- 1Université Côte d'Azur, CNRS, BCL, Nice, France
- 2Brussels Centre for Language Studies (BCLS), Vrije Universiteit Brussel (VUB), Brussels, Belgium
- 3Center for Research in Cognitive Neuroscience (CRCN), ULB Neuroscience Institute (UNI), Université Libre de Bruxelles, Brussels, Belgium
Introduction: Recent research has recognized executive dysfunction as another component affected in Primary Progressive Aphasia (PPA). This systematic review aimed to examine what information distinctive neurophysiological markers can provide in the evaluation of executive function (EF) deficits in PPA, and to what effect executive function deficits can be assessed through the characteristics of functional markers.
Methods: We conducted a systematic literature search following the PRISMA guidelines across studies that employed neuropsychological assessments and neurophysiological imaging techniques (EEG, MEG; PET, SPECT, fMRI, fNIRS) to investigate executive dysfunction correlates in PPA.
Results: Findings from nine articles including a total number of 111 individuals with PPA met our inclusion criteria and were synthesized. Although research on the neural correlates of EF deficits is scarce, MEG studies revealed widespread oscillatory slowing, with increased delta and decreased alpha power, where alterations in alpha, theta, and beta activities were significant predictors of executive function deficits. PET findings demonstrated significant correlations between executive dysfunction and hypometabolism in frontal brain regions. fMRI results indicated elevated homotopic connectivity in PPA patients, with a broader and more anterior distribution of abnormal hippocampal connections of which were associated with reduced executive performance.
Conclusion: Our study provides indirect support for the assumption regarding the significance of the frontal regions and inferior frontal junction in executive control and demonstrates that neurophysiological tools can be a useful aid to further investigate clinical-neurophysiological correlations in PPA.
1 Introduction
Primary progressive aphasia (PPA) is a neurodegenerative condition characterized by the presence of a focal and progressive dementia that impacts the language network. PPA is a form of aphasia, an acquired language disorder that impairs the ability to understand and produce language and, consequently, to effectively communicate in daily living. Unlike post-stroke aphasia, PPA is progressive, meaning that language abilities impacted by the aphasic syndrome worsen in time as the disease progresses. The aetiological mechanisms underlying PPA often involve cortical and/or subcortical atrophy caused by Alzheimer’s disease (AD) type amyloidopathy, frontotemporal tauopathy, TDP-43 pathology, or α-synucleinopathy (usually found in Parkinson’s or Lewy body diseases). PPA is often characterized by clinical and neuropathological similarities to AD or frontotemporal lobe dementias; however, by contrast, aphasia is the most prominent and first occurring syndrome in PPA, as the degeneration particularly impacts the left hemisphere language network, including the perisylvian frontotemporal and parietal areas (Gorno-Tempini et al., 2011; Mesulam et al., 2009; Mesulam, 2001, 2003). Atrophy in these areas leads to language impairments commonly observed in aphasia, including difficulties with word retrieval, word finding, naming, repeating, and producing syntactically complex sentences (Gorno-Tempini et al., 2011). While a gradual decline in language abilities characterizes PPA, other cognitive functions are largely preserved in the initial stages of the disorder (Gorno-Tempini et al., 2011; Mesulam et al., 2009; Mesulam, 2001). According to the latest consensus formalized in 2011, there are currently three clinical variants of PPA diagnostically differentiated based on core linguistic, cognitive, and pathological profiles (Gorno-Tempini et al., 2011; see Table 1). A nonfluent/agrammatic variant (nfvPPA), a semantic variant (svPPA), and a logopenic variant (lvPPA). Although nfvPPA and svPPA often are associated with pathologies within the spectrum of frontotemporal lobar degeneration (FTLD; Mesulam et al., 2008; Snowden et al., 2007; Spinelli et al., 2017), and lvPPA more commonly with Alzheimer’s disease (AD) pathology (Grossman, 2010; Harris and Jones, 2014), a direct correspondence between pathology and clinical manifestations is not necessarily warranted.
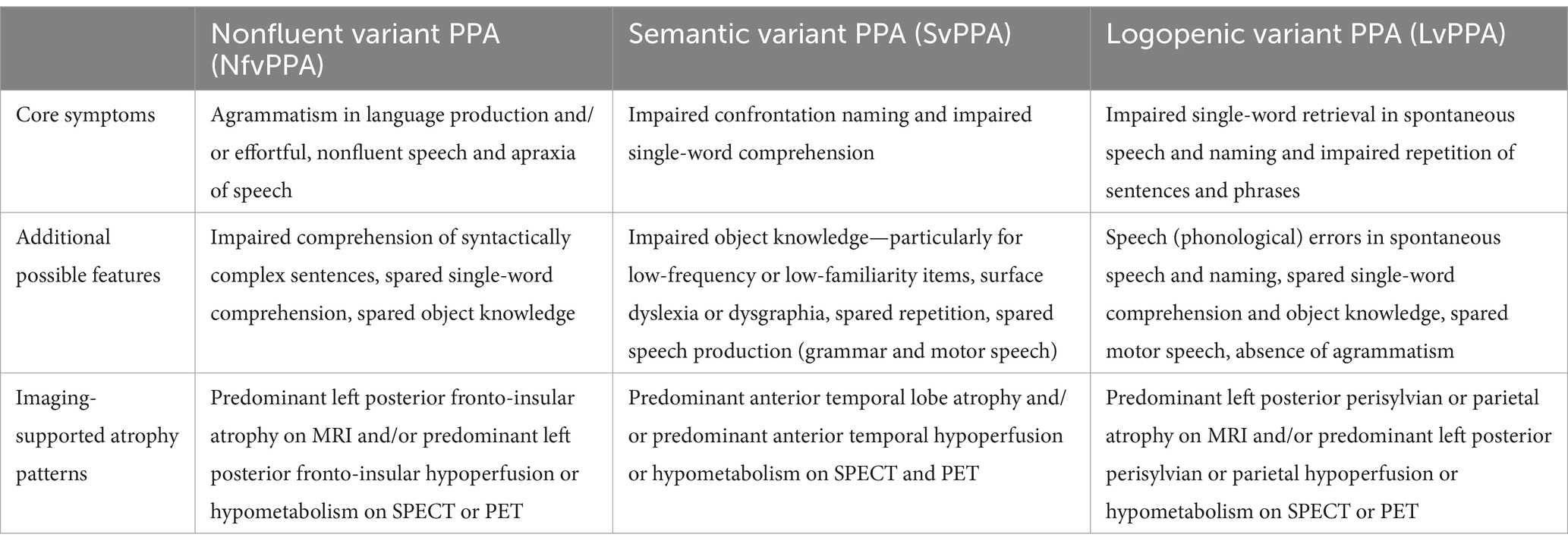
Table 1. The clinical diagnostic criteria for PPA variants (Modified from Gorno-Tempini et al., 2011).
1.1 Executive functions in PPA
While language impairment is the leading characteristic of PPA’s clinical profile, recent research has provided insight into another critical aspect of cognitive decline in individuals with PPA: the deterioration of executive functions (EFs; see also Coemans et al., 2022). Executive functioning refers to several higher-level cognitive abilities that regulate and control the brain’s lower-level cognitive operations to direct behavior toward an intended goal (Alvarez and Emory, 2006; Diamond, 2013; Miyake and Friedman, 2012). Engagement with these functions, also often referred to as executive or cognitive control, requires significant cognitive effort as it involves overriding automatic responses and behaviors. Neuroanatomically, EFs are mostly mediated by the prefrontal cortices and frontal regions, and deficits in these neural networks have been found to have significant implications for EF performance (Kramer et al., 2007; Friedman and Robbins, 2022). Executive processes have also been linked to structures beyond these frontal regions, such as frontoparietal networks (Reineberg et al., 2015), and to both anterior and posterior structures (Bettcher et al., 2016).
