- 1Department of Neurology, Chuncheon Sacred Heart Hospital, Hallym University College of Medicine, Chuncheon-si, Gangwon-do, Republic of Korea
- 2Department of Anesthesiology and Pain Medicine, Chuncheon Sacred Heart Hospital, Hallym University College of Medicine, Chuncheon-si, Gangwon-do, Republic of Korea
- 3Institute of New Frontier Research Team, Hallym University, Chuncheon-si, Gangwon-do, Republic of Korea
- 4Department of Neurology, Seoul National University College of Medicine and Seoul National University Bundang Hospital, Seongnam-si, Gyeonggi-do, Republic of Korea
- 5Department of Radiology and Imaging Sciences, Center for Computational Biology and Bioinformatics, Indiana Alzheimer’s Disease Research Center, Indiana University School of Medicine, Indianapolis, IN, United States
Background: Alzheimer’s disease (AD) is a complex neurodegenerative disorder influenced by various factors, including liver function, which may impact the clearance of amyloid-β (Aβ) in the brain. This study aimed to explore how the apolipoprotein E (APOE) ε4 allele affects the relationship of liver function markers with AD pathology and cognition.
Methods: We analyzed data from two independent cohorts, including 732 participants from the Hallym University Medical Center and 483 from the Alzheimer’s Disease Neuroimaging Initiative, each group consisting of individuals with and without the APOE ε4 allele. Cross-sectional analyses evaluated the associations of liver enzymes (aspartate aminotransferase [AST], alanine aminotransferase [ALT], alkaline phosphatase, total bilirubin, and albumin) with AD diagnosis, amyloid positron emission tomography (PET) burden, and cerebrospinal fluid biomarkers for AD (Aβ42, total tau, and phosphorylated tau181) at baseline. Longitudinally, we investigated the associations between these liver enzymes and changes in cognitive performance over the course of a year. Logistic and linear regression models were used to analyze these associations and mediation analyses were conducted to assess whether age and amyloid PET burden mediated these associations.
Results: Only in the APOE ε4 carrier group, a high AST to ALT ratio and low ALT levels were significantly associated with AD diagnosis, increased amyloid PET burden, and faster longitudinal decline in cognitive function in both cohorts. In particular, the AST to ALT ratio was associated with cerebrospinal fluid Aβ42 levels exclusively in the APOE ε4 carrier group in the Alzheimer’s Disease Neuroimaging Initiative cohort but not with phosphorylated tau181 or total tau levels. Moreover, mediation analyses from both cohorts revealed that in the APOE ε4 carriers group, age did not mediate the associations between liver enzymes and AD diagnosis or amyloid PET burden. However, amyloid PET burden partially mediated the association between liver enzymes and AD diagnosis exclusively in the APOE ε4 carriers group.
Conclusion: This study provides valuable insights into the significant association of the APOE ε4 allele with liver enzymes and their potential role in Aβ-related pathogenesis and cognition in AD. Further research is required to elucidate the underlying mechanisms and potential therapeutic implications of these findings.
1 Introduction
Alzheimer’s Disease (AD) is the most common form of dementia, characterized by progressive neurodegeneration leading to cognitive decline (Long and Holtzman, 2019). AD is characterized by neuropathological markers, including accumulation of extracellular amyloid-β (Aβ) in the form of neuritic plaques and intracellular deposition of hyperphosphorylated tau in neurofibrillary tangles (Long and Holtzman, 2019). AD is a complex neurodegenerative disorder due to its multifactorial etiology, involving a combination of genetic susceptibilities and environmental factors (Hampel et al., 2010).
Despite advances in understanding the molecular pathogenesis of AD, current diagnostic approaches and therapeutic interventions remain inadequate. Recent investigations have focused on the potential interactions between the brain and peripheral organs, particularly the liver, in relation to Aβ clearance (Estrada et al., 2019; Bassendine et al., 2020; Cheng et al., 2020; Huang et al., 2022). This attention to peripheral organ involvement unveils novel avenues for understanding pathophysiology of AD and identifying accessible biomarkers. In particular, liver function enzymes, such as aspartate aminotransferase (AST), alanine aminotransferase (ALT), and alkaline phosphatase (ALP), have been explored for their association with AD, cognitive function, and Aβ accumulation (Kellett et al., 2011; Nho et al., 2019; Lu et al., 2021; Ferri et al., 2022; Han et al., 2022; Li et al., 2022). However, conflicting findings from other studies have challenged this association (Kamada et al., 2016; Vasantharekha et al., 2017). These inconsistencies could be attributed to differences in the study designs, sample sizes, population demographics, methodologies, the specific liver function markers assessed, and the statistical approaches employed. Importantly, previous studies did not account for genetic factors, such as the apolipoprotein E (APOE) genotype, which significantly influences AD pathogenesis. These findings suggest a complex relationship between liver function and AD pathogenesis, prompting the need for more exploration.
Among the genetic factors implicated in AD, the APOE ε4 allele has been identified as the most significant genetic risk factor, increasing disease risk in a gene dose-dependent manner (Farrer et al., 1997). The APOE gene encodes APOE, a glycoprotein with crucial roles in lipid transport and metabolism, both in the brain and periphery (Liu et al., 2013; Chernick et al., 2019; Muñoz et al., 2019). Notably, APOE4 has been shown to inhibit peripheral Aβ clearance and increase Aβ accumulation in the brain, a hallmark of AD pathology (Sharman et al., 2010; Liu et al., 2022). However, the interplay between APOE ε4 status, liver enzyme levels, and AD, particularly in the context of Aβ accumulation and cognitive functions, remains poorly understood. Furthermore, the potential mediation effects of age and Aβ burden on these associations, contingent upon APOE ε4 carrier status, have not been adequately addressed. These considerations are crucial, given the liver function in systemic metabolism and its potential influence on AD pathogenesis.
Our study aimed to address this knowledge gap by investigating the association of APOE ε4 carrier status and blood liver enzymes, including AST, ALT, the AST to ALT ratio, ALP, total bilirubin, and albumin, with AD diagnosis, AD biomarkers, and cognitive performance, in two independent cohorts. Additionally, if such associations were observed, we investigated whether these relationships were independent or mediated by age or Aβ burden.
2 Methods
2.1 Study participants
Two independent cohorts were used in this study: the Hallym University Medical Centers (HUMC) cohort and the Alzheimer’s Disease Neuroimaging Initiative (ADNI) cohort. In the HUMC cohort, we conducted a retrospective analysis of clinical data using the Smart Clinical Data Warehouse, a comprehensive big-data analytical solution specifically designed for clinical applications. This system collects data from four medical university-affiliated hospitals located in various provinces of the Republic of Korea (Hallym Sacred Heart Hospital, Dongtan Sacred Heart Hospital, Kangnam Sacred Heart Hospital, and Chuncheon Sacred Heart Hospital). The Smart Clinical Data Warehouse, underpinned by the QlikView Elite Solution (Qlik, King of Prussia, PA, USA), facilitates in-depth analysis of electronic medical record text data and integrated analysis of static data. The selection of participants for the HUMC cohort was based on clinical visits for symptoms of cognitive decline at one of four associated hospitals under HUMC during the period from November 2015 to June 2023. All participants underwent comprehensive assessments, including physical and neurological examinations, laboratory tests, APOE genotyping, neuropsychological assessments, brain magnetic resonance imaging, and amyloid positron emission tomography (PET) imaging to provide a robust dataset for analysis. We excluded individuals who had not undergone liver function tests within 1 year of their amyloid PET imaging date as well as those with concomitant medical conditions, such as hepatocellular carcinoma and liver cirrhosis (which could significantly affect liver function) or other neurodegenerative disorders, including frontotemporal dementia (Gorno-Tempini et al., 2011; Rascovsky et al., 2011), corticobasal syndrome (Armstrong et al., 2013), Parkinson’s disease dementia (Dubois et al., 2007), and progressive nuclear palsy (Litvan et al., 1996). Additionally, we excluded participants who had outlier values in liver function parameters, including AST, ALT, ALP, total bilirubin, and albumin which exceeded the interquartile range by four times, due to the potential for significant liver function impairment. Following these exclusions, the AST to ALT ratios were calculated from the refined dataset. The study protocol was approved by the Clinical Research Ethics Committee of Chuncheon Sacred Heart Hospital and Hallym University and conformed to the principles outlined in the Declaration of Helsinki.
In the ADNI cohort, the initial phase (ADNI-1) (Petersen et al., 2010) was launched in 2003 by the National Institute on Aging, National Institute of Biomedical Imaging and Bioengineering, Food and Drug Administration, private pharmaceutical companies, and nonprofit organizations. The primary objective was to investigate the feasibility of using serial magnetic resonance imaging, PET, other biological markers, and clinical and neuropsychological assessments as dependable in vivo indicators of AD pathogenesis. Subsequent phases, namely ADNI-GO (ADNIGO, 2009; Aisen et al., 2010), ADNI-2 (Aisen et al., 2015), and ADNI-3 (Weiner et al., 2017), extended the initial phase, allowing for the ongoing follow-up of existing participants and inclusion of new enrollments. A comprehensive description of ADNI, up-to-date information, inclusion and exclusion criteria, clinical and neuroimaging protocols, and a summary of the diagnostic criteria are available at https://www.adni-info.org. Demographic and clinical information, raw neuroimaging data, cerebrospinal fluid (CSF) biomarker data, information on APOE ε4 carrier status, and cognitive scores were obtained from the ADNI Laboratory of Neuro Imaging (LONI) website1 (Saykin et al., 2015). Written informed consent was obtained from all participants at enrollment, including consent for data analysis and sharing. This study was approved by the Institutional Review Board of each participating site. We specifically selected participants underwent both serum liver function tests and APOE genotyping. Furthermore, we excluded participants with outlier values in liver function parameters, including AST, ALT, ALP, total bilirubin, and albumin which exceeded the interquartile range by four times. Subsequent to these exclusions, we calculated the AST to ALT ratios from the refined dataset.
In both the HUMC and ADNI cohorts, all participants underwent neuropsychological assessments annually to provide a longitudinal perspective on changes in cognitive function over time. In contrast, measurements of liver enzymes, CSF biomarkers for AD, and amyloid PET imaging were performed only at baseline.
2.2 Diagnostic status
In the HUMC cohort, participants were categorized as having probable AD based on the National Institute on Aging–Alzheimer’s Association criteria (McKhann et al., 2011), mild cognitive impairment (MCI) according to the National Institute on Aging–Alzheimer’s Association criteria (Albert et al., 2011), or subjective cognitive decline (SCD) in accordance with the guidelines of Jessen et al. (2014).
Participants in the ADNI cohort who met the criteria for a clinical diagnosis of probable AD, MCI, and cognitively normal (CN) older individuals were prospectively followed up with clinical data, neuroimaging studies, and biological samples gathered for molecular biomarker measurements, as previously described (ADNIGO, 2009; ADNI1, 2010; Weiner et al., 2017; ADNI2, 2020). Briefly, the Logical Memory from the Wechsler Memory Scale—Revised (Wechsler, 1987), Mini-Mental State Examination (MMSE) (Folstein et al., 1975), and Clinical Dementia Rating scale (Morris, 1993) were used to determine the diagnostic classifications.
2.3 Amyloid PET imaging
In the HUMC cohort, the amyloid status was determined using amyloid PET imaging with [18F] florbetaben (n = 561) and [18F] flutemetamol (n = 171) tracers. The amyloid PET status was categorized as positive (abnormal) or negative (normal) based on visual ratings by one nuclear medicine physician and one neurologist, both experienced and trained in the field. [18F] florbetaben PET images were classified as positive based on a visual assessment scoring of 2 or 3 according to the brain Aβ plaque load (BAPL) scoring system (Barthel et al., 2011). For [18F] flutemetamol PET images, visual interpretation involved a systematic review across five brain regions: frontal, parietal, posterior cingulate and precuneus, striatum, and lateral temporal areas. A scan was considered positive if any of these regions showed Aβ deposition in either hemisphere (Farrar et al., 2019). The concordance rate between CSF Aβ42 and BAPL scores was 77.4%, using an Aβ42 cutoff of less than 600 pg/mL (Spallazzi et al., 2019). The raters were unaware of the clinical details of the participants but had knowledge of the specific PET tracer used for each image. In case of discordance, the raters held discussions to reach a consensus.
In the ADNI cohort, preprocessed [18F] florbetapir PET scans were obtained from the ADNI LONI site (see text footnote 1), following previously reported methods of PET scan acquisition and processing (Jagust et al., 2010, 2015). [18F] Florbetapir PET standard uptake value ratio (SUVR) values were used to evaluate amyloid deposition by normalizing the intensity using the whole cerebellar reference region.
2.4 Liver function enzymes
In the HUMC cohort, all participants underwent venous blood sample collection following a 12-h overnight fast. These samples were obtained to assess serum levels of liver function enzymes, including AST [normal range, <40 U/L (Neuschwander-Tetri et al., 2008)], ALT [normal range, <40 U/L (Neuschwander-Tetri et al., 2008)], total bilirubin [normal range, 0.2–1.0 mg/dL (Franchini et al., 2010)], ALP [normal range, 30–115 U/L (Lum, 1995)], and albumin [normal range, 3.5–5.0 g/dL (Doweiko and Nompleggi, 1991)]. An AST/ALT ratio of greater than two suggests cirrhosis in various liver diseases (Nyblom et al., 2006). The samples were analyzed using an automated blood analyzer (Beckman Coulter AU5800, Beckman Coulter Inc., Brea, CA, USA), which was operated within the Department of Laboratory Medicine at each of the participating hospitals (Hallym Sacred Heart Hospital, Dongtan Sacred Heart Hospital, Kangnam Sacred Heart Hospital, and Chuncheon Sacred Heart Hospital).
In the ADNI cohort, blood samples were obtained from the participants during fasting and handled according to the established laboratory standard operating procedures of the ADNI (Kang et al., 2015). Results of liver enzymes, including AST, ALT, ALP, total bilirubin, and albumin, were acquired from the ADNI data repository and subsequently incorporated into this study.
2.5 CSF biomarkers
In the ADNI cohort, CSF samples were obtained through lumbar puncture conducted in the morning following an overnight fast. The collected samples were frozen within 1 h of collection and transported on dry ice to the ADNI Biomarker Core Laboratory at the University of Pennsylvania Medical Center. In the laboratory, CSF samples were measured in pristine aliquots using the multiplex xMAP Luminex platform (Luminex Corp, Austin, TX) (Olsson et al., 2005) with immunoassay kit–based reagents containing monoclonal antibodies targeting Aβ42, total tau (t-tau), and phosphorylated tau181 (p-tau181), as previously described (Shaw et al., 2009; Hansson et al., 2018). CSF biomarker data were downloaded from the ADNI LONI website, which is accessible at http://adni.loni.usc.edu. CSF biomarker data were log-transformed to reduce skewness.
