- 1Department of Neurology, Xiangya Hospital, Central South University, Changsha, China
- 2National Clinical Research Center for Geriatric Disorders, Xiangya Hospital, Central South University, Changsha, China
- 3Department of Gastroenterology, Nanfang Hospital, Southern Medical University, Guangzhou, Guangdong, China
Objectives: Physical activity (PA) is considered beneficial in slowing the progression and improving the neurodegenerative disease prognosis. However, the association between PA and neurodegenerative diseases remains unknown. In this study, we conducted a two-sample Mendelian randomization (MR) analysis to estimate the causal association between PA phenotypes and neurodegenerative diseases.
Materials and methods: Genetic variants robustly associated with PA phenotypes, used as instrumental variables, were extracted from public genome-wide association study (GWAS) summary statistics. Neurodegenerative diseases, including amyotrophic lateral sclerosis (ALS), Parkinson’s disease (PD), and Alzheimer’s disease (AD), were considered outcomes. GWAS information was also obtained from the most recent large population study of individuals with European ancestry. Multiple MR methods, pleiotropy tests and sensitivity analyses were performed to obtain a robust and valid estimation.
Results: We found a positive association between moderate-to-vigorous physical activities and ALS based on the inverse variance weighted MR analysis method (OR: 2.507, 95% CI: 1.218–5.160, p = 0.013). The pleiotropy test and sensitivity analysis confirmed the robustness and validity of these MR results. No causal effects of PA phenotypes were found on PD and AD.
Conclusion: Our study indicates a causal effect of PA on the risk of neurodegenerative diseases. Genetically predicted increases in self-reported moderate-to-vigorous PA participation could increase the risk of ALS in individuals of European ancestry. Precise and individualized prescriptions of physical activity should be provided to the elderly population.
Introduction
With the aging of the population, the incidence of neurodegenerative diseases is increasing. Neuronal deterioration and degeneration lead to a plethora of clinical neurological deficits, including motor dysfunction and cognitive decline (Kiernan et al., 2011; Knopman et al., 2021). The most common age-related neurodegenerative diseases include amyotrophic lateral sclerosis (ALS), Parkinson’s disease (PD), and Alzheimer’s disease (AD), all of which exert a vast burden on individual families and society as a whole (Macpherson et al., 2017). To date, there are no curative therapies for neurodegenerative diseases. However, some modifiable factors, including physical activity (PA) and dietary habits, have been considered beneficial in slowing disease progression and improving disease prognosis (Grazioli et al., 2017; Mak et al., 2017; Gubert et al., 2020). Targeting these modifiable factors could also be effective in alleviating symptoms and relieving the abovementioned burdens.
Physical activity has recently attracted neurologists’ attention due to the mounting evidence supporting its role in maintaining brain health (Stillman and Erickson, 2018; Di Liegro et al., 2019; Donofry et al., 2021). Moderate PA has been related to the regulation of neuroplasticity and cell death from molecular perspectives and cognitive and motor functions at the behavioral level (de Sousa Fernandes et al., 2020; Liang et al., 2021; Maugeri et al., 2021). PA can promote autophagy and modulate mitophagy (Almeida et al., 2018), which play an important role in the pathogenesis of neurodegenerative diseases. Therefore, PA is thought to be beneficial for individuals experiencing neurodegenerative diseases, and its protective and preventive effects have been repeatedly noticed (Karssemeijer et al., 2017; Jia et al., 2019; Fan et al., 2020; Mahalakshmi et al., 2020; Kouloutbani et al., 2022). Furthermore, it has been accepted that PA could affect the risk of developing neurodegenerative diseases (Hamer and Chida, 2009; Lian et al., 2019). However, most of the epidemiological findings were obtained from a single center or a small study population (Veldink et al., 2005; Feddermann-Demont et al., 2017). The findings are also not consistent, and thus the association between PA and neurodegenerative diseases remains controversial (Pupillo et al., 2014; Feddermann-Demont et al., 2017; Kivimaki et al., 2019). Hence, large-scale or multicenter investigations focusing on the relationship between PA and neurodegenerative diseases are still required to understand the therapeutic potential of PA.
Mendelian randomization (MR) is a burgeoning analytical approach for exploring the causal relationship between exposures and outcomes. Genetic variants robustly associated with the level of exposure are often used as instrumental variables (IVs) in MR to estimate these causative associations. Unlike traditional randomized controlled trials, MR can identify potential causative factors of diseases, provide more information on whether a particular factor is a cause or a result of a disease, and ascertain whether modifying a specific factor will provide benefits. MR has been widely used in neurological diseases and has discriminated many of their causative factors.
Therefore, we utilized the MR method to investigate and estimate the causal relationship between PA and neurodegenerative diseases in individuals with European ancestry and hypothesize that PA could reduce the risk of neurodegenerative diseases. In our study, a two-sample MR analysis was performed to detect the potential causal association between PA and the risk of neurodegenerative diseases (ALS, PD, and AD) by using genetic variants robustly associated with PA phenotypes, including self-reported and objective accelerometer-based PA levels obtained from published genome-wide association studies (GWASs).
Materials and methods
Genome-wide association study summary statistics for physical activities
Summary statistics for physical activities were obtained from a recently published GWAS of over 377,000 UK biobank participants (Klimentidis et al., 2018). This study identified significant genetic variants associated with PA engagement, which included self-reported and accelerometry-based levels of PA in daily and leisure-time exercise habits. For self-reported PAs, responses to a touchscreen questionnaire similar to the International Physical Activity Questionnaire (Craig et al., 2003) were collected and divided into two phenotypes, listed as self-reported moderate-to-vigorous physical activities (MVPA) and self-reported vigorous physical activities (VPA). For the accelerometry-based PAs, exercise data were recorded from a wrist-worn Axivity-AX3 accelerometer (Doherty et al., 2017). Two measurements derived from the portable device were examined, including the overall acceleration average (OAA) and fraction of accelerations > 425 milligravities (FAA); this cutoff value was determined in accordance with the metabolic equivalents of vigorous physical activities (Hildebrand et al., 2014; Klimentidis et al., 2018).
Genome-wide association study summary statistics for neurodegenerative diseases
The genome-wide association data for ALS were obtained from publicly available GWAS summary statistics, including 20,806 ALS patients and 59,804 control individuals of European ancestry (mainly from the United States, Italian, French, and Belgian) (Nicolas et al., 2018). Both familial and sporadic ALS were included in the GWAS analysis. All patients were diagnosed according to the El Escorial criteria, and all had an onset age older than 18 years. The most recent and largest GWAS of PD involving 482,730 European participants, conducted by the International Parkinson’s Disease Genomics Consortium, was used for the summary statistics for PD outcome. The study detected and reported more than 15 million SNPs in 33,674 PD patients and 449,056 controls (Nalls et al., 2019). We obtained GWAS summary data for AD from a GWAS meta-analysis in a total European population of 63,926 participants (21,982 AD patients and 41,944 cognitively normal controls) performed by the International Genomics of Alzheimer’s Project (Kunkle et al., 2019).
Instrumental variable selection
To screen out significant single-nucleotide polymorphisms (SNPs) associated with PA as valid IVs, a cutoff p-value of 5 × 10–8 with genome-wide significance was chosen. In addition, the threshold of r2 was set as 0.001 to minimize the confounding factors. Consequently, in the PA GWAS, nine genome-wide significant SNPs for MVPA (n = 377,234) and five significant SNPs for VPA (n = 261,055) were separately identified. Furthermore, we identified eight and two SNPs with genome-wide significance for OAA and FAA, respectively. Considering that there were only 2 genome-wide significant SNPs for FAA, a more relaxed cutoff the p-value of 5 × 10–7 was used, and eight SNPs were consequently selected (Choi et al., 2019; Zhuo et al., 2021). In addition, the threshold for minor allele frequency was set to 0.01. When no SNPs of the PA phenotypes were found in the outcome GWAS, they were replaced by their proxies, which were identified from LDlink.1 Furthermore, we removed SNPs associated with confounders and outcomes based on the PhenoScanner V2 database.2 As listed in Supplementary Table 1, SNPs that fulfilled the three core requirements were finally selected as IVs to assess the causal relationship between PA and neurodegenerative diseases.
