- 1Department of Sport Rehabilitation, Xian Physical Education University, Xian, China
- 2Department of Sport Rehabilitation, Shanghai University of Sport, Shanghai, China
- 3College of Kinesiology, Shenyang Sport University, Shenyang, China
Diabetic neuropathic pain (DNP) is a common disease that affects the daily lives of diabetic patients, and its incidence rate is very high worldwide. At present, drug and exercise therapies are common treatments for DNP. Drug therapy has various side effects. In recent years, exercise therapy has received frequent research and increasing attention by many researchers. Currently, the treatment of DNP is generally symptomatic. We can better select the appropriate exercise prescription for DNP only by clarifying the exercise mechanism for its therapy. The unique pathological mechanism of DNP is still unclear and may be related to the pathological mechanism of diabetic neuropathy. In this study, the mechanisms of exercise therapy for DNP were reviewed to understand better the role of exercise therapy in treating DNP.
Introduction
The incidence rate of diabetes worldwide is very high and the number of individuals with diabetes will increase to 642 million by 2040 (Ng et al., 2021). Diabetes affects 8.3% of Americans, and the annual treatment cost of diabetes is US$ 174 billion (Feldman et al., 2019). Diabetic neuropathy is the most common chronic complication in patients with type 1 or 2 diabetes (Javed et al., 2015; Melese et al., 2020) and accounts for 54–59% and 45% of type 1 and type 2 diabetic patients (Zilliox and Russell, 2011), respectively. Pain, abnormal sensation, and decreased stability are the typical clinical symptoms of diabetic neuropathy (Goldberg et al., 2008; Marcovecchio et al., 2010).
The diabetic neuropathic pain (DNP) associated with diabetes is gradually increasing with the prevalence of diabetes (Tesfaye et al., 2010). It is reported that 25% of patients with type 2 diabetic neuropathy will experience pain (Callaghan et al., 2015). In general, symptoms related to DNP are burning, lacerating, tingling and shooting pain, loss of protective sensation, and hyperalgesia (Kirk et al., 2019). DNP hurts patients’ quality of life compared with painless diabetic neuropathy (Van Acker et al., 2009).
Currently, there are no treatments available to completely cure DNP; however, drugs and exercise are its common symptomatic treatments (Pop-Busui et al., 2017). There are three main categories of drugs used to treat DNP: antiepileptics, antidepressants, and non-specific analgesics (Feldman et al., 2019). However, the severe side effects and high cost of drug therapy have greatly limited the treatment of DNP (Tölle et al., 2006). Exercise for pain has received increasing attention in recent years, i.e., exercise for low back pain (Peng et al., 2022). Research on mechanisms related to exercise for pain has also been favored by researchers, i.e., effects of exercise-induced hypoalgesia (Wu et al., 2022). Meanwhile, the effectiveness and safety of exercise have been proven for people with diabetes (Lemaster et al., 2008; Chiang et al., 2016). According to the American Diabetes Association proposal, at least 150 sessions of moderate to vigorous aerobic exercise for adults with diabetes (60 min for children and adolescents) and 2–3 sessions per week of resistance training are need (American Diabetes Association, 2018). More randomized controlled trials are needed to further explore the mechanisms by which exercise reduces DNP in order to select the appropriate exercise prescription for treatment. This review describes the pathological mechanisms associated with exercise for DNP based on recent studies on exercise for DNP.
Mechanisms of exercise in improving diabetic neuropathic pain
Exercise is an integral part of DNP management plan, and it improves blood glucose control, reduces cardiovascular risk factors, reduces obesity, and promotes good health (American Diabetes Association, 2018). Doing exercise for treatment of DNP is increasingly favored by public-health worker. This paper summarizes studies related to the pathological mechanisms of exercise therapy for DNP (Table 1). This is different between exercise can reduce pain and exercise can reduce neuropathic pain in diabetes. Given that the unique mechanism of pain production is unclear, the pathological mechanism of diabetic neuropathy may be related to that of DNP (Calcutt, 2020). Therefore, it was concluded from the pathological mechanism of diabetic neuropathy that exercise may relieve pain related to DNP by affecting blood glucose and intra-epidermal nerve fibers (IENFs), inflammatory response, miRNA, apoptosis, deficit in oxide synthase (NO) synthesis, and dysregulation of voltage-gated calcium channels (VGCCs) (Figure 1).
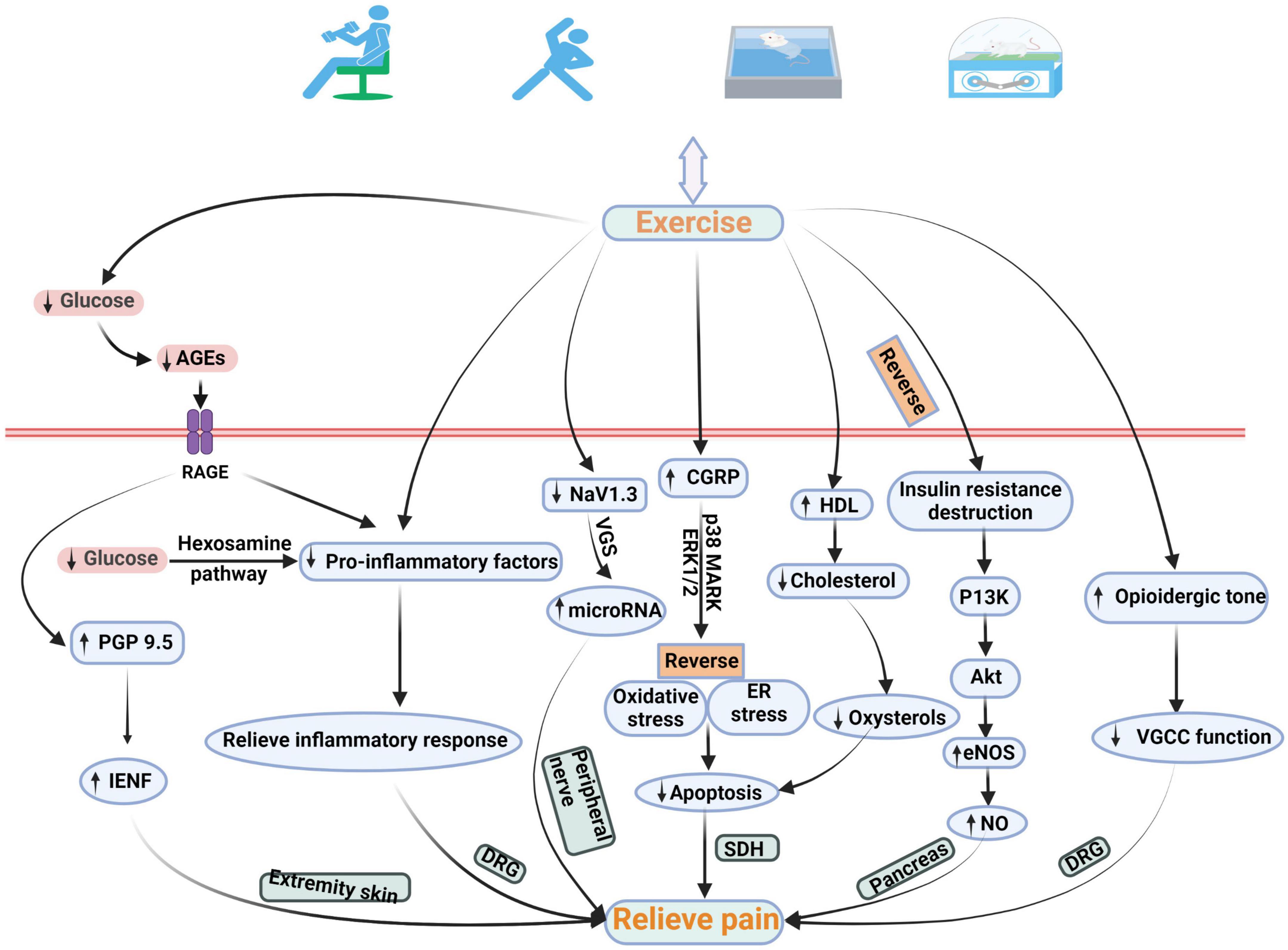
