- 1Rush Alzheimer’s Disease Center, Chicago, IL, United States
- 2Department of Neurological Sciences, Rush University Medical Center, Chicago, IL, United States
- 3Department of Psychiatry and Behavioral Sciences, Rush University Medical Center, Chicago, IL, United States
- 4Department of Diagnostic Radiology and Nuclear Medicine, Rush University Medical Center, Chicago, IL, United States
- 5Department of Biomedical Engineering, Illinois Institute of Technology, Chicago, IL, United States
- 6Department of Preventive Medicine, Rush University Medical Center, Chicago IL, United States
- 7Department of Family Medicine and Neurology, Keck School of Medicine, Los Angeles, CA, United States
- 8Department of Psychology, University of Southern California, Los Angeles, CA, United States
- 9School of Gerontology, University of Southern California, Los Angeles, CA, United States
- 10Department of Orthopedic Surgery, Rush University Medical Center, Chicago, IL, United States
Introduction: Older Black adults experience a high burden of depressive symptoms and cerebrovascular disease but the specific neurobiological substrates underlying the association between late-life depressive symptoms and brain integrity are understudied, particularly in within-group designs.
Methods: Using the Center for Epidemiologic Studies Depression Scale and diffusion-tensor imaging, within-Black variation in the association between late-life depressive symptoms and white matter structural integrity was examined in 297 older Black participants without dementia that were enrolled across three epidemiological studies of aging and dementia. Linear regression models were used to test associations with DTI metrics (fractional anisotropy, trace of the diffusion tensor) as the outcomes and depressive symptoms as the predictor, while adjusting for age, sex, education, scanner, serotonin-reuptake inhibitor use, total volume of white-matter hyperintensities normalized by intracranial volume, and presence of white-matter hyperintensities at the voxel level.
Results: Higher level of self-reported late-life depressive symptoms was associated with greater diffusion-tensor trace (reduced white matter integrity) in connections between commissural pathways and contralateral prefrontal regions (superior and middle frontal/dorsolateral prefrontal cortex), association pathways connecting dorsolateral prefrontal cortex with insular, striatal and thalamic regions, and association pathways connecting the parietal, temporal and occipital lobes and the thalamus.
Discussion: This study demonstrated a discernable pattern of compromised white matter structural integrity underlying late-life depressive symptoms within older Black adults.
Introduction
Depressive symptoms occurring in late life are associated with several adverse health outcomes including cerebrovascular disease and stroke (Pan et al., 2011; Taylor et al., 2013; Aizenstein et al., 2016; Van Agtmaal et al., 2017), cognitive impairment and dementia (Panza et al., 2010; Diniz et al., 2013; Barnes, 2014; Rashidi-Ranjbar et al., 2020), functional disability (Callahan et al., 1998; Wassink-Vossen et al., 2019), morbidity (Lamar et al., 2015; Cummings et al., 2016; Cho and Hamler, 2021), and all-cause mortality (Saz and Dewey, 2001; Wei et al., 2019). Depressive symptoms in older adults have been linked with brain integrity in a number of studies (reviewed in Alexopoulos, 2019) but more work is needed to identify specific neurobiological substrates underlying this association, and this is particularly true for older Black adults who have a high prevalence of late-life depressive symptoms (Skarupski et al., 2005; Pickett et al., 2013) but are understudied generally and in the neurologic (Robbins et al., 2022), neuroimaging (Carmichael and Newton, 2019) and psychiatric (Price et al., 2022) literatures.
Older Black adults experience sociocultural and environmental exposures linked to depression, such as discrimination, poverty, and neighborhood disadvantage (Barnes et al., 2012a; Mezuk et al., 2013; Hastings and Snowden, 2019; Scheuermann et al., 2020), and are disproportionately burdened by depressive symptoms (Skarupski et al., 2005; Walsemann et al., 2009; Liang et al., 2011; Pickett et al., 2013; Reinlieb et al., 2014; but see Erving et al., 2019) and their associated adverse health outcomes (Prabhakaran et al., 2008; Turner et al., 2015; Steenland et al., 2016; Kalyani et al., 2017; Rajan et al., 2019; Deere and Ferdinand, 2020; Cho and Hamler, 2021; Virani et al., 2021). Older Black adults also have a higher brain white-matter hyperintensity burden (Brickman et al., 2008; Morris et al., 2009), a measure thought to generally reflect small vessel-related injury (Schmahmann et al., 2008; Wardlaw et al., 2015), yet the many MRI studies linking late-life depressive symptoms to brain integrity have mostly employed all-White or mixed samples. Focusing on an all-Black sample allows a deeper understanding of within-Black variation in the association between late-life depressive symptoms and brain integrity that could potentially inform the development of programs that reduce racial disparities in affective health (Mezuk et al., 2013; Pickett et al., 2013; Price et al., 2022) and lead to race-relevant targeted treatments.
One candidate neurobiological substrate underlying late-life depressive symptoms is altered brain white matter structural integrity. Reduced white matter structural integrity is commonly measured on in vivo MRI in older adults as visible regions of white matter hyperintensities (WMHs) on T2-weighted or FLAIR images and/or by lower diffusion anisotropy (fractional anisotropy; FA) or higher overall diffusivity (trace) of water molecules on diffusion tensor imaging (DTI). Lower diffusion anisotropy or higher diffusivity may reflect axonal injury or degeneration, vasogenic or cytotoxic edema, myelin disruption, inflammation, or other disturbances in diverse cellular mechanisms (Parekh et al., 2015; Bennett D. A. et al., 2018). These reductions in white matter structural integrity can lead to a “connectopathy” (Filley and Fields, 2016) or an interference of communication across a widely distributed neural circuitry that supports behavior (Schmahmann et al., 2008). Indeed, the link between late-life depressive symptoms and altered brain white matter structural integrity across numerous regions has been well-established in studies using all-White or mixed samples (Lamar et al., 2010; Wen et al., 2014; Smagula and Aizenstein, 2016; Van Agtmaal et al., 2017; Alexopoulos, 2019; Kim and Han, 2021), but to date, this association has not been examined within a large, solely Black, epidemiologic sample. In this study, self-report of late-life depressive symptoms was measured using the Center for Epidemiologic Studies Depression (CES-D) scale (Kohout et al., 1993) and white matter structure was interrogated voxel-wise with DTI, in 297 older Black participants without dementia, from three large epidemiological studies of aging and dementia.
Materials and methods
Participants
Participants were recruited from three parent studies of aging and dementia conducted in the United States: Minority Aging Research Study (MARS; Barnes et al., 2012b), Rush Alzheimer’s Disease Center African American Clinical Core (RADC AA Core; Barnes et al., 2012b), and the Rush Memory and Aging Project (MAP; Bennett D. A. et al., 2018). MARS and RADC AA Core both consist of older Black adults recruited from churches, senior buildings and social clubs and organizations that cater to minority populations. The Rush Memory and Aging Project is a cohort of older diverse lay persons (predominantly White) recruited from retirement communities and subsidized housing facilities across the Chicago metropolitan area. Only Black adults enrolled in MAP were eligible for this analysis. All three studies recruit participants without known dementia at baseline and annual evaluations are harmonized across all studies and include a medical history, neuropsychological testing and neurological evaluation (as previously described; Barnes et al., 2012b; Bennett I. J. et al., 2018). Participants supplied all medications prescribed by a doctor, vitamins, supplements, and over-the-counter remedies and medicines taken in the 2 weeks prior to the annual evaluation. Direct visual inspection of all containers of prescription and over-the-counter agents allowed for medication documentation. Medications were then coded using the Medi-Span Drug Data Base system (Medi-Span Inc., 1995). Three-tesla (3 T) neuroimaging was added to the parent studies between 2009 and 2012. All studies followed the ethical standards set in the 1964 Declaration of Helsinki and its later amendments and were approved by the Institutional Review Board of Rush University Medical Center.
Eligible participants self-identified as Black, successfully completed at least one MRI acquisition that passed quality-control (QC), completed CES-D assessments, and had no history of dementia at or before the first MRI session. To identify these participants, we began with all participants in the three parent studies described above (Figure 1).
