- 1Department of Ophthalmology, Wuhan No. 1 Hospital, Tongji Medical College, Huazhong University of Science and Technology, Wuhan, Hubei, China
- 2Wuhan Fourth Hospital, Wuhan, Hubei, China
Purpose: Since age-related macular degeneration (AMD) is tightly associated with aging and cellular senescence, objective of this study was to investigate the association between plasma levels of senescence-related proteins (SRPs) and risk of AMD.
Design: The whole study was based on two-sample Mendelian randomization (MR) analysis.
Methods: For MR analysis, the primary approach for MR analysis was the inverse-variance weighted (IVW) method and the heterogeneity and pleiotropy of results were tested. The instrumental single-nucleotide polymorphisms (SNPs) associated with 110 SRPs were filtered and selected from a large genome-wide association study (GWAS) for plasma proteome involving 35,559 participants. The GWAS data of AMD was obtained from FinnGen consortium (6,157 AMD cases and 288,237 controls) and further validated by using data from UK Biobank consortium (3,553 AMD cases and 147,089 controls).
Results: The MR results at both discovery and validation stages supported the causality (IVW-P < 0.00045) between plasma levels of 4 SRPs (C3b, CTNNB1, CCL1, and CCL3L1) and the risk of AMD and supported potential causality (IVW-P < 0.05) between other 10 SRPs and risk of AMD. No heterogeneity or pleiotropy in these results was detected.
Conclusion: Our findings supported that high plasma levels of C3b, CTNNB1, CCL1, and CCL3L1 were associated with increased risk of AMD, thereby highlighting the role of systemic inflammation in AMD pathogenesis and providing the rationale for developing new preventative and therapeutic strategies.
1 Introduction
Age-related macular degeneration (AMD) is a neurodegenerative disease involving neuroretina and retinal pigment epithelium (RPE), thereby leading to visual impairment or even blindness (Wong et al., 2014). Studies have reported considerable health burden in patients with AMD, which mainly affects adults aged 40 years and older (Zhu et al., 2019). The number of patients with AMD is continuously increasing and estimated to be about 288 million worldwide by 2,040 (Congdon et al., 2004; Wong et al., 2014).
Intravitreal injection of anti-vascular endothelial growth factor (VEGF), such as ranibizumab (Blodi et al., 2023) and aflibercept (Wykoff et al., 2023), have been used to slow the progression of the neovascular or exudative subtype (nAMD or eAMD). However, for geographic or atrophic AMD, there is no effective treatment available. Therefore, it is necessary to identify risk factors for AMD to help prevent the incidence of AMD and alleviate the burden of this disease on public health. Some intraocular risk factors have been revealed, such as extracellular deposits (Chen et al., 2022). Notably, localized mechanistic studies in the eye have failed to fully elucidate pathogenesis of AMD and the systemic risk factors for AMD were still lacking, thereby limiting the interventions for reducing the risk of AMD.
As a hallmark of aging, cellular senescence is a significant contributor to aging and age-related diseases including Alzheimer’s disease (AD) (Holloway et al., 2023). Previous studies have suggested that oxidative stress, inflammation and RPE senescence may all play a critical role (Kauppinen et al., 2016) in AMD initiation and development. Notably, Saul et al. (2022) has identified a gene set (senescence-related genes, SRGs) for predicting senescence-associated pathways across tissues, which has been used and cited by many researches about age-related diseases (Doolittle et al., 2023; Farr, 2023).
Observational study and randomized clinical trial (RCT) are useful for identifying risk factors of diseases. However, observational studies are vulnerable to reverse causation, residual confounding, and selective bias (Davey Smith and Hemani, 2014). A RCT allows reliable and robust causal inferences to be drawn, but it is costly, time-consuming, and sometimes impractical to conduct one. Mendelian randomization (MR) approaches have opened up opportunities to assess and determine clinically associated characters for multiple diseases (Davey Smith and Hemani, 2014), which examines causal relationships between exposures and outcomes using genetic variants significantly associated with an exposure as instrumental variables. By using MR approaches, the defects of observational study and RCT can be nicely overcome (Davey Smith and Hemani, 2014). In addition, this approach has been used to explore risk factors of multiple eye disorders (Patasova et al., 2021; Choquet et al., 2022).
In this study, we obtained the list of SRGs and explored the causality between the plasma levels of the proteins (senescence-related proteins, SRPs) encoded by these SRGs and the risk of AMD by two-sample MR analysis. The design and findings of this study were summarized in Figure 1. As a results, plasma levels of 4 SRPs (C3b, CCL1, CCL3L1, and CTNNB1) were identified and confirmed to be associated with risk of AMD. It is hoped that these 4 SRPs will serve as new drug targets for intervention.
2 Materials and methods
2.1 Theoretical foundation of MR analysis
The list of SRGs was obtained from Saul et al. (2022) (Supplementary Table 1). The theoretical basis and three basic assumptions of MR analysis (Emdin et al., 2017; Zheng et al., 2017) were shown in Figure 2. In this study, we implemented two-sample MR approaches to judge causation between plasma SRPs and AMD risk.
