- 1Department of Neurological Surgery, Weill Cornell Medicine, New York, NY, United States
- 2Feil Family Brain and Mind Research Institute, Weill Cornell Medicine, New York, NY, United States
- 3Harold and Milliken Hatch Laboratory of Neuroendocrinology, The Rockefeller University, New York, NY, United States
- 4Department of Genetic Medicine, Weill Cornell Medicine, New York, NY, United States
- 5Aligning Science Across Parkinson’s (ASAP) Collaborative Research Network, Chevy Chase, MD, United States
The menopause is a midlife endocrinological process that greatly affects women’s central nervous system functions. Over the last 2 decades numerous clinical studies have addressed the influence of ovarian hormone decline on neurological disorders like Parkinson’s disease and Alzheimer’s disease. However, the findings in support of a role for age at menopause, type of menopause and hormone replacement therapy on Parkinson’s disease onset and its core features show inconsistencies due to the heterogeneity in the study design. Here, we provide a unified overview of the clinical literature on the influence of menopause and ovarian hormones on Parkinson’s disease. We highlight the possible sources of conflicting evidence and gather considerations for future observational clinical studies that aim to explore the neurological impact of menopause-related features in Parkinson’s disease.
Introduction
Parkinson’s disease (PD) is the second most common neurodegenerative disorder, affecting over 6 million people worldwide, and this number is projected to double over the next 20–30 years (Poewe et al., 2017; GBD 2016 Neurology Collaborators, 2018). The disease is characterized primarily by the loss of dopaminergic neurons and presence of Lewy bodies (α-synuclein accumulation within surviving neurons) in the substantia nigra pars compacta (Poewe et al., 2017). The cardinal disease features consist in 4 main motor symptoms: bradykinesia, postural instability, rigidity, and tremor at rest (Poewe et al., 2017; Armstrong and Okun, 2020).
Evidence of a role for sex dimorphism in PD has increased over the last decades, showing that women, compared to men, present a lower PD incidence and prevalence (Wooten, 2004; Bourque et al., 2009; Hirsch et al., 2016; Meoni et al., 2020; Turcano and Savica, 2020), later onset (Haaxma et al., 2007; Meoni et al., 2020), and better motor scores in the Unified PD Rating Scale (UPDRS; Meoni et al., 2020). The influence of biological sex and gonadal hormones on dopamine neurodegeneration (Xing et al., 2017), neuroinflammation (Villa et al., 2016) and oxidative stress (Chainy and Sahoo, 2020) could explain the apparent less susceptibility and milder progression of motor symptoms in women (Cerri et al., 2019). However, how hormones modify PD features in women during menopause and how this compares to men needs further elucidation.
To understand if gonadal hormones play a role in the sexually dimorphic clinical presentation and response to treatment in PD patients (Georgiev et al., 2017), many studies have shown a link with total lifetime exposure to circulating sex hormones (Gatto et al., 2014), reproductive life events that alter ovarian hormone levels, like menopause and pregnancy (Martignoni et al., 2003) and hormone targeted therapies such as use of oral contraceptives (Cartwright et al., 2016) and hormone replacement therapy (HRT; Wang et al., 2015). Altogether, the current clinical literature points toward a trend on a neuroprotective effect of estrogen in PD (Tsang and Ho, 2001); however, inconsistencies between findings have been reported. In this review, we aimed to expand the current understanding of the hormone-PD link observed in patients by discussing the current and most updated clinical evidence. Specifically, we focus on three associations: age at menopause-age at PD onset, type of menopause-PD risk, and HRT use-PD risk. Further, we discuss the possible sources of discrepancies among studies, which meta-analysis studies may not be able to highlight.
Criteria for literature selection
We exhaustively covered the clinical literature, published until July 2021, focusing on the association between menopause and HRT with PD risk using the following key words: Menopause, Early Menopause, Premature Menopause, Perimenopause, Hormone replacement therapy, estrogen, progesterone, AND/OR Parkinson’s disease, Neurodegenerative diseases, in PubMed and MEDLINE databases. The articles included in the main analysis of this review are shown in Table 1. Articles that did not assess the risk of PD or had incomplete data were excluded from the main discussion. Only papers with reported adjusted odds or relative risk ratios (OR/RR) were included in the figures.
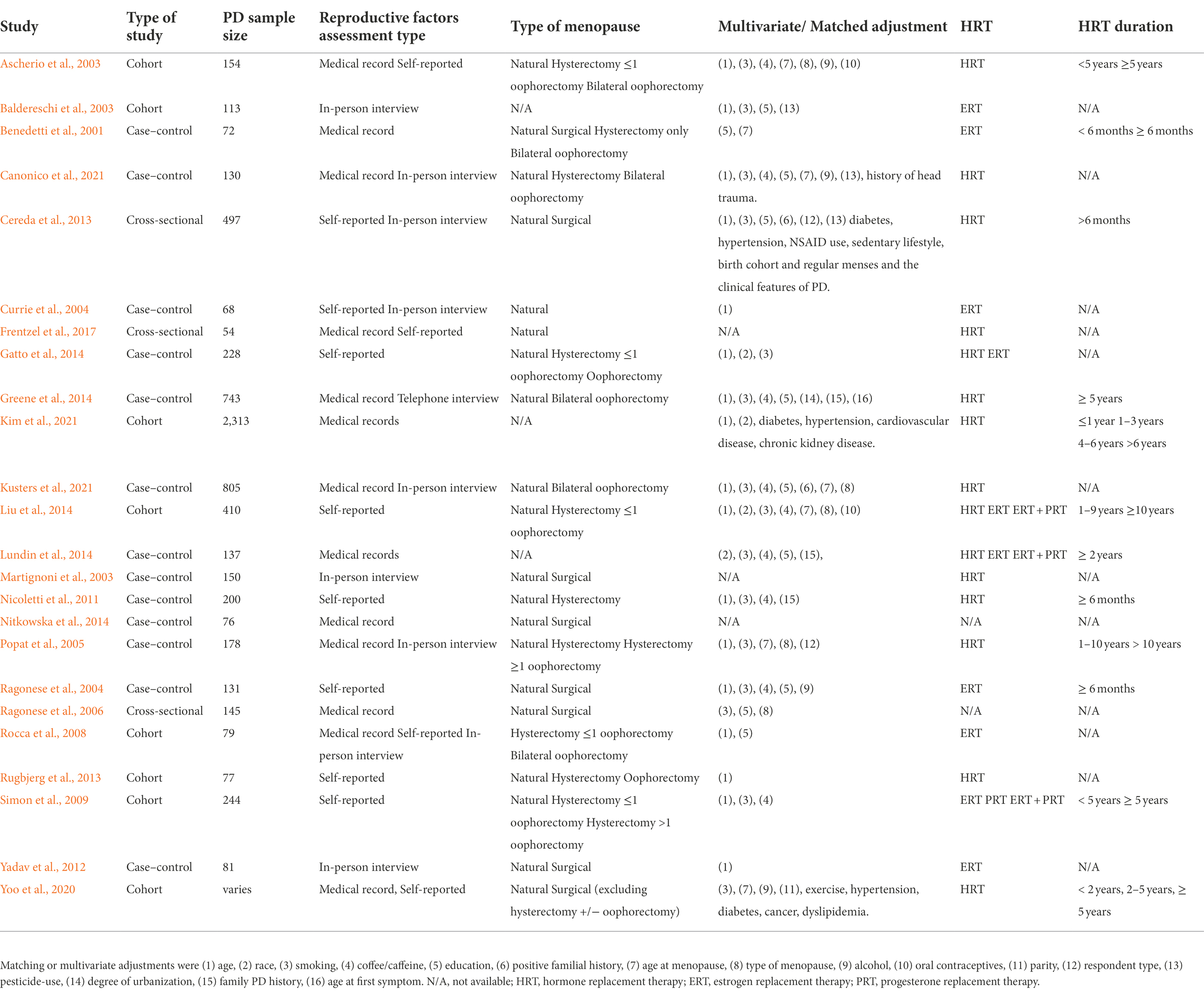
Table 1. Observational studies on menopause and HRT and the association with risk of PD included in the main text.
