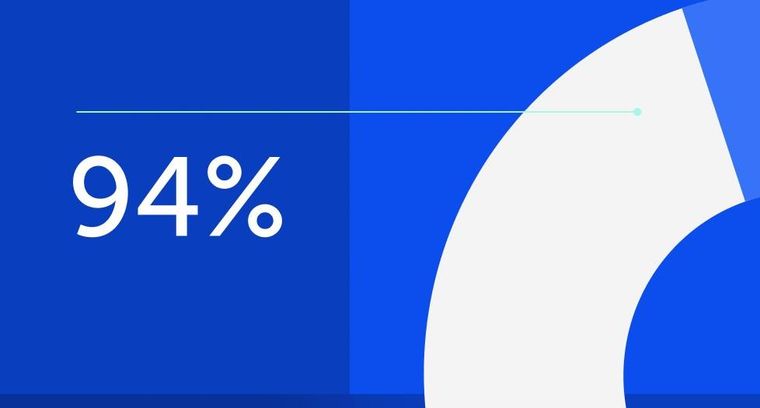
94% of researchers rate our articles as excellent or good
Learn more about the work of our research integrity team to safeguard the quality of each article we publish.
Find out more
MINI REVIEW article
Front. Aging Neurosci., 22 July 2022
Sec. Neuroinflammation and Neuropathy
Volume 14 - 2022 | https://doi.org/10.3389/fnagi.2022.934346
This article is part of the Research TopicRegulation of Neuroinflammation by Multiorgan NetworkView all 5 articles
Major depressive disorder (MDD) is a multifactorial disease affected by several environmental factors. Although several potential onset hypotheses have been identified, the molecular mechanisms underlying the pathogenesis of this disorder remain unclear. Several recent studies have suggested that among many environmental factors, inflammation and immune abnormalities in the brain or the peripheral tissues are associated with the onset of MDDs. Furthermore, several stress-related hypotheses have been proposed to explain the onset of MDDs. Thus, inflammation or immune abnormalities can be considered stress responses that occur within the brain or other tissues and are regarded as one of the mechanisms underlying the stress hypothesis of MDDs. Therefore, we introduce several current advances in inflammation studies in the brain that might be related to the pathophysiology of MDD due to stress exposure in this review.
One of the major mood disorders, major depressive disorder (MDD), is known to show significant morbidity and is associated with social and economic disability [Smith, 2014; Vigo et al., 2016; World Health Organization (WHO), 2020; Wang et al., 2022]. MDD is believed to be a multifactorial disease attributable to both environmental and genetic factors, although the genes responsible for and the molecular mechanisms underlying the pathogenesis of MDD remain unclear (Lopizzo et al., 2015; Tohyama et al., 2015; Miyata et al., 2015a). Although selective serotonin reuptake inhibitors (SSRIs) and serotonin–norepinephrine reuptake inhibitors (SNRIs), which are therapeutic agents for MDD, are based on the most widely accepted hypothesis, the monoamine hypothesis, they do not exhibit adequate therapeutic effects in approximately one-third to half of patients with MDD (Hieronymus et al., 2016; Jesulola et al., 2018; Zhao et al., 2022). The important findings of low-dose ketamine effects have been recently suggested to provide more insights into this discrepancy. Administration of low-dose ketamine increases spine numbers on the dendrite in a short time and shows a sustained antidepressant effect within 1 h not only neurons but also astrocytes (Pryazhnikov et al., 2018; Zanos and Gould, 2018; Stenovec et al., 2021). Thus, this change in the neuronal plasticity and glia functions in the brain might underlie the pathophysiology of MDD and may be related to the monoamine hypothesis.
Considering the relationship between the neuronal plasticity (or synaptic function) and the pathology of MDD, a thorough understanding of the response to inflammation in the brain is important. Several recent studies have suggested that among many environmental factors, inflammation and immune abnormalities in the brain and peripheral tissues are associated with the onset of neuropsychiatric disorders, and inflammation during brain development has been shown to strongly increase the risk of MDD [Goldsmith et al., 2016; Pape et al., 2019; Abdoli et al., 2020; World Health Organization (WHO), 2020; Hansen, 2022; Wang et al., 2022]. In addition, synaptic pruning is induced by increased reactive microglia in the brain of patients with MDD, and nonsteroidal anti-inflammatory drugs (NSAIDs) perform assistant functions in enhancing the therapeutic effects in patients with senile depression (Wohleb et al., 2016; Yuan et al., 2020; Hang et al., 2021; Dawood et al., 2022; Li et al., 2022; Strekalova et al., 2022). Changes in the expression levels of cytokines and chemokines have also been reported to occur in the blood of patients with MDD (Martinuzzi et al., 2021; Poletti et al., 2021; Agarwal et al., 2022).
Among the multiple environmental factors, repeated stressful events are associated with the onset of MDD, and stress has been shown to activate the hypothalamic–pituitary–adrenocortical (HPA) system (Pariante and Lightman, 2008; Nouraei et al., 2018; Ceruso et al., 2020; Hennings et al., 2021). The negative feedback of corticosteroids on the HPA system occurs at the level of the hypothalamus and anterior pituitary via glucocorticoid receptors (GRs). Dysregulation of this negative feedback mechanism has been reported in patients with MDD, which results in hyperactivity of the HPA system and higher basal levels of serum corticosterone (Almeida et al., 2021). In addition, many clinical studies have demonstrated that elevated corticosterone levels trigger depressive symptoms (Short et al., 2016; Raupp-Barcaro et al., 2018). These facts strongly indicate that sustained elevated levels of plasma corticosteroids are a cause of MDD. However, glucocorticoids (humans, cortisol, rodents, and corticosterone) can show both inflammation-aggravating and anti-inflammatory effects. One of the inflammatory cytokines, interleukin-1 (IL-1), inhibits the translocation of the GR from the cytoplasm to the nucleus (Wang et al., 2004). Furthermore, in the activation of the sympathetic nervous system, which is another stress response system, noradrenaline has been reported to act on the microglia to induce IL-1β mRNA expression (Sugama et al., 2019; Tozaki-Saitoh et al., 2020).
As a part of the molecular mechanism of the onset of depression based on this stress hypothesis, microglial activation, and intracerebral inflammation have been shown to be important for the emotional changes caused by chronic stress in rodent models (Furuyashiki et al., 2019). Chronic stress stimulates IL-1β production, and cyclooxygenase (COX) 1 is involved in the biosynthesis of the inflammation-related molecule prostaglandin (PG) E2 following chronic stress exposure (Nie et al., 2018). IL-1β and COX-1 are mainly expressed in microglia in the brain; however, the relationship between the changes in oligodendrocyte and myelin functions and the response to inflammation in the brain is largely unknown. Therefore, this mini-review focuses on the current advances in inflammation studies in the brain that might be related to the pathophysiology of MDD due to stress exposure, which seems to be related to not only neuronal functions but also oligodendrocytes and myelin functions.
Activated microglia have been reported to be involved in various pathologies, including depression, bipolar disorder, and sleep disorder (Stokes et al., 2015; Tay et al., 2018). Stress exposure has been shown to be associated with microglial activation (Walker et al., 2013; Sugama and Kakinuma, 2020). Stress-induced microglial activation involves not only acute stress exposure but also chronic stress exposure. Furthermore, activation of both the HPA axis and the sympathetic nervous system occurs with stress exposure (Elsaafien et al., 2021; Sjörs Dahlman et al., 2021). GR and α- and β-adrenergic receptors (ARs) are expressed in microglia and are involved in the morphological and functional changes of microglia with stress exposure; however, glucocorticoid and noradrenaline functions for microglial activity and inflammation after stress exposure are mediated by contradictory mechanisms (Wohleb et al., 2011; Walker et al., 2013; Delpech et al., 2015; Barnard et al., 2019; Ryan et al., 2020; Brás et al., 2022).
