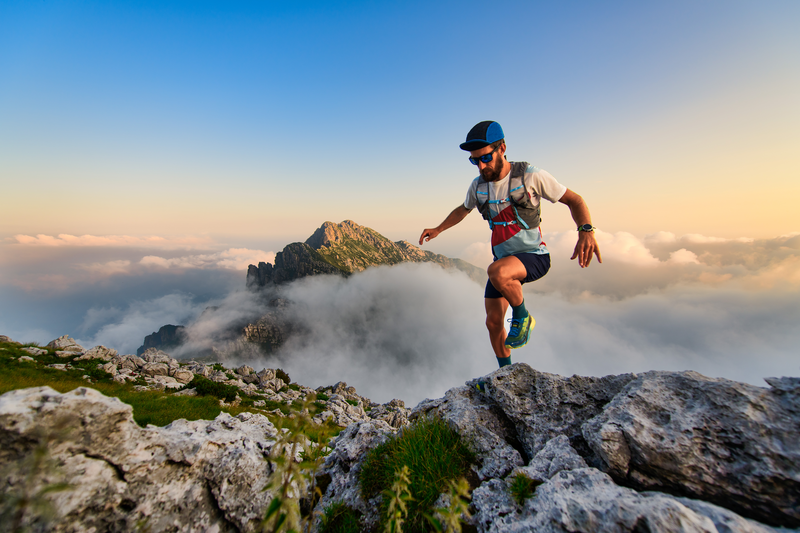
95% of researchers rate our articles as excellent or good
Learn more about the work of our research integrity team to safeguard the quality of each article we publish.
Find out more
SYSTEMATIC REVIEW article
Front. Aging Neurosci. , 26 August 2022
Sec. Neurocognitive Aging and Behavior
Volume 14 - 2022 | https://doi.org/10.3389/fnagi.2022.981002
This article is part of the Research Topic Hippocampal Mechanisms in Aging and Clinical Memory Decline View all 5 articles
Background: Emerging evidence suggests that exercise is a simple and effective method for maintaining brain function.
Aims: This review evaluates the effects of five physical exercises, including aerobic training (AT), high-intensity interval training (HIIT), combined training (CT), resistance training (RT), and AT+RT, on the serum level of brain-derived neurotrophic factor (BDNF) in healthy and non-healthy populations.
Methods: We searched CNKI, PubMed, Embase, Scopus, Medline, Web of Science, and Cochrane Library databases to review randomized controlled studies on exercise interventions for BDNF. Quantitative merging analysis of the resulting data using Bayesian network meta-analysis.
Results: The screening and exclusion of the searched literature resulted in the inclusion of 39 randomized controlled trials containing 5 exercise interventions with a total of 2031 subjects. The AT, RT, AT+RT, HIIT, and CT groups (intervention groups) and the CG group (conventional control group) were assigned to 451, 236, 102, 84, 293, and 865 subjects, respectively. The Bayesian network meta-analysis ranked the effect of exercise on BDNF level improvement in healthy and non-healthy subjects as follows: RT > HIIT > CT > AT+RT > AT > CG. Better outcomes were observed in all five intervention groups than in the CG group, with RT having the most significant effect [MD = 3.11 (0.33, 5.76), p < 0.05].
Conclusions: RT at moderate intensity is recommended for children and older adults in the case of exercise tolerance and is effective in maintaining or modulating BDNF levels for promoting brain health.
Systematic Review Registration: https://inplasy.com, INPLASY202250164.
The central nervous system (CNS) is the primary site of distribution for brain-derived neurotrophic factor (BDNF), the second neurotrophic factor identified after nerve growth factor (NGF) (Binder and Scharfman, 2004). BDNF is most abundant in hippocampal and cortical tissues, and its ability to keep brain cells active promotes the survival, differentiation, and growth of brain neurons (Hans, 1995; Thoenen, 1995; Min-Wook et al., 2005). BDNF not only maintains and promotes neuronal development and differentiation along with nerve growth and regeneration but also engages in a variety of mechanisms such as regenerative repair after neuronal injury and neuronal degeneration prevention. In children and adolescents, BDNF is critical in supporting brain development and plasticity (Iughetti et al., 2011; Wrigglesworth et al., 2019). Physical activity increases the release of BDNF, insulin-like growth factor-1 (IGF-1), fibroblast growth factor 2 (FGF-2), NGF, and vascular endothelial growth factor (VEGF) (Gómez-Pinilla et al., 1997; Fabel et al., 2003; Griffin et al., 2011; Hong et al., 2015; Stein et al., 2018). These promote cerebral angiogenesis and penetrate the blood-brain barrier (BBB) and the blood-cerebrospinal fluid (CSF) barrier, affecting brain health; in the elderly population, reduced levels of BDNF and its receptors may be a major cause of the onset and progression of neurodegenerative diseases (Blesch, 2006; Ruiz-González et al., 2021), resulting in impaired brain health, memory loss, and cognitive decline in the absence of timely and effective interventions. In addition, BDNF is closely associated with cognitive function, whereas physical activity increases BDNF mRNA expression in the hippocampus, which remains high after a short period of cessation of exercise (Neeper et al., 1996; Shawne et al., 1996; Carl and Berchtold, 2002). As a result, changes in BDNF concentrations in the body may be directly linked to the mechanisms through which physical activity reshapes the brain, boosts cognition, and improves neurological disorders (Flöel et al., 2010; Knaepen et al., 2010; Erickson et al., 2011; Nascimento et al., 2015).
Exercise is frequently used in complementary and alternative medicine as a non-pharmacological treatment with hardly any side effects. Exercise therapy has been used extensively in recent years to intervene in nervous system diseases (NSDs), such as cognitive impairment, multiple sclerosis, and Parkinson's disease. There is research evidence indicating that exercise can increase serum BDNF levels, and BDNF is a protective factor for depression and NSDs (Guo et al., 2020). Some studies have shown that aerobic training (AT), resistance training (RT), high-intensity interval training (HIIT), and combined training (CT) do not, however, increase serum BDNF levels (Goekint et al., 2010; Krogh et al., 2014; Nicolini et al., 2019; Abbaspoor et al., 2020). BDNF levels are an important indicator of human brain health, and the overall findings on the effects of exercise therapy on BDNF levels in healthy subjects and patients with NSDs are worthy of further investigation for application in clinical practice. This study collected past RCTs and analyzed the effects of five different exercise interventions on serum BDNF levels in healthy and non-healthy populations using the Bayesian NMA method, yielding comprehensive comparative results (Lumley, 2002). Compared with traditional Meta-analysis, Network meta-analysis (NMA) allows the quantitative comparisons of different interventions for similar health problems to be pooled to integrate direct and indirect comparisons and produce better mixed estimates; provides advantages and disadvantages of the results of different interventions and ranks them, providing an “action guide” for the development of exercise prescriptions. The objective of our study was to understand the effectiveness of exercise in maintaining and promoting brain health in patients with degenerative neurological diseases vs. healthy populations and to provide more comprehensive and effective non-pharmacological evidence for subsequent clinical decisions.
The preferred reporting items for systematic reviews and meta-analyses (PRISMA) were used to conduct the NMA (Hutton et al., 2016). This study was registered with the International Platform of Registered Systematic Review and Meta-analysis Protocols (INLASY) under the unique identification number INPLASY202250164.
CNKI, PubMed, Embase, Scopus, Medline, Web of Science, and the Cochrane Library were among the databases searched. In addition, ongoing and completed trials published between January 1990 and May 2022 were obtained from the American Clinical Trial Registry and the Chinese Clinical Trial Register. A combination of subject terms and free terms were used in the search for comprehensiveness and accuracy, and they were linked using Boolean logic operators. Supplementary material 1 provides a detailed search strategy using the PubMed database as an example.