Statistical analysis
Mendelian randomization has been widely used to explore the causal effect of exposure on outcome (Liao et al., 2022a). Genetic variants that could explain the actual level of exposure are often used as IVs. In MR analysis, as shown in Figure 1, three fundamental assumptions should be satisfied to ensure the validity of the IVs: (1) the chosen IVs should be significantly associated with exposure (PA); (2) the available IVs should not be associated with any confounding factors of the exposure-outcome relationship; and (3) the chosen IVs should not affect the outcome, except possibly via the exposure-outcome relationship. In detail, genetic variants robustly associated with the PA phenotypes (namely, the exposure) were used as IVs, and neurodegenerative diseases, including ALS, PD, and AD, were regarded as outcomes. Thus, a two-sample MR was adopted as the main statistical approach to investigate the causal associations between each IV-exposure and IV-outcome (ALS, PD, AD) pairing.
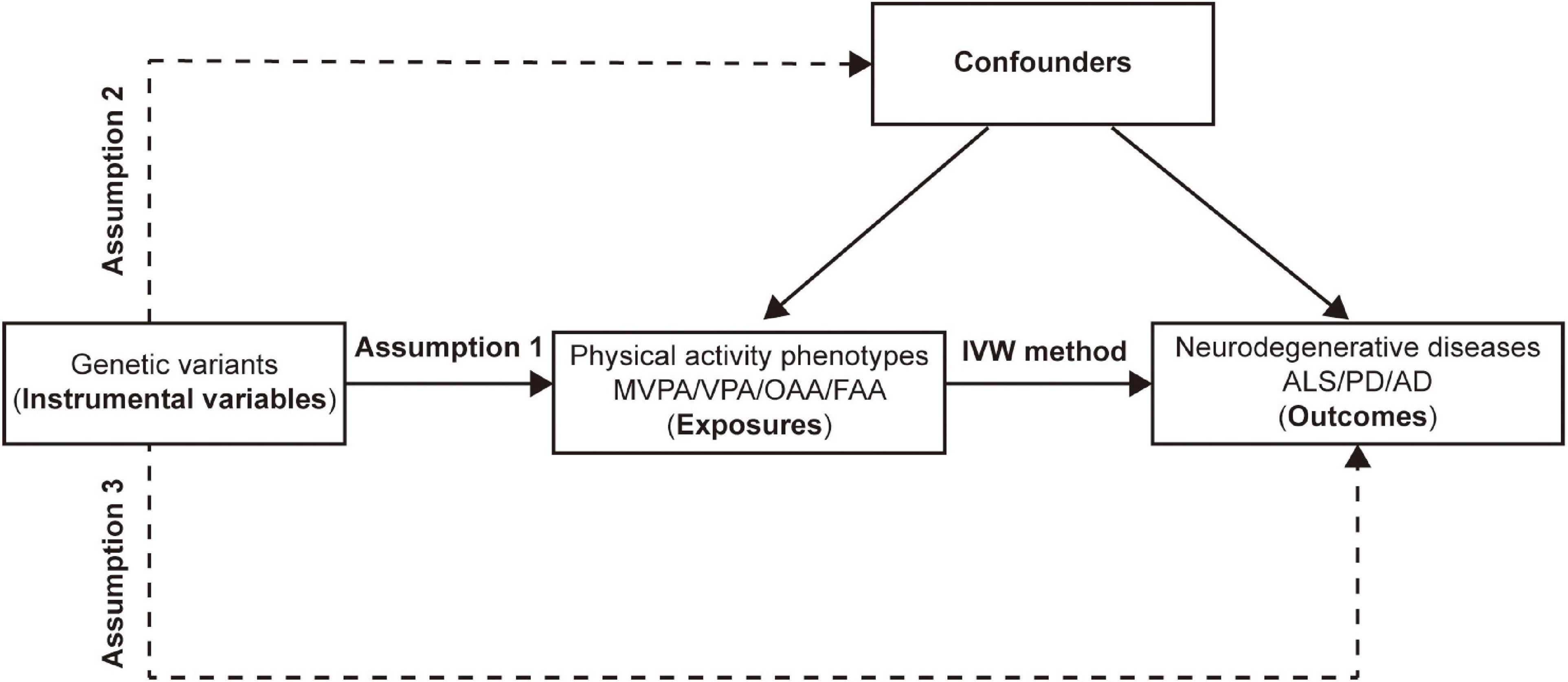
Figure 1. Assumptions and design of the two-sample mendelian randomization in this study. Genetic variants significantly associated with physical activity phenotypes are used as instrumental variables. MVPA, Self-reported moderate-to-vigorous physical activity; VPA, Self-reported vigorous physical activity; OAA, Overall acceleration average; FAA, Fraction of accelerations > 425 milli-gravities; IVW, Inverse variance weighted; ALS, amyotrophic lateral sclerosis; PD, Parkinson’s disease; AD, Alzheimer’s disease.
The inverse variance weighted (IVW) method has been widely used in MR analysis, developed as a meta-analysis of the variant-specific causal estimates and used to obtain a pooled estimation of the causal effect. The IVW method has certain advantages over other similar methods, especially when the IVs enrolled in the analysis were robustly valid. When there were weak IVs, the MR–Egger method could also be applied to obtain a less biased causal estimation. In addition, to minimize the influential impact of abnormal SNPs, the median-based method was performed as a supplement to the IVW method. Finally, the maximum likelihood method was utilized when the populations overlapped.
After MR analysis, sensitivity analysis, including heterogeneity and pleiotropy, was performed. Cochran’s Q statistic was calculated to measure heterogeneity which is widely used (Huang et al., 2021; Liao et al., 2022b). A large Q statistic value indicates that the effect of individual PA phenotype-associated SNPs on outcomes enjoys a relatively large difference, which suggests the existence of heterogeneity and that the findings should be cautiously interpreted. Moreover, a random-effect IVW method was implemented to assess the causal association between the PA phenotypes and outcomes. To explore the directional pleiotropy of the PA phenotype-associated SNPs, the MR–Egger regression test was adopted by comparing the deviation of the MR–Egger intercept from zero, and an intercept p-value of less than 0.05 suggested the presence of horizontal pleiotropy. Furthermore, Mendelian Randomization Pleiotropy RESidual Sum and Outlier (MR-PRESSO) analysis was also performed to identify any possible horizontal pleiotropy (Verbanck et al., 2018). Additionally, leave-one-out analysis was used to determine the potential PA phenotype-associated SNPs that might influence the causal effect and identify potential outliers.
R software (version 4.1.1, for Windows) was used to conduct our two-sample MR. We adopted three main packages for statistical analysis, data output and visualization: (1) ‘‘TwoSampleMR,’’3 (2) ‘‘MRPRESSO,’’4 and (3) ‘‘forestplot.’’5 A two-tailed p-value less than 0.05 was considered statistically significant.
Results
The summarized statistics of all selected SNPs of different PA phenotypes are listed in Supplementary Table 1. Based on the PhenoScanner V2 database, one of the nine MVPA related SNPs was associated with AD, so we omitted this SNP from the MR analysis of the MVPA-AD association.
