- 1Department of Surgery and Medical-Surgical Specialties, University of Catania, Catania, Italy
- 2Clinical Neurophysiology Research Unit, Oasi Research Institute-IRCCS, Troina, Italy
- 3Department of Biomedical and Biotechnological Sciences, University of Catania, Catania, Italy
- 4Department of Neurosciences, Reproductive Sciences and Odontostomatology, University of Naples “Federico II,” Naples, Italy
- 5Unit of Neurology, Department of Neuroscience, Biomedicine and Movement Sciences, University of Verona, Verona, Italy
- 6Laboratory of Clinical Neurosciences, Rambam Medical Center, Haifa, Israel
- 7Neurology Unit, Policlinico University Hospital “G. Rodolico – San Marco,” Catania, Italy
- 8Neurology Unit, Sant’Elia Hospital, ASP Caltanissetta, Caltanissetta, Italy
- 9Department of Medical and Surgical Sciences and Advanced Technologies, University of Catania, Catania, Italy
- 10Unit of Neurology, Neurophysiology and Neurobiology, Department of Medicine and Surgery, Università Campus Bio-Medico di Roma, Rome, Italy
- 11Fondazione Policlinico Universitario Campus Bio-Medico, Rome, Italy
Although primary degenerative diseases are the main cause of dementia, a non-negligible proportion of patients is affected by a secondary and potentially treatable cognitive disorder. Therefore, diagnostic tools able to early identify and monitor them and to predict the response to treatment are needed. Transcranial magnetic stimulation (TMS) is a non-invasive neurophysiological technique capable of evaluating in vivo and in “real time” the motor areas, the cortico-spinal tract, and the neurotransmission pathways in several neurological and neuropsychiatric disorders, including cognitive impairment and dementia. While consistent evidence has been accumulated for Alzheimer’s disease, other degenerative cognitive disorders, and vascular dementia, to date a comprehensive review of TMS studies available in other secondary dementias is lacking. These conditions include, among others, normal-pressure hydrocephalus, multiple sclerosis, celiac disease and other immunologically mediated diseases, as well as a number of inflammatory, infective, metabolic, toxic, nutritional, endocrine, sleep-related, and rare genetic disorders. Overall, we observed that, while in degenerative dementia neurophysiological alterations might mirror specific, and possibly primary, neuropathological changes (and hence be used as early biomarkers), this pathogenic link appears to be weaker for most secondary forms of dementia, in which neurotransmitter dysfunction is more likely related to a systemic or diffuse neural damage. In these cases, therefore, an effort toward the understanding of pathological mechanisms of cognitive impairment should be made, also by investigating the relationship between functional alterations of brain circuits and the specific mechanisms of neuronal damage triggered by the causative disease. Neurophysiologically, although no distinctive TMS pattern can be identified that might be used to predict the occurrence or progression of cognitive decline in a specific condition, some TMS-associated measures of cortical function and plasticity (such as the short-latency afferent inhibition, the short-interval intracortical inhibition, and the cortical silent period) might add useful information in most of secondary dementia, especially in combination with suggestive clinical features and other diagnostic tests. The possibility to detect dysfunctional cortical circuits, to monitor the disease course, to probe the response to treatment, and to design novel neuromodulatory interventions in secondary dementia still represents a gap in the literature that needs to be explored.
Introduction
Background
Primary dementia typically stems from a progressive and degenerative/irreversible neuronal loss, whereas secondary forms are characterized by a progressive, but potentially reversible, cause of cognitive decline. Common examples of primary dementias are Alzheimer’s disease (AD), dementia with Lewy bodies (DLB), fronto-temporal lobar degeneration (FTD), Huntington’s disease (HD), and Creutzfeldt-Jakob’s disease. Among conditions that may cause or mimic dementia, which usually affects younger subjects than primary dementia (Sobów et al., 2007), there are normal-pressure hydrocephalus (NPH), multiples sclerosis (MS), celiac disease (CD) and other immunologically mediated diseases, as well several inflammatory, infective, metabolic, toxic, nutritional, endocrine, hepatic, renal, sleep-related, and rare genetic disorders (Yousuf et al., 2010).
The most relevant aspect of all these conditions is that, if cognitive impairment is early detected and promptly treated, it can be reversed or its course significantly improved, even toward a complete recovery (Lowenthal et al., 2007; Pennisi et al., 2020; Caruso et al., 2022). In this context, a systematic neurophysiological assessment of secondary dementia can aid the diagnosis, follow the course, and predict the response to treatment. Among electrophysiological techniques, transcranial magnetic stimulation (TMS) has emerged as a valuable non-invasive method for the functional evaluation in vivo and in “real time” of the cortico-spinal tract and cortico-cortical connections, capable of revealing neurotransmission abnormalities in several disorders causing cognitive impairment and dementia (Rossini et al., 2007; Cantone et al., 2014; Di Lazzaro et al., 2021).
Transcranial magnetic stimulation
From pioneering applications of TMS to assess the primary motor cortex (M1) functioning and the cortical-spinal tract (Barker et al., 1985; Cantone et al., 2019b; Lanza et al., 2019a, 2020b), researchers have boosted the potentialities of this technique, which is today employed to study cortical excitability, to map connectivity (Dubbioso et al., 2021), and to probe distinctive plastic phenomena (Rothwell, 2018). Overall, this has provided insights into the pathophysiology, treatment, and monitoring of different neurological and neuropsychiatric disorders (Di Pino et al., 2014a; Gomes-Osman et al., 2018; Cantone et al., 2019a).
A functional evaluation of the cortical excitability and cortico-spinal conductivity is performed by applying single-pulse TMS at proper stimulation intensity on the M1, thus eliciting motor evoked potentials (MEPs) that can be recorded from selected muscles of the contralateral side (Di Lazzaro et al., 2001; Hoogendam et al., 2010). The amplitude of MEP expresses a compound index of the output cells excitability within the M1, motor axons, nerve roots, peripheral motor nerves, and muscles (Rossini et al., 2015). The resting motor threshold (rMT), i.e., the minimal stimulation intensity required to elicit MEPs, is considered as a global measure of cortical excitability, since it is an aggregate index of the excitability of motoneuron membranes, neuronal inputs in cortical pyramidal neurons, spinal motoneurons, neuromuscular junctions, and muscle cells (Rossini and Rossi, 2007). Central motor conduction time (CMCT), estimated by subtracting the conduction time along the peripheral nerve (after stimulation of the motor roots) from the MEP cortical-muscular latency, measures the conduction along the cortico-spinal tract (Rossini et al., 2015).
The cortical silent period (cSP), which refers to the electromyographic inhibition of the motor responses occurring after a suprathreshold stimulation of the M1 during a tonic voluntary contraction of contralateral muscles, is an index of the inhibitory intracortical circuit (Chen et al., 1999). This parameter, which normally lasts a few hundred ms, is considered as a dynamic metric of a specific inhibitory intracortical circuitry (Cantello et al., 1992; Werhahn et al., 1999), largely mediated by the gamma-aminobutyric acid (GABA)-B activity (Siebner et al., 1998). The muscle activation and hemisphere stimulation of the same side evokes the ipsilateral SP (iSP), which it is thought to be generated by transcallosal fibers projecting into contralateral GABAergic interneurons (Kobayashi and Pascual-Leone, 2003; Chen et al., 2008; Lanza et al., 2013).
Paired-pulse TMS paradigms allow the assessment of the short-interval intracortical inhibition (SICI) and the intracortical facilitation (ICF) phenomena (Kujirai et al., 1993; Ziemann et al., 1996; Faro et al., 2010). The activity of GABA-A interneurons is the most likely mediator of SICI (Di Lazzaro et al., 2006a; Ferreri et al., 2011), whereas the neurophysiology of ICF is more complex. It probably relates to the activation of a cortical circuit projecting upon cortico-spinal cells different from that preferentially activated by single-pulse TMS. ICF seems to be greatly mediated by excitatory glutamatergic interneurons (Ziemann, 2004; Di Lazzaro et al., 2006c).
Additionally, the short-latency afferent inhibition (SAI) is known to largely reflect central cholinergic activity (Tokimura et al., 2000). Accordingly, in normal conditions, scopolamine (a muscarinic antagonistic) abolishes or reduces SAI (Di Lazzaro et al., 2000), whereas acetylcholine positively stimulates it (Di Lazzaro et al., 2005). Previous evidence suggests that SAI might reflect the integrity of intracortical circuitries underlying sensory-motor integration (Sailer et al., 2003; Martorana et al., 2009; Dubbioso et al., 2017b). SAI provides useful insights in several disorders affecting cognition and movement (Di Lazzaro et al., 2006b,2007; Dubbioso et al., 2019). TMS can also assess the mechanisms underlying synaptic plasticity by means of the paired-associative stimulation (PAS), through the application of magnetic stimuli after a short time interval of exercise or by means of low-frequency repetitive electric stimulation of median nerve coupled with TMS of the contralateral M1 (Carson and Kennedy, 2013). PAS leads to long-term potentiation (LTP)- and long-term depression (LTD)-like phenomena.
Finally, repetitive TMS (rTMS) applied on the same area of the cortex is able to induce temporary changes of cortical excitability, that is suppressed by low frequency stimulations (≤1 Hz) and enhanced by high frequencies (>5 Hz) of stimulation (Chen, 2020). Neurobiological phenomena underlying rTMS share features with the induction of LTP and LTD effects after tetanic stimulation of cortical slices (Wilson et al., 2018), including the correlation with the activity of the N-methyl-D-aspartate (NMDA) receptor (Cheeran et al., 2010), the sensitivity to earlier synaptic activation (Jung and Ziemann, 2009), and the strict link with stimulation frequency (Di Lazzaro et al., 2010). The short-term change in synaptic efficacy and the rapid down-regulation of GABA-related inhibitory circuits are key processes of the calcium- and sodium channel-dependent LTP plasticity (Ziemann et al., 1998a,b). Conversely, by inducing LTD-like changes, rTMS decreases synaptic efficacy (Gladding et al., 2009; Cirillo et al., 2017), both in normal and in pathological conditions (Fisicaro et al., 2020a). The effects of repeated sessions of rTMS persist in time and act by enhancing plasticity or by down-regulating it when it appears inappropriate or even maladaptive (Lefaucheur, 2019). For this reason, translational therapeutic and rehabilitative applications of rTMS can encompass a considerable number of neurological and neuropsychiatric diseases (Lefaucheur et al., 2020; Concerto et al., 2022). Additionally, distinctive rTMS protocols, such as the theta burst stimulation (Li et al., 2019) and quadripulse stimulation (Matsumoto and Ugawa, 2020), can aid toward a further understanding of the complex phenomena of neural plasticity, including the so-called metaplasticity (the “plasticity of synaptic plasticity”) (Di Pino et al., 2014b; Yger and Gilson, 2015; He et al., 2020; Cantone et al., 2021).
Transcranial magnetic stimulation and rTMS protocols are well tolerated and safe. Relatively frequent side effects that have been reported during or after rTMS are transient headache and a discomfort secondary to facial or scalp muscular twitching (Burt et al., 2002), whereas seizure induction represents a very rare adverse event (Anderson et al., 2006). As such, patients with epilepsy or those at risk for epilepsy need to be cautiously managed.
Rationale and aim
Even if not always clinically evident, the involvement of motor areas in dementia has been demonstrated by neuropathological, and neuroimaging studies. The changes in motor areas might be caused by the disease process itself or, more often, might be caused indirectly as a consequence of remodeling mechanisms (Cantone et al., 2014). Indeed, increasing evidence supports the involvement of brain plasticity phenomena in different types of dementia, related to functional and structural changes within a very complex framework (Ferreri and Rossini, 2013).
To date, the implications of the cortical excitability changes observed in dementia are not completely understood. Moreover, while an increasing number of evidences is accumulating over time in AD, in other degenerative dementias (Cantone et al., 2014), and in vascular dementia (VaD) (Cantone et al., 2020), to date a comprehensive summary of the TMS findings in all other dementing processes is lacking. Here, we provide an up-to-date review of available studies, including data about pathophysiology, diagnosis, prognosis, and neuromodulatory interventions.
Methods
A literature search was carried out to find all the relevant studies on TMS and rTMS in secondary dementia or cognitive impairment. A PubMed-based literature review was performed, from database inception to July 14, 2022, by using the search terms “secondary dementia” OR “secondary cognitive impairment” OR “reversible dementia” OR “reversible cognitive impairment” AND “normal pressure hydrocephalus” OR “multiple sclerosis” OR “celiac disease” OR “immune-mediated” OR “endocrine” OR “hepatic” OR “renal” OR “metabolic” OR “nutritional” OR “alcohol” OR “infectious” OR “inflammatory” OR “sleep” OR “genetic” AND “transcranial magnetic stimulation” OR “motor evoked potential” OR “motor cortical excitability.”
Two authors (F.F. and M.C.) independently screened titles and abstracts of the retrieved publications, and disagreements were solved by a third author (M.P.). Articles listed in the references were also reviewed in search of more data. Duplicated entries, retracted publications, studies on other diseases, works on animals or in vitro, non-English written papers, publications that are not research studies (e.g., commentaries, letters, editorials, reviews, etc.), and any other article that did not fit with the primary scope of this review (e.g., intellectual disability and other childhood-onset developmental-related cognitive deficits) were excluded. In particular, patients with vascular cognitive impairment (from mild form to overt VaD) were not included since, although some of them may present a more favorable course and even be partially reversible, to date there is no definitive consensus in the literature concerning the reversibility of this etiology. Moreover, both TMS and rTMS studies in vascular cognitive impairment and its subtypes (including VaD) have been recently reviewed (Cantone et al., 2020; Di Lazzaro et al., 2021). The same holds true for cognitive disorders due to psychiatric diseases (particularly depression) and neurosurgical conditions (e.g., traumatic brain injury, subdural hematoma, brain tumors, etc.).
Results
A total of 475 items were originally retrieved. Of these, 79 peer-reviewed publications were selected according to the inclusion and exclusion criteria and the main findings analyzed clustering within two groups: one on TMS (Table 1) and the other on rTMS studies (Table 2).
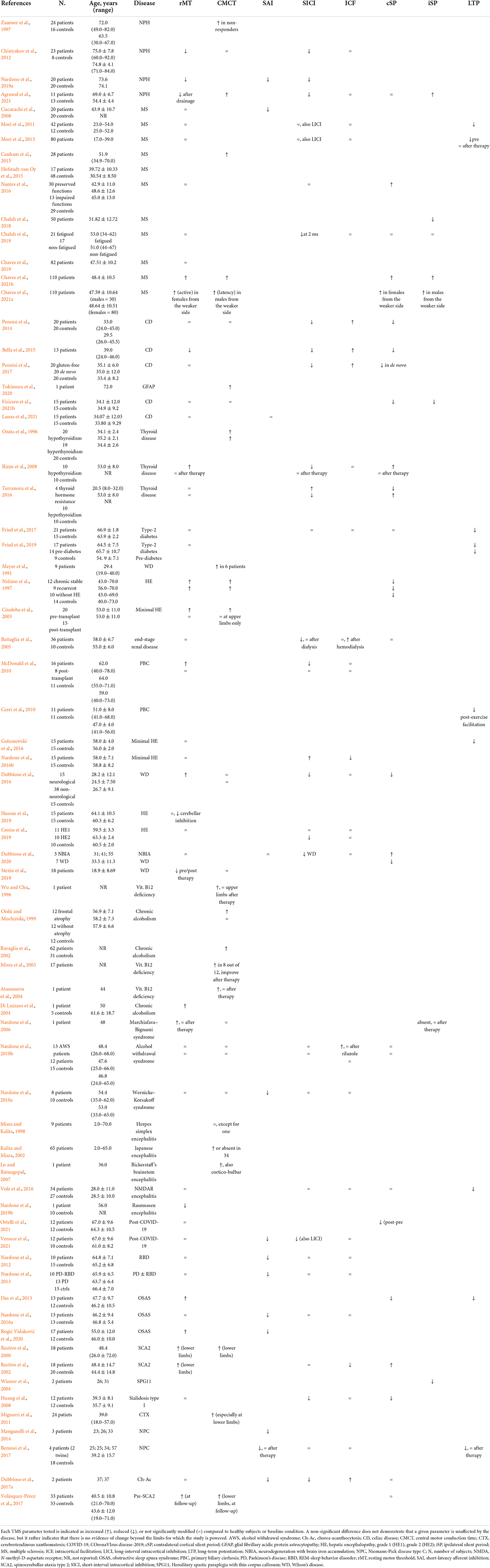
Table 1. Relevant transcranial magnetic stimulation (TMS) findings reported in most common secondary cognitive disorders.
