- Department of Anesthesiology, Shengjing Hospital of China Medical University, Shenyang, Liaoning, China
Extracellular vesicles (EVs) including exosomes are vesicular vesicles with phospholipid bilayer implicated in many cellular interactions and have the ability to transfer multiple types of cargo to cells. It has been found that EVs can package various molecules including proteins and nucleic acids (DNA, mRNA, and noncoding RNA). The discovery of EVs as carriers of proteins and various forms of RNA, such as microRNAs (miRNA) and long noncoding RNAs (lncRNA), has raised great interest in the field of drug delivery. Despite the underlying mechanisms of neuropathic pain being unclear, it has been shown that uncontrolled glial cell activation and the neuroinflammation response to noxious stimulation are important in the emergence and maintenance of neuropathic pain. Many studies have demonstrated a role for noncoding RNAs in the pathogenesis of neuropathic pain and EVs may offer possibilities as carriers of noncoding RNAs for potential in neuropathic pain treatment. In this article, the origins and clinical application of EVs and the mechanism of neuropathic pain development are briefly introduced. Furthermore, we demonstrate the therapeutic roles of EVs in neuropathic pain and that this involve vesicular regulation of glial cell activation and neuroinflammation.
1. Introduction
Neuropathic pain is defined as “pain initiated or caused by a primary lesion or dysfunction of the nervous system” by the International Association for the Study of Pain (IASP). Neuropathic pain can be classified into peripheral and central pain based on the sources of the lesion or disease (Scholz et al., 2019). Nociceptors that are defined as primary afferent sensory neurons can be activated by noxious stimuli and transmit pain signals to SDH (Basbaum et al., 2009). The imbalance between synaptic excitation and inhibition in the SDH leads to aberrant somatosensory signals which are subsequently conveyed to the brainstem and higher brain regions (Tsuda et al., 2017). Up to 8% of the general population suffer from neuropathic pain, which is refractory to recent clinical therapies and seriously affects their quality of life. Therefore, novel, efficacious treatment strategies are urgently needed, but the exact molecular and cellular mechanisms underlying neuropathic pain remain unclear. A large part of cell–cell communications and molecular signaling pathways come into play during regulation of the sensitization of nociceptive pathways. The development and maintenance of neuropathic pain is coupled with the interactions between neurons-glial cells, and neuronal-immune cells (Vicario et al., 2020; Yu et al., 2020b).
EVs were identified as particles naturally released from the cell that are delimited by a lipid bilayer and cannot replicate (Théry et al., 2018). EVs are classified typically based on their size, biogenesis, and biophysical properties: exosomes, microvesicles and apoptotic bodies, many of which show pleiotropic biological functions (Rufino-Ramos et al., 2017; O’Brien et al., 2020). Exosomes are a specific EVs subtype which are considered to be of endosome-origin and ∼30 to ∼200 nm in diameter (Pegtel and Gould, 2019). EVs were initially thought to function only as cellular ‘garbage’ disposals, but with accumulating evidence concerning their origin, composition and transportation, and intercellular signal transmission, it has become clear that EVs have multiple functions. EVs that mediate autocrine, paracrine, and endocrine effects are taken up by surrounding cells or circulate in the blood and are eventually taken up by distal cells (El Andaloussi et al., 2013). EVs with different cargo act as master switches orchestrating both immune and neuronal processes, suggesting that they are involved in many pathophysiological processes such as neuropathic pain (Barile and Vassalli, 2017; Sosanya et al., 2020). EVs derived from different cells contain different cargo compositions including nucleic acids, proteins, lipids, amino acids, and metabolites and participate in numerous physical and pathological processes (Zhang Y. et al., 2019). Studies found that exosomes secreted from specific areas are involved in human neuropathic pain conditions such as mPFC and NAc can enhance allodynia in mouse CCI models (Yu et al., 2020a). In this review, we explore the molecular and cellular mechanisms and therapeutic potential of EVs for neuropathic pain. Because of the hard differentiation, the exosome terminology is used if clarified in the references and the term EV is used if the differentiation is unclear here.
2. Extracellular vesicles
2.1. Biogenesis of EVs
EVs are formed when the plasma membrane double invaginates and eventually generates the MVB, which can be degraded in lysosomes or fuse with cell membranes to release inclusions as EVs (Bebelman et al., 2018; Kalluri and LeBleu, 2020). The biogenesis and transport of EVs are associated with a large number of proteins, including ESCRT, Ras-related proteins GTPase Rab, ceramide, and phospholipids (Trajkovic et al., 2008; Mathieu et al., 2019). Exosomes are made of lipid bilayer structures and appear biconcave or cup-shaped when produced by artificially drying during TEM (Yellon and Davidson, 2014; Lobb et al., 2015). Bilayer lipid vesicles in solution are nearly spherical. The method of purification and processing for EM can lead artifactual observation of different shapes (Chernyshev et al., 2015). EVs generated by cells including neurons, glial cells and immune cells can promote cell-to-cell communication and regulate biological processes after nerve injury (López-Leal et al., 2020). In addition, MSCs including bone marrow MSCs, gingival MSCs, adMSCs, and hucMSCs can release EVs to regulate the pathological progression after nerve injury (Bryk et al., 2022; Zhang Y. U. et al., 2022). EVs derived from different cells contain different signaling molecules and surface antigens as well as different cargos including unique proteins, lipids, and genetic material (Toh et al., 2018). This intercellular communication involves two different mechanisms an ESCRT-dependent mechanisms and an ESCRT-independent mechanisms (D'Agnelli et al., 2020). EVs can affect phenotypic and molecular alterations of receptor cells by direct or indirect delivery of signaling molecules to receptor cells through binding with cell surface receptors and receptor-mediated endocytosis or direct membrane fusion (Meldolesi, 2018; Van Niel et al., 2018; Figure 1).
Within the endosomal system, cargos including proteins and nucleic acids are internalized from extracellular space. Cargos are sorted into early endosomes and then mature into MVB through a process that involves interactions with the Golgi complex. MVB can be degraded in lysosomes or fuse with cell membranes to release inclusions as EVs. The biogenesis and transport of EVs are associated with ESCRT and non-ESCRT. ER, endoplasmic reticulum; MVB, multivesicular bodies.
2.2. Clinical application of EVs
As biomarkers for diagnose and treatments, EVs are at an emerging stage of clinical application. EVs mediating intercellular communication trigger immune responses, inflammatory responses, and many other processes (Harrell et al., 2019; Qiu et al., 2021; Ashrafizadeh et al., 2022). Exosomes presenting in biological fluids and carrying specific membrane proteins are internalized by recipient cell through soluble and juxtacrine signaling events, fusion, and receptor/raft-mediated endocytosis, as well as phagocytosis, and regulate multiple physiological and pathological processes (Hu et al., 2020). The characteristic properties of exosomes during delivery of functional cargos is that they can target specific cells, which also favors their use as therapeutic vehicles (Hessvik and Llorente, 2018). Exosomes affect neuroinflammation and neuropathic pain by delivering proteins, mature miRNAs, and translatable transcripts (Ramanathan and Ajit, 2016). MiR-25-3p, miR-320B, miR-93, miR-126-3p, and RNU48 from serum-derived exosomes in patients with complex regional pain syndrome show significant differential expression (McDonald et al., 2014). Carrying miR-181c-5p containing exosomes repress CCI-induced neuropathic pain through inhibition of neuroinflammation (Zhang Y. U. et al., 2022), which provided the therapeutic rationale for studying EVs to treat neuropathic pain.
