- 1College of Kinesiology, Shenyang Sport University, Shenyang, China
- 2Department of Sport Rehabilitation, Xi’an University of Sport, Xi’an, China
- 3Department of Sport Rehabilitation, Shanghai University of Sport, Shanghai, China
- 4Department of Rehabilitation Medicine, Shanghai Shangti Orthopaedic Hospital, Shanghai, China
Osteoarthritis (OA) is a degenerative joint disease, the prevalence of OA is increasing, and the elderly are the most common in patients with OA. OA has a severe impact on the daily life of patients, this increases the demand for treatment of OA. In recent years, the application of non-invasive brain stimulation (NIBS) has attracted extensive attention. It has been confirmed that NIBS plays an important role in regulating cortical excitability and oscillatory rhythm in specific brain regions. In this review, we summarized the therapeutic effects and mechanisms of different NIBS techniques in OA, clarified the potential of NIBS as a treatment choice for OA, and provided prospects for further research in the future.
Introduction
As a general degenerative disease and the most common form of arthritis, osteoarthritis (OA) is pathologically characterized by cartilage degeneration, subchondral bone sclerosis, and osteophyte formation (Pereira et al., 2015). Cartilage degeneration is also a hallmark of OA (Egloff et al., 2012). The major clinical symptoms of OA are joint pain and activity disorders. The new subchondral bone tissue that results from subchondral bone sclerosis and osteophyte formation contains new blood vessels and nerve fibers, which may be related to OA pathogenesis and pain perception (Walsh et al., 2010). These in turn cause the patient’s dysfunction, poor sleep, and low mood; these conditions seriously affect the quality of life (QOL) of patients. In accordance with statistics, the prevalence rate of OA in people over 60 years old can reach 50%, it in people over 75 years old is as high as 80%, the prevalence in women is higher than that in men, and the disability rate of the disease can reach 53% (Zhang et al., 2020). Patients with OA worldwide are expected to exhibit an increasing trend in the next few decades (Mantovani et al., 2016) partly due to increasing risk factors for OA, such as genetics, obesity, lack of exercise, or exercise injury (Hawker, 2019; Kakouris et al., 2021). The disease not only exerts a huge influence on the QOL of patients but also considerably increases the economic burden of society. At present, however, the complex pathological mechanism of OA is not yet fully known; it mostly involves increased inflammatory components, mechanical overload, altered metabolic changes, and cellular aging (Jeon et al., 2017). In contrast with other inflammatory joint diseases, varying responses to different parts of OA further complicate treatment, and thus, treatment for OA remains a challenge (Hermann et al., 2018).
OA is currently mainly treated with drugs (Guo et al., 2022), mainly non-steroidal anti-infective drugs, which have anti-inflammatory and analgesic effects, but these drugs have many adverse reactions, and long-term use can lead to different degrees of cardiovascular complications (da Costa et al., 2017). Intra-articular injections such as glucocorticoids can reduce joint adhesion and promote cartilage repair, but they only have short-term effects, and long-term injections may cause infection (Kavanaugh et al., 2016). Cartilage protective drugs such as glucosamine can reduce joint edema, maintain synovial fluid viscosity, and inhibit inflammation, but long-term use has serious adverse reactions to gastrointestinal function and is expensive (Arden et al., 2021). Joint-targeted drug therapy only works in the diseased part and has no effect on other normal parts, which is conducive to cartilage repair and pain relief (Zhang et al., 2022). However, the current targeted therapy is still in the clinical trial stage, and the potential adverse reactions are not yet clear. Therefore, it is considered to explore a non-drug-safe treatment method for OA.
OA is also mostly treated with physical therapy, mainly exercise therapy, hydrotherapy, and extracorporeal shock wave therapy (Griffin et al., 2020). Anti-blocking exercise combined with hydrotherapy is prone to adverse events such as worsening pain (Waller et al., 2017) and other symptoms in patients with moderate to severe OA, and hydrotherapy has had only a small short-term clinical effect in patients with OA (Bartels et al., 2016) and does not appear to have a significant effect on the patient’s drug dose or quality of life (Forestier et al., 2016). Extracorporeal shock wave therapy (ESWT) is important for the protection of articular cartilage in patients with early OA, but it can only slow down the course of OA. Moreover, the experimental subjects are mostly mice, and the doses for humans have not been standardized (Chou et al., 2019). Whole-body vibration therapy also does not improve joint stiffness in patients with OA (Qiu et al., 2022).
Bioelectronic medicine uses the body’s electrical signals to improve the diagnosis and treatment of disease. As an emerging bioelectronic medical technology, Non-invasive brain stimulation (NIBS) has been widely used in clinical treatment, particularly for pain (Xiong et al., 2022), neuronal function regulation, brain function cognition, behavior, and other aspects of the evident treatment effect (Miniussi and Ruzzoli, 2013). NIBS not only brings hope for treating diseases that cannot be solved by common drugs and medical means, but also provides drug alternatives with rapid and precise targeting. This paper describes the therapeutic effects and possible mechanisms of six commonly used NIBS methods in OA, namely single pulse transcranial magnetic stimulation (TMS), repetitive transcranial magnetic stimulation (rTMS), transcranial direct current stimulation (tDCS), transcranial alternating current stimulation (tACS), transcranial random noise stimulation (tRNS), transcranial focused ultrasound stimulation (tFUS), to provide directions for future research.
Methods
A comprehensive literature search was conducted in PubMed and Web of Science databases using [Osteoarthritis(Title/Abstract)] OR [OA(Title/Abstract)], [Osteoarthritides(Title/Abstract)] OR [Osteoarthrosis(Title/Abstract)] OR [Osteoarthroses(Title/Abstract)] OR [Arthritis, Degenerative(Title/Abstract)] OR [Arthritides, Degenerative(Title/Abstract)] OR [Degenerative Arthritides(Title/Abstract)] OR [Degenerative Arthritis(Title/Abstract)] OR [Arthrosis(Title/Abstract)] OR [Arthroses(Title/Abstract)] OR [Osteoarthrosis Deformans(Title/Abstract)], [Non-invasive brain stimulation(Title/Abstract)] OR [NIBS(Title/Abstract)], rTMS[Title/Abstract] and Osteoarthritis, Single-pulse TMS[Title/Abstract] and Osteoarthritis, tDCS[Title/Abstract] and Osteoarthritis, tFUS[Title/Abstract] and Osteoarthritis, tACS[Title/Abstract] and Osteoarthritis, tRNS[Title/Abstract] and Osteoarthritis. From the date initially provided until November 2021, it is not limited to randomized controlled trials (RCT). Firstly, irrelevant articles were excluded according to the article titles or abstracts, and after reading and summarizing the remaining articles, the articles with high relevance, in-depth research, or lack of research were selected for inclusion.
Non-invasive brain stimulation
NIBS has become an effective and multi-field treatment technology in recent years. It is used to study the behavioral correlation of specific brain regions; neuronal regulatory function and its related perceptual, cognitive, and behavioral characteristics play a huge role (Plewnia et al., 2015), significantly deepening researchers’ understanding of NIBS. However, NIBS is more dependent on brain state and task content, and some variability can be observed even among or within individuals. And the long-term effects of NIBS have not been explored (Polanía et al., 2018). Recent research trends on NIBS are presented in Figures 1A,B.
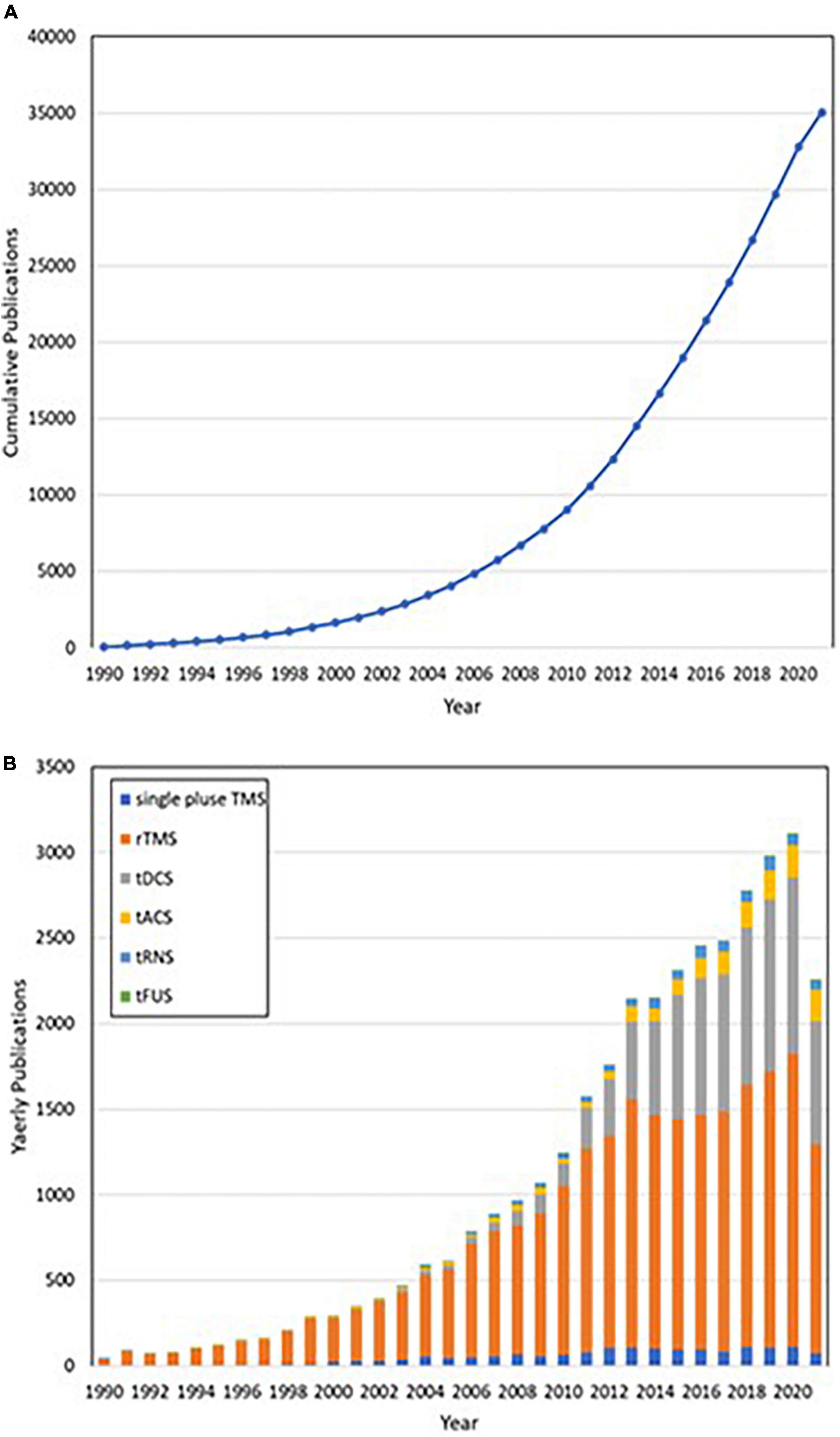
Figure 1. (A) Publications accumulated by year. (B) From 1990 to 2020, publications were classified annually in accordance with different non-invasive brain stimuli in categories that included repetitive transcranial magnetic stimulation (rTMS, orange), single-pulse transcranial magnetic stimulation (single-pulse TMS, blue), transcranial direct current stimulation (tDCS, gray), transcranial alternating current stimulation (tACS, yellow), transcranial random noise stimulation (tRNS, light blue), and transcranial focused ultrasound stimulation (tFUS, green).
