expansion, differentiation, and survival of TAMs and, therefore, may contribute to cancer progression. There were also proportional changes to TAM subsets in elderly versus young tumors, whereby there may be a faster transition from infiltrating monocyte-derived Ly6Chigh TAMs towards mature F4/80high TAMs, with differences in CSF-1R expression. These changes may have implications towards the therapeutic effects of CSF-1/CSF-1R signaling blockade.
The raw data supporting the conclusion of this article will be made available by the authors, without undue reservation.
The animal study was reviewed and approved by the Curtin University Animal Ethics Committee.
All authors designed the project and experiments. CJ was responsible for overall co-ordination of research activity and management of financial support for the project. DN, FP, and CJ provided supervision for research activity planning and study execution. LD and CJ performed the experiments and LD analyzed the data. All authors contributed to writing, reviewing and editing the manuscript.
This work was funded by the Cancer Council Western Australia and Curtin Medical School, Curtin University. Healthy mice included in this study were funded by the National Health and Medical Research Council of Australia.
DN acts as a non-salaried Chief Scientific Officer for Selvax.
The remaining authors declare that the research was conducted in the absence of any commercial or financial relationships that could be construed as a potential conflict of interest.
All claims expressed in this article are solely those of the authors and do not necessarily represent those of their affiliated organizations, or those of the publisher, the editors and the reviewers. Any product that may be evaluated in this article, or claim that may be made by its manufacturer, is not guaranteed or endorsed by the publisher.
The authors acknowledge the Curtin Health Innovation Research Institute and the Curtin Medical School, Curtin University for provision of research facilities and technology platforms utilized in this study. LD was supported by Cancer Council Western Australia Honours and PhD Top-up Scholarships.
The Supplementary Material for this article can be found online at: https://www.frontiersin.org/articles/10.3389/fragi.2022.848925/full#supplementary-material
Bandrés, E., Merino, J., Vázquez, B., Inogés, S., Moreno, C., Subirá, M. L., et al. (2000). The Increase of IFN-γ Production through Aging Correlates with the Expanded CD8+highCD28−CD57+ Subpopulation. Clin. Immunol. 96 (3), 230–235. doi:10.1006/clim.2000.4894
Barrett, J. P., Costello, D. A., O’Sullivan, J., Cowley, T. R., and Lynch, M. A. (2015). Bone Marrow-Derived Macrophages from Aged Rats Are More Responsive to Inflammatory Stimuli. J. Neuroinflamm. 12 (1), 67. doi:10.1186/s12974-015-0287-7
Beerman, I., Bhattacharya, D., Zandi, S., Sigvardsson, M., Weissman, I. L., Bryder, D., et al. (2010). Functionally Distinct Hematopoietic Stem Cells Modulate Hematopoietic Lineage Potential during Aging by a Mechanism of Clonal Expansion. Proc. Natl. Acad. Sci. U.S.A. 107 (12), 5465–5470. doi:10.1073/pnas.1000834107