Age at menopause-age at PD onset and risk of PD
The lower prevalence of PD in women compared to men (Gillies et al., 2014; Picillo et al., 2017; Marras et al., 2018; Meoni et al., 2020) suggests that reproductive life milestones, such as pregnancy, age at menopause, and duration of fertile life may protect women from greater PD deterioration (Martignoni et al., 2002; Miller and Cronin-Golomb, 2010; Gillies et al., 2014), since these determine the cumulative lifetime exposure to endogenous estrogens (Gatto et al., 2014; Yoo et al., 2020). Average age at onset in PD is ~60–65 years old (Poewe et al., 2017). From a clinical perspective, the closest reproductive life event to PD onset is the menopause transition (perimenopause), a period of approximately 5 years affecting women of ~45–55 years old. Perimenopause is typified by erratic fluctuations in hormone levels (Brinton et al., 2015) during a life stage that coincides with the PD prodromal phase (~10–20 years before clinical symptoms emerge). This terminates in a post-menopause stage characterized by low levels of ovarian hormones, estrogen, and progesterone (Harlow et al., 2012). The age at menopause is defined as the age at which a woman has had amenorrhea for 12 months ending the menopausal transition (Harlow et al., 2012). A higher occurrence of PD in post-menopausal versus pre-menopausal women has been reported (Ragonese et al., 2004; Ragonese et al., 2006; Labandeira-Garcia et al., 2016; Lv et al., 2017; Picillo et al., 2017; Jurado-Coronel et al., 2018). Hence, a clear understanding of recommendations, follow-ups, and therapies is needed for physicians treating patients during the perimenopause transition. Nevertheless, current PD treatments follow a one-size-fits-all approach and do not take sex and menopausal stage into account. In the next paragraphs we will highlight the main literature in support of a positive association between menopause and PD risk and age at onset.
We found 16 observational studies that have assessed the association between menopause and PD in the last two decades. 10 case–control, 3 longitudinal cohort and 3 cross-sectional studies (Benedetti et al., 2001; Ragonese et al., 2004; Popat et al., 2005; Ragonese et al., 2006; Nicoletti et al., 2007; Rocca et al., 2008; Simon et al., 2009; Yadav et al., 2012; Cereda et al., 2013; Gatto et al., 2014; Greene et al., 2014; Liu et al., 2014; Nitkowska et al., 2014; Frentzel et al., 2017). 7 case–control studies analyzed the risk of PD in women with early age at menopause (Figure 1). Among these, Benedetti et al. (2001) and Ragonese et al. (2004) have reported higher odds of PD onset in women which reached menopause before 46 years-old. However, the adjusted multiple logistic regression models in these studies did not reach statistical significance. The recent work by Canonico et al. showed a significant association between age at menopause <50 years-old and risk of PD (Canonico et al., 2021). Another report by Nitkowska et al. (2014) showed that while early menopause occurred in only 16% of the control subjects, this number increased to 24% in the PD cohort. Other cohort studies have explored the relationship as well between age at menopause and PD risk. Liu et al. (2014) reported increased odds of PD onset in women with early menopause (<45 years-old). Similarly, Simon et al. (2009) showed a trend toward a decreased risk of PD in women with menopause after 45 years-old, nevertheless these studies did not reach significance. Interestingly, in the cohort study from Rocca et al. (2008), a prominently higher and significant risk of PD was reported in women with premature menopause (<38 years-old) compared to women with early menopause (38–45 years old; Figure 1).
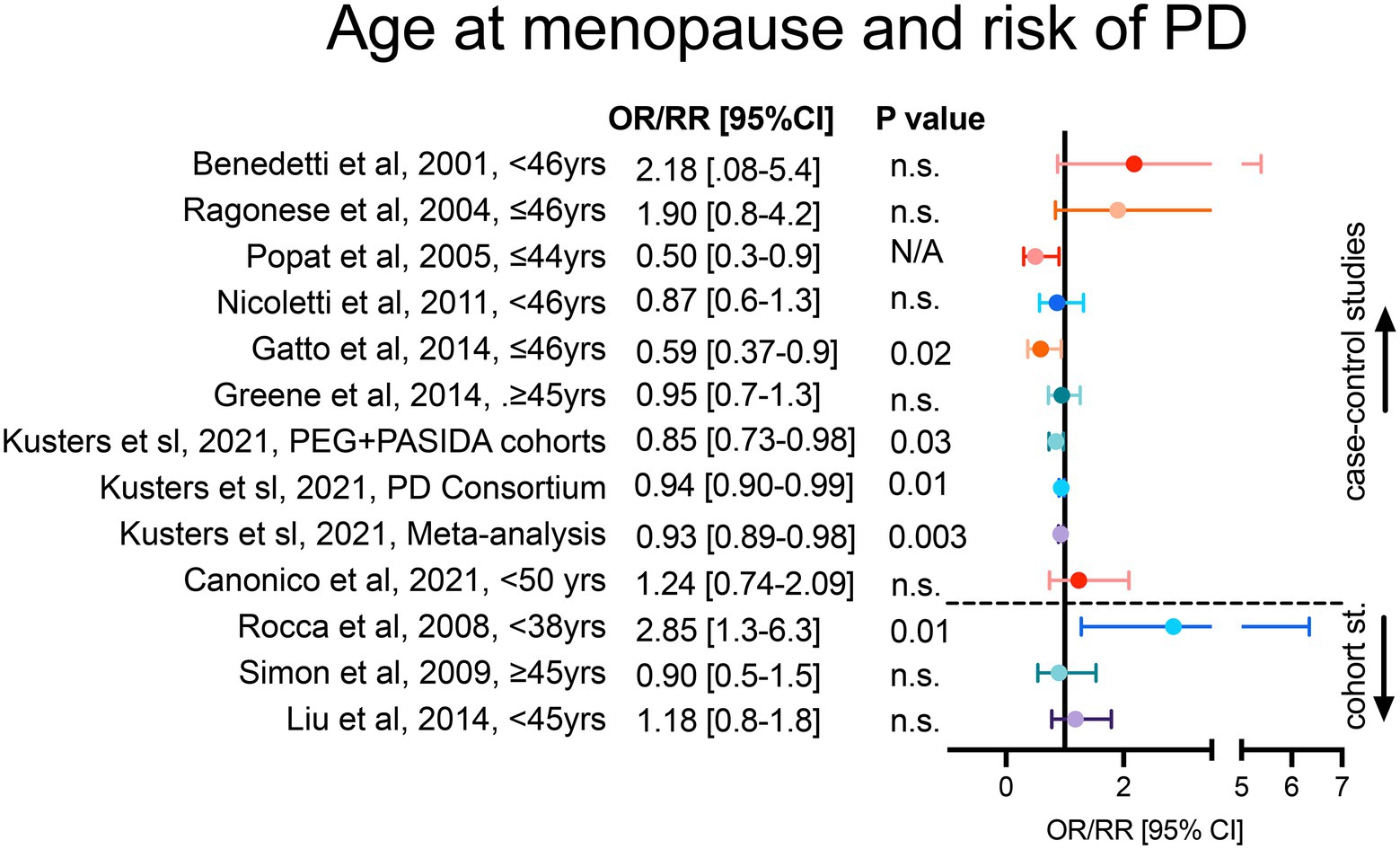
Figure 1. Age at menopause and risk of PD onset. Case–control studies in the upper section and cohort studies in the lower section of the forest plot are represented. For each study, figure reports adjusted OR/RR and 95% CI with level of statistical significance (p value). OR = odds ratio, RR = relative risk, 95% CI = 95% confidence of interval.
In accordance with case–control reports, cohort studies do not always reach statistical significance. Potential points of discrepancy between studies may be the unstandardized research criteria used to classify patients, regression adjustment criteria, and formal representation of the analyses. As an example, the cohort study by Rocca et al. (2008) reported that menopause occurring before 38 years-old is an independent risk factor for PD; however the study analyzed the risk only in women with history of oophorectomy, in contrast to the other 2 mentioned cohort studies by Liu et al. (2014) and Simon et al. (2009), which included patients with natural and surgical menopause. Although these 2 studies were similar (Simon et al., 2009; Liu et al., 2014), the regression analyses were adjusted for different covariates leading to a difficult comparison. Additional support for a significant association between late age at menopause and decrease risk of PD comes from a recent work by Yoo et al., although the data was expressed as a Hazard Ratio rather than OR/RR and patients with history of hysterectomy were excluded from the study (Yoo et al., 2020).