Figure 1. Schematic diagram of the possible pathological mechanism of exercise in the treatment of DNP. Exercise relieves DNP by changing the quantity of IENFs, expression of pro-inflammatory factors, NaV1.3, CGRP, HDL, and eNOS, and VGCC function. AGEs, advanced glycation end products; IENF, intra-epidermal nerve fiber; CGRP, calcitonin gene-related peptide; ASK1, apoptosis signal-regulating kinase 1; p38 MARK, p38 mitogen-activated protein kinase; ERK 1/2, extracellular signal-regulated kinase ½; ER, endoplasmic reticulum; PI3K, phosphatidylinositol-3-kinase; eNOS, endothelial nitric oxide synthase; VGCC, voltage-gated calcium channel.
The role of exercise in glucose control and intra-epidermal nerve fibers
It is known that hyperglycemia is an essential factor that causes diabetes, and that extended hyperglycemia can cause cellular damage (Callaghan et al., 2012). The pathogenesis of DNP remains to be unknown, and hyperglycemia leading to production of advanced glycation end products (AGEs) may be one of the mechanisms for the emergence of pain (Sugimoto et al., 2008). In this signal pathway, glycation of extracellular matrix protein laminin leads to impaired regenerative activity in diabetic neuropathy (Sugimoto et al., 2008). AGEs connect reactive carbohydrate groups to proteins, lipids, or nucleic acids to affect cellular functions (Duran-Jimenez et al., 2009), and AGEs bind to their receptor, initiating NADPH oxidases and generating oxidative stress further damaging neurons, vascular endothelial cells, and thus, leading to reduced IENFs (Vincent et al., 2007; Boric et al., 2013; Do et al., 2020). To quantify intraepidermal nerve fiber density, biopsy of the hairy skin in the lower extremities is a more commonly accepted method (Peltier et al., 2014; Lauria et al., 2010).
A test was conducted to clarify the effects of exercise on controlling the glucose level of diabetic patients. Jensen et al. (2019) conducted 12 weeks of exercise on 50 diabetic patients with musculoskeletal pain, and the results indicated that exercise reduced pain, and that HbA1c (mmol/mol) was decreased from 60 ± 15 to 54 ± 11. These results suggest that exercise effectively improves glucose control in diabetic patients’ neuropathy. Therefore, it is theoretically feasible that exercise can reduce IENF damage by improving blood glucose. Ten weeks of aerobic and resistance exercise interventions on 17 patients with diabetic peripheral neuropathy was conducted by Kluding et al. (2012) After the program, the patients were processed for immunohistochemistry of the right leg proximally at the lateral thigh and distal part of the lateral ankle using a rabbit anti-PGP9.5 primary antibody, and the IENF density was quantified. It was found that exercise significantly reduced pain, lowered HbA1c, and increased PGP 9.5 in the epidermis of the patients, and that the number of branches per fiber in the proximal biopsy site had improved. However, there was no significant increase found in the distal IENF measures or proximal IENF density, and this may be due to some subjects being pain-free before the exercise intervention, limitations of current guidelines on IENF quantification, and testing of IENF density at different time points before and after the exercise intervention (Kluding et al., 2012). Therefore, the specific role of IENFs in DNP needs further study or guidelines to quantify the IENF density to be more detailed and understood. Contradictory findings regarding IENFs in patients with DNP have been reported (Smith et al., 2001; Polydefkis et al., 2003). Another study explained the pathological mechanism of exercise intervention in DNP in terms of neurotrophin and absence of significant change in IENFs in DNP. Groover et al. (2013) developed a DNP mouse model by giving the male C57Bl/6 mice a high-fat diet and then quantifying the IENFs using a rabbit anti-PGP9.5, and the growth factor using an ELISA kit. The quantification of IENF density showed that the high-fat diet and exercise changed the number of PGP9.5-immunopositive fibers. However, the percentage of TrkA/PGP9.5 fibers was 30% lower in the exercise group than in the sedentary control group. The related growth factor and nerve growth factor (NGF) were significantly decreased. Meanwhile, brain-derived neurotrophic factor (BNDF) and glial cell line-derived neurotrophic factor (GDNF) were significantly increased (Groover et al., 2013). The increase in TrkA axons in the DNP mouse model can mediate the increase in NGF (Kashiba et al., 1996), and NGF has an important role in pain management (Pezet and McMahon, 2006). Therefore, this is one of the pathological mechanisms of DNP, and exercise also reduces pain related to DNP by decreasing NGF and TrkA axons. In addition, TrkA-expressing fibers are peptidergic fibers that express nociceptive neuropeptides (Kashiba et al., 1996), and non-peptidergic fibers are sensitive to GDNF (Groover et al., 2013). Therefore, the absence of change in IENFs after exercise intervention in DNP may be due to a decrease in peptidergic fibers and increase in non-peptidergic fibers. However, to determine specific non-peptidergic fibers changes, they should be quantified by markers.
