- 1School of the First Clinical Medicine, Gannan Medical University, Ganzhou, Jiangxi, China
- 2Teaching and Research Section of Clinical Nursing, School of Nursing, Gannan Medical University, Ganzhou, Jiangxi, China
- 3Neurology Department, LuoYang DongFang People’s Hospital, Luoyang Henan, China
- 4Jiangxi Branch of National Clinical Research Center for Geriatric Diseases, The First Affiliated Hospital of Gannan Medical University, Ganzhou, Jiangxi, China
Background: Traditional meta-analysis offers only direct comparative evidence. The optimal cognitive training for poststroke cognitive impairment (PSCI) remains largely undetermined.
Objectives: This study aims to assess and compare the effectiveness of selected cognitive training methods for PSCI patients and to identify and rank the most effective intervention programs.
Methods: Searches were conducted in PubMed, Embase, Cochrane Library, Web of science, China National Knowledge Infrastructure, China Science and Technology Journal Database, Wanfang Database, and China Biomedical Database for randomized controlled trials up to September 30, 2023. Two researchers independently performed literature screening, data extraction, and quality assessment. Network meta-analysis was utilized to synthesize the main findings. The primary outcome focused on the intervention’s impact on subjective cognitive function, with secondary outcomes including effects on activities of daily living, motor function, and functional independence. This study is registered with PROSPERO (CRD42023463282).
Results: Fifty eligible randomized controlled trials were identified, revealing eight distinct interventions. These interventions collectively demonstrate efficacy in enhancing cognition. Traditional cognitive training significantly improves overall cognitive function, daily living function, motor function, and functional independence. In Loewenstein Occupational Therapy Cognitive Assessment, Barthel Index, Fugl-Meyer Assessment, and Functional Independence Measure scales, a combination of computer-based and traditional cognitive training outperformed the conventional control group MD = 29.97 (95%CI: 16.3, 44.2), MD = 18.67 (95%CI: 9.78, 27.45), MD = 28.76 (95%CI: 5.46, 51.79) and MD = 42.2 (95%CI: 5.25, 78.99). In the MMSE scale, virtual reality cognitive training combined with traditional training was most effective MD = 8.01 (95%CI: 3.6, 12.4). On the MoCA scale, the combination of exercise and cognitive training showed superior results MD = 6.68 (95%CI: 2.55, 10.78). Only the combined computer-based and traditional cognitive training, as well as traditional cognitive training alone, significantly enhanced functional independence, with no notable differences in other pairwise interventions.
Conclusion: The network meta-analysis suggests that augmenting traditional training with other modalities may enhance overall effectiveness. Specifically, interventions incorporating computer-based cognitive training appear to surpass other methods in improving cognition, daily living function, motor skills, and functional independence. The findings of this network meta-analysis provide evidence-based guidance for clinical decision-making.
Systematic Review Registration: https://www.crd.york.ac.uk/PROSPERO/, identifier in PROSPERO (CRD42023463282).
1 Introduction
According to the 2020 American Heart Association’s report on heart disease and stroke statistics, the prevalence of stroke in the United States in 2016 was 2.5%, representing 7 million Americans over the age of 20 affected by stroke, resulting in nearly 800,000 stroke events and approximately 150,000 deaths (GBD 2019 Diseases and Injuries Collaborators, 2020). With advances in public awareness and the development of emergency medical services, such as stroke centers, the prognosis for stroke patients has improved globally. However, post-stroke cognitive impairment (PSCI) remains prevalent. PSCI typically manifests within 3 to 6 months post-stroke, encompassing not only defects due to the stroke lesion site but also pre-existing impairments. It primarily affects advanced visual-spatial functions, attention, and executive skills (Rost et al., 2022). PSCI is a leading cause of mortality and disability following a stroke (El Husseini et al., 2023), posing significant health and socioeconomic burdens on individuals, families, and communities.
Cognitive impairment encompasses deficits in functions such as memory, language, visual-spatial abilities, executive functions, calculation, and understanding and judgment. When two or more cognitive domains are impaired, affecting daily or social functioning, it may be classified as dementia. Currently, there is no FDA-approved medication for mild cognitive impairment (MCI). The American Academy of Neurology’s updated practice guideline summary does not recommend drugs for MCI; instead, it advocates regular exercise (grade B) and cognitive intervention (grade C) (Petersen et al., 2018). Cholinesterase inhibitors and the glutamic acid N-methyl-D-aspartate receptor antagonist memantine are the primary approved pharmacological treatments for cognitive impairment (Hermann and Zerr, 2022). Given the limitations and safety concerns of drug therapies, non-pharmacological interventions are recommended as the first-line treatment for dementia. Current clinical studies on non-drug interventions include personalized nursing, physical exercise, massage therapy, cognitive training, transcranial direct current stimulation, transcranial magnetic stimulation, acupuncture, and memory therapy (Li et al., 2021). Cognitive training (CT) involves structured task-based exercises aimed at enhancing cognitive processing. Its primary goal is to improve or maintain cognitive abilities. CT encompasses traditional paper-and-pencil methods, computer-based cognitive training (CBCT), virtual reality cognitive training (VRCT), and multi-component training [e.g., exercise-combined cognitive training (EX-CT)). Research indicates that patients with cognitive impairment generally benefit from cognitive training (Bahar-Fuchs et al., 2019; Li et al., 2022; Papaioannou et al., 2022]. The National Institute on Aging in the United States has found that cognitive training and physical exercise can enhance specific cognitive functions in the elderly. The Finland’s Geriatric Intervention Study to Prevent Cognitive Impairment and Disability demonstrated that combined interventions in nutrition, exercise, cognitive training, and social activities effectively improve or maintain cognitive function in older adults (Oh and Rabins, 2019).
Currently, numerous clinical studies worldwide have investigated various cognitive training treatments for PSCI (Pang et al., 2018; Cho and Lee, 2019; Sun et al., 2022). Earlier systematic reviews and meta-analyses have documented the therapeutic impacts of specific cognitive training methods on PSCI patients. For instance, a systematic review and meta-analysis by Xinming Chen et al. concluded that VRCT significantly enhances cognitive rehabilitation and daily living function recovery in PSCI patients, based on 21 randomized controlled trials (Chen et al., 2022). Another meta-analysis by Ruifeng Sun et al. demonstrated that combined cognitive training and exercise interventions markedly improve executive function and working memory in PSCI patients (Sun et al., 2021). Although previous meta-analyses suggest that CBCT might be more beneficial for cognition than VRCT (Xiao et al., 2022), these findings remain tentative. The most effective cognitive training type for enhancing cognitive abilities and quality of life in PSCI patients is still undetermined, as no study has concurrently compared multiple cognitive training models. Consequently, healthcare professionals face challenges in prescribing the most effective cognitive interventions for their patients. Network meta-analysis, which integrates both direct and indirect evidence to simultaneously compare the efficacy of multiple interventions, can rank these interventions based on different outcomes, thus providing evidence-based data to support medical decision-making. Therefore, the primary goal of this study is to compare and rank the effects of various cognitive training methods on the cognition and quality of life of PSCI patients by synthesizing existing evidence through network meta-analysis.
2 Methods
This study adhered to the Preferred Reporting Items for Systematic Reviews and Meta-analysis (PRISMA) guidelines (Page et al., 2021) and the Cochrane Handbook for Systematic Reviews of Interventions. The network meta-analysis was pre-registered in PROSPERO (CRD42023463282).
2.1 Data sources and retrieval
The systematic literature search was conducted using PubMed, Embase, Cochrane Library, Web of science, China National Knowledge Infrastructure, China Science and Technology Journal Database, Wanfang Database, and the Chinese Biomedical Database, covering the period from each database’s inception to September 30,2023. Additionally, a secondary search was conducted through the references of pertinent studies and reviews. The detailed search strategies are outlined in Supplementary Appendix 1.
2.2 Inclusion criteria
The inclusion criteria were formulated based on the PICOS (population, interventions, controls, outcomes, study design) framework.
2.2.1. Population
The study population comprised stroke patients with cognitive impairment who met established diagnostic criteria. Inclusion criteria included: ① Age ≥ 18 years. ② No significant cognitive dysfunction prior to stroke. ③ Ability to participate in neuropsychological assessments and various cognitive training programs.
2.2.2 Intervention methods
Interventions examined in this study encompass traditional cognitive training, CBCT, VRCT, and multi-component cognitive training (including EX-CT), and multi-component cognitive training (including exercise combined with cognitive training). These interventions were applied either individually or in combination.
2.2.3 Control
Control groups in this study included participants receiving only exercise, traditional rehabilitation therapy, or alternate forms of cognitive training.
2.2.4 Results
The primary outcomes focused on cognitive function, encompassing overall cognitive function, visual-spatial and executive functions, naming, language abilities, memory, attention, abstract thinking, delayed recall, and orientation. Cognitive function was assessed using scales such as the Montreal cognitive assessment (MoCA), mini-mental state ex-amination (MMSE), and Loewenstein Occupational Therapy Cognitive Assessment (LOTCA).
Secondary outcomes included improvements in daily living abilities, motor function, and functional independence. The Modified Barthel Index (MBI) or Barthel Index (BI) assessed activities of daily living, the Fugl-Meyer Assessment (FMA) evaluated motor function, and the Functional Independence Measure (FIM) scale was used for functional independence assessment.
2.2.5 Research design
The study design was restricted to randomized controlled trial.
2.3 Data selection and extraction
All retrieved literature was imported into EndNote X9. Duplicates were eliminated, and studies were preliminarily selected based on titles and abstracts. Subsequently, it is necessary to download and read the full texts to include primary studies that meet the research criteria. Before data extraction, an electronic information extraction form tailored to this project’s standards is used for evaluation. Two researchers (a and b) independently extracted data from the included studies, covering characteristics such as the first author’s name, year of publication, research location (country), citation, study type, participant characteristics, experimental and control group interventions, intervention duration, frequency, participant age, gender, and outcome measures. Discrepancies in literature screening and data extraction were resolved through discussion between the two researchers.
2.4 Literature quality evaluation
Two researchers independently assessed the risk of bias in all included studies using the Rob2.0 tool, as recommended in the Cochrane Handbook (Sterne et al., 2019). The assessment encompassed randomization methods, blinding, allocation concealment, intervention allocation, compliance, missing outcome data, outcome measurement, and selective outcome reporting. Each category was classified as low risk, unclear, or high risk. Any disagreements were discussed and resolved by the two researchers.
2.5 Statistical analysis
2.5.1 Analysis methods
For network meta-analysis, RStudio 4.3.1 software with the gemtc package (version 1.0-1) and JAGS software were used, employing the Markov Chain Monte Carlo method within a Bayesian framework. Four Markov chains were implemented for simulation analysis, with an initial value of 2.5, a refinement iteration step of 1, 5000 pre-simulation iterations for annealing, and 20,000 iterations to achieve model convergence. The deviation information standard (DIC) was utilized to compare model fit and overall consistency (a DIC difference of less than five between consistency and inconsistency models indicated the use of a consistency model). In the presence of closed loops, the node-splitting method was applied to assess local consistency.
All dichotomous outcomes were expressed as risk ratio (RR), with the corresponding 95 % CI reported. A statistically significant difference was considered when the value (GBD 2019 Diseases and Injuries Collaborators, 2020) was not included in the 95% CI. The random effects model within a Bayesian framework was employed to simultaneously analyze the efficacy of all treatment regimens. The analysis results comprised network diagrams for each outcome index, cumulative probability ranking diagrams, league tables, and ‘correction-comparison’ funnel plots. The surface under the cumulative ranking curve (SUCRA) served as an index for cumulative ranking probability, ranking the interventions’ advantages and disadvantages based on the SUCRA value. The closer the value to 100%, the more effective the intervention was deemed. Pairwise and Network Meta-Analysis were conducted using Stata 15.1 and R software (VER.4.3.1).