3. Pathogenesis of neuropathic pain
Neuropathic pain constitutes a significant proportion of all chronic pain suffers. Most patients with neuropathic pain complain of spontaneous pain, hyperalgesia (abnormal hypersensitivity to stimuli), and allodynia (nociceptive responses to non-noxious stimuli under normal states). Studies have shown that the disappearance of myelin breakdown after peripheral nerve injury-induced inflammation is critical for axon regeneration (Ying et al., 2018). Their presence impedes nerve repair. The pain signals are transmitted into the central nervous system, including the SDH by the primary afferent nociceptors on DRGs (Navarro et al., 2007; Chen et al., 2020). Moreover, with SDH synthesizing and releasing a variety of classical inflammatory mediators, nociceptor sensitization leads to ectopic firing and erroneous conduction velocities which changes the sensitization of supraspinal nociceptor neurons through ascending transmission, and finally causes neuropathic pain (Macone and Otis, 2018). The sprouting nociceptive fibers terminate in an unusual way in the denervated skin, with significant allodynia occurring months after nerve injury (Gangadharan et al., 2022). Evidence has added to a growing appreciation of the complex link between neuropathic pain and nerve regeneration (Xie et al., 2017). Changes in ion channels, activation of immune cells, compounds released by glial cells, and epigenetic modifications are all involved in neuropathic pain (Finnerup et al., 2021). Furthermore, mast cells, macrophages, neutrophils, and their released mediators are associated with neuropathic pain. Agtr2-expressing macrophages at the site of nerve injury triggers neuropathic pain caused predominantly by immune cells (Shepherd et al., 2018).
Neuroinflammation which is a localized form of inflammation in the peripheral nervous and central nervous systems is becoming increasingly implicated in neuropathic pain (Ji et al., 2014; Jiang et al., 2020). In the DRG, nerve injury not only causes neuronal changes but also leads to the activation of peripheral glial cells such as Schwann cells and satellite glial cells, eventually leading to neuroinflammation and neuropathic pain (Ji et al., 2013; Yuan et al., 2020). Activated central glial cells also cause diverse alterations that change neural excitability, leading to the development of neuropathic pain (Kohno and Tsuda, 2021).
The discovery of extensive epigenetic regulation such as noncoding RNA modification, DNA methylation, and histone acetylation provides a novel perspective on the mechanism of neuropathic pain. Noncoding RNAs, are an important class of RNA molecule that typically does not encode detectable proteins, may regulate neuropathic pain via multiple and complex mechanisms. Peripheral noxious stimuli drives expressional changes in noncoding RNAs, such as miRNA, lncRNA, and circRNA, and these changes are associated with the aberrant expression of its target mRNA and the occurrence and development of neuropathic pain (Li L. et al., 2019; Zhang S. B. et al., 2019). Specifically, miRNA and lncRNA can be functionally shuttled between cells via exosomes and are termed exosomal shuttle RNA (Valadi et al., 2007), and how these exosomes function as exosomal shuttle RNA carriers in neuropathic pain represents our next research question.
4. Extracellular vesicles regulate neuropathic pain
4.1. Extracellular vesicles regulate neuropathic pain via glial cell activation
Glial cells, also known as neuroglia, modulate neurotransmission at the synaptic level. Now it has been found that glial cells play an important role in neuroinflammation and neuropathic pain (Tsuda and Inoue, 2016; Hirbec et al., 2020). Glial cells are located in DRGs, spinal cord and brain, and show a series of changes such as a shifting phenotype and release of inflammatory mediators in response to a damaging signal within the nervous system to drive central and peripheral sensitization and participate in neuropathic pain evolution (Chen et al., 2018; Matsuda et al., 2019).
Microglia (macrophages residing in the central nervous system) are sensors for the detection of abnormal alterations in response to internal and external insults in the central nervous system (Ji et al., 2016). Studies have found that in SCI models exosomes were delivered to the injury site and significantly enhance axonal regeneration, reduce cell apoptosis, and reduce activation of microglia and astrocytes (Guo et al., 2019). MSC-derived EVs can promote phenotype transformation and function of macrophages/microglia from pro-inflammatory M1 to anti-inflammatory M2 types. The activation of glial cells requires the participation of pro-inflammatory factors which may be related to miRNAs in exosomes, such as miR-34a-5p, miR-21, and miR146a-5p (Domenis et al., 2018). Previous studies have shown that TBI is associated with neuropathic pain (Leung, 2020). Exosomes mediate neuron-glial cell intercellular communication and following TBI, microglia reduce the release of exosomes containing miR-5,121 to inhibit neurite outgrowth and synapse recovery of neurons (Zhao et al., 2021).
The expression of lncGm37494 targeting miR-130b-3p to regulate microglia polarization is increased in exosomes secreted by ADSCs under hypoxia, and has been suggested that exosomes can repair SCI by delivering lncGm37494 (Shao et al., 2020). Intravenous administration of hucMSC-derived exosomes significantly inhibits the transcription levels of astrocytes and microglia, suggesting a reduced inflammatory state from SCI (Kang and Guo, 2022), and single and continuous intrathecal infusion of hucMSC-derived exosomes after SNL can prevent and reverse neuropathic pain. Moreover, it has been found that the analgesic effects of exosomes may involve their actions on neurons and glial cells (Shiue et al., 2019).
As the most abundant glial cell in the peripheral nervous system, Schwann cells undergo phenotypic modulation, proliferate and interact with nociceptive neurons by releasing glial mediators (growth factors and cytokines) after nerve injury. These dramatic alterations in Schwann cells promote nerve regeneration and eventually influence neuropathic pain (Dubový, 2011; Wei et al., 2019). During neuropathic pain, many receptors on Schwann cells are differentially expressed, such as P2X4R, HCAR2 (Boccella et al., 2019; Su et al., 2019). Recent studies have proved the presence of growth factors such as BDNF, NGF, FGF-1, IGF-1, and GDNF in adMSC exosomes. Furthermore, adMSCs-derived exosomes which internalized into the Schwann cells exhibited the ability to promote Schwann cell proliferation in vitro and enhance nerve regeneration following SNI in vivo (Bucan et al., 2019), and interestingly, BDNF and NGF have been identified to correlate with neuropathic pain (Dai et al., 2020; Wu et al., 2021). In addition, exosomal miR-21 was shown to promote the Schwann cell proliferation and the expression of NGF, BDNF, and GDNF after peripheral nerve injury. And exosomal miR-21 secreted by Schwann cells can promote neurite outgrowth and functional repair after SNI (Liu Y. P. et al., 2022; Figure 2).
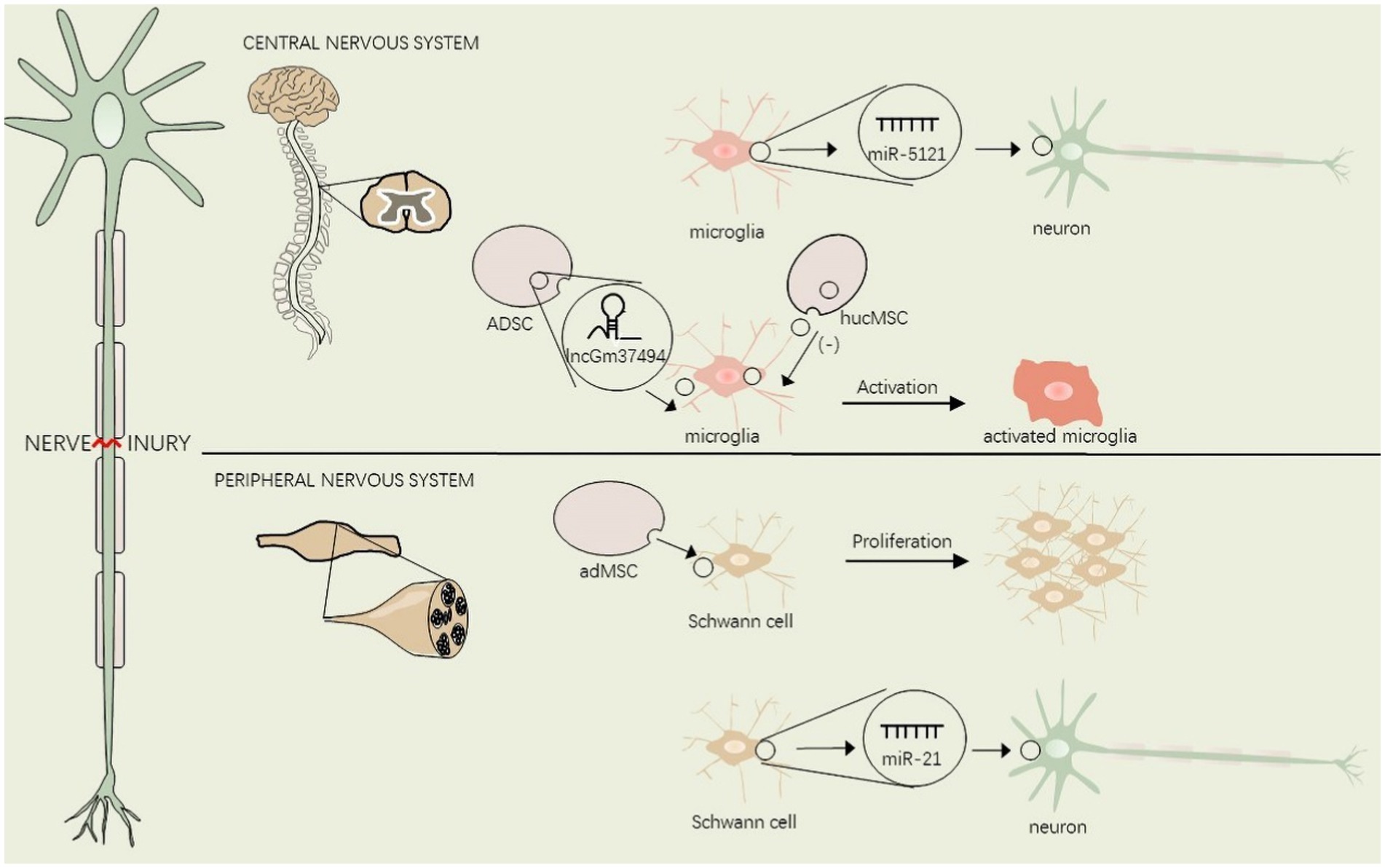
Figure 2. EVs regulate glial cell activation in the central and peripheral nervous system after nerve injury.