Physical activity with amyotrophic lateral sclerosis
For the MVPA phenotype, no evidence of heterogeneity was present according to Cochran’s Q statistic (Q = 5.270, p = 0.384). As shown in Figures 2A, 3A, we found evidence of a positive association between MVPA and ALS based on the IVW method (OR: 2.507, 95% CI: 1.218–5.160, p = 0.013), indicating that a genetically determined increased MVPA engagement could increase the risk of ALS. The maximum likelihood method yielded a similar positive effect (OR: 1.669, 95% CI: 1.220–5.309, p = 0.013). In addition, the other MR results shared similar patterns of effect (Table 1), although the statistical significance was absent (MR–Egger OR: 3.433, 95% CI: 0.218–54.024, p = 0.421; simple median OR: 1.772, 95% CI: 0.631–4.974, p = 0.278; weighted median OR: 1.939, 95% CI: 0.709–5.300, p = 0.197). No directional pleiotropy was suggested by the MR–Egger intercept with an intercept value of −0.005 and p-value of 0.826 (Table 2). MR-PRESSO analysis also indicated that there was no horizontal pleiotropy (Table 2), so there were no outliers for any IVs (global test: p = 0.813). The leave-one-out test showed that the causal estimation of genetically predicted MVPA on ALS risk was mostly not influenced by a single SNP, implying the robustness and validity of our results. For the VPA phenotype, our results suggested a consistent trend of the causal estimation that VPA engagement could promote the onset of ALS across different statistical methods, although the p-values were not significant (Figure 2B and Table 1). No evidence of heterogeneity or pleiotropy was shown (shown in Table 2). For OAA, according to the maximum likelihood method, an inverse association between OAA and ALS risk was revealed (OR: 0.951, 95% CI: 0.907–0.997, p = 0.036). However, other MR methods yielded different trends (Table 2). For FAA, our MR analysis indicated no causal association between FAA and ALS risk by either the IVW method or other methods (shown in Table 1 and Figure 3A).
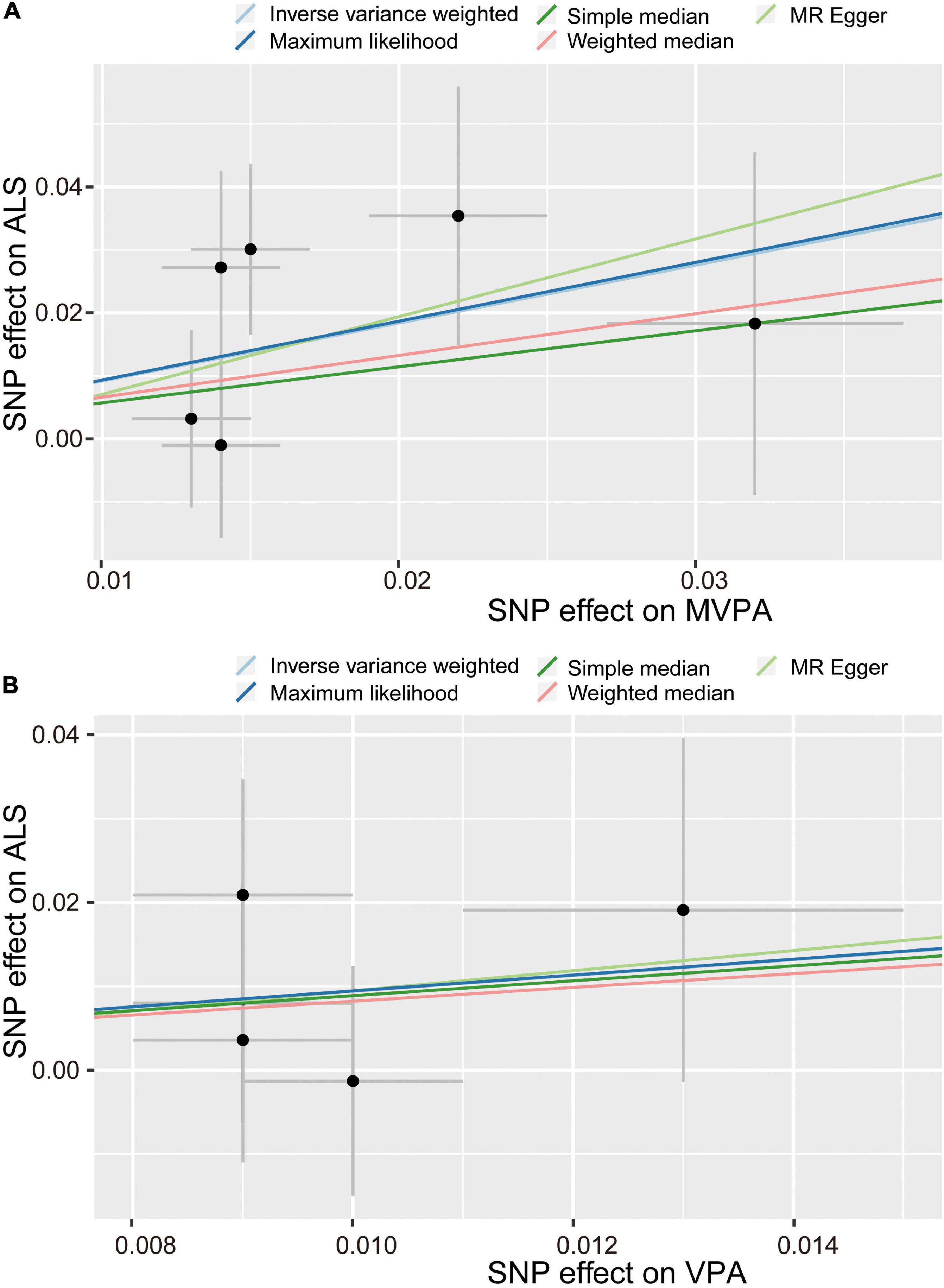
Figure 2. Scatter plot of causal effect of MVPA (A) and VPA (B) on ALS. MVPA, Self-reported moderate-to-vigorous physical activity; VPA, Self-reported vigorous physical activity; SNP, single nucleotide polymorphism; ALS, amyotrophic lateral sclerosis.
