- 1Department of Nursing, The First Affiliated Hospital of Chongqing Medical University, Chongqing, China
- 2Department of Geriatrics, The First Affiliated Hospital of Chongqing Medical University, Chongqing, China
- 3Department of General Practice, The First Affiliated Hospital of Chongqing Medical University, Chongqing, China
- 4Department of Histology and Embryology, Faculty of Basic Medical Sciences, Chongqing Medical University, Chongqing, China
- 5Laboratory of Stem Cell and Tissue Engineering, Chongqing Medical University, Chongqing, China
- 6Institute of Neuroscience, Chongqing Medical University, Chongqing, China
Objective: To investigate the effects of sport stacking on the overall cognition and brain function in patients with mild Alzheimer's disease (AD) and mild cognitive impairment (MCI).
Methods: A single-blind randomized controlled design was performed using sport stacking for 30 min, 5 days/week for 12 weeks. Forty-eight subjects with mild AD or MCI were randomly divided into the sport stacking group (T-mAD = 12, T-MCI = 12) and the active control group (C-mAD = 11, C-MCI = 13). Auditory Verbal Learning Test (AVLT), Alzheimer's Disease Cooperative Study–Activities of Daily Living scale (ADCS-ADL), Geriatric Depression Scale (GDS-30), and Pittsburgh Sleep Quality Index (PSQI) were performed, the level of amyloid β-protein-40 (Aβ-40), Aβ-42, brain-derived neurotrophic factor (BDNF), insulin-like growth factor-1(IGF-1), tumor necrosis factor-alpha (TNF-α), Interleukin-6 (IL-6), and soluble trigger receptor expressed on myeloid cells 2 (sTREM2) in plasma were tested, and brain functional connectivity in resting state and activation under finger movement task were analyzed by functional near-infrared spectroscopy (fNIRS).
Results: Thirty-nine patients completed the trial. After 4 weeks, we found a significant increase in AVLT score in T-MCI (6.36 ± 5.08 vs. −1.11 ± 4.23, p = 0.004), and T-mAD group (4.60 ± 4.77 vs. −0.11 ± 2.89, p = 0.039). After 12 weeks, there was a significantly improved in AVLT (9.64 ± 4.90 vs. −0.33 ± 6.10, p = 0.002) and ADCS-ADL (3.36 ± 3.59 vs. −1.89 ± 2.71, p = 0.003) in T-MCI. There was a significant improvement in AVLT (5.30 ± 5.42 vs. 0.44 ± 2.40) in T-mAD (p < 0.05). Plasma levels of BDNF were upregulated in both T-MCI and T-mAD, and IGF-1 increased in T-MCI (P < 0.05) compared to the control groups. The functional connectivity in MCI patients between DLPFC.R and SCA.R, SMA.L, and SCA.R was decreased. In contrast, in mAD patients, the brain regional function connection was increased between DLPFC.R and Broca's.L. The activation of channel 36 located in the left primary somatosensory cortex was significantly increased after 12-week training, which was correlated with the improved AVLT and the increase of BDNF.
Conclusion: Our findings suggested that sport stacking is effective for patients with MCI and mild AD, possibly through increasing the expression of neuroprotective growth factors and enhancing neural plasticity to improve neurocognitive performance.
Clinical Trial Registration: https://www.ClinicalTrials.gov, ChiCTR.org.cn, identifier: ChiCTR-2100045980.
Introduction
Mild cognitive impairment (MCI) is an intermediate state between normal aging and dementia, with about 15% would progress to dementia in 2 years (Petersen et al., 2018), and one-third (32%) develop Alzheimer's disease (AD) within 5 years (Ward et al., 2013). As predicted, the number of people with dementia will reach to 78 million by 2030 (Gauthier et al., 2021). However, there is still a lack of effective pharmacological treatment today for AD, the most common cause of dementia. In recent years, non-pharmacologic treatments have drawn wide attention by showing their significant role in improving and maintaining cognitive function, quality of life, and daily function in patients with different severity of cognitive impairment. Moreover, a recent review found non-pharmacologic treatments seemed to be more effective than pharmacologic treatments for agitation and aggression in people with dementia (Watt et al., 2019). Aerobic exercise, the mainly studied physical activity among non-pharmacological treatments, has been shown to enhance the cognitive function of people with AD, reduce the risk of AD and other dementia, and delay the onset or progression (Groot et al., 2016; Hamer et al., 2018; Song et al., 2018). However, regular aerobic exercise may be difficult for dementia patients to adhere (Yágüez et al., 2011) for poor interest (Maltais et al., 2019) and low participation of the elderly (Padala et al., 2017).
Sport stacking is a new sport that began in the early 1980s. Participants use 12 specialized cups with both hands to make a pyramid (“up stacking”) and then return the cups into stacks (“down stacking”). The whole process must be in predetermined sequences (Hart et al., 2005). Sport stacking could be seen more suitable for patients with cognitive impairment because it is combined with the game and physical activity, which can trigger a high willingness to participate (Park, 2017). Previous studies showed that sport stacking was beneficial in many aspects, such as hand-eye coordination (Hart et al., 2006), reaction time (Liggins et al., 2007), bilateral coordination (Rhea et al., 2006), and dual hemispheric brain activity (Hart and Bixby, 2005). Some studies have applied sport stacking to stroke patients and found significant improvement in reaction time (Tretriluxana et al., 2014). Despite its beneficial role for cognitive improvements in other diseases, there is still a lack of evidence for the effect of sport stacking on people with dementia.
Growing evidence suggests that AD is a multifactorial disease that affects the central nervous system and systemic processes (Morris et al., 2014). More specifically, increased inflammation and production of reactive oxygen species (ROS) might also play an essential role in the pathophysiology of MCI and AD (Gilgun-Sherki et al., 2001; Rosenberg, 2005; Koyama et al., 2013). Evidence shows that physical activity appears to have a positive effect on inflammation, oxidation, and neurotrophic biomarkers by enhancing the antioxidant activity of plasma and reducing the serum expression of proinflammatory cytokines, which may affect the destructive effects of oxidative stress and inflammation in nerve tissue (Stigger et al., 2019). Physical exercise has been shown to produce an increase in brain-derived neurotrophic factor (BDNF) and variable response to insulin-like growth factor-1 (IGF-1) (Anderson-Hanley et al., 2018). However, the evidence for the effect of sport stacking on these biomarkers as well as AD biomarkers is unknown.
Functional Near-Infrared Spectroscopy (fNIRS) is a new brain mechanism functional imaging technology which can perform advanced cognition and interactive behavior in natural situations. It makes up for the limitations of detection tools such as single-photon emission computed tomography (SPECT), positron emission tomography (PET), and functional magnetic resonance imaging (fMRI) (Liu et al., 2011; Yeung and Chan, 2020). fNIRS can evaluate the activation of different brain regions by observing the changes of oxygenated hemoglobin (Oxy-Hb), deoxyhemoglobin (Deoxy-Hb), and total hemoglobin (Total-Hb) concentration curves in different brain regions during the cognitive process. Hoshi (2011) indicated that monitoring the changing trend of blood oxygen concentration in the prefrontal cortex while completing cognitive tasks could objectively reflect the subjects' cognitive level. Since the abilities of language comprehension, execution of an action, working memory, and movements are needed in fulfilling sport stacking, the brain regions of interest (ROI) were selected in corresponding to these functions. Recent studies have shown that the dorsolateral prefrontal cortex (DLPFC) is a crucial area for processing various behavioral tasks, and specifically, the right DLPFC (DLPRC.R) modulates the direction of these tasks (Xia et al., 2021) and semantic cognition (Herbet et al., 2018). Broca's area, a prefrontal region that was demonstrated to not only be involved in language production and comprehension but also play a role in several non-language-related functions such as working memory, execution, and perception of action (Clos et al., 2013; Kepinska et al., 2018). In addition, the subcentral area (SCA), also known as the subcentral motor cortex, and left supplementary motor area (SMA.L) are correlated with hand movements and grip (White et al., 2013; Auer et al., 2018; Eichert et al., 2021). Therefore, we select DLPFC.R, SCA.R, SMA.L, and Broca's area as the ROIs in this study.
This study aimed to investigate the effects of sport stacking on the neurocognitive performances, molecular biomarkers, and brain function performances in patients with mild AD and MCI. We hypothesized that sport stacking would effectively improve the cognitive function of patients with mild AD and MCI, potentially through divergent molecular factors (e.g., neuroprotective growth factors and cytokines) and the changes in activation of brain areas examined by fNIRS.
Materials and Methods
Study Design
The current study was designed as a single-blind randomized controlled trial (RCT) of 12 weeks of sport stacking vs. a non-exercise and clinic routine management control group. Participants were included from May 2021 to September 2021. The Medical Ethics Committee of the First Affiliated Hospital of Chongqing Medical University approved the research protocol. The study was conducted in compliance with the Declaration of Helsinki's ethical standards (World Medical Association, 2013). It was registered on the Chinese Clinical Trial Registry (Registration No.: ChiCTR2100045980). Participants all agreed to participate in the study and gave written informed consent.
Participants
Eligible elderly participants with a clinical diagnosis of dementia (DSM-IV) or NINCDS-ADRDA Alzheimer's Criteria would be recruited from the geriatric memory clinic at the First Affiliated Hospital of Chongqing Medical University between May 2021 and September 2021. Eligibility criteria for inclusion were: 1) between 60 and 90 years of age; 2) a Clinical Dementia Rating (CDR) score of 0.5 or 1; 3) at least 3 months of stable doses if receiving antidementia medication or mood-stabilizing medication; 4) basic communication skills and normal vision and hearing; and 5) informed consent. Exclusion criteria were: 1) severe psychiatric illness and the use of antidepressants; 2) alcohol or drug abuse; 3) participation in exercise more than twice weekly on a regular basis; and 4) any medical condition precluding participation in the exercise program (e.g., severe cardiovascular, musculoskeletal, or neurological disease).
Sample Size
Cognition (ADAS-Cog) was the primary outcome measure. We calculated that a sample size of 40 participants would provide 80% power (at a two-tailed α-level of 0.05) for detecting differences between groups for an effect size of 0.46 in the ADAS-Cog (Orrell et al., 2012). Assuming a 20% attrition rate, the study recruited a total of 48 participants (24 per group). Sample size calculation was performed using G*Power 3.1.9 (Faul et al., 2007).