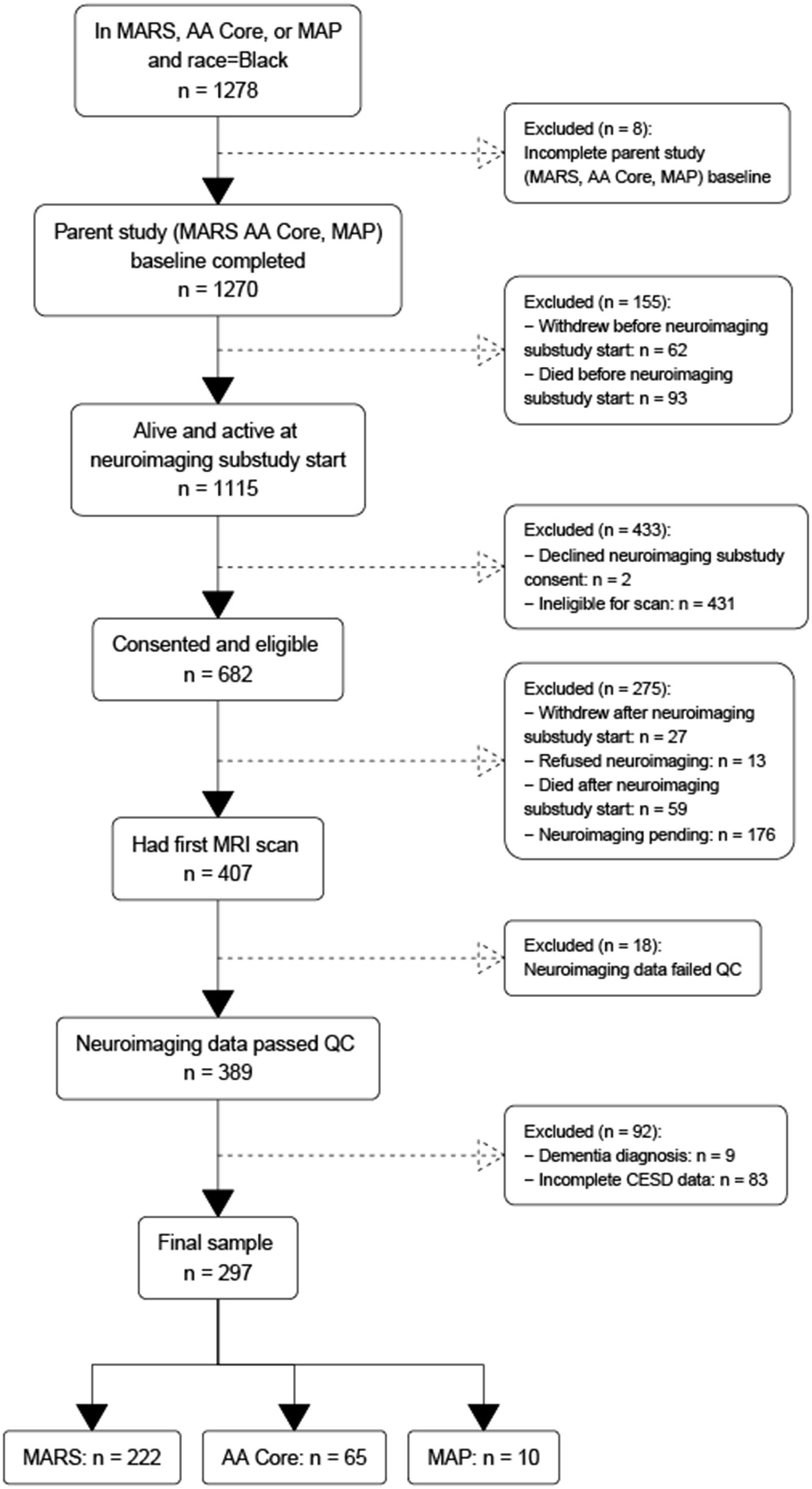
Figure 1. Flowchart of participant recruitment across three parent epidemiological studies of aging and dementia conducted in the United States.
This yielded a group of 1,270 participants that had completed baseline evaluation at the time of analysis (analytic baseline). Of these 1,270 participants, 62 withdrew and 93 died before analytic baseline, leaving a sample of 1,115 participants. Of these 1,115, 431 were ineligible to participate for various reasons (e.g., MRI contraindications such as metal in the body and claustrophobia, living out of the geographic area, physical limitations such as frailty or cognitive limitations such as dementia) and another two declined to consent, leaving 682 that were consented. Of these 682 participants, another 40 withdrew or refused, 59 died before they had their first scan, and 176 had their first scan pending, leaving 407 participants that received their initial MRI scan. Of these 407, 18 scans failed quality control resulting in 389 participants with MRI data. Of these 389 participants, nine were excluded with a diagnosis of dementia based on standard clinical evaluation (Barnes et al., 2012b; Bennett D. A. et al., 2018) and NINCDS/ADRDA diagnostic criteria (McKhann et al., 1984), and 83 were excluded for missing CES-D data. The final sample comprised 297 participants: 222 from MARS, 65 from RADC AA Core, and 10 from MAP. To examine for selection bias, we compared the analytic sample to (1) Black participants across all cohorts regardless of neuroimaging status, (2) Black participants that left the parent study before neuroimaging was begun, and (3) Black participants that were eligible for neuroimaging but were not scanned. The analytic sample had milder depressive symptoms, higher MMSE scores (within the normal range of 24 and above) and less SSRI medication use.
Assessment of depressive symptoms
The 10-item version of the Center for Epidemiologic Studies Depression (CES-D; Kohout et al., 1993) scale was used to measure late-life depressive symptoms. This version was derived from the original 20-item version (Radloff, 1977), which has been shown to have measurement equivalency in Black adults (Nguyen et al., 2004). The 10-item version has similar factor structure as the 20-item version and acceptable reliability (Kohout et al., 1993).
Image acquisition
MRI data were collected on a 3 T Philips MRI scanner and a 3 T Siemens scanner using a 3D magnetization prepared rapid acquisition gradient echo (MPRAGE) sequence, a 2D T2-weighted fluid-attenuated inversion recovery (FLAIR) sequence, and a 2D spin-echo echo-planar diffusion-weighted sequence. The parameters on the 3 T Philips scanner were: for MPRAGE TR = 8 ms, TE = 3.7 ms, TI = 955 ms, flip-angle = 8°, field of view = 240 mm × 228 mm, 181 sagittal slices, acquired voxel size = 1 × 1 × 1 mm3, and an acceleration factor of 2; for FLAIR TR = 9 s, TE = 90 ms, TI = 2,500 ms, field of view = 220 mm × 220 mm, 35 axial slices, acquired voxel size = 0.9 × 1.1 × 4 mm3, and an acceleration factor of 1.6; for DTI TR = 10.7 s, TE = 55 ms, field of view = 224 mm × 224 mm, 65 axial slices, acquired voxel size = 2 × 2 × 2 mm3, b = 1,000 s/mm2 for 40 diffusion directions and 6 b = 0 s/mm2 volumes. The parameters on the 3 T Siemens scanner were: for MPRAGE TR = 2.3 s, TE = 2.98 ms, TI = 900 ms, flip-angle = 9°, field of view = 256 mm × 256 mm, 176 sagittal slices, acquired voxel size = 1 × 1 × 1 mm3, and an acceleration factor of 2; for FLAIR TR = 9 s, TE = 150 ms, TI = 2,490 ms, field of view = 220 mm × 220 mm, 35 axial slices, acquired voxel size = 0.9 × 0.9 × 4 mm3, and an acceleration factor of 2; for DTI TR = 8.1 s, TE = 85 ms, field of view = 224 mm × 224 mm, 65 axial slices, acquired voxel size = 2 × 2 × 2 mm3, b = 1,000 s/mm2 for 40 diffusion directions and 6 b = 0 s/mm2 volumes.
Image processing
Fractional anisotropy and the trace of the diffusion tensor, two of the most commonly used measures derived from the diffusion tensor (Alexander et al., 2007), were used to characterize white matter structural integrity. Correction of distortions in the diffusion-weighted volumes caused by eddy currents and magnetic field non-uniformities, bulk-motion correction, B-matrix reorientation, tensor fitting, and generation of FA and trace maps were accomplished with TORTOISE (http://www.tortoisedti.org; Basser and Pierpaoli, 1996; LeBihan et al., 2001; Pierpaoli et al., 2020). White matter lesions appearing hyperintense in T2-weighted images (white matter hyperintensities; WMHs) were segmented for each participant based on both MPRAGE and FLAIR data (FSL, FMRIB, University of Oxford, United Kingdom) using BIANCA (Griffanti et al., 2016), and a mask (0 and 1 s) was generated (voxels with WMH were given values of 1). The WMH mask of each participant was transformed to the space of the corresponding processed DTI data based on the transformation of the FLAIR image volume to the b = 0 s/mm2 volume.