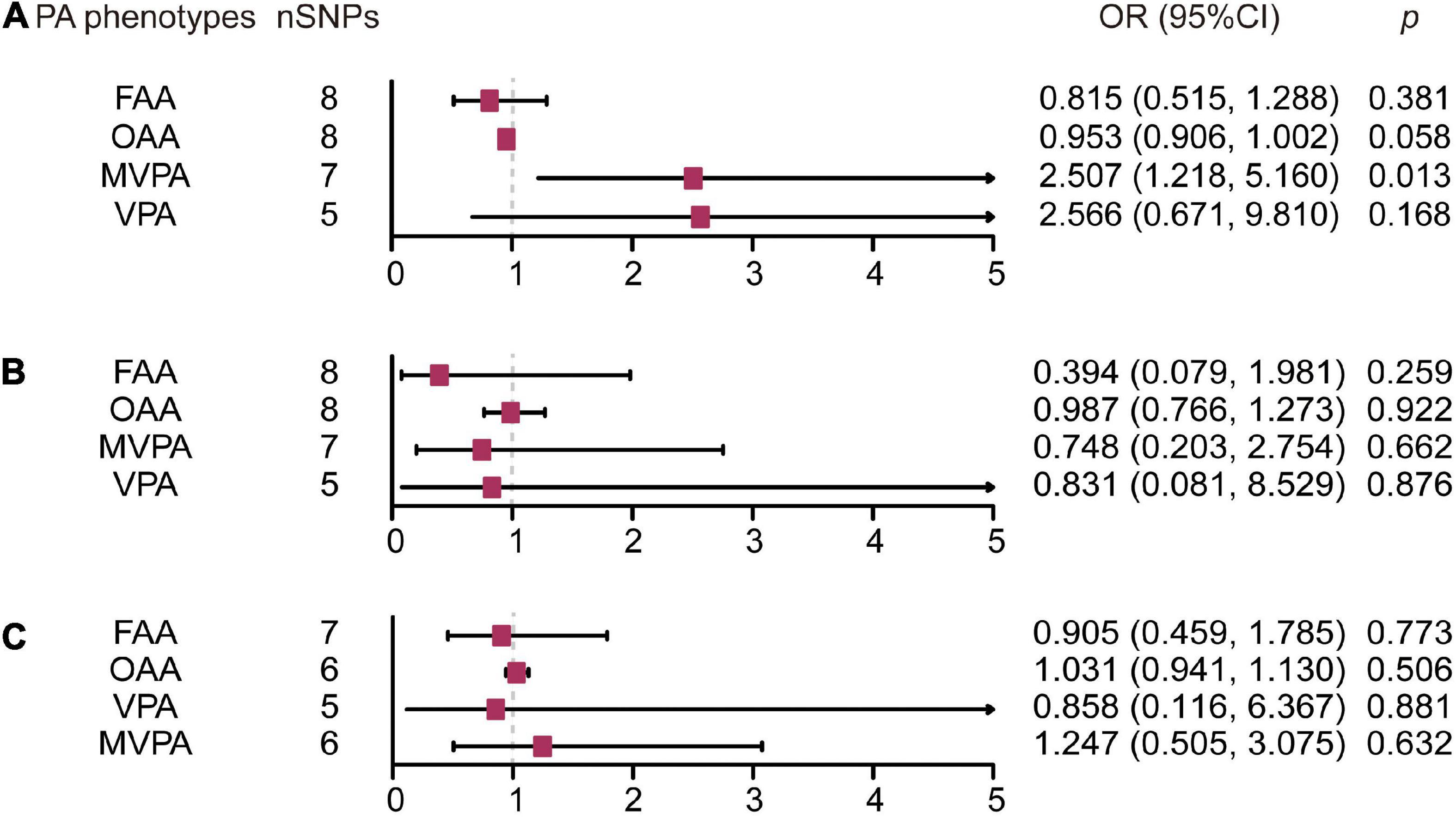
Figure 3. Forest plot of causal effect of physical activity phenotypes on amyotrophic lateral sclerosis (A), Parkinson’s disease (B), and Alzheimer’s disease (C) estimated by the inverse variance weighted method. PA, physical activity; SNP, single nucleotide polymorphism; MVPA, Self-reported moderate-to-vigorous physical activity; VPA, Self-reported vigorous physical activity; OAA, Overall acceleration average; FAA, Fraction of accelerations > 425 milli-gravities; OR, odds ratio; CI, confidence interval.
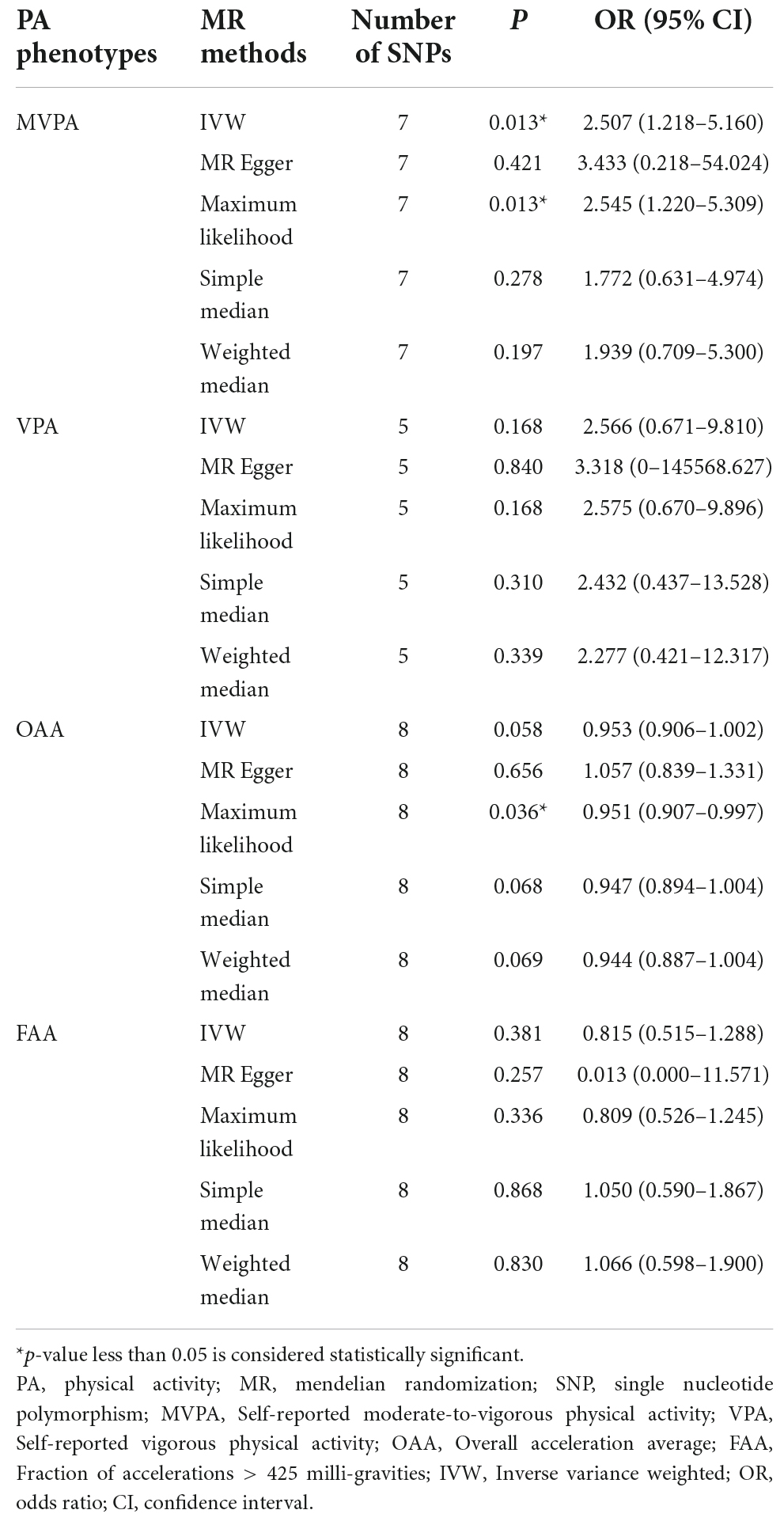
Table 1. Estimated association between physical activity phenotypes and amyotrophic lateral sclerosis.
