- 1Graduate Program in Biological Sciences, Federal University of Goiás, Goiânia, Brazil
- 2Araguaia Valley University Center (UNIVAR), Barra do Garças, Brazil
- 3Cardiovascular Translational Research Center, University of South Carolina, Columbia, SC, United States
- 4Department of Physiology, Medical College of Georgia at Augusta University, Augusta, GA, United States
- 5Institute of Biological Sciences and Health, Federal University of Mato Grosso, Barra do Garças, Brazil
Cardiovascular disease (CVD) is still the leading cause of illness and death in the Western world. Cardiovascular aging is a progressive modification occurring in cardiac and vascular morphology and physiology where increased endothelial dysfunction and arterial stiffness are observed, generally accompanied by increased systolic blood pressure and augmented pulse pressure. The effects of biological sex on cardiovascular pathophysiology have long been known. The incidence of hypertension is higher in men, and it increases in postmenopausal women. Premenopausal women are protected from CVD compared with age-matched men and this protective effect is lost with menopause, suggesting that sex-hormones influence blood pressure regulation. In parallel, the heart progressively remodels over the course of life and the pattern of cardiac remodeling also differs between the sexes. Lower autonomic tone, reduced baroreceptor response, and greater vascular function are observed in premenopausal women than men of similar age. However, postmenopausal women have stiffer arteries than their male counterparts. The biological mechanisms responsible for sex-related differences observed in cardiovascular aging are being unraveled over the last several decades. This review focuses on molecular mechanisms underlying the sex-differences of CVD in aging.
Introduction
Advances in health-assistance, the progress of modern medicine and access to environmental sanitation are some factors favoring the aging population around the world, as observed in the past few decades. According to the World Health Organization (WHO), “elderly” is defined as a chronological age of 60 years old or older (Steverson, 2018). However, chronological age is not a precise marker for the aging process, since biologically, human aging occurs due to cellular damage occurring over the life span. The accumulation of physical, environmental, and social factors regulates a variety of molecular mechanisms enabling aging processes (Davalli et al., 2016). The understanding of the aging process was improved by the Strehler’s enunciation (Strehler, 1977) of the so-called 4 rules of aging. This rule states that aging processes are universal, progressive, intrinsic and deleterious (De La Fuente, 2008), and therefore, will occur in a different pattern among individuals.
The current estimate is that 1 in 11 people in the world is over the age of 65 and by the year 2050, this incidence may increase to 1 in 6 people. Therefore, these numbers bring to the light the necessity of a deeper public discussion regarding older people all over the world.
Aging is a complex degenerative biological process where, over time, the accumulation of multiple irreversible injuries occurs both in molecular and cellular levels, increasing the risk of diseases and eventually, leading to death (Strehler, 1977; De La Fuente, 2008; Bai, 2018). Although aging is not a disease, it can strongly increase the chances for the appearance of degenerative diseases (Bai, 2018).
There are several theories proposed to explain the aging process and they can be divided into two large groups: (Steverson, 2018) genetic programming, and (Davalli et al., 2016) epigenetic changes (Hayflick, 2007). In spite of what is published, there is no conclusive evidence that genes directly drive the changes seen during aging. Therefore, random events (epigenetic) appear to play a major role in the aging process through morphological, physiological and behavioral modifications occurring at all levels of organization (De La Fuente, 2008).
Over the course of life, the functional status of a healthy individual can be predicted by biological parameters (aging’s biomarkers). In fact, they are better predictors for morbidity and mortality compared to chronological age [(CA) – the number of years a person has been alive]. Unlike CA, the biological age [(BA) - how old a person seems to be] is calculated based on genetic, environmental, and disease factors (Bai, 2018). The aging process is complex and thus, aging biomarkers are composed of multiple genes, proteins, and metabolites. The aging biomarkers are divided based on their primary function or physiological target. Alternatively, these biomarkers may have impact on the phenotype and functional evaluation of aging (Bai, 2018).
As age progresses, cells, tissues, and organs start to decline and molecular hallmarks of aging can be seen. Among these, some are related to DNA alterations, including epigenetic changes, genomic instability and telomere loss. Others changes are related to stem cell depletion, , mitochondrial dysfunction, protein loss, malnutrition, intercellular communication changes and oxidative stress (Kuilman et al., 2010; López-Otín et al., 2013). Further, senescence produces proinflammatory and matrix-degrading molecules, in a process which originates in the senescence-associated secretory phenotype [SASP; (distinctive secretome consisting of secretion of many factors, including several cytokines, growth factors, proteases and chemokines)].
Hence, tissue-repair capacity is lost during senescence in progenitor cells, mainly due to mechanisms related to cell cycle disarrangements. However, senescence includes other markers, besides cell cycle disarrangements (Childs et al., 2015; Calcinotto et al., 2019). Of importance, several markers of senescence can be identified, such as higher activity of senescence-associated β-galactosidase (SA-β-gal) (Ravelojaona et al., 2009); augmented levels of cell cycle inhibitors, including cyclin-dependent kinase inhibitor 1 (p21Cip1) (García-Fernández et al., 2014), cyclin-dependent kinase inhibitor 2A (p16INK4a) (Serrano et al., 1997) and cyclin-dependent kinase inhibitor 1B (p27Kip1); as well as expression of plasminogen activator inhibitor-1 (PAI-1) (Vaughan et al., 2017), tumor protein (p53) (Serrano et al., 1997) and ARF tumor suppressor (p19ARF) (Serrano et al., 1997). Moreover, changes in cellular structures are visible, in addition to accumulation of several subproducts, including: lipofuscin (Georgakopoulou et al., 2013), embryonic chondrocyte-expressed 1 (DEC1), decoy death receptor 2 (DCR2) (Collado et al., 2005), senescence-associated heterochromatin foci formation (Narita et al., 2003), DNA damage foci (Hewitt et al., 2012), senescence-associated distension of satellites (Swanson et al., 2013) and, upregulation of some microRNAs (miRNAs) (Calcinotto et al., 2019).
The risk factors for cardiovascular diseases (CVD) are directly affected by factors related to biological sex and gender differences are related to different rates of mortality among sexes. Yet, the literature supports the idea that biological age and vascular senescence directly impacts sexual dimorphism, and therefore, these factors may represent major mechanisms related to sex differences in the pathogenesis of cardiovascular diseases (Colafella and Denton, 2018).
In this regard, accumulating studies have shown sex-dimorphism in longevity phenotypes. Interestingly, most of the centenarians are women, and female neonates are more likely to survive to childhood (Colafella and Denton, 2018). Indeed, females display increased life expectancy compared to males in several countries (Rochelle et al., 2015). In addition to humans, female laboratory rats live longer than their male counterpart (Berg and Simms, 1960; Viña et al., 2005). Global data for 2015–2020 show that life expectancy for women exceeds 4.8 years of that for men. It is expected that in 2050, the female population aged 65 or over will represent about 54%. The proportion of the female population aged 80 and over will decrease from 61% (2019 data) to 59% in 2050 (United Nations, 2019). Besides all the evidence regarding sex-differences in aging, there is an urgency for more studies directly comparing aging-related mechanisms between sexes (Viña et al., 2005).
In the following paragraphs, some mechanisms involved in cardiovascular aging will be discussed, and evidence relating them to sex-differences will be presented.
Aging Mechanisms
Aging is a complex process, involving several mechanisms, such as genomic instability, telomere attrition, epigenetic alterations, loss of proteostasis, deregulated nutrient-sensing, mitochondrial dysfunction, cellular senescence, stem cell exhaustion, altered intercellular communication, inflammation, immune aging, oxidative stress, autophagy and noncoding RNAs [for more details, see reviews (López-Otín et al., 2013; Aunan et al., 2016; Dodig et al., 2019; Guerville et al., 2020)]. Considering the complexity of the issue, we limit our review to the most studied mechanisms related to aging, presented in Figure 1. Evidence linking sex-differences in mechanisms are listed in Table 1 and Figure 2.
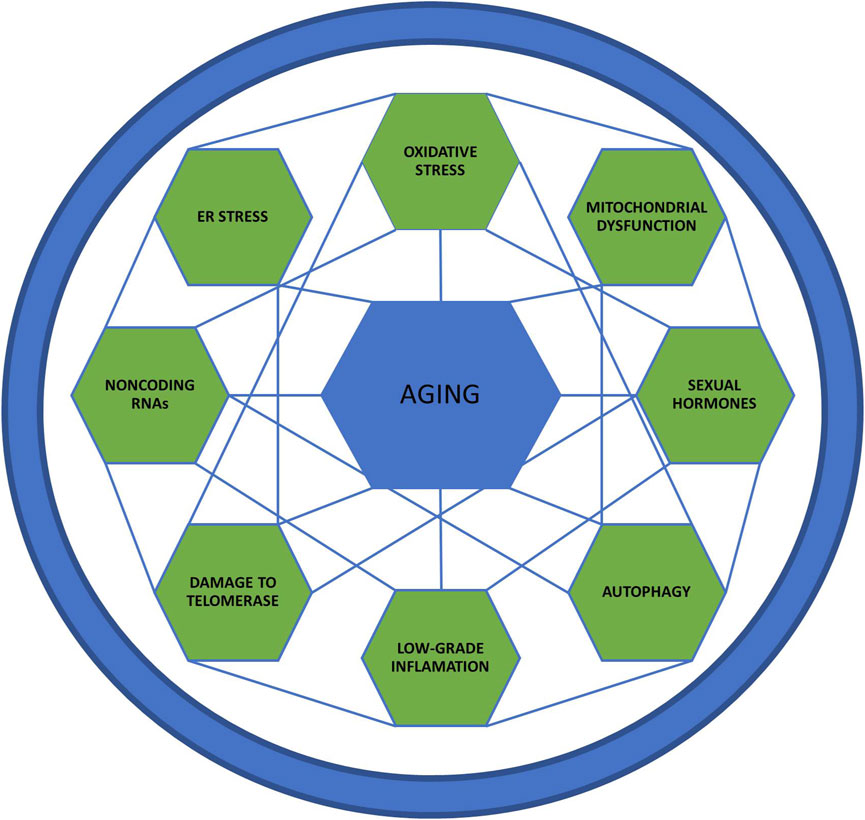
FIGURE 1. Aging mechanisms. This figure represents the aging mechanisms and the interrelationship between the several intrinsic factors that affect this process.
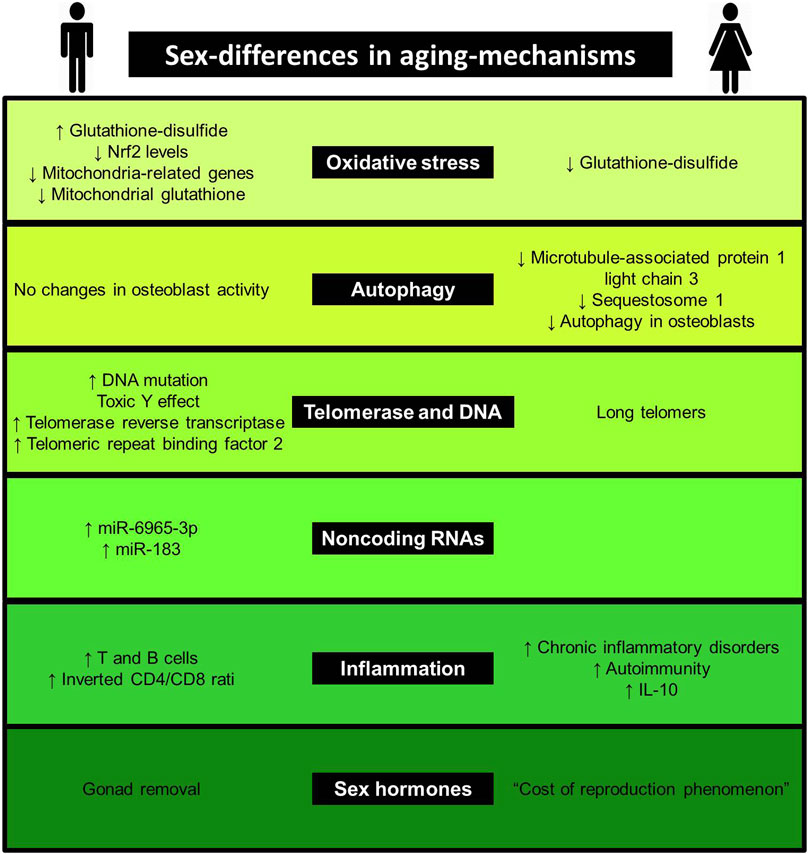
FIGURE 2. Sex differences in aging mechanisms. This figure shows evidence linking sex-differences to aging-mechanisms.
Oxidative Stress-Related Mechanisms
Oxidative stress occurs due to the imbalance between overproduction of reactive oxygen species [(ROS), produced by enzymes including NAD(P)H oxidases (Nox), xanthine oxidase (XO), uncoupled nitric oxide synthase (NOS), mitochondrial oxidase, myeloperoxidase (MPO)] and reduced antioxidant mechanisms [superoxide dismutase (SOD), catalase, glutathione peroxidase (GPx)]. The Free Radical Theory of Aging, described in the 1950s by Harman, first described ROS-accumulation linked to biomolecular damage, favoring cell senescence (Harman, 1956), and the concept that ROS accumulation results in vascular dysfunction during aging is well accepted (Genestra, 2007; Liguori et al., 2018). Currently, it is also known that accumulation of reactive nitrogen species (RNS) is also a player in vascular aging (Daiber et al., 2020).
Excessive ROS and RNS elicit oxidation of proteins, DNA and lipids, leading to cellular dysfunction. ROS are also involved in several vascular signaling pathways related to pro-inflammatory cytokine production (El Assar et al., 2013), contractility/relaxation imbalance (Taniyama and Griendling, 2003), calcium homeostasis (Kozai et al., 2014; Liguori et al., 2018), function and morphology, apoptosis (Dimmeler and Zeiher, 2000), senescence and autophagy, among others (Harvey et al., 2015).
Further, in aging, repair systems decline. Of importance, proteasomes, which enzymes are responsible for degradation of damaged proteins, as well as antioxidant enzymes might be modulated by Nrf2/EpRE intracellular signaling. Accumulating data suggest that declining efficiency of Nrf2/EpRE signaling results in age-dependent decline in the antioxidant enzyme (Zhang et al., 2015). In fact, it has been reported that in older rats decreased nuclear Nrf2 protein levels, as well as decreased antioxidant enzymes occurs in several tissues (Shih and Yen, 2007), including liver (Suh et al., 2004), aorta (Ungvari et al., 2011) and carotid arteries. Similar findings have been reported for VSMCs (Ungvari et al., 2011) from monkeys compared to young controls. Moreover, aging phenotypes are observed with the deletion of Nrf2 , favoring hearing loss (Hoshino et al., 2011), skin aging (Hirota et al., 2011) and decreased serum testosterone level (Chen et al., 2015) from Nrf2-knockout mice.
Mitochondrial dysfunction and endoplasmic reticulum stress may also be important sources of ROS, favoring vascular aging. The endoplasmic reticulum (ER) is an organelle, where folding of secreted proteins occurs, synthesis of lipids and sterols takes place and calcium is stored. ER stress occurs when the demand for protein folding and the capacity of the ER for protein folding are misaligned. ROS production elicits prolonged ER stress, favoring apoptosis (Gong et al., 2017). Yet, aging elicits ER stress evoking oxidative damage and endothelial dysfunction (Lenna et al., 2014).
The major function of mitochondria is to provide energy metabolism through the production of adenosine triphosphate (ATP), by oxidative phosphorylation (Payne and Chinnery, 2015). In addition, mitochondria are involved in other cellular processes, including the formation of reactive species (Abate et al., 2020), β-oxidation of fatty acids (Houten et al., 2016), protein metabolism (Hewton et al., 2021), regulation of apoptosis (Abate et al., 2020), maintenance of calcium concentration in the mitochondrial matrix (Finkel et al., 2015), and others. Aging impacts mitochondrial function, favoring damage to mitochondrial DNA (mtDNA). mtDNA differs from nuclear DNA (nDNA) in that it has a circular organization and differentiated coding. Mitochondria are sources of ROS and RNS, which cause damage to mtDNA because unlike nDNA, it is not protected by histones (Gaziev et al., 2014). The mtDNA is susceptible to mutation in the elderly heart, however, despite the mutations, it has been shown not to affect its copy number. In aging, there is an elevation of mitochondrial mutations, since the enzyme 8-oxodeoxyguandine glycosylase responsible for repairing damaged mtDNA is inactivated (Lesnefsky et al., 2016; Hoppel et al., 2017). The increased propensity to stress in the elderly heart has a close relationship with altered mitochondrial metabolism. With aging, the heart has impaired metabolic flexibility, decreasing the ability to oxidize fatty acids and, increasing the dependence on using the glycolytic pathway (Hyyti et al., 2010; Lopaschuk et al., 2010). In addition, there is a decrease in the activity of complexes III and IV responsible for the weakening of breathing. Defective mitochondria increases the production of ROS, oxidative damage and cell death (Müller-Höcker, 1992). The elevation of ROS further exacerbates mitochondrial damage, called the vicious cycle of the mitochondria, by interrupting the cycle of tricarboxylic acid and the electron transport chain (Lu and Finkel, 2008).
Increased Autophagy
Autophagy is a cellular process of self-degradation with the purpose of maintaining homeostasis, degrading proteins, and renewing organelles (Salabei and Hill, 2015). Cellular aging promotes the accumulation of damaged proteins and organelles that cannot be regulated efficiently by autophagy as this process is impacted by age (Nair and Ren, 2012). Although autophagy is important for cell survival, its excess also promotes cell death (Yu et al., 2006). Therefore, the plasticity of vascular cells should be a well-controlled process.
Evidence shows that aging contributes to a gradual decrease in autophagy and activity of the ubiquitin protein system, resulting in functional deterioration of the organism (Ma et al., 2011). Autophagy is initiated by Beclin-1 and phosphatidylinositol 3-kinase class III (PI-3K). A complex between Beclin-1 and PI3K-III plays an important function in membrane traffic and restructuring involved in autophagy, phagocytosis and endocytosis (Zhong et al., 2009). The autophagy genes ATG5, ATG7 and BECN1 are downregulated in the brain of older people when compared to young people (Lipinski et al., 2010). In cardiomyocytes, the main autophagic signaling pathways are MLC/FAK/AKT/mTOR-mediated inhibitory pathways and Beclin 1 activation pathways (Fernández et al., 2018).
Due to aging, there is a decrease in cardiac autophagy, resulting in loss of homeostasis in cardiac tissue and consequent dysfunction (Taneike et al., 2010). The regulation of autophagy occurs positively by AMP-dependent protein kinase (AMPK) and, conversely, Akt and mTOR downregulate AMPK through the phosphorylation of their targets as p70s6k (Sarkar et al., 2009). Of importance, the interruption of mTOR is the main factor for the reduction of longevity (Blagosklonny, 2008). Moreover, it has been demonstrated that rapamycin, a specific inhibitor of mTOR, was able to increase lifespan in several species (McNab, 2003; Kapahi et al., 2004; Powers et al., 2006; Harrison et al., 2009) due to its ability to suppress cell growth and proliferation (Gao et al., 2020). In humans, inhibition of mTOR pathway improves age-related disorders such as heart disease (McCormick et al., 2011).
Thus, genetic modifications are alternatives to increase autophagy and favor cardiac function, the removal of dysfunctional organelles and poorly folded proteins (Taneike et al., 2010). Akt/mTORC1 inhibition, related to increased autophagy and; increased HSP27 expression, related to reduced accumulation of LC3-II and p62; are mechanisms linked to the attenuation of damage related to cardiac aging. These changes related to autophagy are able to improve contraction, reduce oxidative stress and resist cardiomyocyte senescence (Lin et al., 2016; Gao et al., 2020). In addition, microRNAs such as miR-497-3p, miR-26b-5p or miR-204-5p when negatively regulated increase myocardial autophagy (Qi et al., 2020).
Damage to Telomerase
Telomeres are non-coding, repetitive sequences of nucleotides, physically localized in the terminal part of chromosomes and associated specialized proteins that interact with DNA to form nucleoproteins (De Lange et al., 2006). Aging is mainly determined by the length of telomeres and cells have a limited number of divisions. After each division the telomere is shorter until it reaches an indivisible state known as replicative senescence (Chan and Blackburn, 2004; Martin, 2011).
Among the processes related to telomere shortening are stress, chemical damage, nuclease action or age progression. The activity of telomerase, a specialized RNA-dependent polymerase, compensates for this loss in a mechanism that replicates the ends of linear DNA molecules. The telomerase RNA (TERC) and telomerase reverse transcriptase (TERT) forms a telomerase complex (Lane and Martin, 2010; Scarabino et al., 2019).
When investigating the relationships of TERC and TERT genetic variations associated with aging, it was observed that decreased life expectancy may be related to genotypes including TERT VNTR MNS16A L/L and TERTOs rs2853691 A/G or G/G (Scarabino et al., 2019). In addition to telomerase activity, another factor that interferes with the shortening of the telomere is the loss of protection and stability due to irreparable damage to DNA. This occurs by destabilizing Shelterin complex components such as telomeric factor 1 and 2 (TRF1 and TRF2) (Martínez et al., 2009; Ryan et al., 2010; Porro et al., 2014).
In unprotected telomeres, structure regulation and processing by lncRNA TERRA (RNA containing telomeric repetition) transcribed from telomeres occurs (Porro et al., 2014). The elevation of TERRA expression may be linked to the senescence process. After DNA damage, there is an increase in TERRA signaling by RNA polymerase II, which is linked to the chromatin structure. In damaged telomeres, a p53-dependent increase in the level of EARTH occurs (Caslini et al., 2009; Porro et al., 2010; Porro et al., 2014).
In some cases, as in human peritoneal mesothelial cells (HPMCs), telomerase is active, however, they have short telomeres (3.5 kbps). Reduced telomerase activity plus accompanying mitochondrial dysfunction, resulting in ROS production may explain this phenomenon. Thus, oxidative stress contributes deeply to disruption of non-telomeric regions, promoting premature aging (Ksiazek et al., 2007; Passos et al., 2007).