Our previous studies have indicated that elevation of glucocorticoid levels by chronic stress exposure induces the expression of adhesion molecules in oligodendrocytes via the activation of the PI3K–PDK1–SGK1–NDRG1 pathway, which causes excess arborization of oligodendrocytes (Miyata et al., 2011). However, no reduction in the number of oligodendrocytes was observed in the corpus callosum. We also found that the nodes and paranodes of Ranvier in the corpus callosum were narrower and oligodendrocyte activity decreased after chronic stress exposure (Miyata et al., 2015b, 2016). However, we could not find microglial activation in the corpus callosum for 24 h after the last stress exposure (Miyata et al., 2011). A recent report suggested that microglia are transiently activated for 1.5–4 h after social defeat stress, and this microglial activation is enhanced in the hippocampus and medial prefrontal cortex (PFC) of mice exposed to chronic stress (Nie et al., 2018; Furuyashiki and Kitaoka, 2019). Thus, this stress response to microglia is transient and shows specificity for different brain regions; therefore, further investigation of the relationship between the elevation of plasma glucocorticoid levels, the inflammatory response in the brain and peripheral tissues, and the depression-like pathology is required.
The contradictory mechanisms of glucocorticoids function in both microglial activity and inflammation after stress exposure. Previous reports have presented several hypotheses regarding this controversial phenomenon. The priming hypothesis indicates that the inflammation state is exacerbated by glucocorticoid treatment of microglia before lipopolysaccharide (LPS) stimulation; however, microglial activation is inhibited by glucocorticoid treatment of microglia after LPS stimulation (Frank et al., 2010; Carrillo-de Sauvage et al., 2013). The next hypothesis postulates a dependency on glucocorticoid concentration. Treatment of microglia with a low concentration of glucocorticoids indicates suppression of inflammation; however, treatment with a high concentration of glucocorticoids for microglia promotes inflammation (MacPherson et al., 2005; Liu et al., 2018b).
The contradictory mechanisms underlying noradrenaline also function in both microglial activity and inflammation after stress exposure. The noradrenaline signal was shown to induce p38 MAPK- and ERK1/2-dependent inflammatory responses in the absence of LPS stimulation; however, after LPS stimulation, noradrenaline stimulates cAMP and suppresses the inflammatory response in a PKA-dependent manner (Qian et al., 2009; Takahashi et al., 2018; Zhang et al., 2018; Ryan et al., 2020).
The cyclic GMP–AMP synthase (cGAS)–stimulator of interferon genes (STING) pathway is one of the mechanisms by which DNA appears in the cytoplasm, induces innate immune systems, and strongly induces type I interferon (IFN-I) (Margolis et al., 2017; Sprooten et al., 2019; Taguchi and Mukai, 2019; Yang et al., 2022). In recent years, the response to autologous DNA leakage into the cytoplasm and the abnormal activation of the cGAS-STING pathway have been reported to be involved in many neurodegenerative and inflammatory diseases (Jauhari et al., 2020; Pal et al., 2020; Barrett et al., 2021; Jassim et al., 2021; Hinkle et al., 2022).
The IFN therapy has been established as a useful treatment for many diseases such as hepatitis C, hepatitis B, and multiple myeloma (Ettari et al., 2016; Ye and Chen, 2021; Cao et al., 2022; Liu, H. et al., 2022). However, IFN-I treatment is known to induce various neuropsychiatric symptoms, and depression is known to occur at a high frequency of 30% or more (Tovey and Lallemand, 2010; Pinto and Andrade, 2016; Liu et al., 2020). Nevertheless, the core symptoms of IFN-induced depression are reported to be physical symptoms, such as sleep disorders and loss of appetite, and the mood symptoms that are observed in general depression are difficult to identify in the foreground, with few reports describing the molecular mechanisms underlying these differences. Thus, although the relationship between the cGAS-STING pathway and the onset of depression has not yet been reported, the primary goal of future research is the involvement of chronic stress in the endoplasmic reticulum and the Golgi apparatus functions as the molecular mechanism of INF-I production by TBK1-IRF3 pathway activation and the pathology of INF-I-specific depressive symptoms.
Aging is well-known to cause changes leading to immune system decline, a process called “immunosenescence” (Pietrobon et al., 2020). In addition, a previous report has shown that the elderly have mild chronic inflammation and that inflammatory proteins and growth factors are constantly increasing in the elderly brain and a senescence-associated secretory phenotype (SASP) mediates the transport of these factors extracellularly (Gamage et al., 2020; Liao et al., 2021; Blagosklonny, 2022). However, the increased levels of inflammatory cytokines, such as TNF-α, IL-1, and IL-6, are very low in the elderly, and this mild chronic inflammation in the elderly, called “inflammaging,” is one of the health indicators of the elderly (Rea et al., 2018; Pietrobon et al., 2020). Thus, these phenomena in the elderly might be related to the activation of the SASP by transcription factors, such as NF-κB and C/EBP-β, and the onset of senile depression (Bruxel et al., 2019; Saito et al., 2021).
Inflammasomes are protein complexes that play a central role in innate immunity (Man and Kanneganti, 2016; Gasteiger et al., 2017; Challagundla et al., 2022; Dong et al., 2022). Inflammasome signals have been reported to cause the activation of caspase-1 and increase the secretion of TNF-α and IL-1β, which are inflammatory cytokines in microglia and may be associated with depressive symptoms (Zhu et al., 2017; Slusarczyk et al., 2018; Li et al., 2021; Rao et al., 2021; Liu Q. et al., 2022).
Nucleotide-binding, leucine-rich repeat, pyrin domain containing 3 (NLRP3) has been reported to function as a “hub” for the pathophysiology of various neuropsychiatric disorders, including MDD (Iwata et al., 2016; Yamanashi et al., 2017; Zhang et al., 2022). A previous report has indicated that an increase in the ATP concentration in the PFC and hippocampus was observed after restraint stress or chronic mild stress as a model for the onset of depression and that ATP binds to the P2X7 receptor in microglia (Ribeiro et al., 2019; Lan et al., 2020; Drinkall et al., 2022). Next, this P2X7 signal decreases the concentration of intracellular potassium, inducing the formation of a protein complex between the NLRP3 oligomer, apoptosis-associated speck-like protein containing a CARD (ASC), and pro-caspase 1 (Yang et al., 2018; von Muecke-Heim et al., 2021). The suppression of inflammation in this inflammasome pathway might be a function of the antidepressant effect; however, many P2X7 receptor inhibitors have low central transferability, and IL-1β-neutralizing antibodies are extremely expensive (Iwata et al., 2016; Wang et al., 2016; Yue et al., 2017; Ren et al., 2021).
Brain inflammation after chronic stress exposure involves not only the induction of inflammatory cytokines from microglia but also multiple peripheral reactions, such as dysfunction of the blood–brain barrier, involvement of IL-1 receptors in vascular endothelial cells, and promotion of monocyte migration from the peripheral tissues into the brain (Chaves-Filho et al., 2019; Kitaoka, 2022).
Nonsteroidal anti-inflammatory drugs inhibit COX-1 and COX-2 functions and decrease prostaglandin and thromboxane levels, thus inducing a reduction in TNF-α and IL-6 levels (Wohleb et al., 2016; Yuan et al., 2020; Hang et al., 2021; Li et al., 2022). COX-1 selective inhibitors that suppress inflammation in the brain can inhibit depressive symptoms after chronic stress exposure (Guevara et al., 2015; Brkic et al., 2020). COX-1 is expressed selectively in the microglia, and it has been suggested to be involved in inflammation control and depression by microglial regulation. Toll-like receptors (TLRs) are highly expressed in microglia. Chronic stress exposure induces the TLR2/4-dependent inflammatory cytokines IL-1α and TNF-α in the microglia of PFC (Nie et al., 2018). Furthermore, TLR2/4 is not only required for enhanced expression of inflammatory cytokines in PFCs but also essential for PGE2 production in the subcutaneous region (Nie et al., 2019). The COX-2 selective inhibitor celecoxib inhibits prostaglandin and downstream cytokine production (Strekalova et al., 2022). Thus, several clinical studies have shown that celecoxib enhances the antidepressant effect; however, some studies have indicated that celecoxib might increase brain inflammation (Baune, 2017; Lang and Walter, 2017; Alboni et al., 2018; Strumila et al., 2022). These conflicting results may be resolved through more detailed basic and clinical studies in the future (Luning Prak et al., 2022).
Corticosterone, which is closely related to chronic stress responses, increases the number of neutrophils in the bone marrow (Sarjan and Yajurvedi, 2018). Catecholamines, which are chronic stress response factors, promote the proliferation and recruitment of hematopoietic stem cells in the bone marrow (Hanns et al., 2019). Thus, blood neutrophil and monocyte counts increase in mice exposed to chronic stress.