Interestingly, to reduce certain biases common in observational studies, a recent work by Kusters et al. proposed the use of Mendelian randomization (MR) analyses to address the association of menopause age and PD risk (Kusters et al., 2021). The authors applied a MR to identify genetic variants linked to menopause and PD and used the 8 identified single nucleotide polymorphisms as an instrumental variable to demonstrate a significant inversed association between menopause age and risk of PD (Kusters et al., 2021). This suggests that non modifiable factors, such as genetic variants, in concomitance with the menopause might influence the risk of PD.
One case–control (Yadav et al., 2012), and 3 cross-sectional (Ragonese et al., 2006; Cereda et al., 2013; Frentzel et al., 2017) studies have explored the linear association between age at menopause and age at PD onset. The cross-sectional studies conducted by Yadav et al. (2012) and Frentzel et al. (2017) reported a significant positive correlation. Yadav et al. showed a positive correlation between age at menopause and age at PD onset (R = 0.55, p = 0.001), analyzing age-matched PD and healthy females in their case–control study (Yadav et al., 2012). Whereas, Frentzel et al. (2017) analyzed age-matched PD females and males, reporting also a positive correlation between age at menopause and age at PD onset (beta = 0.370, p < 0.01, adjusted R2 = 0.121). Likewise, Ragonese et al. (2006) (beta = 0.25, SE = 0.15, p = 0.003) and Cereda et al. (2013) (Coeff. = 13.03, SE =5.62, p = 0.021). Despite the heterogeneity in sample size and inclusion criteria of these 4 studies, the authors analyzed the association between age at PD onset and age at menopause using numerical variables instead of binary categorizations, which led to more consistent and statistically significant results.
Type of menopause and risk of PD
To better understand how changes in levels of endogenous estrogen in menopausal women are associated with PD, previous works have looked at risk of PD in women with a history of surgical menopause. These studies suggested that abrupt decline of estrogen in women undergoing hysterectomy and uni/bilateral oophorectomy, commonly termed surgical menopause, may lead to a higher risk of PD, compared to women that experience a natural gradual change in estrogen levels during the menopause transition. However, the current evidence on this matter is in part inconsistent. In fact, it has been suggested that perimenopause acts as a neurological transition period, rendering the brain particularly susceptible to neurodegeneration (Brinton et al., 2015). Thus, the debate on whether the risk of PD neurodegeneration is triggered by the abrupt and complete loss of ovarian hormones, in the surgical menopause, or by erratic hormonal fluctuations during a critical window of time, such as the perimenopause, is still very active.
Among the clinical studies that have investigated the role of menopause in PD, case control studies by Benedetti et al. (2001) and Ragonese et al. (2004) have found opposite association between risk of PD onset and surgical menopause, although the type of surgical menopause was not specified. Interestingly, the risk of PD onset seems to be linked to the type of surgical menopause performed. In Canonico et al. a significant association between bilateral oophorectomy, but not hysterectomy, and risk of PD was found (Canonico et al., 2021). The prevalence of bilateral oophorectomy in controls was 9% in comparison to nearly 25% in PD cases. Furthermore, hysterectomy performed before 45 years-old increases the risk of PD onset, as reported by Nicoletti et al. (2011) and Popat et al. (2005) and in the cohort study by Rugbjerg et al. (2013). However, the findings were not significant in adjusted models. Divergent results have been reported regarding hysterectomy combined with unilateral oophorectomy. While some reports (Ascherio et al., 2003; Simon et al., 2009; Gatto et al., 2014; Liu et al., 2014) indicate no association with the risk of PD onset, studies by Benedetti et al. (2001) and Rocca et al. (2008), reported a significant risk of PD onset (up to three-fold higher). Instead, hysterectomy combined with bilateral oophorectomy has shown a protective effect in most case control and cohort studies (Ascherio et al., 2003; Popat et al., 2005; Simon et al., 2009; Rugbjerg et al., 2013; Gatto et al., 2014; Greene et al., 2014), with the exception of Rocca et al. (2008). Altogether, these studies point towards an increased risk of PD onset when hysterectomy is combined with unilateral oophorectomy and, at younger age (Figures 2A,B).
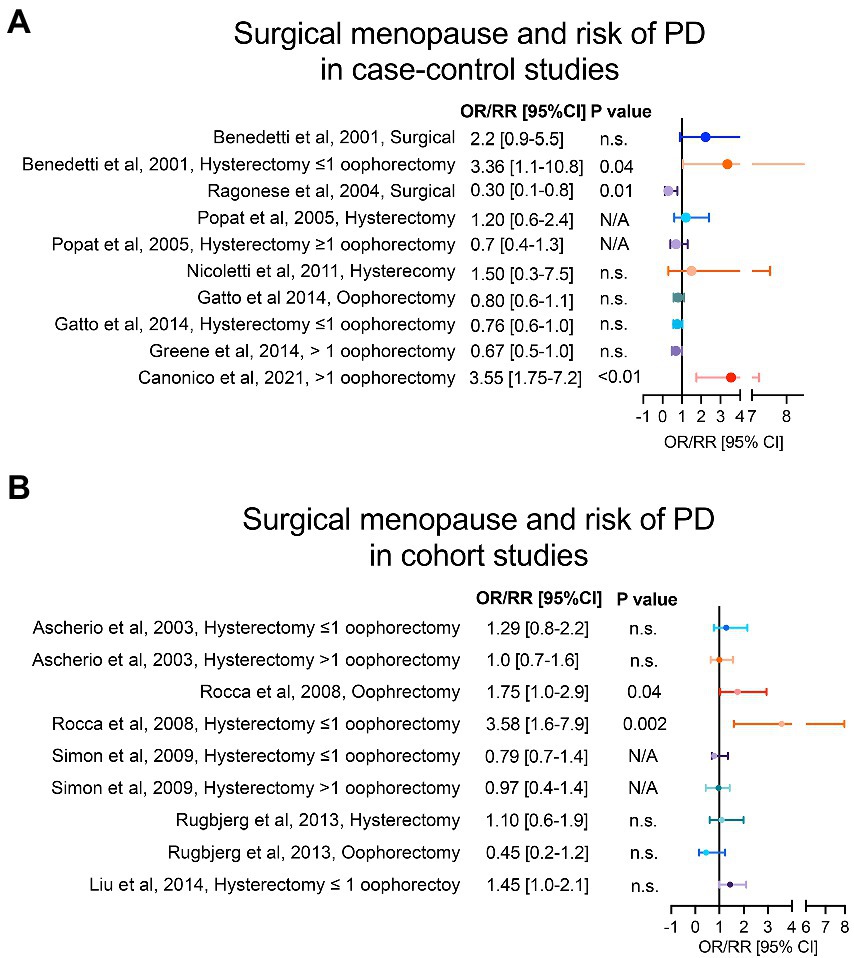
Figure 2. Type of menopause and risk of PD. Panel (A) shows case–control studies and panel (B) cohort studies that have analysed the risk of PD in women with surgical menopause compared to women with natural menopause. For each study, we reported the adjusted OR/RR and 95% CI with level of statistical significance (p value). OR = odds ratio, RR = relative risk, 95% CI = 95% confidence of interval.
Despite the studies mentioned above suggest an increased risk of PD in women who underwent surgical menopause, compared to those who experienced a physiological menopause, the evidence about type of menopause (i.e., natural vs. surgical) and its relation to the risk of PD onset remains conflicting. The source of inconsistent findings between these studies might be related to important confounding factors. Particularly, the underlying condition that prompts the surgical indication of hysterectomy and/or oophorectomy, and the medical management of the different type of menopause. The most common indications for hysterectomy are leiomyoma and abnormal uterine bleeding, known to be deeply related to progesterone and estrogen abnormalities (Vilos et al., 2015; Jewson et al., 2020). Hence, even though both ovaries are preserved in this surgical procedure, this is preceded by hormonal dysfunctions (Torrealday et al., 2017). When bilateral oophorectomy is performed, with or without uterus resection, women commonly receive preventive exogenous gonadal hormones (Domchek and Rebbeck, 2007). This may be a confounding factor when evaluating the association between bilateral oophorectomy and risk of PD onset and may explain the protective trend observed in some studies. Regarding the increased risk found for hysterectomy combined with unilateral oophorectomy, previous evidence has shown ovarian failure in the contralateral ovary following unilateral oophorectomy (Farquhar et al., 2005), paralleled by a loss of blood supply to the remaining ovary due to the uterus resection (Ahn et al., 2002). Furthermore, although higher risk of early ovarian failure has been reported in patients with history of unilateral oophorectomy (Rosendahl et al., 2017), most of these women do not receive HRT (Read et al., 2010). Finally, it’s worth mentioning that a possible age-dependent effect, found in linear trend analyses of age at surgical menopause and risk of PD onset (Rocca et al., 2008), adds more variability to the mentioned findings. This suggests that age stratification should be analyzed in more depth in future studies.