2.6 Comprehensive neuropsychological assessment
In the HUMC cohort, a comprehensive series of neuropsychological assessments were performed longitudinally to evaluate individuals across two distinct cognitive domains: language and memory functions. The raw scores for each participant based on their performance on individual cognitive tests were converted into standardized z-scores, which were adjusted for age, sex, and education norms (Kang et al., 2003). For each participant, based on their performance on individual cognitive tests, the z-scores for language and memory functions were calculated. Specifically, language function was assessed using the Korean Boston Naming Test (Kim and Na, 1999) and memory function was evaluated using the Seoul Verbal Learning Test 20-min delayed recall test.
In the ADNI cohort, composite scores were used to assess language and memory functions. For the language assessment, a composite score was calculated using the Boston Naming Test (Kaplan et al., 2001), animal and vegetable fluency, language components of the MMSE (Folstein et al., 1975), memory tasks of the Alzheimer’s Disease Assessment Schedule–Cognition (ADAS-Cog) (Mohs et al., 1997), and phonemic fluency and sentence repetition of the Montreal Cognitive Assessment (Nasreddine et al., 2005). The memory function was assessed using a composite score that incorporated memory-related tasks from the ADAS-Cog (Mohs et al., 1997), the Rey Auditory Verbal Learning Test (Rey, 1958), memory components of the MMSE (Folstein et al., 1975), and the Logical Memory task (Wechsler, 1987). These composite scores were standardized to have a mean value of 0 and a standard deviation of 1.20, allowing for a consistent and comparative assessment of cognitive function across different individuals in the ADNI cohorts.
2.7 Statistical analysis
We performed a comprehensive analysis to compare the baseline characteristics of individuals in both the amyloid-negative and amyloid-positive groups as well as in the APOE ε4 carrier and APOE ε4 non-carrier groups. We used chi-squared tests to examine categorical variables and Mann–Whitney U tests or t-test to assess continuous variables, as appropriate.
We conducted a logistic regression analysis to investigate the association of each liver enzyme with AD diagnosis and amyloid PET status. To analyze the association between liver enzymes and longitudinal changes in cognitive performance, we used linear mixed-effects models (Pinheiro and Bates, 2006). We conducted linear regression analyses to investigate the association of liver enzymes with amyloid PET global SUVR and CSF biomarkers for AD, including Aβ42, p-tau181, and t-tau.
In accordance with established methodologies (Hayes, 2009), we performed mediation analyses of liver function markers demonstrating a significant correlation with AD diagnosis and amyloid PET burden to investigate whether the identified association was potentially mediated by age. Additionally, we conducted mediation analyses focusing on liver function markers revealing a significant correlation with AD diagnosis to investigate whether the amyloid PET burden potentially mediated this association. To conduct these analyses, we used the Mediation R package, which allowed us to evaluate indirect effects through a bootstrapping approach involving the generation of 10,000 non-parametric simulations (Tingley et al., 2014).
In the HUMC cohort, covariates for association analysis with AD diagnosis, amyloid PET status, and CSF biomarkers for AD as well as for mediation analysis included age, sex, hypertension, diabetes mellitus, dyslipidemia, and statin use for both APOE ε4 carrier and APOE ε4 non-carrier groups. Similarly, in the ADNI cohort, we included covariates such as age, sex, and body mass index (BMI) for both APOE ε4 carrier and APOE ε4 non-carrier groups. For the association analysis with cognitive performance, education was included along with the previously mentioned covariates.
All statistical analyses were performed using R version 4.2.0 (The R Foundation for Statistical Computing, Vienna, Austria). Statistical significance was defined as p < 0.05, with adjustments for multiple comparisons. For multiple testing adjustments, we used the Benjamini–Hochberg procedure for false discovery rate correction (Benjamini and Hochberg, 1995).
3 Results
3.1 Demographics and clinical characteristics
In the HUMC cohort, a total of 770 participants were initially included. Exclusions were made for 28 participants with outlier values in liver function parameters (AST above 63, ALT above 62, ALP above 189, total bilirubin above 1.7, and albumin below 2.8), four with hepatocellular carcinoma, and six with liver cirrhosis. Subsequently, the remaining participants were categorized into the APOE ε4 carrier (N = 218 [29.8%]) and APOE ε4 non-carrier (N = 514) groups. The APOE ε4 carrier group comprised 26 patients with SCD, 87 with MCI, and 105 with probable AD. The median age of the participants in this group was 74 years, and 70.6% were females. The APOE ε4 non-carrier group comprised 45 patients with SCD, 282 with MCI, and 187 with probable AD. The median age of the participants in this group was 74 years, and 66.1% were females. There were no significant differences in age, sex, and frequency of hypertension, diabetes mellitus, dyslipidemia, or statin use between the two groups.
In the ADNI cohort, a total of 498 participants were initially included. After exclusion of 15 participants with outlier values in liver function parameters (AST above 52, ALT above 63, ALP above 145, total bilirubin above 1.5, and albumin below 3.8), the cohort was divided into the APOE ε4 carrier (N = 200 [41.4%]) and APOE ε4 non-carrier (N = 283) groups. The APOE ε4 carrier group included 95 individuals with CN, 66 with MCI, and 39 with probable AD. The median age of the participants in this group was 70 years, and 55.0% were females. The APOE ε4 non-carrier group included 183 individuals with CN, 82 with MCI, and 18 with probable AD. The median age of the participants in this group was 70 years, and 54.4% were females. There were no significant differences in age, sex, or education level between the two groups. However, the APOE ε4 carrier group showed poorer performance in memory and language functions compared to the APOE ε4 non-carrier group in the HUMC cohort. Similarly, the APOE ε4 carrier group in the ADNI cohort exhibited lower performance in memory function than the APOE ε4 non-carrier group. Regarding detailed baseline characteristics, Tables 1, 2 present a comparison of participants in the HUMC and ADNI cohorts, respectively.
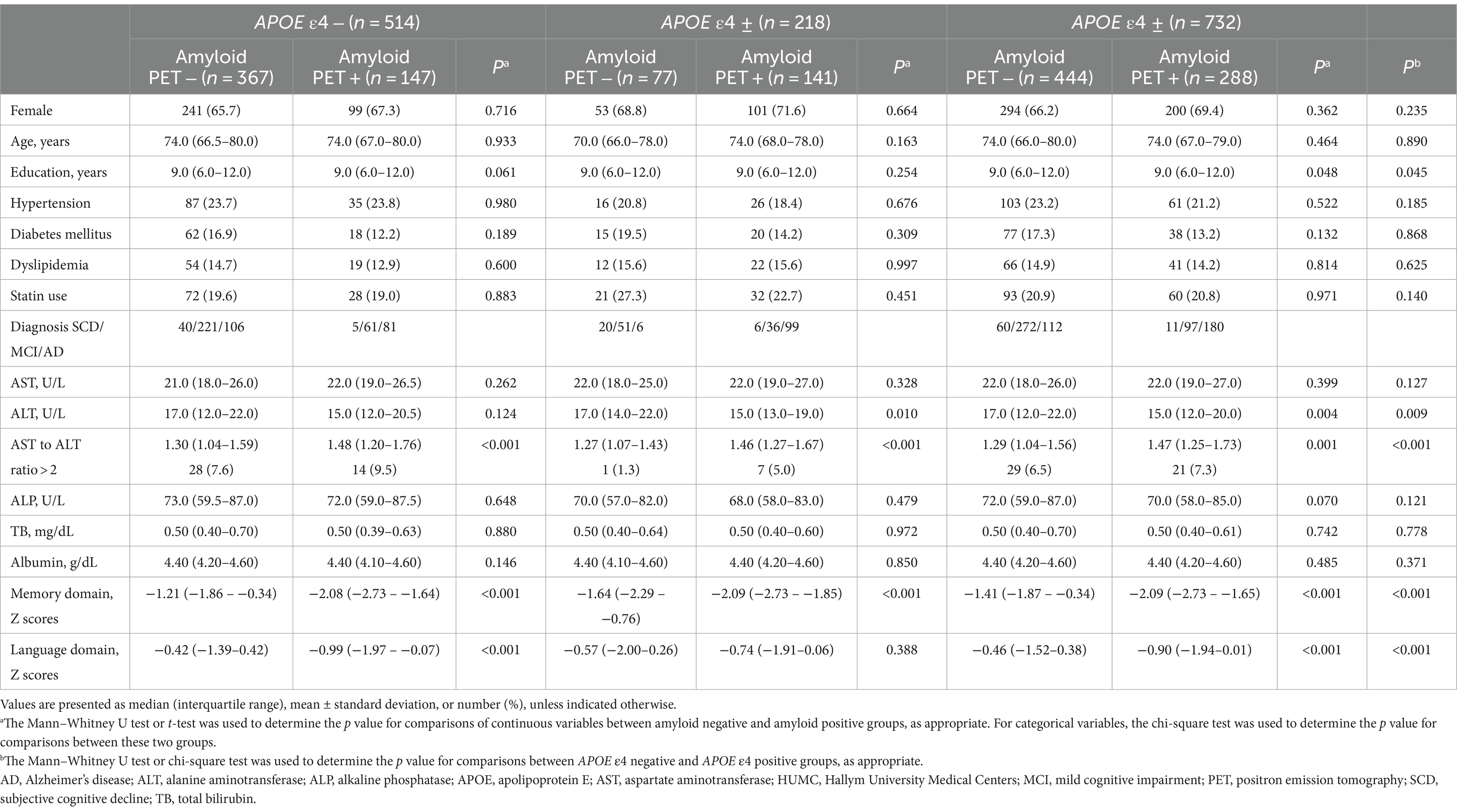
Table 1. Demographic and clinical characteristics, and laboratory results of participants in the HUMC cohort.
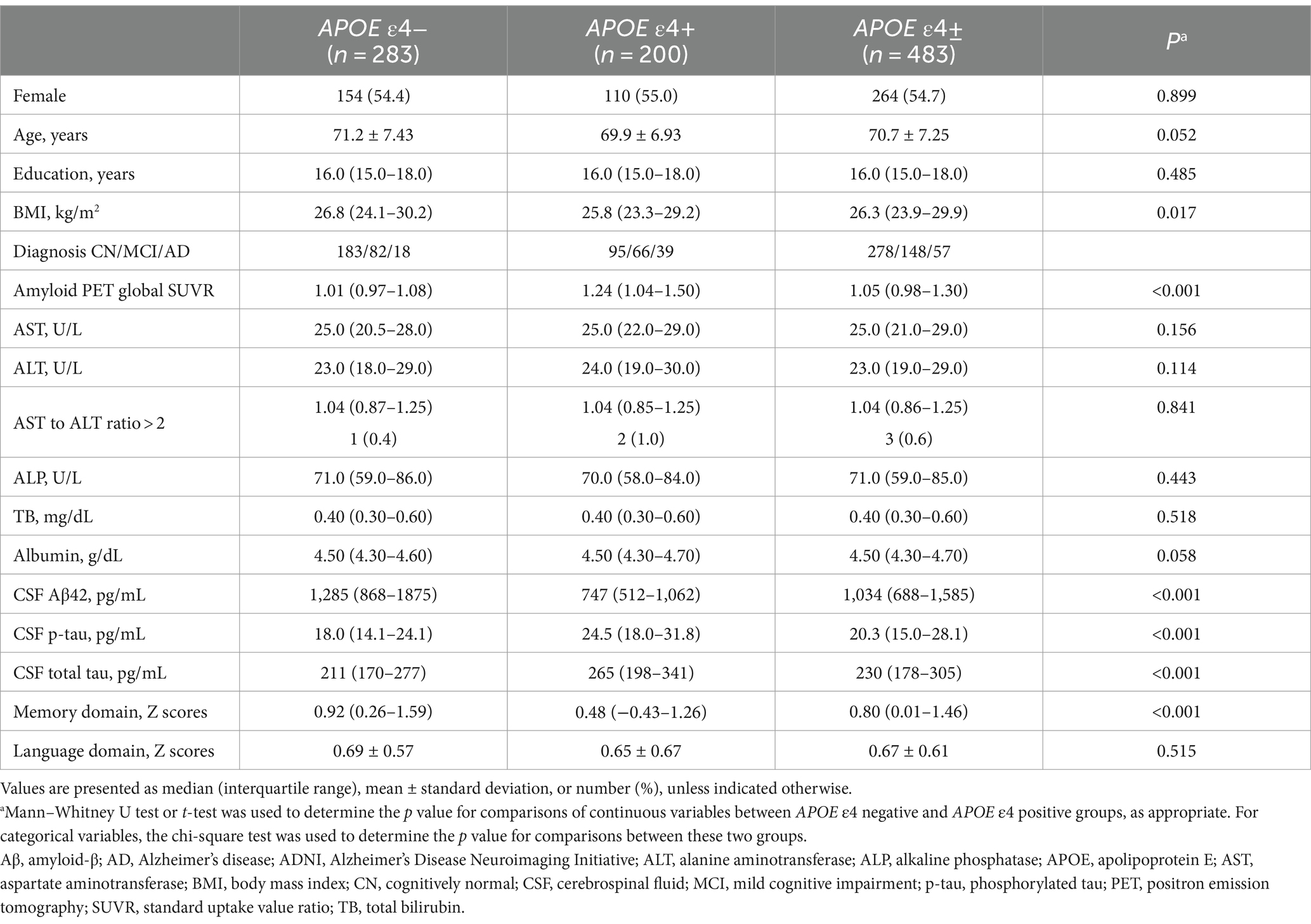
Table 2. Demographic and clinical characteristics, and laboratory results of participants in the ADNI cohort.
