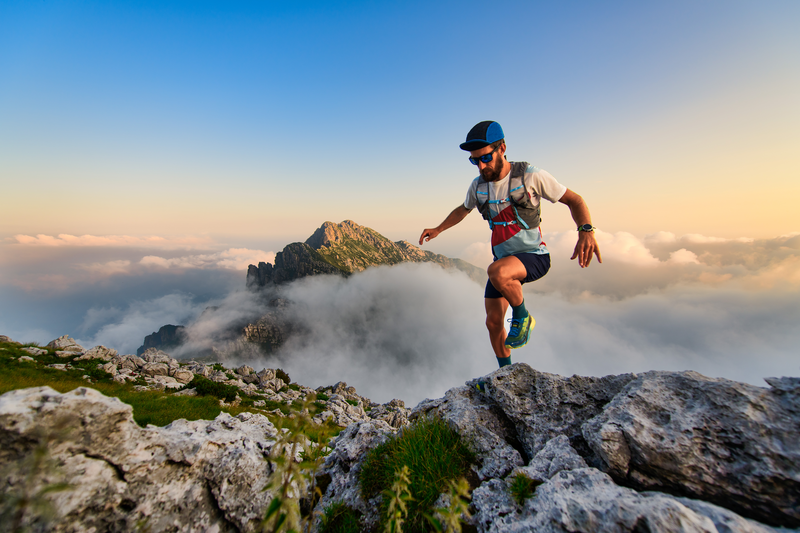
94% of researchers rate our articles as excellent or good
Learn more about the work of our research integrity team to safeguard the quality of each article we publish.
Find out more
OPINION article
Front. Aging Neurosci. , 26 May 2022
Sec. Cellular and Molecular Mechanisms of Brain-aging
Volume 14 - 2022 | https://doi.org/10.3389/fnagi.2022.902191
This article is part of the Research Topic The Many Faces of Brain Aging View all 8 articles
Aging is a major risk factor for developing neuroinflammation. As it progresses, neuroinflammation can cause neuron death in the brain, particularly in the hippocampus (Miyashita, 2004; Stephenson et al., 2018). This brain region is crucial for learning and memory function. Hence, aged humans who experience loss of neurons in this region exhibit frequent tendency of memory loss (Landfield et al., 1996; Bach et al., 1999; Miller and O’Callaghan, 2003; Eichenbaum, 2004; Panegyres, 2004; Gallagher et al., 2010). Aging and its association for the development of numerous brain diseases are continuously increasing in prevalence (Wyss-Coray, 2016). Increased plasma, cerebrospinal fluid (CSF), and brain level of alpha-synuclein (α-syn) and their association to microglial cells activation, pro-inflammatory cytokines production, neurodegeneration, and cognitive deficits have been observed in aged humans (Li et al., 2004; Chu and Kordower, 2007; Fjorback et al., 2007; Goldman et al., 2018; Stephenson et al., 2018). However, the exact mechanism by which such α-syn abnormalities trigger neuroinflammation in aged humans are poorly defined. Studies have shown that irregular accumulations and distributions of α-syn and/or the development of α-syn- reactive immunoglobulin G (IgG) autoantibodies are linked to the brain production of pro-inflammatory cytokines, i.e., interleukin1 beta (IL1β), IL6, and tumor necrosis factor alfa (TNFα), which lead to neuron death and memory deficits in several age-related neurodegenerative diseases, i.e., Alzheimer’s (AD), Parkinson’s (PD), multiple system atrophy (MSA), rapid eye movement sleep behavior disorder (RBD), frontotemporal lobar dementia (PLD), and dementia with Lewy bodies (LBD) (Spillantini et al., 1997; Baba et al., 1998; Zhang et al., 2005; Croisier and Graeber, 2006; Loeffler et al., 2006; Papachroni et al., 2007; van Rooijen et al., 2009; Mazzulli et al., 2011; Smith et al., 2012; Sardi et al., 2013; Kim et al., 2014; Allen Reish and Standaert, 2015; Pandey et al., 2016; Akhtar et al., 2018; Scott et al., 2018; Gregersen et al., 2021). Therefore, this current study explored the involvement of α-syn, α-syn-reactive IgG autoantibodies, and Fc gamma receptors (FcγRs) function in aging human nervous system.