Increased expression of CCL2, a CCR2 ligand and one of the chemokines involved in the mobilization of monocytes in the blood, was observed in microglia after chronic stress exposure, and increased expression of IL-1β was observed in monocytes in the brain (Slusarczyk et al., 2015; Zhao et al., 2016; McKim et al., 2018; Trojan et al., 2019). This increase in monocyte-derived Il-1β expression and activation by caspase-1 is associated with anxiety symptoms (Wohleb et al., 2015; McKim et al., 2018).
The relationship between IL-6 and MDD pathology has been reported previously (Maes et al., 2016; Ting et al., 2020; Kuo et al., 2021). Serum IL-6 concentration was significantly higher in patients with MDD and decreased only in the SSRI and SNRI reaction groups (Fei et al., 2021). Thus, high IL-6 levels in the blood are associated with intractable depression, and IL-6 levels in the blood may predict the therapeutic response to SSRIs and/or SNRIs.
Coronaviruses can damage the central nervous system (CNS) via direct invasion. These viruses enter via the blood-circulation pathway, neuronal pathway, and binding to angiotensin-converting enzyme-2 (ACE-2) receptors (Wu et al., 2020; Kumar et al., 2021; Hornick et al., 2022; Thye et al., 2022). Furthermore, some patients with COVID-19 show cytokine dysregulation, such as TNF-α, IL-1β, IL-6, and IFN-γ, which are well known to be associated with MDD (Liu et al., 2021; Lorkiewicz and Waszkiewicz, 2021). Cytokine-release syndrome or COVID-19 infection-induced inflammatory responses may be associated with chronic stress-related inflammatory responses that prolong and increase post-viral symptoms (Troyer et al., 2020). Furthermore, some COVID-19 survivors show a relationship between disruption of the HPA axis and several psychiatric symptoms, including anxiety, depression, and post-traumatic stress disorder (PTSD) (Hu et al., 2020; Badenoch et al., 2021; Mohammadkhanizadeh and Nikbakht, 2021; Hornick et al., 2022; Thye et al., 2022).
Many diseases of the nervous system attack nonneuronal cells and indirectly affect neuronal function and integrity. We have already demonstrated for the first time both in vivo and in vitro that exposure to chronic stress leads to alterations in oligodendrocyte activity and disrupts axon-myelin adhesion at the paranodes and nodes of Ranvier, as evidenced by the diffuse distribution of key proteins and reduced axonal activity (Miyata et al., 2011, 2015b, 2016). We further found changes in some factors involved in the inflammatory response based on the results of our recent study. Therefore, we have continued investigating the relationship between brain inflammation and depressive pathology.
Compromised white matter/myelin integrity has been reported not only in patients with MDD but also in animal models of MDD (Miyata et al., 2016; Miyata, 2019; Greenberg et al., 2021). Furthermore, in brain imaging and postmortem evaluations of the human brain, patients with MDD have been found to show abnormalities in the white matter and/or oligodendrocytes (Czéh and Nagy, 2018; Vostrikov and Uranova, 2020). Interestingly, stress exposure in animal models can decrease the number of oligodendrocytes in the cortex and amygdala, indicating potential links between disturbed myelination and MDD (Liu et al., 2018a; Kokkosis et al., 2022; Madeira et al., 2022). In contrast, it has been reported that astrocytes are related to neuroinflammation, and astrocyte density in the frontal region is reduced in chronic stress conditions (Banasr et al., 2010; Hattori et al., 2017; Takarada-Iemata et al., 2018; Roboon et al., 2021).
A primary goal of future studies is to understand the mechanisms of several brain/peripheral inflammations, which are discussed in this review, and the molecular mechanisms of neurons, oligodendrocytes/myelin, astrocytes, microglia cells, and vascular systems functional changes in chronic stress-exposed mouse models (Figure 1). Therefore, it will be intriguing to examine whether some factors involved in the inflammatory response, which were found in our studies, play roles in the compromised white matter/myelin integrity that has been reported.
Figure 1. Expected mechanisms of the onset of MDD by the effects of intracerebral inflammation due to environmental stress exposure.
SM, YI, SS, and MT contributed to the search, assessment of available literature, and interpreted the results of previous studies. SM wrote the manuscript. All authors have approved the final version of the manuscript prior to submission.
This study was supported in part by Grants from the Japan Society for the Grant-in-Aid for Scientific Research (C) 19K06916, 16K07073, AMED iD3 Grant Number DNW-14010, the AMED Translational Research Grant Number A-131, the Japan Foundation for Applied Enzymology, and the KINDAI COVID-19 Control Support Project.
We would like to thank the Central Research Facilities, Kindai University Faculty of Medicine Center for Animal Experiments, Instrumental Analyses, and Morphological Analyses for their Technical Support and Editage (www.editage.com) for English language editing. We are grateful to Mrs. M. Kaneita and Mrs. Y. Yamamoto for their technical support.
The authors declare that the research was conducted in the absence of any commercial or financial relationships that could be construed as a potential conflict of interest.
All claims expressed in this article are solely those of the authors and do not necessarily represent those of their affiliated organizations, or those of the publisher, the editors and the reviewers. Any product that may be evaluated in this article, or claim that may be made by its manufacturer, is not guaranteed or endorsed by the publisher.
Abdoli, A., Taghipour, A., Pirestani, M., Jahromi, M. A. M., Roustazadeh, A., Mir, H., et al. (2020). Infections, inflammation, and risk of neuropsychiatric disorders: the neglected role of “co-infection”. Heliyon. 6, e05645. doi: 10.1016/j.heliyon.2020.e05645
Agarwal, K., Manza, P., Chapman, M., Nawal, N., Biesecker, E., McPherson, K., et al. (2022). Inflammatory markers in substance use and mood disorders: a neuroimaging perspective. Front. Psychiatry. 13, 863734. doi: 10.3389/fpsyt.2022.863734
Alboni, S., Benatti, C., Capone, G., Tascedda, F., and Brunello, N. (2018). Neither all anti-inflammatory drugs nor all doses are effective in accelerating the antidepressant-like effect of fluoxetine in an animal model of depression. J. Affect. Disord. 235, 124–128. doi: 10.1016/j.jad.2018.04.063
Almeida, F. B., Pinna, G., and Barros, H. M. T. (2021). The role of HPA axis and allopregnanolone on the neurobiology of major depressive disorders and PTSD. Int. J. Mol. Sci. 22, 5495. doi: 10.3390/ijms22115495
Badenoch, J. B., Rengasamy, E. R., Watson, C., Jansen, K., Chakraborty, S., Sundaram, R. D., et al. (2021). Persistent neuropsychiatric symptoms after COVID-19: a systematic review and meta-analysis. Brain. Commun. 4: fcab297. doi: 10.1101/2021.04.30.21256413
Banasr, M., Chowdhury, G. M. I., Terwilliger, R., Newton, S. S., Duman, R. S., Behar, K. L., et al. (2010). Glial pathology in an animal model of depression: reversal of stress-induced cellular, metabolic and behavioral deficits by the glutamate-modulating drug riluzole. Mol. Psychiatry. 15, 501–511. doi: 10.1038/mp.2008.106
Barnard, D. F., Gabella, K. M., Kulp, A. C., Parker, A. D., Dugan, P. B., and Johnson, J. D. (2019). Sex differences in the regulation of brain IL-1β in response to chronic stress. Psychoneuroendocrinology. 103, 203–211. doi: 10.1016/j.psyneuen.2019.01.026
Barrett, J. P., Knoblach, S. M., Bhattacharya, S., Gordish-Dressman, H., Stoica, B. A., and Loane, D. J. (2021). Traumatic brain injury induces cGAS activation and type I interferon signaling in aged mice. Front. Immunol. 12:710608. doi: 10.3389/fimmu.2021.710608
Baune, B. T. (2017). Are non-steroidal anti-inflammatory drugs clinically suitable for the treatment of symptoms in depression-associated inflammation? Curr. Top. Behav. Neurosci. 31, 303–319. doi: 10.1007/7854_2016_19
Blagosklonny, M. V. (2022). Cell senescence, rapamycin and hyperfunction theory of aging. Cell. Cycle. Mar 31, 1–12. doi: 10.1080/15384101.2022.2054636
Brás, J. P., Guillot de Suduiraut, I., Zanoletti, O., Monari, S., Meijer, M., Grosse, J., et al. (2022). Stress-induced depressive-like behavior in male rats is associated with microglial activation and inflammation dysregulation in the hippocampus in adulthood. Brain Behav. Immun. 99, 397–408. doi: 10.1016/j.bbi.2021.10.018
Brkic, Z., Zivanovic, A., and Adzic, M. (2020). Sex-specific effects of lipopolysaccharide on hippocampal mitochondrial processes in neuroinflammatory model of depression. Neuroscience. 451, 174–183. doi: 10.1016/j.neuroscience.2020.09.059
Bruxel, M. A., Tavares, A. M. V., Neto, L. D. Z., de Souza Borges, V., Schroeder, H. T., Bock, P. M., et al. (2019). Chronic whole-body heat treatment relieves atherosclerotic lesions, cardiovascular and metabolic abnormalities, and enhances survival time restoring the anti-inflammatory and anti-senescent heat shock response in mice. Biochimie. 156, 33–46. doi: 10.1016/j.biochi.2018.09.011
Cao, W., Xie, S., Zhang, L., Bi, X., Lin, Y., Yang, L., et al. (2022). Expression of functional molecule on plasmacytoid dendritic cells is associated with HBsAg loss in HBeAg-positive patients during PEG-IFN α-2a treatment. Front. Immunol. 13, 891424. doi: 10.3389/fimmu.2022.891424
Carrillo-de Sauvage, M. Á., Maatouk, L., Arnoux, I., Pasco, M., Sanz, D. A., Delahaye, M., et al. (2013). Potent and multiple regulatory actions of microglial glucocorticoid receptors during CNS inflammation. Cell. Death Differ. 20, 1546–1557. doi: 10.1038/cdd.2013.108
Ceruso, A., Martínez-Cengotitabengoa, M., Peters-Corbett, A., Diaz-Gutierrez, M. J., and Martínez-Cengotitabengoa, M. (2020). Alterations of the HPA axis observed in patients with major depressive disorder and their relation to early life stress: a systematic review. Neuropsychobiology. 79, 417–427. doi: 10.1159/000506484
Challagundla, N., Saha, B., and Agrawal-Rajput, R. (2022). Insights into inflammasome regulation: cellular, molecular, and pathogenic control of inflammasome activation. Immunol. Res. 2022. doi: 10.1007/s12026-022-09286-9. [Epub ahead of print].