A range of models have been proposed to clarify the disposition of EFs. Therein, the ‘unity and diversity’ model established by Miyake and colleagues distinguishes three core EF components; (a) ‘Shifting between tasks or mental sets’ (henceforth shifting), (b) ‘Updating and monitoring of working memory representations’ (henceforth updating), and (c) ‘Inhibition of prepotent responses’ (henceforth inhibition; Miyake et al., 2000, pp. 55–57). According to the model, ‘unity’ refers to the idea that EFs are not entirely independent of each other but instead share some inherent similarities. ‘Diversity’ refers to the idea that each function holds its unique features—a concept shown through differential performance contributions on cognitive tasks. Hence, overall, the unity and diversity model posits how the three core EFs described are related but still separable.
As mentioned, non-linguistic cognitive domains have been assumed to remain intact in the initial phases of PPA, leading to a pervasive dismissal of these variables in research and diagnostic procedures (Mesulam, 2001). However, recent studies have found evidence for EF deficits in all three variants of PPA (Basaglia-Pappas et al., 2023; Coemans et al., 2022; Kamath et al., 2019). Therein, the reported data show impairments in all three EF components. For example, using Trail Making Test part B and Stroop Color-Word Test to assess shifting and inhibition, Chen et al. (2018) found svPPA patients to have impaired performance in both tasks compared to healthy controls. Within this study, svPPA patients with predominant atrophy in the left hemisphere further exhibited greater impairments on the Stroop test than those with right-hemisphere variant of svPPA. Inhibition, assessed with the Stroop task, has also been impaired in lvPPA patients (Matias-Guiu et al., 2019) and nfvPPA patients (Gajardo-Vidal et al., 2024).
Following the framework of Miyake and colleagues, Coemans et al. (2022) recently conducted a meta-analysis to systematically investigate measures of EFs in PPA. The authors first evaluated and categorized commonly used EF tasks based on their functional component classification. They found that many frequently reported EF tests are limited in their association with EFs due to their dependencies on a multifaceted range of cognitive abilities. Therein, task applicability was determined by the task characteristics’ alignment with the principles proposed by the unity and diversity model. Tasks reported to align with the Miyake model are provided in Table 2. Overall, their study demonstrated poorer performance of PPA patients as a group on the EFs composite (standing for the three components) but also for inhibition and shifting separately. However, they could only include effect sizes for ‘updating’ for six studies, making it the more underreported component, and this could possibly explain why the effects of this component did not always reach significance. PPA variant was a significant moderator of the effects found for EF composite analysis, with performance of lvPPA and nfvPPA being worse than that of svPPA. The authors linked these differences to the degeneration patterns and underlying neuropathologies that can be attested for the PPA variants. Disease duration was identified as a moderator for the overall EFs composite, as well as for shifting and inhibition separately. ‘Patient age at assessment’ was a significant moderator for the overall EFs composite as well as for shifting, but not for inhibition, suggesting that older patients with PPA performed more poorly on EF tests. Interestingly, task modality (i.e., verbal/non-verbal) was not found to influence the observed effects of executive performance deficits. This discovery, showing that task modality exerts no moderating effect on EF deficits in PPA is noteworthy, as it delineates these deficits as being inherent to EFs rather than a secondary outcome of language impairments. Additionally, it suggests that EF impairments can be reliably assessed through various task modalities, thereby supporting the robustness of these findings across conditions.
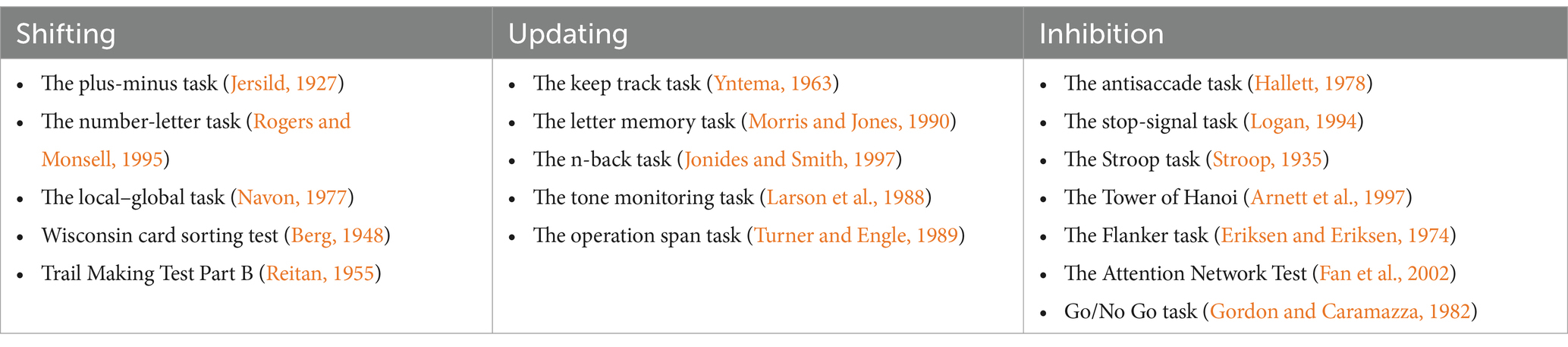
Table 2. Classification of tasks according to EF components (Modified from Coemans et al., 2022).
Moreover, two other meta-analyses further investigated measures of neuropsychological functions in PPA (Kamath et al., 2019, 2020). Kamath et al. (2019) comprehensively compared patients with nfvPPA, svPPA, and behavioral variant frontotemporal dementia (bv-FTD). The authors found similar effect sizes for executive dysfunction in nfvPPA and bv-FTD patients, highlighting a commonality in these variants’ impairments. However, svPPA patients exhibited a notably lower effect size for executive function deficits, indicating a distinction in cognitive profiles (Kamath et al., 2019). These findings are consistent with those of Coemans et al. (2022), who found comparable EF performance deficits in lvPPA and nfvPPA patients, which were further significantly worse than those of patients with svPPA. Kamath et al. (2020), having focused on lvPPA, observed that deficits in visual set-shifting were as conspicuous as language impairments in lvPPA patients. However, due to a limited number of applicable studies, a comprehensive assessment of other EF subdomains or direct comparisons with the other PPA variants were not possible within this study, emphasizing the need for further research on the cognitive intricacies of different PPA variants and their corresponding EF profiles.
1.2 Functional neuroimaging and neurophysiological techniques
Existing diagnostic criteria for PPA (Gorno-Tempini et al., 2011) are grounded in behavioral profiles. In the disorder’s early stages, these clinical manifestations can overlap between variants, making it difficult to differentiate them (Gorno-Tempini et al., 2008; Mesulam et al., 2014). However, emerging data shows a potential for variant differentiation by incorporating functional imaging and physiological techniques. In general, functional neuroimaging and related techniques attempt to measure neuronal activity. The applied techniques measure resting-state/baseline levels of brain activity or the changes in brain function that occur when actively engaging in specific tasks, allowing for the investigation of regions responsible for cognitive processes. However, the way these techniques are employed varies. Within PPA research, techniques such as magnetoencephalography (MEG) are employed to monitor changes in magnetic fields related to shifts in neurophysiological responses in the brain. Ranasinghe et al. (2014) and Shah-Basak et al. (2019) conducted studies focusing on the power spectra of neural oscillations in specific frequency ranges in PPA patients. Oscillatory bands refer to distinct frequency ranges of brain wave activity, each associated with various aspects of neural processing. These bands include delta (<4 Hz), theta (4–8 Hz), alpha (8–12 Hz), beta (12–30 Hz), gamma (30–80 Hz), and high gamma (>80 Hz). Their findings revealed a notable slowing in neural oscillatory activity, particularly characterized by increased delta and decreased alpha power, which not only served as a unique predictor for PPA but also significantly correlated with the severity of executive function deficits, as indicated by changes in alpha, theta, and beta activity.
