- 1University of Delaware, Kinesiology and Applied Physiology, Newark, DE, United States
- 2University of Colorado, Anschutz Medical Campus, Aurora, CO, United States
- 3Denver Veterans Administrative Medical Center, Geriatric Research Education and Clinical Center, Aurora, CO, United States
Aging is a primary risk factor for cardiovascular disease (CVD), which is the leading cause of death in developed countries. Globally, the population of adults over the age of 60 is expected to double by the year 2050. CVD prevalence and mortality rates differ between men and women as they age in part due to sex-specific mechanisms impacting the biological processes of aging. Measures of vascular function offer key insights into cardiovascular health. Changes in vascular function precede changes in CVD prevalence rates in men and women and with aging. A key mechanism underlying these changes in vascular function is the endothelin (ET) system. Studies have demonstrated sex and sex hormone effects on endothelin-1 (ET-1), and its receptors ETA and ETB. However, with aging there is a dysregulation of this system resulting in an imbalance between vasodilation and vasoconstriction. Thus, ET-1 may play a role in the sex differences observed with vascular aging. While most research has been conducted in pre-clinical animal models, we describe more recent translational data in humans showing that the ET system is an important regulator of vascular dysfunction with aging and acts through sex-specific ET receptor mechanisms. In this review, we present translational evidence (cell, tissue, animal, and human) that the ET system is a key mechanism regulating sex-specific changes in vascular function with aging, along with therapeutic interventions to reduce ET-mediated vascular dysfunction associated with aging. More knowledge on the factors responsible for the sex differences with vascular aging allow for optimized therapeutic strategies to attenuate CVD risk in the expanding aging population.
Introduction
Aging is a primary risk factor for cardiovascular disease (CVD), which is the leading cause of death in developed countries. Currently, 13% of the world population and 16% of the population in the United States is over the age of 65 (Ortman et al., 2014; Bank, 2019). Moreover, globally, the population of adults over the age of 60 is expected to double by the year 2050 (Ortman et al., 2014). The prevalence of CVD increases with age, but there are important sex differences in both prevalence and mortality (Virani et al., 2020). Young apparently healthy premenopausal women generally exhibit a favorable cardiovascular risk profile compared with age-matched men, largely attributed to the protective role of ovarian hormones (Iorga et al., 2017). However, the menopause transition is associated with an accelerated risk of CVD in women, matching or exceeding that of men (Dosi et al., 2014), and is thought to be due in part to changes in sex hormones. Understanding the mechanisms contributing to the sex differences in CVD with aging is important given differences in disease onset and mortality.
The endothelium is a monolayer of endothelial cells that creates a barrier between the blood and vascular smooth muscle cells (VSMC), forming the lumen of the blood vessel. To maintain homeostasis, the endothelium produces and releases both vasodilating and vasoconstricting factors, the most potent being nitric oxide (NO) and endothelin-1 (ET-1), respectively. Endothelial dysfunction, which precedes the development of cardiovascular pathologies such as atherosclerosis is a non-traditional risk factor for CVD (Vanhoutte et al., 2017). Endothelial dysfunction is characterized by a reduced vasodilatory capacity, primarily mediated by reduced NO bioavailability, a pro-inflammatory, and pro-thrombotic state of the vasculature (Bonetti et al., 2003). Measures of endothelial function are considered a biomarker for cardiovascular health, and functional changes in conduit and resistance vessels reflect changes in the coronary arteries (Muller-Delp, 2013; Schnabel et al., 2013; Huxley and Kemp, 2018), serving as a useful surrogate measure of coronary endothelial function.
Aging is associated with declines in endothelial function. Notably, there are sex differences in age-related declines in endothelial function. Young women tend to have greater endothelium-dependent dilation compared to men (Schnabel et al., 2013). In men, the decline in endothelial function, as measured by flow-mediated dilation (FMD), begins around the 4th decade of the life; however, this is delayed in women by ∼10 years occuring around the 5th decade of life (Celermajer et al., 1994), which is around the time of menopause (Figure 1). After menopause, the rate of decline is much greater in women compared to men as they age (Celermajer et al., 1994). These sex-specific declines in endothelial function with aging mirror the observed increase in CVD prevalence with aging. Importantly, endothelial function when used as a surrogate marker for cardiovascular health is a useful tool for studying sex differences in vascular aging.
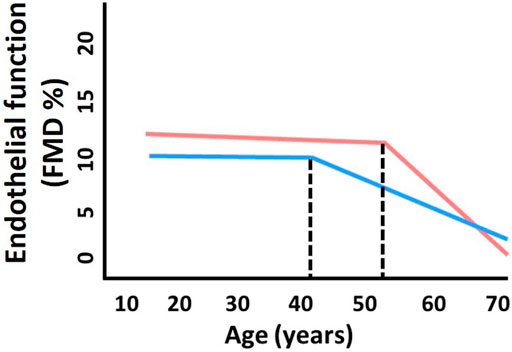
FIGURE 1. Sex differences in age-related declines in endothelial function. The decline in endothelial function (as measured by flow-mediated dilation, FMD) in men (blue) begins around the 4th decade of the life. This inflection point is shifted ∼10 years later in women (pink) to around the 5th decade of life, which is around the time of menopause. Thereafter, the rate of decline is greater in women compared to men with aging. Adapted from Celermajer et al. (1994).
The mechanisms underlying sex differences in the age-related decline in endothelial function remain under investigation, but accumulating evidence indicates that the ET system is a key sex-specific regulator of vascular dysfunction associated with aging. The ET system is active in many tissues such as neural, kidney, lung, and vasculature (Khimji and Rockey, 2010) and plays a role in cardiovascular physiology (Lerman et al., 1995) and numerous pathologies such as vascular dysfunction (Iglarz and Clozel, 2007), atherosclerosis (Ihling et al., 2001), hypertension (Cardillo et al., 1999), coronary artery disease (Kolettis et al., 2013; Best and Lerman, 2000) and heart failure (Ibrahim et al., 2018). Sex hormones can regulate the ET system at the cellular level leading to functional changes in the vasculature. Eloquent reviews have been published on sex differences in the ET system in hypertension, cardiovascular, and renal diseases (Montezano et al., 2008; Gohar and Pollock, 2017). Moreover, sex hormones can directly impact the ET system as previously reviewed (Mikkola et al., 1998; Tostes et al., 2003; Kittikulsuth et al., 2013). This review will focus on the ET system as a major contributor to sex-specific differences in vascular aging. We will review the role of ET-1 in vascular aging from a translational perspective, and update information on sex differences in the ET system with an emphasis on the therapeutic role of ET-1 in vascular adaptations to aerobic exercise.
Aging also increases the risk for CVD through other adverse effects on the arterial wall, including VSMC growth, apoptosis, vascular hypertrophy and enhanced matrix metalloproteinase activity, which can lead to arterial remodeling and stiffening (Folkow and Svanborg, 1993; McEniery et al., 2005; Cockcroft and Mancia, 2012). In contrast to age-associated endothelial dysfunction, there are disparities in the literature on whether there are sex differences in the age-related increase in arterial stiffness, with some studies reporting a similar progression in the age-related increase in arterial stiffness, a steeper progression in men after the age of 50, and greater age-related rates of aortic and carotid artery stiffening in women (DuPont et al., 2019). The disparities in the literature can be attributed to methodology, however, there is a lack of knowledge on how changes in sex hormones contribute to age-related arterial stiffening in both women and men. Although the focus of this review will be on endothelial function, we wish to refer the reader to these excellent reviews on arterial stiffness (Safar, 2018; DuPont et al., 2019).
Regulation of Endothelin
The ET peptide is a potent vasoconstrictor primarily released from endothelial cells (Yanagisawa et al., 1988). There are three variants (ET-1, ET-2, ET3) of the vasoactive peptide with ET-1 being the most biologically active. ET-1 acts locally in a paracrine and autocrine manner due to its abluminal secretion and location of its receptors (Wagner et al., 1992; Davenport et al., 1990), and approximately 60% of circulating ET-1 is cleared in each pass by ETB receptors located on the lungs, kidney and liver, thus plasma concentrations are kept low (Frelin and Guedin, 1994; Dupuis et al., 1996; Johnström et al., 2005).
ET-1 synthesis is regulated through gene transcription. Gene transcription results in prepro-ET-1 mRNA which is cleaved by the protein convertase, furin, to yield Big ET-1 (Peto et al., 1996). Big ET-1 is a 38 amino acid peptide and is the precursor to the 21 amino acid long, biologically active ET-1 peptide (Russell et al., 1998). Big ET-1 can be cleaved by either of two endothelin converting enzymes, ECE-1 or ECE-2 (Turner and Murphy, 1996). ECE-1 has four splice variants and is present on both the plasma membrane and intracellular compartments of endothelial cells (Xu et al., 1994; Schweizer et al., 1997; Valdenaire et al., 1999). Additionally, there are mechanisms of ET-1 generation that are independent of ECE; ET-11-31 is an inactive intermediate which is converted to active ET-1 neprilysin (Wypij et al., 1992; Maguire and Davenport, 2004). For a comprehensive review of endothelin synthesis, we refer the reader to the following publications (D’Orléans-Juste et al., 2003; Houde et al., 2016).
Following synthesis, the peptide and its precursor Big ET-1 are stored in intracellular vesicles such as Weibel-Palade bodies (Kavashima et al., 1999). A majority of the peptide is stored as Big ET-1 and is converted to the biologically active peptide during exocytosis. ET-1 has both a constitutive and regulated pathway for release (Seed et al., 2012). In the constitutive pathway, secretory vessels containing Big-ET1, ET-1, ECE-1, and ECE-2 are trafficked to the membrane for release towards the VSMC (Kuruppu et al., 2007). In the regulated pathway, ET-1 is stored in Weibel-Palade bodies of endothelial cells (Russell et al., 1998). Upon stimulation, trafficking to the membrane occurs and ET-1 is released abluminally providing an immediate increase in the local concentration of ET-1. This local surge in ET-1 following stimulation is important to its vasoactive properties in regulating vascular tone.
Although the endothelium is the primary source of ET-1, there are also non-endothelial sources of ET-1 that include VSMCs and immune cells such as monocytes, neutrophils, and mast cells. VSMCs release ET-1 at a rate that is 100× less than endothelial cells and do not utilize the same storage mechanisms of ET-1 as endothelial cells (Kanse et al., 1991). Despite the lower release of ET-1 from VSMC compared to endothelial cells, ET-1 expression can still be upregulated in VSMC in pathological conditions such as atherosclerosis (Haug et al., 1996). The cells can act through various feedback loops to influence ET-1 production, as demonstrated by the ability of VSMC to inhibit endothelial cell ET-1 production through a NO-mediated mechanism (Di Luozzo et al., 2000). Additionally, vascular cells can respond in unique ways to various ET-1 secretagogues. For example, endothelial cell and VSMC respond differently to ET-1 secretagogues, such as cortisol, which can increase VSMC ET-1 expression while not impacting endothelial cell ET-1 production (Kanse et al., 1991). Non-vascular cells such as immune cells express ET receptors and can release ET-1 to interact locally with the endothelium and VSMC (Iwasa et al., 1999; Elisa et al., 2015; Shinagawa et al., 2017). Specifically, immune cells interact with the endothelium and can increase local inflammation and lead to development of atherosclerotic plaques (Iwasa et al., 1999). Thus, the regulation of ET-1 is an amalgamation of factors including the stimuli, cell types stimulated, and individual cellular responses to secretagogues as well as local cell-to-cell feedback mechanisms.
ET-1 plays an essential role in regulating vascular tone through a balance of endothelial cell and VSMC receptor activation. In the vasculature, both the ETA and ETB receptors are expressed on VSMC and mediate vasoconstriction (Ivey et al., 2008; Nilsson et al., 2008). The vascular smooth muscle ETA receptor is the primary receptor driving the potent vasoconstrictor effects of ET-1 in healthy vasculature, which are long lasting. The signaling cascade involves phospholipase C (PLC), inositol triphosphate (IP3), and diacylglycerol (DAG) leading to an increase in intracellular calcium release (Avedanian et al., 2010). Other signaling pathways activated include the phospholipase D (PLD), phospholipase A2 (PLA2), and mitogen activated protein kinase (MAPK) contributing to the indirect effects of ET-1 such as cellular migration and proliferation (Khalil, 2011). ETA receptors also undergo posttranslational modification such as palmitoylation (Horstmeyer et al., 1996), however they do not undergo ligand-induced phosphorylation which contributes to their prolonged pressor effects (Cramer et al., 1997). The VSMC ETB receptor also leads to vasoconstriction acting through the PLC pathway (Wynne et al., 2009). The ETB receptors are secondary to the ETA receptor-mediated contraction of the VSMC, and expression of ETB receptors is low in non-pathological conditions. However, in pathological conditions ETB receptor expression is upregulated and can contribute to increased vascular tone (Donnelly, 2017).
The ETB receptors are also located on endothelial cells. Endothelial ETB receptors balance the vasoconstrictor effects of VSMC, promote vasodilation via enhancing NO and prostacyclin (PGI2), and act as a clearance receptor to help remove local ET-1 (Hirata et al., 1993). The ETB receptor has numerous sites for posttranslational modifications including phosphorylation, palmitoylation, and glycosylation which can alter its functional properties (Davenport et al., 2016). ETB receptors are deactivated via phosphorylation causing a reduction in activity within 5 minutes (Cramer et al., 1997) and are internalized and trafficked to an endosomal/lysosomal pathway for degradation (Bremnes et al., 2000). These properties of the ETB receptor enable it to act as a clearance receptor and attenuate the vasoconstrictor effects of ET-1 (Kelland et al., 2010). Additionally, the endothelial cell ETB receptor mediates vasodilation through activation of intracellular signaling pathways including PLC, phosphatidylinositol 3- kinase (PI3K), and second messengers IP3 and DAG leading to endothelial nitric oxide synthase (eNOS) phosphorylation and subsequent NO production (Shihoya et al., 2016). However, when there is increased oxidative stress, posttranslational modification of the ETB receptor can lead to attenuated NO production which can promote vascular pathologies (Maron et al., 2012). The impact of endothelin receptors on the vasculature depends on their level of expression and functional capacity, both of which can independently be impacted by aging, sex, and sex hormones.