Chaves-Filho, A. J. M., Macedo, D. S., de Lucena, D. F., and Maes, M. (2019). Shared microglial mechanisms underpinning depression and chronic fatigue syndrome and their comorbidities. Behav. Brain. Res. 372, 111975. doi: 10.1016/j.bbr.2019.111975
Czéh, B., and Nagy, S. A. (2018). Clinical findings documenting cellular and molecular abnormalities of glia in depressive disorders. Front. Mol. Neurosci. 11, 56. doi: 10.3389/fnmol.2018.00056
Dawood, S., Bano, S., and Badawy, A. (2022). Inflammation and serotonin deficiency in major depressive disorder: molecular docking of antidepressant and anti-inflammatory drugs to tryptophan and indoleamine 2,3-dioxygenases. Biosci. Rep. 42, BSR20220426. doi: 10.1042/BSR20220426
Delpech, J. C., Madore, C., Nadjar, A., Joffre, C., Wohleb, E. S., and Layé, S. (2015). Microglia in neuronal plasticity: influence of stress. Neuropharmacology. 96, 19–28. doi: 10.1016/j.neuropharm.2014.12.034
Dong, H., Zhao, B., Chen, J., Liu, Z., Li, X., Li, L., et al. (2022). Mitochondrial calcium uniporter promotes phagocytosis-dependent activation of the NLRP3 inflammasome. Proc. Natl. Acad. Sci. U. S. A. 119, e2123247119. doi: 10.1073/pnas.2123247119
Drinkall, S., Lawrence, C. B., Ossola, B., Russell, S., Bender, C., Brice, N. B., et al. (2022). The two pore potassium channel THIK-1 regulates NLRP3 inflammasome activation. Glia. Mar. 30, 174. doi: 10.1002/glia.24174
Elsaafien, K., Kirchner, M. K., Mohammed, M., Eikenberry, S. A., West, C., Scott, K. A., et al. (2021). Identification of novel cross-talk between the neuroendocrine and autonomic stress axes controlling blood pressure. J. Neurosci. 41, 4641–4657. doi: 10.1523/JNEUROSCI.0251-21.2021
Ettari, R., Previti, S., Bitto, A., Grasso, S., and Zappalà, M. (2016). Immunoproteasome-selective inhibitors: a promising strategy to treat hematologic malignancies, autoimmune and inflammatory diseases. Curr. Med. Chem. 23, 1217–1238. doi: 10.2174/0929867323666160318173706
Fei, L., Santarelli, G., D'Anna, G., Moretti, S., Mirossi, G., Patti, A., et al. (2021). Can SSRI/SNRI antidepressants decrease the 'cytokine storm' in the course of COVID-19 pneumonia? Panminerva. Med. 2021. doi: 10.23736/S0031-0808.21.04436-0. [Epub ahead of print].
Frank, M. G., Miguel, Z. D., Watkins, L. R., and Maier, S. F. (2010). Prior exposure to glucocorticoids sensitizes the neuroinflammatory and peripheral inflammatory responses to E. coli lipopolysaccharide. Brain. Behav. Immun. 24, 19–30. doi: 10.1016/j.bbi.2009.07.008
Furuyashiki, T., Akiyama, S., and Kitaoka, S. (2019). Roles of multiple lipid mediators in stress and depression. Int. Immunol. 31, 579–587. doi: 10.1093/intimm/dxz023
Furuyashiki, T., and Kitaoka, S. (2019). Neural mechanisms underlying adaptive and maladaptive consequences of stress: roles of dopaminergic and inflammatory responses. Psychiatry. Clin. Neurosci. 73, 669–675. doi: 10.1111/pcn.12901
Gamage, R., Wagnon, I., Rossetti, I., Childs, R., Niedermayer, G., Chesworth, R., et al. (2020). Cholinergic modulation of glial function during aging and chronic neuroinflammation. Front. Cell. Neurosci. 14, 577912. doi: 10.3389/fncel.2020.577912
Gasteiger, G., D'Osualdo, A., Schubert, D. A., Weber, A., Bruscia, E. M., and Hartl, D. (2017). Cellular innate immunity: an old game with new players. J. Innate. Immun. 9, 111–125. doi: 10.1159/000453397
Goldsmith, D., Rapaport, M., and Miller, B. (2016). A meta-analysis of blood cytokine network alterations in psychiatric patients: comparisons between schizophrenia, bipolar disorder and depression. Mol. Psychiatr. 21, 1696. doi: 10.1038/mp.2016.3
Greenberg, T., Bertocci, M. A., Versace, A., Lima Santos, J. P., Chase, H. W., Siffler, R., et al. (2021). Depression and anxiety mediate the relationship between frontotemporal white matter integrity and quality of life in distressed young adults. J. Psychiatr. Res. 132, 55–59. doi: 10.1016/j.jpsychires.2020.10.001
Guevara, C., Fernandez, A. C., Cardenas, R., and Suarez-Roca, H. (2015). Reduction of spinal PGE2 concentrations prevents swim stress-induced thermal hyperalgesia. Neurosci. Lett. 591, 110–114. doi: 10.1016/j.neulet.2015.02.035
Hang, X., Zhang, Y., Li, J., Li, Z., Zhang, Y., Ye, X., et al. (2021). Comparative efficacy and acceptability of anti-inflammatory agents on major depressive disorder: a network meta-analysis. Front Pharmacol. 12, 691200. doi: 10.3389/fphar.2021.691200
Hanns, P., Paczulla, A. M., Medinger, M., Konantz, M., and Lengerke, C. (2019). Stress and catecholamines modulate the bone marrow microenvironment to promote tumorigenesis. Cell. Stress. 3, 221–235. doi: 10.15698/cst2019.07.192
Hansen, N. (2022). Immunopsychiatry - innovative technology to characterize disease activity in autoantibody-associated psychiatric diseases. Front. Immunol. 13, 867229. doi: 10.3389/fimmu.2022.867229
Hattori, T., Kaji, M, Ishii, H., Jureepon, R., Takarada-Iemata, M., Minh Ta, H., et al. (2017). CD38 positively regulates postnatal development of astrocytes cell-autonomously and oligodendrocytes non-cell-autonomously. Glia. 65, 974–989. doi: 10.1002/glia.23139
Hennings, J. M., Ising, M., Uhr, M., Holsboer, F., and Lucae, S. (2021). Effects of weariness of life, suicide ideations and suicide attempt on HPA axis regulation in depression. Psychoneuroendocrinology. 131, 105286. doi: 10.1016/j.psyneuen.2021.105286
