- 1Neuropsychology Unit, IRCCS Istituto Centro San Giovanni di Dio Fatebenefratelli, Brescia, Italy
- 2IRCCS Fondazione Don Carlo Gnocchi – ONLUS, Milan, Italy
- 3Department of Psychology, Università Cattolica del Sacro Cuore, Milan, Italy
- 4Service of Statistics, IRCCS Istituto Centro San Giovanni di Dio Fatebenefratelli, Brescia, Italy
- 5IRCCS Centro Neurolesi “Bonino Pulejo”, Messina, Italy
- 6MAC Memory Clinic and Molecular Markers Laboratory, IRCCS Istituto Centro San Giovanni di Dio Fatebenefratelli, Brescia, Italy
- 7Università Degli Studi eCAMPUS, Novedrate, Italy
- 8Istituto Universitario Studi Superiori IUSS, Pavia, Italy
- 9IRCCS Fondazione Mondino, Pavia, Italy
- 10Department Neuroscience and Neurorehabilitation, IRCCS San Raffaele Roma, Rome, Italy
Background: In recent years, an increasing number of studies have examined the potential efficacy of cognitive training procedures in individuals with normal ageing and mild cognitive impairment (MCI).
Objective: The aims of this study were to (i) evaluate the efficacy of the cognitive Virtual Reality Rehabilitation System (VRRS) combined with anodal transcranial direct current stimulation (tDCS) applied to the left dorsolateral prefrontal cortex compared to placebo tDCS stimulation combined with VRRS and (ii) to determine how to prolong the beneficial effects of the treatment. A total of 109 subjects with MCI were assigned to 1 of 5 study groups in a randomized controlled trial design: (a) face-to-face (FTF) VRRS during anodal tDCS followed by cognitive telerehabilitation (TR) (clinic-atDCS-VRRS+Tele@H-VRRS); (b) FTF VRRS during placebo tDCS followed by TR (clinic-ptDCS-VRRS+Tele@H-VRRS); (c) FTF VRRS followed by cognitive TR (clinic-VRRS+Tele@H-VRRS); (d) FTF VRRS followed by at-home unstructured cognitive stimulation (clinic-VRRS+@H-UCS); and (e) FTF cognitive treatment as usual (clinic-TAU).
Results: An improvement in episodic memory was observed after the end of clinic-atDCS-VRRS (p < 0.001). We found no enhancement in episodic memory after clinic-ptDCS-VRRS or after clinic-TAU.
Moreover, the combined treatment led to prolonged beneficial effects (clinic-atDCS-VRRS+Tele@H-VRRS vs. clinic-ptDCS-VRRS+Tele@H-VRRS: p = 0.047; clinic-atDCS-VRRS+Tele@H-VRRS vs. clinic-VRRS+Tele@H-VRRS: p = 0.06).
Discussion: The present study provides preliminary evidence supporting the use of individualized VRRS combined with anodal tDCS and cognitive telerehabilitation for cognitive rehabilitation.
Clinical trial registration: https://clinicaltrials.gov/study/NCT03486704?term=NCT03486704&rank=1, NCT03486704.
1 Introduction
The Global Burden of Diseases study indicated that chronic neurological disorders (CNDs) are among the leading causes of disease burden worldwide, and the rehabilitation of CNDs appears to be a key factor for managing health problems, preventing disability, and reducing the social/economic impact of these diseases (GBD 2017 DALYs and HALE Collaborators, 2018; GBD 2019 Diseases and Injuries Collaborators, 2020; GBD 2021 Nervous System Disorders Collaborators, 2024).
Research suggests that early rehabilitation interventions for CNDs result in improved clinical outcomes; nevertheless, to date, rehabilitation is a very specialized and not always provided by the National Health Service, and it is typically performed in the advanced stage of the disease (Cieza et al., 2021).
Due to the growing need for early rehabilitation services for CNDs among the ageing population, a radical transformation of the health care system and the identification of new ways to strengthen care are necessary.
Cognitive deficits are a common consequence of neurodegenerative and other neurological disorders. The rehabilitation of neuropsychological deficits represents an expanding area of neurological rehabilitation (Taub et al., 2002; Stuss et al., 2008; Parsons, 2016; Maggio et al., 2023). Cognitive difficulties have gained increased amounts of attention in recent years. These disorders can cause significant personal, social, and functional burdens as well as difficulties with activities of daily living. Furthermore, non-pharmacological interventions to prevent and treat cognitive deficits in patients with neurodegenerative disease have been widely studied in recent years. Among non-pharmacological interventions, cognitive training is a potential approach for improving cognitive function and delaying cognitive decline (Woods and Britton, 1977; Cappa et al., 2003, 2005; Clare and Woods, 2004; Cotelli et al., 2006; Clare et al., 2010; Kortte and Rogalski, 2013; Bahar-Fuchs et al., 2013a,b, 2019; Gates and Sachdev, 2014; Hong et al., 2015; Clare, 2017; Rai et al., 2018; Kudlicka et al., 2023).
A critical aspect of cognitive rehabilitation programs is that the most promising interventions involve intensive in-person sessions that are unlikely to be cost-effective or feasible for large-scale implementation (Corbett et al., 2015; Matamala-Gomez et al., 2020; Realdon et al., 2023).
There is a need to provide alternative services dedicated to people at risk of developing neurocognitive disorders, services that can be responsive to the increased demand and at the same time reduce health care costs (Bharucha et al., 2009; Astell et al., 2019; Moyle, 2019). Equitable access to services, improved quality of care, continuous intervention, and promotion of self-management are some of the benefits that can result from the provision of digital medicine and telerehabilitation services (Cherney and van Vuuren, 2012; Cotelli et al., 2019; Maggio et al., 2024).
The delivery of rehabilitation via a variety of technologies appears to be an attractive approach for overcoming the limitations of high-intensity face-to-face (FTF) rehabilitation interventions (Brennan et al., 2011; Realdon et al., 2016; Pitt et al., 2019; Isernia et al., 2020; Rossetto et al., 2023; Pagliari et al., 2024). Moreover, telerehabilitation has been shown to have comparable outcomes to traditional in-person service delivery (Brennan et al., 2002; Rosen, 2004; Poon et al., 2005; Mashima and Doarn, 2008; Kairy et al., 2009; Cherney and van Vuuren, 2012; Jelcic et al., 2014; Vermeij et al., 2016; Antonietti et al., 2017; Burton and O'Connell, 2018; Cotelli et al., 2019; Isernia et al., 2019; Alaimo et al., 2021).
Mild cognitive impairment (MCI) is a condition associated with memory loss and with risk of cognitive decline (Petersen et al., 1999; Petersen, 2004; Petersen et al., 2014; Livingston et al., 2017; Frisoni et al., 2023). In a previous randomized controlled trial (RCT) performed by our group (Manenti et al., 2020a), we reported that cognitive function rehabilitation intervention involving the FTF Virtual Reality Rehabilitation System (VRRS) led to improved memory, language, and visuo-constructive skills compared with FTF treatment as usual. In addition, in the same participants, a cognitive telerehabilitation intervention was associated with greater maintenance of the improvements achieved than home-based unstructured stimulation.
Neurorehabilitation is a rapidly renewing field, and the change is being fuelled by the introduction of cutting-edge technologies, such as digital health technologies and noninvasive brain stimulation techniques, which enable personalized treatment approaches.
In this regard, in recent years, the use of neuromodulation techniques, such as transcranial direct current stimulation (tDCS), has emerged because of their ability to modify cortical plasticity by increasing excitability in cortical neurons within a specific network, improving cognitive abilities (Brunoni et al., 2012; Dayan et al., 2013; Antal et al., 2017; Lefaucheur et al., 2017; Menardi et al., 2022) in neurodegenerative disease (Cotelli et al., 2012, 2020; Manenti et al., 2020b; Saxena and Pal, 2021). Studies have suggested that the use of noninvasive techniques coupled with cognitive intervention is more effective than cognitive training or noninvasive brain stimulation applied alone (Hsu et al., 2015; Brem et al., 2020; Cotelli et al., 2020; Nissim et al., 2020; Pergher et al., 2022).
Consistent with this hypothesis, this study aimed to (i) evaluate the efficacy of Face-to-Face cognitive VRRS combined with anodal tDCS applied to the left dorsolateral prefrontal cortex (DLPFC) on episodic memory compared to placebo tDCS stimulation combined with Face-to-Face cognitive VRRS and FTF cognitive treatment as usual and (ii) determine how to prolong the beneficial effects of the treatment using a telerehabilitation approach. To achieve these objectives, we recruited a sample of subjects with MCI who underwent FTF VRRS combined with anodal or placebo tDCS followed by cognitive telerehabilitation, and we analysed the collected data along with those acquired in our previous study (Manenti et al., 2020a).
2 Materials and methods
Participants were recruited at the IRCCS Istituto Centro San Giovanni di Dio Fatebenefratelli of Brescia, the IRCCS Fondazione Don Carlo Gnocchi Onlus of Milan, and the IRCCS Centro Neurolesi Bonino-Pulejo of Messina from April 2018 to November 2022 (see Figure 1).
2.1 Study design
In this randomized, multicentre, active-controlled study, both the investigators and the outcome assessors were blinded to the treatment assigned to the participants. The study was approved by the local ethics committees (Ethics Statement numbers 48/2017 and 41/2020), conducted in accordance with the Declaration of Helsinki, and reported according to CONSORT guidelines (Boutron et al., 2008, 2017), and the trial was registered on clinicaltrials.gov (NCT number: NCT03486704). The CONSORT checklist is provided in the Supplementary materials.
All participants were fully aware of the aims of the study; written informed consent was obtained. A total of 109 subjects with MCI were randomly assigned to one of five experimental conditions: (a) Face-to-Face VRRS during anodal tDCS followed by cognitive telerehabilitation-TR (clinic-atDCS-VRRS+Tele@H-VRRS); (b) Face-to-Face VRRS during placebo tDCS followed by cognitive telerehabilitation (clinic-ptDCS-VRRS+Tele@H-VRRS); (c) Face-to-Face VRRS followed by telerehabilitation (clinic-VRRS+Tele@H-VRRS); (d) Face-to-Face VRRS followed by at-home unstructured cognitive stimulation (clinic-VRRS+@H-UCS); and (e) Face-to-Face cognitive treatment as usual (clinic-TAU).
Stratified randomization was performed by AG and NSB based on age and scores on the Mini Mental State Examination (MMSE; Folstein et al., 1975). Details of the allocated group were given to the researcher who wrote the treatment on cards contained in sequentially numbered, opaque, and sealed envelopes.
The original study protocol (Ethic Statement number 48/2017; Manenti et al., 2020a) was amended (Ethics Statement number 41/2020) by adding two experimental groups (clinic-atDCS-VRRS+Tele@H-VRRS; clinic-ptDCS-VRRS+Tele@H-VRRS) to evaluate the efficacy of cognitive VRRS combined with anodal tDCS applied to the left DLPFC compared to that of placebo tDCS stimulation combined with VRRS on episodic memory (Marion and Althouse, 2023). See Figure 2 Panel A.
