- 1Department of Clinical Nutrition, Second Affiliated Hospital of Fujian Medical University, Quanzhou, China
- 2The School of Public Health, Fujian Medical University, Fuzhou, Fujian, China
Migraine is a chronic, recurrent neurovascular disorder characterized by episodes closely associated with neurovascular hypersensitivity. Oxidative stress can worsen the hypersensitive state of the central nervous system, which in turn can trigger pro-inflammatory factors that result in neurogenic inflammation. Topiramate is frequently used as a preventative measure for migraines, but there is currently little empirical data to support its efficacy through pathways related to neurogenic inflammation and oxidative stress. This review provides an overview of current knowledge regarding the etiology, inducements, pathophysiology, and available treatments for migraine, with a focus on the clinical and experimental evidence of neurogenic inflammation and oxidative stress in migraine. It also delves into the antioxidant and anti-inflammatory qualities of topiramate, clarifying the possible ways in which topiramate affects these pathways to lessen migraine symptoms.
1 Introduction
Migraine manifests as a chronic neurovascular disease characterized by unilateral or bilateral throbbing severe headaches, predominantly unilateral, recurring with symptoms such as nausea, vomiting, photophobia, and phonophobia that impair autonomic nervous system functions (Steiner and Stovner, 2023) many sufferers cannot perform normal or work activities. according to the 2019 global burden of disease Study, migraine’s incidence ranks fifth among people aged 25–49 (Vos et al., 2020). Surveys across various regions in China among adults aged 18–65 years show an annual prevalence of headache as 28.5%, with most cases being primary migraines frequently seen in middle-aged women (Yu et al., 2013, 2012, 2011). Epidemiological studies indicate that urban residents, people over thirty, and women are likely risk factors for migraines (Yu et al., 2011). The annual medical costs for migraine in China amount to 331.7 billion, causing significant economic losses and severely affecting the quality of life for patients (Yu et al., 2011).
Migraine is a multifactorial disorder closely related to oxidative stress and neurogenic inflammation. Oxidative stress is a process of oxidative damage to the body or cells caused by the excessive accumulation of reactive oxygen species (ROS). When oxidative stress occurs, it activates pro-inflammatory factors in the body, which in turn induces neurogenic inflammation. There is no cure for migraine, and current medications primarily provide symptomatic relief and may not be suitable for all patients (Yamanaka et al., 2021). Therefore, migraine patients who meet the criteria for prophylaxis should undergo standardized prophylactic treatment, which can reduce the severity of their migraine attacks and have a higher overall efficacy. Topiramate has been shown to be an effective and well-tolerated prophylactic medication for the treatment of migraine (Afshari et al., 2012), but the mechanism has not yet been clarified.
Therefore, this review elucidates the etiology, inducements, pathogenesis and treatment modalities of migraine, the clinical evidence that migraine is associated with oxidative stress and neurogenic inflammation, as well as the experimental evidence of antioxidant and anti-inflammatory effects of topiramate, which is of great significance for further research and exploration of the possible mechanisms of topiramate in the treatment of migraine by modulating oxidative stress and neurogenic inflammatory pathways. Furthermore, we offer recommendations for future research. In this review, we aim to provide a deeper theoretical foundation and empirical support for using topiramate to treat migraines.
2 Literature search strategy
Two electronic databases, including PubMed and Web of Science, were searched from January 1998 to August 2024. Search items included Migraine, Headache, Pathophysiology, Pathogenesis, Oxidative stress, Inflammation, Neurogenic inflammation, Topiramate, Topamax, Etiology, Inducement, inducements, Treatment, Preventive therapy, Neuroprotection, Inheritance, Genetic variation, Ferroptosis, Iron, DNA, and Lipid peroxidation. The aim was to gather research related to the etiology, inducements, pathogenesis, and treatment of migraines, with a particular focus on the roles of oxidative stress and inflammation in migraine and the therapeutic potential of topiramate therein.
3 Pathogenesis and treatment of migraine
3.1 Etiology and inducements of migraine
Migraine is a complex disorder with diverse etiologies, and various types of migraines may arise from different triggers. Additionally, a single trigger can result in multiple types of migraine attacks. In China, approximately 44% of migraine patients experience inadequate symptom relief following acute treatment (Zhang et al., 2023). Therefore, accurately identifying the specific causes and triggers of migraines and tailoring treatments accordingly is crucial for accelerating symptom relief and reducing patient suffering.
Migraine has a significant genetic component, with heritability estimates ranging from 50 to 80% (Yeh et al., 2024; Khan et al., 2021). The common subtypes of migraine are primarily polygenic, involving complex interactions among multiple genetic variants, each contributing modestly to disease susceptibility (van den Maagdenberg et al., 2019). Genetic factors influence key clinical features of migraines, such as earlier onset age, higher frequency in male patients, and increased medication usage (Pelzer et al., 2019). Recent studies have identified over 100 genetic loci associated with migraines, which impact neurotransmission, inflammatory responses, and pain perception (Grangeon et al., 2023). For instance, genes involved in synaptic plasticity regulation, such as SH2D5 and NPTX2, may contribute to migraine chronicity (Winsvold et al., 2018). Additionally, the TRPM8 gene, which responds to cold stimuli, has been linked to an increased risk of chronic migraine in individuals carrying the TRPM8 rs10166942 T allele (Ling et al., 2019).
Regarding inducements, sleep disorders are significant precipitating factors for migraine attacks. The brainstem-cortex network plays a critical role in the migraine pathway, with conditions such as insomnia, obstructive sleep apnea, sleep-related movement disorders, and REM sleep disturbances closely associated with migraine onset (Vgontzas and Pavlović, 2018). Stress is another key trigger; when stress exceeds an individual’s coping capacity, migraines may be triggered through the activation of the autonomic nervous system, particularly the hypothalamic-pituitary-adrenal axis. This activation leads to the release of cortisol and other stress hormones, which can initiate or exacerbate migraine episodes (Stubberud et al., 2021). Dietary compounds are also closely linked to migraine occurrence. For example, consuming processed meats containing nitrates and nitrites, which are converted into nitric oxide (NO) in the body, can cause vasodilation, potentially altering cerebral blood flow and triggering migraines (Gonzalez et al., 2016). Endocrine factors significantly contribute to migraine pathogenesis, as evidenced by the pronounced gender difference, with women being more frequently affected. The fluctuation in estrogen levels, particularly from the onset of menarche, increases the risk of migraines in women by approximately threefold compared to men (Jacome, 2001).
3.2 Pathophysiological mechanisms of migraine
The pathophysiological mechanisms of migraine are complex, and multiple hypotheses exist. Currently, two main theories are widely accepted, including the trigeminovascular system (TGVS) theory and the cortical spreading depression (CSD) theory.