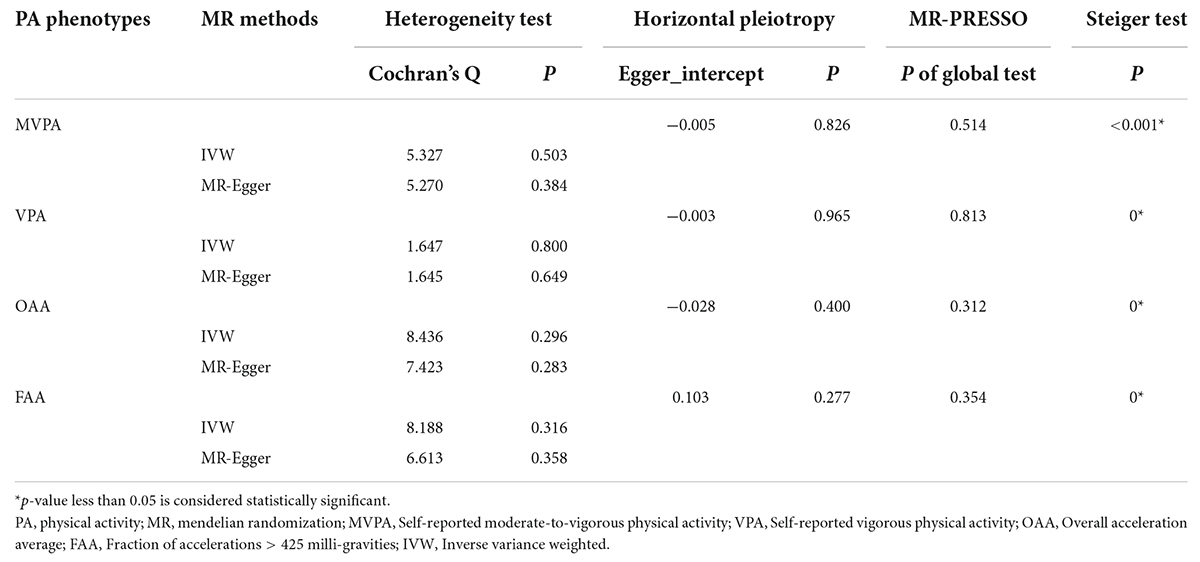
Table 2. Sensitivity test of the mendelian randomization analysis between physical activity phenotypes and amyotrophic lateral sclerosis.
Physical activity with Parkinson’s disease
For the self-reported PA phenotype, including MVPA and VPA, shown in Supplementary Table 4, there was no evidence of heterogeneity as measured by the Q statistics (MVPA Q = 8.211, p = 0.145; VPA Q = 6.558, p = 0.087). Multiple MR methods, including the IVW method, showed that the trend of the effect of MVPA on PD risk was consistent that the risk of PD tended to decrease when the genetically determined MVPA engagement increased despite the association was non-significant (Supplementary Table 2 and Figure 3B). Similar findings were also observed in the causal effect estimation between VPA and PD. MR–Egger intercept and MR-PRESSO analyses found no evidence for any pleiotropy in the MVPA-PD and VPA-PD associations, indicating the stability of our analysis (Supplementary Table 4). For accelerometry-based PA, heterogeneity was detected in both the OAA and FAA phenotypes, as indicated by the large Q statistics (OAA Q = 89.134, p = 0.000; FAA Q = 33.208, p < 0.001). Thus, a random-effects model for the IVW method was utilized to investigate the causal relation between these two phenotypes and PD (Supplementary Table 2). Neither OAA nor FAA exerted a causal effect on the possibility of developing PD (OAA OR: 0.987, 95% CI 0.766–1.273, p = 0.922; FAA OR: 0.394, 95% CI: 0.079–1.981, p = 0.259).
Physical activity and Alzheimer’s disease
There was no evidence of heterogeneity in the MVPA-AD relationship (Q = 2.730, p = 0.604); thus, the IVW, MR–Egger, median-based and maximum likelihood methods were all used to evaluate the causation of MVPA on AD (Supplementary Table 4). Demonstrated in Supplementary Table 3 and Figure 3C, it was implied that there was no significant causal association, and the effect pattern varied for the different methods (IVW: OR: 1.247, 95% CI: 0.505–3.075, p = 0.632; MR Egger OR: 19.620, 95% CI: 0.96–401.027, p = 0.125; weighted median OR: 1.288, 95% CI: 0.430–3.853, p = 0.651; simple median OR: 0.995, 95% CI: 0.337–2.932; p = 0.992). As heterogeneity was detected in the VPA-AD, OAA-AD, and FAA-AD relationships, the random-effects IVW method was used to estimate whether these were causal relationships (Supplementary Table 3 and Figure 3C). The analysis showed VPA and FAA were both inversely associated with AD risk, although the association was not significant (VPA OR: 0.858, 95% CI: 0.116–6.367, p = 0.881; FAA OR: 0.905, 95% CI: 0.459–1.785, p = 0.773), while OAA was positively related to AD risk but also not significant (OAA OR: 1.031, 95% CI: 0.941–1.13, p = 0.506).
Discussion
In this study, we conducted two-sample MR to investigate the causal relationship between PA and neurodegenerative diseases. Although it has been previously reported that PA could reduce the risk of developing neurodegenerative diseases, our results demonstrated that different physical phenotypes exert different effect on neurodegenerative diseases and there was only a causal association between MVPA and ALS. It was revealed that genetically determined increased MVPA engagement could significantly increase the risk of ALS.
Amyotrophic lateral sclerosis is a rare and fatal neurodegenerative disease caused by degeneration of the motor neurons (Shi et al., 2022). The effect of PA on ALS continues to be controversial. Some studies have revealed that PA is not a risk factor for ALS and that increased PA does not increase susceptibility to ALS (Valenti et al., 2005; Pupillo et al., 2014; Feddermann-Demont et al., 2017). However, these studies included limited populations, the conclusions were not validated. Recently, some studies with a larger population size have shown opposite results. A multicenter case–control study suggested that a higher risk of ALS is associated with PA in leisure time and occupational activities after adjustment for confounders (Visser et al., 2018). Furthermore, a cross-sectional study also indicated that patients who reported at least moderate engagement in PA (three times a week) during early adulthood were more likely to be diagnosed with ALS than those who did not (Raymond et al., 2021). Our analysis also supports that more involvement in PA is associated with ALS risk.
Among the selected methods in our analysis, with the validity of the IVs and the absence of pleiotropy, the IVW method was the most efficient (Zhu, 2021). Specifically, in the analysis of MVPA, the causal relationship was significant in two different analytical approaches including the IVW and the maximum likelihood, and the direction of effect remained similar. However, in the analysis of OAA, the significant result was only yielded by the maximum likelihood methods and the tendency were not consistent by different methods which weakened the validity and robustness of the results. Thus, our results demonstrated that more MVPA engagement was casually related with ALS risk. It needs intensive investigation whether PA could alter the risk of ALS by interfering with some already known ALS etiologies. It has been reported that different intensities of PA could induce various glucose demands which leads to the alteration of the muscle metabolism and autophagy flux, and provide different effect in ALS mice (Desseille et al., 2017). Besides, different intensities of PA could also the alter the sizes of recruited motoneurons and modulate the neurotrophic signal in the neuromuscular junctions (Just-Borras et al., 2020). Anyway, the underlying molecular mechanisms between different PA phenotypes and ALS risk still require further exploration. Uncovering the relationship between PA and ALS will be beneficial in the development of more precise guidance for ALS patients.
In addition, we explored the effect of PA on PD. In contrast to the effect of PA on ALS, our results showed that PA was not causally associated with PD risk but genetically predicted increased engagement in MVPA and VPA were inclined to protect against PD. Previous study has found that there is no obvious difference in PA levels between PD and healthy controls (Amara et al., 2019), which is inconsistent with our results. Besides, higher PA levels are associated with slower PD progression (Amara et al., 2019). Various studies have proposed that physical activity interventions could be effective in alleviating the motor and non-motor symptoms of PD, and in improving multiple outcomes in people with PD (Morberg et al., 2014; Okun, 2017; Cheng and Su, 2020; Hidalgo-Agudo et al., 2020). Our results did not invalidate these former studies despite no causality was found between PA and PD risk. Besides, a future investigation of the causal relationship between PA and PD progression are needed. On the other hand, it should be noted that PA could directly interfere with the PD-related pathophysiological process (Fan et al., 2020). In a PD rat model, early moderate treadmill running practice mitigates the accumulation of alpha-synuclein, which contributes to PD pathogenesis and motor dysfunction, and maintains levels of tyrosine hydroxylase in the substantia nigra, which plays an essential role in the metabolism of dopamine (Almeida et al., 2018). Additionally, it is suggested in PD mice model that PA could induce neuroprotection against cell death by promoting enhanced autophagy and neuronal regeneration (Jang et al., 2018), and by regulating both mitochondrial function and neuroinflammation (Tuon et al., 2015). Therefore, disclosing the role of PA in PD risk and progression would be of great benefit and could promote the prescription of PA as an efficient treatment method for PD.