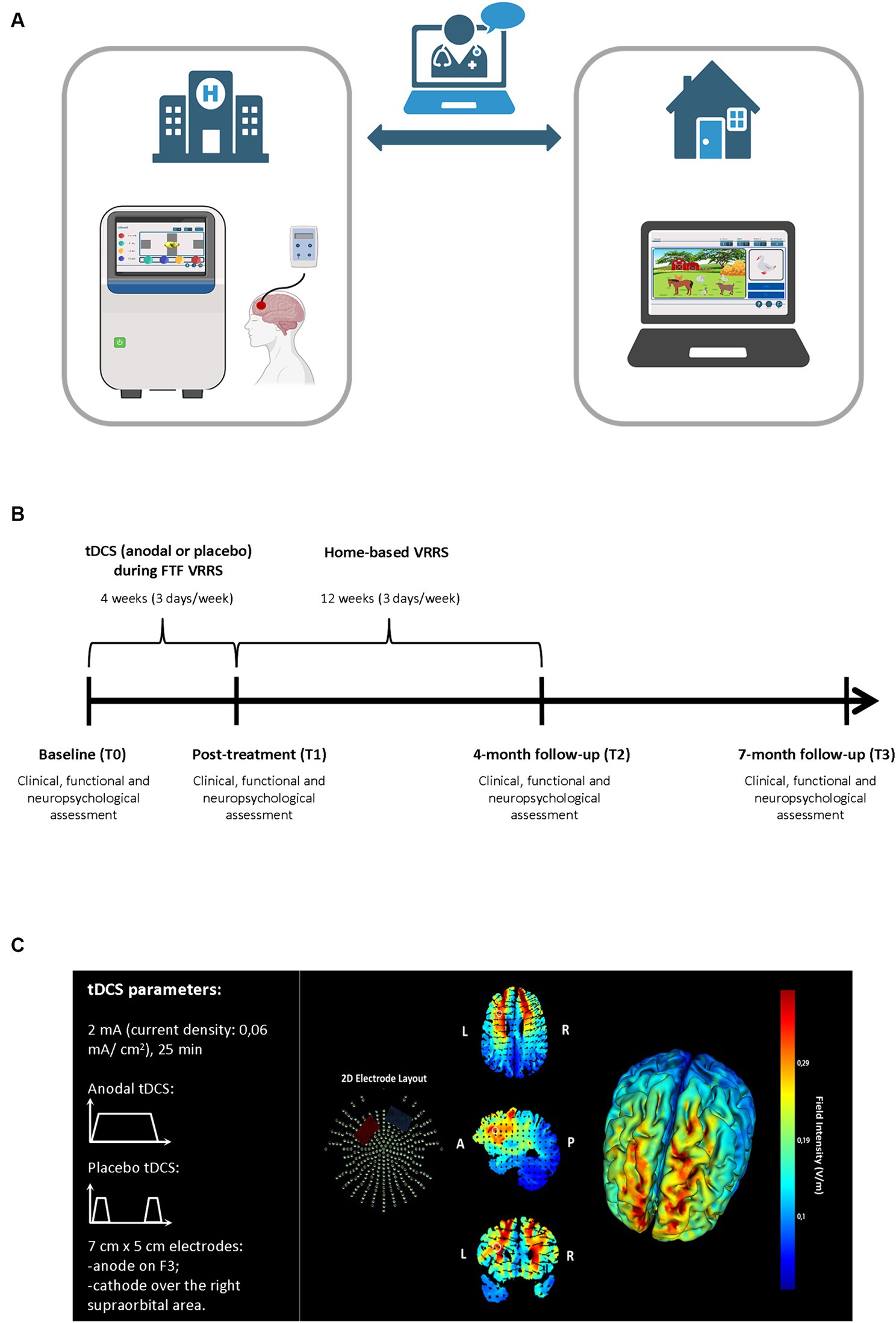
Figure 2. Experimental procedure for the face-to-face cognitive virtual reality rehabilitation system combined with anodal tDCS followed by cognitive telerehabilitation (clinic-atDCS-VRRS+Tele@H-VRRS) and for face-to-face cognitive virtual reality rehabilitation system combined with placebo tDCS followed by cognitive telerehabilitation (clinic-ptDCS-VRRS+Tele@H-VRRS). (A) Face-to-face (FTF) cognitive virtual reality rehabilitation system (VRRS) combined with tDCS followed by cognitive telerehabilitation. (B) Timeline for the experimental protocol of the FTF cognitive virtual reality rehabilitation system (VRRS) combined with anodal or placebo tDCS followed by cognitive telerehabilitation. (C) Current flow model of tDCS montage (anode over F3 and cathode over the right supraorbital area), using two 7×5 cm sponge pads represented in axial, sagittal and coronal views from the Male 1 model in the Soterix HD Targets software (Soterix Medical). Arrows represent the direction of current flow.
The study protocol was carried out with no changes from the above amendments.
2.2 Participants
A total of 109 older adults who met the Petersen criteria for MCI (Petersen, 2011) and who were followed up annually for at least 2 years before enrolment were recruited. The inclusion criteria were as follows: (a) subjective complaints by the subject, a reliable informant or an expert clinician; (b) defective performance in at least one cognitive domain; (c) MMSE score greater than or equal to 24/30 (Folstein et al., 1975); (d) global Clinical Dementia Rating (CDR) score less than or equal to 1 (Morris, 1997); (e) preservation of functional autonomy; (f) absence of criteria for a diagnosis of dementia according to the Diagnostic and Statistical Manual of Mental Disorders (DSM-5; American Psychiatric Association, 2014); and (g) absence of depressive and anxiety symptoms. The exclusion criteria were as follows: other neurological or psychiatric disorders, visual or auditory perception disorders, history of traumatic brain injury, brain tumor or stroke, and alcohol abuse. No other cognitive training was administered during the duration of the present study (from baseline to the last follow-up assessment). Any contraindication for tDCS, such as a history of seizures, major head trauma, past brain surgery, brain metal implant, or a pacemaker, excluded the participant from the allocation to groups involving tDCS application.
The sample size calculation was based on a prior study assessing the effect of VRRS on MMSE scores in patients with Alzheimer’s disease (AD; Jelcic et al., 2014). Considering a significance level (α) of 0.05, a power (1-β) = 80 (two-tailed independent t test), and a dropout rate of 35%, the estimated sample size was twenty participants for each group.
2.3 Assessment procedures
Detailed records of previous medical events/visits and current medication, the Clinical Dementia Rating (CDR) scale (Morris, 1997), the Edinburgh Handedness Inventory (EHI; Oldfield, 1971), and the Cognitive Reserve Index questionnaire (CRIq; Nucci et al., 2012) were completed exclusively at the baseline assessment. Moreover, a comprehensive clinical, functional, and neuropsychological evaluation (approximately 90 min) was carried out for all groups at baseline (T0), at the end of FTF treatment (T1, 1 month from baseline), and at four (T2) and 7 months (T3) from baseline by expert neuropsychologists blinded to the treatment allocation of the participants.
Clinical and functional assessments included the Everyday Memory Questionnaire (EMQ; Sunderland et al., 1986; Calabria et al., 2011) for the evaluation of subjective memory complaints, basic (BADL) and instrumental activity of daily living (IADL) scales (Katz, 1983; Lawton and Brody, 1988) to assess the degree of autonomy in activities of daily living, the Geriatric Depression Scale (GDS; Yesavage et al., 1982) for depressive symptoms, the Neuropsychiatric Inventory (NPI; Cummings et al., 1994; Binetti et al., 1998) for neuropsychiatric symptoms and the Quality of Life in Alzheimer’s Disease (QOL-AD) scale (Bianchetti et al., 2017) for a measure of quality of life.
The standardized neuropsychological battery comprised the Mini Mental State Examination (MMSE; Folstein et al., 1975) for the assessment of global cognition as well as the following cognitive tests, which covered a broad range of cognitive abilities: the Rey Auditory Verbal Learning Test (RAVLT) for immediate and delayed recall (Carlesimo et al., 1996); the Free and Cued Selective Reminding Test (FCSRT; Frasson et al., 2011) and the Rey–Osterrieth complex figure (ROCF) test-recall (Caffarra et al., 2002) for episodic memory; Raven’s Colored Progressive Matrices for nonverbal reasoning (Basso et al., 1987); verbal fluency (phonemic and semantic; Novelli et al., 1986) and action and object naming subtests from the Battery for Aphasic Deficit Analysis (BADA; Miceli et al., 1994) for language production; the ROCF test-copy (Caffarra et al., 2002) for visuo-constructive abilities; and the Trail Making Test (TMT) part A and part B (Giovagnoli et al., 1996) for attention and executive functions (Lezak et al., 2012; see Tables 1, 2 for details).
The participants who received FTF VRRS treatment (clinic-atDCS-VRRS+Tele@H-VRRS, clinic-ptDCS-VRRS+Tele@H-VRRS, clinic-VRRS+Tele@H-VRRS and clinic-VRRS+@H-UCS) underwent an assessment of system usability via the System Usability Scale (SUS; Brooke, 1996; Bangor et al., 2008, 2009; Peres et al., 2013) at T1. Moreover, we recorded the SUS scores at T2 in the subjects who underwent home-based treatment (clinic-atDCS-VRRS+Tele@H-VRRS, clinic-ptDCS-VRRS+Tele@H-VRRS, clinic-VRRS+Tele@H-VRRS and clinic-VRRS+@H-UCS).
2.4 Treatment
Participants received an FTF treatment that could be followed by a home-based treatment, according to their group allocation. The different types of FTF and home-based treatments outlined in this study protocol are described in the following paragraphs.
2.4.1 Face-to-face treatment
All the participants enrolled in the study received 12 sessions of FTF cognitive training. According to the experimental group allocation, participants could undergo one of four treatments during FTF treatment: (a) FTF VRRS (clinic-VRRS) or (b) FTF cognitive treatment as usual (clinic-TAU); (c) FTF VRRS during anodal tDCS (clinic-atDCS-VRRS); (d) FTF VRRS during placebo tDCS (clinic-ptDCS-VRRS).
2.4.1.1 Clinic-VRRS
Twelve 60-min sessions (over 4 weeks) of individualized cognitive rehabilitation using VRRS1 were administered to participants assigned to the clinic-VRRS.
The FTF cognitive VRRS included 12 exercises designed to enhance memory, visuospatial abilities, attention and executive functions. In each treatment session, the participant worked with six exercises, 10 min each, so that each exercise was completed six times over the 12 clinic-VRRS sessions. In all the sessions, except for the first and last sessions, strategies aimed at improving the subject’s performance were suggested by the researcher. Each training session ended with feedback on performance, and a detailed report was available. Clinic-VRRS treatment was tailored to the participant’s baseline characteristics: the starting level was adjusted using an adaptive staircase procedure. Progress was continuously monitored by the researcher, and each exercise adaptively progressed in difficulty.
2.4.1.2 Clinic-TAU
Participants assigned to the clinic-TAU group received 12 60-min sessions of group cognitive stimulation in the clinic. During these group sessions, metacognitive training aimed at learning cognitive strategies and using external aids, reminiscence therapy, reality orientation therapy, and paper and pencil exercises was proposed by mental health professionals.
2.4.1.3 Clinic-atDCS-VRRS or clinic-ptDCS-VRRS
To evaluate the efficacy of the cognitive VRRS combined with anodal tDCS applied to the left DLPFC, all of the individuals allocated to the clinic-atDCS-VRRS+Tele@H-VRRS or clinic-ptDCS-VRRS+Tele@H-VRRS group received tDCS stimulation over the left DLPFC (Anodal or Placebo, based on the assigned group) during the FTF VRRS cognitive training, starting at the beginning of the training (Figure 2 Panel A and B).
A tDCS stimulator (BrainStim, EMS, Bologna, Italy) delivered a constant low-intensity (2 mA) current for 25 min (with a ramping period of 10 s at the beginning and at the end of the tDCS session) through two saline-soaked sponge electrodes (7 cm x 5 cm, current density: 0.06 mA/cm2) (Bikson et al., 2016; Antal et al., 2017). An electroconductive gel was applied under the electrodes to reduce impedance, as in previous studies (Manenti et al., 2013; Sandrini et al., 2014, 2016). Participants and researchers were blinded to the tDCS condition applied: the anodal (active) or placebo stimulation mode was selected by entering a code.
The targeted region was the left DLPFC: the anode electrode was placed over F3, according to the 10–20 EEG international system, and the cathode electrode was located over the right supraorbital area. Figure 2 Panel C shows a graphical representation of the computerized modeling of tDCS-induced current flow.2 In the Placebo tDCS condition, the current was turned off 10 s after the beginning and was turned on for 10 s at the end of the stimulation period so that the participants could not distinguish between anodal and placebo stimulation (Manenti et al., 2013). Sensations induced by tDCS were assessed immediately after the stimulation session (Fertonani et al., 2015).
2.4.2 Home-based treatment
According to the experimental group allocation, participants could undergo one of two treatments during home-based treatment: (a) cognitive telerehabilitation-TR (Tele@H-VRRS); (b) at-home unstructured cognitive stimulation (@H-UCS); or no home-based treatment.
2.4.2.1 Tele@H-VRRS
After the end of FTF treatment, the participants assigned to Tele@H-VRRS received thirty-six 60-min sessions (3 sessions/week over 3 months) of home-based cognitive VRRS treatment (see text footnote 1, respectively). Twelve exercises different from those used in FTF VRRS training and designed to enhance memory, visuospatial abilities, attention and executive functions were selected. Each treatment session comprised six exercises (10 min each), and each exercise was completed 18 times over the thirty-six home-based VRRS sessions. Task difficulty adaptively progressed, and performance was continuously monitored by the researcher.
The VRRS has telerehabilitation functionalities, thus enabling the use of the same functionalities applied in the FTF treatment and the adjustment of exercise characteristics. Before beginning the home-based treatment, the researcher scheduled all 36 sessions of the individualized cognitive training exercise on the subject’s tablet. Moreover, a dedicated role-playing session was conducted with both the participant and his/her caregiver in order to familiarize with the technology. Specifically, the researcher shown they how to use the technological device and they were introduced to all the cognitive exercises included in the home-based treatment.
During home-based treatment, the researcher provided continuous assistance for technical difficulties, and the task difficulty of the individualized cognitive training exercises was remotely adapted once a week via a telerehabilitation platform by the clinician. Each participant received a home-based kit including a tablet that allowed access to a daily individualized training program, a detailed VRRS tablet manual, an exercise instructions booklet, and a diary.
2.4.2.2 @H-UCS
Subjects assigned to @H-UCS were requested to work on detailed activities (paper and pencil exercises, creative manual activities, reading newspapers and magazines, watching documentaries, crosswords, and sudoku) 60 min a day, 3 times a week over 12 weeks (36 sessions in total). Participants received an instruction booklet and a diary.
2.5 Statistical methods
Summary statistics are expressed as the means and standard deviations. Comparisons of sociodemographic features, the scales completed exclusively at the baseline assessment and the system usability scale (SUS) scores between groups were evaluated by parametric (t tests) or corresponding nonparametric (Kruskal-Wallis) tests. The perceptions of sensation scores were compared between the anodal and placebo tDCS groups using the Mann–Whitney test.
