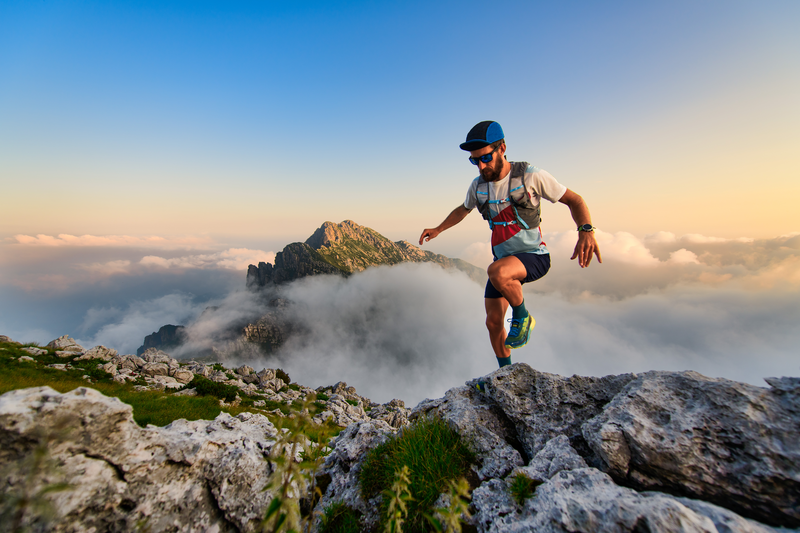
95% of researchers rate our articles as excellent or good
Learn more about the work of our research integrity team to safeguard the quality of each article we publish.
Find out more
ORIGINAL RESEARCH article
Front. Aging Neurosci. , 11 January 2024
Sec. Alzheimer's Disease and Related Dementias
Volume 15 - 2023 | https://doi.org/10.3389/fnagi.2023.1326747
Background: Amyloid beta (Aβ) plaque density was examined in the amygdala of rhesus macaques, to elucidate the influence of age, diet and hormonal environment.
Methods: Luminex technology was used to measure cerebrospinal fluid (CSF) concentrations of Aβ40 and Aβ42 across three decades, while immunohistochemistry was used to examine Aβ plaque density in the amygdala.
Results: Aβ40 was found to be the predominant isoform of Aβ in the CSF, but neither Aβ40 or Aβ42 concentrations showed an age-related change, and the ratio of Aβ42 to Aβ40 showed only a marginal increase. Significantly fewer Aβ plaques were detected in the amygdala of old ovariectomized animals if they received estradiol HRT (p < 0.001); similar results were obtained regardless of whether they had been maintained on a regular monkey chow for ∼48 months or on a high-fat, high-sugar, Western-style diet for ∼30 months.
Conclusion: The results demonstrate that HRT involving estrogen can reduce Aβ plaque load in a cognitive brain region of aged non-human primates. The results from this translational animal model may therefore have clinical relevance to the treatment of AD in post-menopausal women, whether used alone, or as a supplement to current pharmacological and monoclonal antibody-based interventions.
Amyloid beta protein (Aβ) has long been considered a pathological marker of Alzheimer’s disease (AD) and related dementias, and is generally found in pathological plaque deposits as two major forms, Aβ40 and Aβ42 (De-Paula et al., 2012; Alzheimer’s Dement, 2023). However, many subjects with extensive Aβ plaques do not show obvious dementia (Chételat et al., 2011, 2012). Consequently, it is unclear if Aβ plaques play a causal role in triggering AD or if they simply reflect a secondary response to damage and/or inflammation resulting from insults, such as bacterial/viral infections and pollutants. Nevertheless, there is much support for an amyloid cascade hypothesis, which postulates that abnormal accumulation of Aβ plaques within the brain initiates a sequence of events that ultimately lead to AD dementia (Karran et al., 2011; Selkoe and Hardy, 2016; Tolar et al., 2020). Based on this hypothesis many pharmaceutical interventions have targeted reduction of Aβ, or facilitation of its clearance, but most have failed in Phase III clinical trials. More encouragingly, antibody-based interventions have efficacy at reducing Aβ accumulation, and have even received fast-track FDA-approval (Sevigny et al., 2016; Tian Hui Kwan et al., 2020; Avgerinos et al., 2021; Decourt et al., 2021; Shi et al., 2022; Mead and Fox, 2023; van Dyck et al., 2023). On the other hand, the long-term safety of these pharmacological and immunological interventions is unclear, nor are individual differences in treatment response. Consequently, there is a need for additional interventions that can help to reduce Aβ load within the brain, especially in those areas involved in cognitive functions such as the prefrontal cortex, hippocampus and amygdala.
One such intervention that has previously been proposed involves estrogen hormone replacement therapy (HRT). This stems from the observation that the risk of developing AD is greater in women than in men (Viña and Lloret, 2010; Laws et al., 2018; Scheyer et al., 2018) and that HRT can improve cognitive function after menopause (Tang et al., 1996; Kawas et al., 1997; Yaffe et al., 1998; Zandi et al., 2002; Yaffe, 2003). On the other hand, some clinical studies have failed to show any beneficial effects of HRT (Seshadri et al., 2001) or have suggested that HRT increases the risk for developing AD, depending on when the HRT is initiated (Shumaker et al., 2003; Shao et al., 2012; Pourhadi et al., 2023). Consequently, the therapeutic potential of supplementary estrogen for AD is unclear as is the contribution of the sex-steroid environment to the development of Aβ plaques. Slow progress in resolving this issue stems partly from a paucity of experimental animal models, other than transgenic rodents, in which to examine environmental factors that contribute to the formation of Aβ plaques. In this regard, the rhesus macaque represents a valuable animal model with more immediate translational potential to the clinic (Messaoudi et al., 2011; Colman, 2018; Stonebarger et al., 2021). Like humans, these long-lived non-human primates (NHPs) show similar brain organization and development. Also, like women, female rhesus macaques show menstrual cycles and eventually undergo menopause (Gilardi et al., 1997; Downs and Urbanski, 2006: Sorwell and Urbanski, 2013; Luna et al., 2020). Furthermore, old rhesus macaques, like elderly humans, progressively express Aβ plaques within the brain (Heilbroner and Kemper, 1990; Martin et al., 1991; Uno, 1993; Sloane et al., 1997; Stonebarger et al., 2020). A major advantage of rhesus macaque studies, however, is that the animals can be maintained under tightly controlled environmental conditions and their brains can be obtained with a zero post-mortem interval.