The inclusion criteria for the study were determined according to the PICOS criteria (Liberati et al., 2009). The detailed inclusion/exclusion criteria are reported in Tables 1, 2.
Two researchers (BJ-Z and ZS-W) read the titles and abstracts to initially screen the literature and reviewed the remaining literature to select studies that met the inclusion criteria. Subsequently, the screening results of the two researchers were exchanged and compared to confirm whether their results were consistent. Disagreements were discussed and addressed by the research team. Corresponding authors were contacted by email for data not published in the literature. In addition, data presented in graph form were extracted using GetDate software. The extracted information comprised basic information about the included studies (first author and year of publication), subject characteristics (age and sample size), and information on the exercise interventions (means of intervention for the test and control groups, number of interventions, intervention duration, and conclusions).
Two researchers (BJ-Z and ZS-W) independently evaluated the methodology of a single RCT using the Cochrane Collaboration tool (Cumpston et al., 2019). They were evaluated based on random sequence generation, allocation concealment, participant personnel blinding, outcome assessment blinding, incomplete outcome data, selection bias, and other biases.
The effects of the five interventions were statistically analyzed using the R-Studio 4.1 and Addis 1.16.5 softwares, and the network diagram and sequence diagram of various interventions were plotted. NMA was started by R language programming, and Bayesian Markov Chain Monte Carlo (MCMC) algorithm was invoked by relevant instructions to analyze and map the results of random-effects model data (van Valkenhoef et al., 2013; Lin et al., 2014). The iterative convergence was evaluated using the potential scaling reduction factor (PSRF), and the statistical requirement was met when PSRF approached 1 (Van Valkenhoef et al., 2012). The results of the random-effects model data were previously evaluated and processed by the Bayesian MCMC algorithm invoked by relevant ADDIS statistical software instructions; P < 0.05 and 95% confidence intervals (95% CI) were used as the criteria for statistical difference. Due to the inclusion of less than 7 items of resistance training, and some studies did not report single load intensities and durations (Fragala et al., 2014; Deus et al., 2021), therefore no dose effect analysis was performed.
According to the search strategy, 6,914 publications were initially obtained. After deleting duplicate studies using EndnoteX8, 39 publications were included. The specific search process is shown in Figure 1.
This study comprised 39 RCTs with a total of 2031 subjects ranging in age from 15.60 to 92.3 years. The AT, RT, AT+RT, HIIT, and CT groups (intervention groups) and CG group (conventional control group) were assigned to 451, 236, 102, 84, 293, and 865 subjects, respectively. The primary interventions were (1) AT, which included treadmill training, cycling, and aerobic gymnastics; (2) RT, which included large muscle group training, leg press, leg curl, leg extension, vertical traction, arm curl, and chest press; (3) AT+RT; (4) HIIT; and (5) CT, integrating RT, AT, balance training, and agility training. There was a wide variation in the interventions included in the study. The duration of interventions ranged from 15 to 75 min, with the majority lasting between 30 and 60 min. In addition, the frequency of training ranged from 2 to 7 times per week, with the minimum and maximum intervention durations of 3 weeks and 6 months, respectively. In addition, forearm venous blood samples were collected from each subject before and after the exercise intervention, and finally, BDNF concentrations were determined using enzyme-linked immunosorbent assay (ELISA); 36 studies examined serum samples from subjects and 3 studies examined plasma samples from subjects (Seifert et al., 2010; Vaughan et al., 2014; Nascimento et al., 2015). The detailed inclusion characteristics are shown in Table 3.
Of the 39 RCTs included, 16 trials reported a random sequence (random number table, random envelope, and computer), while the remaining 23 did not specify the random assignment method; 13 trials reported the random assignment concealment method: 10 trials were double-blinded, six trials were single-blinded, and one trial did not implement the blinding procedure. In addition, the remaining 22 trials did not report the blinding method. All trials reported the primary outcome indicators completely, with no selective reporting. Furthermore, all trials did not report other biases. The Cochrane Risk of Bias Assessment Tool was used to conduct a methodological assessment of the included trials, and the detailed results are shown in Figure 2.
The five physical activities constructed a CG-centered mesh evidence map (Figure 3) with a total of six intervention nodes and two closed loops: CG–AT–RT–AT+RT and CG–AT–HIIT (Figure 3). The nodal analysis suggested that the P-values were all greater than 0.05 (Table 4), indicating good agreement between direct and indirect comparisons among the 39 included trials. Therefore, the result data for the consistency test was used in this study.
Figure 3. Reticulated evidence map of the effect of different exercise modalities on serum BDNF levels. Each node (solid circle) represents only one type of rehabilitation treatment. The size of the nodes is proportional to the number of subjects involved in the particular treatment intervention (i.e., sample size). The solid line connects the treatment with a direct comparison whose thickness is proportional to the number of trials.
Total BDNF scores were reported in 39 trials. We compared the intervention outcomes between various intervention groups and the control group using MD (as the effect size) and 95% CI. Subjects in the RT [MD = 3.11 (0.33, 5.76), P < 0.05] and CT groups [MD = 1.68 (0.13, 3.29), P < 0.05] had significantly better outcomes than those in the control group. In addition, all exercise treatments significantly improved BDNF. However, other two-by-two comparisons between intervention groups did not show statistically significant differences (P > 0.05, Table 6).
To investigate which exercise strategy had the greatest effect on serum BDNF levels in healthy and non-healthy populations, the intervention effects of the five non-pharmacological treatments were subjected to probability ranking. Statistical evidence revealed that the effect of exercise on BDNF level improvement in healthy and non-healthy people was ranked as follows: RT > HIIT > CT > AT+RT > AT > CG (Figure 4 and Tables 5, 6).
In addition to direct comparisons, some of the trials included in NMA involved indirect comparisons and subjects that had not yet undergone a comparison. Therefore, relevant adjustments were required for control groups with different publication biases. In the funnel plot, studies with small sample sizes have low precision and are scattered all over the bottom of the funnel plot; studies with large sample sizes have high precision and are concentrated at the top of the funnel plot (Galbraith, 1988). The results suggested that the distribution of trials on both sides of the vertical line at X = 0 is symmetrical, and the balance line exhibits a slight inclination pattern (low on the left and high on the right) (Figure 5). The scatter of interventions was concentrated at the top of the funnel plot, with only one point at the bottom of the funnel plot, suggesting a low likelihood of publication bias in the included studies.
According to the retrieved information, this is the first RCT Bayesian Network Meta-analysis using a multiple comparison approach to study the effect of physical activity interventions on BDNF levels in healthy vs. non-healthy populations. Compared to traditional Meta-analysis (Marinus et al., 2019; Ruiz-González et al., 2021), the current study gives a more definitive answer—resistance training is the best exercise to increase peripheral BDNF in both older and younger individuals (healthy or diseased individuals) (see Supplementary material 2 for statistical results). Statistical evidence suggests that exercise is effective in improving peripheral BDNF levels in adolescents and older adults (healthy and diseased individuals) for all types of exercise, and our findings are consistent with those of the Ruiz-González et al. (2021) study. The current study explains the controversy that exists regarding the effects of different exercise interventions and provides more convincing evidence that physical activity promotes brain health.