3 Results
3.1 Baseline characteristics and quality of included studies
Initially, 4440 studies were screened. After removing duplicates, 3758 articles were reviewed, with 3484 excluded based on title and abstract. Consequently, 283 articles were assessed in full text, adhering strictly to the inclusion and exclusion criteria. Ultimately, 47 studies met the inclusion criteria. Additional searches in the references of published systematic reviews identified three more eligible studies, resulting in 50 randomized controlled trials included (Chen et al., 2006, 2019, 2020; Liu et al., 2006, 2019, 2022; Ou et al., 2007; Shin et al., 2008; Yang and Liu, 2008; Wang et al., 2009, 2013; Li and Gan, 2010; Kim et al., 2011; Zhang et al., 2012, 2018, 2020; Feng et al., 2014; Ye et al., 2014; Zucchella et al., 2014; Xu et al., 2015; Yoo et al., 2015; Li M. et al., 2016; Li X. et al., 2016; Cao et al., 2017; Gong, 2017; Jiang et al., 2017; Li et al., 2017; Lv et al., 2017; Maier et al., 2017; De Luca et al., 2018; Prokopenko et al., 2018, 2019; Xiao, 2018; Mao et al., 2019; Wang, 2019; Xiao and Liang, 2019; Yeh et al., 2019, 2022; Faria et al., 2020; Fu et al., 2020; Jung et al., 2020; Shao, 2021; Xuefang et al., 2021; Ho et al., 2022; Liao et al., 2022; Shang et al., 2022; Sun et al., 2022; Xia et al., 2022; Zhao et al., 2020, 2022). The specific screening process is depicted in Figure 1. These studies encompassed 3063 participants, with an average sample size of 61 subjects (range 11–149) and an age range of 42–74 years. Of these, 1274 (41.6%) were female and 1789 (58.4%) male. The geographic distribution of the 50 trials included 74% from China, 6% from Taiwan, 6% from South Korea, 4% each from Italy and Russia, and 2% each from the United States, Portugal, and Spain. Attempts to collect racial data were largely unsuccessful due to infrequent reporting. Eleven different cognitive interventions were administered, including: (1) VRCT (n = 2). (2) VRCT combined with traditional cognitive training (n = 6). (3) VRCT combined with CBCT (n = 1). (4) CBCT (n = 17). (5) CBCT combined with traditional cognitive training (n = 9). (6) Cognitive training (n = 42). (7) Exercise combined with CBCT (n = 2). (8) Exercise combined with traditional cognitive training (n = 1). The baseline characteristics of the subjects are shown in Table 1.
3.2 Quality evaluation of research methods
This study included 50 papers. While most studies detailed their randomization process, 13 did not specify the method of random sequence generation. Only one study (Sun et al., 2022), described allocation concealment, leading to the classification of the others as having an ‘unknown risk’ of bias in this regard. Due to the nature of the interventions, blinding of participants was not feasible. A total 13 studies described blinding methods, while the remaining were assessed as having an unknown risk. Concerning baseline data consistency, all studies reported comparable baseline data with no statistical differences, thus receiving a ‘low risk’ rating. In terms of outcome data completeness, most studies accounted for all participants at the time of outcome reporting, aligning with the number initially included and were thus rated as ‘low risk’. However, seven studies reported data loss or dropout rates exceeding 5% of the original sample size, which was assessed as ‘unknown risk’. Three studies were evaluated as ‘unknown risk’ due to the inaccessibility of their original statistical methods. The detailed methodological quality assessment is provided in a table, and the risk bias assessment is illustrated in Figure 2.
3.3 Results analysis
In total, 50 randomized controlled trials involving 3063 participants were included to assess the impact of various cognitive training methods on cognitive function in patients with PSCI. The network structure diagram, representing various interventions across different outcome indices, is presented in Figure 3. The line thickness in the diagram correlates with the number of pairwise intervention comparisons - the thicker the line, the greater the number of comparisons. Circle size corresponds to the sample size involved in each intervention. The study analyzed both the consistency and inconsistency of data across each outcome index. A difference of less than 5 in the DIC results between the consistency and inconsistency models suggested data consistency for each outcome. Closed loops were observed in the MoCA, MMSE, LOTCA, BI, and FIM scales network diagrams. The node-splitting method was employed for local inconsistency tests on these closed-loop outcomes, yielding P-values all above 0.05, indicating no significant local inconsistency among interventions forming these loops. Publication bias for the included studies was assessed using comparison-correction funnel plots for all outcomes, which demonstrated basic symmetry and a roughly symmetric distribution of studies on both sides of the central line, suggesting a low risk of publication bias (Figure 4).
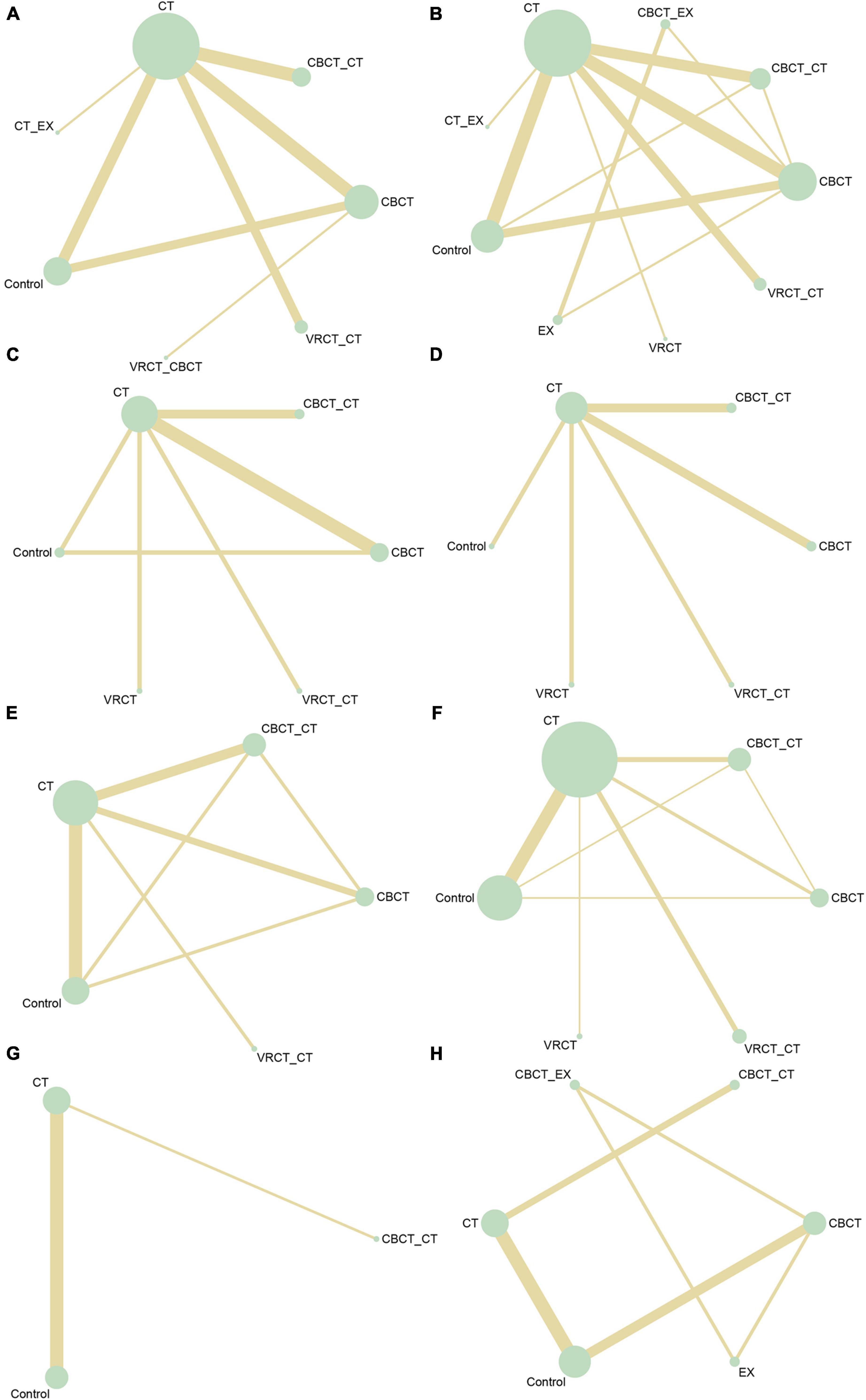
Figure 3. Network intervention comparison network diagram. (A) MMSE. (B) MoCA. (C) Visuo-executive, attention, abstraction, memory, and orientation function in MoCA. (D) Naming and language function in MoCA. (E) LOTCA. (F) BI. (G) FMA. (H) FIM.
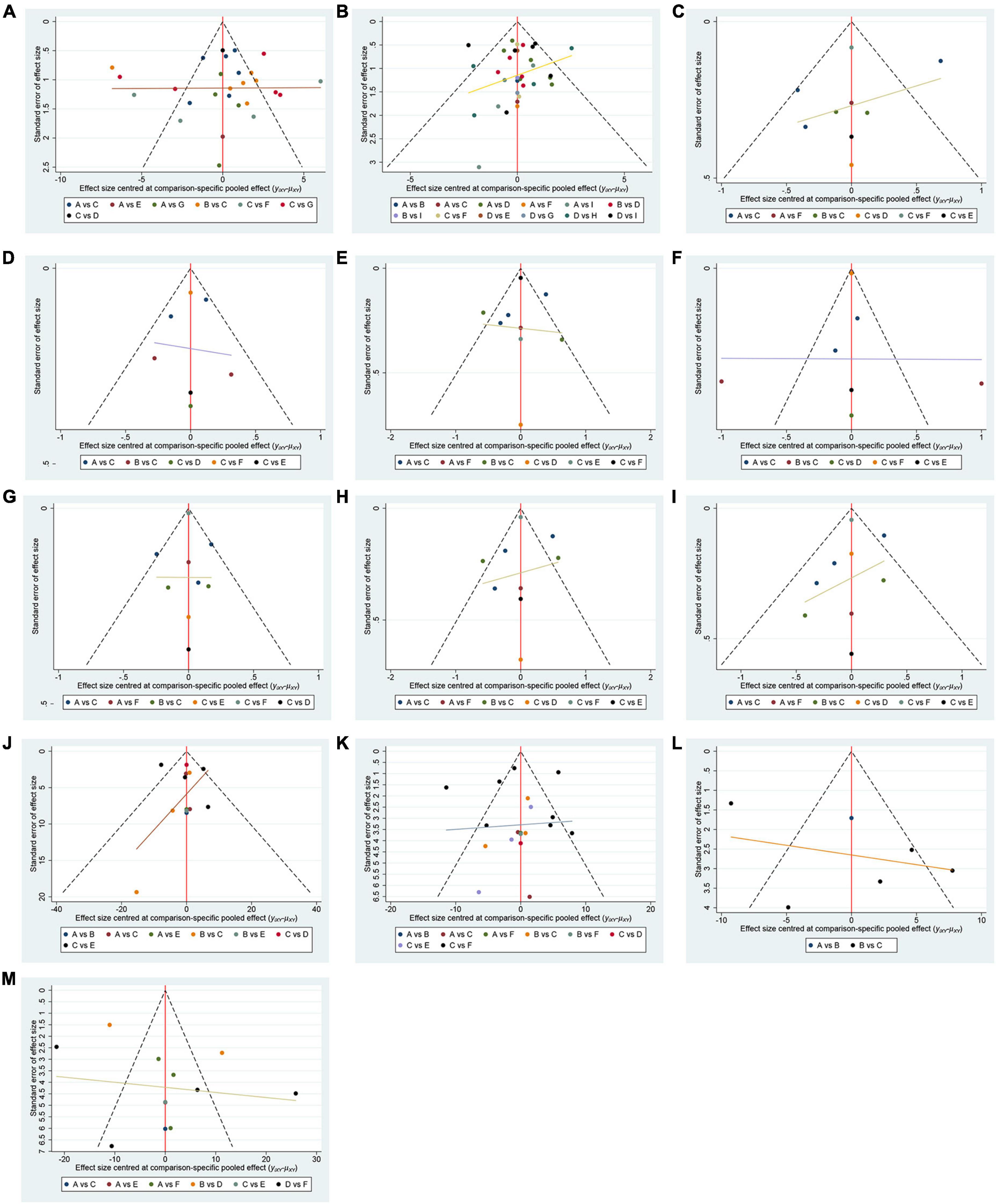
Figure 4. Funnel plot. (A) MMSE; A(reference): CBCT; B:CBCT_CT; C:CT; D:CT_EX; E:VRCT_CBCT; F:VRCT_CT; G:Control. (B) MoCA; A(reference):CBCT; B:CBCT_CT; C:CBCT_EX; D:CT; E:CT_EX; F:EX; G:VRCT; H:VRCT_CT; I:Control. (C) visuo-executive function in MoCA; A (reference):CBCT; B:CBCT_CT; C:CT; D:Control; E:VRCT; F:VRCT_CT. (D) naming function in MoCA; A (reference):CBCT; B:CBCT_CT; C:CT; D:Control; E:VRCT; F:VRCT_CT. (E) attention function in MoCA; A (reference):CBCT; B:CBCT_CT; C:CT; D:Control; E:VRCT; F:VRCT_CT. (F) language function in MoCA; A (reference):CBCT; B:CBCT_CT; C:CT; D:Control; E:VRCT; F:VRCT_CT. (G) abstraction function in MoCA; A (reference):CBCT; B:CBCT_CT; C:CT; D:Control; E:VRCT; F:VRCT_CT. (H) memory function in MoCA; A (reference):CBCT; B:CBCT_CT; C:CT; D:Control; E:VRCT; F:VRCT_CT. (I) orientation function in MoCA;; A (reference):CBCT; B:CBCT_CT; C:CT; D:Control; E:VRCT; F:VRCT_CT. (J) LOTCA; A (reference):CBCT; B:CBCT_CT; C:CT; D:VRCT_CT; E:Control. (K) BI; A(reference):CBCT; B:CBCT_CT; C:CT; D:VRCT; E:VRCT_CT; F:Control. (L) FMA; A (reference):CBCT_CT; B:CT; C:Control. (M) FIM; A(reference):CBCT; B:CBCT_CT; C:CBCT_EX; D:CT; E:EX; F:Control.