We previously observed that estradiol hormone replacement therapy (HRT) lowered gene expression of amyloid precursor protein (APP) in the dorsal raphe nucleus of old female rhesus macaques (Bethea et al., 2016) and more recently showed that HRT significantly affects the transcriptome of the amygdala (Cervera-Juanes et al., 2022); specifically, pathway enrichment analysis identified inhibition of neuro-inflammation in the amygdala with HRT, whereas amyloid processing was enriched. This suggests that HRT may exert a protective effect against neuro-inflammation and Aβ deposition within cognitive brain regions such as the amygdala, and could explain why old ovariectomized rhesus macaques show cognitive benefits when subjected to estradiol HRT (Rapp et al., 2003; Tinkler and Voytko, 2005; Lacreuse, 2006; Voytko et al., 2008; Kohama et al., 2016).
Consequently, the aim of the present study was to examine age-related development of Aβ plaques in the rhesus macaque brain; specifically, in the amygdala, a brain area that is rich in estrogen receptors and plays an important role in learning and memory (Shughrue et al., 1997; Pau et al., 1998; Gundlah et al., 2000). In addition, we set out to test the hypothesis that estrogen HRT can beneficially affect amygdala Aβ plaque density in “surgically menopausal” old females (i.e., old rhesus macaques that had undergone ovariectomy). To address these aims, we performed the following series of related studies, using archived tissues from previous unrelated rhesus macaque studies: (1) Measurement of Aβ40 and Aβ42 concentrations in the cerebral spinal fluid (CSF) to see if they reliably show age-related changes; (2) Identification of the stage of life at which Aβ plaques begin to develop within the amygdala; and (3) Examination of the effect of estradiol HRT on amygdala Aβ plaque density in old ovariectomized females, either maintained on a regular monkey chow or fed a high-fat high-sugar Western-style diet (WSD). Preliminary results were recently presented in abstract form (Urbanski et al., 2022; Appleman et al., 2023).
Cerebrospinal fluid (CSF) and brain tissue was collected at necropsy from rhesus macaques (Macaca mulatta) that had been involved in various unrelated Institutional Animal Care and Use Committee approved research projects. Previously, the animals had been maintained on photoperiods comprising 12 h light and 12 h of darkness per day, and cared for in accordance with National Research Council’s Guide for the Care and Use of Laboratory Animals. Euthanasia was performed following an established protocol recommended by the American Veterinary Medical Association Guidelines for the Euthanasia of Animals, and post-mortem tissues, including CSF, were subsequently obtained through the ONPRC Tissue Distribution Program.
In Experiment 1, CSF was collected at necropsy from the cisterna magna, from 44 animals (males and females), aged 8–31 years. The samples were snap frozen in liquid nitrogen, stored at −80°C, and subsequently assayed for Aβ40 and Aβ42 by Myriad RBM (Austin, TX, USA) using Luminex technology and Human CustomMAP (HMPC109). Both Aβ40 and Aβ42, concentrations were measured as single determinations. The least detectable doses (LDD) were 0.018 and 0.055 ng/ml, respectively, and the intra-assay coefficients of variation were 6.5 and 4.0%.
At necropsy, brains were flushed with 0.9% saline, and the left temporal lobes dissected and immersion fixed in 4% paraformaldehyde for 5–9 days at 4°C. Next, the tissue blocks were rinsed in 0.1 M phosphate buffer and immersed in cryoprotectant (0.1M phosphate buffer with 10% glycerol and 2% DMSO) for 1–2 days, and then immersed in cryoprotectant containing a higher of glycerol (0.1M phosphate buffer with 20% glycerol and 2% DMSO) for 3–4 days. The tissue blocks were then flash frozen in isopentane at −75°C, and stored at −20°C. Frozen coronal sections (30 μm) were subsequently cut through the amygdala and at least 4 sections (spaced 900 μm apart) from each animal were immunohistochemically stained for Aβ, to serve as biological replicates.
The immunohistochemistry was performed on free-floating amygdala sections using a standard avidin-biotin-peroxidase procedure (VECTASTAIN ABC kit; Vector Laboratories, Burlingame, CA, USA) and 3,3’-diaminobenzadine tetrahydrochloride (Sigma-Aldrich, St. Louis, MO, USA) as the chromogen. Importantly, the procedure involved incubation of sections with one of two widely used primary mouse monoclonal antibodies against Aβ, either 10D5 (Creative Biolabs, Shirley, NY, USA) or 4G8 (Biolegend, San Diego, CA, USA), at a concentration of 1:5,000 for ∼48 h at 4°C. In both cases, goat anti-mouse IgG-biotin was used as the secondary antibody (Jackson ImmunoResearch, West Grove, PA, USA).
In Experiment 2, Aβ antibody 10D5 was used to immunohistochemically stain amygdala sections from 16 adult males and females, with 4 representatives from each of the following age groups: (1) < 9 years, (2) 10–19 years, (3) 20–29 years, and (4) > 30 years.
In Experiment 3, Aβ antibody 4G8 was used to immunohistochemically stain amygdala sections from 6 young (8–15 years) and 6 old (23–28 years) males that had previously served as controls in an unrelated study (Urbanski, 2017).
In Experiment 4, Aβ antibody 4G8 was used to immunohistochemically stain amygdala sections from 12 ovariectomized females, aged 20–28 years, that had been maintained on a regular monkey chow diet. As previously described (Kohama et al., 2016), all of the animals had been ovariectomized approximately 48 months prior to necropsy and 5 of them received immediate estradiol hormone replacement therapy (HRT) in the form of subcutaneous elastomer implants; the implants were replaced every few months so as to maintain serum estradiol concentrations at levels slightly above those typically observed during the late follicular phase of the menstrual cycle (Downs and Urbanski, 2006; Sorwell and Urbanski, 2013).
In Experiment 5, Aβ antibody 4G8 was used to immunohistochemically stain amygdala sections from 14 ovariectomized females, aged 20–23 years, that had been maintained on a high-fat, high-sugar Western-style diet (WSD), starting at the time of surgery and continuing until time of necropsy approximately 30 months later (Urbanski et al., 2017; Purnell et al., 2019). Five of these animals began receiving estradiol HRT immediately in the form of subcutaneous elastomer implants that were replaced every few months, so as to maintain plasma estradiol concentrations at a mid- to late late-follicular level; in 4 other animals, the estradiol HRT was delayed for 12 months after ovariectomy as part of the original experimental design (Purnell et al., 2019).