The hippocampus, the body's memory switch, regulates storage conversion and long-term memory orientation. Magnetic resonance imaging (MRI) suggests that the hippocampus in the brain decreases in size with age or the progression of depression and other NSDs in adulthood (Driscoll et al., 2003; Von Bohlen und Halbach, 2010), resulting in a reduction in learning, memory, and emotional control. In addition, the reduction in hippocampal volume causes a range of adverse events, such as a decrease in the number of brain neurons (Issa et al., 1990) and synaptic connections (Geinisman et al., 1995), as well as a decrease in BDNF levels (Lommatzsch et al., 2005). Studies in adolescents have confirmed that children with intellectual deficits and learning disabilities have a positive correlation between their learning ability and BDNF levels; BDNF mediates neurogenesis, and exercise improves BDNF levels to improve learning ability (Ghafori et al., 2018), which is one of the reasons for improved executive and cognitive abilities in the elderly. Numerous animal studies have indicated that exercise increases serum BDNF and IGF-1 levels in vivo, and the structural, functional, and cognitive effects of these factors on the hippocampal region are beneficial (Bechara and Kelly, 2013; Cetinkaya et al., 2013). Active athletes had higher BDNF levels than sedentary individuals (Correia et al., 2011). This confirms that sport is medicine. There is a long-standing consensus on the efficacy of exercise to enhance cardiorespiratory fitness, promote brain health, and prevent and mitigate a range of neurodegenerative diseases, such as Alzheimer's disease and Parkinson's disease (Bangsbo et al., 2019; Mintzer et al., 2019). However, determining which exercise has the best effect on people's BDNF levels is the focus of this study. Statistical results suggested that RT has the best effect on BDNF levels (Figure 4). RT has great potential to increase BDNF levels; in addition, BDNF released during skeletal muscle contraction flows to the brain and activates multiple signaling pathways (Deus et al., 2021). Cathepsin B (CTSB) levels are elevated in the gastrocnemius muscle and plasma following exercise stimulation; this type of muscle-secreted factor can cross the BBB to modulate BDNF concentrations, promoting brain plasticity and ultimately improving cognitive and memory function (Moon et al., 2016). Furthermore, CTSB has neuroprotective effects, and brain atrophy was present in mice whose CTSB genes were knocked out (Felbor et al., 2002). Therefore, exercise-induced increase in CTSB levels is a crucial protective factor. Several studies have also found a correlation between a decrease in muscle mass or an increase in body fat percentage and memory loss and cognitive decline (Beeri et al., 2021; Anand et al., 2022; De Las Heras et al., 2022). RT prevents muscle atrophy and increases the expression of muscle secretory factors, exerting a better effect on brain health than other forms of physical activity. In peripheral cells and organs BDNF is mainly expressed in muscle tissue (Huang et al., 2014), it is also found in the spinal cord and lymphocyte tissues (Gómez-Pinilla et al., 2001; Casoli et al., 2014). BDNF binds specifically to tropomyosin receptor kinase B (TrkB) to regulate the development, survival, and differentiation of brain neurons and stimulates neurogenesis in the hippocampal region (Gray et al., 2006; Araya et al., 2013; Figure 6). In summary, bones and muscles are important tissues for peripheral BDNF secretion, and resistance training causes more intense stress in bones and muscles, which stimulates BDNF expression in peripheral tissues, transport to the brain via blood circulation, and effects on the brain after crossing the BBB.
In addition to BDNF, IGF-1 plays a vital role in maintaining brain health by actively regulating brain function through the BBB (Lewitt and Boyd, 2019). Changes in IGF-1 levels are positively correlated with changes in hippocampal volume (Hvid et al., 2017), which provides nutritional support to neurons and reverses brain aging (Sonntag et al., 1999). Furthermore, beneficial secreted factors, such as IGF-1, are widely expressed during human growth and development but their levels are decreased in adulthood. IGF-1 is predominantly expressed in the liver but is also found in other tissues such as the bone and skeletal muscle. Exercise stimulation increases IGF-1 secretion (Ye et al., 2020). A systematic review by Ye et al. (2020) reported a significant increase in serum IGF-1 levels in subjects older than 60 years who engaged in RT. Similarly, a 12-year longitudinal study suggested that loss of skeletal muscle mass with age was associated with a decrease in serum IGF-1 levels (Frontera et al., 2000). Skeletal muscle is the primary tissue source for systemic IGF-1 production during high-intensity physical activity (Ye et al., 2020). Therefore, physical activity is extremely essential in maintaining brain health.
Research on the effects of BDNF on brain function has greatly benefited from the identification of single nucleotide polymorphisms (SNPs) in the gene encoding human BDNF, which converts valine to methionine at codon 66 (Val66Met), and from in vitro incubation experiments demonstrating that altered amino acid polymorphisms at codon 66 in the BDNF gene affect the intracellular distribution, packaging and release of BDNF protein in vitro. packaging and release in vitro (Egan et al., 2003; Lu et al., 2013). In addition, the BDNF Val66Met polymorphism may affect plasma BDNF concentrations and TrkB receptor activity in peripheral tissues, and may impair the regulated secretion and intracellular transport of BDNF (Lemos Jr et al., 2016; Trombetta et al., 2020). It has been shown that individuals carrying the BDNFMet allele have a reduced exercise gain effect, which seems to explain the consistent lack of significant BDNF changes after exercise in some subjects, regardless of their health (Lu et al., 2013; Lemos Jr et al., 2016). Notably, when participants possessed high levels of physical activity, their memory and cognitive performance remained consistently high whether they carried the BDNFMet allele or the BDNFVal allele. It is not difficult to find that maintaining good exercise habits can offset the potential cognitive disadvantage caused by the BDNF Val66Met polymorphism.
In addition to RT, HIIT, CT, AT+RT, and AT also have beneficial effects on other body functions and peripheral blood BDNF levels. Although some studies confirmed that exercise has no significant effect on BDNF levels, there is a lack of follow-up studies to demonstrate whether there is a decrease in BDNF levels after cessation of exercise. The current study showed that all five interventions achieved effective outcomes, suggesting that all types of exercise are beneficial. However, we observed that the effect of exercise on BDNF levels in the healthy population was not significant, which may be attributed to the consistently higher levels of serum BDNF in the healthy population. The increase in BDNF in healthy individuals may have plateaued because physical activity cannot drive an unrestricted increase in BDNF, demonstrating that regular physical activity maintains function-specific homeostasis in the body (Liu et al., 2014). BDNF levels were negatively correlated with aerobic capacity in healthy populations (Rezola-Pardo et al., 2020) and higher levels of fitness prevented a decrease in hippocampal volume (Erickson et al., 2011), which plausibly explains the non-significant effect of exercise on BDNF levels in healthy populations.Neither physical exercise nor other interventions can repair what is not damaged. Finally, we used the quantitative difference(QD) statistical method proposed by research group of Liu et al. (2017); Sun et al. (2019) to reanalyze the geometric mean (Waldinger et al., 2008; Moser et al., 2020) (calculated from the baseline mean and endpoint means) of the intervention results of the 39 papers included in this network meta-analysis. The study interventions of Yin et al. (2022) and Seifert et al. (2010) were found to have a significant effect on BDNF levels in the chronically inactive population, with QD values greater than α at the level of cells, molecules or central nervous systems. Suggesting that CT and AT may have more significant activation of specific signal transduction pathways in the non-exercise habit group, thereby inducing BDNF expression in skeletal muscle cells. It is important to note that the reasons for its QD value failed to exceed α at the level of cells, molecules or central nervous systems from reanalysis of the other 37 studies may be related to the rigor of the study design, the failure to investigate the previous exercise habits of the intervention population, or the recruitment of a population with active exercise behavior prior to the intervention, which will be verified by our group in a follow-up study. Therefore, exercise may be a specific factor to prevent the decline of BDNF level or keep it at an optimal level. Furthermore, exercise positively regulates BDNF and IGF-1 levels, which is beneficial for neuronal growth, development, and survival. Consequently, it acts as a crucial protective factor in mediating exercise-induced improvements in cognition and reducing depression to prevent the continued progression of other neurodegenerative diseases.
The mechanism by which exercise regulates BDNF and IGF-1 levels to improve brain health is unknown. We speculate, as active stimulation, exercise mediates stress in skeletal muscle or other tissues and organs in response to injury or inflammation, thereby initiating the repair process. BDNF and IGF-1 cross the BBB for the repair of injured and aging brain tissues.