Randomization and Blind
Forty-eight patients who met the inclusion criteria were randomized 1:1 ratio to receive either the intervention (sport stacking) or control (clinic routine management) via a computer-generated randomization sequence by a statistician. Allocation concealment was ensured since the randomization was performed by a research assistant who was not involved in the assessment or intervention. This was a single-blind study in which study participants were blinded to the group allocation.
Procedure
In the sport stacking group, participants and their caregivers were taught how to stack the cups in a specific sequence with the correct technique using the lesson plans recommended in the Speed Stacks® instructor guide (Speed Stacks, 2014) by an experienced instructor at the geriatric clinic or online from baseline to the 3rd month.
Participants needed a set of stacking tools to practice, including 12 cups, a timer, and a stacking mat. To facilitate the self-practice at home, a set of audiovisual videos showing the skills trained in all lessons was provided as a reminder to guide the participants' self-practice. The whole training lessons consist of 3 patterns, including 3–3–3, 3–6–3, and the Cycle. Because of the characteristics of elderly participants with dementia, three lessons were divided into 7 stages, including 3, 3–3–3, 6, 3–6–3, 3–6–3 & 6–6, 1–10–1, and the Cycle. The difficulty of the stages increased per level to ensure that the training remained cognitively challenging. Participants were asked to practice sport stacking at least 30 min a day and at least 5 days a week for a total of 3 months at home. Meanwhile, the participants were asked to record each time they finished sport stacking and the duration of their daily self-practice at home. The caregivers were encouraged to assist the participants in the practice and compliance recording process. The participants or their caregivers were asked to video-tape the participants' self-practice on the first and last day of each week to the researcher for collecting feedback. These videos could be used to check the participants' mastery of the sport stacking mode and correct their inaccuracies promptly. Adherence to the intervention was calculated by the number of pages of the self-recording logbook and the videos of participants' self-practice.
In the control group, all participants (MCI and mAD) received routine management from the Memory Clinic, mainly including (1) Regular medication; (2) Basic health education (medication, diet, exercise, etc.).
Experimental Procedure and Measurements
The neuropsychological evaluations were administrated at three timepoints, namely baseline before randomization, immediately after 4 weeks and after 12 weeks of sport stacking training. Blood tests and fNIRS measurements were conducted at baseline and 12 weeks. The assessments were carried out at the Memory Clinic, Department of Geriatrics, The First Affiliated Hospital of Chongqing Medical University. Under certain special circumstances, the neuropsychological evaluations of some of the participants were carried out online. And trained research assistants were blind to group allocation.
Neuropsychological Evaluation
The Mini-mental State Examination (MMSE; Folstein et al., 1975), and the Alzheimer's Disease Assessment Scale-Cognitive Subscale (ADAS-Cog; Mohs et al., 1983) were used to evaluate the global cognition, behavior, and ability to manage daily life. The Auditory Verbal Learning Task (AVLT; Arnáiz et al., 2004) was used to assess their memory. We used the clock drawing test (CDT; Sunderland et al., 1989) to assess executive functions. The 30-Geriatric Depression Scale (GDS-30; Yesavage et al., 1982) was used to rate the severity of depressive symptoms. The Alzheimer's Disease Cooperative Study–Activities of Daily Living scale (ADCS-ADL; Galasko et al., 1997) was used to assess activities of daily living function of patients with AD and MCI. The Pittsburgh Sleep Quality Index (PSQI; Buysse et al., 1989) was used to measure sleep quality.
Neurobiological Measurements
A 10-mL blood sample was obtained by a qualified nurse from the antecubital vein prior to and after the intervention. The blood samples were drawn to analyze serum amyloid β-protein (e.g., Aβ-40, Aβ-42), neuroprotective growth factors (e.g., BDNF, IGF-1), cytokines (e.g., TNF-α, IL-6), and markers of microglia activation and reactive oxygen species (e.g., sTREM2) levels. The blood samples were centrifuged at 3,000 rpm for 15 min, and serum was isolated and kept at −80°C until the next step. The levels of serum amyloid β-protein, neuroprotective growth factors, inflammatory cytokines, and plasma sTREM2 were analyzed using the Human ELISA kit (Shanghai Jianglai Industrial Limited by Share Ltd., Shanghai, China).
fNIRS Tasks, Acquisition and Analysis
Resting State and Finger Movement Task
The 8-min resting state at the sitting position took place in a sound-attenuated room. During the resting state recording, the participants were required to stay still and keep their eyes closed without falling asleep.
The finger movement task refers to a simple finger sequence (SFS) (Anwar et al., 2016), which requires the participants to sequentially tap the index, middle, ring, and the fourth finger against the thumb on both hands simultaneously. A block design was used in which subjects were asked to perform the finger movement task (SFS) for 20 s followed by 20 s rest for 6 repeat times (Figure 1A).
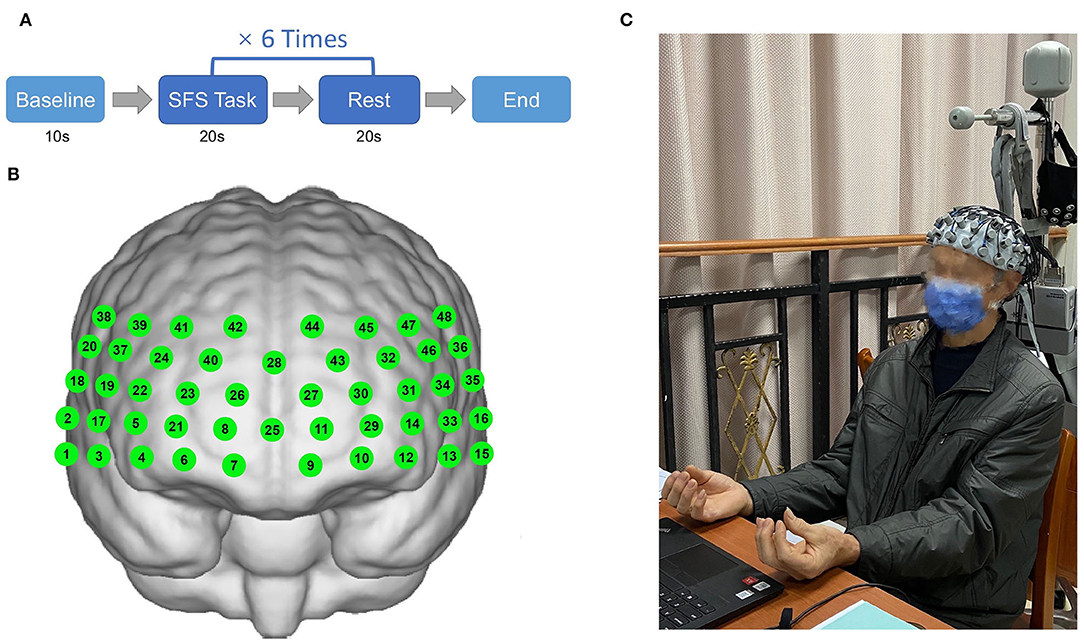
Figure 1. Illustration of finger movement task (simple finger sequence) paradigm (A) and positions of 48 channels (B,C) (Informed consent was obtained from the patient).
fNIRS Acquisition
In this experiment, the hemoglobin concentrations were measured using a multi-channel fNIRS system (NirScan, Danyang Huichuang Medical Equipment Co. Ltd, China). The sampling frequency was 11 Hz, and the wavelengths were 760 and 850 nm, according to the requirements of the internationally-used 10/20 electrode distribution system. We used the FPz channel (10/20 international system) as the center of the middle probe; a total of 31 SD probes (consisting of 15 sources and 16 detectors) with a fixed 3-cm inter-probe distance were placed to cover each subject's bilateral prefrontal cortex (PFC) and temporal cortices, and the lowest probes were positioned along with the Fp1–Fp2 line (Figures 1B,C). A total of 48 NIRS channels were established. The channels and the corresponding brain regions are consistent with a previous study (Liu et al., 2022).
Pre-processing
We used the NirSpark software package v1.7.3 (Danyang Huichuang Medical Equipment Co. Ltd, China) to analyze NIRS data. Data were preprocessed via the following steps. Motion artifacts were corrected by a moving SD and a cubic spline interpolation method. All differential path-length factors (DPF) were set to 6.0. According to previous studies (Li et al., 2021; Zhang et al., 2021), a bandpass filter with cut-off frequencies of 0.01–0.20 Hz was used to minimize noise, global trends, and biological signals (e.g., respiration and cardiac activity). The modified Beer-Lambert law was applied to convert optical densities into changes in Oxy-Hb and Deoxy-Hb concentrations. We used Oxy-Hb as our primary indicator in the following analysis because the Oxy-Hb signal generally has a better signal-to-noise ratio than Deoxy-Hb (Strangman et al., 2002).
Block Average
SFS block waveforms were calculated with a pre-baseline range set of 0–10 s and a block range set of 0–40. We used a 20 s task period of constructing phrases as the time window to analyze mean Oxy-Hb during the task and compare 20 s task period with 20 s rest period to analyze the Oxy-Hb change between task and baseline.
GLM Analysis
The generalized linear model (GLM) was used to analyze the Oxy-Hb time series data. The t-test was performed on the baseline and task state signals for each channel of each subject. The canonical hemodynamic response function (HRF) with time and dispersion derivative was selected as the basic function of the GLM. Through calculating the match between the experimental HRF value and the designs, the GLM can derive a value of activation coefficient β value representing the intensity of activation triggered by the task in the subject's cerebral cortex.
Functional Connectivity Analysis
The regions of interest (ROI) were selected as right dorsolateral prefrontal cortex (DLPFC.R) (channel 26, 28, and 40), left Broca's Area (Broca's. L) (channel 30, 31, and 32), left supplementary motor area cortex (SMA.L) (channel 46), and right subcentral area (SCA.R) (channel 17). For each resting-state dataset, functional connectivity (FC) was analyzed by performing Spearman's correlation between the time series of each channel-to-channel pair, resulting in a 48 × 48 matrix of R-values (Supplementary Figure 1).