Lesions of the nervous system from the peripheral nociceptor to the spinal cord and brain may give rise to neuropathic pain. This figure shows the interaction between EVs and glial cells in the central and peripheral nervous system. adMSC, adipose-derived mesenchymal stem cells, hucMSC, human umbilical cord mesenchymal stem cells, ADSC, Adipose tissue-derived mesenchymal stem/stromal cells.
4.2. Extracellular vesicles regulate neuropathic pain via neuroinflammation
Neuroinflammatory activation of glial cells and immune cells after nerve injury is being increasingly implicated in neuropathic pain (Chambel et al., 2020; Karri et al., 2022). EVs pose tremendous anti-inflammatory capacity following nerve injury and the roles of EVs in neuropathic pain will be a particularly interesting area of future inquiry.
EF-MSCs secrete EVs to repress NLRP3 inflammasome activation and to inhibit the expression of inflammatory cytokines related to neuroinflammation after SCI (Huang et al., 2020). Macrophages change their polarization to anti-inflammatory phenotype which can effectively control the development of inflammation (Ma et al., 2020). HucMSC-derived exosomes can stimulate bone marrow-derived macrophages to transform into M2 type, which can also inhibit inflammation through decreasing inflammatory factors such as TNF-α, MIP-1α, IL-6 and IFN-γ (Sun et al., 2018). MSC derived exosomes can relieve microglia/macrophage and astrocyte-mediated neuroinflammation in rats after TBI (Zhang et al., 2020). Studies suggest a critical role for proteins in the mediation of signaling mechanisms underlying neuropathic pain after SNI. Furthermore, the compliment protein C5a and ICAM-1 were found to be significantly up-regulated in EVs purified from SNI mouse serum (Jean-Toussaint et al., 2020). C5a can help induce the expression of inflammatory factors in microglia and promote the development of their inflammatory status (Liu et al., 2018). Furthermore, C5a and its receptor C5aR can simultaneously participate in the process of neuropathic pain (Quadros and Cunha, 2016).
MSC-derived EVs can facilitate macrophage/microglia polarization from M1 to M2 phenotype. miR-21 and miR-19b in EVs derived from differentiated PC12 cells and MSCs can suppress apoptosis of neurons by decreasing the expression of PTEN which can be applied during post-SCI repair (Xu et al., 2019). We found that miR-216a-5p inside MSC-derived exosomes targeted TLR4 and shifted this M1/M2 polarization to inhibit neuroinflammation after SCI which was mediated by microglial activation (Liu et al., 2020). Exosomes derived from huc-MSC were found to deliver miR-199a-3p/miR-145-5p to neurons causing attenuation of the release of inflammatory factors and promotion of neuronal neurite outgrowth following SCI (Wang et al., 2021). circRNA is a type of noncoding RNA that forms a covalently closed continuous loop. Many of them are enriched in EVs, but whether EV circRNAs regulate neuropathic pain is still a shortage of investigation (Marangon et al., 2023). Circ_003564, a recently discovered circRNA, acted as a critical effector in BMSC-exosome-mediated pyroptosis in the SCI model and these exosomes containing circ_003564 may contribute to treatment efficacy in SCI (Zhao et al., 2022). lncRNA H19 in BMSC derived exosomes can further regulate microglia polarization to release neuroinflammatory signals via sponging miR-29b-3p (Zong et al., 2021). Significantly, the treatment using lncRNA TCTN2-modified exosomes significantly improved functional in vivo recovery in SCI rats and alleviated LPS-induced inflammation in vitro (Liu J. et al., 2022; Figure 3).
This figure illustrates that multiple cells secrete EVs to glial cell and immune cell to mediate neuroinflammation after nerve injury. EF-MSC: epidural fat-mesenchymal stem cells, MSC: mesenchymal stem cells, hucMSC: human umbilical cord mesenchymal stem cells.
4.3. Extracellular vesicles regulates neuropathic pain via miRNA
Diverse evidence has shown that RNA molecules inside EVs are generally less than 300 nt in length which is smaller than human mRNA, indicating a role for noncoding RNA (Chen et al., 2010). Furthermore, miRNAs represent an important cargo type in EVs can obstruct the expression of target genes by inhibiting target mRNA translation and accelerate mRNA degradation. These miRNA are protected from RNase by the exosomes or cellular membranes even in extreme cases (Koga et al., 2011). The miRNAs enriched in EVs are phagocytosed by receptor cells and can mediate cell-to-cell communication thus participating in the occurrence and maintenance of neuropathic pain (Sosanya et al., 2020).
The expression levels of miR-21 in exosomes derived from blood serum is significantly increased in the pSNL model, and exosomal miR-21 is likely to be a diagnostic biomarker for neuropathic pain (Hori et al., 2016). This confirmed that SNI induced upregulation of miR-21-5p, let7b, miR-124, and miR-134 expression in the lumbar DRG and DRG sensory neurons can release exosomes containing miR-21-5p which were subsequently internalized by macrophages and converting them to the M1 type. The study also indicated that intrathecal injection of the miR-21 antagomir inhibits hyperalgesia and relieves neuropathic pain (Simeoli et al., 2017).
Intravenous and intrathecal administration of MSCs effectively relieves neuropathic pain following CCI or SCI (Yousefifard et al., 2016; Al-Massri et al., 2019) and MSC exosome therapy has shown the same functions as MSCs therapy, such as anti-inflammation, regulation of the immune system, and regulation of cell differentiation (Lee et al., 2021). In CCI rats, miR-181c-5p from MSC-derived exosomes were found to be significantly downregulated in a time-dependent manner. Intrathecal administration of exosomal miR-181c-5p which targets microglia and inhibits the secretion of inflammatory factors alleviated neuropathic pain and the neuroinflammatory response after CCI (Zhang Y. U. et al., 2022).
In the CCI model, downregulated miR-183 in the sciatic nerve targeting transcription factor FoxO1 and tight junction protein claudin-5 promoted mechanical hypersensitivity. Exosomal miR-183 was found decreased from sera of complex regional pain syndrome patients and can regulate neuropathic pain state via regulating microvascular barrier (Reinhold et al., 2022). In conclusion, our study assesses exosomal noncoding RNAs as one modifier of neuropathic pain (Table 1).