Maintaining optimal levels of BDNF is critical for physical and brain health. Modern advances in biology have made it easier to extract or synthesize BDNF from other animal tissues. However, the special structure of the BBB prevents harmful substances from entering the CNS, and also reduces the penetration of exogenous drugs through the BBB, and affects the treatment of brain diseases. This is attributed to the lack of curative drugs for other degenerative neurological diseases such as depression and Alzheimer's disease. Endogenous BDNF produced by exercise, on the other hand, can bind specifically to TrkB, which crosses the BBB for the repair of injured and aging brain tissues. In future studies, we will focus on the effect of exercise on BBB permeability and the ameliorative effect of exogenous BDNF on degenerative neurological disorders when used after exercise. In the end, more evidence of high-intensity interval training interventions is desired to determine if resistance training is the optimal intervention.
This study also has several limitations. In the quantitative consolidation, the means and standard deviations presented partially in the form of graphs were extracted through GetData, which may have led to some errors. Additionally, only Chinese and English databases were searched. Finally, small sample studies were included.
Bayesian Network Meta-Analysis evidence suggests that better outcomes were observed in all five intervention groups than that in the conventional control group. The effect of exercise on BDNF level improvement in healthy and non-healthy populations was ranked as follows: RT > HIIT > CT > AT+RT > AT > CG. Exercise has a polypill effect, consequently activating endogenous disease resistance mechanisms. Physical activity as a positive non-pharmacological stimulus enables organs or tissues to initiate the release of endogenous drugs to fight internal diseases of the organism due to aging. RT at moderate intensity is recommended for children and older adults in the case of exercise tolerance, which is more effective in maintaining or increasing BDNF levels for brain health compared to other exercise types. In summary, exercise is a simple and effective way to maintain brain function and promote brain remodeling. Finally, exercise prescriptions should take into account the exercise preferences of the target population to avoid noncompliance.
The original contributions presented in the study are included in the article/Supplementary material, further inquiries can be directed to the corresponding author/s.
TL and FM designed this study. BZ and ZW ran the search strategy, collected data, and assess the quality of studies. LZ and GH rechecked data. BZ performed analysis and wrote the manuscript. TL, CC, and JH rechecked. BL uses get date software to extract graphical data. ZW, TL, and FM edited. ZW lead into QD statistical method and geometric mean in this discussion. All listed authors reviewed and revised the manuscript.
This work was supported by the National Key Research and Development Program of China (2017YFB0403800).
We thank the reviewers for their contribution to the successful publication of this study. Thank to Figdraw platform for providing drawing tools. We also thank Bullet Edits Limited for the linguistic editing and proofreading of the manuscript.
Author FM was employed by Qinghai Institute of Sports Science Limited Company.
The remaining authors declare that the research was conducted in the absence of any commercial or financial relationships that could be construed as a potential conflict of interest.
All claims expressed in this article are solely those of the authors and do not necessarily represent those of their affiliated organizations, or those of the publisher, the editors and the reviewers. Any product that may be evaluated in this article, or claim that may be made by its manufacturer, is not guaranteed or endorsed by the publisher.
The Supplementary Material for this article can be found online at: https://www.frontiersin.org/articles/10.3389/fnagi.2022.981002/full#supplementary-material
CNS, central nervous system; BDNF, brain-derived neurotrophic factor; NGF, nerve growth factor; IGF-1, insulin-like growth factor; FGF-2, fibroblast growth factor 2; VEGF, vascular endothelial growth factor; BBB, blood-brain barrier; CSF, cerebrospinal fluid; NSD, nervous system disease; AT, aerobic training; RT, resistance training; HIIT, high-intensity interval training; CT, combined training; RCT, randomized controlled trial; NMA, network meta-analysis; PRISMA, preferred reporting items for systematic review and meta-analyses; MCMC, Bayesian Markov Chain Monte Carlo; PSRF, potential scaling reduction factor; ELISA, enzyme-linked immunosorbent assay; MRI, magnetic resonance imaging; CTSB, cathepsin B; TrkB, tropomyosin receptor kinase B; QD, quantitative difference; HRR, heart rate reserve; VO2max, maximum oxygen uptake; HRmax, maximum heart rate; THR, target heart rate; MVP, maximum velocity performance; SNPs, single nucleotide polymorphisms.
Abbaspoor, E., Zolfaghari, M., Ahmadi, B., and Khodaei, K. (2020). The effect of combined functional training on BDNF, IGF-1, and their association with health-related fitness in the multiple sclerosis women. Growth Horm. IGF Res. 52, 101320. doi: 10.1016/j.ghir.2020.101320
Anand, S. S., Friedrich, M. G., Lee, D. S., Awadalla, P., Després, J. P., Desai, D., et al. (2022). Canadian alliance of healthy hearts and minds (CAHHM) and the prospective urban and rural epidemiological (PURE) study investigators. Evaluation of adiposity and cognitive function in adults. JAMA Netw Open. 5, e2146324. doi: 10.1001/jamanetworkopen.2021.46324
Araya, A. V., Orellana, X., Godoy, D., Soto, L., and Fiedler, J. (2013). Effect of exercise on circulating levels of brain-derived neurotrophic factor (BDNF) in overweight and obese subjects. Horm. Metab. Res. 45, 541–544. doi: 10.1055/s-0032-1333237
Arrieta, H., Rezola-Pardo, C., Kortajarena, M., Hervás, G., Gil, J., Yanguas, J. J., et al. (2020). The impact of physical exercise on cognitive and affective functions and serum levels of brain-derived neurotrophic factor in nursing home residents: a randomized controlled trial. Maturitas 131, 72–77. doi: 10.1016/j.maturitas.2019.10.014
Bangsbo, J., Blackwell, J., Boraxbekk, C.J., Caserotti, P., Dela, F., Evans, A. B., et al. (2019). Copenhagen Consensus statement 2019: physical activity and ageing. Br. J. Sports Med. 53, 856–858. doi: 10.1136/bjsports-2018-100451
Bechara, R. G., and Kelly, Á. M. (2013). Exercise improves object recognition memory and induces BDNF expression and cell proliferation in cognitively enriched rats. Behav. Brain Res. 245, 96–100. doi: 10.1016/j.bbr.2013.02.018
Beeri, M. S., Leugrans, S. E., Delbono, O., Bennett, D. A., and Buchman, A. S. (2021). Sarcopenia is associated with incident Alzheimer's dementia, mild cognitive impairment, and cognitive decline. J. Am. Geriatr. Soc. 69, 1826–1835. doi: 10.1111/jgs.17206