The role of exercise in inflammatory response
Under normal physiological conditions, inflammatory response is the immune system’s response when a harmful stimulus causes damage to the body (Weiss, 2008). In recent years, evidence has been emerging from related research that inflammatory response has a significant role in the pathogenesis of DNP (Pop-Busui et al., 2016). For example, increased pro-inflammatory factors tumor necrosis factor-α (TNF-α), interleukin-10 (IL-10), and C-reactive proteins (CRPs) lead to an inflammatory response associated with neuropathy in diabetes (Shoelson et al., 2006; Pop-Busui et al., 2016). Galloway and Chattopadhyay (2013) constructed the DNP rat model and observed the expression of pro-inflammatory factors and found that compared to rats with no pain, overexpression of a large number of pro-inflammatory factors in NDP rats such as TNF-α, IL-1, 6, 13, and 17, chemokines such as MIP1 and 3, Regulated on Activation, Normal T Cell Expressed and Secreted (RANTES), Fractalkine, and cell adhesion molecule sICAM in dorsal root ganglion (DRG) that are associated with pain phenotype remarkably increased. These results suggested that the pro-inflammatory factors in DRG play a non-negligible role in DNP (Galloway and Chattopadhyay, 2013).
Exercise inhibits inflammatory response by reducing pro-inflammatory factors, thus, reducing pain in DNP, which is the common activity mechanism in treating DNP. Ma et al. (2019) established a DNP rat model by intraperitoneal injection of streptozotocin (STZ) and made DNP rats perform exercise on a treadmill. Paw withdrawal thresholds (PWTs) were significantly increased in the STZ rats by the exercise compared to the control group, and relief of pain was accompanied by decreases in levels of IL-1β, IL-6, and TNF-a. This suggests that exercise modulates pro-inflammatory factor signaling pathways, hence reducing the inflammatory response and further inhibiting DNP. Furthermore, blocking individual pro-inflammatory factor receptors elevated PWTs. This result illustrated the side effects of inflammatory response on regulation of DNP (Ma et al., 2019). Similarly, Yoon et al. (2015) exerted moderate-intensity exercise on DNP rats and they used the level of TNF-α and IL-1β in the DRG as the observations. The results showed that the exercise inhibited hyperalgesia and recess the development of cold allodynia through the TRPM8 and TRPV1 ion channels. Meanwhile, immunohistochemical studies on DRG also showed a significant decrease in TNF-α and IL-1β in the diabetic exercise group. It is an important mechanism in changing the pain threshold in DNP test animals (Yoon et al., 2015). Interestingly, an exercise intervention study on hyperalgesia and allodynia in DNP rats showed that exercise reduced diabetic-associated thermal hyperalgesia and increased heat shock protein 72 (Hsp72) (Chen et al., 2013). Hsp72 has a neuroprotective role in neuropathic pain in rats after peripheral nerve injury (Chen et al., 2012). However, unlike the above study, the expressions of TNF-α and IL-6 have no difference between the exercise and sedentary groups after the exercise intervention, which may be due to the acute pro-inflammatory effect of the short-term exercise (only 2 weeks of exercise intervention). This is because the acute pro-inflammatory effect after each exercise session causes a transient increase in pro-inflammatory factors such as TNF-α, IL-1β, and IL-6 (Nemet et al., 2002; Galassetti et al., 2006; Chen et al., 2013). However, further investigation is needed to know the specific mechanistic relationships among exercise duration, pain, and pro-inflammatory factors. In conclusion, exercise can modulate DNP inflammatory factors and suppress inflammatory responses, consequently reducing pain in DNP.
The role of exercise in micro-ribonucleic acids
Micro-ribonucleic acids (microRNAs) are short categories of non-coding RNAs and they regulate the expression of their target genes by repressing protein translation (Bartel, 2004; Winter et al., 2009). Previous studies have shown that abnormal expression of microRNAs in white blood cells, the sural nerve, and skin is associated with painful peripheral neuropathies (Leinders et al., 2017). Chen et al. (2014) showed that enhanced NaV1.3 expression exacerbated neuropathic pain, and that among microRNAs, miR-96 alleviated neuropathic pain by decreasing the expression of NaV1.3.
Aghdam et al. (2018) established a DNP rat model by intraperitoneal injection of STZ and with a high-fat diet for 1 month to elucidate the effect of exercise on miR-96 and NaV1.3. Rats were subjected to 10 weeks of swimming exercise, after which real-time quantitative PCR was conducted to measure the expression of miR-96 and NaV1.3. The results showed that the swimming exercise group’s thermal pain threshold was remarkably prolonged compared with that of the control group. The swimming exercise reversed the enhanced expression of NaV1.3 caused by diabetes, whereas increasing expression levels and the swimming exercise decreased NaV1.3 expression in the healthy rats. These results indicated that miR-96/NaV1.3 mRNA is a possible mechanism for a protective effect of swimming exercise on diabetic thermal hyperalgesia. In healthy rats, exercise can regulate pain related to DNP through gene regulation pathways (Aghdam et al., 2018).