3.3.1 Overall cognitive function
3.3.1.1 MMSE scale
The MMSE scale was the focus of 27 studies, encompassing 1497 participants. The network intervention comparison network diagram is depicted in Figure 3A. Four studies assessed the impact of CBCT (Shin et al., 2008; Ye et al., 2014; Prokopenko et al., 2018, 2019), five evaluated traditional cognitive training (Li and Gan, 2010; Zhang et al., 2012; Zucchella et al., 2014; Gong, 2017; Zhao et al., 2020), and 18 directly compared different cognitive training methods (Kim et al., 2011; Wang et al., 2013; Zucchella et al., 2014; Li M. et al., 2016; Cao et al., 2017; Jiang et al., 2017; Lv et al., 2017; Maier et al., 2017; De Luca et al., 2018; Xiao and Liang, 2019; Fu et al., 2020; Jung et al., 2020; Zhang et al., 2020; Zhao et al., 2020; Shao, 2021; Xuefang et al., 2021; Ho et al., 2022; Liao et al., 2022) MMSE score outcomes revealed that the groups receiving CBCT (MD = 4.3, 95% CI:1.44, 7.16, P < 0.05), computer-based combined with traditional cognitive training group (MD = 7.86, 95% CI: 3.85, 11.87, P < 0.05), VRCT combined with traditional cognitive training (MD = 8.01, 95% CI: 3.4, 12.6, P < 0.05), and traditional cognitive training (MD = 3.59, 95% CI: 0.9, 6.26, P < 0.05) alone all outperformed the non-cognitive training control group. Additionally, the group with combined computer-based and traditional cognitive training showed greater improvements than the group with traditional cognitive training (MD = 4.28, 95% CI: 1.3, 7.24, P < 0.05), as did the group combining VRCT and traditional cognitive training when compared to the traditional training group alone (MD = 4.43, 95% CI: 0.68, 8.16, P < 0.05), with these differences being statistically significant. No significant differences were observed in other pairwise comparisons (Figure 5A). Cumulative probability results indicated that the top three interventions for improving MMSE scores were likely VRCT combined with traditional cognitive training (SUCRAs: 85.63%), CBCT combined with traditional cognitive training (SUCRAs: 85.18%), and exercise combined with traditional cognitive training (SUCRAs: 55.12%) (Figure 6A).
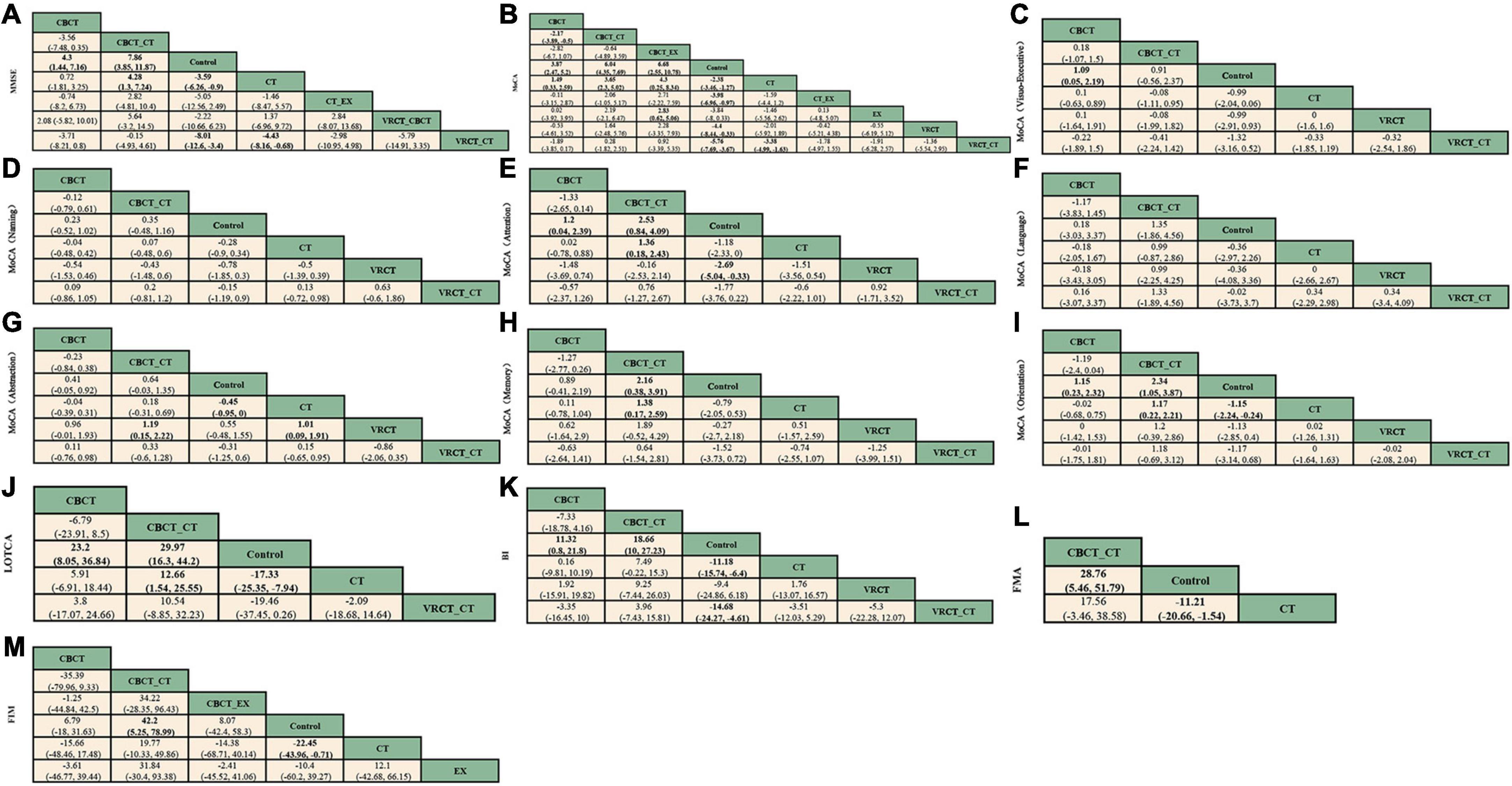
Figure 5. League table. (A) MMSE. (B) MoCA. (C) visuo-executive function in MoCA. (D) naming function in MoCA. (E) attention function in MoCA. (F) language function in MoCA. (G) abstraction function in MoCA. (H) memory function in MoCA. (I) orientation function in MoCA. (J) LOTCA. (K) BI. (L) FMA. (M) FIM.
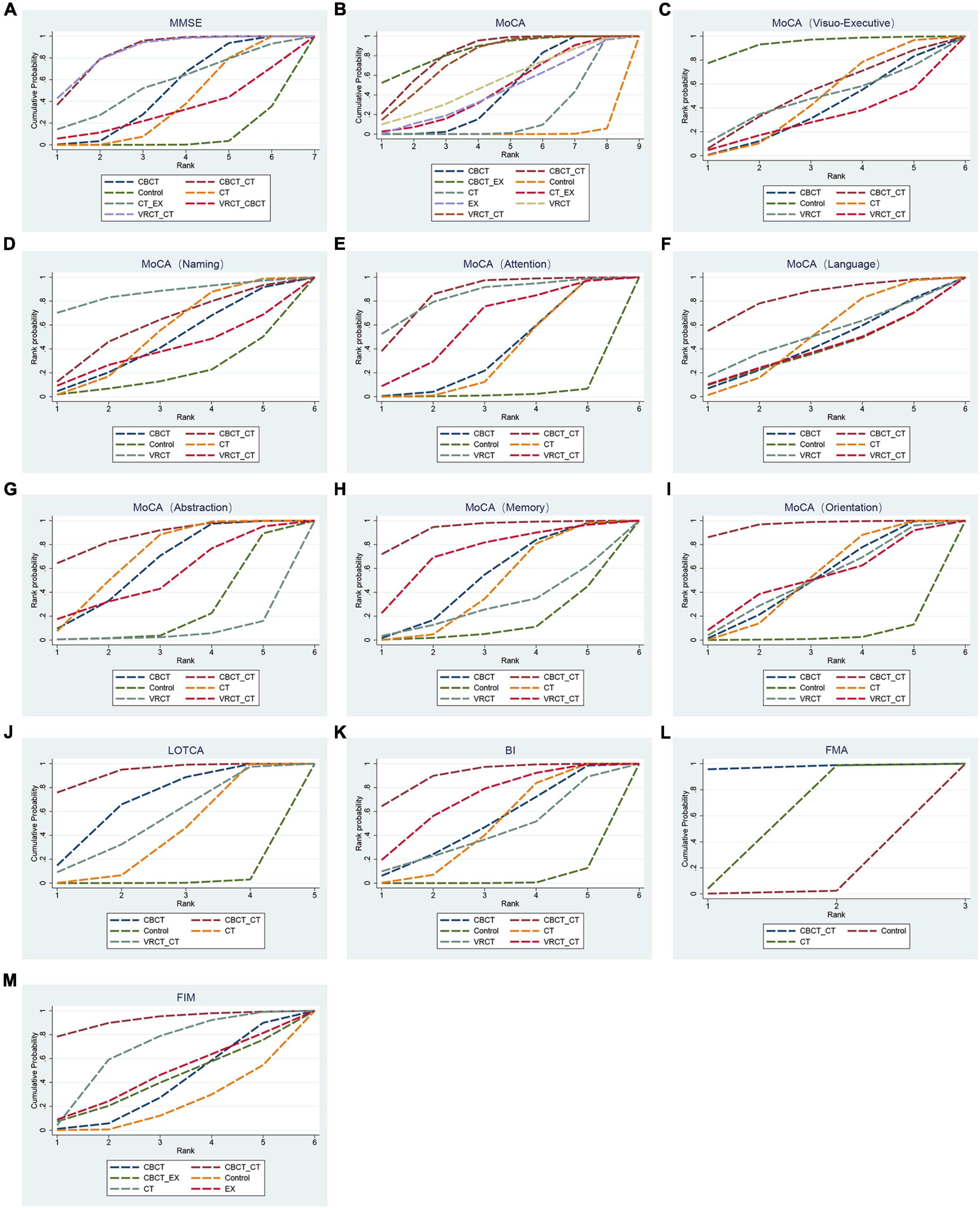
Figure 6. Cumulative sorting chart. (A) MMSE. (B) MoCA. (C) visuo-executive function in MoCA. (D) naming function in MoCA. (E) attention function in MoCA. (F) language function in MoCA. (G) abstraction function in MoCA. (H) memory function in MoCA. (I) orientation function in MoCA. (J) LOTCA. (K) BI. (L) FMA. (M) FIM.