Statistical Analysis
All statistical analyses were performed by IBM SPSS ver. 26.0 (IBM Corp., Armonk, USA). The NirSpark software package v1.7.3, GraphPad Prism 9, and Photoshop software were used to generate figures and graphs. The Shapiro–Wilk test and the Levene test were used to determine the normality and homoscedasticity of the data. All data expressed as mean ± standard deviation (SD). All the (outcome) variables were analyzed for differences between control and intervention groups using independent-sample Student's t-tests and the Mann–Whitney U-test for continuous variables, and Fisher's exact tests for categorical variables. The between-group difference in the change of score of the continuous variables from baseline to follow-up at 4 and 12 weeks were analyzed by using delta(Δ)-linear mixed models (Δ = change from baseline), where “change from baseline” represents the difference between the baseline and follow-up groups for each diagnosis, after adjusted for the premeasurement covariates (age and sex) for primary and secondary endpoint outcomes. Unpaired t-tests were used to compare mean and Oxy-Hb changes between mild AD and MCI cohorts, and paired t-tests were used to compare the R-values of FC and β-value of task activation between baseline and 12-week follow-up in T-mAD and T-MCI group. A p < 0.05 was considered statistically significant, and all p-values were two-tailed. The statistical results were corrected for multiple comparisons across channels by the false discovery rate (FDR). Additionally, we calculated the Pearson correlation coefficient between changes in brain region activation (β-value), BDNF levels, and neuropsychological performances (AVLT) in the T-mAD group.
Results
A total of 48 patients participated in the study, and 39 patients completed the study (21 in the intervention group and 18 in the control group). During this period, 39 patients completed neuropsychological assessments, 32 patients gave blood samples, and 29 completed measurements of fNIRS at baseline and 12-week follow-up (mAD = 15, MCI = 1 4 ).
Characteristics of the Study Population
A total of 48 eligible patients, 23 with MCI and 25 with mild AD, were enrolled in the study. Twenty-four were assigned to the intervention group (IG) and 24 to the control group (CG). Before the end of the trial, 9 participants withdrew (2 loss of interest; 2 refused to hospital; 2 no time; 1 poor health; 1 unable to contact and 1 other reasons), resulting in a drop-out rate of 18.8%. Thirty-Nine patients [26 female (66.7%); mean (SD) age, 73 (6.9) years] completed the study at last (IG: n = 21; CG: n = 18). The Consolidated Standards of Reporting Trials (CONSORT) flowchart outlining the number of participants from screening to study completion at 12-week follow-up is shown in Figure 2. There were no significant statistical differences in characteristics or neuropsychological assessment results between the groups at baseline. Baseline characteristics of the study population are shown in Table 1.
Neuropsychological Tests
As shown in Tables 2, 3, after the 4-week intervention, there was a significant improvement in AVLT in T-MCI (6.36 ± 5.08 vs. −1.11 ± 4.23) and T-mAD (4.60 ± 4.77 vs. −0.11 ± 2.89) compared with the corresponding control group (P < 0.05). After the 12-week intervention, there was a significantly improved AVLT (9.64 ± 4.90 vs. −0.33 ± 6.10) and ADCS-ADL (3.36 ± 3.59 vs. −1.89 ± 2.71) in T-MCI, and there was a significant improvement in AVLT (5.30 ± 5.42 vs. 0.44 ± 2.40) in T-mAD compared with the corresponding control group (p < 0.05).
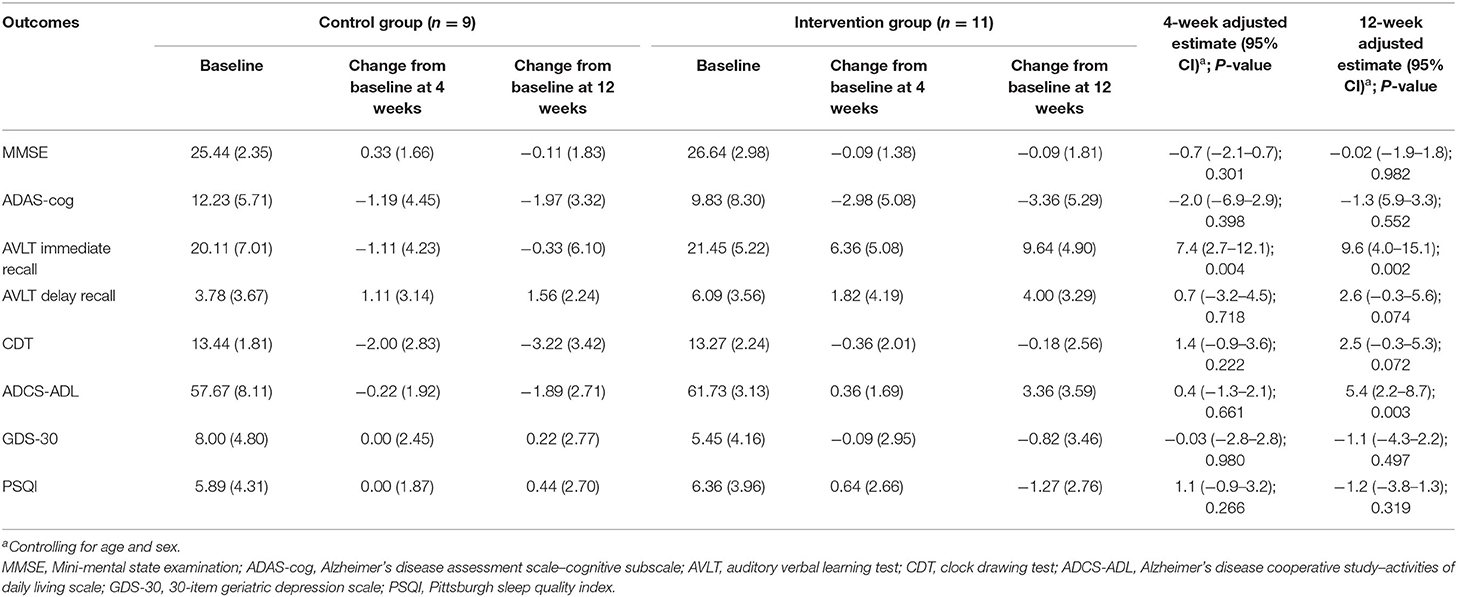
Table 2. Adjusted comparison of neuropsychological outcomes between changes from baseline at 4 and 12 weeks after intervention in patients with MCI (n = 20).
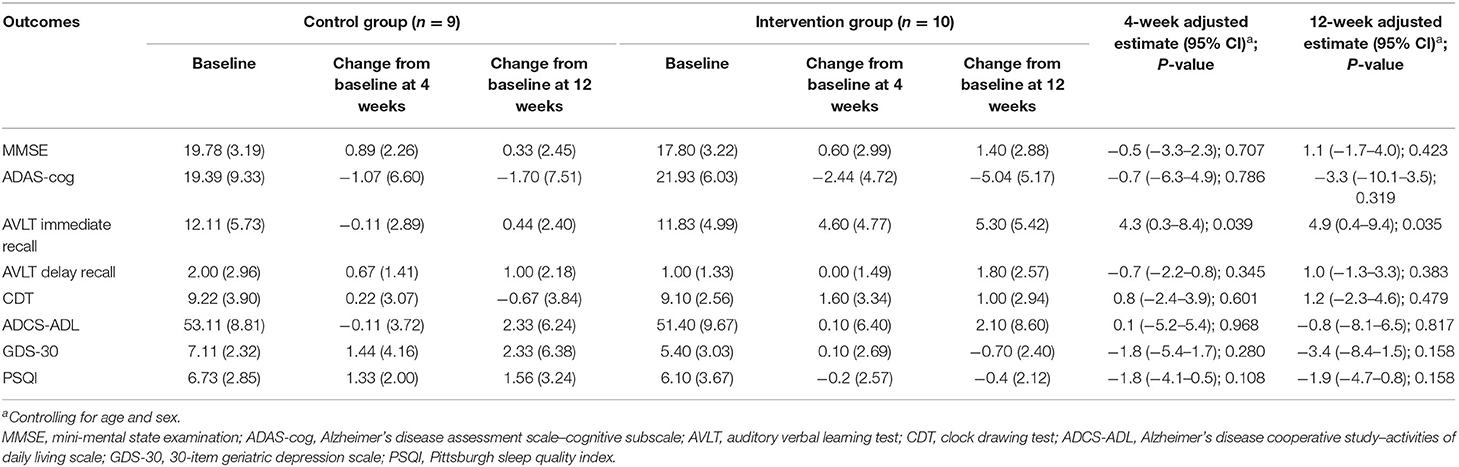
Table 3. Adjusted comparison of neuropsychological outcomes between changes from baseline at 4 and 12 weeks after intervention in patients with mild AD (n = 19).
Neurobiological Tests
As shown in Tables 4, 5, most inflammatory markers remained unchanged after sport stacking. After the 12-week intervention, there was a significant improvement in BDNF in T-MCI (41.6 ± 24.3 vs. −7.5 ± 55.2 ng/ml) and T-mAD (29.9 ± 33.4 vs. −23.5 ± 25.5 ng/ml), as compared with the corresponding control group (p < 0.05). We found a significantly increased IGF-1 (2.7 ± 5.3 vs. −4.7 ± 11.6 ng/ml) and IL-6 (0.5 ± 0.2 vs. 1.0 ± 0.2 pg/ml) in T-MCI compared with the control group (p < 0.05).
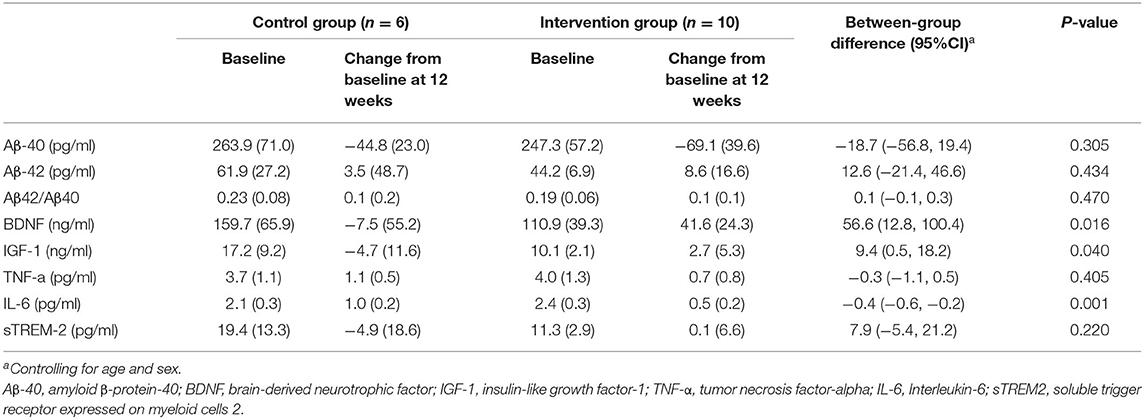
Table 4. Adjusted comparison of neurobiological measurements between changes from baseline at 12 weeks after intervention in patients with MCI (n = 16).