Alpha-synuclein (α-syn) is a protein composed of a 140-amino acids with a total molecular weight of approximately 14 kDa, and is encoded by α-syn gene (SNCA) (Maroteaux et al., 1988; Spillantini et al., 1995). α-syn expression has been observed in different regions of the brain (e.g., hippocampus, presynaptic terminals of neocortex, and substantia nigra), in several nerve cells, (e.g., neurons, astrocytes, and oligodendrocytes), CSF, serum, plasma, and hematopoietic cells (Borghi et al., 2000; Richter-Landsberg et al., 2000; Mori et al., 2002; El-Agnaf et al., 2003; Kim et al., 2004; Miller et al., 2004; Barbour et al., 2008; Scherzer et al., 2008). Neuronal α-syn has been linked to the regulation of dopamine biosynthesis (Perez et al., 2002), synaptic vesicle mobility and recycling (Murphy et al., 2000; Burré et al., 2010; Scott and Roy, 2012), neurotransmitter release (Nemani et al., 2010), lipid metabolism (Jenco et al., 1998; Jo et al., 2000; Payton et al., 2004; Castagnet et al., 2005; Golovko et al., 2005; Narayanan et al., 2005; Barceló-Coblijn et al., 2007), and inflammatory responses (Sharon et al., 2003). The abnormal production and aggregation of α-syn trigger microglial cell activation and the massive generation of several pro-inflammatory cytokines (e.g., IL-1β, TNF-α, and IL-6), causing neurodegeneration and cognitive deficits in aged humans (Li et al., 2004; Chu and Kordower, 2007; Fjorback et al., 2007; Goldman et al., 2018; Stephenson et al., 2018). The identification of the mechanism by which such α-syn abnormalities develop brain inflammation in aged humans could also be helpful for better understanding of the disease mechanisms and development of anti-neuroinflammatory treatments for both healthy individuals and patients with brain diseases.
Immunoglobulin G (IgG), with a molecular weight of ∼150 kDa, is an antibody produced by plasma B cells in response to antigens (Bandilla et al., 1969). The serum concentration of IgG ranges from 7 to 18 mg/ml in healthy adults (Gonzalez-Quintela et al., 2008). The IgG antibody is composed of four polypeptide chains: two identical gamma (γ) heavy (H) chains of 50 kDa and two identical kappa (κ) or lambda (λ) light (L) chains of 25 kDa, connected together by the inter-chain disulfide bonds (Cobb, 2020). IgG- H chain comprises N-terminal variable (VH) and three constant domains (CH1, CH2, CH3), with the supplementary hinge region between the CH1 and CH2 domains (Cobb, 2020). Likewise, each of the IgG- L chain consists of an N-terminal variable domain (VL) and a constant domain (CL). The assembly of the IgG-L chain variable (VL) and constant (CL1) domains to the conforming IgG-H chain variable (VH) and constant (CH1) domains results the formation of the fragment antigen binding arm (Fab), which is responsible for the binding of antigens (Kabat et al., 1978; Potter, 1983). Two H chain – L chain heterodimers (HL) combine into a single antibody molecule through disulfide bonds (S = S) in the hinge region and non-covalent interactions between the CH3 domains. The portion of the antibody formed by the lower hinge region and the CH2/CH3 domains is called fragment crystalline (Fc) (Vidarsson et al., 2014). The Fab region of the IgG binds to specific antigen, whereas Fc region of the IgG binds to IgG receptor termed as Fc gamma R (FcγR) which is expressed on the surface of various immune cells (Karsten et al., 2012; Pandey, 2013; Pandey et al., 2017; Ben Mkaddem et al., 2019). Humans have four subclasses of IgG, (e.g., IgG1, IgG2, IgG3, and IgG4), all of which plays a critical role in biology of the health and disease (Schur, 1988; Pandey et al., 2003; Pandey and Agrawal, 2004; Iankov et al., 2006; Pandey, 2013; Vidarsson et al., 2014). Elevated levels of IgG autoantibodies to several brain elements (e.g., amyloid beta, myelin, optic nerve antigen, glutamic acid decarboxylase) have been observed in serum and/or CSF of patients with AD, multiple sclerosis (MS), cerebellar ataxias, Batten, autoimmune glaucoma, Stiff-person syndrome, and major depressive disorders (Polman and Killestein, 2006; Seehafer et al., 2011; Joachim et al., 2013; Maftei et al., 2013; Mitoma et al., 2017). Similarly, α-syn- specific IgG auto antibodies have been observed to have a role in the induction of neuroinflammation in PD, MSA, and RBD (Loeffler et al., 2006; Papachroni et al., 2007; Smith et al., 2012; Akhtar et al., 2018; Scott et al., 2018; Gregersen et al., 2021). Studies have also found significance in the levels of α-syn reactive IgG auto antibodies in aged patients’ serum and CSF (Smith et al., 2012; Heinzel et al., 2014; Brudek et al., 2017; Horvath et al., 2017; Akhtar et al., 2018; Folke et al., 2019). Additionally, age dependent increases of α-syn reactive IgG auto antibodies have been observed in humans (Koehler et al., 2013). Based on these findings, it is suggested that the abnormal production or aggregation of α-syn may be a trigger for production of IgG antibodies to α-syn. The ligation of such α-syn specific IgG antibodies to α-syn causes the formation of α-syn specific IgG immune complexes (α – syn – IgG ICs) in aged humans.