The analyses were carried out using a modified intention-to-treat (mITT) approach. Specifically, given the lack of a unanimous consensus on this definition, as illustrated by Abraha and Montedori (2010), the inclusion criteria were based on the presence of at least a baseline assessment (four subjects had only a baseline assessment). According to the Cochrane guidelines for randomized trials,3 when dealing with a relatively low percentage of missing values [between 5% (small) and 20% (large); in our case, the percentage of missing values was 8% for all variables, except for TMT], it is reasonable to include participants with some missing values. Ultimately, the decision was made to retain the actual scores without replacement, as the type of analysis applied to the outcomes (generalized linear modeling, which allows the inclusion of all available observations without listwise deletion) and the reduced percentage of missing values in different conditions significantly reduce the risk of bias due to missingness in result interpretation.
Consistent with our first aim, we evaluated the efficacy of FTF cognitive VRRS combined with anodal tDCS compared to that of placebo tDCS stimulation combined with VRRS and of FTF cognitive treatment as usual for episodic memory (RAVLT and FCSRT scores).
For this purpose, we considered three groups of subjects—those who received clinic-atDCS-VRRS+Tele@H-VRRS, clinic-ptDCS-VRRS+Tele@H-VRRS or clinic-TAU—and compared their scores at all the time points (T0, T1, T2, T3). Based on the inherent distribution profiles of the variables (Gaussian, negative binomial, gamma, or Tweedie), we employed generalized linear mixed models (GLMMs) to examine the variations in scores between the three groups and across four distinct time points (T0, T1, T2, T3). Each model incorporated a distinct test score as the dependent variable, while independent variables included time, group, and the interaction term time*group. We treated time, group, and their interaction as fixed effects, while we considered the subjects as random effects. We implemented a repeated measure setting with an AR1 (first order autoregressive) covariance matrix. The AR1 structure explicitly models the correlation between repeated measures on the same subject, assuming that measurements taken closer in time are more highly correlated than those taken further apart. This structure helps in capturing the within-subject variability. Additionally, robust standard errors were requested for the fixed effects covariance estimates when necessary. This approach provides more reliable standard errors and test statistics when data assumptions like homoscedasticity or normality are violated. Post hoc assessments underwent correction using the sequential Bonferroni method. This method, differently from the classical Bonferroni method, is based on the ranking of p-values. Sequential Bonferroni-adjusted p-values were calculated. If this adjusted p-value was less than 0.05, it meant the result was still statistically significant even after the correction. When multiple comparisons were involved, we reported the sequential Bonferroni-adjusted p-value together with the non-adjusted one. Statistical analyses were performed using SPSS version 29.0, and R software (R Core Team, 2013) was used for the creation of graphical representations.
Consistent with our second aim, we assessed the possibility of prolonging the beneficial effects obtained after FTF treatment using a telerehabilitation approach. For this purpose, we considered all the experimental groups (clinic-atDCS-VRRS+Tele@H-VRRS, clinic-ptDCS-VRRS+Tele@H-VRRS, clinic-TAU, clinic-VRRS+Tele@H-VRRS, and clinic-VRRS+@H-UCS), and we compared the changes between T0 and T3 (i.e., the last follow-up visit). To achieve our objective, for the outcomes that improved significantly from T0 to T1, we calculated the difference (referred to as deltaT) between the scores recorded at time T3 and those at baseline (T0). DeltaT served as the dependent variable for our analysis. The distribution of deltaT closely resembled a beta distribution, characterized by its symmetrical shape and evidence of overdispersion. Consequently, we opted for beta regression, adjusting the distribution’s mean to zero to facilitate this process and considering group (with five different levels, one for each condition) as a predictor of the deltaT outcome. To enhance the interpretability of the regression coefficients, we applied an exponential transformation to them. The transformed coefficients should be understood as odds ratios, offering insights into the relationship between our independent variables and the observed changes in scores.
3 Results
A total of 247 subjects were evaluated for inclusion in this study. Ultimately, 138 subjects were excluded (80 subjects did not meet the inclusion criteria, 41 subjects declined to participate mostly due to the COVID-19 pandemic, and 17 for other reasons), whereas 109 subjects were deemed eligible for participation.
These 109 subjects were randomized into five experimental groups: 23 participants were allocated to the clinic-atDCS-VRRS+Tele@H-VRRS group; 21 participants were allocated to the clinic-ptDCS-VRRS+Tele@H-VRRS group; 20 participants were allocated to the clinic-VRRS+Tele@H-VRRS group; 23 subjects were allocated to the clinic-VRRS+@H-UCS group; and 22 participants were allocated to the clinic-TAU group (see Figure 1).
3.1 Participants
We enrolled 109 subjects with MCI, 72 (66%) with amnestic MCI and 37 (34%) subjects with nonamnestic MCI (Petersen, 2011). In particular, the current sample included (i) 36 subjects with amnestic single-domain MCI (aMCI-s), (ii) 36 subjects with amnestic multiple-domain MCI (aMCI-m), (iii) 26 subjects with nonamnestic single-domain MCI (naMCI-s), and (iv) 11 subjects with nonamnestic multiple-domain MCI (naMCI-m).
The five groups did not differ in terms of age (p = 0.268), education (p = 0.687), MMSE score (p = 0.961), EHI score (p = 0.311), CRI-Total Score (p = 0.422), CRI-Education score (p = 0.969), CRI-Working Activity score (p = 0.959), CRI-Leisure Time score (p = 0.068), or CDR scale score (p = 0.639), but there was a significant difference in sex (p = 0.013). See Table 1.
3.2 Face-to-face cognitive virtual reality rehabilitation system combined with anodal tDCS efficacy
Descriptive statistics for the clinical, functional, and neuropsychological evaluation scores at each time point are shown in Table 2.
The results of the GLMMs are presented in Table 3. The only outcome that manifested significant differences between the three groups (clinic-atDCS-VRRS+Tele@H-VRRS, clinic-ptDCS-VRRS+Tele@H-VRRS, and clinic-TAU) from T0 to T1 and over the four time points (T0, T1, T2, and T3) was the immediate free recall (IFR) score of the FCSRT (interaction group*time: p = 0.002). In particular, the analyses revealed improvement from T0 to T1 (p = 1.03*10–5; p adj. = 0.00037) and maintenance at T3 (T0 to T3, p = 1.06*10–5; p adj. = 0.00037) only in the clinic-atDCS-VRRS+Tele@H-VRRS group (Figure 3A). Significant Group*Time interactions were also found for RAVLT-Immediate Recall (p = 0.002) and BADA-Objects Naming (p = 0.016), but none of the three groups improved or deteriorated significantly from T0 to T1. In particular, the analyses showed a decrease in RAVLT-Immediate Recall scores from T0 to T3 (p = 0.0005; p adj. = 0.017) only in the clinic-ptDCS-VRRS+Tele@H-VRRS group, while none of the groups showed a significant improvement or decrease over the four timepoints regarding BADA-Objects Naming.
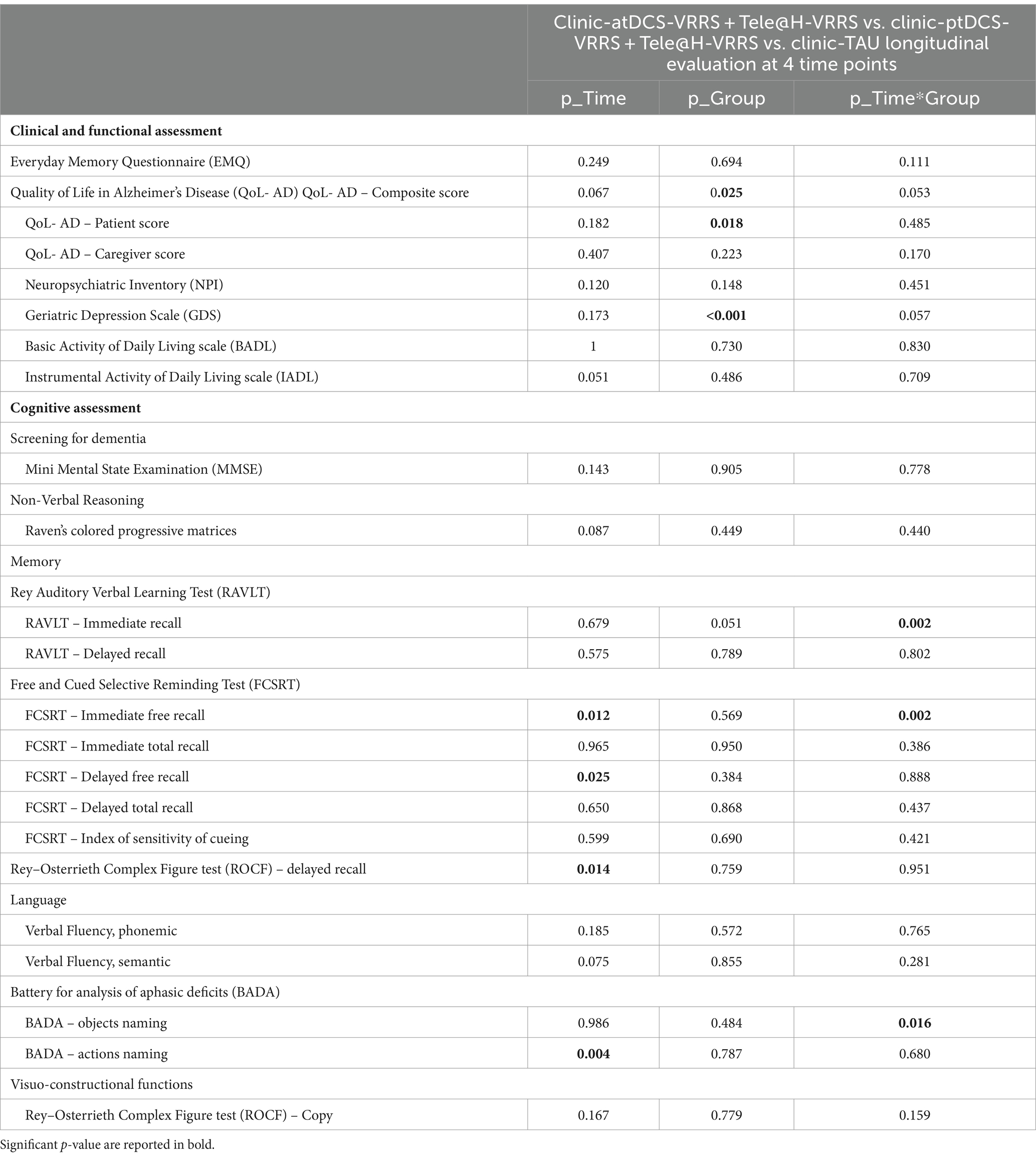
Table 3. Generalized linear mixed models results for clinical, functional, and neuropsychological evaluation.