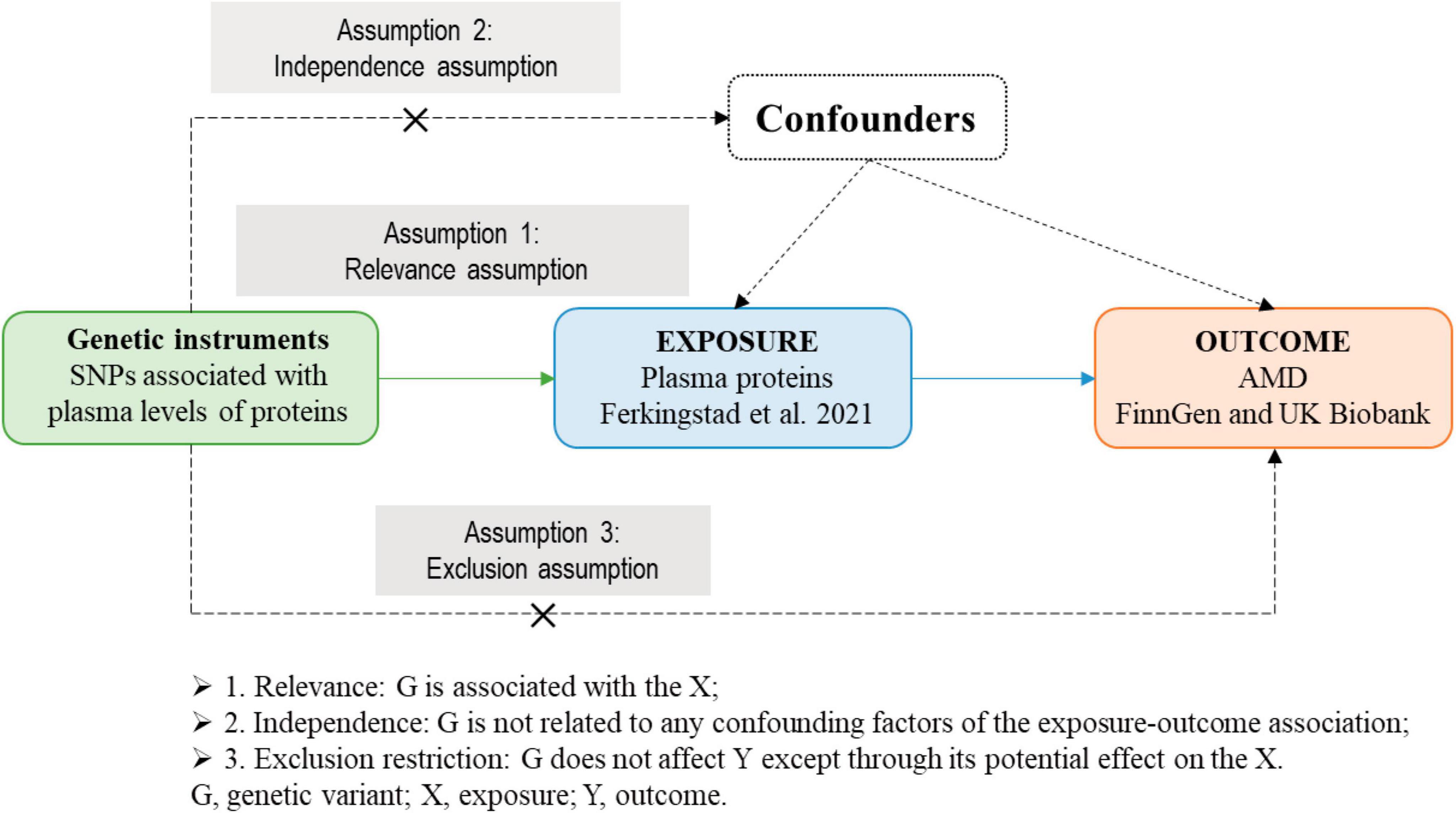
Figure 2. The theoretical basis and three basic assumptions of MR analysis. MR, Mendelian randomization.
2.2 Data source for exposures and outcomes
The summary level genome-wide association studies (GWAS) data of SRPs were obtained from Ferkingstad et al. (2021), who have conducted a large-scale GWAS project on plasma proteome involving 35,559 participants and 4,907 plasma proteins. The GWAS data of AMD were obtained from FinnGen (6,157 cases and 288,237 controls) (Kurki et al., 2023) and UK Biobank consortium (3,553 cases and 147,089 controls) (Sudlow et al., 2015). The AMD cases were defined by H7 in International Classification of Disease-10 (ICD-10) and 3,625 in ICD-9. The FinnGen consortium data was used for discovery and data from UK Biobank consortium was for replication and validation. The flowchart of this study is presented in Figure 3.
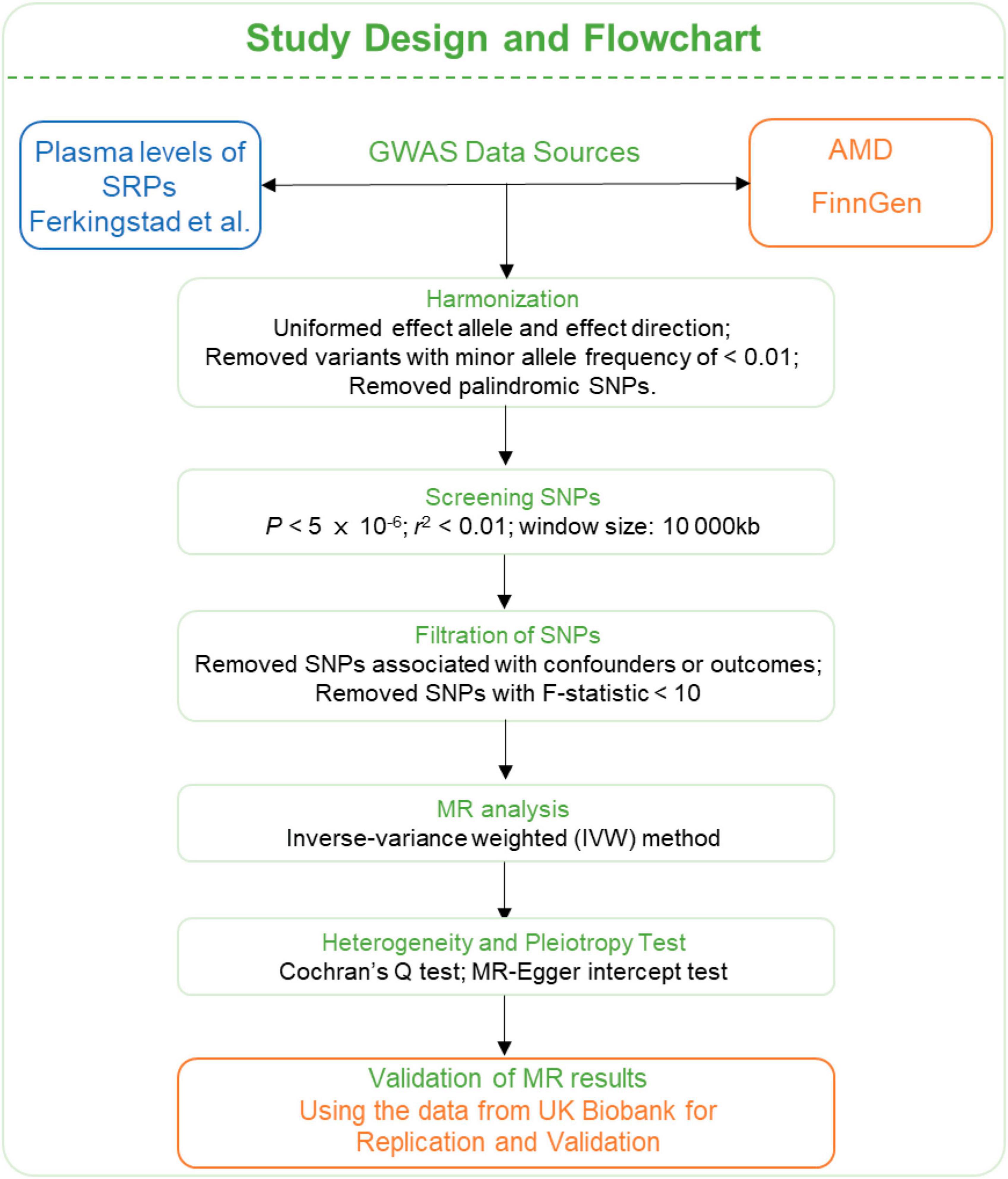
Figure 3. Flowchart of the MR analyses. MR: Mendelian randomization; SRPs, senescence-related proteins; AMD, age-related macular degeneration; GWAS, genome-wide association study; SNP, single nucleotide polymorphism; IVW, inverse-variance weighted.
