- 1Leonard Davis School of Gerontology, University of Southern California, Los Angeles, CA, United States
- 2Department of Cell Biology and Physiology, KU Diabetes Institute and Department of Internal Medicine-Division of Endocrinology, University of Kansas Alzheimer's Disease Research Center, University of Kansas Medical Center, Kansas City, KS, United States
- 3Kansas Center for Metabolism and Obesity Research, Kansas City, MO, United States
- 4Center for Children's Healthy Lifestyles and Nutrition, Kansas City, MO, United States
- 5Kansas City VA Medical Center, Kansas City, MO, United States
- 6Department of Neurology, University of Kansas Alzheimer's Disease Center, University of Kansas Medical Center, Kansas City, KS, United States
- 7Department of Biochemistry and Molecular Biology, University of Kansas Alzheimer's Disease Center, University of Kansas Medical Center, Kansas City, KS, United States
Editorial on the Research Topic
Systemic implications of Alzheimer's disease
In the past decade, it has become increasingly evident that Alzheimer's disease (AD) risk can be modulated by multiple systemic factors. For example, diabetes, cardiovascular disease, and low cardiorespiratory fitness are associated with increased risk for AD, highlighting the previously undescribed role for whole body metabolism in AD pathogenesis (Kopf and Frolich, 2009; Baker et al., 2010; Morris et al., 2014, 2017, 2020; Vidoni et al., 2015; Gaitan et al., 2019; Nho et al., 2019; Tini et al., 2020). Growing evidence supports metabolic changes are observed within the brain and systemic tissues in AD, including from blood, skin, muscle, and adipose tissue (Parker et al., 1990; Parker, 1991; Kish et al., 1992; Curti et al., 1997; Bosetti et al., 2002; Cardoso et al., 2004; Arbones-Mainar et al., 2010; Mosconi et al., 2011; Fisar et al., 2016, 2019; Morris et al., 2021; Kim et al., 2022). Furthermore, recent work describes both liver and kidney function correlate with cognition and AD pathological biomarkers (Nho et al., 2019; Stocker et al., 2023). Overall, these findings highlight the role of systemic physiology in AD.
Our goal for this Research Topic was to highlight the role of systemic changes in AD. Although further studies are needed to understand if systemic changes are a cause or a consequence of AD, understanding systemic contributions to AD can provide novel targets to modify risk through lifestyle changes. Promoting studies which provide integral discussion on systemic physiology in AD is imperative to understand disease etiology and mitigate risk.
A seminal study in 2019 highlighted the association of elevated plasma liver enzyme biomarkers with poor cognition, increased AD risk, elevated brain Aβ burden, and reduced brain glucose metabolism (Nho et al., 2019). As a follow up to this study, in this issue Wu et al. examined the association of liver function with cognitive impairment using the Shenzhen aging-related disorder cohort. The study leveraged a cohort of 7,201 people over the age of 60 years old to examine cognition and blood liver enzyme biomarkers, alanine aminotransferase (ALT) and aspartate aminotransferase (AST). Wu et al. reported higher AST to ALT ratio in the cognitive impairment group compared to the non-impaired group. The cognitive impairment group also had significantly increased age, lower education level, and more female participants. Overall, this study highlights an association of reduced liver function with cognition, a new area of research for the field. Further studies are warranted to understand the role systemic liver physiology contributes to brain aging, cognition, and AD risk.
Renal function is associated with cognitive impairment, however the relationship between renal function and AD biomarkers is currently poorly understood. The cross-sectional study by Zhang et al. examined associations of estimated glomerular filtration rate (eGFR) with plasma biomarkers for AD. While plasma Aβ biomarkers did not associate with renal function measured by eGFR, plasma Tau and neurofilament light (NfL) biomarkers did. These data suggest that reduced renal function is associated with increased markers of neurodegeneration. Renal and hepatic function are directly associated with whole body metabolism, and these studies directly support a role for systemic metabolism in regulating brain aging.