In Experiments 2 and 3, four immunohistochemically-labeled coronal amygdala sections were analyzed from each animal, while in Experiments 4 and 5, five sections were analyzed; the selected section were spaced at 900-μm intervals, centered around the central amygdala. The slide-mounted stained sections were scanned using a Leica Aperio AT2 Slide Scanner (Leica Microsystems, Buffalo Grove, IL, USA). Next, the region of interest (ROI) was selected, using QuPath 0.3.2, and exported to NIH Image J 1.53k using the Extensions feature in QuPath. In Image J, the amygdala was carefully outlined using the Polygon Tool and transformed to an 8-bit, black and white image; the threshold of this image was adjusted until the amyloid plaques became visible. Analysis of the particles was then performed using a particle size threshold set above 250 pixels; this enabled the detection of Aβ plaque density without interference of cellular membrane-bound amyloid precursor protein. The pixel area of each plaque in the amygdala region was summed to calculate the total area covered by plaques; this result was then multiplied by 100 and divided by the total area of the amygdala to obtain the percentage of the amygdala area covered by Aβ plaques. This was then averaged across the amygdala sections from each animal.
Results were analyzed using GB-STAT software (Dynamic Microsysyems, Silverspring, MD, USA). Spearman’s correlation was used to analyze relationships between the following: (1) Aβ40 and Aβ42 concentrations; (2) age and Aβ40 concentrations; (3) age and Aβ42 concentrations; and (4) age and Aβ42 to Aβ40 concentration ratios. Mean concentrations of Aβ40 and Aβ42 in the CSF were compared using paired Student’s t-test, while group means in experiments involving immunohistochemistry were compared using unpaired Student’s t-test.
Concentrations of Aβ40 and Aβ42 in the CSF were significantly (p < 0.001) correlated but showed increased divergence at higher concentrations (Figure 1A). Although no obvious age-related increase or decrease in CSF concentrations was detected in either form of Aβ (Figures 1B, C), the mean concentration of Aβ40 was significantly (p < 0.001) higher than that of Aβ42. The CSF concentration ratio of Aβ42 to Aβ40 showed only a marginally significant (p = 0.049), age-related increase (Figure 1D).
Figure 1. Scatterplot of amyloid beta (Aβ) concentration in the cerebral spinal fluid (CSF) of rhesus macaques. Males and females are represented by filled and open circles, respectively, and red lines depict linear regressions. A significant (p < 0.001) correlation between CSF concentrations of Aβ40 and Aβ42 (A). No significant age-related increase in CSF concentrations of Aβ40 (B) or Aβ42 (C). A marginally significant (p = 0.049) age-related increase in CSF Aβ42 to Aβ40 ratio (D). The lack of a marked increase in the concentration ratio of Aβ42 to Aβ40 in the oldest animals is consistent with the observation that rhesus macaques do not develop full-blown AD during their normal lifespan.
Aβ plaques are usually undetectable in the brains of rhesus macaques before the age of ∼20 years (Uno, 1993). In the present study, no animals younger than 20 years had Aβ plaques that exceed 0.1% of the amygdala area, whereas all of the animals older than 30 years did (Figure 2). Consequently, we considered 0.1% to represent a significant threshold in Aβ plaque development. Moreover, because animals aged 20–29 years showed variable levels of Aβ expression, this age group served as the focus of the subsequent experiments involving manipulation of diet and the sex-steroid environment.
Figure 2. Age-related increase in the expression of amyloid beta (Aβ) plaques in the rhesus macaque amygdala. Each bar represents the mean percentage of males and females (n = 4, for each cohort) showing Aβ density greater than 0.1% at different decades of life. None of the animals younger than 20 years showed a significant number of Aβ plaques, whereas all of the animals older than 30 years of age did. Animals aged 20–29 years showed variable levels of Aβ expression, and so this age group served as the focus of the subsequent studies.
Firstly, we wanted to corroborate the age-related increase in Aβ plaque density, and so we examined Aβ plaque density in another cohort of animals, comprising 6 young (8–15 years) and 6 old (23–28 years) males, taking advantage of available archived brain tissue from an unrelated study. The immunohistochemistry in this and all subsequent experiments utilized an alternate widely used Aβ primary antibody (4G8), instead of 10D5. As expected, none of the young males showed a significant number of Aβ plaques in the amygdala. In contrast, 50% of the older animals showed some plaques but in only one animal did the plaque density occupy > 0.1% of the amygdala area (Figures 3A, B).
Figure 3. Immunohistological staining of amyloid beta (Aβ) plaques in the amygdala of young and old gonad-intact male rhesus macaques. Examples are depicted from (A) an 11-year-old and (B) a 23-year-old animal, while being maintained on a regular monkey chow. Scale bar = 100 μm for all panels; the respective insets show 2x magnified images of the central portion of the amygdala. Taken together with data shown in Figure 5A, the results demonstrate an age-related development of amyloid beta (Aβ) plaques in the amygdala.
Examples of positive Aβ immunohistological staining in the amygdala of aged ovariectomized female rhesus macaques are shown in Figure 4. Regardless of dietary manipulation (i.e., standard monkey chow or WSD), there was a clear difference in Aβ plaque density observed in ovariectomized (Ovx) animals compared to those receiving estradiol HRT (Ovx + E) (Figures 4A, C vs. Figures 4B, D). More than half of the Ovx animals in both dietary treatment groups showed significant amygdala expression of Aβ plaques, whereas only one Ovx + E animal did (Figures 5B, C), similar to the old males (Figure 5A). Because the Ovx animals from the two dietary studies (i.e., regular diet and WSD) had significantly different post-ovariectomy intervals (i.e., ∼48 months versus ∼30 months) it was not possible to determine with any certainty if the amygdala plaque density was significantly enhanced by a WSD. Overall, however, there appeared to be no obvious effect of diet, and so the data from the two dietary studies were pooled. Despite similarity in mean age between the Ovx and Ovx + E animals (Figure 6A), the density of Aβ plaques was significantly (p < 0.001) lower in the latter group and more similar to that observed in the age-matched gonad-intact males (Figure 6B).