Clinically, common NIBS technologies include TMS (Ansari et al., 2021) and transcranial electrical stimulation (tES) (Abhishek and Doherty, 2013; Terranova et al., 2018). Among TMS techniques, single-pulse and rTMS are more commonly used. Meanwhile, tES can be divided into tACS and tDCS. In addition, tFUS (Badran et al., 2020) and tRNS (Battaglini et al., 2020) are also commonly used methods of NIBS (O’Connell et al., 2018).
TMS is a late-model neurophysiological technique based on the theory of the principle of electromagnetic induction (Barker et al., 1985; Hallett, 2007). Its principle is to use the rapidly changing magnetic field that emerges when a high-voltage current passes through a coil, acting on a certain region of the cerebral cortex, or the corresponding skull surface; hence, the magnetic field produces an induction current, which, in turn, changes the neural cell excitability in that region (Klomjai et al., 2015). Such changes can be recorded on effectors through the neural pathway. TMS is divided into three categories: single pulse TMS, double pulse TMS, and rTMS (Auvichayapat and Auvichayapat, 2009; Ansari et al., 2021). There is also an emerging TMS model called Theta Burst Stimulation (TBS). rTMS is a new neuroelectrophysiological technology. rTMS not only affects the local function of the cerebral cortex, but relatively distant cortical function can also achieve cortical function reconstruction. High-frequency TMS can enhance the metabolism of nerve cells, while low-frequency TMS can inhibit nerve cell metabolism (Du et al., 2019). Thus rTMS can produce lasting changes in cortical excitability (Pell et al., 2011) and has become a new treatment for neurological disorders. However, rTMS remains in the preliminary exploration stage. Its treatment effect and optimal treatment parameters have not been scientifically confirmed, and its treatment mechanism also requires further research and discussion (D’Agati et al., 2010). Single-pulse TMS emits only one stimulation pulse at a time; such pulse can depolarize neurons and cause a measurable response (Mathew and Danion, 2018), primarily for the detection of neural pathways, i.e., motion-evoked potential or measurement of a motor threshold. Single-pulse magnetic stimulation can also be used to detect central conduction time, and resting time, accurately map the motor cortex, and measure the motor evoked potential amplitude related to motor cortical conduction (Zorn et al., 2012). However, the aforementioned studies, have no targeted disease or standardized parameters. Dual-pulse magnetic stimulation refers to the emission of two consecutive pulses or one pulse pair after each issued instruction; it can be used in the research on cortical excitability (de Goede et al., 2020). TBS is similar to the frequency of theta waves in the hippocampus of the brain and is a special type of rTMS. It uses short pulses in clumps, with an intra-frequency of 50 Hz and an inter-frequency of 5 Hz (Ferrarelli and Phillips, 2021), which has the advantages of short stimulation time, long duration of therapeutic effect, and closer to the physiological state of neurophysiological activity. Different TBS models have different effects on neuronal excitability. Intermittent TBS (iTBS) acts by continuous stimulation for 2 s with 8 s intervals, which can increase neuronal excitability. On the other hand, continuous TBS (cTBS) provides continuous stimulation at a frequency of 5 Hz, which reduces neuronal excitability (Bai et al., 2022). This article mainly describes two ways of treating OA, rTMS and Single-pulse TMS.
tDCS (Ahn et al., 2019a) is a pair of electrodes that use constant, low-intensity current (1–2 mA) on specific brain regions to regulate cortical neural activity (Cucca et al., 2019). It is widely used in the clinical treatment of Parkinsonism and other neurological diseases; it can also be used to improve motor, language, cognitive, and swallowing functions (Santos Ferreira et al., 2019). However, the exact mechanism of tDCS is not yet completely clear. Its clinical application remains in the incremental research phase, and a single mechanism cannot explain the multiple effects of tDCS; moreover, no unified scheme is available for the choice of stimulation intensity, time, and location of tDCS (Palm et al., 2016a). Currently, for tDCS, the widely recognized mechanism of action is the effect on membrane potential and ion channels, synaptic plasticity, cortical excitability (Nitsche and Paulus, 2000), bilateral hemispheric excitability, and regulation of local cortical and brain network connections (Lapenta et al., 2018).
tACS (Antal et al., 2008) is a special mode of NIBS that transmits sinusoidal alternating current electricity to the scalp, mostly affecting the excitability of cortical neurons (Bland and Sale, 2019). The currently recognized action mechanism of tACS is to regulate brain function by guiding brain oscillations (Antal et al., 2008) and inducing synaptic plasticity over a long period to regulate cognitive processes (Elyamany et al., 2021); it can also link cellular neuronal activity to brain network mechanisms to recover disturbed brain oscillations and perfect behavioral outcomes (Del Felice et al., 2019). tACS can be used as a practical means to judge the diagnosis, classification, and prognosis of mental diseases. tACS is relatively safe and non-invasive (Matsumoto and Ugawa, 2017); hence, it exhibits considerable potential in basic research and as a tool for clinical care. However, information on how tACS ultimately affects neural activity (Vieira et al., 2020) is minimal, and the two practical factors of tACS and electric field are difficult to focus on accurately, which is a common problem in tACS; thus, this technique should be further optimized and studied (Wu et al., 2021).
tRNS is a type of TMS, which exhibits the potential to induce improvements with a lasting perception when combined with assignments such as a contrast detection test (Terney et al., 2008). Nevertheless, the mechanism of these long-range improvements is not yet fully determined. tRNS can strengthen contrast sensitivity after one training cycle; however, this early onset also depends on the characteristics of the stimulus (Battaglini et al., 2020). tRNS can sense the effect of duration (Mioni et al., 2018). Interference with persistent neuronal oscillations can ultimately generate neuroplasticity effects if suitable parameters are applied (Paulus, 2011).
tFUS has a higher spatial resolution and can reach deeper organizations compared with other NIBS methods (di Biase et al., 2019). tFUS acting on the brain can modulate human cortical function (Legon et al., 2014). For example, two 10-min tFUS in the front of the thalamus will induce analgesic effects in healthy individuals (Badran et al., 2020). tFUS can also be used to regulate emotion regulatory networks in the prefrontal cortex (Goh et al., 2020; Sanguinetti et al., 2020). Low-intensity tFUS enhances the neuromodulatory effect of human autonomous motor-related cortical activity (Yu et al., 2021). Through an in-depth study, tFUS was found to be safe, and adverse reactions rarely occur. However, under high stimulation intensity or rate, tFUS may lead to bleeding, cell death or injury, and accidental opening of the blood–brain barrier. Thus, the study of tFUS is necessary to set up a good and secure framework for the application and promotion of clinical treatment of tFUS (Pasquinelli et al., 2019).
The six methods of NIBS are commonly used in clinical treatment, and they exhibit unique characteristics, including their respective sites of action, as indicated in Figure 2.