ET-1, Aging, and Sex
Aging, sex, and sex hormones are associated with changes in ET-1 synthesis, expression, release from endothelial cells, and circulating plasma levels, all which can contribute to vascular dysfunction (Tokunaga et al., 1992; Kumazaki et al., 1994; Donato et al., 2009). Moreover, ETA and ETB receptor expression and function differ between sexes, are modulated by changes in sex hormones, and impacted by aging (Van Guilder et al., 2007; Stauffer et al., 2010; Wenner et al., 2017; Sebzda et al., 2018; Stanhewicz et al., 2018). Directly measuring ET-1 and its receptors at the cell and tissue level can provide unique insights into the molecular mechanisms that are underlying changes in the ET system with aging. Increased ET-1 production and release with aging may be associated with increased receptor expression and activation, leading to a shift in vascular tone away from favoring vasodilation and towards a vasoconstrictor phenotype. This vasoconstrictor phenotype is pro-atherosclerotic and contributes to CVD onset and progression (Vanhoutte et al., 2009). Thus, the ET system may be used as a sex-specific biomarker of vascular health and serve as a molecular target for treatments to reduce age-related CVD.
Plasma ET-1
Plasma levels of ET-1 increase with age and are elevated in middle-aged and older adults compared to young adults (Miyauchi et al., 1992). In women there is a stepwise increase in plasma ET-1 between young, middle, and older adults (Maeda et al., 2003). In men, there is also higher plasma ET-1 in older compared to younger adults (Donato et al., 2009). Plasma ET-1 levels have also been shown to be higher in men compared to women in both young and middle-aged adults (Miyauchi et al., 1992; Polderman et al., 1993). Although plasma ET-1 is higher in men, an 8-year observational study of Swedish adults (30–74 years) reported a strong association between plasma ET-1 and incident CVD in women, while observing no differences in baseline plasma ET-1 between men who did or did not develop incident CVD (Daka et al., 2015). Furthermore, a longitudinal observational cohort study showed plasma ET-1 was a predictor of 10-year all-cause mortality in a general population of healthy middle-aged and older adults (Yokoi et al., 2012). This finding was further supported in a study of polish centenarians in which probability of 1-year survival was associated with ET-1, suggesting a lower plasma ET-1 is a significant independent predictor for longer survival in centenarians (Szewieczek et al., 2015). Throughout aging those with cardiovascular risk factors, such as high blood pressure and atherosclerosis, appear to have elevated plasma ET-1 compared to their age-matched healthy controls (Lerman et al., 1991; Bossard et al., 2015) which could increase their risk of adverse outcomes (Zhang et al., 2017). Additionally, pathologies that are predictive of future CVD such as erectile dysfunction in men and preeclampsia in women, as well as smokers (Hirai et al., 2004), patients with insulin resistance (Piatti et al., 1996), or hyperlipoproteinemia (Haak et al., 1994) also demonstrate increased plasma ET-1, further supporting the importance of the ET system in CVD (El Melegy et al., 2005; George and Granger, 2011). This elevated plasma ET-1 in CVD is likely mediated by increased ET-1 production (synthesis and release) rather than a decrease in clearance (Parker and Thiessen, 2004).
The precursors (such as Big ET-1) and intermediates of ET-1 have also been measured in the plasma, and higher levels are associated with the presence of CVD pathology and severity (Leslie et al., 2004). The 31-amino acid intermediate of ET-1 (ET-11-31) is produced through a chymase enzymatic reaction and is also biologically active although less potent than ET-1 produced through its traditional pathway (Leslie et al., 2004b; Maguire et al., 2001; Fecteau et al., 2005). Plasma levels of ET-11-31 were higher in patients with atherosclerosis compared to healthy patients (Suzaki et al., 2003). ET-11-31 is converted to active ET-1 by NEP, and inhibition of NEP can regulate the activity of ET-11-31. A detailed review of ET-11-31 and cardiovascular pathologies, which further describe the impact and significance of ET-11-31 has previously been published (D’Orléans-Juste et al., 2008). Additionally, C-terminal pro-ET-1 (CT-proET-1) is crucial for ETA and ETB receptor activity, and can be found in the plasma and is elevated in cardiovascular pathologies and associated with organ damage in numerous physiological systems (Habib et al., 2010; Drion et al., 2012; Buendgens et al., 2017). Patients with high levels of CT-proET-1 also had higher mortality suggesting there could be a threshold effect or dose-response relation to disease severity and plasma CT-proET-1 (Buendgens et al., 2017). An additional benefit of measuring CT-proET-1 is the increased stability compared to ET-1 (Xu et al., 1994; Buendgens et al., 2017). Each peptide independently appears to be associated with CVD and collectively measuring plasma concentrations of ET-1, its variant ET-11-31, and its precursors Big ET-1 and CT-proET-1 could provide a more comprehensive assessment of the ET system and its potential impact on cardiovascular health.
Vascular ET-1 Expression and Sensitivity
Despite the benefits of measuring plasma ET-1, plasma concentrations of ET-1 may not always be reflective of tissue or cellular ET-1; local concentrations of ET-1 are tissue dependent and can be 100-fold higher than plasma concentrations (Battistini et al., 1993; Davenport et al., 2016). In animal models, aging is associated with increased expression of prepro-ET-1 mRNA, increased expression of ECE-1, greater stimulated release of ET-1, (Goel et al., 2010) and greater ET-1 expression in the coronary artery (Granado et al., 2014). Aging increases the number of secretagogues that can stimulate the ET system leading to increased activity. Numerous studies using either aging models in cell culture or using cells from older adults demonstrate an increased release of ET-1 in older compared to younger groups (Tokunaga et al., 1992; Kumazaki et al., 1994; Khatib et al., 2007; Wang et al., 2015). A greater concentration of ET-1 may contribute to the reduced sensitivity to ET-1, as noted by a reduced vasoconstrictor response to exogenous ET-1, although this may differ across vascular beds (Weinheimer et al., 1990; Homma et al., 1992; Donoso et al., 1994; Donato et al., 2005).
There is evidence in both animals and humans of reductions in ET-1 sensitivity with aging, which could suggest age-related increases in ET-1 expression and augmented endothelin-mediated vasoconstrictor tone. In humans, blunted vasoconstrictor responses to exogenous ET-1 have been reported in older compared to younger men (Van Guilder et al., 2007), suggesting increased vasoconstrictor tone attributable in part by ET-1 (Thijssen et al., 2007; Van Guilder et al., 2007; Westby et al., 2011). Moreover, in older men there is greater arterial and venous endothelial cellexpression of ET-1 compared to young men (Donato et al., 2009). Notably, there was an inverse relation between FMD and endothelial ET-1 expression, suggesting that the age-related decline in endothelial function in older men may be related to a greater expression of ET-1 and increased ET-1 signaling. Taken together, we speculate that the increased expression of ET-1 in endothelial cells (Donato et al., 2009) coupled with the blunted vasoconstrictor response to exogenous ET-1 in older men (Van Guilder et al., 2007) could suggest greater bioavailability of ET-1 in older adults. Importantly, comparable studies have yet to be published regarding the impact of aging on cellular ET-1 expression in women as well as if sensitivity to exogenous ET-1 is altered with aging in women. Sex differences in cellular secretion of ET-1 have been previously demonstrated. In a model examining male and female HUVECs from twins, males had higher levels of ET-1 secretion at rest. However, when stimulated with VEGF females had a significant increase in secreted ET-1, an effect that was not observed in the male cells, suggesting sex differences in the response to ET-1 secretagogues (Lorenz et al., 2019). Thus, there may be inherent biological differences in ET-1 release that lead to females secreting more ET-1 upon stimulation which may exacerbate endothelin-mediated dysfunction when ET-1 secretagogues increase with aging.
ET-1 Interaction With Other Vasoactive Substances
ET-1 interacts with a number of other substances within the vasculature, such as NO, PGI2, and thromboxane. NO is a regulator of ET-1, and increased NO can downregulate ET-1 production (Bilsel et al., 2000; Tan et al., 2007). This finding is further supported through addition of L-NMMA, a NO inhibitor which led to increased ET-1 production (Akishita et al., 1998). Overexpression of ET-1 can modulate the production of vasodilators, while also exacerbating its pressor effects leading to increased vascular tone and reduced vasodilatory capacity. This can be observed in part through ET-1-mediated reduction in NO bioavailability due to impaired NO production and reduced expression of eNOS (Sud and Black, 2009). ET-1 also mediates superoxide production through activation of NADPH oxidase which can further inhibit vasodilator capacity (Sud and Black, 2009). With aging the increase in ET-1 is associated with a corresponding reduction in NO, both of which contribute to the age-associated decline in endothelial function. Moreover, prostanoid production shifts with aging to favor vasoconstriction (Seals et al., 2011; Bachschmid et al., 2013), and ET-1 upregulates cyclooxygenase-2 expression to favor inflammation and vasoconstriction (Lin et al., 2014). Sex hormones, ET-1 and aging are known to act on the vascular cyclooxygenase system and thus modulate vascular reactivity (Mikkola et al., 1998; Davidge 2001). Aging is also associated with an increased inflammation, characterized by increased proinflammatory cytokines including TNF-α, IL-6, and VCAM-1 as well as CRP and NF-kB (Donato et al., 2008; Donato et al., 2007). These inflammatory cytokines can stimulate ET-1 synthesis, further exacerbating the production of inflammatory cytokines and endothelial dysfunction. More comprehensive reviews on aging and NO can be found here (Seals et al., 2011; Cau et al., 2012; Bachschmid et al., 2013; Sverdlov et al., 2014).
ETA and ETB Receptors
As previously mentioned, ETA and ETB receptors are located on VSMC, and the ETB receptor is also located on the endothelium. The ratio of receptors is important in mediating the functional responses to ET-1. There is a shift in ETA:ETB receptor expression with aging in male Sprague-Dawley rats which can lead to functional changes (Del Ry et al., 2008). In animal models of hypertension and hypercholesterolemia, the ratio of endothelial to smooth muscle ETB receptors is attenuated favoring a vasoconstrictor profile (Kakoki et al., 1999), and can contribute to ET-mediated vascular dysfunction. Importantly with aging there is an increased likelihood for developing hypertension and hypercholesterolemia, thus the changes in the ET system could be compounded by age as well as CVD pathologies.
In humans, sex and aging can both impact receptor density and function. The ETB receptor mediates vasoconstrictor tone in young men, whereas it mediates vasodilator tone in young women (Kellogg et al., 2001; Wenner et al., 2017). These sex differences are also apparent in older adults; Stauffer and colleagues demonstrated older men have greater ETA mediated vasoconstrictor tone compared to women (Stauffer et al., 2010). Interestingly, older postmenopausal women had greater increases in forearm blood flow to dual antagonism of the ETA and ETB receptor compared to ETA alone, suggesting ETB receptors play a larger role in mediating vascular function in older women while ETA receptors play a larger role in older men (Stauffer et al., 2010). Additionally, receptor density is different between older men and women such that older men have a greater density of ETA receptors as well as a higher ratio of ETA:ETB receptors in the saphenous vein compared to women (Ergul et al., 1998). A lack of ETB-mediated vasodilation and increased ETA-mediated vasoconstriction can reduce the vasodilatory capacity contributing to vascular dysfunction. The shift in receptor expression is compounded by changes in ET-1 expression that further exacerbates ET-mediated dysfunction. Collectively, these differences in receptor expression result in functional changes that contribute to age-related endothelial dysfunction.
Women
There has been an increasing amount of evidence pointing to changes in ETB receptor function as a mechanism contributing to vascular dysfunction in women. Our lab has provided additional support demonstrating that the ETB receptor promotes vasodilation in young women however this effect is lost following menopause (Figure 2A) (Wenner et al., 2017). This switch in ETB receptor function in postmenopausal women suggests that the ETB receptor is a primary driver of ET-mediated vascular dysfunction in women (Wenner et al., 2017). Moreover, we have recently shown reduced ETB receptor expression on endothelial cells in postmenopausal compared to young premenopausal women (Figure 2B) (Kuczmarski et al., 2020). Additionally, we reported a positive relation between FMD and ETB receptor expression further suggesting a role for the ETB receptor in contributing to vascular function (Figure 2C). Indeed, these studies suggest there is attenuated ETB mediated dilation in postmenopausal women in part due to lower endothelial ETB receptor expression, and possibly an increased vascular smooth muscle cell ETB-mediated constriction.