Figure 4. Immunohistological staining of amyloid beta (Aβ) plaques in the amygdala of aged ovariectomized female rhesus macaques. Randomized examples are depicted from (A) an ovariectomized (Ovx) animal and (B) an ovariectomized estrogen treated (Ovx + E) animal maintained on a regular monkey chow. Randomized examples are also depicted from (C) an ovariectomized (Ovx) animal and (D) an ovariectomized estrogen treated (Ovx + E) animal maintained on a high fat, high sugar Western-style diet (WSD). Scale bar = 100 μm for all panels; the respective insets show 2x magnified images of the central portion of the amygdala. Taken together with data shown in Figures 5B, C, the results demonstrate a significant effect of estradiol on amyloid beta (Aβ) plaque density in the amygdala.
Figure 5. Amyloid beta (Aβ) plaque density in the amygdala of young and old male rhesus macaques maintained on a regular monkey chow (A), as well as in the amygdala of aged ovariectomized females (B) and aged ovariectomized females that were maintained on a Western-style diet (WSD) (C). Each data point represents the mean value from individuals. Data from young (Y-male) and old (O-male) males are depicted as open and black squares, respectively. Untreated ovariectomized (Ovx) animals are depicted by open circles, while data from ovariectomized animals that were subjected to estrogen HRT (Ovx + E) are depicted either by black or gray circles; in the latter case, the estrogen replacement was delayed for 12 months after ovariectomy and start of maintenance on a WSD. None of the young males showed any amygdala Aβ plaques, but 50% of the old males did; however, the density of these plaques was markedly lower than in the age-matched Ovx females. Overall, 67% of the Ovx controls showed amygdala Aβ plaque density equal or greater than 0.1%, compared to only 7% for the Ovx + E animals, thereby suggesting that loss of estrogens may play a role in promoting age-related development of Aβ plaques.
Figure 6. Effect of HRT on amyloid beta (Aβ) plaque density in the amygdala of aged ovariectomized female rhesus macaques; pooled data are shown from animals that were maintained on either the regular monkey chow or WSD. For reference, data from age-matched gonad-intact males (O-male) are also depicted. Mean values are represented by bars, with the number of animals per group indicated at the base of each bar; SEMs are represented by vertical lines. (A) The mean age of animals in the ovariectomized (Ovx) control group was not statistically (NS) different from that of the ovariectomized animals that were subjected to estrogen HRT (Ovx + E). (B) The mean Aβ plaque density was significantly lower in the amygdala of HRT treated ovariectomized (Ovx + E) animals compared to the untreated ovariectomized (Ovx) controls. ***p < 0.001, Student’s t-test. The results show that loss of estrogens likely contributes to increased Aβ plaque denisity in the amygdala, and that estradiol HRT has the potential to attenuate this increase.
Alzheimer’s disease (AD) is the most common form of dementia, affecting about 6.7 million people in the USA aged over 65 years; it also remains the fifth-leading cause of death among this age group (2023). And yet, there is still no effective cure for AD. Some pharmaceutical interventions, such as donepezil, provide temporary relief from AD symptoms, by blocking the breakdown of acetylcholine (Marucci et al., 2021; Rong et al., 2021). While others, such as memantine, regulate the activity of the excitatory neurotransmitter glutamate (McKeage, 2009; Kishi et al., 2017; Guo et al., 2020). However, none of these interventions has been shown to effectively retard the progression of the disease. More recently, aducanumab and lecanemab, two monoclonal antibody-based therapies designed to decrease the amount of Aβ plaques in the brain have become available (Sevigny et al., 2016; Shi et al., 2022; Mead and Fox, 2023; van Dyck et al., 2023). However, their effectiveness at improving cognitive function remains unclear and there is concern about potential negative side effects (Tian Hui Kwan et al., 2020; Avgerinos et al., 2021; Decourt et al., 2021).
The main goal of the present study was to investigate the therapeutic potential of endocrine-based interventions at reducing the amount of Aβ plaques in the brain, namely, estradiol-based hormone replacement therapy (HRT). Because of the conflicting evidence from clinical studies regarding the beneficial effects of estrogens at reducing the risk of AD (Tang et al., 1996; Kawas et al., 1997; Yaffe et al., 1998; Seshadri et al., 2001; Zandi et al., 2002; Shumaker et al., 2003; Yaffe, 2003; Shao et al., 2012; Pourhadi et al., 2023), we focused our studies on a pragmatic translational non-human primate model of aging.
A more proximate goal, however, was to establish if development of Aβ plaques within the brain follows a similar time course in both rhesus macaques and humans. To do this, we first examined CSF concentrations of Aβ across the first three decades of the rhesus macaque life. As expected, based on previous non-human primate studies (Gearing et al., 1996), we found the concentration of Aβ40 in the monkey CSF to be significantly higher than that of Aβ42, and agreeing with clinical studies that established Aβ40 to be the predominant isoforms of Aβ in humans (Gu and Guo, 2013; Dumurgier et al., 2015; Lehmann et al., 2018). Unlike AD patients (Fukumoto et al., 1996), however, the CSF concentration of Aβ42 or the concentration ratio of Aβ42 to Aβ40 in the oldest rhesus macaques did not show a marked increase. This finding is consistent with the observation that rhesus macaques do not develop full-blown AD during their normal lifespan, despite clearly showing an age-dependent increase in Aβ plaque density and showing mild age-related cognitive decline (without neuronal loss) (Stonebarger et al., 2020, 2021).
