- 1Population Health and Host-Pathogen Interactions Programs, Texas Biomedical Research Institute, San Antonio, TX, United States
- 2School of Public Health in Brownsville, University of Texas Health Houston, Brownsville, TX, United States
- 3South Texas Diabetes and Obesity Institute, University of Texas Rio Grande Valley, Edinburg, TX, United States
- 4Department of Cell Systems and Anatomy, UT-Health San Antonio, San Antonio, TX, United States
- 5Soutwest National Primate Research Center, Texas Biomedical Research Institute, San Antonio, TX, United States
Respiratory infections are one of the top causes of death in the elderly population, displaying susceptibility factors with increasing age that are potentially amenable to interventions. We posit that with increasing age there are predictable tissue-specific changes that prevent the immune system from working effectively in the lung. This mini-review highlights recent evidence for altered local tissue environment factors as we age focusing on increased tissue oxidative stress with associated immune cell changes, likely driven by the byproducts of age-associated inflammatory disease. Potential intervention points are presented.
General Introduction
The elderly population will double to 2 billion by 2050 (Publications, 2019). Age-associated cellular decline begins to be notable in our 40s and accelerates in our 60s (Jones et al., 2002; Massudi et al., 2012; López-Otín et al., 2013; Schneider et al., 2021). While aging represents a complex progression involving many physiological and physical changes in metabolism and endurance, the field of aging is advancing steadily, with greater understanding of the changes in cellular processes, and the critical role that tissue-specific health plays in how we age. However, the molecular events driving age-dependent physiological and physical decline and the molecular thresholds at the tissue and cellular levels that determine the point(s) of no return or so-called tipping point(s), resulting in non-recoverable tissue or systemic dysfunction are largely unknown. Here, we will highlight how and when some aspects of age-associated cellular decline occur in the pulmonary space, a critical organ that interfaces directly with the environment and is prone to infections. Such information can help develop therapeutic strategies to enhance healthy pulmonary aging in a timely manner to mitigate or delay deleterious events.
The Lung Alveolar Environment During Aging
As we age, there are changes in the physical environment and mechanical function of the lung that influence breathing and contribute to the increased susceptibility of the elderly to many infections (Schneider et al., 2021; Dyer, 2012; Vaz Fragoso and Lee, 2012; Haynes, 2020). Aging-related changes in the respiratory system generally include structural changes in the thoracic cage and lung parenchyma, abnormal lung function including ventilation and gas exchange abnormalities, decreased exercise capacity, airway nerve impairment, and reduced respiratory muscle strength and elasticity; each of which affect the cough reflex, sneezing or breathing (Figure 1) (Schneider et al., 2021). These progressive changes cause a decline in lung function and impaired immunological responses (Janssens et al., 1999; Lowery et al., 2013; Sharma and Goodwin, 2006; van Oostrom et al., 2018; Chalise, 2019; Thomas et al., 2019; Cho and Stout-Delgado, 2020; Haynes, 2020). To avoid cumulative damage, lung-resident cells rely on a robust homeostatic balance of stress response and associated inflammatory pathways; however, there is likely a tipping point (Figure 2), where age-associated changes finally overwhelm these control mechanisms leading to an increasing oxidative environment and irreversible damage (Moliva et al., 2014; Schöttker et al., 2015; Schneider et al., 2021). These factors combined with reduced lung-specific homeostatic immune activities facilitate entry of pathogens into the upper and lower respiratory tract leading to an increased propensity for infection (Vaz Fragoso and Lee, 2012; Haynes, 2020).
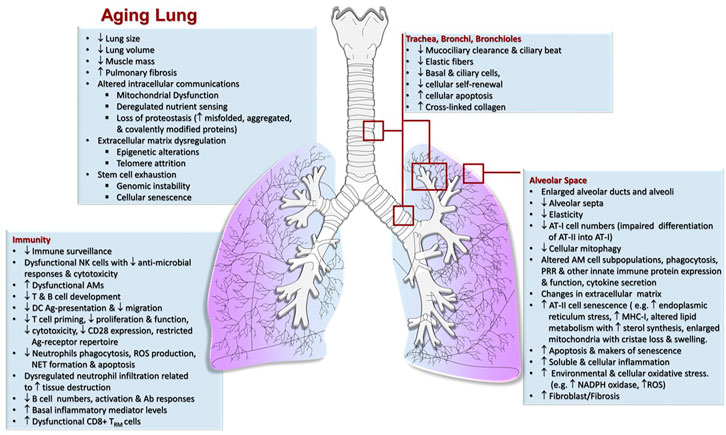
FIGURE 1. Characteristics of the Aging lung. Mechanical, physiological and immunological (increased inflammation/immunosenescence) changes in the lung that take place in elderly individuals increasing the risk of airway clearance failure and susceptibility to respiratory infections.
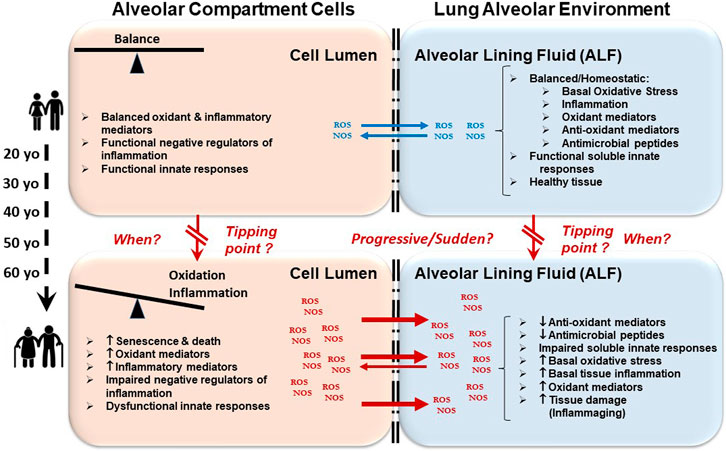
FIGURE 2. Oxidative and inflammatory environment in the lung leads to immunological impartment and susceptibility to respiratory infections. As we age, cellular senescence occurs. There are also alterations in cellular metabolic processes involving energy production and consumption among others. These events lead to an accumulation of oxidative stressors (ROS/NOS) in the lumen of alveolar compartment cells (left boxes) causing increased cellular inflammation that cannot be efficiently regulated. Subsequently, this accumulation of oxidative stressors outflows into the alveolar environment (e.g., upon cell death) (right boxes). This outflow causes lung tissue inflammation and damage, and also drives the oxidation and impairment of lung soluble and cellular immunomodulators. Altogether, these events contribute to the elderly becoming susceptible to acute and chronic respiratory infections and diseases.
The Lung Mucosa
The alveolus is lined with lung mucosa, composed of a surfactant lipid layer and an aqueous-hypophase called alveolar lining fluid (ALF) (Torrelles and Schlesinger, 2017). ALF contains innate soluble components with the primary role of maintaining proper pulmonary function (Notter, 2000; Fronius et al., 2012; Torrelles and Schlesinger, 2017). Our studies and others indicate that lung tissue in the elderly (in humans and mice) has a high basal inflammation and oxidative stress that leads to dysfunction of critical innate soluble and cellular components that could drive host susceptibility to respiratory infections and diseases [e.g., influenza, pneumonia, tuberculosis (TB)] (Toapanta and Ross, 2009; Shivshankar et al., 2011; Canan et al., 2014; Moliva et al., 2014; Piergallini and Turner, 2018a; Keilich et al., 2019; Bulut et al., 2020; Harpur et al., 2021). Thus, defining when and how these changes occur at the cellular and molecular level is critical to understand age-associated lung-specific pathologies and aging in general. Published studies demonstrate that the elderly have basal inflammation (e.g., elevated levels of TNF-α, IL-6, IL-1β, and IL-12), as well as increased protein oxidation with elevated levels of proteins with carbonyl and nitrotyrosine residues indicative of oxidation by reactive oxygen (ROS) and nitrogen (RNS) species, respectively, and reduced protein function in the lung (Moliva et al., 2014; Moliva et al., 2019). Indeed, studies focused on studying the lung mucosa composition in the elderly showed increased levels of innate surfactant proteins and components of the complement system, but with diminished function (Moliva et al., 2014). Dysfunction of innate soluble components of the lung mucosa is linked directly to high levels of oxidative stress [(e.g., elevated levels of ROS/RNS and myeloperoxidase (MPO)], which impair their function, and oxidizes critical surfactant lipids involved in keeping the lung alveolar space integrity and functionality (Moliva et al., 2014).