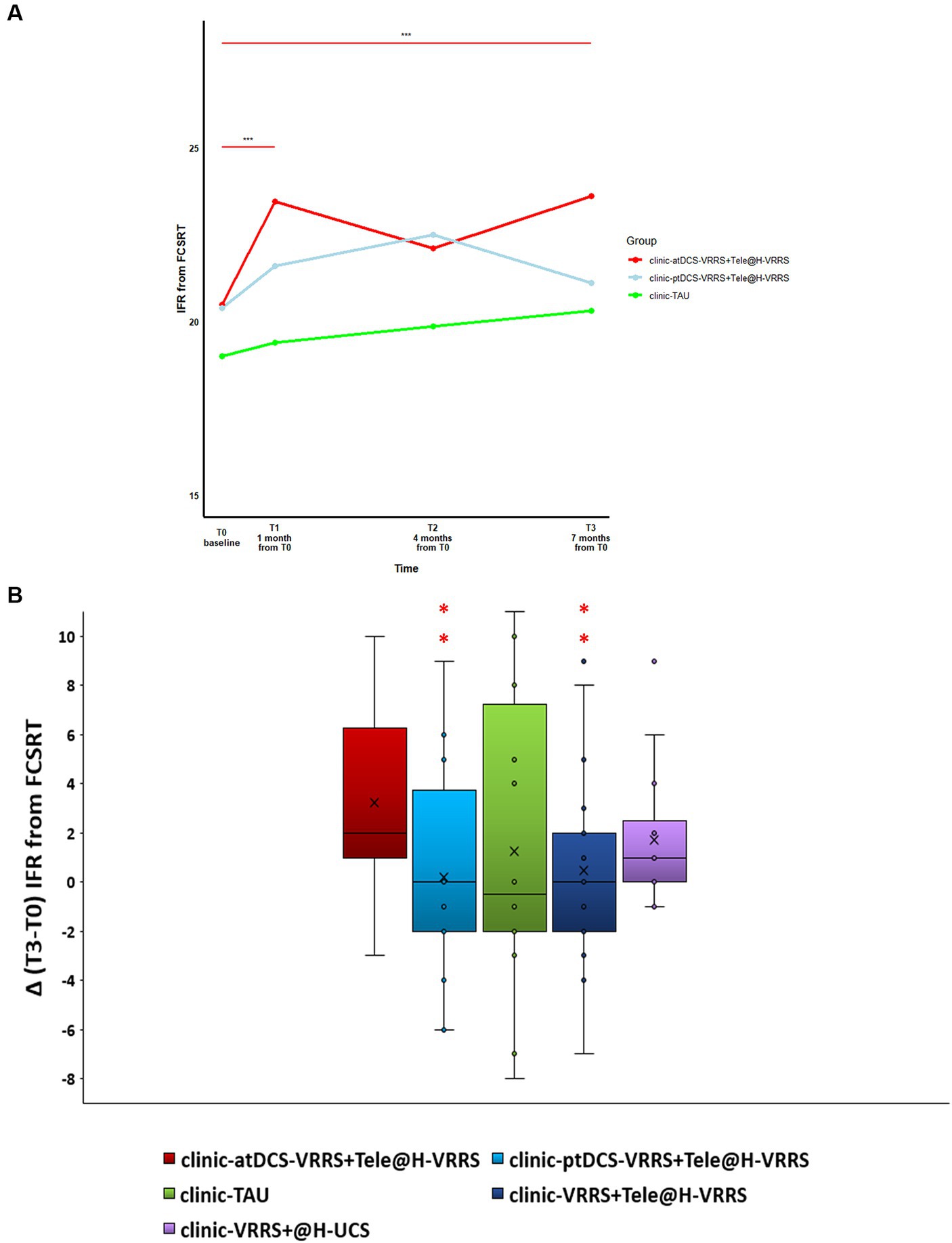
Figure 3. (A) Effects of face-to-face (FTF) cognitive virtual reality rehabilitation system (VRRS) combined with anodal tDCS followed by cognitive telerehabilitation (clinic-atDCS-VRRS+Tele@H-VRRS) vs. FTF cognitive VRRS combined with placebo tDCS followed by cognitive telerehabilitation (clinic-ptDCS-VRRS+Tele@H-VRRS) vs. FTF cognitive treatment as usual (clinic-TAU) on the immediate free recall (IFR) score of the Free and Cued Selective Reminding Test (FCSRT). Asterisks indicate significant comparisons for clinic-atDCS-VRRS+Tele@H-VRRS from T0 to T1 (p < 0.001) and from T0 to T3 (p < 0.001). (B) Long-term beneficial effects of face-to-face VRRS during anodal tDCS followed by cognitive telerehabilitation (clinic-atDCS-VRRS+Tele@H-VRRS). Box-plot of the delta deviations (differences between the scores recorded at time T3 and those at baseline T0) for the different conditions are reported. Asterisks indicate conditions that differed significantly from those of the clinic-atDCS-VRRS+Tele@H-VRRS group.
3.3 Long-term beneficial effects of face-to-face cognitive VRRS during anodal tDCS followed by cognitive telerehabilitation
Consistent with our second aim, we assessed the possibility of prolonging the beneficial effects obtained after FTF treatment with a beta regression analysis.
Regarding the neuropsychological assessment, as reported earlier, the outcome that behaved in a significantly different way in the three groups over time was the IFR of the FCSRT.
The analyses showed that the gains in episodic memory (FCSRT, IFR score) were maintained up to the 7-month (T3) follow-up only in the clinic-atDCS-VRRS+Tele@H-VRRS group. Specifically, the comparison between the effects of clinic-based ptDCS-VRRS combined with Tele@H-VRRS and clinic-based atDCS-VRRS combined with Tele@H-VRRS revealed an estimated effect of −0.5887 for clinic-ptDCS-VRRS+Tele@H-VRRS compared to clinic-atDCS-VRRS+Tele@H-VRRS (p = 0.047; odds ratio = 0.56) when clinic-atDCS-VRRS+Tele@H-VRRS was used as the reference group. Moreover, clinic-VRRS+Tele@H-VRRS had an estimated effect of −0.53 compared to that of clinic-atDCS-VRRS+Tele@H-VRRS (p = 0.06; odds ratio = 0.59), using clinic-atDCS-VRRS+Tele@H-VRRS as the reference group (Figure 3B). Additionally, the model showed a pseudo R-squared value of 0.088, explaining approximately 8.8% of the variability in the outcome variable.
3.4 System usability scale
Interestingly, the SUS, administered at T1 to the four groups who received clinic-VRRS, showed good usability performance of the clinic-VRRS system (72.2, SD 11.6), and the SUS scores obtained at T2 in the three groups that underwent home-based treatment (Tele@H-VRRS and @H-UCS) from T1 and T2 highlighted good usability performance (74.4, SD 9.4) of the VRRS telerehabilitation system (Bangor et al., 2008).
3.5 tDCS-perceptual sensations questionnaire
tDCS perceptual sensation questionnaire scores reported during anodal tDCS were compared with those reported during placebo tDCS (clinic-atDCS-VRRS+Tele@H-VRRS vs. clinic-ptDCS-VRRS+Tele@H-VRRS group), showing comparable tDCS-induced sensations in the two stimulation conditions (clinic-atDCS-VRRS+Tele@H-VRRS: 1.39 SD 0.90, clinic-ptDCS-VRRS+Tele@H-VRRS: 1.69 SD 0.98, U = 172.5, p = 0.230). Overall, only a few subjects reported low-intensity perceptual sensations related to the application of tDCS (burning, itching, and tingling).
4 Discussion
Episodic memory refers to the memory of past life events (Tulving, 1983) and displays the greatest degree of age-related decline (Rönnlund et al., 2005; Salthouse, 2011; Vestergren and Nilsson, 2011), a process that is accelerated in pathological conditions such as MCI and AD.
Non-pharmacological interventions to prevent and treat cognitive deficits and the associated difficulties with activities of daily living in neurodegenerative disease patients have gained attention in recent years. Among these interventions, cognitive training offers a potential approach for dementia prevention and for the improvement of cognitive functions (Cappa et al., 2003; Cotelli et al., 2006; Bahar-Fuchs et al., 2013a, 2013b; Yao et al., 2020; Hu et al., 2022). A critical aspect of cognitive training programs is that the most promising interventions involve intensive in-person sessions that are unlikely to be cost-effective or feasible for large-scale implementation (Botsis et al., 2008; Brennan et al., 2009; Corbetta et al., 2015). Within the framework of non-pharmacological interventions, the use of technology to assist people at risk of developing cognitive disorders or mild dementia at home has gradually gained importance (Realdon et al., 2016; Rossetto et al., 2023). Moreover, in recent years, tDCS has been considered a promising, noninvasive neuromodulation technique for individuals suffering from MCI (Palimariciuc et al., 2023). Since some evidence suggests that it might be worth exploring new cognitive rehabilitation approaches, we applied cognitive rehabilitation training combined with tDCS and telerehabilitation herein (Isernia et al., 2019; Nousia et al., 2021; Menengi̇ç et al., 2022; Torpil et al., 2023).
In particular, we evaluated the efficacy of cognitive virtual reality rehabilitation system (VRRS) combined with anodal transcranial direct current stimulation (tDCS) applied to the left dorsolateral prefrontal cortex (DLPFC) compared to that of placebo tDCS combined with VRRS, and we assessed the possibility of prolonging the beneficial effects. Referring to long-term memory, we targeted the left DLPFC, in line with previous studies that used noninvasive brain stimulation techniques to prove the involvement of this area in memory abilities (Fletcher and Henson, 2001; Rossi et al., 2001, 2004, 2006; Sandrini et al., 2003, 2013, 2014, 2016, 2019, 2020; Manenti et al., 2010, 2011, 2012, 2013, 2016, 2017, 2020b; Sandrini and Cohen, 2014; Brambilla et al., 2015; Krebs et al., 2020; Vaqué-Alcázar et al., 2021). Some researchers have used multiple sessions of tDCS to induce long-lasting effects in subjects with MCI (Yun et al., 2016; Fileccia et al., 2019; Gomes et al., 2019; Sandrini et al., 2020; Gu et al., 2022), but long-term effects of the intervention have been recorded in few studies (Murugaraja et al., 2017; Das et al., 2019; Gomes et al., 2019; Lu et al., 2019; Gu et al., 2022). At the neuronal level, in subjects with MCI, tDCS has been shown to increase regional cerebral metabolism in multiple brain regions, including the insula, hippocampus, and parahippocampus (Yun et al., 2016; Fregni et al., 2021).
Here, we found that an innovative in-person cognitive neurorehabilitation approach (FTF VRRS during anodal tDCS - clinic-atDCS-VRRS) was able to enhance episodic memory in MCI patients. The rationale for the application of tDCS in MCI and AD patients is based on modulating cortical excitability with a combined approach, which involves the induction of neuroplasticity through the activation of impaired cognitive functions in conjunction with tDCS. Therefore, the combined application of specific cognitive training and tDCS is essential for inducing synaptic plasticity mechanisms (Zimerman et al., 2013; Hsu et al., 2015; Prehn and Flöel, 2015; Yun et al., 2016; Gu et al., 2022).
In addition, we observed that MCI subjects who had received face-to-face cognitive rehabilitation combined with neuromodulation followed by asynchronous tablet telerehabilitation treatment (clinic-atDCS-VRRS+Tele@H-VRRS) maintained improvements in memory at the 7-month follow-up. Telerehabilitation technologies allow services to be provided remotely in patients’ homes, allowing access to health care to individuals living in rural settings or with mobility difficulties (Rogante et al., 2010; Brennan et al., 2011; Peretti et al., 2017; De Cola et al., 2020; Lawson et al., 2020; Maresca et al., 2020; Cruse et al., 2022). In addition, the telerehabilitation modality offers the advantage of providing rehabilitation within the natural environment of the patient’s home, making the treatment more realistic and possibly more generalizable to the person’s daily life (McCue et al., 2010).
In line with our previous results, this study confirms the feasibility of telerehabilitation in subjects with MCI, which is likely related to participants engagement in a telerehabilitation design involving asynchronous researcher–patient interactions (Matamala-Gomez et al., 2020). Overall, high rates of participant agreement, recruitment, and treatment adherence supported the feasibility of both in-person cognitive neurorehabilitation treatment (clinic-atDCS-VRRS) and telerehabilitation with home-based cognitive VRRS interventions (Tele@H-VRRS). Moreover, the analyses of system usability demonstrated the good usability of the VRRS applied in clinic (clinic-VRRS) and at home (Tele@H-VRRS). The present results are in line with recent findings and meta-analyses published on the efficacy and feasibility of a cognitive telerehabilitation program in individuals with MCI (Poon et al., 2005; Vermeij et al., 2016; Burton and O’Connell, 2018; Mosca et al., 2020; Nousia et al., 2021; Cacciante et al., 2022; Bernini et al., 2023; Chan et al., 2024).
In particular, MCI patients who received VRRS cognitive treatment in the clinic associated with anodal tDCS followed by VRRS tablet telerehabilitation showed greater maintenance of treatment gains in Immediate Free Recall (IFR) on the Free and Cued Selective Reminding Test (FCSRT), and these enhancements were maintained over a 7-month follow-up. The FCSRT (Buschke, 1984; Grober et al., 1997) is the memory test recommended by the International Working Group on AD (Dubois et al., 2007) for the detection of significant and progressive episodic memory impairment (Lemos et al., 2015). The FCSRT assesses verbal episodic memory with controlled learning and semantic cueing. This test has been shown to be useful in predicting the presence of dementia (Grober et al., 2000), in distinguishing AD from other dementias (Pillon et al., 1994; Pasquier et al., 2001) and in predicting the progression from MCI to AD (Sarazin et al., 2007; Frasson et al., 2011; Clerici et al., 2017). Interestingly, FCSRT scores have been shown to correlate with structural measures of hippocampal atrophy (Sarazin et al., 2010). Specifically, the treatment proposed in this paper involving the combination of anodal tDCS and cognitive training followed by TR improved the IFR of the FCRST in MCI patients, and this increase remained stable at the 7-month follow-up. While the present findings emphasize the importance of telerehabilitation for treating cognitive deficits to slow the progression of the disease, standardization of methodological aspects of the studies is required to obtain more homogenous data and to determine the optimal type and dose of cognitive telerehabilitation (Maggio et al., 2024).
We acknowledge that our study has some limitations. First, a larger sample size might allow us to account for individual differences that could influence the efficacy of the treatment; consequently, the findings should be confirmed in larger samples. Furthermore, in future trials, we will consider a cost-effectiveness analysis of the telerehabilitation approach (Dávalos et al., 2009) and the possibility of planning longer follow-up visits to follow the progress of the improvement obtained after treatment over a longer period of time. Moreover, the lack of control conditions applying tDCS over different cortical areas might represent further limitations of the present study. Furthermore, the placement of the reference electrode in a cephalic region (either anode or cathode) with an equally sized anodal electrode can induce reference-specific effects (anodal/ cathodal) in parallel to the cathodal/anodal effects of the active electrode. Finally, further studies could test for possible learning effects due to the repetition of the tests at several time points, even if the specificity of the results on episodic memory and the selective recording of improvements in the FTF VRRS during anodal tDCS (clinic-atDCS-VRRS) effect, recorded in only one group of subjects, suggest that the cognitive improvements observed in our study cannot be solely accounted for by task practice effects.