To test whether HRT could offer protection against development of Aβ plaques in an estrogen receptor rich part of the brain (Shughrue et al., 1997; Pau et al., 1998; Gundlah et al., 2000), we used a surgically menopausal animal model. Although female rhesus macaques clearly undergo menopause during old age (Gilardi et al., 1997; Downs and Urbanski, 2006; Sorwell and Urbanski, 2013; Luna et al., 2020), the exact age of when this occurs is quite variable between animals. In contrast, ovariectomized old (i.e., > 20 years) female rhesus macaques reliably have extremely low circulating levels of estradiol, like those observed after menopause, and thus surgically menopausal animals represent a more precise experimental model. Furthermore, we examined archived brains from two independent studies involving different diets. In one study the animals had been ovariectomized and maintained on a regular monkey chow for ∼48 months, while in the other study ovariectomized animals had been maintained for ∼30 months on a high-fat, high-sugar Western-style diet (WSD); the latter being an attempt to mimic a common diet of many post-menopausal women in the USA. In both cases, Aβ plaque density was greater in ovariectomized animals that did not receive estradiol HRT, although it should be emphasized that this Aβ plaque density was much lower than what is typically observed in the brains of AD patients (Stonebarger et al., 2020, 2021). Furthermore, the attenuated plaque density in the Ovx + E animals more closely resembled the density observed in age-matched, gonad-intact males. Because old males do not show a marked attenuation of estrogen concentrations, compared to post-menopausal females (Downs and Urbanski, 2006; Urbanski et al., 2014), this finding gives further support to the view that a lack of estrogen contributes to increased Aβ plaque density within the brain. Overall, the present results are consistent with findings from previous studies using transgenic mouse models of AD, which showed sex-differences in accumulation of Aβ plaques in the brain due to estrogen and showed that administration of estradiol was associated with lower Aβ peptide levels compared to untreated controls (Tschiffely et al., 2016; Hu et al., 2023). On the other hand, the present study was performed using a long-lived primate that more closely resembles humans in terms of its behavior, anatomy, physiology and endocrinology, and therefore represents a more translational animal model of aging, especially post-menopausal women.
It is unclear from the present experiments if the HRT prevented or delayed formation of the Aβ plaques, increased their clearance, or a combination of both. It is also unclear if a similar result would have been observed had the HRT been initiated several years after the ovariectomy. On the one hand, results from the Women’s Health Initiative study suggest that many benefits of HRT may be lost if it is delayed by several years after the onset of menopause (Lobo et al., 2014). On the other hand, it is encouraging that HRT in our WSD study showed beneficial effects even in individuals in which it had been delayed for 12 months after the ovariectomy.
Taken together, the present results demonstrate that estradiol HRT can significantly reduce Aβ plaque load in the amygdala of surgically menopausal females, even when consuming a WSD. Because of concern about adverse peripheral side effects associated with conventional HRT, it is unclear if a hormonal-based therapies would gain acceptance by post-menopausal women (German-Castelan et al., 2023). One possible way to overcome this potential problem, however, could be to rely on brain-selective prodrugs, such as 10β,17β-dihydroxyestra-1,4-dien-3-one (DHED) (Tschiffely et al., 2016), as alternatives to traditionally used estrogens. Additional research would be required to demonstrate their efficacy and safety, but they may ultimately prove to be more acceptable endocrine approaches to treating or delaying the onset of AD in humans.
The results demonstrate that HRT involving estrogen can reduce Aβ plaque load in a cognitive brain region of aged non-human primates. They may therefore have clinical relevance to the treatment of AD in post-menopausal women, especially if it can be shown that HRT can also reduce Aβ plaque load in the prefrontal cortex and hippocampus. Whether used alone, or as a supplement to pharmacological and monoclonal antibody-based interventions, estrogen-based HRT could represent a more natural intervention aimed at preventing, delaying, or decreasing Aβ pathology within the brain.
The original contributions presented in this study are included in this article, further inquiries can be directed to the corresponding author.
The animal study was approved by the ONPRC Institutional Animal Use and Care Committee. The study was conducted in accordance with the local legislation and institutional requirements.
HU: Conceptualization, Funding acquisition, Writing–original draft. M-LA: Methodology, Writing–review and editing. JT: Methodology, Writing–review and editing. AW: Methodology, Writing–review and editing. BN: Methodology, Writing–review and editing. RC-J: Writing –review and editing. SK: Writing–review and editing.
The author(s) declare financial support was received for the research, authorship, and/or publication of the article. This work was funded by the National Institutes of Health Grants: K01 AG078407, P30 AG066518, P51 OD011092, R24 OD011895, RF1 AG062220 and S10 OD025002.
We would like to acknowledge the contributions of Dr. Sathya Srinivasan in the ONPRC Integrated Pathology Core for his assistance with the image analysis.
The authors declare that the research was conducted in the absence of any commercial or financial relationships that could be construed as a potential conflict of interest.
The author(s) declared that they were an editorial board member of Frontiers, at the time of submission. This had no impact on the peer review process and the final decision.
All claims expressed in this article are solely those of the authors and do not necessarily represent those of their affiliated organizations, or those of the publisher, the editors and the reviewers. Any product that may be evaluated in this article, or claim that may be made by its manufacturer, is not guaranteed or endorsed by the publisher.
AD, Alzheimer’s disease; A β , Amyloid beta; APP, amyloid precursor protein; CSF, cerebrospinal fluid; DMSO, dimethyl sulfoxide; FDA, Food & Drug Administration; HRT, hormone replacement therapy; NHP, non-human primate; ONPRC, Oregon National Primate Research Center; Ovx, ovariectomized; Ovx+E, ovariectomized estrogen treated; WSD, Western-style diet.
Alzheimer’s Dement (2023). Alzheimer’s disease facts and figures. Alzheimer’s Dement. 19, 1598–1695. doi: 10.1002/alz.13016
Appleman, M. L., Lomniczi, A., Nilaver, B., Kohama, S. G., and Urbanski, H. F. (2023). Hormone replacement therapy reduces amyloid beta pathology in aged rhesus monkeys. Endo 2023 abstract #6208.