Bianchi, C., and Bianchi, T. (2007). Malignant Mesothelioma: Global Incidence and Relationship with Asbestos. Ind. Health 45 (3), 379–387. doi:10.2486/indhealth.45.379
Bouchlaka, M. N., Sckisel, G. D., Chen, M., Mirsoian, A., Zamora, A. E., Maverakis, E., et al. (2013). Aging Predisposes to Acute Inflammatory Induced Pathology after Tumor Immunotherapy. J. Exp. Med. 210 (11), 2223–2237. doi:10.1084/jem.20131219
Cho, R. H., Sieburg, H. B., and Muller-Sieburg, C. E. (2008). A New Mechanism for the Aging of Hematopoietic Stem Cells: Aging Changes the Clonal Composition of the Stem Cell Compartment but Not Individual Stem Cells. Blood 111 (12), 5553–5561. doi:10.1182/blood-2007-11-123547
Cioce, M., Canino, C., Goparaju, C., Yang, H., Carbone, M., and Pass, H. I. (2014). Autocrine CSF-1R Signaling Drives Mesothelioma Chemoresistance via AKT Activation. Cell Death Dis. 5 (4), e1167. doi:10.1038/cddis.2014.136
Cortez-Retamozo, V., Etzrodt, M., Newton, A., Rauch, P. J., Chudnovskiy, A., Berger, C., et al. (2012). Origins of Tumor-Associated Macrophages and Neutrophils. Proc. Natl. Acad. Sci. U.S.A. 109 (7), 2491–2496. doi:10.1073/pnas.1113744109
Cortez-Retamozo, V., Etzrodt, M., Newton, A., Ryan, R., Pucci, F., Sio, S. W., et al. (2013). Angiotensin II Drives the Production of Tumor-Promoting Macrophages. Immunity 38 (2), 296–308. doi:10.1016/j.immuni.2012.10.015
Crane, M. J., Daley, J. M., van Houtte, O., Brancato, S. K., Henry, W. L., and Albina, J. E. (2014). The Monocyte to Macrophage Transition in the Murine Sterile Wound. PLOS ONE 9 (1), e86660. doi:10.1371/journal.pone.0086660
de-Brito, N. M., da-Costa, H. C., Simões, R. L., and Barja-Fidalgo, C. (2019). Lipoxin-Induced Phenotypic Changes in CD115+LY6Chi Monocytes TAM Precursors Inhibits Tumor Development. Front. Oncol. 9, 540. doi:10.3389/fonc.2019.00540
Delneste, Y., Charbonnier, P., Herbault, N., Magistrelli, G., Caron, G., Bonnefoy, J.-Y., et al. (2003). Interferon-γ Switches Monocyte Differentiation from Dendritic Cells to Macrophages. Blood 101 (1), 143–150. doi:10.1182/blood-2002-04-1164
Ding, W., Tan, Y., Qian, Y., Xue, W., Wang, Y., Jiang, P., et al. (2019). Clinicopathologic and Prognostic Significance of Tumor-Associated Macrophages in Patients with Hepatocellular Carcinoma: A Meta-Analysis. PLOS ONE 14 (10), e0223971. doi:10.1371/journal.pone.0223971
Duong, L., Radley-Crabb, H. G., Gardner, J. K., Tomay, F., Dye, D. E., Grounds, M. D., et al. (2018). Macrophage Depletion in Elderly Mice Improves Response to Tumor Immunotherapy, Increases Anti-tumor T Cell Activity and Reduces Treatment-Induced Cachexia. Front. Genet. 9, 526. doi:10.3389/fgene.2018.00526
Duong, L., Radley, H., Lee, B., Dye, D., Pixley, F., Grounds, M., et al. (2021). Macrophage Function in the Elderly and Impact on Injury Repair and Cancer. Immun. Ageing 18, 4. doi:10.1186/s12979-021-00215-2