The role of exercise in apoptosis
Apoptosis is an automatic, evolutionarily conserved, and genetically determined form of programmed cell death that is necessary for maintaining homeostasis and growth in animal tissues (Cheng and Ferrell, 2018). Pancreatic β cells, which are responsible for insulin production, are associated with ER stress-induced apoptosis caused by oxidative stress in diabetic neuropathy. The main action pathway is the apoptosis signal-regulating kinase 1 (ASK1)/p38 mitogen-activated protein kinase (MAPK) signaling pathway (Callaghan et al., 2012; Yamaguchi et al., 2013). Inhibition of the ASK1 signaling pathway reduces the chances of apoptosis pancreatic β cell death (Pepin et al., 2014). In general, the role of calcitonin gene-related peptide (CGRP) in diabetic patients is to control blood glucose, thus reducing the release of insulin (Russell et al., 2014), and insulin increase in diabetic rats is accompanied by loss of CGRP-containing fibers (Hashikawa-Hobara et al., 2012). In an earlier study, CGRP was localized in pancreatic β cells (Westermark et al., 1987), and CGRP prevented apoptosis of vascular smooth muscle cells caused by oxidative stress by activating the extracellular signal-regulated kinase 1/2 (ERK1/2) and p38 MAPK signaling pathways (Schaeffer et al., 2003). Therefore, the mechanism of apoptosis associated with CGRP is involved in the pathological mechanism of DNP. An STZ injection-induced diabetic rat model was established by Nascimento et al. (2012) to research on the role of exercise in DNP and CGRP levels in DRG. A tail-flick apparatus was used to assess nociceptive injury changes. Meanwhile, the immunohistochemical procedure and optical densitometry were performed to measure the level and intensity of CGRP. The results showed that exercise reduced the hyperalgesia and reversed the diabetes-induced decrease in CGRP in the DRG levels of diabetic rats (Nascimento et al., 2012). Therefore, the exercise mechanism for nociceptive hyperalgesia may be increase in CGRP, which reverses the pancreatic β-cell death induced by oxidative and ER stresses through ASK1, p38 MARK, and ERK1/2. That is to control the release of insulin, and then control blood glucose, and finally reduce pain related to DNP. In addition, compared with before the exercise intervention, another study has found an 8.6% increase in HDL cholesterol after the exercise intervention (Lohmann et al., 2010). HDL decrease and LDL increase are inducing factors of oxidative stress, and cholesterol can lead to apoptosis when oxidized to oxysterols (refer to Callaghan et al., 2012 for details). Therefore, exercise may alleviate pain by improving the apoptosis caused by dyslipidemia.
The role of exercise in the deficit of nitric oxide synthesis
Nerve dysfunction is a common complication of experimental diabetes caused by reduced endothelial function and defective neural blood flow (Mrugacz et al., 2021). This may present clinically as DNP (Callaghan et al., 2012; Calcutt, 2020). Reduced diabetic neural blood flow is caused by hyperglycemia leading to reduce nitric oxide (NO) in vascular endothelial cells and further affecting impaired nitric oxide synthase (NOS). Insulin can increase NO by activating intracellular enzymes phosphatidylinositol-3 kinase (PI3K) and protein kinase B (Akt) to further activate endothelial NOS (eNOS) to increase NO, and restore neurological reactivity associated with neural blood flow (Callaghan et al., 2012). This insulin effect of improving vascular reactivity is disrupted by insulin resistance (Wallis et al., 2002). However, a related study has found that exercise can reverse this damage (Rattigan et al., 2001). To verify that exercise ameliorates decreased vascular nerve reactivity and reduced NO signaling that contributes to peripheral nerve dysfunction, Olver et al. (2014) established a diabetic rat model by STZ injection, performed insulin treatment and exercise training, and then observed motor nerve conduction velocity (MNCV) and eNOS expression in the rat’s sciatic nerve. The results revealed that the diabetes sedentary (DS) rats had lower MNCV than the control sedentary (CS) and diabetes exercise-trained (DX) rats, and that the eNOS expression of the DX rats was remarkably higher than that of the DS rats. These results suggested that motor nerve and vascular nerve functions are impaired in diabetic rats, and that exercise can improve this impairment. The effect of the exercise was found to be higher in the CX rats than in the DX rats in terms of MNCV and eNOS expression. In addition, the expression of MNCV and eNOS was higher in the CX rats than in the DX rats. This suggests that medium to high blood glucose reduces vasa nervorum and nerve function in rats (Olver et al., 2014). Therefore, exercise maybe can reverse the deficit of NO synthesis by reversing the reduction of eNOS expression in nerves caused by diabetes, thus partially restoring peripheral nerve dysfunction and reducing pain.
The role of exercise in the dysregulation of voltage-gated calcium channel
Pain related to DNP can be relieved by following strict glycemic control (refer to section “The role of exercise in glucose control and IENF” for details). However, in some cases, strict glycemic control alone does not relieve pain (Calcutt, 2002). Dysregulation of ion channel function is thought to be a cellular mechanism of DNP (Gooch and Podwall, 2004). Among them, VGCCs help in mediating neuropathic pain (Julius and Basbaum, 2001; Zamponi et al., 2009). T-type VGCCs involved in the modulation of neuronal excitability (Todorovic and Jevtovic-Todorovic, 2006) have an important role in DNP (Jagodic et al., 2007). Unlike T-type VGCCs, N-type VGCCs are primarily involved in the release of injurious neurotransmitters like glutamate and substance P, thus propagating the pain. To investigate the mechanisms associated with treatment of DNP by exercise through high- and low voltage-activated Ca2 + channel function in DRG neurons, a DNP rat model was established by Shankarappa et al. (2011) by STZ injection and administration of forced exercise. The results showed that the forced exercise remarkably delayed the tactile hypersensitivity (a semiquantitative behavioral measure of painful neuropathy) in DNP rats, and that this may be due to an analgesic mechanism independent of glycemic control because altering blood glucose levels does not change tactile sensitivity in exercising rats. In addition, this study found that exercise significantly increased the dose of naloxone required to achieve tactile hypersensitivity and attenuated diabetes-related changes in the function of VGCCs. Previous studies have shown that the analgesic mechanism of opioid receptors is related to inhibition of VGCC function (Pettinger et al., 2013). These results suggested that exercise may alter the function of VGCC for analgesic purposes in part by altering opioidergic tone.