Avgerinos, K. I., Ferrucci, L., and Kapogiannis, D. (2021). Effects of monoclonal antibodies against amyloid-β on clinical and biomarker outcomes and adverse event risks: A systematic review and meta-analysis of phase III RCTs in Alzheimer’s disease. Ageing Res. Rev. 68:101339. doi: 10.1016/j.arr.2021.101339
Bethea, C. L., Kohama, S. G., Reddy, A. P., and Urbanski, H. F. (2016). Ovarian steroids regulate gene expression in the dorsal raphe of old female macaques. Neurobiol. Aging 37, 179–191. doi: 10.1016/j.neurobiolaging.2015.10.004
Cervera-Juanes, R., Darakjian, P., Ball, M., Kohama, S. G., and Urbanski, H. F. (2022). Effects of estradiol supplementation on the brain transcriptome of old rhesus macaques maintained on an obesogenic diet. GeroScience 44, 229–252. doi: 10.1007/s11357-021-00453-8
Chételat, G., Villemagne, V. L., Pike, K. E., Ellis, K. A., Ames, D., Masters, C. L., et al. (2012). Relationship between memory performance and β-amyloid deposition at different stages of Alzheimer’s disease. Neurodegener. Dis. 10, 141–144. doi: 10.1159/000334295
Chételat, G., Villemagne, V. L., Pike, K. E., Ellis, K. A., Bourgeat, P., Jones, G., et al. (2011). Independent contribution of temporal beta-amyloid deposition to memory decline in the pre-dementia phase of Alzheimer’s disease. Brain 134, 798–807. doi: 10.1093/brain/awq383
Colman, R. J. (2018). Non-human primates as a model for aging. Biochim Biophys Acta Mol. Basis Dis. 1864, 2733–2741. doi: 10.1016/j.bbadis.2017.07.008
Decourt, B., Boumelhem, F., Pope, E. D. 3rd, Shi, J., Mari, Z., and Sabbagh, M. N. (2021). Critical appraisal of amyloid lowering agents in AD. Curr. Neurol. Neurosci. Rep. 21:39. doi: 10.1007/s11910-021-01125-y
De-Paula, V. J., Radanovic, M., Diniz, B. S., and Forlenza, O. V. (2012). Alzheimer’s disease. Subcell. Biochem. 65, 329–352. doi: 10.1007/978-94-007-5416-4_14
Downs, J. L., and Urbanski, H. F. (2006). Neuroendocrine changes in the aging reproductive axis of female rhesus macaques (Macacaca mulatta). Biol. Reprod. 75, 539–546. doi: 10.1095/biolreprod.106.051839
Dumurgier, J., Schraen, S., Gabelle, A., Vercruysse, O., Bombois, S., Laplanche, J. L., et al. (2015). Cerebrospinal fluid amyloid-β 42/40 ratio in clinical setting of memory centers: A multicentric study. Alzheimers Res. Ther. 7:30. doi: 10.1186/s13195-015-0114-5
Fukumoto, H., Asami-Odaka, A., Suzuki, N., Shimada, H., Ihara, Y., and Iwatsubo, T. (1996). Amyloid beta protein deposition in normal aging has the same characteristics as that in Alzheimer’s disease. Predominance of A beta 42(43) and association of A beta 40 with cored plaques. Am. J. Pathol. 148, 259–265.
Gearing, M., Tigges, J., Mori, H., and Mirra, S. S. (1996). A beta40 is a major form of beta-amyloid in nonhuman primates. Neurobiol. Aging 17, 903–908. doi: 10.1016/s0197-4580(96)00164-9
German-Castelan, L., Shanks, H. R. C., Gros, R., Saito, T., Saido, T. C., Saksida, L. M., et al. (2023). Sex-dependent cholinergic effects on amyloid pathology: A translational study. Alzheimer’s Dement. 1–18. doi: 10.1002/alz.13481
Gilardi, K. V., Shideler, S. E., Valverde, C. R., Roberts, J. A., and Lasley, B. L. (1997). Characterization of the onset of menopause in the rhesus macaque. Biol. Reprod. 57, 335–340. doi: 10.1095/biolreprod57.2.335
Gu, L., and Guo, Z. (2013). Alzheimer’s Aβ42 and Aβ40 peptides form interlaced amyloid fibrils. J. Neurochem. 126, 305–311. doi: 10.1111/jnc.12202
Gundlah, C., Kohama, S. G., Mirkes, S. J., Garyfallou, V. T., Urabnski, H. F., and Bethea, C. L. (2000). Distribution of estrogen receptor beta (ERβ) mRNA in hypothalamus, midbrain and temporal lobe of spayed macaque: Continued expression with hormone replacement. Brain Res. Mol. Brain Res. 76, 191–204. doi: 10.1016/s0006-8993(99)02475-0
Guo, J., Wang, Z., Liu, R., Huang, Y., Zhang, N., and Zhang, R. (2020). Memantine, donepezil, or combination therapy: What is the best therapy for Alzheimer’s disease? A network meta-analysis. Brain Behav. 10:e01831. doi: 10.1002/brb3.1831
Heilbroner, P. L., and Kemper, T. L. (1990). The cytoarchitectonic distribution of senile plaques in three aged monkeys. Acta Neuropathol. 81, 60–65. doi: 10.1007/BF00662638
Hu, Y.-T., Chen, X.-L., Zhang, Y.-N., McGurran, H., Stormmesand, J., Breeuwsma, N., et al. (2023). Sex differences in hippocampal β-amyloid accumulation in the triple-transgenic mouse model of Alzheimer’s disease and the potential role of local estrogens. Front. Neurosci. 17:1117584. doi: 10.3389/fnins.2023.1117584
Karran, E., Mercken, M., and De Strooper, B. (2011). The amyloid cascade hypothesis for Alzheimer’s disease: An appraisal for the development of therapeutics. Nature 11, 698–712. doi: 10.1038/nrd3505
Kawas, C., Resnick, S., Morrison, A., Brookmeyer, R., Corrada, M., Zonderman, A., et al. (1997). A prospective study of estrogen replacement therapy and the risk of developing Alzheimer’s disease: The Baltimore Longitudinal Study of Aging. Neurology 48, 1517-1521. Err. Neurol. 51:654. doi: 10.1212/wnl.48.6.1517