3.2 Association analysis of liver enzymes with amyloid PET burden and diagnostic status
In the HUMC cohort, ALT levels (estimate for logistic regression coefficients = −0.076, standard error (SE) = 0.027, p = 0.01) were significantly lower in the amyloid-positive group (median value: ALT, 15 U/L; interquartile range (IQR): ALT, 13–19 U/L) compared to the amyloid-negative group (median value: ALT, 17 U/L; IQR: ALT, 14–22 U/L) in the APOE ε4 carrier group after adjustment for multiple comparisons (Table 3). In addition, the AST to ALT ratio (estimate = 2.412, SE = 0.574, p < 0.001) was significantly higher in the amyloid-positive group (median value: AST to ALT ratio, 1.46; IQR: AST to ALT ratio, 1.27–1.67) compared to the amyloid-negative group (median value: AST to ALT ratio, 1.27; IQR: AST to ALT ratio, 1.07–1.43) within the APOE ε4 carrier group. Regarding diagnostic status, ALT levels (estimate = −0.195, SE = 0.048, p < 0.001) were significantly decreased, while the AST to ALT ratio (estimate = 5.506, SE = 1.236, p < 0.001) was significantly increased in AD compared with SCD in APOE ε4 carrier group (Table 4). However, the APOE ε4 non-carrier group did not demonstrate significant associations between any of the six liver function markers and the diagnostic status or amyloid PET positivity.
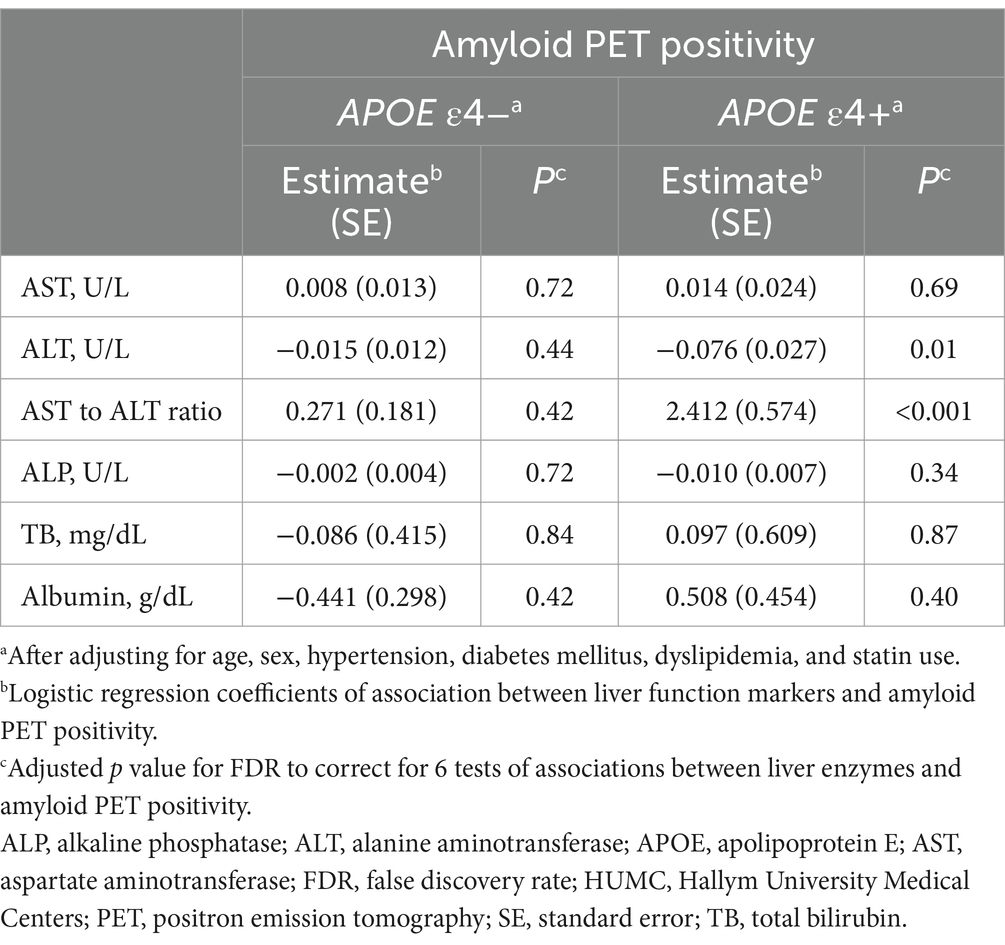
Table 3. Results of the association analysis of liver function markers with amyloid PET positivity in the HUMC cohort.
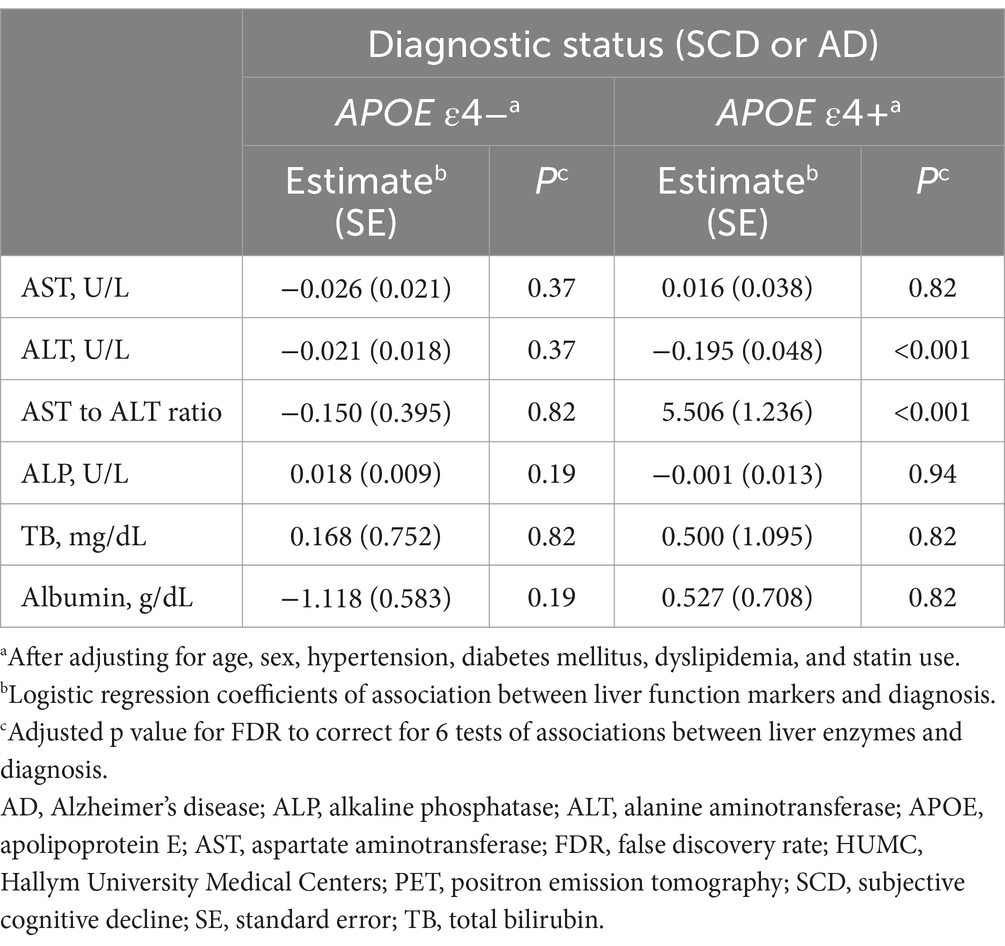
Table 4. Results of the association analysis of liver function markers with AD diagnosis in the HUMC cohort.
In the ADNI cohort, lower ALT levels (β for linear regression coefficients [SE] = −0.006 [0.002], p = 0.02) and higher ALP levels (β [SE] = 0.002 [0.001], p = 0.046) and an AST to ALT ratio (β [SE] = 0.166 [0.061], p = 0.02) were significantly associated with higher values of amyloid PET global SUVR in the APOE ε4 carrier group after adjustment for multiple comparisons (median value: ALT, 23 U/L; ALP, 71 U/L; AST to ALT ratio, 1.04; IQR: ALT, 19–29 U/L; ALP, 59–85 U/L; AST to ALT ratio, 0.86–1.25) (Table 5 and Figure 1). Regarding diagnostic status, ALT levels were significantly decreased (estimate = −0.071, SE = 0.029, p = 0.03), while the AST to ALT ratio and ALP levels were significantly increased in AD compared with CN (AST to ALT ratio: estimate = 1.583, SE = 0.630, p = 0.03; ALP: estimate = 0.036, SE = 0.012, p = 0.02) in the APOE ε4 carrier group (Table 6). However, there were no significant associations between any of the six liver function markers and the diagnostic status or amyloid PET global SUVR in the APOE ε4 non-carrier group.
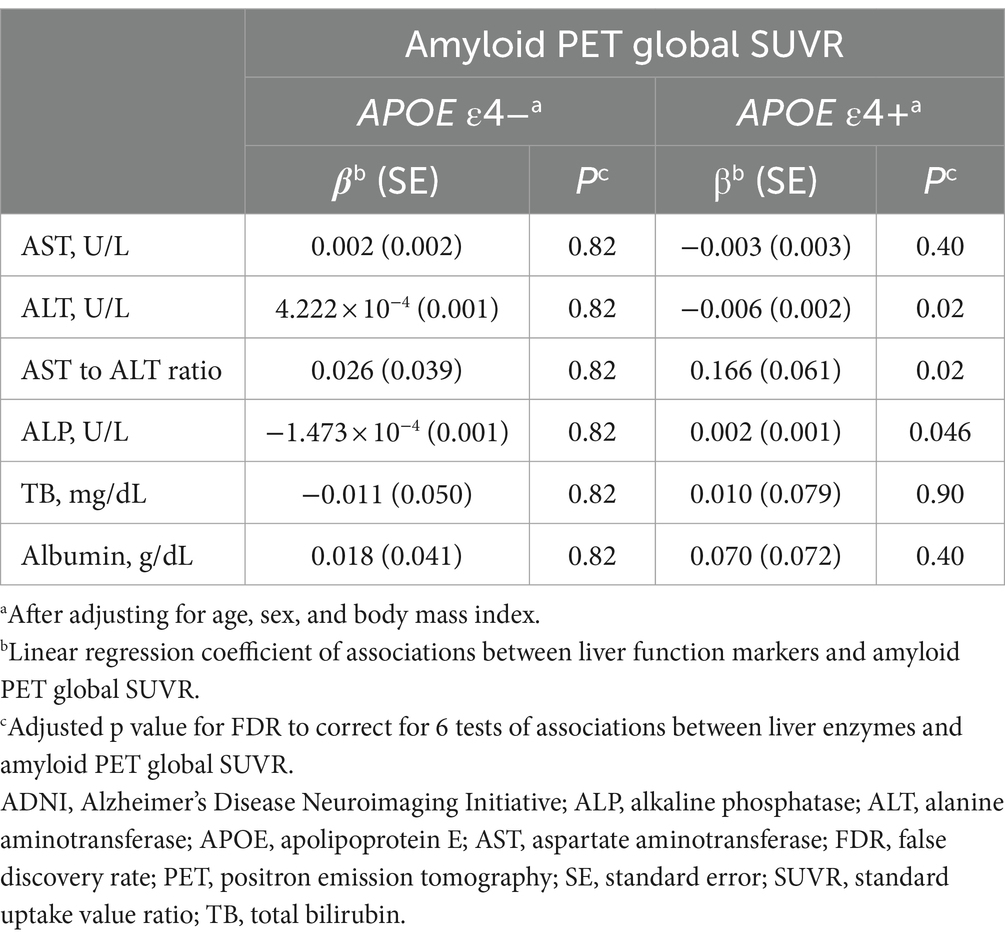
Table 5. Results of the association analysis of liver function markers with amyloid PET global SUVR in the ADNI cohort.
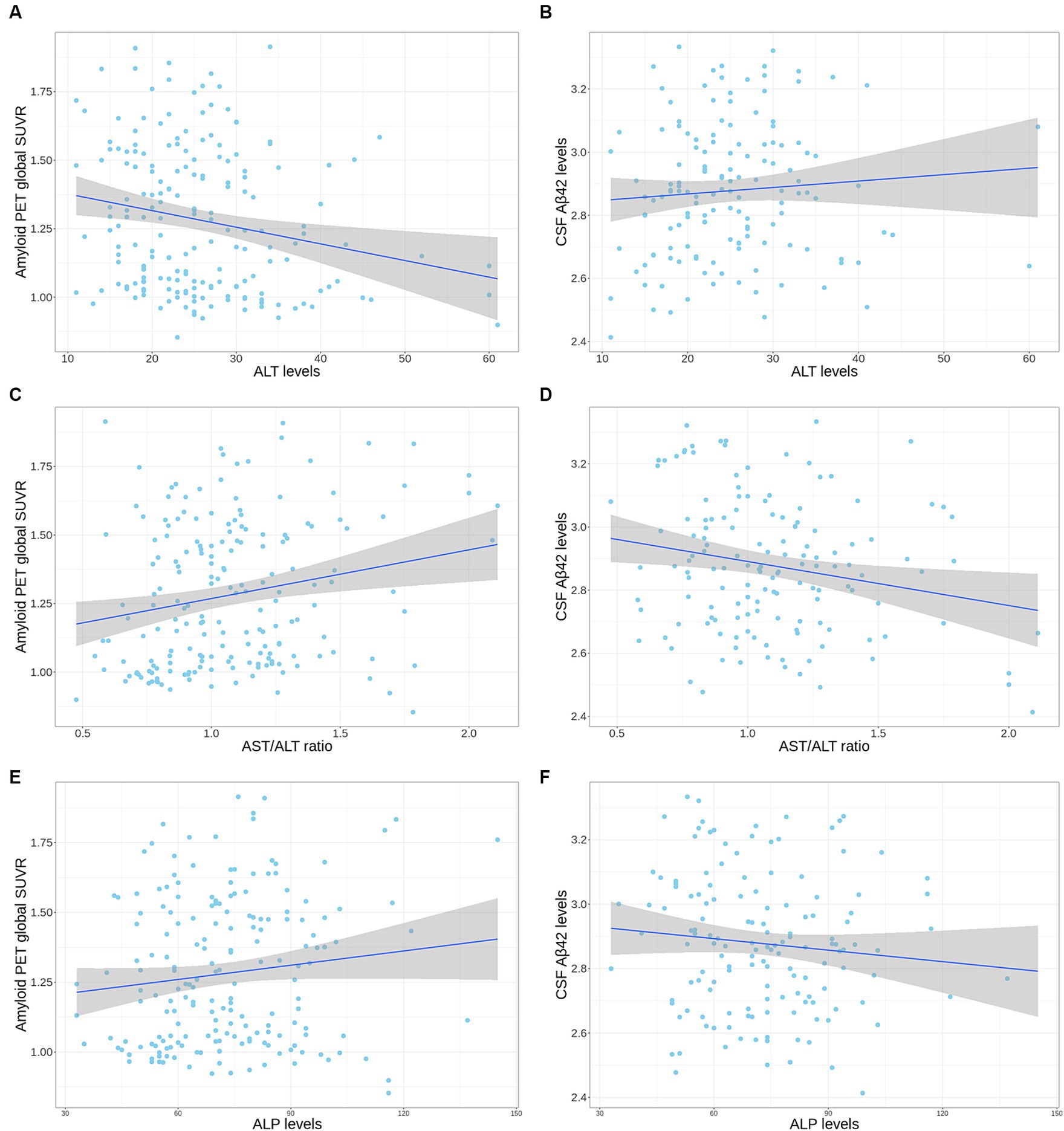
Figure 1. Correlation plot of liver enzymes levels with amyloid PET global SUVR and CSF Aβ42 levels in the APOE ε4 carrier group from the ADNI cohort. (A,B) The scatter plot represents a correlation of ALT levels with amyloid PET global SUVR (A) and CSF Aβ42 levels (B). (C,D) The scatter plot represents a correlation of AST to ALT ratio with amyloid PET global SUVR (C) and CSF Aβ42 levels (D). (E,F) The scatter plot represents a correlation of ALP levels with amyloid PET global SUVR (E) and CSF Aβ42 levels (F). The gray zone around the linear regression line represents the 95% confidence interval. Aβ, amyloid-β; ADNI, Alzheimer’s Disease Neuroimaging Initiative; ALT, alanine aminotransferase; ALP, alkaline phosphatase; APOE, apolipoprotein E; CSF, cerebrospinal fluid; PET, positron emission tomography; SUVR, standard uptake value ratio.