Binder, D. K., and Scharfman, H. E. (2004). Brain-derived neurotrophic factor. Growth Factors.22, 123–131. doi: 10.1080/08977190410001723308
Blesch, A. (2006). Neurotrophic factors in neurodegeneration. Brain Pathol. 16, 295–303. doi: 10.1111/j.1750-3639.2006.00036.x
Briken, S., Rosenkranz, S. C., Keminer, O., Patra, S., Ketels, G., Heesen, C., et al. (2016). Effects of exercise on Irisin, BDNF and IL-6 serum levels in patients with progressive multiple sclerosis. J. Neuroimmunol. 299, 53–58. doi: 10.1016/j.jneuroim.2016.08.007
Busch, A. J., Webber, S. C., Richards, R. S., Bidonde, J., Schachter, C. L., Schafer, L. A., et al. (2013). Resistance exercise training for fibromyalgia. Cochrane Database Syst Rev. 2013, CD010884. doi: 10.1002/14651858.CD010884
Byun, J. E., and Kang, E. B. (2016). The effects of senior brain health exercise program on basic physical fitness, cognitive function and BDNF of elderly women: a feasibility study. J. Exerc. Nutrition Biochem. 20, 8–18. doi: 10.20463/jenb.2016.06.20.2.2
Carl, W. C., and Berchtold, N. C. (2002). Exercise: a behavioral intervention to enhance brain health and plasticity. Trends Neurosci. 2, 295–301. doi: 10.1016/s0166-2236(02)02143-4
Casoli, T., Giuli, C., Balietti, M., Giorgetti, B., Solazzi, M., Fattoretti, P., et al. (2014). Effect of cognitive training on the expression of brain-derived neurotrophic factor in lymphocytes of mild cognitive impairment patients. Rejuvenation Res. 17, 235–238. doi: 10.1089/rej.2013.1516
Cetinkaya, C., Sisman, A. R., Kiray, M., Camsari, U. M., Gencoglu, C., Baykara, B., et al. (2013). Positive effects of aerobic exercise on learning and memory functioning, which correlate with hippocampal IGF-1 increase in adolescent rats. Neurosci. Lett. 549, 177–181. doi: 10.1016/j.neulet.2013.06.012
Chaabene, H., Prieske, O., Herz, M., Moran, J., Höhne, J., Kliegl, R., et al. (2021). Home-based exercise programmes improve physical fitness of healthy older adults: a PRISMA-compliant systematic review and meta-analysis with relevance for COVID-19. Ageing Res. Rev. 67, 101265. doi: 10.1016/j.arr.2021.101265
Cho, S. Y., and Roh, H. T. (2016). Effects of aerobic exercise training on peripheral brain-derived neurotrophic factor and eotaxin-1 levels in obese young men. J. Phys. Ther. Sci. 28, 1355–1358. doi: 10.1589/jpts.28.1355
Correia, P. R., Scorza, F. A., Gomes da Silva, S., Pansani, A., Toscano-Silva, M., de Almeida, A.C., et al. (2011). Increased basal plasma brain-derived neurotrophic factor levels in sprint runners. Neurosci. Bull. 27, 325–329. doi: 10.1007/s12264-011-1531-5
Cumpston, M., Li, T., Page, M. J., Chandler, J., Welch, V. A., Higgins, J. P., et al. (2019). Updated guidance for trusted systematic reviews: a new edition of the cochrane handbook for systematic reviews of interventions. Cochrane Database Syst. Rev. 10, ED000142. doi: 10.1002/14651858.ED000142
Damirchi, A., Tehrani, B. S., Alamdari, K. A., and Babaei, P. (2014). Influence of aerobic training and detraining on serum BDNF, insulin resistance, and metabolic risk factors in middle-aged men diagnosed with metabolic syndrome. Clin. J. Sport Med. 24, 513–518. doi: 10.1097/JSM.0000000000000082
De Las Heras, B., Rodrigues, L., Cristini, J., Weiss, M., Prats-Puig, A., Roig, M., et al. (2022). Does the brain-derived neurotrophic factor Val66Met polymorphism modulate the effects of physical activity and exercise on cognition? Neuroscientist. 28, 69–86. doi: 10.1177/1073858420975712
De Lima, N. S., De Sousa, R. A. L., Amorim, F. T., Gripp, F., Diniz, E., Magalhães, C.O., et al. (2022). Moderate-intensity continuous training and high-intensity interval training improve cognition, and BDNF levels of middle-aged overweight men. Metab. Brain Dis. 37, 463–471. doi: 10.1007/s11011-021-00859-5
Deus, L. A., Corrêa, H. L., Neves, R. V. P., Reis, A. L., Honorato, F. S., Silva, V. L., et al. (2021). Are resistance training-induced BDNF in hemodialysis patients associated with depressive symptoms, quality of life, antioxidant capacity, and muscle strength? an insight for the muscle-brain-renal axis. Int. J. Environ. Res. Public Health. 18, 11299. doi: 10.3390/ijerph182111299
Driscoll, I., Hamilton, D. A., Petropoulos, H., Yeo, R. A., Brooks, W. M., Baumgartner, R. N., et al. (2003). The aging hippocampus: cognitive, biochemical and structural findings. Cereb. Cortex. 13, 1344–1351. doi: 10.1093/cercor/bhg081
Egan, M. F., Kojima, M., Callicott, J. H., Goldberg, T. E., Kolachana, B. S., Bertolino, A., et al. (2003). The BDNF val66met polymorphism affects activity-dependent secretion of BDNF and human memory and hippocampal function. Cell 112, 257–269. doi: 10.1016/S0092-8674(03)00035-7
Elham, E., and Masoud, E. (2018). Interleukin-10 and brain-derived neurotrophic factor responses to the Mat Pilates training in women with multiple sclerosis. Sci. Med. 28, ID31668. doi: 10.15448/1980-6108.2018.4.31668
Erickson, K. I., Voss, M. W., Prakash, R. S., Basak, C., Szabo, A., Chaddock, L., et al. (2011). Exercise training increases size of hippocampus and improves memory. Proc. Natl. Acad. Sci. U.S.A. 108, 3017–3022. doi: 10.1073/pnas.1015950108
Fabel, K., Fabel, K., Tam, B., Kaufer, D., Baiker, A., Simmons, N., et al. (2003). is necessary for exercise-induced adult hippocampal neurogenesis. Eur. J. Neurosci. 18, 2803–2812. doi: 10.1111/j.1460-9568.2003.03041.x
Felbor, U., Kessler, B., Mothes, W., Goebel, H. H., Ploegh, H. L., Bronson, R. T., et al. (2002). Neuronal loss and brain atrophy in mice lacking cathepsins B and L. Proc. Natl. Acad. Sci. U.S.A. 99, 7883–7888. doi: 10.1073/pnas.112632299
Flöel, A., Ruscheweyh, R., Krüger, K., Willemer, C., Winter, B., Völker, K., et al. (2010). Physical activity and memory functions: are neurotrophins and cerebral gray matter volume the missing link? Neuroimage 49, 2756–2763. doi: 10.1016/j.neuroimage.2009.10.043
Fragala, M. S., Beyer, K. S., Jajtner, A. R., Townsend, J. R., Pruna, G. J., Boone, C. H., et al. (2014). Resistance exercise may improve spatial awareness and visual reaction in older adults. J. Strength Cond. Res. 28, 2079–2087. doi: 10.1519/JSC.0000000000000520
Frazzitta, G., Maestri, R., Ghilardi, M. F., Riboldazzi, G., Perini, M., Bertotti, G., et al. (2014). Intensive rehabilitation increases BDNF serum levels in parkinsonian patients: a randomized study. Neurorehabil. Neural Repair. 28, 163–168. doi: 10.1177/1545968313508474
Frontera, W. R., Hughes, V. A., Fielding, R. A., Fiatarone, M. A., Evans, W. J., Roubenoff, R., et al. (2000). Aging of skeletal muscle: a 12-yr longitudinal study. J. Appl. Physiol. 88, 1321–1326. doi: 10.1152/jappl.2000.88.4.1321
Galbraith, R. F. A. (1988). note on graphical presentation of estimated odds ratios from several clinical trials. Stat. Med. 7, 889–894. doi: 10.1002/sim.4780070807
Geinisman, Y., Detoledo-Morrell, L., Morrell, F., and Heller, R. E. (1995). Hippocampal markers of age-related memory dysfunction: behavioral, electrophysiological and morphological perspectives. Prog Neurobiol. 45, 223–252. doi: 10.1016/0301-0082(94)00047-L