The role of energy metabolism in AD is highly cited. Energy disruption is noted across multiple tissues including brain, muscle, skin, and blood. Multiple studies suggest that AD pathologies like Aβ and Tau can disrupt mitochondrial function and energy metabolism (Hansson Petersen et al., 2008; Swerdlow et al., 2014, 2017; Reddy and Oliver, 2019; Weidling et al., 2020; Wilkins et al., 2022; Zysk et al., 2023). Systemic mitochondrial dysfunction and disrupted energy metabolism are noted in AD subjects, even in tissues where AD pathologies are lacking. This suggests disrupted energy metabolism is upstream of AD pathologies, as proposed in the mitochondrial cascade hypothesis of AD (Swerdlow et al., 2014). Modulating energy metabolism and mitochondrial has become a major target for AD therapeutics. Pre-clinical studies and clinical studies support the principle that aerobic capacity and fitness can modulate energy metabolism.
Aerobic capacity and fitness (or the lack there-of) are risk factors for not only systemic diseases, like diabetes and cardiovascular disease, but also for cognitive decline and AD. To study the effects of intrinsic aerobic capacity, rat models were selectively bred for their capacity for voluntary wheel running (Kerr et al.). This breeding scheme yielded low voluntary wheel runner rats with low aerobic capacity and high voluntary wheel runner rats with high aerobic capacity. Kerr et al. examined cognition, hippocampal neurogenesis, and mitochondrial function in these rat models selectively bred for intrinsic aerobic capacity and physical activity. High aerobic capacity and physical activity benefited neurogenesis, hippocampal size, brain glucose metabolism, and brain mitochondrial respiration in female rats. These effects were not observed in male rats. Physical inactivity and aerobic capacity have heritable effects on brain health and aging, with increased susceptibility in females.
Thyroid function has been implicated in AD risk, especially in women. The thyroid is an essential organ that secretes hormones critical for energy metabolism homeostasis. Based on prior findings associating thyroid function with AD, Ma et al. described an association of dementia risk and cognitive impairment with thyroid disease using a meta-analysis systemic review (PROSPERO: CRD42021290105). Fifteen studies were included in the meta-analysis which suggested that hyperthyroidism and subclinical hyperthyroidism are associated with increased dementia risk, while hypothyroidism was not associated with dementia risk. This meta-analysis highlights a potential role of hyperthyroidism in dementia risk and the need to understand the relevance to disease pathogenesis.
Beyond changes in energy metabolism, systemic inflammation is also implicated in AD. Oral hygiene is an important factor in systemic inflammation. Furthermore, oral health is an important indicator for cardiorespiratory health and can directly impact risk for infections (Kotronia et al., 2021). Occlusal support is an indicator of dental health including tooth loss. Occlusal support allows mastication (chewing), food mixing with salivary and gastric enzymes facilitating digestion and nutrient absorption. Da et al. assessed cognitive function and occlusal support in community dwelling adults aged over 60 years using a cross-sectional study design. Individuals with poor occlusal support had an increased odds ratio for cognitive impairment when compared to those with good occlusal support. Data were adjusted for age, sex, education level, cigarette smoking, alcohol drinking, cardiovascular disease, and diabetes. Age mediated most of the association of occlusal support and cognitive impairment. Further studies are needed to understand the association of age, occlusal support, and cognitive impairment.
Collectively, the articles in this Research Topic emphasize the importance of understanding systemic contributions to AD (Figure 1). Understanding how systemic risk factors over lifespan can impact brain aging and cognition is critical to the field of AD. Systemic risk factors, such as diabetes, cardiovascular disease, and physical activity are modifiable and could greatly impact the impact of AD on the aging population.