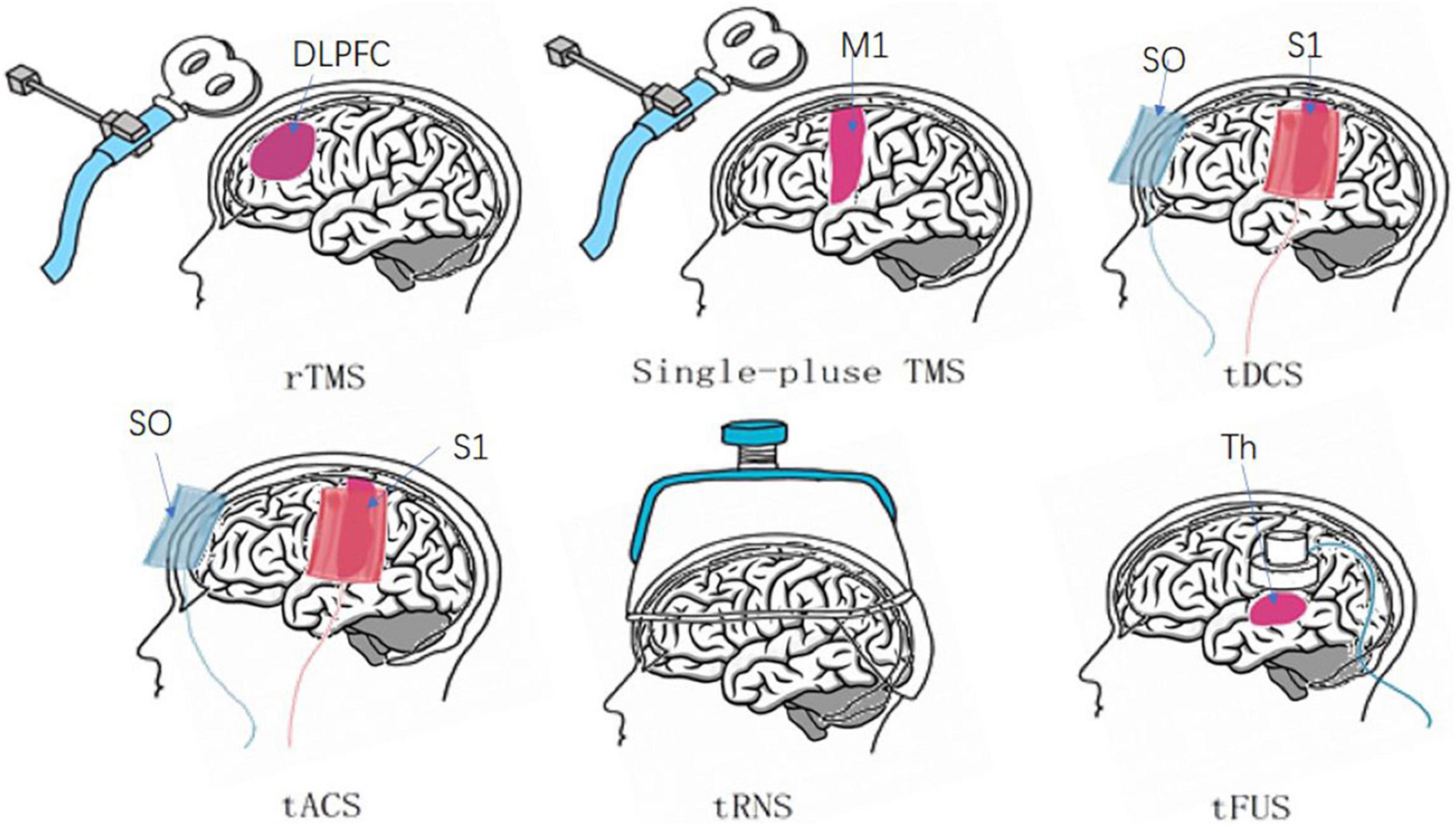
Figure 2. Six major non-invasive brain stimuli and their site of action. rTMS generally functions in the dorsolateral PFC (DLPFC) (Lefaucheur et al., 2020). Single-pulse TMS uses frequent sites of action, such as the primary motor cortex (M1) (Savoie et al., 2020). tDCS and tACS cathode and anode positions: contralateral orbit (SO), primary sensory cortex (S1) (Marlow et al., 2013; May et al., 2021). tRNS: cerebral cortex. tFUS: thalamus (Th) (Palm et al., 2016b; Badran et al., 2020).
Therapeutic effects of non-invasive brain stimulation on osteoarthritis
Relieve pain
Pain is a common symptom in patients with OA (Perrot, 2015), and with an increase in incidence in OA and the risk factors that affect OA, pain places heavy burdens on the daily life of patients with OA and society. The treatment of pain of patients with OA is particularly important. NIBS has been shown to exhibit a therapeutic function on the pain brought by OA in many studies. For example, Jean-Paul Nguyen et al. (2019) performed a monthly rTMS treatment of 10 Hz in the right motor cortex of an elderly female patient suffering from left knee OA. One week after the third treatment, the pain was significantly reduced, and after 1 year of follow-up, the effect of pain reduction continued. Ahn et al. (2017) performed a tDCS intervention of 2 mA per day in middle-aged and elderly patients with knee OA, placing the anode on the primary motor cortex (M1) and the cathode on the contralateral supraorbital (SO). After five interventions, the patient’s NRS scores decreased significantly and the Short-Form McGill Pain Questionnaire (SF-MPQ-2) scores also improved. The therapeutic effect of pain relief was found to be maintained after the 3-week follow-up. Ahn et al. (2018) also evaluated the pain sensitivity of 400 elderly patients with knee OA and conducted five tDCS interventions. Their results showed that the experimental pain sensitivity of the patients was reduced, and tDCS exerted a beneficial effect on the improvement of clinical pain. tFUS is frequently used in chronic neuropathic pain. However, targeted studies on the pain of OA are few. Research on tACS, Trns, and single-pulse TMS with regard to the pain of OA remains in its infancy and requires further exploration.
Alleviate symptoms of depression
Depression and other bad moods are closely related to OA. The occurrence of OA may aggravate depressive symptoms (Zheng et al., 2021), and the long-term accumulation of these symptoms may aggravate the pain grade and joint dysfunction of patients with OA (Rathbun et al., 2018, 2020). NIBS has been widely used in research related to depressive symptoms. Depression assessment, such as the Hamilton Depression Rating Scale, was used as a secondary evaluation index in the research of Nguyen et al. (2019) on the program of rTMS intervention in the treatment of OA. Patients with 20 years of OA showed a significant increase in their HAD scores after ten rTMS interventions. This suggests that rTMS is effective in treating depression in patients with OA. Ahn et al. (2019b) evaluated the efficacy of remotely supervised tDCS for the treatment of OA patients, elderly KOA patients did not experience significant improvement in anxiety and depression scores after 10 consecutive tDCS interventions. This may be the result of various factors, such as autonomously improper use of tDCS. Luz-Santos et al. (2017) and Tavares et al. (2018) also studied tDCS for depression in OA patients, although the results were not available. This requires further research by researchers. The other basic NIBS methods have minimal research on the depressive symptoms of OA, and more studies are still required to confirm their result.
Improve joint motor function
The articular cartilage, as an important basic structure of joints, is the major structure affected by OA, which can cause joint deformities, joint swelling, and a decrease in the range of motion (Abhishek and Doherty, 2013), resulting in limited motor function in patients with OA. In severe cases, joint replacement surgery should be considered. Drug therapy is mostly used in the early treatment of OA, but the efficacy of drug therapy is limited (Cao et al., 2022). The safety and non-invasive characteristics of NIBS make it indispensable in the auxiliary treatment of OA. In a randomized controlled trial of tDCS combined with conventional physical therapy on the functional ability of patients with OA, Fatemeh Rahimi et al. (2021) used the anode of tDCS to intervene in the left primary motor cortex (Ahn et al., 2017), primary sensory cortex (Ahn et al., 2018), and dorsolateral PFC. The subjects were evaluated functionally with the Keen Injury and OA Outcome Score rating scale, the range of motion of the knee joint, 30-s chair standing, and 4 × 10-m walking training before and after treatment. The results show that various functional indicators were significantly improved after tDCS intervention. The strength of the muscles around the joints is also an important factor affecting joint function. Quadriceps weakness is a typical symptom of KOA. Kittelson et al. (2014) performed TMS stimulation on subjects with this symptom. After stimulation, quadriceps torque was reduced, resting motion threshold was also decreased, and the Western Ontario and McMaster Universities OA Index (Luz-Santos et al., 2017) scores increased. All these findings suggested the improvement of the motor function of subjects. The effects of tACS, tRNS and other methods on joint motor function have not yet been confirmed.
Quality of life
As a common degenerative disease of the elderly, OA is becoming increasingly in many young individuals at present, and it is one of the major factors that affect the daily activities and QOL of patients (Sabashi et al., 2021). Studies have shown that (Paget et al., 2021) the QOL of young people is more affected by OA than that of older patients in ankle OA. In the research protocol of Tavares et al. (2018) on elderly KOA patients with defective endogenous pain-inhibitory systems, health-related QOL (HRQOL) was included in the evaluation indicators of OA subjects after tDCS intervention. In the subsequent trial (Tavares et al., 2021), 2 mA tDCS intervention at the stimulation site of M1 and SO for 20 min at 15 times in 3 weeks did not significantly improve the HRQOL and WOMAC scores, and did not exert an evident effect on the QOL of patients with OA. However, in patients with OA after total knee replacement surgery, the SF-36 score was significantly improved, and the QOL of the patients was also improved after 6 weeks of treatment with electroacupuncture and exercise therapy combined with tDCS (Li et al., 2021). The curative effect of NIBS in the QOL of OA patients remains uncertain, and other factors should be excluded for further research. The detailed process and results of other research are provided in Table 1.
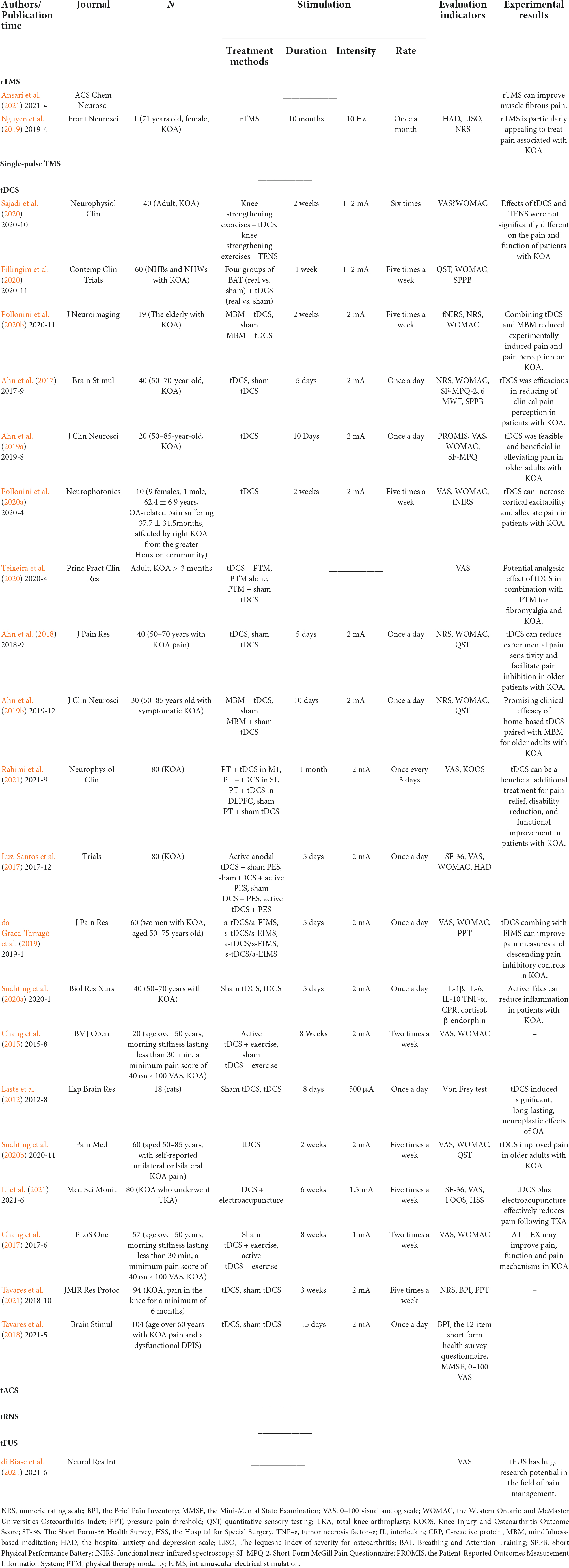
Table 1. Characteristics of the research literature of the six NIBS methods for the treatment of OA.