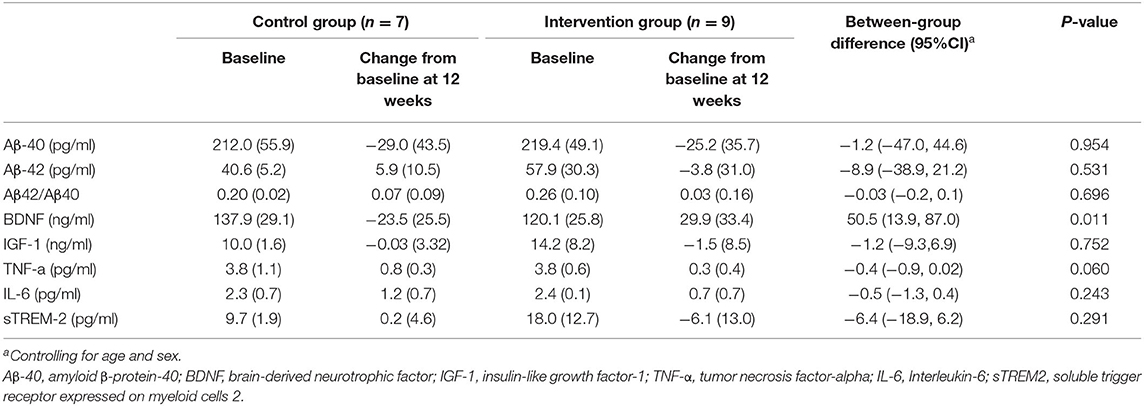
Table 5. Adjusted comparison of neurobiological measurements between changes from baseline at 12 weeks after intervention in patients with mild AD (n = 16).
Inter-cohort fNIRS Analysis
We firstly compared the mean Oxy-Hb concentration during the SFS task between mild AD cohort and MCI cohort, as well as the Oxy-Hb change between task and baseline. As is presented in Figures 3A,B,D, compared to MCI patients, significant lower Oxy-Hb concentrations during the task were exhibited in mild AD patients in channel 2, 4, 8, and 43 [mean with 95% CI, mAD vs. MCI, CH2: −0.0058 (−0.0205, 0.0088) vs. 0.0348 (0.0122, 0.0573), p = 0.006; CH4: −0.0054 (−0.0229, 0.01200) vs. 0.0329 (0.0137, 0.0521), p = 0.019; CH8: −0.0006 (−0.0142, 0.0129) vs. 0.0408 (0.0235, 0.0581), p = 0.008; CH43: −0.0053 (−0.0137, 0.0032) vs. 0.0354 (0.0178, 0.0530), p = 0.0004; all after FDR corrected]. Next, the Oxy-Hb change (task-rest) during SFS task in mild AD and MCI cohorts were shown in Figures 3C,E. Compared with MCI subjects, mild AD patients showed significant lower differences of Oxy-Hb level between the task and rest in channel 25 and channel 43 [mean with 95% CI, mAD vs. MCI, CH25: −0.0042 (−0.0208, 0.0126) vs. 0.0397 (0.0228, 0.0567), p = 0.0005; CH43: −0.0001 (−0.0101, 0.0098) vs. 0.0287 (0.0156, 0.0418), p = 0.0007; all after FDR corrected].
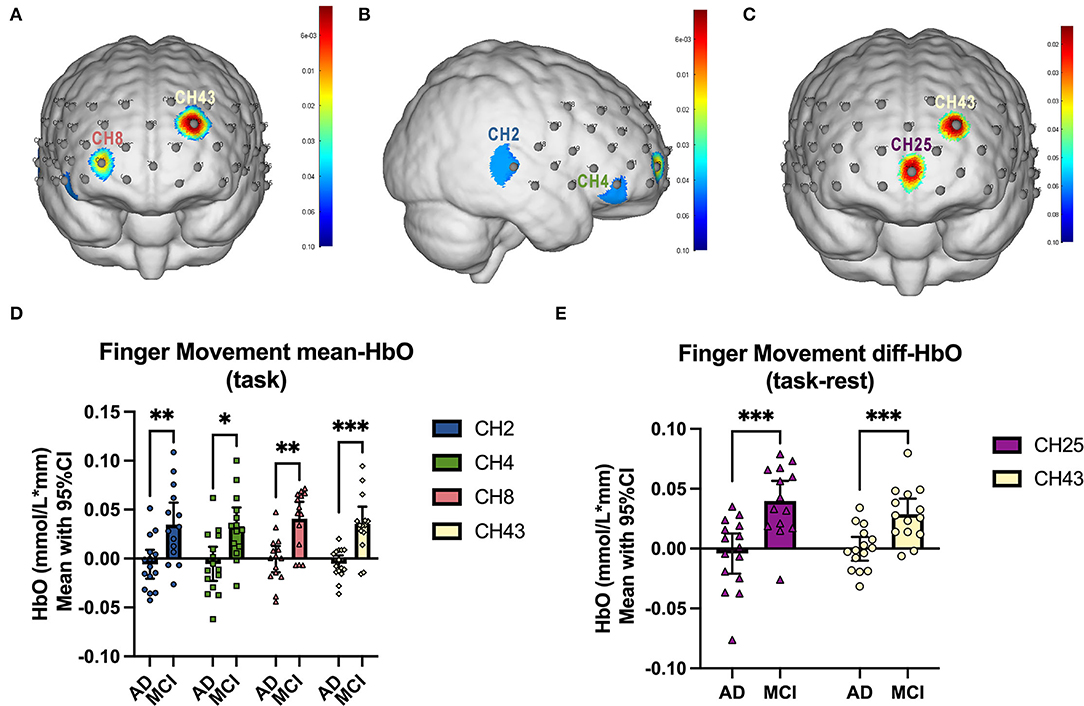
Figure 3. fNIRS comparison between mAD and MCI subjects. (A,B) The front and right lateral view of significant channels in mean Oxy-Hb comparison, and the statistics were presented in (D). (C) The front view of significant channels in diff-Oxy-Hb (task-rest) comparison, and the statistics were presented in (E). *p < 0.05; **p < 0.01; ***p < 0.001.
Functional Connectivity Change After Intervention
In both T-MCI and T-mAD groups, the changes of R-values among every two ROIs for each subject between baseline and 12-week follow-up were compared by paired t-test and were corrected by FDR. As is shown in Figure 4A, compared to baseline, patients with MCI (n = 10) showed significant decrease of FC between SCA.R and SMA.L (mean of R, baseline vs. follow-up: 0.5040 vs. 0.2744, p = 0.038, FDR corrected), and between SCA.R and DLPFC.R (mean of R, baseline vs. follow-up: 0.3454 vs. 0.1786, p = 0.038, FDR corrected) after sport stacking training for 12 weeks. As for T-mAD group (n=8), a significant increase of FC was analyzed between DLPFC.R and Broca's.L after sport stacking intervention (mean of R, baseline vs. follow-up: 0.1085 vs. 0.3727, p = 0.024, FDR corrected) (Figure 4B).
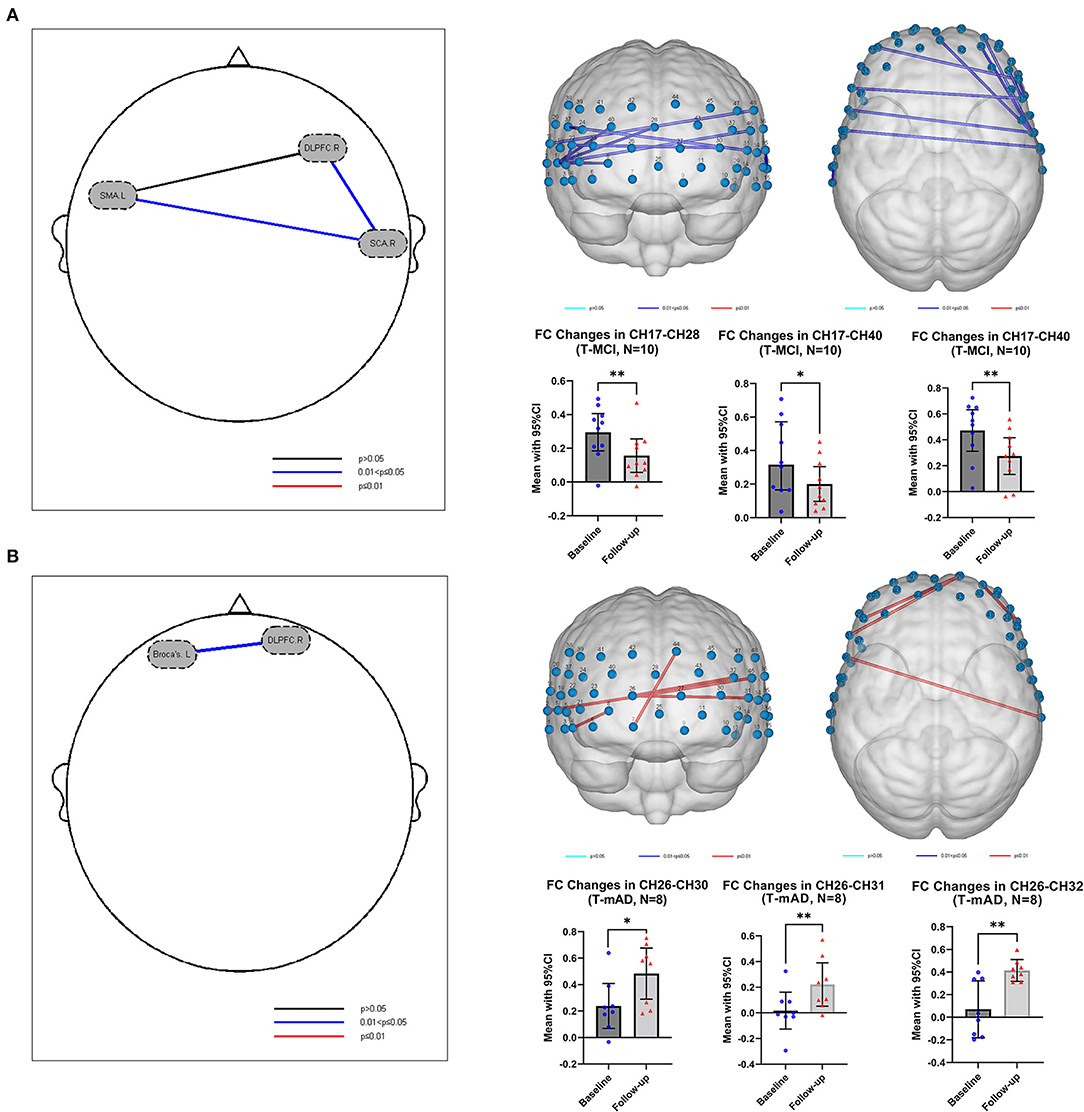
Figure 4. Functional connectivity change after intervention. (A) The functional connectivity change in T-MCI (n = 10) after sport stacking training for 12 weeks. (B) The functional connectivity change in T-mAD (n = 8) after sport stacking training for 12 weeks. Each dot represents the FC value of each channel in T-MCI and T-mAD participants at baseline and at follow-up. *p < 0.05. No significant differences between intervention groups and control groups were found. **p < 0.01.