Hormone replacement therapy and risk of PD
The molecular weight of endogenous gonadal hormones allows easy diffusion across the blood–brain barrier (Diotel et al., 2018). Likewise for exogenous steroids (Cipolla et al., 2009), although their preventive or detrimental potential on the neurons remains unclear (Simpkins et al., 2005). Exogenous steroids, also known as HRT, are commonly prescribed to women to relieve menopausal symptoms (Valdes and Bajaj, 2020). Conventional HRT includes both estrogen and progesterone hormones with various formulations and exerting different specificity of effects on the gonadal-brain axis (Schipper, 2016; Del Rio et al., 2018).
Several studies have explored the role of HRT on the risk of PD onset. A trend toward an increased risk of PD onset in women that received HRT, without distinction of formulation type, was observed in case–control and cohort studies (Ascherio et al., 2003; Martignoni et al., 2003; Popat et al., 2005; Nicoletti et al., 2007; Simon et al., 2009; Rugbjerg et al., 2013; Greene et al., 2014; Liu et al., 2014), as shown in Figures 3A,B. However, only in the study from Gatto et al. (2014) the results reached statistical significance in adjusted models. A more detailed assessment of the HRT formulations evidences a modest increased risk of PD in users of estrogen replacement therapy (ERT) in 2 cohort studies (Simon et al., 2009; Liu et al., 2014) in contrast to 6 case–control studies (Benedetti et al., 2001; Baldereschi et al., 2003; Currie et al., 2004; Ragonese et al., 2004; Gatto et al., 2014; Lundin et al., 2014) that showed a trend for a protective or no effect of ERT. The discrepancies between cohort and case–control studies may be explained by lack of analyses regarding the age at therapy initiation, type or stage of menopause, as well as the ERT subtype. Importantly, the combination of ERT with progesterone or progesterone-like replacement therapy (PRT) in Liu et al. (2014) and Lundin et al. (2014) showed a significant increased risk of PD onset. Moreover, Simon et al. (2009) reported higher odds of PD risk in a small sample of women receiving PRT alone compared to women receiving ERT alone or combined therapy.
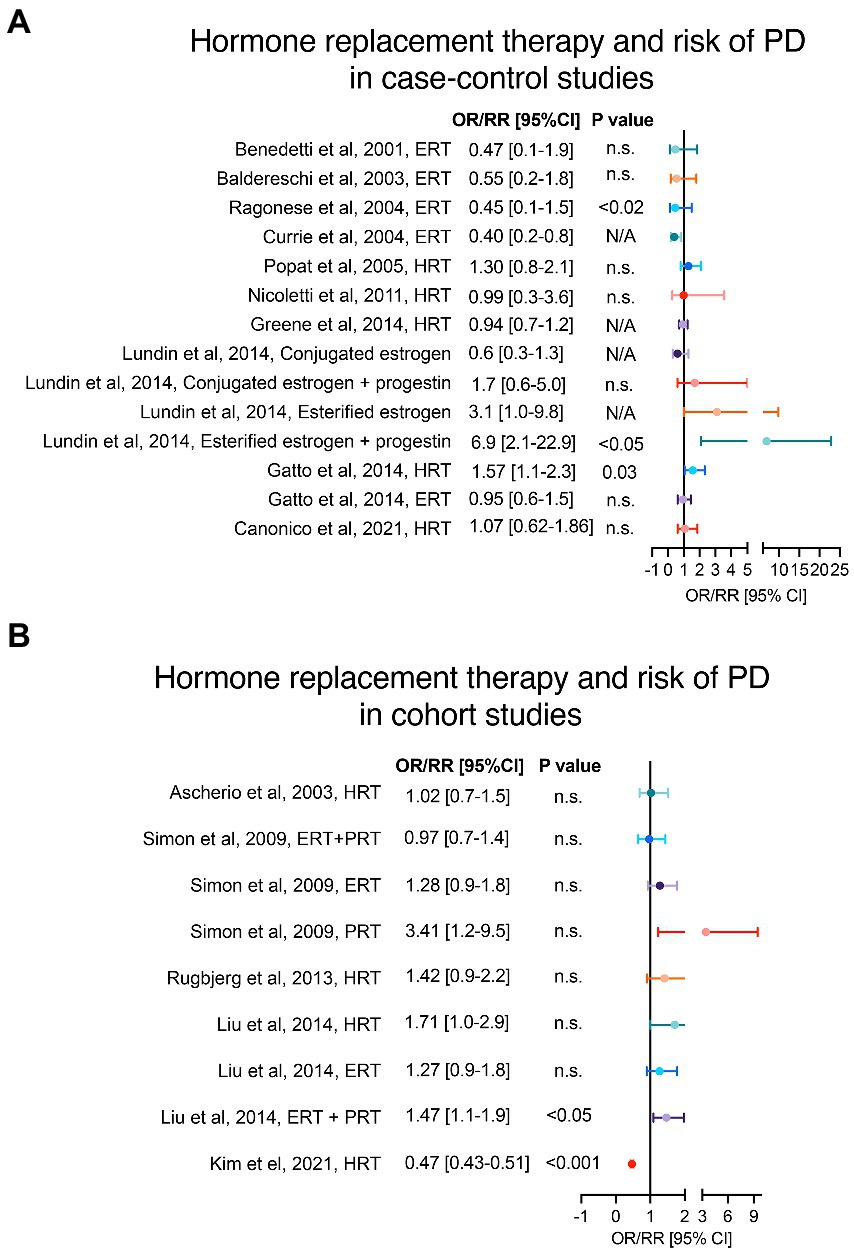
Figure 3. Hormone replacement therapy and risk of PD. Panels (A,B) show case–control and cohort studies, respectively, that have analysed the risk of PD in women with history of HRT. Adjusted OR/RR and 95% CI with level of statistical significance (p value). OR = odds ratio, RR = relative risk, 95% CI = 95% confidence of interval, HRT = hormone replacement therapy, ERT = estrogen replacement therapy, PRT = progesterone replacement therapy.
In support of this, a recent retrospective analysis by Kim et al. showed that sources of discrepancy in the effect of HRT on different neurodegenerative disorders, including PD, may be related to route and duration of HRT administration (Kim et al., 2021), whereas a significantly reduced relative risk (RR) of PD was reported in women taking oral, but not transdermal, therapy. Additionally, this work supports the importance of including large sample sizes in this type of studies (Kim et al., 2021).
Regarding the ERT findings, it is important to highlight that there are two common formulations: the esterified estrogens and the conjugated estrogens. The esterified estrogens are predominantly estrone, whereas conjugated estrogens are a mixture of more biologically active estrogens (like 17b-estradiol) with greater affinity for estrogen receptors than estrone (Lundin et al., 2014); nevertheless, these two formulations share the same FDA indications (Harper-Harrison and Shanahan, 2020). Lundin et al. (2014) showed that the trend toward an increased or decreased risk of PD onset is inverse depending on whether esterified or conjugated formulations are administered. Another interesting observation in the Benedetti et al. (2001) study was the opposite ERT contribution to PD in women depending on the menopausal type (i.e., natural vs. surgical). This association was supported by a similar observation in the study by Popat et al. (2005) in which women with natural menopause that received HRT had lower odds of developing PD, while women with oophorectomy plus hysterectomy on HRT had higher risk of PD onset. Additionally, studies on dementia and Alzheimer’s disease, like the one by Whitmer et al. (2011), support the “window of opportunity hypothesis” that the use of HRT in midlife (before or during early menopause) only may be neuroprotective, whereas HRT initiation in late life could have deleterious effects and worsen the neurodegenerative processes (Marras and Saunders-Pullman, 2014; Kim and Brinton, 2021). Thus, we suggest that a stratification analysis of age at HRT initiation may clarify discrepancies seen in previous clinical observational studies and, moreover, could shed lights on possible age/time-dependent mechanisms of hormones in the central nervous system.