Dykstra, B., Olthof, S., Schreuder, J., Ritsema, M., and de Haan, G. (2011). Clonal Analysis Reveals Multiple Functional Defects of Aged Murine Hematopoietic Stem Cells. J. Exp. Med. 208 (13), 2691–2703. doi:10.1084/jem.20111490
Fox, S. A., Loh, S. S., Mahendran, S. K., and Garlepp, M. J. (2012). Regulated Chemokine Gene Expression in Mouse Mesothelioma and Mesothelial Cells: TNF-α Upregulates Both CC and CXC Chemokine Genes. Oncol. Rep. 28 (2), 707–713. doi:10.3892/or.2012.1809
Franceschi, C., Bonafè, M., Valensin, S., Olivieri, F., De Luca, M., Ottaviani, E., et al. (2000). Inflamm-Aging: An Evolutionary Perspective on Immunosenescence. Ann. N. Y Acad. Sci. 908, 244–254. doi:10.1111/j.1749-6632.2000.tb06651.x
Franceschi, C., Capri, M., Monti, D., Giunta, S., Olivieri, F., Sevini, F., et al. (2007). Inflammaging and Anti-inflammaging: a Systemic Perspective on Aging and Longevity Emerged from Studies in Humans. Mech. Ageing Dev. 128 (1), 92–105. doi:10.1016/j.mad.2006.11.016
Franklin, R. A., Liao, W., Sarkar, A., Kim, M. V., Bivona, M. R., Liu, K., et al. (2014). The Cellular and Molecular Origin of Tumor-Associated Macrophages. Science 344 (6186), 921–925. doi:10.1126/science.1252510
Gomez, C. R., Karavitis, J., Palmer, J. L., Faunce, D. E., Ramirez, L., Nomellini, V., et al. (2010). Interleukin-6 Contributes to Age-Related Alteration of Cytokine Production by Macrophages. Mediators Inflamm. 2010, 1–7. doi:10.1155/2010/475139
Guilbert, L. J., Tynan, P. W., and Stanley, E. R. (1986). Uptake and Destruction of125I-CSF-1 by Peritoneal Exudate Macrophages. J. Cel. Biochem. 31 (3), 203–216. doi:10.1002/jcb.240310303
Gyori, D., Lim, E. L., Grant, F. M., Spensberger, D., Roychoudhuri, R., Shuttleworth, S. J., et al. (2018). Compensation between CSF1R+ Macrophages and Foxp3+ Treg Cells Drives Resistance to Tumor Immunotherapy. JCI Insight 3 (11), e120631. doi:10.1172/jci.insight.120631
Hearps, A. C., Martin, G. E., Angelovich, T. A., Cheng, W.-J., Maisa, A., Landay, A. L., et al. (2012). Aging Is Associated with Chronic Innate Immune Activation and Dysregulation of Monocyte Phenotype and Function. Aging Cell 11 (5), 867–875. doi:10.1111/j.1474-9726.2012.00851.x
Ho, T. T., Warr, M. R., Adelman, E. R., Lansinger, O. M., Flach, J., Verovskaya, E. V., et al. (2017). Autophagy Maintains the Metabolism and Function of Young and Old Stem Cells. Nature 543 (7644), 205–210. doi:10.1038/nature21388
Jackaman, C., Bundell, C. S., Kinnear, B. F., Smith, A. M., Filion, P., van Hagen, D., et al. (2003). IL-2 Intratumoral Immunotherapy Enhances CD8+ T Cells that Mediate Destruction of Tumor Cells and Tumor-Associated Vasculature: a Novel Mechanism for IL-2. J. Immunol. 171 (10), 5051–5063. doi:10.4049/jimmunol.171.10.5051
Jackaman, C., Cornwall, S., Graham, P. T., and Nelson, D. J. (2011). CD40‐activated B Cells Contribute to Mesothelioma Tumor Regression. Immunol. Cel. Biol. 89 (2), 255–267. doi:10.1038/icb.2010.88
Jackaman, C., Radley‐Crabb, H. G., Soffe, Z., Shavlakadze, T., Grounds, M. D., and Nelson, D. J. (2013). Targeting Macrophages Rescues Age‐related Immune Deficiencies in C57 BL/6J Geriatric Mice. Aging Cell 12 (3), 345–357. doi:10.1111/acel.12062