3.2.1 TGVS theory
According to the TGVS theory, which combines blood vessels, nerves, and neurotransmitters, the trigeminal nerve and peri-vascular nerve fibers both inside and outside the skull serve as the main pathways for transmitting pain in migraines. Prior to the onset of migraine pain, it is essential to activate and sensitize the TGVS (Goadsby et al., 2009). The dura mater, intracranial vessels, trigeminal nerve perivascular fibers, trigeminal ganglion (TG), caudal subnucleus of the trigeminal nucleus caudalis (TNC), hypothalamus, and cerebral cortex are all part of the system (Charles and Brennan, 2010). A noxious stimulus stimulates the trigeminal nerve fibers, which in turn activate nociceptors at the fiber terminals. This process releases vasoactive peptides, including substance P, neurokinin A, and calcitonin gene-related peptide (CGRP). In the dura mater and other regions supplied by the trigeminal nerve, this results in neurogenic inflammation due to excessive vasodilation, mast cell degranulation, plasma protein extravasation, and the release of inflammatory mediators such as serotonin. Central sensitization results from the noxious stimulus being transmitted to the secondary neurons in the trigeminal cervical complex via the afferent fibers of the trigeminal nerve. It then travels to the thalamus’s tertiary neurons, which in turn send pain signals to the frontal lobe, cingulate gyrus, and parietal lobe (Ho et al., 2010; Figure 1A).
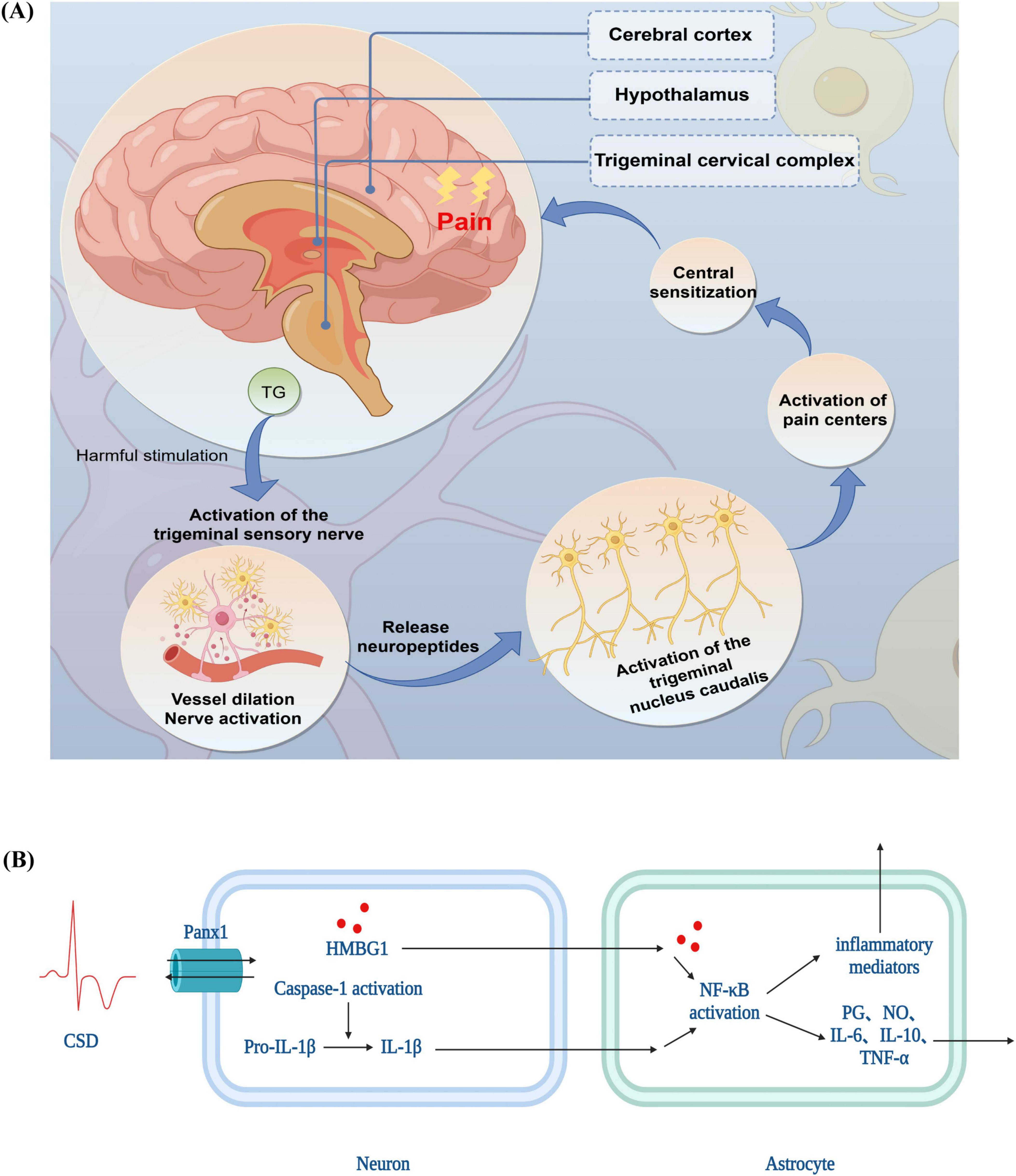
Figure 1. The pathophysiological mechanisms of migraine (A) trigeminovascular system theory (B) cortical spreading depression theory. TG, trigeminal ganglion; CSD, cortical spreading depression theory; PANX1, Pannexin 1; HMGB1, high mobility group box-1 protein; IL, interleukin; NF-κB, nuclear factor kappa-B; TNF-α, tumor necrosis factor; NO, nitric oxide; PG, prostaglandin.
3.2.2 CSD theory
Trigeminal afferent activation sets off the pain pathway, which sends information to the TG, the TNC, and finally to the brainstem and cortical structures involved in pain processing, resulting in pain (Zhang et al., 2011).
Alterations in the concentrations of intracellular and extracellular ions are linked to the production of CSD. Specifically, K+ and H+ move both inside and outside the cell, while Na+, Ca2+, and Cl– are associated with the intracellular movement of water molecules. This movement is caused by cellular swelling and a relative narrowing of extracellular gaps, which in turn generate slow-conducting waves of depolarization in multiple neurons and glial cells, resulting in the temporary inhibition of synaptic activity (Kowalska et al., 2021). For example, intracellular Ca2+ overload induces a transient oxidative stress in the organism and promotes the generation of ROS, which activate injurious signaling through transient receptor potential subtype anchor protein 1 channels (Takano et al., 2007); at the same time, changes in intracellular Ca2+ lead to the opening of neuronal pannexin 1 channels to open and activate cystatinase 1, followed by the release of high mobility group box-1 protein and the activation of nuclear factor kappa-B signaling pathway in astrocytes (Karatas et al., 2013), which triggers substantial inflammatory response, producing a variety of inflammatory cytokines such as interleukin (IL)-1β (Ghaemi et al., 2018), IL-6 (Ghaemi et al., 2018) and tumor necrosis factor (TNF)-α (Ghaemi et al., 2018) as well as Toll-like receptor (Ghaemi et al., 2016) production, which prolongs trigeminal nerve stimulation, leading to sensitization of the dura mater and triggering of headache (Figure 1B).