Largely, neurodegenerative dementias exhibit specific disease-related metabolic reduction patterns (Ishii, 2014). Positron Emission Tomography (PET) is a neuroimaging tool that visualizes brain metabolic processes and pathologies by detecting gamma rays from radioactively labeled tracers. Hypometabolism, characterized by a decline in metabolic rate or activity in the brain, is indicated by reduced glucose uptake and utilization in specific brain regions, suggesting a decrease in neuronal activity or dysfunction in these areas. Within the scope of PPA research, Raczka et al. (2010) identified a significant correlation between executive dysfunction and hypometabolism in various frontomedian and left frontolateral regions, as well as the left insula, left globus pallidus, caudate, and thalamus. Complementing these findings, Desgranges et al. (2007) observed hypometabolism, correlating with moderate deficits in executive function abilities and behavioral changes in svPPA patients, predominantly in the orbitofrontal areas. Similarly, Schroeter et al. (2012) reported that executive deficits in PPA were primarily linked with prefrontal hypometabolism. Collectively, emphasizing a reliance on the frontal brain structures in PPA for executive control functions.
Functional Magnetic Resonance Imaging (fMRI) measures brain activity by observing changes in the brain’s vascular hemodynamic responses as an indirect measure of neuronal activity (Logothetis, 2008). In a study by Tao et al. (2022), patients with PPA exhibited significantly higher homotopic connectivity values. This suggests that there is an increased synchronized activity between homotopic regions, that is, structurally similar areas located symmetrically in opposite hemispheres of the brain. Initially, the PPA group demonstrated a more extensive and anteriorly distributed pattern of abnormal hyperconnectivity. This means that abnormal brain synchronization was not only higher, but also spread over a larger area – specifically, toward anterior brain regions. A pattern which indicates that the disruption in brain functions affects a range of brain regions, particularly those located toward frontal areas. For the significantly abnormal connections, there was a notable correlation between elevated functional connectivity (FC) and diminished executive performance, in this case, evidenced by prolonged reaction times in the Trail Making Test. This suggests that maintained EF task performance in PPA patients is associated with homotopic FC levels more akin to those observed in the control group.
1.3 The current review
Summarizing the available literature shows that, in contrast to the common belief, patients with PPA exhibit significant declines in EFs compared to healthy controls across EF composites (Coemans et al., 2022; Kamath et al., 2020). Notably, the extent of these deficits varies with PPA variant and disease duration (Basaglia-Pappas et al., 2023; Coemans et al., 2022; Kamath et al., 2019). Additionally, the impact of these deficits remains consistent regardless of task modality (Coemans et al., 2022), highlighting the need for further research to enhance diagnostic and treatment approaches in clinical practice. Given that PPA is a relatively rare neurocognitive condition with large individual differences, understanding the broader context is essential. Hence, this systematic review consolidates studies investigating EF dysfunction in PPA using electrophysiological and functional neuroimaging techniques to draw conclusions on functional markers of EF dysfunction in PPA.
Given the profound personal impact and clinical importance of EFs, the emerging evidence of their impairment in PPA, and the current fragmentation of functional neuroimaging findings, the objectives for this review are (1) to examine what information distinctive functional markers can provide in the evaluation of executive function deficits in PPA, and (2) to what effect executive function deficits can be assessed through the characteristics of functional markers.
2 Methods
2.1 Identification of articles and search strategies
This systematic review followed the Preferred Reporting for Systematic Reviews and Meta-analyses guidelines (PRISMA; Moher et al., 2009). Studies from the online publication databases PubMed and Web of Science were searched for literature relevant to the review, with the last search conducted in November 2023. Each title and abstract were scanned, and relevant articles reporting on functional neuroimaging in PPA were retrieved. Only full-text articles published in peer-reviewed journals in English were retained. The following search string was utilized for the systematic review in the databases PubMed and Web of Science:
(((Primary progressive aphas*) OR (PPA) OR (progressive aphasia) OR (semantic dementia) OR (semantic variant) OR (logopenic variant) OR (agrammatic variant) OR (nonfluent variant) OR (progressive nonfluent aphasia) OR (PNFA)) AND ((EEG) OR (electrophysiological) OR (MEG) OR (magnetoencephalography) OR (PET) OR (positron emission tomography) OR (fMRI) OR (SPECT) OR (single-photon emission computerised tomography) OR (fNIRS) OR (functional near-infrared spectroscopy)) AND ((executive function*) OR (executive control) OR (neuropsych*) OR (inhibit*) OR (shift*) OR (updat*)))
This search revealed 3,518 articles. Additionally, individual references were examined to obtain further relevant studies that were not accessible through the online search, equating to a total of 3,537 articles. Primary inclusion criteria for further analysis were (1) a PPA diagnosis, which was open to various diagnostic criteria and did not adhere to any one specific, standardized set of guidelines; (2) the inclusion of neuropsychological testing including the assessment of EF components, and (3) the reported use of one of the following functional assessments: electroencephalography (EEG), magnetoencephalography (MEG), positron emission tomography (PET), single-photon emission computerized tomography (SPECT), functional magnetic resonance imaging (fMRI) or functional near-infrared spectroscopy (fNIRS), see Figure 1 for the PRISMA flow diagram.
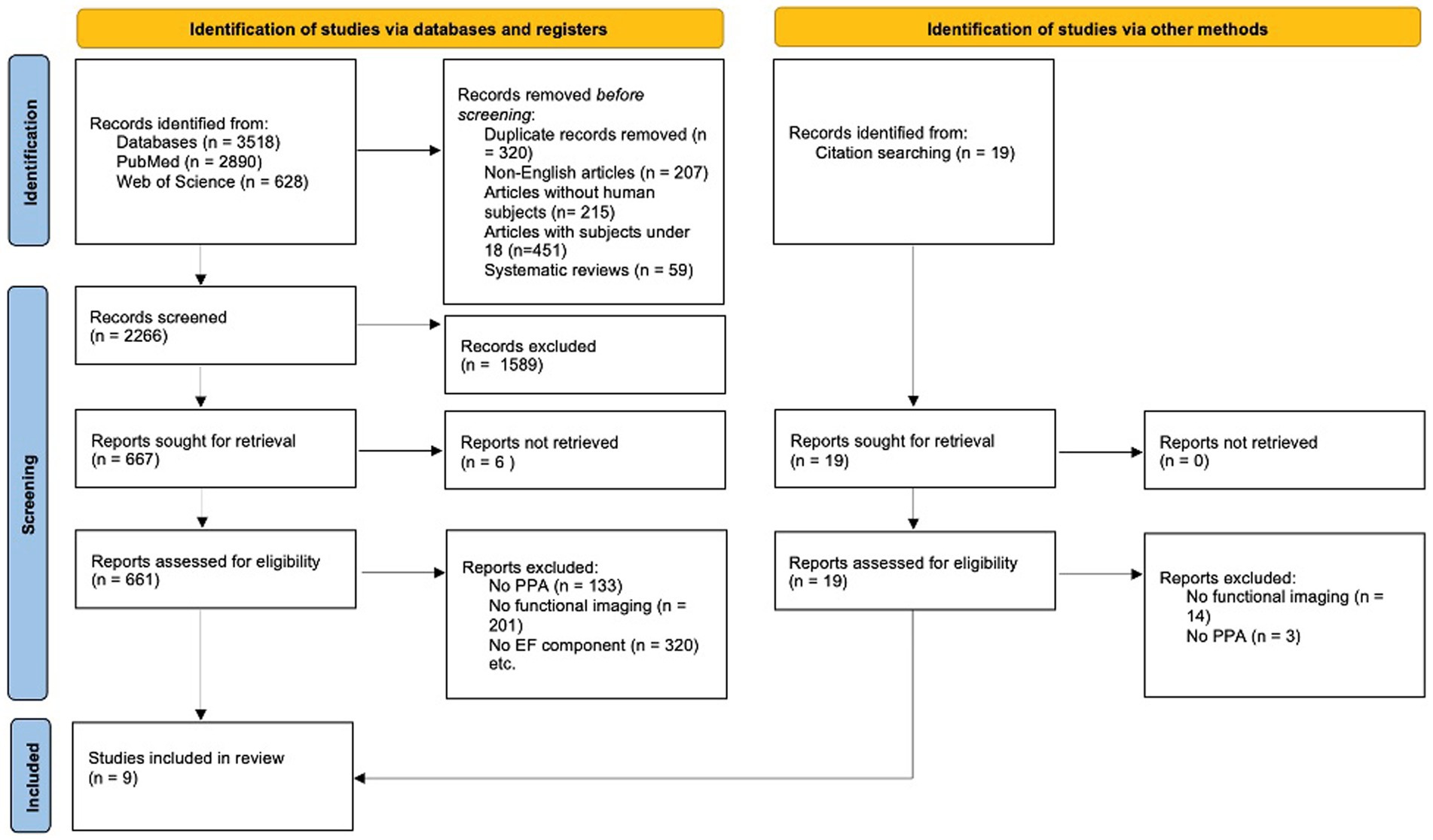
Figure 1. PRISMA flow diagram illustrating the process of study selection for the systematic review. The diagram outlines the number of records identified, included, and excluded at each review stage.