Change of Brain Activation After Intervention
In SFS task fNIRS analysis, the β value derived from GLM from each channel represents cortical activation. We compared the β value of each channel for each T-MCI, T-mAD, C-MCI and C-mAD subjects between baseline and 12-weeek follow-up. We only found that in T-mAD group, CH36 area was significantly activated after sport stacking intervention, which overlaps cortex of left supramarginal gyrus (SMG.L) [mean with 95% CI of β, baseline vs. follow-up: 0.0119 (0.0004, 0.02329) vs. 0.0535 (0.03401, 0.0730), p = 0.0003, FDR corrected] (Figure 5), however, no significant differences between baseline and follow-up were found in the other three groups. Further, we correlated the changes of β value in CH36 of T-mAD subjects with their improved performance of ALVT (difference value) and changes of BDNF levels by Pearson Correlation analysis, and we found that the change of β value in CH36 was correlated with BDNF levels (coefficient value r = −0.780, p = 0.039) and with changes of AVLT scores (r = −0.875, p = 0.004), and AVLT performance was also correlated with increasement of BDNF levels (r = 0.763, p = 0.046). No significant differences were found in T-MCI, C-MCI and C-mAD groups, and no significant differences were found between T-MCI and C-MCI groups, and between T-mAD and C-mAD groups at follow-up.
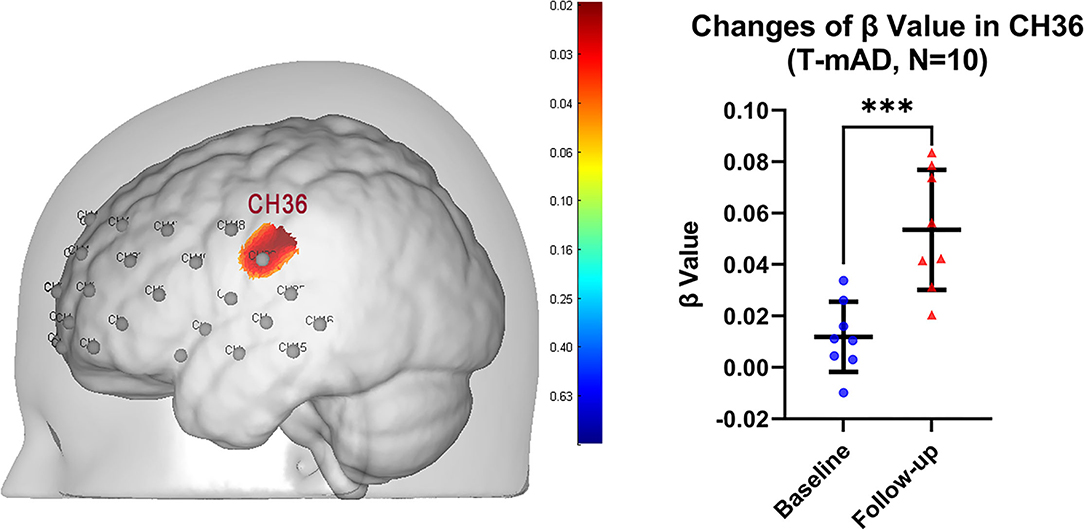
Figure 5. Change of brain activation after intervention in T-mAD subjects. CH36 area was significantly activated after sport stacking intervention (left) and statistical results (right). ***p < 0.001. Each dot represents the individual β value of T-mAD participants in CH36 at baseline and at follow-up. No significant brain activation differences in T-MCI subjects were found, and no significant differences between intervention groups and control groups were found.
Discussion
The present study was aimed at investigating the effects of sport stacking on cognitive performances in individuals with mild AD and MCI. There was apparent evidence of improved cognitive function in tests of AVLT and ADCS-ADL. Our results showed that the intervention effectively improved episodic memory of patients with mild AD and MCI and improved the activities of living of patients with MCI. Moreover, this 12-week sport stacking added to usual care successfully increased the expression of some neuroprotective growth factors, including BDNF in both mild AD and MCI patients and IGF-1 in MCI subjects. More importantly, we found the functional connectivity in MCI patients between DLPFC.R and SCA.R as well as between SMA.L and SCA.R decreased after training. In contrast, in mild AD patients, the brain regional function connection was increased between DLPFC.R and Broca's L. In addition, the activation of channel 36, which was located in the left primary somatosensory cortex, was significantly increased after 12-week of sport stacking, and this increased activation was correlated with the improved cognitive function (AVLT) as well as the increased level of BDNF. These findings support the hypothesis that sport stacking would improve the cognitive function of patients with mild AD and MCI and that sport stacking would have an upregulating effect on anti-inflammatory cytokines and neuronal plasticity.
Sport stacking, which combines game and exercise, is a new sport. Our study indicated that a significant increase in the score of AVLT was measured in the sport stacking group after 12 weeks which means our training could improve all patients' episodic memory. Our results were consistent with that reported in a randomized controlled study of Hagovska and Nagyova (2017), which showed that cognitive training combined with physical training could significantly improve AVLT scores, indicating greater improvement of the memory in older people with mild cognitive impairment. A systematic study of Law et al. (2014) presented similar results in the improvement of general cognitive functions and memory in older adults after the intervention of combined exercise and cognitive training. Similar studies in the literature also found improvements in the previously mentioned cognitive domains (Phirom et al., 2020). In addition, there is growing evidence that the combination of physical and cognitive activities may have synergistic effects (Kraft, 2012; Gheysen et al., 2018). Although physical exercise contributes to plasticity, cognitive activities lead to changes in plasticity (Fissler et al., 2013). This combined benefit from exercise and cognitive stimulation would be consistent with previous animal research, which showed that this cognitive benefit had been found to be from different mechanisms (cell proliferation and cell survival, respectively, Olson et al., 2006; Fabel et al., 2009). And this combined-effect hypothesis indicated that simultaneous exercise and cognitive interventions could further increase cognitive benefits. Similarly, in line with previous studies, these findings showed that when physical exercise was cognitively challenging, the cognitive benefits were greater than traditional exercise (Anderson-Hanley et al., 2012).
Besides improvements in cognitive performances, activities of living of patients with MCI, as assessed with the ADCS-ADL, ameliorated. Sport stacking is a coordinated exercise of hands and eyes. Hand movement can improve hand function, stimulate brain function, reduce the occurrence and development of brain-related diseases (Geng, 2012), and enhance the learning ability of students with intellectual disabilities (Qu et al., 2012). Nyberg et al. found that finger tapping can stimulate the motor cortex in the brain (Nyberg et al., 2006). Finger exercise can also maintain or improve the ability of daily living and self-care and handling tools in patients with dementia (Zhang et al., 2010). Wang and Kui (2014) also suggested that finger movement could improve ADL by promoting blood circulation in the brain and the central nervous system, thereby improving brain function.
However, we did not find significant mAD results in the intervention group for ADL and other cognitive domains, probably due to the short duration of our intervention, while the overall score of patients with mild AD is changing in the direction of improvement. There is evidence that the severity of neurocognitive impairment can regulate the cognitive effect of combined cognitive and sports training (Bamidis et al., 2015). The increase in the severity of neurocognitive impairment may decrease the effect of the intervention (Bamidis et al., 2015). This can be explained by a reduction in the structural brain capacity of participants with more severe neurocognitive impairment (e.g., reduced number of neurons and synapses), which may result in limited resources for training-induced benefits (Bamidis et al., 2015). As a result, it may be more difficult to induce cognitive benefits in people with dementia than in people with MCI or healthy older people.
Our results indicate that the concentration of BDNF in peripheral blood increased significantly in all patients who participated in 12-week sport stacking, and the concentration of IGF-1 in peripheral blood only increased significantly in patients with MCI. Consistent with the results of our study, previous research has suggested that multicomponent exercise could increase BDNF concentrations (Wang et al., 2020), and physical exercise has been shown to increase IGF-1 levels in patients with MCI (Baker et al., 2010). Exercise-related upregulation of BDNF and IGF-1 may help to offset the age-related decline in synaptogenesis, neurogenesis, angiogenesis, synaptic plasticity, and learning and memory, thus making the brain more flexible in dealing with age-related structural and functional neurodegeneration (Cotman and Berchtold, 2002, 2007). Together, these findings suggest that the production of BDNF and IGF-1 may be a mechanism responsible for cognitive improvement after sport stacking. In addition, our results show that non-strenuous exercise games such as sport stacking can improve BDNF and IGF-1 levels, which is of great significance for the exercise program of the elderly because the health status of the elderly is often unable to do strenuous exercise and hard to stick to.
Regarding IL-6, our results indicate that the change of IL-6 concentration in blood serum increased in both intervention groups, whereas only changes from the MCI group became statistically significant. Consistent with the results of our study, Behrendt et al. showed that both open and closed skill exercises were equally efficient in acutely increasing the IL-6 serum levels in healthy older adults (Behrendt et al., 2021). However, Forti et al. (2014) showed results, contrary to ours, that IL-6 levels significantly decreased after 12 weeks of strength training in 20 older adults. It is reported that peripheral IL-6 concentration increases sharply during physical exercise and returns to the baseline level within 24 h (Fischer, 2006). In this case, IL-6 is considered to have anti-inflammatory effects and may be a factor in reducing the risk of chronic inflammation and neurological diseases by exercising regularly (Funk et al., 2011; Smart et al., 2011). As IL-6 plays multiple roles in a variety of biological processes of the human body (Norman et al., 2018; Ellingsgaard et al., 2019), further research is needed to better understand the relationship between IL-6 and exercise and its impact on neurocognitive processes.