Statistical approach
CES-D analyses
Cumulative CES-D scores were averaged over the period beginning at parent study baseline up to and including MRI analytic baseline. Higher cumulative CES-D scores reflected more depressive symptoms. Persons with a history of any reported SSRI use over this period were identified.
DTI analyses
The association of DTI-derived metrics (FA, trace) with depressive symptoms was analyzed voxel-wise along the white matter skeleton (Smith et al., 2006). DTI data from individual participants were non-linearly transformed to the space of the IIT Human Brain Atlas (v.5.0; www.nitrc.org/projects/iit; Zhang and Arfanakis, 2018) using DR-TAMAS. The resulting spatial transformations were then applied to the corresponding FA maps, and local FA maxima were projected onto the IIT Human Brain Atlas (v.5.0) white matter skeleton using TBSS (Smith et al., 2006). The same projection parameters were used to project the trace and WMH mask values from the same voxels as the local FA maxima. Linear regression was then used to test the association of FA along the white matter skeleton (outcome) with depressive symptoms (predictor), while adjusting for age, sex, education, scanner, the total volume of WMHs normalized by the intracranial volume, and the presence of WMHs at the voxel level. SSRI use was added as an additional covariate in a second model. Models were then rerun using the trace of the diffusion tensor along the white matter skeleton (outcome) with depressive symptoms (predictor). The analysis was conducted using FSL Permutation Analysis of Linear Models (PALM; Winkler et al., 2015), assuming different variances across scanners and using two exchangeability blocks (one per scanner; i.e. permutations occurred only between participants imaged on the same scanner). Statistical inference was based on 1,000 permutations of the data, and tail approximation was used to accelerate the analysis (Winkler et al., 2016). Associations were considered significant at p < 0.05, with Family Wise Error Rate (FWER) correction. The Threshold-Free Cluster Enhancement (TFCE; Smith and Nichols, 2009) method was used to define clusters of significance. The regionconnect software1 was used to determine the most probable connections passing through clusters showing significant effects, according to the connectivity information contained in the IIT Human Brain Atlas (v.5.0; developed using high angular resolution diffusion imaging probabilistic tractography; Figure 2).
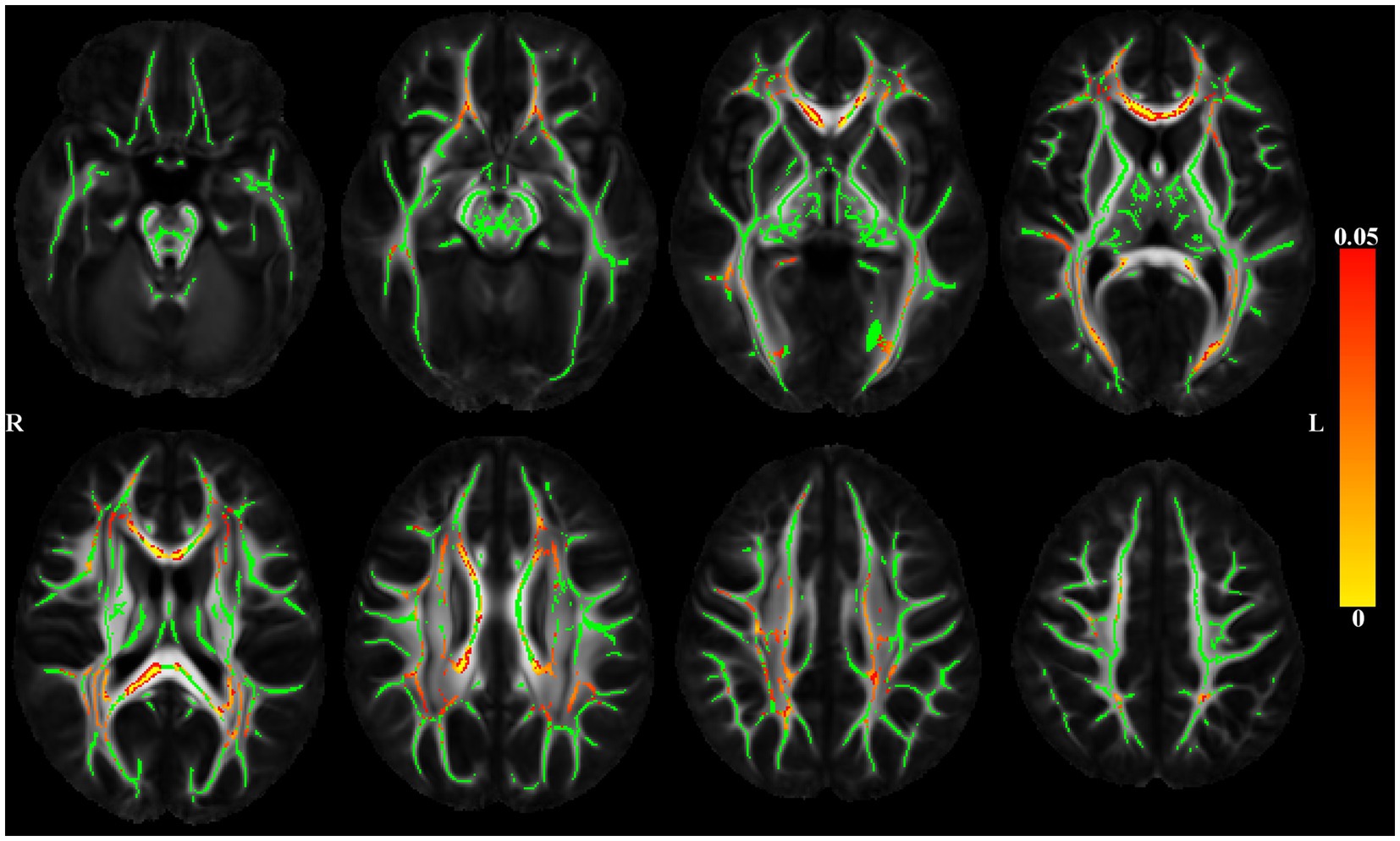
Figure 2. Maps of p values (warm color scale) showing voxels of the white matter skeleton (green color) with significant positive associations (p < 0.05) between depressive symptoms and the trace of the diffusion tensor, in linear regression models adjusted for age, education, sex, scanner, total volume of WMHs normalized by intracranial volume and the presence of WMH at the voxel level.
Results
Sample characteristics
Sample characteristics are shown in Table 1. Of the 297 persons in the analytic sample, 82% were female, had a mean age at the time of scan of 76 years (SD = 6 years) and a mean education of 15 years (SD = 3 years). Global cognition was within normal range (MMSE = 28/30, SD = 1.8). Participants reported an average of 1.7 depressive symptoms (SD = 2.0). Approximately 4% of the sample had a history of SSRI use.
Voxel-based DTI and late-life depressive symptoms
Figure 2 shows significant positive associations between late-life depressive symptoms and the trace of the diffusion tensor, adjusted for demographics and WMHs. Higher level of reported late-life depressive symptoms was associated with greater trace (higher diffusivity) throughout the brain. Adding SSRI use as a covariate did not change the results (data not shown).
Regionconnect generated lists of the most probable white matter connections traversing the clusters shown in Figure 2 that had significant associations between depressive symptoms and trace of the diffusion tensor. Clusters 1 and 2 were dominated by association pathways connecting the superior frontal and rostral middle frontal cortices (two regions encompassing the dorsolateral prefrontal cortex, DLPFC) with each other and with the insula, as well as with subcortical regions including the caudate, putamen, thalamus. Clusters 3 and 4 were dominated by association pathways connecting the parietal, temporal and occipital lobes and the thalamus. Cluster 5 was dominated by commissural pathways connecting mainly the superior frontal, rostral middle frontal, and superior parietal lobes across hemispheres (Table 2).
No associations were found between late-life depressive symptoms and white matter fractional anisotropy.