3.3.1.2 MoCA scale
In total, 27 randomized controlled trials involving 1680 participants analyzed the MoCA scores of PSCI patients pre- and post-intervention. The intervention comparison network diagram is shown in Figure 3B. Four studies investigated the impact of CBCT (Ye et al., 2014; Prokopenko et al., 2018, 2019; Wang, 2019), five focused on traditional cognitive training (Li and Gan, 2010; Feng et al., 2014; Li X. et al., 2016; Gong, 2017; Zhang et al., 2018), and 18 compared different cognitive training methods (Cao et al., 2017; Xiao, 2018; Chen et al., 2019; Liu et al., 2019, 2022; Mao et al., 2019; Wang, 2019; Xiao and Liang, 2019; Yeh et al., 2019, 2022; Faria et al., 2020; Shao, 2021; Xuefang et al., 2021; Ho et al., 2022; Liao et al., 2022; Shang et al., 2022; Sun et al., 2022; Zhao et al., 2022). Of these, 26 studies used a total score system for assessment, while one study scored only certain aspects of the scale. MOCA score outcomes indicated that groups receiving CBCT combined with traditional cognitive training (MD = 6.04, 95% CI: 4.35, 7.69, P < 0.05), CBCT alone (MD = 3.87, 95% CI:2.47, 5.2, P < 0.05), exercise combined with CBCT (MD = 6.68, 95% CI:2.55, 10.78, P < 0.05), VRCT combined with traditional cognitive training (MD = 5.76, 95% CI:3.67, 7.69, P < 0.05), VRCT (MD = 4.44, 95% CI:0.33, 8.44, P < 0.05), traditional cognitive training combined with exercise (MD = 3.98, 95% CI:0.97, 6.96, P < 0.05), and traditional cognitive training alone were all superior to the control group (MD = 2.38, 95% CI: 1.27, 3.46, P < 0.05). Moreover, the group with combined computer-based and traditional cognitive training outperformed the traditional cognitive training group (MD = 3.65, 95% CI: 2.3, 5.02, P < 0.05) and CBCT group (MD = 2.17, 95% CI: 0.5, 3.89, P < 0.05) alone. The computer-based (MD = 1.49, 95% CI: 0.33, 2.59, P < 0.05), exercise combined with computer-based (MD = 4.3, 95% CI: 0.25, 8.34, P < 0.05), and VRCT combined with traditional cognitive training groups (MD = 3.38, 95% CI: 1.63, 4.99, P < 0.05) all showed superior results compared to the traditional cognitive training group, and the group combining exercise with CBCT (MD = 2.83, 95% CI:0.62, 5.06, P < 0.05) also outperformed the exercise group alone, with these differences being statistically significant. No significant differences were found in other pairwise comparisons (Figure 5B). The ranking of interventions is provided in Figure 4. Cumulative probability results identified the top three interventions as exercise combined with traditional cognitive training (SUCRAs: 85.40%), CBCT combined with traditional cognitive training (SUCRAs: 81.54%), and VRCT combined with traditional cognitive training (SUCRAs: 76.30%) (Figure 6B).
Nine studies reported therapeutic effects across seven distinct cognitive domains (Xiao, 2018; Zhang et al., 2018; Liu et al., 2019; Xiao and Liang, 2019; Faria et al., 2020; Shao, 2021; Shang et al., 2022; Xia et al., 2022; Zhao et al., 2022). In visual-spatial and executive function, the intervention comparison network diagram is shown in Figure 3C. The CBCT group (MD = 1.09, 95% CI:0.05, 2.19, P < 0.05) outperformed the conventional control group, with a statistically significant difference (Figure 5C). The cumulative sorting chart is shown in Figure 6C. Regarding naming and language function, the intervention comparison network diagram is shown in Figure 3D. No intervention demonstrated a significant impact on the recovery of these functions in PSCI patients (Figures 5D, E). The cumulative sorting chart is shown in Figures 6D, E.
For attention abilities, the intervention comparison network diagram is shown in Figure 3C. The groups receiving CBCT combined with traditional cognitive training (MD = 2.53, 95% CI:0.84, 4.09, P < 0.05), CBCT alone (MD = 1.2, 95% CI:0.04, 2.39, P < 0.05), and VRCT (MD = 2.69, 95% CI:0.33, 5.04, P < 0.05) all showed superior results compared to the conventional control group, with these differences being statistically significant (Figure 5F). Cumulative probability results suggest that CBCT combined with traditional cognitive training (SUCRAs: 84.17%) and VRCT (SUCRAs: 83.46%) might be the most effective interventions for improving attention abilities (Figure 6F).
In terms of abstract function, the intervention comparison network diagram is shown in Figure 3C. The traditional cognitive training group (MD = 0.45, 95% CI:0,0.95, P < 0.05) exceeded the conventional control group’s performance, and both the CBCT combined with traditional cognitive training (MD = 1.19, 95% CI:0.15, 2.22, P < 0.05) and the cognitive training groups (MD = 1.01, 95% CI:0.09, 1.91, P < 0.05) outperformed the VRCT group (Figure 5G). The cumulative sorting chart is shown in Figure 6G.
For delayed recall, the intervention comparison network diagram is shown in Figure 3C. The group combining computer-based and traditional cognitive training showed better results than both the conventional control (MD = 2.16, 95% CI:0.38, 3.91, P < 0.05) and traditional cognitive training groups (MD = 1.38, 95% CI:0.17, 2.59, P < 0.05) (Figure 5H). The cumulative sorting chart is shown in Figure 6H. In orientation function, the intervention comparison network diagram is shown in Figure 3C. The groups receiving CBCT combined with traditional cognitive training (MD = 2.34, 95% CI:1.05, 3.87, P < 0.05), CBCT alone (MD = 1.15, 95% CI:0.23, 2.32, P < 0.05), and traditional cognitive training (MD = 1.15, 95% CI:0.24, 2.24, P < 0.05) all surpassed the conventional control group. Additionally, the combination of computer-based and traditional cognitive training was more effective than traditional cognitive training alone (MD = 1.17, 95% CI:0.22, 2.21, P < 0.05), with a statistically significant difference (Figure 5I). The cumulative sorting chart is shown in Figure 6I.
In conclusion, based on cumulative probability outcomes, CBCT combined with traditional cognitive training is the leading intervention for enhancing attention, abstract function, memory, and orientation, as measured by the MoCA Scale.
3.3.1.3 LOTCA scale
Eight studies, encompassing 476 participants, were included for analysis. The intervention comparison network diagram is displayed in Figure 3E. One study assessed the impact of CBCT (Wang, 2019), three studies evaluated traditional cognitive training (Chen et al., 2006, 2020; Wang et al., 2009), and four studies directly compared various cognitive training methods (Wang et al., 2013; Li M. et al., 2016; Wang, 2019; Xuefang et al., 2021). LOTCA score outcomes indicated that the groups receiving CBCT combined with traditional cognitive training (MD = 29.97, 95% CI:16.3, 44.2, P < 0.05), CBCT alone (MD = 23.2, 95% CI:8.05, 36.84, P < 0.05), and traditional cognitive training (MD = 17.33, 95% CI:7.94, 25.35, P < 0.05) all surpassed the control group, with these differences being statistically significant (Figure 5J). Cumulative probability results identified CBCT combined with traditional cognitive training (SUCRAs: 92.64%), CBCT (SUCRAs: 67.16%), and VRCT combined with traditional cognitive training (SUCRAs: 51.00%) as the top three interventions (Figure 6J). The combination of computer-based and traditional cognitive training demonstrated the most substantial impact on LOTCA scale scores.
3.3.1.4 Neurocognitive and neuropsychological assessment
Neurocognitive and neuropsychological testing serve as crucial instruments for evaluating cognitive domains, encompassing a broad spectrum of cognitive functions such as, but not limited to, perception and visuospatial function, motor control, attention, memory, executive function, language, and intellectual quotient (Zucchella et al., 2018). In studies that employed Digit span (forward and backward) tests to detect patients’ short-term auditory memory and working memory capacity, six out of eight studies revealed that Digit span tests demonstrated certain clinical amelioration subsequent to the administration of these cognitive training (Zucchella et al., 2014; Yoo et al., 2015; Xiao, 2018; Jung et al., 2020; Ho et al., 2022; Liu et al., 2022). Moreover, Digit span backward test often showed statistical significance more often than Digit span forward test. Two studies demonstrated no significant enhancement (Kim et al., 2011; Faria et al., 2020). In assessments employing the Trail-making test (parts A and B) to investigate attention processes in individuals with PSCI, outcomes from five of the eight studies indicated that there was also a clinical improvement in attention following these cognitive training interventions, particularly in the Trail-making test parts A outcome (Zucchella et al., 2014; Maier et al., 2017; Xiao, 2018; Faria et al., 2020; Liu et al., 2022). Three studies did not demonstrate significant improvement following these cognitive interventions (Kim et al., 2011; Yoo et al., 2015; De Luca et al., 2018). Additionally, one study discovered that in the improvement phase Digit span tests, the combination of VRCT with traditional cognitive training demonstrated superior clinical efficacy compared to the traditional cognitive training group alone, and this difference was statistically significant (Jung et al., 2020). In another study, the traditional cognitive training group exhibited advantages over traditional rehabilitation therapy in both the Digit span tests and Trail-making test assessments (Zucchella et al., 2014).
3.3.2 Function of daily living
Fifteen studies, involving 937 patients, reported on the effects of various interventions on the BI scores of PSCI patients. The intervention comparison network diagram is illustrated in Figure 3F. One study investigated the impact of CBCT (Wang, 2019), seven studies focused on traditional cognitive training (Liu et al., 2006; Ou et al., 2007; Wang et al., 2009; Zhang et al., 2012, 2018; Feng et al., 2014; Zhao et al., 2020), and eight studies directly compared different cognitive training methods (Maier et al., 2017; Xiao, 2018; Chen et al., 2019; Liu et al., 2019, 2022; Mao et al., 2019; Wang, 2019; Xiao and Liang, 2019). BI index results showed that the groups receiving CBCT combined with traditional cognitive training (MD = 18.66, 95% CI:10, 27.23, P < 0.05), VRCT combined with traditional cognitive training (MD = 14.68, 95% CI:4.61, 24.27, P < 0.05), CBCT alone (MD = 11.32, 95% CI:0.8, 21.8, P < 0.05), and traditional cognitive training (MD = 11.18, 95% CI:6.4, 15.74, P < 0.05) all achieved higher scores than the control group, with these differences being statistically significant. No significant differences were found in other pairwise comparisons (Figure 5K). Cumulative probability results revealed that CBCT combined with traditional cognitive training (SUCRAs: 90.19%), VRCT combined with traditional cognitive training (SUCRAs: 69.36%), and CBCT (SUCRAs: 49.59%) were the top-ranked interventions (Figure 6K), suggesting their greater effectiveness in improving the daily living functions of PSCI patients.