In this study, the differences of mean and difference of Oxy-Hb concentration between mild AD and MCI subjects indicated that the cortical blood flow and neuronal activity in mAD patients were significantly reduced, consistent with previous studies (Herrmann et al., 2008; Haberstumpf et al., 2022). One of the important findings of this study is the different outcomes of FC analysis in T-mAD and T-MCI during resting state, compared with baseline and 12-week follow-up. There was a decrease of FC between DLPFC.R and other ROIs in MCI, while an increase was displayed in subjects with mild AD. The DLPFC is an associative cortical region that is often described as a functional hub enabling a host of higher-order processes, including working memory (McKendrick et al., 2014), mentalizing, attention, and response inhibition (Rodrigo et al., 2014). In functional studies of healthy participants, faster processing speeds have been related to reduced directed functional connectivity and activation of the DLPFC (Motes et al., 2018). Based on these studies and our results, we speculated that the decrease of FC in between ROIs in MCI patients may correlate with the neuronal plasticity changes after sport stacking. Although DLPFC activation is commonly observed to increase in a parametric manner with workload (Ayaz et al., 2012), increased DLPFC activation may also occur as a compensatory mechanism to reductions in available neural resources (Stuss and Knight, 2002) or alternatively, inefficient utilization of neural resources (Neubauer and Fink, 2009). which may indicate that patients with MCI may have higher FC values between these ROIs at baseline because of functional compensation in these brain areas, but after sport stacking intervention, the neural remodeling, while improving cognitive function, also regulated the compensation of brain regions and thus reduced FC between ROIs were found. Our study also found the increased activation in CH36, overlapping the cortex of SMG.L, in mild AD subjects under the SFS task and was correlated with increased cognitive performance and upregulated level of BDNF. A previous study has demonstrated that SMG.L is involved in goal orientated cognition (Smallwood et al., 2021). This may prove that sport stacking may arouse a multi-system effect to improve cognition through enhancing neuronal plasticity and boosting the production of BDNF.
The present study has some limitations. Firstly, the sample size in our study is small and it might lead to statistical errors. In order to explore the further impact of sport stacking on the elderly with dementia and avoid statistical errors in the process of data analysis, future studies should expand the sample size; secondly, the period of our intervention was only 12 weeks, and we did not see any significant improvement in outcome indicators other than episodic memory in all patients, probably because the intervention time was relatively short; thirdly, although sport stacking was confirmed to be effective for individuals with MCI and AD, larger trials comparing sport stacking with other active control interventions such as aerobic exercise need to confirm or refute our findings. Finally, our results are restricted to patients with mild AD and MCI and cannot be generalized to those with more dementia types.
Although our study had these limitations, results still suggested the effectiveness of sport stacking and its benefits on participants' memory and activities of daily living, possibly via upregulation of BDNF and IGF-1. What's more, our study provides a new method for non-pharmaceutical treatment for patients with early stages of cognitive impairment.
Conclusion
Our findings suggested that sport stacking is effective for patients with MCI and mild AD, possibly through increasing the expression of neuroprotective growth factors and enhancing neural plasticity to improve neurocognitive performance.
Data Availability Statement
The raw data supporting the conclusions of this article will be made available by the authors, without undue reservation.
Ethics Statement
The studies involving human participants were reviewed and approved by Ethics Committee of the First Affiliated Hospital of Chongqing Medical University. The patients/participants provided their written informed consent to participate in this study.
Author Contributions
FD, YLü, ZY, WZ, YT, JL, DL, JY, and HJ contributed to study design, implementation, and interpretation. ZY, WZ, JS, YLi, and XL contributed to the management of data. ZY, DL, and WZ contributed to the analysis and interpretation of neuropsychological data. ZY, S-sZ, and YT contributed to biomarker data analysis and interpretation. WZ contributed to fNIRS data analysis and interpretation. ZY and WZ contributed to the drafting of the manuscript. FD, YLü, ZY, and WZ contributed to the critical revision of the manuscript. All the authors contributed to the data collection. All authors contributed to the article and approved the submitted version.
Funding
This work was supported by the Intelligent Medicine Project of Chongqing Medical University (Grant No. ZHYX2019003) and the Nursing Research Fund Project of the First Affiliated Hospital of Chongqing Medical University (Grant No. HLJJ2015-06 and HLJJ2020-06). This study was supported by the Geriatric Memory Clinic at the First Affiliated Hospital of Chongqing Medical University, the Department of Histology and Embryology and Laboratory of Stem Cell and Tissue Engineering in Chongqing Medical University, Chongqing, China.
Conflict of Interest
The authors declare that the research was conducted in the absence of any commercial or financial relationships that could be construed as a potential conflict of interest.
Publisher's Note
All claims expressed in this article are solely those of the authors and do not necessarily represent those of their affiliated organizations, or those of the publisher, the editors and the reviewers. Any product that may be evaluated in this article, or claim that may be made by its manufacturer, is not guaranteed or endorsed by the publisher.
Supplementary Material
The Supplementary Material for this article can be found online at: https://www.frontiersin.org/articles/10.3389/fnagi.2022.910261/full#supplementary-material
References
Anderson-Hanley, C., Arciero, P. J., Brickman, A. M., Nimon, J. P., Okuma, N., Westen, S. C., et al. (2012). Exergaming and older adult cognition: a cluster randomized clinical trial. Am. J. Prev. Med. 42, 109–119. doi: 10.1016/j.amepre.2011.10.016
Anderson-Hanley, C., Barcelos, N. M., Zimmerman, E. A., Gillen, R. W., Dunnam, M., Cohen, B. D., et al. (2018). The aerobic and cognitive exercise study (ACES) for community-dwelling older adults with or at-risk for mild cognitive impairment (MCI): neuropsychological, neurobiological and neuroimaging outcomes of a randomized clinical trial. Front. Aging Neurosci. 10, 76. doi: 10.3389/fnagi.2018.00076
Anwar, A. R., Muthalib, M., Perrey, S., Galka, A., Granert, O., Wolff, S., et al. (2016). Effective connectivity of cortical sensorimotor networks during finger movement tasks: a simultaneous fNIRS, fMRI, EEG Study. Brain Topogr. 29, 645–660. doi: 10.1007/s10548-016-0507-1
Arnáiz, E., Almkvist, O., Ivnik, R. J., Tangalos, E. G., Wahlund, L. O., Winblad, B., et al. (2004). Mild cognitive impairment: a cross-national comparison. J. Neurol. Neurosurg. Psychiatry 75, 1275–1280. doi: 10.1136/jnnp.2003.015032
Auer, T., Dewiputri, W. I., Frahm, J., and Schweizer, R. (2018). Higher-order brain areas associated with real-time functional mri neurofeedback training of the somato-motor cortex. Neuroscience 378, 22–33. doi: 10.1016/j.neuroscience.2016.04.034
Ayaz, H., Shewokis, P. A., Bunce, S., Izzetoglu, K., Willems, B., and Onaral, B. (2012). Optical brain monitoring for operator training and mental workload assessment. Neuroimage 59, 36–47. doi: 10.1016/j.neuroimage.2011.06.023
Baker, L. D., Frank, L. L., Foster-Schubert, K., Green, P. S., Wilkinson, C. W., McTiernan, A., et al. (2010). Effects of aerobic exercise on mild cognitive impairment: a controlled trial. Arch. Neurol. 67, 71–79. doi: 10.1001/archneurol.2009.307
Bamidis, P. D., Fissler, P., Papageorgiou, S. G., Zilidou, V., Konstantinidis, E. I., Billis, A. S., et al. (2015). Gains in cognition through combined cognitive and physical training: the role of training dosage and severity of neurocognitive disorder. Front. Aging Neurosci. 7, 152. doi: 10.3389/fnagi.2015.00152
Behrendt, T., Kirschnick, F., Kröger, L., Beileke, P., Rezepin, M., Brigadski, T., et al. (2021). Comparison of the effects of open vs. closed skill exercise on the acute and chronic BDNF, IGF-1 and IL-6 response in older healthy adults. BMC Neurosci. 22, 71. doi: 10.1186/s12868-021-00675-8
Buysse, D. J., Reynolds, C. F. 3rd, Monk, T. H., Berman, S. R., and Kupfer, D. J. (1989). The Pittsburgh Sleep Quality Index: a new instrument for psychiatric practice and research. Psychiatry Res. 28, 193–213. doi: 10.1016/0165-1781(89)90047-4
Clos, M., Amunts, K., Laird, A. R., Fox, P. T., and Eickhoff, S. B. (2013). Tackling the multifunctional nature of Broca's region meta-analytically: co-activation-based parcellation of area 44. Neuroimage 83, 174–188. doi: 10.1016/j.neuroimage.2013.06.041
Cotman, C. W., and Berchtold, N. C. (2002). Exercise: a behavioral intervention to enhance brain health and plasticity. Trends Neurosci. 25, 295–301. doi: 10.1016/S0166-2236(02)02143-4
Cotman, C. W., and Berchtold, N. C. (2007). Physical activity and the maintenance of cognition: learning from animal models. Alzheimers Dement. 3, S30–S37. doi: 10.1016/j.jalz.2007.01.013
Eichert, N., Watkins, K. E., Mars, R. B., and Petrides, M. (2021). Morphological and functional variability in central and subcentral motor cortex of the human brain. Brain Struct. Funct. 226, 263–279. doi: 10.1007/s00429-020-02180-w
Ellingsgaard, H., Hojman, P., and Pedersen, B. K. (2019). Exercise and health — emerging roles of IL-6. Curr. Opin. Physiol. 10, 49–54. doi: 10.1016/j.cophys.2019.03.009
Fabel, K., Wolf, S. A., Ehninger, D., Babu, H., Leal-Galicia, P., and Kempermann, G. (2009). Additive effects of physical exercise and environmental enrichment on adult hippocampal neurogenesis in mice. Front. Neurosci. 3, 50. doi: 10.3389/neuro.22.002.2009
Faul, F., Erdfelder, E., Lang, A. G., and Buchner, A. (2007). G*Power 3: a flexible statistical power analysis program for the social, behavioral, and biomedical sciences. Behav. Res. Methods 39, 175–191. doi: 10.3758/BF03193146
Fischer, C. P. (2006). Interleukin-6 in acute exercise and training: what is the biological relevance? Exerc. Immunol. Rev. 12, 6–33.