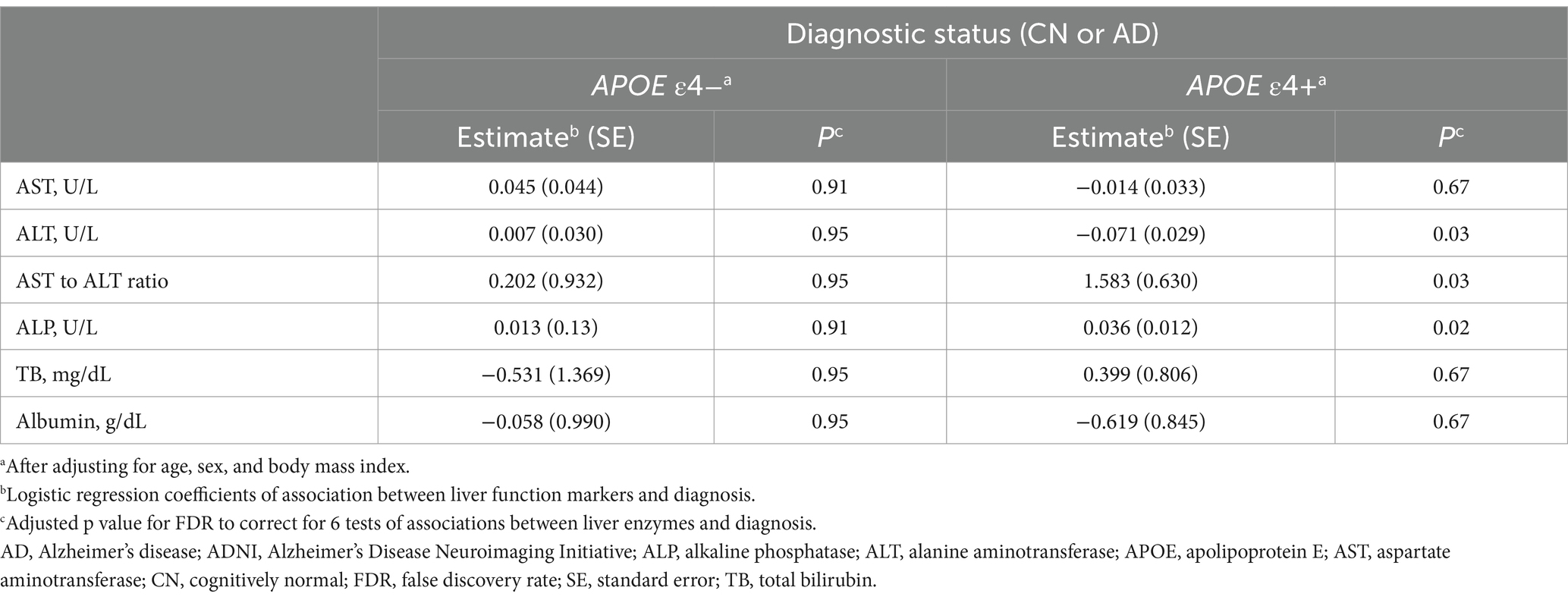
Table 6. Results of the association analysis of liver function markers with AD diagnosis in the ADNI cohort.
3.3 Association analysis of liver enzymes with CSF biomarkers for AD
In the ADNI cohort, a high AST to ALT ratio was significantly associated with low CSF Aβ42 levels (β [SE] = −0.157 [0.055], p = 0.03) after adjustment for multiple comparisons (Table 7 and Figure 1). These associations were not observed with CSF p-tau181 and t-tau levels. Additionally, none of the six liver function markers showed significant associations with any CSF biomarkers for AD in the APOE ε4 non-carrier group.
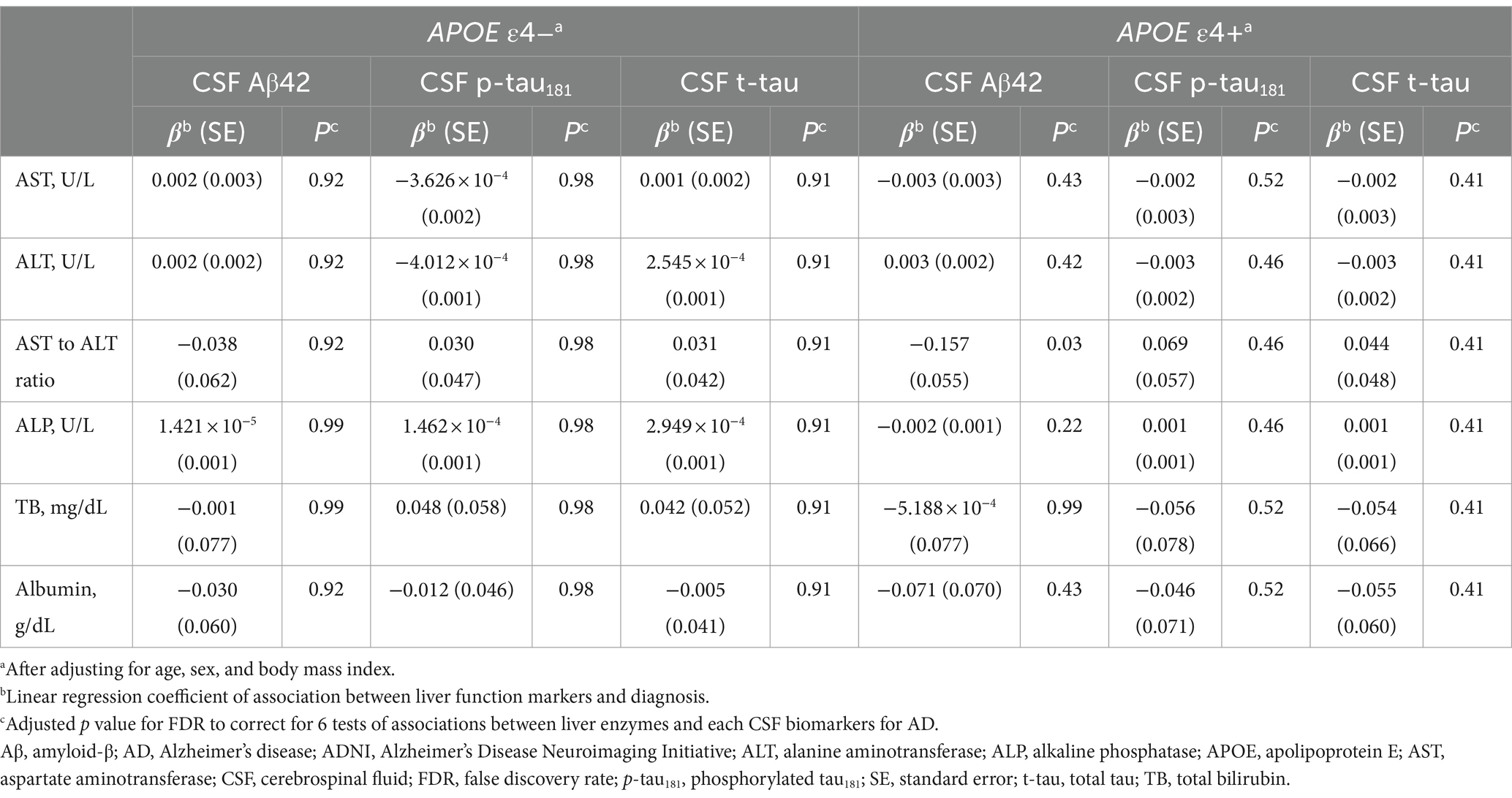
Table 7. Results of the association analysis between liver function markers and CSF biomarkers for AD in the ADNI cohort.
3.4 Association analysis of liver enzymes with cognition
In the HUMC cohort, across all participants including those with SCD, MCI, and AD, low ALT levels were significantly associated with faster longitudinal decline in memory function (β [SE] = 0.035 [0.012], p = 0.029) in the APOE ε4 carrier group after adjustment for multiple comparisons (Table 8). Additionally, a high AST to ALT ratio was significantly associated with faster longitudinal decline in memory function (β [SE] = −1.012 [0.212], p < 0.001) in the APOE ε4 carrier group. There were no significant associations between cognitive performance and any of the six liver function markers in the APOE ε4 non-carrier group.
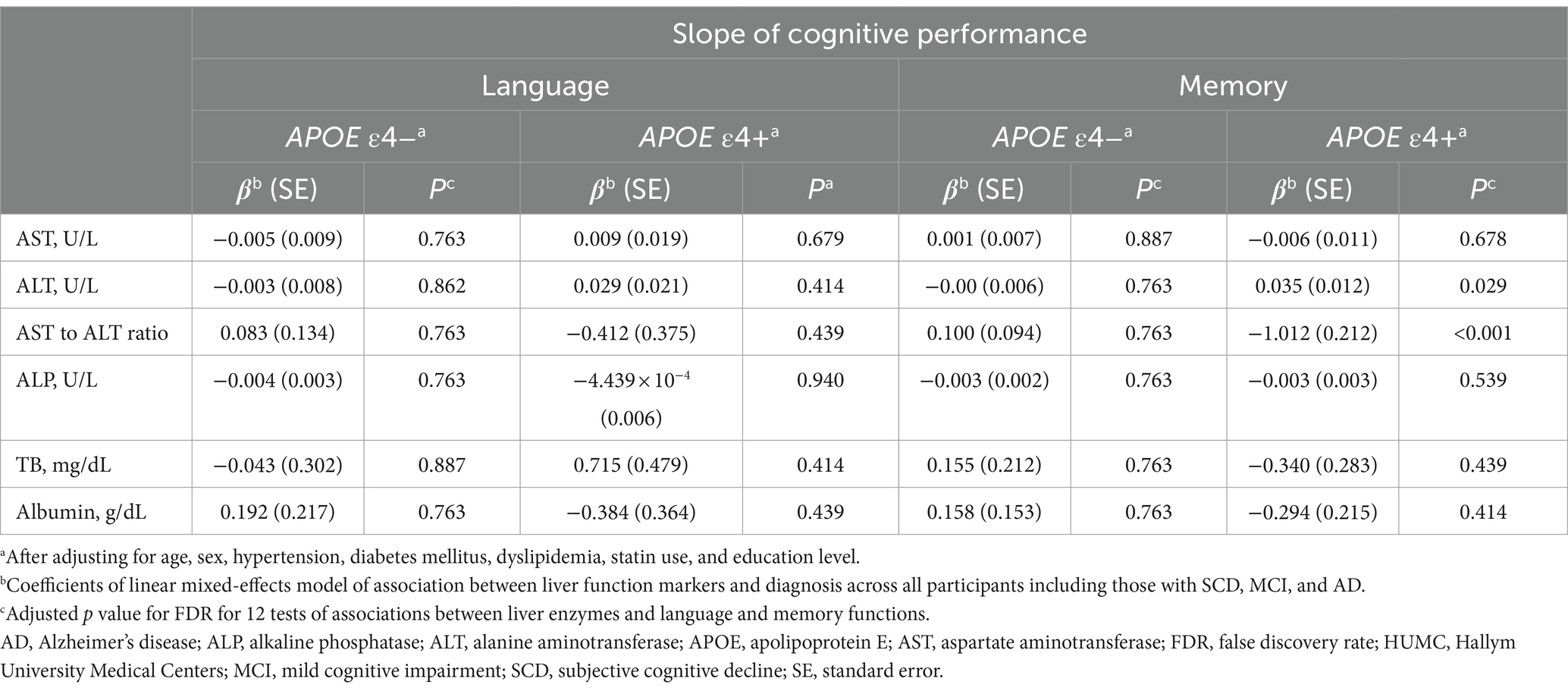
Table 8. Results of the association analysis of liver function markers with cognition in the HUMC cohort.
In the ADNI cohort, among all participants, including those with CN, MCI, and AD, low ALT levels were significantly associated with faster longitudinal decline in memory function (β [SE] = 0.002 [0.001], p = 0.023) in the APOE ε4 carrier group after adjustment for multiple comparisons (Table 9). Moreover, a high AST to ALT ratio and ALP levels showed significant associations with faster longitudinal decline in language (AST to ALT ratio: β [SE] = −0.596 [0.236], p = 0.029; ALP: β [SE] = −0.011 [0.004], p = 0.023) and memory (AST to ALT ratio: β [SE] = −0.653 [0.224], p = 0.023; ALP: β [SE] = −0.001 [0.003], p = 0.023) functions in the APOE ε4 carrier group. However, there were no significant associations between cognitive performance and any of the six liver function markers in the APOE ε4 non-carrier group.