3.3.3 Motor function
Six studies, using FMA score to assess the effects of motor function, totaling 426 participants, were included for analysis. The intervention comparison network diagram is presented in Figure 3G. Five studies evaluated the impact of traditional cognitive training (Chen et al., 2006, 2020; Ou et al., 2007; Wang et al., 2009; Li and Gan, 2010), and one study directly compared CBCT combined with traditional cognitive training to traditional cognitive training alone (Lv et al., 2017). FMA score results indicated that both the group receiving CBCT combined with traditional cognitive training (MD = 28.76, 95% CI:5.46, 51.79, P < 0.05) and the traditional cognitive training group (MD = 11.21, 95% CI:1.54, 20.66, P < 0.05) outperformed the control group, with statistically significant differences (Figure 5L). The combined computer-based and traditional cognitive training approach ranked as the most effective intervention (Figure 6L), demonstrating a pronounced effect on improving motor function in patients with PSCI.
3.3.4 Functional independence
Ten studies, using FIM score to assess the effects of functional independence, encompassing 560 patients, were included in this segment. The intervention comparison network diagram is depicted in Figure 3H. Three studies assessed the impact of CBCT (Shin et al., 2008; Ye et al., 2014; Yoo et al., 2015), four studies focused on traditional cognitive training (Chen et al., 2006, 2020; Liu et al., 2006; Yang and Liu, 2008; Zucchella et al., 2014), and three studies directly compared different cognitive training methods (Lv et al., 2017; Liu et al., 2019; Yeh et al., 2022). The results showed that both the group receiving CBCT combined with traditional cognitive training (MD = 42.2, 95% CI:5.25, 78.99, P < 0.05) and the traditional cognitive training group (MD = 22.45, 95% CI:0.71, 43.96, P < 0.05) significantly outperformed the conventional control group in enhancing functional independence. There were no notable differences in other pairwise interventions (Figure 5M), with the combination of computer-based and traditional cognitive training (SUCRAs: 92.16%) proving to be the most effective (Figure 6M).
4 Discussion
4.1 Explanation of the results and comparison with previous studies
To our knowledge, this study represents the first network meta-analysis comparing various cognitive training treatments for PSCI patients. It encompassed 50 randomized controlled trials involving 3017 subjects, analyzing nine cognitive interventions. Our study found that cognitive training can positively affect cognitive function, daily living function, functional independence and motor function in PSCI patients. The combination of CBCT and traditional cognitive training demonstrated the most significant impact on overall cognitive function improvement as assessed by the MMSE and LOTCA scales, ranking second only to the exercise combined CBCT group as assessed by the MoCA scale. The combined CBCT and traditional cognitive training group showed significant improvements in attention, abstract ability, memory, and orientation compared to other training groups. Furthermore, it ranked highest in terms of impact on daily living function as measured by the BI index, FMA-based daily living function, and FMI-based functional independence. This study primarily revolved around these simplified cognitive screening instruments and life function scales, which facilitate rapid and straightforward assessments of patients’ cognitive functions. Although the obtained results might be approximate, such screening tests are adequate when scores are low and the patient’s medical history strongly indicates dementia, along with staging and monitoring of cognitive impairment. Of course, within the literature we examined, several studies utilizing a variety of neurocognitive and neuropsychological measures have been conducted to uncover the potential benefits of cognitive training on various cognitive dimensions in patients suffering from PSCI. However, due to the heterogeneity of neuropsychological tests employed across studies, the limited recurrence of the same test, and the subtle variances in the focus of interventions and anticipated outcomes, we did not extract the results of specific tests individually and perform meta-analyses. Instead, we adopted a more macro and inclusive methodological strategy, aiming to capture the overall trend of cognitive training enhancing cognitive function in PSCI patients as a whole. By reviewing these articles, we discovered that the majority of studies employed the Digit Span (forward and backward) tests to assess patients’ short-term auditory memory and working memory capacity, as well as the Trail-Making Test (parts A and B) to evaluate the attention process of PSCI patients. Following the administration of these cognitive interventions, there was an improvement in clinical efficacy, but the statistically significant difference between groups was rare. Additionally, our study revealed that CBCT in conjunction with traditional cognitive training yielded a more significant impact on enhancing attention and memory compared to traditional cognitive training or rehabilitation alone. This finding contrasts slightly with the results of De Luca et al. (2018) and Xiao (2018) studies, which did not demonstrate a distinct advantage for the CBCT combined with traditional cognitive training group. This might be associated with the scale employed. The assessment of memory and attention using the MoCA scale is frequently simplistic and approximate, which can easily exaggerate or overlook the cognitive function scores of patients, leading to conspicuous data disparities. Conversely, neuropsychological tests are typically more meticulous and intricate, enabling the assignment of more precise scores and minimizing potential errors.
The inaugural ‘Adult Stroke Rehabilitation Guideline’ issued jointly by the American Heart Association (AHA) and the American Stroke Association (ASA) in 2016 recommended cognitive function rehabilitation training for post-stroke patients at a Level IA (Winstein et al., 2016). Jeffrey M. Rogers et al.’s systematic review highlighted that cognitive training effectively enhances cognitive function in PSCI patients. Our study’s findings align with these perspectives. In the 50 randomized controlled trials employing various cognitive training interventions for PSCI patients, we observed that both traditional cognitive training and CBCT combined with traditional cognitive training improved all study outcome indicators. These interventions notably enhanced overall cognitive function and daily living abilities. Furthermore, compared to traditional cognitive training alone, combined interventions often yielded greater cognitive benefits and effectiveness. Specifically, CBCT combined with traditional cognitive training emerged as the most effective in enhancing attention, abstract function, memory, orientation, daily living function, motor function, and functional independence. Our analysis revealed consistent results between the MMSE and MoCA scales. The top three interventions were identified as a combination of cognitive training and CBCT with traditional cognitive training, VRCT combined with traditional cognitive training, and exercise combined with CBCT. It suggests that these three combined interventions have advantages in improving the overall cognitive function of PSCI patients.
CBCT offers personalized and adaptive content tailored to the patient’s specific cognitive impairments, adjusting in real-time to the feedback received during training sessions. This adaptability ensures that tasks are neither too simple nor too complex, thereby enhancing patient engagement and concentration in the training process (Chuang et al., 2023; Maggio et al., 2023). The study observed that older adults demonstrate greater enthusiasm and compliance for CBCT compared to general cognitive training. Effective cognitive training requires participants to fully commit to the regimen to achieve an adequate ‘training dose’ and thereby reap the benefits (Turunen et al., 2019), which supports the finding that CBCT tends to result in better outcomes. The research indicates that computer games used in this training not only enhance visual-spatial abilities but also improve attention. This is achieved by training the spatial and temporal resolution of attention in these games, thus enhancing attention control and task focus (Bavelier and Green, 2019). Research by Richard E. Mayer suggests that games focusing on a single cognitive skill can enhance cognitive functions such as memory, attention, and spatial cognition. This enhancement requires participants to repeatedly practice the skill in varied environments and with progressively increasing challenges (Mayer, 2019). Cognitive training operates on repeated practice in specific cognitive domains, using tasks involving particular skills to achieve training objectives. Cognitive training is founded on the repetition of specific cognitive domain training, and the intended outcome is realized through the execution of tasks that necessitate specific skills. This renders CBCT, a training approach that incorporates computer games, more effective in enhancing the attention, memory, and visuospatial abilities of PSCI patients. This is in line with the fact that CBCT, alone or in conjunction with traditional cognitive training, can enhance visuospatial and executive function, attention, memory, and orientation. However, despite their adaptability, these technological tools cannot entirely replace traditional cognitive training, especially for more severe and subacute cases, where the role of neuropsychiatrists and speech therapists is crucial. In fact, combining computer-based with traditional cognitive training often results in optimal outcomes (Maggio et al., 2023). A meta-analysis of 32 studies involving 1837 subjects corroborates this study’s findings, revealing that combining computer-based and traditional cognitive training significantly enhances overall cognitive function and daily life function more than conventional cognitive training alone (Nie et al., 2022). In summary, CBCT synergistic intervention demonstrates effectiveness in enhancing cognitive function and mitigating neurological deficits in patients with PSCI. The underlying mechanism is potentially associated with enhanced cerebral blood flow, increased serum BDNF levels, suppressed VILIP-1 and NSE expression, and the repair of damaged neurons. These factors contribute to improving patient prognosis and accelerating the recovery process (Xuefang et al., 2021).
VRCT, akin to CBCT, offers high adaptability by providing personalized training tailored to the participant’s cognitive and physical conditions. This training method features enhanced ecological validity, creating a three-dimensional, digital environment where subjects engage in cognitive tasks within virtual recreations of familiar daily activities (Moreno et al., 2019). Ana Lúcia Faria et al.’s study highlighted that VRCT yields greater cognitive benefits than traditional methods (Faria et al., 2020), aligning with this study’s findings. The effectiveness of VRCT may stem from its immersive experience. By engaging participants in situational daily life scenarios, it offers multisensory, natural, and realistic stimulation. This immersion significantly heightens patients’ action awareness, self-identity, and self-cognition, fostering high interest and enjoyment in VR usage (Choi et al., 2019), thereby comprehensively exercising their abilities and enhancing task success through timely feedback (Faria et al., 2020). Additionally, the gaming aspect of VRCT can also improve cognitive functions. However, it’s noteworthy that participants tend to learn better on desktop computer screens compared to VR games (Mayer, 2019), potentially explaining why CBCT is more effective than its VR counterpart. To elucidate the advantages of VRCT, researchers have proposed various mechanisms. Carrieri et al. suggested that VR can stimulate the reactivation and enhancement of diverse cortical functions, particularly the dorsolateral/ventrolateral prefrontal cortex, optimize sensory cortex efficiency, and boost cognitive function (Carrieri et al., 2016). Flannery et al. noted that VRCT training activates brain metabolism, increases cerebral blood flow, and enhances neurotransmitter release (Flannery, 2002).
Sports combined with cognitive training encompasses two modalities: sequential exercise and cognitive training, and dual-task cognitive training, which concurrently emphasizes physical and cognitive training. This approach is more apt for enhancing the comprehensive skills required in daily life. Prior research indicates that such training is more advantageous in improving overall cognition, working memory, and executive function in elderly individuals with cognitive impairment than conventional treatments (Guo et al., 2020). I-Ching Chuang et al. discovered that exercise combined with cognitive training is more beneficial in enhancing overall cognitive function, particularly in visual-spatial working memory and language memory, compared to either physical or cognitive training alone. However, this approach does not demonstrate significant advantages in daily living abilities (Chuang et al., 2023), aligning with this study’s findings. It is posited that both simultaneous and sequential exercise and cognitive training may impact different cognitive aspects (McEwen et al., 2018). While there is ongoing debate regarding the superiority of each training mode, it is established that exercise combined with cognitive training constitutes an effective intervention. This efficacy could be attributed to exercise’s role in elevating brain neurotrophic factors in the peripheral blood (Marinus et al., 2019), crucial for the survival, maintenance, and regeneration of specific brain neuron populations (Allen et al., 2013). There is evidence indicating that physical exercise enhances the volume of the prefrontal cortex and the prehippocampus, fosters nerve and angiogenesis, and plays a crucial role in reducing cardiovascular risk factors. Furthermore, it has been discovered that the combination of exercise and cognitive training often yields more substantial benefits than either exercise or cognitive training alone. The neurological and cognitive benefits derived from exercise may be associated with increased environmental exposure during cognitive challenge (Karssemeijer et al., 2017). While environmental enrichment is considered to enhance language development in young children, improve cognitive abilities, and reduce the risk of dementia. Increased physical engagement in the form of exercise can enhance cognitive performance, mitigate memory impairment, and decrease the likelihood of Alzheimer’s disease in older adults (Figuracion and Lewis, 2021). In 2016, the inaugural “Adult Stroke Rehabilitation Guidelines” jointly published by the AHA and the ASA also issued Grade-A recommendations for cognitive activities within environmentally enriched settings (Winstein et al., 2016). However, only three related studies were included. Additionally, only the cognitive outcome of the MoCA scale demonstrates that exercise in conjunction with CBCT yields a more favorable effect than CBCT combined with traditional cognitive training. Further high-quality randomized controlled studies are required to ascertain the superiority and inferiority of exercise in combination with CBCT and CBCT in conjunction with traditional cognitive training in enhancing cognitive function and daily living abilities.