The FcγRs are the membrane molecules belonging to the immune tyrosine activation motif (ITAM)-associated receptor family, which recognize the Fc region of the different sub classes of humans IgG (IgG1, IgG2, IgG3, and IgG4) (Pandey, 2013; Ben Mkaddem et al., 2019). These subclasses of IgG have variable affinities for binding to the corresponding FcγRs (Pandey, 2013). Based on function, human FcγRs are divided into two classes, namely, activating FcγRs (e.g., FcγRI, FcγRIIa, FcγRIIc, and FcγRIIIa) and inhibitory FcγR, i.e., FcγRIIb or FcγRIIIb; GPI linked decoy (Ravetch and Bolland, 2001; Pandey, 2013). Activating FcγRs (apart from human FcγRIIa) signal through the specific Fcγ chain that contains ITAM. The ligation of IgG-ICs, by activating FcγRs, causes the tyrosine phosphorylation of ITAM to the cytoplasmic chain by the SRC kinase family (Src, Fyn, Fgr, Hck, and Lyn) (Ghazizadeh et al., 1994; Wang et al., 1994; Ravetch and Bolland, 2001; Pandey, 2013). Resultant phosphorylated ITAM bind to the spleen tyrosine kinase (STK), causing the STK phosphorylation. The phosphorylated STK causes a downstream activation of the linker for activation of T cells (LAT), multimolecular adaptor complexes (MAC), and the phosphoinositide 3-kinase (PI3K). Such activation of LAT- MAC - PI3K pathway leads to the development of membrane-docking sites for Bruton’s tyrosine kinase (BTK), along with the recruitment of phosphoinositide base (PI) and phospholipase C-gamma (PLC-γ) (Pandey, 2013; Pandey et al., 2017). The PLC-γ is the multi-domain phosphodiesterase which catalyzes the conversion of the phosphatidylinositol (3,4,5)-trisphosphate [PI(3,4,5)P3] to three byproducts, i.e., phosphoinositol 4,5 diphosphate [PI(4,5)2], Inositol 1,4,5 triphosphate (IP3), and diacyl glycerol (DAG), which galvanizes the calcium ion (Ca++) mobilization and cause the activation of rat sarcoma virus (RAS) – rapidly accelerated fibrosarcoma (RAF) - mitogen-activated protein kinase (MAPK) pathway. This then leads to cellular activation, pro-inflammatory cytokines production, and the tissue damage that may entail degenerative diseases (Rafiq et al., 2002; Nakashima et al., 2004; Matsubara et al., 2006; Nimmerjahn and Ravetch, 2008; Karsten et al., 2012). In contrast, inhibitory FcγRIIb crosslinking with IgG-ICs triggers the activation of immunoreceptor tyrosine inhibitory motif (ITIM)- downstream signaling and causes the tyrosine phosphorylation of ITIM by Lyn kinase, leading to the conversion of SH2 containing phosphatidylinositol 5 phosphatase (SHIP) to phosphorylated SHIP. Furthermore, the reaction between the phosphorylated SHIP and PI (3, 4, 5) P3 causes the suppression of PI (3, 4, 5) P3- mediated induction of cellular activation (Nimmerjahn and Ravetch, 2008; Pandey, 2013). Overall, the IgG-ICs ligation with activating FcγR causes the transduction of inflammatory signaling through ITAM, causing cellular activation, pro-inflammatory cytokines production, and cell death, which are all inhibited by the IgG-ICs- inhibitory FcγRIIb-ITIM-downstream signaling in humans (Nimmerjahn and Ravetch, 2007, 2008; Wang, 2019). These observations suggested that the proportion of activating vs. inhibitory FcγRs (A/I ratio) determines the magnitude of IgG-ICs-induced inflammatory cell responses (Ravetch and Lanier, 2000; Shushakova et al., 2002; Godau et al., 2004; Ricklin et al., 2012). The steady state human microglial cells showed lower expression of ITAM-FcγRs (e.g., FcγRI, FcγRIIa, FcγRIIb, and FcγRIIIa). In addition, compared to inhibitory FcγR, increased expression of activating FcγRs have been observed in choroid-retinal epithelial cells, hippocampal tissues, microglial cells, and neurons of healthy individuals and elderly patients with other neurodegenerative diseases (Peress et al., 1993; Ulvestad et al., 1994b; Mohamed et al., 2002; Andoh and Kuraishi, 2004; Orr et al., 2005; Kam et al., 2013; Zotova et al., 2013; Murinello et al., 2014; Yue et al., 2019). Additionally, age-associated increased production of α-syn reactive IgG auto antibodies and higher microglial cells expression of activating FcγR have been observed in the CNS regions of the healthy humans (Hart et al., 2012; Koehler et al., 2013). Based on these findings, it is speculated that the robust crosslinking between α-syn – IgG-ICs and the corresponding activating FcγR causes the activation of ITAM and its downstream signaling cascades, which trigger the microglial cells activation and massive generation of pro-inflammatory cytokines that lead to neuron death and cognitive defects.