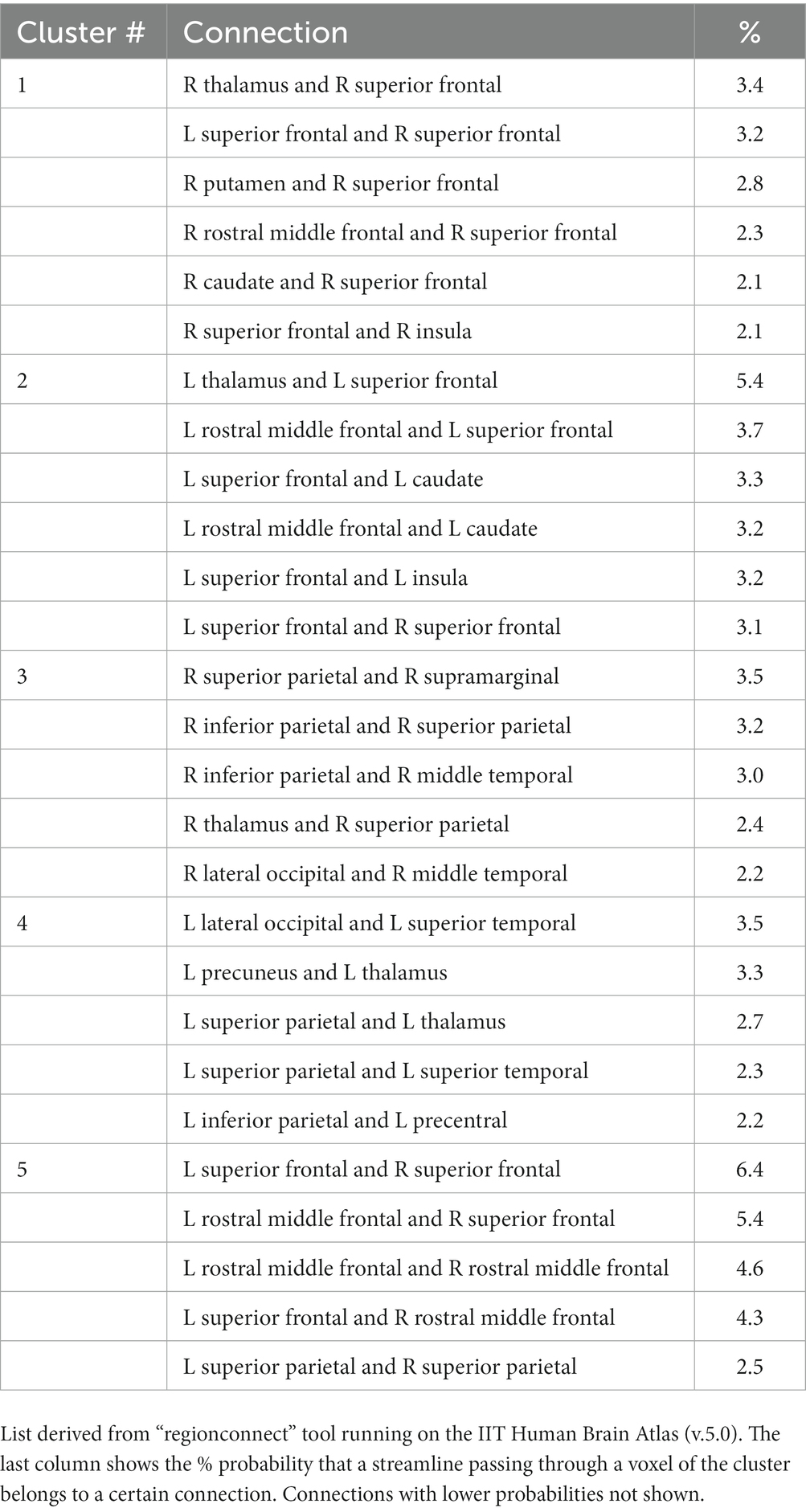
Table 2. List of most probable connections passing through the white matter clusters showing significant associations of depressive symptoms and trace of the diffusion tensor adjusting for age, education, sex, scanner, total volume of WMHs normalized by intracranial volume, and the presence of WMH at the voxel level as seen in Figure 2.
Discussion
This study examined the association between late-life depressive symptoms and white matter structural integrity in a large epidemiological sample of older Black participants without dementia. The results showed that, after correcting for age and other relevant covariates, higher level of self-reported late-life depressive symptoms was associated with reduced white matter integrity across numerous pathways, a well-established finding in the general literature. For a finer localization, regionconnect was used to identify the most probable connections running through white matter regions with significant findings. The connections that emerged included commissural pathways between contralateral prefrontal regions (superior and middle frontal/DLPFC) and association pathways connecting DLPFC with insular, striatal and thalamic regions, as well as association pathways connecting the parietal, temporal and occipital lobes and the thalamus. The associations emerged with the trace of the diffusion tensor indicating increased mobility of water molecules and suggesting degeneration of normal barriers to free diffusion as one of these white matter mechanisms. Significant associations between depressive symptoms and trace, but not FA, may have been observed for two reasons. First, depressive symptoms may be associated with a relatively uniform increase in diffusivity in all directions, which can increase the trace without affecting FA. Second, the biological changes in trace that were associated with depressive symptoms may have been more substantial than any biological changes in FA. Thus, for the available statistical power, trace changes may have been sufficiently high to exceed the value of p threshold for significance, while FA changes may have not been high enough to reach significance.
The results suggest that reduced white matter integrity in a DLPFC-striatal-limbic-thalamic circuit forms a prominent segment of the neurobiological substrate underlying depressive symptoms in older Black adults. This circuitry is critical for cognitive (particularly executive) function, motor/psychomotor function, and mood regulation (Leh et al., 2007; Drevets et al., 2008; Wen et al., 2014; Kim and Han, 2021), and reductions in white matter integrity along its fibers and projections is commonly linked to late-life depressive symptoms in the general literature (Wen et al., 2014; Smagula and Aizenstein, 2016; Van Agtmaal et al., 2017; Alexopoulos, 2019; Coloigner et al., 2019; Kim and Han, 2021). Another prominent segment of this neurobiological substrate emerged in regions of intra-parietal connections and in connections between parietal/temporal/occipital regions and thalamic nuclei. This posterior circuitry contributes to a vast array of complex functions (Culham and Kanwisher, 2001), many of which are relevant to depressive symptoms including sensory and affective aspects of pain (Benuzzi et al., 2008), reactivity to environmental cues (Meyer and Bucci, 2016), and self-awareness of action (Blakemore and Frith, 2003), and, along with connections to DLPFC and related regions, this segment comprises a frontoparietal circuit that has been implicated in the cognitive and emotional symptoms of late-life depression (Tadayonnejad and Ajilore, 2014; Alexopoulos, 2019). Although associations of late-life depressive symptoms with reduced white matter integrity did not emerge in this study for direct connections between DLPFC and parietal regions, the results clearly showed the association in connections to these two high-traffic regions (Bullmore and Sporns, 2009; Gong et al., 2009).
The association between late-life depressive symptoms and white-matter diffusion characteristics was robust even when DTI models were adjusted for whole-brain and voxel-wise WMHs, suggesting that altered white-matter diffusion above and beyond visible macrostructural injury is at play in this association. In the current study, the association emerged with the trace of the diffusion tensor which assesses the amount of diffusion of water molecules without regard for directionality. Higher trace may signal lower structural integrity of tissue, possibly due to degeneration of normal barriers to free diffusion. Increased trace has been shown to be related to higher systolic blood pressure in healthy older (White) men with normal-appearing white matter (Maclullich et al., 2009), suggesting that alterations in white matter structural integrity due to vascular risk factors may occur before there is any evidence of other structural injury. Here we showed that subclinical depressive symptoms were associated with altered white-matter diffusion after controlling for white-matter hyperintensities. It is possible that pre-visible alterations in white matter structural integrity in healthy older adults, and in particular healthy older Black adults who are at higher risk for vascular conditions, could make an early contribution to a continuum of white-matter alterations that interrupt connectivity between regions in a highly distributed network that supports affective health, and create a predisposition to late-life depressive symptoms. Longitudinal studies will be necessary to examine this hypothesis.