Nonetheless, the findings in this study are encouraging, providing preliminary evidence in support of individualized VRRS treatment coupled with tDCS and telerehabilitation for cognitive rehabilitation. Our results should pave the way for future studies aimed at identifying optimal treatment protocols for individuals with MCI. The combination of innovative technologies such as telerehabilitation and transcranial direct current stimulation may be particularly relevant for obtaining the best possible enhancement in subjects with limited access to therapy due to geographical distance, transport difficulties or a lack of local services.
In conclusion, although further research is needed, there is promising evidence for the implementation of transcranial current stimulation and telerehabilitation components in cognitive rehabilitation programs dedicated to individuals with MCI (Cotelli et al., 2019; Cacciante et al., 2022). Further studies are needed on the organizational aspects of TR service delivery, reimbursement for remotely delivered services, and ways to provide training for the involved health care personnel. A further development is the delivery of neurorehabilitation programs using noninvasive brain stimulation technology at home (Charvet et al., 2015, 2020; Pilloni et al., 2022).
Data availability statement
The raw data supporting the conclusions of this article will be made available by the authors, without undue reservation.
Ethics statement
The studies involving humans were approved by the local Ethics Committees. The studies were conducted in accordance with the local legislation and institutional requirements. The participants provided their written informed consent to participate in this study.
Author contributions
RM: Methodology, Conceptualization, Data curation, Investigation, Writing – original draft, Writing – review & editing. FB: Methodology, Conceptualization, Data curation, Investigation, Writing – review & editing. IP: Data curation, Investigation, Writing – original draft, Writing – review & editing. EG: Data curation, Investigation, Writing – original draft, Writing – review & editing. EC: Data curation, Investigation, Writing – original draft, Writing – review & editing. CA: Data curation, Investigation, Writing – review & editing. FR: Data curation, Investigation, Writing – review & editing. ST: Data curation, Investigation, Writing – review & editing. CP: Data curation, Investigation, Writing – review & editing. AG: Formal analysis, Writing – original draft, Writing – review & editing. NSB: Formal analysis, Writing – original draft, Writing – review & editing. RC: Data curation, Investigation, Writing – review & editing. VC: Investigation, Writing – review & editing. GB: Investigation, Writing – review & editing. AQ: Conceptualization, Methodology, Investigation, Writing – review & editing. PB: Conceptualization, Methodology, Investigation, Writing – review & editing. SC: Conceptualization, Methodology, Investigation, Writing – review & editing. PR: Investigation, Writing – review & editing. MC: Data curation, Investigation, Conceptualization, Methodology, Writing – original draft, Writing – review & editing.
Funding
The author(s) declare that financial support was received for the research, authorship, and/or publication of this article. This work has been supported by the Italian Ministry of Health (Ricerca Corrente and Rete IRCCS delle Neuroscienze e della Neuroriabilitazione – Teleneuroriabilitazione–RCR-2022-23682290; RCR-2019-23669119-008).
Conflict of interest
The authors declare that the research was conducted in the absence of any commercial or financial relationships that could be construed as a potential conflict of interest.
Publisher’s note
All claims expressed in this article are solely those of the authors and do not necessarily represent those of their affiliated organizations, or those of the publisher, the editors and the reviewers. Any product that may be evaluated in this article, or claim that may be made by its manufacturer, is not guaranteed or endorsed by the publisher.
Supplementary material
The Supplementary material for this article can be found online at: https://www.frontiersin.org/articles/10.3389/fnagi.2024.1414593/full#supplementary-material
Footnotes
2. ^Soterix Medical; https://soterixmedical.com.
3. ^https://training.cochrane.org/handbook/current/chapter-08
References
Abraha, I., and Montedori, A. (2010). Modified intention to treat reporting in randomised controlled trials: systematic review. BMJ 340:c2697. doi: 10.1136/bmj.c2697
Alaimo, C., Campana, E., Stoppelli, M. R., Gobbi, E., Baglio, F., Rossetto, F., et al. (2021). Cognitive tele-enhancement in healthy older adults and subjects with subjective memory complaints: a review. Front. Neurol. 12:650553. doi: 10.3389/fneur.2021.650553
American Psychiatric Association (2014). Manuale diagnostico e statistico dei disturbi mentali. Milano: Raffaele Cortina Editore.
Antal, A., Alekseichuk, I., Bikson, M., Brockmöller, J., Brunoni, A. R., Chen, R., et al. (2017). Low intensity transcranial electric stimulation: safety, ethical, legal regulatory and application guidelines. Clin. Neurophysiol. 128, 1774–1809. doi: 10.1016/j.clinph.2017.06.001
Antonietti, A., Gandolla, M., Rossini, M., Molteni, F., Pedrocchi, A., and Consortium, A. (2017). Interference between cognitive and motor recovery in elderly dementia patients through a holistic tele-rehabilitation platform. In: Wireless Mobile Communication and Healthcare: 6th International Conference, MobiHealth 2016, Milan, Italy, November 14–16, 2016, Proceedings 6: Springer, pp. 359–366.
Astell, A. J., Bouranis, N., Hoey, J., Lindauer, A., Mihailidis, A., Nugent, C., et al. (2019). Technology and dementia: the future is now. Dement. Geriatr. Cogn. Disord. 47, 131–139. doi: 10.1159/000497800
Bahar-Fuchs, A., Clare, L., and Woods, B. (2013a). Cognitive training and cognitive rehabilitation for mild to moderate Alzheimer's disease and vascular dementia. Cochrane Database Syst. Rev. 2013:Cd003260. doi: 10.1002/14651858.CD003260.pub2
Bahar-Fuchs, A., Clare, L., and Woods, B. (2013b). Cognitive training and cognitive rehabilitation for persons with mild to moderate dementia of the Alzheimer's or vascular type: a review. J Alzheimers Res. Ther. 5, 35–14. doi: 10.1186/alzrt189
Bahar-Fuchs, A., Martyr, A., Goh, A. M., Sabates, J., and Clare, L. (2019). Cognitive training for people with mild to moderate dementia. Cochrane Database Syst. Rev. 3:Cd013069. doi: 10.1002/14651858.CD013069.pub2
Bangor, A., Kortum, P. T., and Miller, J. T. (2008). An empirical evaluation of the system usability scale. Int. J. Hum. Comput. Interact. 24, 574–594. doi: 10.1080/10447310802205776
Bangor, A., Kortum, P., and Miller, J. (2009). Determining what individual SUS scores mean: adding an adjective rating scale. J. Usability Stud. 4, 114–123.
Basso, A., Capitani, E., and Laiacona, M. (1987). Raven's coloured progressive matrices: normative values on 305 adult normal controls. Funct. Neurol. 2, 189–194
Bernini, S., Panzarasa, S., Quaglini, S., Costa, A., Picascia, M., Cappa, S. F., et al. (2023). HomeCoRe system for telerehabilitation in individuals at risk of dementia: a usability and user experience study. Front. Med. (Lausanne) 10:1129914. doi: 10.3389/fmed.2023.1129914
Bharucha, A. J., Anand, V., Forlizzi, J., Dew, M. A., Reynolds, C. F. 3rd, Stevens, S., et al. (2009). Intelligent assistive technology applications to dementia care: current capabilities, limitations, and future challenges. Am. J. Geriatr. Psychiatry 17, 88–104. doi: 10.1097/JGP.0b013e318187dde5
Bianchetti, A., Cornali, C., Ranieri, P., and Trabucchi, M. (2017). Quality of life in patients with mild dementia. Validation of the Italian version of the quality of life Alzheimer’s disease (QoL-AD) scale. Official J. Ital. Soc. Gerontol. Geriatr. 137:11.
Bikson, M., Grossman, P., Thomas, C., Zannou, A. L., Jiang, J., Adnan, T., et al. (2016). Safety of transcranial direct current stimulation: evidence based update 2016. Brain Stimul. 9, 641–661. doi: 10.1016/j.brs.2016.06.004
Binetti, G., Mega, M. S., Magni, E., Padovani, A., Rozzini, L., Bianchetti, A., et al. (1998). Behavioral disorders in Alzheimer disease: a transcultural perspective. Arch. Neurol. 55, 539–544. doi: 10.1001/archneur.55.4.539
Botsis, T., Demiris, G., Pedersen, S., and Hartvigsen, G. (2008). Home telecare technologies for the elderly. J. Telemed. Telecare 14, 333–337. doi: 10.1258/jtt.2008.007002
Boutron, I., Altman, D. G., Moher, D., Schulz, K. F., and Ravaud, P. (2017). CONSORT statement for randomized trials of nonpharmacologic treatments: a 2017 update and a CONSORT extension for nonpharmacologic trial abstracts. Ann. Intern. Med. 167, 40–47. doi: 10.7326/m17-0046
Boutron, I., Moher, D., Altman, D. G., Schulz, K. F., and Ravaud, P. (2008). Extending the CONSORT statement to randomized trials of nonpharmacologic treatment: explanation and elaboration. Ann. Intern. Med. 148, 295–309. doi: 10.7326/0003-4819-148-4-200802190-00008
Brambilla, M., Manenti, R., Ferrari, C., and Cotelli, M. (2015). Better together: left and right hemisphere engagement to reduce age-related memory loss. Behav. Brain Res. 293, 125–133. doi: 10.1016/j.bbr.2015.07.037
Brem, A. K., Di Iorio, R., Fried, P. J., Oliveira-Maia, A. J., Marra, C., Profice, P., et al. (2020). Corticomotor plasticity predicts clinical efficacy of combined Neuromodulation and cognitive training in Alzheimer's disease. Front. Aging Neurosci. 12:200. doi: 10.3389/fnagi.2020.00200
Brennan, D., Georgeadis, A., and Baron, C. (2002). Telerehabilitation tools for the provision of remote speech-language treatment. Top. Stroke Rehabil. 8, 71–78. doi: 10.1310/u7kv-dy7u-q6qp-lvbp
Brennan, D. M., Mawson, S., and Brownsell, S. (2009). Telerehabilitation: enabling the remote delivery of healthcare, rehabilitation, and self management. Stud. Health Technol. Inform. 145, 231–248. doi: 10.3233/978-1-60750-018-6-231
Brennan, D. M., Tindall, L., Theodoros, D., Brown, J., Campbell, M., Christiana, D., et al. (2011). A blueprint for telerehabilitation guidelines—October 2010. J. Telemed. E Health 17, 662–665. doi: 10.1089/tmj.2011.0036
Brooke, J. (1996). “SUS-A quick and dirty usability scale” in Usability evaluation in industry. ed. J. Brooke, vol. 189 (Milton Park: Taylor and Francis), 4–7.