2.3 Selection of genetic instruments
Instrumental variables (single-nucleotide polymorphisms, SNPs) were selected via the following criteria: (i) with genome-wide significance (P < 5 × 10–6) and (ii) pruned by linkage disequilibrium (r2 < 0.01 and within 10 000 kb from the index variant). PhenoScanner (Staley et al., 2016) is an online platform with comprehensive information about genotype-phenotype association. We examined whether the obtained instrumental SNPs were associated with the outcomes and the potential confounders and subsequently remove the associated ones.
2.4 Causality estimated by MR analysis
Mendelian randomization’s validity depends on the crucial assumption of no pleiotropy (Lawlor et al., 2008). Therefore, we used the random-effect inverse-variance weighted (IVW) method (Burgess et al., 2016) as the primary method and performed Cochran’s Q test and MR-Egger intercept test to evaluate the heterogeneity and detect pleiotropy (Bowden et al., 2019).
2.5 Identification of druggable targets
A list of druggable genes were obtained from Freshour et al. (2021) (Drug-Gene Interaction Database, DGIdb V.4.2.01) (Supplementary Table 2). DGIdb provides information on drug-gene interactions and druggable genes from publications, databases and other web-based sources. We downloaded the “Categories Data” (released in February 2022), including all genes in the druggable categories in the DGIdb, from all sources.
2.6 Statistical analysis
We performed all the analyses in R (version 4.0.1) using the TwoSampleMR (Hemani et al., 2018) R packages. The code for MR analysis is accessible at https://mrcieu.github.io/TwoSampleMR/articles/index.html. All statistical tests are 2 sided. Results with IVW-P < 0.05 was considered nominally significant and IVW-P < 0.00045 was taken as statistically significant.
3 Results
3.1 MR results in the discovery stage
The list of 110 SRPs was in Table 1. In the discovery stage, based on MR results, 27 SRPs were suggestively associated and 7 SRPs were significantly associated with risk of AMD (Figures 4, 5; Supplementary Table 3). The 7 SRPs significantly associated with AMD risk were: C3b (odds ratio [OR] = 6.66, 95% confidence interval [CI]: 2.71–16.37, IVW-P = 3.60E-5), CD9 (OR = 2.51, 95% CI: 1.62–3.88, IVW-P = 3.88E-5), CTNNB1 (OR = 1.91, 95% CI: 1.37–2.66, IVW-P = 1.52E-4), CCL24 (OR = 4.27, 95% CI: 1.72–10.56, IVW-P = 1.69E-4), CCL1 (OR = 1.95, 95% CI: 1.37–2.76, IVW-P = 1.76E-4), CCL3L1 (OR = 1.86, 95% CI: 1.3–2.66, IVW-P = 4.13E-4), PECAM1 (OR = 2.4, 95% CI: 1.45–3.97, IVW-P = 4.21E-4). The MR-Egger intercept test and Cochran’s Q test all suggested no apparent heterogeneity or pleiotropy in these 7 results (Heterogeneity-P > 0.05 and Pleiotropy-P > 0.05).
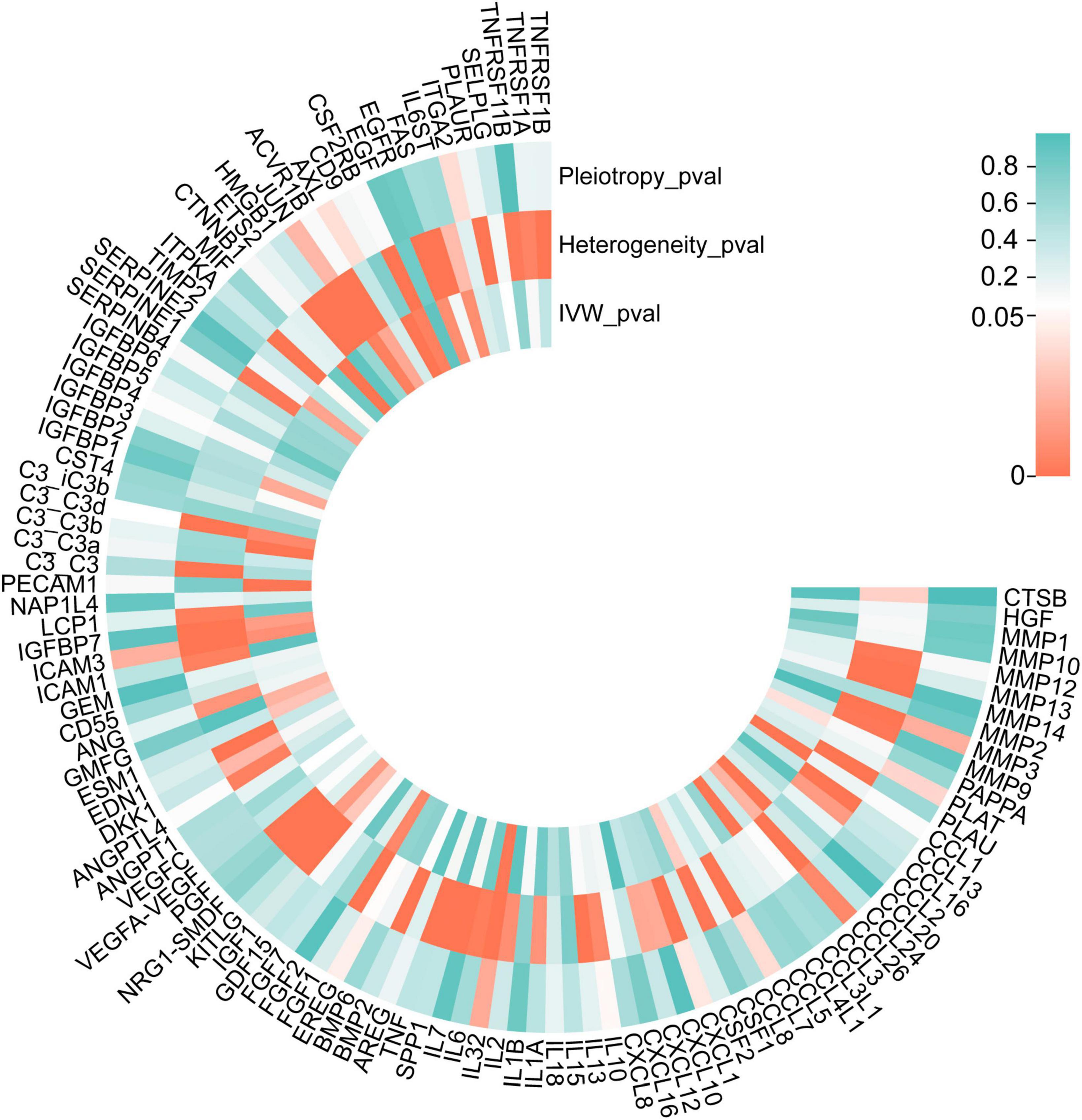
Figure 4. P-values of IVW, heterogeneity test, and pleiotropy test of SRPs and AMD risk in discovery stage. MR: Mendelian randomization; SRPs, senescence-related proteins; AMD, age-related macular degeneration; IVW, inverse-variance weighted.