Mechanisms of non-invasive brain stimulation for osteoarthritis
Controlling central sensitization
OA has a complicated physiological mechanism. Studies have shown that (Fingleton et al., 2015; Perrot, 2015; Vincent, 2020) nerve mediators such as the nerve growth factor (NGF) and central sensitization are closely related to the severity of OA pain. An excitatory–inhibitory mechanism has been proven in the corticospinal system that is related to the degree of pain and the disorder of pain downward control (Passard et al., 2007). rTMS can inhibit the corticospinal system’s excitability by stimulating it, modifying the central pain regulation system (Tarragó et al., 2016), and activating a large number of structures involved in pain processing bilaterally, leading to the long-term relief of chronic extensive pain. The high-frequency rTMS of the motor cortex can also restore the endoscopic suppression control associated with pain relief, and further reduce the pain of OA. tDCS interrupts mechanical pain processing, regulates high-order neuronal circuits that are altered by central sensitization, and weakens the mechanical stimulation response of spinal dorsal horn neurons (Meeker et al., 2019), controlling central sensitization and alleviating pain. Single-pulse TMS can also act on the motor cortex of the brain to increase the overall excitability of the corticospinal cord and the inner periphery of the patient (Woods et al., 2016), reducing quadriceps torque and improving motor function.
Reducing inflammatory factors
Inflammation has been proven to play an important role in OA (Sokolove and Lepus, 2013). Compared with healthy people, OA patients have higher levels of inflammatory factors, such as the tumor necrosis factor-α (Hong et al., 2020), interleukin-1β (Cao et al., 2022), and IL-6 (Wang and He, 2018). The increase of these inflammatory factors may damage the articular cartilage, synovium, and other structures (Mathiessen and Conaghan, 2017); it can also cause the release of adipokines such as leptin, which indirectly causes OA. rTMS can act on microglia, induce the capability of synaptic plasticity, promote the secretion of the anti-inflammatory factor IL-10, and inhibit the production of pro-inflammatory factors, reducing inflammatory response (Lenz et al., 2021). Under the stimulation of cathodic tDCS, endogenous neural stem cells (NSCs) exhibit a tendency to increase (Ryu et al., 2009), inducing a strong regenerative response and reducing the inflammatory response. Neuritis has beneficial and harmful effects on the prevention of secondary tissue damage (Stoll et al., 2002), regeneration, and recovery. Therefore, the parameters of NIBS should be controlled to promote the beneficial aspects of inducing the recruitment of endogenous NSCs and minimizing inflammation as much as possible (Mabuchi et al., 2000). Promoting the production of factors is conducive to the normal growth of chondrocytes and slows down the development of OA.
Influencing gene expression
Mitochondria are the cell organelles where genetic genes are replicated and transcribed; they also have their gene-mitochondrial (Blanco et al., 2018), and the variation of mtDNA directly affects OA phenotypes (Blanco et al., 2018). Therefore, different gene expressions exert varying effects on the OA process. Among them, rTMS can effectively affect the regulation of astrocytes related to the expression of pro-inflammatory or anti-inflammatory genes (Hong et al., 2020) and may change the cell membrane potential and cell function of astrocytes (Ruohonen and Karhu, 2012) to produce anti-inflammatory mediators for the neuroprotection effect, further reducing inflammation response (Liddelow et al., 2017). rTMS can promote the increase of Nrf2 nuclear metastasis (Tian et al., 2020), and Nrf2 can activate genes via antioxidant response, which can effectively protect cells under inflammatory conditions and can also inhibit the expression of signal channels that induce inflammation, effectively reducing the inflammatory response. In addition, tDCS can stimulate the regulation of osteopontin (Rabenstein et al., 2015, 2019). OPN can increase the survival, proliferation, migration, and neuronal differentiation of endogenous NSC (Rabenstein et al., 2015); enhance the proliferation and migration of neuronal precursors after cerebral ischemia (Denhardt et al., 2001), and play an important role in organizational balance, wound healing, immune regulation, and other aspects. These conditions can alleviate secondary damage in patients with OA, promote cartilage repair, and accelerate functional recovery in patients with OA. Although tACS, tFUS, and other methods still lack research on OA gene expression, NIBS plays a role in the pathological mechanism of OA gene expression.
Improving cortex excitability
Studies suggest that higher cortical excitability may be a mechanism of OA and is closely linked to chronic pain and motor capacity in OA patients (Kittelson et al., 2014; Simis et al., 2021). OA patients with lower cortical inhibition had higher degrees of pain and a more obvious decrease in quadriceps motor function. As one of the NIBS methods for stimulating nerve cells in the superficial brain area (Fan et al., 2021), rTMS can regulate cortical excitability and recovery through different frequency stimulations (Barker, 1991). Among the rehabilitation studies based on the hypothesis of cross-hemisphere competition (Chen and Seitz, 2001), low-frequency rTMS may cause a short-term decline in the excitability of the unaffected hemisphere cortex (Wang et al., 2019), balance the excitability of the cerebellum, improve patients’ learning ability, and increase walking ability. By contrast, high-frequency rTMS can enhance the cortical excitability of the affected hemisphere during exercise (Goh et al., 2020), reducing competition and gradually improving the exercise capacity of patients. The improvement of specific motor functions by tDCS is also related to changes in the excitability of the motor cortex. The tDCS acting on M1 can increase the activity of the cerebral cortex, reduce the body’s response time, and better activate a patient’s motor function (Stagg et al., 2012). Therefore, NIBS might alleviate the symptoms of OA by improving cortical hyperexcitability.
Adjusting endocrine
Obesity is an important risk factor for OA, and obesity is driven by complex endocrine mechanisms (Steidle-Kloc et al., 2018). Under the intervention of rTMS, the serum lipid level is significantly reduced (Ren et al., 2017), while the contents of thyroid-stimulating hormone and thyroxine are increased simultaneously. This finding indicates that rTMS can regulate serum lipid metabolism by changing the hypothalamic–pituitary–thyroid axis (George et al., 1996). Therefore, rTMS has the functions of regulating endocrine, improving PFC metabolic activity (Trojak et al., 2011), and regulating serum metabolic activity of lipid levels, thereby stabilizing blood lipid levels, reducing the incidence of obesity, and ultimately decreasing the effect of obesity on OA. tDCS is also extremely important in the control of obesity, but its specific mechanism remains unclear (Araujo et al., 2018), and the endocrine regulation mechanism that affects OA should be further explored.
In summary, on the basis of its role in complex pathophysiological mechanisms, NIBS can be further applied to OA to test its therapeutic mechanism and efficacy of OA. We summarize the mechanism and effects of six commonly used NIBS methods on OA, as shown in Figures 3, 4.
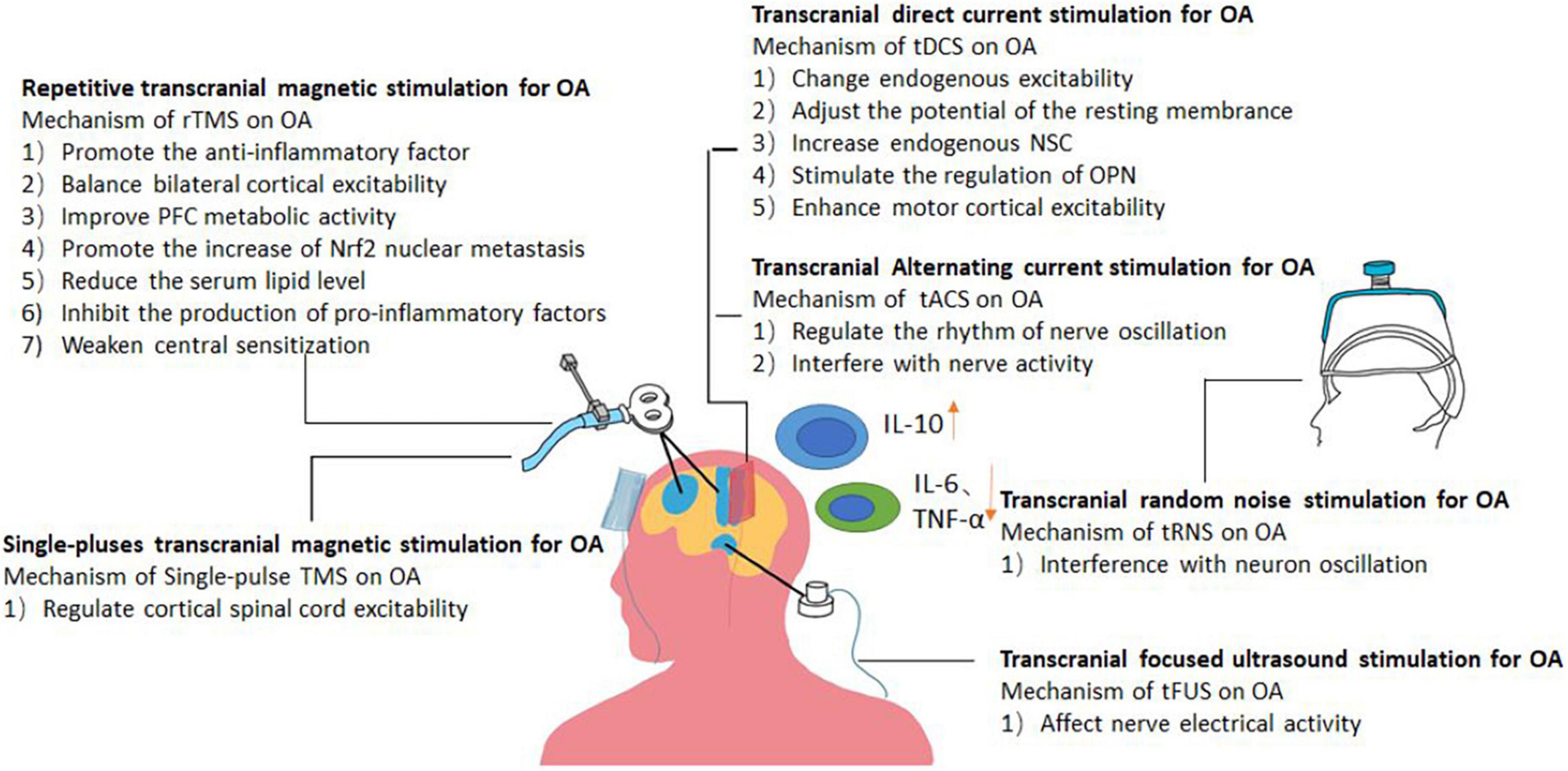
Figure 3. Picture showing the likely mechanism of action of different forms of NIBS in the treatment of osteoarthritis.