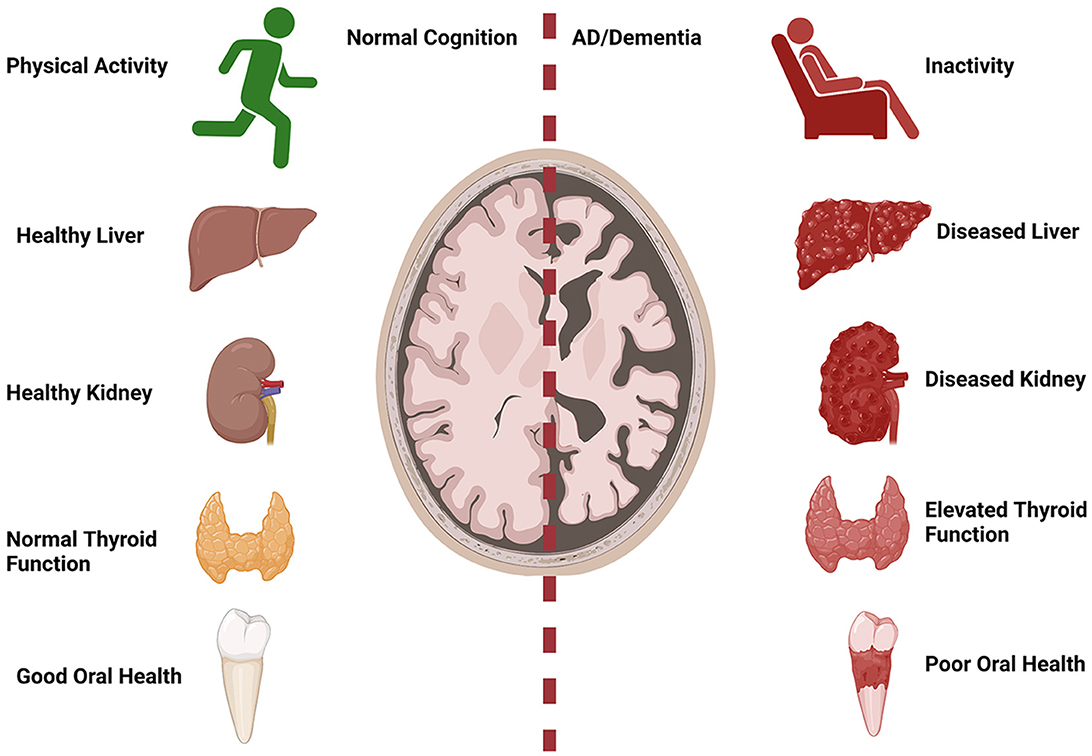
Figure 1. Systemic implications for AD. Normal cognition with aging is associated with increased physical activity, healthy liver and kidney function, normal thyroid function, and good oral health. AD/dementia risk is increased with physical inactivity, abnormal liver and kidney function, hyperthyroidism, and poor oral health. Created with BioRender.com.
Author contributions
All authors listed have made a substantial, direct, and intellectual contribution to the work and approved it for publication.
Funding
This work was funded by (R01AG077536 to CJC), (R01 KD121497, R01AG069781, U01AG070928-01, and P20GM144269 to JPT), and (R01AG078186 and R00AG056600 to HMW).
Conflict of interest
The authors declare that the research was conducted in the absence of any commercial or financial relationships that could be construed as a potential conflict of interest.
Publisher's note
All claims expressed in this article are solely those of the authors and do not necessarily represent those of their affiliated organizations, or those of the publisher, the editors and the reviewers. Any product that may be evaluated in this article, or claim that may be made by its manufacturer, is not guaranteed or endorsed by the publisher.
References
Arbones-Mainar, J. M., Johnson, L. A., Altenburg, M. K., Kim, H. S., and Maeda, N. (2010). Impaired adipogenic response to thiazolidinediones in mice expressing human apolipoproteinE4. FASEB J. 24, 3809–3818. doi: 10.1096/fj.10-159517
Baker, L. D., Frank, L. L., Foster-Schubert, K., Green, P. S., Wilkinson, C. W., McTiernan, A., et al. (2010). Aerobic exercise improves cognition for older adults with glucose intolerance, a risk factor for Alzheimer's disease. J. Alzheimers. Dis. 22, 569–579. doi: 10.3233/JAD-2010-100768
Bosetti, F., Brizzi, F., Barogi, S., Mancuso, M., Siciliano, G., Tendi, E. A., et al. (2002). Cytochrome c oxidase and mitochondrial F1F0-ATPase (ATP synthase) activities in platelets and brain from patients with Alzheimer's disease. Neurobiol. Aging 23, 371–376. doi: 10.1016/S0197-4580(01)00314-1
Cardoso, S. M., Proenca, M. T., Santos, S., Santana, I., and Oliveira, C. R. (2004). Cytochrome c oxidase is decreased in Alzheimer's disease platelets. Neurobiol. Aging 25, 105–110. doi: 10.1016/S0197-4580(03)00033-2
Curti, D., Rognoni, F., Gasparini, L., Cattaneo, A., Paolillo, M., Racchi, M., et al. (1997). Oxidative metabolism in cultured fibroblasts derived from sporadic Alzheimer's disease (AD) patients. Neurosci. Lett. 236, 13–16. doi: 10.1016/S0304-3940(97)00741-6
Fisar, Z., Hansikova, H., Krizova, J., Jirak, R., Kitzlerova, E., Zverova, M., et al. (2019). Activities of mitochondrial respiratory chain complexes in platelets of patients with Alzheimer's disease and depressive disorder. Mitochondrion. 48, 67–77. doi: 10.1016/j.mito.2019.07.013
Fisar, Z., Hroudova, J., Hansikova, H., Spacilova, J., Lelkova, P., Wenchich, L., et al. (2016). Mitochondrial respiration in the platelets of patients with Alzheimer's disease. Curr. Alzheimer Res. 13, 930–941. doi: 10.2174/1567205013666160314150856