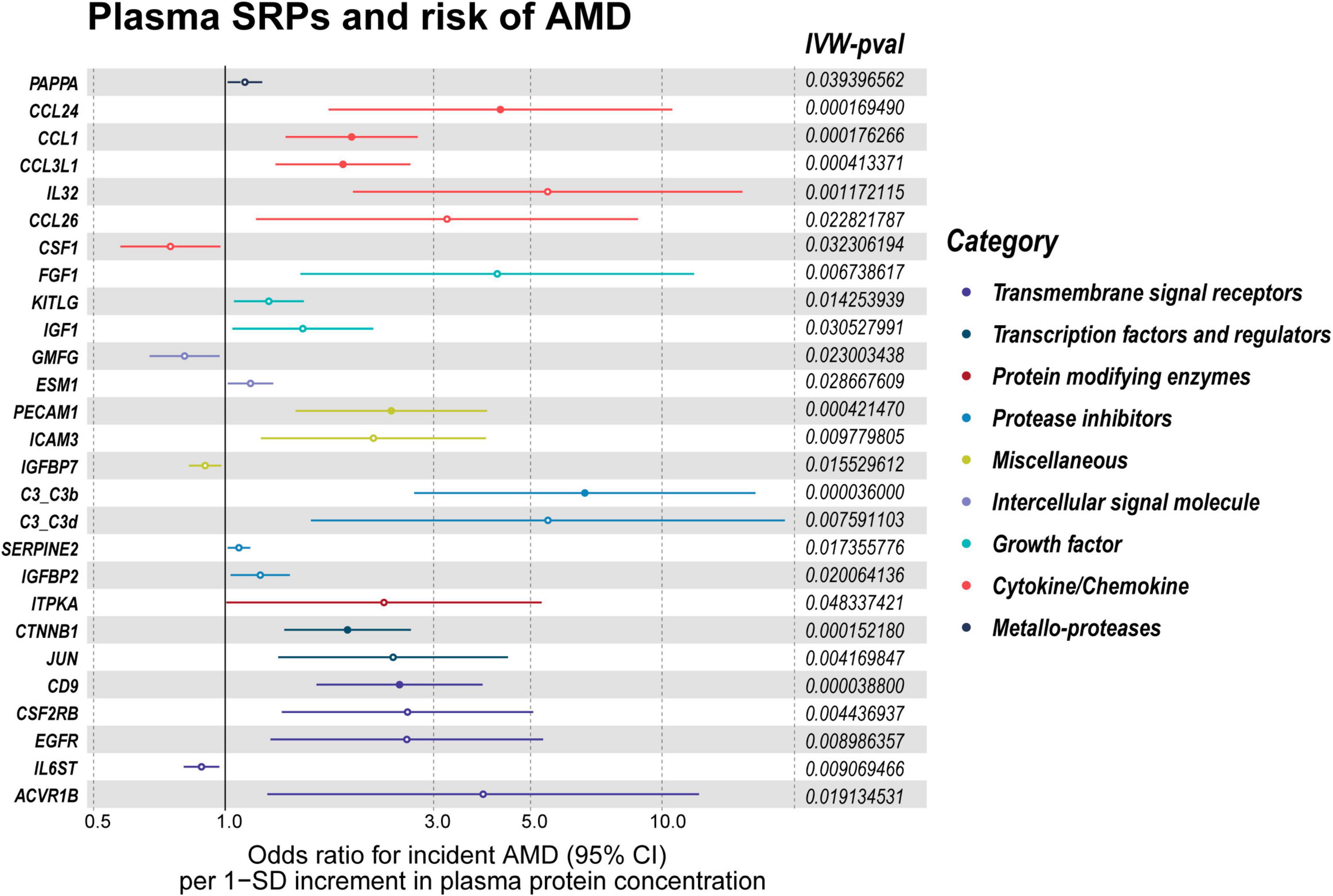
Figure 5. Forest plot of the MR results in discovery stage. MR: Mendelian randomization; SRPs, senescence-related proteins; AMD, age-related macular degeneration; IVW, inverse-variance weighted. P < 0.05 was considered nominally significant and P < 0.00045 was considered statistically significant.
3.2 MR results in the validation stage
In the validation stage, based on MR results, 18 SRPs were suggestively associated and 5 SRPs were significantly associated with risk of AMD (Figures 6, 7; Supplementary Table 4). The 5 SRPs significantly associated with AMD risk were: CCL1 (OR = 1.012, 95% CI: 1.007–1.018, IVW-P = 3.44E-6), CCL3L1 (OR = 1.012, 95% CI: 1.006–1.018, IVW-P = 3.1E-5), CTNNB1 (OR = 1.012, 95% CI: 1.006–1.018, IVW-P = 3.89E-5), CSF2RB (OR = 1.019, 95% CI: 1.009–1.029, IVW-P = 8.18E-5), C3b (OR = 1.024, 95% CI: 1.012–1.036, IVW-P = 1.37E-4). The MR-Egger intercept test and Cochran’s Q test also suggested no apparent heterogeneity or pleiotropy in these 5 results (Heterogeneity-P > 0.05 and Pleiotropy-P > 0.05). Notably, the effect of C3b, CCL1, CCL3L1, and CTNNB1 on the risk of AMD was validated in this stage. The related Gene Ontology (GO) annotations and related diseases of these 4 proteins were showed in Table 2.