Kishi, T., Matsunaga, S., Oya, K., Nomura, I., Ikuta, T., and Iwata, N. (2017). Memantine for Alzheimer’s disease: An updated systematic review and meta-analysis. J. Alzheimers Dis. 60, 401–425. doi: 10.3233/JAD-170424
Kohama, S. G., Renner, L., Landauer, N., Weiss, A. R., Urbanski, H. F., Park, B., et al. (2016). Effect of ovarian hormone therapy on cognition in the aged female rhesus macaque. J. Neurosci. 36, 10416–10424. doi: 10.1523/JNEUROSCI.0909-16.2016
Lacreuse, A. (2006). Effects of ovarian hormones on cognitive function in nonhuman primates. Neuroscience 138, 859–867. doi: 10.1016/j.neuroscience.2005.09.006
Laws, K. R., Irvine, K., and Gale, T. M. (2018). Sex differences in Alzheimer’s disease. Curr. Opin. Psychiatry 31, 133–139. doi: 10.1097/YCO.0000000000000401
Lehmann, S., Delaby, C., Boursier, G., Catteau, C., Ginestet, N., Tiers, L., et al. (2018). Relevance of Aβ42/40 ratio for detection of Alzheimer disease pathology in clinical routine: The PLMR scale. Front. Aging Neurosci. 10:138. doi: 10.3389/fnagi.2018.00138
Lobo, R. A., Davis, S. R., De Villiers, T. J., Gompel, A., Henderson, V. W., Hodis, H. N., et al. (2014). Prevention of diseases after menopause. Climacteric 17, 540–556. doi: 10.3109/13697137.2014.933411
Luna, S. L., Brown, D. I., Kohama, S. G., and Urbanski, H. F. (2020). Lack of effect of short-term DHEA supplementation on the perimenopausal ovary. Biol. Reprod. 103, 1209–1216. doi: 10.1093/biolre/ioaa160
Martin, L. J., Sisodia, S. S., Koo, E. H., Cork, L. C., Dellovade, T. L., Weidemann, A., et al. (1991). Amyloid precursor protein in aged nonhuman primates. Proc. Natl. Acad. Sci. U.S.A. 88, 1461–1465. doi: 10.1073/pnas.88.4.1461
Marucci, G., Buccioni, M., Ben, D. D., Lambertucci, C., Volpini, R., and Amenta, F. (2021). Efficacy of acetylcholinesterase inhibitors in Alzheimer’s disease. Neuropharmacology 190:108352. doi: 10.1016/j.neuropharm.2020.108352
McKeage, K. (2009). Memantine: A review of its use in moderate to severe Alzheimer’s disease. CNS Drugs. 23, 881–897. doi: 10.2165/11201020-000000000-00000
Mead, S., and Fox, N. C. (2023). Lecanemab slows Alzheimer’s disease: Hope and challenges. Lancet Neurol. 22, 106–108. doi: 10.1016/S1474-4422(22)00529-4
Messaoudi, I., Urbanski, H. F., and Kohama, S. G. (2011). “Integrative models of aging in the nonhuman primate,” in Monkeys: Biology, behavior and disorders, ed. R. M. Williams (New York, NY: Nova Science Publishers), 1–54.
Pau, C. Y., Pau, K. Y., and Spies, H. G. (1998). Putative estrogen receptor beta and alpha mRNA expression in male and female rhesus macaques. Mol. Cell Endocrinol. 146, 59–68. doi: 10.1016/s0303-7207(98)00197-x
Pourhadi, N., Mørch, L. S., Holm, E. A., Torp-Pedersen, C., and Meaidi, A. (2023). Menopausal hormone therapy and dementia: Nationwide, nested case-control study. BMJ 381:e072770. doi: 10.1136/bmj-2022-072770
Purnell, J. Q., Urbanski, H. F., Kievit, P., Roberts, C. T., and Bethea, C. L. (2019). Estradiol replacement timing and obesogenic diet effects on body composition and metabolism in postmenopausal macaques. Endocrinology 160, 899–914. doi: 10.1210/en.2018-00884
Rapp, P. R., Morrison, J. H., and Roberts, J. A. (2003). Cyclic estrogen replacement improves cognitive function in aged ovariectomized rhesus monkeys. J. Neurosci. 23, 5708–5714. doi: 10.1523/JNEUROSCI.23-13-05708.2003
Rong, X., Jiang, L., Qu, M., Hassan, S. S. U., and Liu, Z. (2021). Enhancing therapeutic efficacy of donepezil by combined therapy: A comprehensive review. Curr. Pharm. Des. 27, 332–344. doi: 10.2174/1381612826666201023144836
Scheyer, O., Rahman, A., Hristov, H., Berkowitz, C., Isaacson, R. S., Diaz Brinton, R., et al. (2018). Female sex and Alzheimer’s risk: The menopause connection. J. Prev. Alzheimers Dis. 5, 225–230. doi: 10.14283/jpad.2018.34
Selkoe, D. J., and Hardy, J. (2016). The amyloid hypothesis of Alzheimer’s disease at 25 years. EMBO Mol. Med. 8, 595–608. doi: 10.15252/emmm.201606210
Seshadri, S., Zornberg, G. L., Derby, L. E., Myers, M. W., Jick, H., and Drachman, D. A. (2001). Postmenopausal estrogen replacement therapy and the risk of Alzheimer disease. Arch. Neurol. 58, 435–440. doi: 10.1001/archneur.58.3.435
Sevigny, J., Chiao, P., Bussière, T., Weinreb, P. H., Williams, L., Maier, M., et al. (2016). The antibody aducanumab reduces Aβ plaques in Alzheimer’s disease. Nature 537, 50–56. doi: 10.1038/nature19323
Shao, H., Breitner, J. C., Whitmer, R. A., Wang, J., Hayden, K., and Wengreen, H. (2012). Hormone therapy and Alzheimer disease dementia: New findings from the Cache County Study. Neurology 79, 1846–1852. doi: 10.1212/WNL.0b013e318271f823
Shi, M., Chu, F., Zhu, F., and Zhu, J. (2022). Impact of anti-amyloid-β monoclonal antibodies on the pathology and clinical profile of Alzheimer’s disease: A focus on aducanumab and lecanemab. Front. Aging Neurosci. 14:870517. doi: 10.3389/fnagi.2022.870517
Shughrue, P. J., Lane, M. V., and Merchenthaler, I. (1997). Comparative distribution of estrogen receptor-alpha and -beta mRNA in the rat central nervous system. J. Comp. Neurol. 388, 507–525.