Studies addressing the impact of the human lung mucosa from the elderly on microbial pathogenesis are very limited. Upon reaching the alveolar space, respiratory pathogens come in close contact with the lung mucosa before and after their encounter with host alveolar compartment cells [e.g., type 1 and 2 epithelial cells (ATs) and alveolar macrophages (AMs)]. Our previous studies show that homeostatic innate soluble components of the ALF can quickly alter the cell envelope surface of pathogens upon contact, influencing subsequent pathogen-host cell interactions and infection outcomes (Arcos et al., 2011; Arcos et al., 2015; Arcos et al., 2017; Hill et al., 2017; Scordo et al., 2017; Scordo et al., 2019; Haynes, 2020). Alterations of a pathogen cell envelope is mainly due to homeostatic hydrolytic activities (hydrolases), primarily responsible for maintaining lung health, that modify pathogens and influence their subsequent interactions with host cells (Arcos et al., 2011; Hill et al., 2017; Torrelles and Schlesinger, 2017). In this context we have demonstrated that ALF from healthy 60 + year old individuals is dysfunctional, with loss of homeostatic hydrolytic capacity and impaired innate soluble responses linked to high local oxidative stress, compared to healthy 18- to 45-year-old individuals (Moliva et al., 2014). Importantly, a pathogen such as Mycobacterium tuberculosis exposed to ALF from the elderly demonstrates increased virulence in vitro and in vivo indicating that impaired innate responses in the lung may play a critical role in susceptibility to respiratory infection as we age (Moliva et al., 2019). In this context, two major cell populations in the alveolar space are AMs and ATs. Our studies indicate that after exposure to ALF from the elderly, M. tuberculosis doubles its intracellular growth rate in AMs, further indicating the impact of ALF dysfunction as we age (Moliva et al., 2019). Thus, efforts to mitigate oxidative stress in the lungs prior to a so-called tipping point(s) may reduce inflammation and aid in functional recovery of important innate immune-modulators against respiratory pathogens (Piergallini and Turner, 2018b; Iddir et al., 2020; Michaeloudes et al., 2020).
Evidence for a higher oxidative environment of the elderly lung is also supported by proteomics studies in other tissues and in-vitro studies with purified mitochondria from old mice (Moliva et al., 2014; Stauch et al., 2015; Ingram and Chakrabarti, 2016; Gómez-Serrano et al., 2018; Moliva et al., 2019; Ubaida-Mohien et al., 2019; Yang et al., 2019). However, the mechanism behind elevated oxidative stress in the lungs as we age is still not completely understood. Based on studies in other tissues, the oxidative pulmonary environment may be attributed in part to mitochondrial dysfunction. Mitochondria are primarily responsible for producing ATP (Anderson et al., 2019) and are found in greater numbers in tissues and organs where energy needs are high (e.g., muscle, heart, brain, liver and kidney). However, few studies have focused on the lung despite its direct exposure to oxygen, large consumption of energy (Liu and Summer, 2019), and high risk of dysfunction and disease in older age as mitochondrial function declines (Short et al., 2005; Kim et al., 2015; Haas, 2019; Sekhar M.D. et al., 2019). Thus, interventions to address the management of mitochondria-induced oxidative stressors (e.g. reactive oxygen and nitrogen oxidative species, ROS, NOS) in the lung tissue should mitigate lung oxidative stress and inflammation, and lead to improved resistance of the elderly to respiratory infections.
Alveolar Macrophages During Aging
Aging is associated with a weakening of immune function, called cellular immunosenescence. In addition to this process there is a baseline level of dysfunctional inflammation in old age termed inflammaging (Franceschi et al., 2000; Moliva et al., 2014; Frasca and Blomberg, 2016; Fulop et al., 2017; Lafuse et al., 2019; Moliva et al., 2019). The relationship between inflammaging and dysfunctional innate immunity is understood poorly, especially in the lung. These changes are thought to be critical to understanding why the elderly are at an increased risk of developing and succumbing to malignancies, autoimmunity (Linton and Dorshkind, 2004; Pawelec et al., 2010), and infectious diseases such as TB (Piergallini and Turner, 2018b; Lafuse et al., 2019; Moliva et al., 2019).
AMs live in a unique tissue environment and we are only now gaining insight into the local environmental factors that impact AM phenotype and function (Guth et al., 2009; Davies and Taylor, 2015; Allard et al., 2018; Papp et al., 2018; Hoeksema and Glass, 2019; Lafuse et al., 2019). AM responses enable us to maintain health (clearance of invaders) and at the same time, must dampen pro-inflammatory responses to maintain alveolar structure to enable gas exchange. Our failure to understand the cellular and molecular events underlying AM development and biology creates a critical barrier in our attempts to develop new treatment strategies that target the lung. AMs, which constitute >95% of the cells recovered by bronchoalveolar lavage in healthy individuals, are thought to play a central role in lung inflammaging due to their unique regulation by molecular factors in the lung environment.
Indeed, our studies and others have demonstrated an AM inflammatory signature in the elderly (Canan et al., 2014; Wong et al., 2017; Lafuse et al., 2019; Duong et al., 2021). Specifically, AMs from old mice express higher mRNA levels of the macrophage migration inhibitory factor (MIF), TNF-α, IFN-β, IL-10, IL-12p40, and CCL2 than young mice, and old mice also contain higher levels of MIF, IL-1β, IFN-β, and CCL2 in their alveolar lining fluid. Further, old mice have two distinct AM subpopulations, a major CD11c+ CD11b− population and a minor CD11c+ CD11b+ population; the latter is increased significantly in old mice (∼4-fold). Expression of pattern recognition receptors, antigen presentation molecules, and activation markers such as CD206, TLR2, CD16/CD32, MHC class II, and CD86 is higher in CD11c+ CD11b+ AMs, and these cells express monocytic markers such as Ly6C, CX3CR1, and CD115, suggesting monocytic origin. Old mouse purified CD11c+ CD11b+ AMs phagocytose significantly more M. tuberculosis, and express higher RNA levels of genes favoring M. tuberculosis intracellular growth. Thus, the lungs of old mice contain two distinct AM subpopulations, a defined resident subpopulation and an immigrating CD11c+ CD11b+ AM subpopulation expressing monocytic markers, a unique inflammatory signature, with enhanced M. tuberculosis phagocytosis capacity and M. tuberculosis survival. Our findings suggest that this CD11c+ CD11b+ AM subpopulation could be targeted by respiratory pathogens as a niche for replication and survival within the lung of the elderly population driving their susceptibility to respiratory infections.
Nuclear receptors (NRs) function as cellular transcription factors enabling macrophages and other myeloid cells to sense their local environment and shape their immune and metabolic responses (Murphy and Crean, 2015; Leopold Wager et al., 2019a; Bichiou et al., 2021). Drugs targeting NRs represent 16% of all drugs approved for sale in the United States in 2019, demonstrating their feasibility as host-directed therapies (HDTs) for disease management (Ricote and Glass, 2007; Santos et al., 2017; Boudreaux et al., 2019; Zhang et al., 2020). NRs are increasingly appreciated for their importance during aging (Speeckaert et al., 2014; Paillasse and de Medina, 2015; Cisneros and García-Aguirre, 2020; Xu et al., 2020). Most past studies have focused on specific NRs in cell lines or murine cells, thus their function and interactions within primary cells and the lung environment are largely unexplored. Studies using human and murine macrophages (Zollner and Trauner, 2009; Dhiman et al., 2018; Leopold Wager et al., 2019a; Leopold Wager et al., 2019b; Ning et al., 2019; Zhao et al., 2019; Zhao et al., 2020) in cancer (Zhou et al., 2014; Dhiman et al., 2018; Zhao et al., 2019), auto-immunity (Zollner and Trauner, 2009; Lee et al., 2011; Ning et al., 2019; Olson et al., 2020) and infectious diseases (Renga et al., 2012; Leopold Wager et al., 2019a; Leopold Wager et al., 2019b) have highlighted the importance of NRs in maintaining tissue homeostasis (Nagy et al., 2013; Zhao et al., 2020; Alatshan and Benkő, 2021). Several NRs are postulated to dampen pro-inflammatory networks (De Bosscher et al., 2006; Huang and Glass, 2010; Mandard and Patsouris, 2013; Li et al., 2015; Wang et al., 2017; Duez and Pourcet, 2021; Pulakazhi Venu et al., 2021; Vuttaradhi et al., 2021). Thus, NR dysfunction during aging may remove the brakes to pro-inflammatory and oxidative stress pathways with associated altered mitochondrial function as discussed above. Since NRs regulate metabolism and inflammation in a tissue-specific manner, studying NR expression and activity as well as their involvement in altering lung macrophage lipid metabolism, cellular energy homeostasis and protective immune responses during aging could help define new biological pathways that play important roles in host responses in the lung as we age.