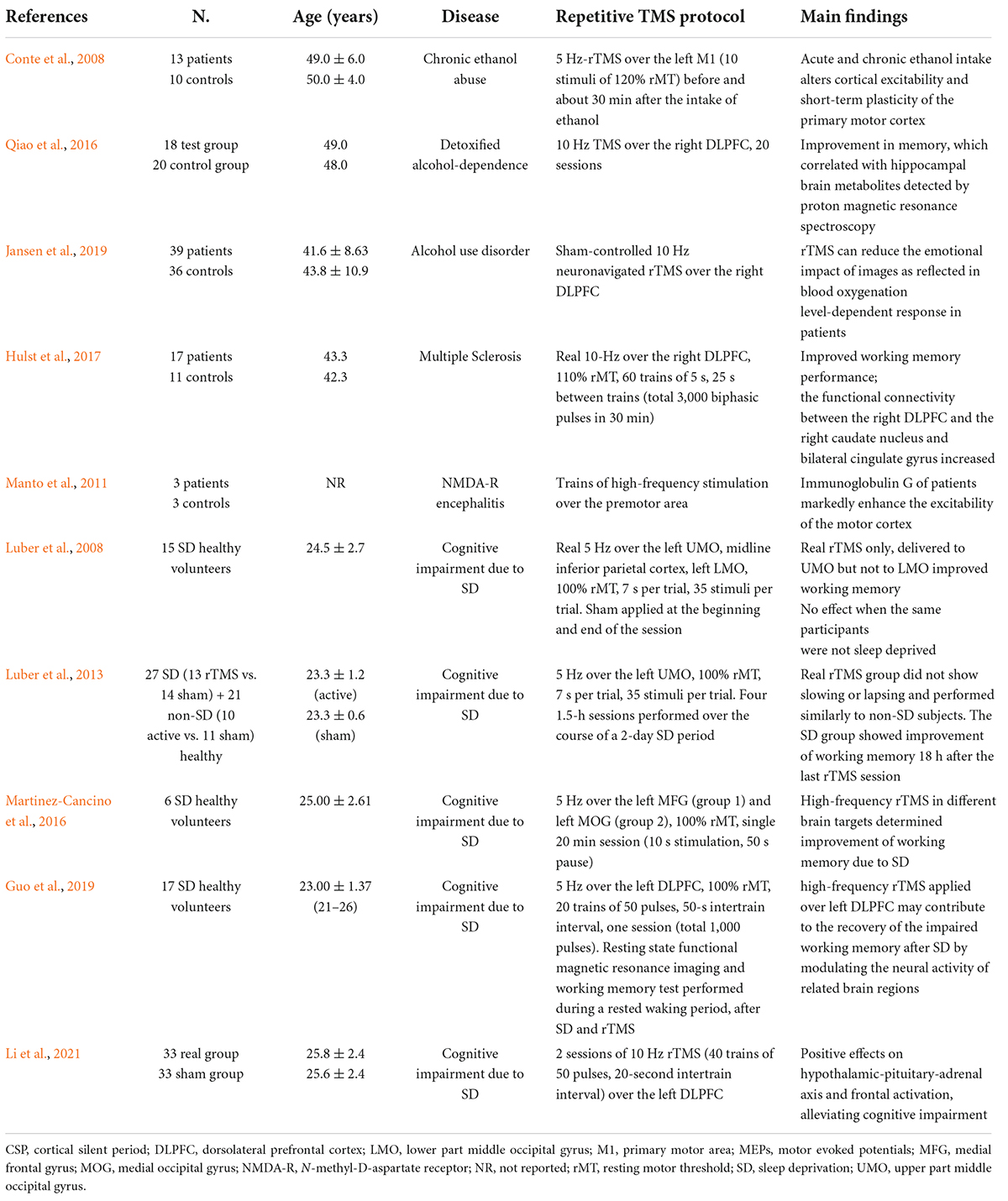
Table 2. Relevant repetitive transcranial magnetic stimulation (rTMS) studies in non-vascular secondary dementia or cognitive disorders.
The TMS group included 4 studies on NPH (Zaaroor et al., 1997; Chistyakov et al., 2012; Nardone et al., 2019a; Agrawal et al., 2021), 11 on MS (Cucurachi et al., 2008; Mori et al., 2011, 2013; Canham et al., 2015; Hofstadt-van Oy et al., 2015; Nantes et al., 2016; Chalah et al., 2018, 2019; Chaves et al., 2019, 2021a,b), 6 on CD and other immune-mediated disorders (Pennisi et al., 2014; Bella et al., 2015; Pennisi et al., 2017; Tokimura et al., 2020; Fisicaro et al., 2021b; Lanza et al., 2021), 5 on endocrine disorders (Ozata et al., 1996; Rizzo et al., 2008; Terranova et al., 2016; Fried et al., 2017, 2019), 13 on liver and renal diseases (Meyer et al., 1991; Nolano et al., 1997; Córdoba et al., 2003; Battaglia et al., 2005; Cerri et al., 2010; McDonald et al., 2010; Dubbioso et al., 2016; Golaszewski et al., 2016; Nardone et al., 2016b; Groiss et al., 2019; Hassan et al., 2019; Stezin et al., 2019; Dubbioso et al., 2020), 9 on nutritional and alcohol-related disorders (Wu and Chu, 1996; Oishi and Mochizuki, 1999; Ravaglia et al., 2002; Misra et al., 2003; Atanassova et al., 2004; Di Lazzaro et al., 2004; Nardone et al., 2006, 2010b, 2016a), 7 on infectious or inflammatory diseases (Misra and Kalita, 1998; Kalita and Misra, 2002; Lo and Ratnagopal, 2007; Volz et al., 2016; Nardone et al., 2019b; Ortelli et al., 2021; Versace et al., 2021), 5 on sleep disorders (Nardone et al., 2012, 2013, 2016a; Das et al., 2013; Rogić Vidaković et al., 2020), and 9 on rare genetic diseases (Restivo et al., 2000, 2002; Winner et al., 2004; Huang et al., 2008; Mignarri et al., 2011; Manganelli et al., 2014; Benussi et al., 2017; Dubbioso et al., 2017a; Velázquez-Pérez et al., 2018). The other group consisted of 10 articles applying rTMS in different clinical contexts (Conte et al., 2008; Luber et al., 2008, 2013; Manto et al., 2011; Martinez-Cancino et al., 2016; Qiao et al., 2016; Hulst et al., 2017; Guo et al., 2019; Jansen et al., 2019; Li et al., 2021).
Figure 1 contains the flow diagram showing the search strategy, the number of records identified, the numbers of excluded studies, and the number of those eventually included (Moher et al., 2009).
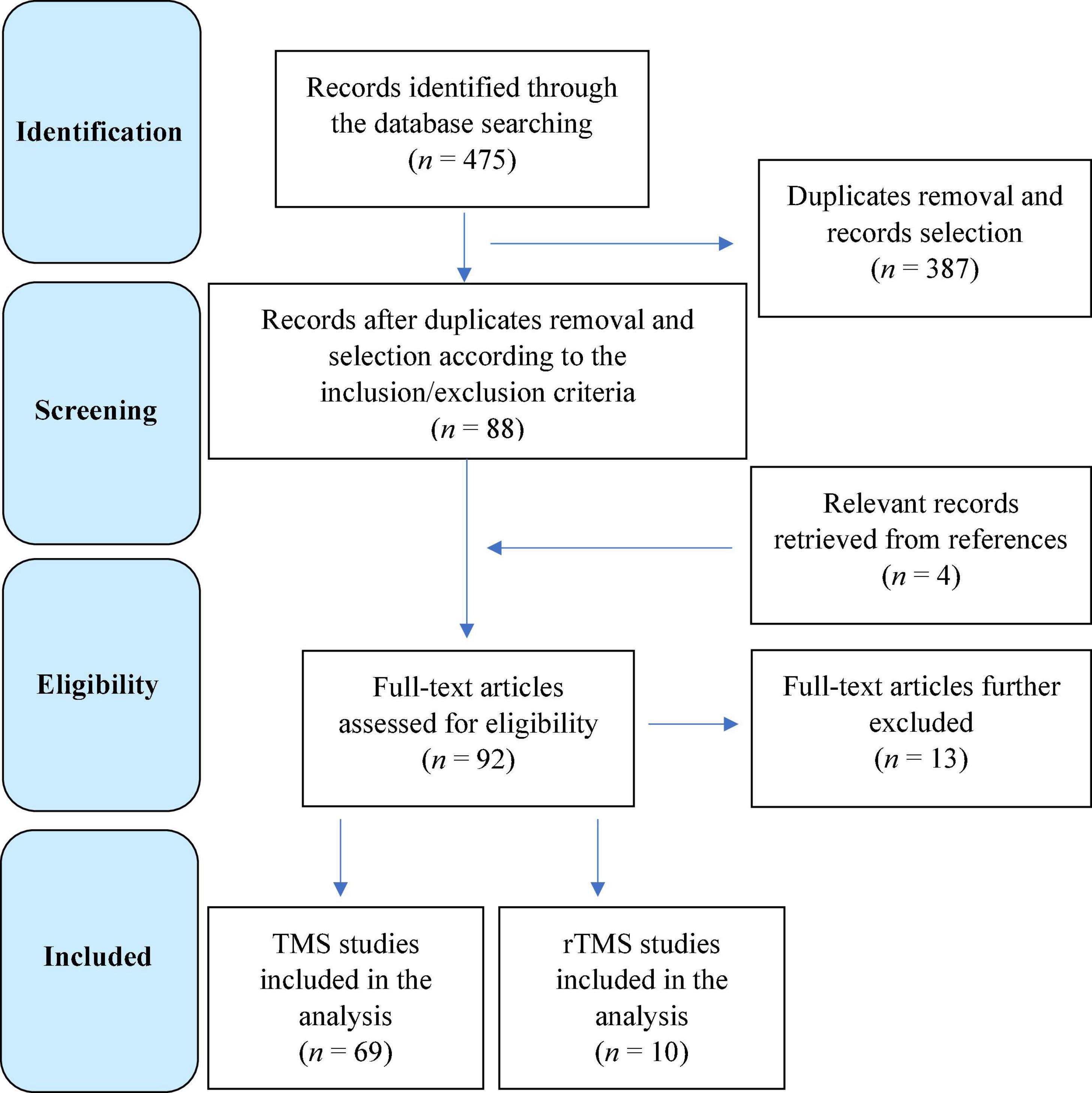
Figure 1. Flow diagram showing the search strategy, the number of records identified, and the number of included/excluded transcranial magnetic stimulation (TMS) and repetitive TMS studies (Moher et al., 2009).
Normal-pressure hydrocephalus
Idiopathic NPH, one of the main causes of secondary dementia, is characterized by the clinical triad of gait disturbance, cognitive dysfunction, and urinary incontinence. When NPH is promptly diagnosed, surgical ventricular shunt allows a rapid and substantial improvement of walking and cognitive status, till a complete resolution in some cases (Wang et al., 2020). For this reason, methods providing objective evidence of developing NPH are essential for differential diagnoses, selection criteria, and surgical decisions.
The first TMS study in patients with NPH (Zaaroor et al., 1997) demonstrated that the gait disturbance is not related to an impairment of conduction along the cortico-spinal tract, whereas another study (Chistyakov et al., 2012) showed a M1 disinhibition in terms of a significant decrease of rMT and SICI. The very low rMT from the leg motor area was the first and most prominent finding in the early stage of disease, whereas the decrease of rMT and SICI was not significant from the hand muscles (Nardone et al., 2019a). In the latter study, the significant reduction of SAI may indicate an impairment of cholinergic circuits and suggest that some patients with apparent idiopathic NPH may develop AD during the disease course. Overall, these data, although limited, indicate that NPH can determine a hyperexcitability of the motor cortex, mostly affecting the leg cortical representation in the early phases and a more widespread cortical pathology in the later stage. Such a pattern of electrocortical changes might be considered as a neurophysiological marker of NPH and a useful hint in differential diagnosis. Finally, a very recent study has evaluated the effect of lumbar drainage on cortical excitability in 11 NPH patients compared to 13 healthy controls (Agrawal et al., 2021). The results demonstrated a significant reduction in the lower limb rMT after lumbar drainage, along with a trend toward improvement in SICI and a shortening of the CMCT at lower limb. As a whole, these results seem to suggest a temporary increase in cortical excitability after lumbar drainage for NPH (Agrawal et al., 2021).
Taken together, TMS can guide the selection of those patients who will benefit from shunting, thus providing prognostic information. Moreover, from a pathophysiological perspective, these results support the view that an altered control of motor output, rather than the pure impairment of central motor conduction, is responsible for gait disturbances in NPH and that recovery of cortico-spinal excitability following ventricular shunt placement can correlate with clinical improvement.
Interestingly, the impairment of cholinergic neurons that can contribute to cognitive decline and gait impairment may also indicate the occurrence of AD pathology in some patients with NPH. Based on the observation that some cases of NPH developed AD during their course (Espay et al., 2017) and the poorer outcome than would be expected in patients with NPH alone (Pomeraniec et al., 2016), recent studies suggest the overlapping AD pathology in about 30% of NPH cases. Indeed, both NPH and AD build-up of brain metabolic wastes and amyloid-β plaques, perivascular reactive astrogliosis, and mislocalization of astrocyte aquaporin-4, thus suggesting that amyloid deposition might cause cholinergic dysfunction also in NPH (Graff-Radford and Jones, 2019). Moreover, in both AD and NPH, human studies have recently implicated an impaired glia-lymphatic (glymphatic) system, whose function facilitates brain fluid clearance and waste removal via the glia-supported perivascular channels (Reeves et al., 2020).
Multiple sclerosis
Given that neurophysiological changes occur early in MS, TMS parameters may represent biomarkers of disease progression and response to drugs or rehabilitative interventions. To date, however, these changes have been only weakly associated with cognitive impairment (Simpson and Macdonell, 2015). A previous study (Cucurachi et al., 2008) found that a reduction in SAI was significantly related to cognitive dysfunction in MS. The study also showed that SAI improved after rivastigmine, as observed in AD. A recent review suggested a link between reduced acetylcholine expression, neuroinflammation, and cognitive deficits in MS (Polachini et al., 2018), although more evidence is required. Another study on 28 primary progressive MS patients found that evoked potentials, including MEPs, generally show a moderate correlation with clinical and functional status, but there was no association with any index of cognitive impairment (Canham et al., 2015).
A considerable amount of TMS investigations in MS showed significant abnormalities, especially regarding SICI and ICF, however, it is still unclear whether they could represent biomarker of disease progression, compensatory mechanisms, or treatment-related changes (Ayache et al., 2014, 2016; Ayache and Chalah, 2017). Moreover, longer cSP duration (Nantes et al., 2016) or triple stimulation technique (Hofstadt-van Oy et al., 2015) did not correlate with cognitive performance in relapsing-remitting MS. However, central mechanisms of fatigue, that can, at least in part, play a role in cognitive performances, appeared related to changes in cortical excitability, especially increased interhemispheric inhibition (Chalah et al., 2018) or SICI (Chalah et al., 2019), as well as attenuation of premotor-motor functional connectivity (Ruiu et al., 2020).
A recent TMS investigation aimed at identifying novel markers predictive of objective and subjective clinical features in 82 subjects, mostly affected by relapsing-remitting MS (Chaves et al., 2019). By means of a ratio considering the stronger and weaker side, a correlation between symptom severity and disability of MS and asymmetric brain excitability was found. Namely, subjects were less cognitively and physically impaired whether showing a cortical-spinal excitability asymmetry, being the weaker side more excitable than the stronger. The authors argued that the asymmetry might be a consequence of the hyperexcitability state secondary to a more prominent neuroinflammation in early MS impairing the weaker side (Chaves et al., 2019). Conversely, less inflammatory and more degenerative component might justify the shift of this excitability asymmetry in the later stages of the disease (Dutta and Trapp, 2014).
Very recently, a detailed single-pulse TMS protocol has been applied in a large cohort of MS patients with the aim to establish and validate a core-set of TMS variables that could predict typical clinical outcomes, including cognitive processing speed (Chaves et al., 2021b). Delayed and longer iSP, longer cSP, and higher rMT (all indicating a lower intercortical, intracortical, and cortico-spinal excitability) were found, especially in the hemisphere corresponding to the weaker hand. Moreover, greater interhemispheric asymmetry correlated with poorer performance in most of clinical outcomes (assessed by the Walking Speed, the nine-hole peg test from both the stronger hand and the weaker hand, fatigue, and symbol digit modality test), although TMS metrics were more related to motor than non-motor outcome measures (Chaves et al., 2021b).