5. Conclusion and perspectives
The main manifestations of neuropathic pain such as spontaneous pain, hyperalgesia, and hyperalgesia, will last for long periods (Kuner and Flor, 2016). Primary sensory neurons show alternations in ion channels and pain-related gene expression, and after receiving noxious stimuli in the peripheral nervous system. Then pain signals are transmitted upwards to the spinal cord and brain, resulting in central sensitization which is an increased responsiveness to nociceptive neurons to normal or subthreshold afferent input in the central nervous system (Zhang J. et al., 2021). Currently there is no general consensus for the underlying mechanism of neuropathic pain as it is complex and multifactorial. In recent years cell transplantation therapy has received widespread attention in the clinical literature. Pluripotent stem cell-derived GABAergic neuron transplants were shown to relieve neuropathic pain induced by both peripheral and central nerve injuries (Dugan et al., 2020; Manion et al., 2020). However, concerns have been raised about the safety of cell transplantation therapy for clinical use, because cell-therapy presents inherent risks, such as microvascular obstruction, malignant tumor formation, and high cost (Jeong et al., 2011; Lou et al., 2017). The occurrence and development of neuropathic pain is accompanied by intercellular signal transduction. EVs were demonstrated to promote cell activation, regulate neuroinflammation and immune response, and promote angiogenesis and axonal growth after nerve injury (Yu et al., 2021). Exosomes, representing a subtype of EVs, have also been a popular studied paracrine topic during the past two decades and are used as a treatment strategies for neuropathic pain (Dong et al., 2019). Exosomes are efficient and safe nanocarriers for pain-targeted gene therapy via intranasal delivery and tail vein injection because of their small molecular weight, ease of transit across the blood–brain barrier and other advantages (Sun et al., 2018; Guo et al., 2019). The use of exosomes could radically eliminate the risk of rejection and offer substantial neuroprotective effects with potential immunoprotective and anti-inflammatory roles (Shojaei et al., 2019).
EVs hold great potential for clinical application with their excellent biocompatibility and bi-layered lipid structures, but the clinical application of EVs faces many challenges, including low yield, impurity and loading efficiency (Tran et al., 2020). Engineered EVs carrying therapeutic molecules hold promise as alternative therapies. The barriers to translation of exosomes remain the difficulty to precisely target the specific cell while limiting off-target biodistribution and the presence of naturally incorporated cellular genetic impurities with potential immunogenicity (Ha et al., 2016; Bunggulawa et al., 2018; Gurung et al., 2021). Specific, loosely associated, proteins and lipids may be lost or masked during exosome isolation, and typical isolation protocols are not specific for exosomes which may influence their targeting behaviors (Kooijmans et al., 2016). With the expanded interest in the field, studies focus on an easy and efficient strategy to broaden, alter or enhance exosome targeting capability. Previous reports have suggested that surface modifications can increase the delivery ability of exosomes (Armstrong et al., 2017; Tian et al., 2018). The low yield of exosomes is a bottleneck for clinical translation. It has shown that knocking down of Rab4 gene and optimization of culture conditions such as supplementing red cell membrane particles can augment the production of exosomes per cell without sacrificing the therapeutic efficacy (Zhang R. et al., 2022).
Glial cells have been found to be a vital component of neuropathic pain and are widely activated during the pain conditions. It is demonstrated that glial cells proliferate, change their morphology and function, change the expression of pain-related genes, and release diffusible factors to affect other cells after a noxious stimulation (Li T. et al., 2019). Activated glial cells release inflammatory factors, such as TNF-α and IL-6, to promote neuroinflammation, and lead to constant painful irritation of the sensory nerves. Studies have provided evidence that microglia can shed new light on the underlying molecular and cellular mechanisms of neuropathic pain (Inoue and Tsuda, 2018). EVs secreted by peripheral macrophages regulate microglial polarization and increase microglia autophagy after SCI via the PI3K/AKT/mTOR signaling pathway (Zhang B. et al., 2021).
As a hot topic in epigenetic research, noncoding RNAs can be encapsulated by EVs and target receptor cells in a paracrine manner to regulate a variety of diseases processes. Recently, several studies have demonstrated that vesicular miRNAs can regulate neuropathic pain. Exosome-encapsulated miR-21 can regulate the occurrence and development of neuropathic pain (Simeoli et al., 2017). Compelling evidence supports the existence of lncRNA and circRNA in body fluids have further clinical implications (Wang et al., 2019; Liu J. et al., 2022). However, the effects and clinical application of vesicular lncRNA and circRNA in neuropathic pain need further exploration.
Author contributions
KZ conceived the idea and wrote the initial draft of the manuscript. PL and YJ prepared the figures and table. ML and JJ performed the analysis with constructive discussions. All authors read and approved the final manuscript.
Funding
The work was supported by Natural Science Foundation of Liaoning Province (2022-YGJC-37) and 345 Talent Project of Shengjing Hospital.
Conflict of interest
The authors declare that the research was conducted in the absence of any commercial or financial relationships that could be construed as a potential conflict of interest.
Publisher’s note
All claims expressed in this article are solely those of the authors and do not necessarily represent those of their affiliated organizations, or those of the publisher, the editors and the reviewers. Any product that may be evaluated in this article, or claim that may be made by its manufacturer, is not guaranteed or endorsed by the publisher.
Abbreviations
EVs, extracellular vesicles; ESE, early-sorting endosome; MVBs, multivesicular bodies; CCI, chronic constriction injury; SCI, spinal cord injury; SNI, spared nerve injury; ICAM-1, intercellular adhesion molecule-1; adMSCs, adipose-derived mesenchymal stem cells; ADSC, Adipose tissue-derived mesenchymal stem/stromal cells; EF-MSC, epidural fat-mesenchymal stem cells; NF200, neurofilament protein 200; GAP-43, growth-associated protein-43; hucMSC, human umbilical cord mesenchymal stem cells; mPFC, medial prefrontal cortex; NAc, nucleus accumbens; TNF-α, tumor necrosis factor-alpha; IL-6, interleukin-6; SDH, spinal dorsal horn; TBI, traumatic brain injury; AT2R, type 2 angiotensin II receptor; NGF, Nerve growth factor; BMSC, bone marrow mesenchymal stem cell; TEM, transmission electron microscopy; circRNA, circular RNA.