Hieronymus, F., Emilsson, J. F., Nilsson, S., and Eriksson, E. (2016). Consistent superiority of selective serotonin reuptake inhibitors over placebo in reducing depressed mood in patients with major depression. Mol. Psychiatry. 21, 523–530. doi: 10.1038/mp.2015.53
Hinkle, J. T., Patel, J., Panicker, N., Karuppagounder, S. S., Biswas, D., Belingon, B., et al. (2022). STING mediates neurodegeneration and neuroinflammation in nigrostriatal α-synucleinopathy. Proc. Natl. Acad. Sci. U. S. A. 119, e2118819119. doi: 10.1073/pnas.2118819119
Hornick, M. G., Olson, M. E., and Jadhav, A. L. (2022). SARS-CoV-2 psychiatric sequelae: a review of neuroendocrine mechanisms and therapeutic strategies. Int. J. Neuropsychopharmacol. 25, 1–12. doi: 10.1093/ijnp/pyab069
Hu, S., Tucker, L., Wu, C., and Yang, L. (2020). Beneficial effects of exercise on depression and anxiety during the Covid-19 pandemic: a narrative review. Front. Psychiatry. 11, 587557. doi: 10.3389/fpsyt.2020.587557
Iwata, M., Ota, K. T., Li, X. Y., Sakaue, F., Li, N., Dutheil, S., et al. (2016). Psychological stress activates the inflammasome via release of adenosine triphosphate and stimulation of the purinergic type 2X7 receptor. Biol. Psychiatry. 80, 12–22. doi: 10.1016/j.biopsych.2015.11.026
Jassim, A. H., Inman, D. M., and Mitchell, C. H. (2021). Crosstalk between dysfunctional mitochondria and inflammation in glaucomatous neurodegeneration. Front. Pharmacol. 12, 699623. doi: 10.3389/fphar.2021.699623
Jauhari, A., Baranov, S. V., Suofu, Y., Kim, J., Singh, T., Yablonska, S., et al. (2020). Melatonin inhibits cytosolic mitochondrial DNA-induced neuroinflammatory signaling in accelerated aging and neurodegeneration. J. Clin. Invest. 130, 3124–3136. doi: 10.1172/JCI135026
Jesulola, E., Micalos, P., and Baguley, I. J. (2018). Understanding the pathophysiology of depression: from monoamines to the neurogenesis hypothesis model- are we there yet? Behav. Brain Res. 341, 79–90. doi: 10.1016/j.bbr.2017.12.025
Kitaoka, S. (2022). Inflammation in the brain and periphery found in animal models of depression and its behavioral relevance. J. Pharmacol. Sci. 148, 262–266. doi: 10.1016/j.jphs.2021.12.005
Kokkosis, A. G., Madeira, M. M., Mullahy, M. R., and Tsirka, S. E. (2022). Chronic stress disrupts the homeostasis and progeny progression of oligodendroglial lineage cells, associating immune oligodendrocytes with prefrontal cortex hypomyelination. Mol. Psychiatry. 27, 2833–2848. doi: 10.1038/s41380-022-01512-y
Kumar, S., Veldhuis, A., and Malhotra, T. (2021). Neuropsychiatric and cognitive sequelae of COVID-19. Front. Psychol. 12, 577529. doi: 10.3389/fpsyg.2021.577529
Kuo, C. Y., Lin, C. H., and Lane, H. Y. (2021). Molecular basis of late-life depression. Int. J. Mol. Sci. 22, 7421. doi: 10.3390/ijms22147421
Lan, T., Bai, M., Chen, X., Wang, Y., Li, Y., Tian, Y., et al. (2020). iTRAQ-based proteomics implies inflammasome pathway activation in the prefrontal cortex of CSDS mice may influence resilience and susceptibility. Life Sci. 262, 118501. doi: 10.1016/j.lfs.2020.118501
Lang, U. E., and Walter, M. (2017). Depression in the context of medical disorders: new pharmacological pathways revisited. Neurosignals. 25, 54–73. doi: 10.1159/000482001
Li, B., Yang, W., Ge, T., Wang, Y., and Cui, R. (2022). Stress induced microglial activation contributes to depression. Pharmacol. Res. 179, 106145. doi: 10.1016/j.phrs.2022.106145
Li, Y., Song, W., Tong, Y., Zhang, X., Zhao, J., Gao, X., et al. (2021). Isoliquiritin ameliorates depression by suppressing NLRP3-mediated pyroptosis via miRNA-27a/SYK/NF-κB axis. J. Neuroinflammation. 18, 1. doi: 10.1186/s12974-020-02040-8
Liao, Z., Yeo, H. L., Wong, S. W., and Zhao, Y. (2021). Cellular senescence: mechanisms and therapeutic potential. Biomedicines. 9, 1769. doi: 10.3390/biomedicines9121769
Liu, H., Yang, X. L., Dong, Z. R., Chen, Z. Q., Hong, J. G., Wang, D. X., et al. (2022). Clinical benefits of direct-acting antivirals therapy in hepatitis C virus patients with hepatocellular carcinoma: a systematic review and meta-analysis. J. Gastroenterol. Hepatol. 2022, 915. doi: 10.1111/jgh.15915
Liu, J., Dietz, K., Hodes, G. E., Russo, S. J., and Casaccia, P. (2018a). Widespread transcriptional alternations in oligodendrocytes in the adult mouse brain following chronic stress. Dev. Neurobiol. 78, 152–162. doi: 10.1002/dneu.22533
Liu, J., Mustafa, S., Barratt, D. T., and Hutchinson, M. R. (2018b). Corticosterone preexposure increases NF-κB translocation and sensitizes IL-1β responses in BV2 microglia-like cells. Front. Immunol. 9, 3. doi: 10.3389/fimmu.2018.00003
Liu, J. J., Wei, Y. B., Strawbridge, R., Bao, Y., Chang, S., Shi, L., et al. (2020). Peripheral cytokine levels and response to antidepressant treatment in depression: a systematic review and meta-analysis. Mol. Psychiatry. 25, 339–350. doi: 10.1038/s41380-019-0474-5
Liu, K., Yang, T., Peng, X. F., Lv, S. M., Ye, X. L., Zhao, T. S., et al. (2021). A systematic meta-analysis of immune signatures in patients with COVID-19. Rev. Med. Virol. 31, e2195. doi: 10.1002/rmv.2195
Liu, Q., Zhang, M. M., Guo, M. X., Zhang, Q. P., Li, N. Z., Cheng, J., et al. (2022). Inhibition of microglial NLRP3 with MCC950 attenuates microglial morphology and NLRP3/caspase-1/IL-1β signaling in stress-induced mice. J. Neuroimmune. Pharmacol. 2022. doi: 10.1007/s11481-021-10037-0. [Epub ahead of print].