Conversely, CSD might be linked to dysfunction in the mitochondria. It is hypothesized that CSD, which results in migraine, may be caused by increased neuronal excitability (Sparaco et al., 2006). Insufficient energy production in the brain can result from mitochondrial damage, and migraine is a reaction to either an excess of oxidative stress or an inadequate amount of brain energy (Fila et al., 2019). Migraine production may be caused by impaired mitochondrial oxidative metabolism, which interferes with regular neuronal activity (Stuart and Griffiths, 2012). Thus, impaired oxidative metabolism results in decreased energy production, increased cortical excitability, and CSD, which in turn causes migraines when mitochondrial damage or dysfunction occurs in the brain.
3.3 Clinical prevention and management of migraine
While migraines are an incurable condition, they can be successfully avoided and managed. When treating migraines, two factors should be taken into account: first, pre-treatment education for patients, which includes avoiding migraine triggers and improving lifestyle choices; and second, pharmacological interventions, which include both preventive and acute-phase therapeutic medications for daily use during migraine attacks. Migraine prevention involves several strategies, including trigger avoidance and lifestyle modifications. Additionally, consensus guidelines (Ailani et al., 2021) recommend considering preventive pharmacologic therapy for patients experiencing four or more migraine headache days per month, or for those with two or more migraine headache days per month associated with substantial disability despite the use of acute medication. Common preventive medications for migraine include antiepileptic drugs, calcium channel blockers, β-blockers, anti-CGRP monoclonal antibodies, and botulinum toxin A. Among these, topiramate has been clinically proven to be effective for migraine prevention and is recommended as a first-line treatment (Irimia et al., 2012). Additionally, topiramate offers the added benefits of weight reduction might via improving insulin resistance in myocytes and adipocytes (Yang et al., 2024), potentially lowering the risk of complications associated with migraines and positively impacting overall patient health. However, the mechanism underlying its preventive effect is still unclear. Thus, more investigation is required to pinpoint the exact mechanisms by which topiramate prevents migraines, with the goal of offering fresh theoretical and experimental data to elucidate and improve the mechanisms of topiramate in migraine prevention.
4 Overview of topiramate
Topiramate is a naturally occurring monosaccharide dextro sulfide that is sulfamate-substituted. Its strong water solubility, easy blood-brain barrier passage, high bioavailability (81–95%), long half-life of approximately 19–25 h, and occasional adverse reactions, like tiredness, anorexia, upper respiratory tract infections, nausea, diarrhea, cognitive impairment (difficulty remembering things, poor concentration, etc.), and weight loss, are some of its beneficial properties.
The mechanism by which topiramate affects the body is by markedly increasing the activity of inhibitory neurotransmitters. Its five main mechanisms of action are as follows:
(1) Increases the threshold for migraine attacks by activating different sites on the gamma-aminobutyric acid (GABA) receptors in brain neural tissue, decreasing neuronal excitability, boosting membrane hyperpolarization, and decreasing focal discharges. These actions improve the chloride ion currents mediated by GABA (Herrero et al., 2002).
(2) Selectively inhibits voltage-dependent Na+ channels, which reduces or stops the release of neurotransmitters and vasoactive peptides, inhibits action potential propagation, and lessens focal discharges (DeLorenzo et al., 2000).
(3) It minimizes sustained membrane depolarization, raises the threshold for migraines, inhibits the release of CGRP and glutamate from the trigeminal vascular nerve endings, blocks high-voltage activated Ca2+ channels, and reduces or prevents the release of neurotransmitters and vasoactive peptides (Dodick, 2018).
(4) Reduces glutamate release as well as discharges by antagonistically binding to the AMPA/kainate receptors while having no discernible effect on the N-methyl-D-aspartic acid receptor subtypes (Gibbs et al., 2000).
(5) Reduces the levels of excitatory neurotransmitters and increases the transmission of inhibitory neurotransmitters by inhibiting carbonic anhydrase (CA), especially CA II and CA IV (White, 2005).
Topiramate attenuates dural vasodilation primarily by inhibiting nitric oxide-mediated release of CGRP from preganglionic fibers of the trigeminal nerve (Akerman and Goadsby, 2005). This may be the primary reason topiramate is not used to treat acute migraine attacks, but rather as a preventive medication for migraine. Studies have shown that topiramate cannot act directly on blood vessels or inhibit CGRP-mediated vasodilation. Nonetheless, topiramate is a very effective medication for migraine prophylaxis; however, the exact mechanism of topiramate treatment of migraine deserves further investigation, as there is less experimental evidence of topiramate prophylactic treatment of migraine.
5 The relationship between migraine, oxidative stress, and neurogenic inflammation
According to the theory of migraine genesis, the majority of migraine triggers have the ability to cause oxidative stress in the body. For example, alcohol is metabolized partially by alcohol dehydrogenase and partially by cytochrome P450-2E1, a dietary factor linked to migraines. Oxidative stress is triggered by the production of superoxide ions and hydrogen peroxide (Valencia-Olvera et al., 2014) in the former pathway. High alcohol consumption activates microglia and dramatically increases ROS production (Qin and Crews, 2012), which can, in extreme situations, affect the fluidity of the mitochondrial membrane, resulting in functional changes and an increase in oxidant production (Haorah et al., 2011). Furthermore, environmental particulate matter has the ability to cross the blood-brain barrier and penetrate the nasal mucosa epithelium, activating the nervous system by binding to particular receptors and producing oxidative stress (Lucchini et al., 2012). Thus, it is postulated that the factors that cause migraines have a similar capacity to increase oxidative stress, which in turn facilitates the onset of migraines.
According to the TGVS theory, neurogenic inflammation is regarded as one of the most direct mechanisms of migraine onset from the perspective of migraine pathophysiology. Moreover, CSD can be brought on by variations in the concentrations of intracellular and extracellular ions, which can result in brief oxidative stress and the release of neurogenic inflammatory factors. According to research, migraines may be brought on by CSD activation (Kissoon and Cutrer, 2017). Thus, among migraines, oxidative stress, and neurogenic inflammation exists a believed intricate linkage. Clinical evidence pertaining to migraines, oxidative stress, and neurogenic inflammation, along with experimental evidence of topiramate’s antioxidative and anti-inflammatory effects, provide strong hypothetical support for the idea that topiramate may treat migraines by blocking oxidative stress and neurogenic inflammation pathways.
5.1 Relationship between migraine and oxidative stress
5.1.1 Clinical evidence of oxidative stress
5.1.1.1 Antioxidant capacity
In contrast to the control group, patients with migraine without aura had higher total oxidative status (TOS), higher oxidative stress index (OSI), and lower total antioxidant status (TAS) (Jornayvaz and Shulman, 2010). In a different study, it was discovered that migraineurs had a much lower total antioxidant capacity (TAC) than controls, but that TAC would rise following therapeutic intervention (Tripathi et al., 2018). About 40% of patients with recurrent migraines had lower TAC levels (Gross et al., 2021). TAS, TOS, and OSI levels were not significantly different between migraine patients and controls in some studies (Eren et al., 2015; Geyik et al., 2016). This suggests that variations in the techniques used, biological samples analyzed, timing of sampling, and subject selection may be the cause of discrepancies (Geyik et al., 2016; Table 1).