References
Al-Massri, K. F., Ahmed, L. A., and El-Abhar, H. S. (2019). Mesenchymal stem cells therapy enhances the efficacy of pregabalin and prevents its motor impairment in paclitaxel-induced neuropathy in rats: role of Notch1 receptor and Jak/stat signaling pathway. Behav. Brain Res. 360, 303–311. doi: 10.1016/j.bbr.2018.12.013
Armstrong, J. P., Holme, M. N., and Stevens, M. M. (2017). Re-engineering extracellular vesicles as smart Nanoscale therapeutics. ACS Nano 11, 69–83. doi: 10.1021/acsnano.6b07607
Ashrafizadeh, M., Kumar, A. P., Aref, A. R., Zarrabi, A., and Mostafavi, E. (2022). Exosomes as promising nanostructures in diabetes mellitus: from insulin sensitivity to ameliorating diabetic complications. Int. J. Nanomedicine 17, 1229–1253. doi: 10.2147/IJN.S350250
Barile, L., and Vassalli, G. (2017). Exosomes: therapy delivery tools and biomarkers of diseases. Pharmacol. Ther. 174, 63–78. doi: 10.1016/j.pharmthera.2017.02.020
Basbaum, A. I., Bautista, D. M., Scherrer, G., and Julius, D. (2009). Cellular and molecular mechanisms of pain. Cells 139, 267–284. doi: 10.1016/j.cell.2009.09.028
Bebelman, M. P., Smit, M. J., Pegtel, D. M., and Baglio, S. R. (2018). Biogenesis and function of extracellular vesicles in cancer. Pharmacol. Ther. 188, 1–11. doi: 10.1016/j.pharmthera.2018.02.013
Boccella, S., Guida, F., De Logu, F., De Gregorio, D., Mazzitelli, M., Belardo, C., et al. (2019). Ketones and pain: unexplored role of hydroxyl carboxylic acid receptor type 2 in the pathophysiology of neuropathic pain. FASEB J. 33, 1062–1073. doi: 10.1096/fj.201801033R
Bryk, M., Karnas, E., Mlost, J., Zuba-Surma, E., and Starowicz, K. (2022). Mesenchymal stem cells and extracellular vesicles for the treatment of pain: current status and perspectives. Br. J. Pharmacol. 179, 4281–4299. doi: 10.1111/bph.15569
Bucan, V., Vaslaitis, D., Peck, C. T., Strauß, S., Vogt, P. M., and Radtke, C. (2019). Effect of exosomes from rat adipose-derived mesenchymal stem cells on neurite outgrowth and sciatic nerve regeneration after crush injury. Mol. Neurobiol. 56, 1812–1824. doi: 10.1007/s12035-018-1172-z
Bunggulawa, E. J., Wang, W., Yin, T., Wang, N., Durkan, C., Wang, Y., et al. (2018). Recent advancements in the use of Exosomes as drug delivery systems. J. Nanobiotechnol. 16:81. doi: 10.1186/s12951-018-0403-9
Chambel, S. S., Tavares, I., and Cruz, C. D. (2020). Chronic pain after spinal cord injury: is there a role for neuron-immune dysregulation? Front. Physiol. 11:748. doi: 10.3389/fphys.2020.00748
Chen, T. S., Lai, R. C., Lee, M. M., Choo, A. B., Lee, C. N., and Lim, S. K. (2010). Mesenchymal stem cell secretes microparticles enriched in pre-Micrornas. Nucleic Acids Res. 38, 215–224. doi: 10.1093/nar/gkp857
Chen, T., Li, Y., Ni, W., Tang, B., Wei, Y., Li, J., et al. (2020). Human neural stem cell-conditioned medium inhibits inflammation in macrophages via Sirt-1 signaling pathway in vitro and promotes sciatic nerve injury recovery in rats. Stem Cells Dev. 29, 1084–1095. doi: 10.1089/scd.2020.0020
Chen, G., Zhang, Y. Q., Qadri, Y. J., Serhan, C. N., and Ji, R. R. (2018). Microglia in pain: detrimental and protective roles in pathogenesis and resolution of pain. Neuron 100, 1292–1311. doi: 10.1016/j.neuron.2018.11.009
Chernyshev, V. S., Rachamadugu, R., Tseng, Y. H., Belnap, D. M., Jia, Y., Branch, K. J., et al. (2015). Size and shape characterization of hydrated and desiccated exosomes. Anal. Bioanal. Chem. 407, 3285–3301. doi: 10.1007/s00216-015-8535-3
D'agnelli, S., Gerra, M. C., Bignami, E., and Arendt-Nielsen, L. (2020). Exosomes as a new pain biomarker opportunity. Mol. Pain 16:1744806920957800. doi: 10.1177/1744806920957800
Dai, W. L., Yan, B., Bao, Y. N., Fan, J. F., and Liu, J. H. (2020). Suppression of peripheral Ngf attenuates neuropathic pain induced by chronic constriction injury through the Tak1-Mapk/Nf-Κb signaling pathways. Cell Commun. Signal 18:66. doi: 10.1186/s12964-020-00556-3
Domenis, R., Cifù, A., Quaglia, S., Pistis, C., Moretti, M., Vicario, A., et al. (2018). Pro inflammatory stimuli enhance the immunosuppressive functions of adipose mesenchymal stem cells-derived exosomes. Sci. Rep. 8:13325. doi: 10.1038/s41598-018-31707-9
Dong, R., Liu, Y., Yang, Y., Wang, H., Xu, Y., and Zhang, Z. (2019). Msc-derived Exosomes-based therapy for peripheral nerve injury: a novel therapeutic strategy. Biomed. Res. Int. 2019:6458237. doi: 10.1155/2019/6458237
Dubový, P. (2011). Wallerian degeneration and peripheral nerve conditions for both axonal regeneration and neuropathic pain induction. Ann. Anat. 193, 267–275. doi: 10.1016/j.aanat.2011.02.011
Dugan, E. A., Jergova, S., and Sagen, J. (2020). Mutually beneficial effects of intensive exercise and Gabaergic neural progenitor cell transplants in reducing neuropathic pain and spinal pathology in rats with spinal cord injury. Exp. Neurol. 327:113208. doi: 10.1016/j.expneurol.2020.113208
El Andaloussi, A., Mäger, I., Breakefield, X. O., and Wood, M. J. (2013). Extracellular vesicles: biology and emerging therapeutic opportunities. Nat. Rev. Drug Discov. 12, 347–357. doi: 10.1038/nrd3978
Finnerup, N. B., Kuner, R., and Jensen, T. S. (2021). Neuropathic pain: from mechanisms to treatment. Physiol. Rev. 101, 259–301. doi: 10.1152/physrev.00045.2019
Gangadharan, V., Zheng, H., Taberner, F. J., Landry, J., Nees, T. A., Pistolic, J., et al. (2022). Neuropathic pain caused by miswiring and abnormal end organ targeting. Nature 606, 137–145. doi: 10.1038/s41586-022-04777-z
Guo, S., Perets, N., Betzer, O., Ben-Shaul, S., Sheinin, A., Michaelevski, I., et al. (2019). Intranasal delivery of Mesenchymal stem cell derived Exosomes loaded with phosphatase and Tensin homolog Sirna repairs complete spinal cord injury. ACS Nano 13, 10015–10028. doi: 10.1021/acsnano.9b01892
Gurung, S., Perocheau, D., Touramanidou, L., and Baruteau, J. (2021). The exosome journey: from biogenesis to uptake and intracellular Signalling. Cell Commun. Signal 19:47. doi: 10.1186/s12964-021-00730-1
Ha, D., Yang, N., and Nadithe, V. (2016). Exosomes as therapeutic drug carriers and delivery vehicles across biological membranes: current perspectives and future challenges. Acta Pharm. Sin. B 6, 287–296. doi: 10.1016/j.apsb.2016.02.001
Harrell, C. R., Jovicic, N., Djonov, V., Arsenijevic, N., and Volarevic, V. (2019). Mesenchymal stem cell-derived Exosomes and other extracellular vesicles as new remedies in the therapy of inflammatory diseases. Cells 8:8. doi: 10.3390/cells8121605
Hessvik, N. P., and Llorente, A. (2018). Current knowledge on exosome biogenesis and release. Cell. Mol. Life Sci. 75, 193–208. doi: 10.1007/s00018-017-2595-9
Hirbec, H., Déglon, N., Foo, L. C., Goshen, I., Grutzendler, J., Hangen, E., et al. (2020). Emerging technologies to study glial cells. Glia 68, 1692–1728. doi: 10.1002/glia.23780