The association between late-life depressive symptoms and reduced white matter integrity demonstrated in this study is particularly relevant to Black adults who have higher rates of vascular risk factors and vascular disease (Carnethon et al., 2017; Brothers et al., 2019) and higher WMH burden (Brickman et al., 2008; Whitlow et al., 2015; Zahodne et al., 2015) that could potentially diminish connectivity along these circuits and lead to compromise in cognition, motor/psychomotor function, and mood. Indeed, vascular depression is clinically defined by the presence of cognitive impairment, psychomotor retardation, depressive symptoms, and MRI-evidence of vascular disease (Krishnan et al., 1997; Alexopoulos, 2019), and Black adults may thus be predisposed to this diagnosis (Reinlieb et al., 2014), especially in late-life when age and common vascular risk factors such as hypertension and diabetes increase risk of WMHs (Gottesman et al., 2010; Nyquist et al., 2014; Whitlow et al., 2015; Hughes et al., 2018; Waldron-Perrine et al., 2018). However, little remains known about the construct of vascular depression and its correlates in older Black adults due to a stark paucity of research (Bogoian and Dotson, 2022). To validate the construct of vascular depression within Black adults, and to understand its correlates to the extent that race-relevant interventions could potentially be developed, will require more studies that include neuroimaging with behavioral measures and employ large epidemiologic all-Black samples.
It is important to note that the CES-D scale used in this study measures subclinical depressive symptoms that do not reach the threshold of clinically-diagnosed major depressive disorder (MDD). Subclinical depressive symptoms increase the risk of developing MDD (Cuijpers and Smit, 2004; Tuithof et al., 2018) and share many of the same adverse outcomes (Beekman et al., 1997; Ayuso-Mateos et al., 2010), suggesting that they occupy a transitional position between euthymic mood and MDD (Zhang et al., 2020). However, not all persons with subclinical depressive symptoms go on to develop MDD (Tuithof et al., 2018), and it is possible that the coupling of depressive symptoms with altered brain structure may aid in identifying those who are most at risk of developing MDD and its attendant adverse outcomes. Indeed, older Black adults report high levels of subclinical depressive symptoms but have low rates of diagnosed MDD. This “race paradox in mental health” (Mezuk et al., 2013; Barnes and Bennett, 2014) may be explained by individual differences in sociodemographic and psychosocial risk and protective factors including socioeconomic status, access to mental health treatment, various sources of stress exposure (discrimination, trauma), and psychological resiliency that influence the probability of MDD diagnosis in Black adults (Tobin, 2021). Individual differences in brain integrity might add further explanatory power; perhaps it is only those older Black adults who have certain stress exposures concomitant with compromised white matter integrity that are at highest risk of transitioning to a diagnosis of MDD. While longitudinal studies are needed to replicate and validate this association, it may hold promise as a race-relevant prognostic feature of movement along the spectrum of late-life depression, potentially aiding in early detection of those individuals who are at risk of MDD, and thus optimizing treatment and reducing racial disparities in affective and brain health.
This study had some limitations. First, the models did not adjust for health-related or sociocultural factors. The purpose of this study was to first determine if there is indeed a link between late-life depressive symptoms and reduced white matter structural integrity within older Black adults. Having accomplished that, future studies will examine potential cognitive, medical, sociocultural, biological, and lifestyle moderators of this important association. Further, the regions identified here can be examined in future studies as potential biological mediators of associations between late-life depressive symptoms and important adverse outcomes. Second, the participants in this study reside in the Chicagoland area and are mostly women with an education beyond high school, and thus the results may not generalize to the US community-dwelling, or international older Black population. Third, the study was cross-sectional. Longitudinal studies showing a link between change in depressive symptoms with change in MRI are required before making any conclusions regarding direction of causality. Finally, even though the average number of depressive symptoms was low in the analytic sample, the frequency was not that different from other published work (Skarupski et al., 2005; Barnes et al., 2009, 2012a,b; Wilson et al., 2010; Turner et al., 2015), and could reflect a healthy volunteer effect in cohort studies. Importantly, despite the low frequency of depressive symptoms, a clear pattern of association with reduced structural integrity was demonstrable within a minoritized population that is underrepresented in research and underserved clinically. Future studies are needed to build upon this finding. These limitations notwithstanding, this study had important strengths. First, neuroimaging studies focusing on variation within Black samples are sorely needed but remain exceedingly rare. Second, the sample, which was well-characterized, was drawn from three large and well-established epidemiologic studies of aging and dementia whose recruitment and data collection procedures are harmonized. Third, by controlling for WMHs on a whole-brain and voxel-wise basis, we were able to measure white matter structural compromise over and above the effects of WMHs. Finally, using regionconnect software, we were able to identify the most probable white matter connections traversing regions where abnormal structural integrity was linked to late-life depressive symptoms in older Black adults.
In conclusion, the results of this study demonstrated that late-life depressive symptoms are associated with a discernable pattern of reduced white matter structural integrity in older Black adults. Future studies are needed to build upon these findings with the goal of deepening understanding of within-Black variability in brain-behavior relationships and ultimately applying that knowledge to reduce racial disparities in affective health and treatment.
Data availability statement
The datasets presented in this study can be found in online repositories. The names of the repository/repositories and accession number (s) can be found at: www.radc.edu.
Ethics statement
The studies involving human participants were reviewed and approved by Institutional Review Board of Rush University Medical Center. The patients/participants provided their written informed consent to participate in this study.
Author contributions
DF, KA, SL, and LB: study concept and design and drafting of the manuscript. LB, DB, and KA: acquisition of data. DF, KA, SL, SZ, and LB: analysis and interpretation of data. DF, KA, SL, SZ, ML, SH, VP, NK, DB, and LB: revision of the manuscript for important intellectual content. SL and SZ: statistical analysis. KA, ML, SH, VP, LB, and DB: obtained funding. All authors contributed to the article and approved the submitted version.
Funding
This work was supported by National Institute on Aging grants R01AG56405, R01AG22018, P30AG10161, P30AG72975, R01AG17917, R01AG064233, R01AG052200, R01AG055430, R01AG062711, and K01AG064044; National Institute of Neurological Disorders and Stroke UH3NS100599; and by the Illinois Department of Public Health.
Acknowledgments
Woojeong Bang, Jennifer Poirier, and Alysha Hodges provided statistical programming. We are indebted to the altruism of the participants of MARS, RADC AA Core, and MAP. More information regarding obtaining MARS, RADC AA Core, and/or MAP data for research use can be found at the RADC Research Resource Sharing Hub (www.radc.rush.edu).
Conflict of interest
The authors declare that the research was conducted in the absence of any commercial or financial relationships that could be construed as a potential conflict of interest.
Publisher’s note
All claims expressed in this article are solely those of the authors and do not necessarily represent those of their affiliated organizations, or those of the publisher, the editors and the reviewers. Any product that may be evaluated in this article, or claim that may be made by its manufacturer, is not guaranteed or endorsed by the publisher.