Aging and its association with developing several of the age-related brain diseases such as AD, PD, MSA, RBD, Huntington (HD), amyotrophic lateral sclerosis (ALS), and PLD are continuously increasing (Budni et al., 2015; Wyss-Coray, 2016). Despite putting considerable effort, the mechanism that underlie age-related development of neuroinflammation, neurodegeneration, and cognitive decline has been poorly defined.
Mice IgG (IgG1, IgG2a/c, IgG2b, and IgG3) and their matching receptors, (e.g., FcγRI, FcγRIIb, FcγRIII and FcγRIV) are different from human IgG, (e.g., IgG1, IgG2, IgG3, and IgG 4) and receptors, (e.g., FcγRI, FcγRIIa, FcγRIIc, FcγRIIIa, FcγRIIb, and FcγRIIIb) (Pandey, 2013). Human or mice FcγRI bind only to the monomeric IgG, but all the other FcγR in mice, (e.g., FcγRIIb, FcγRIII and FcγRIV) and human, (e.g., FcγRIIa,FcγRIIb, FcγRIIIa and FcγRIIIb) can bind to IgG-ICs (Nimmerjahn and Ravetch, 2006). Mice IgG2a/c-ICs, when cross linked to FcγRIII/FcγRIV, causes immune inflammation (Clynes et al., 1999; Yuasa et al., 1999; Karsten et al., 2012; Pandey, 2013). However, mice IgG1-ICs, when crosslinked to FcγRIIb, develops protection against inflammation (Schur, 1988; Karsten et al., 2012; Pandey, 2013; Vidarsson et al., 2014). In contrast, human activating FcγR interaction with IgG1-ICs triggers immune inflammation, but their inhibitory FcγR ligation to IgG4-ICs protects against inflammation (Pandey, 2013). Moreover, the effector function of human IgG1 is like murine IgG2a/c. Similarly, the effector function of human IgG4 is more alike to mouse IgG1 (Pandey, 2013). Mice studies have shown the higher expression of FcγRIV and FcγRIIb receptors in females (Gal-Oz et al., 2019). However, human studies have revealed almost similar level expression of FcγRIIIa in male and females (Huang et al., 2021).
Despite having several of the indicated differences in IgG and their corresponding FcγRs in mice and humans, both mice and human studies have shown that the IgG-ICs cross linking to activating FcγR causes tissue inflammation (Clynes et al., 1999; Yuasa et al., 1999; Karsten et al., 2012; Pandey, 2013) and that IgG-ICs interaction to inhibitory FcγR protects IgG-ICs-activating FcγR axis-induced tissue inflammation (Nimmerjahn and Ravetch, 2007; Karsten et al., 2012; Pandey, 2013). Mice and human studies have also shown that the co-expression of FcyRs activation and inhibition are essential for establishment of the threshold that controls the activation of IgG-ICs – FcγR-mediated effector functions and the disturbances of such balance between the activating and inhibitory FcγR as well as their crosslinking to the corresponding IgG/IgG subclasses-ICs mechanistically provoke the immune inflammations and cause tissue damage in several inflammatory diseases (Nimmerjahn and Ravetch, 2007; Karsten et al., 2012; Pandey, 2013; Pandey et al., 2017).
Brain reactive antigens, autoantibodies, and their link to FcγR- mediated microglial cells activation, pro-inflammatory cytokines, (e.g., IL-1β, IL6, IL18, and TNFα) production, neurons death, and the memory and learning defects have been observed in several neurological diseases (Polinsky et al., 1991; Ulvestad et al., 1994a,b; Terryberry et al., 1998; Gruden et al., 2007; Vyshkina and Kalman, 2008; Okun et al., 2010; Lunnon et al., 2011; Cribbs et al., 2012; Satoh et al., 2012; Vacirca et al., 2012; Joachim et al., 2013; Kam et al., 2013; Murinello et al., 2014; Paris et al., 2014; Schweig et al., 2017, 2019; Yang et al., 2019). The number of the growth and neurotrophic factors, i.e., brain-derived neurotrophic factor (BDNF), nerve growth factor (NGF), and the glial cell line-derived neurotrophic factor (GDNF) are essential for pruning, myelination, differentiation, synaptic and neuronal growth, survival of neurons, and the scalp skin homeostasis (Huang and Reichardt, 2001; Skoff et al., 2003; Adly et al., 2006, 2016; Caldeira et al., 2007; Skaper, 2012; Mitra et al., 2021). Studies have shown the link between the abnormal brain formation of BDNF, NGF, and GDNF and the neurodegeneration that comes with age-related brain diseases (Grassi-Oliveira et al., 2008; Komulainen et al., 2008; Numakawa et al., 2018; Lima Giacobbo et al., 2019). However, the exact mechanism by which α-syn- induced initiation of IgG ICs - activating FcγRs axis triggers neuronal cells activation, pro-inflammatory cytokines production, and neurodegeneration was not explored in human nervous system aging. To resolve all these critical topics, direct in vivo and ex vivo functional studies demonstrating the α-syn-IgG-ICs – activating and/or inhibitory FcγRs axis- downstream signaling and their impact in controlling microglial cells and/or neurons activation, IL-1β, IL6, IL18, TNFα, BDNF, NGF, and GDNF production as well as their combined influence on the status of neurons health and memory function are needed for mice and/or humans with different ages and genders.