2.2 Data extraction
Baseline information was collected from each study; this included publication year and patient characteristics, namely, sex, age at examination, disease duration when provided, and employed diagnostic criteria for PPA; subtype is provided when identified. Additionally, the methodological approaches for each study were extracted. This included data on neuropsychological assessments, data analysis, and neurophysiological data.
3 Results
3.1 Study search and characteristics
The study characteristics from all nine studies are provided in Table 3. Hundred and twelve patients were included in the review (59 nfvPPA, 23 svPPA, 11 lvPPA, and 18 unidentified/mixed variants). Five studies included nfvPPA patients (Cooke et al., 2003; Mandelli et al., 2018; Shah-Basak et al., 2019; Tao et al., 2022), three studies included lvPPA patients (Ranasinghe et al., 2014; Schroeter et al., 2012; Shah-Basak et al., 2019), three studies included svPPA patients (Desgranges et al., 2007; Raczka et al., 2010; Schroeter et al., 2012), and two studies included undefined-PPA patients (Schroeter et al., 2012; Utianski et al., 2019). Six of the included studies employed the Gorno-Tempini et al. (2011) classification of PPA and its variants (Mandelli et al., 2018; Ranasinghe et al., 2014; Schroeter et al., 2012; Shah-Basak et al., 2019; Tao et al., 2022; Utianski et al., 2019), while four studied followed the Neary et al. (1998) diagnostic criteria for frontotemporal dementia (FTD) and its subtypes (Cooke et al., 2003; Desgranges et al., 2007; Raczka et al., 2010; Schroeter et al., 2012). The sex distribution was 51.8% female and 48.2% male, with two studies not disclosing this information. The age range of patients at examination in the included studies varied, ranging from 63.2 to 77.0 years per study, with the mean age across all included studies being 67.4 years. The mean duration of the disease post-onset was 3.4 years, ranging from 1.3 to 5.4 years; one study did not disclose this information (Cooke et al., 2003). Seven studies included age-matched or senior controls (Cooke et al., 2003; Desgranges et al., 2007; Mandelli et al., 2018; Raczka et al., 2010; Ranasinghe et al., 2014; Shah-Basak et al., 2019; Tao et al., 2022), while one study additionally included healthy younger controls (Shah-Basak et al., 2019). One study used neuropsychological data from Mayo’s Older Americans Normative Studies (MOANS) as a reference in place of a control group, providing age- and education-adjusted standard scores to assess patient performance (Utianski et al., 2019). In another study, the control group included individuals with subjective memory complaints that were not confirmed by neuropsychological and clinical evaluations. The subjects exhibited normal neuropsychological performance with only expected age-related cognitive decline. This control group was also utilized to avoid ethical concerns associated with using healthy subjects in [18F] fluorodeoxyglucose-PET studies due to radiation exposure (Schroeter et al., 2012).
3.2 EF measures
Several measures of EFs were used. Therein, studies utilized a combination of tests or basic neuropsychological batteries. The most common tests were the Trail making test-B or Modified trails (TMT-B; Reitan, 1955; Desgranges et al., 2007; Mandelli et al., 2018; Raczka et al., 2010; Ranasinghe et al., 2014; Shah-Basak et al., 2019; Tao et al., 2022; Utianski et al., 2019), and the Stroop test (Stroop, 1935; see Cooke et al., 2003; Desgranges et al., 2007; Raczka et al., 2010; Schroeter et al., 2012; Utianski et al., 2019), used to test shifting and inhibition, respectively. Other tests included the Running span tasks (Desgranges et al., 2007; Ranasinghe et al., 2014; Shah-Basak et al., 2019) and verbal fluency tasks, tests that arguably offer only a restricted assessment of EFs based on the theoretical framework of Miyake et al. (2000).
3.3 Neural correlates of EF measures
Ranasinghe et al. (2014) and Shah-Basak et al. (2019) investigated variations in the power spectra of neural oscillations within specific frequency ranges through MEG. They found that patients with PPA exhibited widespread oscillatory slowing. Notably, this slowing in oscillatory activity, especially the increase in delta and decrease in alpha power, was identified as a unique predictor for PPA. Furthermore, abnormalities or changes in the alpha, theta, and beta activity significantly predicted the severity level (EF scores) of executive function deficits in individuals with PPA. A summary of all findings is presented in Table 4.
The PET studies found hypometabolism consistent with a moderate deficit in EF abilities in SD patients to be concentrated explicitly in the orbitofrontal areas (Desgranges et al., 2007). This hypometabolism was more extensive than grey matter loss in the temporal lobes. Moreover, significant correlations between specific brain regions and principal components were found when looking into the neurodegenerative patterns of PPA-U (Utianski et al., 2019). Therein, principal components were based on EF test scores measured by the Trail Making Test Part B (TMT-B). The second principal component showed a positive correlation between the component and activity in the bilateral inferior frontal lobes, left sensorimotor region, and right cerebellum, contrasted by negative correlations in medial and lateral temporal lobes and lateral parietal lobes. The third principal component showed a positive correlation in the left lateral and medial temporal lobes, indicating asymmetry with minimal right hemisphere involvement, and negative correlations in sensorimotor and lateral parietal regions. Furthermore, significant links were drawn between executive dysfunction and hypometabolism in various frontomedian areas (including the anterior cingulate and mid cingulate gyrus, anterior medial frontal cortex), left frontolateral regions (such as the frontopolar cortex, inferior, middle, and superior frontal gyri), left insula, left globus pallidus, caudate, and thalamus (Raczka et al., 2010). This was further associated with executive dysfunction with hypometabolism in the left frontolateral cortices, encompassing the inferior frontal junction area, previously found to be involved with cognitive control (Jourdan Moser et al., 2009). In Schroeter et al. (2012) executive deficits were also predominantly associated with prefrontal hypometabolism. Overall, the inferior frontal junctions emerged as the most consistently significant regions across various EF tasks, highlighting executive control’s reliance on frontal brain structures.