One particularly important NR regulating AM development and function is peroxisome proliferator-activated receptor gamma (PPARγ), where our recent work has identified novel targetable PPARγ effectors that regulate inflammation and cell death pathways (Arnett et al., 2018). PPARγ endogenous ligands include eicosanoids, which are critical determinants of cellular metabolism and immune responses that exert both anti- and pro-inflammatory actions (Nagy et al., 2012; Dennis and Norris, 2015; Arnett et al., 2018; Bougarne et al., 2018), and likely play important roles in controlling cellular aging. Indeed, PPARs regulate gene expression through multiple mechanisms, including heterodimerizing with the retinoid X receptor (RXR), and binding to PPAR response elements in promoters. PPARs also trans-repress NF-κB and other transcription factors to negatively regulate pro-inflammatory pathways and stabilize the NR co-repressor NCoR, to repress gene expression (Ricote and Glass, 2007). Using macrophage-specific PPARγ knockout mice (Odegaard et al., 2007), we showed that PPARγ specifically in lung macrophages is important for controlling bacterial growth (e.g. M. tuberculosis) and limiting inflammation in vivo (Guirado et al., 2018). These findings highlight a critical role of PPARγ in controlling inflammation, yet the impact of aging on PPARγ and expression and activity of other NRs is still unclear.
Based on our knowledge in the field (Oren et al., 1963; O'Neill et al., 2016; Viola et al., 2019), several NRs are postulated to dampen pro-inflammatory networks (De Bosscher et al., 2006; Huang and Glass, 2010; Mandard and Patsouris, 2013; Papi et al., 2014; Leopold Wager et al., 2019a; Klepsch et al., 2019; Duez and Pourcet, 2021). Thus, it is plausible that NR expression and activity are altered across the continuum of age and NRs become dysfunctional; where age-related NR changes can induce aberrant lung macrophage inflammation, oxidative stress, lipid metabolism, and energy programs. Thus, modulation of NR activity with agonists or antagonists could restore healthy immune homeostasis to slow or reverse the lung health issues associated with aging, allowing for more robust protection against lung infections and inflammatory diseases.
Other Cellular Changes in the Alveolar Compartment During Aging
As we age, alveolar compartment cells become senescent due to the shortening of telomeres and the accumulation of DNA lesions driven by free oxidative radicals generated in the lung environment. AMs, interstitial macrophages, neutrophils and dendritic cells lose their microbicidal and antigen presenting capacities (Wenisch et al., 2000; Uyemura et al., 2002; Stout et al., 2005). Notably, neutrophils exhibit an alteration of their homeostatic functions that may contribute to the susceptibility to lung infections of the elderly (Barkaway et al., 2021). These include decreased phagocytosis, reduced production of ROS and extracellular traps (NETS) formation (Hazeldine et al., 2014), as well as reduced apoptosis and chemotaxis accompanied with dysregulated migration (Niwa et al., 1989; Fulop et al., 2004). Natural killer (NK) cells, which also constitute a first line of defense against virus-infected and malignant cells (Hazeldine and Lord, 2013) change with age. In particular, NK cells become dysfunctional for secretion of regulatory and anti-microbial responses, cytotoxic capacity, and elimination of transformed and senescent cells (Hazeldine and Lord, 2013). These alterations have implications for age-associated immune responses in the alveolar space of elderly individuals. Indeed, several studies implicate NK dysfunction to increased cases of TB reactivation in the elderly, as well as poor resolution of inflammatory disorders and increased incidence of respiratory infections (Hazeldine and Lord, 2013).
With regard to adaptive immunity, the number of B cells decrease during aging leading to reduced immunoglobulin diversity and affinity (Han et al., 2003; McElhaney et al., 2016; Frasca et al., 2020). Age associated inflammation is also known to impact B cell function (Hagen and Derudder, 2020), impacting germinal center formation and cytokine mediated signaling. Systemic T cell proliferation is impaired, repertoire diversity decreases, homeostasis is modified, intracellular signal transduction capability is dysregulated, and T cells produce less cytokines and are less cytotoxic (Fülöp et al., 1999; Voehringer et al., 2002; Ouyang et al., 2003; Naylor et al., 2005). Indeed, CD4 and CD8 T cells show age-related changes in function (Maue et al., 2009; Lorenzo et al., 2018; Haynes, 2020), with changes in CD4 T cells during aging (Haynes and Swain, 2012; Lee et al., 2012) influencing both CD8 T function and B cell antibody production in response to influenza (Maue et al., 2009; Toapanta and Ross, 2009; Lorenzo et al., 2018). For CD4 T cells, intrinsic defects promote delayed adaptive immune responses and recovery from influenza infection, but adoptive transfer studies have also shown that extrinsic factors also contribute to poor T cell function in the lung. This eludes to the influence of the myriad of changes in the alveolar space, such as oxidation of innate molecules or altered AM function, with increasing age that have wide reaching effect including an influence on adaptive immune function. Significant to lung function in older age, memory T cell populations start to lose their function, most notable in tissue specific resident memory CD8 T cells (CD8 TRM cells) (Schenkel et al., 2014; McMaster et al., 2015; Goplen et al., 2020) (Hakim and Gress, 2007). CD8 TRM cells from old mice have been shown to drive lung fibrosis following influenza, SARS-CoV-2 and chikungunya infections, potentially via TGFβ, likely delaying recovering of lung function and promoting conditions for secondary infection and other age associated lung disorders (Uhrlaub et al., 2016; Goplen et al., 2020; Goplen et al., 2021; Shenoy and Mizgerd, 2021). Interestingly, within the lung environment, we have also shown that resident effector CD8+ T cells demonstrate a robust innate-like response, driven by an IL-12 dependent but antigen-independent secretion of IFNγ (Vesosky et al., 2006a; Vesosky et al., 2006b; Rottinghaus et al., 2009; Vesosky et al., 2009). Such CD8 effector cells were shown to play a role in early innate control of M. tuberculosis infection in old mice (Vesosky et al., 2006a; Rottinghaus et al., 2009; Vesosky et al., 2009). The relevance of such cells is not clear, but it is thought to be a compensatory mechanism to establish an additional defense barrier against respiratory pathogens in the elderly (Piergallini and Turner, 2018b), but could equally be one that interferes with optimal antigen specific immunity or perhaps contributes to lung tissue damage that has long term consequences on pulmonary function with increasing age. The study of adaptive immune cells specifically in the lung during aging, in the context of chronic disease such as chronic obstructive pulmonary disease or asthma, is an as yet understudied area (Lee et al., 2012) but lung tissue specificity and age-associated changes likely also play a contributing role to the ability of T and B cells to exert their immune control as we age.
Discussion
Aging is accompanied by a decline in immune efficacy resulting in increased vulnerability to infections. There is increasing evidence that immune dysfunction linked to exacerbated inflammatory reactivity is associated with failure in organ systems (ex: lung, brain, skeletal muscle) leading to functional decline, morbidity and mortality. Several of these syndromes and diseases such as frailty, dementia, cardiovascular disease and cancer, result in elderly persons being dependent on others for daily living. Immune decline occurs at different ages, and can be influenced by host genetics as well as external “perturbations” such as metabolic status (e.g., dysglycemia) and lifetime exposure to pathogens. The mechanisms underlying these alterations are poorly understood; where it is unclear which changes are primary consequences of aging, and which are compensatory. Furthermore, it is unclear if inflammaging begins in the periphery and spills over into organs such as the lung or vice versa. Answering these questions is critical for the development and implementation of therapeutics that delay, prevent or restore an individual’s age-related decrease in immunity, including its associated failure of organ systems. Elucidation of these interactions is best accomplished through longitudinal studies spanning decades, which are logistically challenging and costly. Alternatives are cross-sectional studies in individuals of all ages, or short longitudinal assessments of individuals challenged by an “insult” to their immune system that accelerates aging of their immune cells. These studies in humans present interpretation challenges due to high levels of variability in factors of interest that may underlie responsivity to challenges, for example patients with undiagnosed dysglycemia which is the most prevalent metabolic perturbation in the elderly (Chia et al., 2018; Scordo et al., 2021).