It was previously reported that PA could improve cognitive function (Anderson-Hanley et al., 2012; Holthoff et al., 2015; Palta et al., 2019; Zhou et al., 2022), but the relationship between PA and AD risk remains unclear. Our analysis showed that there was no causal effect of either self-reported PA or accelerometry-based PA levels on AD onset, and the extent of engagement in PA was not a risk factor for AD. Our analysis is consistent with study results derived from meta-analysis of 19 prospective observational cohort studies. It contains more than 400,000 participants with a follow-up of at least 10 years demonstrated that less PA was not associated with a higher risk of dementia and AD (Kivimaki et al., 2019). Besides, existing evidence supporting that PA could reduce the risk of AD or dementia is insufficient (Brasure et al., 2018).
It is worth noting that the effect of PA on ALS, PD, and AD varies, which means that the roles of PA in neurodegenerative disease might differ from each other, and that there is no one-size-fits-all PA prescription for neurodegenerative diseases. It is necessary for clinicians to offer individualized and precise PA guidance for individuals with neurodegenerative disease.
There are some major strengths in our study. First, we explored for the first time the causal effect of different phenotypes of PA on neurodegenerative diseases by two-sample MR analysis. Second, we adopted multiple MR methods, including inverse variance weighted analysis, the MR–Egger method, the median-based method and the maximum likelihood method, to robustly estimate causal relationships, MR–Egger regression and MR-PRESSON to detect pleiotropy, and leave-one-out as the sensitivity test to examine the validity of the results. Third, the random-effect IVW method was implemented to assess the causal association if heterogeneity was present.
However, our MR analysis has some limitations. First, since the GWAS databases of PA and neurodegenerative diseases were all derived from individuals of European ancestry, the universality of our results is limited. Further studies are required to demonstrate whether our results are consistent for other ancestries. Second, although we ruled out SNPs associated with confounders in the MR analysis, some other unknown confounders are present that might influence the causal estimation. Third, PA engagement is determined not only by genetic factors but also by other factors; thus, it should be noted that our results could only explain the causal association of PA with neurodegenerative diseases. Fourth, the included SNPs significantly associated with PA are limited, which could decrease the statistical validity of our findings while minimizing the confounding influence and pleiotropy. Lastly, as the information of ALS, PD and AD phenotypes including genetic mutations are insufficient, the causality of PA on different phenotypes are unable to valuate. Therefore, further investigations to identify more genetic loci associated with PA engagement are needed.
Conclusion
To our knowledge, our MR analysis is the first to indicate a causal effect of PA on the risk of neurodegenerative diseases. Genetically predicted increases in MVPA participation could increase the risk of ALS in individuals of European ancestry. Precise and individualized prescriptions of physical activity should be provided to the elder population.
Data availability statement
The original contributions presented in this study are included in the article/Supplementary material, further inquiries can be directed to the corresponding author.
Author contributions
KH conceived the project. QL and KH extracted data and performed the analysis. QL wrote the manuscript. All authors polished the manuscript and critically revised it for valuable intellectual content and approved the final manuscript.
Funding
This work was supported by the Science and Technology Innovation Program of Hunan Province, China (Grant No. 2021RC2023, KH), the China Postdoctoral Science Foundation (Grant No. 2021M703638, KH), and the National Natural Science Foundation of China (Grant No. 82201557, KH).
Conflict of interest
The authors declare that the research was conducted in the absence of any commercial or financial relationships that could be construed as a potential conflict of interest.
Publisher’s note
All claims expressed in this article are solely those of the authors and do not necessarily represent those of their affiliated organizations, or those of the publisher, the editors and the reviewers. Any product that may be evaluated in this article, or claim that may be made by its manufacturer, is not guaranteed or endorsed by the publisher.
Supplementary material
The Supplementary Material for this article can be found online at: https://www.frontiersin.org/articles/10.3389/fnagi.2022.991140/full#supplementary-material
Abbreviations
ALS, amyotrophic lateral sclerosis; PD, Parkinson’s disease; AD, Alzheimer’s disease; PA, physical activity; MR, mendelian randomization; SNP, single nucleotide polymorphism; MVPA, self-reported moderate-to-vigorous physical activity; VPA, self-reported vigorous physical activity; OAA, overall acceleration average; FAA, fraction of accelerations > 425 milli-gravities; IVW, inverse variance weighted; OR, odds ratio; CI, confidence interval.
Footnotes
- ^ https://ldlink.nci.nih.gov/
- ^ http://www.phenoscanner.medschl.cam.ac.uk/
- ^ https://github.com/MRCIEU/TwoSampleMR
- ^ https://github.com/rondolab/MR-PRESSO
- ^ https://cran.r-project.org/web/packages/forestplot/index.html
References
Almeida, M. F., Silva, C. M., Chaves, R. S., Lima, N. C. R., Almeida, R. S., Melo, K. P., et al. (2018). Effects of mild running on substantia nigra during early neurodegeneration. J. Sports Sci. 36, 1363–1370. doi: 10.1080/02640414.2017.1378494
Amara, A. W., Chahine, L., Seedorff, N., Caspell-Garcia, C. J., Coffey, C., Simuni, T., et al. (2019). Self-reported physical activity levels and clinical progression in early parkinson’s disease. Parkinsonism Relat. Disord. 61, 118–125. doi: 10.1016/j.parkreldis.2018.11.006