Jackaman, C., Yeoh, T. L., Acuil, M. L., Gardner, J. K., and Nelson, D. J. (2016). Murine Mesothelioma Induces Locally-Proliferating IL-10+TNF-α+CD206−CX3CR1+M3 Macrophages that Can Be Selectively Depleted by Chemotherapy or Immunotherapy. OncoImmunology 5 (6), e1173299. doi:10.1080/2162402x.2016.1173299
Kanagasundaram, V., Christy, E., Hamilton, A. J., and Jaworowski, A. (1998). Different Pathways of colony-stimulating Factor 1 Degradation in Macrophage Populations Revealed by Wortmannin Sensitivity. Biochem. J. 330 (Pt 1), 197–202. doi:10.1042/bj3300197
Larsson, A., Carlsson, L., Gordh, T., Lind, A.-L., Thulin, M., and Kamali-Moghaddam, M. (2015). The Effects of Age and Gender on Plasma Levels of 63 Cytokines. J. Immunol. Methods 425, 58–61. doi:10.1016/j.jim.2015.06.009
Linehan, E., Dombrowski, Y., Snoddy, R., Fallon, P. G., Kissenpfennig, A., and Fitzgerald, D. C. (2014). Aging Impairs Peritoneal but Not Bone Marrow-Derived Macrophage Phagocytosis. Aging Cell 13 (4), 699–708. doi:10.1111/acel.12223
Lira-Junior, R., Åkerman, S., Gustafsson, A., Klinge, B., and Boström, E. A. (2017). Colony Stimulating Factor-1 in Saliva in Relation to Age, Smoking, and Oral and Systemic Diseases. Sci. Rep. 7 (1), 7280. doi:10.1038/s41598-017-07698-4
Lou, J., Low-Nam, S. T., Kerkvliet, J. G., and Hoppe, A. D. (2014). Delivery of the CSF-1R to the Lumen of Macropinosomes Promotes its Destruction in Macrophages. J. Cel. Sci. 127 (Pt 24), 5228–5239. doi:10.1242/jcs.154393
Magkouta, S. F., Vaitsi, P. C., Pappas, A. G., Iliopoulou, M., Kosti, C. N., Psarra, K., et al. (2021). CSF1/CSF1R Axis Blockade Limits Mesothelioma and Enhances Efficiency of Anti-PDL1 Immunotherapy. Cancers 13 (11), 2546. doi:10.3390/cancers13112546
Mahbub, S., Deburghgraeve, C. R., and Kovacs, E. J. (2012). Advanced Age Impairs Macrophage Polarization. J. Interferon Cytokine Res. 32 (1), 18–26. doi:10.1089/jir.2011.0058
Movahedi, K., Laoui, D., Gysemans, C., Baeten, M., Stangé, G., Van den Bossche, J., et al. (2010). Different Tumor Microenvironments Contain Functionally Distinct Subsets of Macrophages Derived from Ly6C(high) Monocytes. Cancer Res. 70 (14), 5728–5739. doi:10.1158/0008-5472.Can-09-4672
O’Brien, S. A., Orf, J., Skrzypczynska, K. M., Tan, H., Kim, J., DeVoss, J., et al. (2021). Activity of Tumor-Associated Macrophage Depletion by CSF1R Blockade Is Highly Dependent on the Tumor Model and Timing of Treatment. Cancer Immunol. Immunother. 70 (8), 2401–2410. doi:10.1007/s00262-021-02861-3
Pawelec, G., and Solana, R. (1997). Immunosenescence. Immunol. Today 18 (11), 514–516. doi:10.1016/s0167-5699(97)01145-6
Pawelec, G. (2012). Hallmarks of Human “Immunosenescence”: Adaptation or Dysregulation? Immun. Ageing 9 (1), 15. doi:10.1186/1742-4933-9-15
Pioli, P. D., Casero, D., Montecino-Rodriguez, E., Morrison, S. L., and Dorshkind, K. (2019). Plasma Cells Are Obligate Effectors of Enhanced Myelopoiesis in Aging Bone Marrow. Immunity 51 (2), 351–366.e6. doi:10.1016/j.immuni.2019.06.006