5.1.1.2 Oxidative stress markers
In migraine patients, levels of malondialdehyde (MDA) are significantly elevated, especially in cases of migraine with aura, reflecting increased lipid peroxidation and oxidative stress damage (Aytaç et al., 2014; Bernecker et al., 2011; Gupta et al., 2009; Tuncel et al., 2008). Additionally, the level of 4-hydroxynonenal (4-HNE) is markedly higher in migraine patients compared to controls, further supporting the presence of oxidative stress (Bernecker et al., 2011; Table 1).
The levels of antioxidant enzymes are notably reduced in migraine patients. Compared to controls, catalase (CAT) and superoxide dismutase (SOD) levels are significantly lower in the migraine group (Aytaç et al., 2014). In patients with migraine with aura, SOD activity is significantly higher than in those without aura, although there is no significant correlation between SOD activity and the duration of migraine attacks (Tuncel et al., 2008; Table 1).
A study examining plasma samples from 50 migraine patients and 30 healthy volunteers found that plasma levels of 8-hydroxy-2’-deoxyguanosine were significantly higher in migraine patients compared to controls, indicating a connection between migraine and oxidative stress-related DNA damage (Geyik et al., 2016; Table 1).
5.1.2 Topiramate as an antioxidant
Topiramate exhibits complex and context-dependent effects on oxidative stress. In a mouse model of diabetes, topiramate effectively protected the brain from diabetes-induced damage by reducing 4-HNE levels and increasing glutathione (GSH) levels (Price et al., 2015). Similarly, topiramate decreased its MDA levels and increased GSH levels in a study on the prevention of flap necrosis after plastic and reconstructive surgery (Ahmadzadeh et al., 2022).
However, in a study on the potential toxic effects of topiramate on the liver and kidney of male mice, topiramate significantly decreased the activities of the antioxidant enzymes SOD and CAT, while increasing MDA and NO levels (El Makawy et al., 2022). In a pentylenetetrazole-induced convulsion model in mice, topiramate pretreatment decreased GSH levels and increased MDA levels, suggesting that TPM negatively regulates oxidative stress (Agarwal et al., 2011). In vitro study found that topiramate increased MDA, NO and ROS production in astrocytes, showing a dose-dependent negative effect (Pavone and Cardile, 2003).
These findings suggest that topiramate may exert dual effects on oxidative stress, offering neuroprotection in certain contexts while exacerbating oxidative stress under different conditions and dosages. The evidence regarding its impact on oxidative stress in migraine models remains limited, underscoring the need for careful consideration of dosages of topiramate and application conditions in the prophylactic treatment of migraines. Balancing its neuroprotective potential against the risk of exacerbating oxidative stress is crucial for ensuring safe and effective treatment. Further research is necessary to optimize its clinical use and maximize therapeutic benefits.
5.2 The relationship between migraine and neurogenic inflammation
5.2.1 Clinical evidence of neurogenic inflammation
Increased blood expression of cytokines has been reported in migraineurs, both adults and children. Adult migraine patients have elevated blood levels of TNF-α, IL-1β, IL-6, IL-18, and IL-10 during migraine episodes (Dönder et al., 2021; Perini et al., 2005; Sarchielli et al., 2006), whereas children with migraines have elevated levels of IL-1α (Boćkowski et al., 2009). Even aggressive interventions fail to alleviate the condition when TNF-α levels in the cerebrospinal fluid of migraine patients are elevated but normal in the blood (Rozen and Swidan, 2007). This could be one of the causes of intractable migraines. Patients with migraines also have higher blood levels of TNF-α (Wang et al., 2015). Furthermore, it has been discovered that patients with migraine have higher blood levels of inflammatory markers linked to vascular diseases, such as homocysteine (Oterino et al., 2010), suggesting that a neuroinflammatory cascade is activated during the migraine pathogenesis (Table 2).
5.2.2 Topiramate as an anti-inflammatory agent
Topiramate has demonstrated neuroprotective effects in various pathological models, particularly through its anti-inflammatory properties. In the context of early brain injury following subarachnoid hemorrhage (SAH), topiramate significantly reduced the elevated levels of TNF-α, IL-1β, and IL-6 induced by SAH, suggesting that topiramate may exert neuroprotection via its anti-inflammatory effects (Tian et al., 2015). Similarly, in a diabetic neuropathy mouse model, topiramate administration (10 or 30 mg/kg) led to a significant, dose-dependent reduction in spinal cord levels of TNF-α and IL-1β (Attia et al., 2023). In a methylphenidate-induced rat model, topiramate significantly decreased the levels of IL-1β, TNF-α, and brain-derived neurotrophic factor in isolated hippocampal cells (Motaghinejad et al., 2016).
In an experimental model of abdominal aortic aneurysm, topiramate markedly downregulated pro-inflammatory M1 macrophage activity, reduced the expression of TNF-α, IL-1β, and matrix metalloproteinase-9, and promoted a phenotypic shift of macrophages from M1 to M2, thereby mitigating pro-inflammatory activity and enhancing tissue repair processes (Attia et al., 2023).
These studies collectively suggest that topiramate exerts significant neuroprotective effects across various pathological models through its potent anti-inflammatory properties. These findings not only reinforce the potential of topiramate as a neuroprotective agent but also provide a theoretical foundation for its application in migraine models.
6 Conclusion
This review comprehensively examines the causes, progression, and treatment strategies for migraine, with a particular emphasis on the roles of oxidative stress and neurogenic inflammation. It highlights the antioxidative and anti-inflammatory properties of topiramate in migraine management. Both neurogenic inflammation and oxidative stress are key factors in migraine development, and topiramate’s neuroprotective potential through its anti-inflammatory and antioxidative effects is noted. However, further experimental studies are required to substantiate the mechanism by which topiramate modulates these pathways and to confirm its efficacy in treating migraines. This review offers a thorough analysis and deeper understanding of the pathological mechanisms underlying migraine and the potential therapeutic benefits of topiramate.
Evidence has demonstrated that iron deposition occurs in the brains of migraine patients (Domínguez et al., 2019). These iron ions can exacerbate oxidative stress by catalyzing Fenton and Haber-Weiss reactions, leading to the production of ROS and reactive nitrogen species, which subsequently cause damage to brain cells (Fila et al., 2024). Although migraines are not typically classified as neurodegenerative diseases marked by extensive cell death, studies have reported the occurrence of apoptosis and pyroptosis, both forms of programmed cell death (Wang et al., 2022). The accumulation of iron in the brain may induce lipid peroxidation through oxidative stress, potentially triggering ferroptosis. In a nitroglycerin-induced migraine model in rats, we have observed similar iron accumulation in serum (unpublished data). Ferroptosis, a distinct form of cell death driven by oxidative stress, has been implicated in the pathogenesis of various central nervous system disorders, including Parkinson’s disease (Zhao et al., 2020), Alzheimer’s disease (Lane et al., 2021), Huntington’s disease (Skouta et al., 2014), epilepsy (Cai and Yang, 2021), and traumatic brain injury (Rui et al., 2021). Consequently, inhibiting ferroptosis in neural cells has emerged as a promising therapeutic approach for treating central nervous system disorders including migraine.
