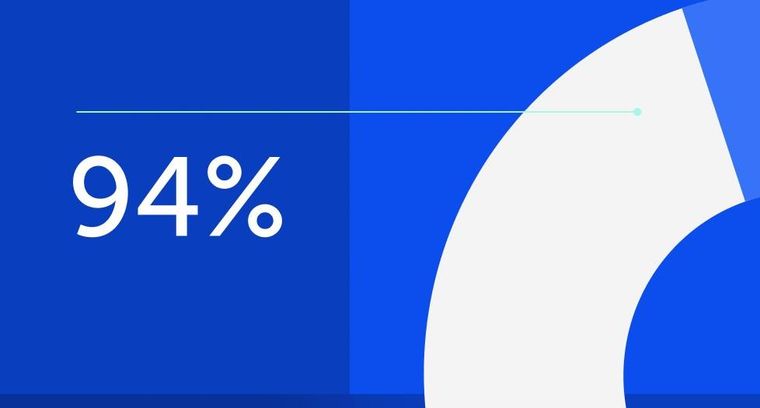
94% of researchers rate our articles as excellent or good
Learn more about the work of our research integrity team to safeguard the quality of each article we publish.
Find out more
PERSPECTIVE article
Front. Aging, 19 October 2021
Sec. Interventions in Aging
Volume 2 - 2021 | https://doi.org/10.3389/fragi.2021.740582
This article is part of the Research TopicScreening for Aging InterventionsView all 5 articles
The increase in our molecular understanding of the biology of aging, coupled with a recent surge in investment, has led to the formation of several companies developing pharmaceuticals to slow aging. Research using the tiny nematode worm Caenorhabditis elegans was the first to show that mutations in single genes can extend lifespan, and subsequent research has shown that this model organism is uniquely suited to testing interventions to slow aging. Yet, with a few notable exceptions, C. elegans is not in the standard toolkit of longevity companies. Here we discuss the paths to overcome the barriers to using C. elegans in industrial drug discovery. We address the predictive power of C. elegans for human aging, how C. elegans research can be applied to specific challenges in the typical drug discovery pipeline, and how standardised and quantitative assays will help C. elegans fulfil its potential in the biotech and pharmaceutical industry. We argue that correct application of this model and its knowledge base will significantly accelerate progress to slow human aging.
As we start to understand aging on a molecular level, there is an increasing drive to find pharmaceutical interventions that slow aging. The case to do so is strong: slowing aging would delay the onset of many chronic diseases, for most of which we have no effective therapy (e.g. Alzheimer’s disease). Furthermore, studies suggest that two current pharmaceuticals, metformin—a diabetes drug, and rapamycin—an immunosuppressant, could be repurposed to slow aging (Soukas et al., 2019; Selvarani et al., 2021). A recent boom in biotech investment has resulted in several companies raising huge sums of money to develop new drugs to tackle aging. Yet, these companies face tremendous challenges. In this Perspective, we will discuss these challenges and how the nematode Caenorhabditis elegans can assist research in industrial drug discovery. C. elegans has a special place in the history of aging research because it was the first animal where single gene mutations resulted in a substantial increase in lifespan. Yet despite this success, C. elegans is not used routinely in industrial research and development. We will discuss why not and how careful application of C. elegans research could help address the key challenges of drug discovery for aging.
The primary question for any drug candidate is whether it slows aging, and thus compounds need to be tested for efficacy in a biological model that ages as soon as possible in the development pipeline. Measuring aging in cultured mammalian cells is almost impossible. The closest model available is measuring senescence in primary human fibroblasts (Hayflick and Moorhead 1961). Aging can be accelerated in these cells by inducing molecular damage (Petrova et al., 2016). However, these experiments are limited by availability of donor tissue, inconsistency of the source (every person is different—genetically, age, medical history), and variations in methodology (Smith et al., 2002). Proceeding directly to testing compounds for aging in mice is slow and expensive, as mice have a lifespan of about 3 years. Aging can be measured at earlier ages in the mouse but there is not a consensus on the age range to use, and experiments last a minimum of several months (Jackson et al., 2017). Thus, C. elegans, with a 2–3 weeks lifespan, is an attractive model to efficiently test compounds for their ability to slow aging before proceeding to mice. Large populations can be tested, which helps deal with the high variation in aging between individuals found in all animal models (Petrascheck and Miller 2017).
All drugs have to be shown to be safe, but for a drug to slow aging there are specific considerations. The side effects of approved drugs are tolerated by regulatory authorities because the benefit outweighs the risk. In the case of slowing aging, an essentially healthy person could be exposed to a drug for decades, so the drug must show impeccable safety to stand a chance of approval. Currently the FDA and other authorities do not recognise aging as an indication, and thus cannot approve a drug to treat it, even if the drug is shown to be safe and effective. However, this position may change. Many are looking to the Targeting Aging with Metformin trial (TAME), approved by the FDA (Barzilai et al., 2016). If successful, this trial may lead to regulatory changes. For now, most drug developers are instead targeting specific age-related diseases with the hope that these drugs will also slow aging in general.
Before regulators will allow progression to clinical trials, there must be a preclinical research phase with standard requirements (European Medicines Agency 2008; Federal Drug Administration 2010). As part of this phase, drugs must be tested for safety in two mammalian species. This expensive step is bound by regulation that also makes experiments time-consuming to arrange. It would be desirable to eliminate drugs with signs of toxicity before proceeding to this point. Some toxicity work can be performed in in vitro cell culture but these methods do not reveal toxicity to the whole organism and in particular, do not reveal developmental and reproductive toxicity, or toxicity from prolonged chronic exposure. C. elegans research has no regulatory restrictions and acute, developmental and reproductive toxicity can be studied in the same experiment (Hunt 2016). Chronic toxicity can be detected in worms as they age. In studies conducted so far, the ranking of toxicity of a series of compounds in C. elegans follows results in mammals closely even if the absolute concentrations are not directly comparable (Williams and Dunesbery 1988; Harlow et al., 2016). Thus, trials in C. elegans can provide early warnings of safety issues.
It is important that the chosen model is appropriate for the specific drug development problem to be solved and that the drug developer can justify the choice to their stakeholders. These stakeholders may include their investors, who are required to support further drug development, and their partners in biotech and pharma who may ultimately license the intellectual property and bring the drug to the clinic. It will also include the regulators, who will examine the results of the R&D process before allowing progression to clinical trials. For C. elegans, it is very reasonable to question why a drug that extends the lifespan of an animal that only lives for a few weeks would be expected to slow aging in an animal that lives for several decades. Yet many interventions, either genetic disruption of conserved pathways or treatment with compounds, slow aging in very different model organisms: C. elegans, Drosophila, mice and even yeast (Johnson et al., 2013; Bhullar and Hubbard 2015; Taormina et al., 2019). For example, metformin and rapamycin extend lifespan in C. elegans (Cabreiro et al., 2013; Robida-Stubbs et al., 2013). The insulin/IGF signalling (IIS) pathway was initially discovered in C. elegans using genetics screens. Its relevance to humans has been supported by studies showing association of longevity with lower circulating IGF-1 levels or a specific allele of FOXO3, a homologue of the C. elegans gene daf-16 (Suh et al., 2008; Willcox et al., 2008; van der Spoel et al., 2015). These results suggest a common property of interventions that slow aging—that they work through universal properties of cell biology and/or animal physiology. It is yet to be seen if this will be true for all drugs that will ultimately be found to slow human aging. However, it is a good working assumption that if a compound slows aging in C. elegans it has a better chance of success than one that does not. Therefore, this information can be crucial in the decision-making process to prioritise drugs for further development and preclinical trials.
Target conservation is an important issue for drug development. C. elegans has a similar number of protein-coding genes as humans (approx. 20,000) (Willyard 2018) and many well conserved homologues (Mack et al., 2018). Most proteins involved in cell biology and metabolism have clear homologues in C. elegans, albeit often C. elegans has fewer isoforms of the same protein. For example, C. elegans has only one Class IA PI 3-kinase (AGE-1), whereas humans have three (Kriplani et al., 2015). Conservation can be very high at the amino acid level, particularly in conserved regions such as the active site, but needs to be evaluated on a protein-by-protein basis. Compounds are usually designed to interact with a particular area of a target enzymes and if there is insufficient conservation in the C. elegans homologue, this area or the entire protein can be replaced by the human gene using Cas-CRISPR technology (McDiarmid et al., 2018; Vincencio and Cerón 2021).
Scepticism about animal models is prevalent in the field of neurodegenerative disease because there have been some high-profile failures of drugs that produced positive results in lab mammals but failed clinical trials in humans - most notably BACE inhibitors for Alzheimer’s disease (Egan et al., 2018; Panza et al., 2018). These drugs inhibit the enzyme that cleaves the amyloid beta peptide, which can then form amyloid plaques in the brain (Vassar et al., 1999). In Phase III clinical trials, these drugs reduced plaque formation but did not slow cognitive decline. Many believe the underlying hypothesis was wrong, or that the drugs needed to be administered before patients showed symptoms. Regardless, the failure of animal models to predict the failure of these drugs needs to be examined. It does not mean that animal models cannot be used to develop further therapies but companies are attracted to the vastly improved tools developed for performing genetic studies with cultured human cells such as CRISPR and induced pluripotent stem cells. However, unlike C. elegans, these models do not age and do not simulate the whole organism context, and thus do not yet replace animal models of aging.
The other advantage of using an animal model is that any side effects of compounds can be seen in the context of multi-organ physiology. The long-lived C. elegans IIS mutants show a small reduction in fertility, which in the case of the age-1(hx546) mutant is only seen during cycles of starvation and feeding (Walker et al., 2000; Jenkins et al., 2004). Long-lived IIS mutants in flies and mice also show infertility. Thus, the C. elegans model is a useful predictor of the whole organism effects of interventions that slow aging. It allows the most promising interventions to be identified at an early stage of drug development and can even reveal methods to avoid side effects—for example by timing the intervention to a later age (Dillin et al., 2002). C. elegans research will never be translatable at all aspects of the drug development pipeline but can be very useful at specific stages depending on the project.
Industrial drug developers need confidence in the data they use to make key decisions. Academic research produces new biological insights that provide the underlying rationale for drug discovery. However, the workflow in academic research groups is not usually well suited for the systematic testing of lead compounds. Furthermore, there is a known issue of reproducibility between labs. Differences in outcome between labs are often caused by subtle differences in methodology that are not captured in the methods reported in basic research papers. In the field of aging, methodological differences are particularly important because multiple environmental factors can influence the aging and survival of lab animals. Many features of the academic C. elegans community ensure some degree of standardisation, such as the distribution of common strains, the ability to freeze strains and the use of common methods, along with a collaborative culture in the field. However, our own experience has found that there are many small differences in methods between labs, such as how media is prepared, how the bacteria used to feed the worms are grown, and the time between making media and using it. Some labs have tried to overcome these issues through publishing extremely detailed methods such sources of reagents including batch numbers (Lithgow et al., 2017).
Another issue that can affect reproducibility is the manual scoring of assays to measure aging in C. elegans, such as the standard lifespan assay. Transferring worms from one plate to another and accurately recording the time of death requires skill, and is vulnerable to subjective interpretation. Automation has the potential to provide objective quantifiable results. Automation covers many areas of the process including worm handling, worm observation and downstream data analysis. Several groups have invented automated methods to quantify aging in C. elegans (Stroustrup et al., 2013, Xian et al., 2013, Li et al., 2015, Churgin et al., 2017, Letizia et al., 2018, Saberi-Bosari et al., 2018, Benedetto et al., 2019, Rahman et al., 2020, Le et al., 2020, reviewed in Felker et al. (2020), Puchalt et al. (2021). These include The Lifespan Machine, which monitors lifespan of C. elegans on Petri dishes in an adapted commercial flat-bed scanner. It has been adopted by the Caenorhabditis Interventions Testing Program, an NIH-funded consortium of academic labs that tests compounds for aging effects (Banse et al., 2019). Another technology is the WormMotel (Churgin et al., 2017), which was adopted by the EU ‘Ageing with elegans’ consortium. Our group has invented a distributed imaging technology (Tataridas-Pallas et al., 2021) to measure how worms slow down with age while they are on Petri dishes (Zavagno et al., unpublished). This method can detect effects on aging within 7–10 days and produces data on how a compound influences animal movement. As well as providing an objective readout of C. elegans aging, we have found another benefit: recording how and where worms are moving on the Petri dish from the beginning of the experiment has shown that small differences in media preparation can influence the initial behaviour of the worms and is likely to affect experimental outcomes. This feedback has allowed us to further standardize our procedures to prepare worms, media and bacteria.
There have been several previous attempts to involve C. elegans in pharmaceutical drug discovery. Biotech companies including Nemapharm, Exelixis, Elixir Pharmaceuticals and DevGen formed in the late 1990s using C. elegans (Wells 1998; McCarthy 2004, 2005). Many of these companies partnered with, or were acquired by, larger pharma or biotech companies but none produced a sustainable C. elegans drug development programme. We argue that the drug discovery landscape has changed considerably over the last 2 decades, with a much larger ecosystem of biotechs involved and a greater role for contract research organisations (CROs). Specialised C. elegans CROs provide an easier option to companies that want to engage in C. elegans research but cannot invest in establishing an in-house facility, which requires considerable expertise and specialist equipment. Until recently the only outsourcing option was to work with an academic C. elegans lab, but in the last few years a small number of companies have been offering C. elegans research services (Butlerijs and Braeckmann 2020).
Once a compound has been shown to slow aging in C. elegans in a way that suggests it will be safe, further possibilities of this genetic model open up. The first is to help establish the mechanism of action. Regulators are much more likely to approve compounds if the mechanism of action is understood. It also allows drug developers to make rational decisions about optimising drug design and generating new derivatives. Mechanism of action can be studied in a number of ways in C. elegans: for example by measuring effects on gene expression either globally via RNAseq, or specifically by measuring downstream targets of known pathways, often by fluorescent reporters in live worms. Further work includes testing the involvement in known pathways or suspected target proteins using mutants or RNA interference. Furthermore, proteotomics, lipodomics and metabolomics are widely used in C. elegans research and can illuminate mechanism of action. In fact, there is a wealth of C. elegans techniques which cannot be adequately covered in this perspective (Chen et al., 2015; Apfeld and Alper 2019; Kropp et al., 2021). High throughput assessment of aging in C. elegans opens the possibility that potential targets and downstream pathways can be identified using genetics or through random mutagenesis screening. Secondly, once an effect is found, it can be developed into an assay with increased throughput, allowing hundreds or thousands of drug derivatives created by medicinal chemistry to be tested for their ability to slow aging. A similar approach has been used to screen libraries of drugs in C. elegans to find those that slow aging (Petrascheck and Miller 2017; Butlerijs and Braeckmann 2020) Our opinion is that the possibilities for C. elegans to help aging drug discovery has only just begun.
The development of drugs that slow aging faces considerable challenges. To even be considered for clinical trials, evidence is required that the compound is both effective and incredibly safe over a long period. It also important to find out as much as possible about the mechanism of action. In this perspective we have argued that research with C. elegans can assist with several of these challenges before the compound is tested in a mammalian model, thereby improving the chances that a lead compound will pass through later stages of drug development. We think that in the future, as C. elegans research is shown to accelerate drug discovery, it will drive further adoption of the technology. In fact, C. elegans was recently used to find an existing drug that could be repurposed effectively and had beneficial effects in treating a child with a genetic phosphomannomutase 2 deficiency (Iyer et al., 2019; Perlstein et al., 2021). Inspiring success stories like this one increase the interest in using C. elegans for drug discovery.
It is important to stress that C. elegans cannot solve all the problems of early-stage drug development and there are several disease states that cannot be well translated to this model. However, whole organism aging does seem to be a field in which translation works well. C. elegans must also be used in conjunction with several other distinct technological approaches. A key consideration for anyone aiming to use C. elegans in drug development is to identify the major obstacles specific to each pipeline and tailor C. elegans research to address them where relevant. There may well be several steps in the pipeline in which C. elegans can be applied and the earlier in the pipeline the number of chemical series or lead compounds is reduced, the more time and money can be saved later. Our perspective, from our experience with engaging with industrial end users, is that increasing the interaction between the scientists driving the industrial programme and the C. elegans scientists designing the research will lead to better use of this extraordinary model to accelerate progress in longevity drug development.
DW wrote the main text. GZ contributed to the writing and created.
GZ is funded by European Regional Development Fund Intensive Industrial Innovation Programme, and Magnitude Biosciences Ltd.
DW is a founder and shareholder of Magnitude Biosciences Ltd. GZ receives partial funding from Magnitude Biosciences Ltd.
All claims expressed in this article are solely those of the authors and do not necessarily represent those of their affiliated organizations, or those of the publisher, the editors and the reviewers. Any product that may be evaluated in this article, or claim that may be made by its manufacturer, is not guaranteed or endorsed by the publisher.
We would like to thank Adelaide Raimundo and Mira Nair for their helpful feedback on the manuscript.
Apfeld, J., and Alper, S. (2019). What Can We Learn about Human Disease from the Nematode C. elegans?. Methods Mol. Biol. 1706, 53–75. doi:10.1007/978-1-4939-7471-9_4
Banse, S. A., Lucanic, M., Sedore, C. A., Coleman-Hulbert, A. L., Plummer, W. T., Chen, E., et al. (2019). Automated Lifespan Determination across Caenorhabditis Strains and Species Reveals Assay-specific Effects of Chemical Interventions. GeroScience 41, 945–960. doi:10.1007/s11357-019-00108-9
Barzilai, N., Crandall, J. P., Kritchevsky, S. B., and Espeland, M. A. (2016). Metformin as a Tool to Target Aging. Cel Metab. 23 (6), 1060–1065. doi:10.1016/j.cmet.2016.05.011
Benedetto, A., Bambade, T., Au, C., Tullet, J. M. A., Monkhouse, J., Dang, H., et al. (2019). New Label‐free Automated Survival Assays Reveal Unexpected Stress Resistance Patterns duringC. Elegansaging. Aging Cell 18, e12998. doi:10.1111/acel.12998
Bhullar, K. S., and Hubbard, B. P. (2015). Lifespan and Healthspan Extension by Resveratrol. Biochim. Biophys. Acta (Bba) - Mol. Basis Dis. 1852 (6), 1209–1218. doi:10.1016/j.bbadis.2015.01.012
Butlerijs, S., and Braeckmann, B. P. (2020). Phenotypic Screening in C. elegans as a Tool for the Discovery of New Geroprotective Drugs. Pharmaceuticals 13 (8), 164. doi:10.3390/ph13080164
Cabreiro, F., Au, C., Leung, K.-Y., Vergara-Irigaray, N., Cochemé, H. M., Noori, T., et al. (2013). Metformin Retards Aging in C. elegans by Altering Microbial Folate and Methionine Metabolism. Cell 153 (1), 228–239. doi:10.1016/j.cell.2013.02.035
Chen, X., Barclay, J. W., Burgoyne, R. D., and Morgan, A. (2015). Using C. elegans to Discover Therapeutic Compounds for Ageing-Associated Neurodegenerative Diseases. Chem. Cent. J. 9, 65. doi:10.1186/s13065-015-0143-y
Churgin, M. A., Jung, S.-K., Yu, C.-C., Chen, X., Raizen, D. M., and Fang-Yen, C. (2017). Longitudinal Imaging of Caenorhabditis Elegans in a Microfabricated Device Reveals Variation in Behavioral Decline during Aging. ELife 6, e26652. doi:10.7554/eLife.26652
Dillin, A., Crawford, D. K., and Kenyon, C. (2002). Timing Requirements for insulin/IGF-1 Signaling in C. elegans. Science 298 (5594), 830–834. doi:10.1126/science.1074240
Egan, M. F., Kost, J., Tariot, P. N., Aisen, P. S., Cummings, J. L., Vellas, B., et al. (2018). Randomized Trial of Verubecestat for Mild-To-Moderate Alzheimer's Disease. N. Engl. J. Med. 378 (18), 1691–1703. doi:10.1056/nejmoa1706441
European Medicines Agency (2008). M 3 (R2) Non-clinical Safety Studies for the Conduct of Human Clinical Trials and Marketing Authorization for Pharmaceuticals. Available at: https://www.ema.europa.eu/en/documents/scientific-guideline/ich-m-3-r2-non-clinical-safety-studies-conduct-human-clinical-trials-marketing-authorization_en.pdf (Accessed July 7, 2021).
Federal Drug Administration (2010). M3(R2) Nonclinical Safety Studies for the Conduct of Human Clinical Trials and Marketing Authorization for Pharmaceuticals. Available at: https://www.fda.gov/media/71542/download (Accessed July 7, 2021).
Felker, D. P., Robbins, C. E., and McCormick, M. A. (2020). Automation of C. elegans Lifespan Measurement. Translational Med. Aging 4, 1–10. doi:10.1016/j.tma.2019.12.001
Harlow, P. H., Perry, S. J., Widdison, S., Daniels, S., Bondo, E., Lamberth, C., et al. (2016). The Nematode Caenorhabditis elegans as a Tool to Predict Chemical Activity on Mammalian Development and Identify Mechanisms Influencing Toxicological Outcome. Sci. Rep. 6, 22965. doi:10.1038/srep22965
Hayflick, L., and Moorhead, P. S. (1961). The Serial Cultivation of Human Diploid Cell Strains. Exp. Cel Res. 25 (3), 585–621. doi:10.1016/0014-4827(61)90192-6
Hunt, P. R. (2016). The C. elegans Model in Toxicity Testing. J. Appl. Toxicol. 37 (1), 50–59. doi:10.1002/jat.3357
Iyer, S., Sam, F. S., DiPrimio, N., Preston, G., Verheijen, J., Murthy, K., et al. (2019). Repurposing the Aldose Reductase Inhibitor and Diabetic Neuropathy Drug Epalrestat for the Congenital Disorder of Glycosylation PMM2-CDG. Dis. Model. Mech. 12 (11), dmm 040584. doi:10.1242/dmm.040584
Jackson, S. J., Andrews, N., Ball, D., Bellantuono, I., Gray, J., Hachoumi, L., et al. (2017). Does Age Matter? the Impact of Rodent Age on Study Outcomes. Lab. Anim. 51 (2), 160–169. doi:10.1177/0023677216653984
Jenkins, N. L., McColl, G., and Lithgow, G. J. (2004). Fitness Cost of Extended Lifespan in Caenorhabditis elegans. Proc. Biol. Sci. 271, 2523–2526. doi:10.1098/rspb.2004.2897
Johnson, S. C., Rabinovitch, P. S., and Kaeberlein, M. (2013). mTOR Is a Key Modulator of Ageing and Age-Related Disease. Nature 493 (7432), 338–345. doi:10.1038/nature11861
Kriplani, N., Hermida, M. A., Brown, E. R., and Leslie, N. R. (2015). Class I PI 3-kinases: Function and Evolution. Adv. Biol. Regul. 59, 53–64. doi:10.1016/j.jbior.2015.05.002
Kropp, P. A., Bauer, R., Zafra, I., Graham, C., and Golden, A. (2021). Caenorhabditis elegans for Rare Disease Modeling and Drug Discovery: Strategies and Strengths. Dis. Model. Mech. 14 (8), dmm049010. doi:10.1242/dmm.049010
Le, K. N., Zhan, M., Cho, Y., Wan, J., Patel, D. S., and Lu, H. (2020). An Automated Platform to Monitor Long-Term Behavior and Healthspan in Caenorhabditis elegans under Precise Environmental Control. Commun. Biol. 3, 297. doi:10.1038/s42003-020-1013-2
Letizia, M. C., Cornaglia, M., Trouillon, R., Sorrentino, V., Mouchiroud, L., Bou Sleiman, M. S., et al. (2018). Microfluidics-enabled Phenotyping of a Whole Population of C. elegans Worms over Their Embryonic and post-embryonic Development at Single-Organism Resolution. Microsyst Nanoeng 4, 6. doi:10.1038/s41378-018-0003-8
Li, S., Stone, H. A., and Murphy, C. T. (2015). A Microfluidic Device and Automatic Counting System for the Study of C. elegans Reproductive Aging. Lab. Chip 15 (2), 524–531. doi:10.1039/c4lc01028k
Lithgow, G. J., Driscoll, M., and Phillips, P. (2017). A Long Journey to Reproducible Results. Nature 548, 387–388. doi:10.1038/548387a
Mack, H. I. D., Heimbucher, T., and Murphy, C. T. (2018). The Nematode Caenorhabditis elegans as a Model for Aging Research. Drug Discov. Today Dis. Models 27, 3–13. doi:10.1016/j.ddmod.2018.11.001
McCarthy, A. A. (2004). Elixir Pharmaceuticals:Targeting Molecular Sources of Aging. Chem. Biol. 11, 733–734. doi:10.1016/s1074-5521(04)00155-3
McCarthy, A. A. (2005). Exelixis: Integrated Drug-Discovery and Development Platform for Human Therapeutics. Chem. Biol. 12, 407–408. doi:10.1016/j.chembiol.2005.04.004
McDiarmid, T. A., Au, V., Loewen, A. D., Liang, J., Mizumoto, K., Moerman, D. G., et al. (2018). CRISPR-Cas9 Human Gene Replacement and Phenomic Characterization in Caenorhabditis elegans to Understand the Functional Conservation of Human Genes and Decipher Variants of Uncertain Significance. Dis. Model. Mech. 11 (12), dmm036517. doi:10.1242/dmm.036517
Panza, F., Lozupone, M., Solfrizzi, V., Sardone, R., Piccininni, C., Dibello, V., et al. (2018). BACE Inhibitors in Clinical Development for the Treatment of Alzheimer's Disease. Expert Rev. Neurotherapeutics 18 (11), 847–857. doi:10.1080/14737175.2018.1531706
Perlstein, E. O., Lao, J., Sam, F., Diprimo, N., Parton, Z., Tsang, H., et al. (2021). “Methods for Treating Congenital Disorders of Glycosylation,”. US Patent US17/064, 441. filed 6/10/2020, patent pending.
Petrascheck, M., and Miller, D. L. (2017). Computational Analysis of Lifespan Experiment Reproducibility. Front. Genet. 8, 92. doi:10.3389/fgene.2017.00092
Petrova, N. V., Velichko, A. K., Razin, S. V., and Kantidze, O. L. (2016). Small Molecule Compounds that Induce Cellular Senescence. Aging Cell 15 (6), 999–1017. doi:10.1111/acel.12518
Puchalt, J. C., Sánchez-Salmerón, A.-J., Ivorra, E., Llopis, S., Martínez, R., and Martorell, P. (2021). Small Flexible Automated System for Monitoring Caenorhabditis elegans Lifespan Based on Active Vision and Image Processing Techniques. Sci. Rep. 11, 12289. doi:10.1038/s41598-021-91898-6
Rahman, M., Edwards, H., Birze, N., Gabrilska, R., Rumbaugh, K. P., Blawzdziewicz, J., et al. (2020). NemaLife Chip: a Micropillar-Based Microfluidic Culture Device Optimized for Aging Studies in Crawling C. elegans. Sci. Rep. 10, 16190. doi:10.1038/s41598-020-73002-6
Robida-Stubbs, S., Glover-Cutter, K., Lamming, D. W., Mizunuma, M., Narasimhan, S. D., Neumann-Haefelin, E., et al. (2013). TOR Signaling and Rapamycin Influence Longevity by Regulating SKN-1/Nrf and DAF-16/FoxO. Cell Metab 15 (5), 713–724. doi:10.1016/j.cmet.2012.04.007
Saberi-Bosari, S., Huayta, J., and San-Miguel, A. (2018). A Microfluidic Platform for Lifelong High-Resolution and High Throughput Imaging of Subtle Aging Phenotypes in C. elegans. Lab. Chip 18 (20), 3090–3100. doi:10.1039/c8lc00655e
Selvarani, R., Mohammed, S., and Richardson, A. (2021). Effect of Rapamycin on Aging and Age-Related Diseases-Past and Future. GeroScience 43, 1135–1158. doi:10.1007/s11357-020-00274-1
Smith, J. R., Venable, S., Roberts, T. W., Metter, E. J., Monticone, R., and Schneider, E. L. (2002). Relationship between In Vivo Age and In Vitro Aging: Assessment of 669 Cell Cultures Derived from Members of the Baltimore Longitudinal Study of Aging. Journals Gerontol. Ser. A: Biol. Sci. Med. Sci. 57 (6), B239–B246. doi:10.1093/gerona/57.6.b239
Soukas, A. A., Hao, H., and Wu, L. (2019). Metformin as Anti-aging Therapy: Is it for Everyone? Trends Endocrinol. Metab. 30 (10), 745–755. doi:10.1016/j.tem.2019.07.015
Stroustrup, N., Ulmschneider, B. E., Nash, Z. M., López-Moyado, I. F., Apfeld, J., and Fontana, W. (2013). The Caenorhabditis elegans Lifespan Machine. Nat. Methods 10, 665–670. doi:10.1038/nmeth.2475
Suh, Y., Atzmon, G., Cho, M. O., Hwang, D., Liu, B., Leahy, D. J., et al. (2008). Functionally Significant Insulin-like Growth Factor I Receptor Mutations in Centenarians. Proc. Natl. Acad. Sci. U S A. 105 (9), 3438–3442. doi:10.1073/pnas.0705467105
Taormina, G., Ferrante, F., Vieni, S., Grassi, N., Russo, A., and Mirisola, M. G. (2019). Longevity: Lesson from Model Organisms. Genes 10 (7), 518. doi:10.3390/genes10070518
Tataridas-Pallas, N., Thompson, M. A., Howard, A., Brown, I., Ezcurra, M., Wu, Z., et al. (2021). Neuronal SKN-1B Modulates Nutritional Signalling Pathways and Mitochondrial Networks to Control Satiety. Plos Genet. 17 (3), e1009358. doi:10.1371/journal.pgen.1009358
van der Spoel, E., Rozing, M. P., Houwing-Duistermaat, J. J., Slagboom, P. E., Beekman, M., de Craen, A. J. M., et al. (2015). Association Analysis of Insulin-like Growth Factor-1 axis Parameters with Survival and Functional Status in Nonagenarians of the Leiden Longevity Study. Aging 7 (11), 956–963. doi:10.18632/aging.100841
Vassar, R., Bennett, B. D., Babu-Khan, S., Kahn, S., Mendiaz, E. A., Denis, P., et al. (1999). Beta-Secretase Cleavage of Alzheimer's Amyloid Precursor Protein by the Transmembrane Aspartic Protease BACE. Science 286 (5440), 735–741. doi:10.1126/science.286.5440.735
Vincencio, J., and Cerón, J. (2021). A Living Organism in Your CRISPR Toolbox: Caenorhabditis elegans Is a Rapid and Efficient Model for Developing CRISPR-Cas Technologies. CRISPR J. 4 (1), 32–42. doi:10.1089/crispr.2020.0103
Walker, D. W., McColl, G., Jenkins, N. L., Harris, J., and Lithgow, G. J. (2000). Evolution of Lifespan in C. elegans. Nature 405, 296–297. doi:10.1038/35012693
Wells, W. A. (1998). High-throughput Worms. Chem. Biol. 5, R147–R148. doi:10.1016/s1074-5521(98)90174-0
Willcox, B. J., Donlon, T. A., He, Q., Chen, R., Grove, J. S., Yano, K., et al. (2008). FOXO3A Genotype Is Strongly Associated with Human Longevity. Proc. Natl. Acad. Sci. 105 (37), 13987–13992. doi:10.1073/pnas.0801030105
Williams, P. L., and Dusenbery, D. B. (1988). Using the Nematode Caenorhabditis elegans to Predict Mammalian Acute Lethality to Metallic Salts. Toxicol. Ind. Health 4 (4), 469–478. doi:10.1177/074823378800400406
Willyard, C. (2018). New Human Gene Tally Reignites Debate. Nature 558, 354–355. doi:10.1038/d41586-018-05462-w
Xian, B., Shen, J., Chen, W., Sun, N., Qiao, N., Jiang, D., et al. (2013). WormFarm: a Quantitative Control and Measurement Device toward Automated Caenorhabditis elegans Aging Analysis. Aging Cell 12 (3), 398–409. doi:10.1111/acel.12063
Keywords: aging, C. elegans, drug discovery, efficacy, toxicity, industry, regulatory
Citation: Weinkove D and Zavagno G (2021) Applying C. elegans to the Industrial Drug Discovery Process to Slow Aging. Front. Aging 2:740582. doi: 10.3389/fragi.2021.740582
Received: 13 July 2021; Accepted: 29 September 2021;
Published: 19 October 2021.
Edited by:
Christian G. Riedel, Karolinska Institutet, SwedenReviewed by:
Eva Gabriella Kevei, University of Reading, United KingdomCopyright © 2021 Weinkove and Zavagno. This is an open-access article distributed under the terms of the Creative Commons Attribution License (CC BY). The use, distribution or reproduction in other forums is permitted, provided the original author(s) and the copyright owner(s) are credited and that the original publication in this journal is cited, in accordance with accepted academic practice. No use, distribution or reproduction is permitted which does not comply with these terms.
*Correspondence: David Weinkove, ZGF2aWQud2VpbmtvdmVAZHVyaGFtLmFjLnVr
Disclaimer: All claims expressed in this article are solely those of the authors and do not necessarily represent those of their affiliated organizations, or those of the publisher, the editors and the reviewers. Any product that may be evaluated in this article or claim that may be made by its manufacturer is not guaranteed or endorsed by the publisher.
Research integrity at Frontiers
Learn more about the work of our research integrity team to safeguard the quality of each article we publish.