Future perspectives
Although NIBS has been proven to be effective and feasible in the treatment of OA, there are still some limitations that need to be improved. First, existing research has focused on the tDCS and rTMS methods. Studies on OA treatment of single-pulse TMS, tACS, tRNS, and tFUS are few. Second, although NIBS, as a mature treatment technique, has been extensively applied to the treatment of clinical diseases in many fields in recent years, the treatment of OA is still in its infancy. No uniform treatment standard for regulating the treatment of OA by NIBS is available. Moreover, when OA occurs in different joints, it will exhibit different symptoms and require more targeted treatment. In addition, current NIBS studies have mostly focused on the pain symptoms of OA, and the treatment of other symptoms lacks evidence to confirm. In summary, the understanding of NIBS in the treatment of OA is limited, and more in-depth exploration can be performed from the aforementioned aspects, such that OA can be treated with more standardized NIBS, enabling OA patients to participate better in family and social life, and reduce social burdens.
Conclusion
With the continuous research on NIBS, the feasibility and effectiveness of NIBS in the treatment of OA have been confirmed by research. This paper summarizes the current research progress of NIBS for OA, describes the main clinical effects and possible mechanisms of NIBS in treating OA, and provides a basis for further research on NIBS in the therapy of OA in the future. However, the treatment protocol of NIBS for the treatment of OA is not unified, which provides a research direction for future research.
Author contributions
X-QW and X-AZ: draft conception, project administration, and funding acquisition. H-QZ, X-QW, X-AZ, and JL: writing – review and editing. All authors contributed to the article and approved the submitted version.
Funding
This research was supported by the Innovative Talents Support Program for Universities of Liaoning Province (No. WR2019024), the Shanghai Frontiers Science Research Base of Exercise and Metabolic Health, and the Shanghai Key Lab of Human Performance (Shanghai University of Sport) (No. 11DZ2261100).
Conflict of interest
The authors declare that the research was conducted in the absence of any commercial or financial relationships that could be construed as a potential conflict of interest.
Publisher’s note
All claims expressed in this article are solely those of the authors and do not necessarily represent those of their affiliated organizations, or those of the publisher, the editors and the reviewers. Any product that may be evaluated in this article, or claim that may be made by its manufacturer, is not guaranteed or endorsed by the publisher.
References
Abhishek, A., and Doherty, M. (2013). Diagnosis and clinical presentation of osteoarthritis. Rheum. Dis. Clin. North Am. 39, 45–66.
Ahn, H., Sorkpor, S., Miao, H., Zhong, C., Jorge, R., Park, L., et al. (2019a). Home-based self-administered transcranial direct current stimulation in older adults with knee osteoarthritis pain: An open-label study. J. Clin. Neurosci. 66, 61–65. doi: 10.1016/j.jocn.2019.05.023
Ahn, H., Suchting, R., Woods, A. J., Miao, H., Green, C., Cho, R. Y., et al. (2018). Bayesian analysis of the effect of transcranial direct current stimulation on experimental pain sensitivity in older adults with knee osteoarthritis: Randomized sham-controlled pilot clinical study. J. Pain Res. 11, 2071–2082. doi: 10.2147/JPR.S173080
Ahn, H., Woods, A. J., Kunik, M. E., Bhattacharjee, A., Chen, Z., Choi, E., et al. (2017). Efficacy of transcranial direct current stimulation over primary motor cortex (anode) and contralateral supraorbital area (cathode) on clinical pain severity and mobility performance in persons with knee osteoarthritis: An experimenter- and participant-blinded, randomized, sham-controlled pilot clinical study. Brain Stimul. 10, 902–909. doi: 10.1016/j.brs.2017.05.007
Ahn, H., Zhong, C., Miao, H., Chaoul, A., Park, L., Yen, I. H., et al. (2019b). Efficacy of combining home-based transcranial direct current stimulation with mindfulness-based meditation for pain in older adults with knee osteoarthritis: A randomized controlled pilot study. J. Clin. Neurosci. 70, 140–145. doi: 10.1016/j.jocn.2019.08.047
Ansari, A. H., Pal, A., Ramamurthy, A., Kabat, M., Jain, S., and Kumar, S. (2021). Fibromyalgia pain and depression: An update on the role of repetitive transcranial magnetic stimulation. ACS Chem. Neurosci. 12, 256–270. doi: 10.1021/acschemneuro.0c00785
Antal, A., Boros, K., Poreisz, C., Chaieb, L., Terney, D., and Paulus, W. (2008). Comparatively weak after-effects of transcranial alternating current stimulation (tACS) on cortical excitability in humans. Brain Stimul. 1, 97–105. doi: 10.1016/j.brs.2007.10.001
Araujo, C., Fitz, R. C., Nogara, D. A., Schestatsky, P., and Gerchman, F. (2018). Effect of transcranial direct current stimulation associated with hypocaloric diet on weight loss and metabolic profile in overweight or obesity: study protocol for a double-blind, randomized controlled clinical trial. Trials 19:386.
Arden, N. K., Perry, T. A., Bannuru, R. R., Bruyère, O., Cooper, C., Haugen, I. K., et al. (2021). Non-surgical management of knee osteoarthritis: Comparison of ESCEO and OARSI 2019 guidelines. Nat. Rev. Rheumatol. 17, 59–66. doi: 10.1038/s41584-020-00523-9
Auvichayapat, P., and Auvichayapat, N. (2009). Basic principle of transcranial magnetic stimulation. J. Med. Assoc. Thail. 92, 1560–1566.
Badran, B. W., Caulfield, K. A., Stomberg-Firestein, S., Summers, P. M., Dowdle, L. T., Savoca, M., et al. (2020). Sonication of the anterior thalamus with MRI-Guided transcranial focused ultrasound (tFUS) alters pain thresholds in healthy adults: A double-blind, sham-controlled study. Brain Stimul. 13, 1805–1812.
Bai, Z., Zhang, J., and Fong, K. N. K. (2022). Effects of transcranial magnetic stimulation in modulating cortical excitability in patients with stroke: A systematic review and meta-analysis. J. Neuroeng. Rehabil. 19:24. doi: 10.1186/s12984-022-00999-4
Barker, A. T. (1991). An introduction to the basic principles of magnetic nerve stimulation. J. Clin. Neurophysiol. 8, 26–37. doi: 10.1097/00004691-199101000-00005
Barker, A. T., Jalinous, R., and Freeston, I. L. (1985). Non-invasive magnetic stimulation of human motor cortex. Lancet 1, 1106–1107.
Bartels, E. M., Juhl, C. B., Christensen, R., Hagen, K. B., Danneskiold-Samsøe, B., Dagfinrud, H., et al. (2016). Aquatic exercise for the treatment of knee and hip osteoarthritis. Cochrane Database Syst. Rev. 3:Cd005523.
Battaglini, L., Contemori, G., Penzo, S., and Maniglia, M. (2020). tRNS effects on visual contrast detection. Neurosci. Lett. 717:134696. doi: 10.1016/j.neulet.2019.134696
Blanco, F. J., Valdes, A. M., and Rego-Pérez, I. (2018). Mitochondrial DNA variation and the pathogenesis of osteoarthritis phenotypes. Nat. Rev. Rheumatol. 14, 327–340.
Bland, N. S., and Sale, M. V. (2019). Current challenges: The ups and downs of tACS. Expe. Brain Res.. 237, 3071–3088. doi: 10.1007/s00221-019-05666-0
Cao, R., Yu, H., Long, H., Zhang, H., Hao, C., Shi, L., et al. (2022). Low deacetylation degree chitosan oligosaccharide protects against IL-1β induced inflammation and enhances autophagy activity in human chondrocytes. J. Biomater. Sci. Polym. Ed. 33, 517–531. doi: 10.1080/09205063.2021.1996962
Chang, W. J., Bennell, K. L., Hodges, P. W., Hinman, R. S., Liston, M. B., and Schabrun, S. M. (2015). Combined exercise and transcranial direct current stimulation intervention for knee osteoarthritis: Protocol for a pilot randomised controlled trial. BMJ Open. 5:e008482. doi: 10.1136/bmjopen-2015-008482
Chang, W. J., Bennell, K. L., Hodges, P. W., Hinman, R. S., Young, C. L., Buscemi, V., et al. (2017). Addition of transcranial direct current stimulation to quadriceps strengthening exercise in knee osteoarthritis: A pilot randomised controlled trial. PLoS One 12:e0180328. doi: 10.1371/journal.pone.0180328
Chen, R., and Seitz, R. J. (2001). Changing cortical excitability with low-frequency magnetic stimulation. Neurology 57, 379–380.