Pixley, F. J., and Stanley, E. R. (2004). CSF-1 Regulation of the Wandering Macrophage: Complexity in Action. Trends Cel. Biol. 14 (11), 628–638. doi:10.1016/j.tcb.2004.09.016
Pixley, F. J. (2012). Macrophage Migration and its Regulation by CSF-1. Int. J. Cel Biol. 2012, 1–12. doi:10.1155/2012/501962
Puchta, A., Naidoo, A., Verschoor, C. P., Loukov, D., Thevaranjan, N., Mandur, T. S., et al. (2016). TNF Drives Monocyte Dysfunction with Age and Results in Impaired Anti-pneumococcal Immunity. Plos Pathog. 12 (1), e1005368. doi:10.1371/journal.ppat.1005368
Pyonteck, S. M., Akkari, L., Schuhmacher, A. J., Bowman, R. L., Sevenich, L., Quail, D. F., et al. (2013). CSF-1R Inhibition Alters Macrophage Polarization and Blocks Glioma Progression. Nat. Med. 19 (10), 1264–1272. doi:10.1038/nm.3337
Ramachandran, P., Pellicoro, A., Vernon, M. A., Boulter, L., Aucott, R. L., Ali, A., et al. (2012). Differential Ly-6C Expression Identifies the Recruited Macrophage Phenotype, Which Orchestrates the Regression of Murine Liver Fibrosis. Proc. Natl. Acad. Sci. U.S.A. 109 (46), E3186. doi:10.1073/pnas.1119964109
Ramsey, J. M., Schwarz, E., Guest, P. C., van Beveren, N. J. M., Leweke, F. M., Rothermundt, M., et al. (2012). Molecular Sex Differences in Human Serum. PLoS One 7 (12), e51504. doi:10.1371/journal.pone.0051504
Ries, C. H., Cannarile, M. A., Hoves, S., Benz, J., Wartha, K., Runza, V., et al. (2014). Targeting Tumor-Associated Macrophages with Anti-CSF-1r Antibody Reveals a Strategy for Cancer Therapy. Cancer Cell 25 (6), 846–859. doi:10.1016/j.ccr.2014.05.016
Rossi, D. J., Bryder, D., Zahn, J. M., Ahlenius, H., Sonu, R., Wagers, A. J., et al. (2005). Cell Intrinsic Alterations Underlie Hematopoietic Stem Cell Aging. Proc. Natl. Acad. Sci. U.S.A. 102 (26), 9194–9199. doi:10.1073/pnas.0503280102
Rovida, E., Paccagnini, A., Del Rosso, M., Peschon, J., and Dello Sbarba, P. (2001). TNF-α-Converting Enzyme Cleaves the Macrophage Colony-Stimulating Factor Receptor in Macrophages Undergoing Activation. J. Immunol. 166 (3), 1583–1589. doi:10.4049/jimmunol.166.3.1583
Ryder, M., Gild, M., Hohl, T. M., Pamer, E., Knauf, J., Ghossein, R., et al. (2013). Genetic and Pharmacological Targeting of CSF-1/CSF-1R Inhibits Tumor-Associated Macrophages and Impairs BRAF-Induced Thyroid Cancer Progression. PLOS ONE 8 (1), e54302. doi:10.1371/journal.pone.0054302
Sanford, D. E., Belt, B. A., Panni, R. Z., Mayer, A., Deshpande, A. D., Carpenter, D., et al. (2013). Inflammatory Monocyte Mobilization Decreases Patient Survival in Pancreatic Cancer: a Role for Targeting the CCL2/CCR2 axis. Clin. Cancer Res. 19 (13), 3404–3415. doi:10.1158/1078-0432.Ccr-13-0525
Shand, F. H. W., Ueha, S., Otsuji, M., Koid, S. S., Shichino, S., Tsukui, T., et al. (2014). Tracking of Intertissue Migration Reveals the Origins of Tumor-Infiltrating Monocytes. Proc. Natl. Acad. Sci. U.S.A. 111 (21), 7771–7776. doi:10.1073/pnas.1402914111
Shi, C., and Pamer, E. G. (2011). Monocyte Recruitment during Infection and Inflammation. Nat. Rev. Immunol. 11 (11), 762–774. doi:10.1038/nri3070