Regarding the improvement of motor function, interventions involving CBCT combined with traditional cognitive training are significant. The identical outcomes were obtained in the research, where the functional independence rating served as the outcome index. It should be highlighted that only CBCT in combination with traditional cognitive training, traditional cognitive training, and the conventional control group were incorporated in the study evaluating motor function. Both interventions demonstrated significant improvements compared to the conventional control group. The enhancement of motor function induced by CBCT and traditional cognitive training might be associated with the following reasons. One is the unique training mode of CBCT. CBCT engages participants in using specialized buttons and joysticks to exercise the movements of the wrist, thumb, and index finger of the impaired hand. This approach allows participants to enhance hand flexibility while engaging in cognitive tasks, thereby improving limb coordination in post-stroke patients (Otfinowski et al., 2006), which may account for the reason why the combination of CBCT with traditional cognitive training enhance motor function more effectively than traditional cognitive training alone. It was widely recognized that there is a connection between movement disorders and cognitive decline, although the underlying mechanism remains incompletely understood (Kobayashi-Cuya et al., 2018). In a prospective cohort study conducted by Zhangyu Wang et al., MRI was used to evaluate brain structural volume (including total brain volume, total white matter, total gray matter, hippocampus volume, and white matter high signal) and statistically analyzed in conjunction with motor function and cognitive function data. Ultimately, it was demonstrated that motor impairment is associated with declines in cognitive functions such as overall cognition, episodic memory, semantic memory, working memory, visuospatial ability and perceptual speed (Wang et al., 2023). Paul Rinne et al. identified a consistent and strong correlation between attention control and motor performance. They posit that poor attention control following stroke is one of the causes of limb paralysis after stroke, furthermore they suggest that attention control itself may be a therapeutic target for improving motor disorders post-stroke (Rinne et al., 2018). Additionally, previous research has shown that most patients with left hemisphere damage and a minority with right hemisphere damage exhibit impaired ipsilateral hand dexterity within a month post-infarction involving the parietal or posterior frontal lobes. This impairment may be linked to post-stroke cognitive impairment affecting action perception and control (Sunderland et al., 1999). Paul Rinne and colleagues interpreted this to mean that motor function impairment on the same side of the stroke focus was selectively associated with the lesion load on the attention network, but not with the anterior corticospinal tract (Rinne et al., 2018). In view of the relationship between motor function and cognitive function, it is possible that with the improvement of cognitive function, the motor function of PSCI patients will also be restored. Although the link/correlation is not clear and not necessarily causative.
In the assessment of cognitive domains using the MOCA scale, it is indicated that aside from language and naming functions, various cognitive training methods positively influence the improvement of visual-spatial and executive functions, attention, abstraction, delayed recall, and orientation. CBCT combined with traditional cognitive training is most effective in enhancing attention, abstract function, memory, and orientation. These results fit with the advantages brought by the unique cognitive training mode of CBCT. However, our findings suggest that various cognitive training programs did not show any advantage in language or naming. In contrast, computer-assisted cognitive training demonstrates superior benefits in improving visual-spatial and executive functions. This slightly diverges from Anastasia Nousia et al.’s study, which found that CBCT enhances language fluency, naming, and delayed memory (Nousia et al., 2021). Our study exclusively analyzed the various cognitive areas of the MoCA scale, suggesting the necessity for multiple outcome indicators within each cognitive domain, as improvements in a few indicators for the same function are less convincing than enhancements across multiple indicators within one function.
Our results align with most studies under similar outcome indicators, yet they diverge from some. A study affirmed the efficacy of CBCT and cognitive training in enhancing cognition in PSCI patients but did not demonstrate the superiority of CBCT in cognitive rehabilitation of post-stroke patients (Mingming et al., 2022). Compared to our study, their sample was smaller, encompassing only four studies from 2013-2021 with 96 participants, which may introduce bias. A network meta-analysis involving 1047 PSCI patients from 21 RCTs indicated that computer-based and VR-based cognitive training were superior to conventional training in MOCA, with the computer-based group showing the best therapeutic effect. However, this did not reach statistical significance under the MMSE index (Xiao et al., 2022). This study posits that, aside from combined interventions, CBCT surpasses conventional training in MoCA, possibly due to the limited number of studies directly comparing VRCT with traditional training included in this research.
4.2 Advantages and limitations of this study
This research entailed an extensive review of studies assessing the efficacy of various cognitive training methods in improving cognitive function in patients with post-stroke cognitive impairment (PSCI). The outcome measures included assessments of cognitive function, daily living, motor function, and functional independence. Given the large sample size and the narrow confidence intervals in this network meta-analysis, we consider the results to be reliable. A key strength of this study is its analysis of diverse cognitive training modalities and detailed examination of different cognitive domains, aspects not covered in previous meta-analyses of cognitive training for PSCI. However, there are several limitations. Firstly, there was no analysis of the heterogeneity of baseline characteristics. The included studies varied in intervention duration and frequency, total intervention time, measurement timings, and the computer platforms used in computer-based and VRCT, contributing to significant heterogeneity. Secondly, only three studies on sports cognitive training were included, with a relatively small sample size, raising concerns about the applicability and accuracy of the results. Thirdly, although all included randomized controlled trials provided conventional drug therapy and rehabilitation training in addition to cognitive training interventions, and studies significantly impacted by drug effects on cognitive function were excluded, the specific roles of these treatments remain ambiguous. This lack of clarity is due to insufficient details about the types and dosages of drugs used post-stroke. Fourthly, the influence of VRCT, which often involves whole-body engagement, is challenging to separate into effects attributable to cognitive training versus physical exercise. Fifthly, the included studies did not adequately describe allocation concealment, and since blinding participants to the interventions was unfeasible, only a few studies blinded the raters. Therefore, the study may contain some subjective biases. Finally, since 66% of the original studies included in this study were in Chinese, the extrapolation of the conclusions of this study has certain limitations.
5 Conclusion
Findings from this study demonstrate that supplementing traditional cognitive training with additional training modalities yields superior outcomes in enhancing the cognitive function, daily living skills, functional independence, and motor capabilities in patients with PSCI compared to exclusive cognitive training. Specifically, the influence of an intervention combining CBCT outperforms others in enhancing cognition, daily living function, motor skills, and functional independence. However, an increased number of high-quality, multi-center, large-sample randomized controlled trials are needed in future studies to validate the efficacy of cognitive training. These trials should provide evidence-based data for healthcare professionals to conduct professional assessments based on patients’ specific conditions, and selectively implement these potential non-pharmaceutical interventions in the daily care and rehabilitation of patients with PSCI.
Data availability statement
The original contributions presented in the study are included in the article/Supplementary material, further inquiries can be directed to the corresponding author.
Author contributions
LZ: Writing−original draft. XH: Writing−original draft. JW: Writing−review and editing. FW: Writing−review and editing. JL: Writing−review and editing. NL: Writing−review and editing.
Funding
The author(s) declare financial support was received for the research, authorship, and/or publication of the article. This work was supported by the Key Projects of the Technology Program of the Jiangxi Traditional Chinese Medicine Administration (2023Z030), and the key research and development program of Ganzhou Municipal Science and Technology Bureau (Ganzhou Science and Technology Foundation [2020] No. 60).
Conflict of interest
The authors declare that the research was conducted in the absence of any commercial or financial relationships that could be construed as a potential conflict of interest.
Publisher’s note
All claims expressed in this article are solely those of the authors and do not necessarily represent those of their affiliated organizations, or those of the publisher, the editors and the reviewers. Any product that may be evaluated in this article, or claim that may be made by its manufacturer, is not guaranteed or endorsed by the publisher.
Supplementary material
The Supplementary Material for this article can be found online at: https://www.frontiersin.org/articles/10.3389/fnagi.2024.1374546/full#supplementary-material
References
Allen, S., Watson, J., Shoemark, D., Barua, N., and Patel, N. K. (2013). GDNF, NGF and BDNF as therapeutic options for neurodegeneration. Pharmacol. Ther. 138, 155–175. doi: 10.1016/j.pharmthera.2013.01.004
Bahar-Fuchs, A., Martyr, A., Goh, A., Sabates, J., and Clare, L. (2019). Cognitive training for people with mild to moderate dementia. Cochrane Database Syst. Rev. 3:Cd013069. doi: 10.1002/14651858.CD013069
Bavelier, D., and Green, C. (2019). Enhancing attentional control: Lessons from action video games. Neuron 104, 147–163. doi: 10.1016/j.neuron.2019.09.031
Cao, H., Xia, W., Zheng, C., and Wang, J. (2017). “The effect of computer-assisted cognitive training on the rehabilitation of different degrees of cognitive impairment after stroke,” in Proceedings of the 18th national conference on physical medicine and rehabilitation of the Chinese medical association, (Shenyang), 182–182.