Finally, two studies have assessed brain plasticity in MS with cognitive impairment using the intermittent theta-burst stimulation (iTBS) protocol. In the first study (Mori et al., 2011), altered cortical plasticity was observed in cognitively impaired patients, along with a direct correlation between liquoral content of amyloid-ß1–42 and the amount of LTP-like effect produced by iTBS. This indicates that neuroinflammation in MS might alter the metabolism of amyloid-ß with a reduction of its liquoral amount, thus producing defective cognitive functioning and maladaptive plasticity (Mori et al., 2011). In the second study, the authors probed if early started interferon (IFN) ß-1a (which is able to decrease the inflammatory component) at high dosage improved cognition and cortical functioning in 80 drug-free relapsing-remitting MS patients (Mori et al., 2013). Before treatment, those with gadolinium-enhancing (Gd +) lesions on brain magnetic resonance imaging (MRI) scored worse on cognitive tests and exhibited less pronounced plasticity after iTBS when compared with Gd-negative (Gd-) lesion individuals. After 6 months of treatment, both iTBS-related plasticity and cognition ameliorated in Gd + patients and were stable in those Gd-, thus suggesting that maladaptive plasticity and cognitive deficits might recover after IFN ß-1a at high dosage in de novo relapsing-remitting MS patients with Gd + lesions (Mori et al., 2013).
Based on the above-mentioned findings, although MEPs have demonstrated a good detection of disease progression and a reliable correlation with clinical disability, they have not been used as a measure of efficacy in clinical trials testing neuroprotective agents (Fernández, 2021). As such, TMS procedures need to be standardized and data interpretation made comparable between centers. For instance, introducing an automated and objective definition of MEP morphology could help in overcoming some of the barriers that currently limit a broader adoption of TMS (Yperman et al., 2020a). Another example could be the use of machine learning methods for MEP analysis, this approach showed predictive performance, although larger multi-center datasets are required in decision-making process and in the design of new treatments (Yperman et al., 2020b).
Very recently, however, it has been shown that a sex-specific disruption in cortico-spinal excitability may exist in MS (Chaves et al., 2021a): namely, males were more likely to have cognitive impairment as measured by the Montreal Cognitive Assessment (MoCA), whereas greater TMS asymmetry was noted in females, who demonstrated higher active MS and longer cSP in the hemisphere corresponding to the weaker hand. Males, but not females, exhibited asymmetry of nerve conduction latency (delayed MEP latency in the hemisphere corresponding to the weaker hand) and a relationship between delayed onset of iSP (measured in the hemisphere corresponding to the weaker hand) and MoCA, thus suggesting a cross-callosal disruption (Chaves et al., 2021a).
Celiac disease and other immune-mediated disorders
Adult patients with CD can present several neurological and neuropsychiatric symptoms, including cognitive complaints, which may range from a mild “brain fog” to an overt dementia. The few TMS studies available converge on a “hyperexcitable celiac brain” (reduced SICI and cSP, increased ICF), which partially recovers when a sustained period of gluten-free diet (GFD) is adopted (Pennisi et al., 2014, 2017; Bella et al., 2015). As such, since GFD may exert a neuroprotective role, it needs to be adopted very early, though its effects on neurological manifestations (and particularly on cognitive features) is debated yet (Lanza et al., 2018a). More recently, the excitability of transcallosal interhemispheric connections, assessed by the latency and duration of iSP, was explored in GFD-naïve CD subjects with respect to healthy individuals (Fisicaro et al., 2021b). The iSP resulted to be significantly shorter in CD and a direct correlation between iSP duration and cognitive score was found, compatible with an interhemispheric disinhibition and adding support to the impairment of GABA-mediated cortical and callosal circuitries (Fisicaro et al., 2021b). Very recently, central cholinergic functioning explored by SAI of the motor cortex resulted to be not affected in de novo CD patients compared to age-matched healthy controls, although the patients presented a statistically significant worse score at MoCA (Lanza et al., 2021). This finding might add support to the vascular inflammation hypothesis of the “gluten encephalopathy,” which seems to be due to an etiology different from that of the cholinergic dysfunction (Lebwohl et al., 2016).
Abnormal evoked potentials, including prolongation of MEP conduction times, have been recently reported in a 72-year-old female developing progressive impairment of cognition and gait, eventually leading to the diagnosis of autoimmune glial fibrillary acidic protein astrocytopathy (GFAP) based on the typical MRI findings of periventricular radial linear Gd enhancement and longitudinally extensive lesions in the spinal cord, and the anti-GFAP antibody detected in the cerebrospinal fluid (Tokimura et al., 2020).
No TMS study was performed in other immunologically mediated disorders, including primary or secondary vasculitis with central nervous system (CNS) involvement (Mansueto et al., 2022).
Endocrine disorders
Prolonged CMCT was reported in patients with both hypo- and hyperthyroidism, although no correlation was found with levels of free thyroxine (FT4), free 3, 3′, 5-L-triiodothyronine (FT3), and thyroid stimulating hormone (TSH), as well as with onset age and disease severity or duration. Notably, TMS changes did not entirely improve after restoration of euthyroidism (Ozata et al., 1996). A more extensive cortical excitability study was carried out in 10 patients with overt hypothyroidism and 10 age-matched healthy controls (Rizzo et al., 2008). At baseline, patients showed decreased cortical excitability, with increased rMT and decreased steepness of MEP recruitment curves. These changes were paralleled by longer cSP and decreased SICI. After 3 months of replacement therapy, all parameters restored to normal values, except for SICI, which needed 6 months of therapy to normalize (Rizzo et al., 2008). A more recent study has confirmed that thyroid hormones influence cortical inhibitory circuits. Accordingly, in overt hypothyroidism, cSP was prolonged and SICI decreased, whereas those with thyroid hormone resistance (elevated level of thyroid hormones but unsuppressed TSH) showed the opposite (Terranova et al., 2016). In patients with hypothyroidism, an impaired SICI [which is a measure of GABA-A receptor-mediated activity (Ziemann et al., 2015; Cash et al., 2017)] supports the hypothesis that thyroid hormones not only modulate cortical excitability but they can also influence the cortical inhibitory circuits.
As known, type-2 diabetes mellitus (T2DM) accelerates cognitive aging and increases risk of dementia. A previous prospective cross-sectional study (Fried et al., 2017) on 21 adults with T2DM and 15 demographically similar non-diabetic controls explored M1 LTP-like plasticity by comparing the MEP amplitude before and after iTBS. An abnormal cortico-motor plasticity that correlated with reduced verbal learning was found in patients. Moreover, since both iTBS after-effects and the Rey Auditory Verbal Learning Task performance are both NMDA receptor-dependent measures, their relationship in T2DM may reflect an impaired glutamatergic neurotransmission (Fried et al., 2017). In a subsequent study combining TMS with magnetic resonance spectroscopy (MRS) (Fried et al., 2019), neuroplastic mechanisms were found to be impaired also in pre-diabetes, whereas in T2DM patients reduced cortico-motor plasticity was associated with lower cortical glutamatergic metabolite concentration. These findings suggest that glutamate-mediated neurotransmission might represent a therapeutic target (Fried et al., 2019).
To our knowledge, no study is available in cognitive disorders due to other endocrine diseases.
Hepatic and renal diseases
Motor evoked potentials in hepatic encephalopathy (HE) are able to detect and monitor alterations in neurotransmission, likely related to myelin changes and microadenoma (Amodio and Montagnese, 2015). A single study reported a significant increase in rMT and CMCT, a reduction of the MEP/compound muscle action potential amplitude ratio, and a shorter cSP, thus suggesting that the inhibitory interneuronal network dysfunction plays a role in HE patients (Nolano et al., 1997).
Given that a previous experimental study reported that hyperammonemia leads to opposite changes of GABAergic neurotransmission with an increase in the cerebellum and a decrease in the cerebral cortex, the cerebellum GABA-mediated activity has been examined in 15 HE subjects with different disease severity (Hassan et al., 2019). The authors utilized a different form of paired-pulse TMS, i.e., the cerebellar inhibition (CBI), which is based on the evidence that a conditioning magnetic stimulus on the cerebellum reduces the MEP amplitude elicited by the test stimulus on contralateral M1 at 5–7 ms interstimulus intervals. CBI is considered to be generated by the activity of cerebellar Purkinje cells and subsequent GABA-mediated suppression of the dentato-thalamo-cortical tract (Ugawa et al., 1991; Groiss and Ugawa, 2012). This paradigm, applied in subjects with disorders affecting the cerebellum, such as spino-cerebellar ataxias, late onset ataxias, acquired cerebellar diseases, and multiple system atrophy, has revealed decreased CBI secondary to a damage in the cerebello-thalamo-cortical tract or in the cerebellum itself (Ugawa et al., 1994a,b, 1997; Grimaldi et al., 2014). HE patients showed a decreased cerebellar inhibition (in terms of increased GABA-mediated activity of Purkinje cells) that was related to disease severity, suggesting a dysfunction of the efferent cerebellar pathways (Hassan et al., 2019). A recent investigation supports this result showing a reduction of GABArgic inhibition, correlated with HE severity, although this was associated with an overall reduced cortico-spinal excitability (Groiss et al., 2019).
Of note, in minimal HE (i.e., the earliest form of HE presenting with psychomotor slowing and subtle impairment of cognition), paired-pulse TMS showed a significant increase of SICI and a reduction of ICF, suggesting an imbalance between excitatory and inhibitory intracortical mechanisms toward an increased inhibitory transmission (Nardone et al., 2016b). Furthermore, associative sensory-motor index of plasticity, an indirect measure of motor learning assessed by PAS protocol, resulted to be impaired in minimal HE patients with respect to age-matched healthy controls (Golaszewski et al., 2016). Finally, T2-MRI hyperintensity observed along the cortico-spinal tract in cirrhotic patients were found to relate to functional abnormalities (including increased rMT, decreased MEP amplitude, and prolonged CMCT, all more marked in the lower extremities) and to revert (except for CMCT at lower limbs) after liver transplantation. These findings suggest that mild cerebral edema along the cortico-spinal pathway may cause a neuronal dysfunction, clinically manifesting in minimal HE (Córdoba et al., 2003).
Wilson disease (WD) is a potentially treatable, inherited metabolism disorder characterized by the pathological accumulation of copper. Although the clinical course can vary, a progressive liver disease is a common feature, as well as some neurological (including cognitive) and psychiatric symptoms. With early diagnosis and treatment, the prognosis is favorable; however, it is of pivotal importance to diagnose patients before the onset of serious symptoms, such those related to CNS dysfunction (Członkowska et al., 2018). A systematic review of TMS in WD concluded for abnormal MEP features in 20–70% of participants, whereas motor responses could not be recorded in 7.6–66.7% of patients. Four studies reported significantly increased cortical excitability and prolonged CMCT, whereas one study found absent or prolonged MEP latency in 2/3 of patients (Bembenek et al., 2015). Interestingly, early diagnosis and prompt therapy of WD can prevent the occurrence of neurological signs, even at subclinical levels, as demonstrated by the normality of brain MRI, clinical scales, cognitive tests, and TMS measures of cortical excitability, including rMT, SICI, and cSP (Dubbioso et al., 2016). However, a previous study on 9 patients (6 of them with prolonged CMCT, reduced MEP amplitude, or absent responses in at least one of the examined muscles) revealed that in one patient only the abnormal responses normalized following treatment with penicillamine (Meyer et al., 1991). A reduction of cortical excitability, characterized by shortening of cSP and increasing of SICI and rMT, occurs when neurological signs become manifest (Dubbioso et al., 2016, 2020). Another recent study has described the longitudinal changes of rMT and CMCT in 18 WD patients. A progressive decrease of rMT was observed, whereas CMCT did not improve despite chelation therapy, thus suggesting that rMT may serve as a marker of chelation efficacy (Stezin et al., 2019).
Primary biliary cirrhosis (PBC) is a female-predominant autoimmune disease associated with fatigue, memory impairment, and sleep disturbances. It has been shown that PBC patients have impaired central activation and abnormal SICI (suggesting CNS abnormalities beyond the voluntary control) and that ICI and SICF were related to daytime somnolence (McDonald et al., 2010). However, both transplanted and non-transplanted patients showed similar abnormalities, raising questions about the mechanisms underpinning these changes and the neurological dysfunction in PBC (McDonald et al., 2010). Finally, compared to healthy controls, female patients did not show post-exercise depression of cortical excitability after voluntary submaximal tonic contraction of finger flexor muscles until exhaustion (Cerri et al., 2010). This might indicate an impairment of the neural mechanisms underlying central fatigue that occur in some PBC patients (Cerri et al., 2010).
Changes of cortical excitability have been also reported in the end-stage renal disease, with the recovery of SICI after hemodialysis, peritoneal dialysis, and transplantation (Battaglia et al., 2005).
Nutritional and alcoholic-related disorders
Central motor conduction time was found to be prolonged in cognitive decline due to vitamin B12 deficiency (Atanassova et al., 2004), although its prolongation might be related to a degeneration/demyelination of the lateral spinal columns. Another study showed that MEPs and MRI changes were consistent with myelin alteration and that the outcome at 6 months correlated with MEP changes (Misra et al., 2003). Given that the central motor pathway may respond to hydroxocobalamin therapy, MEPs can be useful in the monitoring of functional recovery following replacement treatment (Wu and Chu, 1996). To date, no study has been carried out in cognitive disorder due to other vitamin deficiency.
Five studies investigated the central motor conductivity in alcohol-related cognitive disorders: CMCT was significantly prolonged in the chronic alcoholics with frontal lobe atrophy, with a significant positive correlation between CMCT and atrophy severity (Oishi and Mochizuki, 1999). Additionally, in chronic alcoholism, the lack of TMS-evoked I waves pointed toward an impairment of intracortical neural circuitry (Di Lazzaro et al., 2004), whereas a significant CMCT prolongation correlated with frontal skills impairment on neuropsychological testing (Ravaglia et al., 2002). In one study comparing patients with the alcohol withdrawal syndrome, patients with chronic alcohol abuse, and healthy subjects, ICF was increased in the first group only, although it could be restored after administration of the glutamate receptor antagonist riluzole. Other parameters, such as rMT, SICI, and cSP, were not affected, supporting the hypothesis of a selective glutamatergic dysfunction during alcohol withdrawal (Nardone et al., 2010b).
In Marchiafava-Bignami’s syndrome, iSP abnormalities have been reported, along with an extensive abnormal signal intensity throughout the entire corpus callosum at MRI, thus suggesting a severe callosal demyelination (Nardone et al., 2006). Finally, a reduction of SAI was described in patients with amnesia due to Wernicke-Korsakoff syndrome (WKS), thus demonstrating the impairment of cholinergic cortical networks involved in SAI. However, none of the correlations between SAI and neuropsychological tests reached a statistical significance (Nardone et al., 2010a), suggesting that cholinergic dysfunction could not account for the memory disorder in these patients and that damage to the cholinergic system alone was not sufficient to cause a persisting amnesic disorder. It is likely that multiple neurochemical changes, including interactions between cholinergic and non-cholinergic neuromodulatory systems, underlie this amnesic syndrome (Nardone et al., 2010a). Accordingly, recent “pharmaco-TMS” evidence reveals that SAI can be influenced by other neurotransmitters (such as GABA) in addition to acetylcholine (Turco et al., 2018), and this should be considered when interpreting the lack of correlation between SAI values and neuropsychological scores observed by Nardone and colleagues (Nardone et al., 2010a).
Infectious and inflammatory diseases
A previous review in Herpes simplex encephalitis concluded that changes in evoked potential, including MEPs, are infrequent and, if present, an association with HIV infection should be suspected (Misra and Kalita, 1998). No study is available in other infectious diseases of the CNS.
Cortical excitability after PAS was found to be decreased in anti-NMDA receptor encephalitis (Volz et al., 2016). Also, the lower PAS-induced plasticity significantly correlated with the modified Rankin Scale and with a lower functional connectivity within the motor network. Follow-up assessments, available in 6 patients, demonstrated a parallel improvement of PAS-induced plasticity and functional status. These findings indicate that neurophysiological mechanisms, including cortical excitability, LTP-like plasticity, GABAergic and glutamatergic functioning, are altered in patients with anti-NMDA receptor encephalitis and they can be helpful in diagnostic, prognostic, and response to therapy (Volz et al., 2016).
Bickerstaff’s brainstem encephalitis (BBE) is a rare post-infectious neurological disease characterized by external ophthalmoplegia, ataxia, lower limb areflexia, extensor plantar response, and disturbance of consciousness (drowsiness and stupor, till coma). Cortico-hypoglossal and cortico-spinal conduction abnormalities were also reported in BBE, thus further supporting the multifaceted manifestations of anti-GQ1b IgG antibody-positive spectrum disorders (Lo and Ratnagopal, 2007). Finally, compared to other evoked potentials, MEP changes occurred more frequently in Japanese encephalitis, also with a prognostic value (Kalita and Misra, 2002).