Conclusion and outlook
At present, the number of patients with diabetes mellitus is very large, and one-thirds of them present with DNP (Abbott et al., 2011). The pathological mechanism of DNP is still unclear, the above mechanisms have not been fully confirmed, and a large number of RCTs are needed to verify them in the future. Exercise therapy for DNP is receiving increasing attention. Various exercises are effective in improving DNP, including resistance exercise, aerobic exercise, swimming exercise, etc. Naturally, not all exercises have a good analgesic effect. For example, in terms of exercise duration, a short-term exercise may not improve DNP (Cheung et al., 2009); in terms of exercise intensity, a low-intensity exercise has a good analgesic effect (Johnson and Takemoto, 2019). However, excessive exercise intensity reduces IENF, thus leading to severe pain (Do et al., 2020). This review describes the possible mechanisms of exercise in treating DNP through a possible relationship of diabetic neuropathy with the pathological mechanisms of DNP, which provide a basis for future exercise treatment of DNP.
Author contributions
Y-LZ and BG: draft conception, project administration, and funding acquisition. JL, H-QZ, BG, and Y-LZ: writing, reviewing, and editing. All authors contributed to the article and approved the submitted version.
Funding
This work was supported by the Shanghai Key Lab of Human Performance (Shanghai University of Sport) (11DZ2261100), Shanghai Frontiers Science Research Base of Exercise and Metabolic Health, Talent Development Fund of Shanghai Municipal (2021081), Shanghai Clinical Research Center for Rehabilitation Medicine (21MC1930200), and Real-Time Monitoring of Personal Exercise Data and Health Assessment, Special Project of Key R&D Program of Ministry of Science and Technology (2020YFC2006903).
Acknowledgments
We thank all the participants and clinical researchers involved in the publications cited in this review and peer reviewers who contributed to the continuous improvement of this article.
Conflict of interest
The authors declare that the research was conducted in the absence of any commercial or financial relationships that could be construed as a potential conflict of interest.
Publisher’s note
All claims expressed in this article are solely those of the authors and do not necessarily represent those of their affiliated organizations, or those of the publisher, the editors and the reviewers. Any product that may be evaluated in this article, or claim that may be made by its manufacturer, is not guaranteed or endorsed by the publisher.
References
Abbott, C. A., Malik, R. A., van Ross, E. R., Kulkarni, J., and Boulton, A. J. (2011). Prevalence and characteristics of painful diabetic neuropathy in a large community-based diabetic population in the U.K. Diabet. Care 34, 2220–2224. doi: 10.2337/dc11-1108
Aghdam, A. M., Shahabi, P., Karimi-Sales, E., Ghiasi, R., Sadigh-Eteghad, S., Mahmoudi, J., et al. (2018). Swimming exercise induced reversed expression of miR-96 and Its Target Gene NaV1.3 in diabetic peripheral neuropathy in rats. Chin. J. Physiol. 61, 124–129. doi: 10.4077/CJP.2018.BAG531
American Diabetes Association (2018). 4. Lifestyle management: Standards of medical care in diabetes-2018. Diabet. Care 41(Suppl 1), S38–S50. doi: 10.2337/dc18-S004
Bartel, D. P. (2004). MicroRNAs: Genomics, biogenesis, mechanism, and function. Cell 116, 281–297. doi: 10.1016/S0092-8674(04)00045-5
Boric, M., Skopljanac, I., Ferhatovic, L., Jelicic Kadic, A., Banozic, A., and Puljak, L. (2013). Reduced epidermal thickness, nerve degeneration and increased pain-related behavior in rats with diabetes type 1 and 2. J. Chem. Neuroanat. 53, 33–40. doi: 10.1016/j.jchemneu.2013.10.001
Calcutt, N. A. (2002). Potential mechanisms of neuropathic pain in diabetes. Int. Rev. Neurobiol. 50, 205–228. doi: 10.1016/S0074-7742(02)50078-7
Calcutt, N. A. (2020). Diabetic neuropathy and neuropathic pain: A (con)fusion of pathogenic mechanisms? Pain 161(Suppl 1), S65–S86. doi: 10.1097/j.pain.0000000000001922
Callaghan, B. C., Cheng, H. T., Stables, C. L., Smith, A. L., and Feldman, E. L. (2012). Diabetic neuropathy: Clinical manifestations and current treatments. Lancet Neurol. 11, 521–534. doi: 10.1016/S1474-4422(12)70065-0
Callaghan, B. C., Price, R. S., and Feldman, E. L. (2015). Distal symmetric polyneuropathy: A review. JAMA 314, 2172–2181. doi: 10.1001/jama.2015.13611
Chen, H. P., Zhou, W., Kang, L. M., Yan, H., Zhang, L., Xu, B. H., et al. (2014). Intrathecal miR-96 inhibits Nav1.3 expression and alleviates neuropathic pain in rat following chronic construction injury. Neurochem. Res. 39, 76–83. doi: 10.1007/s11064-013-1192-z
Chen, Y. W., Chiu, C. C., Hsieh, P. L., Hung, C. H., and Wang, J. J. (2015). Treadmill training combined with insulin suppresses diabetic nerve pain and cytokines in rat sciatic nerve. Anesth. Analg. 121, 239–246. doi: 10.1213/ANE.0000000000000799
Chen, Y. W., Hsieh, P. L., Chen, Y. C., Hung, C. H., and Cheng, J. T. (2013). Physical exercise induces excess hsp72 expression and delays the development of hyperalgesia and allodynia in painful diabetic neuropathy rats. Anest. Analg. 116, 482–490. doi: 10.1213/ANE.0b013e318274e4a0