Carrieri, M., Petracca, A., Lancia, S., Basso Moro, S., Brigadoi, S., Spezialetti, M., et al. (2016). Prefrontal cortex activation upon a demanding virtual hand-controlled task: A new Frontier for neuroergonomics. Front. Hum. Neurosci. 10:53. doi: 10.3389/fnhum.2016.00053
Chen, J., Mu, Y., Huang, X., Jiang, C., and Wang, K. (2019). Rehabilitation effect of computer –assisted cognitive training on attention disorder in stroke patients. Anhui Med. J. 40, 865–868. doi: 10.3969/j.issn.1000-0399.2019.08.006
Chen, S., Jiang, Q., Liu, P., Huang, D., and Ding, J. (2006). Effect of cognitive rehabilitation on the functional independence of hemiplegic patients with stroke. Chin. J. Clin. Rehabil. 10, 14–16. doi: 10.3321/j.issn:1673-8225.2006.18.006
Chen, X., Chen, S., and Li, J. (2020). Effect of cognitive training on recovery of limb function and daily function in stroke patients. Chin. Ment. Health J. 34, 299–304. doi: 10.3969/j.issn.1000-6729.2020.4.001
Chen, X., Liu, F., Lin, S., Yu, L., and Lin, R. (2022). Effects of virtual reality rehabilitation training on cognitive function and activities of daily living of patients with poststroke cognitive impairment: A systematic review and meta-analysis. Arch. Phys. Med. Rehabil. 103, 1422–1435. doi: 10.1016/j.apmr.2022.03.012
Cho, D., and Lee, S. (2019). Effects of virtual reality immersive training with computerized cognitive training on cognitive function and activities of daily living performance in patients with acute stage stroke: A preliminary randomized controlled trial. Medicine (Baltimore) 98:e14752. doi: 10.1097/md.0000000000014752
Choi, H., Shin, W., and Bang, D. (2019). Mirror therapy using gesture recognition for upper limb function, neck discomfort, and quality of life after chronic stroke: A single-blind randomized controlled trial. Med. Sci. Monit. 25, 3271–3278. doi: 10.12659/msm.914095
Chuang, I., Chiau, H., Liao, W., Wu, Y., Chang, C., and Wu, C. (2023). Effects of computer-based cognitive training combined with physical training for older adults with cognitive impairment: A four-arm randomized controlled trial. Digit. Health 9:20552076231203633. doi: 10.1177/20552076231203633
De Luca, R., Russo, M., Naro, A., Tomasello, P., Leonardi, S., Santamaria, F., et al. (2018). Effects of virtual reality-based training with BTs-Nirvana on functional recovery in stroke patients: Preliminary considerations. Int. J. Neurosci. 128, 791–796. doi: 10.1080/00207454.2017.1403915
El Husseini, N., Katzan, I., Rost, N., Blake, M., Byun, E., Pendlebury, S., et al. (2023). Cognitive impairment after ischemic and hemorrhagic stroke: A scientific statement from the American Heart association/American stroke association. Stroke 54, e272–e291. doi: 10.1161/str.0000000000000430
Faria, A., Pinho, M., and Bermúdez, I. B. S. (2020). A comparison of two personalization and adaptive cognitive rehabilitation approaches: A randomized controlled trial with chronic stroke patients. J. Neuroeng. Rehabil. 17:78. doi: 10.1186/s12984-020-00691-5
Feng, Q., Jin, Y., Zhou, G., and Wang, C. (2014). The effect of nurse led reinforcement cognitive intervention on non dementia cognitive impairment in stroke patients. Chin. J. Gerontol. 21, 6043–6045. doi: 10.3969/j.issn.1005-9202.2014.21.047
Figuracion, K., and Lewis, F. (2021). Environmental enrichment: A concept analysis. Nurs. Forum 56, 703–709. doi: 10.1111/nuf.12565
Flannery, R. (2002). Treating learned helplessness in the elderly dementia patient: Preliminary inquiry. Am. J. Alzheimers Dis. Other Dement. 17, 345–349. doi: 10.1177/153331750201700605
Fu, K., Liu, Y., Sun, L., Fan, F., Liu, Y., Jiang, G., et al. (2020). The effect of virtual reality training on cognitive and balance functions in stroke recovery patients. Chin. Manip. Qi Gong Ther. 11, 34–36. doi: 10.19787/j.issn.1008-1879.2020.01.012
GBD 2019 Diseases and Injuries Collaborators (2020). Global burden of 369 diseases and injuries in 204 countries and territories, 1990-2019: A systematic analysis for the global burden of disease study 2019. Lancet 396, 1204–1222. doi: 10.1016/s0140-6736(20)30925-9
Gong, W. J. (2017). Effect of motor imagery therapy on cognitive function of patients with stroke. Chin. J. Contemp. Neurol. Neurosurg. 17:5. doi: 10.3969/j.issn.1672-6731.2017.06.005
Guo, W., Zang, M., Klich, S., Kawczyński, A., Smoter, M., and Wang, B. (2020). Effect of combined physical and cognitive interventions on executive functions in OLDER adults: A meta-analysis of outcomes. Int. J. Environ. Res. Public Health 17:6166. doi: 10.3390/ijerph17176166
Hermann, P., and Zerr, I. (2022). Rapidly progressive dementias – aetiologies, diagnosis and management. Nat. Rev. Neurol. 18, 363–376. doi: 10.1038/s41582-022-00659-0
Ho, H., Chen, M., Tsai, C., and Chen, H. (2022). Effects of computerized cognitive training on cognitive function, activity, and participation in individuals with stroke: A randomized controlled trial. NeuroRehabilitation 51, 79–89. doi: 10.3233/nre-210271
Jiang, R., Chen, Y., Luo, L., and Pan, C. (2017). Effects of diagnosis and treatment system of cognitive disorders ZM3.1 on cognitive impairment after stroke. Chin. J. Rehabil. Med. 32, 414–418. doi: 10.3969/j.issn.1001-1242.2017.04.007
Jung, H., Daneault, J., Nanglo, T., Lee, H., Kim, B., Kim, Y., et al. (2020). Effectiveness of a serious game for cognitive training in chronic stroke survivors with mild-to-moderate cognitive impairment: A pilot randomized controlled trial. Appl. Sci. 10:6703. doi: 10.3390/app10196703
Karssemeijer, E., Aaronson, J., Bossers, W., Smits, T., Olde Rikkert, M., and Kessels, R. (2017). Positive effects of combined cognitive and physical exercise training on cognitive function in older adults with mild cognitive impairment or dementia: A meta-analysis. Ageing Res. Rev. 40, 75–83. doi: 10.1016/j.arr.2017.09.003
Kim, B., Chun, M., Kim, L., and Park, J. (2011). Effect of virtual reality on cognition in stroke patients. Ann. Rehabil. Med. 35, 450–459. doi: 10.5535/arm.2011.35.4.450
Kobayashi-Cuya, K., Sakurai, R., Suzuki, H., Ogawa, S., Takebayashi, T., and Fujiwara, Y. (2018). Observational evidence of the association between handgrip strength, hand dexterity, and cognitive performance in community-dwelling older adults: A systematic review. J. Epidemiol. 28, 373–381. doi: 10.2188/jea.JE20170041
Li, H., and Gan, L. (2010). Application of cognitive training in rehabilitation of patients with cognitive disorder after cerebral apoplexy. Chin. Nurs. Res. 24, 1273–1274. doi: 10.3969/j.issn.1009-6493.2010.14.032
Li, H., Hu, Z., Gong, Z., Wang, J., and Wang, Q. (2017). Application of cognitive training in motor function recovery of stroke patients with cognitive impairment and hemiplegia. Chin. Nurs. Res. 31, 232–234. doi: 10.3969/j.issn.1009-6493.2017.02.032
Li, X., Lu, S., An, Y., Wang, D., Zhang, J., Li, X., et al. (2016). Attention training for patients with vascular cognitive impairment after cerebral infarction. J. Nurs. Sci. 31, 8–10. doi: 10.3870/j.issn.1001-4152.2016.11.008
Li, M., Shan, D., Xiao, L., and Chen, Q. (2016). Curative effect of computer assisted cognitive rehabilitation system for cognitive impairment after stroke. J. Kunm. Med. Univers. 37, 78–80. doi: 10.3969/j.issn.1003-4706.2016.10.019
Li, R., Geng, J., Yang, R., Ge, Y., and Hesketh, T. (2022). Effectiveness of computerized cognitive training in delaying cognitive function decline in people with mild cognitive impairment: Systematic review and meta-analysis. J. Med. Internet Res. 24:e38624. doi: 10.2196/38624
Li, W., Xu, X., Wu, F., Ni, Y., Lan, J., and Hu, X. (2021). Comparative efficacy of non-pharmacological interventions on behavioural and psychological symptoms in elders with dementia: A network meta-analysis. Nurs. Open 8, 2922–2931. doi: 10.1002/nop2.1049
Liao, P., Zhou, F., Wu, L., and Li, X. (2022). The impact of computer-aided cognitive rehabilitation system on cognitive function in patients with post-stroke cognitive impairment. J. Minim. Invasive Med. 17, 645–648. doi: 10.11864/j.issn.1673.2022.05.28
Liu, S., Ni, C., Han, R., Li, H., Sun, H., Gao, S., et al. (2006). Community based rehabilitation of cognitive deficits in stroke patients. Chin. J. Phys. Med. Rehabil. 28, 814–817. doi: 10.3760/j:issn:0254-1424.2006.12.008
Liu, Z. J., Zeng, Q., and He, H. G. (2019). Clinical effect of computer-assisted cognitive training on post-stroke cognitive impairment. Guangdong Med. J. 40, 1401–1404. doi: 10.13820/j.cnki.gdyx.20186802
Liu, Z., He, Z., Yuan, J., Lin, H., Fu, C., Zhang, Y., et al. (2022). Application of immersive virtual-reality-based puzzle games in elderly patients with post-stroke cognitive impairment: A pilot study. Brain Sci. 31:13. doi: 10.3390/brainsci13010079
Lv, C., Hu, L., Zhao, D., and Ma, Y. (2017). Observation on the therapeutic effect of computer-aided cognitive training on cognitive impairment in stroke patients. Chin. J. Integr. Med. Cardio Cerebrovasc. Dis. 15, 3196–3199. doi: 10.3969/j.issn.1672-1349.2017.24.035
Maggio, M., De Bartolo, D., Calabrò, R., Ciancarelli, I., Cerasa, A., Tonin, P., et al. (2023). Computer-assisted cognitive rehabilitation in neurological patients: State-of-art and future perspectives. Front. Neurol. 14:1255319. doi: 10.3389/fneur.2023.1255319
Maier, M., Banuelos, N., Ballester, B., Duarte, E., and Verschure, P. (2017). Conjunctive rehabilitation of multiple cognitive domains for chronic stroke patients in virtual reality. IEEE Int. Conf. Rehabil. Robot. 2017, 947–952. doi: 10.1109/icorr.2017.8009371
Mao, L. Y., Chen, Y., Tang, L., Liu, L., and Feng, N. J. (2019). Effects of mirror visual feedback on cognition and upper limb function for stroke patients. Chin. J. Rehabil. Theory Pract. 25, 284–288. doi: 10.3969/j.issn.1006.9771.2019.03.007
Marinus, N., Hansen, D., Feys, P., Meesen, R., Timmermans, A., and Spildooren, J. (2019). The impact of different types of exercise training on peripheral blood brain-derived neurotrophic factor concentrations in older adults: A meta-analysis. Sports Med. 49, 1529–1546. doi: 10.1007/s40279-019-01148-z
Mayer, R. (2019). Computer games in education. Annu. Rev. Psychol. 70, 531–549. doi: 10.1146/annurev-psych-010418-102744
McEwen, S., Siddarth, P., Rahi, B., Kim, Y., Mui, W., Wu, P., et al. (2018). Simultaneous aerobic exercise and memory training program in older adults with subjective memory impairments. J. Alzheimers Dis. 62, 795–806. doi: 10.3233/jad-170846
Mingming, Y., Bolun, Z., Zhijian, L., Yingli, W., and Lanshu, Z. (2022). Effectiveness of computer-based training on post-stroke cognitive rehabilitation: A systematic review and meta-analysis. Neuropsychol. Rehabil. 32, 481–497. doi: 10.1080/09602011.2020.1831555
Moreno, A., Wall, K., Thangavelu, K., Craven, L., Ward, E., and Dissanayaka, N. N. (2019). A systematic review of the use of virtual reality and its effects on cognition in individuals with neurocognitive disorders. Alzheimers Dement. 5, 834–850. doi: 10.1016/j.trci.2019.09.016
Nie, P., Liu, F., Lin, S., Guo, J., Chen, X., Chen, S., et al. (2022). The effects of computer-assisted cognitive rehabilitation on cognitive impairment after stroke: A systematic review and meta-analysis. J. Clin. Nurs. 31, 1136–1148. doi: 10.1111/jocn.16030
Nousia, A., Martzoukou, M., Siokas, V., Aretouli, E., Aloizou, A., Folia, V., et al. (2021). Beneficial effect of computer-based multidomain cognitive training in patients with mild cognitive impairment. Appl. Neuropsychol. Adult 28, 717–726. doi: 10.1080/23279095.2019.1692842
Oh, E., and Rabins, P. (2019). Dementia. Ann. Intern. Med. 171, Itc33–Itc48. doi: 10.7326/aitc201909030
Otfinowski, J., Jasiak-Tyrkalska, B., Starowicz, A., and Reguła, K. (2006). [Computer-based rehabilitation of cognitive impairments and motor arm function of patients with hemiparesis after stroke]. Neurol. Neurochir. Pol. 40, 112–118.