Gaitan, J. M., Boots, E. A., Dougherty, R. J., Oh, J. M., Ma, Y., Edwards, D. F., et al. (2019). Brain glucose metabolism, cognition, and cardiorespiratory fitness following exercise training in adults at risk for Alzheimer's disease. Brain Plast 5, 83–95. doi: 10.3233/BPL-190093
Hansson Petersen, C. A., Alikhani, N., Behbahani, H., Wiehager, B., Pavlov, P. F., Alafuzoff, I., et al. (2008). The amyloid beta-peptide is imported into mitochondria via the TOM import machinery and localized to mitochondrial cristae. Proc. Natl. Acad. Sci. USA. 105, 13145–13150. doi: 10.1073/pnas.0806192105
Kim, S., Yi, H. A., Won, K. S., Lee, J. S., and Kim, H. W. (2022). Association between visceral adipose tissue metabolism and Alzheimer's disease. Pathol. Metabol. (2022) 12:030258. doi: 10.3390/metabo12030258
Kish, S. J., Bergeron, C., Rajput, A., Dozic, S., Mastrogiacomo, F., Chang, L. J., et al. (1992). Brain cytochrome oxidase in Alzheimer's disease. J. Neurochem. 59, 776–779. doi: 10.1111/j.1471-4159.1992.tb09439.x
Kopf, D., and Frolich, L. (2009). Risk of incident Alzheimer's disease in diabetic patients: a systematic review of prospective trials. J. Alzheimers. Dis. 16, 677–685. doi: 10.3233/JAD-2009-1011
Kotronia, E., Brown, H., Papacosta, A. O., Lennon, L. T., Weyant, R. J., Whincup, P. H., et al. (2021). Oral health and all-cause, cardiovascular disease, and respiratory mortality in older people in the UK and USA. Sci. Rep. 11, 16452. doi: 10.1038/s41598-021-95865-z
Morris, J. K., Honea, R. A., Vidoni, E. D., Swerdlow, R. H., and Burns, J. M. (2014). Is Alzheimer's disease a systemic disease? Biochim. Biophys. Acta 1842, 1340–1349. doi: 10.1016/j.bbadis.2014.04.012
Morris, J. K., McCoin, C. S., Fuller, K. N., John, C. S., Wilkins, H. M., Green, Z. D., et al. (2021). Mild cognitive impairment and donepezil impact mitochondrial respiratory capacity in skeletal muscle. Function (Oxf). (2021) 2, zqab045. doi: 10.1093/function/zqab045
Morris, J. K., Vidoni, E. D., Johnson, D. K., Van Sciver, A., Mahnken, J. D., Honea, R. A., et al. (2017). Aerobic exercise for Alzheimer's disease: a randomized controlled pilot trial. PLoS ONE. 12, e0170547. doi: 10.1371/journal.pone.0170547
Morris, J. K., Zhang, G., Dougherty, R. J., Mahnken, J. D., John, C. S., Lose, S. R., et al. (2020). Collective effects of age, sex, genotype, and cognitive status on fitness outcomes. Alzheimers Dement (Amst). 12, e12058. doi: 10.1002/dad2.12058
Mosconi, L., de Leon, M., Murray, J., Lu, E. L., Javier, E., McHugh, P., et al. (2011). Reduced mitochondria cytochrome oxidase activity in adult children of mothers with Alzheimer's disease. J. Alzheimers. Dis. 27, 483–490. doi: 10.3233/JAD-2011-110866
Nho, K., Kueider-Paisley, A., Ahmad, S., MahmoudianDehkordi, S., Arnold, M., and Risacher, S. L. (2019). Alzheimer's disease neuroimaging, and c. the Alzheimer disease metabolomics, association of altered liver enzymes with Alzheimer disease diagnosis, cognition, neuroimaging measures, and cerebrospinal fluid biomarkers. JAMA Netw Open. 2, e197978. doi: 10.1001/jamanetworkopen.2019.7978