Although the publication of the Women’s Health Initiative data in 2002 supported that HRT increases the risk of stroke and breast cancer (Rossouw et al., 2002), a recent national survey study reported that 37% of women are current or former HRT users (Gass et al., 2015). Therefore, a rigorous assessment of the HRT doses and different formulations in regard to the type of menopause, age of menopause, duration of HRT, medical indication of HRT, and other factors that can interact with HRT, such as caffeine consumption (Kim et al., 2017), is needed to improve recommendations for women in menopause. Even more, evidence from some of the mentioned observational studies regarding HRT suggests that estrogens may not be the only gonadal hormones capable of affecting the course of PD. Hence, the role of progesterone in influencing the central nervous system directly, through its neuronal receptors, or indirectly, through its action on the peripheral systems, requires further elucidation (Bourque et al., 2019; Cardia et al., 2019; Jarras et al., 2020; Kim et al., 2021).
In Lundin et al. the type of progestin used was the synthetic progesterone formulation medroxy-progesterone acetate (MPA), whereas this was not specified in Liu et al. and Simon et al (Simon et al., 2009; Liu et al., 2014; Lundin et al., 2014). Pre-clinical cell and animal models have shown progesterone to be neuroprotective, but not MPA (Singh and Su, 2013). Preclinical data suggest that progesterone may be neuroprotective in PD by increasing dopaminergic neurotransmission, exerting anti-inflammatory activity, and modulating several other neurotransmitter systems (including glutamatergic, GABAergic, norepinephrine, serotonin, and acetylcholine; Callier et al., 2001; Kritzer et al., 2003; Casas et al., 2013; Barth et al., 2015; Litim et al., 2017). Differently from endogenous progesterone, MPA is detrimental to neurons as it can induce glutamate toxicity and counteract the neuroprotective and neurotrophic effects of 17beta-estradiol (E2; Nilsen et al., 2006; Singh and Su, 2013). This is of particular importance as MPA is often the progestin used in HRT (Kim et al., 2021) and could therefore explain the trend towards increased risk of PD observed in the 3 abovementioned studies (Simon et al., 2009; Liu et al., 2014; Lundin et al., 2014).
Future perspectives and conclusions
Throughout this review, we have highlighted that within the same type of studies the conflicting evidence underlines the different methods of data collection, patient’s classification, and regression models. Similarly, in different types of observational studies the discrepancies may relate to bias in the population inclusion criteria and sample size. Our work emphasizes the importance of considering a uniform standard criterion to adjust regression models with a consistent statistical and clinical judgment. We believe that despite some inconsistent results, the current findings support a role for menopause on the risk of PD onset. This is an exciting research field for scientists working in basic, pre-clinical and clinical sciences aiming to elucidate the underlying mechanisms in PD and promoting better strategies to manage menopausal patients accordingly to their risk profile.
The effect of gonadal steroids on the brain dopamine system has been the subject of numerous pre-clinical publications in the past several decades. Pre-clinical studies have thus far led the way to elucidate the effect of gonadal steroids more consistently on dopamine containing neurons in wild type and parkinsonian animals, especially in toxin-based rodent models of PD (Dluzen et al., 1996; Miller et al., 1998; Grandbois et al., 2000; Quinlan et al., 2013; Gillies et al., 2014; Smith and Dahodwala, 2014; Almey et al., 2015; Rodriguez-Perez et al., 2015; Almey et al., 2016; Labandeira-Garcia et al., 2016; Jurado-Coronel et al., 2018). Several works support the hypothesis that menopause may constitute a triggering risk factor, which interaction with other risk factors and other possible pathological processes may modify the onset of PD. For instance, pre-clinical studies using rotenone and MPTP toxin-induced animal models of PD showed that ovariectomy abolishes the neuroprotective advantage observed in the substantia nigra and striatum of females as compared to males with PD. Conversely, treatment with estrogen reduces dopaminergic neurodegeneration in the substantia nigra and restores dopaminergic transmission (Disshon and Dluzen, 2000; Mitra et al., 2015; Shen et al., 2017; Makav and Eroglu, 2021). Similarly, clinical studies are starting to elucidate the combined effect of menopause with other PD risk factors. Among postmenopausal women, sleep disturbances were associated with approximately 10–30% increased PD risk after ∼16 years follow-up; although prospective cohort studies that include both men and women of diverse backgrounds are required to confirm these findings (Beydoun et al., 2022).
Other nuances important to mention include the notion that, as suggested by the considerable number of studies reviewed in this article, the physiological changes and pathological mechanisms involved in PD neurodegeneration may adapt distinctively at different stages of the menopause process. Moreover, recent studies have approached the concept that perimenopause does not equal the simple loss of estrogen, but that it represents a period of gonadal endocrine imbalance and neurological transition during which the nigro-striatal circuit is more susceptible to PD neurodegeneration (Brinton et al., 2015).
Author contributions
SU, SM, RM, and TM: conceptualization, methodology, resources, investigation, writing—draft preparation, review, and editing. RM and TM: funding acquisition. RM: project administration. All authors contributed to the article and approved the submitted version.
Funding
This research was funded in whole or in part by the Aligning Science Across Parkinson’s (ASAP-020608; RM) through the Michael J. Fox Foundation for Parkinson’s Research (MJFF), the American Parkinson’s Disease Association (APDA 190167-01; RM), and the National Institute of Health grants R21AG064455 (RM), HL098351 (TM), and DA08259 (TM).
Conflict of interest
The authors declare that the research was conducted in the absence of any commercial or financial relationships that could be construed as a potential conflict of interest.
Publisher’s note
All claims expressed in this article are solely those of the authors and do not necessarily represent those of their affiliated organizations, or those of the publisher, the editors and the reviewers. Any product that may be evaluated in this article, or claim that may be made by its manufacturer, is not guaranteed or endorsed by the publisher.