EF measures of executive dysfunction explored through fMRI show that PPA patients have elevated homotopic values (Tao et al., 2022), meaning that the PPA group showed more synchronized activity across homotopic regions. At the baseline, the distribution of abnormal HC connections was more widespread and anterior in the PPA group. For the significantly abnormal connections, elevated FC values correlated with lower executive performance, in this case, through longer Trail reaction times, showing that better task performance was associated with homotopic FC levels closer to that of the control group. The study further included a calculation of structural damage to examine its relation to FC abnormalities as a global Grey matter volume (GMV) measure. This analysis showed that abnormal homotopic FC levels showed behavioral correlations consistent with their anatomical distribution - therein, medial frontal homotopic abnormalities were associated with EF performance in PPA. Mandelli et al. (2018) revealed a significant negative correlation between task performance and the FC between the right and left opercular inferior frontal gyrus (opIFG). Higher FC was linked to fewer errors, indicating better EF performance. No significant correlation was found with volume loss or nodal metrics within these nodes. Not all fMRI studies showed significant correlations between PPA and executive function deficits. One study only found significant EF impairments for a group of five non-aphasic patients with dysexecutive and social impairments (EXEC; Cooke et al., 2003); however, additional recruitment of medial frontal regions was found in the nfvPPA variant.
3.4 Methodological approaches
3.4.1 Data acquisition and processing
Two studies employed MEG to record neural oscillatory activity (Ranasinghe et al., 2014; Shah-Basak et al., 2019) while simultaneously incorporating T1-weighted MRI scans for MEG source modeling. MEG source analyses were conducted by computing power spectral densities using the multitaper method with a focus on delta, theta, alpha, and beta frequency bands (Shah-Basak et al., 2019) and functional connectivity analyses focusing on the alpha frequency band of specific data epochs (Ranasinghe et al., 2014).
Four studies utilized fluorodeoxyglucose PET (FDG-PET; Desgranges et al., 2007; Raczka et al., 2010; Schroeter et al., 2012; Utianski et al., 2019). Some controlled for environmental influences by minimizing external stimuli and body movement in their methodological approach (Desgranges et al., 2007; Utianski et al., 2019), while the other PET studies did not report on this (Raczka et al., 2010; Schroeter et al., 2012). The typical doses of 18FDG utilized ranged from 367 to 576 MBq (Raczka et al., 2010; Schroeter et al., 2012; Utianski et al., 2019), while one study used a smaller dose of 111 to 185 MBq (Desgranges et al., 2007). In the studies, dynamic scans were conducted, with each study adopting different protocols for the timing of image acquisition post-injection, predominantly a 10-min after a 30–50-min uptake period. Statistical Parametric Mapping for voxel-based analysis (Raczka et al., 2010; Schroeter et al., 2012) and 3-dimensional stereotactic surface projections were employed to assess hypometabolism (Utianski et al., 2019).
Three studies utilized fMRI using a 3 T scanner (Cooke et al., 2003; Mandelli et al., 2018; Tao et al., 2022). Functional connectivity (FC) was calculated using pre-processed time-series data. FC estimation included Pearson’s correlation and Fisher’s z-transform, with the functional connectomes subsequently averaged.
3.4.2 Statistical analysis
Statistical analyses included using Pearson’s correlation and FDR correction (Ranasinghe et al., 2014), ANOVAs and post-hoc t-tests with Holm’s correction for group comparisons (Shah-Basak et al., 2019), Spearman tests and ROI analysis (Desgranges et al., 2007), general linear models with significance determined by Gaussian fields theory (Raczka et al., 2010; Schroeter et al., 2012), PCA and Spearman’s method (Utianski et al., 2019), and linear regression, and FDR adjustment, while also exploring the impact on behavioral scores with regression analyses (Tao et al., 2022).
4 Discussion
The clinical profile of PPA and its subtypes is predominately focused on isolated deficits in language domains. In diagnostic guidelines, the loss of speech and other language symptoms are the main causes of impaired daily functioning in individuals with PPA, while other cognitive abilities such as EFs have been described to remain relatively intact in the early stages of the disorder (Mesulam, 2001; Mesulam et al., 2009; Gorno-Tempini et al., 2011). Thus, currently, the executive profile of PPA is not considered under the consensual diagnostic criteria (i.e., Gorno-Tempini et al., 2011) and has rarely been a primary focus of research in this population. However, though language abilities remain the most salient aspect of impairment, executive dysfunction related to all variants of PPA has been reported (see Coemans et al., 2022), even in the early stages. The present systematic review aimed to examine what information functional markers provide in the evaluation of EF deficits in PPA and to what effect EF deficits can be assessed through the characteristics of functional markers. While the existing literature on neurophysiological imaging modalities concerning PPA is scarce, studies examining the neural correlates of executive dysfunction were identified for three neurophysiological techniques, MEG, PET, and fMRI. Overall, the findings of this review add to the argument of affected EFs in individuals diagnosed with PPA, further challenging the prevailing assumption of mostly unaffected EFs in this population. In the following sections, the relationship between EF deficits in PPA and their neural correlates will be discussed, focusing on the EF components shifting, updating, and inhibition and how these deficits are reflected through changes in neural activity patterns across distinct brain regions.
4.1 Neural correlates associated with EF deficits
A summary of EF impairments in PPA and associated neural correlates can be found in Table 5. Several brain regions were linked to EF deficits in individuals with PPA, including the orbitofrontal areas, frontomedian areas, left frontolateral regions, left insula, left globus pallidus, caudate, and thalamus. These findings suggest that specific neural networks are implicated in EFs, with significant overlap in regions traditionally associated with language processing, particularly in the frontal and temporal lobes. This indicates that EF deficits in PPA may not be entirely separable from language impairments, as both components rely on interconnected neural connections. Therein, the extent of executive dysfunction varies across PPA variants, including nfvPPA, svPPA, lvPPA, and U-PPA, suggesting variant-specific patterns of neural degeneration that impact both language and EFs.
4.2 Alignment of EF deficits in PPA with the unity and diversity model
The findings from 111 PPA patients reveal significant impairments in EFs when compared to healthy controls across all three EF composites conceptualized by Miyake et al. (2000). However, to what extent these deficits can be directly attributed to executive impairments or are secondary to language impairments remains a critical question. While multiple studies have included tests for the neural correlates of ‘shifting’ and ‘inhibition’, the component ‘updating’ is relatively underrepresented in existing research. This discrepancy could be due to the inherent challenges in isolating updating processes from other cognitive functions, such as working memory, limiting our comprehension of how PPA affects the spectrum of EFs in variants of PPA. Primarily, studies applied the TMT-B and Stroop Test, which, according to the Miyake et al. (2000) framework, aligns with the shifting and inhibition components of EFs. Therefore, the relative underrepresentation of the updating component in PPA demonstrates the necessity for further investigation into the component by employing tasks that more accurately measure these distinct processes. Moreover, The Digit Span Backwards and Running Span tasks have frequently been used to test updating. However, as these tasks focus more strongly on working memory capacities rather than the continuous processes of renewing and replacing information, they do not effectively align with the principles of the Unity and Diversity model. This misalignment shows a critical need for incorporating different tasks that can more accurately reflect the updating component, such as the n-back task, which directly challenges the ability to continuously update information. Nonetheless, it is important to note that research is currently limited for all three EF components.