Lopizzo, N., Bocchio Chiavetto, L., Cattane, N., Plazzotta, G., Tarazi, F. I., Pariante, C. M., et al. (2015). Gene-environment interaction in major depression: focus on experience-dependent biological systems. Front. Psychiatry. 6, 68. doi: 10.3389/fpsyt.2015.00068
Lorkiewicz, P., and Waszkiewicz, N. (2021). Biomarkers of Post-COVID Depression. J. Clin. Med. 10, 4142. doi: 10.3390/jcm10184142
Luning Prak, E. T., Brooks, T., Makhoul, W., Beer, J. C., Zhao, L., Girelli, T., et al. (2022). No increase in inflammation in late-life major depression screened to exclude physical illness. Transl. Psychiatry. 12, 118. doi: 10.1038/s41398-022-01883-4
MacPherson, A., Dinkel, K., and Sapolsky, R. (2005). Glucocorticoids worsen excitotoxin-induced expression of pro-inflammatory cytokines in hippocampal cultures. Exp. Neurol. 194, 376–383. doi: 10.1016/j.expneurol.2005.02.021
Madeira, M. M., Hage, Z., and Tsirka, S. E. (2022). Beyond myelination: possible roles of the immune proteasome in oligodendroglial homeostasis and dysfunction. Front. Neurosci. 16, 867357. doi: 10.3389/fnins.2022.867357
Maes, M., Nowak, G., Caso, J. R., Leza, J. C., Song, C., Kubera, M., et al. (2016). Toward omics-based, systems biomedicine, and path and drug discovery methodologies for depression-inflammation research. Mol. Neurobiol. 53, 2927–2935. doi: 10.1007/s12035-015-9183-5
Man, S. M., and Kanneganti, T. D. (2016). Converging roles of caspases in inflammasome activation, cell death and innate immunity. Nat. Rev. Immunol. 16, 7–21. doi: 10.1038/nri.2015.7
Margolis, S. R., Wilson, S. C., and Vance, R. E. (2017). Evolutionary origins of cGAS-STING signaling. Trends. Immunol. 38, 733–743. doi: 10.1016/j.it.2017.03.004
Martinuzzi, E., Barbosa, S., Courtet, P., Olié, E., Guillaume, S., Ibrahim, E. C., et al. (2021). Blood cytokines differentiate bipolar disorder and major depressive disorder during a major depressive episode: initial discovery and independent sample replication. Brain. Behav. Immun. Health. 13, 100232. doi: 10.1016/j.bbih.2021.100232
McKim, D. B., Weber, M. D., Niraula, A., Sawicki, C. M., Liu, X., Jarrett, B. L., et al. (2018). Microglial recruitment of IL-1β-producing monocytes to brain endothelium causes stress-induced anxiety. Mol. Psychiatry. 23, 1421–1431. doi: 10.1038/mp.2017.64
Miyata, S. (2019). Cytoskeletal signal-regulated oligodendrocyte myelination and remyelination. Adv. Exp. Med. Biol. 1190, 33–42. doi: 10.1007/978-981-32-9636-7_3
Miyata, S., Hattori, T., Shimizu, S., Ito, A., and Tohyama, M. (2015a). Disturbance of oligodendrocyte function plays a key role in the pathogenesis of schizophrenia and major depressive disorder. Biomed. Res. Inter. 2015, 492367. doi: 10.1155/2015/492367
Miyata, S., Koyama, Y., Takemoto, K., Yoshikawa, K., Ishikawa, T., Taniguchi, M., et al. (2011). Plasma corticosterone activates SGK1 and induces morphological changes in oligodendrocytes in corpus callosum. PLoS ONE. 6, e19859. doi: 10.1371/journal.pone.0019859
Miyata, S., Taniguchi, M., Koyama, Y., Shimizu, S., Tanaka, T., Yasuno, F., et al. (2016). Association between chronic stress-induced structural abnormalities in Ranvier nodes and reduced oligodendrocyte activity in major depression. Sci. Rep. 6, 23084. doi: 10.1038/srep23084
Miyata, S., Yoshikawa, K., Taniguchi, M., Ishikawa, T., Tanaka, T., Shimizu, S., et al. (2015b). Sgk1 regulates desmoglein 1 expression levels in oligodendrocytes in the mouse corpus callosum after chronic stress exposure. Biochem. Biophys. Res. Commun. 464, 76–82. doi: 10.1016/j.bbrc.2015.05.109
Mohammadkhanizadeh, A., and Nikbakht, F. (2021). Investigating the potential mechanisms of depression induced-by COVID-19 infection in patients. J. Clin. Neurosci. 91, 283–287. doi: 10.1016/j.jocn.2021.07.023
Nie, X., Kitaoka, S., Shinohara, M., Kakizuka, A., Narumiya, S., and Furuyashiki, T. (2019). Roles of Toll-like receptor 2/4, monoacylglycerol lipase, and cyclooxygenase in social defeat stress-induced prostaglandin E 2 synthesis in the brain and their behavioral relevance. Sci. Rep. 9, 17548. doi: 10.1038/s41598-019-54082-5
Nie, X., Kitaoka, S., Tanaka, K., Segi-Nishida, E., Imoto, Y., Ogawa, A., et al. (2018). The innate immune receptors TLR2/4 mediate repeated social defeat stress-induced social avoidance through prefrontal microglial activation. Neuron. 99, 464–479. doi: 10.1016/j.neuron.2018.06.035
Nouraei, H., Firouzabadi, N., Mandegary, A., Zomorrodian, K., Bahramali, E., Shayesteh, M. R. H., et al. (2018). Glucocorticoid receptor genetic variants and response to fluoxetine in major depressive disorder. J. Neuropsychiatry. Clin. Neurosci. 30, 45–50. doi: 10.1176/appi.neuropsych.16120322
Pal, S., Rao, G. N., and Pal, A. (2020). High glucose-induced ROS accumulation is a critical regulator of ERK1/2-Akt-tuberin-mTOR signalling in RGC-5 cells. Life Sci. 256, 117914. doi: 10.1016/j.lfs.2020.117914
Pape, K., Tamouza, R., Leboyer, M., and Zipp, F. (2019). Immunoneuropsychiatry—novel perspectives on brain disorders. Nat. Rev. Neurol. 15, 317–328. doi: 10.1038/s41582-019-0174-4
Pariante, C. M., and Lightman, S. L. (2008). The HPA axis in major depression: classical theories and new developments. Trends Neurosci. 31, 464–468. doi: 10.1016/j.tins.2008.06.006
Pietrobon, A. J., Teixeira, F. M. E., and Sato, M. N. (2020). I mmunosenescence and Inflammaging: risk factors of severe COVID-19 in older people. Front. Immunol. 11, 579220. doi: 10.3389/fimmu.2020.579220
Pinto, E. F., and Andrade, C. (2016). Interferon-related depression: a primer on mechanisms, treatment, and prevention of a common clinical problem. Curr. Neuropharmacol. 14, 743–748. doi: 10.2174/1570159X14666160106155129
Poletti, S., Vai, B., Mazza, M. G., Zanardi, R., Lorenzi, C., Calesella, F., et al. (2021). A peripheral inflammatory signature discriminates bipolar from unipolar depression: a machine learning approach. Prog. Neuropsychopharmacol. Biol Psychiatry. 105, 110136. doi: 10.1016/j.pnpbp.2020.110136
Pryazhnikov, E., Mugantseva, E., Casarotto, P., Kolikova, J., Fred, S. M., Toptunov, D., et al. (2018). Longitudinal two-photon imaging in somatosensory cortex of behaving mice reveals dendritic spine formation enhancement by subchronic administration of low-dose ketamine. Sci. Rep. 8, 6464. doi: 10.1038/s41598-018-24933-8
Qian, L., Hu, X., Zhang, D., Snyder, A., Wu, H. M., Li, Y., et al. (2009). beta2 Adrenergic receptor activation induces microglial NADPH oxidase activation and dopaminergic neurotoxicity through an ERK-dependent/protein kinase A-independent pathway. Glia. 57, 1600–1609. doi: 10.1002/glia.20873
Rao, J., Qiao, Y., Xie, R., Lin, L., Jiang, J., Wang, C., et al. (2021). Fecal microbiota transplantation ameliorates stress-induced depression-like behaviors associated with the inhibition of glial and NLRP3 inflammasome in rat brain. J. Psychiatr. Res. 137, 147–157. doi: 10.1016/j.jpsychires.2021.02.057
Raupp-Barcaro, I. F., Vital, M. A., Galduróz, J. C., and Andreatini, R. (2018). Potential antidepressant effect of amantadine: a review of preclinical studies and clinical trials. Braz. J. Psychiatry. 40, 449–458. doi: 10.1590/1516-4446-2017-2393