Hori, N., Narita, M., Yamashita, A., Horiuchi, H., Hamada, Y., Kondo, T., et al. (2016). Changes in the expression of Il-6-mediated Micrornas in the dorsal root ganglion under neuropathic pain in mice. Synapse 70, 317–324. doi: 10.1002/syn.21902
Hu, Q., Su, H., Li, J., Lyon, C., Tang, W., Wan, M., et al. (2020). Clinical applications of exosome membrane proteins. Precis. Clin. Med. 3, 54–66. doi: 10.1093/pcmedi/pbaa007
Huang, J. H., Fu, C. H., Xu, Y., Yin, X. M., Cao, Y., and Lin, F. Y. (2020). Extracellular vesicles derived from epidural fat-mesenchymal stem cells attenuate Nlrp3 inflammasome activation and improve functional recovery after spinal cord injury. Neurochem. Res. 45, 760–771. doi: 10.1007/s11064-019-02950-x
Inoue, K., and Tsuda, M. (2018). Microglia in neuropathic pain: cellular and molecular mechanisms and therapeutic potential. Nat. Rev. Neurosci. 19, 138–152. doi: 10.1038/nrn.2018.2
Jean-Toussaint, R., Tian, Y., Chaudhuri, A. D., Haughey, N. J., Sacan, A., and Ajit, S. K. (2020). Proteome characterization of small extracellular vesicles from spared nerve injury model of neuropathic pain. J. Proteome 211:103540. doi: 10.1016/j.jprot.2019.103540
Jeong, J. O., Han, J. W., Kim, J. M., Cho, H. J., Park, C., Lee, N., et al. (2011). Malignant tumor formation after transplantation of short-term cultured bone marrow Mesenchymal stem cells in experimental myocardial infarction and diabetic neuropathy. Circ. Res. 108, 1340–1347. doi: 10.1161/CIRCRESAHA.110.239848
Ji, R. R., Berta, T., and Nedergaard, M. (2013). Glia and pain: is chronic pain a Gliopathy? Pain 154, S10–S28. doi: 10.1016/j.pain.2013.06.022
Ji, R. R., Chamessian, A., and Zhang, Y. Q. (2016). Pain regulation by non-neuronal cells and inflammation. Science 354, 572–577. doi: 10.1126/science.aaf8924
Ji, R. R., Xu, Z. Z., and Gao, Y. J. (2014). Emerging targets in Neuroinflammation-driven chronic pain. Nat. Rev. Drug Discov. 13, 533–548. doi: 10.1038/nrd4334
Jiang, B. C., Liu, T., and Gao, Y. J. (2020). Chemokines in chronic pain: cellular and molecular mechanisms and therapeutic potential. Pharmacol. Ther. 212:107581. doi: 10.1016/j.pharmthera.2020.107581
Kalluri, R., and LeBleu, V. S. (2020). The biology, function, and biomedical applications of exosomes. Science 367:eaau6977. doi: 10.1126/science.aau6977
Kang, J., and Guo, Y. (2022). Human umbilical cord Mesenchymal stem cells derived Exosomes promote neurological function recovery in a rat spinal cord injury model. Neurochem. Res. 47, 1532–1540. doi: 10.1007/s11064-022-03545-9
Karri, J., Doan, J., Vangeison, C., Catalanotto, M., Nagpal, A. S., and Li, S. (2022). Emerging evidence for Intrathecal management of neuropathic pain following spinal cord injury. Front. Pain Res. 3:933422. doi: 10.3389/fpain.2022.933422
Koga, Y., Yasunaga, M., Moriya, Y., Akasu, T., Fujita, S., Yamamoto, S., et al. (2011). Exosome can prevent Rnase from degrading Microrna in feces. J. Gastrointest. Oncol. 2, 215–222. doi: 10.3978/j.issn.2078-6891.2011.015
Kohno, K., and Tsuda, M. (2021). Role of microglia and P2x4 receptors in chronic pain. Pain Rep. 6:E864. doi: 10.1097/PR9.0000000000000864
Kooijmans, S. A. A., Schiffelers, R. M., Zarovni, N., and Vago, R. (2016). Modulation of tissue tropism and biological activity of exosomes and other extracellular vesicles: new Nanotools for cancer treatment. Pharmacol. Res. 111, 487–500. doi: 10.1016/j.phrs.2016.07.006
Kuner, R., and Flor, H. (2016). Structural plasticity and reorganisation in chronic pain. Nat. Rev. Neurosci. 18, 20–30. doi: 10.1038/nrn.2016.162
Lee, B. C., Kang, I., and Yu, K. R. (2021). Therapeutic features and updated clinical trials of mesenchymal stem cell (Msc)-derived Exosomes. J. Clin. Med. 10:711. doi: 10.3390/jcm10040711
Leung, A. (2020). Addressing chronic persistent headaches after Mtbi as a neuropathic pain state. J. Headache Pain 21:77. doi: 10.1186/s10194-020-01133-2
Li, T., Chen, X., Zhang, C., Zhang, Y., and Yao, W. (2019). An update on reactive astrocytes in chronic pain. J. Neuroinflammation 16:140. doi: 10.1186/s12974-019-1524-2
Li, L., Shao, J., Wang, J., Liu, Y., Zhang, Y., Zhang, M., et al. (2019). Mir-30b-5p attenuates Oxaliplatin-induced peripheral neuropathic pain through the voltage-gated Sodium Channel Na(V)1.6 in rats. Neuropharmacology 153, 111–120. doi: 10.1016/j.neuropharm.2019.04.024
Liu, J., Lin, M., Qiao, F., and Zhang, C. (2022). Exosomes derived from Lncrna Tctn2-modified mesenchymal stem cells improve spinal cord injury by Mir-329-3p/Igf1r Axis. J. Mol. Neurosci. 72, 482–495. doi: 10.1007/s12031-021-01914-7
Liu, W., Rong, Y., Wang, J., Zhou, Z., Ge, X., Ji, C., et al. (2020). Exosome-shuttled Mir-216a-5p from hypoxic preconditioned Mesenchymal stem cells repair traumatic spinal cord injury by shifting microglial M1/M2 polarization. J. Neuroinflammation 17:47. doi: 10.1186/s12974-020-1726-7
Liu, Y., Xu, S. Q., Long, W. J., Zhang, X. Y., and Lu, H. L. (2018). C5ar antagonist inhibits occurrence and progression of complement C5a induced inflammatory response of microglial cells through activating P38mapk and Erk1/2 signaling pathway. Eur. Rev. Med. Pharmacol. Sci. 22, 7994–8003. doi: 10.26355/eurrev_201811_16428
Liu, Y. P., Yang, Y. D., Mou, F. F., Zhu, J., Li, H., Zhao, T. T., et al. (2022). Exosome-mediated Mir-21 was involved in the promotion of structural and functional recovery effect produced by electroacupuncture in sciatic nerve injury. Oxid. Med. Cell. Longev. 2022:7530102. doi: 10.1155/2022/7530102
Lobb, R. J., Becker, M., Wen, S. W., Wong, C. S., Wiegmans, A. P., Leimgruber, A., et al. (2015). Optimized exosome isolation protocol for cell culture supernatant and human plasma. J. Extracell. Vesicles 4:27031. doi: 10.3402/jev.v4.27031
López-Leal, R., Díaz-Viraqué, F., Catalán, R. J., Saquel, C., Enright, A., Iraola, G., et al. (2020). Schwann cell reprogramming into repair cells increases Mirna-21 expression in Exosomes promoting axonal growth. J. Cell Sci. :jcs239004:133. doi: 10.1242/jcs.239004
Lou, G., Chen, Z., Zheng, M., and Liu, Y. (2017). Mesenchymal stem cell-derived Exosomes as a new therapeutic strategy for liver diseases. Exp. Mol. Med. 49:E346. doi: 10.1038/emm.2017.63
Ma, Z., Wang, Y., and Li, H. (2020). Applications of extracellular vesicles in tissue regeneration. Biomicrofluidics 14:011501. doi: 10.1063/1.5127077
Macone, A., and Otis, J. A. D. (2018). Neuropathic pain. Semin. Neurol. 38, 644–653. doi: 10.1055/s-0038-1673679
Manion, J., Khuong, T., Harney, D., Littleboy, J. B., Ruan, T., Loo, L., et al. (2020). Human induced pluripotent stem cell-derived Gabaergic interneuron transplants attenuate neuropathic pain. Pain 161, 379–387. doi: 10.1097/j.pain.0000000000001733