Parker, W. D. (1991). Cytochrome oxidase deficiency in Alzheimer's disease. Ann. N. Y. Acad. Sci. 640, 59–64. doi: 10.1111/j.1749-6632.1991.tb00191.x
Parker, W. D., Filley, C. M., and Parks, J. K. (1990). Cytochrome oxidase deficiency in Alzheimer's disease. Neurology 40, 1302–1303. doi: 10.1212/WNL.40.8.1302
Reddy, P. H., and Oliver, D. M. (2019). Amyloid beta and phosphorylated tau-induced defective autophagy and mitophagy in Alzheimer's disease. Cells. (2019) 8:488. doi: 10.3390/cells8050488
Stocker, H., Beyer, L., Trares, K., Perna, L., Rujescu, D., Holleczek, B., et al. (2023). Association of kidney function with development of Alzheimer disease and other dementias and dementia-related blood biomarkers. JAMA Netw Open 6, e2252387. doi: 10.1001/jamanetworkopen.2022.52387
Swerdlow, R. H., Burns, J. M., and Khan, S. M. (2014). The Alzheimer's disease mitochondrial cascade hypothesis: progress and perspectives. Biochim. Biophys. Acta 1842, 1219–1231. doi: 10.1016/j.bbadis.2013.09.010
Swerdlow, R. H., Koppel, S., Weidling, I., Hayley, C., and Wilkins, J. i. Y. (2017). Mitochondria cybrids, aging, Alzheimer's disease. Prog. Mol. Biol. Transl. Sci. 146, 259–302. doi: 10.1016/bs.pmbts.2016.12.017
Tini, G., Scagliola, R., Monacelli, F., La Malfa, G., Porto, I., Brunelli, C., et al. (2020). Alzheimer's disease and cardiovascular disease: a particular association. Cardiol. Res. Pract. 2020, 2617970. doi: 10.1155/2020/2617970
Vidoni, E. D., Johnson, D. K., Morris, J. K., Van Sciver, A., Greer, C. S., Billinger, S. A., et al. (2015). Dose-response of aerobic exercise on cognition: a community-based, pilot randomized controlled trial. PLoS ONE. 10, e0131647. doi: 10.1371/journal.pone.0131647
Weidling, I. W., Wilkins, H. M., Koppel, S. J., Hutfles, L., Wang, X., Kalani, A., et al. (2020). Mitochondrial DNA manipulations affect tau oligomerization. J. Alzheimers. Dis. 77, 149–163. doi: 10.3233/JAD-200286
Wilkins, H. M., Troutwine, B. R., Menta, B. W., Manley, S. J., Strope, T. A., Lysaker, C. R., et al. (2022). Mitochondrial membrane potential influences amyloid-beta protein precursor localization and amyloid-beta secretion. J. Alzheimers. Dis. 85, 381–394. doi: 10.3233/JAD-215280
Keywords: Alzheimer's disease, systemic, cardiovascular, diabetes, fitness, liver, kidney
Citation: Cortes CJ, Thyfault JP and Wilkins HM (2023) Editorial: Systemic implications of Alzheimer's disease. Front. Aging Neurosci. 15:1219987. doi: 10.3389/fnagi.2023.1219987
Received: 09 May 2023; Accepted: 12 May 2023;
Published: 23 May 2023.
Edited and reviewed by: Agustin Ibanez, Latin American Brain Health Institute (BrainLat), Chile
Copyright © 2023 Cortes, Thyfault and Wilkins. This is an open-access article distributed under the terms of the Creative Commons Attribution License (CC BY). The use, distribution or reproduction in other forums is permitted, provided the original author(s) and the copyright owner(s) are credited and that the original publication in this journal is cited, in accordance with accepted academic practice. No use, distribution or reproduction is permitted which does not comply with these terms.
*Correspondence: Heather M. Wilkins, aHdpbGtpbnMmI3gwMDA0MDtrdW1jLmVkdQ==