References
Ahn, E. H., Bai, S. W., Song, C. H., Kim, J. Y., Jeong, K. A., Kim, S. K., et al. (2002). Effect of hysterectomy on conserved ovarian function. Yonsei Med. J. 43, 53–58. doi: 10.3349/ymj.2002.43.1.53
Almey, A., Milner, T. A., and Brake, W. G. (2015). Estrogen receptors in the central nervous system and their implication for dopamine-dependent cognition in females. Horm. Behav. 74, 125–138. doi: 10.1016/j.yhbeh.2015.06.010
Almey, A., Milner, T. A., and Brake, W. G. (2016). Estrogen receptor alpha and G-protein coupled estrogen receptor 1 are localized to GABAergic neurons in the dorsal striatum. Neurosci. Lett. 622, 118–123. doi: 10.1016/j.neulet.2016.04.023
Armstrong, M. J., and Okun, M. S. (2020). Diagnosis and treatment of Parkinson disease: a review. JAMA 323, 548–560. doi: 10.1001/jama.2019.22360
Ascherio, A., Chen, H., Schwarzschild, M. A., Zhang, S. M., Colditz, G. A., and Speizer, F. E. (2003). Caffeine, postmenopausal estrogen, and risk of Parkinson's disease. Neurology 60:790. doi: 10.1212/01.WNL.0000046523.05125.87
Baldereschi, M., di Carlo, A., Vanni, P., Ghetti, A., Carbonin, P., Amaducci, L., et al. (2003). Lifestyle-related risk factors for Parkinson’s disease: a population-based study. Acta Neurol. Scand. 108, 239–244. doi: 10.1034/j.1600-0404.2003.00128.x
Barth, C., Villringer, A., and Sacher, J. (2015). Sex hormones affect neurotransmitters and shape the adult female brain during hormonal transition periods. Front. Neurosci. 9:37. doi: 10.3389/fnins.2015.00037
Benedetti, M. D., Maraganore, D. M., Bower, J. H., McDonnell, S. K., Peterson, B. J., Ahlskog, J. E., et al. (2001). Hysterectomy, menopause, and estrogen use preceding Parkinson's disease: an exploratory case-control study. Mov. Disord. 16, 830–837. doi: 10.1002/mds.1170
Beydoun, H. A., Naughton, M. J., Beydoun, M. A., Shadyab, A. H., Brunner, R. L., Chen, J. C., et al. (2022). Association of sleep disturbance with Parkinson disease: evidence from the Women's Health Initiative. Menopause 29, 255–263. doi: 10.1097/GME.0000000000001918
Bourque, M., Dluzen, D. E., and Di Paolo, T. (2009). Neuroprotective actions of sex steroids in Parkinson's disease. Front. Neuroendocrinol. 30, 142–157. doi: 10.1016/j.yfrne.2009.04.014
Bourque, M., Morissette, M., and Di Paolo, T. (2019). Repurposing sex steroids and related drugs as potential treatment for Parkinson's disease. Neuropharmacology 147, 37–54. doi: 10.1016/j.neuropharm.2018.04.005
Brinton, R. D., Yao, J., Yin, F., Mack, W. J., and Cadenas, E. (2015). Perimenopause as a neurological transition state. Nat. Rev. Endocrinol. 11, 393–405. doi: 10.1038/nrendo.2015.82
Callier, S., Morissette, M., Grandbois, M., Pélaprat, D., and di Paolo, T. (2001). Neuroprotective properties of 17beta-estradiol, progesterone, and raloxifene in MPTP C57Bl/6 mice. Synapse 41, 131–138. doi: 10.1002/syn.1067
Canonico, M., Pesce, G., Bonaventure, A., le Noan-Lainé, M., Benatru, I., Ranoux, D., et al. (2021). Increased risk of Parkinson's disease in women after bilateral oophorectomy. Mov. Disord. 36, 1696–1700. doi: 10.1002/mds.28563
Cardia, M. C., Carta, A. R., Caboni, P., Maccioni, A. M., Erbì, S., Boi, L., et al. (2019). Trimethyl chitosan hydrogel nanoparticles for progesterone delivery in neurodegenerative disorders. Pharmaceutics 11:657. doi: 10.3390/pharmaceutics11120657
Cartwright, B., Robinson, J., Seed, P. T., Fogelman, I., and Rymer, J. (2016). Hormone replacement therapy versus the combined Oral contraceptive pill in premature ovarian failure: a randomized controlled trial of the effects on bone mineral density. J. Clin. Endocrinol. Metab. 101, 3497–3505. doi: 10.1210/jc.2015-4063
Casas, S., Giuliani, F., Cremaschi, F., Yunes, R., and Cabrera, R. (2013). Neuromodulatory effect of progesterone on the dopaminergic, glutamatergic, and GABAergic activities in a male rat model of Parkinson's disease. Neurol. Res. 35, 719–725. doi: 10.1179/1743132812Y.0000000142
Cereda, E., Barichella, M., Cassani, E., Caccialanza, R., and Pezzoli, G. (2013). Reproductive factors and clinical features of Parkinson's disease. Parkinsonism Relat. Disord. 19, 1094–1099. doi: 10.1016/j.parkreldis.2013.07.020
Cerri, S., Mus, L., and Blandini, F. (2019). Parkinson’s disease in women and men: What's the difference? J. Parkinsons Dis. 9, 501–515. doi: 10.3233/JPD-191683
Chainy, G. B. N., and Sahoo, D. K. (2020). Hormones and oxidative stress: an overview. Free Radic. Res. 54, 1–26. doi: 10.1080/10715762.2019.1702656
Cipolla, M. J., Godfrey, J. A., and Wiegman, M. J. (2009). The effect of ovariectomy and estrogen on penetrating brain arterioles and blood-brain barrier permeability. Microcirculation 16, 685–693. doi: 10.3109/10739680903164131
Currie, L. J., Harrison, M. B., Trugman, J. M., Bennett, J. P., and Wooten, G. F. (2004). Postmenopausal estrogen use affects risk for Parkinson disease. Arch. Neurol. 61, 886–888. doi: 10.1001/archneur.61.6.886
Del Rio, J. P., Alliende, M. I., Molina, N., Serrano, F. G., Molina, S., and Vigil, P. (2018). Steroid hormones and their action in Women's brains: the importance of hormonal balance. Front. Public Health 6:141. doi: 10.3389/fpubh.2018.00141
Diotel, N., Charlier, T. D., Lefebvre d’Hellencourt, C., Couret, D., Trudeau, V. L., Nicolau, J. C., et al. (2018). Steroid transport, local synthesis, and signaling within the brain: roles in neurogenesis, neuroprotection, and sexual behaviors. Front. Neurosci. 12:84. doi: 10.3389/fnins.2018.00084
Disshon, K. A., and Dluzen, D. E. (2000). Estrogen reduces acute striatal dopamine responses in vivo to the neurotoxin MPP+ in female, but not male rats. Brain Res. 868, 95–104. doi: 10.1016/S0006-8993(00)02329-5
Dluzen, D. E., McDermott, J. L., and Liu, B. (1996). Estrogen as a neuroprotectant against MPTP-induced neurotoxicity in C57/B1 mice. Neurotoxicol. Teratol. 18, 603–606. doi: 10.1016/0892-0362(96)00086-4
Domchek, S. M., and Rebbeck, T. R. (2007). Prophylactic oophorectomy in women at increased cancer risk. Curr. Opin. Obstet. Gynecol. 19, 27–30. doi: 10.1097/GCO.0b013e32801195da
Farquhar, C. M., Sadler, L., Harvey, S. A., and Stewart, A. W. (2005). The association of hysterectomy and menopause: a prospective cohort study. BJOG 112, 956–962. doi: 10.1111/j.1471-0528.2005.00696.x
Frentzel, D., Judanin, G., Borozdina, O., Klucken, J., Winkler, J., and Schlachetzki, J. C. M. (2017). Increase of reproductive life span delays age of onset of Parkinson's disease. Front. Neurol. 8:397. doi: 10.3389/fneur.2017.00397
Gass, M. L., Stuenkel, C. A., Utian, W. H., LaCroix, A., Liu, J. H., Shifren, J. L., et al. (2015). Use of compounded hormone therapy in the United States: report of the North American Menopause Society survey. Menopause 22, 1276–1285. doi: 10.1097/GME.0000000000000553
Gatto, N. M., Deapen, D., Stoyanoff, S., Pinder, R., Narayan, S., Bordelon, Y., et al. (2014). Lifetime exposure to estrogens and Parkinson's disease in California teachers. Parkinsonism Relat. Disord. 20, 1149–1156. doi: 10.1016/j.parkreldis.2014.08.003
GBD 2016 Neurology Collaborators (2018). Global, regional, and national burden of Parkinson's disease, 1990-2016: a systematic analysis for the global burden of disease study 2016. Lancet Neurol. 17, 939–953. doi: 10.1016/S1474-4422(18)30499-X
Georgiev, D., Hamberg, K., Hariz, M., Forsgren, L., and Hariz, G. M. (2017). Gender differences in Parkinson's disease: a clinical perspective. Acta Neurol. Scand. 136, 570–584. doi: 10.1111/ane.12796
Gillies, G. E., Pienaar, I. S., Vohra, S., and Qamhawi, Z. (2014). Sex differences in Parkinson's disease. Front. Neuroendocrinol. 35, 370–384. doi: 10.1016/j.yfrne.2014.02.002
Grandbois, M., Morissette, M., Callier, S., and di Paolo, T. (2000). Ovarian steroids and raloxifene prevent MPTP-induced dopamine depletion in mice. NeuroReport 11, 343–346. doi: 10.1097/00001756-200002070-00024
Greene, N., Lassen, C. F., Rugbjerg, K., and Ritz, B. (2014). Reproductive factors and Parkinson's disease risk in Danish women. Eur. J. Neurol. 21, 1168–1e68. doi: 10.1111/ene.12450
Haaxma, C. A., Bloem, B. R., Borm, G. F., Oyen, W. J. G., Leenders, K. L., Eshuis, S., et al. (2007). Gender differences in Parkinson's disease. J. Neurol. Neurosurg. Psychiatry 78, 819–824. doi: 10.1136/jnnp.2006.103788
Harlow, S. D., Gass, M., Hall, J. E., Lobo, R., Maki, P., Rebar, R. W., et al. (2012). Executive summary of the stages of reproductive aging workshop + 10: addressing the unfinished agenda of staging reproductive aging. J. Clin. Endocrinol. Metab. 97, 1159–1168. doi: 10.1210/jc.2011-3362
Harlow, S. D., Gass, M., Hall, J. E., Lobo, R., Maki, P., Rebar, R. W., et al. (2012). Executive summary of the stages of reproductive aging workshop + 10: addressing the unfinished agenda of staging reproductive aging. Menopause 19, 387–395. doi: 10.1097/gme.0b013e31824d8f40
Harper-Harrison, G., and Shanahan, M. M., Hormone replacement therapy, in stat pearls. 2020: Treasure Island (FL).