Chou, W. Y., Cheng, J. H., Wang, C. J., Hsu, S. L., Chen, J. H., and Huang, C. Y. (2019). Shockwave targeting on subchondral bone is more suitable than articular cartilage for knee osteoarthritis. Int. J. Med. Sci. 16, 156–166. doi: 10.7150/ijms.26659
Cucca, A., Sharma, K., Agarwal, S., Feigin, A. S., and Biagioni, M. C. (2019). Tele-monitored tDCS rehabilitation: Feasibility, challenges and future perspectives in Parkinson’s disease. J. Neuroeng. Rehabil. 16:20. doi: 10.1186/s12984-019-0481-4
D’Agati, D., Bloch, Y., Levkovitz, Y., and Reti, I. (2010). rTMS for adolescents: Safety and efficacy considerations. Psychiatry Res. 177, 280–285.
da Costa, B. R., Reichenbach, S., Keller, N., Nartey, L., Wandel, S., Jüni, P., et al. (2017). Effectiveness of non-steroidal anti-inflammatory drugs for the treatment of pain in knee and hip osteoarthritis: A network meta-analysis. Lancet. 390, e21–e33.
da Graca-Tarragó, M., Lech, M., Angoleri, L. D. M., Santos, D. S., Deitos, A., Brietzke, A. P., et al. (2019). Intramuscular electrical stimulus potentiates motor cortex modulation effects on pain and descending inhibitory systems in knee osteoarthritis: A randomized, factorial, sham-controlled study. J. Pain Res. 12, 209–221. doi: 10.2147/JPR.S181019
de Goede, A. A., Cumplido-Mayoral, I., and van Putten, M. (2020). Spatiotemporal dynamics of single and paired pulse TMS-EEG responses. Brain Topogr. 33, 425–437. doi: 10.1007/s10548-020-00773-6
Del Felice, A., Castiglia, L., Formaggio, E., Cattelan, M., Scarpa, B., Manganotti, P., et al. (2019). Personalized transcranial alternating current stimulation (tACS) and physical therapy to treat motor and cognitive symptoms in Parkinson’s disease: A randomized cross-over trial. Neuroimage Clin. 22:101768. doi: 10.1016/j.nicl.2019.101768
Denhardt, D. T., Noda, M., O’Regan, A. W., Pavlin, D., and Berman, J. S. (2001). Osteopontin as a means to cope with environmental insults: Regulation of inflammation, tissue remodeling, and cell survival. J. Clin. Invest. 107, 1055–1061. doi: 10.1172/JCI12980
di Biase, L., Falato, E., and Di Lazzaro, V. (2019). Transcranial Focused Ultrasound (tFUS) and Transcranial Unfocused Ultrasound (tUS) Neuromodulation: From theoretical principles to stimulation practices. Front. Neurol. 10:549. doi: 10.3389/fneur.2019.00549
di Biase, L., Falato, E., Caminiti, M. L., Pecoraro, P. M., Narducci, F., and Di Lazzaro, V. (2021). Focused Ultrasound (FUS) for chronic pain management: Approved and potential applications. Neurol. Res. Int. 2021:8438498. doi: 10.1155/2021/8438498
Du, J., Yang, F., Hu, J., Hu, J., Xu, Q., Cong, N., et al. (2019). Effects of high- and low-frequency repetitive transcranial magnetic stimulation on motor recovery in early stroke patients: Evidence from a randomized controlled trial with clinical, neurophysiological and functional imaging assessments. Neuroimage Clin. 21:101620. doi: 10.1016/j.nicl.2018.101620
Egloff, C., Hügle, T., and Valderrabano, V. (2012). Biomechanics and pathomechanisms of osteoarthritis. Swiss Med. Wkly. 142:w13583.
Elyamany, O., Leicht, G., Herrmann, C. S., and Mulert, C. (2021). Transcranial alternating current stimulation (tACS): From basic mechanisms towards first applications in psychiatry. Eur. Arch. Psychiatry Clin. Neurosci. 271, 135–156. doi: 10.1007/s00406-020-01209-9
Fan, H., Song, Y., Cen, X., Yu, P., Bíró, I., and Gu, Y. (2021). The effect of repetitive transcranial magnetic stimulation on lower-limb motor ability in stroke patients: A systematic review. Front. Hum. Neurosci. 15:620573. doi: 10.3389/fnhum.2021.620573
Ferrarelli, F., and Phillips, M. L. (2021). Examining and modulating neural circuits in psychiatric disorders with transcranial magnetic stimulation and electroencephalography: Present practices and future developments. Am. J. Psychiatry 178, 400–413. doi: 10.1176/appi.ajp.2020.20071050
Fillingim, R. B., Woods, A. J., Ahn, H., Wu, S. S., Redden, D. T., Lai, S., et al. (2020). Pain relief for osteoarthritis through combined treatment (PROACT): Protocol for a randomized controlled trial of mindfulness meditation combined with transcranial direct current stimulation in non-Hispanic black and white adults with knee osteoarthritis. Contemp. Clin. Trials 98:106159. doi: 10.1016/j.cct.2020.106159
Fingleton, C., Smart, K., Moloney, N., Fullen, B. M., and Doody, C. (2015). Pain sensitization in people with knee osteoarthritis: A systematic review and meta-analysis. Osteoarthritis Cartilage 23, 1043–1056.
Forestier, R., Erol Forestier, F. B., and Francon, A. (2016). Spa therapy and knee osteoarthritis: A systematic review. Ann. Phys. Rehabil. Med. 59, 216–226.
George, M. S., Wassermann, E. M., Williams, W. A., Steppel, J., Pascual-Leone, A., Basser, P., et al. (1996). Changes in mood and hormone levels after rapid-rate transcranial magnetic stimulation (rTMS) of the prefrontal cortex. J. Neuropsychiatry Clin. Neurosci. 8, 172–180. doi: 10.1176/jnp.8.2.172
Goh, H. T., Connolly, K., Hardy, J., McCain, K., and Walker-Batson, D. (2020). Single session of repetitive transcranial magnetic stimulation to left dorsolateral prefrontal cortex increased dual-task gait speed in chronic stroke: A pilot study. Gait Posture. 78, 1–5. doi: 10.1016/j.gaitpost.2020.02.020
Griffin, T. M., Batushansky, A., Hudson, J., and Lopes, E. B. P. (2020). Correlation network analysis shows divergent effects of a long-term, high-fat diet and exercise on early stage osteoarthritis phenotypes in mice. J. Sport Health Sci. 9, 119–131. doi: 10.1016/j.jshs.2019.05.008
Guo, X., Lou, J., Wang, F., Fan, D., and Qin, Z. (2022). Recent advances in nano-therapeutic strategies for osteoarthritis. Front. Pharmacol. 13:924387. doi: 10.3389/fphar.2022.924387
Hermann, W., Lambova, S., and Muller-Ladner, U. (2018). Current treatment options for osteoarthritis. Curr. Rheumatol. Rev. 14, 108–116.
Hong, Y., Liu, Q., Peng, M., Bai, M., Li, J., Sun, R., et al. (2020). High-frequency repetitive transcranial magnetic stimulation improves functional recovery by inhibiting neurotoxic polarization of astrocytes in ischemic rats. J. Neuroinflammation 17:150. doi: 10.1186/s12974-020-01747-y
Jeon, O. H., Kim, C., Laberge, R. M., Demaria, M., Rathod, S., Vasserot, A. P., et al. (2017). Local clearance of senescent cells attenuates the development of post-traumatic osteoarthritis and creates a pro-regenerative environment. Nat. Med. 23, 775–781. doi: 10.1038/nm.4324
Kakouris, N., Yener, N., and Fong, D. T. P. (2021). A systematic review of running-related musculoskeletal injuries in runners. J. Sport Health Sci. 10, 513–522.
Kavanaugh, T. E., Werfel, T. A., Cho, H., Hasty, K. A., and Duvall, C. L. (2016). Particle-based technologies for osteoarthritis detection and therapy. Drug Deliv. Transl. Res. 6, 132–147. doi: 10.1007/s13346-015-0234-2
Kittelson, A. J., Thomas, A. C., Kluger, B. M., and Stevens-Lapsley, J. E. (2014). Corticospinal and intracortical excitability of the quadriceps in patients with knee osteoarthritis. Expe. Brain Res. 232, 3991–3999. doi: 10.1007/s00221-014-4079-6
Klomjai, W., Katz, R., and Lackmy-Vallée, A. (2015). Basic principles of transcranial magnetic stimulation (TMS) and repetitive TMS (rTMS). Ann. Phys. Rehabil. Med. 58, 208–213.
Lapenta, O. M., Marques, L. M., Rego, G. G., Comfort, W. E., and Boggio, P. S. (2018). tDCS in Addiction and impulse control disorders. J. ECT. 34, 182–192.
Laste, G., Caumo, W., Adachi, L. N., Rozisky, J. R., de Macedo, I. C., Filho, P. R., et al. (2012). After-effects of consecutive sessions of transcranial direct current stimulation (tDCS) in a rat model of chronic inflammation. Exp. Brain Res. 221, 75–83. doi: 10.1007/s00221-012-3149-x
Lefaucheur, J. P., Aleman, A., Baeken, C., Benninger, D. H., Brunelin, J., Di Lazzaro, V., et al. (2020). Evidence-based guidelines on the therapeutic use of repetitive transcranial magnetic stimulation (rTMS): An update (2014-2018). Clin. Neurophysiol. 131, 474–528.
Legon, W., Sato, T. F., Opitz, A., Mueller, J., Barbour, A., Williams, A., et al. (2014). Transcranial focused ultrasound modulates the activity of primary somatosensory cortex in humans. Nat. Neurosci. 17, 322–329.
Lenz, M., Eichler, A., and Vlachos, A. (2021). Monitoring and modulating inflammation-associated alterations in synaptic plasticity: Role of brain stimulation and the blood-brain interface. Biomolecules 11:359. doi: 10.3390/biom11030359
Li, X., Yu, W., Li, H., Wang, B., and Xu, J. (2021). Prospective, single-center comparison of transcranial direct current stimulation plus electroacupuncture and standard analgesia in patients after total knee arthroplasty: Effect on rehabilitation and functional recovery. Med. Sci. Monitor 27:e930363. doi: 10.12659/MSM.930363
Liddelow, S. A., Guttenplan, K. A., Clarke, L. E., Bennett, F. C., Bohlen, C. J., Schirmer, L., et al. (2017). Neurotoxic reactive astrocytes are induced by activated microglia. Nature 541, 481–487.