Recently, the motor output has been probed in a 56-year-old man affected by acquired unilateral hemispheric atrophy secondary to Rasmussen encephalitis (Nardone et al., 2019b). Namely, the amplitude of the ipsilateral MEPs was higher than those of ten age-matched healthy individuals, thus demonstrating a reinforcement of motor ipsilateral projections from motor cortex unaffected to the affected limb (Nardone et al., 2019b).
Lastly, a non-negligible amount of subjects complains of cognitive impairment, apathy, and fatigue after COronaVIrus Disease-2019 infection (COVID-19) (Fisicaro et al., 2021a). The evaluation of rMT, MEP amplitude, and cSP duration from the left M1, before and 2 min after a fatiguing isometric pinching task, pointed out an overall GABAergic impairment, possibly representing the correlate of fatigue and explaining apathy and executive deficits observed in post-COVID-19 patients (Ortelli et al., 2021). A subsequent study explored the activity of inhibitory intracortical circuits and sensory-motor interactions in patients complaining of fatigue and presenting executive dysfunction after resolution of COVID-19 with neurological complications (Versace et al., 2021). TMS studies revealed a marked reduction of SICI, a disruption of long-interval intracortical inhibition (reflecting GABA-B-mediated inhibition), and a decrease of SAI, thus suggesting a dysfunction of GABAergic and cholinergic activity in the M1 in patients who recovered from COVID-19 with cognitive manifestations (Versace et al., 2021).
Sleep disorders
Transcranial magnetic stimulation changes have been reported in obstructive sleep apnea syndrome (OSAS), restless legs syndrome (RLS), insomnia, and sleep-deprived (SD) healthy subjects. Overall, OSAS tends to exhibit an increased motor cortex inhibition while the opposite is observed in RLS (Lanza et al., 2015), whereas excitability seems to be in favor of a profile of cortical disinhibition in chronic insomnia and SD individuals. Brain plasticity abnormalities have been demonstrated both in OSAS and RLS (Das et al., 2013; Lanza et al., 2018b,c, 2022b). SAI was significantly reduced in apneic patients compared to healthy controls, and SAI level was strongly correlated with neuropsychological scores, suggesting that cognitive deficits in OSAS may be, at least in part, related to impaired cholinergic neurotransmission, presumably caused by the intermitted nocturnal hypoxemia (Nardone et al., 2016a). A more recent navigated TMS study demonstrated an increased rMT and reduced SAI in OSAS, thus confirming previous findings of impaired cortical afferent inhibition in these patients (Rogić Vidaković et al., 2020).
In patients with idiopathic REM-sleep behavior disorder (RBD), which is a strong predictor of neurodegeneration (Mahowald and Schenck, 2018), mean SAI was found to be significantly reduced (Figorilli et al., 2021). SAI also correlated with episodic verbal memory and executive functions, supporting the hypothesis of cholinergic dysfunction and risk of cognitive impairment in some RBD patients (Nardone et al., 2012). SAI abnormalities were also revealed in RBD with a diagnosis of Parkinson’s disease (PD), thus suggesting that RBD-related cholinergic dysfunction may be a significant determinant of cognitive impairment in PD (Nardone et al., 2013; Fisicaro et al., 2020b). In RBD patients still asymptomatic for a parkinsonian syndrome, changes of ICF and, to a lesser extent, of SICI has been observed and these changes might precede neurodegeneration. Moreover, SICI correlated with muscle tone alteration, adding support the RBD model of retrograde influence on the cortex from the brainstem (Lanza et al., 2020a). Very recently, a direct TMS comparison between 15 patients with idiopathic RBD and 15 with PD with RBD, both age-matched to 15 healthy participants, showed that both patients’ groups shared a reduced ICF, thus suggesting the involvement of glutamatergic transmission not only in subjects at risk for degeneration but also in those with an overt α-synucleinopathy (Lanza et al., 2022a).
Rare genetic diseases
Niemann-Pick disease type C (NPC), a rare autosomal recessive lysosomal storage disorder, presents with a wide range of neurological manifestations, including cognitive impairment (Iodice et al., 2015). In three patients affected by the adult form of NPC, an alteration of SAI reduction was found (Manganelli et al., 2014), thus suggesting that cholinergic changes might play a role in the development of cognitive impairment in NPC (Manganelli et al., 2014) and strengthening the similarity with AD (Esposito et al., 2019). Another recent TMS study on two patients carrying a homozygous mutation in the NPC1 gene and in two heterozygous family members found that baseline SAI and LTP-like plasticity were impaired. After 12 months of treatment with miglustat, a considerable improvement of SAI and LTP-like plasticity was observed in the two patients, suggesting that these biomarkers might support the diagnosis and provide prognostic insights (Benussi et al., 2017). A further study showed that long term therapy with miglustat did not arrest the cognitive decline or improve the reduction of SAI, although it could stabilize other neurological manifestations (Tozza et al., 2018).
In chorea-acanthocytosis, an autosomal recessive neurodegenerative disorder characterized by adult-onset chorea, acanthocytes in the peripheral blood, and HD-like neuropsychiatric symptoms, a striking alteration of SICI was found, supporting the functional disruption of GABA-mediated networks (Dubbioso et al., 2017a). The authors also demonstrated a reduction of SAI, suggesting that cognitive and psychiatric disturbs might be underpinned by central cholinergic dysfunction (Dubbioso et al., 2017a).
A recent TMS study compared patients with neurodegeneration with brain iron accumulation (NBIA), such as patients with aceruloplasminemia and Kufor-Rakeb disease (PARK-9), and those with neurological WD. NBIA exhibited abnormal prolongation of cSP with respect to WD. On the contrary, WD displayed higher rMT and reduced cSP and SICI (Dubbioso et al., 2016, 2020). SAI, tested in NBIA only, was normal, suggesting a non-cholinergic origin of cognitive impairment in these subjects (Dubbioso et al., 2020).
In patients with spinocerebellar ataxias type 2 (SCA2), cortico-spinal tract involvement has been frequently reported (Restivo et al., 2000; Giardina et al., 2020), even in the pre-symptomatic stage (Velázquez-Pérez et al., 2017), and it seems to progress significantly during the pre-ataxic stage (Velázquez-Pérez et al., 2018). A first study (Restivo et al., 2002) found that mean cSP duration and rMT were significantly increased in the patient group. Conversely, SICI showed no significant difference between patients and controls, whereas at longer interstimulus intervals the expected facilitation of test responses resulted significantly less marked in patients. Notably, these alterations were related to the worsening of the general clinical status. The authors speculated that changes of motor cortex excitability in SCA2 might represent a slow neurodegenerative process characterized by a gradual loss of cerebellar neurons, eventually leading to an imbalance between inhibitory and excitatory circuits within the motor system (Restivo et al., 2002).
Similarly, TMS revealed cortico-spinal alterations even in subjects with mild clinical signs of cortico-spinal tract involvement in cerebrotendinous xanthomatosis (a rare neurometabolic disease due to defective activity of sterol 27-hydroxylase, with plasma and tissue cholestanol storage) (Mignarri et al., 2011), the clinical phenotype of which is characterized by both systemic manifestations and neurological signs, including cognitive decline. Namely, lower-limb CMCT was increased in 15 out of 24 (62.5%) patients, with interside difference abnormal in 9 (37.5%), whereas upper-limb CMCT was prolonged in 9 out of 24 (37.5%) with interside difference abnormal in 12 out of 24 (50%). MEPs at rest were absent in 13 out of 24 (54.2%) patients from lower limbs and in 4 out of 24 (16.7%) from upper limbs (Mignarri et al., 2011). Interestingly, MEPs revealed pyramidal tract alterations also in patients with subclinical signs only (i.e., pyramidal signs without disability), although no significant correlation was found between MEP alterations and Pyramidal Function Scale. Replacement treatment can prevent the progression of pyramidal damage, especially if started early (Mignarri et al., 2011).
A progressive axonal degeneration was proven to occur in the cortico-cortical projections (lack of transcallosal inhibition) and cortico-spinal tract (reduced MEP amplitude) in hereditary spastic paraplegia with thin corpus callosum (SPG11 gene) in two sisters with cognitive impairment (Winner et al., 2004). Lastly, in sialidosis type I, a rare inherited neurodegenerative disorder caused by mutations in the NEU1 gene, the slope of input/output was significantly increased, whereas SICI and cSP duration were reduced in patients compared to controls, suggesting that the major clinical changes take place above the brainstem (Huang et al., 2008).
Therapeutic applications
To date only one study assessed the effects of high frequency-rTMS over the right dorsolateral prefrontal cortex (DLPFC) on working memory in a small sample of MS patients compared to healthy controls (Hulst et al., 2017). The authors reported that task accuracy improved after real but not sham (fictitious) stimulation. Moreover, the task-related functional connectivity between the right DLPFC and right caudate nucleus and bilateral cingulate gyrus increased, suggesting a rTMS-induced change in network efficiency in cognitively impaired MS subjects (Hulst et al., 2017).
Few studies were performed in cerebellar degeneration (Lanza et al., 2019b), reporting an improvement in cognitive performance attributed to an enhanced motor function and resource utilization. Cerebellar rTMS might also have enhanced prefrontal functions through the cerebellar projections into this area, which, in turn, improved cognitive abilities (Pope and Miall, 2014).
Anecdotally, patients with alcohol-related cognitive deficit showed no LTP-like effect after 5 Hz-rTMS over the left M1, while the cSP (which was already increased at baseline) remained unchanged (Conte et al., 2008). The positive effect of high-frequency rTMS over the right DLPFC on the cognitive regulation of emotional processing, reappraisal, and craving in alcohol use disorder has been recently observed in 39 patients and 36 healthy controls in an rTMS/functional MRI study, thus implying that rTMS can reduce the emotional impact of images, as reflected in blood oxygenation level-dependent response in these patients (Jansen et al., 2019). The positive effect of high-frequency rTMS over the same cortical target (right DLPFC) on memory was also noted and appeared to correlate with increased in brain metabolites detected by proton (MRS) in recently detoxified alcohol-dependent patients (Qiao et al., 2016).
At a short latency between a peripheral nerve (conditioning) stimulus and a second stimulus (called test stimulus) applied contralaterally on motor cortex, the corticomotor response is increased due to facilitation, a phenomenon called afferent facilitation of the motor response. Following high-frequency rTMS over the prefrontal areas of severe subacute NMDA receptor antibody encephalitis, the afferent facilitation of motor responses increased by immunoglobulin (Ig)-G administration, highlighting that IgG may induce hyperexcitability (Manto et al., 2011).
Finally, the effect of rTMS on cognitive impairment induced by SD has been assessed. A first study in 2008 demonstrated that 5 Hz-rTMS over the upper-middle occipital cortex resulted in a reduction of the sleep-induced reaction time deficit, without a corresponding decrease in accuracy (Luber et al., 2008). Few years later, the same research group showed the beneficial effects of rTMS after 4 sessions of concurrent rTMS/task performance given over the course of 2 days of SD (Luber et al., 2013). Similar results were reported by other authors, with 5 Hz-rTMS over the left middle occipital gyrus, resulting in greater accuracy and shorter response times after SD (Martinez-Cancino et al., 2016; Guo et al., 2019). Lastly, a recent study has evaluated the effect of high-frequency rTMS on reversing the negative effects of SD for 24 h in 66 healthy individuals randomized into “real” rTMS group and “sham” group (Li et al., 2021). The authors showed that SD induced cognitive impairment, increase of anxiety, depression, cortisol levels, and brain-derived neurotrophic factor (BDNF) levels, whereas decreased frontal blood activation. Notably, rTMS improved the hyperactivity of the hypothalamic-pituitary-adrenal axis, decreased the frontal blood activation induced by SD, and reduced the consumption of plasma proBDNF (Li et al., 2021).
Discussion
Summary of the evidences
A first consideration that can be drawn from the present review in that, while in degenerative dementia neurophysiological alterations might mirror specific, and possibly primary, neuropathological changes (and hence be used as early biomarkers), this pathogenic link appears to be overall weaker for secondary forms of dementia, in which neurotransmitter dysfunction is more likely related to a systemic or diffuse neural damage. In these cases, therefore, an effort toward the understanding of pathological mechanisms of cognitive impairment should be made, also by investigating the relationship between functional alterations of brain circuits and the specific mechanisms of neuronal damage triggered by the causative disease.
A common factor that seems to emerge is that the development of hyperexcitability/disinhibition of cortical circuits may be secondary to several noxious conditions, and, as such, it might represent a compensatory phenomenon that might prevent the development of an excitatory dysfunction; alternatively, it might be the consequence of a predominant impairment of inhibitory interneurons. This hypothesis contrasts with the view of hyperexcitability as a possible causative factor in some neurodegenerative disorders, such as AD and dementia in motor neuron diseases (Ranieri et al., 2020; Di Lazzaro et al., 2021). Interestingly, a hyperexcitability is also suggested in a few secondary dementias for which more homogeneous studies are available. Namely, in NPH, where hypoperfusion is hypothesized to underlie the onset of clinical manifestations, TMS showed a reduced GABAergic transmission and a hyperexcitability of the motor cortex (Chistyakov et al., 2012; Nardone et al., 2019a).
On the other hand, in MS neuroinflammation is the main noxious factor and it has been related to cortical hyperexcitability before the occurrence of structural alterations (Simpson and Macdonell, 2015; Chaves et al., 2019). In a recent large series (Chaves et al., 2021b), measures of intracortical inhibition (i.e., cSP and iSP) did not correlate with cognitive changes, while reduced cortico-spinal excitability (i.e., increased rMT and reduced MEP amplitude) correlated with both motor impairment and cognition, possibly indicating that the later stages of the disease are more affected by neurodegeneration and greater disability. Nevertheless, sex-specific disruption in cortico-spinal excitability and hemispheric asymmetry have been recently found in MS, pointing to interhemispheric disruption as a potential biomarker of cognitive impairment and a possible target for neuromodulation treatments (Chaves et al., 2021a).
Other intriguing considerations arise from the comparison of NPH and MS. As known, the most relevant pathophysiological mechanism underlying cognitive decline in MS implies the degeneration of cortical and subcortical networks. NPH may be also characterized by some neurodegenerative mechanisms, which, at least in part, seem to overlap with those observed in some primary dementia (including AD and FTD). Similarly, a common mechanism for neurodegeneration in MS and AD has been suggested, likely due to an increased amyloid precursor protein expression in the axons around MS plaques (Chandra, 2015). In this context, blood flow and pulsation propagation changes have been demonstrated in MS patients, which are similar to those of NPH patients, and consistent with an underlying pulse wave encephalopathy component in MS (Bateman et al., 2016). Moreover, although cytokine production and immune activation have been associated with various degenerative and neuroimmune disorders (such as MS), inflammatory changes have been reported also in NPH. The enlarged brain ventricles may indeed activate the production of interleukin (IL)-1β, IL-6, IL-10, IL-21, and tumor necrosis factor-α, with a pattern similar to that observed in MS, thus reflecting an inflammatory component in NPH as well (Sosvorova et al., 2015). Translationally, this might be viewed as an example of how improve the classification of secondary dementias according to the putative etiological contribution of neurodegeneration processes, although current classifications do not fully consider it, except for the possibility of “mixed dementia” (e.g., of vascular and degenerative origin).
Among immune-mediated disorders, CD might affect CNS through multiple pathogenic factors in addition to the cross-reacting antibodies and immune complex deposition, including direct neurotoxicity and, especially in severe cases or in non-GFD patients, nutrients deficiency secondary to malabsorption. In this case, the predominant neurophysiological pattern is a hyperexcitability characterized by reduced GABAergic inhibition and facilitated glutamatergic transmission. However, hyperexcitability does not seem to be similarly associated with hyperammonemia-related damage in HE, where inconsistent results have been reported about changes in SICI, with reduced inhibition possibly characterizing only the severe stages (Groiss et al., 2019). Finally, in other cognitive disorders associated with mechanisms involving specific molecular targets (such as the anti-NMDA receptor encephalitis), circuitry dysfunction appears more strictly related to these pathogenic factors, in which an altered LTP-like linked to NMDA receptor-dependent plasticity has been demonstrated (Volz et al., 2016).
Of note, SAI is known to be reduced in primary cholinergic forms of dementia, such as AD and DLB (Di Lazzaro et al., 2021). Few reports indicate a reduction of SAI also in some secondary dementias, thus leading to hypothesize that central cholinergic dysfunction may contribute to cognitive decline. Interestingly, some of these alterations can be reverted by the specific treatment targeting the neuropathological mechanisms underlying these disorders, e.g., surgical drainage in NPH, immunomodulatory therapy in MS and autoimmune encephalitis, or GFD in CD.