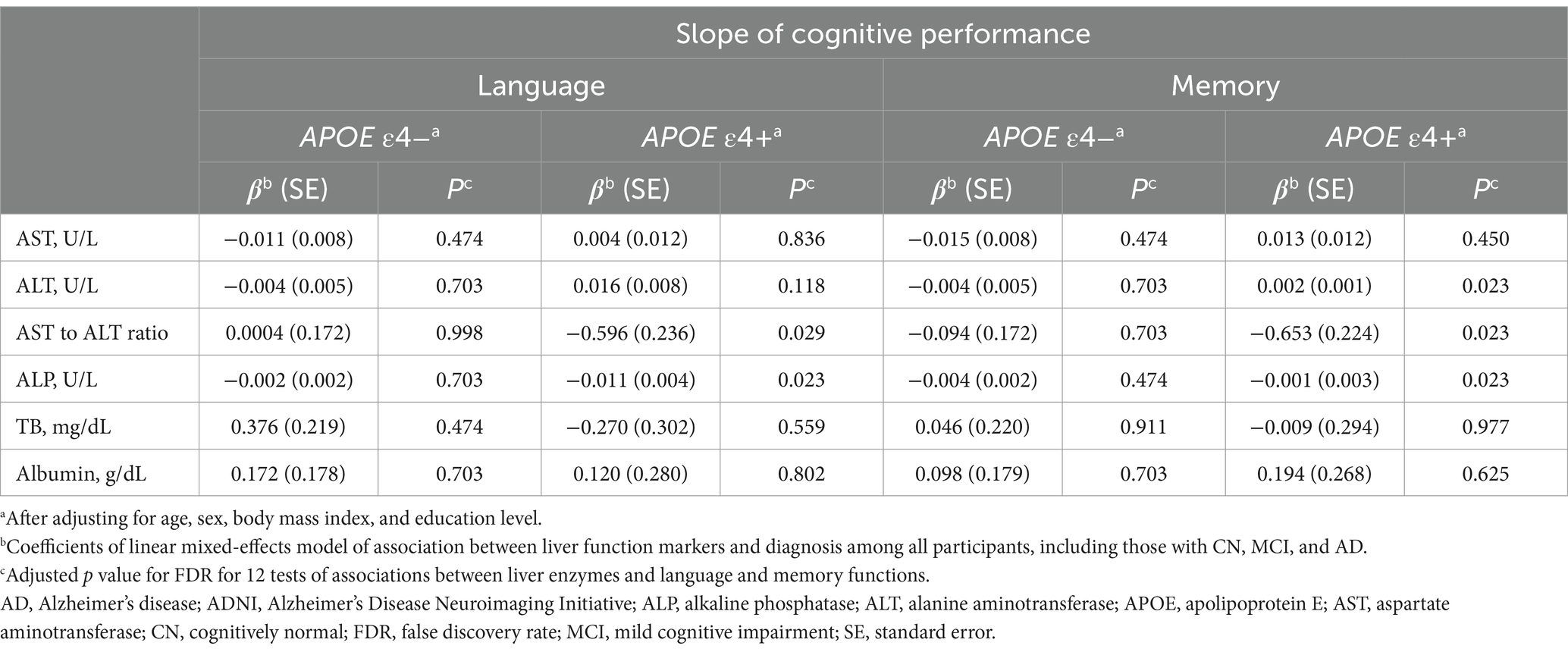
Table 9. Results of the association analysis of liver function markers with cognition in the ADNI cohort.
3.5 Mediation analysis of liver function markers on amyloid PET burden or AD diagnosis
In the HUMC cohort, mediation analyses revealed that the associations of ALT levels and the AST to ALT ratio with amyloid PET positivity (total effect: ALT; β = −0.009, p = 0.003; AST to ALT ratio; β = 0.379, p < 0.001; mediating effect: ALT; β = −0.001, p = 0.158; AST to ALT ratio; β = 0.018, p = 0.360) and AD diagnosis (total effect: ALT; β = −0.001, p < 0.001; AST to ALT ratio; β = 0.456, p < 0.001; mediating effect: ALT; β = −0.00004, p = 0.468; AST to ALT ratio; β = 0.009, p = 0.750) in the APOE ε4 carrier group were not mediated by age (Figure 2 and Table 10). Furthermore, the association between the AST to ALT ratio and AD diagnosis was significantly mediated by amyloid PET positivity (total effect: β = 0.451, p < 0.001; mediating effect: β = 0.184, p < 0.001), indicating a substantial indirect effect in the APOE ε4 carrier group.
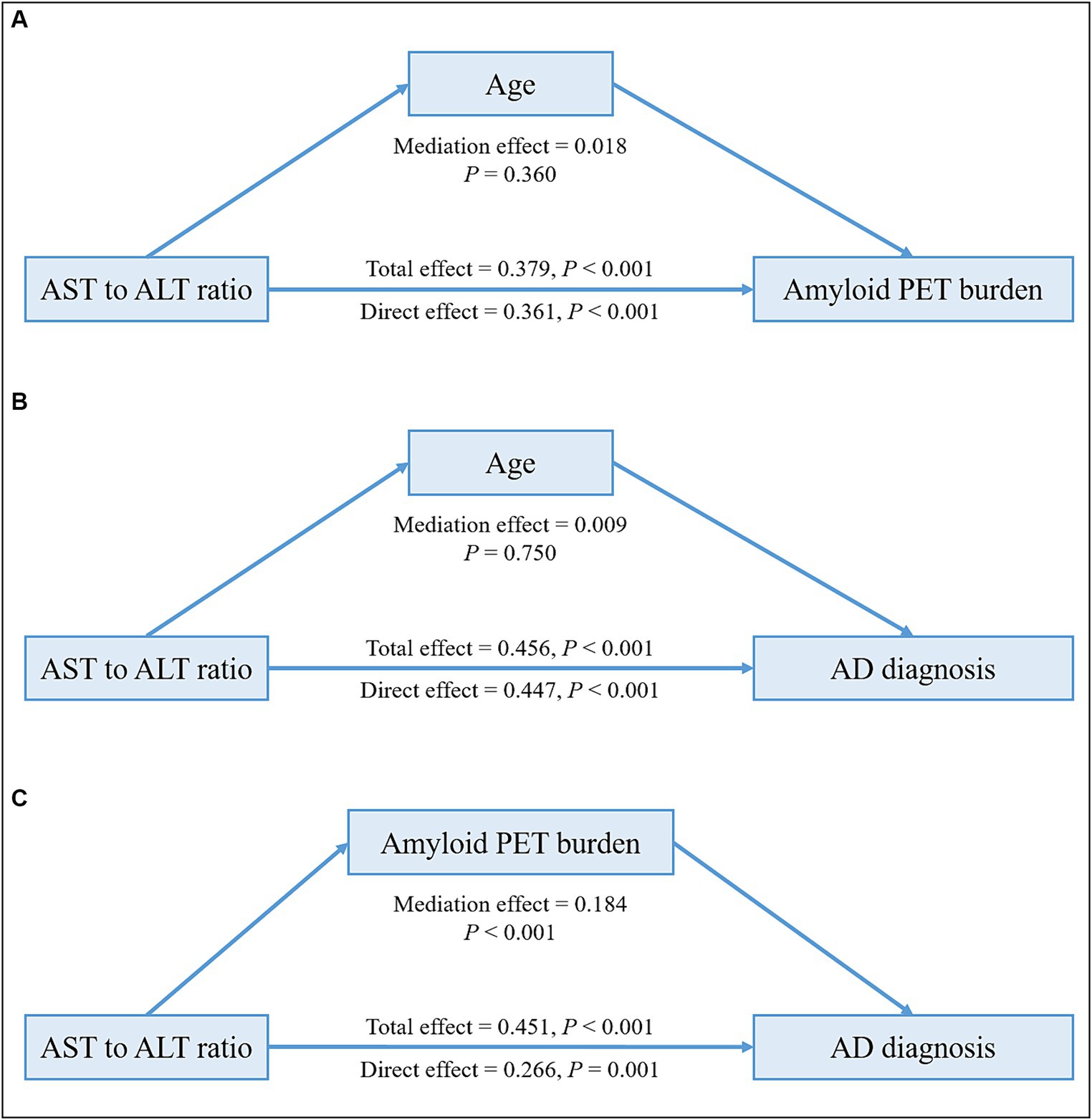
Figure 2. Mediation analysis of liver function markers on amyloid PET burden or diagnosis among the APOE ε4 carrier group in the HUMC cohort. Mediation effects of liver function markers on amyloid PET burden through age (A), on AD diagnosis through age (B), and on AD diagnosis through amyloid PET burden (C) in the APOE ε4 carrier group. All analyses were adjusted for age, sex, hypertension, diabetes mellitus, dyslipidemia, and statin use. AD, Alzheimer’s disease; ALT, alanine aminotransferase; APOE, apolipoprotein E; AST, aspartate aminotransferase; HUMC, Hallym University Medical Centers; PET, positron emission tomography.
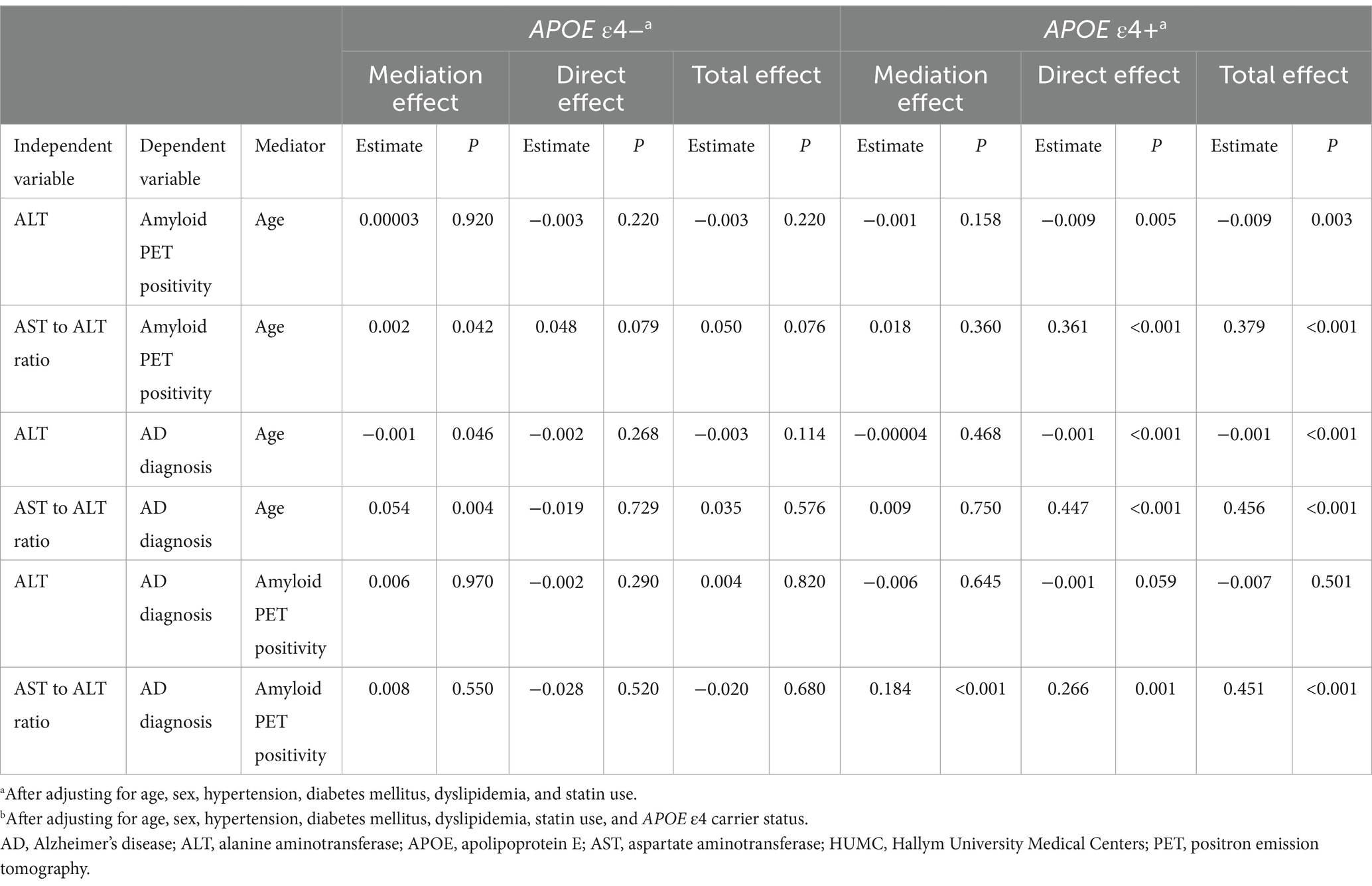
Table 10. Results of the mediation analysis of liver function markers on amyloid PET positivity or diagnosis in the HUMC cohort.
In the ADNI cohort, mediation analyses revealed that the associations of ALT levels and the AST to ALT ratio with amyloid PET global SUVR (total effect: ALT; β = −0.006, p = 0.002; AST to ALT ratio; β = 0.178, p = 0.012; mediating effect: ALT; β = −0.0002, p = 0.464; AST to ALT ratio; β = 0.013, p = 0.416) and AD diagnosis (total effect: ALT; β = −0.013, p = 0.009; AST to ALT ratio; β = 0.189, p = 0.009; mediating effect: ALT; β = 0.0001, p = 0.922; AST to ALT ratio; β = 0.005, p = 0.806) were not mediated by age in the APOE ε4 carrier group (Figure 3 and Table 11). The associations of AD diagnosis with ALT (total effect: β = −0.013, p = 0.017; mediating effect: β = −0.007, p = 0.002) and the AST to ALT ratio (total effect: β = 0.184, p = 0.032; mediating effect: β = 0.106, p = 0.024) were significantly mediated by amyloid PET global SUVR, indicating a notable indirect effect in the APOE ε4 carrier group. Likewise, in the case of ALP levels, the association with AD diagnosis was not mediated by age but was significantly mediated by amyloid PET global SUVR (total effect: β = 0.0012, p = 0.002; mediating effect: β = 0.0004, p = 0.039), exclusively in the APOE ε4 carrier group (Table 11).