Ghafori, R., He Irani, A., and Taghiaghadsi, M. (2018). Effect of motor exercises on serum level of brain-derived neurotrophic factor and executive function in children with dysgraphia. J. Kermanshah Univ. Med. Sci. 22, e79187. doi: 10.5812/jkums.79187
Gibala, M. J., Little, J. P., Macdonald, M. J., and Hawley, J. A. (2012). Physiological adaptations to low-volume, high-intensity interval training in health and disease. J. Physiol. 590, 1077–1084. doi: 10.1113/jphysiol.2011.224725
Goekint, M., De Pauw, K., Roelands, B., Njemini, R., Bautmans, I., Mets, T., et al. (2010). Strength training does not influence serum brain-derived neurotrophic factor. Eur. J. Appl. Physiol. 110, 285–293. doi: 10.1007/s00421-010-1461-3
Goldfield, G. S., Kenny, G. P., Prud'homme, D., Holcik, M., Alberga, A.S., Fahnestock, M., et al. (2018). Effects of aerobic training, resistance training, or both on brain-derived neurotrophic factor in adolescents with obesity: the hearty randomized controlled trial. Physiol. Behav. 191, 138–145. doi: 10.1016/j.physbeh.2018.04.026
Gómez-Pinilla, F., Dao, L., and So, V. (1997). Physical exercise induces FGF-2 and its mRNA in the hippocampus. Brain Res. 764, 1–8. doi: 10.1016/S0006-8993(97)00375-2
Gómez-Pinilla, F., Ying, Z., Opazo, P., Roy, R. R., and Edgerton, V. R. (2001). Differential regulation by exercise of BDNF and NT-3 in rat spinal cord and skeletal muscle. Eur. J. Neurosci. 13, 1078–1084. doi: 10.1046/j.0953-816x.2001.01484.x
Gray, J., Yeo, G. S., Cox, J. J., Morton, J., Adlam, A. L., Keogh, J. M., et al. (2006). Hyperphagia, severe obesity, impaired cognitive function, and hyperactivity associated with functional loss of one copy of the brain-derived neurotrophic factor (BDNF) gene. Diabetes 55, 3366–3371. doi: 10.2337/db06-0550
Griffin, É. W., Mullally, S., Foley, C., Warmington, S. A., O'Mara, S. M., Kelly, A. M., et al. (2011). Aerobic exercise improves hippocampal function and increases BDNF in the serum of young adult males. Physiol. Behav. 104, 934–941. doi: 10.1016/j.physbeh.2011.06.005
Guo, L. Y., Lozinski, B., and Yong, V. W. (2020). Exercise in multiple sclerosis and its models: Focus on the central nervous system outcomes. J. Neurosci. Res. 98, 509–523. doi: 10.1002/jnr.24524
Heisz, J. J., Clark, I. B., Bonin, K., Paolucci, E. M., Michalski, B., Becker, S., et al. (2017). The effects of physical exercise and cognitive training on memory and neurotrophic factors. J. Cogn. Neurosci. 29, 1895–1907. doi: 10.1162/jocn_a_01164
Hong, Y. P., Lee, H. C., and Kim, H. T. (2015). Treadmill exercise after social isolation increases the levels of NGF, BDNF, and synapsin I to induce survival of neurons in the hippocampus, and improves depression-like behavior. J. Exerc. Nutrition Biochem. 19, 11–18. doi: 10.5717/jenb.2015.19.1.11
Hsu, C. C., Fu, T. C., Huang, S. C., Chen, C. P., and Wang, J. S. (2021). Increased serum brain-derived neurotrophic factor with high-intensity interval training in stroke patients: a randomized controlled trial. Ann. Phys. Rehabil. Med. 64, 101385. doi: 10.1016/j.rehab.2020.03.010
Huang, T., Larsen, K. T., Ried-Larsen, M., Mller, N. C., and Andersen, L. B. (2014). The effects of physical activity and exercise on brain-derived neurotrophic factor in healthy humans: a review. Scand. J. Med. Sci. Sports. 24, 1–10. doi: 10.1111/sms.12069
Hutton, B., Catalá-López, F., and Moher, D. (2016). La extensión de la declaración PRISMA para revisiones sistemáticas que incorporan metaanálisis en red: PRISMA-NMA [The PRISMA statement extension for systematic reviews incorporating network meta-analysis: PRISMA-NMA]. Med. Clin. (Barc) 147, 262–266. doi: 10.1016/j.medcli.2016.02.025
Hvid, L. G., Nielsen, M. K. F., Simonsen, C., Andersen, M., and Caserotti, P. (2017). Brain-derived neurotrophic factor (BDNF) serum basal levels is not affected by power training in mobility-limited older adults: a randomized controlled trial. Exp. Gerontol. 93, 29–35. doi: 10.1016/j.exger.2017.03.019
Imboden, C., Gerber, M., Beck, J., Eckert, A., Lejri, I., Pühse, U., et al. (2021). Aerobic exercise and stretching as add-on to inpatient treatment for depression have no differential effects on stress-axis activity, serum-BDNF, TNF-alpha and objective sleep measures. Brain Sci. 11, 411. doi: 10.3390/brainsci11040411
Issa, A. M., Rowe, W., Gauthier, S., and Meaney, M. J. (1990). Hypothalamic-pituitary-adrenal activity in aged, cognitively impaired and cognitively unimpaired rats. J. Neurosci. 10, 3247–3254. doi: 10.1523/JNEUROSCI.10-10-03247.1990
Iughetti, L., Casarosa, E., Predieri, B., Patianna, V., and Luisi, S. (2011). Plasma brain-derived neurotrophic factor concentrations in children and adolescents. Neuropeptides 45, 205–211. doi: 10.1016/j.npep.2011.02.002
Jeon, Y. K., and Ha, C. H. (2017). The effect of exercise intensity on brain derived neurotrophic factor and memory in adolescents. Environ. Health Prev. Med. 22, 27. doi: 10.1186/s12199-017-0643-6
Kerling, A., Kück, M., Tegtbur, U., Grams, L., Weber-Spickschen, S., Hanke, A., et al. (2017). Exercise increases serum brain-derived neurotrophic factor in patients with major depressive disorder. J. Affect. Disord. 215, 152–155. doi: 10.1016/j.jad.2017.03.034
Kim, H., Suzuki, T., Kim, M., Kojima, N., Ota, N., Shimotoyodome, A., et al. (2015). Effects of exercise and milk fat globule membrane (MFGM) supplementation on body composition, physical function, and hematological parameters in community-dwelling frail Japanese women: a randomized double blind, placebo-controlled, follow-up trial. PLoS ONE 10, e0116256. doi: 10.1371/journal.pone.0116256
Knaepen, K., Goekint, M., Heyman, E. M., and Meeusen, R. (2010). Neuroplasticity - exercise-induced response of peripheral brain-derived neurotrophic factor: a systematic review of experimental studies in human subjects. Sports Med. 40, 765–801. doi: 10.2165/11534530-000000000-00000
Kohanpour, M. A., Peeri, M., and Azar Ba Yjani, M. A. (2017). The effects of aerobic exercise with lavender essence use on cognitive state and serum brain-derived neurotrophic factor levels in elderly with mild cognitive impairment. Br. J. Pharmacol. 6, 80–84. Available online at: http://www.herbmedpharmacol.com
Krogh, J., Rostrup, E., Thomsen, C., Elfving, B., Videbech, P., Nordentoft, M., et al. (2014). The effect of exercise on hippocampal volume and neurotrophines in patients with major depression: a randomized clinical trial. J. Affect. Disord. 165, 24–30. doi: 10.1016/j.jad.2014.04.041
Ledreux, A., Håkansson, K., Carlsson, R., Kidane, M., Columbo, L., Terjestam, Y., et al. (2019). Differential effects of physical exercise, cognitive training, and mindfulness practice on serum BDNF levels in healthy older adults: a randomized controlled intervention study. J. Alzheimers Dis. 71, 1245–1261. doi: 10.3233/JAD-190756
Lemos, J. R. Jr, Alves, C.R., de Souza, S.B., Marsiglia, J.D., Silva, M.S., Pereira, A.C., et al. (2016). Peripheral vascular reactivity and serum BDNF responses to aerobic training are impaired by the BDNF Val66Met polymorphism. Physiol. Genomics 48, 116–123. doi: 10.1152/physiolgenomics.00086.2015