We postulate that there is a gradual increase in inflammation and altered metabolism across the aging continuum, potentially initiated in the pulmonary space, which is directly exposed to environmental insults, that culminates in a cascade of organ and systemic dysfunctions, reaching a peak or tipping point(s) where inflammation and oxidation can no longer be reversed by interventions (Figure 2). Modulating cellular oxidative stress-related factors that occur during inflammaging in the alveolus prior to the tipping point(s) may allow for the restoration and maintenance of healthy immune and metabolic homeostasis during aging.
Author Contributions
All authors listed have made a substantial, direct, and intellectual contribution to the work and approved it for publication.
Funding
This work was supported by a National Institutes of Health-National Institute on Aging (NIA) program project grant JT (P01-AG051428).
Conflict of Interest
The authors declare that the research was conducted in the absence of any commercial or financial relationships that could be construed as a potential conflict of interest.
Publisher’s Note
All claims expressed in this article are solely those of the authors and do not necessarily represent those of their affiliated organizations, or those of the publisher, the editors, and the reviewers. Any product that may be evaluated in this article, or claim that may be made by its manufacturer, is not guaranteed or endorsed by the publisher.
Acknowledgments
We would like to thank our laboratory members for their dedication to research on aging.
References
Alatshan, A., and Benkő, S. (2021). Nuclear Receptors as Multiple Regulators of NLRP3 Inflammasome Function. Front. Immunol. 12, 630569. doi:10.3389/fimmu.2021.630569
Allard, B., Panariti, A., and Martin, J. G. (2018). Alveolar Macrophages in the Resolution of Inflammation, Tissue Repair, and Tolerance to Infection. Front. Immunol. 9, 1777. doi:10.3389/fimmu.2018.01777
Anderson, A. J., Jackson, T. D., Stroud, D. A., and Stojanovski, D. (2019). Mitochondria-hubs for Regulating Cellular Biochemistry: Emerging Concepts and Networks. Open Biol. 9 (8), 190126. doi:10.1098/rsob.190126
Arcos, J., Diangelo, L. E., Scordo, J. M., Sasindran, S. J., Moliva, J. I., Turner, J., et al. (2015). Lung Mucosa Lining Fluid Modification ofMycobacterium Tuberculosisto Reprogram Human Neutrophil Killing Mechanisms. J. Infect. Dis. 212 (6), 948–958. doi:10.1093/infdis/jiv146
Arcos, J., Sasindran, S. J., Fujiwara, N., Turner, J., Schlesinger, L. S., and Torrelles, J. B. (2011). Human Lung Hydrolases DelineateMycobacterium Tuberculosis-Macrophage Interactions and the Capacity to Control Infection. J.I. 187 (1), 372–381. doi:10.4049/jimmunol.1100823
Arcos, J., Sasindran, S. J., Moliva, J. I., Scordo, J. M., Sidiki, S., Guo, H., et al. (2017). Mycobacterium tuberculosis Cell wall Released Fragments by the Action of the Human Lung Mucosa Modulate Macrophages to Control Infection in an IL-10-dependent Manner. Mucosal Immunol. 10 (5), 1248–1258. doi:10.1038/mi.2016.115
Arnett, E., Weaver, A. M., Woodyard, K. C., Montoya, M. J., Li, M., Hoang, K. V., et al. (2018). PPARγ Is Critical for Mycobacterium tuberculosis Induction of Mcl-1 and Limitation of Human Macrophage Apoptosis. Plos Pathog. 14 (6), e1007100. doi:10.1371/journal.ppat.1007100
Barkaway, A., Rolas, L., Joulia, R., Bodkin, J., Lenn, T., Owen-Woods, C., et al. (2021). Age-related Changes in the Local Milieu of Inflamed Tissues Cause Aberrant Neutrophil Trafficking and Subsequent Remote Organ Damage. Immunity 54 (7), 1494–1510. doi:10.1016/j.immuni.2021.04.025
Bichiou, H., Bouabid, C., Rabhi, I., and Guizani-Tabbane, L. (2021). Transcription Factors Interplay Orchestrates the Immune-Metabolic Response of Leishmania Infected Macrophages. Front. Cel. Infect. Microbiol. 11, 660415. doi:10.3389/fcimb.2021.660415
Boudreaux, S. P., Duren, R. P., Call, S. G., Nguyen, L., Freire, P. R., Narayanan, P., et al. (2019). Drug Targeting of NR4A Nuclear Receptors for Treatment of Acute Myeloid Leukemia. Leukemia 33 (1), 52–63. doi:10.1038/s41375-018-0174-1
Bougarne, N., Weyers, B., Desmet, S. J., Deckers, J., Ray, D. W., Staels, B., et al. (2018). Molecular Actions of PPARα in Lipid Metabolism and Inflammation. Endocr. Rev. 39 (5), 760–802. doi:10.1210/er.2018-00064
Bulut, O., Kilic, G., Domínguez-Andrés, J., and Netea, M. G. (2020). Overcoming Immune Dysfunction in the Elderly: Trained Immunity as a Novel Approach. Int. Immunol. 32 (12), 741–753. doi:10.1093/intimm/dxaa052
Canan, C. H., Gokhale, N. S., Carruthers, B., Lafuse, W. P., Schlesinger, L. S., Torrelles, J. B., et al. (2014). Characterization of Lung Inflammation and its Impact on Macrophage Function in Aging. J. Leukoc. Biol. 96 (3), 473–480. doi:10.1189/jlb.4a0214-093rr
Chalise, H. N. (2019). Aging: Basic Concept. Am. J. Biomed. Sci. Res. 1 (1), 8–10. doi:10.34297/ajbsr.2019.01.000503
Chia, C. W., Egan, J. M., and Ferrucci, L. (2018). Age-Related Changes in Glucose Metabolism, Hyperglycemia, and Cardiovascular Risk. Circ. Res. 123 (7), 886–904. doi:10.1161/circresaha.118.312806
Cho, S. J., and Stout-Delgado, H. W. (2020). Aging and Lung Disease. Annu. Rev. Physiol. 82, 433–459. doi:10.1146/annurev-physiol-021119-034610
Cisneros, B., and García-Aguirre, I. (2020). Nuclear Protein export Pathway in Aging Therapeutics. Aging 12 (6), 4682–4684. doi:10.18632/aging.102948
Davies, L. C., and Taylor, P. R. (2015). Tissue‐resident Macrophages: Then and Now. Immunology 144 (4), 541–548. doi:10.1111/imm.12451
De Bosscher, K., Vanden Berghe, W., and Haegeman, G. (2006). Cross-talk between Nuclear Receptors and Nuclear Factor κB. Oncogene 25 (51), 6868–6886. doi:10.1038/sj.onc.1209935
Dennis, E. A., and Norris, P. C. (2015). Eicosanoid Storm in Infection and Inflammation. Nat. Rev. Immunol. 15 (8), 511–523. doi:10.1038/nri3859
Dhiman, V. K., Bolt, M. J., and White, K. P. (2018). Nuclear Receptors in Cancer - Uncovering New and Evolving Roles through Genomic Analysis. Nat. Rev. Genet. 19 (3), 160–174. doi:10.1038/nrg.2017.102
Duez, H., and Pourcet, B. (2021). Nuclear Receptors in the Control of the NLRP3 Inflammasome Pathway. Front. Endocrinol. 12, 630536. doi:10.3389/fendo.2021.630536