Topiramate has been proposed as a migraine preventive medication because it inhibits ferroptosis via the glutathione peroxidase 4 (GPX4), GSH, and cystine/glutamate antiporter (System Xc-). In particular, topiramate may control the flow of glutamate and cysteine through System Xc-, which could impact GSH synthesis and improve the ability of GPX4 to change harmful lipid hydroperoxides into safe lipid alcohols. This could prevent ferroptosis and lessen inflammation caused by the release of lipid metabolites and damage-associated molecular patterns (Figure 2).
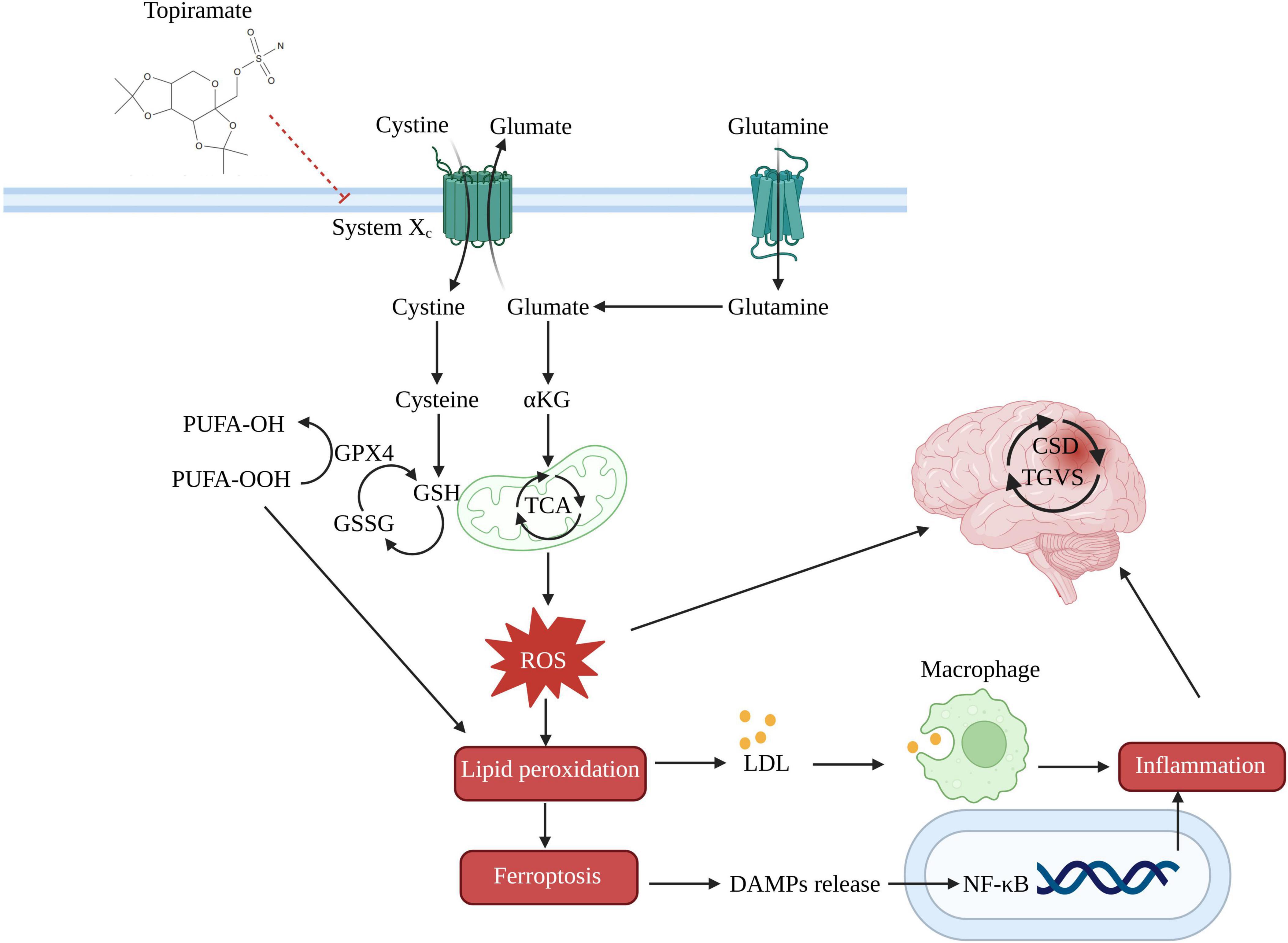
Figure 2. Potential mechanism of topiramate in treating migraine by the ferroptosis pathway. System Xc-, cystine/glutamate antiporter; GSSG, oxidized glutathione; α-KG, alpha-ketoglutarate; TCA, tricarboxylic acid cycle; PUFA-OH, lipid alcohols; PUFA-OOH, lipid hydroperoxides; LDL, low-density lipoprotein; DAMPs, damage-associated molecular patterns.
Investigating ferroptosis not only expands our knowledge of the intricate mechanisms underlying migraines, but it also creates new opportunities for locating possible targets for treatment. In the future, research should focus on ferroptosis pathways to identify targets for migraine preventive treatments. Additionally, further exploration of the role of topiramate in modulating this pathway, particularly its dose-dependent effects, is necessary. This could lead to the development of new strategies for migraine treatment.
Author contributions
Q-WC: Formal analysis, Investigation, Methodology, Validation, Visualization, Writing – original draft, Writing – review and editing. R-TM: Investigation, Methodology, Visualization, Writing – original draft. C-YK: Conceptualization, Funding acquisition, Investigation, Project administration, Visualization, Writing – original draft, Writing – review and editing.
Funding
The author(s) declare that financial support was received for the research, authorship, and/or publication of this article. This study was supported by a grant from the Second Affiliated Hospital of Fujian Medical University (Serial No. YJ202301).
Conflict of interest
The authors declare that the research was conducted in the absence of any commercial or financial relationships that could be construed as a potential conflict of interest.
Publisher’s note
All claims expressed in this article are solely those of the authors and do not necessarily represent those of their affiliated organizations, or those of the publisher, the editors and the reviewers. Any product that may be evaluated in this article, or claim that may be made by its manufacturer, is not guaranteed or endorsed by the publisher.