Chen, Y. W., Li, Y. T., Chen, Y. C., Li, Z. Y., and Hung, C. H. (2012). Exercise training attenuates neuropathic pain and cytokine expression after chronic constriction injury of rat sciatic nerve. Anesth. Analg. 114, 1330–1337. doi: 10.1213/ANE.0b013e31824c4ed4
Cheng, X., and Ferrell, J. E. Jr. (2018). Apoptosis propagates through the cytoplasm as trigger waves. Science (New York, NY) 361, 607–612. doi: 10.1126/science.aah4065
Cheung, N. W., Cinnadaio, N., Russo, M., and Marek, S. (2009). A pilot randomised controlled trial of resistance exercise bands in the management of sedentary subjects with type 2 diabetes. Diabetes Res. Clin. Pract. 83, e68–e71. doi: 10.1016/j.diabres.2008.12.009
Chiang, S. S., Lee, C. L., Liu, H. C., Wang, J. S., Lee, I. T., Song, Y. M., et al. (2016). Physical activity and albuminuria were associated with painful diabetic polyneuropathy in type 2 diabetes in an ethnic Chinese population. Clin. Chim. Acta Int. J. Clin. Chem. 462, 55–59. doi: 10.1016/j.cca.2016.08.022
Do, J. G., Noh, S. U., Chae, S. W., Yoon, K. J., and Lee, Y. T. (2020). Excessive walking exercise precipitates diabetic neuropathic foot pain: Hind paw suspension treadmill exercise experiment in a rat model. Sci. Rep. 10:10498. doi: 10.1038/s41598-020-67601-6
Duran-Jimenez, B., Dobler, D., Moffatt, S., Rabbani, N., Streuli, C. H., Thornalley, P. J., et al. (2009). Advanced glycation end products in extracellular matrix proteins contribute to the failure of sensory nerve regeneration in diabetes. Diabetes 58, 2893–2903. doi: 10.2337/db09-0320
Feldman, E. L., Callaghan, B. C., Pop-Busui, R., Zochodne, D. W., Wright, D. E., Bennett, D. L., et al. (2019). Diabetic neuropathy. Nat. Rev. Dis. Primers 5:41. doi: 10.1038/s41572-019-0092-1
Galassetti, P. R., Iwanaga, K., Crisostomo, M., Zaldivar, F. P., Larson, J., and Pescatello, A. (2006). Inflammatory cytokine, growth factor and counterregulatory responses to exercise in children with type 1 diabetes and healthy controls. Pediatr. Diabet. 7, 16–24. doi: 10.1111/j.1399-543X.2006.00140.x
Galloway, C., and Chattopadhyay, M. R. E. T. R. A. C. T. E. D. (2013). Increases in inflammatory mediators in DRG implicate in the pathogenesis of painful neuropathy in Type 2 diabetes. Cytokine 63, 1–5. doi: 10.1016/j.cyto.2013.04.009
Goldberg, A., Russell, J. W., and Alexander, N. B. (2008). Standing balance and trunk position sense in impaired glucose tolerance (IGT)-related peripheral neuropathy. J. Neurol. Sci. 270, 165–171. doi: 10.1016/j.jns.2008.03.002
Gooch, C., and Podwall, D. (2004). The diabetic neuropathies. Neurologist 10, 311–322. doi: 10.1097/01.nrl.0000144733.61110.25
Groover, A. L., Ryals, J. M., Guilford, B. L., Wilson, N. M., Christianson, J. A., and Wright, D. E. (2013). Exercise-mediated improvements in painful neuropathy associated with prediabetes in mice. Pain 154, 2658–2667. doi: 10.1016/j.pain.2013.07.052
Hashikawa-Hobara, N., Hashikawa, N., Zamami, Y., Takatori, S., and Kawasaki, H. (2012). The mechanism of calcitonin gene-related peptide-containing nerve innervation. J. Pharmacol. Sci. 119, 117–121. doi: 10.1254/jphs.12R02CP
Heidari, M., Zolaktaf, V., Ghasemi, G., and Nejadian, S. L. (2021). Integrated exercise and glycemic and peripheral sensation control in diabetic neuropathy: A single-blind, randomized controlled trial. Int. J. Prev. Med. 12:169. doi: 10.4103/ijpvm.IJPVM_306_20
Jagodic, M. M., Pathirathna, S., Nelson, M. T., Mancuso, S., Joksovic, P. M., Rosenberg, E. R., et al. (2007). Cell-specific alterations of T-type calcium current in painful diabetic neuropathy enhance excitability of sensory neurons. J. Neurosci. 27, 3305–3316. doi: 10.1523/JNEUROSCI.4866-06.2007
Javed, S., Alam, U., and Malik, R. A. (2015). Treating diabetic neuropathy: Present strategies and emerging solutions. Rev. Diabet. Stud. RDS. 12, 63–83. doi: 10.1900/RDS.2015.12.63
Jensen, T. M., Eriksen, S. B. M., Larsen, J. S., Aadahl, M., Rasmussen, S. S., Olesen, L. B., et al. (2019). Exercise training is associated with reduced pains from the musculoskeletal system in patients with type 2 diabetes. Diabet. Res. Clin. Pract. 154, 124–129.
Johnson, C. E., and Takemoto, J. K. A. (2019). Review of beneficial low-intensity exercises in diabetic peripheral neuropathy patients. J. Pharm. Pharm. Sci. 22, 22–27. doi: 10.18433/jpps30151
Julius, D., and Basbaum, A. I. (2001). Molecular mechanisms of nociception. Nature 413, 203–210. doi: 10.1038/35093019
Kashiba, H., Ueda, Y., and Senba, E. (1996). Coexpression of preprotachykinin-A, alpha-calcitonin gene-related peptide, somatostatin, and neurotrophin receptor family messenger RNAs in rat dorsal root ganglion neurons. Neuroscience 70, 179–189. doi: 10.1016/0306-4522(95)00334-F
Kirk, J. K., Hunter, J. C., Mihalko, S. L., Danhauer, S. C., and Shumaker, S. A. (2019). Perspectives of pain in patients with type 2 diabetes. Exp. Rev. Endocrinol. Metabol. 14, 215–219. doi: 10.1080/17446651.2019.1592674
Kluding, P. M., Pasnoor, M., Singh, R., Jernigan, S., Farmer, K., Rucker, J., et al. (2012). The effect of exercise on neuropathic symptoms, nerve function, and cutaneous innervation in people with diabetic peripheral neuropathy. J. Diabet. Complicat. 26, 424–429. doi: 10.1016/j.jdiacomp.2012.05.007
Lauria, G., Bakkers, M., Schmitz, C., Lombardi, R., Penza, P., Devigili, G., et al. (2010). Intraepidermal nerve fiber density at the distal leg: A worldwide normative reference study. J. Peripher. Nerv. Syst. JPNS. 15, 202–207.