Duong, L., Radley, H., Lee, B., Dye, D., Pixley, F., Grounds, M., et al. (2021). Macrophage Function in the Elderly and Impact on Injury Repair and Cancer. Immun. Ageing 18 (1), 4. doi:10.1186/s12979-021-00215-2
Dyer, C. (2012). The Interaction of Ageing and Lung Disease. Chron. Respir. Dis. 9 (1), 63–67. doi:10.1177/1479972311433766
Franceschi, C., Bonafè, M., Valensin, S., Olivieri, F., De Luca, M., Ottaviani, E., et al. (2000). Inflamm-aging. An Evolutionary Perspective on Immunosenescence. Ann. N. Y Acad. Sci. 908, 244–254. doi:10.1111/j.1749-6632.2000.tb06651.x
Frasca, D., Blomberg, B. B., Garcia, D., Keilich, S. R., and Haynes, L. (2020). Age‐related Factors that Affect B Cell Responses to Vaccination in Mice and Humans. Immunol. Rev. 296 (1), 142–154. doi:10.1111/imr.12864
Frasca, D., and Blomberg, B. B. (2016). Inflammaging Decreases Adaptive and Innate Immune Responses in Mice and Humans. Biogerontology 17 (1), 7–19. doi:10.1007/s10522-015-9578-8
Fronius, M., Clauss, W. G., and Althaus, M. (2012). Why Do We Have to Move Fluid to Be Able to Breathe? Front. Physio. 3, 146. doi:10.3389/fphys.2012.00146
Fulop, T., Larbi, A., Dupuis, G., Le Page, A., Frost, E. H., Cohen, A. A., et al. (2017). Immunosenescence and Inflamm-Aging as Two Sides of the Same Coin: Friends or Foes? Front. Immunol. 8, 1960. doi:10.3389/fimmu.2017.01960
Fülöp, T., Gagné, D., Goulet, A.-C., Desgeorges, S., Lacombe, G., Arcand, M., et al. (1999). Age-related Impairment of P56lck and ZAP-70 Activities in Human T Lymphocytes Activated through the TcR/CD3 Complex. Exp. Gerontol. 34 (2), 197–216. doi:10.1016/s0531-5565(98)00061-8
Fulop, T., Larbi, A., Douziech, N., Fortin, C., Guérard, K.-P., Lesur, O., et al. (2004). Signal Transduction and Functional Changes in Neutrophils with Aging. Aging Cell 3 (4), 217–226. doi:10.1111/j.1474-9728.2004.00110.x
Gómez-Serrano, M., Camafeita, E., Loureiro, M., and Peral, B. (2018). Mitoproteomics: Tackling Mitochondrial Dysfunction in Human Disease. Oxid Med. Cel Longev 2018, 1435934. doi:10.1155/2018/1435934
Goplen, N. P., Wu, Y., Son, Y. M., Li, C., Wang, Z., Cheon, I. S., et al. (2020). Tissue-resident CD8+ T Cells Drive Age-Associated Chronic Lung Sequelae after Viral Pneumonia. Sci. Immunol. 5 (53). doi:10.1126/sciimmunol.abc4557
Goplen, N. P., Cheon, I. S., and Sun, J. (2021). Age-Related Dynamics of Lung-Resident Memory CD8+ T Cells in the Age of COVID-19. Front. Immunol. 12, 636118. doi:10.3389/fimmu.2021.636118
Guirado, E., Rajaram, M. V., Chawla, A., Daigle, J., La Perle, K. M., Arnett, E., et al. (2018). Deletion of PPARγ in Lung Macrophages Provides an Immunoprotective Response against M. tuberculosis Infection in Mice. Tuberculosis 111, 170–177. doi:10.1016/j.tube.2018.06.012
Guth, A. M., Janssen, W. J., Bosio, C. M., Crouch, E. C., Henson, P. M., and Dow, S. W. (2009). Lung Environment Determines Unique Phenotype of Alveolar Macrophages. Am. J. Physiology-Lung Cell Mol. Physiol. 296 (6), L936–L946. doi:10.1152/ajplung.90625.2008
Haas, R. H. (2019). Mitochondrial Dysfunction in Aging and Diseases of Aging. Biology (Basel) 8 (2). doi:10.3390/biology8020048
Hagen, M., and Derudder, E. (2020). Inflammation and the Alteration of B-Cell Physiology in Aging. Gerontology 66 (2), 105–113. doi:10.1159/000501963
Hakim, F. T., and Gress, R. E. (2007). Immunosenescence: Deficits in Adaptive Immunity in the Elderly. Tissue Antigens 70 (3), 179–189. doi:10.1111/j.1399-0039.2007.00891.x
Han, S., Yang, K., Ozen, Z., Peng, W., Marinova, E., Kelsoe, G., et al. (2003). Enhanced Differentiation of Splenic Plasma Cells but Diminished Long-Lived High-Affinity Bone Marrow Plasma Cells in Aged Mice. J. Immunol. 170 (3), 1267–1273. doi:10.4049/jimmunol.170.3.1267
Harpur, C. M., Le Page, M. A., and Tate, M. D. (2021). Too young to die? How aging affects cellular innate immune responses to influenza virus and disease severity. Virulence 12 (1), 1629–1646. doi:10.1080/21505594.2021.1939608
Haynes, L. (2020). Aging of the Immune System: Research Challenges to Enhance the Health Span of Older Adults. Front. Aging 1, 1–2. doi:10.3389/fragi.2020.602108
Haynes, L., and Swain, S. L. (2012). Aged-related Shifts in T Cell Homeostasis lead to Intrinsic T Cell Defects. Semin. Immunol. 24 (5), 350–355. doi:10.1016/j.smim.2012.04.001
Hazeldine, J., Harris, P., Chapple, I. L., Grant, M., Greenwood, H., Livesey, A., et al. (2014). Impaired Neutrophil Extracellular Trap Formation: a Novel Defect in the Innate Immune System of Aged Individuals. Aging Cell 13 (4), 690–698. doi:10.1111/acel.12222
Hazeldine, J., and Lord, J. M. (2013). The Impact of Ageing on Natural Killer Cell Function and Potential Consequences for Health in Older Adults. Ageing Res. Rev. 12 (4), 1069–1078. doi:10.1016/j.arr.2013.04.003
Hill, P. J., Scordo, J. M., Arcos, J., Kirkby, S. E., Wewers, M. D., Wozniak, D. J., et al. (2017). Modifications of Pseudomonas aeruginosa Cell Envelope in the Cystic Fibrosis Airway Alters Interactions with Immune Cells. Sci. Rep. 7 (1), 4761. doi:10.1038/s41598-017-05253-9
Hoeksema, M. A., and Glass, C. K. (2019). Nature and Nurture of Tissue-specific Macrophage Phenotypes. Atherosclerosis 281, 159–167. doi:10.1016/j.atherosclerosis.2018.10.005
Huang, W., and Glass, C. K. (2010). Nuclear Receptors and Inflammation Control: Molecular Mechanisms and Pathophysiological Relevance. Atvb 30 (8), 1542–1549. doi:10.1161/atvbaha.109.191189
Iddir, M., Brito, A., Dingeo, G., Fernandez Del Campo, S. S., Samouda, H., La Frano, M. R., et al. (2020). Strengthening the Immune System and Reducing Inflammation and Oxidative Stress through Diet and Nutrition: Considerations during the COVID-19 Crisis. Nutrients 12 (6). doi:10.3390/nu12061562
Ingram, T., and Chakrabarti, L. (2016). Proteomic Profiling of Mitochondria: what Does it Tell Us about the Ageing Brain? Aging 8 (12), 3161–3179. doi:10.18632/aging.101131
Janssens, J. P., Pache, J. C., and Nicod, L. P. (1999). Physiological Changes in Respiratory Function Associated with Ageing. Eur. Respir. J. 13 (1), 197–205. doi:10.1034/j.1399-3003.1999.13a36.x
Jones, D. P., Mody, V. C., Carlson, J. L., Lynn, M. J., and Sternberg, P. (2002). Redox Analysis of Human Plasma Allows Separation of Pro-oxidant Events of Aging from Decline in Antioxidant Defenses. Free Radic. Biol. Med. 33 (9), 1290–1300. doi:10.1016/s0891-5849(02)01040-7