References
Afshari, D., Rafizadeh, S., and Rezaei, M. (2012). A comparative study of the effects of low-dose topiramate versus sodium valproate in migraine prophylaxis. Int. J. Neurosci. 122, 60–68. doi: 10.3109/00207454.2011.626908
Agarwal, N. B., Agarwal, N. K., Mediratta, P. K., and Sharma, K. K. (2011). Effect of lamotrigine, oxcarbazepine and topiramate on cognitive functions and oxidative stress in PTZ-kindled mice. Seizure 20, 257–262. doi: 10.1016/j.seizure.2010.12.006
Ahmadzadeh, M., Esmaeilzadeh, Z., Khezri, M. R., Jafari, A., and Ghasemnejad-Berenji, M. (2022). The promising effect of topiramate on random-pattern skin flap survival in rats. Aesthetic Plast. Surg. 46, 2548–2555. doi: 10.1007/s00266-022-02969-6
Ailani, J., Burch, R. C., Robbins, M. S., and Board of Directors of the American Headache Society (2021). The American headache society consensus statement: Update on integrating new migraine treatments into clinical practice. Headache 61, 1021–1039. doi: 10.1111/head.14153
Akerman, S., and Goadsby, P. J. (2005). Topiramate inhibits trigeminovascular activation: An intravital microscopy study. Br. J. Pharmacol. 146, 7–14. doi: 10.1038/sj.bjp.0706290
Attia, M. A., Soliman, N., Eladl, M. A., Bilasy, S. E., El-Abaseri, T. B., Ali, H. S., et al. (2023). Topiramate affords neuroprotection in diabetic neuropathy model via downregulating spinal GFAP/inflammatory burden and improving neurofilament production. Toxicol. Mech. Methods 33, 563–577. doi: 10.1080/15376516.2023.2196687
Aytaç, B., Coşkun, Ö, Alioğlu, B., Durak, Z. E., Büber, S., Tapçi, E., et al. (2014). Decreased antioxidant status in migraine patients with brain white matter hyperintensities. Neurol. Sci. 35, 1925–1929. doi: 10.1007/s10072-014-1864-8
Bernecker, C., Ragginer, C., Fauler, G., Horejsi, R., Möller, R., Zelzer, S., et al. (2011). Oxidative stress is associated with migraine and migraine-related metabolic risk in females: Oxidative stress and migraine. Eur. J. Neurol. 18, 1233–1239. doi: 10.1111/j.1468-1331.2011.03414.x
Boćkowski, L., Sobaniec, W., and Żelazowska-Rutkowska, B. (2009). Proinflammatory Plasma Cytokines in Children With Migraine. Pediatr. Neurol. 41, 17–21. doi: 10.1016/j.pediatrneurol.2009.02.001
Cai, Y., and Yang, Z. (2021). Ferroptosis and its role in epilepsy. Front. Cell. Neurosci. 15:696889. doi: 10.3389/fncel.2021.696889
Charles, A., and Brennan, K. C. (2010). The neurobiology of migraine. Handb. Clin. Neurol. 97, 99–108. doi: 10.1016/S0072-9752(10)97007-3
DeLorenzo, R. J., Sombati, S., and Coulter, D. A. (2000). Effects of topiramate on sustained repetitive firing and spontaneous recurrent seizure discharges in cultured hippocampal neurons. Epilepsia 41, 40–44. doi: 10.1111/j.1528-1157.2000.tb02170.x
Domínguez, C., López, A., Ramos-Cabrer, P., Vieites-Prado, A., Pérez-Mato, M., Villalba, C., et al. (2019). Iron deposition in periaqueductal gray matter as a potential biomarker for chronic migraine. Neurology 92, e1076–e1085. doi: 10.1212/WNL.0000000000007047
Dönder, A., Cafer, V., Yilmaz, A., Aslanhan, H., and Arikanoğlu, A. (2021). Investigation of serum vaspin, visfatin, chemerin and IL-18 levels in migraine patients. Arq. Neuropsiquiatr. 79, 789–794. doi: 10.1590/0004-282X-ANP-2020-0425
El Makawy, A. I., Mabrouk, D. M., Ibrahim, F. M., and Ahmed, K. A. (2022). Genotoxic, biochemical and histopathological studies to assessment the topiramate hepatorenal toxicity in mice. Drug Chem. Toxicol. 45, 103–112. doi: 10.1080/01480545.2019.1660364
Eren, Y., Dirik, E., Neşelioğlu, S., and Erel, Ö (2015). Oxidative stress and decreased thiol level in patients with migraine: Cross-sectional study. Acta Neurol. Belg. 115, 643–649. doi: 10.1007/s13760-015-0427-y
Fila, M., Pawłowska, E., and Blasiak, J. (2019). Mitochondria in migraine pathophysiology - does epigenetics play a role? Arch. Med. Sci. AMS 15, 944–956. doi: 10.5114/aoms.2019.86061
Fila, M., Przyslo, L., Derwich, M., Luniewska-Bury, J., Pawlowska, E., and Blasiak, J. (2024). Potential of ferroptosis and ferritinophagy in migraine pathogenesis. Front. Mol. Neurosci. 17:1427815. doi: 10.3389/fnmol.2024.1427815
Geyik, S., Altunısık, E., Neyal, A. M., and Taysi, S. (2016). Oxidative stress and DNA damage in patients with migraine. J. Headache Pain 17:10. doi: 10.1186/s10194-016-0606-0
Ghaemi, A., Alizadeh, L., Babaei, S., Jafarian, M., Khaleghi Ghadiri, M., Meuth, S. G., et al. (2018). Astrocyte-mediated inflammation in cortical spreading depression. Cephalalgia 38, 626–638. doi: 10.1177/0333102417702132
Ghaemi, A., Sajadian, A., Khodaie, B., Lotfinia, A. A., Lotfinia, M., Aghabarari, A., et al. (2016). Immunomodulatory effect of toll-like receptor-3 ligand poly I:C on Cortical spreading depression. Mol. Neurobiol. 53, 143–154. doi: 10.1007/s12035-014-8995-z
Gibbs, J. W., Sombati, S., DeLorenzo, R. J., and Coulter, D. A. (2000). Cellular actions of topiramate: Blockade of kainate-evoked inward currents in cultured hippocampal neurons. Epilepsia 41, 10–16. doi: 10.1111/j.1528-1157.2000.tb02164.x
Goadsby, P. J., Charbit, A. R., Andreou, A. P., Akerman, S., and Holland, P. R. (2009). Neurobiology of migraine. Neuroscience 161, 327–341. doi: 10.1016/j.neuroscience.2009.03.019
Gonzalez, A., Hyde, E., Sangwan, N., Gilbert, J. A., Viirre, E., and Knight, R. (2016). Migraines are correlated with higher levels of nitrate-, nitrite-, and nitric oxide-reducing oral microbes in the american gut project cohort. mSystems 1, e105–e116. doi: 10.1128/mSystems.00105-16
Grangeon, L., Lange, K. S., Waliszewska-Prosół, M., Onan, D., Marschollek, K., Wiels, W., et al. (2023). Genetics of migraine: Where are we now? J. Headache Pain 24:12. doi: 10.1186/s10194-023-01547-8
Gross, E. C., Putananickal, N., Orsini, A.-L., Vogt, D. R., Sandor, P. S., Schoenen, J., et al. (2021). Mitochondrial function and oxidative stress markers in higher-frequency episodic migraine. Sci. Rep. 11:4543. doi: 10.1038/s41598-021-84102-2
Gupta, R., Pathak, R., Bhatia, M. S., and Banerjee, B. D. (2009). Comparison of oxidative stress among migraineurs, tension-type headache subjects, and a control group. Ann. Indian Acad. Neurol. 12, 167–172. doi: 10.4103/0972-2327.56316