Rea, I. M., Gibson, D. S., McGilligan, V., McNerlan, S. E., Alexander, H. D., and Ross, O. A. (2018). Age and age-related diseases: role of inflammation triggers and cytokines. Front. Immunol. 9, 586. doi: 10.3389/fimmu.2018.00586
Ren, C., Li, L. X., Dong, A. Q., Zhang, Y. T., Hu, H., Mao, C. J., et al. (2021). Depression induced by chronic unpredictable mild stress increases susceptibility to Parkinson's disease in mice via neuroinflammation mediated by P2X7 receptor. ACS. Chem. Neurosci. 12, 1262–1272. doi: 10.1021/acschemneuro.1c00095
Ribeiro, D. E., Roncalho, A. L., Glaser, T., Ulrich, H., Wegener, G., and Joca, S. (2019). P2X7 Receptor signaling in stress and depression. Int. J. Mol. Sci. 20, 2778. doi: 10.3390/ijms20112778
Roboon, J., Hattori, T., Ishii, H., Takarada-Iemata, M., Nguyen, D. T., Heer, C. D., et al. (2021). Inhibition of CD38 and supplementation of nicotinamide riboside ameliorate lipopolysaccharide-induced microglial and astrocytic neuroinflammation by increasing NAD. J. Neurochem. 158, 311–327. doi: 10.1111/jnc.15367
Ryan, K. M., Boyle, N. T., Harkin, A., and Connor, T. J. (2020). Dexamethasone attenuates inflammatory-mediated suppression of β2-adrenoceptor expression in rat primary mixed glia. J. Neuroimmunol. 338, 577082. doi: 10.1016/j.jneuroim.2019.577082
Saito, Y., Miyajima, M., Yamamoto, S., Sato, T., Miura, N., Fujimiya, M., et al. (2021). Accumulation of senescent neural cells in murine lupus with depression-like behavior. Front. Immunol. 12, 692321. doi: 10.3389/fimmu.2021.692321
Sarjan, H. N., and Yajurvedi, H. N. (2018). Chronic stress induced duration dependent alterations in immune system and their reversibility in rats. Immunol. Lett. 197, 31–43. doi: 10.1016/j.imlet.2018.02.008
Short, A. K., Fennell, K. A., Perreau, V. M., Fox, A., O'Bryan, M. K., Kim, J. H., et al. (2016). Elevated paternal glucocorticoid exposure alters the small noncoding RNA profile in sperm and modifies anxiety and depressive phenotypes in the offspring. Transl. Psychiatry. 6, e837. doi: 10.1038/tp.2016.109
Sjörs Dahlman, A., Jonsdottir, I. H., and Hansson, C. (2021). The hypothalamo-pituitary-adrenal axis and the autonomic nervous system in burnout. Handb. Clin. Neurol. 182, 83–94. doi: 10.1016/B978-0-12-819973-2.00006-X
Slusarczyk, J., Trojan, E., Głombik, K., Budziszewska, B., Kubera, M., Lasoń, W., et al. (2015). Prenatal stress is a vulnerability factor for altered morphology and biological activity of microglia cells. Front. Cell. Neurosci. 9, 82. doi: 10.3389/fncel.2015.00082
Slusarczyk, J., Trojan, E., Głombik, K., Piotrowska, A., Budziszewska, B., Kubera, M., et al. (2018). Targeting the NLRP3 inflammasome-related pathways via tianeptine treatment-suppressed microglia polarization to the M1 phenotype in lipopolysaccharide-stimulated cultures. Int. J. Mol. Sci. 19, 1965. doi: 10.3390/ijms19071965
Sprooten, J., Agostinis, P., and Garg, A. D. (2019). Type I interferons and dendritic cells in cancer immunotherapy. Int. Rev. Cell. Mol. Biol. 348, 217–262. doi: 10.1016/bs.ircmb.2019.06.001
Stenovec, M., Li, B., Verkhratsky, A., and Zorec, R. (2021). Ketamine action on astrocytes provides new insights into rapid antidepressant mechanisms. Adv. Neurobiol. 26, 349–365. doi: 10.1007/978-3-030-77375-5_14
Stokes, L., Spencer, S. J., and Jenkins, T. A. (2015). Understanding the role of P2X7 in affective disorders-are glial cells the major players? Front. Cell. Neurosci. 9, 258. doi: 10.3389/fncel.2015.00258
Strekalova, T., Pavlov, D., Trofimov, A., Anthony, D. C., Svistunov, A., Proshin, A., et al. (2022). Hippocampal over-expression of cyclooxygenase-2 (COX-2) is associated with susceptibility to stress-induced anhedonia in mice. Int. J. Mol. Sci. 23, 2061. doi: 10.3390/ijms23042061
Strumila, R., Lengvenyte, A., Olie, E., Courtet, P., and Guillaume, S. (2022). Null effect of vortioxetine augmentation with celecoxib should not be generalized to other antidepressants. Eur. Neuropsychopharmacol. 55, 84–85. doi: 10.1016/j.euroneuro.2021.11.001
Sugama, S., and Kakinuma, Y. (2020). Stress and brain immunity: microglial homeostasis through hypothalamus-pituitary-adrenal gland axis and sympathetic nervous system. Brain. Behav. Immun. Health. 7, 100111. doi: 10.1016/j.bbih.2020.100111
Sugama, S., Takenouchi, T., Hashimoto, M., Ohata, H., Takenaka, Y., and Kakinuma, Y. (2019). Stress-induced microglial activation occurs through β-adrenergic receptor: noradrenaline as a key neurotransmitter in microglial activation. J. Neuroinflammation. 16, 266. doi: 10.1186/s12974-019-1632-z
Taguchi, T., and Mukai, K. (2019). Innate immunity signalling and membrane trafficking. Curr. Opin. Cell. Biol. 59, 1–7. doi: 10.1016/j.ceb.2019.02.002
Takahashi, K., Nakagawasai, O., Nemoto, W., Kadota, S., Isono, J., Odaira, T., et al. (2018). Memantine ameliorates depressive-like behaviors by regulating hippocampal cell proliferation and neuroprotection in olfactory bulbectomized mice. Neuropharmacology. 137, 141–155. doi: 10.1016/j.neuropharm.2018.04.013
Takarada-Iemata, M., Yoshikawa, A., Ta, H. M., Okitani, N., Nishiuchi, T., Aida, Y., et al. (2018). N-myc downstream-regulated gene 2 protects blood-brain barrier integrity following cerebral ischemia. Glia. 66, 1432–1446. doi: 10.1002/glia.23315
Tay, T. L., Béchade, C., D'Andrea, I., St-Pierre, M. K., Henry, M. S., Roumier, A., et al. (2018). Microglia gone rogue: impacts on psychiatric disorders across the lifespan. Front. Mol. Neurosci. 10, 421. doi: 10.3389/fnmol.2017.00421
Thye, A. Y., Law, J. W., Tan, L. T., Pusparajah, P., Ser, H. L., Thurairajasingam, S., et al. (2022). Psychological symptoms in COVID-19 patients: insights into pathophysiology and risk factors of long COVID-19. Biology. 11, 61. doi: 10.3390/biology11010061
Ting, E. Y., Yang, A. C., and Tsai, S. J. (2020). Role of interleukin-6 in depressive disorder. Int. J. Mol. Sci. 21, 2194. doi: 10.3390/ijms21062194
Tohyama, M., Miyata, S., Hattori, T., Shimizu, S., and Matsuzaki, S. (2015). Molecular basis of major psychiatric diseases such as schizophrenia and depression. Anat. Sci. Int. 90, 137–143. doi: 10.1007/s12565-014-0269-3
Tovey, M. G., and Lallemand, C. (2010). Safety, tolerability, and immunogenicity of interferons. Pharmaceuticals. 3, 1162–1186. doi: 10.3390/ph3041162
Tozaki-Saitoh, H., Sasaki, I., Yamashita, T., Hosoi, M., Kato, T. A., and Tsuda, M. (2020). Involvement of exchange protein directly activated by cAMP and tumor progression locus 2 in IL-1β production in microglial cells following activation of β-adrenergic receptors. J. Pharmacol. Sci. 143, 133–140. doi: 10.1016/j.jphs.2020.03.004
Trojan, E., Chamera, K., Bryniarska, N., Kotarska, K., Leśkiewicz, M., Regulska, M., et al. (2019). Role of chronic administration of antidepressant drugs in the prenatal stress-evoked inflammatory response in the brain of adult offspring rats: involvement of the NLRP3 inflammasome-related pathway. Mol. Neurobiol. 56, 5365–5380. doi: 10.1007/s12035-018-1458-1