Keilich, S. R., Bartley, J. M., and Haynes, L. (2019). Diminished Immune Responses with Aging Predispose Older Adults to Common and Uncommon Influenza Complications. Cell Immunol. 345, 103992. doi:10.1016/j.cellimm.2019.103992
Kim, S.-J., Cheresh, P., Jablonski, R., Williams, D., and Kamp, D. (2015). The Role of Mitochondrial DNA in Mediating Alveolar Epithelial Cell Apoptosis and Pulmonary Fibrosis. Ijms 16 (9), 21486–21519. doi:10.3390/ijms160921486
Klepsch, V., Moschen, A. R., Tilg, H., Baier, G., and Hermann-Kleiter, N. (2019). Nuclear Receptors Regulate Intestinal Inflammation in the Context of IBD. Front. Immunol. 10, 1070. doi:10.3389/fimmu.2019.01070
Lafuse, W. P., Rajaram, M. V. S., Wu, Q., Moliva, J. I., Torrelles, J. B., Turner, J., et al. (2019). Identification of an Increased Alveolar Macrophage Subpopulation in Old Mice that Displays Unique Inflammatory Characteristics and Is Permissive toMycobacterium tuberculosisInfection. J.I. 203 (8), 2252–2264. doi:10.4049/jimmunol.1900495
Lee, J. M., Lee, Y. K., Mamrosh, J. L., Busby, S. A., Griffin, P. R., Pathak, M. C., et al. (2011). A Nuclear-receptor-dependent Phosphatidylcholine Pathway with Antidiabetic Effects. Nature 474 (7352), 506–510. doi:10.1038/nature10111
Lee, N., Shin, M. S., and Kang, I. (2012). T-cell Biology in Aging, with a Focus on Lung Disease. Journals Gerontol. Ser. A: Biol. Sci. Med. Sci. 67A (3), 254–263. doi:10.1093/gerona/glr237
Leopold Wager, C. M., Arnett, E., and Schlesinger, L. S. (2019). Macrophage Nuclear Receptors: Emerging Key Players in Infectious Diseases. Plos Pathog. 15 (3), e1007585–e. doi:10.1371/journal.ppat.1007585
Leopold Wager, C. M., Arnett, E., and Schlesinger, L. S. (2019). Mycobacterium tuberculosis and Macrophage Nuclear Receptors: what We Do and Don't Know. Tuberculosis 116, S98–S106. doi:10.1016/j.tube.2019.04.016
Li, L., Liu, Y., Chen, H.-z., Li, F.-w., Wu, J.-f., Zhang, H.-k., et al. (2015). Impeding the Interaction between Nur77 and P38 Reduces LPS-Induced Inflammation. Nat. Chem. Biol. 11 (5), 339–346. doi:10.1038/nchembio.1788
Linton, P. J., and Dorshkind, K. (2004). Age-related Changes in Lymphocyte Development and Function. Nat. Immunol. 5 (2), 133–139. doi:10.1038/ni1033
Liu, G., and Summer, R. (2019). Cellular Metabolism in Lung Health and Disease. Annu. Rev. Physiol. 81, 403–428. doi:10.1146/annurev-physiol-020518-114640
López-Otín, C., Blasco, M. A., Partridge, L., Serrano, M., and Kroemer, G. (2013). The Hallmarks of Aging. Cell 153 (6), 1194–1217. doi:10.1016/j.cell.2013.05.039
Lorenzo, E. C., Bartley, J. M., and Haynes, L. (2018). The Impact of Aging on CD4+ T Cell Responses to Influenza Infection. Biogerontology 19 (6), 437–446. doi:10.1007/s10522-018-9754-8
Lowery, E. M., Brubaker, A. L., Kuhlmann, E., and Kovacs, E. J. (2013). The Aging Lung. Clin. Interv. Aging 8, 1489–1496. doi:10.2147/CIA.S51152
Mandard, S., and Patsouris, D. (2013). Nuclear Control of the Inflammatory Response in Mammals by Peroxisome Proliferator-Activated Receptors. PPAR Res. 2013, 613864. doi:10.1155/2013/613864
Massudi, H., Grant, R., Braidy, N., Guest, J., Farnsworth, B., and Guillemin, G. J. (2012). Age-associated Changes in Oxidative Stress and NAD+ Metabolism in Human Tissue. PLoS One 7 (7), e42357. doi:10.1371/journal.pone.0042357
Maue, A. C., Yager, E. J., Swain, S. L., Woodland, D. L., Blackman, M. A., and Haynes, L. (2009). T-cell Immunosenescence: Lessons Learned from Mouse Models of Aging. Trends Immunol. 30 (7), 301–305. doi:10.1016/j.it.2009.04.007
McElhaney, J. E., Kuchel, G. A., Zhou, X., Swain, S. L., and Haynes, L. (2016). T-cell Immunity to Influenza in Older Adults: A Pathophysiological Framework for Development of More Effective Vaccines. Front. Immunol. 7, 41. doi:10.3389/fimmu.2016.00041
McMaster, S. R., Wilson, J. J., Wang, H., and Kohlmeier, J. E. (2015). Airway-Resident Memory CD8 T Cells Provide Antigen-specific Protection against Respiratory Virus Challenge through Rapid IFN-γ Production. J.I. 195 (1), 203–209. doi:10.4049/jimmunol.1402975
Michaeloudes, C., Bhavsar, P. K., Mumby, S., Xu, B., Hui, C. K. M., Chung, K. F., et al. (2020). Role of Metabolic Reprogramming in Pulmonary Innate Immunity and its Impact on Lung Diseases. J. Innate Immun. 12 (1), 31–46. doi:10.1159/000504344
Moliva, J. I., Duncan, M. A., Olmo-Fontánez, A., Akhter, A., Arnett, E., Scordo, J. M., et al. (2019). The Lung Mucosa Environment in the Elderly Increases Host Susceptibility to Mycobacterium tuberculosis Infection. J. Infect. Dis. 220 (3), 514–523. doi:10.1093/infdis/jiz138
Moliva, J. I., Rajaram, M. V. S., Sidiki, S., Sasindran, S. J., Guirado, E., Pan, X. J., et al. (2014). Molecular Composition of the Alveolar Lining Fluid in the Aging Lung. Age 36 (3), 9633. doi:10.1007/s11357-014-9633-4
Murphy, E., and Crean, D. (2015). Molecular Interactions between NR4A Orphan Nuclear Receptors and NF-Κb Are Required for Appropriate Inflammatory Responses and Immune Cell Homeostasis. Biomolecules 5 (3), 1302–1318. doi:10.3390/biom5031302
Nagy, L., Szanto, A., Szatmari, I., and Széles, L. (2012). Nuclear Hormone Receptors Enable Macrophages and Dendritic Cells to Sense Their Lipid Environment and Shape Their Immune Response. Physiol. Rev. 92 (2), 739–789. doi:10.1152/physrev.00004.2011
Nagy, Z. S., Czimmerer, Z., Szanto, A., and Nagy, L. (2013). Pro-inflammatory Cytokines Negatively Regulate PPARγ Mediated Gene Expression in Both Human and Murine Macrophages via Multiple Mechanisms. Immunobiology 218 (11), 1336–1344. doi:10.1016/j.imbio.2013.06.011
Naylor, K., Li, G., Vallejo, A. N., Lee, W.-W., Koetz, K., Bryl, E., et al. (2005). The Influence of Age on T Cell Generation and TCR Diversity. J. Immunol. 174 (11), 7446–7452. doi:10.4049/jimmunol.174.11.7446
Ning, L., Lou, X., Zhang, F., and Xu, G. (2019). Nuclear Receptors in the Pathogenesis and Management of Inflammatory Bowel Disease. Mediators Inflamm. 2019, 2624941. doi:10.1155/2019/2624941
Niwa, Y., Kasama, T., Miyachi, Y., and Kanoh, T. (1989). Neutrophil Chemotaxis, Phagocytosis and Parameters of Reactive Oxygen Species in Human Aging: Cross-Sectional and Longitudinal Studies. Life Sci. 44 (22), 1655–1664. doi:10.1016/0024-3205(89)90482-7
Notter, R. H. (2000). Lung Surfactants: Basic Science and Clinical Applications. New York: Marcel Dekker, 1–444.