Haorah, J., Floreani, N. A., Knipe, B., and Persidsky, Y. (2011). Stabilization of superoxide dismutase by acetyl-l-carnitine in human brain endothelium during alcohol exposure: Novel protective approach. Free Radic. Biol. Med. 51, 1601–1609. doi: 10.1016/j.freeradbiomed.2011.06.020
Herrero, A. I, Olmo, N., González-Escalada, J. R., and Solís, J. M. (2002). Two new actions of topiramate: Inhibition of depolarizing GABAA-mediated responses and activation of a potassium conductance. Neuropharmacology 42, 210–220. doi: 10.1016/S0028-3908(01)00171-X
Ho, T. W., Edvinsson, L., and Goadsby, P. J. (2010). CGRP and its receptors provide new insights into migraine pathophysiology. Nat. Rev. Neurol. 6, 573–582. doi: 10.1038/nrneurol.2010.127
Irimia, P., Carmona-Abellán, M., and Martínez-Vila, E. (2012). Chronic migraine: A therapeutic challenge for clinicians. Expert Opin. Emerg. Drugs 17, 445–447. doi: 10.1517/14728214.2012.726612
Jornayvaz, F. R., and Shulman, G. I. (2010). Regulation of mitochondrial biogenesis. Essays Biochem. 47, 69–84. doi: 10.1042/bse0470069
Karatas, H., Erdener, S. E., Gursoy-Ozdemir, Y., Lule, S., Eren-Koçak, E., Sen, Z. D., et al. (2013). Spreading depression triggers headache by activating neuronal panx1 channels. Science 339, 1092–1095. doi: 10.1126/science.1231897
Khan, J., Asoom, L. I. A., Sunni, A. A., Rafique, N., Latif, R., Saif, S. A., et al. (2021). Genetics, pathophysiology, diagnosis, treatment, management, and prevention of migraine. Biomed. Pharmacother. 139:111557. doi: 10.1016/j.biopha.2021.111557
Kissoon, N. R., and Cutrer, F. M. (2017). Aura and other neurologic dysfunction in or with migraine. Headache J. Head Face Pain 57, 1179–1194. doi: 10.1111/head.13101
Kowalska, M., Prendecki, M., Piekut, T., Kozubski, W., and Dorszewska, J. (2021). Migraine: Calcium channels and glia. Int. J. Mol. Sci. 22:2688. doi: 10.3390/ijms22052688
Lane, D. J. R., Metselaar, B., Greenough, M., Bush, A. I., and Ayton, S. J. (2021). Ferroptosis and NRF2: An emerging battlefield in the neurodegeneration of Alzheimer’s disease. Essays Biochem. 65, 925–940. doi: 10.1042/EBC20210017
Ling, Y.-H., Chen, S.-P., Fann, C. S.-J., Wang, S.-J., and Wang, Y.-F. (2019). TRPM8 genetic variant is associated with chronic migraine and allodynia. J. Headache Pain 20:115. doi: 10.1186/s10194-019-1064-2
Lucchini, R. G., Dorman, D. C., Elder, A., and Veronesi, B. (2012). Neurological impacts from inhalation of pollutants and the nose-brain connection. Neurotoxicology 33, 838–841. doi: 10.1016/j.neuro.2011.12.001
Motaghinejad, M., Motevalian, M., and Shabab, B. (2016). Neuroprotective effects of various doses of topiramate against methylphenidate induced oxidative stress and inflammation in rat isolated hippocampus. Clin. Exp. Pharmacol. Physiol. 43, 360–371. doi: 10.1111/1440-1681.12538
Oterino, A., Toriello, M., Valle, N., Castillo, J., Alonso-Arranz, A., Bravo, Y., et al. (2010). The relationship between homocysteine and genes of folate-related enzymes in migraine patients. Headache J. Head Face Pain 50, 99–168. doi: 10.1111/j.1526-4610.2009.01484.x
Pavone, A., and Cardile, V. (2003). An in vitro study of new antiepileptic drugs and astrocytes. Epilepsia 44, 34–39. doi: 10.1046/j.1528-1157.44.s10.5.x
Pelzer, N., Louter, M. A., van Zwet, E. W., Nyholt, D. R., Ferrari, M. D., van den Maagdenberg, A. M., et al. (2019). Linking migraine frequency with family history of migraine. Cephalalgia Int. J. Headache 39, 229–236. doi: 10.1177/0333102418783295
Perini, F., D’Andrea, G., Galloni, E., Pignatelli, F., Billo, G., Alba, S., et al. (2005). Plasma cytokine levels in migraineurs and controls. Headache J. Head Face Pain 45, 926–931. doi: 10.1111/j.1526-4610.2005.05135.x
Price, T. O., Farr, S. A., Niehoff, M. L., Ercal, N., Morley, J. E., and Shah, G. N. (2015). Protective effect of topiramate on hyperglycemia-induced cerebral oxidative stress, pericyte loss and learning behavior in diabetic mice. Int. Libr. Diabetes Metab. 1, 6–12.
Qin, L., and Crews, F. T. (2012). NADPH oxidase and reactive oxygen species contribute to alcohol-induced microglial activation and neurodegeneration. J. Neuroinflamm. 9:5. doi: 10.1186/1742-2094-9-5
Rozen, T., and Swidan, S. Z. (2007). Elevation of CSF tumor necrosis factor α levels in new daily persistent headache and treatment refractory chronic migraine. Headache J. Head Face Pain 47, 1050–1055. doi: 10.1111/j.1526-4610.2006.00722.x
Rui, T., Wang, H., Li, Q., Cheng, Y., Gao, Y., Fang, X., et al. (2021). Deletion of ferritin H in neurons counteracts the protective effect of melatonin against traumatic brain injury-induced ferroptosis. J. Pineal Res. 70:e12704. doi: 10.1111/jpi.12704
Sarchielli, P., Alberti, A., Baldi, A., Coppola, F., Rossi, C., Pierguidi, L., et al. (2006). Proinflammatory cytokines, adhesion molecules, and lymphocyte integrin expression in the internal jugular blood of migraine patients without aura assessed ictally. Headache J. Head Face Pain 46, 200–207. doi: 10.1111/j.1526-4610.2006.00337.x
Skouta, R., Dixon, S. J., Wang, J., Dunn, D. E., Orman, M., Shimada, K., et al. (2014). Ferrostatins inhibit oxidative lipid damage and cell death in diverse disease models. J. Am. Chem. Soc. 136, 4551–4556. doi: 10.1021/ja411006a
Sparaco, M., Feleppa, M., Lipton, R., Rapoport, A., and Bigal, M. (2006). Mitochondrial dysfunction and migraine: Evidence and hypotheses. Cephalalgia 26, 361–372. doi: 10.1111/j.1468-2982.2005.01059.x
Steiner, T. J., and Stovner, L. J. (2023). Global epidemiology of migraine and its implications for public health and health policy. Nat. Rev. Neurol. 19, 109–117. doi: 10.1038/s41582-022-00763-1
Stuart, S., and Griffiths, L. R. (2012). A possible role for mitochondrial dysfunction in migraine. Mol. Genet. Genom. 287, 837–844. doi: 10.1007/s00438-012-0723-7
Stubberud, A., Buse, D. C., Kristoffersen, E. S., Linde, M., and Tronvik, E. (2021). Is there a causal relationship between stress and migraine? Current evidence and implications for management. J. Headache Pain 22:155. doi: 10.1186/s10194-021-01369-6