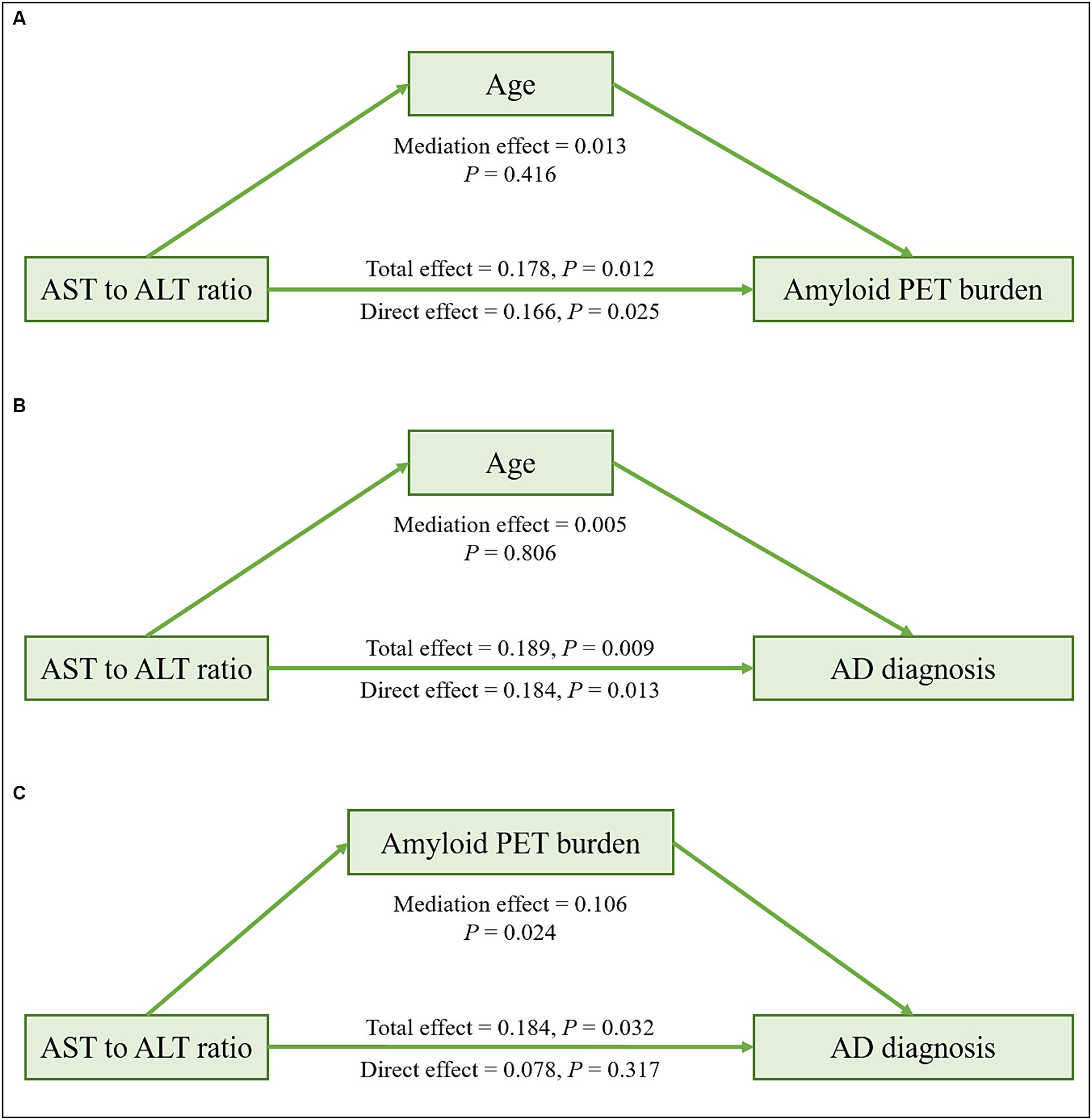
Figure 3. Mediation analysis of liver function markers on amyloid PET burden or diagnosis among the APOE ε4 carrier group in the ADNI cohort. Mediation effects of liver function markers on amyloid PET burden through age (A), on AD diagnosis through age (B), and on AD diagnosis through amyloid PET burden (C) in the APOE ε4 carrier group. All analyses were adjusted for age, sex, and body mass index. AD, Alzheimer’s disease; ADNI, Alzheimer’s Disease Neuroimaging Initiative; ALT, alanine aminotransferase; APOE, apolipoprotein E; AST, aspartate aminotransferase; PET, positron emission tomography.
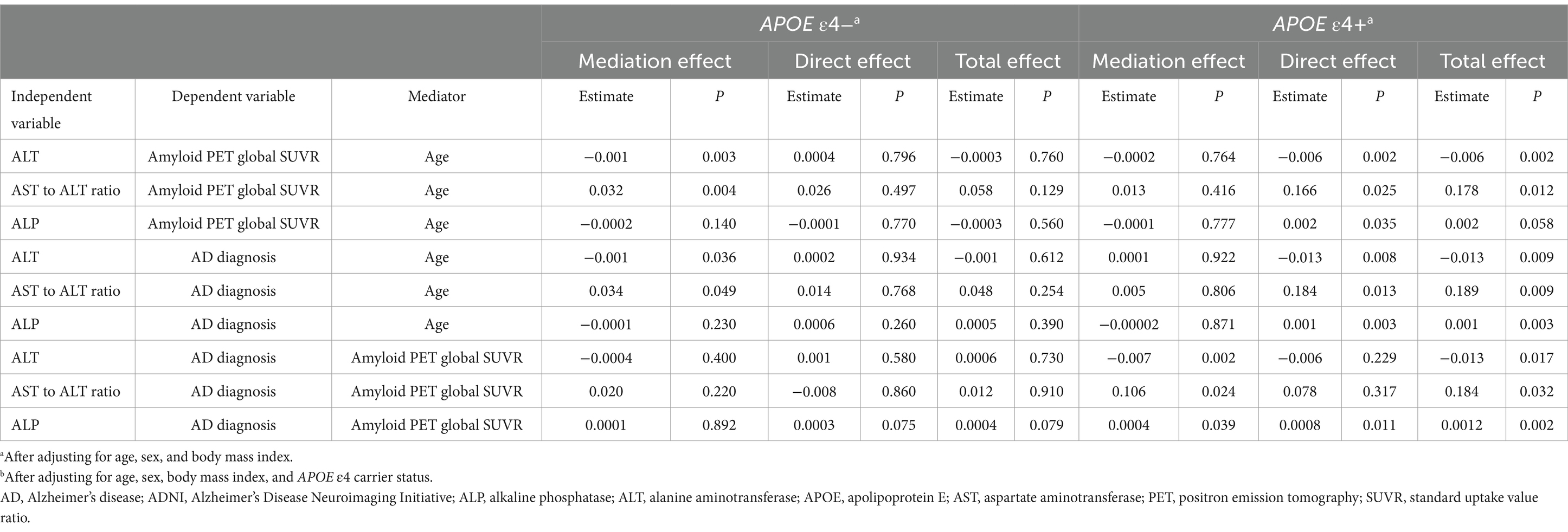
Table 11. Results of the mediation analysis of liver function markers on amyloid PET global SUVR or diagnosis in the ADNI cohort.
4 Discussion
In this study, we comprehensively investigated the association of liver function markers with AD diagnosis, amyloid PET burden, CSF biomarkers for AD, and cognition in two independent cohorts. A particularly novel aspect of this study was its emphasis on how this association might differ based on the presence of the APOE ε4 allele.
The findings shed light on significant associations between liver enzyme levels, particularly ALT levels and the AST to ALT ratio, with AD diagnosis, amyloid PET burden, and cognitive function, especially among individuals carrying the APOE ε4 allele. However, these associations were not observed in those without the APOE ε4 allele. Furthermore, in the ADNI cohort, we observed that ALP levels were significantly associated with AD diagnosis, amyloid PET burden, and cognition, but this association was specific to the APOE ε4 carrier group. Additionally, the AST to ALT ratio was significantly associated with CSF Aβ42 levels in the ADNI cohort, especially in the APOE ε4 carrier group, but no such correlation was found for p-tau181 or t-tau levels. These findings strongly suggest that the presence of the APOE ε4 allele may play a crucial role in Aβ accumulation and cognitive decline in AD, potentially through its impact on liver function. The relationship between the APOE ε4 allele and liver enzymes might reflect underlying changes in hepatic lipid metabolism, which could subsequently influence cerebral Aβ aggregation and contribute to the neuropathology of AD (D’Alonzo et al., 2023).
Intriguingly, our mediation analyses, conducted in two independent cohorts, demonstrated that age did not mediate the associations of liver enzymes with amyloid PET burden and AD diagnosis in the APOE ε4 carrier group. Importantly, the associations between liver enzymes and AD diagnosis were partially mediated by the amyloid PET burden, and this mediation effect was particularly observed in the APOE ε4 carrier group.
This study has provided insights into the association of the APOE ε4 allele and serum liver enzymes with AD pathogenesis and longitudinal changes in cognition, as evidenced by the analysis of two independent cohorts. Moreover, our findings also highlighted that the effect of liver enzymes on AD was mediated through amyloid PET burden, with the impact of this mediation varying depending on whether an individual carries the APOE ε4 allele.
The APOE gene encodes APOE, a 35-kDa glycoprotein with widespread expression throughout the human body that serves as a key lipid transporter (Muñoz et al., 2019). Notably, the APOE ε4 allele is recognized as the most potent genetic risk factor for AD, and its influence increases in a gene dose-dependent manner (Farrer et al., 1997). In comparison with the APOE ε3 or ε2 allele, the APOE ε4 allele significantly increases the risk of AD by promoting the accumulation of Aβ in the brain (Castellano et al., 2011). Astrocytes are the primary source of APOE, which aids in the transportation of cholesterol to neurons via APOE receptors within the brain (Liu et al., 2013). Conversely, in peripheral tissues, hepatocytes are the primary producers of APOE, which is released into the bloodstream to regulate cholesterol metabolism in an isoform-dependent manner (Chernick et al., 2019). APOE, primarily generated by the liver, is distinct from the form found in the brain and separated by the blood–brain barrier (Chernick et al., 2019). Despite their physical separation, mounting evidence suggests that peripheral APOE could potentially influence insulin signaling, neuroinflammation, and synaptic function in the brain (Lane-Donovan et al., 2016; Giannisis et al., 2022). Moreover, plasma levels of APOE isoforms have been found to correlate with regional brain volume, cerebral glucose metabolism, and cognitive performance (Nielsen et al., 2017). In mice models, ApoE4 has been shown to impede the Aβ peripheral clearance (Sharman et al., 2010), while a biologically inspired nanostructure known as ApoE3-reconstituted high-density lipoprotein, which exhibits a strong binding affinity to Aβ, has been found to restore memory deficits by accelerating Aβ clearance (Song et al., 2014). Moreover, expression of ApoE4 in the liver has been found to exacerbate brain amyloid pathology, whereas liver-expressed ApoE3 has demonstrated beneficial effects on brain function and mitigated amyloid deposition in a mouse model (Liu et al., 2022). Despite these intriguing associations, there is no consensus regarding the exact relationship between the APOE allele and AD pathogenesis. For instance, Huynh et al. reported that the deletion of ApoE in the hepatocytes of APP/PS1 mice, resulting in decreased plasma ApoE levels but no change in brain ApoE levels, did not influence the amount of amyloid plaques (Huynh et al., 2019). Furthermore, there is supporting evidence that serum-based liver function markers, including AST, ALT, and ALP levels and the AST to ALT ratio, are associated with AD diagnosis, poor cognitive performance, and increased Aβ deposition (Kellett et al., 2011; Nho et al., 2019; Lu et al., 2021; Ferri et al., 2022; Han et al., 2022; Li et al., 2022). However, there is a lack of studies investigating the effects of the APOE ε4 allele on the association of liver function markers with AD pathogenesis and cognition in humans. Some of these concerns are partially addressed by the findings of our study, which showed that ALT levels and the AST to ALT ratio were significantly associated with AD diagnosis, Aβ accumulation, and cognition but only in the APOE ε4 carrier group across the two independent cohorts. Notably, such a correlation was not evident when assessing p-tau181 or t-tau levels. In particular, our mediation analysis revealed that the brain Aβ burden partially mediated the association between liver function markers and AD, exclusively in APOE ε4 carrier group. These findings indicate that liver function is associated with the accumulation of Aβ in the brain in AD, and this relationship depends on an individual’s APOE ε4 carrier status.
The primary endogenous peripheral receptor responsible for regulating plasma Aβ, thereby preventing Aβ access to the brain, is circulating low-density lipoprotein receptor (LDL)-related protein 1 (LRP1) (Tamaki et al., 2006; Zlokovic et al., 2010). In the liver, LRP1, in conjunction with LDL receptor, plays a crucial role in the clearance of circulating Aβ and APOE-containing particles from the bloodstream (Zlokovic et al., 2010; Van De Sluis et al., 2017). The decrease in LRP-1 expression is implicated in age-related decline in hepatic Aβ clearance (Tamaki et al., 2006). This impaired degradation of Aβ in the liver may lead to increased accumulation of Aβ in the brain (Maarouf et al., 2018). In our mediation analysis, we observed that the association of liver function markers with both brain Aβ burden and AD was not mediated by age, in the APOE ε4 carrier group. These results suggest that AD pathogenesis in APOE ε4 carriers is not predominantly driven by aging-related hepatic changes but rather through distinct mechanisms that impair hepatic Aβ clearance. As elucidated by D’Alonzo et al., the impact of the APOE ε4 allele on liver function involves impaired catabolism of lipoproteins, thereby increasing exposure to circulating lipoprotein-Aβ, which may lead to the Aβ aggregation in the brain and enhanced AD risk (D’Alonzo et al., 2023). Additionally, small high-density lipoproteins particles have been shown to influence brain Aβ levels, contributing to reduced AD risk through improved Aβ clearance and vascular function (Martinez et al., 2023).
The implications of liver function in the pathogenesis of AD offer intriguing insights into the potential therapeutic targets for AD. For instance, the notable therapeutic effects of the ayurvedic agent, Withania somnifera, achieved by increasing levels of liver LRP, suggests that targeting peripheral Aβ clearance may provide a unique approach to rapidly eliminate Aβ in AD transgenic mice (Sehgal et al., 2012). Statins have also demonstrated the potential to reduce the risk of AD by upregulating hepatic LRP1 and LDL receptor expression, which is mediated by sterol response element-binding protein-2 (Moon et al., 2011; Zissimopoulos et al., 2017). Additionally, transthyretin, a transporter protein primarily produced in the liver and released into the bloodstream, is downregulated in AD (Han et al., 2011). Given its role as a carrier of Aβ at the blood–brain barrier and in the liver, particularly via LRP1, transthyretin may offer valuable insights into the development of therapeutic strategies for AD (Alemi et al., 2016, 2017).