O'Neill, L. A. J., Kishton, R. J., and Rathmell, J. (2016). A Guide to Immunometabolism for Immunologists. Nat. Rev. Immunol. 16 (9), 553–565. doi:10.1038/nri.2016.70
Odegaard, J. I., Ricardo-Gonzalez, R. R., Goforth, M. H., Morel, C. R., Subramanian, V., Mukundan, L., et al. (2007). Macrophage-specific PPARγ Controls Alternative Activation and Improves Insulin Resistance. Nature 447 (7148), 1116–1120. doi:10.1038/nature05894
Olson, W. J., Jakic, B., and Hermann‐Kleiter, N. (2020). Regulation of the Germinal center Response by Nuclear Receptors and Implications for Autoimmune Diseases. FEBS J. 287 (14), 2866–2890. doi:10.1111/febs.15312
Oren, R., Farnham, A. E., Saito, K., Milofsky, E., and Karnovsky, M. L. (1963). Metabolic Patterns in Three Types of Phagocytizing Cells. J. Cel Biol 17, 487–501. doi:10.1083/jcb.17.3.487
Ouyang, Q., Wagner, W. M., Voehringer, D., Wikby, A., Klatt, T., Walter, S., et al. (2003). Age-associated Accumulation of CMV-specific CD8+ T Cells Expressing the Inhibitory Killer Cell Lectin-like Receptor G1 (KLRG1). Exp. Gerontol. 38 (8), 911–920. doi:10.1016/s0531-5565(03)00134-7
Paillasse, M. R., and de Medina, P. (2015). The NR4A Nuclear Receptors as Potential Targets for Anti-aging Interventions. Med. Hypotheses 84 (2), 135–140. doi:10.1016/j.mehy.2014.12.003
Papi, A., De Carolis, S., Bertoni, S., Storci, G., Sceberras, V., Santini, D., et al. (2014). PPARγ and RXR Ligands Disrupt the Inflammatory Cross-Talk in the Hypoxic Breast Cancer Stem Cells Niche. J. Cel. Physiol 229 (11), 1595–1606. doi:10.1002/jcp.24601
Papp, A. C., Azad, A. K., Pietrzak, M., Williams, A., Handelman, S. K., Igo, R. P., et al. (2018). AmpliSeq Transcriptome Analysis of Human Alveolar and Monocyte-Derived Macrophages over Time in Response to Mycobacterium tuberculosis Infection. PLoS One 13 (5), e0198221. doi:10.1371/journal.pone.0198221
Pawelec, G., Derhovanessian, E., and Larbi, A. (2010). Immunosenescence and Cancer. Crit. Rev. Oncology/Hematology 75 (2), 165–172. doi:10.1016/j.critrevonc.2010.06.012
Piergallini, T. J., and Turner, J. (2018). Tuberculosis in the Elderly: Why Inflammation Matters. Exp. Gerontol. 105, 32–39. doi:10.1016/j.exger.2017.12.021
Piergallini, T. J., and Turner, J. (2018). Tuberculosis in the Elderly: Why Inflammation Matters. Exp. Gerontol. 105, 32–39. doi:10.1016/j.exger.2017.12.021
Pulakazhi Venu, V. K., Alston, L., Iftinca, M., Tsai, Y.-C., Stephens, M., Warriyar K. V., V., et al. (2021). Nr4A1 Modulates Inflammation-Associated Intestinal Fibrosis and Dampens Fibrogenic Signaling in Myofibroblasts. Am. J. Physiology-Gastrointestinal Liver Physiol. 321 (3), G280–G297. doi:10.1152/ajpgi.00338.2019
Renga, B., Francisci, D., D’Amore, C., Schiaroli, E., Carino, A., Baldelli, F., et al. (2012). HIV-1 Infection Is Associated with Changes in Nuclear Receptor Transcriptome, Pro-inflammatory and Lipid Profile of Monocytes. BMC Infect. Dis. 12, 274. doi:10.1186/1471-2334-12-274
Ricote, M., and Glass, C. (2007). PPARs and Molecular Mechanisms of Transrepression. Biochim. Biophys. Acta (Bba) - Mol. Cel Biol. Lipids 1771 (8), 926–935. doi:10.1016/j.bbalip.2007.02.013
Rottinghaus, E. K., Vesosky, B., and Turner, J. (2009). Interleukin-12 Is Sufficient to Promote Antigen-independent Interferon-Gamma Production by CD8 T Cells in Old Mice. Immunology 128 (1 Suppl. l), e679–90. doi:10.1111/j.1365-2567.2009.03061.x
Santos, R., Ursu, O., Gaulton, A., Bento, A. P., Donadi, R. S., Bologa, C. G., et al. (2017). A Comprehensive Map of Molecular Drug Targets. Nat. Rev. Drug Discov. 16, 19–34. doi:10.1038/nrd.2016.230
Schenkel, J. M., Fraser, K. A., Beura, L. K., Pauken, K. E., Vezys, V., and Masopust, D. (2014). Resident Memory CD8 T Cells Trigger Protective Innate and Adaptive Immune Responses. Science 346 (6205), 98–101. doi:10.1126/science.1254536
Schneider, J. L., Rowe, J. H., Garcia-de-Alba, C., Kim, C. F., Sharpe, A. H., and Haigis, M. C. (2021). The Aging Lung: Physiology, Disease, and Immunity. Cell 184 (8), 1990–2019. doi:10.1016/j.cell.2021.03.005
Schöttker, B., Brenner, H., Jansen, E. H., Gardiner, J., Peasey, A., Kubínová, R., et al. (2015). Evidence for the Free Radical/oxidative Stress Theory of Ageing from the CHANCES Consortium: a Meta-Analysis of Individual Participant Data. BMC Med. 13, 300. doi:10.1186/s12916-015-0537-7
Scordo, J. M., Aguillón-Durán, G. P., Ayala, D., Quirino-Cerrillo, A. P., Rodríguez-Reyna, E., Mora-Guzmán, F., et al. (2021). A Prospective Cross-Sectional Study of Tuberculosis in Elderly Hispanics Reveals that BCG Vaccination at Birth Is Protective whereas Diabetes Is Not a Risk Factor. PLoS One 16 (7), e0255194. doi:10.1371/journal.pone.0255194
Scordo, J. M., Arcos, J., Kelley, H. V., Diangelo, L., Sasindran, S. J., Youngmin, E., et al. (2017). Mycobacterium tuberculosis Cell Wall Fragments Released upon Bacterial Contact with the Human Lung Mucosa Alter the Neutrophil Response to Infection. Front. Immunol. 8, 307. doi:10.3389/fimmu.2017.00307
Scordo, J. M., Olmo-Fontánez, A. M., Kelley, H. V., Sidiki, S., Arcos, J., Akhter, A., et al. (2019). The Human Lung Mucosa Drives Differential Mycobacterium tuberculosis Infection Outcome in the Alveolar Epithelium. Mucosal Immunol. 12, 795–804. doi:10.1038/s41385-019-0156-2
Sekhar M.D., R. V. (2019). “Oxidation Damage Accumulation Aging Theory (The Novel Role of Glutathione),” in Encyclopedia of Gerontology and Population Aging. Editors D. Gu,, and M. E. Dupre (Cham: Springer International Publishing), 1–9. doi:10.1007/978-3-319-69892-2_51-1
Sharma, G., and Goodwin, J. (2006). Effect of Aging on Respiratory System Physiology and Immunology. Clin. Interventions Aging 1 (3), 253–260. doi:10.2147/ciia.2006.1.3.253
Shenoy, A. T., and Mizgerd, J. P. (2021). Seedy CD8+ TRM Cells in Aging Lungs Drive Susceptibility to Pneumonia and Sequelae. Cell Mol Immunol 18 (4), 787–789. doi:10.1038/s41423-020-00629-w
Shivshankar, P., Boyd, A. R., Le Saux, C. J., Yeh, I.-T., and Orihuela, C. J. (2011). Cellular Senescence Increases Expression of Bacterial Ligands in the Lungs and Is Positively Correlated with Increased Susceptibility to Pneumococcal Pneumonia. Aging Cell 10 (5), 798–806. doi:10.1111/j.1474-9726.2011.00720.x
Short, K. R., Bigelow, M. L., Kahl, J., Singh, R., Coenen-Schimke, J., Raghavakaimal, S., et al. (2005). Decline in Skeletal Muscle Mitochondrial Function with Aging in Humans. Proc. Natl. Acad. Sci. 102 (15), 5618–5623. doi:10.1073/pnas.0501559102
Speeckaert, M. M., Vanfraechem, C., Speeckaert, R., and Delanghe, J. R. (2014). Peroxisome Proliferator-Activated Receptor Agonists in a Battle against the Aging Kidney. Ageing Res. Rev. 14, 1–18. doi:10.1016/j.arr.2014.01.006
Stauch, K. L., Purnell, P. R., Villeneuve, L. M., and Fox, H. S. (2015). Proteomic Analysis and Functional Characterization of Mouse Brain Mitochondria during Aging Reveal Alterations in Energy Metabolism. Proteomics 15 (9), 1574–1586. doi:10.1002/pmic.201400277
Stout, R. D., Jiang, C., Matta, B., Tietzel, I., Watkins, S. K., and Suttles, J. (2005). Macrophages Sequentially Change Their Functional Phenotype in Response to Changes in Microenvironmental Influences. J. Immunol. 175 (1), 342–349. doi:10.4049/jimmunol.175.1.342