Leinders, M., Üçeyler, N., Thomann, A., and Sommer, C. (2017). Aberrant microRNA expression in patients with painful peripheral neuropathies. J. Neurol. Sci. 380, 242–249. doi: 10.1016/j.jns.2017.07.041
Lemaster, J. W., Mueller, M. J., Reiber, G. E., Mehr, D. R., Madsen, R. W., and Conn, V. S. (2008). Effect of weight-bearing activity on foot ulcer incidence in people with diabetic peripheral neuropathy: Feet first randomized controlled trial. Phys. Ther. 88, 1385–1398. doi: 10.2522/ptj.20080019
Lohmann, H., Siersma, V., and Olivarius, N. F. (2010). Fitness consultations in routine care of patients with type 2 diabetes in general practice: An 18-month non-randomised intervention study. BMC Fam. Pract. 11:83. doi: 10.1186/1471-2296-11-83
Ma, X. Q., Qin, J., Li, H. Y., Yan, X. L., Zhao, Y., and Zhang, L. J. (2019). Role of exercise activity in alleviating neuropathic pain in diabetes via inhibition of the pro-inflammatory signal pathway. Biol. Res. Nurs. 21, 14–21. doi: 10.1177/1099800418803175
Ma, X., Liu, S., Liu, D., Wang, Q., Li, H., and Zhao, Z. (2020). Exercise intervention attenuates neuropathic pain in diabetes via mechanisms of mammalian target of rapamycin (mTOR). Arch. Physiol. Biochem. 126, 41–48. doi: 10.1080/13813455.2018.1489851
Marcovecchio, M. L., Tossavainen, P. H., and Dunger, D. B. (2010). Prevention and treatment of microvascular disease in childhood type 1 diabetes. Bri. Med. Bull. 94, 145–164.
Melese, H., Alamer, A., Hailu Temesgen, M., and Kahsay, G. (2020). Effectiveness of exercise therapy on gait function in diabetic peripheral neuropathy patients: A systematic review of randomized controlled trials. Diabetes Metab. Syndr. Obesity Targets. Ther. 13, 2753–2764. doi: 10.2147/DMSO.S261175
Mrugacz, M., Bryl, A., and Zorena, K. (2021). Retinal vascular endothelial cell dysfunction and neuroretinal degeneration in diabetic patients. J. Clin. Med. 10:458. doi: 10.3390/jcm10030458
Nadi, M., Bambaeichi, E., and Marandi, S. M. (2019). Comparison of the effect of two therapeutic exercises on the inflammatory and physiological conditions and complications of diabetic neuropathy in female patients. Diabetes Metab. Syndr. Obes. 12, 1493–1501. doi: 10.2147/DMSO.S206454
Nascimento, P. S., Lovatel, G. A., Ilha, J., Xavier, L. L., Schaan, B. D., and Achaval, M. (2012). Exercise alleviates hypoalgesia and increases the level of calcitonin gene-related peptide in the dorsal horn of the spinal cord of diabetic rats. Clinics (Sao Paulo, Brazil). 67, 1087–1091. doi: 10.6061/clinics/2012(09)17
Nemet, D., Oh, Y., Kim, H. S., Hill, M., and Cooper, D. M. (2002). Effect of intense exercise on inflammatory cytokines and growth mediators in adolescent boys. Pediatrics 110, 681–689. doi: 10.1542/peds.110.4.681
Ng, A. C. T., Delgado, V., Borlaug, B. A., and Bax, J. J. (2021). Diabesity: The combined burden of obesity and diabetes on heart disease and the role of imaging. Nat. Rev. Cardiol. 18, 291–304. doi: 10.1038/s41569-020-00465-5
Olver, T. D., McDonald, M. W., Grisé, K. N., Dey, A., Allen, M. D., Medeiros, P. J., et al. (2014). Exercise training enhances insulin-stimulated nerve arterial vasodilation in rats with insulin-treated experimental diabetes. Am. J. Physiol. Regul. Integr. Comp. Physiol. 306, R941–R950. doi: 10.1152/ajpregu.00508.2013
Peltier, A., Goutman, S. A., and Callaghan, B. C. (2014). Painful diabetic neuropathy. BMJ (Clin. Res. Ed) 348:G1799.
Peng, M. S., Wang, R., Wang, Y. Z., Chen, C. C., Wang, J., Liu, X. C., et al. (2022). Efficacy of therapeutic aquatic exercise vs physical therapy modalities for patients with chronic low back pain: A randomized clinical trial. JAMA Netw. Open. 5:e2142069. doi: 10.1001/jamanetworkopen.2021.42069
Pepin, E., Higa, A., Schuster-Klein, C., Bernard, C., Sulpice, T., Guardiola, B., et al. (2014). Deletion of apoptosis signal-regulating kinase 1 (ASK1) protects pancreatic beta-cells from stress-induced death but not from glucose homeostasis alterations under pro-inflammatory conditions. PLoS One 9:e112714. doi: 10.1371/journal.pone.0112714
Pettinger, L., Gigout, S., Linley, J. E., and Gamper, N. (2013). Bradykinin controls pool size of sensory neurons expressing functional δ-opioid receptors. J. Neurosci. 33, 10762–10771. doi: 10.1523/JNEUROSCI.0123-13.2013
Pezet, S., and McMahon, S. B. (2006). Neurotrophins: Mediators and modulators of pain. Ann. Rev. Neurosci. 29, 507–538. doi: 10.1146/annurev.neuro.29.051605.112929
Polydefkis, M., Griffin, J. W., and McArthur, J. (2003). New insights into diabetic polyneuropathy. JAMA 290, 1371–1376. doi: 10.1001/jama.290.10.1371
Pop-Busui, R., Ang, L., Holmes, C., Gallagher, K., and Feldman, E. L. (2016). Inflammation as a therapeutic target for diabetic neuropathies. Curr. Diabet. Rep. 16:29.
Pop-Busui, R., Boulton, A. J., Feldman, E. L., Bril, V., Freeman, R., Malik, R. A., et al. (2017). Diabetic neuropathy: A position statement by the American diabetes association. Diabet. Care 40, 136–154. doi: 10.2337/dc16-2042
Rattigan, S., Wallis, M. G., Youd, J. M., and Clark, M. G. (2001). Exercise training improves insulin-mediated capillary recruitment in association with glucose uptake in rat hindlimb. Diabetes 50, 2659–2665. doi: 10.2337/diabetes.50.12.2659
Russell, F. A., King, R., Smillie, S. J., Kodji, X., and Brain, S. D. (2014). Calcitonin gene-related peptide: Physiology and pathophysiology. Physiol. Rev. 94, 1099–1142.