Excessive ROS production favors the migration of hTERT from the nucleus to the cytosol, reducing telomerase activity and consequently promoting aging of endothelial cells. The use of antioxidants and other active ingredients can delay this process. N-acetylcysteine incubation decreased ROS formation, preventing damage to mtDNA. In addition, incubation with atorvastatin had similar effects (Haendeler et al., 2004; Santos et al., 2004; de Punder et al., 2019). It is important to note that despite the beneficial effects of antioxidants against ROS in several animal models and in vitro studies, many large-scale randomized controlled trials have provided inconsistent results on the prevention of chronic diseases and lifespan in humans (Sadowska-Bartosz and Bartosz, 2014).
Metabolic stress affects the size of the telomeres. For example, a decrease in serum omega-3 levels has a correlation with telomere size, which can be a marker of aging (Farzaneh-Far et al., 2010).
Evidence from humans studies show that elevated ROS contributes to chronic inflammatory diseases and shortening of telomeres (Finley et al., 2006; Letsolo et al., 2017). Acceleration in the telomere shortening is drastically observed in oxidative stress conditions by several mechanisms: 1) single strand breaks (SSB) mediating the collapse of replication fork and the loss of telomere; 2) increased cell division and telomere shortening due cell death and/or senescence-related process, 3) multi-telomeric foci at chromatid ends termed fragile telomeres, due to unreplicated ssDNA accumulation (Von Zglinicki, 2002; Sfeir et al., 2009).
Noncoding RNAs
Epigenetic alterations act as a link between the intrinsic genetic landscape and extrinsic environmental influences, including DNA methylation or hydroxymethylation, histone modifications, and chromatin remodeling including microRNAs (miRNAs), and noncoding RNAs (ncRNAs) (Greco and Condorelli, 2015; Venkatesh and Workman, 2015; Zhang et al., 2018). Noncoding RNAs are a class of regulatory ncRNAs and can provide important data for the aging process. They have a transcription length greater than 200 nucleotides and can be separated according to their length into short and long ncRNAs (Jin et al., 2019).
Circular RNA (circRNA), a recently reported type of noncoding RNA, has been shown to play a role in both cellular senescence and cellular survival, and some recent studies make the assumption that circRNA contributes to the pathogeneses of age-related diseases, making them possible biomarkers (Cai et al., 2019). Age-related diseases are related to processes of proliferation and cell survival, which are also controlled by ncRNAs, modulating some pathways via cyclin-dependent kinase inhibitor 1 (p21), cyclin-dependent kinase 2 (CDK2) and mammalian forkhead transcription factors (Maiese, 2016).
Studies have profiled the changes in lncRNAs in CVD and aging. Cardiac-specific lncRNAs from mouse hearts with myocardial infarction have been associated with maladaptive cardiac pathological remodeling and some of these lncRNAs (CDKN2BAS1/ANRIL, RMRP, RNY5, SOX2-OT, SRA1 EGOT, H19, HOTAIR, and LOC285194/TUSC7) are changed (2,204 genes are up-regulated and 1,338 genes are down-regulated) in individuals with dilated cardiomyopathy and aortic stenosis [116]. Moreover, expression of the myosin heavy-chain-associated RNA transcripts (Myheart or Mhrt, a conserved lncRNAs involved in the antisense direction) increases as age progresses and acts as a safeguard for cardiac health in mice and humans (Greco et al., 2016).
Besides lncRNAs, circRNAs have been linked to aging-associated genes, such as circANRIL. In fact, circRNAs lead to greater proliferation of SMCs during atherosclerosis, and they are increased in patients with coronary artery diseases (Holdt et al., 2016; Ghafouri-Fard et al., 2021).
Another gene involved in decreasing longevity is the LMNA that encodes laminin A and the detection of its mutation can be used to track damage by age (Zhavoronkov et al., 2012). Mutations in genes that encode receptors, kinases, substrates of metabolic pathways of insulin/IGF-1, PI3K, TOR, MAPK can also occur, resulting in an increase or decrease in life span (Clancy et al., 2001; Narasimhan et al., 2009; Zhavoronkov et al., 2012).
In patients with myocardial infarction, the lncRNAs called aHIF, KCNQ1OT1, MALAT1 are more expressed while those of ANRIL are decreased when compared to healthy people. In mice with the same condition, MIRT1 and MIRT2 are stimulated and correlated with genes involved in left ventricular remodeling and ejection fraction (Vausort et al., 2014; Zangrando et al., 2014).
In heart failure, common in aging, there is positive regulation of some lncRNAs such as NRON (NFAT non-coding repressor) and MHRT (RNA transcripts associated with the myosin heavy chain) (Vausort et al., 2014) and mitochondrial LIPCAR (uc022bqs.1) with chimeric fusion transcription of the 5′ end of COX2 and 3′ end of CYTB (Kumarswamy et al., 2014). The MHRT-Brg1/BAF complex can protect the heart from hypertrophy and insufficiency (Han et al., 2014).
In hypertensive patients there is an increase in plasma levels of lncRNA-AK098656 with VSMCs-dominant specific for humans. This lncRNA binds myosin heavy chain proteins and fibronectin-1, facilitating their degradation. AK098656 may be an efficient therapeutic target for hypertensive control (Jin et al., 2018).
Low-Grade Inflammation
Aging is also characterized by a chronic low-grade inflammation state, also known as “inflammaging”, shifting to the production of pro-inflammatory cytokines (Rea et al., 2018), chemokines, and adhesion molecules (De Martinis et al., 2005; Vasto et al., 2007; Calder et al., 2017). The immunological decline increases the susceptibility to various ailments such as cardiovascular diseases, cancer and, infections which are higher in older people (Weyand and Goronzy, 2016).
With age, the body starts to maintain a chronic pro-inflammatory state, as it is constantly in a stressful environment. Among the inflammatory predictors of aging are an increase in CD8+ T cells, decreased CD4+ T cells and CD19+ B cells, and mitogen-induced inhibition of T cell proliferation. High levels of serum interleukin -6 (IL-6) is a reliable marker of disability and mortality in the older people. Other cytokines such as interleukin-10 (IL-10, anti-inflammatory) and tumor necrosis factor alfa (TNF-α, inflammatory) can also be considered serum markers of aging (Butcher and Lord, 2004; De Martinis et al., 2005). Although necessary, when there is an excess of inflammatory responses, a stimulus to human aging occurs (Xia et al., 2017). In an experiment with older horses, there was an increase in the expression of interleukin-1 beta (IL-1 β), interleukin-15 (IL-15), interleukin-18 (IL-18) and TNF-α in peripheral blood (Xia et al., 2017).
The general balance of cytokines is important in aging, as well as alterations in the gene-promoting regions of these cytokines, thus determining susceptibility to age-related diseases (Lio et al., 2003). A study showed that Italian male centenarians display a more frequent single nucleotide polymorphism (SNP) in the IL-6 gene promoter region. In addition, they also have an increased occurrence of SNP -1082G in the IL-10 gene 5′ flanking region. Therefore, polymorphisms of inflammatory cytokine genes can regulate immunoinflammatory responses (Pes et al., 2004).
A low-grade state of inflammation is observed in aging, further affecting apoptosis and autophagy (Calder et al., 2017). Impaired autophagy can trigger the appearance of a pro-inflammatory phenotype and activation of the inflammasome (Calder et al., 2017), a multiprotein complex that is activated in response to microbial invasion or damage‐associated molecular patterns (DAMPs) in innate immune cells (Guo et al., 2015).
Aberrant activation of NLR family pyrin domain containing 3 protein (NLRP3) inflammasome leads to the production of inflammatory cytokines IL-1β and IL-18 and contributes to amplification of pathological inflammation. Of importance, inflammasome is activated during aging and aging-related disease (He et al., 2020). In fact, ablation of NLRP3-inflammasome protected mice from age-associated changes in the heart. Moreover, old NLRP3 KO mice showed an inhibition of the PI3K/AKT/mTOR pathway and improvement in autophagy, compared with old wild-type mice, suggesting that NLRP3-inflammasome suppression improves longevity and prevents cardiac aging in male mice (Marín-Aguilar et al., 2020).
Sex Differences in Cardiovascular Aging
During aging, cardiovascular structure and function start a progressive decline. Heart and vasculature gradually show homeostatic imbalance, vascular stiffening, fibrosis, and increased left ventricular (LV) wall thickness leading to accentuated tissue adaptations and decreased stress tolerance (Stern et al., 2003). All the aging mechanisms previously described in this review have been shown to participate in cardiovascular aging.
It is well known that men display increased adverse cardiovascular events compared to pre-menopausal women. However, this statistic inverts after menopause, where this female cardiovascular protection is lost (Pollow et al., 2019; Virani et al., 2020). Moreover, the development of CVD coincides with the decline of female sex hormones such as estrogens (Zhao et al., 2018).
Interestingly, decreased plasma levels of testosterone have been linked with age-related CVD pathways in men (Traish et al., 2009), indicating that abnormal levels of testosterone may have deleterious effects in the cardiovascular system (Lopes et al., 2012). Further, lower testosterone levels, when observed in elderly men, are related to an increased occurrence of heart diseases and modification in body composition. However, hypogonadism is related to augmented cardiovascular risk (Guay, 2009). Regardles, mechanisms involved in cardiometabolic maintenance are clearly regulated by male sex steroids, demonstrating an important role during aging (Traish et al., 2009; Barrientos et al., 2020).
Conversely, men with reduced testosterone levels displayed decreased death risk (Laughlin et al., 2008). And, supraphysiological levels of testosterone may contribute to CVD risk (Gagliano-Jucá and Basaria, 2019). Testosterone impacts vascular function, by overexpression of pro-inflammatory cytokines, arterial thickness and by reducing NO synthesis and bioavailability (Lopes et al., 2012). Mesenteric arteries from old Wistar rats, treated with testosterone displayed augmented oxidative stress and inflammation possibly to due increased leucocyte migration, favoring increased cardiovascular risk (Chignalia et al., 2015). Further, apoptosis and migration of VSMCS are processes induced by testosterone, via ROS formation and MAPK activation, respectively (Lopes et al., 2014).
A prospective cohort of post-menopausal women in the Multi-Ethnic Study of Atherosclerosis showed an association between higher total testosterone/estradiol ratio and increased risk on incident CVD, as well as total testosterone and bioavailable testosterone levels (Wang et al., 2012; Zhao et al., 2018).
The loss of renal function with age or disease can drive an increase in blood pressure (Hall, 2003). A study conducted with hypertensive and normotensive postmenopausal women reported a decrease in sex hormones and increase in sensitivity to sodium intake (Tominaga et al., 1991). Of note, evidence shows increased sodium/hydrogen exchanger 3 (NHE3) expression in the proximal portion of the renal tubule in males compared to females, whereas epithelial sodium channel (ENaC) and sodium/chloride co-transporter (NCC) are higher in distal portions of the renal tubule from females, compared to male rats, favoring sodium excretion in females (Veiras et al., 2017).
Obesity is a risk factor for CVD and has been linked to telomere shortening (Batsis et al., 2018). Interestingly, telomere shortening can induce permanent cellular senescence (Colafella and Denton, 2018), but this process occurs earlier in men, even though telomere length is similar between sexes in later life (Barrett and Richardson, 2011). Moreover, kidneys from male and female rats displayed age-related telomere shortening, however, males were more affected than females. Further, p53 and p21 (senescent markers) expression significantly increased in males, but not in females whereas augmented antioxidants mechanisms (such as SOD, glutathione peroxidase and glutathione reductase) were observed in the cortex from older female (Tarry-Adkins et al., 2006).
CVD-treatment, targeting peculiarities between the sexes, may be an important topic for further investigations. Many medications are commonly used to prevent CVD before it occurs, as well as to prevent existing disease from getting worse. In this regard, treatments for cardiovascular disease are more common in women than men in primary prevention, but the reverse is seen in secondary prevention (Walli-Attaei et al., 2020). Interestingly, a meta-analysis showed that women were significantly less likely to be prescribed with aspirin, statins, and angiotensin-converting enzyme inhibitors, compared to men (Zhao et al., 2020). Therefore, it is fair to say that guideline-recommended medications to treat CVDs are less frequently prescribed to women. Another important point is that women may not be receiving the same degree of health assistance compared to men (Okunrintemi et al., 2018), as revealed by a survey conducted with more than 11 million women, using data from the Medical Expenditure Panel Survey, from the Department of Health and Human Service. The authors also speculate that physicians may have a dubious view of similar symptoms when reported by men, compared to women”
It is reasonable to state that the CVD’s treatment does not often consider the peculiarities inherent to the binomial sex and age. Aging brings a decline in basal physiological functions and in some cases, the patients are as vulnerable to the pharmacological treatments as they are to the CVD (Stuck et al., 2015). Strategies to improve the identification of avoidable risk factors in an aging population, including the prediction of adverse drug reactions, will bring some light to these patients receiving a safer treatment.
In this regard a large gap for the evaluation and selection of safer treatments is still an open question, and personalized medicine is becoming a strong market niche. Personalized medicine goes for the selection of therapies offering the highest safety, ensuring patient care (Vogenberg et al., 2010). On the other hand, making it suitable for a large portion of the population is still a challenge, considering that most CVD’s protocols still work with the concept that “one size fits all” (Riondino et al., 2019).
Age-Sex Implications in Cardiac Structure
Cardiac remodeling is a process continuously influenced by age and cardiac hypertrophy is common in both sexes (Kane and Howlett, 2018). Over the lifetime, left ventricular (LV) mass and volume are reduced in both sexes. However, structural evaluations suggest bigger LV mass and wall thickness in men, compared to women (Salton et al., 2002; Lieb et al., 2009). Of importance, in women, changes observed in the wall thickness are more accelerated, especially in the face of hypertensive conditions and diabetes (Cheng et al., 2010). Even while receiving anti-hypertensive-drugs, women have higher risk to develop LV hypertrophy, compared to men (Izzo et al., 2017). In fact, the format of remodeling that occurs in women present a more concentric remodeling over the years (Cheng et al., 2010; Merz and Cheng, 2016; Singh et al., 2019; Miller et al., 2020) and this tendency favors diastolic dysfunction and a higher incidence of heart failure with preserved ejection function in women (Gori et al., 2014).
Extracellular Matrix Deposition
Extracellular matrix deposition is a hallmark for cardiac remodeling in aging and sex differences are also observed. A study conducted in non-diseased human hearts showed an age-dependent sex-specific regulation of extracellular matrix components (Dworatzek et al., 2016). Young women have lower collagen type I, III and VI, tissue inhibitor of metalloproteinase 3 than men. Interestingly, the expression of these proteins was higher in older women, compared to men. In fact, differences in the expression of female cardiac extracellular matrix genes, with excess collagen and other proteins in the LV, and estrogen deficiency in menopause (Dworatzek et al., 2016; Merz and Cheng, 2016). Indeed, cardiac extracellular matrix proteins, including collagen, are greater in the LV of older women than in older men, suggesting a role on heart fibrosis (Dworatzek et al., 2016).
Estrogen
Estrogen seems to regulate extracellular matrix deposition, suggesting a distinct molecular mechanism occurring between the sexes. In vitro experiments conducted in primary human aortic smooth muscle cells exposed to estrogen resulted in decreased collagen, and increased elastin (Natoli et al., 2005). In postmenopausal women, estrogen contributed to decreased LV mass (Lim et al., 1999) and elicited increased baroreflex sensitivity (Huikuri et al., 1996). Yet, estrogen regulates cardiac modulators related to hypertrophy, including natriuretic peptide (Van Eickels et al., 2001; Babiker et al., 2004; Pedram et al., 2005; Pedram et al., 2008), endothelin (Pedram et al., 2005) and, PI3K/PKB-signaling (Camper-Kirby et al., 2001; Bhuiyan et al., 2007). Of importance, LV mass and wall thickness are related to polymorphisms occurring in the ESR2 gene in women, but not in men (Peter et al., 2005).
Other Mechanisms
Other possible links to cardiac remodeling are the reduced number of ventricular myocytes, usually observed both in humans and animals, probably caused by several mechanisms. First, decreased stem cell regeneration is observed in men, whereas cardiac stem cells are more expressed in women (Olivetti et al., 1995). Additionally, apoptosis is an event more frequently observed in men facing cardiovascular diseases, compared to women (Boddaert et al., 2005). Yet, other processes such as cardiomyocyte necrosis and autophagy, hypertrophy and fibroblast proliferation are more prominent in men than in women (Zhang et al., 2007; Leon and Gustafsson, 2016; Kane and Howlett, 2018).
The robust body of evidence implicating age- and sex-related cardiac remodeling makes this is an exciting area for future exploration, aiming to define other mechanisms that might be implicated in these events.
Age-Sex Implications in the Cardiac Function
Electrical Activity
A decreased number of cells in the pacemaker has been established during aging, and this is related to a decline in gene expression involved in the sinoatrial function, such as changes in many ion channels and ion-homeostasis related genes (Tellez et al., 2011; Mirza et al., 2012; Kane and Howlett, 2018). The heart decreases its electrical conductance with older age and a slower conduction is seen through the atrium and atrioventricular node. Thus, the occurrence of bradycardia and the necessity of an artificial pacemaker system increases, especially in men (Méndez-Bailón et al., 2017; Chamandi et al., 2018).
One plausible explanation for reduced conduction may be the reduced expression of connexins, involved in myocyte cell connections, in addition to electrophysiological changes in the atrial myocytes (Bonda et al., 2016; Jansen et al., 2017). Experiments using ischemia/reperfusion in hearts isolated from mice, demonstrated that expression of mitochondrial connexin-43 has a protective cardiac effect, and that post-ischemic estrogen treatment reduced infarct size while increasing connexin-43 expression in mitochondria. Further, mitochondrial Cx43 displayed a protective role in female hearts leading to a decreased myocardial ischemia/reperfusion compared to that in age-matched males (Wang et al., 2020). Pacemaker nucleus records, obtained in the electric fish Apteronotus leptorhynchus, showed a sex-dimorphism under the influence of steroid hormones (Zupanc, 2020). Pronounced sex-differences in morphology and in the formation of a syncytium by the astrocytes were observed. A larger syncytium area covering the pacemaker cells was present in females, along with greater connexin-43 expression, suggesting a stronger gap-junction in females, compared to males.
Systolic and Diastolic Function
Implications coming from sex-related differences in the heart structure also impact cardiac function, such as systolic function, which clinically, may be frequently accessed by the left ventricular ejection fraction (LVEF), a value that is generally higher in women, compared to men (Chung et al., 2006; Tadic et al., 2019). Older women present higher ejection fraction than men, along with higher right ventricular fractional area and a negative global longitudinal strain, besides greater tricuspid regurgitation velocities. Moreover, abnormal ventricular end-diastolic and end-systolic volumes was higher in women than men. However, overall women had better survival outcomes with pulse pressure as a key determinant and, conversely, heart rate and B-type natriuretic peptide were associated with poorer outcome in men (Beale et al., 2019).
Despite some evidence showing increased ejection fraction in aged women, other studies have shown preserved ejection fraction and, therefore, a better knowledge of cardiac structure and function abnormalities during heart failure may help diagnose people with high risk for death due to CVD (Shah et al., 2014; Merrill et al., 2019). In fact, among old patients (49% were women) with preserved ejection fraction, LV hypertrophy and higher pulmonary artery pressure and LV filling pressure were predictive of heart failure, hospitalization and cardiovascular death (Shah et al., 2014).
Cardiac function also differs and men have defects in the systolic pump earlier, while diastolic function is little compromised (Gebhard et al., 2013). On the other hand, women have greater diastolic involvement, greater systolic torsion and LV shortening, in addition to increased ejection fraction (Yoneyama et al., 2012; Gebhard et al., 2013). In older age, the systolic function is reduced, also evidenced by the decreased capacity of the ventricular myocytes to contract (Fannin et al., 2014; Kane and Howlett, 2018).
Diastolic dysfunction has shown to be a hallmark pathological intermediate in the development of HFpEF. In fact, a cohort study in ethnic Asians demonstrated that prevalence of diastolic dysfunction as also others diastolic parameters increase with advanced age and it is greater in women overall than men (Chang et al., 2021).
The maintenance of optimal output involves development of greater systolic stiffness in the LV (Chen et al., 1998). Interestingly, end-systolic elastance is higher, mainly, in older females (Saba et al., 1999; Redfield et al., 2005). The mechanism behind this event may be related to alterations in chamber geometry. Of importance, aging is associated with impaired subendocardial function (Lumens et al., 2006) due to reduced global longitudinal shortening (Støylen et al., 2020) where arterial stiffness is a greater contributor, especially in women (Bell et al., 2017; Yoshida et al., 2020).
Further, female obesity results in heart failure with preserved ejection fraction (HFpEF) (Kitzman and Shah, 2016). This condition occurs due to ischemic events in the heart’s circulation, especially in vessels of large caliber, resulting in reduced contraction of the ventricles (Murphy et al., 2020). This and other evidence show that the occurrence of CVD in obese patients is most dangerous for women. Some mechanisms may explain the association between increased adiposity and HFpEF. Augmented adiposity promotes inflammation which induces dysregulation of the nitric oxide-cyclic guanosine monophosphate-protein kinase G signaling cascade which in turn leads to mitochondrial disruption and endothelial dysfunction (Paulus and Tschöpe, 2013). Additionally, greater activation of the angiotensin-aldosterone signaling pathway can cause myocardial injury (Kitzman and Shah, 2016). Moreover, estrogenic vasodilatory effects as well as myocardial energy substrates changes during post-menopause and they have been proposed as important contributors to the pathogenesis of HFpEF, once its prevalence increases in postmenopausal women (Sabbatini and Kararigas, 2020; Gökçe et al., 2003; Voutilainen et al., 1993).