However, based on the elevated brain expression of α-syn- reactive IgG auto antibodies and the higher expression of activating FcγR in aged humans (Hart et al., 2012; Koehler et al., 2013; Heinzel et al., 2014; Brudek et al., 2017; Horvath et al., 2017; Akhtar et al., 2018; Folke et al., 2019), this report suggests that the α- syn- reactive IgG auto antibodies and their immunological reaction to α-syn are the basis of the formation of the α-syn – IgG-ICs in the aged human brains. Furthermore, the strong interaction between such α syn- IgG-ICs and activation of FcγR fules the activating FcγR- downstream signaling that causes the microglial cells and/or neurons activation, pro-inflammatory cytokines production, and the neurons death in aged humans (Figure 1A). Also, downregulation of inhibitory FcγR in aging permits poor communication between α syn IgG – ICs and the corresponding inhibitory FcγR and their downstream ITIM and SHIP signaling that principally controls the α-syn – IgG-ICs – activating FcγR axis- downstream signaling-mediated microglial cells and/or neurons activation, pro-inflammatory cytokines generation, and the neurons death in aged humans (Figure 1B). Targeting the α syn- IgG-ICs – activating FcγR axis and/or their downstream signaling by which microglial and/or neuronal cells activate and fuel brain inflammation and develop memory and learning defects could help to understand the disease mechanisms and development of the alternative anti-neuroinflammatory treatments for aged humans.
Figure 1. Alpha-synuclein-specific IgG immune complexes (α-syn-IgG-ICs) – Fc gamma receptor (FcγR) function in induction of neuroinflammation in aged humans. During aging, α-syn- IgG-ICs interaction with the activating FcγR activates the SRC family kinases, (e.g., Src, Fyn, Fgr, Hck, and Lyn). These enzymes trigger the phosphorylation of immune-tyrosine activation motif (ITAM) and cause the recruitment of SH2 domain having Syk kinases, which lead to the phosphorylation of spleen tyrosine kinase (STK). Such alteration in STK causes the activation of the linker for activation of T cells (LAT)- multimolecular adaptor complexes (MAC)- phosphoinositide 3-kinase (PI3K) – Bruton’s tyrosine kinase (BTK) – phosphoinositide base (PI) + phospholipase C-gamma (PLC-γ) – phosphatidylinositol (3,4,5)-trisphosphate: PI(3,4,5)P3 – phosphoinositol 4,5 diphosphate [PI(4,5)2] + Inositol 1,4,5 triphosphate (IP3) + diacyl glycerol (DAG) – calcium (Ca++) – rat sarcoma virus (RAS) – rapidly accelerated fibrosarcoma (RAF) – mitogen-activated protein kinase (MAPK) pathway and cause increased activation of microglial cells and/or neurons and pro-inflammatory cytokines generation, which lead to neuron death in aged humans (A). Furthermore, due to aging-induced down regulation of inhibitory FcγR, α syn-IgG-ICs fail to establish proper binding to the inhibitory FcγR and, in turn, the inhibitory FcγR- downstream LYN kinases- phosphorylated immunoreceptor tyrosine inhibitory motif (ITIM) – phosphorylated SH2-containing-phosphatidylinositol-5′-phosphatase (SHIP) anti-inflammatory pathway do not control the α syn- IgG-ICs – activated FcγRs axis- mediated microglial cells and/or neurons activation, pro-inflammatory cytokines generation, and the neurons death in aged humans (B).
MP has intellectualized and wrote the study, conducted the literature search, performed the proofreading, editing, and approval of final version of the manuscript.
This work was supported by the research awards and grants to MP from the Division of Human Genetics, Cincinnati Children’s Hospital Medical Center, Cincinnati, OH, United States, Just-In-Time Core Grant Award; Center for Clinical and Translational Science, University of Cincinnati, Cincinnati, OH, United States (NIH/NCRR Grant Number UL1TR001425), Research, Innovation and Pilot (RIP) Funding Award; Cincinnati Children’s Research Foundation (CCRF), Cincinnati, OH, United States (CCRF Grant Number 31-41642-584000), Michael J. Fox Foundation, New York, NY, United States (MJFF Grant Number- 16452 and 010292), and Orphan Disease Center, University of Pennsylvania, Philadelphia, PA, United States (MDBR Grant Number-21-121-MPS).
The author declares that the research was conducted in the absence of any commercial or financial relationships that could be construed as a potential conflict of interest.