Footnotes
References
Aizenstein, H. J., Baskys, A., Boldrini, M., Butters, M. A., Diniz, B. S., Jaiswal, M. K., et al. (2016). Vascular depression consensus report-a critical update. BMC Med. 14:161. doi: 10.1186/s12916-016-0720-5
Alexander, A. L., Lee, J. E., Lazar, M., and Field, A. S. (2007). Diffusion tensor imaging of the brain. Neurotherapeutics 4, 316–329. doi: 10.1016/j.nurt.2007.05.011
Alexopoulos, G. S. (2019). Mechanisms and treatment of late-life depression. Transl. Psychiatry 9:188. doi: 10.1038/s41398-019-0514-6
Ayuso-Mateos, J. L., Nuevo, R., Verdes, E., Naidoo, N., and Chatterji, S. (2010). From depressive symptoms to depressive disorders: the relevance of thresholds. Br. J. Psychiatry 196, 365–371. doi: 10.1192/bjp.bp.109.071191
Barnes, D. M. (2014). Depression and distress in blacks and whites in the US: results of a systematic review. Compr. Psychiatry 8:e46. doi: 10.1016/j.comppsych.2014.08.004
Barnes, L. L., and Bennett, D. A. (2014). Alzheimer’s disease in African Americans: risk factors and challenges for the future. Health Aff. 33, 580–586. doi: 10.1377/hlthaff.2013.1353
Barnes, L. L., Lewis, T. T., Begeny, C. T., Yu, L., Bennett, D. A., and Wilson, R. S. (2012a). Perceived discrimination and cognition in older African Americans. J. Int. Neuropsychol. Soc. 18, 856–865. doi: 10.1017/S1355617712000628
Barnes, L. L., Mendes de Leon, C. F., Bienias, J. L., Wilson, R. S., Everson-Rose, S. A., and Evans, D. A. (2009). Hostility and change in cognitive function over time in older blacks and whites. Psychosom. Med. 71, 652–658. doi: 10.1097/PSY.0b013e3181a651b3
Barnes, L. L., Shah, R. C., Aggarwal, N. T., Bennett, D. A., and Schneider, J. A. (2012b). The minority aging research study: ongoing efforts to obtain brain donation in African Americans without dementia. Curr. Alzheimer Res. 9, 734–745. doi: 10.2174/156720512801322627
Basser, P. J., and Pierpaoli, C. (1996). Microstructural and physiological features of tissues elucidated by quantitative-diffusion-tensor MRI. J. Magn. Reson. B 111, 209–219. doi: 10.1006/jmrb.1996.0086
Beekman, A. T., Deeg, D. J., Braam, A. W., Smit, J. H., and van Tilburg, W. (1997). Consequences of major and minor depression in later life: a study of disability, well-being and service utilization. Psychol. Med. 27, 1397–1409. doi: 10.1017/S0033291797005734
Bennett, D. A., Buchman, A. S., Boyle, P., Barnes, L. L., Wilson, R. S., and Schneider, J. A. (2018). Religious orders study and rush memory and aging project. J. Alzheimers Dis. 64, S161–S189. doi: 10.3233/JAD-179939
Bennett, I. J., Madden, D. J., Vaidya, C. J., Howard, D. V., and Howard, J. H. (2018). Age-related differences in multiple measures of white matter integrity: a diffusion tensor imaging study of healthy aging. Hum. Brain Mapp. 31, 378–390. doi: 10.1002/hbm.20872
Benuzzi, F., Fausta, L., Duzzi, D., Nichelli, P. F., and Porro, C. A. (2008). Does it look painful or disgusting? Ask your parietal and cingulate cortex. J. Neurosci. 28, 923–931. doi: 10.1523/JNEUROSCI.4012-07.2008
Blakemore, S.-J., and Frith, C. (2003). Self-awareness and action. Curr. Op Neurobiol. 13, 219–224. doi: 10.1016/S0959-4388(03)00043-6
Bogoian, H. R., and Dotson, V. M. (2022). Vascular depression in black Americans: a systematic review of the construct and its cognitive, functional, and psychosocial correlates. Clin. Neuropsychol. 36, 431–461. doi: 10.1080/13854046.2021.1933188
Brickman, A. M., Schupf, N., Manly, J. J., Luchsinger, J. A., Andrews, H., Tang, M. X., et al. (2008). Brain morphology in older African Americans, Caribbean Hispanics, and whites from northern Manhattan. Arch. Neurol. 65, 1053–1061. doi: 10.1001/archneur.65.8.1053
Brothers, R. M., Fadel, P. J., and Keller, D. M. (2019). Racial disparities in cardiovascular disease risk: mechanisms of vascular dysfunction. Am. J. Physiol. Heart Circ. Physiol. 317, H777–H789. doi: 10.1152/ajpheart.00126.2019
Bullmore, E., and Sporns, O. (2009). Complex brain networks: graph theoretical analysis of structural and functional systems. Nat. Rev. Neurosci. 10, 186–198. doi: 10.1038/nrn2575
Callahan, C. M., Wolinsky, F. D., Stump, T. E., Nienaber, N. A., Hui, S. L., and Tierney, W. M. (1998). Mortality, symptoms, and functional impairment in late-life depression. J. Gen. Intern. Med. 13, 746–752. doi: 10.1046/j.1525-1497.1998.00226.x
Carmichael, O., and Newton, R. (2019). Brain MRI findings related to Alzheimer’s disease in older African American adults. Prog. Mol. Biol. Trans. Sci. 165, 3–23. doi: 10.1016/bs.pmbts.2019.04.002
Carnethon, M. R., Pu, J., Howard, G., Albert, M. A., Anderson, C. A. M., Bertoni, A. G., et al. (2017). Cardiovascular health in African Americans: a scientific statement from the American Heart Association. Circulation 136, e393–e423. doi: 10.1161/CIR.0000000000000534
Cho, S., and Hamler, T. C. (2021). Depressive symptoms and multimorbidity: is there an association for older black Americans? J. Aging Health 33, 310–316. doi: 10.1177/0898264320981244
Coloigner, J., Batail, J.-M., Commowick, O., Corouge, I., Robert, G., Barillot, C., et al. (2019). White matter abnormalities in depression: a categorical and phenotypic diffusion MRI study. NeuroImage Clin. 22:101710. doi: 10.1016/j.nicl.2019.101710
Cuijpers, P., and Smit, F. (2004). Subthreshold depression as a risk indicator for major depressive disorder: a systematic review of prospective studies. Acta Psychiatr. Scand. 109, 325–331. doi: 10.1111/j.1600-0447.2004.00301.x
Culham, J. C. C., and Kanwisher, N. G. (2001). Neuroimaging of cognitive functions in human parietal cortex. Curr. Op Neurobiol. 11, 157–163. doi: 10.1016/S0959-4388(00)00191-4
Cummings, D. M., Kirian, K., Howard, G., Howard, V., Yuan, Y., Munter, P., et al. (2016). Consequences of comorbidity of elevated stress and/or depressive symptoms and incident cardiovascular outcomes in diabetes: results from the reasons for geograhic and racial differences in stroke (REGARDS) study. Diabetes Care 39, 101–109. doi: 10.2337/dc15-1174
Deere, B. P., and Ferdinand, K. C. (2020). Hypertension and race/ethnicity. Curr. Opin. Cardiol. 35, 342–350. doi: 10.1097/HCO.0000000000000742
Diniz, B. S., Butters, M. A., Albert, S. M., Dew, M. A., and Reynolds, C. F. (2013). Late-life depression and risk of vascular dementia and Alzheimer’s disease: systematic review and meta-analysis of community-based cohort studies. Br. J. Psychiatry 202, 329–8335. doi: 10.1192/bjp.bp.112.118307
Drevets, W. C., Price, J. L., and Furey, M. L. (2008). Brain structural and functional abnormalities in mood disorders: implications for neurocircuitry models of depression. Brain Struct. Funct. 213, 93–118. doi: 10.1007/s00429-008-0189-x
Erving, C. L., Thomas, C. S., and Frazier, C. (2019). Is the black-white mental health paradox consistent across gender and psychiatric disorders? Am. J. Epidemiol. 188, 314–322. doi: 10.1093/aje/kwy224
Filley, C. M., and Fields, R. D. (2016). White matter and cognition: making the connection. J. Neurophysiol. 116, 2093–2104. doi: 10.1152/jn.00221.2016
Gong, G., He, Y., Concha, L., Lebel, C., Gross, D. W., Evans, A. C., et al. (2009). Mapping anatomical connectivity patterns of human cerebral cortex using in vivo diffusion tensor imaging tractography. Cereb. Cortex 19, 524–536. doi: 10.1093/cercor/bhn102
Gottesman, R. F., Coresh, J., Catellier, D. J., Sharrett, A. R., Rose, K. M., Coker, L. H., et al. (2010). Blood pressure and white-matter disease progression in a biethnic cohort: atherosclerosis risk in communities (ARIC) study. Stroke 41, 3–8. doi: 10.1161/STROKEAHA.109.566992