In fact, hormone therapy (HT) decreased LV mass by 20% in postmenopausal women (Lim et al., 1999) and also reduced LV mass index in hypertensive women (Light et al., 2001). In the same way, ovariectomized animals treated with GPR30 (estrogen receptor) agonist (G1) displayed improved LV diastolic function, collagen deposition, atrial natriuretic factor, cardiac NAD(P)H oxidase 4 (NOX4) expression and, inhibited angiotensin II-induced hypertrophy in H9c2 cardiomyocytes, whereas GPR30 antagonist inhibited the protective effects on this hypertrophy (Wang et al., 2012).
Estradiol and Estrogen
Isolated hearts from both adult female and male rats treated with estradiol leads to the development of cardioprotection against infarction, though a reduced effect was observed in hearts from male rats (Sovershaev et al., 2006). The mechanism behind this protection relies on the activation of the PI3K/GSK3β, favoring mitochondrial protection. Further, estradiol is able to induce S-nitrosylated proteins and greater NO-signaling activation, which are known to be cardioprotective (Deschamps and Murphy, 2009). Estrogen deficiency via ovariectomy (OVX) in hypertensive female rats increases oxidative stress as well as cardiac inflammation which result in diastolic dysfunction and myocardial fibrosis (Mori et al., 2011).
Further, estradiol protects isolated hearts from female SHR against IR injury via GPR30 leading to Nocth-1 activation. Through non-nuclear estrogen receptors, phosphoinositol 3 kinase-dependent and mitochondrial adenosine triphosphate (ATP)-sensitive potassium channels survival (Rocca et al., 2018) leads to endothelial nitric oxide synthase (e-NOS) activation and S-nitrosylation (Shao et al., 2016) which play a key role in post-translational modification in cardioprotection (Menazza et al., 2017).
Although several studies showing a cardio-protective role for premenopausal hormones, conflicting evidence exists regarding the efficacy of hormone therapy in postmenopausal women (Korzick and Lancaster, 2013).
Increased collagen deposition and LV stiffness as well as concentric remodeling are associated with female sex and age (Hoshida et al., 2016). Among the female hormones, estrogen plays a key role during age-related diastolic dysfunction in women. Indeed, this hormone is a vasodilator (Reis et al., 1994) acting at cellular Ca2+ handling sites, which could impact diastolic performance (Oneglia et al., 2020). Further, it was demonstrated in rats that OVX decreased expression of phosphorylated phospholamban [PLB (facilitates sarco(endo)plasmic reticulum Ca2+-ATPase 2a (SERCA2a) activity)] which in turns reduced lusitropy and increased cardiac filling pressures. Of note, the worst diastolic dysfunction caused by OVX was demonstrated in older rats than middle-age (Alencar et al., 2017). Furthermore, estrogen treatment in a primate model of menopause preserved diastolic function due calcium homeostasis (Michalson et al., 2018).
Oxidative Stress
Another mechanism contributing to diastolic dysfunction is increased oxidative stress (Kumar et al., 2019). Decreased ROS production was seen in female rat heart upon ischemia/reperfusion compared with age-matched male hearts via posttranslational modification of mitochondrial proteins (Lagranha et al., 2010). Indeed, preclinical ischemia/reperfusion studies with OVX demonstrated that estrogen induces ATP production and electron transport chain activity (Lancaster et al., 2012), downregulates mitochondrial apoptotic pathways (Fliegner et al., 2010) and upregulates mitochondrial antioxidants (Liu et al., 2014). Further, this hormone was able to reduce ROS formation, prevent energy dysregulation and improve diastolic function (Chen et al., 2015) in treated OVX mouse model of hypertrophic cardiomyopathy. Of importance, ROS acts as a scavenger to nitric oxide, a key regulator of normal diastolic function (Silberman et al., 2010).
Gonadectomy
As already mentioned, circulating estrogen and testosterone levels decrease with age and there is a link between sex hormones and CVD. Interestingly, long-term gonadectomy in older male mice slowed isovolumic relaxation time promoting diastolic dysfunction in the aging heart. The mechanism behind this dysfunction may due to higher cardiac expression of PLB protein in older mice with gonadectomy compared to control mice. The increase in PLB interferes with Ca2+ uptake, prolonging its uptake in the sarcoplasmic reticulum and prolonged transient Ca2+ decay in cardiac cells from aging gonadectomy mice. Further, in hearts from aging gonadectomy mice, there is a reduction in phosphorylation of the regulatory myosin light chain ELC (Ayaz et al., 2019).
Other Mechanisms
Growing evidence has linked polyunsaturated fatty acids (PUFAs) and cardiac homeostasis. PUFAs are metabolized through numerous metabolic pathways, including cytochrome P450 (CYP) monooxygenase (Jamieson et al., 2017). The progression of CVDs such as hypertension and atherosclerosis has been implicated in the hydrolysis and inactivation of epoxy metabolites by soluble epoxide hydrolase (sEH) (Harris and Hammock, 2013). When compared to aged wild-type (WT) mice, male sEH null mice had preserved diastolic function and females had preserved systolic function. Furthermore, the latter preserved Sirt-3 activity, mitochondrial ultrastructure and SOD activity levels. Increased age-related carbonyl levels have been demonstrated in male WT and sEH null mice (Jamieson et al., 2020).
Age-Sex Implications in the Cardiac Vasculature
Cardiac Vasculature Thickening
In human, macroscopic changes such as thickening of the aortic valve leaflets, accumulation of lipids and calcification of the aortic valve are observed in both sexes during aging. Carotid intima-media thickening, coronary artery calcification and the formation of atherosclerotic plaques are more prevalent in men than in adult and middle-aged women; however, as they become older, women start to present greater dysfunctions (Kelley et al., 2011; Merz and Cheng, 2016). Men tend to have a higher risk of plaque (Ota et al., 2010; Merz and Cheng, 2016). Interestingly, women with coronary artery disease were less likely to undergo optimal secondary prevention with antiplatelet and lipid-lowering therapies than their male counterparts (Madan et al., 2020).
When considering the scientific evidence on the increased risk of CVD in old age, it is important to consider changes in cardiac functional and structural aspects (Kane and Howlett, 2018). Mortality in the western world is mainly caused by CVDs, a factor that is increasing globally (Heidenreich et al., 2011). In the formation and expansion of plaque in atherosclerosis, senescence inducers, such as telomere shortening and oxidative stress are produced in vascular smooth muscle cells and endothelial cells (Wang and Bennett, 2012). As a result, senescent endothelial cells are prone to apoptosis, endothelial layer “leakiness”, oxidized LDL extravasation and, decreased NO secretion (Hogg and Kalyanaraman, 1999; Zhang et al., 2002; Krouwer et al., 2012). Further, with aging, the vasculature displays alterations such as aortic stiffening and thus, vascular aging may be considered a prodromal stage of atherosclerotic diseases (Zhang et al., 2018).
Estradiol
The incidence of HFpEF and HFrEF in both sexes, increases with age. In women, especially postmenopausal women, there is a higher HFpEF index, while men have a higher prevalence of HFrEF (Beale et al., 2018; Sickinghe et al., 2019). Whereas HFrEF is related to larger vessels developing after an ischemic event (Sickinghe et al., 2019), HFpEF is gradual, involving the microvasculature of the heart (Lee et al., 2016).
A mechanism contributing to this phenomenon may be through 17β-estradiol (E2). In fact, E2 is able to regulate eNOS activity, increase soluble guanylyl cyclase (sGC) to elevate cyclic GMP (cGMP) concentrations leading to activation of protein kinase G (PKG) (Nevzati et al., 2015). Further, E2 also acts via protein kinases PI3K and Akt signaling (Gourdy et al., 2018). Through p38/MAPK signaling, E2 inhibited the proliferation of VSM cells only in female mice (Pellegrini et al., 2014). Furthermore, the inflammatory regulation of the initial atherosclerotic plaque is prevented by the activation of ER-α, decreasing the deposition of lipoproteins and, consequently, the formation of fatty streaks (Elhage et al., 1997).
With aging, perivascular fibrosis (formation of fibrosis around blood vessels) increases (Nosalski et al., 2020) and this fibrosis formation can be influenced by estrogen (Sickinghe et al., 2019). In women, the inhibition of collagen I and III production occurs through activation of ER-α cardiac fibroblasts by E2, whereas in men the E2 to ER-β binding stimulates collagen production (Dworatzek et al., 2019). Cardiac fibrosis in men occurs due to the androgenic influence of the up-regulation of TGF-β, resulting in extracellular matrix deposition (Kong et al., 2014).
Interleukins
Comorbidities such as diabetes, obesity and hypertension share the ability to induce a systemic inflammatory state which was recently shown to be accompanied by a larger deterioration of the cardiac cells function and structure in HFPEF (Mohammed et al., 2012; Paulus and Tschöpe, 2013). In fact, high circulating levels of interleukin-6 (IL-6) and tumor necrosis factor α (TNF-α) were observed in a cross-sectional study of HFPEF patients regardless the sex (Kalogeropoulos et al., 2010).
Sex Differences in Vascular Aging
Vascular aging increases the risk of CVD, despite being an independent risk factor for age-related diseases. Further, women often present with acute myocardial infarction later than men (Nanna et al., 2019) due the protective role of circulating estrogens against vessel lipid accumulation and endothelial dysfunction (Chakrabarti et al., 2014).
Among the vascular abnormalities presented are decreased vessel elasticity, increased vascular stiffness and pulse wave velocity (PWV), decreased vascular elasticity, increased lumen, increased senescence of vascular cells, compromised vascular homeostasis and, vascular remodeling (Tian and Li, 2014; Donato et al., 2015; Ding et al., 2018; Cao et al., 2020). In addition, endogenous antioxidant capacity is decreased and reactive oxygen species are elevated, altering the redox balance (Gliemann et al., 2016). With aging, large-arterial stiffness and wave reflections increase and this is particularly higher in women (Smulyan et al., 2001; Mitchell et al., 2004).
Vascular Cell Senescence
Cellular senescence is heterogeneous and cell specific, triggered by critical stressors including DNA damage, oncogenes, among others (Gliemann et al., 2016). Vascular senescence is a pathophysiological process of structural and functional changes including dysregulation of vascular tone, increased endothelium permeability, arterial stiffness, impairment of angiogenesis and vascular repair (Mitchell et al., 2004).
Vascular endothelial cell (EC) senescence is observed in both inflammation and aging and is associated with vascular dysfunction, leading to CVD in the aging individual (Smulyan et al., 2001). Senescent ECs show attenuated endothelial nitric oxide (NO) production, increased endothelin‐1 (ET-1) production, elevated inflammation, increased expression of adhesion molecules VCAM-1 and ICAM-1 and increased cell apoptosis, as well as increased activation of NF-κB (Colpani and Spinetti, 2019). In fact, a randomized study involving 541 men and women, aged 70–82 years, demonstrated that elevated levels of plasminogen activator and Von Willebrand factor, as markers of EC injury and dysfunction, were associated with lower cerebral blood flow in older adults at high risk for CVD (Mitchell et al., 2004).
Interestingly, a study with cell culture-based bioassay on primary human arterial endothelial cells from both men and women with peripheral artery disease showed that cellular ROS production was higher in women than in men, suggesting increased endothelial oxidative stress (Gardner et al., 2015; Uryga and Bennett, 2016; Jia et al., 2019; Sun and Feinberg, 2021).
Vascular Stiffness
The molecular mechanisms involved with vascular stiffness resulting from fibrosis and extracellular matrix are stimulated by vasoactive molecules such as endothelin-1, aldosterone and angiotensin II, commonly associated with aging (Harvey et al., 2016). The pathways related to pathophysiological changes in the vascular endothelium include activation of the transforming growth factor-β1, increased expression and activation of matrix metalloproteinases, galectin-3 overload, SMAD signaling, activation of the renin angiotensin-aldosterone system and, activation of inflammatory and fibrotic signaling pathways (p38 MAPK, TGF-β) (Qi et al., 2011; Yaghooti et al., 2011; Wang et al., 2012; Calvier et al., 2013; Song et al., 2015).
With aging, arterial stiffness increases in both sexes (Mitchell et al., 2004), however, women display a greater stiffening after menopause, which corroborates with the decrease in estrogen levels. Further, with aging, large-artery stiffness and wave reflections increase and this is particularly higher in women (Smulyan et al., 2001; Mitchell et al., 2004). In fact, women taking hormone replacement therapy (HRT) displayed a decrease in the carotid‐femoral PWV (Rajkumar et al., 1997). A study with ovariectomized monkeys showed that 18 months of estrogen treatment was able to reduce arterial stiffness (Adams et al., 1990). Testosterone deficiency in men without CVD is associated with increased carotid-femoral PWV, probably promoting premature vascular aging due to increased arterial stiffness (Vlachopoulos et al., 2014). Another evidence of low testosterone levels is microvascular dysfunction in middle-aged men (Corrigan et al., 2015). Moreover, hypogonadal men who use testosterone replacement therapy show an improvement in arterial stiffness (Yaron et al., 2009).
Wall shear stress is the drag exerted by flowing blood on the vessel wall, playing an important role in the production of vasoactive substances by endothelial cells. Interestingly, a longitudinal observational study reported no significantly differences between sexes regarding mean shear stress in 12 years of observation, however, it was shown that peak shear stress decreases significantly only in men. Further, arterial stiffness increases with aging with women displaying a greater result (+74.5% in women and +28.0% in men) (Irace et al., 2012). In the same way, mean wall shear stress from neck vessels decreased with age and it is significantly higher in females than in males, possibly due to a decrease in flow (Zhao et al., 2015).
In addition, women have less coronary flow reserve than men and have more functional than structural coronary abnormalities (Shaw et al., 2009; Collins et al., 2020). The Working Study Group on micro- and macro- circulation of the Italian Society of Hypertension (SIIA) found that the vascular wall/lumen ratio tends to increase with age in both sexes (Bruno et al., 2018; Rizzoni et al., 2019). Older women have less baroreflex sensitivity than older men, presenting greater carotid artery stiffness (Lacolley et al., 2017). Finally, PWV increases in both sexes, however, it is more pronounced in men over 50 years of age (AlGhatrif et al., 2013). When comparing telomere lengths between men and women, it was found that with advancing age, men with shorter telomeres were more prone to high blood pressure and high PWV values (Laina et al., 2018).
Oxidative Stress and Inflammation
Key mechanisms such as oxidative stress and inflammation may contribute to the vascular aging process via decreased testosterone levels in both women and men (Moreau et al., 2020). Augmented ROS, acting as inflammatory mediators, impair endothelial function and worsen arterial stiffness by decreasing NO production, degrading elastin, and increasing collagen and calcium deposition. Even in apparently healthy adults, aging causes a progressive decline in macro and microvascular endothelial function. Furthermore, there are gender differences in the rate of this decline (Moreau et al., 2020).
In fact, male rats treated with testosterone showed increased antioxidant activity (catalase and superoxide dismutase) which was lost with castration (Ahlbom et al., 2001; Kłapcińska et al., 2008; Eleawa et al., 2013). Similarly, in humans, decreased testosterone concentration was correlated with pro-inflammatory cytokines in older men (Barud et al., 2002; Malkin et al., 2004). Moreover, hypogonadal older men who take testosterone supplementation displayed decreased circulating levels of TNF-α and increased levels of IL-10 (Malkin et al., 2004).
In early and late postmenopausal, high testosterone concentrations elevated levels of inflammatory markers and C-reactive protein (Sowers et al., 2005; Maturana et al., 2008; Maggio et al., 2011). Experiments in ovariectomized (OVX) spontaneously hypertensive rats showed an increased relaxation in aorta after treatment with estrogen compared to OVX rats, however, this protection was abolished when the animals received estrogen plus testosterone, activating NADPH-oxidase subunit p47phox and consequently increasing the production of superoxide (Costa et al., 2015).
Moreover, infusion of vitamin C (antioxidant) in women who underwent OVX restored vasodilatory responses to acetylcholine (Virdis et al., 2000) and increased FMD in estrogen-deficient postmenopausal women (Moreau et al., 2013). Aerobic exercise increases FMD in older men, but only postmenopausal women who are receiving E2 therapy change, probably due to oxidative stress (Moreau et al., 2013).
Vascular homeostasis is due to the balance between endothelial vasoconstrictor and vasodilator factors, thus, endothelial dysfunction can result in CVD. Adequate NO synthesis and release by endothelial cells are predictors of a good endothelial response to stimuli (Sandoo et al., 2015). The accumulation of oxidative stressors, common during aging, triggers a decrease in the production of NO, and may be responsible for the inactivation of SIRT1. Consequently, this inhibition interferes with the growth of endothelial cells due to the increase in acetylation of p53 (Ota et al., 2010; Cencioni et al., 2013). Greater suppression of NO contributes to arterial stiffness in rodent models and elderly women, reducing endothelium-dependent dilation (Durrant et al., 2009; Donato et al., 2018).
In advanced aging and in both sexes, oxidative stress and inflammation can modulate endothelial dysfunction by raising blood pressure, blood glucose, obesity and sodium intake. Thus, changes in the endothelium may occur even in the absence of a related disease (Donato et al., 2015; Tesauro et al., 2017). However, the main cause of morbidity and mortality in women is CVD (Collins et al., 2020). Differences in vascular pathophysiology between sexes have been identified in current advanced cardiac imaging techniques (Maas et al., 2011). Common conditions among women such as polycystic ovary syndrome, pre-eclampsia, autoimmune diseases, anemia, hyperuricemia and menopause can accelerate atherosclerosis, promoting endothelial dysfunction (Lian and Keaney, 2010; Collins et al., 2020). In pre-menopause, a decrease in endothelial function begins, accompanied by a worsening after menopause or another period of prolonged estrogen deficiency (Bechlioulis et al., 2010; Moreau et al., 2012). During the menopause transition, due to the loss of the antioxidant, anti-inflammatory, anti-proliferative and dilating effects of estradiol on the vascular wall, there is a decrease in endothelial function and an increase in arterial stiffness (Moreau, 2018).
Estrogen
Estrogen influences vascular protection by positively regulating NO (Jespersen et al., 2012; Wanitschek et al., 2016; Collins et al., 2020). Such relationship can be observed in women who have had an ovariectomy, where they develop a reduction in endothelium-dependent dilation (Virdis et al., 2000). In women, estradiol acts on estrogen receptors (ERs) present in the vasculature by releasing NO via eNOS activation through both genomic and non-genomic mechanisms. Moreover, the greater vascular relaxation by estradiol includes greater β-adrenergic and lower α-adrenergic sensitivity (Duckles and Miller, 2010). After menopause, there is an increased risk of CVD due to the loss of this protection and a reduction in the action of the β-adrenergic receptor for vascular tone (Stice et al., 2009).
Mineralocorticoid Receptors
Another mechanism contributing to sex differences in vascular aging is through mineralocorticoid receptors (MRs) which act in response to aldosterone by modulating renal sodium reabsorption and then regulating blood pressure (Kim et al., 2018). It was demonstrated that vascular stiffness increases with age in both sexes, however, later in life of females, correlating with the timing of increased vascular MR expression. Further, this augmented vascular stiffness is prevented in smooth muscle cell-specific MR deletion (SMC-MR) deficient males and females. Interestingly, vascular fibrosis increases later in life in females and is attenuated by SMC-MR deletion in males only (DuPont et al., 2021). Angiotensin II (Ang II) signaling modulates constriction in the vasculature and is known to increase with aging (Wang et al., 2010). Of importance, MR contributes to Ang II-induced vasoconstriction only in males whereas in females, the mechanisms are distinct (DuPont et al., 2021).
The renin-angiotensin system (RAS) plays an important role in the development of CVD in aging. Recently, angiotensin converting enzyme 2 (ACE2) was shown to produce angiotensin (Strehler, 1977; Hayflick, 2007; De La Fuente, 2008; López-Otín et al., 2013; Davalli et al., 2016; Bai, 2018; Steverson, 2018) [Ang-(1–7)], vasodilator/antiproliferative peptide witch has opposite effects to Ang II (Kim et al., 2018; DuPont et al., 2021).
Ang II promotes cell senenescence in HUVEC culture, by increasing senescence-associated galactosidase, DNA damage and adhesion molecule expression which were inhibited by activation of Ang-(1–7) through G protein-coupled receptor Mas (MasR) (Wang et al., 2010). Additionally, Ang-(1–7) causes vasodilator effects in rats via activation of angiotensin type 2 receptors (AT(2)R). Interestingly, a study demonstrated that aorta from aged rats displayed increased AT(2)R, MasR, and ACE2, suggesting that Ang (Strehler, 1977; Hayflick, 2007; De La Fuente, 2008; López-Otín et al., 2013; Davalli et al., 2016; Bai, 2018; Steverson, 2018)-mediated depressor effects are preserved in aged animals (Rubio-Ruíz et al., 2014).
Further, resveratrol, an antioxidant drug, was able to protect against arterial aging through reduced activity of the ACE-Ang II axis and stimulation of the ACE2-Ang-(1-7)-ATR2-MasR axis (Bosnyak et al., 2012; Santos et al., 2013; Kim et al., 2018; Romero et al., 2019).
Age-related changes in vascular responses to angiotensin-(1–7) were demonstrated in both male and female mice. In this study, the Ang-(1–7) vasodilatory effect was absent in aorta from old females compared to young females, which was restored by estradiol replacement. Further, the treatment was able to decrease production of ROS and normalize levels of NO. Regarding male mice, Ang-(1–7) induced a dose-dependent vasodilator effect in aorta regardless of whether the artery was from young or old mice (Costa-Fraga et al., 2018).
The endothelin system is also impacted by aging and a role in sex difference has been demonstrated. It is well known that endothelin receptors (ETR) mediate vasoconstriction and vasodilation in arteries (Van Guilder et al., 2007). Interestingly, a study evaluating endothelial function in men showed a key role performed by ETAR in vasodilation and vasoconstriction. In fact, older men displayed blunted responses to acetylcholine which was improved by co-infusion of BQ-123 (ETAR antagonist), suggesting that, at least in part, reduction in endothelial function with age is through ETAR (Westby et al., 2011). In contrast, in postmenopausal women endothelial function decline due to a loss of ETBR-mediated dilation, since ETBR antagonist (BQ-788) restored vasodilation in postmenopausal women (Wenner et al., 2017).