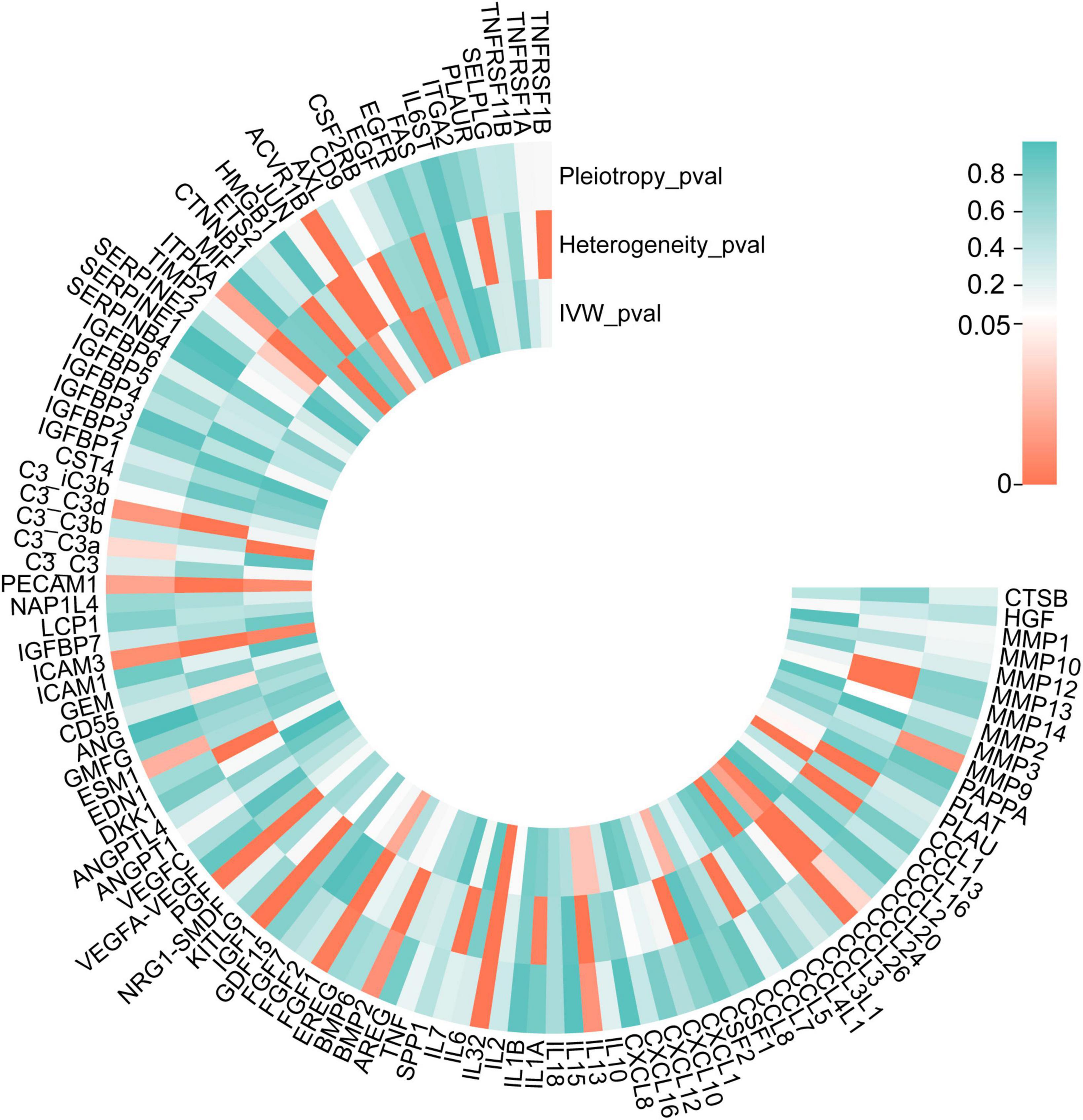
Figure 6. P-values of IVW, heterogeneity test and pleiotropy test of SRPs and AMD risk in validation stage. MR: Mendelian randomization; SRPs, senescence-related proteins; AMD, age-related macular degeneration; IVW, inverse-variance weighted.
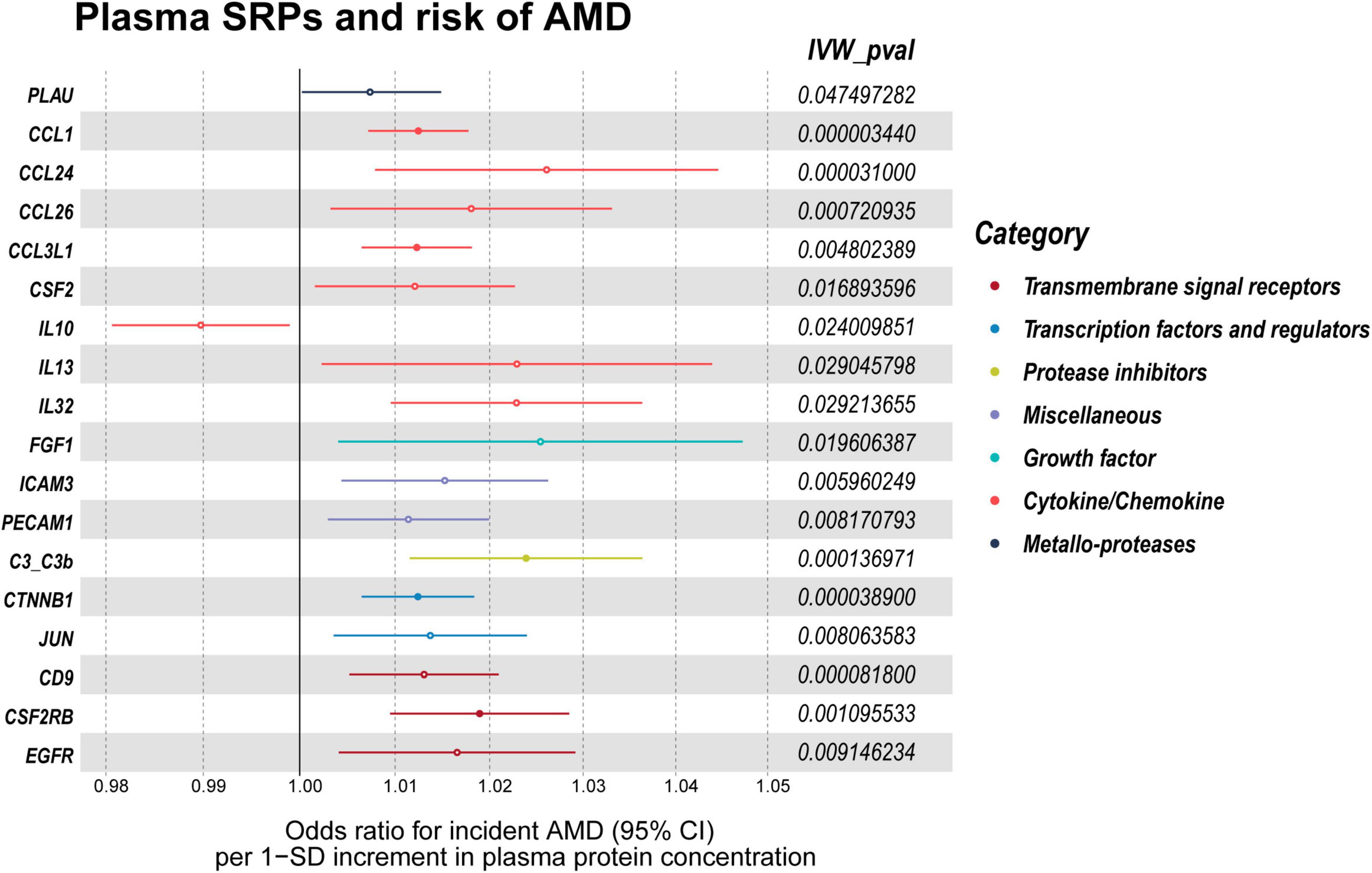
Figure 7. Forest plot of the MR results in validation stage. MR: Mendelian randomization; SRPs, senescence-related proteins; AMD, age-related macular degeneration; IVW, inverse-variance weighted. P < 0.05 was considered nominally significant and P < 0.00045 was considered statistically significant.