Schaeffer, C., Vandroux, D., Thomassin, L., Athias, P., Rochette, L., and Connat, J. L. (2003). Calcitonin gene-related peptide partly protects cultured smooth muscle cells from apoptosis induced by an oxidative stress via activation of ERK1/2 MAPK. Biochim. Biophys. Acta 1643, 65–73. doi: 10.1016/j.bbamcr.2003.09.005
Shankarappa, S. A., Piedras-Rentería, E. S., and Stubbs, E. B. Jr. (2011). Forced-exercise delays neuropathic pain in experimental diabetes: Effects on voltage-activated calcium channels. J. Neurochem. 118, 224–236. doi: 10.1111/j.1471-4159.2011.07302.x
Shoelson, S. E., Lee, J., and Goldfine, A. B. (2006). Inflammation and insulin resistance. J. Clin. Invest. 116, 1793–1801. doi: 10.1172/JCI29069
Smith, A. G., Ramachandran, P., Tripp, S., and Singleton, J. R. (2001). Epidermal nerve innervation in impaired glucose tolerance and diabetes-associated neuropathy. Neurology 57, 1701–1704. doi: 10.1212/wnl.57.9.1701
Sugimoto, K., Yasujima, M., and Yagihashi, S. (2008). Role of advanced glycation end products in diabetic neuropathy. Curr. Pharm. Des. 14, 953–961.
Tesfaye, S., Boulton, A. J., Dyck, P. J., Freeman, R., Horowitz, M., Kempler, P., et al. (2010). Diabetic neuropathies: Update on definitions, diagnostic criteria, estimation of severity, and treatments. Diabetes Care 33, 2285–2293. doi: 10.2337/dc10-1303
Todorovic, S. M., and Jevtovic-Todorovic, V. (2006). The role of T-type calcium channels in peripheral and central pain processing. CNS Neurol. Disorders Drug Targets 5, 639–653.
Tölle, T., Xu, X., and Sadosky, A. B. (2006). Painful diabetic neuropathy: A cross-sectional survey of health state impairment and treatment patterns. J. Diabet. Complicat. 20, 26–33.
Van Acker, K., Bouhassira, D., De Bacquer, D., Weiss, S., Matthys, K., Raemen, H., et al. (2009). Prevalence and impact on quality of life of peripheral neuropathy with or without neuropathic pain in type 1 and type 2 diabetic patients attending hospital outpatients clinics. Diabet. Metabol. 35, 206–213. doi: 10.1016/j.diabet.2008.11.004
Vincent, A. M., Perrone, L., Sullivan, K. A., Backus, C., Sastry, A. M., Lastoskie, C., et al. (2007). Receptor for advanced glycation end products activation injures primary sensory neurons via oxidative stress. Endocrinology 148, 548–558. doi: 10.1210/en.2006-0073
Wallis, M. G., Wheatley, C. M., Rattigan, S., Barrett, E. J., Clark, A. D., and Clark, M. G. (2002). Insulin-mediated hemodynamic changes are impaired in muscle of Zucker obese rats. Diabetes 51, 3492–3498.
Westermark, P., Wernstedt, C., O’Brien, T. D., Hayden, D. W., and Johnson, K. H. (1987). Islet amyloid in type 2 human diabetes mellitus and adult diabetic cats contains a novel putative polypeptide hormone. Am. J. Pathol. 127, 414–417.
Winter, J., Jung, S., Keller, S., Gregory, R. I., and Diederichs, S. (2009). Many roads to maturity: microRNA biogenesis pathways and their regulation. Nat. cell Biol. 11, 228–234. doi: 10.1038/ncb0309-228
Wu, B., Zhou, L., Chen, C., Wang, J., Hu, L. I., and Wang, X. (2022). Effects of exercise-induced hypoalgesia and its neural mechanisms. Med. Sci. Sports Exerc. 54, 220–231. doi: 10.1249/MSS.0000000000002781
Yamaguchi, K., Takeda, K., Kadowaki, H., Ueda, I., Namba, Y., Ouchi, Y., et al. (2013). Involvement of ASK1-p38 pathway in the pathogenesis of diabetes triggered by pancreatic ß cell exhaustion. Biochim. Biophys. Acta 1830, 3656–3663. doi: 10.1016/j.bbagen.2013.01.029
Yoo, M., D’Silva, L. J., Martin, K., Sharma, N. K., Pasnoor, M., LeMaster, J. W., et al. (2015). Pilot study of exercise therapy on painful diabetic peripheral neuropathy. Pain Med. 16, 1482–1489.
Yoon, H., Thakur, V., Isham, D., Fayad, M., and Chattopadhyay, M. (2015). Moderate exercise training attenuates inflammatory mediators in DRG of Type 1 diabetic rats. Exp. Neurol. 267, 107–114. doi: 10.1016/j.expneurol.2015.03.006
Zamponi, G. W., Lewis, R. J., Todorovic, S. M., Arneric, S. P., and Snutch, T. P. (2009). Role of voltage-gated calcium channels in ascending pain pathways. Brain Res. Rev. 60, 84–89. doi: 10.1016/j.brainresrev.2008.12.021
Keywords: exercise, diabetic neuropathic pain, mechanism, therapy, review
Citation: Zhu H-Q, Luo J, Gou B and Zheng Y-L (2022) Mechanisms of exercise for diabetic neuropathic pain. Front. Aging Neurosci. 14:975453. doi: 10.3389/fnagi.2022.975453
Received: 22 June 2022; Accepted: 08 September 2022;
Published: 12 October 2022.
Edited by:
Dongsheng Xu, Shanghai University of Traditional Chinese Medicine, ChinaReviewed by:
Wenzhan Tu, Second Affiliated Hospital and Yuying Children’s Hospital of Wenzhou Medical University, ChinaChunlong Liu, Guangzhou University of Chinese Medicine, China
Copyright © 2022 Zhu, Luo, Gou and Zheng. This is an open-access article distributed under the terms of the Creative Commons Attribution License (CC BY). The use, distribution or reproduction in other forums is permitted, provided the original author(s) and the copyright owner(s) are credited and that the original publication in this journal is cited, in accordance with accepted academic practice. No use, distribution or reproduction is permitted which does not comply with these terms.
*Correspondence: Bo Gou, 85244025@qq.com; Yi-Li Zheng, zhengyili2008@163.com