Closing Remarks
This review considered many aspects of how biological sex influences cardiovascular pathophysiology. The following conclusions can be made: 1) sex is an important variable that defines cardiovascular structure and function in health and disease; 2) biological sex must be considered for all study designs and approaches; 3) sex steroid hormones define phenotypic responses and therefore, hormonal status should be considered in the design of animal and human experiments, drug protocols and other interventions; 4) when there is a difference between the sexes in cardiovascular pathophysiology, much can be learned by examining the sex that is least or most protected; 5) extrapolation between sexes regarding cardiovascular physiology and pathology should not be made; and 6) drugs for treatment of cardiovascular conditions in elderly men and women should be personalized.
Author Contributions
RW and FG designed the review; RW, FP and JS revised the paper; VD and JM wrote the paper.
Funding
Financial support for this work was provided by the NIDDK Diabetic Complications Consortium (DK076169 and DK115255 to RW); the National Institutes of Health (HL-134604 to RW); Fundação de Amparo a Pesquisa do Estado de Mato Grosso (FAPEMAT, 0324552/2018 to FG.); Conselho Nacional de Desenvolvimento Científico e Tecnológico (CNPq, 305823/2015-9 to FG); Coordenação de Aperfeiçoamento de Pessoal de Nível Superior (CAPES, 88881.190484/2018-01 Scholarship to VD).
Conflict of Interest
The authors declare that the research was conducted in the absence of any commercial or financial relationships that could be construed as a potential conflict of interest.
Publisher’s Note
All claims expressed in this article are solely those of the authors and do not necessarily represent those of their affiliated organizations, or those of the publisher, the editors and the reviewers. Any product that may be evaluated in this article, or claim that may be made by its manufacturer, is not guaranteed or endorsed by the publisher.
References
Abate, M., Festa, A., Falco, M., Lombardi, A., Luce, A., Grimaldi, A., et al. (2020). Mitochondria as Playmakers of Apoptosis, Autophagy and Senescence. Semin. Cel Develop. Biol. 98 (March), 139–153. doi:10.1016/j.semcdb.2019.05.022
Adams, M. R., Kaplan, J. R., Manuck, S. B., Koritnik, D. R., Parks, J. S., Wolfe, M. S., et al. (1990). Inhibition of Coronary Artery Atherosclerosis by 17-beta Estradiol in Ovariectomized Monkeys. Lack of an Effect of Added Progesterone. Arteriosclerosis 10 (6), 1051–1057. doi:10.1161/01.atv.10.6.1051
Ahlbom, E., Prins, G. S., and Ceccatelli, S. (2001). Testosterone Protects Cerebellar Granule Cells from Oxidative Stress-Induced Cell Death through a Receptor Mediated Mechanism. Brain Res. 892 (2), 255–262. doi:10.1016/s0006-8993(00)03155-3
Alencar, A. K., da Silva, J. S., Lin, M., Silva, A. M., Sun, X., Ferrario, C. M., et al. (2017). Effect of Age, Estrogen Status, and Late-Life GPER Activation on Cardiac Structure and Function in the Fischer344×Brown Norway Female Rat. Gerona 72 (2), 152–162. doi:10.1093/gerona/glw045
AlGhatrif, M., Strait, J. B., Morrell, C. H., Canepa, M., Wright, J., Elango, P., et al. (2013). Longitudinal Trajectories of Arterial Stiffness and the Role of Blood Pressure. Hypertension 62 (5), 934–941. doi:10.1161/hypertensionaha.113.01445
Aunan, J. R., Watson, M. M., Hagland, H. R., and Søreide, K. (2016). Molecular and Biological Hallmarks of Ageing. Br. J. Surg. 103 (2), e29–e46. doi:10.1002/bjs.10053
Ayaz, O., Banga, S., Heinze-Milne, S., Rose, R. A., Pyle, W. G., and Howlett, S. E. (2019). Long-term Testosterone Deficiency Modifies Myofilament and Calcium-Handling Proteins and Promotes Diastolic Dysfunction in the Aging Mouse Heart. Am. J. Physiology-Heart Circulatory Physiol. 316 (4), H768–H780. doi:10.1152/ajpheart.00471.2018
Babiker, F. A., De Windt, L. J., Van Eickels, M., Thijssen, V., Bronsaer, R. J. P., Grohé, C., et al. (2004). 17β-Estradiol Antagonizes Cardiomyocyte Hypertrophy by Autocrine/Paracrine Stimulation of a Guanylyl Cyclase A Receptor-Cyclic Guanosine Monophosphate-dependent Protein Kinase Pathway. Circulation 109 (2), 269–276. doi:10.1161/01.cir.0000105682.85732.bd
Bai, X. (2018). Biomarkers of Aging. Adv. Exp. Med. Biol. 1086, 217–234. doi:10.1007/978-981-13-1117-8_14
Barrett, E. L. B., and Richardson, D. S. (2011). Sex Differences in Telomeres and Lifespan. Aging Cell 10 (6), 913–921. doi:10.1111/j.1474-9726.2011.00741.x
Barrientos, G., Llanos, P., Basualto-Alarcón, C., and Estrada, M. (2020). Androgen-Regulated Cardiac Metabolism in Aging Men. Front. Endocrinol. (Lausanne) 11 (May), 316–413. doi:10.3389/fendo.2020.00316
Barud, W., Palusiński, R., Bełtowski, J., and Wójcicka, G. (2002). Inverse Relationship between Total Testosterone and Anti-oxidized Low Density Lipoprotein Antibody Levels in Ageing Males. Atherosclerosis 164 (2), 283–288. doi:10.1016/s0021-9150(02)00069-2
Batsis, J. A., Mackenzie, T. A., Vasquez, E., Germain, C. M., Emeny, R. T., Rippberger, P., et al. (2018). Association of Adiposity, Telomere Length and Mortality: Data from the NHANES 1999-2002. Int. J. Obes. 42 (2), 198–204. doi:10.1038/ijo.2017.202
Beale, A. L., Nanayakkara, S., and Kaye, D. M. (2019). Impact of Sex on Ventricular-Vascular Stiffness and Long-Term Outcomes in Heart Failure with Preserved Ejection Fraction: Topcat Trial Substudy. J. Am. Heart Assoc. 8 (13), e012190–8. doi:10.1161/JAHA.119.012190
Beale, A. L., Meyer, P., Marwick, T. H., Lam, C. S. P., and Kaye, D. M. (2018). Sex Differences in Cardiovascular Pathophysiology. Circulation 138 (2), 198–205. doi:10.1161/circulationaha.118.034271
Bechlioulis, A., Kalantaridou, S. N., Naka, K. K., Chatzikyriakidou, A., Calis, K. A., Makrigiannakis, A., et al. (2010). Endothelial Function, but Not Carotid Intima-media Thickness, Is Affected Early in Menopause and Is Associated with Severity of Hot Flushes. J. Clin. Endocrinol. Metab. 95 (3), 1199–1206. doi:10.1210/jc.2009-2262
Bell, V., McCabe, E. L., Larson, M. G., Rong, J., Merz, A. A., Osypiuk, E., et al. (2017). Relations between Aortic Stiffness and Left Ventricular Mechanical Function in the Community. J. Am. Heart Assoc. 6 (1), 1–10. doi:10.1161/JAHA.116.004903
Berchtold, N. C., Cribbs, D. H., Coleman, P. D., Rogers, J., Head, E., Kim, R., et al. (2008). Gene Expression Changes in the Course of normal Brain Aging Are Sexually Dimorphic. Pnas 105 (40), 15605–15610. doi:10.1073/pnas.0806883105
Berg, B. N., and Simms, H. S. (1960). Nutrition and Longevity in the Rat. J. Nutr. 71, 255–263. doi:10.1093/jn/71.3.255
Bhuiyan, M. S., Shioda, N., and Fukunaga, K. (2007). Ovariectomy Augments Pressure Overload-Induced Hypertrophy Associated with Changes in Akt and Nitric Oxide Synthase Signaling Pathways in Female Rats. Am. J. Physiol. Endocrinol. Metab. 293 (6), E1606–E1614. doi:10.1152/ajpendo.00246.2007
Blagosklonny, M. V. (2008). Aging: ROS or TOR. Cell Cycle 7 (21), 3344–3354. doi:10.4161/cc.7.21.6965
Boddaert, J., Mallat, Z., Fornes, P., Esposito, B., Lecomte, D., Verny, M., et al. (2005). Age and Gender Effects on Apoptosis in the Human Coronary Arterial wall. Mech. Ageing Dev. 126 (6–7), 678–684. doi:10.1016/j.mad.2005.01.001
Bonda, T. A., Szynaka, B., Sokołowska, M., Dziemidowicz, M., Winnicka, M. M., Chyczewski, L., et al. (2016). Remodeling of the Intercalated Disc Related to Aging in the Mouse Heart. J. Cardiol. 68 (3), 261–268. doi:10.1016/j.jjcc.2015.10.001
Bosnyak, S., Widdop, R. E., Denton, K. M., and Jones, E. S. (2012). Differential Mechanisms of Ang (1-7)-mediated Vasodepressor Effect in Adult and Aged Candesartan-Treated Rats. Int. J. Hypertens. 2012, 1–9. doi:10.1155/2012/192567
Bruno, R. M., Grassi, G., Seravalle, G., Savoia, C., Rizzoni, D., and Virdis, A. (2018). Age- and Sex-specific Reference Values for media/lumen Ratio in Small Arteries and Relationship with Risk Factors. Hypertension 71 (6), 1193–1200. doi:10.1161/hypertensionaha.117.10634
Butcher, S. K., and Lord, J. M. (2004). Stress Responses and Innate Immunity: Aging as a Contributory Factor. Aging Cell 3 (4), 151–160. doi:10.1111/j.1474-9728.2004.00103.x
Cai, H., Li, Y., Niringiyumukiza, J. D., Su, P., and Xiang, W. Circular RNA Involvement in Aging: An Emerging Player with Great Potential, Mech. Ageing Develop. 178, (2019). 16–24. doi:10.1016/j.mad.2018.11.002
Calcinotto, A., Kohli, J., Zagato, E., Pellegrini, L., Demaria, M., and Alimonti, A. (2019). Cellular Senescence: Aging, Cancer, and Injury. Physiol. Rev. 99 (2), 1047–1078. doi:10.1152/physrev.00020.2018
Calder, P. C., Bosco, N., Bourdet-Sicard, R., Capuron, L., Delzenne, N., Doré, J., et al. (2017). Health Relevance of the Modification of Low Grade Inflammation in Ageing (Inflammageing) and the Role of Nutrition. Ageing Res. Rev. 40, 95–119. doi:10.1016/j.arr.2017.09.001
Calvier, L., Miana, M., Reboul, P., Cachofeiro, V., Martinez-Martinez, E., De Boer, R. A., et al. (2013). Galectin-3 Mediates Aldosterone-Induced Vascular Fibrosis. Arterioscler Thromb. Vasc. Biol. 33 (1), 67–75. doi:10.1161/atvbaha.112.300569
Camper-Kirby, D., Welch, S., Walker, A., Shiraishi, I., Setchell, K. D. R., Schaefer, E., et al. (2001). Myocardial Akt Activation and Gender. Circ. Res. 88 (10), 1020–1027. doi:10.1161/hh1001.090858
Camus, M. F., Clancy, D. J., and Dowling, D. K. (2012). Mitochondria, Maternal Inheritance, and Male Aging. Curr. Biol. 22 (18), 1717–1721. doi:10.1016/j.cub.2012.07.018
Camuzard, O., Santucci-Darmanin, S., Breuil, V., Cros, C., Gritsaenko, T., Pagnotta, S., et al. (2016). Sex-specific Autophagy Modulation in Osteoblastic Lineage: A Critical Function to Counteract Bone Loss in Female. Oncotarget 7 (41), 66416–66428. doi:10.18632/oncotarget.12013
Cao, Q., Wu, J., Wang, X., and Song, C. (2020). Noncoding RNAs in Vascular Aging. Oxidative Med. Cell Longevity 2020, 1–14. doi:10.1155/2020/7914957
Caslini, C., Connelly, J. A., Serna, A., Broccoli, D., and Hess, J. L. (2009). MLL Associates with Telomeres and Regulates Telomeric Repeat-Containing RNA Transcription. Mol. Cel Biol 29 (16), 4519–4526. doi:10.1128/mcb.00195-09
Cencioni, C., Spallotta, F., Martelli, F., Valente, S., Mai, A., Zeiher, A., et al. (2013). Oxidative Stress and Epigenetic Regulation in Ageing and Age-Related Diseases. Int. J. Mol. Sci. 14 (9), 17643–17663. doi:10.3390/ijms140917643
Chakrabarti, S., Morton, J. S., and Davidge, S. T. (2014). Mechanisms of Estrogen Effects on the Endothelium: An Overview. Can. J. Cardiol. 30 (7), 705–712. doi:10.1016/j.cjca.2013.08.006
Chamandi, C., Barbanti, M., Munoz-Garcia, A., Latib, A., Nombela-Franco, L., Gutiérrez-Ibanez, E., et al. (2018). Long-Term Outcomes in Patients with New Permanent Pacemaker Implantation Following Transcatheter Aortic Valve Replacement. JACC: Cardiovasc. Interventions 11 (3), 301–310. doi:10.1016/j.jcin.2017.10.032
Chan, S. R. W. L., and Blackburn, E. H. (2004). Telomeres and Telomerase. Phil. Trans. R. Soc. Lond. B 359 (1441), 109–122. doi:10.1098/rstb.2003.1370
Chang, S.-N., Sung, K.-T., Huang, W.-H., Lin, J.-W., Chien, S.-C., Hung, T.-C., et al. (2021). Sex, Racial Differences and Healthy Aging in Normative Reference Ranges on Diastolic Function in Ethnic Asians: 2016 ASE Guideline Revisited. J. Formos. Med. Assoc., 1–16. doi:10.1016/j.jfma.2020.12.024
Chen, C.-H., Nakayama, M., Nevo, E., Fetics, B. J., Maughan, W. L., and Kass, D. A. (1998). Coupled Systolic-Ventricular and Vascular Stiffening with Age. J. Am. Coll. Cardiol. 32 (5), 1221–1227. doi:10.1016/s0735-1097(98)00374-x
Chen, H., Jin, S., Guo, J., Kombairaju, P., Biswal, S., and Zirkin, B. R. (2015). Knockout of the Transcription Factor Nrf2: Effects on Testosterone Production by Aging Mouse Leydig Cells. Mol. Cell Endocrinol. 409, 113–120. doi:10.1016/j.mce.2015.03.013
Chen, Y., Zhang, Z., Hu, F., Yang, W., Yuan, J., Cui, J., et al. (2015). 17β-estradiol Prevents Cardiac Diastolic Dysfunction by Stimulating Mitochondrial Function: A Preclinical Study in a Mouse Model of a Human Hypertrophic Cardiomyopathy Mutation. J. Steroid Biochem. Mol. Biol. 147, 92–102. doi:10.1016/j.jsbmb.2014.12.011
Cheng, S., Xanthakis, V., Sullivan, L. M., Lieb, W., Massaro, J., Aragam, J., et al. (2010). Correlates of Echocardiographic Indices of Cardiac Remodeling over the Adult Life Course. Circulation 122 (6), 570–578. doi:10.1161/circulationaha.110.937821
Chignalia, A. Z., Oliveira, M. A., Debbas, V., Dull, R. O., Laurindo, F. R. M., Touyz, R. M., et al. (2015). Testosterone Induces Leucocyte Migration by NADPH Oxidase-Driven ROS- and COX2-dependent Mechanisms. Clin. Sci. 129 (1), 39–48. doi:10.1042/cs20140548
Childs, B. G., Durik, M., Baker, D. J., and Van Deursen, J. M. (2015). Cellular Senescence in Aging and Age-Related Disease: From Mechanisms to Therapy. Nat. Med. 21 (12), 1424–1435. doi:10.1038/nm.4000
Chuaire-Noack, L., Sánchez-Corredor, M. C., and Martínez-Agüero, M. (2014). Revisiting the X-Chromosome Inactivation and its Impact on Female Longevity. Adv. Biosci. Biotechnol. 05 (06), 572–583. doi:10.4236/abb.2014.56067
Chung, A. K., Das, S. R., Leonard, D., Peshock, R. M., Kazi, F., Abdullah, S. M., et al. (2006). Women Have Higher Left Ventricular Ejection Fractions Than Men Independent of Differences in Left Ventricular Volume. Circulation 113 (12), 1597–1604. doi:10.1161/circulationaha.105.574400
Clancy, D. J., Gems, D., Harshman, L. G., Oldham, S., Stocker, H., Hafen, E., et al. (2001). Extension of Life-Span by Loss of CHICO, a Drosophila Insulin Receptor Substrate Protein. Science 292 (5514), 104–106. doi:10.1126/science.1057991
Cluckey, T. G., Nieto, N. C., Rodoni, B. M., and Traustadóttir, T. (2017). Preliminary Evidence that Age and Sex Affect Exercise-Induced hTERT Expression. Exp. Gerontol. 96, 7–11. doi:10.1016/j.exger.2017.06.003
Colafella, K. M. M., and Denton, K. M. (2018). Sex-specific Differences in Hypertension and Associated Cardiovascular Disease. Nat. Rev. Nephrol. 14 (3), 185–201. doi:10.1038/nrneph.2017.189
Collado, M., Gil, J., Efeyan, A., Guerra, C., Schuhmacher, A. J., Barradas, M., et al. (2005). Senescence in Premalignant Tumours. Nature 436 (7051), 642. doi:10.1038/436642a
Collins, P., Maas, A., Prasad, M., Schierbeck, L., and Lerman, A. (2020). Endothelial Vascular Function as a Surrogate of Vascular Risk and Aging in Women. Mayo Clinic Proc. 95 (3), 541–553. doi:10.1016/j.mayocp.2019.07.001
Colpani, O., and Spinetti, G. (2019). MicroRNAs Orchestrating Senescence of Endothelial and Vascular Smooth Muscle Cells. Vasc. Biol. 1 (1), H75–H81. doi:10.1530/vb-19-0017
Corrigan, F. E., Al Mheid, I., Eapen, D. J., Hayek, S. S., Sher, S., Martin, G. S., et al. (2015). Low Testosterone in Men Predicts Impaired Arterial Elasticity and Microvascular Function. Int. J. Cardiol. 194, 94–99. doi:10.1016/j.ijcard.2015.05.065
Costa, T. J., Ceravolo, G. S., Dos Santos, R. A., De Oliveira, M. A., Araújo, P. X., Giaquinto, L. R., et al. (2015). Association of Testosterone with Estrogen Abolishes the Beneficial Effects of Estrogen Treatment by Increasing ROS Generation in Aorta Endothelial Cells. Am. J. Physiol. Heart Circ. Physiol. 308 (7), H723–H732. doi:10.1152/ajpheart.00681.2014
Costa-Fraga, F. P., Goncalves, G. K., Souza-Neto, F. P., Reis, A. M., Capettini, L. A., Santos, R. A., et al. (2018). Age-related Changes in Vascular Responses to Angiotensin-(1-7) in Female Mice. J. Renin Angiotensin Aldosterone Syst. 19 (3), 1470320318789332. doi:10.1177/1470320318789332
Daiber, A., Steven, S., Vujacic-Mirski, K., Kalinovic, S., Oelze, M., Di Lisa, F., et al. (2020). Regulation of Vascular Function and Inflammation via Cross Talk of Reactive Oxygen and Nitrogen Species from Mitochondria or NADPH Oxidase-Implications for Diabetes Progression. Int. J. Mol. Sci. 21 (10), 1–30. doi:10.3390/ijms21103405
Davalli, P., Mitic, T., Caporali, A., Lauriola, A., and D’Arca, D. (2016). ROS, Cell Senescence, and Novel Molecular Mechanisms in Aging and Age-Related Diseases. Oxidative Med. Cell Longevity 2016, 1–18. doi:10.1155/2016/3565127
De La Fuente, M. (2008). Role of Neuroimmunomodulation in Aging. Neuroimmunomodulation 15 (4–6), 213–223. doi:10.1159/000156465
De Lange, T., Lundblad, V., and Blackburn, E. (2006). Telomeres. Second. New York: Cold Spring Harbor Laboratory Press, 21–48.