Luz-Santos, C., Ribeiro Camatti, J., Barbosa Paixão, A., Nunes Sá, K., Montoya, P., Lee, M., et al. (2017). Additive effect of tDCS combined with Peripheral Electrical Stimulation to an exercise program in pain control in knee osteoarthritis: Study protocol for a randomized controlled trial. Trials 18:609. doi: 10.1186/s13063-017-2332-6
Mabuchi, T., Kitagawa, K., Ohtsuki, T., Kuwabara, K., Yagita, Y., Yanagihara, T., et al. (2000). Contribution of microglia/macrophages to expansion of infarction and response of oligodendrocytes after focal cerebral ischemia in rats. Stroke 31, 1735–1743. doi: 10.1161/01.str.31.7.1735
Mantovani, V., Maccari, F., and Volpi, N. (2016). Chondroitin Sulfate and glucosamine as disease modifying Anti- Osteoarthritis Dru gs (DMOADs). Curr. Med. Chem. 23, 1139–1151. doi: 10.2174/0929867323666160316123749
Marlow, N. M., Bonilha, H. S., and Short, E. B. (2013). Efficacy of transcranial direct current stimulation and repetitive transcranial magnetic stimulation for treating fibromyalgia syndrome: A systematic review. Pain Pract. 13, 131–145.
Mathew, J., and Danion, F. R. (2018). Ups and downs in catch-up saccades following single-pulse TMS-methodological considerations. PLoS One 13:e0205208. doi: 10.1371/journal.pone.0205208
Mathiessen, A., and Conaghan, P. G. (2017). Synovitis in osteoarthritis: Current understanding with therapeutic implications. Arthritis Res. Ther. 19:18.
Matsumoto, H., and Ugawa, Y. (2017). Adverse events of tDCS and tACS: A review. Clin. Neurophysiol. Pract. 2, 19–25.
May, E. S., Hohn, V. D., Nickel, M. M., Tiemann, L., Gil Ávila, C., Heitmann, H., et al. (2021). Modulating brain rhythms of pain using transcranial alternating current stimulation (tACS) - A sham-controlled study in healthy human participants. J. Pain 22, 1256–1272. doi: 10.1016/j.jpain.2021.03.150
Meeker, T. J., Keaser, M. L., Khan, S. A., Gullapalli, R. P., Seminowicz, D. A., and Greenspan, J. D. (2019). Non-invasive motor cortex neuromodulation reduces secondary hyperalgesia and enhances activation of the descending pain modulatory network. Front. Neurosci. 13:467. doi: 10.3389/fnins.2019.00467
Miniussi, C., and Ruzzoli, M. (2013). Transcranial stimulation and cognition. Handb. Clin. Neurol. 116, 739–750.
Mioni, G., Grondin, S., Mapelli, D., and Stablum, F. A. (2018). tRNS investigation of the sensory representation of time. Sci. Rep. 8:10364. doi: 10.1038/s41598-018-28673-7
Nguyen, J. P., Dixneuf, V., Esnaut, J., Moreno, A. S., Malineau, C., Nizard, J., et al. (2019). The value of high-frequency repetitive transcranial magnetic stimulation of the motor cortex to treat central pain sensitization associated with knee osteoarthritis. Front. Neurosci. 13:388. doi: 10.3389/fnins.2019.00388
Nitsche, M. A., and Paulus, W. (2000). Excitability changes induced in the human motor cortex by weak transcranial direct current stimulation. J. Physiol. 527 Pt 3, 633–639.
O’Connell, N. E., Marston, L., Spencer, S., DeSouza, L. H., and Wand, B. M. (2018). Non-invasive brain stimulation techniques for chronic pain. Cochrane Database Syst. Rev. 4:Cd008208.
Paget, L. D. A., Tol, J. L., Kerkhoffs, G., and Reurink, G. (2021). Health-related quality of life in ankle osteoarthritis: A case-control study. Cartilage 13(1_suppl), 1438S–1444S.
Palm, U., Ayache, S. S., Padberg, F., and Lefaucheur, J. P. (2016a). [Transcranial direct current stimulation (tDCS) for depression: Results of nearly a decade of clinical research]. Encephale 42, 39–47.
Palm, U., Chalah, M. A., Padberg, F., Al-Ani, T., Abdellaoui, M., Sorel, M., et al. (2016b). Effects of transcranial random noise stimulation (tRNS) on affect, pain and attention in multiple sclerosis. Restor. Neurol. Neurosci. 34, 189–199. doi: 10.3233/RNN-150557
Pasquinelli, C., Hanson, L. G., Siebner, H. R., Lee, H. J., and Thielscher, A. (2019). Safety of transcranial focused ultrasound stimulation: A systematic review of the state of knowledge from both human and animal studies. Brain Stimul. 12, 1367–1380. doi: 10.1016/j.brs.2019.07.024
Passard, A., Attal, N., Benadhira, R., Brasseur, L., Saba, G., Sichere, P., et al. (2007). Effects of unilateral repetitive transcranial magnetic stimulation of the motor cortex on chronic widespread pain in fibromyalgia. Brain 130(Pt 10), 2661–2670.
Paulus, W. (2011). Transcranial electrical stimulation (tES - tDCS; tRNS, tACS) methods. Neuropsychol. Rehabil.. 21, 602–617.
Pell, G. S., Roth, Y., and Zangen, A. (2011). Modulation of cortical excitability induced by repetitive transcranial magnetic stimulation: Influence of timing and geometrical parameters and underlying mechanisms. Prog. Neurobiol. 93, 59–98. doi: 10.1016/j.pneurobio.2010.10.003
Plewnia, C., Schroeder, P. A., and Wolkenstein, L. (2015). Targeting the biased brain: Non-invasive brain stimulation to ameliorate cognitive control. Lancet Psychiatry 2, 351–356.
Polanía, R., Nitsche, M. A., and Ruff, C. C. (2018). Studying and modifying brain function with non-invasive brain stimulation. Nat. Neurosci. 21, 174–187.
Pollonini, L., Miao, H., and Ahn, H. (2020a). Longitudinal effect of transcranial direct current stimulation on knee osteoarthritis patients measured by functional infrared spectroscopy: A pilot study. Neurophotonics 7:025004. doi: 10.1117/1.NPh.7.2.025004
Pollonini, L., Montero-Hernandez, S., Park, L., Miao, H., Mathis, K., and Ahn, H. (2020b). Functional Near-Infrared Spectroscopy to Assess Central Pain Responses in a Nonpharmacologic Treatment Trial of Osteoarthritis. J. Neuroimaging 30, 808–814. doi: 10.1111/jon.12782
Qiu, C. G., Chui, C. S., Chow, S. K. H., Cheung, W. H., and Wong, R. M. Y. (2022). Effects of whole-body vibration therapy on knee Osteoarthritis: A systematic review and meta-analysis of randomized controlled trials. J. Rehabil. Med. 54:jrm00266. doi: 10.2340/jrm.v54.2032
Rabenstein, M., Hucklenbroich, J., Willuweit, A., Ladwig, A., Fink, G. R., Schroeter, M., et al. (2015). Osteopontin mediates survival, proliferation and migration of neural stem cells through the chemokine receptor CXCR4. Stem Cell Res. Ther. 6:99. doi: 10.1186/s13287-015-0098-x
Rabenstein, M., Unverricht-Yeboah, M., Keuters, M. H., Pikhovych, A., Hucklenbroich, J., Vay, S. U., et al. (2019). Transcranial current stimulation alters the expression of immune-mediating genes. Front. Cell. Neurosci. 13:461. doi: 10.3389/fncel.2019.00461
Rahimi, F., Nejati, V., Nassadj, G., Ziaei, B., and Mohammadi, H. K. (2021). The effect of transcranial direct stimulation as an add-on treatment to conventional physical therapy on pain intensity and functional ability in individuals with knee osteoarthritis: A randomized controlled trial. Neurophysiol. Clin. 51, 507–516. doi: 10.1016/j.neucli.2021.06.002
Rathbun, A. M., Shardell, M. D., Ryan, A. S., Yau, M. S., Gallo, J. J., Schuler, M. S., et al. (2020). Association between disease progression and depression onset in persons with radiographic knee osteoarthritis. Rheumatology 59, 3390–3399.
Rathbun, A. M., Stuart, E. A., Shardell, M., Yau, M. S., Baumgarten, M., and Hochberg, M. C. (2018). Dynamic effects of depressive symptoms on osteoarthritis knee pain. Arthritis Care Res. 70, 80–88.
Ren, W., Ma, J., Li, J., Zhang, Z., and Wang, M. (2017). Repetitive Transcranial Magnetic Stimulation (rTMS) modulates lipid metabolism in aging adults. Front. Aging Neurosci. 9:334. doi: 10.3389/fnagi.2017.00334
Ruohonen, J., and Karhu, J. (2012). tDCS possibly stimulates glial cells. Clin. Neurophysiol. 123, 2006–2009.
Ryu, J. K., Cho, T., Wang, Y. T., and McLarnon, J. G. (2009). Neural progenitor cells attenuate inflammatory reactivity and neuronal loss in an animal model of inflamed AD brain. J. Neuroinflammation 6:39. doi: 10.1186/1742-2094-6-39
Sabashi, K., Ishida, T., Matsumoto, H., Mikami, K., Chiba, T., Yamanaka, M., et al. (2021). Dynamic postural control correlates with activities of daily living and quality of life in patients with knee osteoarthritis. BMC Musculoskelet. Disord. 22:287. doi: 10.1186/s12891-021-04164-1
Sajadi, S., Karimi, M., Forogh, B., Raissi, G. R., Zarnegar, F., and Ahadi, T. (2020). Randomized clinical trial comparing of transcranial direct current stimulation (tDCS) and transcutaneous electrical nerve stimulation (TENS) in knee osteoarthritis. Neurophysiol. Clin. 50, 367–374. doi: 10.1016/j.neucli.2020.08.005
Sanguinetti, J. L., Hameroff, S., Smith, E. E., Sato, T., Daft, C. M. W., Tyler, W. J., et al. (2020). Transcranial focused ultrasound to the right prefrontal cortex improves mood and alters functional connectivity in humans. Front. Hum. Neurosci. 14:52. doi: 10.3389/fnhum.2020.00052
Santos Ferreira, I., Teixeira Costa, B., Lima Ramos, C., Lucena, P., Thibaut, A., and Fregni, F. (2019). Searching for the optimal tDCS target for motor rehabilitation. J. Neuroeng. Rehabil. 16:90.