Singh, P., Goode, T., Dean, A., Awad, S. S., and Darlington, G. J. (2011). Elevated Interferon Gamma Signaling Contributes to Impaired Regeneration in the Aged Liver. The Journals Gerontol. Ser. A 66A (9), 944–956. doi:10.1093/gerona/glr094
Sinha, S. K., Miikeda, A., Fouladian, Z., Mehrabian, M., Edillor, C., Shih, D., et al. (2021). Local M-CSF (Macrophage Colony-Stimulating Factor) Expression Regulates Macrophage Proliferation and Apoptosis in Atherosclerosis. Atvb 41 (1), 220–233. doi:10.1161/atvbaha.120.315255
Stanley, E. R., and Chitu, V. (2014). CSF-1 Receptor Signaling in Myeloid Cells. Cold Spring Harbor Perspect. Biol. 6 (6), a021857. doi:10.1101/cshperspect.a021857
Strohacker, K., Breslin, W. L., Carpenter, K. C., and McFarlin, B. K. (2012). Aged Mice Have Increased Inflammatory Monocyte Concentration and Altered Expression of Cell-Surface Functional Receptors. J. Biosci. 37 (1), 55–62. doi:10.1007/s12038-011-9169-z
Suehiro, A., Imagawa, T., Hosokawa, H., Suehiro, M., Ohe, Y., and Kakishita, E. (1999). Age Related Elevation of Serum Macrophage colony Stimulating Factor (M-CSF) Level. Arch. Gerontol. Geriatr. 29 (1), 13–20. doi:10.1016/S0167-4943(99)00015-1
Thevaranjan, N., Puchta, A., Schulz, C., Naidoo, A., Szamosi, J. C., Verschoor, C. P., et al. (2017). Age-Associated Microbial Dysbiosis Promotes Intestinal Permeability, Systemic Inflammation, and Macrophage Dysfunction. Cell Host Microbe 21 (4), 455–466.e4. doi:10.1016/j.chom.2017.03.002
Tomay, F., Wells, K., Duong, L., Tsu, J. W., Dye, D. E., Radley-Crabb, H. G., et al. (2018). Aged Neutrophils Accumulate in Lymphoid Tissues from Healthy Elderly Mice and Infiltrate T- and B-Cell Zones. Immunol. Cel Biol. 96 (8), 831–840. doi:10.1111/imcb.12046
Van Overmeire, E., Stijlemans, B., Heymann, F., Keirsse, J., Morias, Y., Elkrim, Y., et al. (2015). M-CSF and GM-CSF Receptor Signaling Differentially Regulate Monocyte Maturation and Macrophage Polarization in the Tumor Microenvironment. Cancer Res. 76, 35–42. doi:10.1158/0008-5472.CAN-15-0869
Wang, H., Shao, Q., Sun, J., Ma, C., Gao, W., Wang, Q., et al. (2016). Interactions between colon Cancer Cells and Tumor-Infiltrated Macrophages Depending on Cancer Cell-Derived colony Stimulating Factor 1. OncoImmunology 5 (4), e1122157. doi:10.1080/2162402X.2015.1122157
Yagi, T., Baba, Y., Okadome, K., Kiyozumi, Y., Hiyoshi, Y., Ishimoto, T., et al. (2019). Tumour-associated Macrophages Are Associated with Poor Prognosis and Programmed Death Ligand 1 Expression in Oesophageal Cancer. Eur. J. Cancer 111, 38–49. doi:10.1016/j.ejca.2019.01.018
Yang, M., Li, Z., Ren, M., Li, S., Zhang, L., Zhang, X., et al. (2018). Stromal Infiltration of Tumor-Associated Macrophages Conferring Poor Prognosis of Patients with Basal-like Breast Carcinoma. J. Cancer 9 (13), 2308–2316. doi:10.7150/jca.25155
Yeung, Y.-G., and Stanley, E. R. (2003). Proteomic Approaches to the Analysis of Early Events in Colony-stimulating Factor-1 Signal Transduction. Mol. Cell Proteomics 2 (11), 1143–1155. doi:10.1074/mcp.R300009-MCP200