4.3 Neurophysiological insights into PPA’s executive deficits
In the current review, MEG studies, detecting changes in brain activity with high temporal accuracy, highlighted the presence of a widespread oscillatory slowing in PPA. Therein, EF abilities were impaired, and the severity of these deficits was associated with an increase in delta, theta and alpha power, and decreased beta power, providing a predictor for executive dysfunction through oscillatory abnormalities. Nonetheless, the processes underlying these abnormal oscillatory activities are not well understood, indicating a need for further research. PET imaging revealed hypometabolism in brain regions associated with executive control, such as the frontomedian and left frontolateral regions (Desgranges et al., 2007; Raczka et al., 2010). This hypometabolism, or reduced metabolic activity was further correlated with executive dysfunction. The role of the frontomedial and frontolateral regions, specifically the anterior cingulate cortex and the inferior frontal junction, is commonly associated with cognitive control and EF processes (Brass et al., 2005; Ridderinkhof et al., 2004). In individuals with AD, PET scans have shown a reduced uptake of glucose in certain brain regions as a reflection of decreased neural activity (Camandola and Mattson, 2017; Hoyer, 1985) and worsening hypometabolism has been linked to both progressive cognitive and functional decline (Landau et al., 2011). Additionally, the spatial resolution of fMRI demonstrates abnormal functional connectivity patterns, particularly increased synchronized activity across homotopic regions, which correlated with reduced executive performance (Tao et al., 2022). Abnormalities or changes in homotopic connectivity can be indicative of alterations in functional processes, in the association with neurological or neurodegenerative conditions, or the outcomes of brain injury. Considering these modalities, electrophysiological techniques such as electroencephalography (EEG) could offer additional insights into the neural mechanisms underlying EF deficits in PPA. This is because the high temporal resolution of EEG combined with its accessibility makes it a suitable method for examining the neural dynamics associated with executive dysfunction. Furthermore, a multimodal imaging approach, combining neurophysiological imaging such as MEG, PET, fMRI, or EEG with structural imaging, would offer a more comprehensive understanding of cognitive impairments in PPA.
4.4 Methodological considerations
As a reflection of the rarity of PPA and its variants, the sample sizes in all the reviewed studies were relatively small. This limitation makes it challenging to generalize findings across the PPA variants. PPA typically presents heterogeneity in symptoms; thus, a single-case approach could offer a profound understanding of the relationship between EF deficits and neural correlates by allowing for a more detailed examination of individual differences, thereby providing insights that might be obscured in larger studies. Nonetheless, group studies allowing for the implementation of advanced statistical methods to facilitate comparisons across PPA variants would still optimally surpass single-case approaches by enhancing the generalizability and robustness of findings. Another promising methodological approach identified in the reviewed papers includes PCA analysis, which captures the variability in EF performance and neurophysiological imaging within a small cohort of patients.
4.5 Limitations
This review is not without any limitations. Firstly, as the literature on the neural correlates of executive dysfunction is scarce, only nine studies met the inclusion criteria for the systematic review. Within these studies, the assessment of EFs was based on correlations between EF task performance and neural correlates; no direct task-based activity was available. Secondly, there is a lack of consensus on what task domains unequivocally can be part of executive functioning. There is currently no established standard for defining and evaluating EFs, leading to variability in research findings and task selection across clinical assessments. This review only included studies that used tasks in line with the characteristics of EF components defined by Miyake et al. (2000). However, it is common for studies to report tasks as being indicative of executive functions, though the criteria for how and/or whether these tasks relate to executive processes remain unclear. Therein, it has been argued that given that EFs are not a uniform concept, there has been a lack of a clear standard measure in research, which is essential for comparing and evaluating potential EF measures. A limitation of this review is the underrepresentation of the updating component of executive functions, with most studies focusing on shifting and inhibition, which limits a comprehensive understanding of all EF components in PPA. This is recurrently reported as a limitation (Coemans et al., 2022) and diagnostic practice could benefit from a deeper understanding of PPA (variant) performance on this component. Different diagnostic criteria (Gorno-Tempini et al., 2011; Neary et al., 1998) were used to classify PPA variants within the studies of this systematic review as an unfortunate result of differences in publication dates, potentially affecting comparability across studies. However, although future studies should look at correlations between PPA subtype and executive dysfunctions, there remains to be clear merit in investigating the PPA population as a whole. Another limitation of our study is the inclusion of studies that used composite scores derived from multiple tasks, including tasks of less relevance to EF, which may reduce the ability to clearly link results to each specific executive function component. Moreover, though the review identified positive associations between EFs and neural correlates in PPA, these associations can only be interpreted as indicative. Additionally, in considering the patient groups, the three variants of PPA may show differing characteristics; however, not all included studies separated PPA variants in their analyses. Moreover, the studies included in our analysis did not provide pathological diagnoses for patients; the data provided were based primarily on clinical diagnoses. The functional neuroimaging studies on individuals with PPA are currently very few. This limits us from contemplating further on the functional markers using these techniques, however, as these kinds of functional neuroimaging studies are becoming more widespread, a future study using Coordinate-Based Meta-Analysis would be insightful. Lastly, another limitation of the study is the use of different control groups, including individuals with subjective memory complaints and normative data, which potentially affect the comparability across studies.
5 Conclusion
Though further research is still needed to assess underlying functional mechanisms related to PPA pathology, the present study suggests that reductions in functional connectivity may indicate deficits in the left hemisphere neural networks related to cognitive functions. Although neural differences are complex and difficult to pinpoint to cognitive mechanisms with certainty, the findings support the continued incorporation of all functional assessments of executive dysfunction for PPA patients. Additionally, with widely differencing definitions (or the lack thereof) across studies of what EFs encompass, there is a need for a consensus for EF functions and their assessments. Still, our study indirectly supports Miyake et al.'s (2000) assumption of the frontal regions and inferior frontal junctions relevance for executive control. Moreover, our study suggests that the FTLD spectrum is associated with dysfunction in this area.
Data availability statement
The original contributions presented in the study are included in the article/supplementary material, further inquiries can be directed to the corresponding author.
Author contributions
KT: Conceptualization, Methodology, Writing – original draft, Writing – review & editing. SK: Conceptualization, Writing – original draft, Writing – review & editing. SA: Conceptualization, Writing – original draft, Writing – review & editing.
Funding
The author(s) declare financial support was received for the research, authorship, and/or publication of this article. KT was supported by UCAJEDI Investments in the Future project managed by the National Research Agency (ANR) with the reference number ANR-15-IDEX-01. SA was supported by funding from the European Union’s Horizon 2020 research and innovation programme under a Marie Skłodowska-Curie grant [agreement no. 838602].
Conflict of interest
The authors declare that the research was conducted in the absence of any commercial or financial relationships that could be construed as a potential conflict of interest.
Publisher’s note
All claims expressed in this article are solely those of the authors and do not necessarily represent those of their affiliated organizations, or those of the publisher, the editors and the reviewers. Any product that may be evaluated in this article, or claim that may be made by its manufacturer, is not guaranteed or endorsed by the publisher.