Current limitations and research perspectives
Currently, no distinctive TMS pattern can be identified that is prompt to be used to predict the occurrence or progression of cognitive decline in a specific pathological condition. However, to date, most pathological hypotheses and proposed applications of TMS in secondary dementia remain speculative, mainly due to limiting factors in the collection and analysis of the articles reviewed, that prevent a meta-analysis approach. These mainly consist of the small sample size and heterogeneity of the pathological condition examined. Furthermore, most of the studies are based on inclusion criteria that are not specifically targeted on the presence and quantification of cognitive impairment. Additionally, due to the average small sample size of the individual studies, even in those conditions where a given parameter was consistently affected among different reports (such as the reduction of SICI in NPH and CD), the effect of other potential confounding factors, such as a “publication bias,” cannot be excluded. Therefore, unlike primary forms of dementia, such as AD, DLB, and FTD, where TMS indexes might be not far from a “bench-to-bedside” translation (Di Lazzaro et al., 2021), the heterogeneous and often limited data available in secondary forms of dementia do not allow to foresee a prompt diagnostic translation yet.
Additionally, as emerged from the neurological conditions here reviewed, it is still unclear which TMS abnormality can be related to the disease per se and which would be strictly related to dementia within the same disease. Nonetheless, specific TMS measures of cortical excitability were found to be consistently altered in some of the above-mentioned disorders, such as: rMT in NPH, HE, and OSAS; cSP in MS, CD, HE, and hypothyroidism; SAI in OSAS, RBD, and NPC; SICI in NPH, CD, and hypothyroidism. Moreover, the progression of HE from minimal (without a clear cognitive impairment) to an overt HE can be seen as an example of how differentiate the TMS correlates of a disease from a dementing process in the context of that disease: basically normal TMS profile in minimal HE (normal rMT, normal or slightly increased CMCT) and clearly altered in overt HE (increased rMT, prolonged CMCT). Therefore, these neurophysiological measures might add useful information even in secondary dementia, especially when combined with suggestive clinical features and other diagnostic exams.
Finally, the possibility to detect cortical dysfunction and maladaptive plasticity, to monitor the disease course and complications, to probe the response to treatment, including innovative neuromodulatory interventions, still represents a gap in the literature and a challenge for research. In this scenario, the identification of reliable TMS metrics in secondary dementia will provide not only biomarkers useful for the diagnosis and the prognosis, but also possible targets for non-invasive neuromodulation protocols. Indeed, the possibility to detect cortical dysfunction and maladaptive plasticity will greatly benefit from the recording of pattern consistently altered in specific conditions, that can be better evaluated in experimental models and then possibly applied also to probe the response to pharmacological or neuromodulatory treatments in longitudinal studies. As such, in addition to the already available studies, which have used only high-frequency (excitatory) rTMS in a few disorders (i.e., chronic ethanol abuse, MS, and SD-related cognitive impairment), other protocols using low-frequency (inhibitory) or high-frequency protocols customized on the TMS patterns observed should be prompted. For instance, low frequency rTMS might be applied to reduce hyperexcitability in some disorders (e.g., reduced rMT and SICI in NPH; reduced SICI and cSP and increased ICF in CD), whereas further high frequency protocols might be considered in others with increased rMT and prolonged CMCT (e.g., HE, OSAS, and SCA2).
The research agenda within these promising lines of investigation may include the following:
(i) defining reliable TMS parameters of cortical circuitry dysfunction; this will require to focus on single or pooled measures that show to be consistently altered in a given disease typically associated with cognitive impairment, in order to overcome the issue of undersized studies;
(ii) obtaining further neurophysiological insights on the pathophysiological changes typical of each disorder considered, that might be investigated in experimental models and tested as a target of new pharmacological treatments or neuromodulatory interventions;
(iii) expanding the exploration of functional response to therapies; this applies to all potentially treatable or modifiable conditions and will require longitudinal trials monitoring the course of cognitive impairment and TMS indexes of cortical plasticity or cortico-spinal conductivity.
Author contributions
GL and VD: conceptualization. FF and MC: literature search. RD and FR: data analysis. GL and AC: writing – original draft preparation. MP and AG: writing – review and editing. RB and VD: supervision. All authors contributed to the article and approved the submitted version.
Funding
This research was partially funded by a grant of the “Piano di Incentivi per la Ricerca de Ateneo 2020–2022 (PIACERI)”, Linea 2 – FAMOUS-UNICT (UPB 5C722012125), and Università degli Studi di Catania, Catania (Italy).
Conflict of interest
The authors declare that the research was conducted in the absence of any commercial or financial relationships that could be construed as a potential conflict of interest.
Publisher’s note
All claims expressed in this article are solely those of the authors and do not necessarily represent those of their affiliated organizations, or those of the publisher, the editors and the reviewers. Any product that may be evaluated in this article, or claim that may be made by its manufacturer, is not guaranteed or endorsed by the publisher.
References
Agrawal, A., Bhattacharya, A., Kamble, N., Yadav, R., and Pal, P. K. (2021). Effect of lumbar drainage on cortical excitability in normal pressure hydrocephalus. Can. J. Neurol. Sci. J. Can. Sci. Neurol. 48, 253–258. doi: 10.1017/cjn.2020.169
Amodio, P., and Montagnese, S. (2015). Clinical neurophysiology of hepatic encephalopathy. J. Clin. Exp. Hepatol. 5, S60–S68. doi: 10.1016/j.jceh.2014.06.007
Anderson, B., Mishory, A., Nahas, Z., Borckardt, J. J., Yamanaka, K., Rastogi, K., et al. (2006). Tolerability and safety of high daily doses of repetitive transcranial magnetic stimulation in healthy young men. J. ECT 22, 49–53. doi: 10.1097/00124509-200603000-00011
Atanassova, P. A., Chalakova, N. T., Goranov, S. E., Ilieva, E. M., Sotirova, K. N., and Massaldjieva, R. I. (2004). A case of encephalomyelopolyneuropathy in vitamin B12 deficiency. Folia Med. 46, 52–54.
Ayache, S. S., and Chalah, M. A. (2017). Cortical excitability changes: A mirror to the natural history of multiple sclerosis? Neurophysiol. Clin. Clin. Neurophysiol. 47, 221–223. doi: 10.1016/j.neucli.2017.02.001
Ayache, S. S., Créange, A., Farhat, W. H., Zouari, H. G., Lesage, C., Palm, U., et al. (2016). Cortical excitability changes over time in progressive multiple sclerosis. Funct. Neurol. 30, 257–263. doi: 10.11138/FNeur/2015.30.4.257
Ayache, S. S., Créange, A., Farhat, W. H., Zouari, H. G., Mylius, V., Ahdab, R., et al. (2014). Relapses in multiple sclerosis: Effects of high-dose steroids on cortical excitability. Eur. J. Neurol. 21, 630–636. doi: 10.1111/ene.12356
Barker, A. T., Jalinous, R., and Freeston, I. L. (1985). Non-invasive magnetic stimulation of human motor cortex. Lancet Lond. Engl. 1, 1106–1107. doi: 10.1016/s0140-6736(85)92413-4
Bateman, G. A., Lechner-Scott, J., and Lea, R. A. (2016). A comparison between the pathophysiology of multiple sclerosis and normal pressure hydrocephalus: Is pulse wave encephalopathy a component of MS? Fluids Barriers CNS 13:18. doi: 10.1186/s12987-016-0041-2
Battaglia, F., Quartarone, A., Bagnato, S., Rizzo, V., Morgante, F., Floccari, F., et al. (2005). Brain dysfunction in uremia: A question of cortical hyperexcitability? Clin. Neurophysiol. 116, 1507–1514. doi: 10.1016/j.clinph.2005.02.016
Bella, R., Lanza, G., Cantone, M., Giuffrida, S., Puglisi, V., Vinciguerra, L., et al. (2015). Effect of a gluten-free diet on cortical excitability in adults with celiac disease. PLoS One 10:e0129218. doi: 10.1371/journal.pone.0129218
Bembenek, J. P., Kurczych, K., and Członkowska, A. (2015). TMS-induced motor evoked potentials in Wilson’s disease: A systematic literature review. Bioelectromagnetics 36, 255–266. doi: 10.1002/bem.21909
Benussi, A., Cotelli, M. S., Cosseddu, M., Bertasi, V., Turla, M., Salsano, E., et al. (2017). Preliminary results on long-term potentiation-like cortical plasticity and cholinergic dysfunction after miglustat treatment in niemann-pick disease type C. JIMD Rep. 36, 19–27. doi: 10.1007/8904_2016_33
Burt, T., Lisanby, S. H., and Sackeim, H. A. (2002). Neuropsychiatric applications of transcranial magnetic stimulation: A meta analysis. Int. J. Neuropsychopharmacol. 5, 73–103. doi: 10.1017/S1461145702002791
Canham, L. J. W., Kane, N., Oware, A., Walsh, P., Blake, K., Inglis, K., et al. (2015). Multimodal neurophysiological evaluation of primary progressive multiple sclerosis – An increasingly valid biomarker, with limits. Mult. Scler. Relat. Disord. 4, 607–613. doi: 10.1016/j.msard.2015.07.009
Cantello, R., Gianelli, M., Civardi, C., and Mutani, R. (1992). Magnetic brain stimulation: The silent period after the motor evoked potential. Neurology 42, 1951–1959. doi: 10.1212/wnl.42.10.1951
Cantone, M., Di Pino, G., Capone, F., Piombo, M., Chiarello, D., Cheeran, B., et al. (2014). The contribution of transcranial magnetic stimulation in the diagnosis and in the management of dementia. Clin. Neurophysiol. 125, 1509–1532. doi: 10.1016/j.clinph.2014.04.010
Cantone, M., Lanza, G., Fisicaro, F., Pennisi, M., Bella, R., Di Lazzaro, V., et al. (2020). Evaluation and treatment of vascular cognitive impairment by transcranial magnetic stimulation. Neural Plast. 2020, 1–17. doi: 10.1155/2020/8820881
Cantone, M., Lanza, G., Vinciguerra, L., Puglisi, V., Ricceri, R., Fisicaro, F., et al. (2019b). Age, height, and sex on motor evoked potentials: Translational data from a large Italian cohort in a clinical environment. Front. Hum. Neurosci. 13:185. doi: 10.3389/fnhum.2019.00185
Cantone, M., Lanza, G., Le Pira, A., Barone, R., Pennisi, G., Bella, R., et al. (2019a). Adjunct diagnostic value of transcranial magnetic stimulation in mucopolysaccharidosis-related cervical myelopathy: A pilot study. Brain Sci. 9:200. doi: 10.3390/brainsci9080200
Cantone, M., Lanza, G., Ranieri, F., Opie, G. M., and Terranova, C. (2021). Editorial: Non-invasive brain stimulation in the study and modulation of metaplasticity in neurological disorders. Front. Neurol. 12:721906. doi: 10.3389/fneur.2021.721906
Carson, R. G., and Kennedy, N. C. (2013). Modulation of human corticospinal excitability by paired associative stimulation. Front. Hum. Neurosci. 7:823. doi: 10.3389/fnhum.2013.00823
Caruso, G., Godos, J., Privitera, A., Lanza, G., Castellano, S., Chillemi, A., et al. (2022). Phenolic acids and prevention of cognitive decline: Polyphenols with a neuroprotective role in cognitive disorders and Alzheimer’s disease. Nutrients 14:819. doi: 10.3390/nu14040819
Cash, R. F. H., Noda, Y., Zomorrodi, R., Radhu, N., Farzan, F., Rajji, T. K., et al. (2017). Characterization of glutamatergic and GABAA-mediated neurotransmission in motor and dorsolateral prefrontal cortex using paired-pulse TMS-EEG. Neuropsychopharmacol. Off. Publ. Am. Coll. Neuropsychopharmacol. 42, 502–511. doi: 10.1038/npp.2016.133
Cerri, G., Cocchi, C. A., Montagna, M., Zuin, M., Podda, M., Cavallari, P., et al. (2010). Patients with primary biliary cirrhosis do not show post-exercise depression of cortical excitability. Clin. Neurophysiol. Off. J. Int. Fed. Clin. Neurophysiol. 121, 1321–1328. doi: 10.1016/j.clinph.2009.12.040
Chalah, M. A., Kauv, P., Créange, A., Hodel, J., Lefaucheur, J.-P., and Ayache, S. S. (2019). Neurophysiological, radiological and neuropsychological evaluation of fatigue in multiple sclerosis. Mult. Scler. Relat. Disord. 28, 145–152. doi: 10.1016/j.msard.2018.12.029
Chalah, M. A., Palm, U., Lefaucheur, J.-P., Créange, A., and Ayache, S. S. (2018). Interhermispheric inhibition predicts anxiety levels in multiple sclerosis: A corticospinal excitability study. Brain Res. 1699, 186–194. doi: 10.1016/j.brainres.2018.08.029
Chandra, A. (2015). Role of amyloid from a multiple sclerosis perspective: A literature review. Neuroimmunomodulation 22, 343–346. doi: 10.1159/000375309
Chaves, A. R., Snow, N. J., Alcock, L. R., and Ploughman, M. (2021b). Probing the brain–body connection using transcranial magnetic stimulation (TMS): Validating a promising tool to provide biomarkers of neuroplasticity and central nervous system function. Brain Sci. 11:384. doi: 10.3390/brainsci11030384
Chaves, A. R., Kenny, H. M., Snow, N. J., Pretty, R. W., and Ploughman, M. (2021a). Sex-specific disruption in corticospinal excitability and hemispheric (a)symmetry in multiple sclerosis. Brain Res. 1773:147687. doi: 10.1016/j.brainres.2021.147687
Chaves, A. R., Wallack, E. M., Kelly, L. P., Pretty, R. W., Wiseman, H. D., Chen, A., et al. (2019). Asymmetry of brain excitability: A new biomarker that predicts objective and subjective symptoms in multiple sclerosis. Behav. Brain Res. 359, 281–291. doi: 10.1016/j.bbr.2018.11.005
Cheeran, B., Koch, G., Stagg, C. J., Baig, F., and Teo, J. (2010). Transcranial magnetic stimulation: From neurophysiology to pharmacology, molecular biology and genomics. Neurosci. Rev. J. Bringing Neurobiol. Neurol. Psychiatry 16, 210–221. doi: 10.1177/1073858409349901
Chen, R. (2020). Guideline on therapeutic use of repetitive transcranial magnetic stimulation: Useful but know the methods and limitations. Clin. Neurophysiol. Off. J. Int. Fed. Clin. Neurophysiol. 131, 461–462. doi: 10.1016/j.clinph.2019.11.020
Chen, R., Cros, D., Curra, A., Di Lazzaro, V., Lefaucheur, J.-P., Magistris, M. R., et al. (2008). The clinical diagnostic utility of transcranial magnetic stimulation: Report of an IFCN committee. Clin. Neurophysiol. Off. J. Int. Fed. Clin. Neurophysiol. 119, 504–532. doi: 10.1016/j.clinph.2007.10.014
Chen, R., Lozano, A. M., and Ashby, P. (1999). Mechanism of the silent period following transcranial magnetic stimulation. Evidence from epidural recordings. Exp. Brain Res. 128, 539–542. doi: 10.1007/s002210050878
Chistyakov, A. V., Hafner, H., Sinai, A., Kaplan, B., and Zaaroor, M. (2012). Motor cortex disinhibition in normal-pressure hydrocephalus. J. Neurosurg. 116, 453–459. doi: 10.3171/2011.9.JNS11678
Cirillo, G., Di Pino, G., Capone, F., Ranieri, F., Florio, L., Todisco, V., et al. (2017). Neurobiological after-effects of non-invasive brain stimulation. Brain Stimulat. 10, 1–18. doi: 10.1016/j.brs.2016.11.009
Concerto, C., Lanza, G., Fisicaro, F., Pennisi, M., Rodolico, A., Torrisi, G., et al. (2022). Repetitive transcranial magnetic stimulation for post-traumatic stress disorder: Lights and shadows. World J. Clin. Cases 10, 5929–5933. doi: 10.12998/wjcc.v10.i17.5929
Conte, A., Attilia, M. L., Gilio, F., Iacovelli, E., Frasca, V., Bettolo, C. M., et al. (2008). Acute and chronic effects of ethanol on cortical excitability. Clin. Neurophysiol. 119, 667–674. doi: 10.1016/j.clinph.2007.10.021
Córdoba, J., Raguer, N., Flavià, M., Vargas, V., Jacas, C., Alonso, J., et al. (2003). T2 hyperintensity along the cortico-spinal tract in cirrhosis relates to functional abnormalities. Hepatol. Baltim. Md 38, 1026–1033. doi: 10.1053/jhep.2003.50406
Cucurachi, L., Immovilli, P., Granella, F., Pavesi, G., and Cattaneo, L. (2008). Short-latency afferent inhibition predicts verbal memory performance in patients with multiple sclerosis. J. Neurol. 255, 1949–1956. doi: 10.1007/s00415-008-0041-5
Członkowska, A., Litwin, T., Dusek, P., Ferenci, P., Lutsenko, S., Medici, V., et al. (2018). Wilson disease. Nat. Rev. Dis. Primer 4:21. doi: 10.1038/s41572-018-0018-3
Das, A., Anupa, A. V., and Radhakrishnan, A. (2013). Reduced plastic brain responses to repetitive transcranial magnetic stimulation in severe obstructive sleep apnea syndrome. Sleep Med. 14, 636–640. doi: 10.1016/j.sleep.2013.04.008
Di Lazzaro, V., Bella, R., Benussi, A., Bologna, M., Borroni, B., Capone, F., et al. (2021). Diagnostic contribution and therapeutic perspectives of transcranial magnetic stimulation in dementia. Clin. Neurophysiol 132, 2568–2607. doi: 10.1016/j.clinph.2021.05.035
Di Lazzaro, V., Oliviero, A., Mazzone, P., Insola, A., Pilato, F., Saturno, E., et al. (2001). Comparison of descending volleys evoked by monophasic and biphasic magnetic stimulation of the motor cortex in conscious humans. Exp. Brain Res. 141, 121–127. doi: 10.1007/s002210100863
Di Lazzaro, V., Oliviero, A., Pilato, F., Saturno, E., Dileone, M., Marra, C., et al. (2005). Neurophysiological predictors of long term response to AChE inhibitors in AD patients. J. Neurol. Neurosurg. Psychiatry 76, 1064–1069. doi: 10.1136/jnnp.2004.051334
Di Lazzaro, V., Oliviero, A., Pilato, F., Saturno, E., Dileone, M., Meglio, M., et al. (2004). Direct recording of the output of the motor cortex produced by transcranial magnetic stimulation in a patient with cerebral cortex atrophy. Clin. Neurophysiol. Off. J. Int. Fed. Clin. Neurophysiol. 115, 112–115. doi: 10.1016/s1388-2457(03)00320-1
Di Lazzaro, V., Oliviero, A., Profice, P., Pennisi, M. A., Di Giovanni, S., Zito, G., et al. (2000). Muscarinic receptor blockade has differential effects on the excitability of intracortical circuits in the human motor cortex. Exp. Brain Res. 135, 455–461. doi: 10.1007/s002210000543
Di Lazzaro, V., Pilato, F., Dileone, M., Profice, P., Ranieri, F., Ricci, V., et al. (2007). Segregating two inhibitory circuits in human motor cortex at the level of GABAA receptor subtypes: A TMS study. Clin. Neurophysiol. Off. J. Int. Fed. Clin. Neurophysiol. 118, 2207–2214. doi: 10.1016/j.clinph.2007.07.005
Di Lazzaro, V., Pilato, F., Dileone, M., Ranieri, F., Ricci, V., Profice, P., et al. (2006a). GABAA receptor subtype specific enhancement of inhibition in human motor cortex. J. Physiol. 575, 721–726. doi: 10.1113/jphysiol.2006.114694
Di Lazzaro, V., Pilato, F., Oliviero, A., Dileone, M., Saturno, E., Mazzone, P., et al. (2006c). Origin of facilitation of motor-evoked potentials after paired magnetic stimulation: Direct recording of epidural activity in conscious humans. J. Neurophysiol. 96, 1765–1771. doi: 10.1152/jn.00360.2006
Di Lazzaro, V., Pilato, F., Dileone, M., Saturno, E., Oliviero, A., Marra, C., et al. (2006b). In vivo cholinergic circuit evaluation in frontotemporal and Alzheimer dementias. Neurology 66, 1111–1113. doi: 10.1212/01.wnl.0000204183.26231.23
Di Lazzaro, V., Profice, P., Pilato, F., Dileone, M., Oliviero, A., and Ziemann, U. (2010). The effects of motor cortex rTMS on corticospinal descending activity. Clin. Neurophysiol. Off. J. Int. Fed. Clin. Neurophysiol. 121, 464–473. doi: 10.1016/j.clinph.2009.11.007
Di Pino, G., Pellegrino, G., Assenza, G., Capone, F., Ferreri, F., Formica, D., et al. (2014a). Modulation of brain plasticity in stroke: A novel model for neurorehabilitation. Nat. Rev. Neurol. 10, 597–608. doi: 10.1038/nrneurol.2014.162
Di Pino, G., Pellegrino, G., Capone, F., and Di Lazzaro, V. (2014b). Human cerebral cortex metaplasticity and stroke recovery. Austin J Cerebrovasc Stroke 1:1001.