This study, while providing valuable insights into the relationship between APOE ε4 allele status, liver function tests, and AD biomarkers, is subject to several limitations that must be carefully considered. Firstly, the cross-sectional design of our analyses limits our ability to determine causality or temporal relationships among liver function, APOE ε4 allele status, and AD biomarkers. Moreover, while the HUMC and ADNI cohorts are typical of clinic participants, they may not represent the broader community, necessitating further validation of these results in more diverse socio-economic, educational, and racial groups. Secondly, our study was the inability to incorporate quantitative measures of amyloid PET in the HUMC cohort when investigating the relationship between liver enzymes and the amyloid PET burden. This limitation arises from the absence of imaging data, which restricts the depth of the analysis. However, the practice of visually rating amyloid PET scans remains valuable in the clinical setting. Thirdly, data on the presence of hepatitis were not available in both the HUMC and ADNI cohorts. Additionally, the ADNI cohort did not have data on presence of hypertension, diabetes mellitus, and dyslipidemia, while the HUMC cohort did not have data on BMI, which is an important covariate associated with ALT levels (Ndrepepa and Kastrati, 2019). Furthermore, the HUMC cohort did not have data on the CSF biomarkers for AD. Fourthly, the use of different cognitive tests to generate composite scores for distinct cognitive domains across cohorts, along with variations in diagnostic group frequencies and definitions, may have introduced variability and potential confounding factors into our results. Fifthly, the use of linear regression models in our study to investigate the association between liver enzyme levels and AD outcomes may not have adequately captured potential non-linear relationships. Lastly, our study did not fully address the potential role of gene–environment interactions, such as lifestyle factors like diet and alcohol use, in modulating the influence of APOE ε4 allele on AD risk and liver function, emphasizing the necessity for in-depth study on interventions to reduce AD risk.
5 Conclusion
In summary, our study across two independent cohorts provides valuable insights into the pivotal association of APOE ε4 status and liver enzymes with Aβ-related pathogenesis and cognition in AD. Future research should focus on unraveling the biological pathways at the intersection of liver function and AD, aiming to identify novel therapeutic targets that could mitigate the progression of AD.
Data availability statement
The HUMC datasets used during the current study are not readily available due to their containing information that could compromise the privacy of research participants. Publicly available datasets from the ADNI cohort were analyzed in this study. This data can be found at: http://adni.loni.usc.edu.
Ethics statement
The protocol for the HUMC study has been approved by the Clinical Research Ethics Committee of Chuncheon Sacred Heart Hospital, Hallym University (IRB No. 2023-07-009) and conforms to the provisions of the Declaration of Helsinki. All data sources mentioned in the ADNI studies are publically available summary level information that requires no ethical approval or consent. The ADNI obtained ethics approvals from all sites collecting data in accordance with the standards of the local ethics committee and Institutional Review Board boards.
Author contributions
S-WH: Conceptualization, Data curation, Formal analysis, Investigation, Methodology, Writing – original draft, Writing – review & editing. S-HL: Writing – original draft, Writing – review & editing. JK: Writing – original draft, Writing – review & editing. J-JL: Writing – original draft, Writing – review & editing. YP: Writing – original draft, Writing – review & editing. SK: Writing – original draft, Writing – review & editing. KN: Writing – original draft, Writing – review & editing. J-HS: Conceptualization, Data curation, Formal analysis, Funding acquisition, Investigation, Methodology, Supervision, Writing – original draft, Writing – review & editing.
Funding
The author(s) declare that financial support was received for the research, authorship, and/or publication of this article. This research was supported by the Bio & Medical Technology Development Program of the National Research Foundation (NRF) funded by the Korean government (MSIT) (No. RS-2023-00223501).
Conflict of interest
The authors declare that the research was conducted in the absence of any commercial or financial relationships that could be construed as a potential conflict of interest.
Publisher’s note
All claims expressed in this article are solely those of the authors and do not necessarily represent those of their affiliated organizations, or those of the publisher, the editors and the reviewers. Any product that may be evaluated in this article, or claim that may be made by its manufacturer, is not guaranteed or endorsed by the publisher.
Footnotes
References
ADNI2 (2020). Alzheimer’s Disease Neuroimaging Initiative: ADNI2 procedures manual. Available at: https://adni.loni.usc.edu/wpcontent/uploads/2008/07/adni2-procedures-manual.pdf (Accessed May 21, 2020).
ADNIGO (2009). Alzheimer’s Disease Neuroimaging Initiative: ADNI Grand Opportunities procedures manual. Available at: http://adni.loni.usc.edu/wp-content/uploads/2008/07/ADNI_GO_Procedures_Manual_06102011.pdf
Aisen, P. S., Petersen, R. C., Donohue, M. C., Gamst, A., Raman, R., Thomas, R. G., et al. (2010). Clinical Core of the Alzheimer's disease neuroimaging Initiative: progress and plans. Alzheimers Dement. 6, 239–246. doi: 10.1016/j.jalz.2010.03.006
Aisen, P. S., Petersen, R. C., Donohue, M., and Weiner, M. W.Alzheimer’s Disease Neuroimaging Initiative (2015). Alzheimer’s disease neuroimaging initiative 2 clinical core: progress and plans. Alzheimers Dement. 11, 734–739. doi: 10.1016/j.jalz.2015.05.005
Albert, M. S., DeKosky, S. T., Dickson, D., Dubois, B., Feldman, H. H., Fox, N. C., et al. (2011). The diagnosis of mild cognitive impairment due to Alzheimer's disease: recommendations from the National Institute on Aging-Alzheimer's Association workgroups on diagnostic guidelines for Alzheimer's disease. Alzheimers Dement. 7, 270–279. doi: 10.1016/j.jalz.2011.03.008
Alemi, M., Gaiteiro, C., Ribeiro, C. A., Santos, L. M., Gomes, J. R., Oliveira, S. M., et al. (2016). Transthyretin participates in beta-amyloid transport from the brain to the liver-involvement of the low-density lipoprotein receptor-related protein 1? Sci. Rep. 6:20164. doi: 10.1038/srep20164
Alemi, M., Silva, S. C., Santana, I., and Cardoso, I. (2017). Transthyretin stability is critical in assisting beta amyloid clearance–relevance of transthyretin stabilization in Alzheimer's disease. CNS Neurosci. Ther. 23, 605–619. doi: 10.1111/cns.12707
ADNI1 . (2010). Alzheimer’s Disease Neuroimaging Initiative: ADNI Procedures Manual. Available at: http://adni.loni.usc.edu/wp-content/uploads/2010/09/ADNI_GeneralProceduresManual.pdf
Armstrong, M. J., Litvan, I., Lang, A. E., Bak, T. H., Bhatia, K. P., Borroni, B., et al. (2013). Criteria for the diagnosis of corticobasal degeneration. Neurology 80, 496–503. doi: 10.1212/WNL.0b013e31827f0fd1
Barthel, H., Gertz, H.-J., Dresel, S., Peters, O., Bartenstein, P., Buerger, K., et al. (2011). Cerebral amyloid-β PET with florbetaben (18F) in patients with Alzheimer's disease and healthy controls: a multicentre phase 2 diagnostic study. Lancet Neurol. 10, 424–435. doi: 10.1016/S1474-4422(11)70077-1
Bassendine, M. F., Taylor-Robinson, S. D., Fertleman, M., Khan, M., and Neely, D. (2020). Is Alzheimer’s disease a liver disease of the brain? J. Alzheimers Dis. 75, 1–14. doi: 10.3233/JAD-190848
Benjamini, Y., and Hochberg, Y. (1995). Controlling the false discovery rate: a practical and powerful approach to multiple testing. J. R. Stat. Soc. Ser. B Methodol. 57, 289–300. doi: 10.1111/j.2517-6161.1995.tb02031.x
Castellano, J. M., Kim, J., Stewart, F. R., Jiang, H., DeMattos, R. B., Patterson, B. W., et al. (2011). Human apoE isoforms differentially regulate brain amyloid-β peptide clearance. Sci. Transl. Med. 3:89ra57. doi: 10.1126/scitranslmed.3002156
Cheng, Y., Tian, D.-Y., and Wang, Y.-J. (2020). Peripheral clearance of brain-derived Aβ in Alzheimer's disease: pathophysiology and therapeutic perspectives. Transl. Neurodegener. 9, 1–11. doi: 10.1186/s40035-020-00195-1
Chernick, D., Ortiz-Valle, S., Jeong, A., Qu, W., and Li, L. (2019). Peripheral versus central nervous system APOE in Alzheimer’s disease: interplay across the blood-brain barrier. Neurosci. Lett. 708:134306. doi: 10.1016/j.neulet.2019.134306
D’Alonzo, Z. J., Lam, V., Takechi, R., Nesbit, M., Vaccarezza, M., and Mamo, J. C. (2023). Peripheral metabolism of lipoprotein-amyloid beta as a risk factor for Alzheimer’s disease: potential interactive effects of APOE genotype with dietary fats. Genes Nutr. 18:2. doi: 10.1186/s12263-023-00722-5
Doweiko, J. P., and Nompleggi, D. J. (1991). Reviews: role of albumin in human physiology and pathophysiology. J. Parenter. Enter. Nutr. 15, 207–211. doi: 10.1177/0148607191015002207
Dubois, B., Burn, D., Goetz, C., Aarsland, D., Brown, R. G., Broe, G. A., et al. (2007). Diagnostic procedures for Parkinson's disease dementia: recommendations from the movement disorder society task force. Mov. Disord. 22, 2314–2324. doi: 10.1002/mds.21844
Estrada, L. D., Ahumada, P., Cabrera, D., and Arab, J. P. (2019). Liver dysfunction as a novel player in Alzheimer’s progression: looking outside the brain. Front. Aging Neurosci. 11:174. doi: 10.3389/fnagi.2019.00174
Farrar, G., Molinuevo, J. L., and Zanette, M. (2019). Is there a difference in regional read [18 F] flutemetamol amyloid patterns between end-of-life subjects and those with amnestic mild cognitive impairment? Eur. J. Nucl. Med. Mol. Imaging 46, 1299–1308. doi: 10.1007/s00259-019-04282-y
Farrer, L. A., Cupples, L. A., Haines, J. L., Hyman, B., Kukull, W. A., Mayeux, R., et al. (1997). Effects of age, sex, and ethnicity on the association between apolipoprotein E genotype and Alzheimer disease: a meta-analysis. JAMA 278, 1349–1356. doi: 10.1001/jama.1997.03550160069041
Ferri, E., Rossi, P. D., Scichilone, M., Lucchi, T. A., and Arosio, B. (2022). Liver enzymes in a cohort of community-dwelling older persons: focus on sex contribution. Nutrients 14:4973. doi: 10.3390/nu14234973
Folstein, M. F., Folstein, S. E., and McHugh, P. R. (1975). “Mini-mental state”: a practical method for grading the cognitive state of patients for the clinician. J. Psychiatr. Res. 12, 189–198. doi: 10.1016/0022-3956(75)90026-6
Franchini, M., Targher, G., and Lippi, G. (2010). Serum bilirubin levels and cardiovascular disease risk: a Janus Bifrons? Adv. Clin. Chem. 50, 47–63. doi: 10.1016/S0065-2423(10)50003-9
Giannisis, A., Patra, K., Edlund, A. K., Nieto, L. A., Benedicto-Gras, J., Moussaud, S., et al. (2022). Brain integrity is altered by hepatic APOE ε4 in humanized-liver mice. Mol. Psychiatry 27, 3533–3543. doi: 10.1038/s41380-022-01548-0
Gorno-Tempini, M. L., Hillis, A. E., Weintraub, S., Kertesz, A., Mendez, M., Cappa, S. F., et al. (2011). Classification of primary progressive aphasia and its variants. Neurology 76, 1006–1014. doi: 10.1212/WNL.0b013e31821103e6
Hampel, H., Frank, R., Broich, K., Teipel, S. J., Katz, R. G., Hardy, J., et al. (2010). Biomarkers for Alzheimer's disease: academic, industry and regulatory perspectives. Nat. Rev. Drug Discov. 9, 560–574. doi: 10.1038/nrd3115
Han, S.-H., Jung, E. S., Sohn, J.-H., Hong, H. J., Hong, H. S., Kim, J. W., et al. (2011). Human serum transthyretin levels correlate inversely with Alzheimer's disease. J. Alzheimers Dis. 25, 77–84. doi: 10.3233/JAD-2011-102145
Han, S.-W., Park, Y. H., Jang, E. S., Nho, K., and Kim, S. (2022). Implications of liver enzymes in the pathogenesis of Alzheimer’s disease. J. Alzheimers Dis. 88, 1371–1376. doi: 10.3233/JAD-220343
Hansson, O., Seibyl, J., Stomrud, E., Zetterberg, H., Trojanowski, J., Bittner, T., et al. (2018). Alzheimer's disease neuroimaging Initiative CSF biomarkers of Alzheimer's disease concord with amyloid-β PET and predict clinical progression: a study of fully automated immunoassays in BioFINDER and ADNI cohorts. Alzheimers Dement. 14, 1470–1481. doi: 10.1016/j.jalz.2018.01.010
Hayes, A. F. (2009). Beyond baron and Kenny: statistical mediation analysis in the new millennium. Commun. Monogr. 76, 408–420. doi: 10.1080/03637750903310360
Huang, Z., Lin, H. W., Zhang, Q., and Zong, X. (2022). Targeting Alzheimer’s disease: the critical crosstalk between the liver and brain. Nutrients 14:4298. doi: 10.3390/nu14204298
Huynh, T.-P. V., Wang, C., Tran, A. C., Tabor, G. T., Mahan, T. E., Francis, C. M., et al. (2019). Lack of hepatic apoE does not influence early Aβ deposition: observations from a new APOE knock-in model. Mol. Neurodegener. 14, 1–23. doi: 10.1186/s13024-019-0337-1
Jagust, W. J., Bandy, D., Chen, K., Foster, N. L., Landau, S. M., Mathis, C. A., et al. (2010). The Alzheimer's disease neuroimaging Initiative positron emission tomography core. Alzheimers Dement. 6, 221–229. doi: 10.1016/j.jalz.2010.03.003
Jagust, W. J., Landau, S. M., Koeppe, R. A., Reiman, E. M., Chen, K., Mathis, C. A., et al. (2015). The Alzheimer's disease neuroimaging initiative 2 PET core: 2015. Alzheimers Dement. 11, 757–771. doi: 10.1016/j.jalz.2015.05.001
Jessen, F., Amariglio, R. E., Van Boxtel, M., Breteler, M., Ceccaldi, M., Chételat, G., et al. (2014). A conceptual framework for research on subjective cognitive decline in preclinical Alzheimer’s disease. Alzheimers Dement. 10, 844–852. doi: 10.1016/j.jalz.2014.01.001
Kamada, Y., Hashimoto, R., Yamamori, H., Yasuda, Y., Takehara, T., Fujita, Y., et al. (2016). Impact of plasma transaminase levels on the peripheral blood glutamate levels and memory functions in healthy subjects. BBA Clin. 5, 101–107. doi: 10.1016/j.bbacli.2016.02.004
Kang, J.-H., Korecka, M., Figurski, M. J., Toledo, J. B., Blennow, K., Zetterberg, H., et al. (2015). The Alzheimer’s disease neuroimaging initiative 2 biomarker core: a review of progress and plans. Alzheimers Dement. 11, 772–791. doi: 10.1016/j.jalz.2015.05.003
Kang, Y., Na, D., and Hahn, S. (2003). Seoul neuropsychological screening battery. Seoul: Human Brain Research & Consulting Co.