All claims expressed in this article are solely those of the authors and do not necessarily represent those of their affiliated organizations, or those of the publisher, the editors and the reviewers. Any product that may be evaluated in this article, or claim that may be made by its manufacturer, is not guaranteed or endorsed by the publisher.
The author would like to acknowledge Vanca Pandey for English and Grammar modifications, BioRender.com for creating the figure, and Albert F. Magnusen and Charles D. Loftice for office and laboratory support.
Adly, M. A., Assaf, H. A., Hussein, M. R., and Paus, R. (2006). Analysis of the expression pattern of glial cell line-derived neurotrophic factor, neurturin, their cognate receptors GFRα-1 and GFRα-2, and a common signal transduction element c-Ret in the human scalp skin. J. Cutan. Pathol. 33, 799–808. doi: 10.1111/j.1600-0560.2006.00561.x
Adly, M. A., Assaf, H. A., and Hussein, M. R. A. (2016). Age-associated decrease in GDNF and its cognate receptor GFRα-1 protein expression in human skin. Int. J. Exp. Pathol. 97, 248–256. doi: 10.1111/iep.12175
Akhtar, R. S., Licata, J. P., Luk, K. C., Shaw, L. M., Trojanowski, J. Q., and Lee, V. M. Y. (2018). Measurements of auto-antibodies to α-synuclein in the serum and cerebral spinal fluids of patients with Parkinson’s disease. J. Neurochem. 145, 489–503. doi: 10.1111/jnc.14330
Allen Reish, H. E., and Standaert, D. G. (2015). Role of α-synuclein in inducing innate and adaptive immunity in Parkinson disease. J. Parkinsons Dis. 5, 1–19. doi: 10.3233/JPD-140491
Andoh, T., and Kuraishi, Y. (2004). Direct action of immunoglobulin G on primary sensory neurons through Fc gamma receptor I. FASEB J. 18, 182–184. doi: 10.1096/fj.02-1169fje
Baba, M., Nakajo, S., Tu, P. H., Tomita, T., Nakaya, K., Lee, V. M., et al. (1998). Aggregation of alpha-synuclein in Lewy bodies of sporadic Parkinson’s disease and dementia with Lewy bodies. Am. J. Pathol. 152, 879–884.
Bach, M. E., Barad, M., Son, H., Zhuo, M., Lu, Y. F., Shih, R., et al. (1999). Age-related defects in spatial memory are correlated with defects in the late phase of hippocampal long-term potentiation in vitro and are attenuated by drugs that enhance the cAMP signaling pathway. Proc. Natl. Acad. Sci. U.S.A. 96, 5280–5285. doi: 10.1073/pnas.96.9.5280
Bandilla, K. K., McDuffie, F. C., and Gleich, G. J. (1969). Immunoglobulin classes of antibodies produced in the primary and secondary responses in man. Clin. Exp. Immunol. 5, 627–641.
Barbour, R., Kling, K., Anderson, J. P., Banducci, K., Cole, T., Diep, L., et al. (2008). Red blood cells are the major source of alpha-synuclein in blood. Neurodegener. Dis. 5, 55–59. doi: 10.1159/000112832
Barceló-Coblijn, G., Golovko, M. Y., Weinhofer, I., Berger, J., and Murphy, E. J. (2007). Brain neutral lipids mass is increased in alpha-synuclein gene-ablated mice. J. Neurochem. 101, 132–141. doi: 10.1111/j.1471-4159.2006.04348.x
Ben Mkaddem, S., Benhamou, M., and Monteiro, R. C. (2019). Understanding Fc receptor involvement in inflammatory diseases: from mechanisms to new therapeutic tools. Front. Immunol. 10:811. doi: 10.3389/fimmu.2019.00811
Borghi, R., Marchese, R., Negro, A., Marinelli, L., Forloni, G., Zaccheo, D., et al. (2000). Full length alpha-synuclein is present in cerebrospinal fluid from Parkinson’s disease and normal subjects. Neurosci. Lett. 287, 65–67. doi: 10.1016/s0304-3940(00)01153-8
Brudek, T., Winge, K., Folke, J., Christensen, S., Fog, K., Pakkenberg, B., et al. (2017). Autoimmune antibody decline in Parkinson’s disease and Multiple System Atrophy; a step towards immunotherapeutic strategies. Mol. Neurodegener. 12:44. doi: 10.1186/s13024-017-0187-7
Budni, J., Bellettini-Santos, T., Mina, F., Garcez, M. L., and Zugno, A. I. (2015). The involvement of BDNF, NGF and GDNF in aging and Alzheimer’s disease. Aging Dis. 6, 331–341. doi: 10.14336/AD.2015.0825