Brunoni, A. R., Nitsche, M. A., Bolognini, N., Bikson, M., Wagner, T., Merabet, L., et al. (2012). Clinical research with transcranial direct current stimulation (tDCS): challenges and future directions. Brain Stimul. 5, 175–195. doi: 10.1016/j.brs.2011.03.002
Burton, R. L., and O'Connell, M. E. (2018). Telehealth rehabilitation for cognitive impairment: randomized controlled feasibility trial. JMIR Res. Protoc. 7:e43. doi: 10.2196/resprot.9420
Buschke, H. (1984). Cued recall in amnesia. J. Clin. Exp. Neuropsychol. 6, 433–440. doi: 10.1080/01688638408401233
Cacciante, L., Pietà, C. D., Rutkowski, S., Cieślik, B., Szczepańska-Gieracha, J., Agostini, M., et al. (2022). Cognitive telerehabilitation in neurological patients: systematic review and meta-analysis. Neurol. Sci. 43, 847–862. doi: 10.1007/s10072-021-05770-6
Caffarra, P., Vezzadini, G., Dieci, F., Zonato, F., and Venneri, A. (2002). Rey-Osterrieth complex figure: normative values in an Italian population sample. Neurol. Sci. 22, 443–447. doi: 10.1007/s100720200003
Calabria, M., Manenti, R., Rosini, S., Zanetti, O., Miniussi, C., and Cotelli, M. (2011). Objective and subjective memory impairment in elderly adults: a revised version of the everyday memory questionnaire. Aging Clin. Exp. Res. 23, 67–73. doi: 10.1007/bf03324954
Cappa, S. F., Benke, T., Clarke, S., Rossi, B., Stemmer, B., and van Heugten, C. M. (2003). EFNS guidelines on cognitive rehabilitation: report of an EFNS task force. Eur. J. Neurol. 10, 11–23. doi: 10.1046/j.1468-1331.2003.00537.x
Cappa, S. F., Benke, T., Clarke, S., Rossi, B., Stemmer, B., and van Heugten, C. M. (2005). EFNS guidelines on cognitive rehabilitation: report of an EFNS task force. Eur. J. Neurol. 12, 665–680. doi: 10.1111/j.1468-1331.2005.01330.x
Carlesimo, G. A., Caltagirone, C., and Gainotti, G. (1996). The mental deterioration battery: normative data, diagnostic reliability and qualitative analyses of cognitive impairment. The Group for the Standardization of the mental deterioration battery. Eur. Neurol. 36, 378–384. doi: 10.1159/000117297
Chan, A. T. C., Ip, R. T. F., Tran, J. Y. S., Chan, J. Y. C., and Tsoi, K. K. F. (2024). Computerized cognitive training for memory functions in mild cognitive impairment or dementia: a systematic review and meta-analysis. NPJ Digit. Med. 7:1. doi: 10.1038/s41746-023-00987-5
Charvet, L. E., Kasschau, M., Datta, A., Knotkova, H., Stevens, M. C., Alonzo, A., et al. (2015). Remotely-supervised transcranial direct current stimulation (tDCS) for clinical trials: guidelines for technology and protocols. Front. Syst. Neurosci. 9:26. doi: 10.3389/fnsys.2015.00026
Charvet, L. E., Shaw, M. T., Bikson, M., Woods, A. J., and Knotkova, H. (2020). Supervised transcranial direct current stimulation (tDCS) at home: a guide for clinical research and practice. Brain Stimul. 13, 686–693. doi: 10.1016/j.brs.2020.02.011
Cherney, L. R., and van Vuuren, S. (2012). Telerehabilitation, virtual therapists, and acquired neurologic speech and language disorders. Semin. Speech Lang. 33, 243–257. doi: 10.1055/s-0032-1320044
Cieza, A., Causey, K., Kamenov, K., Hanson, S. W., Chatterji, S., and Vos, T. (2021). Global estimates of the need for rehabilitation based on the global burden of disease study 2019: a systematic analysis for the global burden of disease study 2019. Lancet 396, 2006–2017. doi: 10.1016/s0140-6736(20)32340-0
Clare, L. (2017). Rehabilitation for people living with dementia: a practical framework of positive support. PLoS Med. 14:e1002245. doi: 10.1371/journal.pmed.1002245
Clare, L., Linden, D. E., Woods, R. T., Whitaker, R., Evans, S. J., Parkinson, C. H., et al. (2010). Goal-oriented cognitive rehabilitation for people with early-stage Alzheimer disease: a single-blind randomized controlled trial of clinical efficacy. Am. J. Geriatr. Psychiatry 18, 928–939. doi: 10.1097/JGP.0b013e3181d5792a
Clare, L., and Woods, R. T. (2004). Cognitive training and cognitive rehabilitation for people with early-stage Alzheimer's disease: a review. J. Neuropsychol. Rehabil. 14, 385–401. doi: 10.1080/09602010443000074
Clerici, F., Ghiretti, R., Di Pucchio, A., Pomati, S., Cucumo, V., Marcone, A., et al. (2017). Construct validity of the free and cued selective reminding test in older adults with memory complaints. J. Neuropsychol. 11, 238–251. doi: 10.1111/jnp.12087
Corbett, A., Owen, A., Hampshire, A., Grahn, J., Stenton, R., Dajani, S., et al. (2015). The effect of an online cognitive training package in healthy older adults: an online randomized controlled trial. J. Am. Med. Dir. Assoc. 16, 990–997. doi: 10.1016/j.jamda.2015.06.014
Corbetta, D., Imeri, F., and Gatti, R. (2015). Rehabilitation that incorporates virtual reality is more effective than standard rehabilitation for improving walking speed, balance and mobility after stroke: a systematic review. J. Physiother. 61, 117–124. doi: 10.1016/j.jphys.2015.05.017
Cotelli, M., Calabria, M., and Zanetti, O. (2006). Cognitive rehabilitation in Alzheimer's disease. Aging Clin. Exp. Res. 18, 141–143. doi: 10.1007/bf03327429
Cotelli, M., Manenti, R., Alberici, A., Brambilla, M., Cosseddu, M., Zanetti, O., et al. (2012). Prefrontal cortex rTMS enhances action naming in progressive non-fluent aphasia. Eur. J. Neurol. 19, 1404–1412. doi: 10.1111/j.1468-1331.2012.03699.x
Cotelli, M., Manenti, R., Brambilla, M., Gobbi, E., Ferrari, C., Binetti, G., et al. (2019). Cognitive telerehabilitation in mild cognitive impairment, Alzheimer's disease and frontotemporal dementia: a systematic review. J. Telemed. Telecare 25, 67–79. doi: 10.1177/1357633x17740390
Cotelli, M., Manenti, R., Ferrari, C., Gobbi, E., Macis, A., and Cappa, S. F. (2020). Effectiveness of language training and non-invasive brain stimulation on oral and written naming performance in primary progressive aphasia: a meta-analysis and systematic review. Neurosci. Biobehav. Rev. 108, 498–525. doi: 10.1016/j.neubiorev.2019.12.003
Cruse, N., Piotto, V., Coelho, C., and Behn, N. (2022). Telehealth administration of narrative and procedural discourse: a UK and US comparison of traumatic brain injury and matched controls. Int. J. Lang. Commun. Disord. 59, 519–531. doi: 10.1111/1460-6984.12813
Cummings, J. L., Mega, M., Gray, K., Rosenberg-Thompson, S., Carusi, D. A., and Gornbein, J. (1994). The neuropsychiatric inventory: comprehensive assessment of psychopathology in dementia. Neurology 44, 2308–2314. doi: 10.1212/wnl.44.12.2308
Das, N., Spence, J. S., Aslan, S., Vanneste, S., Mudar, R., Rackley, A., et al. (2019). Cognitive training and transcranial direct current stimulation in mild cognitive impairment: a randomized pilot trial. Front. Neurosci. 13:307. doi: 10.3389/fnins.2019.00307
Dávalos, M. E., French, M. T., Burdick, A. E., and Simmons, S. C. (2009). Economic evaluation of telemedicine: review of the literature and research guidelines for benefit-cost analysis. Telemed. J. E Health 15, 933–948. doi: 10.1089/tmj.2009.0067
Dayan, E., Censor, N., Buch, E. R., Sandrini, M., and Cohen, L. G. (2013). Noninvasive brain stimulation: from physiology to network dynamics and back. Nat. Neurosci. 16, 838–844. doi: 10.1038/nn.3422
De Cola, M. C., Maresca, G., D'Aleo, G., Carnazza, L., Giliberto, S., Maggio, M. G., et al. (2020). Teleassistance for frail elderly people: a usability and customer satisfaction study. Geriatr. Nurs. 41, 463–467. doi: 10.1016/j.gerinurse.2020.01.019
Dubois, B., Feldman, H. H., Jacova, C., DeKosky, S. T., Barberger-Gateau, P., Cummings, J., et al. (2007). Research criteria for the diagnosis of Alzheimer's disease: revising the NINCDS–ADRDA criteria. Lancet Neurol. 6, 734–746. doi: 10.1016/S1474-4422(07)70178-3
Fertonani, A., Ferrari, C., and Miniussi, C. (2015). What do you feel if I apply transcranial electric stimulation? Safety, sensations and secondary induced effects. Clin. Neurophysiol. 126, 2181–2188. doi: 10.1016/j.clinph.2015.03.015
Fileccia, E., Di Stasi, V., Poda, R., Rizzo, G., Stanzani-Maserati, M., Oppi, F., et al. (2019). Effects on cognition of 20-day anodal transcranial direct current stimulation over the left dorsolateral prefrontal cortex in patients affected by mild cognitive impairment: a case-control study. Neurol. Sci. 40, 1865–1872. doi: 10.1007/s10072-019-03903-6
Fletcher, P. C., and Henson, R. N. (2001). Frontal lobes and human memory: insights from functional neuroimaging. Brain 124, 849–881. doi: 10.1093/brain/124.5.849
Folstein, M. F., Folstein, S. E., and McHugh, P. R. (1975). “Mini-mental state”: a practical method for grading the cognitive state of patients for the clinician. J. Psychiatr. Res. 12, 189–198. doi: 10.1016/0022-3956(75)90026-6
Frasson, P., Ghiretti, R., Catricalà, E., Pomati, S., Marcone, A., Parisi, L., et al. (2011). Free and cued selective reminding test: an Italian normative study. Neurol. Sci. 32, 1057–1062. doi: 10.1007/s10072-011-0607-3
Fregni, F., El-Hagrassy, M. M., Pacheco-Barrios, K., Carvalho, S., Leite, J., Simis, M., et al. (2021). Evidence-based guidelines and secondary Meta-analysis for the use of transcranial direct current stimulation in neurological and psychiatric disorders. Int. J. Neuropsychopharmacol. 24, 256–313. doi: 10.1093/ijnp/pyaa051
Frisoni, G. B., Altomare, D., Ribaldi, F., Villain, N., Brayne, C., Mukadam, N., et al. (2023). Dementia prevention in memory clinics: recommendations from the European task force for brain health services. Lancet Reg. Health Eur. 26:100576. doi: 10.1016/j.lanepe.2022.100576
Gates, N. J., and Sachdev, P. (2014). Is cognitive training an effective treatment for preclinical and early Alzheimer's disease? J. Alzheimers Dis. 42, S551–S559. doi: 10.3233/jad-141302
GBD 2017 DALYs and HALE Collaborators (2018). Global, regional, and national disability-adjusted life-years (DALYs) for 359 diseases and injuries and healthy life expectancy (HALE) for 195 countries and territories, 1990-2017: a systematic analysis for the global burden of disease study 2017. Lancet 392, 1859–1922. doi: 10.1016/s0140-6736(18)32335-3
GBD 2019 Diseases and Injuries Collaborators (2020). Global burden of 369 diseases and injuries in 204 countries and territories, 1990-2019: a systematic analysis for the global burden of disease study 2019. Lancet 396, 1204–1222. doi: 10.1016/s0140-6736(20)30925-9
GBD 2021 Nervous System Disorders Collaborators (2024). Global, regional, and national burden of disorders affecting the nervous system, 1990-2021: a systematic analysis for the global burden of disease study 2021. Lancet Neurol. 23, 344–381. doi: 10.1016/S1474-4422(24)00038-3
Giovagnoli, A. R., Del Pesce, M., Mascheroni, S., Simoncelli, M., Laiacona, M., and Capitani, E. (1996). Trail making test: normative values from 287 normal adult controls. Ital. J. Neurol. Sci. 17, 305–309. doi: 10.1007/bf01997792
Gomes, M. A., Akiba, H. T., Gomes, J. S., Trevizol, A. P., de Lacerda, A. L. T., Dias, Á., et al. (2019). Transcranial direct current stimulation (tDCS) in elderly with mild cognitive impairment: a pilot study. Dement. Neuropsychol. 13, 187–195. doi: 10.1590/1980-57642018dn13-020007
Grober, E., Lipton, R. B., Hall, C., and Crystal, H. (2000). Memory impairment on free and cued selective reminding predicts dementia. Neurology 54, 827–832. doi: 10.1212/wnl.54.4.827
Grober, E., Merling, A., Heimlich, T., and Lipton, R. B. (1997). Free and cued selective reminding and selective reminding in the elderly. J. Clin. Exp. Neuropsychol. 19, 643–654. doi: 10.1080/01688639708403750
Gu, J., Li, D., Li, Z., Guo, Y., Qian, F., Wang, Y., et al. (2022). The effect and mechanism of transcranial direct current stimulation on episodic memory in patients with mild cognitive impairment. Front. Neurosci. 16:811403. doi: 10.3389/fnins.2022.811403
Hong, Y. J., Jang, E. H., Hwang, J., Roh, J. H., and Lee, J. H. (2015). The efficacy of cognitive intervention programs for mild cognitive impairment: a systematic review. Curr. Alzheimer Res. 12, 527–542. doi: 10.2174/1567205012666150530201636
Hsu, W. Y., Ku, Y., Zanto, T. P., and Gazzaley, A. (2015). Effects of noninvasive brain stimulation on cognitive function in healthy aging and Alzheimer's disease: a systematic review and meta-analysis. Neurobiol. Aging 36, 2348–2359. doi: 10.1016/j.neurobiolaging.2015.04.016
Hu, M., Hu, H., Shao, Z., Gao, Y., Zeng, X., Shu, X., et al. (2022). Effectiveness and acceptability of non-pharmacological interventions in people with mild cognitive impairment: overview of systematic reviews and network meta-analysis. J. Affect. Disord. 311, 383–390. doi: 10.1016/j.jad.2022.05.043
Isernia, S., Di Tella, S., Pagliari, C., Jonsdottir, J., Castiglioni, C., Gindri, P., et al. (2020). Effects of an innovative Telerehabilitation intervention for people with Parkinson's disease on quality of life, motor, and non-motor abilities. Front. Neurol. 11:846. doi: 10.3389/fneur.2020.00846
Isernia, S., Pagliari, C., Jonsdottir, J., Castiglioni, C., Gindri, P., Gramigna, C., et al. (2019). Efficiency and patient-reported outcome measures from clinic to home: the human empowerment aging and disability program for digital-health rehabilitation. Front. Neurol. 10:1206. doi: 10.3389/fneur.2019.01206
Jelcic, N., Agostini, M., Meneghello, F., Bussè, C., Parise, S., Galano, A., et al. (2014). Feasibility and efficacy of cognitive telerehabilitation in early Alzheimer's disease: a pilot study. Clin. Interv. Aging 9, 1605–1611. doi: 10.2147/cia.S68145
Kairy, D., Lehoux, P., Vincent, C., and Visintin, M. (2009). A systematic review of clinical outcomes, clinical process, healthcare utilization and costs associated with telerehabilitation. Disabil. Rehabil. 31, 427–447. doi: 10.1080/09638280802062553
Katz, S. (1983). Assessing self-maintenance: activities of daily living, mobility, and instrumental activities of daily living. J. Am. Geriatr. Soc. 31, 721–727. doi: 10.1111/j.1532-5415.1983.tb03391.x
Kortte, K. B., and Rogalski, E. J. (2013). Behavioural interventions for enhancing life participation in behavioural variant frontotemporal dementia and primary progressive aphasia. Int. Rev. Psychiatry 25, 237–245. doi: 10.3109/09540261.2012.751017
Krebs, C., Klöppel, S., Heimbach, B., and Peter, J. (2020). Education moderates the effect of tDCS on episodic memory performance in cognitively impaired patients. Brain Stimul. 13, 1396–1398. doi: 10.1016/j.brs.2020.07.008
Kudlicka, A., Martyr, A., Bahar-Fuchs, A., Sabates, J., Woods, B., and Clare, L. (2023). Cognitive rehabilitation for people with mild to moderate dementia. Cochrane Database Syst. Rev. 2023:3388. doi: 10.1002/14651858.CD013388.pub2
Lawson, D. W., Stolwyk, R. J., Ponsford, J. L., McKenzie, D. P., Downing, M. G., and Wong, D. (2020). Telehealth delivery of memory rehabilitation following stroke. J. Int. Neuropsychol. Soc. 26, 58–71. doi: 10.1017/s1355617719000651
Lawton, M., and Brody, E. (1988). Instrumental activities of daily living (Iadl) scale-self-rated version. Psychopharmacol. Bull. 24, 789–791.