Anderson-Hanley, C., Arciero, P. J., Brickman, A. M., Nimon, J. P., Okuma, N., Westen, S. C., et al. (2012). Exergaming and older adult cognition: A cluster randomized clinical trial. Am. J. Prev. Med. 42, 109–119. doi: 10.1016/j.amepre.2011.10.016
Brasure, M., Desai, P., Davila, H., Nelson, V. A., Calvert, C., Jutkowitz, E., et al. (2018). Physical activity interventions in preventing cognitive decline and alzheimer-type dementia: A systematic review. Ann. Intern. Med. 168, 30–38. doi: 10.7326/M17-1528
Cheng, Y. C., and Su, C. H. (2020). Evidence supports PA prescription for parkinson’s disease: Motor symptoms and non-motor features: A scoping review. Int. J. Environ. Res. Public Health 17:2894. doi: 10.3390/ijerph17145024
Choi, K. W., Chen, C. Y., Stein, M. B., Klimentidis, Y. C., Wang, M. J., Koenen, K. C., et al. (2019). Assessment of bidirectional relationships between physical activity and depression among adults: A 2-sample mendelian randomization study. JAMA Psychiatry 76, 399–408. doi: 10.1001/jamapsychiatry.2018.4175
Craig, C. L., Marshall, A. L., Sjostrom, M., Bauman, A. E., Booth, M. L., Ainsworth, B. E., et al. (2003). International physical activity questionnaire: 12-country reliability and validity. Med. Sci. Sports Exerc. 35, 1381–1395. doi: 10.1249/01.MSS.0000078924.61453.FB
de Sousa Fernandes, M. S., Ordonio, T. F., Santos, G. C. J., Santos, L. E. R., Calazans, C. T., Gomes, D. A., et al. (2020). Effects of physical exercise on neuroplasticity and brain function: A systematic review in human and animal studies. Neural Plast. 2020:8856621. doi: 10.1155/2020/8856621
Desseille, C., Deforges, S., Biondi, O., Houdebine, L., D’amico, D., Lamaziere, A., et al. (2017). Specific physical exercise improves energetic metabolism in the skeletal muscle of amyotrophic-lateral- sclerosis mice. Front. Mol. Neurosci. 10:332. doi: 10.3389/fnmol.2017.00332
Di Liegro, C. M., Schiera, G., Proia, P., and Di Liegro, I. (2019). Physical activity and brain health. Genes 10:720. doi: 10.3390/genes10090720
Doherty, A., Jackson, D., Hammerla, N., Plotz, T., Olivier, P., Granat, M. H., et al. (2017). Large scale population assessment of physical activity using wrist worn accelerometers: The UK biobank study. PLoS One 12:e0169649. doi: 10.1371/journal.pone.0169649
Donofry, S. D., Stillman, C. M., Hanson, J. L., Sheridan, M., Sun, S., Loucks, E. B., et al. (2021). Promoting brain health through physical activity among adults exposed to early life adversity: Potential mechanisms and theoretical framework. Neurosci. Biobehav. Rev. 131, 688–703. doi: 10.1016/j.neubiorev.2021.09.051
Fan, B., Jabeen, R., Bo, B., Guo, C., Han, M., Zhang, H., et al. (2020). What and how can physical activity prevention function on parkinson’s disease? Oxid. Med. Cell. Longev. 2020:4293071. doi: 10.1155/2020/4293071
Feddermann-Demont, N., Junge, A., Weber, K. P., Weller, M., Dvorak, J., and Tarnutzer, A. A. (2017). Prevalence of potential sports-associated risk factors in Swiss amyotrophic lateral sclerosis patients. Brain Behav. 7:e00630. doi: 10.1002/brb3.630
Grazioli, E., Dimauro, I., Mercatelli, N., Wang, G., Pitsiladis, Y., Di Luigi, L., et al. (2017). Physical activity in the prevention of human diseases: Role of epigenetic modifications. BMC Genomics 18:802. doi: 10.1186/s12864-017-4193-5
Gubert, C., Kong, G., Renoir, T., and Hannan, A. J. (2020). Exercise, diet and stress as modulators of gut microbiota: Implications for neurodegenerative diseases. Neurobiol. Dis. 134:104621. doi: 10.1016/j.nbd.2019.104621
Hamer, M., and Chida, Y. (2009). Physical activity and risk of neurodegenerative disease: A systematic review of prospective evidence. Psychol. Med. 39, 3–11. doi: 10.1017/S0033291708003681
Hidalgo-Agudo, R. D., Lucena-Anton, D., Luque-Moreno, C., Heredia-Rizo, A. M., and Moral-Munoz, J. A. (2020). Additional physical interventions to conventional physical therapy in parkinson’s disease: A systematic review and meta-analysis of randomized clinical trials. J. Clin. Med. 9:1038. doi: 10.3390/jcm9041038
Hildebrand, M., Van Hees, V. T., Hansen, B. H., and Ekelund, U. (2014). Age group comparability of raw accelerometer output from wrist- and hip-worn monitors. Med. Sci. Sports Exerc. 46, 1816–1824. doi: 10.1249/MSS.0000000000000289
Holthoff, V. A., Marschner, K., Scharf, M., Steding, J., Meyer, S., Koch, R., et al. (2015). Effects of physical activity training in patients with alzheimer’s dementia: Results of a pilot RCT study. PLoS One 10:e0121478. doi: 10.1371/journal.pone.0121478
Huang, K., Bi, F. F., and Yang, H. (2021). A systematic review and meta-analysis of the prevalence of congenital myopathy. Front. Neurol. 12:761636. doi: 10.3389/fneur.2021.761636
Jang, Y. C., Hwang, D. J., Koo, J. H., Um, H. S., Lee, N. H., Yeom, D. C., et al. (2018). Association of exercise-induced autophagy upregulation and apoptosis suppression with neuroprotection against pharmacologically induced parkinson’s disease. J. Exerc. Nutr. Biochem. 22, 1–8. doi: 10.20463/jenb.2018.0001
Jia, R. X., Liang, J. H., Xu, Y., and Wang, Y. Q. (2019). Effects of physical activity and exercise on the cognitive function of patients with alzheimer disease: A meta-analysis. BMC Geriatr. 19:181. doi: 10.1186/s12877-019-1175-2
Just-Borras, L., Hurtado, E., Cilleros-Mane, V., Biondi, O., Charbonnier, F., Tomas, M., et al. (2020). Running and swimming prevent the deregulation of the BDNF/TrkB neurotrophic signalling at the neuromuscular junction in mice with amyotrophic lateral sclerosis. Cell. Mol. Life Sci. 77, 3027–3040. doi: 10.1007/s00018-019-03337-5
Karssemeijer, E. G. A., Aaronson, J. A., Bossers, W. J., Smits, T., Olde Rikkert, M. G. M., and Kessels, R. P. C. (2017). Positive effects of combined cognitive and physical exercise training on cognitive function in older adults with mild cognitive impairment or dementia: A meta-analysis. Ageing Res. Rev. 40, 75–83. doi: 10.1016/j.arr.2017.09.003
Kiernan, M. C., Vucic, S., Cheah, B. C., Turner, M. R., Eisen, A., Hardiman, O., et al. (2011). Amyotrophic lateral sclerosis. Lancet 377, 942–955.