Burré, J., Sharma, M., Tsetsenis, T., Buchman, V., Etherton, M. R., and Südhof, T. C. (2010). Alpha-synuclein promotes SNARE-complex assembly in vivo and in vitro. Science 329, 1663–1667. doi: 10.1126/science.1195227
Caldeira, M. V., Melo, C. V., Pereira, D. B., Carvalho, R., Correia, S. S., Backos, D. S., et al. (2007). Brain-derived neurotrophic factor regulates the expression and synaptic delivery of alpha-amino-3-hydroxy-5-methyl-4-isoxazole propionic acid receptor subunits in hippocampal neurons. J. Biol. Chem. 282, 12619–12628. doi: 10.1074/jbc.M700607200
Castagnet, P. I., Golovko, M. Y., Barceló-Coblijn, G. C., Nussbaum, R. L., and Murphy, E. J. (2005). Fatty acid incorporation is decreased in astrocytes cultured from alpha-synuclein gene-ablated mice. J. Neurochem. 94, 839–849. doi: 10.1111/j.1471-4159.2005.03247.x
Chu, Y., and Kordower, J. H. (2007). Age-associated increases of α-synuclein in monkeys and humans are associated with nigrostriatal dopamine depletion: Is this the target for Parkinson’s disease? Neurobiol. Dis. 25, 134–149. doi: 10.1016/j.nbd.2006.08.021
Clynes, R., Maizes, J. S., Guinamard, R., Ono, M., Takai, T., and Ravetch, J. V. (1999). Modulation of immune complex–induced inflammation in vivo by the coordinate expression of activation and inhibitory Fc Receptors. J. Exp. Med. 189, 179–186. doi: 10.1084/jem.189.1.179
Cobb, B. A. (2020). The history of IgG glycosylation and where we are now. Glycobiology 30, 202–213. doi: 10.1093/glycob/cwz065
Cribbs, D. H., Berchtold, N. C., Perreau, V., Coleman, P. D., Rogers, J., Tenner, A. J., et al. (2012). Extensive innate immune gene activation accompanies brain aging, increasing vulnerability to cognitive decline and neurodegeneration: a microarray study. J. Neuroinflammation 9:179. doi: 10.1186/1742-2094-9-179
Croisier, E., and Graeber, M. B. (2006). Glial degeneration and reactive gliosis in alpha-synucleinopathies: the emerging concept of primary gliodegeneration. Acta Neuropathol. 112, 517–530. doi: 10.1007/s00401-006-0119-z
Eichenbaum, H. (2004). Hippocampus: cognitive processes and neural representations that underlie declarative memory. Neuron 44, 109–120. doi: 10.1016/j.neuron.2004.08.028
El-Agnaf, O. M., Salem, S. A., Paleologou, K. E., Cooper, L. J., Fullwood, N. J., Gibson, M. J., et al. (2003). Alpha-synuclein implicated in Parkinson’s disease is present in extracellular biological fluids, including human plasma. FASEB J. 17, 1945–1947. doi: 10.1096/fj.03-0098fje
Fjorback, W. A., Varming, K., and Jensen, H. P. (2007). Determination of α−synuclein concentration in human plasma using ELISA. Scand. J. Clin. Lab. Invest. 67, 431–435. doi: 10.1080/00365510601161497
Folke, J., Rydbirk, R., Løkkegaard, A., Salvesen, L., Hejl, A.-M., Starhof, C., et al. (2019). Distinct autoimmune anti-α-synuclein antibody patterns in multiple system atrophy and parkinson’s disease. Front. Immunol. 10:2253. doi: 10.3389/fimmu.2019.02253
Gallagher, M., Bakker, A., Yassa, M. A., and Stark, C. E. L. (2010). Bridging neurocognitive aging and disease modification: targeting functional mechanisms of memory impairment. Curr. Alzheimer Res. 7, 197–199. doi: 10.2174/156720510791050867
Gal-Oz, S. T., Maier, B., Yoshida, H., Seddu, K., Elbaz, N., Czysz, C., et al. (2019). ImmGen report: sexual dimorphism in the immune system transcriptome. Nat. Commun. 10:4295. doi: 10.1038/s41467-019-12348-6
Ghazizadeh, S., Bolen, J. B., and Fleit, H. B. (1994). Physical and functional association of Src-related protein tyrosine kinases with Fc gamma RII in monocytic THP-1 cells. J. Biol. Chem. 269, 8878–8884. doi: 10.1016/s0021-9258(17)37050-3
Godau, J., Heller, T., Hawlisch, H., Trappe, M., Howells, E., Best, J., et al. (2004). C5a initiates the inflammatory cascade in immune complex peritonitis. J. Immunol. 173, 3437–3445. doi: 10.4049/jimmunol.173.5.3437
Goldman, J. G., Andrews, H., Amara, A., Naito, A., Alcalay, R. N., Shaw, L. M., et al. (2018). Cerebrospinal fluid, plasma, and saliva in the BioFIND study: relationships among biomarkers and Parkinson’s disease Features. Mov. Disord. 33, 282–288. doi: 10.1002/mds.27232