Marangon, D., Castro, E. S. J. H., and Lecca, D. (2023). Neuronal and glial communication via non-coding Rnas: messages in extracellular vesicles. Int. J. Mol. Sci. 24:470. doi: 10.3390/ijms24010470
Mathieu, M., Martin-Jaular, L., Lavieu, G., and Théry, C. (2019). Specificities of secretion and uptake of exosomes and other extracellular vesicles for cell-to-cell communication. Nat. Cell Biol. 21, 9–17. doi: 10.1038/s41556-018-0250-9
Matsuda, M., Huh, Y., and Ji, R. R. (2019). Roles of inflammation, neurogenic inflammation, and neuroinflammation in pain. J. Anesth. 33, 131–139. doi: 10.1007/s00540-018-2579-4
Mcdonald, M. K., Tian, Y., Qureshi, R. A., Gormley, M., Ertel, A., Gao, R., et al. (2014). Functional significance of macrophage-derived exosomes in inflammation and pain. Pain 155, 1527–1539. doi: 10.1016/j.pain.2014.04.029
Meldolesi, J. (2018). Exosomes and ectosomes in intercellular communication. Curr. Biol. 28, R435–R444. doi: 10.1016/j.cub.2018.01.059
Navarro, X., Vivó, M., and Valero-Cabré, A. (2007). Neural plasticity after peripheral nerve injury and regeneration. Prog. Neurobiol. 82, 163–201. doi: 10.1016/j.pneurobio.2007.06.005
O’Brien, K., Breyne, K., Ughetto, S., Laurent, L. C., and Breakefield, X. O. (2020). RNA delivery by extracellular vesicles in mammalian cells and its applications. Nat. Rev. Mol. Cell Biol. 21, 585–606. doi: 10.1038/s41580-020-0251-y
Pegtel, D. M., and Gould, S. J. (2019). Exosomes. Annu. Rev. Biochem. 88, 487–514. doi: 10.1146/annurev-biochem-013118-111902
Qiu, P., Zhou, J., Zhang, J., Dong, Y., and Liu, Y. (2021). Exosome: the regulator of the immune system in sepsis. Front. Pharmacol. 12:671164. doi: 10.3389/fphar.2021.671164
Quadros, A. U., and Cunha, T. M. (2016). C5a and pain development: an old molecule, a new target. Pharmacol. Res. 112, 58–67. doi: 10.1016/j.phrs.2016.02.004
Ramanathan, S., and Ajit, S. K. (2016). Microrna-based biomarkers in pain. Adv. Pharmacol. 75, 35–62. doi: 10.1016/bs.apha.2015.12.001
Reinhold, A. K., Salvador, E., Förster, C. Y., Birklein, F., and Rittner, H. L. (2022). Microvascular barrier protection by Microrna-183 via Foxo1 repression: a pathway disturbed in neuropathy and complex regional pain syndrome. J. Pain 23, 967–980. doi: 10.1016/j.jpain.2021.12.007
Rufino-Ramos, D., Albuquerque, P. R., Carmona, V., Perfeito, R., Nobre, R. J., and Pereira De Almeida, L. (2017). Extracellular vesicles: novel promising delivery systems for therapy of brain diseases. J. Control. Release 262, 247–258. doi: 10.1016/j.jconrel.2017.07.001
Scholz, J., Finnerup, N. B., Attal, N., Aziz, Q., Baron, R., Bennett, M. I., et al. (2019). The Iasp classification of chronic pain for Icd-11: chronic neuropathic pain. Pain 160, 53–59. doi: 10.1097/j.pain.0000000000001365
Shao, M., Jin, M., Xu, S., Zheng, C., Zhu, W., Ma, X., et al. (2020). Exosomes from Long noncoding Rna-Gm37494-Adscs repair spinal cord injury via shifting microglial M1/M2 polarization. Inflammation 43, 1536–1547. doi: 10.1007/s10753-020-01230-z
Shepherd, A. J., Mickle, A. D., Golden, J. P., Mack, M. R., Halabi, C. M., De Kloet, A. D., et al. (2018). Macrophage angiotensin ii type 2 receptor triggers neuropathic pain. Proc. Natl. Acad. Sci. U. S. A. 115, E8057–E8066. doi: 10.1073/pnas.1721815115
Shiue, S. J., Rau, R. H., Shiue, H. S., Hung, Y. W., Li, Z. X., Yang, K. D., et al. (2019). Mesenchymal stem cell Exosomes as a cell-free therapy for nerve injury-induced pain in rats. Pain 160, 210–223. doi: 10.1097/j.pain.0000000000001395
Shojaei, S., Hashemi, S. M., Ghanbarian, H., Salehi, M., and Mohammadi-Yeganeh, S. (2019). Effect of Mesenchymal stem cells-derived Exosomes on tumor microenvironment: tumor progression versus tumor suppression. J. Cell. Physiol. 234, 3394–3409. doi: 10.1002/jcp.27326
Simeoli, R., Montague, K., Jones, H. R., Castaldi, L., Chambers, D., Kelleher, J. H., et al. (2017). Exosomal cargo including microrna regulates sensory neuron to macrophage communication after nerve trauma. Nat. Commun. 8:1778. doi: 10.1038/s41467-017-01841-5
Sosanya, N. M., Kumar, R., Clifford, J. L., Chavez, R., Dimitrov, G., Srinivasan, S., et al. (2020). Identifying plasma derived extracellular vesicle (Ev) contained biomarkers in the development of chronic neuropathic pain. J. Pain 21, 82–96. doi: 10.1016/j.jpain.2019.05.015
Su, W. F., Wu, F., Jin, Z. H., Gu, Y., Chen, Y. T., Fei, Y., et al. (2019). Overexpression of P2x4 receptor in Schwann cells promotes motor and sensory functional recovery and remyelination via Bdnf secretion after nerve injury. Glia 67, 78–90. doi: 10.1002/glia.23527
Sun, G., Li, G., Li, D., Huang, W., Zhang, R., Zhang, H., et al. (2018). Hucmsc derived Exosomes promote functional recovery in spinal cord injury mice via attenuating inflammation. Mater. Sci. Eng. C Mater. Biol. Appl. 89, 194–204. doi: 10.1016/j.msec.2018.04.006
Théry, C., Witwer, K. W., Aikawa, E., Alcaraz, M. J., Anderson, J. D., Andriantsitohaina, R., et al. (2018). Minimal information for studies of extracellular vesicles 2018 (Misev2018): a position statement of the international society for extracellular vesicles and update of the Misev2014 guidelines. J. Extracell. Vesicles 7:1535750. doi: 10.1080/20013078.2018.1535750
Tian, T., Zhang, H. X., He, C. P., Fan, S., Zhu, Y. L., Qi, C., et al. (2018). Surface functionalized exosomes as targeted drug delivery vehicles for cerebral ischemia therapy. Biomaterials 150, 137–149. doi: 10.1016/j.biomaterials.2017.10.012
Toh, W. S., Lai, R. C., Zhang, B., and Lim, S. K. (2018). Msc exosome works through a protein-based mechanism of action. Biochem. Soc. Trans. 46, 843–853. doi: 10.1042/BST20180079
Trajkovic, K., Hsu, C., Chiantia, S., Rajendran, L., Wenzel, D., Wieland, F., et al. (2008). Ceramide triggers budding of exosome vesicles into multivesicular endosomes. Science 319, 1244–1247. doi: 10.1126/science.1153124
Tran, P. H., Xiang, D., Nguyen, T. N., Tran, T. T., Chen, Q., Yin, W., et al. (2020). Aptamer-guided extracellular vesicle theranostics in oncology. Theranostics 10, 3849–3866. doi: 10.7150/thno.39706
Tsuda, M., and Inoue, K. (2016). Neuron-microglia interaction by purinergic signaling in neuropathic pain following neurodegeneration. Neuropharmacology 104, 76–81. doi: 10.1016/j.neuropharm.2015.08.042
Tsuda, M., Koga, K., Chen, T., and Zhuo, M. (2017). Neuronal and microglial mechanisms for neuropathic pain in the spinal dorsal horn and anterior cingulate cortex. J. Neurochem. 141, 486–498. doi: 10.1111/jnc.14001
Valadi, H., Ekström, K., Bossios, A., Sjöstrand, M., Lee, J. J., and Lötvall, J. O. (2007). Exosome-mediated transfer of Mrnas and Micrornas is a novel mechanism of genetic exchange between cells. Nat. Cell Biol. 9, 654–659. doi: 10.1038/ncb1596
Van Niel, G., D'angelo, G., and Raposo, G. (2018). Shedding light on the cell biology of extracellular vesicles. Nat. Rev. Mol. Cell Biol. 19, 213–228. doi: 10.1038/nrm.2017.125