4 Discussion
Age-related macular degeneration is a neurodegenerative disease predominantly affecting the elders, which can cause vision loss and has a significant impact on the quality of life of affected individuals (Wood et al., 2011). Identifying systemic risk factors for AMD is important for preventing development of this disease. Therefore, this study aimed to explore the relationship between plasma SRPs and AMD risk by two-sample MR analysis, which is a useful tool for assessing and determining clinically associated characters for multiple diseases (Davey Smith and Hemani, 2014). As a results, high plasma levels of 4 SRPs (C3b, CCL1, CCL3L1, and CTNNB1) were identified to be associated with increased risk of AMD.
Blood supply of the retina consists of retinal microcirculatory system and the underlying choriocapillaris (Funk, 1997). Notably, systemic inflammation has been associated with the development of AMD (Sannan, 2023). In this study, the identified 4 SRPs associated with AMD risk are tightly associated with inflammation processes, which further highlighted the important role of systemic inflammation and the high plasma levels of inflammatory mediators in the pathogenesis of AMD.
Complement C3 is a gene that plays a crucial role in the activation of the complement cascade and major effector functions of complement are mediated through C3b (Haapasalo and Meri, 2019). In AMD, the injury of blood-retinal barrier allows leakage of serum proteins, including complement components, into the retina from the underlying choriocapillaris (Katschke et al., 2018). Local complement activation leads to the recruitment of microglia into the lesion, which then produce additional complement components, prune complement-coated synapses away from neurons, depriving neurons of trophic support, and cause neuroinflammation that adds to neuronal damage and loss (Stephan et al., 2012). The elevated level of plasma C3b may promote the development of AMD by facilitating the neuroinflammation in the retina, which explained the observed association between higher plasma level of C3b and the increased risk of AMD. Notably, C3b has been associated with AMD (Helgason et al., 2013) and taken as the therapeutic target for AMD (Yang et al., 2022; Jia et al., 2023). Yang et al. (2022) reported the preclinical assessment and phase 1 clinical outcomes of a bispecific fusion protein (efdamrofusp alfa), which is capable of neutralizing both VEGF isoforms and C3b/C4b, in neovascular AMD (nAMD) treatment. However, recent studies focused on neutralizing local C3b instead of lowering circulating level of it. Considering the identified causal effect of C3b on AMD risk in this study, circulating C3b lowering strategy might be a potential way for reducing AMD risk, which calls for further studies.
The other three AMD-associated proteins identified in this study may also contribute to the development of AMD through the induction of neuroinflammation in the retina. CCL1 belongs to the C-C subfamily of chemokines, which are secreted proteins involved in immunoregulatory and inflammatory processes. It binds to the C-C motif receptor 8 (CCR8). In addition, it has been implicated in various inflammation-associated diseases, including asthma (Hurme et al., 2022), rheumatoid arthritis (White et al., 2013), and multiple sclerosis (Schropp et al., 2023). In asthma, CCL1 is thought to play a role in recruiting inflammatory cells to the airways, leading to airway inflammation and hyperresponsiveness. Targeted drug discovery efforts have focused on developing drugs that can block the interaction between CCL1 and its receptor, with the aim of reducing inflammation and disease progression (Connolly et al., 2012). CCL3L1 encodes a protein that binds to several chemokine receptors, including CCR5 (Urban et al., 2009). CCL3L1 has also been implicated in inflammatory diseases such as rheumatoid arthritis (Nordang et al., 2012). CTNNB1 encodes a protein that is part of a complex of proteins that make up adherens junctions (AJs) (van der Wal and van Amerongen, 2020), which are essential for creating and maintaining epithelial cell layers by regulating cell growth and adhesion between cells. In addition, CTNNB1 is related to cholesterol homeostasis (Chen et al., 2023), which might also be the mechanical basis of the association between plasma CTNNB1 level and AMD risk.
There were some limitations in this MR-designed investigation, despite its many advantages over conventional epidemiological studies. First, this study only included European-ancestry individuals, which suggests that our findings cannot be directly applied to other populations. Second, our findings only revealed the causality between plasma levels of several SRPs and AMD, not the underlying mechanisms, which call for further researches.
We obtained druggable genes from the DGIdb database (Freshour et al., 2021). Importantly, most of the SRGs are druggable genes, including the four genes identified as risk factors for AMD, i.e., C3b, CCL1, CCL3L1, and CTNNB1. Therefore, our study may provide some novel potential drug targets for AMD or the rationale of existing drugs. Still, the results need to be confirmed by further studies and it is hoped that these 4 SRPs will serve as new drug targets for preventing the incidence of AMD.
In conclusion, we provided the genetic evidence that plasma levels of C3b, CTNNB1, CCL1, and CCL3L1 are causally associated with risk of AMD, which highlights the role of systemic inflammation in the pathophysiology of AMD. Given that the genes encoding these four proteins are all druggable targets, the findings may contribute to understanding the pathogenesis of AMD and the development of new therapeutic or preventive strategy for AMD.
Data availability statement
The original contributions presented in this study are included in this article/Supplementary material, further inquiries can be directed to the corresponding author.
Ethics statement
Human subjects or animal subjects were not included in this study. This study used only publicly available, deidentified data from previously published works, making it exempt according to the Wuhan Fourth Hospital Institutional Review Board. Our research adhered to the tenets of the Declaration of Helsinki.