Dubbioso, R., Raffin, E., Karabanov, A., Thielscher, A., and Siebner, H. R. (2017b). Centre-surround organization of fast sensorimotor integration in human motor hand area. NeuroImage 158, 37–47. doi: 10.1016/j.neuroimage.2017.06.063
Dubbioso, R., Esposito, M., Peluso, S., Iodice, R., De Michele, G., Santoro, L., et al. (2017a). Disruption of GABA(A)-mediated intracortical inhibition in patients with chorea-acanthocytosis. Neurosci. Lett. 654, 107–110. doi: 10.1016/j.neulet.2017.06.032
Dubbioso, R., Madsen, K. H., Thielscher, A., and Siebner, H. R. (2021). The myelin content of the human precentral hand knob reflects interindividual differences in manual motor control at the physiological and behavioral level. J. Neurosci. 41, 3163–3179. doi: 10.1523/JNEUROSCI.0390-20.2021
Dubbioso, R., Manganelli, F., Siebner, H. R., and Di Lazzaro, V. (2019). Fast intracortical sensory-motor integration: A window into the pathophysiology of Parkinson’s disease. Front. Hum. Neurosci. 13:111. doi: 10.3389/fnhum.2019.00111
Dubbioso, R., Ranucci, G., Esposito, M., Di Dato, F., Topa, A., Quarantelli, M., et al. (2016). Subclinical neurological involvement does not develop if Wilson’s disease is treated early. Parkinsonism Relat. Disord. 24, 15–19. doi: 10.1016/j.parkreldis.2016.01.024
Dubbioso, R., Ruggiero, L., Esposito, M., Tarantino, P., De Angelis, M., Aruta, F., et al. (2020). Different cortical excitability profiles in hereditary brain iron and copper accumulation. Neurol. Sci. Off. J. Ital. Neurol. Soc. Ital. Soc. Clin. Neurophysiol. 41, 679–685. doi: 10.1007/s10072-019-04147-0
Dutta, R., and Trapp, B. D. (2014). Relapsing and progressive forms of multiple sclerosis: Insights from pathology. Curr. Opin. Neurol. 27, 271–278. doi: 10.1097/WCO.0000000000000094
Espay, A. J., Da Prat, G. A., Dwivedi, A. K., Rodriguez-Porcel, F., Vaughan, J. E., Rosso, M., et al. (2017). Deconstructing normal pressure hydrocephalus: Ventriculomegaly as early sign of neurodegeneration. Ann. Neurol. 82, 503–513. doi: 10.1002/ana.25046
Esposito, M., Dubbioso, R., Tozza, S., Iodice, R., Aiello, M., Nicolai, E., et al. (2019). In vivo evidence of cortical amyloid deposition in the adult form of Niemann Pick type C. Heliyon 5:e02776. doi: 10.1016/j.heliyon.2019.e02776
Faro, A., Giordano, D., Kavasidis, I., Pino, C., Spampinato, C., Cantone, M. G., et al. (2010). “An interactive tool for customizing clinical transacranial magnetic stimulation (TMS) experiments,” in XII Mediterranean Conference on medical and biological engineering and computing 2010 IFMBE proceedings, eds P. D. Bamidis and N. Pallikarakis (Berlin: Springer), 200–203. doi: 10.1007/978-3-642-13039-7_50
Fernández, V. (2021). The use of motor-evoked potentials in clinical trials in multiple sclerosis. J. Clin. Neurophysiol. Off. Publ. Am. Electroencephalogr. Soc. 38, 166–170. doi: 10.1097/WNP.0000000000000734
Ferreri, F., and Rossini, P. M. (2013). TMS and TMS-EEG techniques in the study of the excitability, connectivity, and plasticity of the human motor cortex. Rev. Neurosci. 24, 431–442. doi: 10.1515/revneuro-2013-0019
Ferreri, F., Pasqualetti, P., Määttä, S., Ponzo, D., Ferrarelli, F., Tononi, G., et al. (2011). Human brain connectivity during single and paired pulse transcranial magnetic stimulation. NeuroImage 54, 90–102. doi: 10.1016/j.neuroimage.2010.07.056
Figorilli, M., Lanza, G., Congiu, P., Lecca, R., Casaglia, E., Mogavero, M. P., et al. (2021). Neurophysiological aspects of REM sleep behavior disorder (RBD): A narrative review. Brain Sci. 11:1588. doi: 10.3390/brainsci11121588
Fisicaro, F., Lanza, G., D’Agate, C. C., Ferri, R., Cantone, M., Falzone, L., et al. (2021b). Intracortical and intercortical motor disinhibition to transcranial magnetic stimulation in newly diagnosed celiac disease patients. Nutrients 13:1530. doi: 10.3390/nu13051530
Fisicaro, F., Di Napoli, M., Liberto, A., Fanella, M., Di Stasio, F., Pennisi, M., et al. (2021a). Neurological sequelae in patients with COVID-19: A histopathological perspective. Int. J. Environ. Res. Public. Health 18:1415. doi: 10.3390/ijerph18041415
Fisicaro, F., Lanza, G., Bella, R., and Pennisi, M. (2020a). “Self-neuroenhancement”: The last frontier of noninvasive brain stimulation? J. Clin. Neurol. 16, 158–159. doi: 10.3988/jcn.2020.16.1.158
Fisicaro, F., Lanza, G., Cantone, M., Ferri, R., Pennisi, G., Nicoletti, A., et al. (2020b). Clinical and electrophysiological hints to tms in de novo patients with Parkinson’s disease and progressive supranuclear palsy. J. Pers. Med. 10:274. doi: 10.3390/jpm10040274
Fried, P. J., Pascual-Leone, A., and Bolo, N. R. (2019). Diabetes and the link between neuroplasticity and glutamate in the aging human motor cortex. Clin. Neurophysiol. Off. J. Int. Fed. Clin. Neurophysiol. 130, 1502–1510. doi: 10.1016/j.clinph.2019.04.721
Fried, P. J., Schilberg, L., Brem, A.-K., Saxena, S., Wong, B., Cypess, A. M., et al. (2017). Humans with type-2 diabetes show abnormal long-term potentiation-like cortical plasticity associated with verbal learning deficits. J. Alzheimers Dis. JAD 55, 89–100. doi: 10.3233/JAD-160505
Giardina, F., Lanza, G., Calì, F., and Ferri, R. (2020). Late-onset oro-facial dyskinesia in Spinocerebellar Ataxia type 2: A case report. BMC Neurol. 20:156. doi: 10.1186/s12883-020-01739-8
Gladding, C. M., Fitzjohn, S. M., and Molnár, E. (2009). Metabotropic glutamate receptor-mediated long-term depression: Molecular mechanisms. Pharmacol. Rev. 61, 395–412. doi: 10.1124/pr.109.001735
Golaszewski, S., Langthaler, P. B., Schwenker, K., Florea, C., Christova, M., Brigo, F., et al. (2016). Abnormal cortical synaptic plasticity in minimal hepatic encephalopathy. Brain Res. Bull. 125, 200–204. doi: 10.1016/j.brainresbull.2016.07.011
Gomes-Osman, J., Indahlastari, A., Fried, P. J., Cabral, D. L. F., Rice, J., Nissim, N. R., et al. (2018). Non-invasive brain stimulation: Probing intracortical circuits and improving cognition in the aging brain. Front. Aging Neurosci. 10:177. doi: 10.3389/fnagi.2018.00177
Graff-Radford, N. R., and Jones, D. T. (2019). Normal pressure hydrocephalus. Contin. Minneap. Minn 25, 165–186. doi: 10.1212/CON.0000000000000689
Grimaldi, G., Argyropoulos, G. P., Boehringer, A., Celnik, P., Edwards, M. J., Ferrucci, R., et al. (2014). Non-invasive cerebellar stimulation–a consensus paper. Cerebellum Lond. Engl. 13, 121–138. doi: 10.1007/s12311-013-0514-7
Groiss, S. J., and Ugawa, Y. (2012). Cerebellar stimulation in ataxia. Cerebellum Lond. Engl. 11, 440–442. doi: 10.1007/s12311-011-0329-3
Groiss, S. J., Butz, M., Baumgarten, T. J., Füllenbach, N.-D., Häussinger, D., and Schnitzler, A. (2019). GABA-ergic tone hypothesis in hepatic encephalopathy – Revisited. Clin. Neurophysiol. Off. J. Int. Fed. Clin. Neurophysiol. 130, 911–916. doi: 10.1016/j.clinph.2019.03.011
Guo, Z., Jiang, Z., Jiang, B., McClure, M. A., and Mu, Q. (2019). High-frequency repetitive transcranial magnetic stimulation could improve impaired working memory induced by sleep deprivation. Neural Plast. 2019:7030286. doi: 10.1155/2019/7030286
Hassan, S. S., Baumgarten, T. J., Ali, A. M., Füllenbach, N.-D., Jördens, M. S., Häussinger, D., et al. (2019). Cerebellar inhibition in hepatic encephalopathy. Clin. Neurophysiol. Off. J. Int. Fed. Clin. Neurophysiol. 130, 886–892. doi: 10.1016/j.clinph.2019.02.020
He, W., Fong, P.-Y., Leung, T. W. H., and Huang, Y.-Z. (2020). Protocols of non-invasive brain stimulation for neuroplasticity induction. Neurosci. Lett. 719:133437. doi: 10.1016/j.neulet.2018.02.045
Hofstadt-van Oy, U., Keune, P. M., Muenssinger, J., Hagenburger, D., and Oschmann, P. (2015). Normative data and long-term test-retest reliability of the triple stimulation technique (TST) in multiple sclerosis. Clin. Neurophysiol. Off. J. Int. Fed. Clin. Neurophysiol. 126, 356–364. doi: 10.1016/j.clinph.2014.05.032
Hoogendam, J. M., Ramakers, G. M. J., and Di Lazzaro, V. (2010). Physiology of repetitive transcranial magnetic stimulation of the human brain. Brain Stimulat. 3, 95–118. doi: 10.1016/j.brs.2009.10.005
Huang, Y.-Z., Lai, S.-C., Lu, C.-S., Weng, Y.-H., Chuang, W.-L., and Chen, R.-S. (2008). Abnormal cortical excitability with preserved brainstem and spinal reflexes in sialidosis type I. Clin. Neurophysiol. Off. J. Int. Fed. Clin. Neurophysiol. 119, 1042–1050. doi: 10.1016/j.clinph.2008.01.023
Hulst, H. E., Goldschmidt, T., Nitsche, M. A., de Wit, S. J., van den Heuvel, O. A., Barkhof, F., et al. (2017). rTMS affects working memory performance, brain activation and functional connectivity in patients with multiple sclerosis. J. Neurol. Neurosurg. Psychiatry 88, 386–394. doi: 10.1136/jnnp-2016-314224
Iodice, R., Dubbioso, R., Topa, A., Ruggiero, L., Pisciotta, C., Esposito, M., et al. (2015). Electrophysiological characterization of adult-onset Niemann-Pick type C disease. J. Neurol. Sci. 348, 262–265. doi: 10.1016/j.jns.2014.12.005
Jansen, J. M., van den Heuvel, O. A., van der Werf, Y. D., de Wit, S. J., Veltman, D. J., van den Brink, W., et al. (2019). The effect of high-frequency repetitive transcranial magnetic stimulation on emotion processing, reappraisal, and craving in alcohol use disorder patients and healthy controls: A functional magnetic resonance imaging study. Front. Psychiatry 10:272. doi: 10.3389/fpsyt.2019.00272
Jung, P., and Ziemann, U. (2009). Homeostatic and nonhomeostatic modulation of learning in human motor cortex. J. Neurosci. Off. J. Soc. Neurosci. 29, 5597–5604. doi: 10.1523/JNEUROSCI.0222-09.2009
Kalita, J., and Misra, U. K. (2002). Neurophysiological changes in Japanese encephalitis. Neurol. India 50, 262–266.
Kobayashi, M., and Pascual-Leone, A. (2003). Transcranial magnetic stimulation in neurology. Lancet Neurol. 2, 145–156.