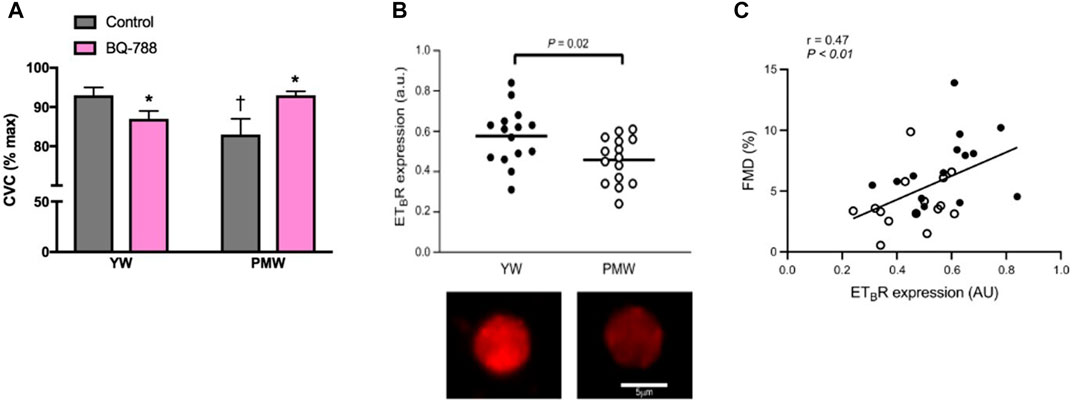
FIGURE 2. Loss of ETB-mediated vasodilation and decreased endothelial ETB receptor expression contribute to age-related declines in endothelial function in women. Cutaneous microvascular conductance (CVC; expressed as % maximal dilation) was measured in young women (YW) and postmenopausal women (PMW) during local heating with microdialysis perfusions of lactated Ringer solution (control; gray bars) or the ETB receptor antagonist BQ-788 (pink bars). ETB receptors mediate dilation in young women (YW), an effect that is lost in postmenopausal women (PMW) (A) Graph from Stanhewicz et al. (2018); data from Wenner et al. (2017). ETB receptor expression [in arbitrary units (a.u.)] in venous endothelial cells collected from healthy young women (YW) and postmenopausal women (PMW). (B, top) Representative images of a venous endothelial cell ETB receptor expression in a YW and PMW with a scale bar representing cell size (B, bottom) Data from Kuczmarski et al. (2020). Pearson correlation showing the significant relation between flow-mediated dilation (FMD, %) and ETB receptor expression [in arbitrary units (AU)] among YW (n = 15, closed circles) and PMW (n = 15, open circles) (C) Data from Kuczmarski et al. (2020).
The loss of estradiol (E2) during menopause could be a factor contributing to the changes in the ET system. Estradiol not only directly impacts ET-1, but also enhances NO and PGI2 (Davidge, 2001). To better understand the impact of sex hormones on the ET system we conducted a study examining ET receptor function during two stages of the menstrual cycle. In healthy young women, during the mid-luteal phase when estrogen and progesterone concentrations are elevated, ET receptor function favored a vasodilatory profile (Sebzda et al., 2018). Thus, both aging and loss of hormones could contribute to phenotypic and functional changes in the ET system in postmenopausal women. Additional work is needed to tease out the individual effects of estradiol and progesterone on ET receptor function.
The use of animal models, specifically ovariectomy (OVX), a form of surgical menopause that reduces levels of ovarian hormones in females, with E2 add-back, have been a useful tool to study the effects of E2 on the ET system. OVX leads to increased plasma and cellular ET-1 levels as well as increased ET-1 mediated constriction (Wang et al., 1997; Rodrigo et al., 2003; Tan et al., 2003). Increased VSMC constriction is mediated by increased ETA mediated tone and/or increased ETB receptor expression (Sudhir et al., 1997; David et al., 2001). E2 add-back following OVX attenuated ET-1 levels and ET-1 mediated constriction (Sudhir et al., 1997; Tan et al., 2003). Some of these actions appear to be through E2 receptors as treatment with the selective estrogen receptor modulator, raloxifene, reduced ET-1 expression compared to the OVX group (Demir et al., 2013). Additionally, both ET receptor antagonism and E2 independently or combined, have a vasoprotective effect in OVX rats following vascular injury (Kitada et al., 2014).
ET receptor expression is also impacted by E2. In an animal model using OVX and E2 add-back, the loss of ovarian hormones increased ET receptor expression, however this response was attenuated when E2 was restored (Gohar et al., 2016). ETA receptor mRNA expression, but not ETB receptor expression, was reduced after treatment with E2 in the aorta of OVX rabbits (Pedersen et al., 2009). In rat aortic VSMCs there was also a reduction in ETA receptor protein expression following E2 treatment; unfortunately, ETB receptor expression was not evaluated in this study (Ting-Huai et al., 2001). In contrast, cardiomyocytes from OVX spontaneously hypertensive rats had decreased ETB receptor mRNA expression following E2 treatment with no change in ETA receptor expression (Nuedling et al., 2003). Another study evaluating the impact of E2 following a myocardial infarction in female rats demonstrated no change in ETA receptor expression but attenuated ETB receptor expression in the left ventricle in the E2 treated group compared to placebo (Smith et al., 2000). Importantly, different tissues have different ratios of endothelin receptor expression, and the model used can also impact ET receptor expression. Gohar et al. examined ETA receptor and ETB receptor mRNA expression in OVX rats treated with E2 and demonstrated tissue specific changes in receptor expression. For example, in the lungs, E2 decreased ETA receptor expression but did not change ETB receptor expression, whereas in the liver, E2 did not change ETA receptor expression but increased ETB receptor expression (Gohar et al., 2016). It has also been shown that E2 attenuates ETA expression on VSMCs in culture (Ting-Huai et al., 2001). Thus, there appears to be tissue dependent actions of E2 on the ET system, such that loss of E2 can lead to dysregulation of the endothelin system and restoration of E2 seems to attenuate the ET system.
Investigation of the underlying mechanistic pathways to explain the role of E2 on the ET system are ongoing. In cell culture models, E2 treatment was able to attenuate ET-1 production in cells stimulated with numerous secretagogues including angiotensin-II, thrombin, TNF-α, and cyclic strain (Bilsel et al., 2000; Juan et al., 2004; Chao et al., 2005; Pearson et al., 2008; Morey et al., 2015). This could be due to E2 directly inhibiting ET-1 production through regulating gene transcription (Bilsel et al., 2000). E2 can act through both its ERα and ERβ receptor in HUVECs to attenuate ET-1 production (Evans et al., 2002). E2 can also modulate mast cell degranulation activity, thereby affecting the source of ET-1 modulating proteases (Maurer et al., 2004; Zierau et al., 2012). The addition of a pure estrogen receptor antagonist, ICI182780, attenuated the effects of E2 on ET-1 production demonstrating the presence of an estrogen receptor-mediated pathway (Akishita et al., 1998). Additionally, short term E2 exposure attenuated the vasoconstrictor effects of ET-1 in porcine coronary artery rings (Teoh et al., 2000). Collectively these studies suggest E2 can attenuate the production, secretion, and constrictor effects of ET-1.
More recently the G-protein estrogen receptor (GPER) has been shown to play a role in modulating the ET system by attenuating the ET-1 vasoconstrictor response in VSMC (Meyer et al., 2012) as well as increase NO production in endothelial cells (Fredette et al., 2018). GPER also can modulate ET-1 as well as ETA and ETB receptor expression in the kidney (Gohar et al., 2020). Indirect effects are mediated by E2 ability to increase NO, which can downregulate ET-1 production (Bilsel et al., 2000; Tan et al., 2007). When NO was inhibited, there was increased ET-1 production providing additional evidence of the regulatory effects of NO (Akishita et al., 1998). Receptor-independent mechanisms have been described using the E2 metabolites, 2-hydroxyestradiol and 2-methoxyestradiol, both of which have inhibitory effects on ET-1 release (Dubey et al., 2001). This finding could have important implications for postmenopausal women who can still produce E2 locally in various tissues. These different pathways suggest some redundancy in the ability of hormones and their metabolites to attenuate the ET system, and while favorable effects of E2 replacement therapy on plasma ET-1 have been reported in postmenopausal women (Best et al., 1998), the underlying mechanisms have yet to be studied in humans. This additional production pathway is an important consideration for women using menopausal hormone therapy (Bolton 2016; Naftolin et al., 2019).
Men
The ET system also contributes to vascular dysfunction in men. In young men ETB and ETA receptors mediate vasoconstrictor tone (Haynes and Webb, 1994; Kellogg et al., 2001). In older men there is greater ETA mediated vasoconstrictor tone compared to younger men, as determined by brachial artery infusion of the ETA receptor antagonist, BQ-123 (Van Guilder et al., 2007). Furthermore, BQ-123 administration improved acetylcholine-mediated vasodilation in older men (Westby et al., 2011), suggesting that ETA-mediated vasoconstriction contributes to the age-related endothelial dysfunction in men (Figure 3A). Both plasma and endothelial cell ET-1 expression (Figure 3B) are increased in older men compared to younger men (Donato et al., 2009), and the vasoconstrictor response to exogenous ET-1 is attenuated in older men compared to younger men (Van Guilder et al., 2007). Thus, there appears to be elevated ET-1 bioavailability as well as increased ETA receptor activation leading to augmented ET-mediated vasoconstrictor tone in older men. These changes are likely due to aging and possibly declines in testosterone, both of which can modulate the ET system and vascular function.
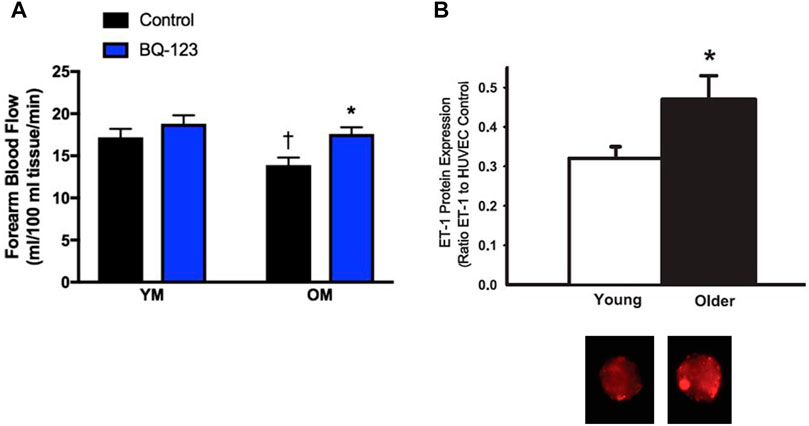
FIGURE 3. ETA-mediated vasoconstriction and increased cellular expression of ET-1 contributes to age-related declines in endothelial function in men. Forearm blood flow responses to acetylcholine were measured in young men (YM) and older men (OM) during saline conditions (control; black bars) or co-infused with the ETA receptor antagonist BQ-123 (blue bars). The age-associated decline in endothelial function was abolished with co-infusion of BQ-123 (A) Graph from Stanhewicz et al. (2018); data from Westby et al. (2011). Endothelial cellular expression of ET-1 (as a ratio of ET-1 to HUVEC control) collected in healthy young and old men. (B, top) and representative images of venous endothelial cell ET-1 expression obtained from brachial arteries in a young and older man (B, bottom) Data from Donato et al. (2009)).
To evaluate the impact of androgens on the vasculature, testosterone treatment has been used in animal models. In a study using aortas from male rabbits, testosterone was found to worsen endothelial function (Hutchison et al., 1997). This effect was also observed during treatment of porcine coronary arteries with testosterone, and further showed augmented vasoconstrictor responses to ET-1 (Teoh et al., 2000). Loss of testosterone through orchidectomy induced a significant increase in ET-1 and ETB receptor expression in the rat portal vein, which was reversed following testosterone replacement, however ETA receptor expression remained unchanged in the presence or absence of testosterone (Rossignoli et al., 2014).
Additional evidence of the impact of testosterone on the ET system is found in cell culture experiments. In human aortic endothelial cells, testosterone increased the number of cells secreting ET-1 and upregulated ET-1 mRNA within 3 h (Pearson et al., 2008). In porcine aortic and human coronary artery endothelial cells testosterone increased ET-1 release in both short term (5 h) and long-term incubation (24 h). Additionally, antagonizing the testosterone receptor using flutamide resulted in attenuated testosterone-mediated ET-1 release (Wilbert-Lampen et al., 2005). However, when haloperidol, a non-selective sigma-1 receptor antagonist was used in combination with testosterone treatment there was significantly less ET-1 produced. Thus, testosterone can act through direct and indirect pathways to increase ET-1 concentrations. The use of hormone therapies in men has been used for conditions such as hypogonadism where low testosterone can be detrimental, however, the use of testosterone therapy with natural aging is more controversial. A recent review of the impact of testosterone on vascular aging can be found here (Moreau et al., 2020).
Pharmacological and Non-Pharmacological Therapies
Given that the ET system contributes to numerous vascular pathologies, its receptors are a target for pharmacological intervention. ET-receptor antagonists (ERA) have been studied in several pathologies; however, the studies were stopped early due to an increase in adverse effects of ERA treatment including increased fluid retention and liver toxicity, with the outcomes falling short of expectations (Ohkita et al., 2012; Dhaun and Webb 2019). Although at least eight small molecule ERA compounds have been developed, only three currently have FDA approval (bosentan, macitentan, and ambrisentan), and are only approved for pulmonary arterial hypertension (PAH). These ERAs are considered a first line therapy for PAH as they can improve symptoms and increase quality of life (Galiè et al., 2008), yet women experience greater benefits to ERAs compared to men (Gabler et al., 2012). Interestingly, the prevalence of PAH is ∼4:1 in women compared to men, and although the survival rate is higher in women, this drops after the age of 60 and is similar to that in men (Mair et al., 2014; Batton et al., 2018). These barriers with ERA efficacy have prompted the development of new, more selective ERAs.
New study designs have helped to increase efficacy by using an enrichment-responder design that identifies participants who demonstrate a response to the drug at low doses and who will potentially be less likely to have an adverse event. The SONAR trial was the first to use this approach in patients with type 2 diabetes and kidney disease, and demonstrated that in responders, Atrasentan, a selective low dose ERA targeting ETA receptors, reduced the risk of renal events (Heerspink et al., 2019). It was noted that there was high variability in the responses to Atrasentan, and in a sub-analysis of the SONAR trial there were sex differences in the pharmacokinetics of Atrasentan such that women had significantly higher area under the plasma concentration curve of plasma Atrasentan compared to men (Koomen et al., 2021). Additionally, there were racial differences observed in both Asian and Black patients compared with North American patients (Koomen et al., 2021). Indeed, given the sexual dimorphism of ET receptor subtype expression and function with aging, the impact of aging and sex on the efficacy of these selective ERA therapeutics should not be ignored.
Novel therapeutics targeting different parts of the ET system as well as novel ways to antagonize receptor function have shown promise in early studies. New methods using immunotherapy to create custom antibodies to ET receptors also show promise for the treatment of PAH. A specific antibody against the ETR-002 epitope of the ETA receptor was able to reduce right ventricle systolic pressure in PAH animals (Dai et al., 2019). Additionally, new molecular constructs, known as ET traps, have been developed which bind and sequester circulating ET-1 with the goal of reducing pathological levels to physiological levels (Jain, Coffey, et al., 2019), and have been shown to work in type 1 diabetics (Jain et al., 2019). These new approaches in drug development, disease selection, and trial design provide opportunities for creating targeted therapies focusing on the ET system.
Exercise
Exercise training also has beneficial effects on health and longevity and can reduce CVD risk. Vascular endothelial function is reduced in middle-aged and older sedentary adults and aerobic exercise training can rescue these impairments. Given the distinct role of ET-1 on age-associated impairments in endothelial function in both men (Westby et al., 2011; Moreau et al., 2020) and women (Tan et al., 2003; Wenner et al., 2017), as well as populations with overt CVD risk factors such as hypertension (Nyberg et al., 2013) or overweight/obesity (Dow et al., 2017), the ability for exercise to reduce ET-1-mediated vascular dysfunction is an important area of study.
Clear evidence demonstrates aerobic exercise training improves ET system function in previously sedentary older men, and similarly, endothelial function is preserved in habitually active older men (DeSouza et al., 2000; Pierce et al., 2011). These findings can be explained, in part, due to the effects of exercise on the endothelin system. In young males, 8 weeks of aerobic exercise training decreased plasma ET-1 concentrations, that was reversed with 8 weeks of detraining (Maeda et al., 2001). Older men who have engaged in lifelong habitual physical activity also appear to have protection against elevations in plasma ET-1 with aging (Nyberg et al., 2013). Moreover, 12 weeks of aerobic exercise training in older sedentary men resulted in a reduction in ETA-mediated vasoconstrictor tone (Van Guilder et al., 2007). These data suggest a favorable effect of aerobic exercise in men to reverse age-related increases in ET-1 system activity and improve or preserve endothelial function with aging.
In women, the impact of aerobic exercise on endothelial function is equivocal, with data showing endothelial function is not consistently preserved (Pierce et al., 2011; Santos-Parker et al., 2017; Gliemann et al., 2020) in habitually active postmenopausal women, or improved after an aerobic training intervention (Pierce et al., 2011; Moreau et al., 2013; Gliemann et al., 2020). Although plasma ET-1 concentration is reduced in older postmenopausal women after aerobic exercise training (Maeda et al., 2003), greater reductions have been observed in premenopausal women (Nyberg et al., 2014). Moreover, the impact of aerobic exercise on ET-1-mediated vasoconstrictor tone in women is not yet known. Determining the sex- and age-specific differences in ET receptor subtype expression and function to modulate endothelial function after aerobic exercise training remains a rich area of research.
E2 is known to modulate the ET system as described above. Recent data indicate that E2 plays an essential role to “transduce” the benefits of exercise on the vasculature in postmenopausal women (Moreau et al., 2013). Therefore, it is likely that E2-deficient postmenopausal women demonstrate a general lack of vascular adaptations to aerobic exercise training compared to men due to loss of endogenous E2 production at menopause (Moreau et al., 2013). These vascular impairments are likely further exacerbated by a concomitant increase in ET-1 system activity and/or dysfunctional ET-1 receptors with age and/or menopause. Identifying windows of opportunity for preventative or early treatment, and optimizing exercise interventions that effectively protect against endothelial impairment, is critical for reducing CVD in women given the stark reduction in vasoprotective sex hormones and endothelial function along with a concomitant rise in prevalence of hypertension and CVD with menopause.
Summary and Future Directions
In summary, sex-specific differences in the ET-1 signaling pathway plays a large role in sex differences observed in endothelial function with aging (Figure 4). In women, aging is associated with a decreased endothelial ETB receptor expression (Kuczmarski et al., 2020) and loss of ETB-mediated vasodilation (Wenner et al., 2017) contributing to endothelial dysfunction (Wenner et al., 2017). These changes in ETB receptor expression and function are likely modulated by sex hormones based off work in cell culture and preclinical animal models, as well as emerging data in humans. In men, aging is associated with overexpression of cellular ET-1 and greater ETA receptor-mediated vasoconstriction, both of which contribute to declines in endothelial function (Figure 4). These sex-specific differences in ET receptor subtype expression and function can modulate ET-1 while also increasing vasoconstrictor tone and exacerbating its pressor effects. However, it remains unclear whether the age-related increases in plasma ET-1 are due to these aforementioned changes in expression of ET-1 or ETA/ETB receptors, or clearance by the ETB receptor, in humans. The local tissue concentrations of ET-1 and expression of ET-1 in VSMC as well as changes in ETA/ETB receptor expression on VSMC in aging men and women is an important area of future investigation. As vascular dysfunction becomes more apparent with aging, the ET system should not be overlooked as an important contributor to sexual dimorphism in the development and progression of age-associated CVD. Moreover, additional work is needed to understand if there is a shift due to aging and/or fluctuations in sex hormones in the different signaling pathways of ETA and ETB receptors.
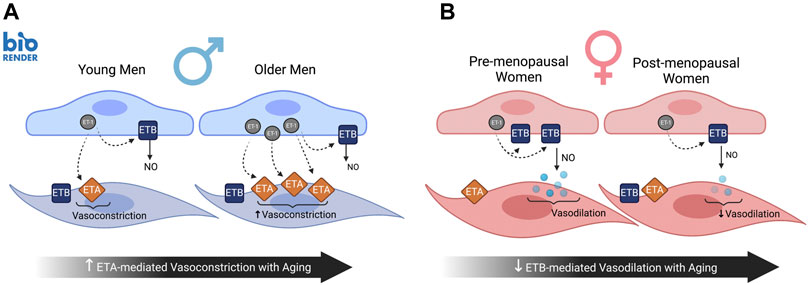
FIGURE 4. Sex differences in ET-1 signaling pathway with aging impacting endothelial function. In men, aging is associated with overexpression of cellular ET-1 and greater ETA receptor mediated vasoconstriction (A), both of which contribute to declines in endothelial function. In contrast, the age-related decline in endothelial function in women is mediated in part by decreased endothelial ETB receptor expression and loss of ETB-mediated vasodilation (B). Created with BioRender.com.
Given the ET system is a critical player in regulating vascular function as well as numerous pathological conditions, the ability to regulate the ET system through lifestyle or pharmacological interventions are valuable therapeutic tools. Finding windows of opportunity for preventative or early treatment is critical to reducing CVD. More research is needed to develop new methods to manipulate production of ET-1 and receptor function as therapeutic targets, since currently approved drugs tend to have numerous side effects. Targeting specific ET receptors based on receptor subtype and tissue location could help to attenuate the ET system and help preserve vascular function without systemic side effects. Lifestyle changes are often first line treatments to prevent, delay, and reverse elevations in CVD risk. ET system activity may be an important mechanism underlying the sex-specific differences in adaptations to aerobic exercise and is an important question that warrants future study. This is critical given that less than 1 in 4 adults report sufficient activity to meet the relevant national physical activity guidelines for both aerobic and muscle strengthening exercise (Gliemann et al., 2020). Finally, understanding the effects of hormone therapy in men and women and the timing of initiating treatment on the ET system may provide valuable information for preventing or delaying the age-associated declines in endothelial function and increased prevalence of CVD.
Author Contributions
AK, LW, KM, and MW contributed to the conception and design of the research; AK, LW, KM, and MW drafted the manuscript; AK, LW, KM, and MW edited and revised the manuscript; AK, LW, KM, and MW approved the final version of the manuscript.
Funding
MW is supported by NIH Grant R01 HL146558.
Conflict of Interest
The authors declare that the research was conducted in the absence of any commercial or financial relationships that could be construed as a potential conflict of interest.
Publisher’s Note
All claims expressed in this article are solely those of the authors and do not necessarily represent those of their affiliated organizations, or those of the publisher, the editors and the reviewers. Any product that may be evaluated in this article, or claim that may be made by its manufacturer, is not guaranteed or endorsed by the publisher.
References
Akishita, M., Kozaki, K., Eto, M., Yoshizumi, M., Ishikawa, M., Toba, K., et al. (1998). Estrogen Attenuates Endothelin-1 Production by Bovine Endothelial Cells via Estrogen Receptor. Biochem. Biophysical Res. Commun. 251 (1), 17–21. doi:10.1006/bbrc.1998.9409
Avedanian, L., Riopel, J., Bkaily, G., Nader, M., D’Orleans-Juste, P., and Jacques, D. (2010). ETA Receptors Are Present in Human Aortic Vascular Endothelial Cells and Modulate Intracellular calciumThis Article Is One of a Selection of Papers Published in the Two-Part Special Issue Entitled 20 Years of Endothelin Research. Can. J. Physiol. Pharmacol. 88 (8), 817–829. doi:10.1139/y10-057
Bachschmid, M. M., Schildknecht, S., Matsui, R., Zee, R., Haeussler, D., A. Cohen, R., et al. (2013). Vascular Aging: Chronic Oxidative Stress and Impairment of Redox Signaling-Consequences for Vascular Homeostasis and Disease. Ann. Med. 45, 17–36. doi:10.3109/07853890.2011.645498
Battistini, B., D'Orléans-Juste, P., and Sirois, P. (1993). Endothelins: Circulating Plasma Levels and Presence in Other Biologic Fluids. Lab. Invest. 68 (6), 600–628.
Batton, K. A., Austin, C. O., Bruno, K. A., Burger, C. D., Shapiro, B. P., and Fairweather, D. (2018). Sex Differences in Pulmonary Arterial Hypertension: Role of Infection and Autoimmunity in the Pathogenesis of Disease. Biol. Sex. Differ. 9 (1), 1–14. doi:10.1186/s13293-018-0176-8
Best, P. J. M., Berger, Peter., Miller, Virginia. M., and Lerman, Amir. (1998). The Effect of Estrogen Replacement Therapy on Plasma Nitric Oxide and Endothelin-1 Levels in Postmenopausal Women. Ann. Intern. Med. 128, 285–288. doi:10.7326/0003-4819-128-4-199802150-00006
Best, P. J. M., and Lerman, A. (2000). Endothelin in Cardiovascular Disease: From Atherosclerosis to Heart Failure. J. Cardiovasc. Pharmacol. 35 (4 Suppl. 2), S61–S63. doi:10.1097/00005344-200000002-00014
Bilsel, A., Moini, H., Tetik, E., Aksungar, F., Kaynak, B., and Ozer, A. (2000). 17β-Estradiol Modulates Endothelin-1 Expression and Release in Human Endothelial Cells. Cardiovasc. Res. 46 (3), 579–584. doi:10.1016/s0008-6363(00)00046-8
Bolton, J. L. (2016). Menopausal Hormone Therapy, Age, and Chronic Diseases: Perspectives on Statistical Trends. Chem. Res. Toxicol. 29 (10), 1583–1590. doi:10.1021/acs.chemrestox.6b00272
Bonetti, P. O., Lerman, L. O., and Lerman, A. (2003). Endothelial Dysfunction. Atvb 23 (2), 168–175. doi:10.1161/01.ATV.0000051384.43104.FC
Bossard, M., Pumpol, K., van der Lely, S., Aeschbacher, S., Schoen, T., Krisai, P., et al. (2015). Plasma Endothelin-1 and Cardiovascular Risk Among Young and Healthy Adults. Atherosclerosis 239 (1), 186–191. doi:10.1016/j.atherosclerosis.2014.12.061
Bremnes, T., Paasche, J. D., Mehlum, A., Sandberg, C., Bremnes, B., and Attramadal, H. (2000). Regulation and Intracellular Trafficking Pathways of the Endothelin Receptors. J. Biol. Chem. 275 (23), 17596–17604. doi:10.1074/jbc.M000142200
Buendgens, L., Yagmur, E., Bruensing, J., Herbers, U., Baeck, C., Trautwein, C., et al. (2017). C-terminal Proendothelin-1 (CT-ProET-1) Is Associated with Organ Failure and Predicts Mortality in Critically Ill Patients. J Intensive Care 5 (1), 1–9. doi:10.1186/s40560-017-0219-y
Cardillo, C., Kilcoyne, C. M., Waclawiw, M., Cannon, R. O., and Panza, J. A. (1999). Role of Endothelin in the Increased Vascular Tone of Patients with Essential Hypertension. Hypertension 33, 753–758. doi:10.1161/01.HYP.0000113293.15186.3b
Cau, S. B. A., Carneiro, F. S., and Tostes, R. C. (2012). Differential Modulation of Nitric Oxide Synthases in Aging: Therapeutic Opportunities. Front. Physio. 3, 1–11. doi:10.3389/fphys.2012.00218
Celermajer, D. S., Sorensen, K. E., Spiegelhalter, D. J., Georgakopoulos, D., Robinson, J., and Deanfield, J. E.. 1994. “Aging Is Associated with Endothelial Dysfunction in Healthy Men Years before the Age-Related Decline in Women”. doi:10.1016/0735-1097(94)90305-0
Çetinkaya Demir, B., Uyar, Y., Özbilgin, K., and Köse, C. (2013). Effect of Raloxifene and Atorvastatin in Atherosclerotic Process in Ovariectomized Rats. J. Obstet. Gynaecol. Res. 39 (1), 229–236. doi:10.1111/j.1447-0756.2012.01969.x
Chao, H.-H., Chen, J. J., Chen, C. H., Lin, H., Cheng, C. F., Lian, W. S., et al. (2005). Inhibition of Angiotensin II Induced Endothelin-1 Gene Expression by 17- -oestradiol in Rat Cardiac Fibroblasts. Heart 91 (5), 664–669. doi:10.1136/hrt.2003.031898
Cockcroft, J., and Mancia, G. (2012). Vascular Aging. J. Hypertens. 30 (Suppl. 1), S1–S2. doi:10.1097/HJH.0b013e328353e4ec
Cramer, H., Müller-Esterl, W., and Schroeder, C. (1997). Subtype-Specific Desensitization of Human Endothelin ETA and ETB Receptors Reflects Differential Receptor Phosphorylation. Biochemistry 36 (43), 13325–13332. doi:10.1021/bi9708848
D'Orléans-Juste, P., Houde, M., Rae, G. A., Bkaily, G., Carrier, E., and Simard, E. (2008). Endothelin-1 (1-31): From Chymase-dependent Synthesis to Cardiovascular Pathologies. Vasc. Pharmacol. 49 (2–3), 51–62. doi:10.1016/j.vph.2008.06.007
D'Orléans-Juste, P., Plante, M., Honoré, J. C., Carrier, E., and Labonté, J. (2003). Synthesis and Degradation of Endothelin-1. Can. J. Physiol. Pharmacol. 81 (6), 503–510. doi:10.1139/y03-032
Dai, Y., Chen, X., Song, X., Chen, X., Ma, W., Lin, J., et al. (2019). Immunotherapy of Endothelin-1 Receptor Type A for Pulmonary Arterial Hypertension. J. Am. Coll. Cardiol. 73 (20), 2567–2580. doi:10.1016/j.jacc.2019.02.067
Daka, B., Olausson, J., Larsson, C. A., Hellgren, M. I., Råstam, L., Jansson, P. A., et al. (2015). Circulating Concentrations of Endothelin-1 Predict Coronary Heart Disease in Women but Not in Men: a Longitudinal Observational Study in the Vara-Skövde Cohort. BMC Cardiovasc. Disord. 15 (1), 1–8. doi:10.1186/s12872-015-0141-y
Davenport, A. P., Hyndman, K. A., Dhaun, N., Southan, C., Kohan, D. E., Pollock, J. S., et al. (2016). Endothelin. Pharmacol. Rev. 68, 357–418. doi:10.1124/pr.115.011833
Davenport, A. P., Ashby, M. J., Easton, P., Ella, S., Bedford, J., Dickerson, C., et al. (1990). A Sensitive Radioimmunoassay Measuring Endothelin-like Immunoreactivity in Human Plasma: Comparison of Levels in Patients with Essential Hypertension and Normotensive Control Subjects. Clin. Sci. 78 (3), 261–264. doi:10.1042/cs0780261
David, F. L., Carvalho, M. H. C., Cobra, A. L. N., Nigro, D., Nigro, D., Fortes, Z. B., et al. (2001). Ovarian Hormones Modulate Endothelin-1 Vascular Reactivity and MRNA Expression in DOCA-Salt Hypertensive Rats. Hypertension 38, 692–696. doi:10.1161/01.hyp.38.3.692
Davidge, S. T. (2001). Prostaglandin H Synthase and Vascular Function. Circ. Res. 89 (8), 650–660. doi:10.1161/HH2001.098351
Del Ry, S., Maltinti, M., Giannessi, D., Cavallini, G., and Bergamini, E. (2008). Age-Related Changes in Endothelin-1 Receptor Subtypes in Rat Heart. Exp. Aging Res. 34 (3), 251–266. doi:10.1080/03610730802070233
DeSouza, C. A., Shapiro, L. F., Clevenger, C. M., Dinenno, F. A., Monahan, K. D., Tanaka, H., et al. (2000). Regular Aerobic Exercise Prevents and Restores Age-Related Declines in Endothelium-dependent Vasodilation in Healthy Men. Circulation 102 (12), 1351–1357. doi:10.1161/01.CIR.102.12.1351
Dhaun, N., and Webb, D. J. (2019). Endothelins in Cardiovascular Biology and Therapeutics. Nat. Rev. Cardiol. 16 (August). doi:10.1038/s41569-019-0176-3
Di Luozzo, G., Bhargava, J., and Powell, R. J. (2000). Vascular Smooth Muscle Cell Effect on Endothelial Cell Endothelin-1 Production. J. Vasc. Surg. 31 (4), 781–789. doi:10.1067/mva.2000.103788
Donato, A. J., Gano, L. B., Eskurza, I., Silver, A. E., Gates, P. E., Jablonski, K., et al. (2009). Vascular Endothelial Dysfunction with Aging: Endothelin-1 and Endothelial Nitric Oxide Synthase. Am. J. Physiol. Heart Circ. Physiol. 297, H425–H432. doi:10.1152/ajpheart.00689.2008.-To
Donato, A. J., Black, A. D., Jablonski, K. L., Gano, L. B., and Seals, D. R. (2008). Aging Is Associated with Greater Nuclear NFκB, Reduced IκBα, and Increased Expression of Proinflammatory Cytokines in Vascular Endothelial Cells of Healthy Humans. Aging Cell 7 (6), 805–812. doi:10.1111/j.1474-9726.2008.00438.x
Donato, A. J., Eskurza, I., Silver, A. E., Pierce, G. L., Seals, D. R., Gates, P. E., et al. (2007). Direct Evidence of Endothelial Oxidative Stress with Aging in Humans. Circ. Res. 100 (11), 1659–1666. doi:10.1161/01.RES.0000269183.13937.e8
Donato, A., Lesniewski, L., and Delp, M. (2005). The Effects of Aging and Exercise Training on Endothelin-1 Vasoconstrictor Responses in Rat Skeletal Muscle Arterioles. Cardiovasc. Res. 66, 393–401. doi:10.1016/j.cardiores.2004.10.023
Donnelly, J. E. D. (2017). Facsm Co-chair, Charles H Hillman, Ph D Co-chair, D Ph, Jennifer L Etnier, etPhysical Activity, Fitness, Cognitive Function, and Academic Achievement in Children: A Systematic Review. Med. Sci. Sports Exerc. 48 (6), 1197–1222. doi:10.1249/MSS.0000000000000901.Physical
Donoso, M. V., Fournier, A., Peschke, H., Faúndez, H., Domenech, R., and Huidobro-Toro, J. P. (1994). Aging Differentially Modifies Arterial Sensitivity to Endothelin-1 and 5-Hydroxytryptamine: Studies in Dog Coronary Arteries and Rat Arterial Mesenteric Bed. Peptides 15 (8), 1489–1495. doi:10.1016/0196-9781(94)90128-7
Dosi, R., Bhatt, N., Shah, P., and Patell, R. (2014). Cardiovascular Disease and Menopause. Jcdr 8 (2), 62–64. doi:10.7860/JCDR/2014/6457.4009
Dow, C. A., Stauffer, B. L. Danielle. L., Brunjes, D. L., Greiner, J. J., and DeSouza, C. A. (2017). Regular Aerobic Exercise Reduces Endothelin-1-Mediated Vasoconstrictor Tone in Overweight and Obese Adults. Exp. Physiol. 102 (9), 1133–1142. doi:10.1113/EP086454
Drion, I., Kleefstra, N., Landman, G. W. D., Alkhalaf, A., Struck, J., Groenier, K. H., et al. (2012). Plasma COOH-Terminal Proendothelin-1: A Marker of Fatal Cardiovascular Events, All-Cause Mortality, and New-Onset Albuminuria in Type 2 Diabetes? (ZODIAC-29). Diabetes Care 35 (11), 2354–2358. doi:10.2337/dc11-2526
Dubey, R. K., Keller, P. J., Imthurn, B., Rosselli, M., and Rosselli, M. (2001). Estradiol Metabolites Inhibit Endothelin Synthesis by an Estrogen Receptor-independent Mechanism. Hypertension 37 (2), 640–644. doi:10.1161/01.hyp.37.2.640K
DuPont, J. J., Kenney, R. M., Patel, A. R., and Jaffe, I. Z. (2019). Sex Differences in Mechanisms of Arterial Stiffness. Br. J. Pharmacol. 176 (21), 4208–4225. doi:10.1111/bph.14624
Dupuis, J., Stewart, D. J., Cernacek, P., and Gosselin, G. (1996). Human Pulmonary Circulation Is an Important Site for Both Clearance and Production of Endothelin-1. Circulation 94 (7), 1578–1584. doi:10.1161/01.cir.94.7.1578
El Melegy, N. T., AliAli, M.-E. M., and Awad, E. M. A. (2005). Plasma Levels of Endothelin-1, Angiotensin II, Nitric Oxide and Prostaglandin E2 in the Venous and Cavernosal Blood of Patients with Erectile Dysfunction. BJU Int. 96 (7), 1079–1086. doi:10.1111/j.1464-410X.2005.05780.x
Elisa, T., Antonio, P., Giuseppe, P., Alessandro, B., Giuseppe, A., Federico, C., et al. (2015). Endothelin Receptors Expressed by Immune Cells Are Involved in Modulation of Inflammation and in Fibrosis: Relevance to the Pathogenesis of Systemic Sclerosis. J. Immunol. Res. 2015, 1–11. doi:10.1155/2015/147616
Ergul, A., Shoemaker, K., Puett, D., and Tackett, R. L. (1998). Gender Differences in the Expression of Endothelin Receptors in Human Saphenous Veins In Vitro. J. Pharmacol. Exp. Ther. 285 (2), 511–517.
Evans, M. J., Harris, H. A., Chris, P., MillerSotirios, K., Miller, C. P., Karathanasis, S. K., et al. (2002). Estrogen Receptors α and β Have Similar Activities in Multiple Endothelial Cell Pathways. Endocrinology 143 (10), 3785–3795. doi:10.1210/en.2002-220356
Fecteau, M.-H., Honore, J.-C., Plante, M., Labonte, J., Rae, G. A., and D’Orleans-Juste, P. (2005). Endothelin-1 (1-31) Is an Intermediate in the Production of Endothelin-1 after Big Endothelin-1 Administration In Vivo. Hypertension 46 (1), 87–92. doi:10.1161/01.HYP.0000170460.24604.23
Folkow, B., and Svanborg, A. (1993). Physiology of Cardiovascular Aging. Physiol. Rev. 73, 725–764. doi:10.1152/physrev.1993.73.4.725
Fredette, N. C., Meyer, M. R., and Prossnitz, E. R. (2018). Role of GPER in Estrogen-dependent Nitric Oxide Formation and Vasodilation. J. Steroid Biochem. Mol. Biol. 176, 65–72. doi:10.1016/j.jsbmb.2017.05.006
Frelin, C., and Guedin, D. (1994). Why Are Circulating Concentrations of Endothelin-1 So Low? Cardiovasc. Res. 28 (11), 1613–1622. doi:10.1093/cvr/28.11.1613
Gabler, N. B., French, B., Strom, B. L., Liu, Z., Palevsky, H. I., Taichman, D. B., et al. (2012). Race and Sex Differences in Response to Endothelin Receptor Antagonists for Pulmonary Arterial Hypertension. Chest 141 (1), 20–26. doi:10.1378/chest.11-0404
Galie, N., Olschewski, H., Oudiz, R. J., Torres, F., Frost, A., Ghofrani, H. A., et al. (2008). Ambrisentan for the Treatment of Pulmonary Arterial Hypertension. Circulation 117 (23), 3010–3019. doi:10.1161/CIRCULATIONAHA.107.742510
George, E. M., and Granger, J. P. (2011). Endothelin: Key Mediator of Hypertension in Preeclampsia. Am. J. Hypertens. 24 (9), 964–969. doi:10.1038/ajh.2011.99
Gliemann, L., Rytter, N., Tamariz-Ellemann, A., Egelund, J., Brandt, N., Carter, H. H., et al. (2020). Lifelong Physical Activity Determines Vascular Function in Late Postmenopausal Women. Med. Sci. Sports Exerc. 52 (3), 627–636. doi:10.1249/MSS.0000000000002180
Gliemann, L., Tamariz-Ellemann, A., Baasch-Skytte, T., Ehlers, T. S., and Gunnarsson, T. P. (2020). Increased Prostacyclin Formation after High-Intensity Interval Training in Late Postmenopausal Women. Eur. J. Appl. Physiol. 120, 1711–1720. doi:10.1007/s00421-020-04405-6
Goel, A., Su, B., Flavahan, S., Lowenstein, C. J., Berkowitz, D. E., Flavahan, N. A., et al. (2010). Increased Endothelial Exocytosis and Generation of Endothelin-1 Contributes to Constriction of Aged Arteries. Circ. Res. 107 (2), 242–251. doi:10.1161/CIRCRESAHA.109.210229
Gohar, E. Y., Daugherty, E. M., Aceves, J. O., Sedaka, R., Obi, I. E. J., Allan, J. M., et al. (2020). Evidence for G‐Protein-Coupled Estrogen Receptor as a Pronatriuretic Factor. Jaha 9 (10), e015110. doi:10.1161/JAHA.119.015110
Gohar, E. Y., and Pollock, D. M. (2018). Sex-Specific Contributions of Endothelin to Hypertension. Curr. Hypertens. Rep. 20 (7). doi:10.1007/s11906-018-0856-0
Gohar, E. Y., Yusuf, C., and Pollock, D. M. (2016). Ovarian Hormones Modulate Endothelin A and B Receptor Expression. Life Sci. 159 (August), 148–152. doi:10.1016/j.lfs.2016.01.010
Granado, M., Rubio, C., Amor, S., Monge, L., Fernández, N., Carreño-Tarragona, G., et al. (2014). Effects of Age and Caloric Restriction on the Cardiac and Coronary Response to Endothelin-1 in Rats. Exp. Gerontol. 60, 183–189. doi:10.1016/j.exger.2014.10.018
Haak, T., März, W., Jungmann, E., Hausser, S., Siekmeier, R., Gross, W., et al. (1994). Elevated Endothelin Levels in Patients with Hyperlipoproteinemia. Clin. Investig. 72 (8), 580–584. doi:10.1007/BF00227448
Habib, A., Al-Omari, M. A., Khaleghi, M., Morgenthaler, N. G., Struck, J., Bergmann, A., et al. (2010). Plasma C-Terminal Pro-endothelin-1 Is Associated with Target-Organ Damage in African Americans with Hypertension. Am. J. Hypertens. 23 (11), 1204–1208. doi:10.1038/ajh.2010.149
Haug, C., Voisard, R., Lenich, A., Baur, R., Höher, M., Osterhues, H., et al. (1996). Increased Endothelin Release by Cultured Human Smooth Muscle Cells from Atherosclerotic Coronary Arteries. Cardiovasc. Res. 31 (5), 807–813. doi:10.1016/0008-6363(96)00012-0
Haynes, W., and Webb, David. J. (1994). Contribution of Endogenous Generation of Endothelin-1 to Basal Vascular Tone. The Lancet 344 (8926), 852–854. doi:10.1016/s0140-6736(94)92827-4
Heerspink, H. J. L., Parving, H. H., Parving, H. H., Andress, D. L., Bakris, G., Correa-Rotter, R., et al. (2019). Atrasentan and Renal Events in Patients with Type 2 Diabetes and Chronic Kidney Disease (SONAR): A Double-Blind, Randomised, Placebo-Controlled Trial. Lancet 393 (10184), 1937–1947. doi:10.1016/S0140-6736(19)30772-X
Hirai, Y., Adachi, H., Fujiura, Y., Hiratsuka, A., Enomoto, M., and Imaizumi, T. (2004). Plasma Endothelin-1 Level Is Related to Renal Function and Smoking Status but Not to Blood Pressure. J. Hypertens. 22 (4), 713–718. doi:10.1097/00004872-200404000-00013
Hirata, Y., Emori, T., Eguchi, S., Kanno, K., Imai, T., Ohta, K., et al. (1993). Endothelin Receptor Subtype B Mediates Synthesis of Nitric Oxide by Cultured Bovine Endothelial Cells. J. Clin. Invest. 91 (4), 1367–1373. doi:10.1172/JCI116338
Homma, S., Miyauchi, T., Sugishita, Y., Goto, K., Sato, M., and Ohshima, N. (1992). Vasoconstrictor Effects of Endothelin-1 on Myocardium Microcirculation Studied by the Langendorff Perfusion Method: Differential Sensitivities Among Microvessels. Microvasc. Res. 43 (2), 205–217. doi:10.1016/0026-2862(92)90017-j
Horstmeyer, A., Cramer, H., Sauer, T., Müller-Esterl, W., and Schroeder, C. (1996). Palmitoylation of Endothelin Receptor A. J. Biol. Chem. 271 (34), 20811–20819. doi:10.1074/jbc.271.34.20811
Houde, M., Desbiens, L., and D’Orléans-Juste, P. (2016). Endothelin-1. Adv. Pharmacol. 77, 143–175. doi:10.1016/bs.apha.2016.05.002
Hutchison, S. J., Sudhir, K., Chou, T. M., Sievers, R. E., Zhu, B. Q., Sievers, R. E., et al. (1997). Testosterone Worsens Endothelial Dysfunction Associated with Hypercholesterolemia and Environmental Tobacco Smoke Exposure in Male Rabbit Aorta 11All Editorial Decisions for This Article, Including Selection of Referees, Were Made by a Guest Editor. This Policy Applies to All Articles with Authors from the University of California San Francisco. J. Am. Coll. Cardiol. 29 (4), 800–807. doi:10.1016/S0735-1097(96)00570-0
Huxley, V. H., and Kemp, S. S. (2018). Sex-Specific Characteristics of the Microcirculation. Adv. Exp. Med. Biol. 1065, 307–328. doi:10.1007/978-3-319-77932-4
Ibrahim, N. E., Shrestha, S., McCarthy, C., Lyass, A., Li, Y., Gaggin, H., et al. (2018). Endothelin-1 Predicts Incident Heart Failure, Incident Myocardial Infarction, Cardiovascular Mortality, and All-Cause Mortality in Patients Undergoing Diagnostic Coronary Angiography: Results from the Catheter Sampled Blood Archive in Cardiovascular Diseases (Casablanca) Study. J. Am. Coll. Cardiol. 71 (11), A773. doi:10.1016/S0735-1097(18)31314-7
Iglarz, M., and Clozel, M. (2007). Mechanisms of ET-1-Induced Endothelial Dysfunction. J. Cardiovasc. Pharmacol. 50, 621–628. doi:10.1161/CIRCULATIONAHA.111.078824
Ihling, C., Szombathy, T., Bohrmann, B., Brockhaus, M., Schaefer, H. E., Loeffler, B. M., et al. (2001). Coexpression of Endothelin-Converting Enzyme-1 and Endothelin-1 in Different Stages of Human Atherosclerosis. Circulation 104 (8), 864–869. doi:10.1161/hc3301.094742
Iorga, A., Moazeni, S., Ruffenach, G., Umar, S., Eghbali, M., and Eghbali, M. (2017). The Protective Role of Estrogen and Estrogen Receptors in Cardiovascular Disease and the Controversial Use of Estrogen Therapy. Biol. Sex. Differ. 8 (1), 33. doi:10.1186/s13293-017-0152-8M
Ivey, M. E., Osman, N., and Little, P. J. (2008). Endothelin-1 Signalling in Vascular Smooth Muscle: Pathways Controlling Cellular Functions Associated with Atherosclerosis. Atherosclerosis 199, 237–247. doi:10.1016/j.atherosclerosis.2008.03.006
Iwasa, S., Fan, J., Shimokama, T., Nagata, M., and Watanabe, T. (1999). Increased Immunoreactivity of Endothelin-1 and Endothelin B Receptor in Human Atherosclerotic Lesions. A Possible Role in Atherogenesis. Atherosclerosis 146, 93–100. doi:10.1016/s0021-9150(99)00134-3
Jain, A., Coffey, C., Mehrotra, V., and Flammer, J. (2019). Endothelin-1 Traps as a Potential Therapeutic Tool: From Diabetes to beyond? Drug Discov. Today 24 (00), 1937–1942. doi:10.1016/j.drudis.2019.07.008
Jain, A., Mehrotra, V., Jha, I., and Jain, A. (2019). In Vivo Studies Demonstrate that Endothelin-1 Traps Are a Potential Therapy for Type I Diabetes. J. Diabetes Metab. Disord. 18 (1), 133–143. doi:10.1007/s40200-019-00400-7
Johnström, P., Fryer, T. D., Richards, H. K., Harris, N. G., Barret, O., Clark, J. C., et al. (2005). Positron Emission Tomography Using 18 F-Labelled Endothelin-1 Reveals Prevention of Binding to Cardiac Receptors Owing to Tissue-specific Clearance by ETB Receptors In Vivo. Br. J. Pharmacol. 144 (1), 115–122. doi:10.1038/sj.bjp.0706064
Juan, S.-H., Chen, J.-J., Chen, C.-H., Lin, H., Cheng, C.-F. Ju. Chi., Liu, J.-C., et al. (2004). 17β-Estradiol Inhibits Cyclic Strain-Induced Endothelin-1 Gene Expression within Vascular Endothelial Cells. Am. J. Physiology-Heart Circulatory Physiol. 287 (3 56-3), H1254–H1261. doi:10.1152/ajpheart.00723.2003
Kakoki, M., Hirata, Y., Hayakawa, H., Tojo, A., Nagata, D., Suzuki, E., et al. (1999). Effects of Hypertension, Diabetes Mellitus, and Hypercholesterolemia on Endothelin Type B Receptor-Mediated Nitric Oxide Release from Rat Kidney. Circulation 99, 1242–1248. doi:10.1161/01.cir.99.9.1242
Kanse, S. M., Takahashi, K., Warren, J. B., Ghatei, M., and Bloom, S. R. (1991). Glucocorticoids Induce Endothelin Release from Vascular Smooth Muscle Cells but Not Endothelial Cells. Eur. J. Pharmacol. 199 (1), 99–101. doi:10.1016/0014-2999(91)90641-3
Kanse, S. M., Takahashi, K., Warren, J. B., Perera, T., Porta, M., Ghatei, M., et al. (1991). Production of Endothelin by Vascular Smooth Muscle Cells. J. Cardiovasc. Pharmacol. 17, S113–S116. doi:10.1097/00005344-199100177-00029
Kayashima, K., Doi, Y., Kudo, H., Kiyonaga, H., and Fujimoto, S. (1999). Effects of Endothelin-1 on Vasoactivity and its Synthesis, Storage, and Acting Sites in the Rat Superior Mesenteric Vasculature: An Ultrastructural and Immunocytochemical Study. Med. Electron Microsc. 32 (1), 36–42. doi:10.1007/s007950050006
Kelland, N. F., Kuc, R. E. D. L, McLean, D. L., AzferGulliver-Sloan, A. F. H., Bagnall, A. J., Gray, G. A., et al. (2010). Endothelial Cell-specific ETB Receptor Knockout: Autoradiographic and Histological Characterisation and Crucial Role in the Clearance of endothelin-1This Article Is One of a Selection of Papers Published in the Two-Part Special Issue Entitled 20 Years of Endothelin Research. Can. J. Physiol. Pharmacol. 88 (6), 644–651. doi:10.1139/Y10-041
Kellogg, D. L., Liu, Y., and Pérgola, P. E. (2001). Selected Contribution: Gender Differences in the Endothelin-B Receptor Contribution to Basal Cutaneous Vascular Tone in Humans. J. Appl. Physiol. 91 (5), 2407–2411. doi:10.1152/jappl.2001.91.5.2407
Khalil, R. A. (2011). Modulators of the Vascular Endothelin Receptor in Blood Pressure Regulation and Hypertension. Cmp 4 (3), 176–186. doi:10.2174/1874467211104030176
Khatib, A. M., Lomri, A., Mitrovic, R. D., and Moldovan, F. (2007). Articular Chondrocyte Aging and Endothelin-1. Cytokine 37 (1), 6–13. doi:10.1016/j.cyto.2007.02.005
Khimji, A.-k., and Rockey, D. C. (2010). Endothelin-Biology and Disease. Cell Signal. 22 (11), 1615–1625. doi:10.1016/j.cellsig.2010.05.002
Kitada, K., Yui, N., Mori, T., Ohkita, M., and Matsumura, Y. (2014). Vasoprotective Effects of an Endothelin Receptor Antagonist in Ovariectomized Female Rats. Life Sci. 118 (2), 379–385. doi:10.1016/j.lfs.2014.01.009
Kittikulsuth, W., Sullivan, J., and Pollock, D. (2013). ET-1 Actions in the Kidney: Evidence for Sex Differences. Br. J. Pharmacol. 168 (2), 318–326. doi:10.1111/j.1476-5381.2012.01922.x
Kolettis, T. M., Barton, M., Langleben, D., and Matsumura, Y. (2013). Endothelin in Coronary Artery Disease and Myocardial Infarction. Cardiol. Rev. 21 (5), 249–256. doi:10.1097/CRD.0b013e318283f65a
Koomen, J. V., Stevens, J., Bakris, G., Correa‐Rotter, R., Hou, F. F., Kitzman, D. W., et al. (2021). Inter‐individual Variability in Atrasentan Exposure Partly Explains Variability in Kidney protection and Fluid Retention Responses: A Post Hoc Analysis of the SONAR Trial. Diabetes Obes. Metab. 23 (2), 561–568. doi:10.1111/dom.14252
Kuczmarski, A. V., Shoemaker, L. N., Hobson, J. C., Edwards, D. G., and Wenner, M. M. (2020). Altered Endothelial ETB Receptor Expression in Postmenopausal Women. Am. J. Physiology-Heart Circulatory Physiol. 319 (1), H242–H247. doi:10.1152/ajpheart.00342.2020
Kumazaki, T., Fujii, T., Kobayashi, M., and Mitsui, Y. (1994). Aging- and Growth-dependent Modulation of Endothelin-1 Gene Expression in Human Vascular Endothelial Cells. Exp. Cel. Res. 211 (1), 6–11. doi:10.1006/excr.1994.1051
Kuruppu, S., Reeve, S., and Ian Smith, A. (2007). Characterisation of Endothelin Converting Enzyme-1 Shedding from Endothelial Cells. FEBS Lett. 581 (23), 4501–4506. doi:10.1016/J.FEBSLET.2007.08.028
Lerman, A., Edwards, B. S., Hallett, J. W., Heublein, D. M., Sandberg, S. M., and Burnett, J. C. (1991). Circulating and Tissue Endothelin Immunoreactivity in Advanced Atherosclerosis. N. Engl. J. Med. 325 (14), 997–1001. doi:10.1056/NEJM199110033251404
Lerman, A., Holmes, D. R., Bell, M. R., Garratt, K. N., Nishimura, R. A., and Burnett, J. C. (1995). Endothelin in Coronary Endothelial Dysfunction and Early Atherosclerosis in Humans. Circulation 92 (9), 2426–2431. doi:10.1161/01.CIR.92.9.2426
Leslie, S. J., Johnston, N., Strachan, F. E., Bagnall, A., Gray, G. A., Newby, D. E., et al. (2004). Endothelin-1[1-31] Is Not Elevated in Men with Chronic Heart Failure. J. Cardiovasc. Pharmacol. 44 (Suppl. 1), S96–S99. doi:10.1097/01.fjc.0000166233.11336.67
Leslie, S. J., Rahman, M. Q., Denvir, M. A., Newby, D. E., and Webb, D. J. (2004). Endothelins and Their Inhibition in the Human Skin Microcirculation: ET[1-31], a New Vasoconstrictor Peptide. Br. J. Clin. Pharmacol. 57 (6), 720–725. doi:10.1111/j.1365-2125.2004.02074.x
Lin, C.-C., Hsieh, H.-L., Chi, P.-L., Hsiao, L.-D., and Yang, C. M. (2014). Upregulation of COX-2/PGE2 by ET-1 Mediated through Ca2+-dependent Signals in Mouse Brain Microvascular Endothelial Cells. Mol. Neurobiol. 49 (3), 1256–1269. doi:10.1007/s12035-013-8597-1
Lorenz, M., Blaschke, B., Benn, A., Hammer, E., Witt, E., Kirwan, J., et al. (2019). Sex-Specific Metabolic and Functional Differences in Human Umbilical Vein Endothelial Cells from Twin Pairs. Atherosclerosis 291 (October), 99–106. doi:10.1016/j.atherosclerosis.2019.10.007
Maeda, S., Miyauchi, T., Kakiyama, T., Sugawara, J., Iemitsu, M., Irukayama-Tomobe, Y., et al. (2001). Effects of Exercise Training of 8 Weeks and Detraining on Plasma Levels of Endothelium-Derived Factors, Endothelin-1 and Nitric Oxide, in Healthy Young Humans. Life Sci. 69 (9), 1005–1016. doi:10.1016/S0024-3205(01)01192-4
Maeda, S., Tanabe, T., Miyauchi, T., Otsuki, T., Sugawara, J., Iemitsu, M., et al. (2003). Aerobic Exercise Training Reduces Plasma Endothelin-1 Concentration in Older Women. J. Appl. Physiol. 95, 336–341. doi:10.1152/japplphysiol.01016.2002.-Endothelial
Maguire, J., and Davenport, A. P. (2004). Alternative Pathway to Endothelin-Converting Enzyme for the Synthesis of Endothelin in Human Blood Vessels. J. Cardiovasc. Pharmacol. 44 (Suppl. 1), S27–S29. doi:10.1097/01.fjc.0000166219.65593.af
Maguire, J. J., Kuc, R. E., and Davenport, A. P. (2001). Vasoconstrictor Activity of Novel Endothelin Peptide, ET-1(1 - 31), in Human Mammary and Coronary Arteriesin Vitro. Br. J. Pharmacol. 134 (6), 1360–1366. doi:10.1038/sj.bjp.0704384
Mair, K. M., Johansen, A. K. Z., Wright, A. F., Wallace, E., and Maclean, M. R. (2014). Pulmonary Arterial Hypertension: Basis of Sex Differences in Incidence and Treatment Response. Br. J. Pharmacol. 171 (3), 567–579. doi:10.1111/bph.12281
Maron, B. A., Zhang, Y.-Y., White, K., Chan, S. Y., Mahoney, C. E., Loscalzo, J., et al. (2012). Aldosterone Inactivates the Endothelin-B Receptor via a Cysteinyl Thiol Redox Switch to Decrease Pulmonary Endothelial Nitric Oxide Levels and Modulate Pulmonary Arterial Hypertension. Circulation 126 (8), 963–974. doi:10.1161/CIRCULATIONAHA.112.094722E
Maurer, M., Wedemeyer, J., Metz, M., Weller, K., Chatterjea, D., Clouthier, D. E., et al. (2004). Mast Cells Promote Homeostasis by Limiting Endothelin-1-Induced Toxicity. Nature 432 (7016), 512–516. doi:10.1038/nature03085M
McEniery, C. M., Yasmin, I. R. H., Hall, I. R., Qasem, A., Wilkinson, I. B., and Cockcroft, J. R. (2005). Normal Vascular Aging: Differential Effects on Wave Reflection and Aortic Pulse Wave Velocity. J. Am. Coll. Cardiol. 46 (9), 1753–1760. doi:10.1016/j.jacc.2005.07.037
Meyer, M. R., Field, A. S., Kanagy, N. L., Barton, M., and Prossnitz, E. R. (2012). GPER Regulates Endothelin-dependent Vascular Tone and Intracellular Calcium. Life Sci. 91 (13–14), 623–627. doi:10.1016/j.lfs.2012.01.007
Mikkola, T., Viinikka, L., and Ylikorkala, O. (1998). Estrogen and Postmenopausal Estrogen/Progestin Therapy: Effect on Endothelium-dependent Prostacyclin, Nitric Oxide and Endothelin-1 Production. Eur. J. Obstet. Gynecol. Reprod. Biol. 79 (1), 75–82. doi:10.1016/S0301-2115(98)00050-5
Miyauchi, T., Yanagisawa, M., Iida, K., Ajisaka, R., Suzuki, N., Fujino, M., et al. (1992). Age- and Sex-Related Variation of Plasma Endothelin-1 Concentration in Normal and Hypertensive Subjects. Am. Heart J. 123 (4), 1092–1093. doi:10.1300/j123v23n03_2210.1016/0002-8703(92)90734-d
Moreau, K. L., Babcock, M. C., and Hildreth, K. L. (2020). Sex Differences in Vascular Aging in Response to Testosterone. Biol. Sex. Differ. 11 (1), 1–14. doi:10.1186/s13293-020-00294-8
Moreau, K. L., Stauffer, B. L., Seals, D. R., and Douglas, W. M. (2013). Essential Role of Estrogen for Improvements in Vascular Endothelial Function with Endurance Exercise in Postmenopausal Women. J. Clin. Endocrinol. Metab. 98, 4507–4515. doi:10.1210/jc.2013-2183
Morey, K. A., Razandi, M., Pedram, A., Hu, R.-M., Prins, A. B., Levin, R. E., et al. (1998). Oestrogen and Progesterone Inhibit the Stimulated Production of Endothelin-1. Biochem. J. 330 (3), 1097–1105. doi:10.1042/bj3301097
Muller-Delp, J. M. (2013). The Coronary Microcirculation in Health and Disease. ISRN Physiol. 22 (2), 221–231. doi:10.1016/j.ccl.2004.02.00510.1155/2013/238979
Naftolin, F., Friedenthal, J., Nachtigall, R., and Nachtigall, L. (2019). Cardiovascular Health and the Menopausal Woman: the Role of Estrogen and when to Begin and End Hormone Treatment. F1000Res 8, 1576. doi:10.12688/f1000research.15548.1
Nilsson, D., Wackenfors, A., Gustafsson, L. L. Edvinsson., Edvinsson, L., Paulsson, P., Ingemansson, R., et al. (2008). Increased ETA and ETB Receptor Contraction in the Left Internal Mammary Artery from Patients with Hypertension. J. Hum. Hypertens. 22 (3), 226–229. doi:10.1038/sj.jhh.1002317
Nuedling, S., Eickels, M. v., Alle´ra, A. A., Alléra, A., Doevendans, P., Meyer, R., et al. (2003). 17β -Estradiol Regulates the Expression of Endothelin Receptor Type B in the Heart. Br. J. Pharmacol. 140, 195–201. doi:10.1038/sj.bjp.0705409
Nyberg, M., Seidelin, K., Andersen, T. R., Overby, N. N., Andersen, T. R., Overby, N. N., et al. (2014). Biomarkers of Vascular Function in Premenopausal and Recent Postmenopausal Women of Similar Age: Effect of Exercise Training. Am. J. Physiol. Regul. Integr. Comp. Physiol. 306, R510–R517. doi:10.1152/ajpregu.00539.2013.-Menopause
Nyberg, M., Mortensen, S. P., and Hellsten, Y. (2013). Physical Activity Opposes the Age-Related Increase in Skeletal Muscle and Plasma Endothelin-1 Levels and Normalizes Plasma Endothelin-1 Levels in Individuals with Essential Hypertension. Acta Physiol. 207 (3), 524–535. doi:10.1111/apha.12048
Ohkita, M., Tawa, M., Kitada, K., and Matsumura, Y. (2012). Pathophysiological Roles of Endothelin Receptors in Cardiovascular Diseases. J. Pharmacol. Sci. 119 (4), 302–313. doi:10.1254/jphs.12r01cr
Ortman, J. M., Velkoff, V., and Howard, H. (2014). “An Aging Nation: The Older Population in the United States.” Economics and Statistics Administration. US Department of Commerce, 1–28. Available at: https://www.census.gov/prod/2014pubs/p25-1140.pdf.
Parker, J. D., and Thiessen, J. J. (2004). Increased Endothelin-1 Production in Patients with Chronic Heart Failure. Am. J. Physiology-Heart Circulatory Physiol. 286 (3), H1141–H1145. doi:10.1152/ajpheart.00239.2001
Pearson, L. J., Yandle, T. G., Nicholls, M. G., and Evans, J. J. (2008). Regulation of Endothelin-1 Release from Human Endothelial Cells by Sex Steroids and Angiotensin-II. Peptides 29 (6), 1057–1061. doi:10.1016/j.peptides.2008.02.003
Pedersen, S. H., Nielsen, L. B., Nina, G. P., Pedersen, N. G., Nilas, L., and Ottesen, B. (2009). Hormone Therapy Modulates ETAmRNA Expression in the Aorta of Ovariectomised New Zealand White Rabbits. Gynecol. Endocrinol. 25 (3), 175–182. doi:10.1080/09513590802549833
Peto, H., Corder, R., Janes, R. W., and Wallace, B. A. (1996). A Molecular Model for Human Big-Endothelin-1 (Big ET-1). FEBS Lett. 394 (2), 191–195. doi:10.1016/0014-5793(96)00951-9
Piatti, P. M., Monti, L. D., Conti, M., Baruffaldi, L., Galli, L., Phan, C. V., et al. (1996). Hypertriglyceridemia and Hyperinsulinemia Are Potent Inducers of Endothelin-1 Release in Humans. Diabetes 45 (3), 316–321. doi:10.2337/diab.45.3.31610.2337/diabetes.45.3.316
Pierce, G. L., Donato, A. J., LaRocca, T. J., Eskurza, I., Silver, A. E., and Seals, D. R. (2011). Habitually Exercising Older Men Do Not Demonstrate Age-Associated Vascular Endothelial Oxidative Stress. Aging Cell 10 (6), 1032–1037. doi:10.1111/j.1474-9726.2011.00748.x
Polderman, K. H., Stehouwer, Coen. D. A., Van Kamp, Gerard. J., van Kamp, G. J., Dekker, G. A., Verheugt, F. W., et al. (1993). Influence of Sex Hormones on Plasma Endothelin Levels. Ann. Intern. Med. 118 (6), 429–432. doi:10.7326/0003-4819-118-6-199303150-00006
Rodrigo, M. C., Martin, D. S., and Eyster, K. M. (2003). Vascular ECE-1 MRNA Expression Decreases in Response to Estrogens. Life Sci. 73 (23), 2973–2983. doi:10.1016/j.lfs.2003.05.001Martin
Rossignoli, P. d. S., De Labio, R. W., Payão, S. L. M., Pereira, O. C. M., and Chies, A. B. (2014). Orchidectomy Enhances the Expression of Endothelin-1 and ETB Receptors in Rat Portal Vein. J. Smooth Muscle Res. 50 (1), 85–92. doi:10.1540/jsmr.50.85
Russell, F. D., Coppell, A. L., and Davenport, A. P. (1998). In Vitro Enzymatic Processing of Radiolabelled Big ET-1 in Human Kidney. Biochem. Pharmacol. 55 (5), 697–701. doi:10.1016/s0006-2952(97)00515-7
Russell, F. D., Skepper, J. N., and Davenport, A. P. (1998). Human Endothelial Cell Storage Granules. Circ. Res. 83, 314–321. doi:10.1161/01.res.83.3.314
Safar, M. E. (2018). Arterial Stiffness as a Risk Factor for Clinical Hypertension. Nat. Rev. Cardiol. 15, 97–105. doi:10.1038/nrcardio.2017.155
Santos-Parker, J. R., Strahler, T. R., Vorwald, V. M., Pierce, G. L., Seals, D. R., and Douglas, R. (2017). Habitual Aerobic Exercise Does Not Protect against Micro- or Macrovascular Endothelial Dysfunction in Healthy Estrogen-Deficient Postmenopausal Women. J. Appl. Physiol. 122 (1), 11–19. doi:10.1152/japplphysiol.00732.2016
Schnabel, R. B., Biener, M. P., Wilde, S., Sinning, C. R., Ojeda, F. M., Zeller, T., et al. (2013). Sex Differences in Noninvasive Vascular Function in the Community. J. Hypertens. 31 (7), 1437–1446. doi:10.1097/HJH.0b013e328360f755
Schweizer, A., Valdenaire, O., Nelböck, P., Deuschle, U., Dumas Milne Edwards, J.-B., Stumpf, J. G., et al. (1997). Human Endothelin-Converting Enzyme (ECE-1): Three Isoforms with Distinct Subcellular Localizations. Biochem. J. 328 (Pt 33), 871–877. doi:10.1042/bj3280871
Seals, D. R., Jablonski, K. L., and Donato, A. J. (2011). Aging and Vascular Endothelial Function in Humans. Clin. Sci. NIH Public Access 120, 357–375. doi:10.1042/CS20100476
Sebzda, K. N., Kuczmarski, A. V., Pohlig, R. T., Shannon, L. L., David, G. E., Edwards, D. G., et al. (2018). Ovarian Hormones Modulate Endothelin-1 Receptor Responses in Young Women. Microcirculation 25, e12490. doi:10.1111/micc.12490
Seed, A., Kuc, R. E., Maguire, J. J., Hillier, C., Johnston, F., Essers, H., et al. (2012). The Dual Endothelin Converting Enzyme/Neutral Endopeptidase Inhibitor SLV-306 (Daglutril), Inhibits Systemic Conversion of Big Endothelin-1 in Humans. Life Sci. 91 (13–14), 743–748. doi:10.1016/J.LFS.2012.03.022
Shihoya, W., Nishizawa, T., Okuta, A., Tani, K., Dohmae, N., Fujiyoshi, Y., et al. (2016). Activation Mechanism of Endothelin ETB Receptor by Endothelin-1. Nature 537, 363–368. doi:10.1038/nature19319
Shinagawa, S., Okazaki, T., Ikeda, M., Yudoh, K., Kisanuki, Y. Y., Yanagisawa, M., et al. (2017). T Cells upon Activation Promote Endothelin 1 Production in Monocytes via IFN-γ and TNF-α. Sci. Rep. 7 (1), 1–8. doi:10.1038/s41598-017-14202-5
Smith, P. J. W., Ornatsky, O., Stewart, D. J., Picard, P., Dawood, F., Wen, W.-H., et al. (2000). Effects of Estrogen Replacement on Infarct Size, Cardiac Remodeling, and the Endothelin System after Myocardial Infarction in Ovariectomized Rats. Circulation 102 (24), 2983–2989. doi:10.1161/01.CIR.102.24.2983
Stanhewicz, A. E., Wenner, M. M., and Stachenfeld, N. S. (2018). Sex Differences in Endothelial Function Important to Vascular Health and Overall Cardiovascular Disease Risk across the Lifespan. Am. J. Physiology-Heart Circulatory Physiol. 315 (6), H1569–H1588. doi:10.1152/ajpheart.00396.2018
Stauffer, B. L., Westby, C. M., Greiner, J. J., Van Guilder, G. P., and Desouza, C. A. (2010). Sex Differences in Endothelin-1-Mediated Vasoconstrictor Tone in Middle-Aged and Older Adults. Am. J. Physiology-Regulatory, Integr. Comp. Physiol. 298 (2), R261–R265. doi:10.1152/ajpregu.00626.2009
Sud, N., and Black, S. M. (2009). Endothelin-1 Impairs Nitric Oxide Signaling in Endothelial Cells through a Protein Kinase Cδ-dependent Activation of STAT3 and Decreased Endothelial Nitric Oxide Synthase Expression. DNA Cel. Biol. 28 (11), 543–553. doi:10.1089/dna.2009.0865
Sudhir, K., Ko, E., Zellner, C., Wong, H. E., and Hutchison, S. J. (1997). Physiological Concentrations of Estradiol Attenuate Endothelin 1-Induced Coronary Vasoconstriction In Vivo. Circulation 96 (10), 3626–3632. doi:10.1161/01.CIR.96.10.3626
Suzaki, Y., Yoshizumi, M., Yamashita, Y., Abe, S., Tsuchiya, K., Satoh, Y., et al. (2003). Determination of Plasma Concentrations of Endothelin-1(1-31) and Endothelin-1 in Healthy Subjects and Patients with Atherosclerosis. J. Cardiovasc. Pharmacol. 41 (Suppl. 1), S83–S87.