Author contributions
SL: Conceptualization, Data curation, Formal analysis, Investigation, Methodology, Visualization, Writing—original draft. MH: Conceptualization, Data curation, Formal analysis, Methodology, Writing—original draft. ZW: Conceptualization, Methodology, Supervision, Writing—review and editing.
Funding
The author(s) declare that no financial support was received for the research, authorship, and/or publication of this article.
Conflict of interest
The authors declare that the research was conducted in the absence of any commercial or financial relationships that could be construed as a potential conflict of interest.
Publisher’s note
All claims expressed in this article are solely those of the authors and do not necessarily represent those of their affiliated organizations, or those of the publisher, the editors and the reviewers. Any product that may be evaluated in this article, or claim that may be made by its manufacturer, is not guaranteed or endorsed by the publisher.
Supplementary material
The Supplementary Material for this article can be found online at: https://www.frontiersin.org/articles/10.3389/fnagi.2024.1322519/full#supplementary-material
Abbreviations
AMD, age-related macular degeneration; SRGs, senescence-related genes; SRPs, senescence-related proteins; RPE, retinal pigment epithelium; MR, Mendelian randomization; SNP, single nucleotide polymorphism; GWAS, genome-wide association study; RCT, randomized clinical trial; PRESSO, Pleiotropy RESidual Sum and Outlier; IVW, inverse variance-weighted; OR, odds ratio; CI, confidence interval.
Footnotes
References
Blodi, B. A., Domalpally, A., Corkery, E., Osborne, A., Blotner, S., Grzeschik, S. M., et al. (2023). Prevalence of macular atrophy in the MARINA study of ranibizumab versus sham for neovascular age-related macular degeneration. Ophthalmol. Retina 7, 661–671. doi: 10.1016/j.oret.2023.03.004
Bowden, J., Del Greco, M. F., Minelli, C., Zhao, Q., Lawlor, D. A., Sheehan, N. A., et al. (2019). Improving the accuracy of two-sample summary-data Mendelian randomization: moving beyond the NOME assumption. Int. J. Epidemiol. 48, 728–742. doi: 10.1093/ije/dyy258
Burgess, S., Dudbridge, F., and Thompson, S. G. (2016). Combining information on multiple instrumental variables in Mendelian randomization: comparison of allele score and summarized data methods. Stat. Med. 35, 1880–1906. doi: 10.1002/sim.6835
Chen, L., Yang, P., and Curcio, C. A. (2022). Visualizing lipid behind the retina in aging and age-related macular degeneration, via indocyanine green angiography (ASHS-LIA). Eye (Lond. Engl.) 36, 1735–1746. doi: 10.1038/s41433-022-02016-3
Chen, P. C., Hsu, C. P., Wang, S. Y., Wu, T. Y., Lin, Y. J., Chen, Y. T., et al. (2023). miR-194 up-regulates cholesterol 7 alpha-hydroxylase expression via β-catenin signaling and aggravates cholestatic liver diseases. Am. J. Pathol. 193, 755–768.
Choquet, H., Khawaja, A. P., Jiang, C., Yin, J., Melles, R. B., Glymour, M. M., et al. (2022). Association between myopic refractive error and primary open-angle glaucoma: a 2-sample mendelian randomization study. JAMA Ophthalmol. 140, 864–871. doi: 10.1001/jamaophthalmol.2022.2762
Congdon, N., O’Colmain, B., Klaver, C. C., Klein, R., Muñoz, B., Friedman, D. S., et al. (2004). Causes and prevalence of visual impairment among adults in the United States. Arch. Ophthalmol. 122, 477–485.
Connolly, S., Skrinjar, M., and Rosendahl, A. (2012). Orally bioavailable allosteric CCR8 antagonists inhibit dendritic cell, T cell and eosinophil migration. Biochem. Pharmacol. 83, 778–787. doi: 10.1016/j.bcp.2011.12.021
Davey Smith, G., and Hemani, G. (2014). Mendelian randomization: genetic anchors for causal inference in epidemiological studies. Hum. Mol. Genet. 23, R89–R98.
Doolittle, M. L., Saul, D., Kaur, J., Rowsey, J. L., Vos, S. J., Pavelko, K. D., et al. (2023). Multiparametric senescent cell phenotyping reveals targets of senolytic therapy in the aged murine skeleton. Nat. Commun. 14:4587.
Emdin, C. A., Khera, A. V., and Kathiresan, S. (2017). Mendelian Randomization. JAMA 318, 1925–1926.
Farr, J. N. (2023). Skeletal senescence with aging and type 2 diabetes. Endocrinol. Metab. (Seoul, Korea) 38, 295–301.
Ferkingstad, E., Sulem, P., Atlason, B. A., Sveinbjornsson, G., Magnusson, M. I., Styrmisdottir, E. L., et al. (2021). Large-scale integration of the plasma proteome with genetics and disease. Nat. Genet. 53, 1712–1721.
Freshour, S. L., Kiwala, S., Cotto, K. C., Coffman, A. C., McMichael, J. F., Song, J. J., et al. (2021). Integration of the drug-gene interaction database (DGIdb 4.0) with open crowdsource efforts. Nucleic Acids Res. 49, D1144–D1151. doi: 10.1093/nar/gkaa1084
Haapasalo, K., and Meri, S. (2019). Regulation of the complement system by pentraxins. Front. Immunol. 10:1750. doi: 10.3389/fimmu.2019.01750
Helgason, H., Sulem, P., Duvvari, M. R., Luo, H., Thorleifsson, G., Stefansson, H., et al. (2013). A rare nonsynonymous sequence variant in C3 is associated with high risk of age-related macular degeneration. Nat. Genet. 45, 1371–1374.
Hemani, G., Zheng, J., Elsworth, B., Wade, K. H., Haberland, V., Baird, D., et al. (2018). The MR-Base platform supports systematic causal inference across the human phenome. Elife 7:e34408. doi: 10.7554/eLife.34408
Holloway, K., Neherin, K., Dam, K. U., and Zhang, H. (2023). Cellular senescence and neurodegeneration. Hum. Genet. 142, 1247–1262.
Hurme, P., Komulainen, M., Tulkki, M., Leino, A., Rückert, B., Turunen, R., et al. (2022). Cytokine expression in rhinovirus- vs. respiratory syncytial virus-induced first wheezing episode and its relation to clinical course. Front. Immunol. 13:1044621. doi: 10.3389/fimmu.2022.1044621
Jia, H., Li, T., Sun, J., Gong, Y., Liu, H., Wang, H., et al. (2023). A novel bispecific fusion protein targeting C3b/C4b and VEGF in patients with nAMD: a randomized, open-label, phase 1b study. Am. J. Ophthalmol. 248, 8–15. doi: 10.1016/j.ajo.2022.11.016
Katschke, K. J. Jr., Xi, H., Cox, C., Truong, T., Malato, Y., Lee, W. P., et al. (2018). Classical and alternative complement activation on photoreceptor outer segments drives monocyte-dependent retinal atrophy. Sci. Rep. 8:7348.