Kujirai, T., Caramia, M. D., Rothwell, J. C., Day, B. L., Thompson, P. D., Ferbert, A., et al. (1993). Corticocortical inhibition in human motor cortex. J. Physiol. 471, 501–519. doi: 10.1113/jphysiol.1993.sp019912
Lanza, G., Aricò, D., Lanuzza, B., Cosentino, F. I. I., Tripodi, M., Giardina, F., et al. (2020a). Facilitatory/inhibitory intracortical imbalance in REM sleep behavior disorder: Early electrophysiological marker of neurodegeneration? Sleep 43:zsz242. doi: 10.1093/sleep/zsz242
Lanza, G., Puglisi, V., Vinciguerra, L., Fisicaro, F., Vagli, C., Cantone, M., et al. (2020b). TMS correlates of pyramidal tract signs and clinical motor status in patients with cervical spondylotic myelopathy. Brain Sci. 10:806. doi: 10.3390/brainsci10110806
Lanza, G., Bella, R., Cantone, M., Pennisi, G., Ferri, R., and Pennisi, M. (2018a). Cognitive impairment and celiac disease: Is transcranial magnetic stimulation a trait d’Union between gut and brain? Int. J. Mol. Sci. 19:2243. doi: 10.3390/ijms19082243
Lanza, G., Cantone, M., Aricò, D., Lanuzza, B., Cosentino, F. I. I., Paci, D., et al. (2018b). Clinical and electrophysiological impact of repetitive low-frequency transcranial magnetic stimulation on the sensory–motor network in patients with restless legs syndrome. Ther. Adv. Neurol. Disord. 11:175628641875997. doi: 10.1177/1756286418759973
Lanza, G., Lanuzza, B., Aricò, D., Cantone, M., Cosentino, F. I. I., Bella, R., et al. (2018c). Impaired short-term plasticity in restless legs syndrome: A pilot rTMS study. Sleep Med. 46, 1–4. doi: 10.1016/j.sleep.2018.02.008
Lanza, G., Bella, R., Giuffrida, S., Cantone, M., Pennisi, G., Spampinato, C., et al. (2013). Preserved transcallosal inhibition to transcranial magnetic stimulation in nondemented elderly patients with leukoaraiosis. BioMed Res. Int. 2013:351680. doi: 10.1155/2013/351680
Lanza, G., Cantone, M., Puglisi, V., Vinciguerra, L., Fisicaro, F., Vagli, C., et al. (2019a). “Mute” plantar response: Does the cortico-spinal tract “speak”? Brain Stimulat. 12, 1579–1580. doi: 10.1016/j.brs.2019.07.008
Lanza, G., Casabona, J. A., Bellomo, M., Cantone, M., Fisicaro, F., Bella, R., et al. (2019b). Update on intensive motor training in spinocerebellar ataxia: Time to move a step forward? J. Int. Med. Res 48:0300060519854626. doi: 10.1177/0300060519854626
Lanza, G., Cosentino, F. I. I., Lanuzza, B., Tripodi, M., Aricò, D., Figorilli, M., et al. (2022a). Reduced intracortical facilitation to TMS in both isolated REM sleep behavior disorder (RBD) and early Parkinson’s disease with RBD. J. Clin. Med. 11:2291. doi: 10.3390/jcm11092291
Lanza, G., DelRosso, L. M., and Ferri, R. (2022b). Sleep and homeostatic control of plasticity. Handb. Clin. Neurol. 184, 53–72. doi: 10.1016/B978-0-12-819410-2.00004-7
Lanza, G., Fisicaro, F., D’Agate, C. C., Ferri, R., Cantone, M., Falzone, L., et al. (2021). Preserved central cholinergic functioning to transcranial magnetic stimulation in de novo patients with celiac disease. PLoS One 16:e0261373. doi: 10.1371/journal.pone.0261373
Lanza, G., Lanuzza, B., Aricò, D., Cantone, M., Cosentino, F. I. I., Pennisi, M., et al. (2015). Direct comparison of cortical excitability to transcranial magnetic stimulation in obstructive sleep apnea syndrome and restless legs syndrome. Sleep Med. 16, 138–142. doi: 10.1016/j.sleep.2014.08.016
Lebwohl, B., Luchsinger, J. A., Freedberg, D. E., Green, P. H. R., and Ludvigsson, J. F. (2016). Risk of dementia in patients with celiac disease: A population-based cohort study. J. Alzheimers Dis. JAD 49, 179–185. doi: 10.3233/JAD-150388
Lefaucheur, J.-P. (2019). Transcranial magnetic stimulation. Handb. Clin. Neurol. 160, 559–580. doi: 10.1016/B978-0-444-64032-1.00037-0
Lefaucheur, J.-P., Aleman, A., Baeken, C., Benninger, D. H., Brunelin, J., Di Lazzaro, V., et al. (2020). Evidence-based guidelines on the therapeutic use of repetitive transcranial magnetic stimulation (rTMS): An update (2014–2018). Clin. Neurophysiol. 131, 474–528. doi: 10.1016/j.clinph.2019.11.002
Li, C.-T., Huang, Y.-Z., Bai, Y.-M., Tsai, S.-J., Su, T.-P., and Cheng, C.-M. (2019). Critical role of glutamatergic and GABAergic neurotransmission in the central mechanisms of theta-burst stimulation. Hum. Brain Mapp. 40, 2001–2009. doi: 10.1002/hbm.24485
Li, S., Zhou, H., Yu, Y., Lyu, H., Mou, T., Shi, G., et al. (2021). Effect of repetitive transcranial magnetic stimulation on the cognitive impairment induced by sleep deprivation: A randomized trial. Sleep Med. 77, 270–278. doi: 10.1016/j.sleep.2020.06.019
Lo, Y. L., and Ratnagopal, P. (2007). Cortico-hypoglossal and corticospinal conduction abnormality in Bickerstaff’s brainstem encephalitis. Clin. Neurol. Neurosurg. 109, 523–525. doi: 10.1016/j.clineuro.2007.03.006
Lowenthal, D. T., Paran, E., Burgos, L., and Williams, L. S. (2007). General characteristics of treatable, reversible, and untreatable dementias. Am. J. Geriatr. Cardiol. 16, 136–142. doi: 10.1111/j.1076-7460.2007.06396.x
Luber, B., Stanford, A. D., Bulow, P., Nguyen, T., Rakitin, B. C., Habeck, C., et al. (2008). Remediation of sleep-deprivation–induced working memory impairment with fMRI-guided transcranial magnetic stimulation. Cereb. Cortex N. Y. NY 18, 2077–2085. doi: 10.1093/cercor/bhm231
Luber, B., Steffener, J., Tucker, A., Habeck, C., Peterchev, A. V., Deng, Z.-D., et al. (2013). Extended remediation of sleep deprived-induced working memory deficits using fMRI-guided transcranial magnetic stimulation. Sleep 36, 857–871. doi: 10.5665/sleep.2712
Mahowald, M. W., and Schenck, C. H. (2018). The “when” and “where” of α-synucleinopathies: Insights from REM sleep behavior disorder. Neurology 91, 435–436. doi: 10.1212/WNL.0000000000006129
Manganelli, F., Dubbioso, R., Iodice, R., Topa, A., Dardis, A., Russo, C. V., et al. (2014). Central cholinergic dysfunction in the adult form of Niemann Pick disease type C: A further link with Alzheimer’s disease? J. Neurol. 261, 804–808. doi: 10.1007/s00415-014-7282-2
Mansueto, G., Lanza, G., Fisicaro, F., Alaouieh, D., Hong, E., Girolami, S., et al. (2022). Central and peripheral nervous system complications of vasculitis syndromes from pathology to bedside: Part 1—central nervous system. Curr. Neurol. Neurosci. Rep 22, 47–69. doi: 10.1007/s11910-022-01172-z
Manto, M., Dalmau, J., Didelot, A., Rogemond, V., and Honnorat, J. (2011). Afferent facilitation of corticomotor responses is increased by IgGs of patients with NMDA-receptor antibodies. J. Neurol. 258, 27–33. doi: 10.1007/s00415-010-5674-5
Martinez-Cancino, D. P., Azpiroz-Leehan, J., Jimenez-Angeles, L., Garcia-Quintanar, A., and Santana-Miranda, R. (2016). Effects of high frequency rTMS on sleep deprivation: A pilot study. Conf. Proc. Annu. Int. Conf. IEEE Eng. Med. Biol. Soc. IEEE Eng. Med. Biol. Soc. Annu. Conf. 2016, 5937–5940. doi: 10.1109/EMBC.2016.7592080
Martorana, A., Mori, F., Esposito, Z., Kusayanagi, H., Monteleone, F., Codecà, C., et al. (2009). Dopamine modulates cholinergic cortical excitability in Alzheimer’s disease patients. Neuropsychopharmacol. Off. Publ. Am. Coll. Neuropsychopharmacol. 34, 2323–2328. doi: 10.1038/npp.2009.60
Matsumoto, H., and Ugawa, Y. (2020). Quadripulse stimulation (QPS). Exp. Brain Res. 238, 1619–1625. doi: 10.1007/s00221-020-05788-w
McDonald, C., Newton, J., Lai, H. M., Baker, S. N., and Jones, D. E. (2010). Central nervous system dysfunction in primary biliary cirrhosis and its relationship to symptoms. J. Hepatol. 53, 1095–1100. doi: 10.1016/j.jhep.2010.05.036
Meyer, B. U., Britton, T. C., Bischoff, C., Machetanz, J., Benecke, R., and Conrad, B. (1991). Abnormal conduction in corticospinal pathways in Wilson’s disease: Investigation of nine cases with magnetic brain stimulation. Mov. Disord. Off. J. Mov. Disord. Soc. 6, 320–323. doi: 10.1002/mds.870060409
Mignarri, A., Rossi, S., Ballerini, M., Gallus, G. N., Del Puppo, M., Galluzzi, P., et al. (2011). Clinical relevance and neurophysiological correlates of spasticity in cerebrotendinous xanthomatosis. J. Neurol. 258, 783–790. doi: 10.1007/s00415-010-5829-4
Misra, U. K., and Kalita, J. (1998). Neurophysiological studies in herpes simplex encephalitis. Electromyogr. Clin. Neurophysiol. 38, 177–182.
Misra, U. K., Kalita, J., and Das, A. (2003). Vitamin B12 deficiency neurological syndromes: A clinical. MRI and electrodiagnostic study. Electromyogr. Clin. Neurophysiol. 43, 57–64.
Moher, D., Liberati, A., Tetzlaff, J., and Altman, D. G. (2009). Preferred reporting items for systematic reviews and meta-analyses: The PRISMA statement. PLoS Med 6:e1000097. doi: 10.1371/journal.pmed.1000097
Mori, F., Kusayanagi, H., Buttari, F., Centini, B., Monteleone, F., Nicoletti, C. G., et al. (2013). Early treatment with high-dose interferon beta-1a reverses cognitive and cortical plasticity deficits in multiple sclerosis. Funct. Neurol. 27, 163–168.
Mori, F., Rossi, S., Sancesario, G., Codecà, C., Mataluni, G., Monteleone, F., et al. (2011). Cognitive and cortical plasticity deficits correlate with altered amyloid-β CSF levels in multiple sclerosis. Neuropsychopharmacology 36, 559–568. doi: 10.1038/npp.2010.187
Nantes, J. C., Zhong, J., Holmes, S. A., Whatley, B., Narayanan, S., Lapierre, Y., et al. (2016). Intracortical inhibition abnormality during the remission phase of multiple sclerosis is related to upper limb dexterity and lesions. Clin. Neurophysiol. Off. J. Int. Fed. Clin. Neurophysiol. 127, 1503–1511. doi: 10.1016/j.clinph.2015.08.011
Nardone, R., Bergmann, J., Brigo, F., Christova, M., Kunz, A., Seidl, M., et al. (2013). Functional evaluation of central cholinergic circuits in patients with Parkinson’s disease and REM sleep behavior disorder: A TMS study. J. Neural Transm. Vienna Austria 1996, 413–422. doi: 10.1007/s00702-012-0888-6
Nardone, R., De Blasi, P., Höller, Y., Brigo, F., Golaszewski, S., Frey, V. N., et al. (2016b). Intracortical inhibitory and excitatory circuits in subjects with minimal hepatic encephalopathy: A TMS study. Metab. Brain Dis. 31, 1065–1070. doi: 10.1007/s11011-016-9848-4
Nardone, R., Bergmann, J., Brigo, F., Höller, Y., Schwenker, K., Florea, C., et al. (2016a). Cortical afferent inhibition reflects cognitive impairment in obstructive sleep apnea syndrome: A TMS study. Sleep Med. 24, 51–56. doi: 10.1016/j.sleep.2016.08.003
Nardone, R., Bergmann, J., Kronbichler, M., Caleri, F., Lochner, P., Tezzon, F., et al. (2010b). Altered motor cortex excitability to magnetic stimulation in alcohol withdrawal syndrome. Alcohol. Clin. Exp. Res. 34, 628–632. doi: 10.1111/j.1530-0277.2009.01131.x
Nardone, R., Bergmann, J., De Blasi, P., Kronbichler, M., Kraus, J., Caleri, F., et al. (2010a). Cholinergic dysfunction and amnesia in patients with Wernicke-Korsakoff syndrome: A transcranial magnetic stimulation study. J. Neural Transm. Vienna Austria 1996, 385–391. doi: 10.1007/s00702-009-0347-1
Nardone, R., Bergmann, J., Kunz, A., Christova, M., Brigo, F., Tezzon, F., et al. (2012). Cortical afferent inhibition is reduced in patients with idiopathic REM sleep behavior disorder and cognitive impairment: A TMS study. Sleep Med. 13, 919–925. doi: 10.1016/j.sleep.2012.03.009
Nardone, R., Golaszewski, S., Schwenker, K., Brigo, F., Maccarrone, M., Versace, V., et al. (2019a). Cholinergic transmission is impaired in patients with idiopathic normal-pressure hydrocephalus: A TMS study. J. Neural Transm. Vienna Austria 1996, 1073–1080. doi: 10.1007/s00702-019-02036-6
Nardone, R., Langthaler, P. B., Orioli, A., Versace, V., Scarano, G. I., Brigo, F., et al. (2019b). Ipsilateral motor evoked potentials in a patient with unihemispheric cortical atrophy due to Rasmussen encephalitis. Neural Regen. Res. 14, 1025–1028. doi: 10.4103/1673-5374.250581
Nardone, R., Venturi, A., Buffone, E., Covi, M., Florio, I., Lochner, P., et al. (2006). Transcranial magnetic stimulation shows impaired transcallosal inhibition in Marchiafava-Bignami syndrome. Eur. J. Neurol. 13, 749–753. doi: 10.1111/j.1468-1331.2006.01302.x
Nolano, M., Guardascione, M. A., Amitrano, L., Perretti, A., Fiorillo, F., Ascione, A., et al. (1997). Cortico-spinal pathways and inhibitory mechanisms in hepatic encephalopathy. Electroencephalogr. Clin. Neurophysiol. 105, 72–78. doi: 10.1016/s0924-980x(96)96571-6
Oishi, M., and Mochizuki, Y. (1999). Frontal lobe atrophy and central motor conduction time in chronic alcoholics. Clin. EEG Electroencephalogr. 30, 76–78. doi: 10.1177/155005949903000209
Ortelli, P., Ferrazzoli, D., Sebastianelli, L., Engl, M., Romanello, R., Nardone, R., et al. (2021). Neuropsychological and neurophysiological correlates of fatigue in post-acute patients with neurological manifestations of COVID-19: Insights into a challenging symptom. J. Neurol. Sci. 420:117271. doi: 10.1016/j.jns.2020.117271
Ozata, M., Ozkardes, A., Dolu, H., Corakçi, A., Yardim, M., and Gundogan, M. A. (1996). Evaluation of central motor conduction in hypothyroid and hyperthyroid patients. J. Endocrinol. Invest. 19, 670–677. doi: 10.1007/BF03349037
Pennisi, G., Lanza, G., Giuffrida, S., Vinciguerra, L., Puglisi, V., Cantone, M., et al. (2014). Excitability of the motor cortex in de novo patients with celiac disease. PLoS One 9:e102790. doi: 10.1371/journal.pone.0102790
Pennisi, M., Lanza, G., Cantone, M., D’Amico, E., Fisicaro, F., Puglisi, V., et al. (2020). Acetyl-L-Carnitine in dementia and other cognitive disorders: A critical update. Nutrients 12:1389. doi: 10.3390/nu12051389
Pennisi, M., Lanza, G., Cantone, M., Ricceri, R., Ferri, R., D’Agate, C. C., et al. (2017). Cortical involvement in celiac disease before and after long-term gluten-free diet: A Transcranial Magnetic Stimulation study. PLoS One 12:e0177560. doi: 10.1371/journal.pone.0177560
Polachini, C. R. N., Spanevello, R. M., Schetinger, M. R. C., and Morsch, V. M. (2018). Cholinergic and purinergic systems: A key to multiple sclerosis? J. Neurol. Sci. 392, 8–21. doi: 10.1016/j.jns.2018.06.020
Pomeraniec, I. J., Bond, A. E., Lopes, M. B., and Jane, J. A. (2016). Concurrent Alzheimer’s pathology in patients with clinical normal pressure hydrocephalus: Correlation of high-volume lumbar puncture results, cortical brain biopsies, and outcomes. J. Neurosurg. 124, 382–388. doi: 10.3171/2015.2.JNS142318
Pope, P. A., and Miall, R. C. (2014). Restoring cognitive functions using non-invasive brain stimulation techniques in patients with cerebellar disorders. Front. Psychiatry 5:33. doi: 10.3389/fpsyt.2014.00033
Qiao, J., Jin, G., Lei, L., Wang, L., Du, Y., and Wang, X. (2016). The positive effects of high-frequency right dorsolateral prefrontal cortex repetitive transcranial magnetic stimulation on memory, correlated with increases in brain metabolites detected by proton magnetic resonance spectroscopy in recently detoxified alcohol-dependent patients. Neuropsychiatr. Dis. Treat. 12, 2273–2278. doi: 10.2147/NDT.S106266
Ranieri, F., Mariotto, S., Dubbioso, R., and Di Lazzaro, V. (2020). Brain stimulation as a therapeutic tool in amyotrophic lateral sclerosis: Current status and interaction with mechanisms of altered cortical excitability. Front. Neurol. 11:605335. doi: 10.3389/fneur.2020.605335
Ravaglia, S., Costa, A., Ratti, M. T., Savoldi, F., Bo, P., and Moglia, A. (2002). Cognitive impairment and central motor conduction time in chronic alcoholics. Funct. Neurol. 17, 83–86.