Hirsch, L., Jette, N., Frolkis, A., Steeves, T., and Pringsheim, T. (2016). The incidence of Parkinson’s disease: a systematic review and meta-analysis. Neuroepidemiology 46, 292–300. doi: 10.1159/000445751
Jarras, H., Bourque, M., Poirier, A. A., Morissette, M., Coulombe, K., di Paolo, T., et al. (2020). Neuroprotection and immunomodulation of progesterone in the gut of a mouse model of Parkinson's disease. J. Neuroendocrinol. 32:e12782. doi: 10.1111/jne.12782
Jewson, M., Purohit, P., and Lumsden, M. A. (2020). Progesterone and abnormal uterine bleeding/menstrual disorders. Best Pract. Res. Clin. Obstet. Gynaecol. 69, 62–73. doi: 10.1016/j.bpobgyn.2020.05.004
Jurado-Coronel, J. C., Cabezas, R., Ávila Rodríguez, M. F., Echeverria, V., García-Segura, L. M., and Barreto, G. E. (2018). Sex differences in Parkinson's disease: features on clinical symptoms, treatment outcome, sexual hormones and genetics. Front. Neuroendocrinol. 50, 18–30. doi: 10.1016/j.yfrne.2017.09.002
Kim, Y. J., and Brinton, R. D. (2021). Precision hormone therapy: identification of positive responders. Climacteric 24, 350–358. doi: 10.1080/13697137.2021.1882418
Kim, I. Y., O’Reilly, É. J., Hughes, K. C., Gao, X., Schwarzschild, M. A., and Ascherio, A. (2017). Differences in Parkinson's disease risk with caffeine intake and postmenopausal hormone use. J. Parkinsons Dis. 7, 677–684. doi: 10.3233/JPD-171175
Kim, Y. J., Soto, M., Branigan, G. L., Rodgers, K., and Brinton, R. D. (2021). Association between menopausal hormone therapy and risk of neurodegenerative diseases: implications for precision hormone therapy. Alzheimers Dement 7:e12174. doi: 10.1002/trc2.12174
Kritzer, M. F., Adler, A., and Bethea, C. L. (2003). Ovarian hormone influences on the density of immunoreactivity for tyrosine hydroxylase and serotonin in the primate corpus striatum. Neuroscience 122, 757–772. doi: 10.1016/S0306-4522(03)00548-7
Kusters, C. D. J., Paul, K. C., Duarte Folle, A., Keener, A. M., Bronstein, J. M., Bertram, L., et al. (2021). Increased menopausal age reduces the risk of Parkinson's disease: a Mendelian randomization approach. Mov. Disord. 36, 2264–2272. doi: 10.1002/mds.28760
Labandeira-Garcia, J. L., Rodriguez-Perez, A. I., Valenzuela, R., Costa-Besada, M. A., and Guerra, M. J. (2016). Menopause and Parkinson’s disease. Interaction between estrogens and brain renin-angiotensin system in dopaminergic degeneration. Front. Neuroendocrinol. 43, 44–59. doi: 10.1016/j.yfrne.2016.09.003
Litim, N., Morissette, M., and Di Paolo, T. (2017). Effects of progesterone administered after MPTP on dopaminergic neurons of male mice. Neuropharmacology 117, 209–218. doi: 10.1016/j.neuropharm.2017.02.007
Liu, R., Baird, D., Park, Y., Freedman, N. D., Huang, X., Hollenbeck, A., et al. (2014). Female reproductive factors, menopausal hormone use, and Parkinson’s disease. Mov. Disord. 29, 889–896. doi: 10.1002/mds.25771
Lundin, J. I., Ton, T. G. N., LaCroix, A. Z., Longstreth, W. T., Franklin, G. M., Swanson, P. D., et al. (2014). Formulations of hormone therapy and risk of Parkinson's disease. Mov. Disord. 29, 1631–1636. doi: 10.1002/mds.26037
Lv, M., Zhang, Y., Chen, G. C., Li, G., Rui, Y., Qin, L., et al. (2017). Reproductive factors and risk of Parkinson’s disease in women: a meta-analysis of observational studies. Behav. Brain Res. 335, 103–110. doi: 10.1016/j.bbr.2017.07.025
Makav, M., and Eroglu, H. A. (2021). Recuperative effect of estrogen on rotenone-induced experimental model of Parkinson’s disease in rats. Environ. Sci. Pollut. Res. Int. 28, 21266–21275. doi: 10.1007/s11356-020-11985-5
Marras, C., and Saunders-Pullman, R. (2014). The complexities of hormonal influences and risk of Parkinson’s disease. Mov. Disord. 29, 845–848. doi: 10.1002/mds.25891
Marras, C., Beck, J. C., Bower, J. H., Roberts, E., Ritz, B., Ross, G. W., et al. (2018). Prevalence of Parkinson’s disease across North America. NPJ Parkinsons Dis. 4:21. doi: 10.1038/s41531-018-0058-0
Martignoni, E., Nappi, R. E., Citterio, A., Calandrella, D., Corengia, E., Fignon, A., et al. (2002). Parkinson’s disease and reproductive life events. Neurol. Sci. 23, S85–S86. doi: 10.1007/s100720200082
Martignoni, E., Nappi, R. E., Citterio, A., Calandrella, D., Zangaglia, R., Mancini, F., et al. (2003). Reproductive life milestones in women with Parkinson's disease. Funct. Neurol. 18, 211–217.
Meoni, S., Macerollo, A., and Moro, E. (2020). Sex differences in movement disorders. Nat. Rev. Neurol. 16, 84–96. doi: 10.1038/s41582-019-0294-x
Miller, I. N., and Cronin-Golomb, A. (2010). Gender differences in Parkinson's disease: clinical characteristics and cognition. Mov. Disord. 25, 2695–2703. doi: 10.1002/mds.23388
Miller, D. B., Ali, S. F., O’Callaghan, J. P., and LAWS, S. C. (1998). The impact of gender and estrogen on striatal dopaminergic neurotoxicity. Ann. N. Y. Acad. Sci. 844, 153–165. doi: 10.1111/j.1749-6632.1998.tb08230.x
Mitra, S., Chakrabarti, N., Dutta, S. S., Ray, S., Bhattacharya, P., Sinha, P., et al. (2015). Gender-specific brain regional variation of neurons, endogenous estrogen, neuroinflammation and glial cells during rotenone-induced mouse model of Parkinson's disease. Neuroscience 292, 46–70. doi: 10.1016/j.neuroscience.2014.12.052
Nicoletti, A., Arabia, G., Pugliese, P., Nicoletti, G., Torchia, G., Condino, F., et al. (2007). Hormonal replacement therapy in women with Parkinson disease and levodopa-induced dyskinesia: a crossover trial. Clin. Neuropharmacol. 30, 276–280. doi: 10.1097/wnf.0b013e318050c9f9
Nicoletti, A., Nicoletti, G., Arabia, G., Annesi, G., de Mari, M., Lamberti, P., et al. (2011). Reproductive factors and Parkinson’s disease: a multicenter case-control study. Mov. Disord. 26, 2563–2566. doi: 10.1002/mds.23951
Nilsen, J., Morales, A., and Brinton, R. D. (2006). Medroxyprogesterone acetate exacerbates glutamate excitotoxicity. Gynecol. Endocrinol. 22, 355–361. doi: 10.1080/09513590600863337
Nitkowska, M., Czyzyk, M., and Friedman, A. (2014). Reproductive life characteristics in females affected with Parkinson’s disease and in healthy control subjects - a comparative study on polish population. Neurol. Neurochir. Pol. 48, 322–327. doi: 10.1016/j.pjnns.2014.08.004
Picillo, M., Nicoletti, A., Fetoni, V., Garavaglia, B., Barone, P., and Pellecchia, M. T. (2017). The relevance of gender in Parkinson's disease: a review. J. Neurol. 264, 1583–1607. doi: 10.1007/s00415-016-8384-9
Poewe, W., Seppi, K., Tanner, C. M., Halliday, G. M., Brundin, P., Volkmann, J., et al. (2017). Parkinson disease. Nat. Rev. Dis. Primers 3:17013. doi: 10.1038/nrdp.2017.13