De Martinis, M., Franceschi, C., Monti, D., and Ginaldi, L. (2005). Inflamm-ageing and Lifelong Antigenic Load as Major Determinants of Ageing Rate and Longevity. FEBS Lett. 579 (10), 2035–2039. doi:10.1016/j.febslet.2005.02.055
de Punder, K., Heim, C., Wadhwa, P. D., and Entringer, S. (2019). Stress and Immunosenescence: The Role of Telomerase. Psychoneuroendocrinology 101, 87–100. doi:10.1016/j.psyneuen.2018.10.019
Deschamps, A. M., and Murphy, E. (2009). Activation of a Novel Estrogen Receptor, GPER, Is Cardioprotective in Male and Female Rats. Am. J. Physiol. Heart Circ. Physiol. 297 (5), H1806–H1813. doi:10.1152/ajpheart.00283.2009
Dimmeler, S., and Zeiher, A. M. (2000). Reactive Oxygen Species and Vascular Cell Apoptosis in Response to Angiotensin II and Pro-atherosclerotic Factors. Regul. Pept. 90 (1–3), 19–25. doi:10.1016/s0167-0115(00)00105-1
Ding, Y.-N., Tang, X., Chen, H.-Z., and Liu, D.-P. (2018). Epigenetic Regulation of Vascular Aging and Age-Related Vascular Diseases. Adv. Exp. Med. Biol. 1086, 55–75. doi:10.1007/978-981-13-1117-8_4
Dodig, S., Čepelak, I., and Pavić, I. (2019). Hallmarks of Senescence and Aging. Biochem. Med. (Zagreb) 29 (3), 030501–030515. doi:10.11613/BM.2019.030501
Donato, A. J., Machin, D. R., and Lesniewski, L. A. (2018). Mechanisms of Dysfunction in the Aging Vasculature and Role in Age-Related Disease. Circ. Res. 123 (7), 825–848. doi:10.1161/circresaha.118.312563
Donato, A. J., Morgan, R. G., Walker, A. E., and Lesniewski, L. A. (2015). Cellular and Molecular Biology of Aging Endothelial Cells. J. Mol. Cell Cardiol. 89, 122–135. doi:10.1016/j.yjmcc.2015.01.021
Duckles, S. P., and Miller, V. M. (2010). Hormonal Modulation of Endothelial NO Production. Pflugers Arch. - Eur. J. Physiol. 459 (6), 841–851. doi:10.1007/s00424-010-0797-1
DuPont, J. J., Kim, S. K., Kenney, R. M., and Jaffe, I. Z. (2021). Sex Differences in the Time Course and Mechanisms of Vascular and Cardiac Aging in Mice: Role of the Smooth Muscle Cell Mineralocorticoid Receptor. Am. J. Physiol. Hear. Circ. Physiol. 320 (1), H169–H180. doi:10.1152/ajpheart.00262.2020
Durrant, J. R., Seals, D. R., Connell, M. L., Russell, M. J., Lawson, B. R., Folian, B. J., et al. (2009). Voluntary Wheel Running Restores Endothelial Function in Conduit Arteries of Old Mice: Direct Evidence for Reduced Oxidative Stress, Increased Superoxide Dismutase Activity and Down-Regulation of NADPH Oxidase. J. Physiol. 587 (13), 3271–3285. doi:10.1113/jphysiol.2009.169771
Dworatzek, E., Baczko, I., and Kararigas, G. (2016). Effects of Aging on Cardiac Extracellular Matrix in Men and Women. Prot. Clin. Appl. 10 (1), 84–91. doi:10.1002/prca.201500031
Dworatzek, E., Mahmoodzadeh, S., Schriever, C., Kusumoto, K., Kramer, L., Santos, G., et al. (2019). Sex-specific Regulation of Collagen I and III Expression by 17β-Estradiol in Cardiac Fibroblasts: Role of Estrogen Receptors. Cardiovasc. Res. 115 (2), 315–327. doi:10.1093/cvr/cvy185
El Assar, M., Angulo, J., and Rodríguez-Mañas, L. (2013). Oxidative Stress and Vascular Inflammation in Aging. Free Radic. Biol. Med. 65, 380–401. doi:10.1016/j.freeradbiomed.2013.07.003
Eleawa, S. M., Sakr, H. F., Hussein, A. M., Assiri, A. S., Bayoumy, N. M. K., and Alkhateeb, M. (2013). Effect of Testosterone Replacement Therapy on Cardiac Performance and Oxidative Stress in Orchidectomized Rats. Acta Physiol. 209 (2), 136–147. doi:10.1111/apha.12158
Elhage, R., Arnal, J.-F., Pieraggi, M.-T., Duverger, N., Fiévet, C., Faye, J.-C., et al. (1997). 17β-Estradiol Prevents Fatty Streak Formation in Apolipoprotein E-Deficient Mice. Arterioscler Thromb. Vasc. Biol. 17 (11), 2679–2684. doi:10.1161/01.atv.17.11.2679
Fannin, J., Rice, K. M., Thulluri, S., Dornon, L., Arvapalli, R. K., Wehner, P., et al. (2014). Age-associated Alterations of Cardiac Structure and Function in the Female F344xBN Rat Heart. Age (Dordr) 36 (4), 9684. doi:10.1007/s11357-014-9684-6
Farzaneh-Far, R., Lin, J., Epel, E. S., Harris, W. S., Blackburn, E. H., and Whooley, M. A. (2010). Association of marine omega-3 Fatty Acid Levels with Telomeric Aging in Patients with Coronary Heart Disease. Jama 303 (3), 250–257. doi:10.1001/jama.2009.2008
Fernández, Á. F., Sebti, S., Wei, Y., Zou, Z., Shi, M., McMillan, K. L., et al. (2018). Disruption of the Beclin 1-BCL2 Autophagy Regulatory Complex Promotes Longevity in Mice. Nature 558 (7708), 136–140. doi:10.1038/s41586-018-0162-7
Finkel, T., Menazza, S., Holmström, K. M., Parks, R. J., Liu, J., Sun, J., et al. (2015). The Ins and Outs of Mitochondrial Calcium. Circ. Res. 116 (11), 1810–1819. doi:10.1161/circresaha.116.305484
Finley, J. C., Reid, B. J., Odze, R. D., Sanchez, C. A., Galipeau, P., Li, X., et al. (2006). Chromosomal Instability in Barrett's Esophagus Is Related to Telomere Shortening. Cancer Epidemiol. Biomarkers Prev. 15 (8), 1451–1457. doi:10.1158/1055-9965.epi-05-0837
Fliegner, D., Schubert, C., Penkalla, A., Witt, H., Kararigas, G., Kararigas, G., et al. (2010). Female Sex and Estrogen Receptor-Beta Attenuate Cardiac Remodeling and Apoptosis in Pressure Overload. Am. J. Physiol. Regul. Integr. Comp. Physiol. 298 (6), R1597–R1606. doi:10.1152/ajpregu.00825.2009
Gökçe, M., Karahan, B., Erdöl, C., Kasap, H., and Ozdemirci, S. (2003). Left Ventricular Diastolic Function Assessment by Tissue Doppler Echocardiography in Relation to Hormonal Replacement Therapy in Postmenopausal Women with Diastolic Dysfunction. Am. J. Ther. 10 (2), 104–111. doi:10.1097/00045391-200303000-00005
Gagliano-Jucá, T., and Basaria, S. (2019). Testosterone Replacement Therapy and Cardiovascular Risk. Nat. Rev. Cardiol. 16 (9), 555–574. doi:10.1038/s41569-019-0211-4
Gao, G., Chen, W., Yan, M., Liu, J., Luo, H., Wang, C., et al. (2020). Rapamycin Regulates the Balance between Cardiomyocyte Apoptosis and Autophagy in Chronic Heart Failure by Inhibiting mTOR Signaling. Int. J. Mol. Med. 45 (1), 195–209. doi:10.3892/ijmm.2019.4407
García-Fernández, R. A., García-Palencia, P., Suarez, C., Sánchez, M. A., Gil-Gómez, G., Sánchez, B., et al. (2014). Cooperative Role between P21cip1/waf1 and P27kip1 in Premature Senescence in Glandular Proliferative Lesions in Mice. Histol. Histopathol 29 (3), 397–406. doi:10.14670/HH-29.397
Gardner, A. W., Parker, D. E., Montgomery, P. S., Sosnowska, D., Casanegra, A. I., Ungvari, Z., et al. (2015). Gender and Racial Differences in Endothelial Oxidative Stress and Inflammation in Patients with Symptomatic Peripheral Artery Disease. J. Vasc. Surg. 61 (5), 1249–1257. doi:10.1016/j.jvs.2014.02.045
Gardner, M., Bann, D., Wiley, L., Cooper, R., Hardy, R., Nitsch, D., et al. (2014). Gender and Telomere Length: Systematic Review and Meta-Analysis. Exp. Gerontol. 51 (1), 15–27. doi:10.1016/j.exger.2013.12.004
Gaziev, A. I., Abdullaev, S., and Podlutsky, A. (2014). Mitochondrial Function and Mitochondrial DNA Maintenance with Advancing Age. Biogerontology 15 (5), 417–438. doi:10.1007/s10522-014-9515-2
Gebhard, C., Stähli, B. E., Gebhard, C. E., Tasnady, H., Zihler, D., Wischnewsky, M. B., et al. (2013). Age- and Gender-dependent Left Ventricular Remodeling. Echocardiography 30 (10), 1143–1150. doi:10.1111/echo.12264
Genestra, M. (2007). Oxyl Radicals, Redox-Sensitive Signalling Cascades and Antioxidants. Cell Signal. 19 (9), 1807–1819. doi:10.1016/j.cellsig.2007.04.009
Gentilini, D., Castaldi, D., Mari, D., Monti, D., Franceschi, C., di Blasio, A. M., et al. (2012). Age-dependent Skewing of X Chromosome Inactivation Appears Delayed in Centenarians' Offspring. Is There a Role for Allelic Imbalance in Healthy Aging and Longevity? Aging Cell 11 (2), 277–283. doi:10.1111/j.1474-9726.2012.00790.x
Georgakopoulou, E. A., Tsimaratou, K., Evangelou, K., Fernandez, M. P. J., Zoumpourlis, V., Trougakos, I. P., et al. (2013). Specific Lipofuscin Staining as a Novel Biomarker to Detect Replicative and Stress‐induced Senescence. A Method Applicable in Cryo-Preserved and Archival Tissues. Aging (Albany NY) 5 (1), 37–50. doi:10.18632/aging.100527
Ghafouri-Fard, S., Gholipour, M., and Taheri, M. (2021). The Emerging Role of Long Non-coding RNAs and Circular RNAs in Coronary Artery Disease. Front. Cardiovasc. Med. 8 (February), 1–16. doi:10.3389/fcvm.2021.632393
Gliemann, L., Nyberg, M., and Hellsten, Y. (2016). Effects of Exercise Training and Resveratrol on Vascular Health in Aging. Free Radic. Biol. Med. 98, 165–176. doi:10.1016/j.freeradbiomed.2016.03.037
Gong, J., Wang, X.-z., Wang, T., Chen, J. J., Xie, X.-y., Hu, H., et al. (2017). Molecular Signal Networks and Regulating Mechanisms of the Unfolded Protein Response. J. Zhejiang Univ. Sci. B 18 (1), 1–14. doi:10.1631/jzus.b1600043
Gori, M., Lam, C. S. P., Gupta, D. K., Santos, A. B. S., Cheng, S., Shah, A. M., et al. (2014). Sex-specific Cardiovascular Structure and Function in Heart Failure with Preserved Ejection Fraction. Eur. J. Heart Fail. 16 (5), 535–542. doi:10.1002/ejhf.67
Gourdy, P., Guillaume, M., Fontaine, C., Adlanmerini, M., Montagner, A., Laurell, H., et al. (2018). Estrogen Receptor Subcellular Localization and Cardiometabolism. Mol. Metab. 15 (May), 56–69. doi:10.1016/j.molmet.2018.05.009
Greco, C. M., and Condorelli, G. (2015). Epigenetic Modifications and Noncoding RNAs in Cardiac Hypertrophy and Failure. Nat. Rev. Cardiol. 12 (8), 488–497. doi:10.1038/nrcardio.2015.71
Greco, S., Zaccagnini, G., Perfetti, A., Fuschi, P., Valaperta, R., Voellenkle, C., et al. (2016). Long Noncoding RNA Dysregulation in Ischemic Heart Failure. J. Transl Med. 14 (1), 1–14. doi:10.1186/s12967-016-0926-5
Guay, A. T. (2009). The Emerging Link between Hypogonadism and Metabolic Syndrome. J. Androl. 30 (4), 370–376. doi:10.2164/jandrol.108.006015
Gubbels Bupp, M. R. (2015). Sex, the Aging Immune System, and Chronic Disease. Cell Immunol. 294 (2), 102–110. doi:10.1016/j.cellimm.2015.02.002
Guerville, F., De Souto Barreto, P., Ader, I., Andrieu, S., Casteilla, L., Dray, C., et al. (2020). Revisiting the Hallmarks of Aging to Identify Markers of Biological Age. J. Prev. Alzheimers Dis. 7 (1), 56–64. doi:10.14283/jpad.2019.50
Guo, D., Ye, Y., Qi, J., Tan, X., Zhang, Y., Ma, Y., et al. (2017). Age and Sex Differences in microRNAs Expression during the Process of Thymus Aging. Acta Biochim. Biophys. Sin (Shanghai). 49 (5), 409–419. doi:10.1093/abbs/gmx029
Guo, H., Callaway, J. B., and Ting, J. P.-Y. (2015). Inflammasomes: Mechanism of Action, Role in Disease, and Therapeutics. Nat. Med. 21 (7), 677–687. doi:10.1038/nm.3893
Haendeler, J., Hoffmann, J., Diehl, J. F., Vasa, M., Spyridopoulos, I., Zeiher, A. M., et al. (2004). Antioxidants Inhibit Nuclear Export of Telomerase Reverse Transcriptase and Delay Replicative Senescence of Endothelial Cells. Circ. Res. 94 (6), 768–775. doi:10.1161/01.res.0000121104.05977.f3
Hall, J. E. (2003). The Kidney, Hypertension, and Obesity. Hypertension 41 (3 II), 625–633. doi:10.1161/01.HYP.0000052314.95497.78
Hamilton, J. B., and Mestler, G. E. (1969). Mortality and Survival: Comparison of Eunuchs with Intact Men and Women in a Mentally Retarded Population. J. Gerontol. 24 (4), 395–411. doi:10.1093/geronj/24.4.395
Han, P., Li, W., Lin, C.-H., Yang, J., Shang, C., Nurnberg, S. T., et al. (2014). A Long Noncoding RNA Protects the Heart from Pathological Hypertrophy. Nature 514 (7520), 102–106. doi:10.1038/nature13596
Harman, D. (1956). Aging: A Theory Based on Free Radical and Radiation Chemistry. J. Gerontol. 11, 298–300. doi:10.1093/geronj/11.3.298
Harris, T. R., and Hammock, B. D. (2013). Soluble Epoxide Hydrolase: Gene Structure, Expression and Deletion. Gene 526 (2), 61–74. doi:10.1016/j.gene.2013.05.008
Harrison, D. E., Strong, R., Sharp, Z. D., Nelson, J. F., Astle, C. M., Flurkey, K., et al. (2009). Rapamycin Fed Late in Life Extends Lifespan in Genetically Heterogeneous Mice. Nature 460 (7253), 392–395. doi:10.1038/nature08221
Harshman, L. G., and Zera, A. J. (2007). The Cost of Reproduction: the Devil in the Details. Trends Ecol. Evol. 22 (2), 80–86. doi:10.1016/j.tree.2006.10.008
Harvey, A., Montezano, A. C., Lopes, R. A., Rios, F., and Touyz, R. M. (2016). Vascular Fibrosis in Aging and Hypertension: Molecular Mechanisms and Clinical Implications. Can. J. Cardiol. 32 (5), 659–668. doi:10.1016/j.cjca.2016.02.070
Harvey, A., Montezano, A. C., and Touyz, R. M. (2015). Vascular Biology of Ageing-Implications in Hypertension. J. Mol. Cell Cardiol. 83, 112–121. doi:10.1016/j.yjmcc.2015.04.011
Hayflick, L. (2007). Biological Aging Is No Longer an Unsolved Problem. Ann. N Y Acad. Sci. 1100, 1–13. doi:10.1196/annals.1395.001
He, M., Chiang, H.-H., Luo, H., Zheng, Z., Qiao, Q., Wang, L., et al. (2020). An Acetylation Switch of the NLRP3 Inflammasome Regulates Aging-Associated Chronic Inflammation and Insulin Resistance. Cel Metab. 31 (3), 580–591. doi:10.1016/j.cmet.2020.01.009
Heidenreich, P. A., Trogdon, J. G., Khavjou, O. A., Butler, J., Dracup, K., Ezekowitz, M. D., et al. (2011). Forecasting the Future of Cardiovascular Disease in the United States. Circulation 123 (8), 933–944. doi:10.1161/cir.0b013e31820a55f5
Hewitt, G., Jurk, D., Marques, F. D., Correia-Melo, C., Hardy, T., Gackowska, A., et al. (2012). Telomeres Are Favoured Targets of a Persistent DNA Damage Response in Ageing and Stress-Induced Senescence. Nat. Commun. 3, 708–709. doi:10.1038/ncomms1708
Hewton, K. G., Johal, A. S., and Parker, S. J. (2021). Transporters at the Interface between Cytosolic and Mitochondrial Amino Acid Metabolism. Metabolites 11 (2), 1–28. doi:10.3390/metabo11020112
Hirokawa, K., Utsuyama, M., Hayashi, Y., Kitagawa, M., Makinodan, T., and Fulop, T. (2013). Slower Immune System Aging in Women versus Men in the Japanese Population. Immun. Ageing 10 (1), 19–9. doi:10.1186/1742-4933-10-19
Hirota, A., Kawachi, Y., Yamamoto, M., Koga, T., Hamada, K., and Otsuka, F. (2011). Acceleration of UVB-Induced Photoageing in Nrf2 Gene-Deficient Mice. Exp. Dermatol. 20 (8), 664–668. doi:10.1111/j.1600-0625.2011.01292.x
Hoffman, J. M., O’Neill, D. G., Creevy, K. E., and Austad, S. N. (2018). Do Female Dogs Age Differently Than Male Dogs? J. Gerontol. A. Biol. Sci. Med. Sci. 73 (2), 150–156. doi:10.1093/gerona/glx061
Hogg, N., and Kalyanaraman, B. (1999). Nitric Oxide and Lipid Peroxidation. Biochim. Biophys. Acta 1411 (2–3), 378–384. doi:10.1016/s0005-2728(99)00027-4
Holdt, L. M., Stahringer, A., Sass, K., Pichler, G., Kulak, N. A., Wilfert, W., et al. (2016). Circular Non-coding RNA ANRIL Modulates Ribosomal RNA Maturation and Atherosclerosis in Humans. Nat. Commun. 7, 12429. doi:10.1038/ncomms12429
Hoppel, C. L., Lesnefsky, E. J., Chen, Q., and Tandler, B. (2017). Mitochondrial Dysfunction in Cardiovascular Aging. Adv. Exp. Med. Biol. 982, 451–464. doi:10.1007/978-3-319-55330-6_24
Hoshida, S., Shinoda, Y., Ikeoka, K., Fukuoka, H., Inui, H., and Watanabe, T. (2016). Age‐ and Sex‐related Differences in Diastolic Function and Cardiac Dimensions in a Hypertensive Population. ESC Heart Fail. 3 (4), 270–277. doi:10.1002/ehf2.12097
Hoshino, T., Tabuchi, K., Nishimura, B., Tanaka, S., Nakayama, M., Ishii, T., et al. (2011). Protective Role of Nrf2 in Age-Related Hearing Loss and Gentamicin Ototoxicity. Biochem. Biophysical Res. Commun. 415 (1), 94–98. doi:10.1016/j.bbrc.2011.10.019
Houten, S. M., Violante, S., Ventura, F. V., and Wanders, R. J. A. (2016). The Biochemistry and Physiology of Mitochondrial Fatty Acid β-Oxidation and its Genetic Disorders. Annu. Rev. Physiol. 78, 23–44. doi:10.1146/annurev-physiol-021115-105045
Huikuri, H. V., Pikkuja¨msa¨, S. M., Airaksinen, K. E. J., Ika¨heimo, M. J., Rantala, A. O., Kauma, H., et al. (1996). Sex-Related Differences in Autonomic Modulation of Heart Rate in Middle-Aged Subjects. Circulation 94 (2), 122–125. doi:10.1161/01.cir.94.2.122
Hyyti, O. M., Ledee, D., Ning, X. H., Ge, M., and Portman, M. A. (2010). Aging Impairs Myocardial Fatty Acid and Ketone Oxidation and Modifies Cardiac Functional and Metabolic Responses to Insulin in Mice. Am. J. Physiol. Heart Circ. Physiol. 299 (3), H868–H875. doi:10.1152/ajpheart.00931.2009
Irace, C., Carallo, C., De Franceschi, M. S., Scicchitano, F., Milano, M., Tripolino, C., et al. (2012). Human Common Carotid wall Shear Stress as a Function of Age and Gender: A 12-year Follow-Up Study. Age (Dordr) 34 (6), 1553–1562. doi:10.1007/s11357-011-9318-1
Isensee, J., Witt, H., Pregla, R., Hetzer, R., Regitz-Zagrosek, V., and Ruiz Noppinger, P. (2008). Sexually Dimorphic Gene Expression in the Heart of Mice and Men. J. Mol. Med. 86 (1), 61–74. doi:10.1007/s00109-007-0240-z
Izzo, R., Losi, M.-A., Stabile, E., Lönnebakken, M. T., Canciello, G., Esposito, G., et al. (2017). Development of Left Ventricular Hypertrophy in Treated Hypertensive Outpatients. Hypertension 69 (1), 136–142. doi:10.1161/hypertensionaha.116.08158
Jamieson, K. L., Keshavarz-Bahaghighat, H., Darwesh, A. M., Sosnowski, D. K., and Seubert, J. M. (2020). Age and Sex Differences in Hearts of Soluble Epoxide Hydrolase Null Mice. Front. Physiol. 11 (February), 48–15. doi:10.3389/fphys.2020.00048
Jamieson, K. L., Endo, T., Darwesh, A. M., Samokhvalov, V., and Seubert, J. M. (2017). Cytochrome P450-Derived Eicosanoids and Heart Function. Pharmacol. Ther. 179, 47–83. doi:10.1016/j.pharmthera.2017.05.005