Griffanti, L., Zamboni, G., Khan, A., Li, L., Bonifacio, G., Sundaresan, V., et al. (2016). BIANCA (brain intensity AbNormality classification algorithm): a new tool for automated segmentation of white matter hyperintensities. NeuroImage 141, 191–205. doi: 10.1016/j.neuroimage.2016.07.018
Hastings, J. F., and Snowden, L. R. (2019). African Americans and Caribbean blacks: perceived neighborhood disadvantage and depression. J. Commun. Psychol. 47, 227–237. doi: 10.1002/jcop.22117
Hughes, T. M., Sink, K. M., Williamson, J. D., Hugenschmidt, C. E., Wagner, B. C., Whitlow, C. T., et al. (2018). Relationships between cerebral structure and cognitive function in African Americans with type 2 diabetes. J. Diabetes Complicat. 32, 916–921. doi: 10.1016/j.jdiacomp.2018.05.017
Kalyani, R. R., Ji, N., Carnethon, M., Bertoni, A. G., Selvin, E., Gregg, E. W., et al. (2017). Diabetes, depressive symptoms, and functional disability in African Americans: the Jackson heart study. J. Diabetes Complicat. 31, 1259–1265. doi: 10.1016/j.jdiacomp.2017.03.003
Kim, Y.-K., and Han, K.-M. (2021). Neural substrates for late-life depression: a selective review of structural neuroimaging studies. Prog. Neuropsychopharmacol. Biol. Psychiatry 104:110010. doi: 10.1016/j.pnpbp.2020.110010
Kohout, F. J., Berkman, L. F., Evans, D. A., and Cornoni-Huntley, J. (1993). Two shorter forms of the CES-D (Center for Epidemiologic Studies Depression) depression symptoms index. J. Aging Health 5, 179–193. doi: 10.1177/089826439300500202
Krishnan, K. R., Hays, J. C., and Blazer, D. G. (1997). MRI-defined vascular depression. Am. J. Psychiatry 154, 497–501. doi: 10.1176/ajp.154.4.497
Lamar, M., Charlton, R. A., Morris, R. G., and Markus, H. S. (2010). The impact of subcortical white matter disease on mood in euthymic older adults: a diffusion tensor imaging study. Am. J. Geriatr. Psychiatry 18, 634–642. doi: 10.1097/JGP.0b013e3181cabad1
Lamar, M., Rubin, L., Ajilore, O., Charlton, R., Zhang, A., Yang, S., et al. (2015). What metabolic syndrome contributes to brain outcomes in African Americans and Caucasian cohorts. Curr. Alzheimer Res. 12, 640–647. doi: 10.2174/1567205012666150701102325
LeBihan, D., Mangin, J. F., Poupon, C., Clark, C. A., Pappata, S., Molko, N., et al. (2001). Diffusion tensor imaging: concepts and applications. J. Magn. Reson. Imaging 13, 534–546. doi: 10.1002/jmri.1076
Leh, S. E., Ptito, A., Chakravarty, M. M., and Strafella, A. P. (2007). Fronto-striatal connections in the human brain: a probabilistic diffusion tractography study. Neurosci. Lett. 419, 113–118. doi: 10.1016/j.neulet.2007.04.049
Liang, J., Xu, X., Quinones, A. R., Bennett, J. M., and Ye, W. (2011). Multiple trajectories of depressive symptoms in middle and late life: racial/ethnic variations. Psychol. Aging 26, 761–777. doi: 10.1037/a0023945
Maclullich, A. M. J., Ferguson, K. J., Reid, L. M., Deary, I. J., Starr, J. M., Seckl, J. R., et al. (2009). Higher systolic blood pressure is associated with increased water diffusivity in normal-appearing white matter. Stroke 40, 3869–3871. doi: 10.1161/STROKEAHA.109.547877
McKhann, G., Drachman, D., Folstein, M., Katzman, R., Price, D., and Stadlan, E. (1984). Clinical diagnosis of Alzheimer’s disease: report of the NINCDS-ADRDA work group under the auspices of Department of Health and Human Services Task Force on Alzheimer’s disease. Neurology 34, 939–944. doi: 10.1212/WNL.34.7.939
Meyer, H. C., and Bucci, D. J. (2016). Neural and behavioral mechanisms of proactive and reactive inhibition. Learn. Mem. 23, 505–514. doi: 10.1101/lm.040501.115
Mezuk, B., Abdou, C. M., Hudson, D., Kershaw, K. N., Rafferty, J. A., Lee, H., et al. (2013). “White box” epidemiology and the social neuroscience of health behaviors: the environmental affordances model. Soc. Ment. Health 3, 79–95. doi: 10.1177/2156869313480892
Morris, Z., Whiteley, W. N., Longstreth, W. T. Jr., Weber, F., Lee, Y. C., Tsushima, Y., et al. (2009). Incidental findings on brain magnetic imaging: systematic review and metaanalysis. BMJ 339:b3016. doi: 10.1136/bmj.b3016
Nguyen, H. T., Kitner-Triolo, M., Evans, M. K., and Zonderman, A. B. (2004). Factorial invariance of the CES-D in low socioeconomic status African Americans compared with a nationally representative sample. Psychiatry Res. 126, 177–187. doi: 10.1016/j.psychres.2004.02.004
Nyquist, P. A., Bilgel, M. S., Gottesman, R., Yanek, L. R., Moy, T. F., Becker, L. C., et al. (2014). Extreme deep white matter hyperintensity volumes are associated with African American race. Cerebrovasc. Dis. 37, 244–250. doi: 10.1159/000358117
Pan, A., Sun, Q., Okereke, O., Rexrode, K. M., and Hu, F. B. (2011). Depression and risk of stroke morbidity and mortality: a meta-analysis and systematic review. JAMA 306, 1241–1249. doi: 10.1001/jama.2011.1282
Panza, F., Frisardi, V., Capurso, C., D’Introno, A., Colacicco, A. M., Imbimbo, B. P., et al. (2010). Late-life depression, mild cognitive impairment, and dementia: possible continuum? Am. J. Geriatr. Psychiatry 18, 98–116. doi: 10.1097/JGP.0b013e3181b0fa13
Parekh, M. B., Gurjarpadhye, A. A., Manoukian, M. A. C., Dubnika, A., Rajadas, J., and Inayathullah, M. (2015). Recent developments in diffusion tensor imaging of brain. Radiol. Open J. 1, 1–12. doi: 10.17140/ROJ-1-101
Pickett, Y. R., Bazelais, K. N., and Bruce, M. L. (2013). Late-life depression in older African Americans: a comprehensive review of epidemiological and clinical data. Int. J. Geriatr. Psychiatry 28, 903–913. doi: 10.1002/gps.3908
Pierpaoli, C, Walker, L, Irfanoglu, MO, Barnett, A, and Batter, PJ. (2020). “TORTOISE: an integrated software package for processing of diffusion MRI data” in Proceedings of the 18th Annual Meeting ISMRM, Stockholm, Sweden.
Prabhakaran, S., Wright, C. B., Yoshita, M., Delapaz, R., Brown, T., DeCarli, C., et al. (2008). Prevalence and determinants of subclinical brain infarction: the northern Manhattan study. Neurology 70, 425–430. doi: 10.1212/01.wnl.0000277521.66947.e5
Price, J. L., Bruce, M. A., and Adinoff, B. (2022). Addressing structural racism in psychiatry with steps to improve psychophysiologic research. JAMA Psychiatry 71, 70–74. doi: 10.1001/jamapsychiatry.2021.2663
Radloff, L. S. (1977). The CES-D scale: a self-report depression scale for research in the general population. Appl. Psychol. Meas. 1, 385–401. doi: 10.1177/014662167700100306
Rajan, K. B., Weuve, J., Barnes, L. L., Wilson, R. S., and Evans, D. A. (2019). Prevalence and incidence of clinically diagnosed Alzheimer’s disease dementia from 1994 to 2012 in a population study. Alzheimers Dement. 15, 1–7. doi: 10.1016/j.jalz.2018.07.216
Rashidi-Ranjbar, N., Miranda, D., Butters, M. A., Mulsant, B. H., and Voineskos, A. N. (2020). Evidence for structural and functional alternations of frontal-executive and corticolimbic circuits in late-life depression and relationship to mild cognitive impairment and dementia: a systematic review. Front. Neurosci. 14:253. doi: 10.3389/fnins.2020.00253
Reinlieb, M. E., Persaud, A., Singh, D., Garcon, E., Rutherford, B. R., Pelton, G. H., et al. (2014). Vascular depression: overrepresented among African Americans? Int. J. Geriatr. Psychiatry 29, 470–477. doi: 10.1002/gps.4029
Robbins, N. M., Charleston, L., Saadi, A., Thayer, Z., Codrington, W. U., and Landry, A. (2022). Black patients matter in neurology. Neurology 99, 106–114. doi: 10.1212/WNL.0000000000200830
Saz, P., and Dewey, M. E. (2001). Depression, depressive symptoms and mortality in persons aged 65 and over living in the community: a systematic review of the literature. Int. J. Geriatr. Psychiatry 16, 622–630. doi: 10.1002/gps.396