Ou, H., Haifang, Y., Li, Y., Zhan, L., and Chen, H. (2007). The effect of cognitive rehabilitation on motor function and activity of daily life(ADL) in hemiplegic patients with stroke. Guangdong Med. J. 28, 1245–1247. doi: 10.3969/j.issn.1001-9448.2007.08.016
Page, M., McKenzie, J., Bossuyt, P., Boutron, I., Hoffmann, T., Mulrow, C., et al. (2021). The PRISMA 2020 statement: An updated guideline for reporting systematic reviews. BMJ 372:n71. doi: 10.1136/bmj.n71
Pang, M., Yang, L., Ouyang, H., Lam, F., Huang, M., and Jehu, D. (2018). Dual-task exercise reduces cognitive-motor interference in walking and falls after stroke. Stroke 49, 2990–2998. doi: 10.1161/strokeaha.118.022157
Papaioannou, T., Voinescu, A., Petrini, K., and Stanton Fraser, D. (2022). Efficacy and moderators of virtual reality for cognitive training in people with dementia and mild cognitive impairment: A systematic review and meta-analysis. J. Alzheimers Dis. 88, 1341–1370. doi: 10.3233/jad-210672
Petersen, R., Lopez, O., Armstrong, M., Getchius, T., Ganguli, M., Gloss, D., et al. (2018). Practice guideline update summary: Mild cognitive impairment: Report of the guideline development, dissemination, and implementation subcommittee of the American academy of neurology. Neurology 90, 126–135. doi: 10.1212/wnl.0000000000004826
Prokopenko, S., Bezdenezhnykh, A., Mozheyko, E., and Petrova, M. M. (2018). A comparative clinical study of the effectiveness of computer cognitive training in patients with post-stroke cognitive impairments without dementia. Psychol. Russia 11, 55–67. doi: 10.11621/PIR.2018.0205
Prokopenko, S., Bezdenezhnykh, A., Mozheyko, E., and Zubrickaya, E. (2019). Effectiveness of computerized cognitive training in patients with poststroke cognitive impairments. Neurosci. Behav. Phys. 49, 539–543. doi: 10.1007/s11055-019-00767-3
Rinne, P., Hassan, M., Fernandes, C., Han, E., Hennessy, E., Waldman, A., et al. (2018). Motor dexterity and strength depend upon integrity of the attention-control system. Proc. Natl. Acad. Sci. U.S.A. 115, E536–E545. doi: 10.1073/pnas.1715617115
Rost, N., Brodtmann, A., Pase, M., van Veluw, S., Biffi, A., Duering, M., et al. (2022). Post-stroke cognitive impairment and dementia. Circ. Res. 130, 1252–1271. doi: 10.1161/circresaha.122.319951
Shang, Y., Li, W., Zhou, Y., Chen, Z., Sun, C., Li, R., et al. (2022). The effect of computer-aided cognitive training on cognitive impairment after stroke. Chin. J. Phys. Med. Rehabil. 44, 700–702. doi: 10.3760/cma.j.issn.0254-1424.2022.08.006
Shao, Q. (2021). Clinical efficacy observation of computer-aided cognitive training system in patients with cognitive impairment after stroke. Zhengzhou: Zhengzhou University.
Shin, S., Kim, J., and Kim, Y. (2008). The effects of a computer-assisted cognition training program (RehaCom®) in stroke patients. Brain Neurorehabil. 1, 181–189. doi: 10.12786/bn.2008.1.2.181
Sterne, J., Savović, J., Page, M., Elbers, R., Blencowe, N., Boutron, I., et al. (2019). RoB 2: A revised tool for assessing risk of bias in randomised trials. BMJ 366, l4898. doi: 10.1136/bmj.l4898
Sun, R., Li, X., Zhu, Z., Li, T., Li, W., Huang, P., et al. (2021). Effects of combined cognitive and exercise interventions on poststroke cognitive function: A systematic review and meta-analysis. Biomed. Res. Int. 2021:4558279. doi: 10.1155/2021/4558279
Sun, R., Li, X., Zhu, Z., Li, T., Zhao, M., Mo, L., et al. (2022). Effects of dual-task training in patients with post-stroke cognitive impairment: A randomized controlled trial. Front. Neurol. 13:1027104. doi: 10.3389/fneur.2022.1027104
Sunderland, A., Bowers, M., Sluman, S., Wilcock, D., and Ardron, M. (1999). Impaired dexterity of the ipsilateral hand after stroke and the relationship to cognitive deficit. Stroke 30, 949–955. doi: 10.1161/01.str.30.5.949
Turunen, M., Hokkanen, L., Bäckman, L., Stigsdotter-Neely, A., Hänninen, T., Paajanen, T., et al. (2019). Computer-based cognitive training for older adults: Determinants of adherence. PLoS One 14:e0219541. doi: 10.1371/journal.pone.0219541
Wang, H., Jiang, Y., and Liu, J. (2009). Effect of cognitive intervention on rehabilitation of cerebral hemorrhage patients. J. Nurs. Sci. 24, 76–77. doi: 10.3870/hlxzz.2009.01.076
Wang, M. (2019). Effect of computer-assisted cognitive rehabilitation combined with group cognitive training on cognitive function of stroke patients. Chin. J. Microcirc. 29, 43–47. doi: 10.3969/j.issn.1005-1740.2019.01.009
Wang, X., Gu, Y., and Liu, M. (2013). Effectiveness of computer-assisted cognitive training system for patients with cognitive function impairment after stroke. Chin. J. Rehabil. 28, 330–332. doi: 10.3870/zgkf.2013.05.003
Wang, Z., Wang, J., Guo, J., Dove, A., Arfanakis, K., Qi, X., et al. (2023). Association of motor function with cognitive trajectories and structural brain differences: A community-based cohort study. Neurology 101, e1718–e1728. doi: 10.1212/wnl.0000000000207745
Winstein, C., Stein, J., Arena, R., Bates, B., Cherney, L., Cramer, S., et al. (2016). Guidelines for adult stroke rehabilitation and recovery: A guideline for healthcare professionals from the American heart association/American stroke association. Stroke 47, e98–e169. doi: 10.1161/str.0000000000000098
Xia, Y., Li, Q., and Fan, K. (2022). The effect of computer-aided cognitive training on the recovery of cognitive and neurological function in patients with ischemic stroke. J. Clin. Nurs. 21, 67–70. doi: 10.3969/j.issn.1671-8933.2022.02.024
Xiao, T. (2018). The impact of computer-aided cognitive training based on information processing theory on cognitive impairment after stroke. Fuzhou: Fujian University of Traditional Chinese Medicine.
Xiao, X., and Liang, B. (2019). The effect of virtual reality training on cognitive function and P300 in stroke recovery patients. Chin. J. Rehabil. Med. 34, 339–341. doi: 10.3969/j.issn.1001-1242.2019.03.019
Xiao, Z., Wang, Z., Ge, S., Zhong, Y., and Zhang, W. (2022). Rehabilitation efficacy comparison of virtual reality technology and computer-assisted cognitive rehabilitation in patients with post-stroke cognitive impairment: A network meta-analysis. J. Clin. Neurosci. 103, 85–91. doi: 10.1016/j.jocn.2022.07.005
Xu, F., Huang, L., Ni, C., Chen, J., Wang, L., Feng, H., et al. (2015). The effect of computer-aided cognitive training on cognitive function in stroke patients. Anhui Med. Pharm. J. 8, 1519–1520. doi: 10.3969/j.issn.1009-6469.2015.08.024
Xuefang, L., Guihua, W., and Fengru, M. (2021). The effect of early cognitive training and rehabilitation for patients with cognitive dysfunction in stroke. Int. J. Methods Psychiatr. Res. 30:e1882. doi: 10.1002/mpr.1882
Yang, H., and Liu, Z. (2008). Effect of cognitive function training on cognitive impairment in patients with stroke. J. Pract. Med. 24, 4058–4059. doi: 10.3969/j.issn.1006-5725.2008.23.026
Ye, H., Yang, S., Chen, L., and Jiang, C. (2014). Effect of computer-assisted cognitive training on cognitive function and functional independence of stroke patients. J. Pract. Med. 17, 2742–2744. doi: 10.3969/j.issn.1006-5725.2014.17.016
Yeh, T., Chang, K., and Wu, C. (2019). The active ingredient of cognitive restoration: A multicenter randomized controlled trial of sequential combination of aerobic exercise and computer-based cognitive training in stroke survivors with cognitive decline. Arch. Phys. Med. Rehabil. 100, 821–827. doi: 10.1016/j.apmr.2018.12.020
Yeh, T., Chang, K., Wu, C., Chen, C., and Chuang, I. (2022). Clinical efficacy of aerobic exercise combined with computer-based cognitive training in stroke: A multicenter randomized controlled trial. Top. Stroke Rehabil. 29, 255–264. doi: 10.1080/10749357.2021.1922045
Yoo, C., Yong, M., Chung, J., and Yang, Y. (2015). Effect of computerized cognitive rehabilitation program on cognitive function and activities of living in stroke patients. J. Phys. Ther. Sci. 27, 2487–2489. doi: 10.1589/jpts.27.2487
Zhang, C., Zhong, X., and Ke, Q. (2018). Observation on the therapeutic effect of cognitive function training combined with rehabilitation nursing on post-stroke cognitive impairment patients. Acad. J. Guangzhou Med. Coll. 46, 112–114. doi: 10.3969/j.issn.2095-9664.2018.03.29
Zhang, J., Wang, Q., Xie, J., Wei, N., and Zhang, H. (2012). The effect of reinforced cognitive training on the daily living activities of cognitive impairment patients after stroke. Chin. J. Rehabil. Theory Pract. 18, 778–780.
Zhang, L., Liu, C., Li, H., and Jian, F. (2020). The effect of computer-assisted cognitive rehabilitation system intervention on cognitive impairment in stroke. Modern Med. J. 48, 1332–1334. doi: 10.3969/j.issn.1671-7562.2020.10.021
Zhao, Z., Wang, X., Shen, N., Tang, H., Liu, J., and Zhang, L. (2020). Rehabilitation effect of cognitive function training on cognitive impairment patients after stroke. Chin. Pharm. 29:1.
Zhao, Z., Zhao, K., and Qu, Y. (2022). Computer-assisted cognition training relieves cognitive dysfunction after a stroke: A multicenter randomized and controlled study. Chin. J. Phys. Med. Rehabil. 44, 978–981. doi: 10.3760/cma.j.issn.0254-1424.2022.11.004
Zucchella, C., Capone, A., Codella, V., Vecchione, C., Buccino, G., Sandrini, G., et al. (2014). Assessing and restoring cognitive functions early after stroke. Funct. Neurol. 29, 255–262.
Keywords: cognitive training, computer-based cognitive training, virtual reality cognitive training, Multi-component training, post-stroke cognitive impairment, Treatment
Citation: Zhou L, Huang X, Wang J, Wang F, Liu J and Liu N (2024) The influence of eight cognitive training regimes upon cognitive screening tool performance in post-stroke survivors: a network meta-analysis. Front. Aging Neurosci. 16:1374546. doi: 10.3389/fnagi.2024.1374546
Received: 22 January 2024; Accepted: 04 July 2024;
Published: 19 July 2024.
Edited by:
Lori G. Cook, The University of Texas at Dallas, United StatesReviewed by:
Rizaldy Taslim Pinzon, Duta Wacana Christian University, IndonesiaMartin Rice, Texas Woman’s University, United States
Copyright © 2024 Zhou, Huang, Wang, Wang, Liu and Liu. This is an open-access article distributed under the terms of the Creative Commons Attribution License (CC BY). The use, distribution or reproduction in other forums is permitted, provided the original author(s) and the copyright owner(s) are credited and that the original publication in this journal is cited, in accordance with accepted academic practice. No use, distribution or reproduction is permitted which does not comply with these terms.
*Correspondence: Nanhai Liu, 13970781916@163.com
†These authors have contributed equally to this work