Thomas, E. T., Guppy, M., Straus, S. E., Bell, K. J. L., and Glasziou, P. (2019). Rate of normal Lung Function Decline in Ageing Adults: a Systematic Review of Prospective Cohort Studies. BMJ Open 9 (6), e028150. doi:10.1136/bmjopen-2018-028150
Toapanta, F. R., and Ross, T. M. (2009). Impaired Immune Responses in the Lungs of Aged Mice Following Influenza Infection. Respir. Res. 10, 112. doi:10.1186/1465-9921-10-112
Torrelles, J. B., and Schlesinger, L. S. (2017). Integrating Lung Physiology, Immunology, and Tuberculosis. Trends Microbiol. 25 (8), 688–697. doi:10.1016/j.tim.2017.03.007
Ubaida-Mohien, C., Lyashkov, A., Gonzalez-Freire, M., Tharakan, R., Shardell, M., Moaddel, R., et al. (2019). Discovery Proteomics in Aging Human Skeletal Muscle Finds Change in Spliceosome, Immunity, Proteostasis and Mitochondria. Elife 8, eLife.49874. doi:10.7554/eLife.49874
Uhrlaub, J. L., Pulko, V., DeFilippis, V. R., Broeckel, R., Streblow, D. N., Coleman, G. D., et al. (2016). Dysregulated TGF-β Production Underlies the Age-Related Vulnerability to Chikungunya Virus. Plos Pathog. 12 (10), e1005891. doi:10.1371/journal.ppat.1005891
Uyemura, K., Castle, S. C., and Makinodan, T. (2002). The Frail Elderly: Role of Dendritic Cells in the Susceptibility of Infection. Mech. Ageing Develop. 123 (8), 955–962. doi:10.1016/s0047-6374(02)00033-7
van Oostrom, S. H., Engelfriet, P. M., Verschuren, W. M. M., Schipper, M., Wouters, I. M., Boezen, M., et al. (2018). Aging-related Trajectories of Lung Function in the General Population-The Doetinchem Cohort Study. PLoS One 13 (5), e0197250. doi:10.1371/journal.pone.0197250
Vaz Fragoso, C. A., and Lee, P. J. (2012). The Aging Lung. Journals Gerontol. Ser. A: Biol. Sci. Med. Sci. 67A (3), 233–235. doi:10.1093/gerona/glr249
Vesosky, B., Flaherty, D. K., Rottinghaus, E. K., Beamer, G. L., and Turner, J. (2006). Age Dependent Increase in Early Resistance of Mice to Mycobacterium tuberculosis Is Associated with an Increase in CD8 T Cells that Are Capable of Antigen Independent IFN-γ Production. Exp. Gerontol. 41 (11), 1185–1194. doi:10.1016/j.exger.2006.08.006
Vesosky, B., Flaherty, D. K., and Turner, J. (2006). Th1 Cytokines Facilitate CD8-T-Cell-Mediated Early Resistance to Infection with Mycobacterium tuberculosis in Old Mice. Infect. Immun. 74 (6), 3314–3324. doi:10.1128/iai.01475-05
Vesosky, B., Rottinghaus, E. K., Davis, C., and Turner, J. (2009). CD8 T Cells in Old Mice Contribute to the Innate Immune Response to Mycobacterium tuberculosis via Interleukin-12p70-dependent and Antigen-independent Production of Gamma Interferon. Infect. Immun. 77 (8), 3355–3363. doi:10.1128/iai.00295-09
Viola, A., Munari, F., Sánchez-Rodríguez, R., Scolaro, T., and Castegna, A. (2019). The Metabolic Signature of Macrophage Responses. Front. Immunol. 10, 1462. doi:10.3389/fimmu.2019.01462
Voehringer, D., Koschella, M., and Pircher, H. (2002). Lack of Proliferative Capacity of Human Effector and Memory T Cells Expressing Killer Cell Lectinlike Receptor G1 (KLRG1). Blood 100 (10), 3698–3702. doi:10.1182/blood-2002-02-0657
Vuttaradhi, V. K., Ezhil, I., Ramani, D., Kanumuri, R., Raghavan, S., Balasubramanian, V., et al. (2021). Inflammation-induced PELP1 Expression Promotes Tumorigenesis by Activating GM-CSF Paracrine Secretion in the Tumor Microenvironment. J. Biol. Chem. 298 (1), 101406. doi:10.1016/j.jbc.2021.101406
Wang, L., Nanayakkara, G., Yang, Q., Tan, H., Drummer, C., Sun, Y., et al. (2017). A Comprehensive Data Mining Study Shows that Most Nuclear Receptors Act as Newly Proposed Homeostasis-Associated Molecular Pattern Receptors. J. Hematol. Oncol. 10 (1), 168. doi:10.1186/s13045-017-0526-8
Wenisch, C., Patruta, S., Daxböck, F., Krause, R., and Hörl, W. (2000). Effect of Age on Human Neutrophil Function. J. Leukoc. Biol. 67 (1), 40–45. doi:10.1002/jlb.67.1.40
Wong, C. K., Smith, C. A., Sakamoto, K., Kaminski, N., Koff, J. L., and Goldstein, D. R. (2017). Aging Impairs Alveolar Macrophage Phagocytosis and Increases Influenza-Induced Mortality in Mice. J.I. 199 (3), 1060–1068. doi:10.4049/jimmunol.1700397
Xu, L., Ma, X., Verma, N., Perie, L., Pendse, J., Shamloo, S., et al. (2020). PPARγ Agonists Delay Age-Associated Metabolic Disease and Extend Longevity. Aging Cell 19 (11), e13267. doi:10.1111/acel.13267
Yang, L., Cao, Y., Zhao, J., Fang, Y., Liu, N., and Zhang, Y. (2019). Multidimensional Proteomics Identifies Declines in Protein Homeostasis and Mitochondria as Early Signals for Normal Aging and Age-Associated Disease in Drosophila*[S]. Mol. Cell Proteomics 18 (10), 2078–2088. doi:10.1074/mcp.ra119.001621
Zhang, C., Zhang, B., Zhang, X., Sun, G., and Sun, X. (2020). Targeting Orphan Nuclear Receptors NR4As for Energy Homeostasis and Diabetes. Front. Pharmacol. 11, 587457. doi:10.3389/fphar.2020.587457
Zhao, L., Zhou, S., and Gustafsson, J. Å. (2019). Nuclear Receptors: Recent Drug Discovery for Cancer Therapies. Endocr. Rev. 40 (5), 1207–1249. doi:10.1210/er.2018-00222
Zhao, L., Gimple, R. C., Yang, Z., Wei, Y., Gustafsson, J. A., and Zhou, S. (2020). Immunoregulatory Functions of Nuclear Receptors: Mechanisms and Therapeutic Implications. Trends Endocrinol. Metab. 31 (2), 93–106. doi:10.1016/j.tem.2019.10.002
Zhou, F., Drabsch, Y., Dekker, T. J. A., de Vinuesa, A. G., Li, Y., Hawinkels, L. J. A. C., et al. (2014). Nuclear Receptor NR4A1 Promotes Breast Cancer Invasion and Metastasis by Activating TGF-β Signalling. Nat. Commun. 5, 3388. doi:10.1038/ncomms4388
Keywords: aging, lung environment, oxidative stress, respiratory infections, tuberculosis
Citation: Torrelles JB, Restrepo BI, Bai Y, Ross C, Schlesinger LS and Turner J (2022) The Impact of Aging on the Lung Alveolar Environment, Predetermining Susceptibility to Respiratory Infections. Front. Aging 3:818700. doi: 10.3389/fragi.2022.818700
Received: 19 November 2021; Accepted: 03 January 2022;
Published: 19 January 2022.
Edited by:
Laura Haynes, University of Connecticut, United StatesReviewed by:
Erica C. Lorenzo, University of Connecticut, United StatesDominique Martin, University of Connecticut, United States
Copyright © 2022 Torrelles, Restrepo, Bai, Ross, Schlesinger and Turner. This is an open-access article distributed under the terms of the Creative Commons Attribution License (CC BY). The use, distribution or reproduction in other forums is permitted, provided the original author(s) and the copyright owner(s) are credited and that the original publication in this journal is cited, in accordance with accepted academic practice. No use, distribution or reproduction is permitted which does not comply with these terms.
*Correspondence: Jordi B. Torrelles, anRvcnJlbGxlc0B0eGJpb21lZC5vcmc=; Blanca I. Restrepo, QmxhbmNhLkkuUmVzdHJlcG9AdXRoLnRtYy5lZHU=; Yidong Bai, QmFpWUB1dGhzY3NhLmVkdQ==; Corinna Ross, Y3Jvc3NAdHhiaW9tZWQub3Jn; Larry S. Schlesinger, bHNjaGxlc2luZ2VyQHR4YmlvbWVkLm9yZw==; Joanne Turner, am9hbm5ldHVybmVyQHR4YmlvbWVkLm9yZw==
†These authors have contributed equally to this work