Lewitt, M. S., and Boyd, G. W. (2019). The role of insulin-like growth factors and insulin-like growth factor-binding proteins in the nervous system. Biochem. Insights 12, 1178626419842176. doi: 10.1177/1178626419842176
Liberati, A., Altman, D. G., Tetzlaff, J., Mulrow, C., Gøtzsche, P. C., Ioannidis, J. P., et al. (2009). The PRISMA statement for reporting systematic reviews and meta-analyses of studies that evaluate health care interventions: explanation and elaboration. PLoS Med. 6, e1000100. doi: 10.1371/journal.pmed.1000100
Lin, L., Jing, Z., and Chu, A. H. (2014). Pcnetmeta: Methods for patient-centered network meta-analysis. Available online at: https://cran.r-project.org/web/packages/pcnetmeta/index.html
Liu, C. Y., Liu, L., Chen, J. G., Peng, Z., and Yang, X. B. (2014). Action-dependent photobiomodulation on health, suboptimal health, and disease. Int. J. Photoenergy 2014, 1–11. doi: 10.1155/2014/832706
Liu, I. T., Lee, W. J., Lin, S. Y., Chang, S. T., Kao, C. L., Cheng, Y. Y., et al. (2020). Therapeutic effects of exercise training on elderly patients with dementia: a randomized controlled trial. Arch. Phys. Med. Rehabil. 101, 762–769. doi: 10.1016/j.apmr.2020.01.012
Liu, T. C., Liu, G., Hu, S. J., Zhu, L., Yang, X. B., Zhang, Q. G., et al. (2017). Quantitative biology of exercise-induced signal transduction pathways. Adv. Exp. Med. Biol. 977, 419–424. doi: 10.1007/978-3-319-55231-6_54
Lommatzsch, M., Zingler, D., Schuhbaeck, K., Schloetcke, K., Zingler, C., Schuff-Werner, P., et al. (2005). The impact of age, weight and gender on BDNF levels in human platelets and plasma. Neurobiol. Aging 26, 115–123. doi: 10.1016/j.neurobiolaging.2004.03.002
Lu, B., Nagappan, G., Guan, X., Nathan, P. J., and Wren, P. (2013). BDNF-based synaptic repair as a disease-modifying strategy for neurodegenerative diseases. Nat. Rev. Neurosci. 14, 401–416. doi: 10.1038/nrn3505
Lumley, T. (2002). Network meta-analysis for indirect treatment comparisons. Stat. Med. 21, 2313–2324. doi: 10.1002/sim.1201
Maass, A., Düzel, S., Brigadski, T., Goerke, M., Becke, A., Sobieray, U., et al. (2016). Relationships of peripheral IGF-1, VEGF and BDNF levels to exercise-related changes in memory, hippocampal perfusion and volumes in older adults. Neuroimage 131, 142–154. doi: 10.1016/j.neuroimage.2015.10.084
Marinus, N., Hansen, D., Feys, P., Meesen, R., Timmermans, A., and Spildooren, J. (2019). The impact of different types of exercise training on peripheral blood brain-derived neurotrophic factor concentrations in older adults: A meta-analysis. Sports Med. 49, 1529–1546. doi: 10.1007/s40279-019-01148-z
Matura, S., Fleckenstein, J., Deichmann, R., Engeroff, T., Füzéki, E., Hattingen, E., et al. (2017). Effects of aerobic exercise on brain metabolism and grey matter volume in older adults: results of the randomised controlled SMART trial. Transl. Psychiatry 7, e1172. doi: 10.1038/tp.2017.135
Mersy, D. J. (1991). Health benefits of aerobic exercise. Postgrad. Med. 90, 103–107. 110–112. doi: 10.1080/00325481.1991.11700983
Mintzer, J., Donovan, K. A., Kindy, A. Z., Lock, S. L., Chura, L. R., Barracca, N., et al. (2019). Lifestyle choices and brain health. Front. Med. 6, 204. doi: 10.3389/fmed.2019.00204
Min-Wook, K., Bang, M. S., Han, T. R., Ko, Y. J., Yoon, B. W., Kim, J. H., et al. (2005). Exercise increased BDNF and trkB in the contralateral hemisphere of the ischemic rat brain. Brain Res. 1052, 16–21. doi: 10.1016/j.brainres.2005.05.070
Moon, H. Y., Becke, A., Berron, D., Becker, B., Sah, N., Benoni, G., et al. (2016). Running-induced systemic cathepsin b secretion is associated with memory function. Cell Metab. 24, 332–340. doi: 10.1016/j.cmet.2016.05.025
Moser, W., Keiser, J., Speich, B., Sayasone, S., Knopp, S., Hattendorf, J., et al. (2020). One mean to rule them all? The arithmetic mean based egg reduction rate can be misleading when estimating anthelminthic drug efficacy in clinical trials. PLoS Negl. Trop. Dis. 14, e0008185. doi: 10.1371/journal.pntd.0008185
Nascimento, C. M., Pereira, J. R., Pires de Andrade, L., Garuffi, M., Ayan, C., Kerr, D. S., et al. (2015). Physical exercise improves peripheral BDNF levels and cognitive functions in mild cognitive impairment elderly with different bdnf Val66Met genotypes. J. Alzheimers Dis. 43, 81–91. doi: 10.3233/JAD-140576
Neeper, S. A., Gómez-Pinilla, F., Choi, J., and Cotman, C. W. (1996). Physical activity increases mRNA for brain-derived neurotrophic factor and nerve growth factor in rat brain. Brain Res. 726, 49–56. doi: 10.1016/0006-8993(96)00273-9
Nicolini, C., Toepp, S., Harasym, D., Michalski, B., Fahnestock, M., Gibala, M. J., et al. (2019). No changes in corticospinal excitability, biochemical markers, and working memory after six weeks of high-intensity interval training in sedentary males. Physiol. Rep. 7, e14140. doi: 10.14814/phy2.14140
Ozkul, C., Guclu-Gunduz, A., Irkec, C., Fidan, I., Aydin, Y., Ozkan, T., et al. (2018). Effect of combined exercise training on serum brain-derived neurotrophic factor, suppressors of cytokine signaling 1 and 3 in patients with multiple sclerosis. J. Neuroimmunol. 316, 121–129. doi: 10.1016/j.jneuroim.2018.01.002
Rezola-Pardo, C., Hervás, G., Arrieta, H., Hernández-de Diego, A., Ruiz-Litago, F., Gil, S.M., et al. (2020). Physical exercise interventions have no effect on serum BDNF concentration in older adults living in long-term nursing homes. Exp. Gerontol. 139, 111024. doi: 10.1016/j.exger.2020.111024
Ruiz, J. R., Gil-Bea, F., Bustamante-Ara, N., Rodríguez-Romo, G., Fiuza-Luces, C., Serra-Rexach, J. A., et al. (2015). Resistance training does not have an effect on cognition or related serum biomarkers in nonagenarians: a randomized controlled trial. Int. J. Sports Med. 36, 54–60. doi: 10.1055/s-0034-1375693
Ruiz-González, D., Hernández-Martínez, A., Valenzuela, P. L., Morales, J. S., and Soriano-Maldonado, A. (2021). Effects of physical exercise on plasma brain-derived neurotrophic factor in neurodegenerative disorders: a systematic review and meta-analysis of randomized controlled trials. Neurosci. Biobehav. Rev. 128, 394–405. doi: 10.1016/j.neubiorev.2021.05.025
Schulz, K. H., Gold, S. M., Witte, J., Bartsch, K., Lang, U. E., Hellweg, R., et al. (2004). Impact of aerobic training on immune-endocrine parameters, neurotrophic factors, quality of life and coordinative function in multiple sclerosis. J. Neurol. Sci. 225, 11–18. doi: 10.1016/j.jns.2004.06.009
Seifert, T., Brassard, P., Wissenberg, M., Rasmussen, P., Nordby, P., Stallknecht, B., et al. (2010). Endurance training enhances BDNF release from the human brain. Am. J. Physiol. Regul. Integr. Comp. Physiol. 298, R372–R377. doi: 10.1152/ajpregu.00525.2009
Shawne, A. N., Gómez-Pinilla, F., Choi, J., and Cotman, C. W. (1996). Physical activity increases mRNA for brain-derived neurotrophic factor and nerve growth factor in rat brain. Brain Res. 726, 49–56.