Yu, W., Chen, J., Xiong, Y., Pixley, F. J., Dai, X.-M., Yeung, Y.-G., et al. (2008). CSF-1 Receptor Structure/function in MacCsf1r-/- Macrophages: Regulation of Proliferation, Differentiation, and Morphology. J. Leukoc. Biol. 84 (3), 852–863. doi:10.1189/jlb.0308171
Yuan, R., Tsaih, S.-W., Petkova, S. B., De Evsikova, C. M., Xing, S., Marion, M. A., et al. (2009). Aging in Inbred Strains of Mice: Study Design and Interim Report on Median Lifespans and Circulating IGF1 Levels. Aging Cell 8 (3), 277–287. doi:10.1111/j.1474-9726.2009.00478.x
Zhang, Y., Cheng, S., Zhang, M., Zhen, L., Pang, D., Zhang, Q., et al. (2013). High-Infiltration of Tumor-Associated Macrophages Predicts Unfavorable Clinical Outcome for Node-Negative Breast Cancer. PLOS ONE 8 (9), e76147. doi:10.1371/journal.pone.0076147
Zhang, L., Li, Z., Skrzypczynska, K. M., Fang, Q., Zhang, W., O’Brien, S. A., et al. (2020). Single-Cell Analyses Inform Mechanisms of Myeloid-Targeted Therapies in Colon Cancer. Cell 181 (2), 442–459.e29. doi:10.1016/j.cell.2020.03.048
Zhu, Y., Knolhoff, B. L., Meyer, M. A., Nywening, T. M., West, B. L., Luo, J., et al. (2014). CSF1/CSF1R Blockade Reprograms Tumor-Infiltrating Macrophages and Improves Response to T-Cell Checkpoint Immunotherapy in Pancreatic Cancer Models. Cancer Res. 74 (18), 5057–5069. doi:10.1158/0008-5472.CAN-13-3723
Keywords: macrophage, monocyte, CSF-1R, aging, cancer, tumor-associated macrophages
Citation: Duong L, Pixley FJ, Nelson DJ and Jackaman C (2022) Aging Leads to Increased Monocytes and Macrophages With Altered CSF-1 Receptor Expression and Earlier Tumor-Associated Macrophage Expansion in Murine Mesothelioma. Front. Aging 3:848925. doi: 10.3389/fragi.2022.848925
Received: 05 January 2022; Accepted: 12 April 2022;
Published: 27 April 2022.
Edited by:
Laura Haynes, University of Connecticut, United StatesReviewed by:
Julia Kzhyshkowska, Heidelberg University, GermanyCopyright © 2022 Duong, Pixley, Nelson and Jackaman. This is an open-access article distributed under the terms of the Creative Commons Attribution License (CC BY). The use, distribution or reproduction in other forums is permitted, provided the original author(s) and the copyright owner(s) are credited and that the original publication in this journal is cited, in accordance with accepted academic practice. No use, distribution or reproduction is permitted which does not comply with these terms.
*Correspondence: Connie Jackaman, Y29ubmllLmphY2thbWFuQGN1cnRpbi5lZHUuYXU=
Disclaimer: All claims expressed in this article are solely those of the authors and do not necessarily represent those of their affiliated organizations, or those of the publisher, the editors and the reviewers. Any product that may be evaluated in this article or claim that may be made by its manufacturer is not guaranteed or endorsed by the publisher.
Research integrity at Frontiers
Learn more about the work of our research integrity team to safeguard the quality of each article we publish.