Shumaker, S. A., Legault, C., Rapp, S. R., Thal, L., Wallace, R. B., Ockene, J. K., et al. (2003). Estrogen plus progestin and the incidence of dementia and mild cognitive impairment in postmenopausal women: The Women’s Health Initiative Memory Study: A randomized controlled trial. JAMA 289, 2651–2662. doi: 10.1001/jama.289.20.2651
Sloane, J. A., Pietropaolo, M. F., Rosene, D. L., Moss, M. B., Peters, A., Kemper, T., et al. (1997). Lack of correlation between plaque burden and cognition in the aged monkey. Acta Neuropathol. 94, 471–478. doi: 10.1007/s004010050735
Sorwell, K. G., and Urbanski, H. F. (2013). Causes and consequences of age-related steroid hormone changes: Insights gained from nonhuman primates. J. Neuroendocrinol. 25, 1062–1069. doi: 10.1111/jne.12064
Stonebarger, G. A., Bimonte-Nelson, H. A., and Urbanski, H. F. (2021). The rhesus macaque as a translational model for neurodegeneration and Alzheimer’s disease. Front. Aging Neurosci. 13:734173. doi: 10.3389/fnagi.2021.734173
Stonebarger, G. A., Urbanski, H. F., Woltjer, R. L., Vaughan, K. L., Ingram, D. K., Schultz, P. L., et al. (2020). Amyloidosis increase is not attenuated by long-term calorie restriction or related to neuron density in the prefrontal cortex of extremely aged rhesus macaques. GeroScience 42, 1733–1749. doi: 10.1007/s11357-020-00259-0
Tang, M. X., Jacobs, D., Stern, Y., Marder, K., Schofield, P., Gurland, B., et al. (1996). Effect of oestrogen during menopause on risk and age at onset of Alzheimer’s disease. Lancet 348, 429–432. doi: 10.1016/S0140-6736(96)03356-9
Tian Hui Kwan, A., Arfaie, S., Therriault, J., Rosa-Neto, P., and Gauthier, S. (2020). Lessons learnt from the second generation of anti-amyloid monoclonal antibodies clinical trials. Dement. Geriatr. Cogn. Disord. 49, 334–348. doi: 10.1159/000511506
Tinkler, G. P., and Voytko, M. L. (2005). Estrogen modulates cognitive and cholinergic processes in surgically menopausal monkeys. Prog. Neuropsychopharmacol. Biol. Psychiatry 29, 423–431. doi: 10.1016/j.pnpbp.2004.12.016
Tolar, M., Abushakra, S., and Sabbagh, M. (2020). The path forward in Alzheimer’s disease therapeutics: Reevaluating the amyloid cascade hypothesis. Alzheimer’s Dement. 16, 1553–1560. doi: 10.1016/j.jalz.2019.09.075
Tschiffely, A. E., Schuh, R. A., Prokai-Tatrai, K., Ottinger, M. A., and Prokai, L. (2016). A comparative evaluation of treatments with 17β-estradiol and its brain-selective prodrug in a double-transgenic mouse model of Alzheimer’s disease. Horm. Behav. 83, 39–44. doi: 10.1016/j.yhbeh.2017.11.015
Uno, H. (1993). The incidence of senile plaques and multiple infarction in aged macaque brain. Neurobiol. Aging 14, 673–674. doi: 10.1016/0197-4580(93)90067-l
Urbanski, H. F. (2017). Effect of androgen supplementation on 24-hour activity-rest patterns of aged male rhesus macaques. Neurobiol. Aging 54, 100–102. doi: 10.1016/j.neurobiolaging.2017.02.020
Urbanski, H. F., Appleman, M. L., Nilaver, B., and Kohama, S. G. (2022). Amyloid beta expression in the amygdala of aged rhesus macaques: Effect of hormone replacement therapy. Soc. Endocrinol. Abstr. 86:345.
Urbanski, H. F., Mueller, K., and Bethea, C. L. (2017). Effect of an obesogenic diet on circadian activity and serum hormones in old monkeys. Endocr. Connect. 6, 380–383. doi: 10.1530/EC-17-0108
Urbanski, H. F., Sorwell, K. G., Garyfallou, V. T., Garten, J., Weiss, A., Renner, L., et al. (2014). Androgen supplementation during aging: Development of a physiologically appropriate protocol. Rejuvenation Res. 17, 150–153. doi: 10.1089/rej.2013.1518
van Dyck, C. H., Swanson, C. J., Aisen, P., Bateman, R. J., Chen, C., Gee, M., et al. (2023). Lecanemab in Early Alzheimer’s Disease. N. Engl. J. Med. 388, 9–21. doi: 10.1056/NEJMoa2212948
Viña, J., and Lloret, A. (2010). Why women have more Alzheimer’s disease than men: Gender and mitochondrial toxicity of amyloid-beta peptide. J. Alzheimers Dis. 20(Suppl. 2), S527–S533. doi: 10.3233/JAD-2010-100501
Voytko, M. L., Higgs, C. J., and Murray, R. (2008). Differential effects on visual and spatial recognition memory of a novel hormone therapy regimen of estrogen alone or combined with progesterone in older surgically menopausal monkeys. Neuroscience 154, 1205–1217. doi: 10.1016/j.neuroscience.2008.04.064
Yaffe, K. (2003). Hormone therapy and the brain: Déjà vu all over again? JAMA 289, 2717–2719. doi: 10.1001/jama.289.20.2717
Yaffe, K., Sawaya, G., Lieberburg, I., and Grady, D. (1998). Estrogen therapy in postmenopausal women: Effects on cognitive function and dementia. JAMA 279, 688–695. doi: 10.1001/jama.279.9.688
Keywords: aging, Alzheimer’s disease, amyloid plaques, hormone replacement therapy (HRT), rhesus macaque, menopause
Citation: Appleman M-L, Thomas JL, Weiss AR, Nilaver BI, Cervera-Juanes R, Kohama SG and Urbanski HF (2024) Effect of hormone replacement therapy on amyloid beta (Aβ) plaque density in the rhesus macaque amygdala. Front. Aging Neurosci. 15:1326747. doi: 10.3389/fnagi.2023.1326747
Received: 23 October 2023; Accepted: 27 December 2023;
Published: 11 January 2024.
Edited by:
Mercè Pallàs, University of Barcelona, SpainReviewed by:
Md. Sohanur Rahman, Florida International University, United StatesCopyright © 2024 Appleman, Thomas, Weiss, Nilaver, Cervera-Juanes, Kohama and Urbanski. This is an open-access article distributed under the terms of the Creative Commons Attribution License (CC BY). The use, distribution or reproduction in other forums is permitted, provided the original author(s) and the copyright owner(s) are credited and that the original publication in this journal is cited, in accordance with accepted academic practice. No use, distribution or reproduction is permitted which does not comply with these terms.
*Correspondence: Henryk F. Urbanski, dXJiYW5za2lAb2hzdS5lZHU=
Disclaimer: All claims expressed in this article are solely those of the authors and do not necessarily represent those of their affiliated organizations, or those of the publisher, the editors and the reviewers. Any product that may be evaluated in this article or claim that may be made by its manufacturer is not guaranteed or endorsed by the publisher.
Research integrity at Frontiers
Learn more about the work of our research integrity team to safeguard the quality of each article we publish.