Sonntag, W. E., Lynch, C. D., Bennett, S. A., Khan, A. S., Thornton, P. L., Cooney, P. T., et al. (1999). Alterations in insulin-like growth factor-1 gene and protein expression and type 1 insulin-like growth factor receptors in the brains of ageing rats. Neuroscience 88, 269–279. doi: 10.1016/S0306-4522(98)00192-4
Stein, A. M., Silva, T. M. V., Coelho, F. G. M., Arantes, F. J., Costa, J. L. R., Teodoro, E., et al. (2018). Physical exercise, IGF-1 and cognition A systematic review of experimental studies in the elderly. Dement Neuropsychol. 12, 114–122. doi: 10.1590/1980-57642018dn12-020003
Sun, S., Hu, C., Pan, J., Liu, C., and Huang, M. (2019). Trait mindfulness is associated with the self-similarity of heart rate variability. Front. Psychol. 10, 314. doi: 10.3389/fpsyg.2019.00314
Szymura, J., Kubica, J., Wiecek, M., and Pera, J. (2020). The immunomodulary effects of systematic exercise in older adults and people with Parkinson's disease. J. Clin. Med. 9, 184. doi: 10.3390/jcm9010184
Thoenen, H. (1995). Neurotrophins and neuronal plasticity. Science 270, 593–598. doi: 10.1126/science.270.5236.593
Trombetta, I. C., DeMoura, J. R., Alves, C. R., Carbonari-Brito, R., Cepeda, F. X., Lemos, J. R. Jr, et al. (2020). Serum levels of BDNF in cardiovascular protection and in response to exercise. Arq. Bras. Cardiol. 115, 263–269. doi: 10.36660/abc.20190368
Van Valkenhoef, G., Lu, G., de Brock, B., Hillege, H., Ades, A. E., Welton, N. J., et al. (2012). Automating network meta-analysis. Res. Synth. Methods 3, 285–299. doi: 10.1002/jrsm.1054
van Valkenhoef, G., Tervonen, T., Zwinkels, T., de Brock, B., and Hillege, H. (2013). ADDIS: a decision support system for evidence-based medicine. Decis. Support Syst. 55, 459–475. doi: 10.1016/j.dss.2012.10.005
Vaughan, S., Wallis, M., Polit, D., Steele, M., Shum, D., Morris, N., et al. (2014). The effects of multimodal exercise on cognitive and physical functioning and brain-derived neurotrophic factor in older women: a randomised controlled trial. Age Ageing 43, 623–629. doi: 10.1093/ageing/afu010
Von Bohlen und Halbach, O. (2010). Involvement of BDNF in age-dependent alterations in the hippocampus. Front. Aging Neurosci. 2, 36. doi: 10.3389/fnagi.2010.00036
Wagner, G., Herbsleb, M., de la Cruz, F., Schumann, A., Brünner, F., Schachtzabel, C., et al. (2015). Hippocampal structure, metabolism, and inflammatory response after a 6-week intense aerobic exercise in healthy young adults: a controlled trial. J. Cereb. Blood Flow Metab. 35, 1570–1578. doi: 10.1038/jcbfm.2015.125
Waldinger, M. D., Zwinderman, A. H., Olivier, B., and Schweitzer, D. H. (2008). Geometric mean IELT and premature ejaculation: appropriate statistics to avoid overestimation of treatment efficacy. J. Sex. Med. 5, 492–499. doi: 10.1111/j.1743-6109.2007.00696.x
Wens, I., Keytsman, C., Deckx, N., Cools, N., Dalgas, U., Eijnde, B. O., et al. (2016). Brain derived neurotrophic factor in multiple sclerosis: effect of 24 weeks endurance and resistance training. Eur. J. Neurol. 23, 1028–1035. doi: 10.1111/ene.12976
Wrigglesworth, J., Ryan, J., Vijayakumar, N., and Whittle, S. (2019). Brain-derived neurotrophic factor DNA methylation mediates the association between neighborhood disadvantage and adolescent brain structure. Psychiatry Res. Neuroimaging 285, 51–57. doi: 10.1016/j.pscychresns.2018.12.012
Ye, G., Xiao, Z., Luo, Z., Huang, X., Abdelrahim, M. E. A., Huang, W., et al. (2020). Resistance training effect on serum insulin-like growth factor 1 in the serum: a meta-analysis. Aging Male 23, 1471–1479. doi: 10.1080/13685538.2020.1801622
Yin, L. -Q., Tang C, -f., Luo, W. Q., Tao, X., Li, F. L., and Li, J. (2022). Effect of multi-mode exercise on cognitive function and neuroplasticity in the elderly with MCI. China Sport Sci. 42, 78–87+97. doi: 10.16469/j.css.202201007
Zimmer, P., Bloch, W., Schenk, A., Oberste, M., Riedel, S., Kool, J., et al. (2018). High-intensity interval exercise improves cognitive performance and reduces matrix metalloproteinases-2 serum levels in persons with multiple sclerosis: a randomized controlled trial. Mult. Scler. 24, 1635–1644. doi: 10.1177/1352458517728342
Keywords: exercise intervention, network meta-analysis, brain-derived neurotrophic factor, brain health, rehabilitation, quantitative difference, geometric mean
Citation: Zhou B, Wang Z, Zhu L, Huang G, Li B, Chen C, Huang J, Ma F and Liu TC (2022) Effects of different physical activities on brain-derived neurotrophic factor: A systematic review and bayesian network meta-analysis. Front. Aging Neurosci. 14:981002. doi: 10.3389/fnagi.2022.981002
Received: 29 June 2022; Accepted: 02 August 2022;
Published: 26 August 2022.
Edited by:
Stephen D. Ginsberg, Nathan Kline Institute for Psychiatric Research, United StatesReviewed by:
Eduardo Henrique Loreti, Centro Universitário da Grande Dourados (UNIGRAN), BrazilCopyright © 2022 Zhou, Wang, Zhu, Huang, Li, Chen, Huang, Ma and Liu. This is an open-access article distributed under the terms of the Creative Commons Attribution License (CC BY). The use, distribution or reproduction in other forums is permitted, provided the original author(s) and the copyright owner(s) are credited and that the original publication in this journal is cited, in accordance with accepted academic practice. No use, distribution or reproduction is permitted which does not comply with these terms.
*Correspondence: Fuhai Ma, NzcwMzkwOTU3QHFxLmNvbQ==; Timon Chengyi Liu, bGl1dGN5QHNjbnUuZWR1LmNu
†These authors have contributed equally to this work and share first authorship
Disclaimer: All claims expressed in this article are solely those of the authors and do not necessarily represent those of their affiliated organizations, or those of the publisher, the editors and the reviewers. Any product that may be evaluated in this article or claim that may be made by its manufacturer is not guaranteed or endorsed by the publisher.
Research integrity at Frontiers
Learn more about the work of our research integrity team to safeguard the quality of each article we publish.