Scheuermann, T. S., Saint Onge, J. M., Ramaswamy, M., Cox, L. S., Ahluwalia, J. S., and Nollen, N. L. (2020). The role of neighborhood experiences in psychological distress among African American and white smokers. Race Soc. Probl. 12, 133–144. doi: 10.1007/s12552-020-09281-5
Schmahmann, J. D., Smith, E. E., Eichler, F. S., and Filley, C. M. (2008). Cerebral white matter: neuroanatomy, clinical neurology, and neurobehavioral correlates. Ann. N. Y. Acad. Sci. 1142, 266–309. doi: 10.1196/annals.1444.017
Skarupski, K. A., Mendes de Leon, C. F., Bienias, J. L., Barnes, L. L., Everson-Rose, S., Wilson, R. S., et al. (2005). Black-white differences in depressive symptoms among older adults over time. J. Gerontol. Psychol. Sci. 608, P136–P142. doi: 10.1093/geronb/60.3.p136
Smagula, S. F., and Aizenstein, H. J. (2016). Brain structural connectivity in late-life major depressive disorder. Biol. Psychiatry Cogn. Neurosci. Neuroimag. 1, 271–277. doi: 10.1016/j.bpsc.2015.11.005
Smith, S. M., Jenkinson, M., Johansen-Berg, H., Rueckert, D., Nichols, T. E., MacKay, C. E., et al. (2006). Tract-based spatial statistics: voxelwise analysis of multi-subject diffusion data. NeuroImage 31, 1487–1505. doi: 10.1016/j.neuroimage.2006.02.024
Smith, S. M., and Nichols, T. E. (2009). Threshold-free cluster enhancement: addressing problems of smoothing, threshold dependence and localization in cluster inference. NeuroImage 44, 83–98. doi: 10.1016/j.neuroimage.2008.03.061
Steenland, K., Goldstein, F. C., Levey, A., and Wharton, W. (2016). A meta-analysis of Alzheimer’s disease incidence and prevalence comparing African-Americans and Caucasians. J. Alzheimers Dis. 50, 71–76. doi: 10.3233/JAD-150778
Tadayonnejad, R., and Ajilore, O. (2014). Brain network dysfunction in late-life depression: a literature review. J. Geriatr. Psychiatry Neurol. 27, 5–12. doi: 10.1177/0891988713516539
Taylor, W. D., Aizenstein, H. J., and Alexopoulos, G. S. (2013). The vascular depression hypothesis: mechanisms linking vascular disease with depression. Mol. Psychiatry 18, 963–974. doi: 10.1038/mp.2013.20
Tobin, C. S. S. (2021). Distinguishing distress from disorder: black-white patterns in the determinants of and links between depressive symptoms and major depression. J. Affect. Disord. 279, 510–517. doi: 10.1016/j.jad.2020.10.035
Tuithof, M., ten Have, M., van Dorsselaer, S., Kleinjan, M., Beekman, A., and de Graaf, R. (2018). Course of subthreshold depression into a depressive disorder and its risk factors. J. Affect. Disord. 241, 206–215. doi: 10.1016/j.jad.2018.08.010
Turner, A. D., Capuano, A. W., Wilson, R. S., and Barnes, L. L. (2015). Depressive symptoms and cognitive decline in older African Americans: two scales and their factors. Am. J. Geriatr. Psychiatry 23, 568–578. doi: 10.1016/j.jagp.2014.08.003
Van Agtmaal, M. J. M., Houben, A. J. H. M., Pouwer, F., Stehouwer, C. D. A., and Schram, M. T. (2017). Association of microvascular dysfunction with late-life depression: a systematic review and meta-analysis. JAMA Psychiat. 74, 729–739. doi: 10.1001/jamapsychiatry.2017.0984
Virani, S. S., Alonso, A., Aparicio, H. J., Benjamin, E. J., Bittencourt, M. S., Callaway, C., et al. (2021). Heart disease and stroke statistics—2021 update: a report from the American Heart Association. Circulation 143, e254–e743. doi: 10.1161/CIR.0000000000000950
Waldron-Perrine, B., Kisser, J. E., Brody, A., Haacke, E. M., Dawood, R., Millis, S., et al. (2018). MRI and neuropsychological correlates in African Americans with hypertension and left ventricular hypertrophy. Am. J. Hypertens. 31, 865–868. doi: 10.1093/ajh/hpy060
Walsemann, K. M., Gee, G. C., and Geronimus, A. T. (2009). Ethnic differences in trajectories of depressive symptoms: disadvantage in family background, high school experiences and adult characteristics. J. Health Soc. Behav. 50, 82–98. doi: 10.1177/002214650905000106
Wardlaw, J. M., Valdes-Hernandez, M. C., and Munoz-Maniega, S. (2015). What are white matter hyperintensities made of? Relevance to vascular cognitive impairment. J. Am. Heart. Assoc. 4:e001140. doi: 10.1161/JAHA.114.001140
Wassink-Vossen, S., Collard, R. M., Wardenaar, K. J., Verhaak, P. F. M., Rhebergen, D., Naarding, P., et al. (2019). Trajectories and determinants of functional limitations in late-life depression: a 2-year prospective cohort study. Eur. Psychiatry 62, 90–96. doi: 10.1016/jeurpsy.2019.09.003
Wei, J., Hou, R., Zhang, X., Xu, H., Xie, L., Chandrasekar, E. K., et al. (2019). The association of late-life depression with all-cause and cardiovascular mortality among community-dwelling older adults: systematic review and meta-analysis. Br. J. Psychiatry 215, 449–455. doi: 10.1192/bjp.2019.74
Wen, M.-C., Steffens, D. C., Chen, M.-K., and Zainal, N. H. (2014). Diffusion tensor imaging studies in late-life depression: systematic review and meta-analysis. Int. J. Geriatr. Psychiatry 29, 1173–1184. doi: 10.1002/gps.4129
Whitlow, C. T., Sink, K. M., Divers, J., Smith, S. C., Xu, J., Palmer, N. D., et al. (2015). Effects of type 2 diabetes on brain structure and cognitive function: African American-diabetes heart study MIND. Am. J. Radiol. 36, 1648–1653. doi: 10.3174/ajnr.A4321
Wilson, R. S., Hoganson, G. M., Rajan, K. B., Barnes, L. L., Mendes de Leon, C. F., and Evans, D. A. (2010). Temporal course of depressive symptoms during the development of Alzheimer disease. Neurology 75, 21–6. doi: 10.1212/WNL.0b013e3181e620c5
Winkler, A. M., Ridgway, G. R., Douaud, G., Nichols, T. E., and Smith, S. M. (2016). Faster permutation inference in brain imaging. NeuroImage 141, 502–516. doi: 10.1016/j.neuroimage.2016.05.068
Winkler, A. M., Webster, M. A., Vidaurre, D., Nichols, T. E., and Smith, S. M. (2015). Multi-level block permutation. NeuroImage 123, 253–268. doi: 10.1016/j.neuroimage.2015.05.092
Zahodne, L. B., Manly, J. J., Narkhede, A., Griffith, E. Y., DeCarli, C., Schupf, N. S., et al. (2015). Structural MRI predictors of late-life cognition differ across African Americans, Hispanics, and whites. Curr. Alzheimer Res. 12, 632–639. doi: 10.2174/1567205012666150530203214
Zhang, S., and Arfanakis, K. (2018). Evaluation of standardized and study-specific diffusion tensor imaging templates of the adult human brain: Template characteristics, spatial normalization accuracy, and detection of small inter-group FA differences. Neuroimage 172, 40–50. doi: 10.1016/j.neuroimage.2018.01.046
Keywords: Black, African-American, late-life depressive symptoms, brain structure, diffusion-tensor imaging
Citation: Fleischman DA, Arfanakis K, Leurgans SE, Zhang S, Lamar M, Han SD, Poole VN, Kim N, Bennett DA and Barnes LL (2023) Late-life depressive symptoms and white matter structural integrity within older Black adults. Front. Aging Neurosci. 15:1138568. doi: 10.3389/fnagi.2023.1138568
Edited by:
Anja Soldan, Johns Hopkins University, United StatesReviewed by:
Derek Archer, Vanderbilt University Medical Center, United StatesElizabeth Moore, Vanderbilt University, United States
Anouk Geraets, University of Luxembourg, Luxembourg
Ana Paula Mendes-Silva, University of Toronto, Canada
Copyright © 2023 Fleischman, Arfanakis, Leurgans, Zhang, Lamar, Han, Poole, Kim, Bennett and Barnes. This is an open-access article distributed under the terms of the Creative Commons Attribution License (CC BY). The use, distribution or reproduction in other forums is permitted, provided the original author(s) and the copyright owner(s) are credited and that the original publication in this journal is cited, in accordance with accepted academic practice. No use, distribution or reproduction is permitted which does not comply with these terms.
*Correspondence: Debra A. Fleischman, RGVicmFfRmxlaXNjaG1hbkBydXNoLmVkdQ==