Lefaucheur, J. P., Antal, A., Ayache, S. S., Benninger, D. H., Brunelin, J., Cogiamanian, F., et al. (2017). Evidence-based guidelines on the therapeutic use of transcranial direct current stimulation (tDCS). Clin. Neurophysiol. 128, 56–92. doi: 10.1016/j.clinph.2016.10.087
Lemos, R., Simões, M. R., Santiago, B., and Santana, I. (2015). The free and cued selective reminding test: validation for mild cognitive impairment and Alzheimer's disease. J. Neuropsychol. 9, 242–257. doi: 10.1111/jnp.12048
Lezak, M., Howieson, D., Bigler, E., and Tranel, D. (2012). Neuropsychological assessment. 5th Edn. Oxford: University Press.
Livingston, G., Sommerlad, A., Orgeta, V., Costafreda, S. G., Huntley, J., Ames, D., et al. (2017). Dementia prevention, intervention, and care. Lancet 390, 2673–2734. doi: 10.1016/s0140-6736(17)31363-6
Lu, H., Chan, S. S. M., Chan, W. C., Lin, C., Cheng, C. P. W., and Linda Chiu Wa, L. (2019). Randomized controlled trial of TDCS on cognition in 201 seniors with mild neurocognitive disorder. Ann. Clin. Transl. Neurol. 6, 1938–1948. doi: 10.1002/acn3.50823
Maggio, M. G., Baglio, F., Arcuri, F., Borgnis, F., Contrada, M., Maldonado Diaz, M. D., et al. (2024). Cognitive Telerehabilitation: an expert consensus paper on current evidence and future perspective. Front. Neurol. 15:1338873. doi: 10.3389/fneur.2024.1338873
Maggio, M. G., De Bartolo, D., Calabrò, R. S., Ciancarelli, I., Cerasa, A., Tonin, P., et al. (2023). Computer-assisted cognitive rehabilitation in neurological patients: state-of-art and future perspectives. Front. Neurol. 14:1255319. doi: 10.3389/fneur.2023.1255319
Manenti, R., Brambilla, M., Petesi, M., Ferrari, C., and Cotelli, M. (2013). Enhancing verbal episodic memory in older and young subjects after non-invasive brain stimulation. Front. Aging Neurosci. 5:49. doi: 10.3389/fnagi.2013.00049
Manenti, R., Cotelli, M., Calabria, M., Maioli, C., and Miniussi, C. (2010). The role of the dorsolateral prefrontal cortex in retrieval from long-term memory depends on strategies: a repetitive transcranial magnetic stimulation study. Neuroscience 166, 501–507. doi: 10.1016/j.neuroscience.2009.12.037
Manenti, R., Cotelli, M., and Miniussi, C. (2011). Successful physiological aging and episodic memory: a brain stimulation study. Behav. Brain Res. 216, 153–158. doi: 10.1016/j.bbr.2010.07.027
Manenti, R., Cotelli, M., Robertson, I. H., and Miniussi, C. (2012). Transcranial brain stimulation studies of episodic memory in young adults, elderly adults and individuals with memory dysfunction: a review. Brain Stimul. 5, 103–109. doi: 10.1016/j.brs.2012.03.004
Manenti, R., Gobbi, E., Baglio, F., Macis, A., Ferrari, C., Pagnoni, I., et al. (2020a). Effectiveness of an innovative cognitive treatment and Telerehabilitation on subjects with mild cognitive impairment: a multicenter, randomized, active-controlled study. Front. Aging Neurosci. 12:585988. doi: 10.3389/fnagi.2020.585988
Manenti, R., Sandrini, M., Brambilla, M., and Cotelli, M. (2016). The optimal timing of stimulation to induce long-lasting positive effects on episodic memory in physiological aging. Behav. Brain Res. 311, 81–86. doi: 10.1016/j.bbr.2016.05.028
Manenti, R., Sandrini, M., Gobbi, E., Binetti, G., and Cotelli, M. (2020b). Effects of transcranial direct current stimulation on episodic memory in amnestic mild cognitive impairment: a pilot study. J. Gerontol. B Psychol. Sci. Soc. Sci. 75, 1403–1413. doi: 10.1093/geronb/gby134
Manenti, R., Sandrini, M., Gobbi, E., Cobelli, C., Brambilla, M., Binetti, G., et al. (2017). Strengthening of existing episodic memories through non-invasive stimulation of prefrontal cortex in older adults with subjective memory complaints. Front. Aging Neurosci. 9:401. doi: 10.3389/fnagi.2017.00401
Maresca, G., Maggio, M. G., De Luca, R., Manuli, A., Tonin, P., Pignolo, L., et al. (2020). Tele-neuro-rehabilitation in Italy: state of the art and future perspectives. Front. Neurol. 11:563375. doi: 10.3389/fneur.2020.563375
Marion, J. D., and Althouse, A. D. (2023). The use of historical controls in clinical trials. JAMA 330, 1484–1485. doi: 10.1001/jama.2023.16182
Mashima, P. A., and Doarn, C. R. (2008). Overview of telehealth activities in speech-language pathology. Telemed. J. E Health 14, 1101–1117. doi: 10.1089/tmj.2008.0080
Matamala-Gomez, M., Maisto, M., Montana, J. I., Mavrodiev, P. A., Baglio, F., Rossetto, F., et al. (2020). The role of engagement in Teleneurorehabilitation: a systematic review. Front. Neurol. 11:354. doi: 10.3389/fneur.2020.00354
McCue, M., Fairman, A., and Pramuka, M. (2010). Enhancing quality of life through telerehabilitation. Phys. Med. Rehabil. Clin. N. Am. 21, 195–205. doi: 10.1016/j.pmr.2009.07.005
Menardi, A., Rossi, S., Koch, G., Hampel, H., Vergallo, A., Nitsche, M. A., et al. (2022). Toward noninvasive brain stimulation 2.0 in Alzheimer's disease. Ageing Res. Rev. 75:101555. doi: 10.1016/j.arr.2021.101555
Menengi̇ç, K. N., Yeldan, İ., Çınar, N., and Şahiner, T. (2022). Effectiveness of motor-cognitive dual-task exercise via telerehabilitation in Alzheimer’s disease: an online pilot randomized controlled study. Clin. Neurol. Neurosurg. 223:107501. doi: 10.1016/j.clineuro.2022.107501
Miceli, G., Laudanna, A., Burani, C., and Capasso, R. (1994). Batteria per l'Analisi dei Deficit Afasici (BADA). CEPSAG: Universita Cattolica del Sacro Cuore, Rome.
Morris, J. C. (1997). Clinical dementia rating: a reliable and valid diagnostic and staging measure for dementia of the Alzheimer type. Int. Psychogeriatr. 9, 173–176. doi: 10.1017/s1041610297004870
Mosca, I. E., Salvadori, E., Gerli, F., Fabbri, L., Pancani, S., Lucidi, G., et al. (2020). Analysis of feasibility, adherence, and appreciation of a newly developed tele-rehabilitation program for people with MCI and VCI. Front. Neurol. 11:583368. doi: 10.3389/fneur.2020.583368
Moyle, W. (2019). The promise of technology in the future of dementia care. Nat. Rev. Neurol. 15, 353–359. doi: 10.1038/s41582-019-0188-y
Murugaraja, V., Shivakumar, V., Sivakumar, P. T., Sinha, P., and Venkatasubramanian, G. (2017). Clinical utility and tolerability of transcranial direct current stimulation in mild cognitive impairment. Asian J. Psychiatr. 30, 135–140. doi: 10.1016/j.ajp.2017.09.001
Nissim, N. R., Moberg, P. J., and Hamilton, R. H. (2020). Efficacy of noninvasive brain stimulation (tDCS or TMS) paired with language therapy in the treatment of primary progressive aphasia: an exploratory Meta-analysis. Brain Sci. 10:597. doi: 10.3390/brainsci10090597
Nousia, A., Martzoukou, M., Siokas, V., Aretouli, E., Aloizou, A. M., Folia, V., et al. (2021). Beneficial effect of computer-based multidomain cognitive training in patients with mild cognitive impairment. Appl. Neuropsychol. Adult 28, 717–726. doi: 10.1080/23279095.2019.1692842
Novelli, G., Papagno, C., Capitani, E., and Laiacona, M. (1986). Tre test clinici di ricerca e produzione lessicale Taratura su sogetti normali Archivio di psicologia, neurologia e psichiatria.
Nucci, M., Mapelli, D., and Mondini, S. (2012). Cognitive reserve index questionnaire (CRIq): a new instrument for measuring cognitive reserve. Aging Clin. Exp. Res. 24, 218–226. doi: 10.1007/BF03654795
Oldfield, R. C. (1971). The assessment and analysis of handedness: the Edinburgh inventory. Neuropsychologia 9, 97–113. doi: 10.1016/0028-3932(71)90067-4
Pagliari, C., Di Tella, S., Jonsdottir, J., Mendozzi, L., Rovaris, M., De Icco, R., et al. (2024). Effects of home-based virtual reality telerehabilitation system in people with multiple sclerosis: a randomized controlled trial. J. Telemed. Telecare 30, 344–355. doi: 10.1177/1357633x211054839
Palimariciuc, M., Oprea, D. C., Cristofor, A. C., Florea, T., Dobrin, R. P., Dobrin, I., et al. (2023). The effects of transcranial direct current stimulation in patients with mild cognitive impairment. Neurol. Int. 15, 1423–1442. doi: 10.3390/neurolint15040092
Parsons, T. D. (2016). Neuropsychological rehabilitation 3.0: state of the science. Clin. Neuropsychol. Technol., 113–132. doi: 10.1007/978-3-319-31075-6_7
Pasquier, F., Grymonprez, L., Lebert, F., and Van der Linden, M. (2001). Memory impairment differs in frontotemporal dementia and Alzheimer's disease. Neurocase 7, 161–171. doi: 10.1093/neucas/7.2.161
Peres, S. C., Pham, T., and Phillips, R. (2013). Validation of the system usability scale (SUS) SUS in the wild. In: Proceedings of the Human Factors and Ergonomics Society Annual Meeting: SAGE Publications Sage CA: Los Angeles, CA, pp. 192–196.