Jansen, H. J., Moghtadaei, M., MacKasey, M., Rafferty, S. A., Bogachev, O., Sapp, J. L., et al. (2017). Atrial Structure, Function and Arrhythmogenesis in Aged and Frail Mice. Sci. Rep. 7 (March), 1–17. doi:10.1038/srep44336
Jespersen, L., Hvelplund, A., Abildstrom, S. Z., Pedersen, F., Galatius, S., Madsen, J. K., et al. (2012). Stable Angina Pectoris with No Obstructive Coronary Artery Disease Is Associated with Increased Risks of Major Adverse Cardiovascular Events. Eur. Heart J. 33 (6), 734–744. doi:10.1093/eurheartj/ehr331
Jia, G., Aroor, A. R., Jia, C., and Sowers, J. R. (2019). Endothelial Cell Senescence in Aging-Related Vascular Dysfunction. Biochim. Biophys. Acta (Bba) - Mol. Basis Dis. 1865 (7), 1802–1809. doi:10.1016/j.bbadis.2018.08.008
Jin, L., Lin, X., Yang, L., Fan, X., Wang, W., Li, S., et al. (2018). AK098656, a Novel Vascular Smooth Muscle Cell-Dominant Long Noncoding RNA, Promotes Hypertension. Hypertension 71 (2), 262–272. doi:10.1161/HYPERTENSIONAHA.117.09651
Jin, L., Song, Q., Zhang, W., Geng, B., and Cai, J. (2019). Roles of Long Noncoding RNAs in Aging and Aging Complications. Biochim. Biophys. Acta (Bba) - Mol. Basis Dis. 1865 (7), 1763–1771. doi:10.1016/j.bbadis.2018.09.021
Kalogeropoulos, A., Georgiopoulou, V., Psaty, B. M., Rodondi, N., Smith, A. L., Harrison, D. G., et al. (2010). Inflammatory Markers and Incident Heart Failure Risk in Older Adults. J. Am. Coll. Cardiol. 55 (19), 2129–2137. doi:10.1016/j.jacc.2009.12.045
Kane, A. E., and Howlett, S. E. (2018). Differences in Cardiovascular Aging in Men and Women. Adv. Exp. Med. Biol. 1065, 389–411. doi:10.1007/978-3-319-77932-4_25
Kapahi, P., Zid, B. M., Harper, T., Koslover, D., Sapin, V., and Benzer, S. (2004). Regulation of Lifespan in Drosophila by Modulation of Genes in the TOR Signaling Pathway. Curr. Biol. 14, 885–890. doi:10.1016/j.cub.2004.03.059
Kelley, R. E., Dasmahapatra, P., Wang, J., Chen, W., Srinivasan, S. R., Fernandez, C., et al. (2011). Prevalence of Atherosclerotic Plaque in Young and Middle-Aged Asymptomatic Individuals. South. Med. J. 104 (12), 803–808. doi:10.1097/smj.0b013e318236c35c
Kim, E. N., Kim, M. Y., Lim, J. H., Kim, Y., Shin, S. J., Park, C. W., et al. (2018). The Protective Effect of Resveratrol on Vascular Aging by Modulation of the Renin-Angiotensin System. Atherosclerosis 270, 123–131. doi:10.1016/j.atherosclerosis.2018.01.043
Kim, S. K., McCurley, A. T., DuPont, J. J., Aronovitz, M., Moss, M. E., Stillman, I. E., et al. (2018). Smooth Muscle Cell-Mineralocorticoid Receptor as a Mediator of Cardiovascular Stiffness with Aging. Hypertension 71 (4), 609–621. doi:10.1161/hypertensionaha.117.10437
Kitzman, D. W., and Shah, S. J. (2016). The HFpEF Obesity Phenotype. J. Am. Coll. Cardiol. 68 (2), 200–203. doi:10.1016/j.jacc.2016.05.019
Klein, S. L., and Flanagan, K. L. (2016). Sex Differences in Immune Responses. Nat. Rev. Immunol. 16 (10), 626–638. doi:10.1038/nri.2016.90
Kong, P., Christia, P., and Frangogiannis, N. G. (2014). The Pathogenesis of Cardiac Fibrosis. Cell. Mol. Life Sci. 71 (4), 549–574. doi:10.1007/s00018-013-1349-6
Korzick, D. H., and Lancaster, T. S. (2013). Age-related Differences in Cardiac Ischemia-Reperfusion Injury: Effects of Estrogen Deficiency. Pflugers Arch. - Eur. J. Physiol. 465 (5), 669–685. doi:10.1007/s00424-013-1255-7
Kozai, D., Ogawa, N., and Mori, Y. (2014). Redox Regulation of Transient Receptor Potential Channels. Antioxid. Redox Signaling 21 (6), 971–986. doi:10.1089/ars.2013.5616
Krouwer, V. J., Hekking, L. H., Langelaar-Makkinje, M., Regan-Klapisz, E., and Post, J. A. (2012). Endothelial Cell Senescence Is Associated with Disrupted Cell-Cell Junctions and Increased Monolayer Permeability. Vasc. Cel 4, 12–10. doi:10.1186/2045-824X-4-12
Ksiazek, K., Passos, J. F., Olijslagers, S., Saretzki, G., Martin-Ruiz, C., and von Zglinicki, T. (2007). Premature Senescence of Mesothelial Cells Is Associated with Non-telomeric DNA Damage. Biochem. Biophysical Res. Commun. 362 (3), 707–711. doi:10.1016/j.bbrc.2007.08.047
Kuilman, T., Michaloglou, C., Mooi, W. J., and Peeper, D. S. (2010). The Essence of Senescence. Genes Develop. 24 (22), 2463–2479. doi:10.1101/gad.1971610
Kumar, A. A., Kelly, D. P., and Chirinos, J. A. (2019). Mitochondrial Dysfunction in Heart Failure with Preserved Ejection Fraction. Circulation 139 (11), 1435–1450. doi:10.1161/circulationaha.118.036259
Kumarswamy, R., Bauters, C., Volkmann, I., Maury, F., Fetisch, J., Holzmann, A., et al. (2014). Circulating Long Noncoding RNA, LIPCAR, Predicts Survival in Patients with Heart Failure. Circ. Res. 114 (10), 1569–1575. doi:10.1161/circresaha.114.303915
Kwekel, J. C., Vijay, V., Han, T., Moland, C. L., Desai, V. G., and Fuscoe, J. C. (2017). Sex and Age Differences in the Expression of Liver microRNAs during the Life Span of F344 Rats. Biol. Sex. Differ. 8 (1), 6–21. doi:10.1186/s13293-017-0127-9
Kłapcińska, B., Jagsz, S., Sadowska-Krepa, E., Górski, J., Kempa, K., and Langfort, J. (2008). Effects of Castration and Testosterone Replacement on the Antioxidant Defense System in Rat Left Ventricle. J. Physiol. Sci. 58 (3), 173–177. doi:10.2170/physiolsci.RP002208
Lacolley, P., Regnault, V., Segers, P., and Laurent, S. (2017). Vascular Smooth Muscle Cells and Arterial Stiffening: Relevance in Development, Aging, and Disease. Physiol. Rev. 97 (4), 1555–1617. doi:10.1152/physrev.00003.2017
Lagranha, C. J., Deschamps, A., Aponte, A., Steenbergen, C., and Murphy, E. (2010). Sex Differences in the Phosphorylation of Mitochondrial Proteins Result in Reduced Production of Reactive Oxygen Species and Cardioprotection in Females. Circ. Res. 106 (11), 1681–1691. doi:10.1161/circresaha.109.213645
Laina, A., Stellos, K., and Stamatelopoulos, K. (2018). Vascular Ageing: Underlying Mechanisms and Clinical Implications. Exp. Gerontol. 109, 16–30. doi:10.1016/j.exger.2017.06.007
Lancaster, T. S., Jefferson, S. J., Hunter, J. C., Lopez, V., Van Eyk, J. E., Lakatta, E. G., et al. (2012). Quantitative Proteomic Analysis Reveals Novel Mitochondrial Targets of Estrogen Deficiency in the Aged Female Rat Heart. Physiol. Genomics 44 (20), 957–969. doi:10.1152/physiolgenomics.00184.2011
Lane, N., and Martin, W. (2010). The Energetics of Genome Complexity. Nature 467 (7318), 929–934. doi:10.1038/nature09486
Lapham, K., Kvale, M. N., Lin, J., Connell, S., Croen, L. A., Dispensa, B. P., et al. (2015). Automated Assay of Telomere Length Measurement and Informatics for 100,000 Subjects in the Genetic Epidemiology Research on Adult Health and Aging (GERA) Cohort. Genetics 200 (4), 1061–1072. doi:10.1534/genetics.115.178624
Laughlin, G. A., Barrett-Connor, E., and Bergstrom, J. (2008). Low Serum Testosterone and Mortality in Older Men. J. Clin. Endocrinol. Metab. 93 (1), 68–75. doi:10.1210/jc.2007-1792
Lee, J. F., Barrett-O'Keefe, Z., Garten, R. S., Nelson, A. D., Ryan, J. J., Nativi, J. N., et al. (2016). Evidence of Microvascular Dysfunction in Heart Failure with Preserved Ejection Fraction. Heart 102 (4), 278–284. doi:10.1136/heartjnl-2015-308403
Lenna, S., Han, R., and Trojanowska, M. (2014). Endoplasmic Reticulum Stress and Endothelial Dysfunction. IUBMB Life 66 (8), 530–537. doi:10.1002/iub.1292
Leon, L. J., and Gustafsson, Å. B. (2016). Staying Young at Heart: Autophagy and Adaptation to Cardiac Aging. J. Mol. Cell Cardiol. 95, 78–85. doi:10.1016/j.yjmcc.2015.11.006
Lesnefsky, E. J., Chen, Q., and Hoppel, C. L. (2016). Mitochondrial Metabolism in Aging Heart. Circ. Res. 118 (10), 1593–1611. doi:10.1161/circresaha.116.307505
Letsolo, B. T., Jones, R. E., Rowson, J., Grimstead, J. W., Keith, W. N., Jenkins, G. J., et al. (2017). Extensive Telomere Erosion Is Consistent with Localised Clonal Expansions in Barrett's Metaplasia. PLoS One 12 (3), e0174833–11. doi:10.1371/journal.pone.0174833
Lian, B. Q., and Keaney, J. F. (2010). Predicting Ischemic Heart Disease in Women. J. Am. Coll. Cardiol. 55 (16), 1697–1699. doi:10.1016/j.jacc.2009.10.074
Lieb, W., Xanthakis, V., Sullivan, L. M., Aragam, J., Pencina, M. J., Larson, M. G., et al. (2009). Longitudinal Tracking of Left Ventricular Mass over the Adult Life Course. Circulation 119 (24), 3085–3092. doi:10.1161/circulationaha.108.824243
Light, K. C., Hinderliter, A. L., West, S. G., Grewen, K. M., Steege, J. F., Sherwood, A., et al. (2001). Hormone Replacement Improves Hemodynamic Profile and Left Ventricular Geometry in Hypertensive and Normotensive Postmenopausal Women. J. Hypertens. 19 (2), 269–278. doi:10.1097/00004872-200102000-00014
Liguori, I., Russo, G., Curcio, F., Bulli, G., Aran, L., Della-Morte, D., et al. (2018). Oxidative Stress, Aging, and Diseases. Clin. Interv. Aging 13, 757–772. doi:10.2147/cia.s158513
Lim, W. K., Wren, B., Jepson, N., Roy, S., and Caplan, G. (1999). Effect of Hormone Replacement Therapy on Left Ventricular Hypertrophy. Am. J. Cardiol. 83 (7), 1132–A9. doi:10.1016/s0002-9149(99)00029-6
Lin, S., Wang, Y., Zhang, X., Kong, Q., Li, C., Li, Y., et al. (2016). HSP27 Alleviates Cardiac Aging in Mice via a Mechanism Involving Antioxidation and Mitophagy Activation. Oxidative Med. Cell Longevity 2016, 1–13. doi:10.1155/2016/2586706
Lio, D., Scola, L., Crivello, A., Candore, G., Bonafè, M., Cavallone, L., et al. (2003). Inflammation, Genetics, and Longevity: Further Studies on the Protective Effects in Men of IL-10 -1082 Promoter SNP and its Interaction with TNF-Alpha -308 Promoter SNP. J. Medgenet 40, 296–299. doi:10.1136/jmg.40.4.296
Lipinski, M. M., Zheng, B., Lu, T., Yan, Z., Py, B. F., Ng, A., et al. (2010). Genome-wide Analysis Reveals Mechanisms Modulating Autophagy in normal Brain Aging and in Alzheimer's Disease. Proc. Natl. Acad. Sci. 107 (32), 14164–14169. doi:10.1073/pnas.1009485107
Liu, H., Yanamandala, M., Lee, T. C., and Kim, J. K. (2014). Mitochondrial P38β and Manganese Superoxide Dismutase Interaction Mediated by Estrogen in Cardiomyocytes. PLoS One 9 (1), e85272–10. doi:10.1371/journal.pone.0085272
Lopaschuk, G. D., Ussher, J. R., Folmes, C. D. L., Jaswal, J. S., and Stanley, W. C. (2010). Myocardial Fatty Acid Metabolism in Health and Disease. Physiol. Rev. 90 (1), 207–258. doi:10.1152/physrev.00015.2009
Lopes, R. A., Neves, K. B., Carneiro, F. S., and Tostes, R. C. (2012). Testosterone and Vascular Function in Aging. Front. Physiol. 3 (April), 89–9. doi:10.3389/fphys.2012.00089
Lopes, R. A., Neves, K. B., Pestana, C. R., Queiroz, A. L., Zanotto, C. Z., Chignalia, A. Z., et al. (2014). Testosterone Induces Apoptosis in Vascular Smooth Muscle Cells via Extrinsic Apoptotic Pathway with Mitochondria-Generated Reactive Oxygen Species Involvement. Am. J. Physiol. Heart Circ. Physiol. 306 (11), H1485–H1494. doi:10.1152/ajpheart.00809.2013
López-Otín, C., Blasco, M. A., Partridge, L., Serrano, M., and Kroemer, G. (2013). The Hallmarks of Aging. Cell 153 (6), 1194–1217. doi:10.1016/j.cell.2013.05.039
Lu, T., and Finkel, T. (2008). Free Radicals and Senescence. Exp. Cel Res. 314 (9), 1918–1922. doi:10.1016/j.yexcr.2008.01.011
Lumens, J., Delhaas, T., Arts, T., Cowan, B. R., and Young, A. A. (2006). Impaired Subendocardial Contractile Myofiber Function in Asymptomatic Aged Humans, as Detected Using MRI. Am. J. Physiol. Heart Circ. Physiol. 291 (4), H1573–H1579. doi:10.1152/ajpheart.00074.2006
Ma, Q., Qiang, J., Gu, P., Wang, Y., Geng, Y., and Wang, M. (2011). Age-related Autophagy Alterations in the Brain of Senescence Accelerated Mouse Prone 8 (SAMP8) Mice. Exp. Gerontol. 46 (7), 533–541. doi:10.1016/j.exger.2011.02.006
Maas, A. H. E. M., Van Der Schouw, Y. T., Regitz-Zagrosek, V., Swahn, E., Appelman, Y. E., Pasterkamp, G., et al. (2011). Red Alert for Women's Heart: the Urgent Need for More Research and Knowledge on Cardiovascular Disease in Women: Proceedings of the Workshop Held in Brussels on Gender Differences in Cardiovascular Disease, 29 September 2010. Eur. Heart J. 32 (11), 1362–1368. doi:10.1093/eurheartj/ehr048
Madan, N., Gajo, E., Sanghani, R. M., and Volgman, A. S. (2020). Sex-Based Considerations in the Evaluation of Chest Pain and Management of Obstructive Coronary Artery Disease. Curr. Atheroscler. Rep. 22 (8), 39. doi:10.1007/s11883-020-00855-7
Maggio, M., Ceda, G. P., Lauretani, F., Bandinelli, S., Corsi, A. M., Giallauria, F., et al. (2011). SHBG, Sex Hormones, and Inflammatory Markers in Older Women. J. Clin. Endocrinol. Metab. 96 (4), 1053–1059. doi:10.1210/jc.2010-1902
Maiese, K. (2016). Disease Onset and Aging in the World of Circular RNAs. J. Transl Sci. 2 (6), 327–329. doi:10.15761/jts.1000158
Malkin, C. J., Pugh, P. J., Jones, R. D., Kapoor, D., Channer, K. S., and Jones, T. H. (2004). The Effect of Testosterone Replacement on Endogenous Inflammatory Cytokines and Lipid Profiles in Hypogonadal Men. J. Clin. Endocrinol. Metab. 89 (7), 3313–3318. doi:10.1210/jc.2003-031069
Marín-Aguilar, F., Lechuga-Vieco, A. V., Alcocer-Gómez, E., Castejón-Vega, B., Lucas, J., Garrido, C., et al. (2020). NLRP3 Inflammasome Suppression Improves Longevity and Prevents Cardiac Aging in Male Mice. Aging Cell 19 (1), e13050–14. doi:10.1111/acel.13050
Martin, G. M. (2011). The Biology of Aging: 1985-2010 and beyond. FASEB j. 25 (11), 3756–3762. doi:10.1096/fj.11-1102.ufm
Martínez, P., Thanasoula, M., Muñoz, P., Liao, C., Tejera, A., McNees, C., et al. (2009). Increased Telomere Fragility and Fusions Resulting from TRF1 Deficiency lead to Degenerative Pathologies and Increased Cancer in Mice. Genes Develop. 23 (17), 2060–2075. doi:10.1101/gad.543509
Maturana, M. A., Breda, V., Lhullier, F., and Spritzer, P. M. (2008). Relationship between Endogenous Testosterone and Cardiovascular Risk in Early Postmenopausal Women. Metabolism 57 (7), 961–965. doi:10.1016/j.metabol.2008.02.012
McCormick, M. A., Tsai, S.-y., and Kennedy, B. K. (2011). TOR and Ageing: A Complex Pathway for a Complex Process. Phil. Trans. R. Soc. B 366 (1561), 17–27. doi:10.1098/rstb.2010.0198
McNab, B. K. (2003). Ecology Shapes Bird Bioenergetics. Nature 426 (6967), 620–621. doi:10.1038/426620b
Menazza, S., Sun, J., Appachi, S., Chambliss, K. L., Kim, S. H., Aponte, A., et al. (2017). Non-nuclear Estrogen Receptor Alpha Activation in Endothelium Reduces Cardiac Ischemia-Reperfusion Injury in Mice. J. Mol. Cell Cardiol. 107, 41–51. doi:10.1016/j.yjmcc.2017.04.004
Méndez-Bailón, M., Muñoz-Rivas, N., Jiménez-García, R., Hernández-Barrera, V., de Miguel-Yanes, J. M., Villalba, N. L., et al. (2017). Women with Atrial Fibrillation and Type 2 Diabetes Have a Higher Incidence of Hospitalization and Undergo Ablation or Pacemaker Implantation Less Frequently Than Men. Eur. J. Intern. Med. 42, 67–73. doi:10.1016/j.ejim.2017.05.008
Merrill, M., Sweitzer, N. K., Lindenfeld, J., and Kao, D. P. (2019). Sex Differences in Outcomes and Responses to Spironolactone in Heart Failure with Preserved Ejection Fraction. JACC: Heart Fail. 7 (3), 228–238. doi:10.1016/j.jchf.2019.01.003
Merz, A. A., and Cheng, S. (2016). Sex Differences in Cardiovascular Ageing. Heart 102 (11), 825–831. doi:10.1136/heartjnl-2015-308769
Michalson, K. T., Groban, L., Howard, T. D., Shively, C. A., Sophonsritsuk, A., Appt, S. E., et al. (2018). Estradiol Treatment Initiated Early after Ovariectomy Regulates Myocardial Gene Expression and Inhibits Diastolic Dysfunction in Female Cynomolgus Monkeys: Potential Roles for Calcium Homeostasis and Extracellular Matrix Remodeling. J. Am. Heart Assoc. 7 (21), e009769–18. doi:10.1161/JAHA.118.009769
Miller, R. J. H., Mikami, Y., Heydari, B., Wilton, S. B., James, M. T., Howarth, A. G., et al. (2020). Sex-specific Relationships between Patterns of Ventricular Remodelling and Clinical Outcomes. Eur. Heart J. Cardiovasc. Imaging 21 (9), 983–990. doi:10.1093/ehjci/jeaa164
Min, K.-J., Lee, C.-K., and Park, H.-N. (2012). The Lifespan of Korean Eunuchs. Curr. Biol. 22 (18), R792–R793. doi:10.1016/j.cub.2012.06.036
Mirza, M., Strunets, A., Shen, W.-K., and Jahangir, A. (2012). Mechanisms of Arrhythmias and Conduction Disorders in Older Adults. Clin. Geriatr. Med. 28 (4), 555–573. doi:10.1016/j.cger.2012.08.005
Mitchell, G. F., Parise, H., Benjamin, E. J., Larson, M. G., Keyes, M. J., Vita, J. A., et al. (2004). Changes in Arterial Stiffness and Wave Reflection with Advancing Age in Healthy Men and Women. Hypertension 43 (6), 1239–1245. doi:10.1161/01.hyp.0000128420.01881.aa
Mohammed, S. F., Borlaug, B. A., Roger, V. L., Mirzoyev, S. A., Rodeheffer, R. J., Chirinos, J. A., et al. (2012). Comorbidity and Ventricular and Vascular Structure and Function in Heart Failure with Preserved Ejection Fraction. Circ. Heart Fail. 5 (6), 710–719. doi:10.1161/circheartfailure.112.968594
Moreau, K. L., Babcock, M. C., and Hildreth, K. L. (2020). Sex Differences in Vascular Aging in Response to Testosterone. Biol. Sex. Differ. 11 (1), 18–14. doi:10.1186/s13293-020-00294-8
Moreau, K. L., Hildreth, K. L., Meditz, A. L., Deane, K. D., and Kohrt, W. M. (2012). Endothelial Function Is Impaired across the Stages of the Menopause Transition in Healthy Women. J. Clin. Endocrinol. Metab. 97 (12), 4692–4700. doi:10.1210/jc.2012-2244
Moreau, K. L. (2018). Intersection between Gonadal Function and Vascular Aging in Women. J. Appl. Physiol. 125 (6), 1881–1887. doi:10.1152/japplphysiol.00117.2018
Moreau, K. L., Stauffer, B. L., Kohrt, W. M., and Seals, D. R. (2013). Essential Role of Estrogen for Improvements in Vascular Endothelial Function with Endurance Exercise in Postmenopausal Women. J. Clin. Endocrinol. Metab. 98 (11), 4507–4515. doi:10.1210/jc.2013-2183
Mori, T., Kai, H., Kajimoto, H., Koga, M., Kudo, H., Takayama, N., et al. (2011). Enhanced Cardiac Inflammation and Fibrosis in Ovariectomized Hypertensive Rats: A Possible Mechanism of Diastolic Dysfunction in Postmenopausal Women. Hypertens. Res. 34 (4), 496–502. doi:10.1038/hr.2010.261
Müller-Höcker, J. (1992). Mitochondria and Ageing. Brain Pathol. 2, 149–158. doi:10.1111/j.1750-3639.1992.tb00683.x
Murphy, S. P., Ibrahim, N. E., and Januzzi, J. L. (2020). Heart Failure with Reduced Ejection Fraction. Jama 324 (5), 488–504. doi:10.1001/jama.2020.10262
Nair, S., and Ren, J. (2012). Autophagy and Cardiovascular Aging. Cell Cycle 11 (11), 2092–2099. doi:10.4161/cc.20317
Nanna, M. G., Hajduk, A. M., Krumholz, H. M., Murphy, T. E., Dreyer, R. P., Alexander, K. P., et al. (2019). Sex-based Differences in Presentation, Treatment, and Complications Among Older Adults Hospitalized for Acute Myocardial Infarction: The SILVER-AMI Study. Circ. Cardiovasc. Qual. Outcomes 12 (10), e005691–13. doi:10.1161/CIRCOUTCOMES.119.005691
Narasimhan, S. D., Yen, K., and Tissenbaum, H. A. (2009). Converging Pathways in Lifespan Regulation. Curr. Biol. 19 (15), R657–R666. doi:10.1016/j.cub.2009.06.013
Narita, M., Nuñez, S., Heard, E., Narita, M., Lin, A. W., Hearn, S. A., et al. (2003). Rb-mediated Heterochromatin Formation and Silencing of E2F Target Genes during Cellular Senescence. Cell 113 (6), 703–716. doi:10.1016/s0092-8674(03)00401-x
Natoli, A. K., Medley, T. L., Ahimastos, A. A., Drew, B. G., Thearle, D. J., Dilley, R. J., et al. (2005). Sex Steroids Modulate Human Aortic Smooth Muscle Cell Matrix Protein Deposition and Matrix Metalloproteinase Expression. Hypertension 46 (5), 1129–1134. doi:10.1161/01.hyp.0000187016.06549.96
Nevzati, E., Shafighi, M., Bakhtian, K. D., Treiber, H., Fandino, J., and Fathi, A. R. (2015). Estrogen Induces Nitric Oxide Production via Nitric Oxide Synthase Activation in Endothelial Cells. Acta Neurochir Suppl. 120, 141–145. doi:10.1007/978-3-319-04981-6_24
Nosalski, R., Mikolajczyk, T., Siedlinski, M., Saju, B., Koziol, J., Maffia, P., et al. (2020). Nox1/4 Inhibition Exacerbates Age Dependent Perivascular Inflammation and Fibrosis in a Model of Spontaneous Hypertension. Pharmacol. Res. 161 (July), 105235. doi:10.1016/j.phrs.2020.105235
Okunrintemi, V., Valero-Elizondo, J., Patrick, B., Salami, J., Tibuakuu, M., Ahmad, S., et al. (2018). Gender Differences in Patient-Reported Outcomes Among Adults with Atherosclerotic Cardiovascular Disease. J. Am. Heart Assoc. 7 (24), e010498. doi:10.1161/JAHA.118.010498
Oliván, S., Calvo, A. C., Manzano, R., Zaragoza, P., and Osta, R. (2014). Sex Differences in Constitutive Autophagy. Biomed. Res. Int. 2014, 1–5. doi:10.1155/2014/652817
Olivetti, G., Giordano, G., Corradi, D., Melissari, M., Lagrasta, C., Gambert, S. R., et al. (1995). Gender Differences and Aging: Effects on the Human Heart. J. Am. Coll. Cardiol. 26 (4), 1068–1079. doi:10.1016/0735-1097(95)00282-8
Oneglia, A., Nelson, M. D., and Merz, C. N. B. (2020). Sex Differences in Cardiovascular Aging and Heart Failure. Curr. Heart Fail. Rep. 17 (6), 409–423. doi:10.1007/s11897-020-00487-7
Ostan, R., Monti, D., Gueresi, P., Bussolotto, M., Franceschi, C., and Baggio, G. (2016). Gender, Aging and Longevity in Humans: An Update of an Intriguing/neglected Scenario Paving the Way to a Gender-specific Medicine. Clin. Sci. 130 (19), 1711–1725. doi:10.1042/cs20160004
Ota, H., Eto, M., Kano, M. R., Kahyo, T., Setou, M., Ogawa, S., et al. (2010). Induction of Endothelial Nitric Oxide Synthase, SIRT1, and Catalase by Statins Inhibits Endothelial Senescence through the Akt Pathway. Arterioscler Thromb. Vasc. Biol. 30 (11), 2205–2211. doi:10.1161/atvbaha.110.210500
Ota, H., Reeves, M. J., Zhu, D. C., Majid, A., Collar, A., Yuan, C., et al. (2010). Sex Differences in Patients with Asymptomatic Carotid Atherosclerotic Plaque. Stroke 41 (8), 1630–1635. doi:10.1161/strokeaha.110.581306
Pansarasa, O., Castagna, L., Colombi, B., Vecchiet, J., Felzani, G., and Marzatico, F. (2000). Age and Sex Differences in Human Skeletal Muscle: Role of Reactive Oxygen Species. Free Radic. Res. 33 (3), 287–293. doi:10.1080/10715760000301451
Passos, J. F., Saretzki, G., and Von Zglinicki, T. (2007). DNA Damage in Telomeres and Mitochondria during Cellular Senescence: Is There a Connection? Nucleic Acids Res. 35 (22), 7505–7513. doi:10.1093/nar/gkm893
Paulus, W. J., and Tschöpe, C. (2013). A Novel Paradigm for Heart Failure with Preserved Ejection Fraction. J. Am. Coll. Cardiol. 62 (4), 263–271. doi:10.1016/j.jacc.2013.02.092
Payne, B. A. I., and Chinnery, P. F. (2015). Mitochondrial Dysfunction in Aging: Much Progress but many Unresolved Questions. Biochim. Biophys. Acta (Bba) - Bioenerg. 1847 (11), 1347–1353. doi:10.1016/j.bbabio.2015.05.022
Pedram, A., Razandi, M., Aitkenhead, M., and Levin, E. R. (2005). Estrogen Inhibits Cardiomyocyte Hypertrophy In Vitro. J. Biol. Chem. 280 (28), 26339–26348. doi:10.1074/jbc.m414409200
Pedram, A., Razandi, M., Lubahn, D., Liu, J., Vannan, M., and Levin, E. R. (2008). Estrogen Inhibits Cardiac Hypertrophy: Role of Estrogen Receptor-β to Inhibit Calcineurin. Endocrinology 149 (7), 3361–3369. doi:10.1210/en.2008-0133
Pellegrini, G. G., Cregor, M., Mcandrews, K., Morales, C. C., Mccabe, L. D., Mccabe, G. P., et al. (2017). NRF2 Regulates Mass Accrual and the Antioxidant Endogenous Response in Bone Differently Depending on the Sex and Age. PLoS One 12 (2), e0171161–18. doi:10.1371/journal.pone.0171161
Pellegrini, M., Bulzomi, P., Lecis, M., Leone, S., Campesi, I., Franconi, F., et al. (2014). Endocrine Disruptors Differently Influence Estrogen Receptor β and Androgen Receptor in Male and Female Rat VSMC. J. Cel. Physiol 229 (8), 1061–1068. doi:10.1002/jcp.24530
Pes, G. M., Lio, D., Carru, C., Deiana, L., Baggio, G., Franceschi, C., et al. (2004). Association between Longevity and Cytokine Gene Polymorphisms. A Study in Sardinian Centenarians. Aging Clin. Exp. Res. 16 (3), 244–248. doi:10.1007/bf03327391
Peter, I., Shearman, A., Vasan, R., Zucker, D., Schmid, C., Demissie, S., et al. (2005). Association of Estrogen Receptor β Gene Polymorphisms with Left Ventricular Mass and Wall Thickness in Women. Am. J. Hypertens. 18 (11), 1388–1395. doi:10.1016/j.amjhyper.2005.05.023
Podolskiy, D. I., Lobanov, A. V., Kryukov, G. V., and Gladyshev, V. N. (2016). Analysis of Cancer Genomes Reveals Basic Features of Human Aging and its Role in Cancer Development. Nat. Commun. 7, 1–12. doi:10.1038/ncomms12157
Pollow, D. P., Uhlorn, J. A., Sylvester, M. A., Romero-Aleshire, M. J., Uhrlaub, J. L., Lindsey, M. L., et al. (2019). Menopause and FOXP3+ Treg Cell Depletion Eliminate Female protection against T Cell-Mediated Angiotensin II Hypertension. Am. J. Physiology-Heart Circulatory Physiol. 317 (2), H415–H423. doi:10.1152/ajpheart.00792.2018
Porro, A., Feuerhahn, S., Delafontaine, J., Riethman, H., Rougemont, J., and Lingner, J. (2014). Functional Characterization of the TERRA Transcriptome at Damaged Telomeres. Nat. Commun. 5, 1–13. doi:10.1038/ncomms6379
Porro, A., Feuerhahn, S., Reichenbach, P., and Lingner, J. (2010). Molecular Dissection of Telomeric Repeat-Containing RNA Biogenesis Unveils the Presence of Distinct and Multiple Regulatory Pathways. Mol. Cel Biol 30 (20), 4808–4817. doi:10.1128/mcb.00460-10
Powers, R. W., Kaeberlein, M., Caldwell, S. D., Kennedy, B. K., and Fields, S. (2006). Extension of Chronological Life Span in Yeast by Decreased TOR Pathway Signaling. Genes Develop. 20 (2), 174–184. doi:10.1101/gad.1381406
Qi, G., Jia, L., Li, Y., Bian, Y., Cheng, J., Li, H., et al. (2011). Angiotensin II Infusion-Induced Inflammation, Monocytic Fibroblast Precursor Infiltration, and Cardiac Fibrosis Are Pressure Dependent. Cardiovasc. Toxicol. 11 (2), 157–167. doi:10.1007/s12012-011-9109-z
Qi, J., Luo, X., Ma, Z., Zhang, B., Li, S., and Zhang, J. (2020). Downregulation of miR-26b-5p, miR-204-5p, and miR-497-3p Expression Facilitates Exercise-Induced Physiological Cardiac Hypertrophy by Augmenting Autophagy in Rats. Front. Genet. 11 (February), 78–13. doi:10.3389/fgene.2020.00078
Rajkumar, C., Kingwell, B. A., Cameron, J. D., Waddell, T., Mehra, R., Christophidis, N., et al. (1997). Hormonal Therapy Increases Arterial Compliance in Postmenopausal Women. J. Am. Coll. Cardiol. 30 (2), 350–356. doi:10.1016/s0735-1097(97)00191-5
Ravelojaona, V., Robert, A. M., and Robert, L. (2009). Expression of Senescence-Associated β-galactosidase (SA-β-Gal) by Human Skin Fibroblasts, Effect of Advanced Glycation End-Products and Fucose or Rhamnose-Rich Polysaccharides. Arch. Gerontol. Geriatr. 48 (2), 151–154. doi:10.1016/j.archger.2007.12.004
Rea, I. M., Gibson, D. S., McGilligan, V., McNerlan, S. E., Alexander, H. D., and Ross, O. A. (2018). Age and Age-Related Diseases: Role of Inflammation Triggers and Cytokines. Front. Immunol. 9 (APR), 1–28. doi:10.3389/fimmu.2018.00586
Redfield, M. M., Jacobsen, S. J., Borlaug, B. A., Rodeheffer, R. J., and Kass, D. A. (2005). Age- and Gender-Related Ventricular-Vascular Stiffening. Circulation 112 (15), 2254–2262. doi:10.1161/circulationaha.105.541078
Reis, S. E., Gloth, S. T., Blumenthal, R. S., Resar, J. R., Zacur, H. A., Gerstenblith, G., et al. (1994). Ethinyl Estradiol Acutely Attenuates Abnormal Coronary Vasomotor Responses to Acetylcholine in Postmenopausal Women. Circulation 89 (1), 52–60. doi:10.1161/01.cir.89.1.52
Riondino, S., Ferroni, P., Roselli, M., and Guadagni, F. (2019). Precision Medicine in the Ageing World: The Role of Biospecimen Sciences. Int. J. Biol. Markers 34 (1), 3–5. doi:10.1177/1724600818816995
Rizzoni, D., Rizzoni, M., Nardin, M., Chiarini, G., Agabiti-Rosei, C., Aggiusti, C., et al. (2019). Vascular Aging and Disease of the Small Vessels. High Blood Press. Cardiovasc. Prev. 26 (3), 183–189. doi:10.1007/s40292-019-00320-w
Rocca, C., Femminò, S., Aquila, G., Granieri, M. C., De Francesco, E. M., Pasqua, T., et al. (2018). Notch1 Mediates Preconditioning protection Induced by GPER in Normotensive and Hypertensive Female Rat Hearts. Front. Physiol. 9 (MAY), 1–11. doi:10.3389/fphys.2018.00521
Rochelle, T. L., Yeung, D. K. Y., Bond, M. H., and Li, L. M. W. (2015). Predictors of the Gender gap in Life Expectancy across 54 Nations. Psychol. Health Med. 20 (2), 129–138. doi:10.1080/13548506.2014.936884
Romero, A., San Hipólito-Luengo, Á., Villalobos, L. A., Vallejo, S., Valencia, I., Michalska, P., et al. (2019). The angiotensin-(1-7)/Mas Receptor axis Protects from Endothelial Cell Senescence via Klotho and Nrf2 Activation. Aging Cell 18 (3), e12913–12. doi:10.1111/acel.12913
Rubio-Ruíz, M. E., Del Valle-Mondragón, L., Castrejón-Tellez, V., Carreón-Torres, E., Díaz-Díaz, E., and Guarner-Lans, V. (2014). Angiotensin II and 1-7 during Aging in Metabolic Syndrome Rats. Expression of AT1, AT2 and Mas Receptors in Abdominal white Adipose Tissue. Peptides 57, 101–108. doi:10.1016/j.peptides.2014.04.021
Ryan, R. P., McCarthy, Y., Andrade, M., Farah, C. S., Armitage, J. P., and Dow, J. M. (2010). Cell-cell Signal-dependent Dynamic Interactions between HD-GYP and GGDEF Domain Proteins Mediate Virulence in Xanthomonas Campestris. Proc. Natl. Acad. Sci. 107 (13), 5989–5994. doi:10.1073/pnas.0912839107
Saba, P. S., Ganau, A., Devereux, R. B., Pini, R., Pickering, T. G., and Roman, M. J. (1999). Impact of Arterial Elastance as a Measure of Vascular Load on Left Ventricular Geometry in Hypertension. J. Hypertens. 17 (7), 1007–1015. doi:10.1097/00004872-199917070-00018
Sabbatini, A. R., and Kararigas, G. (2020). Menopause-Related Estrogen Decrease and the Pathogenesis of HFpEF. J. Am. Coll. Cardiol. 75 (9), 1074–1082. doi:10.1016/j.jacc.2019.12.049
Sadowska-Bartosz, I., and Bartosz, G. (2014). Effect of Antioxidants Supplementation on Aging and Longevity. Biomed. Res. Int. 2014, 1–17. doi:10.1155/2014/404680
Salabei, J. K., and Hill, B. G. (2015). Autophagic Regulation of Smooth Muscle Cell Biology. Redox Biol. 4, 97–103. doi:10.1016/j.redox.2014.12.007
Salton, C. J., Chuang, M. L., O’Donnell, C. J., Kupka, M. J., Larson, M. G., Kissinger, K. V., et al. (2002). Gender Differences and normal Left Ventricular Anatomy in an Adult Population Free of Hypertension. J. Am. Coll. Cardiol. 39 (6), 1055–1060. doi:10.1016/s0735-1097(02)01712-6
Sandoo, A., Veldhuijzen van Zanten, J. J. C., Metsios, G. S., Carroll, D., and Kitas, G. D. (2015). The Endothelium and its Role in Regulating Vascular Tone. Open Cardiovasc. Med. J. 4 (1), 302–312. doi:10.2174/1874192401004010302
Santos, J. H., Meyer, J. N., Skorvaga, M., Annab, L. A., and Van Houten, B. (2004). Mitochondrial hTERT Exacerbates Free-Radical-Mediated mtDNA Damage. Aging Cell 3 (6), 399–411. doi:10.1111/j.1474-9728.2004.00124.x
Santos, R. A., Ferreira, A. J., Verano-Braga, T., and Bader, M. (2013). Angiotensin-converting Enzyme 2, Angiotensin-(1-7) and Mas: New Players of the Renin-Angiotensin System. J. Endocrinol. 216 (2), R1–R53. doi:10.1530/JOE-12-0341
Sarkar, S., Ravikumar, B., Floto, R. A., and Rubinsztein, D. C. (2009). Rapamycin and mTOR-independent Autophagy Inducers Ameliorate Toxicity of Polyglutamine-Expanded Huntingtin and Related Proteinopathies. Cell Death Differ 16 (1), 46–56. doi:10.1038/cdd.2008.110
Scarabino, D., Peconi, M., Pelliccia, F., and Corbo, R. M. (2019). Analysis of the Association between TERC and TERT Genetic Variation and Leukocyte Telomere Length and Human Lifespan-A Follow-Up Study. Genes (Basel) 10 (2), 1–12. doi:10.3390/genes10020082
Serrano, M., Lin, A. W., McCurrach, M. E., Beach, D., and Lowe, S. W. (1997). Oncogenic Ras Provokes Premature Cell Senescence Associated with Accumulation of P53 and p16INK4a. Cell 88 (5), 593–602. doi:10.1016/s0092-8674(00)81902-9
Sfeir, A., Kosiyatrakul, S. T., Hockemeyer, D., MacRae, S. L., Karlseder, J., Schildkraut, C. L., et al. (2009). Mammalian Telomeres Resemble Fragile Sites and Require TRF1 for Efficient Replication. Cell 138 (1), 90–103. doi:10.1016/j.cell.2009.06.021
Shah, A. M., Claggett, B., Sweitzer, N. K., Shah, S. J., Anand, I. S., O’Meara, E., et al. (2014). Cardiac Structure and Function and Prognosis in Heart Failure with Preserved Ejection Fraction. Circ. Heart Fail. 7 (5), 740–751. doi:10.1161/circheartfailure.114.001583
Shao, Q., Fallica, J., Casin, K. M., Murphy, E., Steenbergen, C., and Kohr, M. J. (2016). Characterization of the Sex-dependent Myocardial S-Nitrosothiol Proteome. Am. J. Physiology-Heart Circulatory Physiol. 310 (4), H505–H515. doi:10.1152/ajpheart.00681.2015
Shaw, L. J., Bugiardini, R., and Merz, C. N. B. (2009). Women and Ischemic Heart Disease. J. Am. Coll. Cardiol. 54 (17), 1561–1575. doi:10.1016/j.jacc.2009.04.098
Shih, P.-H., and Yen, G.-C. (2007). Differential Expressions of Antioxidant Status in Aging Rats: The Role of Transcriptional Factor Nrf2 and MAPK Signaling Pathway. Biogerontology 8 (2), 71–80. doi:10.1007/s10522-006-9033-y
Sickinghe, A. A., Korporaal, S. J. A., Den Ruijter, H. M., and Kessler, E. L. (2019). Estrogen Contributions to Microvascular Dysfunction Evolving to Heart Failure with Preserved Ejection Fraction. Front. Endocrinol. (Lausanne) 10 (JULY), 442–449. doi:10.3389/fendo.2019.00442
Silberman, G. A., Fan, T.-H. M., Liu, H., Jiao, Z., Xiao, H. D., Lovelock, J. D., et al. (2010). Uncoupled Cardiac Nitric Oxide Synthase Mediates Diastolic Dysfunction. Circulation 121 (4), 519–528. doi:10.1161/circulationaha.109.883777
Singh, A., Chan, D. C. S., Greenwood, J. P., Dawson, D. K., Sonecki, P., Hogrefe, K., et al. (2019). Symptom Onset in Aortic Stenosis. JACC: Cardiovasc. Imaging 12 (1), 96–105. doi:10.1016/j.jcmg.2017.09.019
Smulyan, H., Asmar, R. G., Rudnicki, A., London, G. M., and Safar, M. E. (2001). Comparative Effects of Aging in Men and Women on the Properties of the Arterial Tree. J. Am. Coll. Cardiol. 37 (5), 1374–1380. doi:10.1016/s0735-1097(01)01166-4
Song, X., Qian, X., Shen, M., Jiang, R., Wagner, M. B., Ding, G., et al. (2015). Protein Kinase C Promotes Cardiac Fibrosis and Heart Failure by Modulating Galectin-3 Expression. Biochim. Biophys. Acta (Bba) - Mol. Cel Res. 1853 (2), 513–521. doi:10.1016/j.bbamcr.2014.12.001
Sovershaev, M. A., Egorina, E. M., Andreasen, T. V., Jonassen, A. K., and Ytrehus, K. (2006). Preconditioning by 17beta-Estradiol in Isolated Rat Heart Depends on PI3-K/PKB Pathway, PKC, and ROS. Am. J. Physiol. Heart Circ. Physiol. 291 (4), H1554–H1562. doi:10.1152/ajpheart.01171.2005
Sowers, M. R., Jannausch, M., Randolph, J. F., McConnell, D., Little, R., Lasley, B., et al. (2005). Androgens Are Associated with Hemostatic and Inflammatory Factors Among Women at the Mid-life. J. Clin. Endocrinol. Metab. 90 (11), 6064–6071. doi:10.1210/jc.2005-0765
Stern, S., Behar, S., and Gottlieb, S. (2003). Cardiology Patient Pages. Aging and Diseases of the Heart. Circulation 108 (14), e99–101. doi:10.1161/01.CIR.0000086898.96021.B9
Steverson, M. (2018). Ageing and Health [Internet]. World Health Organizaton (WHO). Available at: https://www.who.int/news-room/fact-sheets/detail/ageing-and-health.
Stice, J. P., Lee, J. S., Pechenino, A. S., and Knowlton, A. A. (2009). Estrogen, Aging and the Cardiovascular System. Future Cardiol. 5 (1), 93–103. doi:10.2217/14796678.5.1.93
Stochholm, K., Juul, S., and Gravholt, C. H. (2010). Diagnosis and Mortality in 47,XYY Persons: A Registry Study. Orphanet J. Rare Dis. 5 (1), 15–16. doi:10.1186/1750-1172-5-15
Støylen, A., Dalen, H., and Molmen, H. E. (2020). Left Ventricular Longitudinal Shortening: Relation to Stroke Volume and Ejection Fraction in Ageing, Blood Pressure, Body Size and Gender in the HUNT3 Study. Open Hear 7 (2), e001243. doi:10.1136/openhrt-2020-001243
Strindhall, J., Skog, M., Ernerudh, J., Bengner, M., Löfgren, S., Matussek, A., et al. (2013). The Inverted CD4/CD8 Ratio and Associated Parameters in 66-Year-Old Individuals: The Swedish HEXA Immune Study. Age 35 (3), 985–991. doi:10.1007/s11357-012-9400-3
Stuck, A. E., Moser, A., Morf, U., Wirz, U., Wyser, J., Gillmann, G., et al. (2015). Effect of Health Risk Assessment and Counselling on Health Behaviour and Survival in Older People: A Pragmatic Randomised Trial. Plos Med. 12 (10), e1001889–21. doi:10.1371/journal.pmed.1001889
Suh, J. H., Shenvi, S. V., Dixon, B. M., Liu, H., Jaiswal, A. K., Liu, R.-M., et al. (2004). Decline in Transcriptional Activity of Nrf2 Causes Age-Related Loss of Glutathione Synthesis, Which Is Reversible with Lipoic Acid. Proc. Natl. Acad. Sci. 101 (10), 3381–3386. doi:10.1073/pnas.0400282101
Sun, X., and Feinberg, M. W. (2021). Vascular Endothelial Senescence: Pathobiological Insights, Emerging Long Noncoding RNA Targets, Challenges and Therapeutic Opportunities. Front. Physiol. 12 (June), 1–23. doi:10.3389/fphys.2021.693067
Swanson, E. C., Manning, B., Zhang, H., and Lawrence, J. B. (2013). Higher-order Unfolding of Satellite Heterochromatin Is a Consistent and Early Event in Cell Senescence. J. Cel Biol 203 (6), 929–942. doi:10.1083/jcb.201306073
Tadic, M., Cuspidi, C., and Grassi, G. (2019). The Influence of Sex on Left Ventricular Remodeling in Arterial Hypertension. Heart Fail. Rev. 24 (6), 905–914. doi:10.1007/s10741-019-09803-3
Taneike, M., Yamaguchi, O., Nakai, A., Hikoso, S., Takeda, T., Mizote, I., et al. (2010). Inhibition of Autophagy in the Heart Induces Age-Related Cardiomyopathy. Autophagy 6 (5), 600–606. doi:10.4161/auto.6.5.11947
Taniyama, Y., and Griendling, K. K. (2003). Reactive Oxygen Species in the Vasculature. Hypertension 42 (6), 1075–1081. doi:10.1161/01.hyp.0000100443.09293.4f
Tarry-Adkins, J. L., Ozanne, S. E., Norden, A., Cherif, H., and Hales, C. N. (2006). Lower Antioxidant Capacity and Elevated P53 and P21 May Be a Link between Gender Disparity in Renal Telomere Shortening, Albuminuria, and Longevity. Am. J. Physiol. Ren. Physiol 290 (2), F509–F516. doi:10.1152/ajprenal.00215.2005
Tellez, J. O., Mączewski, M., Yanni, J., Sutyagin, P., Mackiewicz, U., Atkinson, A., et al. (2011). Ageing-dependent Remodelling of Ion Channel and Ca2+clock Genes Underlying Sino-Atrial Node Pacemaking. Exp. Physiol. 96 (11), 1163–1178. doi:10.1113/expphysiol.2011.057752
Tesauro, M., Mauriello, A., Rovella, V., Annicchiarico-Petruzzelli, M., Cardillo, C., Melino, G., et al. (2017). Arterial Ageing: from Endothelial Dysfunction to Vascular Calcification. J. Intern. Med. 281 (5), 471–482. doi:10.1111/joim.12605
Tian, X.-L., and Li, Y. (2014). Endothelial Cell Senescence and Age-Related Vascular Diseases. J. Genet. Genomics 41 (9), 485–495. doi:10.1016/j.jgg.2014.08.001
Tominaga, T., Suzuki, H., Ogata, Y., Matsukawa, S., and Saruta, T. (1991). The Role of Sex Hormones and Sodium Intake in Postmenopausal Hypertension. J. Hum. Hypertens. 5 (6), 495–500.
Traish, A. M., Saad, F., Feeley, R. J., and Guay, A. (2009). The Dark Side of Testosterone Deficiency: III. Cardiovascular Disease. J. Androl. 30 (5), 477–494. doi:10.2164/jandrol.108.007245
Ungvari, Z., Bailey-Downs, L., Sosnowska, D., Gautam, T., Koncz, P., Losonczy, G., et al. (2011). Vascular Oxidative Stress in Aging: A Homeostatic Failure Due to Dysregulation of NRF2-Mediated Antioxidant Response. Am. J. Physiol. Heart Circ. Physiol. 301 (2), H363–H372. doi:10.1152/ajpheart.01134.2010
Ungvari, Z., Bailey-Downs, L., Gautam, T., Sosnowska, D., Wang, M., Monticone, R. E., et al. (2011). Age-Associated Vascular Oxidative Stress, Nrf2 Dysfunction, and NF- B Activation in the Nonhuman Primate Macaca mulatta. Journals Gerontol. Ser. A: Biol. Sci. Med. Sci. 66A (8), 866–875. doi:10.1093/gerona/glr092
United Nations (2019). World Population Ageing 2019 [Internet]. Department of Economic and Social Affairs, Population Division. New York, NY: Springer, 1–111. Available at: http://link.springer.com/chapter/10.1007/978-94-007-5204-7_6.
Uryga, A. K., and Bennett, M. R. (2016). Ageing Induced Vascular Smooth Muscle Cell Senescence in Atherosclerosis. J. Physiol. 594 (8), 2115–2124. doi:10.1113/jp270923
Van Eickels, M., Grohé, C., Cleutjens, J. P. M., Janssen, B. J., Wellens, H. J. J., and Doevendans, P. A. (2001). 17β-Estradiol Attenuates the Development of Pressure-Overload Hypertrophy. Circulation 104 (12), 1419–1423. doi:10.1161/hc3601.095577
Van Guilder, G. P., Westby, C. M., Greiner, J. J., Stauffer, B. L., and DeSouza, C. A. (2007). Endothelin-1 Vasoconstrictor Tone Increases with Age in Healthy Men but Can Be Reduced by Regular Aerobic Exercise. Hypertension 50 (2), 403–409. doi:10.1161/hypertensionaha.107.088294
Vasto, S., Candore, G., Balistreri, C. R., Caruso, M., Colonna-Romano, G., Grimaldi, M. P., et al. (2007). Inflammatory Networks in Ageing, Age-Related Diseases and Longevity. Mech. Ageing Develop. 128 (1), 83–91. doi:10.1016/j.mad.2006.11.015
Vaughan, D. E., Rai, R., Khan, S. S., Eren, M., and Ghosh, A. K. (2017). Plasminogen Activator Inhibitor-1 Is a Marker and a Mediator of Senescence. Arterioscler Thromb. Vasc. Biol. 37 (8), 1446–1452. doi:10.1161/atvbaha.117.309451
Vausort, M., Wagner, D. R., and Devaux, Y. (2014). Long Noncoding RNAs in Patients with Acute Myocardial Infarction. Circ. Res. 115 (7), 668–677. doi:10.1161/circresaha.115.303836
Veiras, L. C., Girardi, A. C. C., Curry, J., Pei, L., Ralph, D. L., Tran, A., et al. (2017). Sexual Dimorphic Pattern of Renal Transporters and Electrolyte Homeostasis. J. Am. Soc. Nephrol. 28 (12), 3504–3517. doi:10.1681/asn.2017030295
Venkatesh, S., and Workman, J. L. (2015). Histone Exchange, Chromatin Structure and the Regulation of Transcription. Nat. Rev. Mol. Cel Biol 16 (3), 178–189. doi:10.1038/nrm3941
Viña, J., Borrás, C., Gambini, J., Sastre, J., and Pallardó, F. V. (2005). Why Females Live Longer Than Males? Importance of the Upregulation of Longevity-Associated Genes by Oestrogenic Compounds. FEBS Lett. 579 (12), 2541–2545. doi:10.1016/j.febslet.2005.03.090
Viña, J., Sastre, J., Pallardó, F., and Borrás, C. (2003). Mitochondrial Theory of Aging: Importance to Explain Why Females Live Longer Than Males. Antioxid. Redox Signaling 5 (5), 549–556. doi:10.1089/152308603770310194
Virani, S. S., Alonso, A., Benjamin, E. J., Bittencourt, M. S., Callaway, C. W., Carson, A. P., et al. (2020). Heart Disease and Stroke Statistics-2020 Update: A Report from the American Heart Association. Circulation 141, e139–e596. doi:10.1161/CIR.0000000000000757
Virdis, A., Ghiadoni, L., Pinto, S., Lombardo, M., Petraglia, F., Gennazzani, A., et al. (2000). Mechanisms Responsible for Endothelial Dysfunction Associated with Acute Estrogen Deprivation in Normotensive Women. Circulation 101 (19), 2258–2263. doi:10.1161/01.cir.101.19.2258
Vlachopoulos, C., Ioakeimidis, N., Miner, M., Aggelis, A., Pietri, P., Terentes-Printzios, D., et al. (2014). Testosterone Deficiency: A Determinant of Aortic Stiffness in Men. Atherosclerosis 233 (1), 278–283. doi:10.1016/j.atherosclerosis.2013.12.010
Vogenberg, F. R., Isaacson Barash, C., and Pursel, M. (2010). Personalized Medicine: Part 1: Evolution and Development into Theranostics. P T 35 (10), 560–576.
Von Zglinicki, T. (2002). Oxidative Stress Shortens Telomeres. Trends Biochem. Sci. 27 (7), 339–344. doi:10.1016/s0968-0004(02)02110-2
Voutilainen, S., Hippeläinen, M., Hulkko, S., Karppinen, K., Ventilä, M., and Kupari, M. (1993). Left Ventricular Diastolic Function by Doppler Echocardiography in Relation to Hormonal Replacement Therapy in Healthy Postmenopausal Women. Am. J. Cardiol. 71 (7), 614–617. doi:10.1016/0002-9149(93)90525-h
Walli-Attaei, M., Joseph, P., Rosengren, A., Chow, C. K., Rangarajan, S., Lear, S. A., et al. (2020). Variations between Women and Men in Risk Factors, Treatments, Cardiovascular Disease Incidence, and Death in 27 High-Income, Middle-Income, and Low-Income Countries (PURE): a Prospective Cohort Study. The Lancet 396 (10244), 97–109. doi:10.1016/s0140-6736(20)30543-2
Wang, H., Jessup, J. A., Lin, M. S., Chagas, C., Lindsey, S. H., and Groban, L. (2012). Activation of GPR30 Attenuates Diastolic Dysfunction and Left Ventricle Remodelling in Oophorectomized mRen2.Lewis Rats. Cardiovasc. Res. 94 (1), 96–104. doi:10.1093/cvr/cvs090
Wang, J. C., and Bennett, M. (2012). Aging and Atherosclerosis. Circ. Res. 111 (2), 245–259. doi:10.1161/circresaha.111.261388
Wang, L., Szklo, M., Folsom, A. R., Cook, N. R., Gapstur, S. M., and Ouyang, P. (2012). Endogenous Sex Hormones, Blood Pressure Change, and Risk of Hypertension in Postmenopausal Women: The Multi-Ethnic Study of Atherosclerosis. Atherosclerosis 224 (1), 228–234. doi:10.1016/j.atherosclerosis.2012.07.005
Wang, M., Khazan, B., and G. Lakatta, E. (2010). Central Arterial Aging and Angiotensin II Signaling. Chyr 6 (4), 266–281. doi:10.2174/157340210793611668
Wang, M., Smith, K., Yu, Q., Miller, C., Singh, K., and Sen, C. K. (2020). Mitochondrial Connexin 43 in Sex-dependent Myocardial Responses and Estrogen-Mediated Cardiac protection Following Acute Ischemia/reperfusion Injury. Basic Res. Cardiol. 115 (1). doi:10.1007/s00395-019-0759-5
Wang, M., Zhang, J., Telljohann, R., Jiang, L., Wu, J., Monticone, R. E., et al. (2012). Chronic Matrix Metalloproteinase Inhibition Retards Age-Associated Arterial Proinflammation and Increase in Blood Pressure. Hypertension 60 (2), 459–466. doi:10.1161/hypertensionaha.112.191270
Wanitschek, M. M., Dörler, J., and Alber, H. F. (2016). Chronic Stable Angina. N. Engl. J. Med. 375 (3), 292–92. doi:10.1056/NEJMc1605394
Wenner, M. M., Sebzda, K. N., Kuczmarski, A. V., Pohlig, R. T., and Edwards, D. G. (2017). ETB Receptor Contribution to Vascular Dysfunction in Postmenopausal Women. Am. J. Physiol. Regul. Integr. Comp. Physiol. 313 (1), R51–R57. doi:10.1152/ajpregu.00410.2016
Westby, C. M., Weil, B. R., Greiner, J. J., Stauffer, B. L., and DeSouza, C. A. (2011). Endothelin-1 Vasoconstriction and the Age-Related Decline in Endothelium-dependent Vasodilatation in Men. Clin. Sci. 120 (11), 485–491. doi:10.1042/cs20100475
Weyand, C. M., and Goronzy, J. J. (2016). Aging of the Immune System. Mechanisms and Therapeutic Targets. Ann. Am. Thorac. Soc. 13 Suppl 5 (December), S422–S428. doi:10.1513/AnnalsATS.201602-095AW
Xia, S., Zhang, X., Zheng, S., Khanabdali, R., Kalionis, B., Wu, J., et al. (2017). An Update on Dementia Prevention and Treatment. Aust. N. Z. J. Psychiatry 51 (1), 6. doi:10.1177/0004867417702054
Xu, X., Zhan, M., Duan, W., Prabhu, V., Brenneman, R., Wood, W., et al. (2007). Gene Expression Atlas of the Mouse central Nervous System: Impact and Interactions of Age, Energy Intake and Gender. Genome Biol. 8 (11), R234. doi:10.1186/gb-2007-8-11-r234
Yaghooti, H., Firoozrai, M., Fallah, S., and Khorramizadeh, M. R. (2011). Angiotensin II Induces NF-κB, JNK and P38 MAPK Activation in Monocytic Cells and Increases Matrix Metalloproteinase-9 Expression in a PKC- andRho Kinase-dependent Manner. Braz. J. Med. Biol. Res. 44 (3), 193–199. doi:10.1590/s0100-879x2011007500008
Yan, L., Ge, H., Li, H., Lieber, S., Natividad, F., Resuello, R., et al. (2004). Gender-specific Proteomic Alterations in Glycolytic and Mitochondrial Pathways in Aging Monkey Hearts. J. Mol. Cell Cardiol. 37 (5), 921–929. doi:10.1016/j.yjmcc.2004.06.012
Yaron, M., Greenman, Y., Rosenfeld, J. B., Izkhakov, E., Limor, R., Osher, E., et al. (2009). Effect of Testosterone Replacement Therapy on Arterial Stiffness in Older Hypogonadal Men. Eur. J. Endocrinol. 160 (5), 839–846. doi:10.1530/eje-09-0052
Yoneyama, K., Gjesdal, O., Choi, E.-Y., Wu, C. O., Hundley, W. G., Gomes, A. S., et al. (2012). Age, Sex, and Hypertension-Related Remodeling Influences Left Ventricular Torsion Assessed by Tagged Cardiac Magnetic Resonance in Asymptomatic Individuals. Circulation 126 (21), 2481–2490. doi:10.1161/circulationaha.112.093146
Yoshida, Y., Nakanishi, K., Daimon, M., Ishiwata, J., Sawada, N., Hirokawa, M., et al. (2020). Sex-specific Difference in the Association between Arterial Stiffness and Subclinical Left Ventricular Dysfunction. Eur. Heart J. Cardiovasc. Imaging 81, 1–7. doi:10.1093/ehjci/jeaa156
Yu, L., Wan, F., Dutta, S., Welsh, S., Liu, Z., Freundt, E., et al. (2006). Autophagic Programmed Cell Death by Selective Catalase Degradation. Proc. Natl. Acad. Sci. 103 (13), 4952–4957. doi:10.1073/pnas.0511288103
Zangrando, J., Zhang, L., Vausort, M., Maskali, F., Marie, P. Y., Wagner, D. R., et al. (2014). Identification of Candidate Long Non-coding RNAs in Response to Myocardial Infarction. BMC Genomics 15 (1), 1–14. doi:10.1186/1471-2164-15-460
Zhang, H., Davies, K. J. A., and Forman, H. J. (2015). Oxidative Stress Response and Nrf2 Signaling in Aging. Free Radic. Biol. Med. 88, 314–336. doi:10.1016/j.freeradbiomed.2015.05.036
Zhang, J., Patel, J. M., and Block, E. R. (2002). Enhanced Apoptosis in Prolonged Cultures of Senescent Porcine Pulmonary Artery Endothelial Cells. Mech. Ageing Develop. 123 (6), 613–625. doi:10.1016/s0047-6374(01)00412-2
Zhang, W., Song, M., Qu, J., and Liu, G.-H. (2018). Epigenetic Modifications in Cardiovascular Aging and Diseases. Circ. Res. 123 (7), 773–786. doi:10.1161/circresaha.118.312497
Zhang, X.-P., Vatner, S. F., Shen, Y.-T., Rossi, F., Tian, Y., Peppas, A., et al. (2007). Increased Apoptosis and Myocyte Enlargement with Decreased Cardiac Mass; Distinctive Features of the Aging Male, but Not Female, Monkey Heart. J. Mol. Cell Cardiol. 43 (4), 487–491. doi:10.1016/j.yjmcc.2007.07.048
Zhao, D., Guallar, E., Ouyang, P., Subramanya, V., Vaidya, D., Ndumele, C. E., et al. (2018). Endogenous Sex Hormones and Incident Cardiovascular Disease in Post-Menopausal Women. J. Am. Coll. Cardiol. 71 (22), 2555–2566. doi:10.1016/j.jacc.2018.01.083
Zhao, M., Woodward, M., Vaartjes, I., Millett, E. R. C., Klipstein-Grobusch, K., Hyun, K., et al. (2020). Sex Differences in Cardiovascular Medication Prescription in Primary Care: A Systematic Review and Meta-Analysis. J. Am. Heart Assoc. 9 (11), e014742. doi:10.1161/JAHA.119.014742
Zhao, X., Zhao, M., Amin-Hanjani, S., Du, X., Ruland, S., and Charbel, F. T. (2015). Wall Shear Stress in Major Cerebral Arteries as a Function of Age and Gender-A Study of 301 Healthy Volunteers. J. Neuroimaging 25 (3), 403–407. doi:10.1111/jon.12133
Zhavoronkov, A., Smit-Mcbride, Z., Guinan, K. J., Litovchenko, M., and Moskalev, A. (2012). Potential Therapeutic Approaches for Modulating Expression and Accumulation of Defective Lamin A in Laminopathies and Age-Related Diseases. J. Mol. Med. 90 (12), 1361–1389. doi:10.1007/s00109-012-0962-4
Zhong, Y., Wang, Q. J., Li, X., Yan, Y., Backer, J. M., Chait, B. T., et al. (2009). Distinct Regulation of Autophagic Activity by Atg14L and Rubicon Associated with Beclin 1-Phosphatidylinositol-3-Kinase Complex. Nat. Cel Biol 11 (4), 468–476. doi:10.1038/ncb1854
Zupanc, G. K. H. (2020). Development of a Sexual Dimorphism in a central Pattern Generator Driving a Rhythmic Behavior: The Role of Glia-Mediated Potassium Buffering in the Pacemaker Nucleus of the Weakly Electric Fish Apteronotus Leptorhynchus. Dev. Neurobiol. 80 (1–2), 6–15. doi:10.1002/dneu.22736
Keywords: sex differences, aging mechanism, oxidative stress, autphagy, telomerase, RNA, inflammation
Citation: Dela Justina V, Miguez JSG, Priviero F, Sullivan JC, Giachini FR and Webb RC (2021) Sex Differences in Molecular Mechanisms of Cardiovascular Aging. Front. Aging 2:725884. doi: 10.3389/fragi.2021.725884
Received: 15 June 2021; Accepted: 25 August 2021;
Published: 10 September 2021.
Edited by:
Kerrie L. Moreau, University of Colorado Anschutz Medical Campus, United StatesReviewed by:
Devin Wahl, Colorado State University, United StatesConcepción Peiró, Autonomous University of Madrid, Spain
Copyright © 2021 Dela Justina, Miguez, Priviero, Sullivan, Giachini and Webb. This is an open-access article distributed under the terms of the Creative Commons Attribution License (CC BY). The use, distribution or reproduction in other forums is permitted, provided the original author(s) and the copyright owner(s) are credited and that the original publication in this journal is cited, in accordance with accepted academic practice. No use, distribution or reproduction is permitted which does not comply with these terms.
*Correspondence: Vanessa Dela Justina, dmFuZV9jZXNzYUBob3RtYWlsLmNvbQ==
†These authors have contributed equally to this work