Popat, R. A., van den Eeden, S. K., Tanner, C. M., McGuire, V., Bernstein, A. L., Bloch, D. A., et al. (2005). Effect of reproductive factors and postmenopausal hormone use on the risk of Parkinson disease. Neurology 65, 383–390. doi: 10.1212/01.wnl.0000171344.87802.94
Quinlan, M. G., Almey, A., Caissie, M., LaChappelle, I., Radiotis, G., and Brake, W. G. (2013). Estradiol and striatal dopamine receptor antagonism influence memory system bias in the female rat. Neurobiol. Learn. Mem. 106, 221–229. doi: 10.1016/j.nlm.2013.08.018
Ragonese, P., D’Amelio, M., Callari, G., Salemi, G., Morgante, L., and Savettieri, G. (2006). Age at menopause predicts age at onset of Parkinson’s disease. Mov. Disord. 21, 2211–2214. doi: 10.1002/mds.21127
Ragonese, P., D’Amelio, M., Salemi, G., Aridon, P., Gammino, M., Epifanio, A., et al. (2004). Risk of Parkinson disease in women: effect of reproductive characteristics. Neurology 62, 2010–2014. doi: 10.1212/WNL.62.11.2010
Read, M. D., Edey, K. A., Hapeshi, J., and Foy, C. (2010). The age of ovarian failure following premenopausal hysterectomy with ovarian conservation. Menopause Int. 16, 56–59. doi: 10.1258/mi.2010.010022
Rocca, W. A., Bower, J. H., Maraganore, D. M., Ahlskog, J. E., Grossardt, B. R., de Andrade, M., et al. (2008). Increased risk of parkinsonism in women who underwent oophorectomy before menopause. Neurology 70, 200–209. doi: 10.1212/01.wnl.0000280573.30975.6a
Rodriguez-Perez, A. I., Borrajo, A., Valenzuela, R., Lanciego, J. L., and Labandeira-Garcia, J. L. (2015). Critical period for dopaminergic neuroprotection by hormonal replacement in menopausal rats. Neurobiol. Aging 36, 1194–1208. doi: 10.1016/j.neurobiolaging.2014.10.028
Rosendahl, M., Simonsen, M. K., and Kjer, J. J. (2017). The influence of unilateral oophorectomy on the age of menopause. Climacteric 20, 540–544. doi: 10.1080/13697137.2017.1369512
Rossouw, J. E., Anderson, G. L., Prentice, R. L., LaCroix, A., Kooperberg, C., Stefanick, M. L., et al. (2002). Risks and benefits of estrogen plus progestin in healthy postmenopausal women: principal results from the Women's Health Initiative randomized controlled trial. JAMA 288, 321–333. doi: 10.1001/jama.288.3.321
Rugbjerg, K., Christensen, J., Tjønneland, A., and Olsen, J. H. (2013). Exposure to estrogen and women’s risk for Parkinson’s disease: a prospective cohort study in Denmark. Parkinsonism Relat. Disord. 19, 457–460. doi: 10.1016/j.parkreldis.2013.01.008
Schipper, H. M. (2016). The impact of gonadal hormones on the expression of human neurological disorders. Neuroendocrinology 103, 417–431. doi: 10.1159/000440620
Shen, D., Tian, X., Zhang, B., and Song, R. (2017). Mechanistic evaluation of neuroprotective effect of estradiol on rotenone and 6-OHDA induced Parkinson's disease. Pharmacol. Rep. 69, 1178–1185. doi: 10.1016/j.pharep.2017.06.008
Simon, K. C., Chen, H., Gao, X., Schwarzschild, M. A., and Ascherio, A. (2009). Reproductive factors, exogenous estrogen use, and risk of Parkinson's disease. Mov. Disord. 24, 1359–1365. doi: 10.1002/mds.22619
Simpkins, J. W., Yang, S. H., Wen, Y., and Singh, M. (2005). Estrogens, progestins, menopause and neurodegeneration: basic and clinical studies. Cell. Mol. Life Sci. 62, 271–280. doi: 10.1007/s00018-004-4382-2
Singh, M., and Su, C. (2013). Progesterone-induced neuroprotection: factors that may predict therapeutic efficacy. Brain Res. 1514, 98–106. doi: 10.1016/j.brainres.2013.01.027
Smith, K. M., and Dahodwala, N. (2014). Sex differences in Parkinson's disease and other movement disorders. Exp. Neurol. 259, 44–56. doi: 10.1016/j.expneurol.2014.03.010
Torrealday, S., Kodaman, P., and Pal, L. (2017). Premature ovarian insufficiency - an update on recent advances in understanding and management. F1000Res. 6:2069. doi: 10.12688/f1000research.11948.1
Tsang, K. L., and Ho, S. L. (2001). Estrogen therapy in postmenopausal women with Parkinson's disease. Expert. Rev. Neurother. 1, 135–137. doi: 10.1586/14737175.1.2.135
Turcano, P., and Savica, R. (2020). Sex differences in movement disorders. Handb. Clin. Neurol. 175, 275–282. doi: 10.1016/B978-0-444-64123-6.00019-9
Villa, A., Vegeto, E., Poletti, A., and Maggi, A. (2016). Estrogens, Neuroinflammation, and Neurodegeneration. Endocr. Rev. 37, 372–402. doi: 10.1210/er.2016-1007
Vilos, G. A., Allaire, C., Laberge, P. Y., Leyland, N., Vilos, A. G., Murji, A., et al. (2015). The management of uterine leiomyomas. J. Obstet. Gynaecol. Can. 37, 157–178. doi: 10.1016/S1701-2163(15)30338-8
Wang, P., Li, J., Qiu, S., Wen, H., and du, J. (2015). Hormone replacement therapy and Parkinson's disease risk in women: a meta-analysis of 14 observational studies. Neuropsychiatr. Dis. Treat. 11, 59–66. doi: 10.2147/NDT.S69918
Whitmer, R. A., Quesenberry, C. P., Zhou, J., and Yaffe, K. (2011). Timing of hormone therapy and dementia: the critical window theory revisited. Ann. Neurol. 69, 163–169. doi: 10.1002/ana.22239
Wooten, G. F. (2004). Are men at greater risk for Parkinson’s disease than women? J. Neurol. Neurosurg. Psychiatry 75, 637–639. doi: 10.1136/jnnp.2003.020982
Xing, L., Venables, M. J., and Trudeau, V. L. (2017). Role of aromatase and radial glial cells in neurotoxin-induced dopamine neuron degeneration and regeneration. Gen. Comp. Endocrinol. 241, 69–79. doi: 10.1016/j.ygcen.2016.02.011
Yadav, R., Shukla, G., Goyal, V., Singh, S., and Behari, M. (2012). A case control study of women with Parkinson’s disease and their fertility characteristics. J. Neurol. Sci. 319, 135–138. doi: 10.1016/j.jns.2012.05.026
Keywords: Parkinson’s disease, menopause, hormone replacement therapy, risk, onset
Citation: Unda SR, Marciano S, Milner TA and Marongiu R (2022) State-of-the-art review of the clinical research on menopause and hormone replacement therapy association with Parkinson’s disease: What meta-analysis studies cannot tell us. Front. Aging Neurosci. 14:971007. doi: 10.3389/fnagi.2022.971007
Edited by:
Silvia Fossati, Temple University, United StatesCopyright © 2022 Unda, Marciano, Milner and Marongiu. This is an open-access article distributed under the terms of the Creative Commons Attribution License (CC BY). The use, distribution or reproduction in other forums is permitted, provided the original author(s) and the copyright owner(s) are credited and that the original publication in this journal is cited, in accordance with accepted academic practice. No use, distribution or reproduction is permitted which does not comply with these terms.
*Correspondence: Roberta Marongiu, cm9tMjA0M0BtZWQuY29ybmVsbC5lZHU=
†ORCID: Santiago R. Unda http://orcid.org/0000-0001-7319-6794
Sabina Marciano https://orcid.org/0000-0002-0899-0454
Teresa A. Milner https://orcid.org/0000-0002-0458-6569
Roberta Marongiu https://orcid.org/0000-0002-7609-7000