Peretti, A., Amenta, F., Tayebati, S. K., Nittari, G., and Mahdi, S. S. (2017). Telerehabilitation: review of the state-of-the-art and areas of application. JMIR Rehabil. Assist. Technol. 4:e7. doi: 10.2196/rehab.7511
Pergher, V., Au, J., Alizadeh Shalchy, M., Santarnecchi, E., Seitz, A., Jaeggi, S. M., et al. (2022). The benefits of simultaneous tDCS and working memory training on transfer outcomes: a systematic review and meta-analysis. Brain Stimul. 15, 1541–1551. doi: 10.1016/j.brs.2022.11.008
Petersen, R. C. (2004). Mild cognitive impairment as a diagnostic entity. J. Intern. Med. 256, 183–194. doi: 10.1111/j.1365-2796.2004.01388.x
Petersen, R. C. (2011). Clinical practice. Mild cognitive impairment. N. Engl. J. Med. 364, 2227–2234. doi: 10.1056/NEJMcp0910237
Petersen, R. C., Caracciolo, B., Brayne, C., Gauthier, S., Jelic, V., and Fratiglioni, L. (2014). Mild cognitive impairment: a concept in evolution. J. Intern. Med. 275, 214–228. doi: 10.1111/joim.12190
Petersen, R. C., Smith, G. E., Waring, S. C., Ivnik, R. J., Tangalos, E. G., and Kokmen, E. (1999). Mild cognitive impairment: clinical characterization and outcome. Arch. Neurol. 56, 303–308. doi: 10.1001/archneur.56.3.303
Pillon, B., Deweer, B., Michon, A., Malapani, C., Agid, Y., and Dubois, B. (1994). Are explicit memory disorders of progressive supranuclear palsy related to damage to striatofrontal circuits? Comparison with Alzheimer's, Parkinson's, and Huntington's diseases. Neurology 44, 1264–1270. doi: 10.1212/wnl.44.7.1264
Pilloni, G., Vogel-Eyny, A., Lustberg, M., Best, P., Malik, M., Walton-Masters, L., et al. (2022). Tolerability and feasibility of at-home remotely supervised transcranial direct current stimulation (RS-tDCS): single-center evidence from 6,779 sessions. Brain Stimul. 15, 707–716. doi: 10.1016/j.brs.2022.04.014
Pitt, R., Theodoros, D., Hill, A. J., and Russell, T. (2019). The impact of the telerehabilitation group aphasia intervention and networking programme on communication, participation, and quality of life in people with aphasia. Int. J. Speech Lang. Pathol. 21, 513–523. doi: 10.1080/17549507.2018.1488990
Poon, P., Hui, E., Dai, D., Kwok, T., and Woo, J. (2005). Cognitive intervention for community-dwelling older persons with memory problems: telemedicine versus face-to-face treatment. Int. J. Geriatr. Psychiatry 20, 285–286. doi: 10.1002/gps.1282
Prehn, K., and Flöel, A. (2015). Potentials and limits to enhance cognitive functions in healthy and pathological aging by tDCS. Front. Cell. Neurosci. 9:355. doi: 10.3389/fncel.2015.00355
R Core Team (2013). R: A language and environment for statistical computing. Vienna: R Foundation for Statistical Computing.
Rai, H., Yates, L., and Orrell, M. (2018). Cognitive stimulation therapy for dementia. Clin. Geriatr. Med. 34, 653–665. doi: 10.1016/j.cger.2018.06.010
Realdon, O., Adorni, R., Ginelli, D., Micucci, D., Blasi, V., Bellavia, D., et al. (2023). Embedding the patient-citizen perspective into an operational framework for the development and the introduction of new Technologies in Rehabilitation Care: the Smart&Touch-ID model. Healthcare (Basel) 11:604. doi: 10.3390/healthcare11111604
Realdon, O., Rossetto, F., Nalin, M., Baroni, I., Cabinio, M., Fioravanti, R., et al. (2016). Technology-enhanced multi-domain at home continuum of care program with respect to usual care for people with cognitive impairment: the ability-TelerehABILITation study protocol for a randomized controlled trial. BMC Psychiatry 16:425. doi: 10.1186/s12888-016-1132-y
Rogante, M., Grigioni, M., Cordella, D., and Giacomozzi, C. (2010). Ten years of telerehabilitation: a literature overview of technologies and clinical applications. NeuroRehabilitation 27, 287–304. doi: 10.3233/nre-2010-0612
Rönnlund, M., Nyberg, L., Bäckman, L., and Nilsson, L. G. (2005). Stability, growth, and decline in adult life span development of declarative memory: cross-sectional and longitudinal data from a population-based study. Psychol. Aging 20, 3–18. doi: 10.1037/0882-7974.20.1.3
Rosen, M. J. (2004). Telerehabilitation. Telemed. J. E Health 10, 115–117. doi: 10.1089/tmj.2004.10.115
Rossetto, F., Isernia, S., Realdon, O., Borgnis, F., Blasi, V., Pagliari, C., et al. (2023). A digital health home intervention for people within the Alzheimer's disease continuum: results from the ability-TelerehABILITation pilot randomized controlled trial. Ann. Med. 55, 1080–1091. doi: 10.1080/07853890.2023.2185672
Rossi, S., Cappa, S. F., Babiloni, C., Pasqualetti, P., Miniussi, C., Carducci, F., et al. (2001). Prefrontal [correction of Prefontal] cortex in long-term memory: an “interference” approach using magnetic stimulation. Nat. Neurosci. 4, 948–952. doi: 10.1038/nn0901-948
Rossi, S., Miniussi, C., Pasqualetti, P., Babiloni, C., Rossini, P. M., and Cappa, S. F. (2004). Age-related functional changes of prefrontal cortex in long-term memory: a repetitive transcranial magnetic stimulation study. J. Neurosci. 24, 7939–7944. doi: 10.1523/JNEUROSCI.0703-04.2004
Rossi, S., Pasqualetti, P., Zito, G., Vecchio, F., Cappa, S. F., Miniussi, C., et al. (2006). Prefrontal and parietal cortex in human episodic memory: an interference study by repetitive transcranial magnetic stimulation. Eur. J. Neurosci. 23, 793–800. doi: 10.1111/j.1460-9568.2006.04600.x
Salthouse, T. A. (2011). Neuroanatomical substrates of age-related cognitive decline. J. Psychol. Bull. 137, 753–784. doi: 10.1037/a0023262
Sandrini, M., Brambilla, M., Manenti, R., Rosini, S., Cohen, L. G., and Cotelli, M. (2014). Noninvasive stimulation of prefrontal cortex strengthens existing episodic memories and reduces forgetting in the elderly. Front. Aging Neurosci. 6:289. doi: 10.3389/fnagi.2014.00289
Sandrini, M., Cappa, S. F., Rossi, S., Rossini, P. M., and Miniussi, C. (2003). The role of prefrontal cortex in verbal episodic memory: rTMS evidence. J. Cogn. Neurosci. 15, 855–861. doi: 10.1162/089892903322370771
Sandrini, M., Censor, N., Mishoe, J., and Cohen, L. G. (2013). Causal role of prefrontal cortex in strengthening of episodic memories through reconsolidation. Curr. Biol. 23, 2181–2184. doi: 10.1016/j.cub.2013.08.045
Sandrini, M., and Cohen, L. G. (2014). “Effects of brain stimulation on declarative and procedural memories” in The stimulated brain: Cognitive enhancement using non-invasive brain stimulation. ed. R. C. Kadosh (Amsterdam: Elsevier).
Sandrini, M., Manenti, R., Brambilla, M., Cobelli, C., Cohen, L. G., and Cotelli, M. (2016). Older adults get episodic memory boosting from noninvasive stimulation of prefrontal cortex during learning. Neurobiol. Aging 39, 210–216. doi: 10.1016/j.neurobiolaging.2015.12.010
Sandrini, M., Manenti, R., Gobbi, E., Rusich, D., Bartl, G., and Cotelli, M. (2019). Transcranial direct current stimulation applied after encoding facilitates episodic memory consolidation in older adults. Neurobiol. Learn. Mem. 163:107037. doi: 10.1016/j.nlm.2019.107037
Sandrini, M., Manenti, R., Sahin, H., and Cotelli, M. (2020). Effects of transcranial electrical stimulation on episodic memory in physiological and pathological ageing. Ageing Res. Rev. 61:101065. doi: 10.1016/j.arr.2020.101065
Sarazin, M., Berr, C., De Rotrou, J., Fabrigoule, C., Pasquier, F., Legrain, S., et al. (2007). Amnestic syndrome of the medial temporal type identifies prodromal AD: a longitudinal study. Neurology 69, 1859–1867. doi: 10.1212/01.wnl.0000279336.36610.f7
Sarazin, M., Chauviré, V., Gerardin, E., Colliot, O., Kinkingnéhun, S., de Souza, L. C., et al. (2010). The amnestic syndrome of hippocampal type in Alzheimer's disease: an MRI study. J. Alzheimers Dis. 22, 285–294. doi: 10.3233/jad-2010-091150
Saxena, V., and Pal, A. (2021). Role of transcranial direct current stimulation in the Management of Alzheimer's disease: a Meta-analysis of effects, adherence and adverse effects. Clin. Psychopharmacol. Neurosci. 19, 589–599. doi: 10.9758/cpn.2021.19.4.589
Stuss, D. T., Winocur, G., and Robertson, I. H. (2008). Cognitive neurorehabilitation: Evidence and application. 2nd Edn. New York: Cambridge University Press.
Sunderland, A., Watts, K., Baddeley, A. D., and Harris, J. E. (1986). Subjective memory assessment and test performance in elderly adults. J. Gerontol. 41, 376–384. doi: 10.1093/geronj/41.3.376
Taub, E., Uswatte, G., and Elbert, T. (2002). New treatments in neurorehabilitation founded on basic research. Nat. Rev. Neurosci. 3, 228–236. doi: 10.1038/nrn754
Torpil, B., Pekçetin, E., and Pekçetin, S. (2023). The effectiveness of cognitive rehabilitation intervention with the telerehabilitation method for amnestic mild cognitive impairment: a feasibility randomized controlled trial. J. Telemed. Telecare 1:541. doi: 10.1177/1357633x231189541
Vaqué-Alcázar, L., Mulet-Pons, L., Abellaneda-Pérez, K., Solé-Padullés, C., Cabello-Toscano, M., Macià, D., et al. (2021). tDCS-induced memory reconsolidation effects and its associations with structural and functional MRI substrates in subjective cognitive decline. Front. Aging Neurosci. 13:695232. doi: 10.3389/fnagi.2021.695232
Vermeij, A., Claassen, J. A., Dautzenberg, P. L., and Kessels, R. P. (2016). Transfer and maintenance effects of online working-memory training in normal ageing and mild cognitive impairment. Neuropsychol. Rehabil. 26, 783–809. doi: 10.1080/09602011.2015.1048694
Vestergren, P., and Nilsson, L. G. (2011). Perceived causes of everyday memory problems in a population-based sample aged 39–99. Appl. Cogn. Psychol. 25, 641–646. doi: 10.1002/acp.1734
Woods, R. T., and Britton, P. G. (1977). Psychological approaches to the treatment of the elderly. Age Ageing 6, 104–112. doi: 10.1093/ageing/6.2.104
Yao, S., Liu, Y., Zheng, X., Zhang, Y., Cui, S., Tang, C., et al. (2020). Do nonpharmacological interventions prevent cognitive decline? A systematic review and meta-analysis. Transl. Psychiatry 10:19. doi: 10.1038/s41398-020-0690-4
Yesavage, J. A., Brink, T. L., Rose, T. L., Lum, O., Huang, V., Adey, M., et al. (1982). Development and validation of a geriatric depression screening scale: a preliminary report. J. Psychiatr. Res. 17, 37–49. doi: 10.1016/0022-3956(82)90033-4
Yun, K., Song, I. U., and Chung, Y. A. (2016). Changes in cerebral glucose metabolism after 3 weeks of noninvasive electrical stimulation of mild cognitive impairment patients. Alzheimers Res. Ther. 8:49. doi: 10.1186/s13195-016-0218-6
Zimerman, M., Nitsch, M., Giraux, P., Gerloff, C., Cohen, L. G., and Hummel, F. C. (2013). Neuroenhancement of the aging brain: restoring skill acquisition in old subjects. Ann. Neurol. 73, 10–15. doi: 10.1002/ana.23761
Glossary
Keywords: MCI, telerehabilitation, cognition, tDCS, transcranial direct current stimulation
Citation: Manenti R, Baglio F, Pagnoni I, Gobbi E, Campana E, Alaimo C, Rossetto F, Di Tella S, Pagliari C, Geviti A, Bonfiglio NS, Calabrò RS, Cimino V, Binetti G, Quartarone A, Bramanti P, Cappa SF, Rossini PM and Cotelli M (2024) Long-lasting improvements in episodic memory among subjects with mild cognitive impairment who received transcranial direct current stimulation combined with cognitive treatment and telerehabilitation: a multicentre, randomized, active-controlled study. Front. Aging Neurosci. 16:1414593. doi: 10.3389/fnagi.2024.1414593
Edited by:
Antonio Ivano Triggiani, National Institute of Neurological Disorders and Stroke (NIH), United StatesReviewed by:
John Dimitrios Papatriantafyllou, Third Age Day-Care Center, IASIS, GreeceMarina Bluma, Karolinska Institutet, Sweden
Copyright © 2024 Manenti, Baglio, Pagnoni, Gobbi, Campana, Alaimo, Rossetto, Di Tella, Pagliari, Geviti, Bonfiglio, Calabrò, Cimino, Binetti, Quartarone, Bramanti, Cappa, Rossini and Cotelli. This is an open-access article distributed under the terms of the Creative Commons Attribution License (CC BY). The use, distribution or reproduction in other forums is permitted, provided the original author(s) and the copyright owner(s) are credited and that the original publication in this journal is cited, in accordance with accepted academic practice. No use, distribution or reproduction is permitted which does not comply with these terms.
*Correspondence: Ilaria Pagnoni, aXBhZ25vbmlAZmF0ZWJlbmVmcmF0ZWxsaS5ldQ==