Kellett, K. A., Williams, J., Vardy, E. R., Smith, A. D., and Hooper, N. M. (2011). Plasma alkaline phosphatase is elevated in Alzheimer’s disease and inversely correlates with cognitive function. Int. J. Mol. Epidemiol. Genet. 2, 114–121
Kim, H., and Na, D. L. (1999). BRIEF REPORT normative data on the Korean version of the Boston naming test. J. Clin. Exp. Neuropsychol. 21, 127–133. doi: 10.1076/jcen.21.1.127.942
Lane-Donovan, C., Wong, W. M., Durakoglugil, M. S., Wasser, C. R., Jiang, S., Xian, X., et al. (2016). Genetic restoration of plasma ApoE improves cognition and partially restores synaptic defects in ApoE-deficient mice. J. Neurosci. 36, 10141–10150. doi: 10.1523/JNEUROSCI.1054-16.2016
Li, W., Yue, L., Sun, L., and Xiao, S. (2022). An increased aspartate to alanine aminotransferase ratio is associated with a higher risk of cognitive impairment. Front. Med. 9:780174. doi: 10.3389/fmed.2022.780174
Litvan, I., Agid, Y., Calne, D., Campbell, G., Dubois, B., Duvoisin, R., et al. (1996). Clinical research criteria for the diagnosis of progressive supranuclear palsy (Steele-Richardson-Olszewski syndrome): report of the NINDS-SPSP international workshop. Neurology 47, 1–9. doi: 10.1212/WNL.47.1.1
Liu, C.-C., Kanekiyo, T., Xu, H., and Bu, G. (2013). Apolipoprotein E and Alzheimer disease: risk, mechanisms and therapy. Nat. Rev. Neurol. 9, 106–118. doi: 10.1038/nrneurol.2012.263
Liu, C.-C., Zhao, J., Fu, Y., Inoue, Y., Ren, Y., Chen, Y., et al. (2022). Peripheral apoE4 enhances Alzheimer’s pathology and impairs cognition by compromising cerebrovascular function. Nat. Neurosci. 25, 1020–1033. doi: 10.1038/s41593-022-01127-0
Long, J. M., and Holtzman, D. M. (2019). Alzheimer disease: an update on pathobiology and treatment strategies. Cell 179, 312–339. doi: 10.1016/j.cell.2019.09.001
Lu, Y., Pike, J. R., Selvin, E., Mosley, T., Palta, P., Sharrett, A. R., et al. (2021). Low liver enzymes and risk of dementia: the atherosclerosis risk in communities (ARIC) study. J. Alzheimers Dis. 79, 1775–1784. doi: 10.3233/JAD-201241
Lum, G. (1995). Significance of low serum alkaline phosphatase activity in a predominantly adult male population. Clin. Chem. 41, 515–518. doi: 10.1093/clinchem/41.4.515
Maarouf, C. L., Walker, J. E., Sue, L. I., Dugger, B. N., Beach, T. G., and Serrano, G. E. (2018). Impaired hepatic amyloid-beta degradation in Alzheimer’s disease. PLoS One 13:e0203659. doi: 10.1371/journal.pone.0203659
Martinez, A. E., Weissberger, G., Kuklenyik, Z., He, X., Meuret, C., Parekh, T., et al. (2023). The small HDL particle hypothesis of Alzheimer’s disease. Alzheimers Dement. 19, 391–404. doi: 10.1002/alz.12649
McKhann, G. M., Knopman, D. S., Chertkow, H., Hyman, B. T., Jack, C. R. Jr., Kawas, C. H., et al. (2011). The diagnosis of dementia due to Alzheimer’s disease: recommendations from the National Institute on Aging-Alzheimer’s association workgroups on diagnostic guidelines for Alzheimer’s disease. Alzheimers Dement. 7, 263–269. doi: 10.1016/j.jalz.2011.03.005
Mohs, R. C., Knopman, D., Petersen, R. C., Ferris, S. H., Ernesto, C., Grundman, M., et al. (1997). Development of cognitive instruments for use in clinical trials of antidementia drugs: additions to the Alzheimer’s disease assessment scale that broaden its scope. Alzheimer Dis. Assoc. Disord. 11, 13–21. doi: 10.1097/00002093-199700112-00003
Moon, J. H., Kang, S. B., Park, J. S., Lee, B. W., Kang, E. S., Ahn, C. W., et al. (2011). Up-regulation of hepatic low-density lipoprotein receptor–related protein 1: a possible novel mechanism of antiatherogenic activity of hydroxymethylglutaryl–coenzyme A reductase inhibitor: atorvastatin and hepatic LRP1 expression. Metabolism 60, 930–940. doi: 10.1016/j.metabol.2010.08.013
Morris, J. C. (1993). The clinical dementia rating (CDR): current version and scoring rules. Neurology 43:2412. doi: 10.1212/WNL.43.11.2412-a
Muñoz, S. S., Garner, B., and Ooi, L. (2019). Understanding the role of ApoE fragments in Alzheimer’s disease. Neurochem. Res. 44, 1297–1305. doi: 10.1007/s11064-018-2629-1
Nasreddine, Z. S., Phillips, N. A., Bédirian, V., Charbonneau, S., Whitehead, V., Collin, I., et al. (2005). The Montreal cognitive assessment, MoCA: a brief screening tool for mild cognitive impairment. J. Am. Geriatr. Soc. 53, 695–699. doi: 10.1111/j.1532-5415.2005.53221.x
Ndrepepa, G., and Kastrati, A. (2019). Alanine aminotransferase—a marker of cardiovascular risk at high and low activity levels. J. Lab. Precis. Med. 4:29. doi: 10.21037/jlpm.2019.08.01
Neuschwander-Tetri, B. A., Ünalp, A., Creer, M. H., and Network, N. S. C. R. (2008). Influence of local reference populations on upper limits of normal for serum alanine aminotransferase levels. Arch. Intern. Med. 168, 663–666. doi: 10.1001/archinternmed.2007.131
Nho, K., Kueider-Paisley, A., Ahmad, S., MahmoudianDehkordi, S., Arnold, M., Risacher, S. L., et al. (2019). Association of altered liver enzymes with Alzheimer disease diagnosis, cognition, neuroimaging measures, and cerebrospinal fluid biomarkers. JAMA Netw. Open 2, –e197978. doi: 10.1001/jamanetworkopen.2019.7978
Nielsen, H. M., Chen, K., Lee, W., Chen, Y., Bauer, R. J., Reiman, E., et al. (2017). Peripheral apoE isoform levels in cognitively normal APOE ε3/ε4 individuals are associated with regional gray matter volume and cerebral glucose metabolism. Alzheimers Res. Ther. 9, 1–10. doi: 10.1186/s13195-016-0231-9
Nyblom, H., Björnsson, E., Simrén, M., Aldenborg, F., Almer, S., and Olsson, R. (2006). The AST/ALT ratio as an indicator of cirrhosis in patients with PBC. Liver Int. 26, 840–845. doi: 10.1111/j.1478-3231.2006.01304.x
Olsson, A., Vanderstichele, H., Andreasen, N., de Meyer, G., Wallin, A., Holmberg, B., et al. (2005). Simultaneous measurement of β-amyloid (1–42), total tau, and phosphorylated tau (Thr181) in cerebrospinal fluid by the xMAP technology. Clin. Chem. 51, 336–345. doi: 10.1373/clinchem.2004.039347
Petersen, R. C., Aisen, P. S., Beckett, L. A., Donohue, M. C., Gamst, A. C., Harvey, D. J., et al. (2010). Alzheimer's disease neuroimaging Initiative (ADNI) clinical characterization. Neurology 74, 201–209. doi: 10.1212/WNL.0b013e3181cb3e25
Pinheiro, J., and Bates, D. (2006). Mixed-effects models in S and S-PLUS. New York, NY: Springer Science & Business Media.
Rascovsky, K., Hodges, J. R., Knopman, D., Mendez, M. F., Kramer, J. H., Neuhaus, J., et al. (2011). Sensitivity of revised diagnostic criteria for the behavioural variant of frontotemporal dementia. Brain 134, 2456–2477. doi: 10.1093/brain/awr179
Saykin, A. J., Shen, L., Yao, X., Kim, S., Nho, K., Risacher, S. L., et al. (2015). Genetic studies of quantitative MCI and AD phenotypes in ADNI: progress, opportunities, and plans. Alzheimers Dement. 11, 792–814. doi: 10.1016/j.jalz.2015.05.009
Sehgal, N., Gupta, A., Valli, R. K., Joshi, S. D., Mills, J. T., Hamel, E., et al. (2012). Withania somnifera reverses Alzheimer's disease pathology by enhancing low-density lipoprotein receptor-related protein in liver. Proc. Natl. Acad. Sci. 109, 3510–3515. doi: 10.1073/pnas.1112209109
Sharman, M. J., Morici, M., Hone, E., Berger, T., Taddei, K., Martins, I. J., et al. (2010). APOE genotype results in differential effects on the peripheral clearance of amyloid-β 42 in APOE knock-in and knock-out mice. J. Alzheimers Dis. 21, 403–409. doi: 10.3233/JAD-2010-100141
Shaw, L. M., Vanderstichele, H., Knapik-Czajka, M., Clark, C. M., Aisen, P. S., Petersen, R. C., et al. (2009). Cerebrospinal fluid biomarker signature in Alzheimer's disease neuroimaging initiative subjects. Ann. Neurol. 65, 403–413. doi: 10.1002/ana.21610
Song, Q., Huang, M., Yao, L., Wang, X., Gu, X., Chen, J., et al. (2014). Lipoprotein-based nanoparticles rescue the memory loss of mice with Alzheimer’s disease by accelerating the clearance of amyloid-beta. ACS Nano 8, 2345–2359. doi: 10.1021/nn4058215
Spallazzi, M., Barocco, F., Michelini, G., Immovilli, P., Taga, A., Morelli, N., et al. (2019). CSF biomarkers and amyloid PET: concordance and diagnostic accuracy in a MCI cohort. Acta Neurol. Belg. 119, 445–452. doi: 10.1007/s13760-019-01112-8
Tamaki, C., Ohtsuki, S., Iwatsubo, T., Hashimoto, T., Yamada, K., Yabuki, C., et al. (2006). Major involvement of low-density lipoprotein receptor-related protein 1 in the clearance of plasma free amyloid β-peptide by the liver. Pharm. Res. 23, 1407–1416. doi: 10.1007/s11095-006-0208-7
Tingley, D., Yamamoto, T., Hirose, K., Keele, L., and Imai, K. (2014). Mediation: R package for causal mediation analysis. J. Stat. Softw. 59:1–38.
Van De Sluis, B., Wijers, M., and Herz, J. (2017). News on the molecular regulation and function of hepatic LDLR and LRP1. Curr. Opin. Lipidol. 28, 241–247. doi: 10.1097/MOL.0000000000000411
Vasantharekha, R., Priyanka, H. P., Swarnalingam, T., Srinivasan, A. V., and ThyagaRajan, S. (2017). Interrelationship between mini-mental state examination scores and biochemical parameters in patients with mild cognitive impairment and Alzheimer's disease. Geriatr Gerontol Int 17, 1737–1745. doi: 10.1111/ggi.12957
Weiner, M. W., Veitch, D. P., Aisen, P. S., Beckett, L. A., Cairns, N. J., Green, R. C., et al. (2017). The Alzheimer's disease neuroimaging Initiative 3: continued innovation for clinical trial improvement. Alzheimers Dement. 13, 561–571. doi: 10.1016/j.jalz.2016.10.006
Zissimopoulos, J. M., Barthold, D., Brinton, R. D., and Joyce, G. (2017). Sex and race differences in the association between statin use and the incidence of Alzheimer disease. JAMA Neurol. 74, 225–232. doi: 10.1001/jamaneurol.2016.3783
Zlokovic, B. V., Deane, R., Sagare, A. P., Bell, R. D., and Winkler, E. A. (2010). Low-density lipoprotein receptor-related protein-1: a serial clearance homeostatic mechanism controlling Alzheimer’s amyloid β-peptide elimination from the brain. J. Neurochem. 115, 1077–1089. doi: 10.1111/j.1471-4159.2010.07002.x
Keywords: Alzheimer’s disease, amyloid-β, APOE ε4, cognition, liver enzymes
Citation: Han S-W, Lee S-H, Kim JH, Lee J-J, Park YH, Kim S, Nho K and Sohn J-H (2024) Association of liver function markers and apolipoprotein E ε4 with pathogenesis and cognitive decline in Alzheimer’s disease. Front. Aging Neurosci. 16:1411466. doi: 10.3389/fnagi.2024.1411466
Edited by:
Eileen Ruth Samson Torres, Cornell University, United StatesReviewed by:
Yifei Lu, University of North Carolina at Chapel Hill, United StatesG. William Rebeck, Georgetown University, United States
Copyright © 2024 Han, Lee, Kim, Lee, Park, Kim, Nho and Sohn. This is an open-access article distributed under the terms of the Creative Commons Attribution License (CC BY). The use, distribution or reproduction in other forums is permitted, provided the original author(s) and the copyright owner(s) are credited and that the original publication in this journal is cited, in accordance with accepted academic practice. No use, distribution or reproduction is permitted which does not comply with these terms.
*Correspondence: Jong-Hee Sohn, deepfoci@hallym.or.kr