Fissler, P., Küster, O., Schlee, W., and Kolassa, I. T. (2013). Novelty interventions to enhance broad cognitive abilities and prevent dementia: synergistic approaches for the facilitation of positive plastic change. Prog. Brain Res. 207, 403–434. doi: 10.1016/B978-0-444-63327-9.00017-5
Folstein, M. F., Folstein, S. E., and McHugh, P. R. (1975). “Mini-mental state”. A practical method for grading the cognitive state of patients for the clinician. J. Psychiatr Res. 12, 189–198. doi: 10.1016/0022-3956(75)90026-6
Forti, L. N., Njemini, R., Beyer, I., Eelbode, E., Meeusen, R., Mets, T., et al. (2014). Strength training reduces circulating interleukin-6 but not brain-derived neurotrophic factor in community-dwelling elderly individuals. Age 36, 9704. doi: 10.1007/s11357-014-9704-6
Funk, J. A., Gohlke, J., Kraft, A. D., McPherson, C. A., Collins, J. B., and Jean Harry, G. (2011). Voluntary exercise protects hippocampal neurons from trimethyltin injury: possible role of interleukin-6 to modulate tumor necrosis factor receptor-mediated neurotoxicity. Brain Behav. Immun. 25, 1063–1077. doi: 10.1016/j.bbi.2011.03.012
Galasko, D., Bennett, D., Sano, M., Ernesto, C., Thomas, R., Grundman, M., et al. (1997). An inventory to assess activities of daily living for clinical trials in Alzheimer's disease. The Alzheimer's disease cooperative study. Alzheimer Dis. Assoc. Disord. 11 (Suppl. 2), S33–S39. doi: 10.1097/00002093-199700112-00005
Gauthier, S, Rosa-Neto, P, Morais, JA, and Webster, C. (2021). World alzheimer report 2021. Journey Through the Diagnosis of Dementia. Alzheimer's Disease International; 2021.
Geng, QB, (2012). The effect of finger exercises training in elderly patients with mild cognitive impairment. Guide China Med. 10, 488–489. doi: 10.15912/j.cnki.gocm.2012.24.489
Gheysen, F., Poppe, L., DeSmet, A., Swinnen, S., Cardon, G., De Bourdeaudhuij, I., et al. (2018). Physical activity to improve cognition in older adults: can physical activity programs enriched with cognitive challenges enhance the effects? A systematic review and meta-analysis. Int. J. Behav. Nutr. Phys. Act. 15, 63. doi: 10.1186/s12966-018-0697-x
Gilgun-Sherki, Y., Melamed, E., and Offen, D. (2001). Oxidative stress induced-neurodegenerative diseases: the need for antioxidants that penetrate the blood brain barrier. Neuropharmacology 40, 959–975. doi: 10.1016/S0028-3908(01)00019-3
Groot, C., Hooghiemstra, A. M., Raijmakers, P. G., van Berckel, B. N., Scheltens, P., Scherder, E. J., et al. (2016). The effect of physical activity on cognitive function in patients with dementia: a meta-analysis of randomized control trials. Ageing Res. Rev. 25, 13–23. doi: 10.1016/j.arr.2015.11.005
Haberstumpf, S., Seidel, A., Lauer, M., Polak, T., Deckert, J., and Herrmann, M. J. (2022). Reduced parietal activation in participants with mild cognitive impairments during visual-spatial processing measured with functional near-infrared spectroscopy. J. Psychiatr. Res. 146, 31–42. doi: 10.1016/j.jpsychires.2021.12.021
Hagovska, M., and Nagyova, I. (2017). The transfer of skills from cognitive and physical training to activities of daily living: a randomised controlled study. Eur. J. Ageing 14, 133–142. doi: 10.1007/s10433-016-0395-y
Hamer, M., Muniz Terrera, G., and Demakakos, P. (2018). Physical activity and trajectories in cognitive function: english longitudinal study of ageing. J. Epidemiol. Community Health 72, 477–483. doi: 10.1136/jech-2017-210228
Hart, M. A., and Bixby, W. R. (2005). EEG activation patterns during participation in a cup-stacking task. Res. Q. Exerc. Sport 76. A57.
Hart, M. A., Smith, L. A., and DeChant, A. (2005). Influence of participation in a cup-stacking unit on timing tasks. Percept. Mot. Skills 101, 869–876. doi: 10.2466/pms.101.3.869-876
Hart, M. A., Smith, L. A., and DeChant-Bruennig, A. (2006). Effect of participation in a cup stacking unit on hand-eye coordination of elementary children. Phys. Educ. 63, 154–159. Available online at: https://eric.ed.gov/?id=EJ768180
Herbet, G., Moritz-Gasser, S., and Duffau, H. (2018). Electrical stimulation of the dorsolateral prefrontal cortex impairs semantic cognition. Neurology 90, e1077–e1084. doi: 10.1212/WNL.0000000000005174
Herrmann, M. J., Langer, J. B., Jacob, C., Ehlis, A. C., and Fallgatter, A. J. (2008). Reduced prefrontal oxygenation in Alzheimer disease during verbal fluency tasks. Am. J. Geriatr. Psychiatry 16, 125–135. doi: 10.1097/JGP.0b013e3180cc1fbc
Hoshi, Y. (2011). Towards the next generation of near-infrared spectroscopy. Philos. Trans. A Math. Phys. Eng. Sci. 369, 4425–4439. doi: 10.1098/rsta.2011.0262
Kepinska, O., de Rover, M., Caspers, J., and Schiller, N. O. (2018). Connectivity of the hippocampus and Broca's area during acquisition of a novel grammar. Neuroimage 165, 1–10. doi: 10.1016/j.neuroimage.2017.09.058
Koyama, A., O'Brien, J., Weuve, J., Blacker, D., Metti, A. L., and Yaffe, K. (2013). The role of peripheral inflammatory markers in dementia and Alzheimer's disease: a meta-analysis. J. Gerontol. A Biol. Sci. Med. Sci. 68, 433–440. doi: 10.1093/gerona/gls187
Kraft, E. (2012). Cognitive function, physical activity, and aging: possible biological links and implications for multimodal interventions. Neuropsychol. Dev. Cogn. B Aging Neuropsychol. Cogn. 19, 248–263. doi: 10.1080/13825585.2011.645010
Law, L. L., Barnett, F., Yau, M. K., and Gray, M. A. (2014). Effects of combined cognitive and exercise interventions on cognition in older adults with and without cognitive impairment: a systematic review. Ageing Res. Rev. 15, 61–75. doi: 10.1016/j.arr.2014.02.008
Li, Y., Song, F., Liu, Y., Wang, Y., and Ma, X. (2021). Relevance of emotional conflict and gender differences in the cognitive tasks of digital interface layouts using NIRS technology. IEEE Access 9, 17382–17391. doi: 10.1109/ACCESS.2020.3048737
Liggins, T., Coleman, D., Solis, A., and Yuhua, L. (2007). Effects of a cup-stacking exercise program on motor development for school children. Res. Q. Exerc. Sport 78, A47.
Liu, B., Zhou, J., and Li, F. (2011). Functional near-infrared spectroscopy:an emergingfunctional neuroimaging technology. J. Psychol. Sci. 34, 943–949. doi: 10.16719/j.cnki.1671-6981.2011.04.003
Liu, X., Cheng, F., Hu, S., Wang, B., Hu, C., Zhu, Z., et al. (2022). Cortical activation and functional connectivity during the verbal fluency task for adolescent-onset depression: a multi-channel NIRS study. J. Psychiatr. Res. 147, 254–261. doi: 10.1016/j.jpsychires.2022.01.040
Maltais, M., Rolland, Y., Ha,ý, P. E., Armaingaud, D., Vellas, B., and de Souto Barreto, P. (2019). Six-month observational follow-up on activities of daily living in people with dementia living in nursing homes after a 6-month group based on either exercise or social activities. Aging Clin. Exp. Res. 31, 361–366. doi: 10.1007/s40520-018-0984-z
McKendrick, R., Ayaz, H., Olmstead, R., and Parasuraman, R. (2014). Enhancing dual-task performance with verbal and spatial working memory training: continuous monitoring of cerebral hemodynamics with NIRS. Neuroimage 85 (Pt. 3), 1014–1026. doi: 10.1016/j.neuroimage.2013.05.103
Mohs, R. C., Rosen, W. G., and Davis, K. L. (1983). The Alzheimer's disease assessment scale: an instrument for assessing treatment efficacy. Psychopharmacol. Bull. 19, 448–450.