Kauppinen, A., Paterno, J. J., Blasiak, J., Salminen, A., and Kaarniranta, K. (2016). Inflammation and its role in age-related macular degeneration. Cell. Mol. Life Sci. 73, 1765–1786.
Kurki, M. I., Karjalainen, J., Palta, P., Sipilä, T. P., Kristiansson, K., Donner, K. M., et al. (2023). FinnGen provides genetic insights from a well-phenotyped isolated population. Nature 613, 508–518.
Lawlor, D. A., Harbord, R. M., Sterne, J. A., Timpson, N., and Davey Smith, G. (2008). Mendelian randomization: using genes as instruments for making causal inferences in epidemiology. Stat. Med. 27, 1133–1163.
Nordang, G. B., Carpenter, D., Viken, M. K., Kvien, T. K., Armour, J. A., and Lie, B. A. (2012). Association analysis of the CCL3L1 copy number locus by paralogue ratio test in Norwegian rheumatoid arthritis patients and healthy controls. Genes Immun. 13, 579–582. doi: 10.1038/gene.2012.30
Patasova, K., Khawaja, A. P., Tamraz, B., Williams, K. M., Mahroo, O. A., Freidin, M., et al. (2021). Association between medication-taking and refractive error in a large general population-based cohort. Invest. Ophthalmol. Vis. Sci. 62:15. doi: 10.1167/iovs.62.2.15
Sannan, N. S. (2023). Assessment of aggregate index of systemic inflammation and systemic inflammatory response index in dry age-related macular degeneration: a retrospective study. Front. Med. (Lausanne) 10:1143045. doi: 10.3389/fmed.2023.1143045
Saul, D., Kosinsky, R. L., Atkinson, E. J., Doolittle, M. L., Zhang, X., LeBrasseur, N. K., et al. (2022). A new gene set identifies senescent cells and predicts senescence-associated pathways across tissues. Nat. Commun. 13:4827. doi: 10.1038/s41467-022-32552-1
Schropp, V., Chunder, R., Dietel, B., Tacke, S., and Kuerten, S. (2023). The presence of cerebellar B cell aggregates is associated with a specific chemokine profile in the cerebrospinal fluid in a mouse model of multiple sclerosis. J. Neuroinflammation 20:18. doi: 10.1186/s12974-023-02695-z
Staley, J. R., Blackshaw, J., Kamat, M. A., Ellis, S., Surendran, P., Sun, B. B., et al. (2016). PhenoScanner: a database of human genotype-phenotype associations. Bioinformatics 32, 3207–3209.
Stephan, A. H., Barres, B. A., and Stevens, B. (2012). The complement system: an unexpected role in synaptic pruning during development and disease. Annu. Rev. Neurosci. 35, 369–389.
Sudlow, C., Gallacher, J., Allen, N., Beral, V., Burton, P., Danesh, J., et al. (2015). UK biobank: an open access resource for identifying the causes of a wide range of complex diseases of middle and old age. PLoS Med. 12:e1001779. doi: 10.1371/journal.pmed.1001779
Urban, T. J., Weintrob, A. C., Fellay, J., Colombo, S., Shianna, K. V., Gumbs, C., et al. (2009). CCL3L1 and HIV/AIDS susceptibility. Nat. Med. 15, 1110–1112.
van der Wal, T., and van Amerongen, R. (2020). Walking the tight wire between cell adhesion and WNT signalling: a balancing act for β-catenin. Open Biol. 10:200267.
White, G. E., Iqbal, A. J., and Greaves, D. R. (2013). CC chemokine receptors and chronic inflammation–therapeutic opportunities and pharmacological challenges. Pharmacol. Rev. 65, 47–89. doi: 10.1124/pr.111.005074
Wong, W. L., Su, X., Li, X., Heung, C. M., Klein, R., Cheng, C. Y., et al. (2014). Global prevalence of age-related macular degeneration and disease burden projection for 2020 and 2040: a systematic review and meta-analysis. Lancet Glob. Health 2, e106–e116. doi: 10.1016/S2214-109X(13)70145-1
Wood, J. M., Lacherez, P., Black, A. A., Cole, M. H., Boon, M. Y., and Kerr, G. K. (2011). Risk of falls, injurious falls, and other injuries resulting from visual impairment among older adults with age-related macular degeneration. Invest. Ophthalmol. Vis. Sci. 52, 5088–5092.
Wykoff, C. C., Brown, D. M., Reed, K., Berliner, A. J., Gerstenblith, A. T., Breazna, A., et al. (2023). Effect of high-dose intravitreal aflibercept, 8 mg, in patients with neovascular age-related macular degeneration: the phase 2 CANDELA randomized clinical trial. JAMA Ophthalmol. 141, 834–842. doi: 10.1001/jamaophthalmol.2023.2421
Yang, S., Li, T., Jia, H., Gao, M., Li, Y., Wan, X., et al. (2022). Targeting C3b/C4b and VEGF with a bispecific fusion protein optimized for neovascular age-related macular degeneration therapy. Sci. Transl. Med. 14:eabj2177. doi: 10.1126/scitranslmed.abj2177
Zheng, J., Baird, D., Borges, M. C., Bowden, J., Hemani, G., Haycock, P., et al. (2017). Recent developments in mendelian randomization studies. Curr. Epidemiol. Rep. 4, 330–345.
Keywords: age-related macular degeneration, senescence, Mendelian, biomarker, drug target
Citation: Lei S, Hu M and Wei Z (2024) Identification of systemic biomarkers and potential drug targets for age-related macular degeneration. Front. Aging Neurosci. 16:1322519. doi: 10.3389/fnagi.2024.1322519
Received: 23 October 2023; Accepted: 15 January 2024;
Published: 01 February 2024.
Edited by:
Yiguo Qiu, Dana–Farber Cancer Institute, United StatesReviewed by:
Vivekananda Budamagunta, Nautilus Biotechnology, United StatesHsueh-Te Lee, National Yang Ming Chiao Tung University, Taiwan
Copyright © 2024 Lei, Hu and Wei. This is an open-access article distributed under the terms of the Creative Commons Attribution License (CC BY). The use, distribution or reproduction in other forums is permitted, provided the original author(s) and the copyright owner(s) are credited and that the original publication in this journal is cited, in accordance with accepted academic practice. No use, distribution or reproduction is permitted which does not comply with these terms.
*Correspondence: Zhongtao Wei, d2Vpemhvbmd0YW8yMDIzQDEyNi5jb20=
†These authors have contributed equally to this work