Golovko, M. Y., Faergeman, N. J., Cole, N. B., Castagnet, P. I., Nussbaum, R. L., and Murphy, E. J. (2005). Alpha-synuclein gene deletion decreases brain palmitate uptake and alters the palmitate metabolism in the absence of alpha-synuclein palmitate binding. Biochemistry 44, 8251–8259. doi: 10.1021/bi0502137
Gonzalez-Quintela, A., Alende, R., Gude, F., Campos, J., Rey, J., Meijide, L. M., et al. (2008). Serum levels of immunoglobulins (IgG, IgA, IgM) in a general adult population and their relationship with alcohol consumption, smoking and common metabolic abnormalities. Clin. Exp. Immunol. 151, 42–50. doi: 10.1111/j.1365-2249.2007.03545.x
Grassi-Oliveira, R., Stein, L. M., Lopes, R. P., Teixeira, A. L., and Bauer, M. E. (2008). Low plasma brain-derived neurotrophic factor and childhood physical neglect are associated with verbal memory impairment in major depression–a preliminary report. Biol. Psychiatry 64, 281–285. doi: 10.1016/j.biopsych.2008.02.023
Gregersen, E., Betzer, C., Kim, W. S., Kovacs, G., Reimer, L., Halliday, G. M., et al. (2021). Alpha-synuclein activates the classical complement pathway and mediates complement-dependent cell toxicity. J. Neuroinflammation 18:177. doi: 10.1186/s12974-021-02225-9
Gruden, M. A., Davidova, T. B., Malisauskas, M., Sewell, R. D., Voskresenskaya, N. I., Wilhelm, K., et al. (2007). Differential neuroimmune markers to the onset of Alzheimer’s disease neurodegeneration and dementia: autoantibodies to Abeta((25-35)) oligomers, S100b and neurotransmitters. J. Neuroimmunol. 186, 181–192. doi: 10.1016/j.jneuroim.2007.03.023
Hart, A. D., Wyttenbach, A., Hugh Perry, V., and Teeling, J. L. (2012). Age related changes in microglial phenotype vary between CNS regions: grey versus white matter differences. Brain Behav. Immunity. 26, 754–765. doi: 10.1016/j.bbi.2011.11.006
Heinzel, S., Gold, M., Deuschle, C., Bernhard, F., Maetzler, W., Berg, D., et al. (2014). Naturally occurring alpha-synuclein autoantibodies in Parkinson’s disease: sources of (error) variance in biomarker assays. PLoS One 9:e114566. doi: 10.1371/journal.pone.0114566
Horvath, I., Iashchishyn, I. A., Forsgren, L., and Morozova-Roche, L. A. (2017). Immunochemical detection of α-Synuclein autoantibodies in parkinson’s disease: correlation between Plasma and Cerebrospinal Fluid Levels. ACS Chem. Neurosci. 8, 1170–1176. doi: 10.1021/acschemneuro.7b00063
Huang, E. J., and Reichardt, L. F. (2001). Neurotrophins: roles in neuronal development and function. Annu. Rev. Neurosci. 24, 677–736. doi: 10.1146/annurev.neuro.24.1.677
Huang, Z., Chen, B., Liu, X., Li, H., Xie, L., Gao, Y., et al. (2021). Effects of sex and aging on the immune cell landscape as assessed by single-cell transcriptomic analysis. Proc. Natl. Acad. Sci. U.S.A. 118:e2023216118. doi: 10.1073/pnas.2023216118
Iankov, I. D., Pandey, M., Harvey, M., Griesmann, G. E., Federspiel, M. J., and Russell, S. J. (2006). Immunoglobulin g antibody-mediated enhancement of measles virus infection can bypass the protective antiviral immune response. J. Virol. 80, 8530–8540. doi: 10.1128/JVI.00593-06
Jenco, J. M., Rawlingson, A., Daniels, B., and Morris, A. J. (1998). Regulation of phospholipase D2: selective inhibition of mammalian phospholipase D isoenzymes by alpha- and beta-synucleins. Biochemistry 37, 4901–4909. doi: 10.1021/bi972776r
Jo, E., McLaurin, J., Yip, C. M., St George-Hyslop, P., and Fraser, P. E. (2000). alpha-Synuclein membrane interactions and lipid specificity. J. Biol. Chem. 275, 34328–34334. doi: 10.1074/jbc.M004345200
Joachim, S. C., Reinehr, S., Kuehn, S., Laspas, P., Gramlich, O. W., Kuehn, M., et al. (2013). Immune response against ocular tissues after immunization with optic nerve antigens in a model of autoimmune glaucoma. Mol. Vis. 19, 1804–1814.
Kabat, E. A., Wu, T. T., and Bilofsky, H. (1978). Variable region genes for the immunoglobulin framework are assembled from small segments of DNA—A hypothesis. Proc. Natl. Acad. