- 1International Clinical Research Center, St. Anne’s University Hospital Brno, Brno, Czech Republic
- 2Department of Biology, Faculty of Medicine, Masaryk University, Brno, Czech Republic
- 3Department of Pediatric Oncology, University Hospital Brno, Brno, Czech Republic
- 4Institute of Hematology and Blood Transfusion, Prague, Czech Republic
The population of childhood cancer survivors (CCS) has grown rapidly in recent decades. Although cured of their original malignancy, these individuals are at increased risk of serious late effects, including age-associated complications. An impaired immune system has been linked to the emergence of these conditions in the elderly and CCS, likely due to senescent immune cell phenotypes accompanied by low-grade inflammation, which in the elderly is known as “inflammaging.” Whether these observations in the elderly and CCS are underpinned by similar mechanisms is unclear. If so, existing knowledge on immunosenescent phenotypes and inflammaging might potentially serve to benefit CCS. We summarize recent findings on the immune changes in CCS and the elderly, and highlight the similarities and identify areas for future research. Improving our understanding of the underlying mechanisms and immunosenescent markers of accelerated immune aging might help us to identify individuals at increased risk of serious health complications.
Introduction
Each year, ∼300,000 children are diagnosed with a form of cancer globally. (Steliarova-Foucher et al., 2017). Cancer therapy has made immense progress in recent decades, increasing the 5 year survival rate to up to 85% in countries with advanced healthcare systems (Gatta et al., 2014; Howlader et al., 2020). Yet with this great step forward, a significant problem has emerged: childhood cancer survivors (CCS) have a higher incidence of developing other, severe health conditions compared to their siblings (Armstrong et al., 2014), with up to 75% experiencing at least one late adverse effect and 40% suffering from at least one serious or life-threatening condition in early-mid adulthood (Geenen et al., 2007). The earlier prevalence of certain health complications in CCS compared to their siblings (or control population) (Figure 1) is a phenomenon known as premature aging. These complications include cardiovascular diseases, frailty, and secondary neoplasms (Oeffinger et al., 2006; Armstrong et al., 2013; Ness et al., 2013). Frailty is described by experiencing three or more conditions of established frailty phenotype (low lean muscle mass, exhaustion, low energy expenditure, slowness and weakness), while prefrailty is described by experiencing two of these conditions conditions (Ness et al., 2013). The early onset of health complications for CCS seems to prove the fragile health status of CCS being more similar to elderly (Table 1). The most pronounced comorbidities in CCS span through many tissues and organ systems, such as pulmonary, cardiac and circulatory, genitourinary, nervous and endocrine (Geenen et al., 2007; Reulen et al., 2010; Armstrong et al., 2014; Bhakta et al., 2017). The diseases developing in above mentioned organ systems are accompanied by metabolic changes, higher rate of infections and subsequent malignancy (Reulen et al., 2010; Lorenzi et al., 2011). In CCS, secondary cancer has been described as the most severe comorbid condition compared to cardiovascular or respiratory diseases (Reulen et al., 2010). The emergence of these conditions is thought to be driven by late effects of the often aggressive treatments needed to cure the childhood cancer, (Gallicchio et al., 2008; Fowler et al., 2020), but the precise mechanisms are unknown. However, cancer therapeutics have been already associated with cellular aging through senescence initiation, free radical generation, DNA damage and telomere attrition (Cupit-Link et al., 2017; Wang et al., 2021).
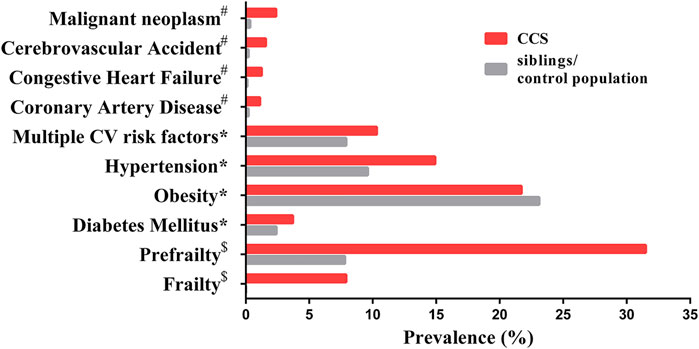
FIGURE 1. The prevalence of age-associated complications in CCS and siblings/control population. Data marked # has a mean age of 26.6 years (range 18–48) for CCS and a mean age of 29.2 years (range 18–56) for siblings (Oeffinger et al., 2006); data marked * has a median age of 33.7 years (range 11–58) in CCS and a median age of 36 years (range 7–62) for siblings (Armstrong et al., 2013); data marked $ has a mean age of 33.6 years (range 18–50) for CCS and a mean age of 29 years (range 18–50) for the control population (Ness et al., 2013). Malignant neoplasm represents secondary neoplasms for CCS and primary neoplasms for siblings/control population. Abbreviation: CV, cardiovascular.
Many conditions affecting CCS in early/mid-adulthood are age-associated; cardiovascular diseases, diabetes mellitus, hypertension, frailty and cancer increase during aging (Partridge et al., 2018). Aging itself is a complex process that ultimately results in the gradual decline of various critical cellular processes, signaling pathways, and regulatory mechanisms, leading to eventual disruption of tissue homeostasis and the emergence of disease (López-Otín et al., 2013). The immune compartment is widely affected by aging through the process of progressive dysregulation of immune function. Alterations to the innate and adaptive immune compartments and aging-associated chronic low-grade inflammation (CLGI), (Solana et al., 2012; Fulop et al., 2013), termed “inflammaging” (Franceschi et al., 2000a), are common. Together, these changes are termed “immunosenescence” and contribute to the emergence of various health conditions, such as frailty, (Pansarasa et al., 2019), type 2 diabetes mellitus (Lee et al., 2019), pulmonary diseases (Murray and Chotirmall, 2015), increased susceptibility to infections, autoimmune disease, and cancer (Pawelec, 1999) in aged individuals. Unsurprisingly, strategies aimed at improving aspects of immunosenescence in the elderly are being actively explored.
The immune systems of young adult CCS exhibit common features with those of the elderly (Azanan et al., 2016; Ariffin et al., 2017). High prevalence of typical aging-associated conditions in CCS suggest that CCS might exhibit premature immunosenescence as a result of their cancer and/or its treatment. Understanding this phenomenon will help determine whether screening for early immunosenescence will enable the risk-stratification of CCS after treatment and thereby facilitate optimal clinical management, e.g. earlier screening for secondary cancer. Furthermore, if conserved mechanisms are at play in both CCS and elderly patients, emerging therapies to ameliorate immunosenescence in the elderly should be assessed for their potential benefit to CCS.
In this review, we discuss the possible adverse effects of childhood cancer treatment on the immune system and the potential links to enduring ill health in CCS. We compare these effects to the senescent immune system in the “healthy” elderly, assessing the evidence for parallel versus distinct mechanisms in these two populations. We conclude by speculating on how we might exploit our knowledge of cellular, molecular and epigenetic mechanisms of immunosenescence to improve health and wellbeing in CCS and we highlight possible directions for future research in the field.
Immune Cell Subsets Affected by Aging and Childhood Cancer Treatment
Many of the initial studies defining immunosenescence were performed in unique cohorts of centenarians, allowing the identification of immunosenescent cellular phenotypes/markers that enable successful aging (Effros et al., 1994; Fagnoni et al., 1996; Ostan et al., 2008). These descriptive studies revealed characteristic frequencies of immunosenescence-associated cell subsets (naïve, memory and terminally differentiated T cells) that are now widely used to describe the immunosenescent phenotype in humans. Stemming from this work, several possible mechanisms were proposed to account for the development of immunosenescence, including 1) decrease in naïve T cells accompanied by expansion of memory T cell subsets with age; (Saule et al., 2006) 2) changes in myeloid cells, particularly monocytes; (Hearps et al., 2012) 3) CLGI; (Fülöp et al., 2019) and 4) chronic infection with pathogens such as cytomegalovirus (CMV) (Olsson et al., 2000; Koch et al., 2007; Kallemeijn et al., 2017).
Immune changes in both the elderly and CCS have now been identified (Table 2); below we consider each immune cell subset in turn, identifying the parallels and differences between the two population groups.
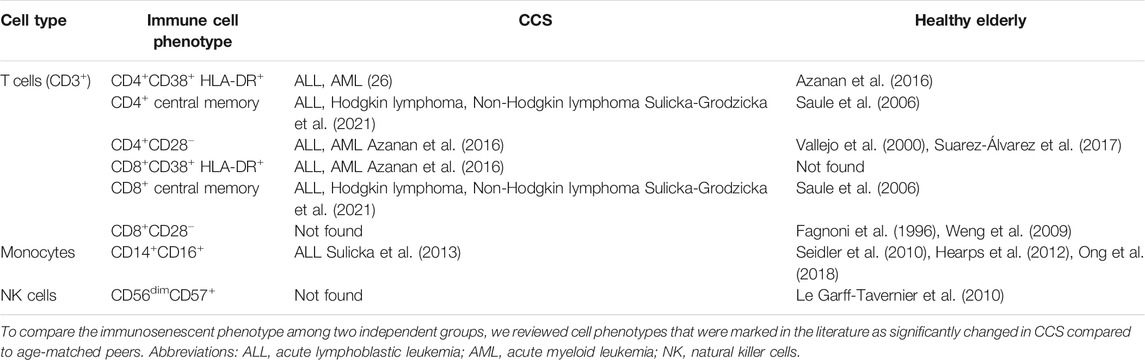
TABLE 2. The phenotypes of cellular subsets associated with senescence/immunosenescence in CCS and the healthy elderly.
Adaptive Immune Cells
T Cells
Age-associated changes in the subpopulation frequencies of CD4+ and CD8+ T cells are well established (Di Mitri et al., 2011; Montecino-Rodriguez et al., 2013; Lanna et al., 2014; Callender et al., 2018). Multi-parameter flow cytometry of circulating T-cell subsets in cohorts of elderly individuals has identified increasing proportions of specific T-cell subsets as major immunosenescence markers. The four main T-cell subsets are distinguished based on the recognition of antigens and are delineated as naive (CD45RA+, CD45RO−, CD27+, CD28+, and CCR7+), central memory (CD45RA−, CD45RO+, CD27+, CD28+, and CCR7+), effector memory (CD45RA−, CD45RO+, CD27−, CD28−, and CCR7−) and terminal effector T cells (CD45RA+, CD45RO−, CD27−, CD28− and CCR7−) (Wang et al., 1995; Boucher et al., 1998; Hendriks et al., 2000; Larbi and Fulop, 2014; Xu and Larbi, 2017). In general, a decrease in naive T cells and an increase in effector memory and terminal effector T cells during normal aging have been established (Saule et al., 2006). Interestingly, even though markers used to determine immunosenescent phenotype are established (Figure 2), T-cell phenotyping strategies using flow cytometry still vary among human studies. (Fagnoni et al., 1996; Kovaiou et al., 2005; Koch et al., 2008; Lázničková et al., 2020).
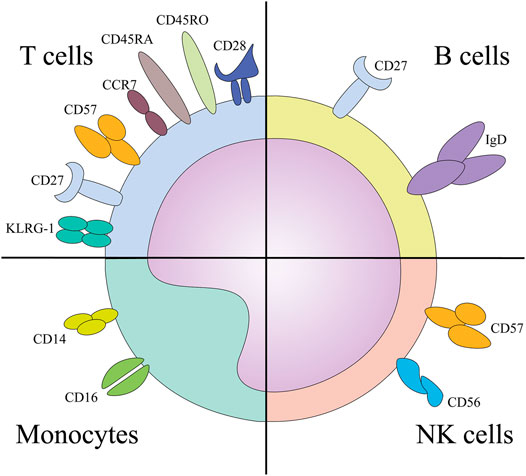
FIGURE 2. The surface markers implicated in the immunosenescence phenotype of designated cells. Abbreviations: KLRG-1, Killer cell lectin like receptor sub family G member 1; NK, natural killer cells.
The marker expression patterns of senescent T cells are consistent with their altered functionality. Instead of expressing co-stimulatory molecules such as CD27 and CD28, (Hendriks et al., 2000; Weng et al., 2009; Parish et al., 2010) senescent T cells express Killer cell lectin-like receptor subfamily G member 1 (KLRG-1) and CD57, (Brenchley et al., 2003; Wherry, 2011) which are associated with low proliferative capacity but high cytotoxic potential (Ibegbu et al., 2005; Kared et al., 2016). Furthermore, these cells exhibit elevated reactive oxygen species (ROS) production and constitutive p38 MAP kinase activation in response to AMP-activated protein kinase (AMPK) and possibly a nutrient-sensing and/or DNA-damage response (Lanna et al., 2014; Akbar et al., 2016). Senescent T cells are also characterized by shorter telomeres, the inability to upregulate telomerase expression, inadequate expression of the DNA damage response machinery, increased cyclin-dependent kinase inhibitor p16INK4a expression, and elevated secretion of inflammatory cytokines (Akbar et al., 2016). Chemotherapy regimens and stem cell transplantation cause T cell senescence through p16INK4a expression in patients treated for hematological malignancy (Wood et al., 2016) and chemotherapy can induce surface expression of CD57 in Vδ2pos T cells as demonstrated in elderly patients with liver metastatic colorectal cancer (Bruni et al., 2019). On top of that, it has been shown that T cells from patients with hematological malignancy show higher clonality and lower T cell diversity based on T cell receptor repertoire analysis by next-generation sequencing than those with solid tumors, when compared to healthy donors above 60 yo (Simnica et al., 2019). Therefore, certain therapeutic approaches may represent a risk for senescence induction in T cells with the potential to compromise the overall health status of cancer survivors. Moreover, a mouse study has uncovered that senescence driven by Ercc1 deletion (encoding a crucial DNA repair protein) in hematopoietic cells drives senescence also in other non-lymphoid organs, e.g. aorta, heart, lung, liver and gastrointestinal tract, and splenocytes from these mice drive senescence in young senescence reporter mice upon transplant (Yousefzadeh et al., 2021).
T-cell frequency studies in CCS are scarce (Table 2), but we recently showed that high-risk neuroblastoma CCS exhibit immunosenescent-like alterations, including a decrease in naive T-cell frequency and an increase in the frequency of memory T cells, accompanied by the transient expression of CD57 in memory T-cell populations within the first 4 years after diagnosis (Lázničková et al., 2020). Other T-cell phenotyping studies in CCS have also detected the loss of CD28 and increase in CD57 in CD4+ T cells (Azanan et al., 2016). The studies of activation status of T cells in CCS and elderly are unparalleled. Increased activation of CD8+ T cells by double positivity for CD38 and HLA-DR has been detected in CCS, (Azanan et al., 2016) while the activation status by only CD38 increased (Sulicka-Grodzicka et al., 2021) and by HLA-DR only decreased in CD8+ T cells in CCS (Chua et al., 2017). In the elderly, the activation status by only CD38 has been found to be decreased and by only HLA-DR increased in CD8+ T cells in elderly (Qin et al., 2016). Interestingly, terminally differentiated CD8+ T cells do not show a senescent phenotype in CCS like in elderly, while the central memory CD8+ and CD4+ T cells increase in both, CCS and elderly (Saule et al., 2006; Sulicka-Grodzicka et al., 2021).
In addition to phenotyping and telomere length analyses, epigenetic remodeling in blood cells contributes to the alterations in genome that accompany aging (Weidner et al., 2014). By this approach, researchers have identified aging-related T-cell epigenetic remodeling in CCS (Daniel et al., 2018). For example, total body irradiation alters the DNA methylation signature of T cells, which has been associated with an increased frequency of type 1 cytokine-producing T cells and increased systemic levels of these cytokines (Daniel et al., 2018). Epigenetic changes might, therefore, explain why radiotherapy confers up to an eight-fold increased risk of a severe or life-threatening medical condition (Oeffinger et al., 2006; Armstrong et al., 2010; Ness et al., 2018).
B Cells
The role of B cells in immunosenescence is relatively under-studied and thus their contribution to this process is elusive. In the peripheral blood, four major B-cell subsets can be distinguished, based on expression patterns of surface molecules: naive (IgD+/CD27−), IgM memory (IgD+/CD27+), switched memory (IgD−/CD27+), and late/exhausted memory (LM, IgD−/CD27−). While total B-cell counts remain relatively unchanged in the peripheral blood in healthy adults, (Blanco et al., 2018) recent findings have indicated a shift in the B-cell subset distribution in the elderly in favor of LM B cells. This shift is accompanied by increased expression of senescence-associated secretory phenotype (SASP) markers, including TNF-α, IL-6, and IL-8 (Frasca et al., 2017a; Frasca et al., 2017b). Quantification of the LM B-cells subset showed a decrease in CSS and naive B cells an increase in CCS compared to age-matched controls, (Sulicka-Grodzicka et al., 2021) showing a deregulation in the B cell compartment in both directions, to healthy peers and to elderly. The accumulation of the LM B-cell subset contributes to lower vaccine protection against influenza in the elderly and is further decreased in obese individuals (Frasca et al., 2016). CCS are often in need of boosters or complete revaccination after cancer therapy due to active treatment in early childhood resulting in incomplete series of vaccinations and no/low immune recovery depending on the type of implemented treatment (Patel et al., 2007; Shetty and Winter, 2012; Han et al., 2018; Choi et al., 2020). In contrast to children with active chemotherapy treatment, patients who had completed chemotherapy and healthy children had similar, stronger vaccination response efficacies (Goossen et al., 2013). Therefore, monitoring of B cell subsets might be an indicator of the B cell ability to cope with infections and eventually infection rate in general but the subset distribution in CCS does not seem to reflect the aging phenotype described in elderly.
In the year 2011, a subset of B cells designated age-associated B cells (ABCs) was shown to accumulate in aged mice (Hao et al., 2011; Rubtsov et al., 2011). These cells are responsive to Toll-like receptor (TLR) 7 and 9 stimulation, actively secrete IL-10 and IL-4, and favor T-cell polarization towards T helper 17 (Th17) cells. In 2019, their possible counterparts have been identified in humans in relation to autoimmune diseases and viral infections (Ma et al., 2019). Whether these B cells also accumulate during normal aging or in CCS is unclear. Therefore, there is a need of verifying this B cell subset in elderly and find the comorbidities associated with this phenotype. The mouse studies (Hao et al., 2011; Rubtsov et al., 2011) imply that comorbidities linked to higher emergence of Th17 cells (e.g. obesity) might be linked with these ABCs (Frasca et al., 2017a; Blanco et al., 2018). Whether ABCs will be applicable to CCS remains elusive but due to the prevalence of comorbidities similar to those in the elderly, chances are the phenotype might be present.
Innate Immune Cells
The profound age-associated changes in the immune system underlying immunosenescence are mainly linked to adaptive immunity. However, research conducted over the past two decades has demonstrated many functional age-related alterations in innate immune cells (Shaw et al., 2013; Pinti et al., 2016; Agrawal et al., 2017; Ortmann and Kolaczkowska, 2018). We outline the main findings on innate immune cells in elderly and CCS below.
Monocytes/Macrophages
Monocyte/macrophage activation status and function are essential for appropriate immune responses against pathogens and transformed cells and for mounting the adaptive immune response. Data from human and animal studies show age-related dysfunction of the monocyte/macrophage system (Solana et al., 2012; Linehan and Fitzgerald, 2015). A detailed overview addressing the impact of ageing on monocyte/macrophage function is beyond the scope of this article and more comprehensive reviews can be found elsewhere (Shaw et al., 2010; Solana et al., 2012). Here we will highlight sporadic recent findings of monocyte/macrophage alteration seen in CCS, potentially associated with the chronic health condition development.
While the total number of monocytes seems to be unaffected by aging, (Seidler et al., 2010) proportional changes in monocyte subsets, including the relative expansion of CD16+ populations, have been observed in healthy males and females (20–84 yo) (Hearps et al., 2012). The CD14+CD16+ monocyte subset is also expanded in CCS (Sulicka et al., 2013) (Table 2). Since CD16+ monocyte subsets are characterized as significant producers of pro-inflammatory cytokines, (Hearps et al., 2012) their proportional increase in the elderly and CCS may contribute to CLGI and thus to the pathogenesis of inflammatory diseases. Moreover, significantly shorter relative telomere length has been determined in monocyte subsets from older individuals. Although information about monocyte telomere length in CCS are not available, shortened leukocyte telomere length has been reported in CCS (Song et al., 2020). Whether telomere shortening affect monocyte function remain to be elucidated. Nevertheless, shorter leukocyte telomeres have been associated with chronic health conditions in elderly and CCS (Spyridopoulos et al., 2009; Song et al., 2020). Although older individuals exhibit dysregulated inflammatory response, (Hearps et al., 2012; Metcalf et al., 2017) no data on monocyte functions, including phagocytosis or TLR stimulation, are available in CCS at the moment. This knowledge gap provides an opportunity for future research studies in the CCS field.
Neutrophils
Neutrophils are professional phagocytes adapted to perform rapid immune action at the site of infection or tissue damage. Even though neutrophil counts remain stable during an individual’s adulthood, (Solana et al., 2012) profound age-related changes at the functional level have been reported. In particular, neutrophils from elderly subjects exhibit reduced migration potential, phagocytosis, bactericidal activity, ROS production, and neutrophil extracellular trap formation (Wenisch et al., 2000; Butcher et al., 2001; Simell et al., 2011; Brubaker et al., 2013; Hazeldine et al., 2014; Sapey et al., 2014; Itagaki et al., 2015; Sauce et al., 2017). By contrast, evidence of increased neutrophil proteinase activity and upregulated degranulation has been observed in older adults (Sapey et al., 2014). Interestingly, in a mouse model, a higher level of neutrophil elastase was associated with a low-grade inflammatory state accompanying obesity (Talukdar et al., 2012). In CCS, a low absolute neutrophil count (neutropenia) has been reported as a consequence of intensive cancer treatment and possess a higher risk of infection (Han et al., 2009). Studies on neutrophil functionality in CCS are lacking but we can speculate that neutrophils of CCS might possess similar defects as seen in elderly. Thus, the impaired effector function of neutrophils might be involved in the increased susceptibility of some CCS to microbial infections.
Dendritic Cells
Dendritic cells (DCs) are professional antigen-presenting cells that can prime naive T-cell activation and effector differentiation. In general, the phenotypes and numbers of DCs are largely unaffected by aging, (Granot et al., 2017) although some studies have reported a marked decrease in plasmacytoid DCs (pDCs) (Pérez-Cabezas et al., 2007; Jing et al., 2009; Garbe et al., 2012; Splunter et al., 2019) and in CD141+ myeloid DCs (mDCs) upon in vitro stimulation with retinoic acid in healthy elderly (Agrawal et al., 2016). Since pDCs play key role in antiviral responses, their reduction in combination with mDC dysfunction may partially explain higher incidence of viral infections in elderly (Jing et al., 2009). The same mechanism might be critical for some CCS, although there are currently no studies regarding DC phenotype and function in CCS.
Concerning DC function, the following alterations have been reported in elderly individuals: decreased capacity to phagocytose antigens, (Agrawal et al., 2007) migrate, (Agrawal et al., 2007) prime naive T cells after stimulation, (Sridharan et al., 2011) respond to TLR stimulation, (Panda et al., 2010) secrete type I and III interferons (Panda et al., 2010; Qian et al., 2011; Sridharan et al., 2011). Furthermore, these cells produce increased levels of pro-inflammatory cytokines, and decreased levels of anti-inflammatory and immune-regulatory cytokines (summarized in review) (Agrawal et al., 2017). Overall, the disruption of DC functions followed by increased pro-inflammatory potential might result not only in higher risk of infections but also to CLGI and loss of tolerance. Thus, DC dysfunction likely contribute to chronic age-associated diseases in elderly and probably in CCS.
Natural Killer Cells
Natural killer (NK) cells are a subset of cytotoxic non-T lymphocytes that promote an early innate immune response by recognizing and killing virus-infected and tumor cells. Thus, quantitative and functional NK cell defect may lower resistance to infections and protecting effect against tumors in elderly/CCS. While the distribution of NK cell subsets seems to be altered in elderly individuals, the data are inconsistent (Pinti et al., 2016). A decrease in CD56bright NK cells as a possible consequence of limited production of new NK cells, (Chidrawar et al., 2006; Le Garff-Tavernier et al., 2010) with a concomitant increase in CD56dim NK cell population, has been reported in the elderly (Le Garff-Tavernier et al., 2010). Similar to T cells, CD57 expression can be used as a marker of replicative senescence in NK cells (White et al., 2014). The aging-related changes in NK cells described in the elderly population have not been studied in CCS. Nevertheless, decreased or absent NK cell cytolytic activity in 25% of patients has been reported in CCS 6 months after completion of leukemia therapy (Perkins et al., 2017). If altered NK cell function is transient or persist for years after therapy completion remains to be determined.
Hematopoietic Stem and Progenitor Cell Aging in the Elderly and Childhood Cancer Survivors
Thus far, we have discussed how age-related alterations of individual immune cell types and their function underlie aging. However, there are also data to suggest that the age-related dysfunction of effector immune cells is in fact inherited from their progenitors. Throughout our lifespan, encounters with various stressors (e.g. allergens, viruses, bacteria, therapy of more or less severe disorders and diseases) progressively impair various cellular repair mechanisms. A consequence of inadequate repair is hematopoietic stem and progenitor cell (HSPCs) aging (Todhunter et al., 2018). Key markers of HSPC aging include higher levels of oxidative stress and DNA damage response rates, expression of p21–p53, senescence-associated β-galactosidase, and shorter telomeres (Fali et al., 2018). Additionally, the expression of genes involved in hematopoiesis, leukocyte activation, and intracellular signaling is downregulated in aged human bone marrow-derived HSPCs (Rundberg Nilsson et al., 2016).
Aged HSPCs in humans are associated with perturbed lymphopoiesis (Kuranda et al., 2011) and increased myeloid cell differentiation, (Pang et al., 2011) underlined by epigenetic changes (Beerman et al., 2013) and an age-related higher frequency of hematopoietic stem cells (HSCs) within the bone marrow (Pang et al., 2011; Farrell et al., 2014). However, data from a study on bone marrow and blood samples from patients undergoing hip replacement surgery suggests lower frequencies of HSCs in both, the bone marrow and peripheral blood in the elderly (Brusnahan et al., 2010).
Another age-related phenomenon of clonal hematopoiesis is associated with a risk of developing hematologic malignancies and an increase in specific somatic mutations in peripheral blood cells with age (Genovese et al., 2014; Jaiswal et al., 2014). These mutations have been further associated with an increased risk of myocardial infarction and coronary heart disease (Jaiswal et al., 2017). However, these mutations occur in a relatively small fraction of individuals (12.5%) as was demonstrated in the study population of Icelanders (11,262 men and women, median age of 74 years) (Zink et al., 2017). However, prevalence of these mutations increased towards 50% in subpopulation of Icelanders >85 years. Interestingly, 10 year survivorship of individuals >80 years of age seems unaffected by carrying the mutations in two of the most common genes associated to clonal hematopoiesis DNMT3A and TET2 (van den Akker et al., 2016) compared to middle-aged population study (Jaiswal et al., 2014).
HSC transplantation is a frequently used therapeutic option in childhood cancer treatment, but only few researchers have assessed its long-term impact on overall immune health in CCS. Telomere length in HSCs rapidly shortens upon transplant while replenishing the pool of blood cells. Recipients of HSC transplants have shorter telomeres in leukocytes compared with their HSC donors, (Wynn et al., 1998; Baerlocher et al., 2009) suggesting a higher turnover of recipient HSCs to ensure immune recovery. A high degree of telomere loss might occur between CD34+CD38− and CD34+CD38+, (Vaziri et al., 1994) while more committed progenitors are more rapidly replenishing the pool of blood cells in recipients (Wynn et al., 1999). Therefore, the higher turnover rate of HSCs may predispose the recipient to an increased risk of accelerated aging of the hematopoietic compartment. This suggestion has been confirmed in serial transplantation assays in vitro, which showed that HSCs with increased cell cycle activity resulted in shorter telomeres, (Allsopp et al., 2001) even though HSCs exhibit some telomerase activity (Morrison et al., 1996). Moreover, HSCs after serial transplantation possess high levels of ROS, (Jang and Sharkis, 2007) which could lead to post-transplant HSCs suffering from oxidative damage to cellular components and altered metabolism and intracellular signaling (Zhang et al., 2016; Forrester et al., 2018; Milkovic et al., 2019). Although the telomere length of leukocytes in HSC transplant recipients and other markers described previously, such as high ROS and altered intracellular signaling, suggest immunosenescence in CCS, there are no studies confirming the presence of HSC aging markers and their fitness in CCS compared to the elderly. Moreover, there are no studies describing HSC aging in CCS comparing individuals with and without HSC transplantation. Even though, HSCs aging could be caused by transplantation followed by higher turnover of HSCs, there can be other likely mechanisms involved. First of all, telomerase complex has been implicated to control hematopoietic stem cell differentiation and senescence in vitro (Jose et al., 2018). Second of all, mesenchymal stromal cells (MSCs), which have been described to be critical for extracellular matrix production in the bone marrow, and thus facilitation of HSCs engraftment and cell fate decision, (Zhao and Liu, 2016; Krater et al., 2017), can likely present another pro-aging scenario regarding HSCs. There has been evidence, that paclitaxel (chemotherapy drug) induces senescence in MSCs in vitro, (Munz et al., 2018) and SASP produced by MSCs can in turn cause senescence of other epithelial cells posing a risk for HSCs upon transplant (Alessio et al., 2019). Moreover, while MSCs are being investigated to improve the engraftment of HSCs and to overcome Graft versus host disease (GvHD) in hematological transplantations, (Lee et al., 2002; Squillaro et al., 2016; Zhao and Liu, 2016; Burnham et al., 2020) concomitant immunosuppressive treatment through inhibition of Nuclear factor of activated T cells (NFAT) signaling, which is traditionally used to prevent GvHD, (Castagna et al., 2016) can induce extracellular matrix remodelling through impaired NFAT signaling in MSCs, (Tidu et al., 2021) and thus can impact the homing and function of HSCs in the bone marrow.
Overall, there are some parallels between the decline in function of naturally aged HSPCs seen in the elderly and the post-transplant phenotype and function of HSCs in CCS. We consider that there is an urgent need for long-term follow-up studies in CCS and their healthy counterparts to directly compare HSPC status and the expression of molecular aging markers. From the resulting data, we can then determine to what extent HSPC aging might be accountable for the complications and comorbidities of CCS observed later in life.
Chronic Low-Grade Inflammation at the Crossroads of Immune Cell Dysregulation in the Elderly and Childhood Cancer Survivors
Frailty is a common feature of aging, (Lu et al., 2016; Wang et al., 2020) but it is also seen in young adult CCS (Collerton et al., 2012; Ness et al., 2013; Ness et al., 2015). This multi-system condition is closely linked with chronic inflammation (Lu et al., 2016). Interestingly, studies in the elderly, particularly those with poor physical function or frailty, have identified the presence of CLGI that is characterized by increased circulating levels of the pro-inflammatory cytokines IL-6, IFN-γ, IL-8, IL-15, CRP, and TNF-α (Bruunsgaard et al., 1999; Franceschi et al., 2000b; Zanni et al., 2003; Gangemi et al., 2005; Koelman et al., 2019). CLGI in this population, referred to as inflammaging, (Franceschi et al., 2000a) is considered a major known contributor to clinical manifestation of age-associated diseases but does not have to be the source of disease development (Fülöp et al., 2019).
The presumed sources of the inflammatory molecules contributing to inflammaging include not only immune cells but also non-immune cells, particularly adipocytes (Mau and Yung, 2018) or senescent cells from outside of the immune system (Childs et al., 2017). Moreover, cell debris, misfolded/misplaced cell molecules, referred to as damage-associated molecular patterns (DAMPs), metabolic processes, age-associated changes in the gut microbiome, and persistent viral infection can all contribute to some degree to the activation of immune cells and the ongoing chronic inflammatory response (Franceschi et al., 2017; Chen and Yung, 2019).
Several researchers have reported a partial CLGI phenotype among CCS (Table 3), characterized by high levels of IL-6, (Azanan et al., 2016; Ariffin et al., 2017; Chua et al., 2017) but not TNF- α, and accompanied by other pro-inflammatory (IL-17a, IL-2) and anti-inflammatory (IL-10) cytokines (Ariffin et al., 2017). Nevertheless, the emerging data on CLGI phenotype is still inconsistent showing also comparable levels of TNF- α, IL-6, soluble high-sensitivity C-reactive protein (hsCRP) to healthy age-matched controls (Sulicka et al., 2013). In addition, expression of the inflammatory marker soluble CRP has been reported in several CCS cohorts (Azanan et al., 2016; Ariffin et al., 2017; Chua et al., 2017). Another sign of CLGI in CCS is the presence of CMV Ig, which is also found in the elderly population, suggesting an age-related immunophenotype (Azanan et al., 2016). Furthermore, recent findings indicate that monitoring DAMPs might have prognostic or predictive value in cancer patients because their increased level signals unfavorable disease progression and survival so could serve as a potential biomarker of the level of tissue damage during/after therapy (Fucikova et al., 2015). To date, no studies on DAMPs in CCS have been published, but future work should focus on this phenomenon in CCS. After therapy, DAMPs evaluation can provide valuable information about the patients’ response to therapy and comparison of different chemotherapeutic regimens and intensity of radiotherapy between patients. In the search for early cancer development markers, further monitoring of DAMPs during regular check-ups could potentially uncover a secondary malignancy occurring frequently as late effect in CCS.
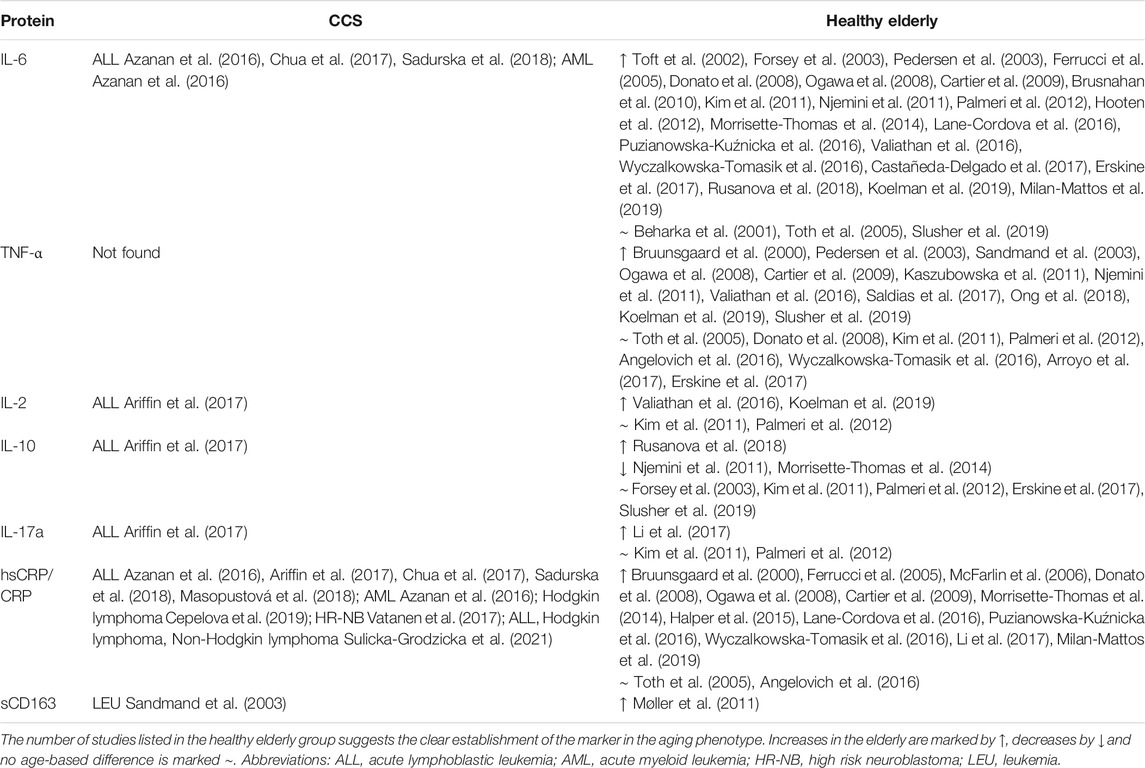
TABLE 3. Immune mediators associated with the senescent/immunosenescent phenotype in cohorts of CCS and the healthy elderly detected in serum/plasma.
While the effects of different therapies might have distinct outcomes in terms of changes to the cellular immune compartment, this convergence of immune dysregulation into CLGI might enable blood inflammatory markers to be used as a relatively simple and straightforward approach to define the levels of damage in individual CCS. While the effect of CLGI in CCS has not been specifically addressed, CLGI is likely to be linked to the risk level for various comorbidities, as evidenced by the number of comorbidities and CLGI markers in the elderly. Therefore, CLGI might present a common ground not only for individuals with chronic inflammatory disorders and elderly, but also for CCS. Thus, CCS with significant evidence of CLGI could be potentially stratified for more intense monitoring and/or early intervention strategies.
Immunosenescence-Targeted Interventions
In CCS, chemotherapy, radiotherapy, and HSC transplants have all been associated with adverse effects on the immune system through telomere shortening, phenotype/epigenetic changes, and cytokine production (Li et al., 2012; Azanan et al., 2016; Ariffin et al., 2017; Vatanen et al., 2017; Daniel et al., 2018). While therapeutics aiming to restore optimal function to the ailing immune system in CCS have yet to be developed, several groups have explored possible strategies to reduce the impact of immunosenescence in the elderly. These findings have been nicely compiled in many review articles on the topic of reversing or retarding aging, (Fulop et al., 2017; Duggal, 2018; Aiello et al., 2019; Weyh et al., 2020) and strategies, such as diet, exercise and pharmacologic therapies are proposed to remediate cancer treatment-related aging of CCS (Guida et al., 2021).
A simple and non-invasive strategy to combat immunosenescence and CLGI is to follow an active lifestyle. Exercise reduces the proportion of CD14+CD16+ monocytes and TNF-α production upon stimuli (Timmerman et al., 2008) and improves the migration of neutrophils towards IL-8 (Bartlett et al., 2016). Exercise has a positive effect on the adaptive immune system. As it increases the frequency of naive T cells and recent thymic emigrants, and lowers T-helper 17 cell polarization in those who exercise compared with less active counterparts (Duggal et al., 2018). Nevertheless, the proportion of CD28−CD57+ CD8 T cells seems unaffected. In general, it has been suggested that exercise induces a systemic anti-inflammatory response in the organism by initially IL-6 and later IL-1Ra and IL-10 expression (Petersen and Pedersen, 1985).
An interesting review by Leal et al. (2018) highlights the involvement of adipokines and myokines in the body’s response to exercise (Steensberg et al., 2003; Fischer et al., 2007; Miyamoto-Mikami et al., 2015). Nowadays, a clinical trial has been established to determine the type of exercise beneficial to the elderly (NCT04534049). The observation of the whole organism response is important, in particular, because of the comorbidities (e.g. diabetes mellitus, metabolic diseases) that are often observed in CCS (Table 1). Thus, regular exercise could also form a preventative measure.
Potential therapeutic approaches targeting the already existing immunosenescent phenotype through metabolic pathways could involve AMPK activators, mechanistic target of rapamycin (mTOR) inhibitors, and caloric restriction (Hortova-Kohoutkova et al., 2021). AMPK activators, mTOR inhibitors, and caloric restriction are all associated with increased life and/or health span (Harrison et al., 2009; Martin-Montalvo et al., 2013; Pifferi et al., 2019). Nevertheless, there are still major knowledge gaps in the crosstalk between AMPK and mTOR related to senescence and aging on the whole body and cell type/subset, as AMPK drives T-cell senescence through p38 kinase (Lanna et al., 2014) and mTOR complex 1 is involved in autophagy induction of CD8+CD28+ T cells but not CD8+CD28− T cells via the T-cell receptor (Arnold et al., 2014). Regarding the mechanisms underlying the benefits of caloric restriction, AMPK and sirtuin 1 (SIRT1), and NAD+-dependent deacetylase seem to mediate the caloric restriction health benefits while triggering autophagy (Cohen et al., 2004; Lee, 2019). Sirtuins might also have protective roles in age-related disease development (Lee, 2019). For example, resveratrol, a SIRT1 activator, has anti-inflammatory effects, and can suppress TLR signaling, reduce pro-inflammatory gene expression, (Malaguarnera, 2019) and decrease age-related changes of CD4 and CD8 T lymphocytes in aged mice (Wong et al., 2011). Although caloric restriction has proven to have beneficial effects on immune cell phenotypes distribution, it may conversely increase susceptibility to infection as has been shown in aged mice (Gardner, 2005; Goldberg et al., 2015). Thus, balancing a proper diet-related strategies or interventions in the AMPK-mTOR pathways have to be in concordance with the organismal health. Inappropriate diet in individuals with obesity and/or metabolic syndrome may increase a risk of infection (Hortova-Kohoutkova et al., 2021).
Another important anti-aging strategy aims to rejuvenate the thymus in elderly (Thomas et al., 2020). The data available on thymus regeneration are so far limited to animal models; although, increased serum levels of IL-7, a thymoprotective cytokine, has been described in trained elderly cyclists (Duggal et al., 2018). Data from an early study showed that IL-7 treatment in the form of a fusion protein restored thymic cellularity and architecture, (Henson et al., 2005) producing additional de novo T cells and increasing thymic output. Another thymic regeneration strategy involves reprogramming fibroblasts with forkhead box N1 (FOXN1) to form thymic epithelial cells and create a functional thymic stroma, (Bredenkamp et al., 2014a) or gene therapy in the form of inducible FOXN1 expression (Bredenkamp et al., 2014b). A combination of the AMPK activator (metformin) and other active substances with the focus on immunosenescence and epigenetic markers in elderly participants is currently used in an ongoing phase 2 clinical trial on thymus regeneration (NCT04375657).
A promising therapeutic approach centers on gut microbiota manipulation. Loss of microbiota diversity is associated with aging and has also been observed in CCS (Table 1). Probiotic supplements are already widely used by cancer patients with questionnaire-based positive results (Ciernikova et al., 2017; Nazir et al., 2018). Further studies on the long-term use of these supplements that evaluate the progression of comorbidities and immunosenescence are needed. Studies on the gradual change of microbiome diversity with aging (Xu et al., 2019) and in CCS (Chua et al., 2017) can serve to optimize probiotic composition.
Some strategies to overcome immunosenescence, namely exercise and probiotic supplements, can be relatively smoothly implemented in CCS post-therapy regimens, and studies on their quality of life and immunosenescence markers are much needed. These strategies can prove to be helpful rather than harmful by off-target effects in both groups, CCS and elderly. Moreover, implementation of exercise in young cancer patients or CCS could have many additional benefits including a motivation to meet with their peers, retrieve emotional and social stability (Gallicchio et al., 2008; Effinger et al., 2019) and thus overall higher quality of life. Unlike CCS, the elderly might have many physical limitations already unable to perform exercise such as longer-distance walking and thus performing exercise presents a rather preventative strategy for exercise-habituated aging individuals. The approved metabolic manipulation strategies can be used in CCS as well as in elderly when advised by and closely examined its suitability to the overall health status by their general practitioners/specialists. Unlike some above-mentioned strategies, thymus regeneration strategies are still in the process of development and thus not approved yet. Nevertheless, this strategy might be more beneficial to the elderly rather than CCS depending on the rate of thymic involution and thymic output. Lastly, so far unapproved strategies based on interfering with metabolic and signaling pathways have to be carefully studied in mouse models and in clinical trials because of possible off-target effects.
Childhood Cancer Survivors Risk Stratification Strategies
Specialised life-long follow-up care programmes for CCS are gradually being established across the world’s healthcare systems (Jacobs and Shulman, 2017; van Kalsbeek et al., 2021). In order to provide personalised, effective and also cost-effective surveillance of late effects, evidence-based strategies and models are sought to stratify CCS by risk of individual late effects. However, current risk-stratification schemes (Wallace et al., 2001; Edgar et al., 2013; Frobisher et al., 2017) are largely based on intensity of treatment, especially cumulative doses of chemotherapy and type and intensity of radiotherapy, and do not suffice to explain many variations in individual responses to treatment.
Given the CLGI is the condition contributing to aetilogy of many late effects, and concurrently is associated with immunosenescence, we suppose that accelerating aging phenotype of CCS might be accompanied by immunosenescence. Understanding the relationship between age-related immunosenescence and immune features of CCS may allow the identification of new markers. Combination of conventional factors (e.g cardiovascular – hypertension, diabetes, dyslipidemia) (Armstrong et al., 2013; Chow et al., 2014) and newly identified markers may improve currently existing risk stratification models. Such a classification of CCS into groups can eventually become the subjects of different prevention regimens/programs. Based on the reviewed literature, several candidate markers could be promising tools to screen CCS cohorts: 1) analysis of serum/plasma for major markers of the senescent phenotype (TNF-α, IL-6, hsCRP, IL-10, IL-2, IL-17A and sCD163), 2) analysis of cellular senescence in the subsets of peripheral blood cells (T cells, NK cells, and monocytes), 3) senescence markers (telomere length, p16INK4a expression, β-galactosidase, epigenetic changes (T cells and other peripheral blood mononuclear cells)) and 4) presence of health complications (Figure 3). Detection of these markers in CCS cohorts is likely to correlate with, and may even predict, the risk of developing serious typical age-related polymorbidities and frailty and would be a useful first step towards a more detailed understanding of the mechanistic link between comorbidities in CCS, immune phenotypes, and premature aging.
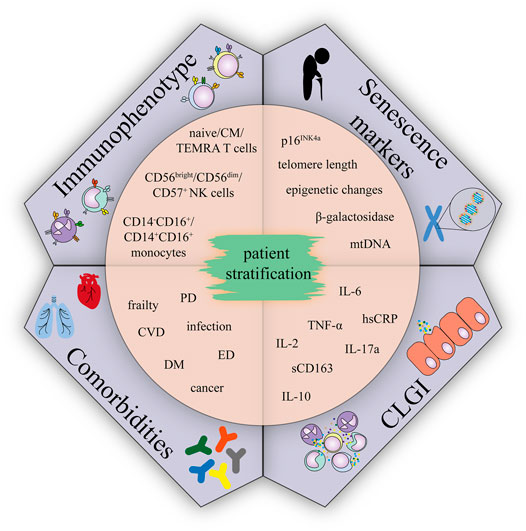
FIGURE 3. Immunophenotype, comorbidities, CLGI, and senescence markers suggested for CCS patient stratification.; Abbreviations: CM, central memory; TEMRA, terminally differentiated effector memory T cells re-expressing CD45RA; CVD, cardiovascular disease; DM, diabetes mellitus; ED, endocrinal disease; PD, pulmonary disease.
One notable effect of cancer therapies, including chemotherapy, (Li et al., 2012) radiotherapy, and bone marrow transplantation, (Schröder et al., 2001) is the disruption of telomere homeostasis. Furthermore, telomere attrition is promoted by an inflammatory environment (Jose et al., 2017) and has been described as among the first systemic hallmarks of aging in the elderly, (Woo et al., 2014) accelerated aging in chronic disorders, (Zhao et al., 2013; Haycock et al., 2014) and aging in CCS (Ness et al., 2018). Significantly shorter leukocyte telomere length has been reported among CCS exposed to various cancer treatment in comparison to non-cancer control (Song et al., 2020) and has been associated with prevalence of chronic health conditions in CCS. Interestingly, favourable health behaviours have been associated with longer leukocyte telomere length of younger CCS. Song et al. (2020) suggests telomere length as a promising aging biomarker which may be involved in strategies for health promotion and disease prevention in CCS particularly with regard to aging-related chronic health conditions.
Here, we hypothesize that the crosstalk of telomere control mechanisms and chronic inflammatory disorders is another crucial factor in the development of accelerated senescence in CCS. The fact that telomere shortening is induced by anticancer therapy, and at the same time clearly correlates as an additional risk for further development of cancer makes telomere length assessment a strong candidate for stratification of CCS at different risks of further adverse effects.
Several systemic markers correlate with the aging process (Herranz and Gil, 2018; Wiley and Campisi, 2016) (Table 4). The markers observed in peripheral blood cells in CCS include apart from telomere shortening also expression of p16INK4a and epigenetic changes. Moreover, p16INK4a expression in T cells correlates with intensity of chemotherapy and frailty in the CCS, and thus cellular senescence has been proposed to be associated with premature aging in CCS (Smitherman et al., 2020). Another established aging marker accessible from plasma, circulating cell-free mitochondrial DNA, has not yet been studied in CCS and neither has been β-galactosidase, a well-known marker for senescence (Dimri et al., 1995). Interestingly, β-galactosidase has been reported in only one study recently in peripheral blood cells (terminally differentiated CD8+ T cells specifically) of healthy elderly, (Martínez-Zamudio et al., 2021) and a study by Spazzafumo et al. (2017) reports increased β-galactosidase activity in plasma of elders (Spazzafumo et al., 2017). Nevertheless, none of those parameters has ultimate informative value without the context of many other parameters (Casella et al., 2019).
In summary, further analysis of candidate markers shared between elderly and CCS might help to develop a risk-stratification profiles for identification of high-risk (intense monitoring and early intervention) and low risk (reduced monitoring) subpopulation groups. The appropriate patient stratification would enable increased screening and pro-active early clinical management of these patients.
Future Perspectives
The parallels between immune senescence in the elderly and immune alterations seen in CCS are, in some cases, quite notable; however, the definition of altered immune states in CCS is incomplete. The specific effects of different cancer treatments on the young immune system are yet to be fully understood, and long-term follow-up studies are overall lacking. Moreover, another hurdle is that the data available for CCS immune cell phenotype and CLGI consist almost exclusively of leukemia survivors, while the comorbidities cover multiple cancer types—leukemias, lymphomas, brain and nervous system tumors etc. The question is, whether the studies were done on leukemia survivors on purpose due to the highest incidence reaching up to 25% of all pediatric neoplasms, (Howlader et al., 2020) and thus achieving a phenotype assessment in a relatively homogeneous group of CCS, or the immune senescence and CLGI occur predominantly in this type of pediatric cancer due to its specific therapeutic regimen including antracyclines and alkylating agents (in 73% of CCS), and radiotherapy (in 52% of CCS) (Azanan et al., 2016; Ariffin et al., 2017). We recently showed that high-risk neuroblastoma CCS exhibited transient signs of an immunosenescent-like phenotype, but this was resolved after 5 years, (Lázničková et al., 2020) illustrating the dynamic nature of immune recovery from cancer treatment and the need for larger longitudinal studies across tumor types. Although more than two-thirds of CCS experience notable ill health in the medium term, one-third do not. As yet, it is unclear whether the immune systems of those patients in better health are more like those of healthy age-matched peers or whether other factors are responsible for the emergence of typical age-related conditions in these young adults. Genetic factors, including those for metabolic diseases, that predispose to obesity, hypertension or diabetes mellitus have not provided sufficient predictive value in CCS, presumably due to low patient numbers in genetic studies and lack of control cohorts (Wilson et al., 2015; Clemens et al., 2018). So far, only one study showed association of leptin receptor-encoding gene polymorphism and obesity in irradiated female CCS (Ross et al., 2004).
Another possible explanation for the differences in the immune features between CCS and healthy peers is that immune alterations may pre-dated treatment, or even the cancer itself. Therefore, future research should be directed to investigate mechanisms behind these alterations resulting in identification of immune parameters allowing prognosis prediction. One such predictive pre-treatment immune cell characteristic has been already attributed to the neutrophil-to-lymphocyte ratio in pediatric patients with solid brain tumors who have elevated neutrophils and decreased lymphocyte counts compared to children with unrelated diagnoses prior to surgery (Yalon et al., 2019). These data remain to be validated for other pediatric cancers; however, data has been collected for various adult cancers (Howard et al., 2019). Nevertheless, it remains important to understand the present state of the immune system status in CCS due to the high number of CCS across the globe and the tight association of the immune system with the development of comorbidities, including possible relapses or secondary cancers.
In summary, we consider that the late adverse effects in CCS are similar to the immunosenescence-related age-associated morbidities observed in the elderly. Our belief is that immunosenescent phenotyping could provide crucial information for patients struggling with the therapy-induced morbidities that occur in CCS. This link is especially relevant considering the growing research emphasis on ways to ameliorate immunosenescence in the elderly. If relevant parallels exist between these two patient groups, then similar strategies could markedly benefit CCS. The increasing numbers of CCS are an undisputable testament to one of the biggest successes of oncological research in previous decades. The challenge moving forward is to ensure that cancer cures are not necessarily accompanied by a future of chronic conditions with the potential to limit both quantity and quality of life.
Author Contributions
PL prepared the figures and tables, and wrote the manuscript. KB prepared the tables and wrote the manuscript. TK advised clinical research interpretations and critically reviewed the manuscript. JF conceptualized, wrote and critically reviewed the manuscript. All authors contributed to manuscript revision, read, and approved the submitted version.
Funding
The work has been funded by European Social Fund and European Regional Development Fund—MAGNET (Grant/Award Number: CZ.02.1.01/0.0/0.0/15_003/0000492) and ENOCH (Grant/Award Number: CZ.02.1.01/0.0/0.0/16_019/0000868) and by the Ministry of Health of the Czech Republic, DRO (Institute of Hematology and Blood Transfusion—UHKT, 00023736).
Conflict of Interest
The authors declare that the research was conducted in the absence of any commercial or financial relationships that could be construed as a potential conflict of interest.
Acknowledgments
The authors would like to thank Lucy Robinson and Jessica Tamanini from Insight Editing London for editing and critical review of the manuscript.
Abbreviations
ABC, Age-associated B cell; AMPK, AMP-activated protein kinase; CCS, Childhood cancer survivor; CLGI, Chronic low-grade inflammation; CMV, Cytomegalovirus; DAMPs, Damage-associated molecular patterns; DC, Dendritic cell; FOXN1, Forkhead box N1; hsCRP, High-sensitivity C-reactive protein; HSC, Hematopoietic stem cell; HSPC, Hematopoietic stem and progenitor cell; KLRG-1, Killer cell lectin-like receptor subfamily G member 1; LM, Late/exhausted memory B cell; NK, Natural killer cell; mDCs, Myeloid dendritic cells; mTOR, Mechanistic target of rapamycin; pDCs, Plasmacytoid dendritic cells; ROS, Reactive oxygen species; SASP, Senescence-associated secretory phenotype; SIRT1, Sirtuin 1; TLR, Toll-like receptor.
References
Agrawal, A., Agrawal, S., and Gupta, S. (2017). Role of Dendritic Cells in Inflammation and Loss of Tolerance in the Elderly. Front. Immunol. 8, 896. doi:10.3389/fimmu.2017.00896
Agrawal, A., Agrawal, S., Cao, J.-N., Su, H., Osann, K., and Gupta, S. (2007). Altered Innate Immune Functioning of Dendritic Cells in Elderly Humans: A Role of Phosphoinositide 3-Kinase-Signaling Pathway. J. Immunol. 178 (11), 6912–6922. doi:10.4049/jimmunol.178.11.6912
Agrawal, S., Ganguly, S., Tran, A., Sundaram, P., and Agrawal, A. (2016). Retinoic Acid Treated Human Dendritic Cells Induce T Regulatory Cells via the Expression of CD141 and GARP Which Is Impaired with Age. Aging 8 (6), 1223–1235. doi:10.18632/aging.100973
Aiello, A., Farzaneh, F., Candore, G., Caruso, C., Davinelli, S., Gambino, C. M., et al. (2019). Immunosenescence and its Hallmarks: How to Oppose Aging Strategically? A Review of Potential Options for Therapeutic Intervention. Front. Immunol. 10, 2247. doi:10.3389/fimmu.2019.02247
Akbar, A. N., Henson, S. M., and Lanna, A. (2016). Senescence of T Lymphocytes: Implications for Enhancing Human Immunity. Trends Immunol. 37 (12), 866–876. doi:10.1016/j.it.2016.09.002
Alessio, N., Aprile, D., Squillaro, T., Di Bernardo, G., Finicelli, M., Melone, M. A., et al. (2019). The Senescence-Associated Secretory Phenotype (SASP) from Mesenchymal Stromal Cells Impairs Growth of Immortalized Prostate Cells but has No Effect on Metastatic Prostatic Cancer Cells. Aging 11 (15), 5817–5828. doi:10.18632/aging.102172
Allsopp, R. C., Cheshier, S., and Weissman, I. L. (2001). Telomere Shortening Accompanies Increased Cell Cycle Activity during Serial Transplantation of Hematopoietic Stem Cells. J. Exp. Med. 193 (8), 917–924. doi:10.1084/jem.193.8.917
Angelovich, T. A., Shi, M. D. Y., Zhou, J., Maisa, A., Hearps, A. C., and Jaworowski, A. (2016). Ex Vivo foam Cell Formation is Enhanced in Monocytes from Older Individuals by Both Extrinsic and Intrinsic Mechanisms. Exp. Gerontol. 80, 17–26. doi:10.1016/j.exger.2016.04.006
Ariffin, H., Azanan, M. S., Abd Ghafar, S. S., Oh, L., Lau, K. H., Thirunavakarasu, T., et al. (2017). Young Adult Survivors of Childhood Acute Lymphoblastic Leukemia Show Evidence of Chronic Inflammation and Cellular Aging. Cancer 123 (21), 4207–4214. doi:10.1002/cncr.30857
Armstrong, G. T., Kawashima, T., Leisenring, W., Stratton, K., Stovall, M., Hudson, M. M., et al. (2014). Aging and Risk of Severe, Disabling, Life-Threatening, and Fatal Events in the Childhood Cancer Survivor Study. J. Clin. Oncol. 32 (12), 1218–1227. doi:10.1200/jco.2013.51.1055
Armstrong, G. T., Oeffinger, K. C., Chen, Y., Kawashima, T., Yasui, Y., Leisenring, W., et al. (2013). Modifiable Risk Factors and Major Cardiac Events Among Adult Survivors of Childhood Cancer. J. Clin. Oncol. 31 (29), 3673–3680. doi:10.1200/jco.2013.49.3205
Armstrong, G. T., Stovall, M., and Robison, L. L. (2010). Long-term Effects of Radiation Exposure Among Adult Survivors of Childhood Cancer: Results from the Childhood Cancer Survivor Study. Radiat. Res. 174 (6), 840–850. doi:10.1667/rr1903.1
Arnold, C. R., Pritz, T., Brunner, S., Knabb, C., Salvenmoser, W., Holzwarth, B., et al. (2014). T Cell Receptor-Mediated Activation Is a Potent Inducer of Macroautophagy in Human CD8+CD28+ T Cells but Not in CD8+CD28− T Cells. Exp. Gerontol. 54, 75–83. doi:10.1016/j.exger.2014.01.018
Arroyo, E., Wells, A. J., Gordon, J. A., Varanoske, A. N., Gepner, Y., Coker, N. A., et al. (2017). Tumor Necrosis Factor-Alpha and Soluble TNF-Alpha Receptor Responses in Young vs. Middle-Aged Males Following Eccentric Exercise. Exp. Gerontol. 100, 28–35. doi:10.1016/j.exger.2017.10.012
Azanan, M. S., Abdullah, N. K., Chua, L. L., Lum, S. H., Abdul Ghafar, S. S., Kamarulzaman, A., et al. (2016). Immunity in Young Adult Survivors of Childhood Leukemia is Similar to the Elderly Rather Than Age-Matched Controls: Role of Cytomegalovirus. Eur. J. Immunol. 46 (7), 1715–1726. doi:10.1002/eji.201646356
Baerlocher, G. M., Rovó, A., Müller, A., Matthey, S., Stern, M., Halter, J., et al. (2009). Cellular Senescence of white Blood Cells in Very Long-Term Survivors After Allogeneic Hematopoietic Stem Cell Transplantation: The Role of Chronic Graft-Versus-Host Disease and Female Donor Sex. Blood 114 (1), 219–222. doi:10.1182/blood-2009-03-209833
Bartlett, D. B., Fox, O., McNulty, C. L., Greenwood, H. L., Murphy, L., Sapey, E., et al. (2016). Habitual Physical Activity Is Associated with the Maintenance of Neutrophil Migratory Dynamics in Healthy Older Adults. Brain Behav. Immun. 56, 12–20. doi:10.1016/j.bbi.2016.02.024
Bates, J. E., Howell, R. M., Liu, Q., Yasui, Y., Mulrooney, D. A., Dhakal, S., et al. (2019). Therapy-Related Cardiac Risk in Childhood Cancer Survivors: An Analysis of the Childhood Cancer Survivor Study. J. Clin. Oncol. 37 (13), 1090–1101. doi:10.1200/jco.18.01764
Beerman, I., Bock, C., Garrison, B. S., Smith, Z. D., Gu, H., Meissner, A., et al. (2013). Proliferation-Dependent Alterations of the DNA Methylation Landscape Underlie Hematopoietic Stem Cell Aging. Cell Stem Cell 12 (4), 413–425. doi:10.1016/j.stem.2013.01.017
Beharka, A. A., Meydani, M., Wu, D., Leka, L. S., Meydani, A., and Meydani, S. N. (2001). Interleukin-6 Production Does Not Increase with Age. J. Gerontol. Ser. A: Biol. Sci. Med. Sci. 56 (2), B81–B88. doi:10.1093/gerona/56.2.b81
Bell, J. T., Tsai, P. C., Yang, T. P., Pidsley, R., Nisbet, J., Glass, D., et al. (2012). Epigenome-Wide Scans Identify Differentially Methylated Regions for Age and Age-Related Phenotypes in a Healthy Ageing Population. PLoS Genet. 8 (4), e1002629. doi:10.1371/journal.pgen.1002629
Bhakta, N., Liu, Q., Ness, K. K., Baassiri, M., Eissa, H., Yeo, F., et al. (2017). The Cumulative burden of Surviving Childhood Cancer: an Initial Report from the St Jude Lifetime Cohort Study (SJLIFE). Lancet 390 (10112), 2569–2582. doi:10.1016/s0140-6736(17)31610-0
Bischoff, C., Graakjaer, J., Petersen, H. C., Hjelmborg, J. v. B., Vaupel, J. W., Bohr, V., et al. (2005). The Heritability of Telomere Length Among the Elderly and Oldest-Old. Twin Res. Hum. Genet. 8 (5), 433–439. doi:10.1375/twin.8.5.433
Blanco, E., Pérez-Andrés, M., Arriba-Méndez, S., Contreras-Sanfeliciano, T., Criado, I., Pelak, O., et al. (2018). Age-Associated Distribution of Normal B-Cell and Plasma Cell Subsets in Peripheral Blood. J. Allergy Clin. Immunol. 141 (6), 2208–2219. doi:10.1016/j.jaci.2018.02.017
Bollati, V., Schwartz, J., Wright, R., Litonjua, A., Tarantini, L., Suh, H., et al. (2009). Decline in Genomic DNA Methylation through Aging in a Cohort of Elderly Subjects. Mech. Ageing Dev. 130 (4), 234–239. doi:10.1016/j.mad.2008.12.003
Boucher, N., Dufeu-Duchesne, T., Vicaut, E., Farge, D., Effros, R. B., and Schächter, F. (1998). CD28 Expression in T Cell Aging and Human Longevity. Exp. Gerontol. 33 (3), 267–282. doi:10.1016/s0531-5565(97)00132-0
Bredenkamp, N., Nowell, C. S., and Blackburn, C. C. (2014b). Regeneration of the Aged Thymus by a Single Transcription Factor. Development 141 (8), 1627–1637. doi:10.1242/dev.103614
Bredenkamp, N., Ulyanchenko, S., O’Neill, K. E., Manley, N. R., Vaidya, H. J., and Blackburn, C. C. (2014a). An Organized and Functional Thymus Generated from FOXN1-Reprogrammed Fibroblasts. Nat. Cell Biol 16 (9), 902–908. doi:10.1038/ncb3023
Brenchley, J. M., Karandikar, N. J., Betts, M. R., Ambrozak, D. R., Hill, B. J., Crotty, L. E., et al. (2003). Expression of CD57 Defines Replicative Senescence and Antigen-Induced Apoptotic Death of CD8+ T Cells. Blood 101 (7), 2711–2720. doi:10.1182/blood-2002-07-2103
Brignardello, E., Felicetti, F., Castiglione, A., Chiabotto, P., Corrias, A., Fagioli, F., et al. (2013). Endocrine Health Conditions in Adult Survivors of Childhood Cancer: the Need for Specialized Adult-Focused Follow-Up Clinics. Eur. J. Endocrinol. 168 (3), 465–472. doi:10.1530/eje-12-1043
Brubaker, A. L., Rendon, J. L., Ramirez, L., Choudhry, M. A., and Kovacs, E. J. (2013). Reduced Neutrophil Chemotaxis and Infiltration Contributes to Delayed Resolution of Cutaneous Wound Infection with Advanced Age. J. Immunol. 190 (4), 1746–1757. doi:10.4049/jimmunol.1201213
Bruni, E., Cazzetta, V., Donadon, M., Cimino, M., Torzilli, G., Spata, G., et al. (2019). Chemotherapy Accelerates Immune-Senescence and Functional Impairments of Vdelta2(pos) T Cells in Elderly Patients Affected by Liver Metastatic Colorectal Cancer. J. Immunother. Cancer 7 (1), 347. doi:10.1186/s40425-019-0825-4
Brusnahan, S. K., McGuire, T. R., Jackson, J. D., Lane, J. T., Garvin, K. L., O'Kane, B. J., et al. (2010). Human Blood and Marrow Side Population Stem Cell and Stro-1 Positive Bone Marrow Stromal Cell Numbers Decline with Age, With an Increase in Quality of Surviving Stem Cells: Correlation with Cytokines. Mech. Ageing Dev. 131 (11-12), 718–722. doi:10.1016/j.mad.2010.10.002
Bruunsgaard, H., Andersen-Ranberg, K., Jeune, B., Pedersen, A. N., Skinhoj, P., and Pedersen, B. K. (1999). A High Plasma Concentration of TNF- Is Associated With Dementia in Centenarians. J. Gerontol. Ser. A: Biol. Sci. Med. Sci. 54 (7), M357–M364. doi:10.1093/gerona/54.7.m357
Bruunsgaard, H., Skinhøj, P., Pedersen, A. N., Schroll, M., and Pedersen, B. K. (2000). Ageing, Tumour Necrosis Factor-Alpha (TNF-α) and Atherosclerosis. Clin. Exp. Immunol. 121 (2), 255–260. doi:10.1046/j.1365-2249.2000.01281.x
Burnham, A. J., Daley-Bauer, L. P., and Horwitz, E. M. (2020). Mesenchymal Stromal Cells in Hematopoietic Cell Transplantation. Blood Adv. 4 (22), 5877–5887. doi:10.1182/bloodadvances.2020002646
Butcher, S. K., Chahal, H., Nayak, L., Sinclair, A., Henriquez, N. V., Sapey, E., et al. (2001). Senescence in Innate Immune Responses: Reduced Neutrophil Phagocytic Capacity and CD16 Expression in Elderly Humans. J. Leukoc. Biol. 70 (6), 881–886. doi:10.1189/jlb.70.6.881
Callender, L. A., Carroll, E. C., Beal, R. W. J., Chambers, E. S., Nourshargh, S., Akbar, A. N., et al. (2018). Human CD8(+) EMRA T Cells Display a Senescence-Associated Secretory Phenotype Regulated by P38 MAPK. Aging Cell 17 (1), e12675. doi:10.1111/acel.12675
Cartier, A., Côté, M., Lemieux, I., Pérusse, L., Tremblay, A., Bouchard, C., et al. (2009). Age-Related Differences in Inflammatory Markers in Men: Contribution of Visceral Adiposity. Metabolism 58 (10), 1452–1458. doi:10.1016/j.metabol.2009.04.025
Casella, G., Munk, R., Kim, K. M., Piao, Y., De, S., Abdelmohsen, K., et al. (2019). Transcriptome Signature of Cellular Senescence. Nucleic Acids Res. 47 (14), 7294–7305. doi:10.1093/nar/gkz555
Castagna, L., Bramanti, S., Furst, S., Giordano, L., Sarina, B., Crocchiolo, R., et al. (2016). Tacrolimus Compared With Cyclosporine A After Haploidentical T-Cell Replete Transplantation With Post-Infusion Cyclophosphamide. Bone Marrow Transpl. 51 (3), 462–465. doi:10.1038/bmt.2015.289
Castañeda-Delgado, J. E., Frausto-Lujan, I., González-Curiel, I., Montoya-Rosales, A., Serrano, C. J., Torres-Juarez, F., et al. (2017). Differences in Cytokine Production during Aging and its Relationship with Antimicrobial Peptides Production. Immunol. Invest. 46 (1), 48–58. doi:10.1080/08820139.2016.1212873
Cepelova, M., Kruseova, J., Luks, A., Capek, V., Cepela, P., Potockova, J., et al. (2019). Accelerated Atherosclerosis, Hyperlipoproteinemia and Insulin Resistance in Long-Term Survivors of Hodgkin Lymphoma During Childhood and Adolescence. Neoplasma 66 (6), 978–987. doi:10.4149/neo_2019_190115n45
Chen, G., and Yung, R. (2019). Meta‐Inflammaging at the Crossroad of Geroscience. Aging Med. 2 (3), 157–161. doi:10.1002/agm2.12078
Chidrawar, S. M., Khan, N., Chan, Y. L. T., Nayak, L., and Moss, P. A. (2006). Ageing is Associated With a Decline in Peripheral Blood CD56bright NK Cells. Immun. Ageing 3, 10. doi:10.1186/1742-4933-3-10
Childs, B. G., Gluscevic, M., Baker, D. J., Laberge, R.-M., Marquess, D., Dananberg, J., et al. (2017). Senescent Cells: An Emerging Target for Diseases of Ageing. Nat. Rev. Drug Discov. 16 (10), 718–735. doi:10.1038/nrd.2017.116
Choi, D. K., Strzepka, J. T., Hunt, S. R., Tannenbaum, V. L., and Jang, I. E. (2020). Vaccination in Pediatric Cancer Survivors: Vaccination Rates, Immune Status, and Knowledge Regarding Compliance. Pediatr. Blood Cancer 67 (10), e28565. doi:10.1002/pbc.28565
Chow, E. J., Baker, K. S., Lee, S. J., Flowers, M. E. D., Cushing-Haugen, K. L., Inamoto, Y., et al. (2014). Influence of Conventional Cardiovascular Risk Factors and Lifestyle Characteristics on Cardiovascular Disease after Hematopoietic Cell Transplantation. J. Clin. Oncol. 32 (3), 191–198. doi:10.1200/jco.2013.52.6582
Chow, E. J., Simmons, J. H., Roth, C. L., Baker, K. S., Hoffmeister, P. A., Sanders, J. E., et al. (2010). Increased Cardiometabolic Traits in Pediatric Survivors of Acute Lymphoblastic Leukemia Treated With Total Body Irradiation. Biol. Blood Marrow Transplant. 16 (12), 1674–1681. doi:10.1016/j.bbmt.2010.05.016
Christensen, B. C., Houseman, E. A., Marsit, C. J., Zheng, S., Wrensch, M. R., Wiemels, J. L., et al. (2009). Aging and Environmental Exposures Alter Tissue-specific DNA Methylation Dependent Upon CpG Island Context. PLoS Genet. 5 (8), e1000602. doi:10.1371/journal.pgen.1000602
Chua, L. L., Rajasuriar, R., Azanan, M. S., Abdullah, N. K., Tang, M. S., Lee, S. C., et al. (2017). Reduced Microbial Diversity in Adult Survivors of Childhood Acute Lymphoblastic Leukemia and Microbial Associations With Increased Immune Activation. Microbiome 5 (1), 35. doi:10.1186/s40168-017-0250-1
Ciernikova, S., Mego, M., Semanova, M., Wachsmannova, L., Adamcikova, Z., Stevurkova, V., et al. (2017). Probiotic Survey in Cancer Patients Treated in the Outpatient Department in a Comprehensive Cancer Center. Integr. Cancer Ther. 16 (2), 188–195. doi:10.1177/1534735416643828
Clemens, E., van der Kooi, A. L. F., Broer, L., van Dulmen-den Broeder, E., Visscher, H., Kremer, L., et al. (2018). The Influence of Genetic Variation on Late Toxicities in Childhood Cancer Survivors: A Review. Crit. Rev. Oncol. Hematol. 126, 154–167. doi:10.1016/j.critrevonc.2018.04.001
Cohen, H. Y., Miller, C., Bitterman, K. J., Wall, N. R., Hekking, B., Kessler, B., et al. (2004). Calorie Restriction Promotes Mammalian Cell Survival by Inducing the SIRT1 Deacetylase. Science 305 (5682), 390–392. doi:10.1126/science.1099196
Collerton, J., Martin-Ruiz, C., Davies, K., Hilkens, C. M., Isaacs, J., Kolenda, C., et al. (2012). Frailty and the Role of Inflammation, Immunosenescence and Cellular Ageing in the Very Old: Cross-Sectional Findings from the Newcastle 85+ Study. Mech. Ageing Dev. 133 (6), 456–466. doi:10.1016/j.mad.2012.05.005
Cupit-Link, M. C., Kirkland, J. L., Ness, K. K., Armstrong, G. T., Tchkonia, T., LeBrasseur, N. K., et al. (2017). Biology of Premature Ageing in Survivors of Cancer. ESMO Open 2 (5), e000250. doi:10.1136/esmoopen-2017-000250
Daniel, S., Nylander, V., Ingerslev, L. R., Zhong, L., Fabre, O., Clifford, B., et al. (2018). T Cell Epigenetic Remodeling and Accelerated Epigenetic Aging Are Linked to Long-Term Immune Alterations in Childhood Cancer Survivors. Clin. Epigenetics 10 (1), 138. doi:10.1186/s13148-018-0561-5
Di Mitri, D., Azevedo, R. I., Henson, S. M., Libri, V., Riddell, N. E., Macaulay, R., et al. (2011). Reversible Senescence in Human CD4+CD45RA+CD27− Memory T Cells. J. Immunol. 187 (5), 2093–2100. doi:10.4049/jimmunol.1100978
Dimri, G. P., Lee, X., Basile, G., Acosta, M., Scott, G., Roskelley, C., et al. (1995). A Biomarker that Identifies Senescent Human Cells in Culture and in Aging Skin In Vivo. Proc. Natl. Acad. Sci. 92 (20), 9363–9367. doi:10.1073/pnas.92.20.9363
Donato, A. J., Black, A. D., Jablonski, K. L., Gano, L. B., and Seals, D. R. (2008). Aging is Associated With Greater Nuclear NFκB, Reduced IκBα, and Increased Expression of Proinflammatory Cytokines in Vascular Endothelial Cells of Healthy Humans. Aging Cell 7 (6), 805–812. doi:10.1111/j.1474-9726.2008.00438.x
Duggal, N. A., Pollock, R. D., Lazarus, N. R., Harridge, S., and Lord, J. M. (2018). Major Features of Immunesenescence, Including Reduced Thymic Output, Are Ameliorated by High Levels of Physical Activity in Adulthood. Aging Cell 17 (2). doi:10.1111/acel.12750
Duggal, N. A. (2018). Reversing the Immune Ageing Clock: Lifestyle Modifications and Pharmacological Interventions. Biogerontology 19 (6), 481–496. doi:10.1007/s10522-018-9771-7
Edgar, A. B., Duffin, K., Borthwick, S., Marciniak-Stepak, P., and Wallace, W. H. (2013). Can Intensity of Long-Term Follow-Up for Survivors of Childhood and Teenage Cancer be Determined by Therapy-Based Risk Stratification? BMJ Open 3 (8). doi:10.1136/bmjopen-2012-002451
Effinger, K. E., Stratton, K. L., Fisher, P. G., Ness, K. K., Krull, K. R., Oeffinger, K. C., et al. (2019). Long-Term Health and Social Function in Adult Survivors of Paediatric Astrocytoma: A Report from the Childhood Cancer Survivor Study. Eur. J. Cancer 106, 171–180. doi:10.1016/j.ejca.2018.10.016
Effros, R. B., Boucher, N., Porter, V., Zhu, X., Spaulding, C., Walford, R. L., et al. (1994). Decline in CD28+ T Cells in Centenarians and in Long-Term T Cell Cultures: a Possible Cause for Both In Vivo and In Vitro Immunosenescence. Exp. Gerontol. 29 (6), 601–609. doi:10.1016/0531-5565(94)90073-6
Erskine, R. M., Tomlinson, D. J., Morse, C. I., Winwood, K., Hampson, P., Lord, J. M., et al. (2017). The Individual and Combined Effects of Obesity- and Ageing-Induced Systemic Inflammation on Human Skeletal Muscle Properties. Int. J. Obes. 41 (1), 102–111. doi:10.1038/ijo.2016.151
Fagnoni, F. F., Vescovini, R., Mazzola, M., Bologna, G., Nigro, E., Lavagetto, G., et al. (1996). Expansion of Cytotoxic CD8 + CD28 − T Cells in Healthy Ageing People, Including Centenarians. Immunology 88 (4), 501–507. doi:10.1046/j.1365-2567.1996.d01-689.x
Fali, T., Fabre-Mersseman, V., Yamamoto, T., Bayard, C., Papagno, L., Fastenackels, S., et al. (2018). Elderly Human Hematopoietic Progenitor Cells Express Cellular Senescence Markers and are More Susceptible to Pyroptosis. JCI Insight 3 (13), e95319. doi:10.1172/jci.insight.95319
Farrell, T. L., McGuire, T. R., Bilek, L. D., Brusnahan, S. K., Jackson, J. D., Lane, J. T., et al. (2014). Changes in the Frequencies of Human Hematopoietic Stem and Progenitor Cells With Age and Site. Exp. Hematol. 42 (2), 146–154. doi:10.1016/j.exphem.2013.11.003
Felicetti, F., Manicone, R., Corrias, A., Manieri, C., Biasin, E., Bini, I., et al. (2011). Endocrine Late Effects After Total Body Irradiation in Patients Who Received Hematopoietic Cell Transplantation During Childhood: A Retrospective Study From a Single Institution. J. Cancer Res. Clin. Oncol. 137 (9), 1343–1348. doi:10.1007/s00432-011-1004-2
Ferrucci, L., Corsi, A., Lauretani, F., Bandinelli, S., Bartali, B., Taub, D. D., et al. (2005). The Origins of Age-Related Proinflammatory State. Blood 105 (6), 2294–2299. doi:10.1182/blood-2004-07-2599
Fischer, C. P., Berntsen, A., Perstrup, L. B., Eskildsen, P., and Pedersen, B. K. (2007). Plasma Levels of Interleukin-6 and C-Reactive Protein Are Associated With Physical Inactivity Independent of Obesity. Scand. J. Med. Sci. Sports 17 (5), 580–587. doi:10.1111/j.1600-0838.2006.00602.x
Forrester, S. J., Kikuchi, D. S., Hernandes, M. S., Xu, Q., and Griendling, K. K. (2018). Reactive Oxygen Species in Metabolic and Inflammatory Signaling. Circ. Res. 122 (6), 877–902. doi:10.1161/circresaha.117.311401
Forsey, R. J., Thompson, J. M., Ernerudh, J., Hurst, T. L., Strindhall, J., Johansson, B., et al. (2003). Plasma Cytokine Profiles in Elderly Humans. Mech. Ageing Dev. 124 (4), 487–493. doi:10.1016/s0047-6374(03)00025-3
Fowler, H., Belot, A., Ellis, L., Maringe, C., Luque-Fernandez, M. A., Njagi, E. N., et al. (2020). Comorbidity Prevalence Among Cancer Patients: A Population-Based Cohort Study of Four Cancers. BMC Cancer 20 (1), 2. doi:10.1186/s12885-019-6472-9
Franceschi, C., Bonafè, M., Valensin, S., Olivieri, F., De Luca, M., Ottaviani, E., et al. (2000a). Inflamm-aging. An Evolutionary Perspective on Immunosenescence. Ann. N. Y Acad. Sci. 908, 244–254. doi:10.1111/j.1749-6632.2000.tb06651.x
Franceschi, C., Bonafè, M., and Valensin, S. (2000b). Human Immunosenescence: The Prevailing of Innate Immunity, the Failing of Clonotypic Immunity, and the Filling of Immunological Space. Vaccine 18 (16), 1717–1720. doi:10.1016/s0264-410x(99)00513-7
Franceschi, C., Garagnani, P., Vitale, G., Capri, M., and Salvioli, S. (2017). Inflammaging and 'Garb-Aging'. Trends Endocrinol. Metab. 28 (3), 199–212. doi:10.1016/j.tem.2016.09.005
Franco, S., Ozkaynak, M. F., Sandoval, C., Tugal, O., Jayabose, S., Engelhardt, M., et al. (2003). Telomere Dynamics in Childhood Leukemia and Solid Tumors: A Follow-Up Study. Leukemia 17 (2), 401–410. doi:10.1038/sj.leu.2402815
Frasca, D., Diaz, A., Romero, M., and Blomberg, B. B. (2017). Human Peripheral Late/Exhausted Memory B Cells Express a Senescent-Associated Secretory Phenotype and Preferentially Utilize Metabolic Signaling Pathways. Exp. Gerontol. 87 (Pt A), 113–120. doi:10.1016/j.exger.2016.12.001
Frasca, D., Diaz, A., Romero, M., D'Eramo, F., and Blomberg, B. B. (2017). Aging Effects on T-Bet Expression in Human B Cell Subsets. Cell Immunol. 321, 68–73. doi:10.1016/j.cellimm.2017.04.007
Frasca, D., Ferracci, F., Diaz, A., Romero, M., Lechner, S., and Blomberg, B. B. (2016). Obesity Decreases B Cell Responses in Young and Elderly Individuals. Obesity 24 (3), 615–625. doi:10.1002/oby.21383
Fried, L. P., Tangen, C. M., Walston, J., Newman, A. B., Hirsch, C., Gottdiener, J., et al. (2001). Frailty in Older Adults: Evidence for a Phenotype. J. Gerontol. Ser. A: Biol. Sci. Med. Sci. 56 (3), M146–M157. doi:10.1093/gerona/56.3.m146
Frobisher, C., Glaser, A., Glaser, A., Levitt, G. A., Cutter, D. J., Winter, D. L., et al. (2017). Risk Stratification of Childhood Cancer Survivors Necessary for Evidence-Based Clinical Long-Term Follow-Up. Br. J. Cancer 117 (11), 1723–1731. doi:10.1038/bjc.2017.347
Fucikova, J., Moserova, I., Urbanova, L., Bezu, L., Kepp, O., Cremer, I., et al. (2015). Prognostic and Predictive Value of DAMPs and DAMP-Associated Processes in Cancer. Front. Immunol. 6, 402. doi:10.3389/fimmu.2015.00402
Fülöp, T., Larbi, A., and Witkowski, J. M. (2019). Human Inflammaging. Gerontology 65 (5), 495–504. doi:10.1159/000497375
Fulop, T., Larbi, A., Witkowski, J. M., Kotb, R., Hirokawa, K., and Pawelec, G. (2013). Immunosenescence and Cancer. Crit. Rev. Oncog 18 (6), 489–513. doi:10.1615/critrevoncog.2013010597
Fulop, T., Witkowski, J. M., Le Page, A., Fortin, C., Pawelec, G., and Larbi, A. (2017). Intracellular Signalling Pathways: Targets to Reverse Immunosenescence. Clin. Exp. Immunol. 187 (1), 35–43. doi:10.1111/cei.12836
Gallicchio, L., Kalesan, B., Hoffman, S. C., and Helzlsouer, K. J. (2008). Non-cancer Adverse Health Conditions and Perceived Health and Function Among Cancer Survivors Participating in a Community-Based Cohort Study in Washington County, Maryland. J. Cancer Surviv. 2 (1), 12–19. doi:10.1007/s11764-008-0046-1
Gangemi, S., Basile, G., Monti, D., Merendino, R. A., Pasquale, G. D., Bisignano, U., et al. (2005). Age-related Modifications in Circulating IL-15 Levels in Humans. Mediators Inflamm. 2005 (4), 245–247. doi:10.1155/mi.2005.245
Garbe, K., Bratke, K., Wagner, S., Virchow, J. C., and Lommatzsch, M. (2012). Plasmacytoid Dendritic Cells and Their Toll-Like Receptor 9 Expression Selectively Decrease With Age. Hum. Immunol. 73 (5), 493–497. doi:10.1016/j.humimm.2012.02.007
Gardner, E. M. (2005). Caloric Restriction Decreases Survival of Aged Mice in Response to Primary Influenza Infection. J. Gerontol. Ser. A: Biol. Sci. Med. Sci. 60 (6), 688–694. doi:10.1093/gerona/60.6.688
Gatta, G., Botta, L., Rossi, S., Aareleid, T., Bielska-Lasota, M., Clavel, J., et al. (2014). Childhood Cancer Survival in Europe 1999-2007: Results of EUROCARE-5-A Population-Based Study. Lancet Oncol. 15 (1), 35–47. doi:10.1016/s1470-2045(13)70548-5
Gavazzi, G., Mallaret, M. R., Couturier, P., Iffenecker, A., and Franco, A. (2002). Bloodstream Infection: Differences Between Young‐Old, Old, and Old‐Old Patients. J. Am. Geriatr. Soc. 50 (10), 1667–1673. doi:10.1046/j.1532-5415.2002.50458.x
Geenen, M. M., Cardous-Ubbink, M. C., Kremer, L. C. M., van den Bos, C., van der Pal, H. J. H., Heinen, R. C., et al. (2007). Medical Assessment of Adverse Health Outcomes in Long-Term Survivors of Childhood Cancer. JAMA 297 (24), 2705–2715. doi:10.1001/jama.297.24.2705
Genovese, G., Kähler, A. K., Handsaker, R. E., Lindberg, J., Rose, S. A., Bakhoum, S. F., et al. (2014). Clonal Hematopoiesis and Blood-Cancer Risk Inferred from Blood DNA Sequence. N. Engl. J. Med. 371 (26), 2477–2487. doi:10.1056/nejmoa1409405
Goldberg, E. L., Romero‐Aleshire, M. J., Renkema, K. R., Ventevogel, M. S., Chew, W. M., Uhrlaub, J. L., et al. (2015). Lifespan‐Extending Caloric Restriction or mTOR Inhibition Impair Adaptive Immunity of Old Mice by Distinct Mechanisms. Aging cell 14 (1), 130–138. doi:10.1111/acel.12280
Goossen, G. M., Kremer, L. C., and van de Wetering, M. D. (2013). Influenza Vaccination in Children Being Treated with Chemotherapy for Cancer. Cochrane Database Syst. Rev. 2013 (8), CD006484. doi:10.1002/14651858.CD006484.pub2
Gramatges, M. M., Liu, Q., Yasui, Y., Okcu, M. F., Neglia, J. P., Strong, L. C., et al. (2014). Telomere Content and Risk of Second Malignant Neoplasm in Survivors of Childhood Cancer: A Report From the Childhood Cancer Survivor Study. Clin. Cancer Res. 20 (4), 904–911. doi:10.1158/1078-0432.ccr-13-2076
Granot, T., Senda, T., Carpenter, D. J., Matsuoka, N., Weiner, J., Gordon, C. L., et al. (2017). Dendritic Cells Display Subset and Tissue-Specific Maturation Dynamics Over Human Life. Immunity 46 (3), 504–515. doi:10.1016/j.immuni.2017.02.019
Guida, J. L., Agurs-Collins, T., Ahles, T. A., Campisi, J., Dale, W., Demark-Wahnefried, W., et al. (2021). Strategies to Prevent or Remediate Cancer and Treatment-Related Aging. J. Natl. Cancer Inst. 113 (2), 112–122. doi:10.1093/jnci/djaa060
Haapanen, M. J., Perälä, M.-M., Salonen, M. K., Guzzardi, M. A., Iozzo, P., Kajantie, E., et al. (2018). Telomere Length and Frailty: The Helsinki Birth Cohort Study. J. Am. Med. Dir. Assoc. 19 (8), 658–662. doi:10.1016/j.jamda.2018.05.011
Halper, B., Hofmann, M., Oesen, S., Franzke, B., Stuparits, P., Vidotto, C., et al. (2015). Influence of Age and Physical Fitness on miRNA-21, TGF-β and its Receptors in Leukocytes of Healthy Women. Exerc. Immunol. Rev. 21, 154–163.
Han, J. H., Harmoney, K. M., Dokmeci, E., Torrez, J., Chavez, C. M., Cordova de Ortega, L., et al. (2018). Dynamic Re-immunization of Off-Treatment Childhood Cancer Survivors: An Implementation Feasibility Study. PLoS One 13 (2), e0191804. doi:10.1371/journal.pone.0191804
Han, J. W., Kwon, S. Y., Won, S. C., Shin, Y. J., Ko, J. H., and Lyu, C. J. (2009). Comprehensive Clinical Follow-Up of Late Effects in Childhood Cancer Survivors Shows the Need for Early and Well-Timed Intervention. Ann. Oncol. 20 (7), 1170–1177. doi:10.1093/annonc/mdn778
Hao, Y., O’Neill, P., Naradikian, M. S., Scholz, J. L., and Cancro, M. P. (2011). A B-Cell Subset Uniquely Responsive to Innate Stimuli Accumulates in Aged Mice. Blood 118 (5), 1294–1304. doi:10.1182/blood-2011-01-330530
Harrison, D. E., Strong, R., Sharp, Z. D., Nelson, J. F., Astle, C. M., Flurkey, K., et al. (2009). Rapamycin Fed Late in Life Extends Lifespan in Genetically Heterogeneous Mice. Nature 460 (7253), 392–395. doi:10.1038/nature08221
Haycock, P. C., Heydon, E. E., Kaptoge, S., Butterworth, A. S., Thompson, A., and Willeit, P. (2014). Leucocyte Telomere Length and Risk of Cardiovascular Disease: Systematic Review and Meta-Analysis. BMJ 349, g4227. doi:10.1136/bmj.g4227
Hazeldine, J., Harris, P., Chapple, I. L., Grant, M., Greenwood, H., Livesey, A., et al. (2014). Impaired Neutrophil Extracellular Trap Formation: a Novel Defect in the Innate Immune System of Aged Individuals. Aging Cell 13 (4), 690–698. doi:10.1111/acel.12222
Hearps, A. C., Martin, G. E., Angelovich, T. A., Cheng, W.-J., Maisa, A., Landay, A. L., et al. (2012). Aging is Associated With Chronic Innate Immune Activation and Dysregulation of Monocyte Phenotype and Function. Aging Cell 11 (5), 867–875. doi:10.1111/j.1474-9726.2012.00851.x
Hendriks, J., Gravestein, L. A., Tesselaar, K., van Lier, R. A. W., Schumacher, T. N. M., and Borst, J. (2000). CD27 Is Required for Generation and Long-Term Maintenance of T Cell Immunity. Nat. Immunol. 1 (5), 433–440. doi:10.1038/80877
Henson, S. M., Snelgrove, R., Hussell, T., Wells, D. J., and Aspinall, R. (2005). An IL-7 Fusion Protein that Shows Increased Thymopoietic Ability. J. Immunol. 175 (6), 4112–4118. doi:10.4049/jimmunol.175.6.4112
Herranz, N., and Gil, J. (2018). Mechanisms and Functions of Cellular Senescence. J. Clin. Invest. 128 (4), 1238–1246. doi:10.1172/jci95148
Hooten, N. N., Fitzpatrick, M., Wood, W. H., De, S., Ejiogu, N., Zhang, Y., et al. (2013). Age-Related Changes in microRNA Levels in Serum. Aging 5 (10), 725–740. doi:10.18632/aging.100603
Hortova-Kohoutkova, M., Laznickova, P., and Fric, J. (2021). How Immune-Cell Fate and Function are Determined by Metabolic Pathway Choice: The Bioenergetics Underlying the Immune Response. BioEssays 43 (2), e2000067.
Howard, R., Kanetsky, P. A., and Egan, K. M. (2019). Exploring the Prognostic Value of the Neutrophil-to-Lymphocyte Ratio in Cancer. Sci. Rep. 9 (1), 19673. doi:10.1038/s41598-019-56218-z
Howlader, N., Noone, A. M., Krapcho, M., Miller, D., Brest, A., Yu, M., et al. (2020). SEER Cancer Statistics Review. Bethesda, MD: National Cancer Institute.
Ibegbu, C. C., Xu, Y.-X., Harris, W., Maggio, D., Miller, J. D., and Kourtis, A. P. (2005). Expression of Killer Cell Lectin-like Receptor G1 on Antigen-Specific Human CD8+ T Lymphocytes during Active, Latent, and Resolved Infection and its Relation With CD57. J. Immunol. 174 (10), 6088–6094. doi:10.4049/jimmunol.174.10.6088
Itagaki, K., Kaczmarek, E., Lee, Y. T., Tang, I. T., Isal, B., Adibnia, Y., et al. (2015). Mitochondrial DNA Released by Trauma Induces Neutrophil Extracellular Traps. PLoS One 10 (3), e0120549. doi:10.1371/journal.pone.0120549
Jacobs, L. A., and Shulman, L. N. (2017). Follow-Up Care of Cancer Survivors: Challenges and Solutions. Lancet Oncol. 18 (1), e19–e29. doi:10.1016/s1470-2045(16)30386-2
Jaiswal, S., Fontanillas, P., Flannick, J., Manning, A., Grauman, P. V., Mar, B. G., et al. (2014). Age-Related Clonal Hematopoiesis Associated With Adverse Outcomes. N. Engl. J. Med. 371 (26), 2488–2498. doi:10.1056/nejmoa1408617
Jaiswal, S., Natarajan, P., Silver, A. J., Gibson, C. J., Bick, A. G., Shvartz, E., et al. (2017). Clonal Hematopoiesis and Risk of Atherosclerotic Cardiovascular Disease. N. Engl. J. Med. 377 (2), 111–121. doi:10.1056/nejmoa1701719
Jang, Y.-Y., and Sharkis, S. J. (2007). A Low Level of Reactive Oxygen Species Selects for Primitive Hematopoietic Stem Cells that May Reside in the Low-Oxygenic Niche. Blood 110 (8), 3056–3063. doi:10.1182/blood-2007-05-087759
Jing, Y., Shaheen, E., Drake, R. R., Chen, N., Gravenstein, S., and Deng, Y. (2009). Aging is Associated with a Numerical and Functional Decline in Plasmacytoid Dendritic Cells, Whereas Myeloid Dendritic Cells are Relatively Unaltered in Human Peripheral Blood. Hum. Immunol. 70 (10), 777–784. doi:10.1016/j.humimm.2009.07.005
Johnson, N. D., and Conneely, K. N. (2019). The Role of DNA Methylation and Hydroxymethylation in Immunosenescence. Ageing Res. Rev. 51, 11–23. doi:10.1016/j.arr.2019.01.011
Jose, S. S., Bendickova, K., Kepak, T., Krenova, Z., and Fric, J. (2017). Chronic Inflammation in Immune Aging: Role of Pattern Recognition Receptor Crosstalk With the Telomere Complex? Front. Immunol. 8, 1078. doi:10.3389/fimmu.2017.01078
Jose, S. S., Tidu, F., Burilova, P., Kepak, T., Bendickova, K., and Fric, J. (2018). The Telomerase Complex Directly Controls Hematopoietic Stem Cell Differentiation and Senescence in an Induced Pluripotent Stem Cell Model of Telomeropathy. Front. Genet. 9, 345. doi:10.3389/fgene.2018.00345
Jylhävä, J., Nevalainen, T., Marttila, S., Jylhä, M., Hervonen, A., and Hurme, M. (2013). Characterization of the Role of Distinct Plasma Cell-free DNA Species in Age-Associated Inflammation and Frailty. Aging Cell 12 (3), 388–397. doi:10.1111/acel.12058
Kallemeijn, M. J., Boots, A. M. H., van der Klift, M. Y., Brouwer, E., Abdulahad, W. H., Verhaar, J. A. N., et al. (2017). Ageing and Latent CMV Infection Impact on Maturation, Differentiation and Exhaustion Profiles of T-Cell Receptor Gammadelta T-Cells. Sci. Rep. 7 (1), 5509. doi:10.1038/s41598-017-05849-1
Kao, T. W., Chen, W. L., Han, D. S., Huang, Y. H., Chen, C. L., and Yang, W. S. (2016). Examining How p16(INK4a) Expression Levels are Linked to Handgrip Strength in the Elderly. Sci. Rep. 6, 31905. doi:10.1038/srep31905
Kared, H., Martelli, S., Ng, T. P., Pender, S. L. F., and Larbi, A. (2016). CD57 in Human Natural Killer Cells and T-Lymphocytes. Cancer Immunol. Immunother. 65 (4), 441–452. doi:10.1007/s00262-016-1803-z
Kaszubowska, L., Kaczor, J. J., Hak, L., Dettlaff-Pokora, A., Szarynska, M., and Kmiec, Z. (2011). Sensitivity of Natural Killer Cells to Activation in the Process of Ageing is Related to the Oxidative and Inflammatory Status of the Elderly. J. Physiol. Pharmacol. 62 (1), 101–109.
Kim, H., Kim, H.-S., Youn, J.-C., Shin, E.-C., and Park, S. (2011). Serum Cytokine Profiles in Healthy Young and Elderly Population Assessed Using Multiplexed Bead-Based Immunoassays. J. Transl. Med. 9, 113. doi:10.1186/1479-5876-9-113
Koch, S., Larbi, A., Derhovanessian, E., Özcelik, D., Naumova, E., and Pawelec, G. (2008). Multiparameter Flow Cytometric Analysis of CD4 and CD8 T Cell Subsets in Young and Old People. Immun. Ageing 5, 6. doi:10.1186/1742-4933-5-6
Koch, S., Larbi, A., Ozcelik, D., Solana, R., Gouttefangeas, C., Attig, S., et al. (2007). Cytomegalovirus Infection: a Driving Force in Human T Cell Immunosenescence. Ann. N. Y. Acad. Sci. 1114, 23–35. doi:10.1196/annals.1396.043
Koelman, L., Pivovarova-Ramich, O., Pfeiffer, A. F. H., Grune, T., and Aleksandrova, K. (2019). Cytokines for Evaluation of Chronic Inflammatory Status in Ageing Research: Reliability and Phenotypic Characterisation. Immun. Ageing 16, 11. doi:10.1186/s12979-019-0151-1
Kovaiou, R. D., Weiskirchner, I., Keller, M., Pfister, G., Cioca, D. P., and Grubeck-Loebenstein, B. (2005). Age-Related Differences in Phenotype and Function of CD4+ T Cells Are Due to a Phenotypic Shift from Naive to Memory Effector CD4+ T Cells. Int. Immunol. 17 (10), 1359–1366. doi:10.1093/intimm/dxh314
Krater, M., Jacobi, A., Otto, O., Tietze, S., Muller, K., Poitz, D. M., et al. (2017). Bone Marrow Niche-Mimetics Modulate HSPC Function via Integrin Signaling. Sci. Rep. 7 (1), 2549. doi:10.1038/s41598-017-02352-5
Kuo, C. L., Pilling, L. C., Kuchel, G. A., Ferrucci, L., and Melzer, D. (2019). Telomere Length and Aging-Related Outcomes in Humans: A Mendelian Randomization Study in 261,000 Older Participants. Aging Cell 18 (6), e13017. doi:10.1111/acel.13017
Kuranda, K., Vargaftig, J., de la Rochere, P., Dosquet, C., Charron, D., Bardin, F., et al. (2011). Age-related Changes in Human Hematopoietic Stem/Progenitor Cells. Aging Cell 10 (3), 542–546. doi:10.1111/j.1474-9726.2011.00675.x
Lane-Cordova, A. D., Ranadive, S. M., Kappus, R. M., Cook, M. D., Phillips, S. A., Woods, J. A., et al. (2016). Aging, not Age-Associated Inflammation, Determines Blood Pressure and Endothelial Responses to Acute Inflammation. J. Hypertens. 34 (12), 2402–2409. doi:10.1097/hjh.0000000000001103
Lanna, A., Henson, S. M., Escors, D., and Akbar, A. N. (2014). The Kinase P38 Activated by the Metabolic Regulator AMPK and Scaffold TAB1 Drives the Senescence of Human T Cells. Nat. Immunol. 15 (10), 965–972. doi:10.1038/ni.2981
Larbi, A., and Fulop, T. (2014). From “Truly Naïve” to “Exhausted Senescent” T Cells: When Markers Predict Functionality. Cytometry 85 (1), 25–35. doi:10.1002/cyto.a.22351
Lázničková, P., Kepák, T., Hortová-Kohoutková, M., Horváth, L., Sheardová, K., Marciniak, R., et al. (2020). Childhood Survivors of High-Risk Neuroblastoma Show Signs of Immune Recovery and not Immunosenescence. Eur. J. Immunol. 50 (12), 2092–2094. doi:10.1002/eji.202048541
Le Garff-Tavernier, M., Béziat, V., Decocq, J., Siguret, V., Gandjbakhch, F., Pautas, E., et al. (2010). Human NK Cells Display Major Phenotypic and Functional Changes Over the Life Span. Aging Cell 9 (4), 527–535. doi:10.1111/j.1474-9726.2010.00584.x
Leal, L. G., Lopes, M. A., and Batista, M. L. (2018). Physical Exercise-Induced Myokines and Muscle-Adipose Tissue Crosstalk: A Review of Current Knowledge and the Implications for Health and Metabolic Diseases. Front. Physiol. 9, 1307. doi:10.3389/fphys.2018.01307
Lee, I. H. (2019). Mechanisms and Disease Implications of Sirtuin-Mediated Autophagic Regulation. Exp. Mol. Med. 51 (9), 1–11. doi:10.1038/s12276-019-0302-7
Lee, J.-J., Nam, C.-E., Cho, S.-H., Park, K.-S., Chung, I.-J., and Kim, H.-J. (2003). Telomere Length Shortening in Non-Hodgkin’s Lymphoma Patients Undergoing Chemotherapy. Ann. Hematol. 82 (8), 492–495. doi:10.1007/s00277-003-0691-4
Lee, S. T., Jang, J. H., Cheong, J.-W., Kim, J. S., Maemg, H.-Y., Hahn, J. S., et al. (2002). Treatment of High-Risk Acute Myelogenous Leukaemia by Myeloablative Chemoradiotherapy Followed by Co-infusion of T Cell-Depleted Haematopoietic Stem Cells and Culture-Expanded Marrow Mesenchymal Stem Cells from a Related Donor with One Fully Mismatched Human Leucocyte Antigen Haplotype. Br. J. Haematol. 118 (4), 1128–1131. doi:10.1046/j.1365-2141.2002.03767.x
Lee, Y.-h., Kim, S. R., Han, D. H., Yu, H. T., Han, Y. D., Kim, J. H., et al. (2019). Senescent T Cells Predict the Development of Hyperglycemia in Humans. Diabetes 68 (1), 156–162. doi:10.2337/db17-1218
Li, P., Hou, M., Lou, F., Björkholm, M., and Xu, D. (2012). Telomere Dysfunction Induced by Chemotherapeutic Agents and Radiation in Normal Human Cells. Int. J. Biochem. Cell Biol. 44 (9), 1531–1540. doi:10.1016/j.biocel.2012.06.020
Li, Q., Ding, S., Wang, Y. M., Xu, X., Shen, Z., Fu, R., et al. (2017). Age-Associated Alteration in Th17 Cell Response is Related to Endothelial Cell Senescence and Atherosclerotic Cerebral Infarction. Am. J. Transl. Res. 9 (11), 5160–5168.
Linehan, E., and Fitzgerald, D. (2015). Ageing and the Immune System: Focus on Macrophages. Eur. J. Microbiol. Immunol. 5 (1), 14–24. doi:10.1556/eujmi-d-14-00035
Liu, W., Liu, Y., Yang, Y., Ou, W., Chen, X., Huang, B., et al. (2018). Metabolic Biomarkers of Aging and Aging-Related Diseases in Chinese Middle-Aged and Elderly Men. J. Nutr. Health Aging 22 (10), 1189–1197. doi:10.1007/s12603-018-1062-0
Liu, Y., Sanoff, H. K., Cho, H., Burd, C. E., Torrice, C., Ibrahim, J. G., et al. (2009). Expression of p16INK4ain Peripheral Blood T-Cells is a Biomarker of Human Aging. Aging Cell 8 (4), 439–448. doi:10.1111/j.1474-9726.2009.00489.x
López-Otín, C., Blasco, M. A., Partridge, L., Serrano, M., and Kroemer, G. (2013). The Hallmarks of Aging. Cell 153 (6), 1194–1217. doi:10.1016/j.cell.2013.05.039
Lorenzi, M. F., Xie, L., Rogers, P. C., Pritchard, S., Goddard, K., and McBride, M. L. (2011). Hospital-related Morbidity Among Childhood Cancer Survivors in British Columbia, Canada: Report of the Childhood, Adolescent, Young Adult Cancer Survivors (CAYACS) Program. Int. J. Cancer 128 (7), 1624–1631. doi:10.1002/ijc.25751
Lu, Y., Tan, C. T. Y., Nyunt, M. S. Z., Mok, E. W. H., Camous, X., Kared, H., et al. (2016). Inflammatory and Immune Markers Associated with Physical Frailty Syndrome: Findings from Singapore Longitudinal Aging Studies. Oncotarget 7 (20), 28783–28795. doi:10.18632/oncotarget.8939
Ma, S., Wang, C., Mao, X., and Hao, Y. (2019). B Cell Dysfunction Associated With Aging and Autoimmune Diseases. Front. Immunol. 10, 318. doi:10.3389/fimmu.2019.00318
Malaguarnera, L. (2019). Influence of Resveratrol on the Immune Response. Nutrients 11 (5). doi:10.3390/nu11050946
Marengoni, A., von Strauss, E., Rizzuto, D., Winblad, B., and Fratiglioni, L. (2009). The Impact of Chronic Multimorbidity and Disability on Functional Decline and Survival in Elderly Persons. A Community-Based, Longitudinal Study. J. Intern. Med. 265 (2), 288–295. doi:10.1111/j.1365-2796.2008.02017.x
Martin-Montalvo, A., Mercken, E. M., Mitchell, S. J., Palacios, H. H., Mote, P. L., Scheibye-Knudsen, M., et al. (2013). Metformin Improves Healthspan and Lifespan in Mice. Nat. Commun. 4, 2192. doi:10.1038/ncomms3192
Martínez-Zamudio, R. I., Dewald, H. K., Vasilopoulos, T., Gittens-Williams, L., Fitzgerald-Bocarsly, P., and Herbig, U. (2021). Senescence-associated β-Galactosidase Reveals the Abundance of Senescent CD8+ T Cells in Aging Humans. Aging Cell 20 (5), e13344. doi:10.1111/acel.13344
Masopustová, A., Jehlička, P., Huml, M., Votava, T., Trefil, L., Kreslová, M., et al. (2018). Plethysmographic and Biochemical Markers in the Diagnosis of Endothelial Dysfunction in Pediatric Acute Lymphoblastic Leukemia Survivors - New Applications. Physiol. Res. 67 (6), 903–909. doi:10.33549/physiolres.933754
Mau, T., and Yung, R. (2018). Adipose Tissue Inflammation in Aging. Exp. Gerontol. 105, 27–31. doi:10.1016/j.exger.2017.10.014
McFarlin, B. K., Flynn, M. G., Campbell, W. W., Craig, B. A., Robinson, J. P., Stewart, L. K., et al. (2006). Physical Activity Status, but not Age, Influences Inflammatory Biomarkers and Toll-Like Receptor 4. Gerontol. A. Biol. Sci. Med. Sci. 61 (4), 388–393. doi:10.1093/gerona/61.4.388
Mertens, A. C., Liu, Q., Neglia, J. P., Wasilewski, K., Leisenring, W., Armstrong, G. T., et al. (2008). Cause-Specific Late Mortality Among 5-Year Survivors of Childhood Cancer: the Childhood Cancer Survivor Study. JNCI J. Natl. Cancer Inst. 100 (19), 1368–1379. doi:10.1093/jnci/djn310
Metcalf, T. U., Wilkinson, P. A., Cameron, M. J., Ghneim, K., Chiang, C., Wertheimer, A. M., et al. (2017). Human Monocyte Subsets are Transcriptionally and Functionally Altered in Aging in Response to Pattern Recognition Receptor Agonists. J. Immunol. 199 (4), 1405–1417. doi:10.4049/jimmunol.1700148
Milan-Mattos, J. C., Anibal, F. F., Perseguini, N. M., Minatel, V., Rehder-Santos, P., Castro, C. A., et al. (2019). Effects of Natural Aging and Gender on Pro-Inflammatory Markers. Braz. J. Med. Biol. Res. 52 (9), e8392. doi:10.1590/1414-431x20198392
Milkovic, L., Cipak Gasparovic, A., Cindric, M., Mouthuy, P. A., and Zarkovic, N. (2019). Short Overview of ROS as Cell Function Regulators and Their Implications in Therapy Concepts. Cells 8 (8), 793. doi:10.3390/cells8080793
Miyamoto-Mikami, E., Sato, K., Kurihara, T., Hasegawa, N., Fujie, S., Fujita, S., et al. (2015). Endurance Training-Induced Increase in Circulating Irisin Levels is Associated With Reduction of Abdominal Visceral Fat in Middle-Aged and Older Adults. PLoS One 10 (3), e0120354. doi:10.1371/journal.pone.0120354
Møller, H. J., Frikke-Schmidt, R., Moestrup, S. K., Nordestgaard, B. G., and Tybjærg-Hansen, A. (2011). Serum Soluble CD163 Predicts Risk of Type 2 Diabetes in the General Population. Clin. Chem. 57 (2), 291–297. doi:10.1373/clinchem.2010.154724
Montecino-Rodriguez, E., Berent-Maoz, B., and Dorshkind, K. (2013). Causes, Consequences, and Reversal of Immune System Aging. J. Clin. Invest. 123 (3), 958–965. doi:10.1172/jci64096
Montiel Rojas, D., Nilsson, A., Ponsot, E., Brummer, R. J., Fairweather-Tait, S., Jennings, A., et al. (2018). Short Telomere Length is Related to Limitations in Physical Function in Elderly European Adults. Front. Physiol. 9, 1110. doi:10.3389/fphys.2018.01110
Morrisette-Thomas, V., Cohen, A. A., Fülöp, T., Riesco, É., Legault, V., Li, Q., et al. (2014). Inflamm-Aging Does Not Simply Reflect Increases in Pro-Inflammatory Markers. Mech. Ageing Dev. 139, 49–57. doi:10.1016/j.mad.2014.06.005
Morrison, S. J., Prowse, K. R., Ho, P., and Weissman, I. L. (1996). Telomerase Activity in Hematopoietic Cells Is Associated With Self-Renewal Potential. Immunity 5 (3), 207–216. doi:10.1016/s1074-7613(00)80316-7
Mostoufi-Moab, S., Seidel, K., Leisenring, W. M., Armstrong, G. T., Oeffinger, K. C., Stovall, M., et al. (2016). Endocrine Abnormalities in Aging Survivors of Childhood Cancer: A Report From the Childhood Cancer Survivor Study. J. Clin. Oncol. 34 (27), 3240–3247. doi:10.1200/jco.2016.66.6545
Munz, F., Lopez Perez, R., Trinh, T., Sisombath, S., Weber, K. J., Wuchter, P., et al. (2018). Human Mesenchymal Stem Cells Lose Their Functional Properties after Paclitaxel Treatment. Sci. Rep. 8 (1), 312. doi:10.1038/s41598-017-18862-1
Murray, M. A., and Chotirmall, S. H. (2015). The Impact of Immunosenescence on Pulmonary Disease. Mediators Inflamm. 2015, 692546. doi:10.1155/2015/692546
Nazir, Y., Hussain, S. A., Abdul Hamid, A., and Song, Y. (2018). Probiotics and Their Potential Preventive and Therapeutic Role for Cancer, High Serum Cholesterol, and Allergic and HIV Diseases. Biomed. Research Int. 2018, 3428437. doi:10.1155/2018/3428437
Ness, K. K., Armstrong, G. T., Kundu, M., Wilson, C. L., Tchkonia, T., and Kirkland, J. L. (2015). Frailty in Childhood Cancer Survivors. Cancer 121 (10), 1540–1547. doi:10.1002/cncr.29211
Ness, K. K., Kirkland, J. L., Gramatges, M. M., Wang, Z., Kundu, M., McCastlain, K., et al. (2018). Premature Physiologic Aging as a Paradigm for Understanding Increased Risk of Adverse Health Across the Lifespan of Survivors of Childhood Cancer. J. Clin. Oncol. 36 (21), 2206–2215. doi:10.1200/jco.2017.76.7467
Ness, K. K., Krull, K. R., Jones, K. E., Mulrooney, D. A., Armstrong, G. T., Green, D. M., et al. (2013). Physiologic Frailty as a Sign of Accelerated Aging Among Adult Survivors of Childhood Cancer: a Report from the St Jude Lifetime Cohort Study. J. Clin. Oncol. 31 (36), 4496–4503. doi:10.1200/jco.2013.52.2268
Njemini, R., Bautmans, I., Onyema, O. O., Van Puyvelde, K., Demanet, C., and Mets, T. (2011). Circulating Heat Shock Protein 70 in Health, Aging and Disease. BMC Immunol. 12, 24. doi:10.1186/1471-2172-12-24
Oeffinger, K. C., Mertens, A. C., Sklar, C. A., Kawashima, T., Hudson, M. M., Meadows, A. T., et al. (2006). Chronic Health Conditions in Adult Survivors of Childhood Cancer. N. Engl. J. Med. 355 (15), 1572–1582. doi:10.1056/nejmsa060185
Ogawa, K., Suzuki, K., Okutsu, M., Yamazaki, K., and Shinkai, S. (2008). The Association of Elevated Reactive Oxygen Species Levels from Neutrophils With Low-Grade Inflammation in the Elderly. Immun. Ageing 5, 13. doi:10.1186/1742-4933-5-13
Olsson, J., Wikby, A., Johansson, B., Löfgren, S., Nilsson, B. O., and Ferguson, F. G. (2000). Age-related Change in Peripheral Blood T-Lymphocyte Subpopulations and Cytomegalovirus Infection in the Very Old: the Swedish Longitudinal OCTO Immune Study. Mech. Ageing Dev. 121 (1–3), 187–201. doi:10.1016/s0047-6374(00)00210-4
Ong, S. M., Hadadi, E., Dang, T. M., Yeap, W. H., Tan, C. T., Ng, T. P., et al. (2018). The Pro-Inflammatory Phenotype of the Human Non-Classical Monocyte Subset is Attributed to Senescence. Cell Death Dis. 9 (3), 266. doi:10.1038/s41419-018-0327-1
Ortmann, W., and Kolaczkowska, E. (2018). Age is the Work of Art? Impact of Neutrophil and Organism Age on Neutrophil Extracellular Trap Formation. Cell Tissue Res. 371 (3), 473–488. doi:10.1007/s00441-017-2751-4
Ostan, R., Bucci, L., Capri, M., Salvioli, S., Scurti, M., Pini, E., et al. (2008). Immunosenescence and Immunogenetics of Human Longevity. Neuroimmunomodulation 15 (4-6), 224–240. doi:10.1159/000156466
Palmeri, M., Misiano, G., Malaguarnera, M., Forte, G. I., Vaccarino, L., Milano, S., et al. (2012). Cytokine Serum Profile in a Group of Sicilian Nonagenarians. J. Immunoassay Immunochem. 33 (1), 82–90. doi:10.1080/15321819.2011.601781
Panda, A., Qian, F., Mohanty, S., van Duin, D., Newman, F. K., Zhang, L., et al. (2010). Age-Associated Decrease in TLR Function in Primary Human Dendritic Cells Predicts Influenza Vaccine Response. J. Immunol. 184 (5), 2518–2527. doi:10.4049/jimmunol.0901022
Pang, W. W., Price, E. A., Sahoo, D., Beerman, I., Maloney, W. J., Rossi, D. J., et al. (2011). Human Bone Marrow Hematopoietic Stem Cells Are Increased in Frequency and Myeloid-Biased With Age. Proc. Natl. Acad. Sci. 108 (50), 20012–20017. doi:10.1073/pnas.1116110108
Pansarasa, O., Pistono, C., Davin, A., Bordoni, M., Mimmi, M. C., Guaita, A., et al. (2019). Altered Immune System in Frailty: Genetics and Diet May Influence Inflammation. Ageing Res. Rev. 54, 100935. doi:10.1016/j.arr.2019.100935
Parish, S. T., Wu, J. E., and Effros, R. B. (2010). Sustained CD28 Expression Delays Multiple Features of Replicative Senescence in Human CD8 T Lymphocytes. J. Clin. Immunol. 30 (6), 798–805. doi:10.1007/s10875-010-9449-7
Partridge, L., Deelen, J., and Slagboom, P. E. (2018). Facing up to the Global Challenges of Ageing. Nature 561 (7721), 45–56. doi:10.1038/s41586-018-0457-8
Patel, S. R., Ortin, M., Cohen, B. J., Borrow, R., Irving, D., Sheldon, J., et al. (2007). Revaccination of Children After Completion of Standard Chemotherapy for Acute Leukemia. Clin. Infect. Dis. 44 (5), 635–642. doi:10.1086/511636
Pawelec, G. (1999). Immunosenescence: Impact in the Young as Well as the Old? Mech. Ageing Dev. 108 (1), 1–7. doi:10.1016/s0047-6374(99)00010-x
Pedersen, M., Bruunsgaard, H., Weis, N., Hendel, H. W., Andreassen, B. U., Eldrup, E., et al. (2003). Circulating Levels of TNF-Alpha and IL-6-relation to Truncal Fat Mass and Muscle Mass in Healthy Elderly Individuals and in Patients with Type-2 Diabetes. Mech. Ageing Develop. 124 (4), 495–502. doi:10.1016/s0047-6374(03)00027-7
Pérez-Cabezas, B., Naranjo-Gómez, M., Fernández, M. A., Grífols, J. R., Pujol-Borrell, R., and Borràs, F. E. (2007). Reduced Numbers of Plasmacytoid Dendritic Cells in Aged Blood Donors. Exp. Gerontol. 42 (10), 1033–1038. doi:10.1016/j.exger.2007.05.010
Perkins, J. L., Chen, Y., Harris, A., Diller, L., Stovall, M., Armstrong, G. T., et al. (2014). Infections Among Long-Term Survivors of Childhood and Adolescent Cancer: A Report From the Childhood Cancer Survivor Study. Cancer 120 (16), 2514–2521. doi:10.1002/cncr.28763
Perkins, J. L., Harris, A., and Pozos, T. C. (2017). Immune Dysfunction After Completion of Childhood Leukemia Therapy. J. Pediatr. Hematol. Oncol. 39 (1), 1–5. doi:10.1097/mph.0000000000000697
Petersen, A. M., and Pedersen, B. K. (1985). The Anti-Inflammatory Effect of Exercise. J. Appl. Physiol. 98 (4), 1154–1162. doi:10.1152/japplphysiol.00164.2004
Pifferi, F., Terrien, J., Perret, M., Epelbaum, J., Blanc, S., Picq, J. L., et al. (2019). Promoting Healthspan and Lifespan with Caloric Restriction in Primates. Commun. Biol. 2, 107. doi:10.1038/s42003-019-0348-z
Pinti, M., Appay, V., Campisi, J., Frasca, D., Fülöp, T., Sauce, D., et al. (2016). Aging of the Immune System: Focus on Inflammation and Vaccination. Eur. J. Immunol. 46 (10), 2286–2301. doi:10.1002/eji.201546178
Pinti, M., Cevenini, E., Nasi, M., De Biasi, S., Salvioli, S., Monti, D., et al. (2014). Circulating Mitochondrial DNA Increases with Age and is a Familiar Trait: Implications for “Inflamm-Aging”. Eur. J. Immunol. 44 (5), 1552–1562. doi:10.1002/eji.201343921
Puzianowska-Kuźnicka, M., Owczarz, M., Wieczorowska-Tobis, K., Nadrowski, P., Chudek, J., Slusarczyk, P., et al. (2016). Interleukin-6 and C-Reactive Protein, Successful Aging, and Mortality: The PolSenior Study. Immun. Ageing 13, 21. doi:10.1186/s12979-016-0076-x
Qian, F., Wang, X., Zhang, L., Lin, A., Zhao, H., Fikrig, E., et al. (2011). Impaired Interferon Signaling in Dendritic Cells from Older Donors Infected In Vitro With West Nile Virus. J. Infect. Dis. 203 (10), 1415–1424. doi:10.1093/infdis/jir048
Qin, L., Jing, X., Qiu, Z., Cao, W., Jiao, Y., Routy, J.-P., et al. (2016). Aging of Immune System: Immune Signature from Peripheral Blood Lymphocyte Subsets in 1068 Healthy Adults. Aging 8 (5), 848–859. doi:10.18632/aging.100894
Reulen, R. C., Winter, D. L., Frobisher, C., Lancashire, E. R., Stiller, C. A., Jenney, M. E., et al. (2010). Long-Term Cause-Specific Mortality Among Survivors of Childhood Cancer. JAMA 304 (2), 172–179. doi:10.1001/jama.2010.923
Ross, J. A., Oeffinger, K. C., Davies, S. M., Mertens, A. C., Langer, E. K., Kiffmeyer, W. R., et al. (2004). Genetic Variation in the Leptin Receptor Gene and Obesity in Survivors of Childhood Acute Lymphoblastic Leukemia: A Report from the Childhood Cancer Survivor Study. J. Clin. Oncol. 22 (17), 3558–3562. doi:10.1200/jco.2004.11.152
Rubtsov, A. V., Rubtsova, K., Fischer, A., Meehan, R. T., Gillis, J. Z., Kappler, J. W., et al. (2011). Toll-like Receptor 7 (TLR7)-Driven Accumulation of a Novel CD11c+ B-Cell Population Is Important for the Development of Autoimmunity. Blood 118 (5), 1305–1315. doi:10.1182/blood-2011-01-331462
Rundberg Nilsson, A., Soneji, S., Adolfsson, S., Bryder, D., and Pronk, C. J. (2016). Human and Murine Hematopoietic Stem Cell Aging is Associated with Functional Impairments and Intrinsic Megakaryocytic/Erythroid Bias. PLoS One 11 (7), e0158369. doi:10.1371/journal.pone.0158369
Rusanova, I., Diaz-Casado, M. E., Fernandez-Ortiz, M., Aranda-Martinez, P., Guerra-Librero, A., Garcia-Garcia, F. J., et al. (2018). Analysis of Plasma MicroRNAs as Predictors and Biomarkers of Aging and Frailty in Humans. Oxid Med. Cell Longev. 2018, 7671850. doi:10.1155/2018/7671850
Sadurska, E., Zaucha-Prażmo, A., Brodzisz, A., Kowalczyk, J., and Ben-Skowronek, I. (2018). Premature Atherosclerosis After Treatment for Acute Lymphoblastic Leukemia in Childhood. Ann. Agric. Environ. Med. 25 (1), 71–76. doi:10.5604/12321966.1230680
Saldias, M. P., Fernandez, C., Morgan, A., Diaz, C., Morales, D., Jana, F., et al. (2017). Aged Blood Factors Decrease Cellular Responses Associated with Delayed Gingival Wound Repair. PLoS One 12 (9), e0184189. doi:10.1371/journal.pone.0184189
Sanders, J. L., Cauley, J. A., Boudreau, R. M., Zmuda, J. M., Strotmeyer, E. S., Opresko, P. L., et al. (2009). Leukocyte Telomere Length is Not Associated With BMD, Osteoporosis, or Fracture in Older Adults: Results From the Health, Aging and Body Composition Study. J. Bone Miner. Res. 24 (9), 1531–1536. doi:10.1359/jbmr.090318
Sandmand, M., Bruunsgaard, H., Kemp, K., Andersen-Ranberg, K., Schroll, M., and Jeune, B. (2003). High Circulating Levels of Tumor Necrosis Factor-α in Centenarians Are Not Associated with Increased Production in T Lymphocytes. Gerontology 49 (3), 155–160. doi:10.1159/000069174
Sapey, E., Greenwood, H., Walton, G., Mann, E., Love, A., Aaronson, N., et al. (2014). Phosphoinositide 3-kinase Inhibition Restores Neutrophil Accuracy in the Elderly: Toward Targeted Treatments for Immunosenescence. Blood 123 (2), 239–248. doi:10.1182/blood-2013-08-519520
Sauce, D., Dong, Y., Campillo-Gimenez, L., Casulli, S., Bayard, C., Autran, B., et al. (2017). Reduced Oxidative Burst by Primed Neutrophils in the Elderly Individuals is Associated With Increased Levels of the CD16bright/CD62Ldim Immunosuppressive Subset. Gerona 72 (2), 163–172. doi:10.1093/gerona/glw062
Saule, P., Trauet, J., Dutriez, V., Lekeux, V., Dessaint, J.-P., and Labalette, M. (2006). Accumulation of Memory T Cells From Childhood to Old Age: Central and Effector Memory Cells in CD4+ versus Effector Memory and Terminally Differentiated Memory Cells in CD8+ Compartment. Mech. Ageing Develop. 127 (3), 274–281. doi:10.1016/j.mad.2005.11.001
Schröder, C. P., Wisman, G. B., de Jong, S., van der Graaf, W. T., Ruiters, M. H., Mulder, N. H., et al. (2001). Telomere Length in Breast Cancer Patients Before and After Chemotherapy With or Without Stem Cell Transplantation. Br. J. Cancer 84 (10), 1348–1353. doi:10.1054/bjoc.2001.1803
Seidler, S., Zimmermann, H. W., Bartneck, M., Trautwein, C., and Tacke, F. (2010). Age-dependent Alterations of Monocyte Subsets and Monocyte-Related Chemokine Pathways in Healthy Adults. BMC Immunol. 11, 30. doi:10.1186/1471-2172-11-30
Shaw, A. C., Goldstein, D. R., and Montgomery, R. R. (2013). Age-Dependent Dysregulation of Innate Immunity. Nat. Rev. Immunol. 13 (12), 875–887. doi:10.1038/nri3547
Shaw, A. C., Joshi, S., Greenwood, H., Panda, A., and Lord, J. M. (2010). Aging of the Innate Immune System. Curr. Opin. Immunol. 22 (4), 507–513. doi:10.1016/j.coi.2010.05.003
Shetty, A. K., and Winter, M. A. (2012). Immunization of Children Receiving Immunosuppressive Therapy for Cancer or Hematopoietic Stem Cell Transplantation. Ochsner J. 12 (3), 228–243.