Vicario, N., Turnaturi, R., Spitale, F. M., Torrisi, F., Zappalà, A., Gulino, R., et al. (2020). Intercellular communication and ion channels in neuropathic pain chronicization. Inflamm. Res. 69, 841–850. doi: 10.1007/s00011-020-01363-9
Wang, Y., Lai, X., Wu, D., Liu, B., Wang, N., and Rong, L. (2021). Umbilical Mesenchymal stem cell-derived Exosomes facilitate spinal cord functional recovery through the Mir-199a-3p/145-5p-mediated Ngf/Trka signaling pathway in rats. Stem Cell Res Ther 12:117. doi: 10.1186/s13287-021-02148-5
Wang, Y., Liu, J., Ma, J., Sun, T., Zhou, Q., Wang, W., et al. (2019). Exosomal CircRNAs: biogenesis, effect and application in human diseases. Mol. Cancer 18:116. doi: 10.1186/s12943-019-1041-z
Wei, Z., Fei, Y., Su, W., and Chen, G. (2019). Emerging role of Schwann cells in neuropathic pain: receptors, glial mediators and myelination. Front. Cell. Neurosci. 13:116. doi: 10.3389/fncel.2019.00116
Wu, Y., Shen, Z., Xu, H., Zhang, K., Guo, M., Wang, F., et al. (2021). Bdnf participates in chronic constriction injury-induced neuropathic pain via transcriptionally activating P2x(7) in primary sensory neurons. Mol. Neurobiol. 58, 4226–4236. doi: 10.1007/s12035-021-02410-0
Xie, W., Strong, J. A., and Zhang, J. M. (2017). Active nerve regeneration with failed target Reinnervation drives persistent neuropathic pain. ENeuro 4:8. doi: 10.1523/ENEURO.0008-17.2017
Xu, G., Ao, R., Zhi, Z., Jia, J., and Yu, B. (2019). Mir-21 and Mir-19b delivered by hMSC-derived EVs regulate the apoptosis and differentiation of neurons in patients with spinal cord injury. J. Cell. Physiol. 234, 10205–10217. doi: 10.1002/jcp.27690
Yellon, D. M., and Davidson, S. M. (2014). Exosomes: nanoparticles involved in cardioprotection? Circ. Res. 114, 325–332. doi: 10.1161/CIRCRESAHA.113.300636
Ying, Z., Pan, C., Shao, T., Liu, L., Li, L., Guo, D., et al. (2018). Mixed lineage kinase domain-like protein MLKL breaks down myelin following nerve injury. Mol. Cell 72, 457–468. doi: 10.1016/j.molcel.2018.09.011
Yousefifard, M., Nasirinezhad, F., Shardi Manaheji, H., Janzadeh, A., Hosseini, M., and Keshavarz, M. (2016). Human bone marrow-derived and umbilical cord-derived Mesenchymal stem cells for alleviating neuropathic pain in a spinal cord injury model. Stem Cell Res Ther 7:36. doi: 10.1186/s13287-016-0295-2
Yu, X., Abdul, M., Fan, B. Q., Zhang, L., Lin, X., Wu, Y., et al. (2020a). The release of Exosomes in the medial prefrontal cortex and nucleus accumbens brain regions of chronic constriction injury (cci) model mice could elevate the pain sensation. Neurosci. Lett. 723:134774. doi: 10.1016/j.neulet.2020.134774
Yu, X., Liu, H., Hamel, K. A., Morvan, M. G., Yu, S., Leff, J., et al. (2020b). Dorsal root ganglion macrophages contribute to both the initiation and persistence of neuropathic pain. Nat. Commun. 11:264. doi: 10.1038/s41467-019-13839-2
Yu, T., Xu, Y., Ahmad, M. A., Javed, R., Hagiwara, H., and Tian, X. (2021). Exosomes as a promising therapeutic strategy for peripheral nerve injury. Curr. Neuropharmacol. 19, 2141–2151. doi: 10.2174/1570159X19666210203161559
Yuan, Q., Liu, X., Xian, Y. F., Yao, M., Zhang, X., Huang, P., et al. (2020). Satellite glia activation in dorsal root ganglion contributes to mechanical Allodynia after selective motor fiber injury in adult rats. Biomed. Pharmacother. 127:110187. doi: 10.1016/j.biopha.2020.110187
Zhang, R., Bu, T., Cao, R., Li, Z., Wang, C., Huang, B., et al. (2022). An optimized exosome production strategy for enhanced yield while without sacrificing cargo loading efficiency. J. Nanobiotechnol. 20:463. doi: 10.1186/s12951-022-01668-3
Zhang, B., Lin, F., Dong, J., Liu, J., Ding, Z., and Xu, J. (2021). Peripheral macrophage-derived exosomes promote repair after spinal cord injury by inducing local anti-inflammatory type microglial polarization via increasing autophagy. Int. J. Biol. Sci. 17, 1339–1352. doi: 10.7150/ijbs.54302
Zhang, S. B., Lin, S. Y., Liu, M., Liu, C. C., Ding, H. H., Sun, Y., et al. (2019). Circanks1a in the spinal cord regulates hypersensitivity in a rodent model of neuropathic pain. Nat. Commun. 10:4119. doi: 10.1038/s41467-019-12049-0
Zhang, Y., Liu, Y., Liu, H., and Tang, W. H. (2019). Exosomes: biogenesis, biologic function and clinical potential. Cell Biosci. 9:19. doi: 10.1186/s13578-019-0282-2
Zhang, J., Rong, L., Shao, J., Zhang, Y., Liu, Y., Zhao, S., et al. (2021). Epigenetic restoration of voltage-gated Potassium Channel Kv1.2 alleviates nerve injury-induced neuropathic pain. J. Neurochem. 156, 367–378. doi: 10.1111/jnc.15117
Zhang, Y. U., Ye, G., Zhao, J., Chen, Y., Kong, L., Sheng, C., et al. (2022). Exosomes carried Mir-181c-5p alleviates neuropathic pain in cci rat models. An. Acad. Bras. Cienc. 94:E20210564. doi: 10.1590/0001-3765202220210564
Zhang, Y., Zhang, Y., Chopp, M., Zhang, Z. G., Mahmood, A., and Xiong, Y. (2020). Mesenchymal stem cell-derived Exosomes improve functional recovery in rats after traumatic brain injury: a dose-response and therapeutic window study. Neurorehabil. Neural Repair 34, 616–626. doi: 10.1177/1545968320926164
Zhao, Y., Chen, Y., Wang, Z., Xu, C., Qiao, S., Liu, T., et al. (2022). Bone marrow mesenchymal stem cell exosome attenuates inflammasome-related pyroptosis via delivering Circ_003564 to improve the recovery of spinal cord injury. Mol. Neurobiol. 59, 6771–6789. doi: 10.1007/s12035-022-03006-y
Zhao, C., Deng, Y., He, Y., Huang, X., Wang, C., and Li, W. (2021). Decreased level of exosomal Mir-5121 released from microglia suppresses Neurite outgrowth and synapse recovery of neurons following traumatic brain injury. Neurotherapeutics 18, 1273–1294. doi: 10.1007/s13311-020-00999-z
Keywords: exosome, neuropathic pain, glial cell, neuroinflammation, noncoding RNA, extracellular vesicles
Citation: Zhang K, Li P, Jia Y, Liu M and Jiang J (2023) Concise review: Current understanding of extracellular vesicles to treat neuropathic pain. Front. Aging Neurosci. 15:1131536. doi: 10.3389/fnagi.2023.1131536
Edited by:
Jagdeep K. Sandhu, National Research Council Canada (NRC), CanadaReviewed by:
Arsalan S. Haqqani, National Research Council Canada (NRC), CanadaDylan Burger, University of Ottawa, Canada
Pranav Sharma, Xosomix LLC, United States
Copyright © 2023 Zhang, Li, Jia, Liu and Jiang. This is an open-access article distributed under the terms of the Creative Commons Attribution License (CC BY). The use, distribution or reproduction in other forums is permitted, provided the original author(s) and the copyright owner(s) are credited and that the original publication in this journal is cited, in accordance with accepted academic practice. No use, distribution or reproduction is permitted which does not comply with these terms.
*Correspondence: Jingjing Jiang, ampqX3NqQDE2My5jb20=