Troyer, E. A., Kohn, J. N., and Hong, S. (2020). Are we facing a crashing wave of neuropsychiatric sequelae of COVID-19? Neuropsychiatric symptoms and potential immunologic mechanisms. Brain. Behav. Immun. 87, 34–39. doi: 10.1016/j.bbi.2020.04.027
Vigo, D., Thornicroft, G., and Atun, R. (2016). Estimating the true global burden of mental illness. Lancet Psych. 3, 171–178. doi: 10.1016/S2215-0366(15)00505-2
von Muecke-Heim, I. A., Ries, C., Urbina, L., and Deussing, J. M. (2021). P2X7R antagonists in chronic stress-based depression models: a review. Eur. Arch. Psychiatry. Clin. Neurosci. 271, 1343–1358. doi: 10.1007/s00406-021-01306-3
Vostrikov, V. M., and Uranova, N. A. (2020). Reduced density of oligodendrocytes and oligodendrocyte clusters in the caudate nucleus in major psychiatric illnesses. Schizophr. Res. 215, 211–216. doi: 10.1016/j.schres.2019.10.027
Walker, F. R., Nilsson, M., and Jones, K. (2013). Acute and chronic stress-induced disturbances of microglial plasticity, phenotype and function. Curr. Drug. Targets. 14, 1262–1276. doi: 10.2174/13894501113149990208
Wang, H., He, Y., Sun, Z., Ren, S., Liu, M., Wang, G., et al. (2022). Microglia in depression: an overview of microglia in the pathogenesis and treatment of depression. J. Neuroinflammation. 19, 132. doi: 10.1186/s12974-022-02492-0
Wang, W., Xiang, Z. H., Jiang, C. L., Liu, W. Z., and Shang, Z. L. (2016). Effects of antidepressants on P2X7 receptors. Psychiatry. Res. 242, 281–287. doi: 10.1016/j.psychres.2016.06.001
Wang, X., Wu, H., and Miller, A. H. (2004). Interleukin 1alpha (IL-1alpha) induced activation of p38 mitogen-activated protein kinase inhibits glucocorticoid receptor function. Mol. Psychiatry 9, 65–75. doi: 10.1038/sj.mp.4001339
Wohleb, E. S., Franklin, T., Iwata, M., and Duman, R. S. (2016). Integrating neuroimmune systems in the neurobiology of depression. Nat. Rev. Neurosci. 17, 497–511. doi: 10.1038/nrn.2016.69
Wohleb, E. S., Hanke, M. L., Corona, A. W., Powell, N. D., Stiner, L. M., Bailey, M. T., et al. (2011). Adrenergic receptor antagonism prevents anxiety-like behavior and microglial reactivity induced by repeated social defeat. J. Neurosci. 31, 6277–6288. doi: 10.1523/JNEUROSCI.0450-11.2011
Wohleb, E. S., McKim, D. B., Sheridan, J. F., and Godbout, J. P. (2015). Monocyte trafficking to the brain with stress and inflammation: a novel axis of immune-to-brain communication that influences mood and behavior. Front. Neurosci. 8, 447. doi: 10.3389/fnins.2014.00447
World Health Organization (WHO). (2020). Depression. World Health Organization. Available from: https://www.who.int/en/news-room/~fact-sheets/detail/depression.
Wu, Y., Xu, X., Chen, Z., Duan, J., Hashimoto, K., Yang, L., et al. (2020). Nervous system involvement after infection with COVID-19 and other coronaviruses. Brain. Behav. Immun. 87, 18–22. doi: 10.1016/j.bbi.2020.03.031
Yamanashi, T., Iwata, M., Kamiya, N., Tsunetomi, K., Kajitani, N., Wada, N., et al. (2017). Beta-hydroxybutyrate, an endogenic NLRP3 inflammasome inhibitor, attenuates stress-induced behavioral and inflammatory responses. Sci. Rep. 7, 7677. doi: 10.1038/s41598-017-08055-1
Yang, J. W., Yang, S. J., Na, J. M., Hahn, H. G., and Cho, S. W. (2018). 3-(Naphthalen-2-yl(propoxy)methyl)azetidine hydrochloride attenuates NLRP3 inflammasome-mediated signaling pathway in lipopolysaccharide-stimulated BV2 microglial cells. Biochem. Biophys. Res. Commun. 495, 151–156. doi: 10.1016/j.bbrc.2017.10.131
Yang, Y., Huang, Y., and Zeng, Z. (2022). Advances in cGAS-STING signaling pathway and diseases. Front. Cell. Dev. Biol. 10, 800393. doi: 10.3389/fcell.2022.800393
Ye, J., and Chen, J. (2021). Interferon and hepatitis B: current and future perspectives. Front. Immunol. 12, 733364. doi: 10.3389/fimmu.2021.733364
Yuan, Z., Chen, Z., Xue, M., Zhang, J., and Leng, L. (2020). Application of antidepressants in depression: a systematic review and meta-analysis. J. Clin. Neurosci. 80, 169–181. doi: 10.1016/j.jocn.2020.08.013
Yue, N., Huang, H., Zhu, X., Han, Q., Wang, Y., Li, B., et al. (2017). Activation of P2X7 receptor and NLRP3 inflammasome assembly in hippocampal glial cells mediates chronic stress-induced depressive-like behaviors. J. Neuroinflammation. 14, 102. doi: 10.1186/s12974-017-0865-y
Zanos, P., and Gould, T. D. (2018). Mechanisms of ketamine action as an antidepressant. Mol. Psychiatry. 23, 801–811. doi: 10.1038/mp.2017.255
Zhang, S., Hu, J., Liu, G., Wu, H., Li, M., Shi, C., et al. (2022). Chronic clomipramine treatment increases hippocampal volume in rats exposed to chronic unpredictable mild stress. Transl. Psychiatry. 12, 245. doi: 10.1038/s41398-022-02006-9
Zhang, X., Hartung, J. E., Bortsov, A. V., Kim, S., O'Buckley, S. C., Kozlowski, J., et al. (2018). Sustained stimulation of β2- and β3-adrenergic receptors leads to persistent functional pain and neuroinflammation. Brain. Behav. Immun. 73, 520–532. doi: 10.1016/j.bbi.2018.06.017
Zhao, Q., Wu, X., Yan, S., Xie, X., Fan, Y., Zhang, J., et al. (2016). The antidepressant-like effects of pioglitazone in a chronic mild stress mouse model are associated with PPARγ-mediated alteration of microglial activation phenotypes. J. Neuroinflammation. 13, 259. doi: 10.1186/s12974-016-0728-y
Zhao, X., Karkare, S., Nash, A. I., Sheehan, J. J., Aboumrad, M., Near, A. M., et al. (2022). Characteristics and current standard of care among veterans with major depressive disorder in the United States: a real-world data analysis. J. Affect. Disord. 307, 184–190. doi: 10.1016/j.jad.2022.03.058
Keywords: major depressive disorders, stress hypothesis, cGAS-STING pathway, inflammasome, immunosenescence
Citation: Miyata S, Ishino Y, Shimizu S and Tohyama M (2022) Involvement of inflammatory responses in the brain to the onset of major depressive disorder due to stress exposure. Front. Aging Neurosci. 14:934346. doi: 10.3389/fnagi.2022.934346
Received: 02 May 2022; Accepted: 27 June 2022;
Published: 22 July 2022.
Edited by:
Tsuyoshi Hattori, Kanazawa University, JapanReviewed by:
Hussein Kadhem Al-Hakeim, University of Kufa, IraqCopyright © 2022 Miyata, Ishino, Shimizu and Tohyama. This is an open-access article distributed under the terms of the Creative Commons Attribution License (CC BY). The use, distribution or reproduction in other forums is permitted, provided the original author(s) and the copyright owner(s) are credited and that the original publication in this journal is cited, in accordance with accepted academic practice. No use, distribution or reproduction is permitted which does not comply with these terms.
*Correspondence: Shingo Miyata, c21peWF0YUBtZWQua2luZGFpLmFjLmpw
Disclaimer: All claims expressed in this article are solely those of the authors and do not necessarily represent those of their affiliated organizations, or those of the publisher, the editors and the reviewers. Any product that may be evaluated in this article or claim that may be made by its manufacturer is not guaranteed or endorsed by the publisher.
Research integrity at Frontiers
Learn more about the work of our research integrity team to safeguard the quality of each article we publish.