Reeves, B. C., Karimy, J. K., Kundishora, A. J., Mestre, H., Cerci, H. M., Matouk, C., et al. (2020). Glymphatic System Impairment in Alzheimer’s Disease and Idiopathic Normal Pressure Hydrocephalus. Trends Mol. Med. 26, 285–295. doi: 10.1016/j.molmed.2019.11.008
Restivo, D. A., Giuffrida, S., Rapisarda, G., Antonuzzo, A., Saponara, R., Reggio, A., et al. (2000). Central motor conduction to lower limb after transcranial magnetic stimulation in spinocerebellar ataxia type 2 (SCA2). Clin. Neurophysiol. Off. J. Int. Fed. Clin. Neurophysiol. 111, 630–635. doi: 10.1016/s1388-2457(99)00305-3
Restivo, D. A., Lanza, S., Saponara, R., Rapisarda, G., Giuffrida, S., and Palmeri, A. (2002). Changes of cortical excitability of human motor cortex in spinocerebellar ataxia type 2. A study with paired transcranial magnetic stimulation. J. Neurol. Sci. 198, 87–92. doi: 10.1016/s0022-510x(02)00086-2
Rizzo, V., Crupi, D., Bagnato, S., Quartarone, A., Benvenga, S., Bartolone, L., et al. (2008). Neural response to transcranial magnetic stimulation in adult hypothyroidism and effect of replacement treatment. J. Neurol. Sci. 266, 38–43. doi: 10.1016/j.jns.2007.08.031
Rogić Vidaković, M., Šoda, J., Jerković, A., Benzon, B., Bakraè, K., Dužević, S., et al. (2020). Obstructive sleep apnea syndrome: A preliminary navigated transcranial magnetic stimulation study. Nat. Sci. Sleep 12, 563–574. doi: 10.2147/NSS.S253281
Rossini, P. M., and Rossi, S. (2007). Transcranial magnetic stimulation: Diagnostic, therapeutic, and research potential. Neurology 68, 484–488. doi: 10.1212/01.wnl.0000250268.13789.b2
Rossini, P. M., Burke, D., Chen, R., Cohen, L. G., Daskalakis, Z., Di Iorio, R., et al. (2015). Non-invasive electrical and magnetic stimulation of the brain, spinal cord, roots and peripheral nerves: Basic principles and procedures for routine clinical and research application. An updated report from an I.F.C.N. Committee. Clin. Neurophysiol. Off. J. Int. Fed. Clin. Neurophysiol. 126, 1071–1107. doi: 10.1016/j.clinph.2015.02.001
Rossini, P. M., Rossi, S., Babiloni, C., and Polich, J. (2007). Clinical neurophysiology of aging brain: From normal aging to neurodegeneration. Prog. Neurobiol. 83, 375–400. doi: 10.1016/j.pneurobio.2007.07.010
Rothwell, J. (2018). Transcranial brain stimulation: Past and future. Brain Neurosci. Adv. 2:2398212818818070. doi: 10.1177/2398212818818070
Ruiu, E., Dubbioso, R., Madsen, K. H., Svolgaard, O., Raffin, E., Andersen, K. W., et al. (2020). Probing context-dependent modulations of ipsilateral premotor-motor connectivity in relapsing-remitting multiple sclerosis. Front. Neurol. 11:193. doi: 10.3389/fneur.2020.00193
Sailer, A., Molnar, G. F., Paradiso, G., Gunraj, C. A., Lang, A. E., and Chen, R. (2003). Short and long latency afferent inhibition in Parkinson’s disease. Brain J. Neurol. 126, 1883–1894. doi: 10.1093/brain/awg183
Siebner, H. R., Dressnandt, J., Auer, C., and Conrad, B. (1998). Continuous intrathecal baclofen infusions induced a marked increase of the transcranially evoked silent period in a patient with generalized dystonia. Muscle Nerve 21, 1209–1212. doi: 10.1002/(SICI)1097-4598(199809)21:9
Simpson, M., and Macdonell, R. (2015). The use of transcranial magnetic stimulation in diagnosis, prognostication and treatment evaluation in multiple sclerosis. Mult. Scler. Relat. Disord. 4, 430–436. doi: 10.1016/j.msard.2015.06.014
Sobów, T., Wojtera, M., and Kłoszewska, I. (2007). Potentially reversible dementias in a memory clinic population. Arch. Psychiatry Psychother. 3, 19–24.
Sosvorova, L., Kanceva, R., Vcelak, J., Kancheva, L., Mohapl, M., Starka, L., et al. (2015). The comparison of selected cerebrospinal fluid and serum cytokine levels in patients with multiple sclerosis and normal pressure hydrocephalus. Neuro Endocrinol. Lett. 36, 564–571.
Stezin, A., Kamble, N., Jhunjhunwala, K., Prasad, S., and Pal, P. K. (2019). Clinical utility of longitudinal measurement of motor threshold in Wilson’s disease. Can. J. Neurol. Sci. J. Can. Sci. Neurol. 46, 251–254. doi: 10.1017/cjn.2018.379
Terranova, C., Rizzo, V., Calamuneri, A., Bartolone, L., Morgante, F., Bruschetta, D., et al. (2016). Cortical excitability in patients with resistance to thyroid hormone compared to patients with hypothyroidism and euthyroid controls: A transcranial magnetic stimulation study. Arch. Ital. Biol. 154, 68–77. doi: 10.12871/00039829201624
Tokimura, H., Di Lazzaro, V., Tokimura, Y., Oliviero, A., Profice, P., Insola, A., et al. (2000). Short latency inhibition of human hand motor cortex by somatosensory input from the hand. J. Physiol. 523, 503–513. doi: 10.1111/j.1469-7793.2000.t01-1-00503.x
Tokimura, R., Matsuda, N., Kobayashi, S., Kimura, A., and Kanai, K. (2020). Abnormal evoked potentials in autoimmune glial fibrillary acidic protein astrocytopathy. eNeurologicalSci 18:100229. doi: 10.1016/j.ensci.2020.100229
Tozza, S., Dubbioso, R., Iodice, R., Topa, A., Esposito, M., Ruggiero, L., et al. (2018). Long-term therapy with miglustat and cognitive decline in the adult form of Niemann-Pick disease type C: A case report. Neurol. Sci. Off. J. Ital. Neurol. Soc. Ital. Soc. Clin. Neurophysiol. 39, 1015–1019. doi: 10.1007/s10072-018-3314-5
Turco, C. V., El-Sayes, J., Locke, M. B., Chen, R., Baker, S., and Nelson, A. J. (2018). Effects of lorazepam and baclofen on short- and long-latency afferent inhibition. J. Physiol. 596, 5267–5280. doi: 10.1113/JP276710
Ugawa, Y., Day, B. L., Rothwell, J. C., Thompson, P. D., Merton, P. A., and Marsden, C. D. (1991). Modulation of motor cortical excitability by electrical stimulation over the cerebellum in man. J. Physiol. 441, 57–72.
Ugawa, Y., Genba-Shimizu, K., Rothwell, J. C., Iwata, M., and Kanazawa, I. (1994a). Suppression of motor cortical excitability by electrical stimulation over the cerebellum in ataxia. Ann. Neurol. 36, 90–96. doi: 10.1002/ana.410360117
Ugawa, Y., Hanajima, R., and Kanazawa, I. (1994b). Motor cortex inhibition in patients with ataxia. Electroencephalogr. Clin. Neurophysiol. 93, 225–229. doi: 10.1016/0168-5597(94)90044-2
Ugawa, Y., Terao, Y., Hanajima, R., Sakai, K., Furubayashi, T., Machii, K., et al. (1997). Magnetic stimulation over the cerebellum in patients with ataxia. Electroencephalogr. Clin. Neurophysiol. 104, 453–458. doi: 10.1016/s0168-5597(97)00051-8
Velázquez-Pérez, L., Rodríguez-Labrada, R., Torres-Vega, R., Ortega-Sánchez, R., Medrano-Montero, J., González-Piña, R., et al. (2018). Progression of corticospinal tract dysfunction in pre-ataxic spinocerebellar ataxia type 2: A two-years follow-up TMS study. Clin. Neurophysiol. Off. J. Int. Fed. Clin. Neurophysiol. 129, 895–900. doi: 10.1016/j.clinph.2018.01.066
Velázquez-Pérez, L., Tünnerhoff, J., Rodríguez-Labrada, R., Torres-Vega, R., Ruiz-Gonzalez, Y., Belardinelli, P., et al. (2017). Early corticospinal tract damage in prodromal SCA2 revealed by EEG-EMG and EMG-EMG coherence. Clin. Neurophysiol. Off. J. Int. Fed. Clin. Neurophysiol. 128, 2493–2502. doi: 10.1016/j.clinph.2017.10.009
Versace, V., Sebastianelli, L., Ferrazzoli, D., Romanello, R., Ortelli, P., Saltuari, L., et al. (2021). Intracortical GABAergic dysfunction in patients with fatigue and dysexecutive syndrome after COVID-19. Clin. Neurophysiol. Off. J. Int. Fed. Clin. Neurophysiol. 132, 1138–1143. doi: 10.1016/j.clinph.2021.03.001
Volz, M. S., Finke, C., Harms, L., Jurek, B., Paul, F., Flöel, A., et al. (2016). Altered paired associative stimulation-induced plasticity in NMDAR encephalitis. Ann. Clin. Transl. Neurol. 3, 101–113. doi: 10.1002/acn3.277
Wang, Z., Zhang, Y., Hu, F., Ding, J., and Wang, X. (2020). Pathogenesis and pathophysiology of idiopathic normal pressure hydrocephalus. CNS Neurosci. Ther 26, 1230–1240. doi: 10.1111/cns.13526
Werhahn, K. J., Kunesch, E., Noachtar, S., Benecke, R., and Classen, J. (1999). Differential effects on motorcortical inhibition induced by blockade of GABA uptake in humans. J. Physiol. 517, 591–597. doi: 10.1111/j.1469-7793.1999.0591t.x
Wilson, M. T., Fulcher, B. D., Fung, P. K., Robinson, P. A., Fornito, A., and Rogasch, N. C. (2018). Biophysical modeling of neural plasticity induced by transcranial magnetic stimulation. Clin. Neurophysiol. Off. J. Int. Fed. Clin. Neurophysiol. 129, 1230–1241. doi: 10.1016/j.clinph.2018.03.018
Winner, B., Uyanik, G., Gross, C., Lange, M., Schulte-Mattler, W., Schuierer, G., et al. (2004). Clinical progression and genetic analysis in hereditary spastic paraplegia with thin corpus callosum in spastic gait gene 11 (SPG11). Arch. Neurol. 61, 117–121. doi: 10.1001/archneur.61.1.117
Wu, T., and Chu, N. S. (1996). Recovery patterns of motor and somatosensory evoked potentials following treatment of vitamin B12 deficiency. J. Formos. Med. Assoc. Taiwan Yi Zhi 95, 157–161.
Yger, P., and Gilson, M. (2015). Models of metaplasticity: A review of concepts. Front. Comput. Neurosci 9:138. doi: 10.3389/fncom.2015.00138
Yousuf, R. M., Fauzi, A. R. M., Wai, K. T., Amran, M., Akter, S., and Ramli, M. (2010). Potentially reversible causes of dementia. Public Health 2:9.
Yperman, J., Becker, T., Valkenborg, D., Hellings, N., Cambron, M., Dive, D., et al. (2020a). Deciphering the morphology of motor evoked potentials. Front. Neuroinformatics 14:28. doi: 10.3389/fninf.2020.00028
Yperman, J., Becker, T., Valkenborg, D., Popescu, V., Hellings, N., Wijmeersch, B. V., et al. (2020b). Machine learning analysis of motor evoked potential time series to predict disability progression in multiple sclerosis. BMC Neurol. 20:105. doi: 10.1186/s12883-020-01672-w
Zaaroor, M., Bleich, N., Chistyakov, A., Pratt, H., and Feinsod, M. (1997). Motor evoked potentials in the preoperative and postoperative assessment of normal pressure hydrocephalus. J. Neurol. Neurosurg. Psychiatry 62, 517–521. doi: 10.1136/jnnp.62.5.517
Ziemann, U. (2004). TMS and drugs. Clin. Neurophysiol. 115, 1717–1729. doi: 10.1016/j.clinph.2004.03.006
Ziemann, U., Chen, R., Cohen, L. G., and Hallett, M. (1998a). Dextromethorphan decreases the excitability of the human motor cortex. Neurology 51, 1320–1324. doi: 10.1212/wnl.51.5.1320
Ziemann, U., Reis, J., Schwenkreis, P., Rosanova, M., Strafella, A., Badawy, R., et al. (2015). TMS and drugs revisited 2014. Clin. Neurophysiol. Off. J. Int. Fed. Clin. Neurophysiol. 126, 1847–1868. doi: 10.1016/j.clinph.2014.08.028
Ziemann, U., Rothwell, J. C., and Ridding, M. C. (1996). Interaction between intracortical inhibition and facilitation in human motor cortex. J. Physiol. 496, 873–881. doi: 10.1113/jphysiol.1996.sp021734
Ziemann, U., Tergau, F., Wischer, S., Hildebrandt, J., and Paulus, W. (1998b). Pharmacological control of facilitatory I-wave interaction in the human motor cortex. A paired transcranial magnetic stimulation study. Electroencephalogr. Clin. Neurophysiol. 109, 321–330. doi: 10.1016/s0924-980x(98)00023-x
Keywords: transcranial magnetic stimulation, dementia, cortical excitability, cognitive impairment, electrophysiology, neuromodulation
Citation: Lanza G, Fisicaro F, Dubbioso R, Ranieri F, Chistyakov AV, Cantone M, Pennisi M, Grasso AA, Bella R and Di Lazzaro V (2022) A comprehensive review of transcranial magnetic stimulation in secondary dementia. Front. Aging Neurosci. 14:995000. doi: 10.3389/fnagi.2022.995000
Received: 15 July 2022; Accepted: 29 August 2022;
Published: 26 September 2022.
Edited by:
Davide Vito Moretti, Association Fatebenefratelli for Research, ItalyReviewed by:
Antonio Suppa, Sapienza University of Rome, ItalyMassimiliano Mirabella, Catholic University of the Sacred Heart Rome, Italy
Copyright © 2022 Lanza, Fisicaro, Dubbioso, Ranieri, Chistyakov, Cantone, Pennisi, Grasso, Bella and Di Lazzaro. This is an open-access article distributed under the terms of the Creative Commons Attribution License (CC BY). The use, distribution or reproduction in other forums is permitted, provided the original author(s) and the copyright owner(s) are credited and that the original publication in this journal is cited, in accordance with accepted academic practice. No use, distribution or reproduction is permitted which does not comply with these terms.
*Correspondence: Giuseppe Lanza, Z2l1c2VwcGUubGFuemExQHVuaWN0Lml0