References
Alvarez, J. A., and Emory, E. (2006). Executive function and the frontal lobes: a Meta-analytic review. Neuropsychol. Rev. 16, 17–42. doi: 10.1007/s11065-006-9002-x
Arnett, P. A., Rao, S. M., Grafman, J., Bernardin, L., Luchetta, T., Binder, J. R., et al. (1997). Executive functions in multiple sclerosis: an analysis of temporal ordering, semantic encoding, and planning abilities. Neuropsychology 11, 535–544. doi: 10.1037//0894-4105.11.4.535
Basaglia-Pappas, S., Laurent, B., Getenet, J.-C., Boulangé, A., Rendón de laCruz, A., Simoes Loureiro, I., et al. (2023). Executive profile of the Logopenic variant of primary progressive aphasia: comparison with the semantic and non-fluent variants and Alzheimer’s disease. Brain Sci. 13:406. doi: 10.3390/brainsci13030406
Berg, E. A. (1948). A simple objective technique for measuring flexibility in thinking. J. Gen. Psychol. 39, 15–22. doi: 10.1080/00221309.1948.9918159
Bettcher, B. M., Mungas, D., Patel, N., Elofson, J., Dutt, S., Wynn, M., et al. (2016). Neuroanatomical substrates of executive functions: beyond prefrontal structures. Neuropsychologia 85, 100–109. doi: 10.1016/j.neuropsychologia.2016.03.001
Brass, M., Derrfuss, J., Forstmann, B., and von Cramon, D. Y. (2005). The role of the inferior frontal junction area in cognitive control. Trends Cogn. Sci. 9, 314–316. doi: 10.1016/j.tics.2005.05.001
Camandola, S., and Mattson, M. P. (2017). Brain metabolism in health, aging, and neurodegeneration. EMBO J. 36, 1474–1492. doi: 10.15252/embj.201695810
Chen, K., Ding, J., Lin, B., Huang, L., Tang, L., Bi, Y., et al. (2018). The neuropsychological profiles and semantic-critical regions of right semantic dementia. NeuroImage. Clin. 19, 767–774. doi: 10.1016/j.nicl.2018.05.035
Clinical and Neuropathological Criteria for Frontotemporal Dementia (1994). The Lund and Manchester Groups. J Neurol Neurosurg Psychiatry, 57, 416–418. doi: 10.1136/jnnp.57.4.416
Coemans, S., Keulen, S., Savieri, P., Tsapkini, K., Engelborghs, S., Chrispeels, N., et al. (2022). Executive functions in primary progressive aphasia: a meta-analysis. Cortex 157, 304–322. doi: 10.1016/j.cortex.2022.10.001
Cooke, A., DeVita, C., Gee, J., Alsop, D., Detre, J., Chen, W., et al. (2003). Neural basis for sentence comprehension deficits in frontotemporal dementia. Brain Lang. 85, 211–221. doi: 10.1016/s0093-934x(02)00562-x
Desgranges, B., Matuszewski, V., Piolino, P., Chételat, G., Mézenge, F., Landeau, B., et al. (2007). Anatomical and functional alterations in semantic dementia: a voxel-based MRI and PET study. Neurobiol. Aging 28, 1904–1913. doi: 10.1016/j.neurobiolaging.2006.08.006
Diamond, A. (2013). Executive functions. Annu. Rev. Psychol. 64, 135–168. doi: 10.1146/annurev-psych-113011-143750
Eriksen, B. A., and Eriksen, C. W. (1974). Effects of noise letters upon the identification of a target letter in a nonsearch task. Percept. Psychophys. 16, 143–149. doi: 10.3758/BF03203267
Fan, J., McCandliss, B. D., Sommer, T., Raz, A., and Posner, M. I. (2002). Testing the efficiency and independence of attentional networks. J. Cogn. Neurosci. 14, 340–347. doi: 10.1162/089892902317361886
Friedman, N. P., and Robbins, T. W. (2022). The role of prefrontal cortex in cognitive control and executive function. Neuropsychopharmacology 47, 72–89. doi: 10.1038/s41386-021-01132-0
Gajardo-Vidal, A., Montembeault, M., Lorca-Puls, D. L., Licata, A. E., Bogley, R., Erlhoff, S., et al. (2024). Assessing processing speed and its neural correlates in the three variants of primary progressive aphasia with a non-verbal tablet-based task. Cortex 171, 165–177. doi: 10.1016/j.cortex.2023.10.011
Gordon, B., and Caramazza, A. (1982). Lexical decision for open- and closed-class words: failure to replicate differential frequency sensitivity. Brain Lang. 15, 143–160. doi: 10.1016/0093-934x(82)90053-0
Gorno-Tempini, M. L., Brambati, S. M., Ginex, V., Ogar, J., Dronkers, N. F., Marcone, A., et al. (2008). The logopenic/phonological variant of primary progressive aphasia. Neurology 71, 1227–1234. doi: 10.1212/01.wnl.0000320506.79811.da
Gorno-Tempini, M. L., Hillis, A. E., Weintraub, S., Kertesz, A., Mendez, M., Cappa, S. F., et al. (2011). Classification of primary progressive aphasia and its variants. Neurology 76, 1006–1014. doi: 10.1212/WNL.0b013e31821103e6
Grossman, M. (2010). Primary progressive aphasia: Clinicopathological correlations. Nat. Rev. Neurol. 6, 88–97. doi: 10.1038/nrneurol.2009.216
Hallett, P. E. (1978). Primary and secondary saccades to goals defined by instructions. Vis. Res. 18, 1279–1296. doi: 10.1016/0042-6989(78)90218-3
Harris, J. M., and Jones, M. (2014). Pathology in primary progressive aphasia syndromes. Curr. Neurol. Neurosci. Rep. 14:466. doi: 10.1007/s11910-014-0466-4
Hoyer, S. (1985). The effect of age on glucose and energy metabolism in brain cortex of rats. Arch. Gerontol. Geriatr. 4, 193–203. doi: 10.1016/0167-4943(85)90001-9
Ishii, K. (2014). PET approaches for diagnosis of dementia. Am. J. Neuroradiol. 35, 2030–2038. doi: 10.3174/ajnr.A3695
Jonides, J., and Smith, E. E. (1997). “The architecture of working memory” in Cognitive neuroscience (Cambridge, MA, US: The MIT Press), 243–276.
Jourdan Moser, S., Cutini, S., Weber, P., and Schroeter, M. L. (2009). Right prefrontal brain activation due to Stroop interference is altered in attention-deficit hyperactivity disorder—a functional near-infrared spectroscopy study. Psychiatry Res. 173, 190–195. doi: 10.1016/j.pscychresns.2008.10.003
Kamath, V., Chaney, G.-A. S., DeRight, J., and Onyike, C. U. (2019). A meta-analysis of neuropsychological, social cognitive, and olfactory functioning in the behavioral and language variants of frontotemporal dementia. Psychol. Med. 49, 2669–2680. doi: 10.1017/S0033291718003604
Kamath, V., Sutherland, E. R., and Chaney, G.-A. (2020). A Meta-analysis of neuropsychological functioning in the Logopenic variant of primary progressive aphasia: comparison with the semantic and non-fluent variants. J. Int. Neuropsychol. Soc. 26, 322–330. doi: 10.1017/S1355617719001115
Kramer, J. H., Quitania, L., Dean, D., Neuhaus, J., Rosen, H. J., Halabi, C., et al. (2007). Magnetic resonance imaging correlates of set shifting. J. Int. Neuropsychol. Soc. 13, 386–392. doi: 10.1017/S1355617707070567
Landau, S. M., Harvey, D., Madison, C. M., Koeppe, R. A., Reiman, E. M., Foster, N. L., et al. (2011). Associations between cognitive, functional, and FDG-PET measures of decline in AD and MCI. Neurobiol. Aging 32, 1207–1218. doi: 10.1016/j.neurobiolaging.2009.07.002
Larson, G. E., Merritt, C. R., and Williams, S. E. (1988). Information processing and intelligence: some implications of task complexity. Intelligence 12, 131–147. doi: 10.1016/0160-2896(88)90012-8
Logan, G. D. (1994). “On the ability to inhibit thought and action: a users’ guide to the stop signal paradigm” in Inhibitory processes in attention, memory, and language. Eds. Dale Dagenbach, Thomas H. Carr. (San Diego: Academic Press), 189–239.