Simell, B., Vuorela, A., Ekström, N., Palmu, A., Reunanen, A., Meri, S., et al. (2011). Aging Reduces the Functionality of Anti-Pneumococcal Antibodies and the Killing of Streptococcus pneumoniae by Neutrophil Phagocytosis. Vaccine 29 (10), 1929–1934. doi:10.1016/j.vaccine.2010.12.121
Simnica, D., Akyuz, N., Schliffke, S., Mohme, M., Wenserski, L. V., Mährle, T., et al. (2019). T Cell Receptor Next-Generation Sequencing Reveals Cancer-Associated Repertoire Metrics and Reconstitution after Chemotherapy in Patients with Hematological and Solid Tumors. Oncoimmunology 8 (11), e1644110. doi:10.1080/2162402x.2019.1644110
Slusher, A. L., Zuniga, T. M., and Acevedo, E. O. (2019). Inflamm-Aging Is Associated with Lower Plasma PTX3 Concentrations and an Impaired Capacity of PBMCs to Express hTERT Following LPS Stimulation. Mediators Inflamm. 2019, 2324193. doi:10.1155/2019/2324193
Smitherman, A. B., Wood, W. A., Mitin, N., Ayer Miller, V. L., Deal, A. M., Davis, I. J., et al. (2020). Accelerated Aging Among Childhood, Adolescent, and Young Adult Cancer Survivors is Evidenced by Increased Expression of p16 INK4a and Frailty. Cancer 126 (22), 4975–4983. doi:10.1002/cncr.33112
Solana, R., Tarazona, R., Gayoso, I., Lesur, O., Dupuis, G., and Fulop, T. (2012). Innate Immunosenescence: Effect of Aging on Cells and Receptors of the Innate Immune System in Humans. Semin. Immunol. 24 (5), 331–341. doi:10.1016/j.smim.2012.04.008
Song, N., Li, Z., Qin, N., Howell, C. R., Wilson, C. L., Easton, J., et al. (2020). Shortened Leukocyte Telomere Length Associates with an Increased Prevalence of Chronic Health Conditions Among Survivors of Childhood Cancer: A Report from the St. Jude Lifetime Cohort. Clin. Cancer Res. 26 (10), 2362–2371. doi:10.1158/1078-0432.ccr-19-2503
Song, Z., von Figura, G., Liu, Y., Kraus, J. M., Torrice, C., Dillon, P., et al. (2010). Lifestyle Impacts on the Aging-Associated Expression of Biomarkers of DNA Damage and Telomere Dysfunction in Human Blood. Aging Cell 9 (4), 607–615. doi:10.1111/j.1474-9726.2010.00583.x
Spazzafumo, L., Mensà, E., Matacchione, G., Galeazzi, T., Zampini, L., Recchioni, R., et al. (2017). Age-Related Modulation of Plasmatic Beta-Galactosidase Activity in Healthy Subjects and in Patients Affected by T2DM. Oncotarget 8 (55), 93338–93348. doi:10.18632/oncotarget.21848
Splunter, M. V., Perdijk, O., Fick-Brinkhof, H., Floris-Vollenbroek, E. G., Meijer, B., Brugman, S., et al. (2019). Plasmacytoid Dendritic Cell and Myeloid Dendritic Cell Function in Ageing: A Comparison between Elderly and Young Adult Women. PLoS One 14 (12), e0225825. doi:10.1371/journal.pone.0225825
Spyridopoulos, I., Hoffmann, J., Aicher, A., Brümmendorf, T. H., Doerr, H. W., Zeiher, A. M., et al. (2009). Accelerated Telomere Shortening in Leukocyte Subpopulations of Patients With Coronary Heart Disease. Circulation 120 (14), 1364–1372. doi:10.1161/circulationaha.109.854299
Squillaro, T., Peluso, G., and Galderisi, U. (2016). Clinical Trials With Mesenchymal Stem Cells: An Update. Cell Transpl. 25 (5), 829–848. doi:10.3727/096368915x689622
Sridharan, A., Esposo, M., Kaushal, K., Tay, J., Osann, K., Agrawal, S., et al. (2011). Age-Associated Impaired Plasmacytoid Dendritic Cell Functions Lead to Decreased CD4 and CD8 T Cell Immunity. Age 33 (3), 363–376. doi:10.1007/s11357-010-9191-3
Steensberg, A., Fischer, C. P., Keller, C., Møller, K., and Pedersen, B. K. (2003). IL-6 Enhances Plasma IL-1ra, IL-10, and Cortisol in Humans. Am. J. Physiol. Endocrinol. Metab. 285 (2), E433–E437. doi:10.1152/ajpendo.00074.2003
Steliarova-Foucher, E., Colombet, M., Ries, L. A. G., Moreno, F., Dolya, A., Bray, F., et al. (2017). International Incidence of Childhood Cancer, 2001-10: A Population-Based Registry Study. Lancet Oncol. 18 (6), 719–731. doi:10.1016/S1470-2045(17)30186-9
Suarez-Álvarez, B., Rodríguez, R. M., Schlangen, K., Raneros, A. B., Márquez-Kisinousky, L., Fernández, A. F., et al. (2017). Phenotypic Characteristics of Aged CD4+ CD28null T Lymphocytes are Determined by Changes in the Whole-Genome DNA Methylation Pattern. Aging Cell 16 (2), 293–303. doi:10.1111/acel.12552
Sulicka, J., Surdacki, A., Mikołajczyk, T., Strach, M., Gryglewska, B., Ćwiklińska, M., et al. (2013). Elevated Markers of Inflammation and Endothelial Activation and Increased Counts of Intermediate Monocytes in Adult Survivors of Childhood Acute Lymphoblastic Leukemia. Immunobiology 218 (5), 810–816. doi:10.1016/j.imbio.2012.09.003
Sulicka-Grodzicka, J., Surdacki, A., Seweryn, M., Mikołajczyk, T., Rewiuk, K., Guzik, T., et al. (2021). Low-grade Chronic Inflammation and Immune Alterations in Childhood and Adolescent Cancer Survivors: A Contribution to Accelerated Aging? Cancer Med. 10 (5), 1772–1782. doi:10.1002/cam4.3788
Talukdar, S., Oh, D. Y., Bandyopadhyay, G., Li, D., Xu, J., McNelis, J., et al. (2012). Neutrophils Mediate Insulin Resistance in Mice Fed a High-Fat Diet through Secreted Elastase. Nat. Med. 18 (9), 1407–1412. doi:10.1038/nm.2885
Teo, Y. V., Capri, M., Morsiani, C., Pizza, G., Faria, A. M. C., Franceschi, C., et al. (2019). Cell-Free DNA as a Biomarker of Aging. Aging Cell 18 (1), e12890. doi:10.1111/acel.12890
Thomas, R., Wang, W., and Su, D. M. (2020). Contributions of Age-Related Thymic Involution to Immunosenescence and Inflammaging. Immun. Ageing 17, 2. doi:10.1186/s12979-020-0173-8
Tidu, F., De Zuani, M., Jose, S. S., Bendíčková, K., Kubala, L., Caruso, F., et al. (2021). NFAT Signaling in Human Mesenchymal Stromal Cells Affects Extracellular Matrix Remodeling and Antifungal Immune Responses. iScience 24 (6), 102683. doi:10.1016/j.isci.2021.102683
Timmerman, K. L., Flynn, M. G., Coen, P. M., Markofski, M. M., and Pence, B. D. (2008). Exercise Training-Induced Lowering of Inflammatory (CD14+CD16+) Monocytes: A Role in the Anti-inflammatory Influence of Exercise? J. Leukoc. Biol. 84 (5), 1271–1278. doi:10.1189/jlb.0408244
Todhunter, M. E., Sayaman, R. W., Miyano, M., and LaBarge, M. A. (2018). Tissue Aging: The Integration of Collective and Variant Responses of Cells to Entropic Forces over Time. Curr. Opin. Cel Biol. 54, 121–129. doi:10.1016/j.ceb.2018.05.016
Toft, A. D., Jensen, L. B., Bruunsgaard, H., Ibfelt, T., Halkjær-Kristensen, J., Febbraio, M., et al. (2002). Cytokine Response to Eccentric Exercise in Young and Elderly Humans. Am. J. Physiol. Cell Physiol. 283 (1), C289–C295. doi:10.1152/ajpcell.00583.2001
Toth, M. J., Matthews, D. E., Tracy, R. P., and Previs, M. J. (2005). Age-related Differences in Skeletal Muscle Protein Synthesis: Relation to Markers of Immune Activation. Am. J. Physiol. Endocrinol. Metab. 288 (5), E883–E891. doi:10.1152/ajpendo.00353.2004
Valiathan, R., Ashman, M., and Asthana, D. (2016). Effects of Ageing on the Immune System: Infants to Elderly. Scand. J. Immunol. 83 (4), 255–266. doi:10.1111/sji.12413
Vallejo, A. N., Schirmer, M., Weyand, C. M., and Goronzy, J. J. (2000). Clonality and Longevity of CD4+CD28null T Cells are Associated With Defects in Apoptotic Pathways. J. Immunol. 165 (11), 6301–6307. doi:10.4049/jimmunol.165.11.6301
van den Akker, E. B., Pitts, S. J., Deelen, J., Moed, M. H., Potluri, S., van Rooij, J., et al. (2016). Uncompromised 10-Year Survival of Oldest Old Carrying Somatic Mutations in DNMT3A and TET2. Blood 127 (11), 1512–1515. doi:10.1182/blood-2015-12-685925
van Kalsbeek, R. J., van der Pal, H. J. H., Hjorth, L., Winther, J. F., Michel, G., Haupt, R., et al. (2021). The European Multistakeholder PanCareFollowUp Project: Novel, Person-Centred Survivorship Care to Improve Care Quality, Effectiveness, Cost-Effectiveness and Accessibility for Cancer Survivors and Caregivers. Eur. J. Cancer 153, 74–85. doi:10.1016/j.ejca.2021.05.030
Vatanen, A., Hou, M., Huang, T., Söder, O., Jahnukainen, T., Kurimo, M., et al. (2017). Clinical and Biological Markers of Premature Aging After Autologous SCT in Childhood Cancer. Bone Marrow Transpl. 52 (4), 600–605. doi:10.1038/bmt.2016.334
Vaziri, H., Dragowska, W., Allsopp, R. C., Thomas, T. E., Harley, C. B., and Lansdorp, P. M. (1994). Evidence for a Mitotic Clock in Human Hematopoietic Stem Cells: Loss of Telomeric DNA with Age. Proc. Natl. Acad. Sci. 91 (21), 9857–9860. doi:10.1073/pnas.91.21.9857
Wallace, W. H. B., Blacklay, A., Eiser, C., Davies, H., Hawkins, M., Levitt, G. A., et al. (2001). Regular Review: Developing Strategies for Long Term Follow up of Survivors of Childhood Cancer. Bmj 323 (7307), 271–274. doi:10.1136/bmj.323.7307.271
Wang, E. C., Moss, P. A., Frodsham, P., Lehner, P. J., Bell, J. I., and Borysiewicz, L. K. (1995). CD8highCD57+ T Lymphocytes in Normal, Healthy Individuals are Oligoclonal and Respond to Human Cytomegalovirus. J. Immunol. 155 (10), 5046–5056.
Wang, S., Prizment, A., Thyagarajan, B., and Blaes, A. (2021). Cancer Treatment-Induced Accelerated Aging in Cancer Survivors: Biology and Assessment. Cancers 13 (3), 427. doi:10.3390/cancers13030427
Wang, X., Lu, Y., Li, C., Larbi, A., Feng, L., Shen, Q., et al. (2020). Associations of Lifestyle Activities and a Heathy Diet With Frailty in Old Age: A Community-Based Study in Singapore. Aging 12 (1), 288–308. doi:10.18632/aging.102615
Weidner, C., Lin, Q., Koch, C., Eisele, L., Beier, F., Ziegler, P., et al. (2014). Aging of Blood Can be Tracked by DNA Methylation Changes at Just Three CpG Sites. Genome Biol. 15 (2), R24. doi:10.1186/gb-2014-15-2-r24
Weng, N.-p., Akbar, A. N., and Goronzy, J. (2009). CD28− T Cells: Their Role in the Age-Associated Decline of Immune Function. Trends Immunol. 30 (7), 306–312. doi:10.1016/j.it.2009.03.013
Wenisch, C., Patruta, S., Daxböck, F., Krause, R., and Hörl, W. (2000). Effect of Age on Human Neutrophil Function. J. Leukoc. Biol. 67 (1), 40–45. doi:10.1002/jlb.67.1.40
Weyh, C., Kruger, K., and Strasser, B. (2020). Physical Activity and Diet Shape the Immune System During Aging. Nutrients 12 (3). doi:10.3390/nu12030622
White, M. J., Nielsen, C. M., McGregor, R. H. C., Riley, E. M., and Goodier, M. R. (2014). Differential Activation of CD 57‐Defined Natural Killer Cell Subsets During Recall Responses to Vaccine Antigens. Immunology 142 (1), 140–150. doi:10.1111/imm.12239
Wiley, C. D., and Campisi, J. (2016). From Ancient Pathways to Aging Cells-Connecting Metabolism and Cellular Senescence. Cell Metab. 23 (6), 1013–1021. doi:10.1016/j.cmet.2016.05.010
Wilson, C. L., Liu, W., Yang, J. J., Kang, G., Ojha, R. P., Neale, G. A., et al. (2015). Genetic and Clinical Factors Associated With Obesity Among Adult Survivors of Childhood Cancer: A Report From the St. Jude Lifetime Cohort. Cancer 121 (13), 2262–2270. doi:10.1002/cncr.29153
Wolff, J. L., Starfield, B., and Anderson, G. (2002). Prevalence, Expenditures, and Complications of Multiple Chronic Conditions in the Elderly. Arch. Intern. Med. 162 (20), 2269–2276. doi:10.1001/archinte.162.20.2269
Wong, Y. T., Gruber, J., Jenner, A. M., Tay, F. E. H., and Ruan, R. (2011). Chronic Resveratrol Intake Reverses Pro-inflammatory Cytokine Profile and Oxidative DNA Damage in Ageing Hybrid Mice. Age 33 (3), 229–246. doi:10.1007/s11357-010-9174-4
Woo, J., Yu, R., Tang, N., and Leung, J. (2014). Telomere Length is Associated with Decline in Grip Strength in Older Persons Aged 65 Years and over. Age 36 (5), 9711. doi:10.1007/s11357-014-9711-7
Wood, W. A., Krishnamurthy, J., Mitin, N., Torrice, C., Parker, J. S., Snavely, A. C., et al. (2016). Chemotherapy and Stem Cell Transplantation Increase p16 INK4a Expression, a Biomarker of T-Cell Aging. EBioMedicine 11, 227–238. doi:10.1016/j.ebiom.2016.08.029
Woodmansey, E. J., McMurdo, M. E. T., Macfarlane, G. T., and Macfarlane, S. (2004). Comparison of Compositions and Metabolic Activities of Fecal Microbiotas in Young Adults and in Antibiotic-Treated and Non-antibiotic-treated Elderly Subjects. Appl. Environ. Microbiol. 70 (10), 6113–6122. doi:10.1128/aem.70.10.6113-6122.2004
Wyczalkowska-Tomasik, A., Czarkowska-Paczek, B., Zielenkiewicz, M., and Paczek, L. (2016). Inflammatory Markers Change with Age, But do Not Fall Beyond Reported Normal Ranges. Arch. Immunol. Ther. Exp. 64 (3), 249–254. doi:10.1007/s00005-015-0357-7
Wynn, R. F., Cross, M. A., Hatton, C., Will, A. M., Lashford, L. S., Dexter, T. M., et al. (1998). Accelerated Telomere Shortening in Young Recipients of Allogeneic Bone-Marrow Transplants. Lancet 351 (9097), 178–181. doi:10.1016/s0140-6736(97)08256-1
Wynn, R., Thornley, I., Freedman, M., and Saunders, E. F. (1999). Telomere Shortening in Leucocyte Subsets of Long-Term Survivors of Allogeneic Bone Marrow Transplantation. Br. J. Haematol. 105 (4), 997–1001. doi:10.1046/j.1365-2141.1999.01450.x
Xu, C., Zhu, H., and Qiu, P. (2019). Aging Progression of Human Gut Microbiota. BMC Microbiol. 19 (1), 236. doi:10.1186/s12866-019-1616-2
Xu, W., and Larbi, A. (2017). Markers of T Cell Senescence in Humans. Int. J. Mol. Sci. 18 (8), 1742. doi:10.3390/ijms18081742
Yalon, M., Toren, A., Jabarin, D., Fadida, E., Constantini, S., and Mehrian-Shai, R. (2019). Elevated NLR May be a Feature of Pediatric Brain Cancer Patients. Front. Oncol. 9, 327. doi:10.3389/fonc.2019.00327
Yousefzadeh, M. J., Flores, R. R., Zhu, Y., Schmiechen, Z. C., Brooks, R. W., Trussoni, C. E., et al. (2021). An Aged Immune System Drives Senescence and Ageing of Solid Organs. Nature 594 (7861), 100–105. doi:10.1038/s41586-021-03547-7
Zanni, F., Vescovini, R., Biasini, C., Fagnoni, F., Zanlari, L., Telera, A., et al. (2003). Marked Increase With Age of Type 1 Cytokines Within Memory and Effector/Cytotoxic CD8+ T Cells in Humans: A Contribution to Understand the Relationship Between Inflammation and Immunosenescence. Exp. Gerontol. 38 (9), 981–987. doi:10.1016/s0531-5565(03)00160-8
Zhang, J., Wang, X., Vikash, V., Ye, Q., Wu, D., Liu, Y., et al. (2016). ROS and ROS-Mediated Cellular Signaling. Oxid. Med. Cell. Longev. 2016, 4350965. doi:10.1155/2016/4350965
Zhao, J., Miao, K., Wang, H., Ding, H., and Wang, D. W. (2013). Association Between Telomere Length and Type 2 Diabetes Mellitus: A Meta-Analysis. PLoS One 8 (11), e79993. doi:10.1371/journal.pone.0079993
Zhao, K., and Liu, Q. (2016). The Clinical Application of Mesenchymal Stromal Cells in Hematopoietic Stem Cell Transplantation. J. Hematol. Oncol. 9 (1), 46. doi:10.1186/s13045-016-0276-z
Keywords: childhood cancer survivor, immunosenescence, low-grade inflammation, accelerated aging, late effects, patient stratification, elderly
Citation: Lázničková P, Bendíčková K, Kepák T and Frič J (2021) Immunosenescence in Childhood Cancer Survivors and in Elderly: A Comparison and Implication for Risk Stratification. Front. Aging 2:708788. doi: 10.3389/fragi.2021.708788
Received: 20 May 2021; Accepted: 05 July 2021;
Published: 19 July 2021.
Edited by:
Moisés Evandro Bauer, Pontifical Catholic University of Rio Grande do Sul, BrazilReviewed by:
Roel De Maeyer, University College London, United KingdomUmberto Galderisi, University of Campania Luigi Vanvitelli, Italy
Copyright © 2021 Lázničková, Bendíčková, Kepák and Frič. This is an open-access article distributed under the terms of the Creative Commons Attribution License (CC BY). The use, distribution or reproduction in other forums is permitted, provided the original author(s) and the copyright owner(s) are credited and that the original publication in this journal is cited, in accordance with accepted academic practice. No use, distribution or reproduction is permitted which does not comply with these terms.
*Correspondence: Jan Frič, jan.fric@fnusa.cz