Kivimaki, M., Singh-Manoux, A., Pentti, J., Sabia, S., Nyberg, S. T., Alfredsson, L., et al. (2019). Physical inactivity, cardiometabolic disease, and risk of dementia: An individual-participant meta-analysis. BMJ 365:l1495. doi: 10.1016/S0140-6736(10)61156-7
Klimentidis, Y. C., Raichlen, D. A., Bea, J., Garcia, D. O., Wineinger, N. E., Mandarino, L. J., et al. (2018). Genome-wide association study of habitual physical activity in over 377,000 UK biobank participants identifies multiple variants including CADM2 and APOE. Int. J. Obes. 42, 1161–1176. doi: 10.1038/s41366-018-0120-3
Knopman, D. S., Amieva, H., Petersen, R. C., Chetelat, G., Holtzman, D. M., Hyman, B. T., et al. (2021). Alzheimer disease. Nat. Rev. Dis. Primers 7:33. doi: 10.1038/s41572-021-00269-y
Kouloutbani, K., Venetsanou, F., Markati, A., Karteroliotis, K. E., and Politis, A. (2022). The effectiveness of physical exercise interventions in the management of neuropsychiatric symptoms in dementia patients: A systematic review. Int. Psychogeriatr. 34, 177–190. doi: 10.1017/S1041610221000193
Kunkle, B. W., Grenier-Boley, B., Sims, R., Bis, J. C., Damotte, V., Naj, A. C., et al. (2019). Genetic meta-analysis of diagnosed alzheimer’s disease identifies new risk loci and implicates Abeta, tau, immunity and lipid processing. Nat. Genet. 51, 414–430. doi: 10.1038/s41588-019-0358-2
Lian, L., Liu, M., Cui, L., Guan, Y., Liu, T., Cui, B., et al. (2019). Environmental risk factors and amyotrophic lateral sclerosis (ALS): A case-control study of ALS in China. J. Clin. Neurosci. 66, 12–18. doi: 10.1016/j.jocn.2019.05.036
Liang, J., Wang, H., Zeng, Y., Qu, Y., Liu, Q., Zhao, F., et al. (2021). Physical exercise promotes brain remodeling by regulating epigenetics, neuroplasticity and neurotrophins. Rev. Neurosci. 32, 615–629. doi: 10.1515/revneuro-2020-0099
Liao, Q., He, J., Tian, F. F., Bi, F. F., and Huang, K. (2022a). A causal relationship between leukocyte telomere length and multiple sclerosis: A mendelian randomization study. Front. Immunol. 13:922922. doi: 10.3389/fimmu.2022.922922
Liao, Q., Zhang, Y., He, J., and Huang, K. (2022b). Global prevalence of myotonic dystrophy: An updated systematic review and meta-analysis. Neuroepidemiology 56, 163–173. doi: 10.1159/000524734
Macpherson, H., Teo, W. P., Schneider, L. A., and Smith, A. E. (2017). A life-long approach to physical activity for brain health. Front. Aging Neurosci. 9:147. doi: 10.3389/fnagi.2017.00147
Mahalakshmi, B., Maurya, N., Lee, S. D., and Bharath Kumar, V. (2020). Possible neuroprotective mechanisms of physical exercise in neurodegeneration. Int. J. Mol. Sci. 21:5895. doi: 10.3390/ijms21165895
Mak, M. K., Wong-Yu, I. S., Shen, X., and Chung, C. L. (2017). Long-term effects of exercise and physical therapy in people with parkinson disease. Nat. Rev. Neurol. 13, 689–703. doi: 10.1038/nrneurol.2017.128
Maugeri, G., D’agata, V., Magri, B., Roggio, F., Castorina, A., Ravalli, S., et al. (2021). Neuroprotective effects of physical activity via the adaptation of astrocytes. Cells 10:1542. doi: 10.3390/cells10061542
Morberg, B. M., Jensen, J., Bode, M., and Wermuth, L. (2014). The impact of high intensity physical training on motor and non-motor symptoms in patients with parkinson’s disease (PIP): A preliminary study. NeuroRehabilitation 35, 291–298. doi: 10.3233/NRE-141119
Nalls, M. A., Blauwendraat, C., Vallerga, C. L., Heilbron, K., Bandres-Ciga, S., Chang, D., et al. (2019). Identification of novel risk loci, causal insights, and heritable risk for parkinson’s disease: A meta-analysis of genome-wide association studies. Lancet Neurol. 18, 1091–1102. doi: 10.1016/S1474-4422(19)30320-5
Nicolas, A., Kenna, K. P., Renton, A. E., Ticozzi, N., Faghri, F., Chia, R., et al. (2018). Genome-wide analyses identify KIF5A as a novel ALS gene. Neuron 97:1268–1283.e6. doi: 10.1016/j.neuron.2018.02.027
Okun, M. S. (2017). Management of parkinson disease in 2017: Personalized approaches for patient-specific needs. JAMA 318, 791–792. doi: 10.1001/jama.2017.7914
Palta, P., Sharrett, A. R., Deal, J. A., Evenson, K. R., Gabriel, K. P., Folsom, A. R., et al. (2019). Leisure-time physical activity sustained since midlife and preservation of cognitive function: The atherosclerosis risk in communities study. Alzheimers Dement. 15, 273–281. doi: 10.1016/j.jalz.2018.08.008
Pupillo, E., Messina, P., Giussani, G., Logroscino, G., Zoccolella, S., Chio, A., et al. (2014). Physical activity and amyotrophic lateral sclerosis: A European population-based case-control study. Ann. Neurol. 75, 708–716. doi: 10.1002/ana.24150
Raymond, J., Mehta, P., Larson, T., Factor-Litvak, P., Davis, B., and Horton, K. (2021). History of vigorous leisure-time physical activity and early onset amyotrophic lateral sclerosis (ALS), data from the national ALS registry: 2010-2018. Amyotroph. Lateral Scler. Frontotemporal Degener. 22, 535–544. doi: 10.1080/21678421.2021.1910308
Shi, G., Shao, S., Zhou, J., Huang, K., and Bi, F. F. (2022). Urinary p75(ECD) levels in patients with amyotrophic lateral sclerosis: A meta-analysis. Amyotroph. Lateral Scler. Frontotemporal Degener. 23, 438–445. doi: 10.1080/21678421.2021.1990345
Stillman, C. M., and Erickson, K. I. (2018). Physical activity as a model for health neuroscience. Ann. N. Y. Acad. Sci. 1428, 103–111. doi: 10.1111/nyas.13669
Tuon, T., Souza, P. S., Santos, M. F., Pereira, F. T., Pedroso, G. S., Luciano, T. F., et al. (2015). Physical training regulates mitochondrial parameters and neuroinflammatory mechanisms in an experimental model of parkinson’s disease. Oxid. Med. Cell. Longev. 2015:261809. doi: 10.1155/2015/261809
Valenti, M., Pontieri, F. E., Conti, F., Altobelli, E., Manzoni, T., and Frati, L. (2005). Amyotrophic lateral sclerosis and sports: A case-control study. Eur. J. Neurol. 12, 223–225. doi: 10.1111/j.1468-1331.2004.00978.x
Veldink, J. H., Kalmijn, S., Groeneveld, G. J., Titulaer, M. J., Wokke, J. H., and Van Den Berg, L. H. (2005). Physical activity and the association with sporadic ALS. Neurology 64, 241–245. doi: 10.1212/01.WNL.0000149513.82332.5C
Verbanck, M., Chen, C. Y., Neale, B., and Do, R. (2018). Detection of widespread horizontal pleiotropy in causal relationships inferred from mendelian randomization between complex traits and diseases. Nat. Genet. 50, 693–698. doi: 10.1038/s41588-018-0099-7
Visser, A. E., Rooney, J. P. K., D’ovidio, F., Westeneng, H. J., Vermeulen, R. C. H., Beghi, E., et al. (2018). Multicentre, cross-cultural, population-based, case-control study of physical activity as risk factor for amyotrophic lateral sclerosis. J. Neurol. Neurosurg. Psychiatry 89, 797–803. doi: 10.1136/jnnp-2017-317724
Zhou, S., Chen, S., Liu, X., Zhang, Y., Zhao, M., and Li, W. (2022). Physical activity improves cognition and activities of daily living in adults with alzheimer’s disease: A systematic review and meta-analysis of randomized controlled trials. Int. J. Environ. Res. Public Health 19:1216. doi: 10.3390/ijerph19031216
Zhu, X. (2021). Mendelian randomization and pleiotropy analysis. Quant. Biol. 9, 122–132. doi: 10.1007/s40484-020-0216-3
Keywords: physical activity, neurodegenerative diseases, amyotrophic lateral sclerosis, Parkinson’s disease, Alzheimer’s disease, Mendelian randomization
Citation: Liao Q, He J and Huang K (2022) Physical activities and risk of neurodegenerative diseases: A two-sample Mendelian randomization study. Front. Aging Neurosci. 14:991140. doi: 10.3389/fnagi.2022.991140
Received: 11 July 2022; Accepted: 08 September 2022;
Published: 23 September 2022.
Edited by:
Dongsheng Xu, Shanghai University of Traditional Chinese Medicine, ChinaReviewed by:
Yihong Ma, Kumamoto University, JapanRobert Newton, Pennington Biomedical Research Center, United States
Paul Arciero, Skidmore College, United States
Copyright © 2022 Liao, He and Huang. This is an open-access article distributed under the terms of the Creative Commons Attribution License (CC BY). The use, distribution or reproduction in other forums is permitted, provided the original author(s) and the copyright owner(s) are credited and that the original publication in this journal is cited, in accordance with accepted academic practice. No use, distribution or reproduction is permitted which does not comply with these terms.
*Correspondence: Kun Huang, aHVhbmdrbkBvdXRsb29rLmNvbQ==