Morris, J. K., Honea, R. A., Vidoni, E. D., Swerdlow, R. H., and Burns, J. M. (2014). Is Alzheimer's disease a systemic disease? Biochim. Biophys. Acta 1842, 1340–1349. doi: 10.1016/j.bbadis.2014.04.012
Motes, M. A., Yezhuvath, U. S., Aslan, S., Spence, J. S., Rypma, B., and Chapman, S. B. (2018). Higher-order cognitive training effects on processing speed-related neural activity: a randomized trial. Neurobiol. Aging 62, 72–81. doi: 10.1016/j.neurobiolaging.2017.10.003
Neubauer, A. C., and Fink, A. (2009). Intelligence and neural efficiency: Measures of brain activation versus measures of functional connectivity in the brain. Intelligence 37, 223–229. doi: 10.1016/j.intell.2008.10.008
Norman, J. E., Rutkowsky, J., Bodine, S., and Rutledge, J. C. (2018). The potential mechanisms of exercise-induced cognitive protection: a literature review. Curr. Pharm. Des. 24, 1827–1831. doi: 10.2174/1381612824666180406105149
Nyberg, L., Eriksson, J., Larsson, A., and Marklund, P. (2006). Learning by doing versus learning by thinking: an fMRI study of motor and mental training. Neuropsychologia 44, 711–717. doi: 10.1016/j.neuropsychologia.2005.08.006
Olson, A. K., Eadie, B. D., Ernst, C., and Christie, B. R. (2006). Environmental enrichment and voluntary exercise massively increase neurogenesis in the adult hippocampus via dissociable pathways. Hippocampus 16, 250–260. doi: 10.1002/hipo.20157
Orrell, M., Yates, L. A., Burns, A., Russell, I., Woods, R. T., Hoare, Z., et al. (2012). Individual Cognitive Stimulation Therapy for dementia (iCST): study protocol for a randomized controlled trial. Trials 13, 172. doi: 10.1186/1745-6215-13-172
Padala, K. P., Padala, P. R., Lensing, S. Y., Dennis, R. A., Bopp, M. M., Roberson, P. K., et al. (2017). Home-Based exercise program improves balance and fear of falling in community-dwelling older adults with mild Alzheimer's disease: a pilot study. J. Alzheimers Dis. 59, 565–574. doi: 10.3233/JAD-170120
Park, C. H. (2017). Experience of sport stacking in Korean older adults. J. Exerc. Rehabil. 13, 43–47. doi: 10.12965/jer.1734922.461
Petersen, R. C., Lopez, O., Armstrong, M. J., Getchius, T. S. D., Ganguli, M., Gloss, D., et al. (2018). Practice guideline update summary: Mild cognitive impairment: report of the guideline development, dissemination, and implementation subcommittee of the American academy of neurology. Neurology 90, 126–135. doi: 10.1212/WNL.0000000000004826
Phirom, K., Kamnardsiri, T., and Sungkarat, S. (2020). Beneficial effects of interactive physical-cognitive game-based training on fall risk and cognitive performance of older adults. Int. J. Environ. Res. Public Health 17, 6079. doi: 10.3390/ijerph17176079
Qu, Y., Zhang, C. H., and Zhang, H. X. (2012). Finger exercises can improve the activities of daily living in elderly patients with mild dementia. Shanghai Nurs. J. 12, 14–16. doi: 10.3969/j.issn.1009-8399.2012.04.004
Rhea, C., Ludwig, K., and Mokha, M. (2006). Changes in upper-limb coordination and kinematics follow-ing a five-week instructional unit on cup stacking. Ind. AHPERD 35, 27–28. doi: 10.1016/S1551-7977(08)70023-2
Rodrigo, A. H., Domenico, S. I., Ayaz, H., Gulrajani, S., Lam, J., and Ruocco, A. C. (2014). Differentiating functions of the lateral and medial prefrontal cortex in motor response inhibition. Neuroimage 85 (Pt. 1), 423–431. doi: 10.1016/j.neuroimage.2013.01.059
Rosenberg, P. B. (2005). Clinical aspects of inflammation in Alzheimer's disease. Int. Rev. Psychiatry 17, 503–514. doi: 10.1080/02646830500382037
Smallwood, J., Bernhardt, B. C., Leech, R., Bzdok, D., Jefferies, E., and Margulies, D. S. (2021). The default mode network in cognition: a topographical perspective. Nat. Rev. Neurosci. 22, 503–513. doi: 10.1038/s41583-021-00474-4
Smart, N. A., Larsen, A. I., Le Maitre, J. P., and Ferraz, A. S. (2011). Effect of exercise training on interleukin-6, tumour necrosis factor alpha and functional capacity in heart failure. Cardiol. Res. Pract. 2011, 532620. doi: 10.4061/2011/532620
Song, D., Yu, D. S. F., Li, P. W. C., and Lei, Y. (2018). The effectiveness of physical exercise on cognitive and psychological outcomes in individuals with mild cognitive impairment: a systematic review and meta-analysis. Int. J. Nurs. Stud. 79, 155–164. doi: 10.1016/j.ijnurstu.2018.01.002
Speed Stacks (2014). Sport Stacking Instructor Guide. Speed Stacks. Available online at: www.SpeedStacks.com/teach
Stigger, F. S., Zago Marcolino, M. A., Portela, K. M., and Plentz, R. D. M. (2019). Effects of exercise on inflammatory, oxidative, and neurotrophic biomarkers on cognitively impaired individuals diagnosed with dementia or mild cognitive impairment: a systematic review and meta-analysis. J. Gerontol. A Biol. Sci. Med. Sci. 74, 616–624. doi: 10.1093/gerona/gly173
Strangman, G., Culver, J. P., Thompson, J. H., and Boas, D. A. (2002). A quantitative comparison of simultaneous BOLD fMRI and NIRS recordings during functional brain activation. Neuroimage 17, 719–731. doi: 10.1006/nimg.2002.1227
Stuss, D. T., and Knight, R. T. (2002). Principles of Frontal Lobe Function. New York, NY: Oxford University Press, 628–652. doi: 10.1093/acprof:oso/9780195134971.001.0001
Sunderland, T., Hill, J. L., Mellow, A. M., Lawlor, B. A., Gundersheimer, J., Newhouse, P. A., et al. (1989). Clock drawing in Alzheimer's disease. A novel measure of dementia severity. J. Am. Geriatr. Soc. 37, 725–729. doi: 10.1111/j.1532-5415.1989.tb02233.x
Tretriluxana, J., Khacharoen, S., Hiengkaew, V., and Prayoonwiwat, N. (2014). Learning of the bimanual cup-stacking task in individuals with chronic stroke improved with dyad training protocol. J. Med. Assoc. Thai. 97 (Suppl. 7), S39–44.
Wang, X., Wang, H., Ye, Z., Ding, G., Li, F., Ma, J., et al. (2020). The neurocognitive and BDNF changes of multicomponent exercise for community-dwelling older adults with mild cognitive impairment or dementia: a systematic review and meta-analysis. Aging 12, 4907–4917. doi: 10.18632/aging.102918
Wang, Y., and Kui, J. K. (2014). Application of acupoint massage in nursing care of elderly patients with dementia. Yunnan J. Trad. Chin. Med. Mater. Med. 35, 81–82. doi: 10.16254/j.cnki.53-1120/r.2014.04.025
Ward, A., Tardiff, S., Dye, C., and Arrighi, H. M. (2013). Rate of conversion from prodromal Alzheimer's disease to Alzheimer's dementia: a systematic review of the literature. Dement. Geriatr. Cogn. Dis. Extra 3, 320–332. doi: 10.1159/000354370
Watt, J. A., Goodarzi, Z., Veroniki, A. A., Nincic, V., Khan, P. A., Ghassemi, M., et al. (2019). Comparative efficacy of interventions for aggressive and agitated behaviors in dementia: a systematic review and network meta-analysis. Ann. Intern. Med. 171, 633–642. doi: 10.7326/M19-0993
White, O., Davare, M., Andres, M., and Olivier, E. (2013). The role of left supplementary motor area in grip force scaling. PLoS ONE 8, e83812. doi: 10.1371/journal.pone.0083812
World Medical Association (2013). World Medical Association declaration of helsinki: ethical principles for medical research involving human subjects. JAMA 310, 2191–2194. doi: 10.1001/jama.2013.281053
Xia, X., Li, Y., Wang, Y., Xia, J., Lin, Y., Zhang, X., et al. (2021). Functional role of dorsolateral prefrontal cortex in the modulation of cognitive bias. Psychophysiology 58, e13894. doi: 10.1111/psyp.13894
Yágüez, L., Shaw, K. N., Morris, R., and Matthews, D. (2011). The effects on cognitive functions of a movement-based intervention in patients with Alzheimer's type dementia: a pilot study. Int. J. Geriatr. Psychiatry 26, 173–181. doi: 10.1002/gps.2510
Yesavage, J. A., Brink, T. L., Rose, T. L., Lum, O., Huang, V., Adey, M., et al. (1982). Development and validation of a geriatric depression screening scale: a preliminary report. J. Psychiatr. Res. 17, 37–49. doi: 10.1016/0022-3956(82)90033-4
Yeung, M. K., and Chan, A. S. (2020). Functional near-infrared spectroscopy reveals decreased resting oxygenation levels and task-related oxygenation changes in mild cognitive impairment and dementia: a systematic review. J. Psychiatr. Res. 124, 58–76. doi: 10.1016/j.jpsychires.2020.02.017
Zhang, C. H., Xu, Y., and Z, H. X. (2010). The effect of sequential care on the ability of daily life for elders with moderate dementia. Shanghai Nurs. J. 10, 30–33. doi: 10.3969/j.issn.1009-8399.2010.01.008
Zhang, N., Yuan, X., Li, Q., Wang, Z., Gu, X., Zang, J., et al. (2021). The effects of age on brain cortical activation and functional connectivity during video game-based finger-to-thumb opposition movement: a functional near-infrared spectroscopy study. Neurosci. Lett. 746, 135668. doi: 10.1016/j.neulet.2021.135668
Keywords: sport stacking, AD, MCI, Alzheimer's disease, mild cognitive impairment, neuropsychological, neurobiological, fNIRS
Citation: Yang Z, Zhang W, Liu D, Zhang S-s, Tang Y, Song J, Long J, Yang J, Jiang H, Li Y, Liu X, Lü Y and Ding F (2022) Effects of Sport Stacking on Neuropsychological, Neurobiological, and Brain Function Performances in Patients With Mild Alzheimer's Disease and Mild Cognitive Impairment: A Randomized Controlled Trial. Front. Aging Neurosci. 14:910261. doi: 10.3389/fnagi.2022.910261
Received: 01 April 2022; Accepted: 20 April 2022;
Published: 12 May 2022.
Edited by:
Ke Zhang, China Medical University, ChinaReviewed by:
Lee Wei Lim, The University of Hong Kong, Hong Kong SAR, ChinaYun Cheng, Fudan University, China
Copyright © 2022 Yang, Zhang, Liu, Zhang, Tang, Song, Long, Yang, Jiang, Li, Liu, Lü and Ding. This is an open-access article distributed under the terms of the Creative Commons Attribution License (CC BY). The use, distribution or reproduction in other forums is permitted, provided the original author(s) and the copyright owner(s) are credited and that the original publication in this journal is cited, in accordance with accepted academic practice. No use, distribution or reproduction is permitted which does not comply with these terms.
*Correspondence: Yang Lü, eWFuZ2x5dSYjeDAwMDQwO2hvc3BpdGFsLmNxbXUuZWR1LmNu; Fu Ding, MjAxNTk4JiN4MDAwNDA7aG9zcGl0YWwuY3FtdS5lZHUuY24=
†These authors have contributed equally to this work and share first authorship