Savoie, F. A., Dallaire-Jean, L., Thénault, F., Whittingstall, K., and Bernier, P. M. (2020). Single-Pulse TMS over the parietal cortex does not impair sensorimotor perturbation-induced changes in motor commands. eNeuro 7, ENEURO.209–ENEURO.219.
Simis, M., Imamura, M., de Melo, P. S., Marduy, A., Pacheco-Barrios, K., Teixeira, P. E. P., et al. (2021). Increased motor cortex inhibition as a marker of compensation to chronic pain in knee osteoarthritis. Sci. Rep. 11:24011. doi: 10.1038/s41598-021-03281-0
Sokolove, J., and Lepus, C. M. (2013). Role of inflammation in the pathogenesis of osteoarthritis: Latest findings and interpretations. Ther. Adv. Musculoskelet. Dis. 5, 77–94. doi: 10.1177/1759720X12467868
Stagg, C. J., Bachtiar, V., O’Shea, J., Allman, C., Bosnell, R. A., Kischka, U., et al. (2012). Cortical activation changes underlying stimulation-induced behavioural gains in chronic stroke. Brain J. Neurol. 135(Pt 1), 276–284.
Steidle-Kloc, E., Dannhauer, T., Wirth, W., and Eckstein, F. (2018). Responsiveness of infrapatellar fat pad volume change to body weight loss or gain: Data from the osteoarthritis initiative. Cells Tissues Organs. 205, 53–62.
Stoll, G., Jander, S., and Schroeter, M. (2002). Detrimental and beneficial effects of injury-induced inflammation and cytokine expression in the nervous system. Adv. Exp. Med. Biol. 513, 87–113.
Suchting, R., Colpo, G. D., Rocha, N. P., and Ahn, H. (2020a). The effect of transcranial direct current stimulation on inflammation in older adults with knee osteoarthritis: A bayesian residual change analysis. Biol. Res. Nurs. 22, 57–63. doi: 10.1177/1099800419869845
Suchting, R., Kapoor, S., Mathis, K. B., and Ahn, H. (2020b). Changes in experimental pain sensitivity from using home-based remotely supervised transcranial direct current stimulation in older adults with knee osteoarthritis. Pain Med. 21, 2676–2683. doi: 10.1093/pm/pnaa268
Tarragó, M., Deitos, A., Brietzke, A. P., Vercelino, R., Torres, I. L. S., Fregni, F., et al. (2016). Descending control of nociceptive processing in knee osteoarthritis is associated with intracortical disinhibition: An exploratory study. Medicine 95:e3353. doi: 10.1097/MD.0000000000003353
Tavares, D. R. B., Okazaki, J. E. F., Rocha, A. P., Santana, M. V. A., Pinto, A., Civile, V. T., et al. (2018). Effects of transcranial direct current stimulation on knee osteoarthritis pain in elderly subjects with defective endogenous pain-inhibitory systems: Protocol for a randomized controlled trial. JMIR Res. Protoc. 7:e11660. doi: 10.2196/11660
Tavares, D. R. B., Okazaki, J. E. F., Santana, M. V. A., Pinto, A., Tutiya, K. K., Gazoni, F. M., et al. (2021). Motor cortex transcranial direct current stimulation effects on knee osteoarthritis pain in elderly subjects with dysfunctional descending pain inhibitory system: A randomized controlled trial. Brain Stimul. 14, 477–487. doi: 10.1016/j.brs.2021.02.018
Teixeira, P. E. P., Alawdah, L., Alhassan, H. A. A., Guidetti, M., Priori, A., Papatheodorou, S., et al. (2020). The Analgesic Effect of Transcranial Direct Current Stimulation (tDCS) combined with Physical Therapy on Common Musculoskeletal Conditions: A Systematic Review and Meta-Analysis. Princ. Pract. Clin. Res. (2015). 6, 23–26. doi: 10.21801/ppcrj.2020.61.5
Terney, D., Chaieb, L., Moliadze, V., Antal, A., and Paulus, W. (2008). Increasing human brain excitability by transcranial high-frequency random noise stimulation. J. Neurosci. 28, 14147–14155.
Terranova, C., Rizzo, V., Cacciola, A., Chillemi, G., Calamuneri, A., Milardi, D., et al. (2018). Is there a future for Non-invasive brain stimulation as a therapeutic tool? Front. Neurol. 9:1146. doi: 10.3389/fneur.2018.01146
Tian, L., Sun, S. S., Cui, L. B., Wang, S. Q., Peng, Z. W., Tan, Q. R., et al. (2020). Repetitive transcranial magnetic stimulation elicits antidepressant- and anxiolytic-like effect via nuclear factor-E2-related Factor 2-mediated anti-inflammation mechanism in rats. Neuroscience 429, 119–133. doi: 10.1016/j.neuroscience.2019.12.025
Trojak, B., Chauvet-Gelinier, J. C., Vergès, B., and Bonin, B. (2011). Significant increase in plasma thyroid-stimulating hormone during low-frequency repetitive transcranial magnetic stimulation. J. Neuropsychiatry Clin. Neurosci. 23:E12. doi: 10.1176/jnp.23.1.jnpe12
Vieira, P. G., Krause, M. R., and Pack, C. C. (2020). tACS entrains neural activity while somatosensory input is blocked. PLoS Biol. 18:e3000834. doi: 10.1371/journal.pbio.3000834
Waller, B., Munukka, M., Rantalainen, T., Lammentausta, E., Nieminen, M. T., Kiviranta, I., et al. (2017). Effects of high intensity resistance aquatic training on body composition and walking speed in women with mild knee osteoarthritis: A 4-month RCT with 12-month follow-up. Osteoarthr. Cartil. 25, 1238–1246.
Walsh, D. A., McWilliams, D. F., Turley, M. J., Dixon, M. R., Fransès, R. E., Mapp, P. I., et al. (2010). Angiogenesis and nerve growth factor at the osteochondral junction in rheumatoid arthritis and osteoarthritis. Rheumatology 49, 1852–1861. doi: 10.1093/rheumatology/keq188
Wang, R. Y., Wang, F. Y., Huang, S. F., and Yang, Y. R. (2019). High-frequency repetitive transcranial magnetic stimulation enhanced treadmill training effects on gait performance in individuals with chronic stroke: A double-blinded randomized controlled pilot trial. Gait Posture 68, 382–387. doi: 10.1016/j.gaitpost.2018.12.023
Wang, T., and He, C. (2018). Pro-inflammatory cytokines: The link between obesity and osteoarthritis. Cytokine Growth Factor Rev. 44, 38–50.
Woods, A. J., Antal, A., Bikson, M., Boggio, P. S., Brunoni, A. R., Celnik, P., et al. (2016). A technical guide to tDCS, and related non-invasive brain stimulation tools. Clin. neurophysiol. 127, 1031–1048. doi: 10.1016/j.clinph.2015.11.012
Wu, L., Liu, T., and Wang, J. (2021). Improving the effect of transcranial alternating current stimulation (tACS): A systematic review. Front. Hum. Neurosci. 15:652393. doi: 10.3389/fnhum.2021.652393
Xiong, H. Y., Zheng, J. J., and Wang, X. Q. (2022). Non-invasive brain stimulation for chronic pain: State of the art and future directions. Front. Mol. Neurosci. 15:888716. doi: 10.3389/fnmol.2022.888716
Yu, K., Liu, C., Niu, X., and He, B. (2021). Transcranial focused ultrasound neuromodulation of voluntary movement-related cortical activity in humans. IEEE Trans. Biomed. Eng. 68, 1923–1931. doi: 10.1109/TBME.2020.3030892
Zhang, M., Hu, W., Cai, C., Wu, Y., Li, J., and Dong, S. (2022). Advanced application of stimuli-responsive drug delivery system for inflammatory arthritis treatment. Mater. Today Bio. 14:100223. doi: 10.1016/j.mtbio.2022.100223
Zhang, Z., Huang, C., Jiang, Q., Zheng, Y., Liu, Y., Liu, S., et al. (2020). Guidelines for the diagnosis and treatment of osteoarthritis in China (2019 edition). Ann. Transl. Med. 8:1213. doi: 10.21037/atm-20-4665
Zheng, S., Tu, L., Cicuttini, F., Zhu, Z., Han, W., Antony, B., et al. (2021). Depression in patients with knee osteoarthritis: Risk factors and associations with joint symptoms. BMC Musculoskelet. Disord. 22:40. doi: 10.1186/s12891-020-03875-1
Keywords: osteoarthritis, NIBS, rTMS, single-pulse TMS, tDCS, tRNS, tACS, tFUS
Citation: Zhu H-Q, Luo J, Wang X-Q and Zhang X-A (2022) Non-invasive brain stimulation for osteoarthritis. Front. Aging Neurosci. 14:987732. doi: 10.3389/fnagi.2022.987732
Received: 06 July 2022; Accepted: 26 August 2022;
Published: 29 September 2022.
Edited by:
Chuhuai Wang, Sun Yat-sen University, ChinaReviewed by:
Ying Shen, The First Affiliated Hospital of Nanjing Medical University, ChinaMin Su, Soochow University, Taiwan
Xiao Bao, Yuebei People’s Hospital, China
Copyright © 2022 Zhu, Luo, Wang and Zhang. This is an open-access article distributed under the terms of the Creative Commons Attribution License (CC BY). The use, distribution or reproduction in other forums is permitted, provided the original author(s) and the copyright owner(s) are credited and that the original publication in this journal is cited, in accordance with accepted academic practice. No use, distribution or reproduction is permitted which does not comply with these terms.
*Correspondence: Xin-An Zhang, zhangxa2725@163.com; Xue-Qiang Wang, wangxueqiang@sus.edu.cn