Takano, T., Tian, G.-F., Peng, W., Lou, N., Lovatt, D., Hansen, A. J., et al. (2007). Cortical spreading depression causes and coincides with tissue hypoxia. Nat. Neurosci. 10, 754–762. doi: 10.1038/nn1902
Tian, Y., Guo, S.-X., Li, J.-R., Du, H.-G., Wang, C.-H., Zhang, J.-M., et al. (2015). Topiramate attenuates early brain injury following subarachnoid haemorrhage in rats via duplex protection against inflammation and neuronal cell death. Brain Res. 1622, 174–185. doi: 10.1016/j.brainres.2015.06.007
Tripathi, G. M., Kalita, J., and Misra, U. K. (2018). A study of oxidative stress in migraine with special reference to prophylactic therapy. Int. J. Neurosci. 128, 318–324. doi: 10.1080/00207454.2017.1374959
Tuncel, D., Tolun, F. I., Gokce, M., İmrek, S., and Ekerbiçer, H. (2008). Oxidative stress in migraine with and without aura. Biol. Trace Elem. Res. 126, 92–97. doi: 10.1007/s12011-008-8193-9
Valencia-Olvera, A. C., Morán, J., Camacho-Carranza, R., Prospéro-García, O., and Espinosa-Aguirre, J. J. (2014). CYP2E1 induction leads to oxidative stress and cytotoxicity in glutathione-depleted cerebellar granule neurons. Toxicol. In Vitro 28, 1206–1214. doi: 10.1016/j.tiv.2014.05.014
van den Maagdenberg, A. M. J. M., Nyholt, D. R., and Anttila, V. (2019). Novel hypotheses emerging from GWAS in migraine? J. Headache Pain 20:5. doi: 10.1186/s10194-018-0956-x
Vgontzas, A., and Pavlović, J. M. (2018). Sleep disorders and migraine: Review of literature and potential pathophysiology mechanisms. Headache 58, 1030–1039. doi: 10.1111/head.13358
Vos, T., Lim, S. S., Abbafati, C., Abbas, K. M., Abbasi, M., Abbasifard, M., et al. (2020). Global burden of 369 diseases and injuries in 204 countries and territories, 1990–2019: A systematic analysis for the global burden of disease study 2019. Lancet 396, 1204–1222. doi: 10.1016/S0140-6736(20)30925-9
Wang, F., He, Q., Ren, Z., Li, F., Chen, W., Lin, X., et al. (2015). Association of serum levels of intercellular adhesion molecule-1 and interleukin-6 with migraine. Neurol. Sci. 36, 535–540. doi: 10.1007/s10072-014-2010-3
Wang, Y., Shan, Z., Zhang, L., Fan, S., Zhou, Y., Hu, L., et al. (2022). P2X7R/NLRP3 signaling pathway-mediated pyroptosis and neuroinflammation contributed to cognitive impairment in a mouse model of migraine. J. Headache Pain 23:75. doi: 10.1186/s10194-022-01442-8
White, H. S. (2005). Molecular pharmacology of topiramate: Managing seizures and preventing migraine. Headache J. Head Face Pain 45, S48–S56. doi: 10.1111/j.1526-4610.2005.4501006.x
Winsvold, B. S., Palta, P., Eising, E., Page, C. M., and International Headache Genetics Consortium (2018). Epigenetic DNA methylation changes associated with headache chronification: A retrospective case-control study. Cephalalgia Int. J. Headache 38, 312–322. doi: 10.1177/0333102417690111
Yamanaka, G., Suzuki, S., Morishita, N., Takeshita, M., Kanou, K., Takamatsu, T., et al. (2021). Experimental and clinical evidence of the effectiveness of riboflavin on migraines. Nutrients 13:2612. doi: 10.3390/nu13082612
Yang, Y.-H., Fan, X.-X., Ye, L., Huang, W.-J., and Ko, C.-Y. (2024). Examining the molecular mechanisms of topiramate in alleviating insulin resistance: A study on C2C12 myocytes and 3T3L-1 adipocytes. Endocrine 85, 168–180. doi: 10.1007/s12020-024-03706-6
Yeh, P.-K., An, Y.-C., Hung, K.-S., and Yang, F.-C. (2024). Influences of genetic and environmental factors on chronic migraine: A narrative review. Curr. Pain Headache Rep. 28, 169–180. doi: 10.1007/s11916-024-01228-4
Yu, S., He, M., Liu, R., Feng, J., Qiao, X., Yang, X., et al. (2013). Headache yesterday in China: A new approach to estimating the burden of headache, applied in a general-population survey in China. Cephalalgia 33, 1211–1217. doi: 10.1177/0333102413490347
Yu, S., Liu, R., Zhao, G., Yang, X., Qiao, X., Feng, J., et al. (2012). The prevalence and burden of primary headaches in China: A population-based door-to-door survey. Headache J. Head Face Pain 52, 582–591. doi: 10.1111/j.1526-4610.2011.02061.x
Yu, S.-Y., Cao, X.-T., Zhao, G., Yang, X.-S., Qiao, X.-Y., Fang, Y.-N., et al. (2011). The burden of headache in China: Validation of diagnostic questionnaire for a population-based survey. J. Headache Pain 12, 141–146. doi: 10.1007/s10194-011-0336-2
Zhang, L., Novick, D., Zhong, S., Li, J., Walker, C., Harrison, L., et al. (2023). Real-world analysis of clinical characteristics, treatment patterns, and patient-reported outcomes of insufficient responders and responders to prescribed acute migraine treatment in China. Pain Ther. 12, 751–769. doi: 10.1007/s40122-023-00494-1
Zhang, X., Levy, D., Kainz, V., Noseda, R., Jakubowski, M., and Burstein, R. (2011). Activation of central trigeminovascular neurons by cortical spreading depression. Ann. Neurol. 69, 855–865. doi: 10.1002/ana.22329
Keywords: topiramate, migraine, oxidative stress, neurogenic inflammation, ferroptosis
Citation: Chen Q-W, Meng R-T and Ko C-Y (2024) Modulating oxidative stress and neurogenic inflammation: the role of topiramate in migraine treatment. Front. Aging Neurosci. 16:1455858. doi: 10.3389/fnagi.2024.1455858
Received: 27 June 2024; Accepted: 19 September 2024;
Published: 02 October 2024.
Edited by:
Kimberly Stieglitz, Roxbury Community College, United StatesReviewed by:
Fawad Khan, Ochsner Health System, United StatesFaten Mohamed Ibrahim, National Research Centre, Egypt
Copyright © 2024 Chen, Meng and Ko. This is an open-access article distributed under the terms of the Creative Commons Attribution License (CC BY). The use, distribution or reproduction in other forums is permitted, provided the original author(s) and the copyright owner(s) are credited and that the original publication in this journal is cited, in accordance with accepted academic practice. No use, distribution or reproduction is permitted which does not comply with these terms.
*Correspondence: Chih-Yuan Ko, eXVhbm1vbW9rb0BnbWFpbC5jb20=
†ORCID: Qiao-Wen Chen, orcid.org/0009-0008-5135-2627; Run-Tian Meng, orcid.org/0009-0001-5777-474X; Chih-Yuan Ko, orcid.org/0000-0002-5767-3041