Logothetis, N. K. (2008). What we can do and what we cannot do with fMRI. Nature 453, 869–878. doi: 10.1038/nature06976
Mandelli, M. L., Welch, A. E., Vilaplana, E., Watson, C., Battistella, G., Brown, J. A., et al. (2018). Altered topology of the functional speech production network in non-fluent/agrammatic variant of PPA. Cortex 108, 252–264. doi: 10.1016/j.cortex.2018.08.002
Matias-Guiu, J. A., Díaz-Álvarez, J., Cuetos, F., Cabrera-Martín, M. N., Segovia-Ríos, I., Pytel, V., et al. (2019). Machine learning in the clinical and language characterisation of primary progressive aphasia variants. Cortex 119, 312–323. doi: 10.1016/j.cortex.2019.05.007
Mesulam, M.-M. (2003). Primary progressive aphasia—a language-based dementia. N. Engl. J. Med. 349, 1535–1542. doi: 10.1056/NEJMra022435
Mesulam, M.-M., Rogalski, E. J., Wieneke, C., Hurley, R. S., Geula, C., Bigio, E. H., et al. (2014). Primary progressive aphasia and the evolving neurology of the language network. Nat. Rev. Neurol. 10, 554–569. doi: 10.1038/nrneurol.2014.159
Mesulam, M., Wicklund, A., Johnson, N., Rogalski, E., Léger, G. C., Rademaker, A., et al. (2008). Alzheimer and frontotemporal pathology in subsets of primary progressive aphasia. Ann. Neurol. 63, 709–719. doi: 10.1002/ana.21388
Mesulam, M., Wieneke, C., Rogalski, E., Cobia, D., Thompson, C., and Weintraub, S. (2009). Quantitative template for subtyping primary progressive aphasia. Arch. Neurol. 66, 1545–1551. doi: 10.1001/archneurol.2009.288
Miyake, A., and Friedman, N. P. (2012). The nature and Organization of Individual Differences in executive functions: four general conclusions. Curr. Dir. Psychol. Sci. 21, 8–14. doi: 10.1177/0963721411429458
Miyake, A., Friedman, N. P., Emerson, M. J., Witzki, A. H., Howerter, A., and Wager, T. D. (2000). The Unity and Diversity of executive functions and their contributions to complex “frontal lobe” tasks: a latent variable analysis. Cogn. Psychol. 41, 49–100. doi: 10.1006/cogp.1999.0734
Moher, D., Liberati, A., Tetzlaff, J., and Altman, D. G.PRISMA Group (2009). Preferred reporting items for systematic reviews and meta-analyses: the PRISMA statement. PLoS Med. 6:e1000097. doi: 10.1371/journal.pmed.1000097
Morris, N., and Jones, D. M. (1990). Memory updating in working memory: the role of the central executive. Br. J. Psychol. 81, 111–121. doi: 10.1111/j.2044-8295.1990.tb02349.x
Navon, D. (1977). Forest before trees: the precedence of global features in visual perception. Cogn. Psychol. 9, 353–383. doi: 10.1016/0010-0285(77)90012-3
Neary, D., Snowden, J., Gustafson, L., Passant, U., Stuss, D., Black, S., et al. (1998). Frontotemporal lobar degeneration—A consensus on clinical diagnostic criteria. Neurology 51, 1546–1554. doi: 10.1212/WNL.51.6.1546
Raczka, K. A., Becker, G., Seese, A., Frisch, S., Heiner, S., Marschhauser, A., et al. (2010). Executive and behavioral deficits share common neural substrates in frontotemporal lobar degeneration—a pilot FDG-PET study. Psychiatry Res. 182, 274–280. doi: 10.1016/j.pscychresns.2010.02.009
Ranasinghe, K. G., Hinkley, L. B., Beagle, A. J., Mizuiri, D., Dowling, A. F., Honma, S. M., et al. (2014). Regional functional connectivity predicts distinct cognitive impairments in Alzheimer’s disease spectrum. NeuroImage. Clinical 5, 385–395. doi: 10.1016/j.nicl.2014.07.006
Reineberg, A. E., Andrews-Hanna, J. R., Depue, B., Friedman, N. P., and Banich, M. T. (2015). Resting-state networks predict individual differences in common and specific aspects of executive function. NeuroImage 104, 69–78. doi: 10.1016/j.neuroimage.2014.09.045
Reitan, R. M. (1955). The relation of the trail making test to organic brain damage. J. Consult. Psychol. 19, 393–394. doi: 10.1037/h0044509
Ridderinkhof, K. R., Ullsperger, M., Crone, E. A., and Nieuwenhuis, S. (2004). The role of the medial frontal cortex in cognitive control. Science 306, 443–447. doi: 10.1126/science.1100301
Rogers, R. D., and Monsell, S. (1995). Costs of a predictible switch between simple cognitive tasks. J. Exp. Psychol. Gen. 124, 207–231. doi: 10.1037/0096-3445.124.2.207
Schroeter, M. L., Vogt, B., Frisch, S., Becker, G., Barthel, H., Mueller, K., et al. (2012). Executive deficits are related to the inferior frontal junction in early dementia. Brain 135, 201–215. doi: 10.1093/brain/awr311
Shah-Basak, P. P., Kielar, A., Deschamps, T., Verhoeff, N. P., Jokel, R., and Meltzer, J. (2019). Spontaneous oscillatory markers of cognitive status in two forms of dementia. Hum. Brain Mapp. 40, 1594–1607. doi: 10.1002/hbm.24470
Snowden, J., Neary, D., and Mann, D. (2007). Frontotemporal lobar degeneration: clinical and pathological relationships. Acta Neuropathol. 114, 31–38. doi: 10.1007/s00401-007-0236-3
Spinelli, E. G., Mandelli, M. L., Miller, Z. A., Santos-Santos, M. A., Wilson, S. M., Agosta, F., et al. (2017). Typical and atypical pathology in primary progressive aphasia variants. Ann. Neurol. 81, 430–443. doi: 10.1002/ana.24885
Stroop, J. R. (1935). Studies of interference in serial verbal reactions. J. Exp. Psychol. 18, 643–662. doi: 10.1037/h0054651
Tao, Y., Tsapkini, K., and Rapp, B. (2022). Inter-hemispheric synchronicity and symmetry: the functional connectivity consequences of stroke and neurodegenerative disease. NeuroImage Clin. 36:103263. doi: 10.1016/j.nicl.2022.103263
Turner, M. L., and Engle, R. W. (1989). Is working memory capacity task dependent? J. Mem. Lang. 28, 127–154. doi: 10.1016/0749-596X(89)90040-5
Utianski, R. L., Botha, H., Martin, P. R., Schwarz, C. G., Duffy, J. R., Clark, H. M., et al. (2019). Clinical and neuroimaging characteristics of clinically unclassifiable primary progressive aphasia. Brain Lang. 197:104676. doi: 10.1016/j.bandl.2019.104676
Keywords: primary progressive aphasia, executive functions, neuropsychology, neurophysiological imaging, MEG, PET, fMRI
Citation: Thomsen K, Keulen S and Arslan S (2024) Functional correlates of executive dysfunction in primary progressive aphasia: a systematic review. Front. Aging Neurosci. 16:1448214. doi: 10.3389/fnagi.2024.1448214
Edited by:
Emilia Vitale, National Research Council (CNR), ItalyReviewed by:
Edoardo Gioele Spinelli, Vita-Salute San Raffaele University, ItalyJuan F. Cardona, University of the Valley, Colombia
Copyright © 2024 Thomsen, Keulen and Arslan. This is an open-access article distributed under the terms of the Creative Commons Attribution License (CC BY). The use, distribution or reproduction in other forums is permitted, provided the original author(s) and the copyright owner(s) are credited and that the original publication in this journal is cited, in accordance with accepted academic practice. No use, distribution or reproduction is permitted which does not comply with these terms.
*Correspondence: Kristin Thomsen, S3Jpc3Rpbi5UaG9tc2VuQHZ1Yi5iZQ==