Sverdlov, A. L., Ngo, D. T. M., Chan, W. P. A., Yuliy, Y., Horowitz, J. D., and Horowitz, Y. Y. (2014). Aging of the Nitric Oxide System: Are We as Old as Our NO? Jaha 3 (4). doi:10.1161/JAHA.114.000973
Szewieczek, J., Francuz, T., Dulawa, J., Legierska, K., Hornik, B., Włodarczyk, I., et al. (2015). Functional Measures, Inflammatory Markers and Endothelin-1 as Predictors of 360-Day Survival in Centenarians. Age 37 (5). doi:10.1007/s11357-015-9822-9
Tan, Z., Wang, T. H., Chen, Y., Lin, G. P., and Pan, J. Y. (2007). Role of NO Signal Pathway in the Inhibitory of 17beta-Estradiol on the Production of Endothelin-1 in Vascular Smooth Muscle Cells. Zhongguo Ying Yong Sheng Li Xue Za Zhi 23 (3), 347–350.
Tan, Z., Wang, T.-H., Yang, D., Fu, X.-D., and Pan, J.-Y. (2003). Mechanisms of 17β-Estradiol on the Production of ET-1 in Ovariectomized Rats. Life Sci. 73 (21), 2665–2674. doi:10.1016/S0024-3205(03)00605-2
Teoh, H., Quan, A., Leung, S. W. S., and ManMan., R. Y. K. (2000). Differential Effects of 17β -estradiol and Testosterone on the Contractile Responses of Porcine Coronary Arteries. Br. J. Pharmacol. 129 (7), 1301–1308. doi:10.1038/sj.bjp.0703164
Thijssen, D. H. J., Rongen, G. A., van Dijk, A., Smits, P., and Hopman, M. T. E. (2007). Enhanced Endothelin-1-Mediated Leg Vascular Tone in Healthy Older Subjects. J. Appl. Physiol. 103 (September), 852–857. doi:10.1152/japplphysiol.00357.2007
Ting-Huai, W., Tan, Z., Pei-Quing, L., Lu, W., Yang, D., and Pan, J-Y. (2001). Down-Regulation of ETA Receptor of Vascular Smooth Muscle Cells by 17b-Estradiol. Acta Physiol. Sincia 43.
Tokunaga, O., Fan, J., Watanabe, T., Kobayashi, M., Kumazaki, T., and Mitsui, Y. (1992). Endothelin. Immunohistologic Localization in Aorta and Biosynthesis by Cultured Human Aortic Endothelial Cells. Lab. Invest. 67 (2), 210–217.
Tostes, R. C., Fortes, Z. B., Montezano, A. C., Touyz, R. M., and Webb, R. C. (2008). Endothelin, Sex and Hypertension. Clin. Sci. 114, 85–97. doi:10.1042/CS20070169
Tostes, R. C., Nigro, D., Fortes, Z. B., and Carvalho, M. H. C. (2003). Effects of Estrogen on the Vascular System. Braz. J. Med. Biol. Res. 36 (9), 1143–1158. doi:10.1590/S0100-879X2003000900002
Turner, A. J., and Murphy, L. J. (1996). Molecular Pharmacology of Endothelin Converting Enzymes. Biochem. Pharmacol. 51 (2), 91–102. doi:10.1016/0006-2952(95)02036-5
Valdenaire, O., Lepailleur-Enouf, D., Egidy, G., Thouard, A., Barret, A., Vranckx, R., et al. (1999). A Fourth Isoform of Endothelin-Converting Enzyme (ECE-1) Is Generated from an Additional Promoter. Molecular Cloning and Characterization. Eur. J. Biochem. 264 (2), 341–349. doi:10.1046/j.1432-1327.1999.00602.x
Van Guilder, G. P., Westby, C. M., Greiner, J. J., Stauffer, B. L., DeSouza, C. A., and Desouza, C. A. (2007). Endothelin-1 Vasoconstrictor Tone Increases with Age in Healthy Men but Can Be Reduced by Regular Aerobic Exercise. Hypertension 50, 403–409. doi:10.1161/HYPERTENSIONAHA.107.088294
Vanhoutte, P. M., Shimokawa, H., Feletou, M., and Tang, E. H. C. (2017). Endothelial Dysfunction and Vascular Disease - a 30th Anniversary Update. Acta Physiol. 219 (1), 22–96. doi:10.1111/apha.12646
Vanhoutte, P. M., ShimokawaTang, H., Tang, E. H. C., and Feletou, M. (2009). Endothelial Dysfunction and Vascular Disease. Acta Physiol. 196, 193–222. doi:10.1111/j.1748-1716.2009.01964.x
Virani, S. S., AlonsoBenjamin, A., Benjamin, E. J., Callaway, C. W., Carson, A. P., Chamberlain, A. M., et al. (2020). Heart Disease and Stroke Statistics-2020 Update: A Report from the American Heart Association. Circulation 141. doi:10.1161/CIR.0000000000000757
Wagner, O. F., Christ, G., Wojta, J., Vierhapper, H., Parzer, S., Nowotny, P. J., et al. (1992). Polar Secretion of Endothelin-1 by Cultured Endothelial Cells. J. Biol. Chem. 267, 16066–16068. doi:10.1016/s0021-9258(18)41966-7
Wang, X., Barber, D. A., Lewis, D. A. Christopher. G., McGregor, C. G. A., Sieck, G. C., Fitzpatrick, L. A., et al. (1997). Gender and Transcriptional Regulation of NO Synthase and ET-1 in Porcine Aortic Endothelial Cells. Am. J. Physiology-Heart Circulatory Physiol. 273 (4), H1962–H1967. doi:10.1152/ajpheart.1997.273.4.H1962
Wang, X., Guo, Z., Ding, Z., Khaidakov, M., Lin, J., Xu, Z., et al. (2015). Endothelin-1 Upregulation Mediates Aging-Related Cardiac Fibrosis. J. Mol. Cell Cardiol. 80, 101–109. doi:10.1016/j.yjmcc.2015.01.001L
Weinheimer, G., Dooley, D. J. M. Cazzonelli., Cazzonelli, M., and Osswald, H. (1990). Aging and Endothelin-1 Induced Vascular Contractions. Experientia 46, 1008–1009. doi:10.1007/BF01940656
Wenner, M. M., Sebzda, K. N., Kuczmarski, A. V., Pohlig, R. T., and Edwards, D. G. (2017). ETBreceptor Contribution to Vascular Dysfunction in Postmenopausal Women. Am. J. Physiology-Regulatory, Integr. Comp. Physiol. 313 (1), R51–R57. doi:10.1152/ajpregu.00410.2016
Westby, C. M., Weil, B. R., Greiner, J. J., and Stauffer, C. A. (2011). Endothelin-1 Vasoconstriction and the Age-Related Decline in Endothelium-dependent Vasodilatation in Men. Clin. Sci. 120 (11), 485–491. doi:10.1042/CS20100475
Wilbert-Lampen, U., Seliger, C., Trapp, A., Straube, F., and Plasse, A. (2005). Female Sex Hormones Decrease Constitutive Endothelin-1 Release via Endothelial Sigma-1/Cocaine Receptors: An Action Independent of the Steroid Hormone Receptors. Endothelium 12 (4), 185–191. doi:10.1080/10623320500227275
Wynne, B. M., Chiao, C.-W., and Webb, R. C. (2009). Vascular Smooth Muscle Cell Signaling Mechanisms for Contraction to Angiotensin II and Endothelin-1. J. Am. Soc. Hypertens. 3 (2), 84–95. doi:10.1016/j.jash.2008.09.002
Wypij, D. M., Nichols, J. S., Nichols, P. J., Lowell Stacy, D., Lowell Stacy, D., Berman, J., et al. (1992). Role of Mast Cell Chymase in the Extracellular Processing of Big-Endothelin-1 to Endothelin-1 in the Perfused Rat Lung. Biochem. Pharmacol. 43 (4), 845–853. doi:10.1016/0006-2952(92)90252-E
Xu, D., Emoto, N., Giaid, A., Slaughter, C., Kaw, S., DeWit, D., et al. (1994). ECE-1: A Membrane-Bound Metalloprotease that Catalyzes the Proteolytic Activation of Big Endothelin-1. Cell 78 (3), 473–485. doi:10.1016/0092-8674(94)90425-1
Yanagisawa, M., Kurihara, H., Kimura, S., Tomobe, Y., Kobayashi, M., Mitsui, Y., et al. (1988). A Novel Potent Vasoconstrictor Peptide Produced by Vascular Endothelial Cells. Nature 332 (31), 411–415. doi:10.1038/332411a0
Yokoi, K., Adachi, H., Hirai, Y., Enomoto, M., Fukami, A., Ogata, K., et al. (2012). Plasma Endothelin-1 Level Is a Predictor of 10-Year Mortality in a General Population. Circ. J. 76 (12), 2779–2784. doi:10.1253/circj.CJ-12-0469
Zhang, C.-L., Xie, S., Qiao, X., An, Y.-M., Zhang, Y., Li, L., et al. (2017). Plasma Endothelin-1-Related Peptides as the Prognostic Biomarkers for Heart Failure. Medicine (United States) 96 (50), e9342. doi:10.1097/MD.0000000000009342
Keywords: endothelin, aging, sex hormone, endothelial function, cardiovascular
Citation: Kuczmarski AV, Welti LM, Moreau KL and Wenner MM (2021) ET-1 as a Sex-Specific Mechanism Impacting Age-Related Changes in Vascular Function. Front. Aging 2:727416. doi: 10.3389/fragi.2021.727416
Received: 18 June 2021; Accepted: 13 August 2021;
Published: 31 August 2021.
Edited by:
Cátia F. Lourenço, University of Coimbra, PortugalReviewed by:
Pedro D’Orléans-Juste, Université de Sherbrooke, CanadaMartin Houde, Leiden University, Netherlands
Copyright © 2021 Kuczmarski, Welti, Moreau and Wenner. This is an open-access article distributed under the terms of the Creative Commons Attribution License (CC BY). The use, distribution or reproduction in other forums is permitted, provided the original author(s) and the copyright owner(s) are credited and that the original publication in this journal is cited, in accordance with accepted academic practice. No use, distribution or reproduction is permitted which does not comply with these terms.
*Correspondence: Megan M. Wenner, mwenner@udel.edu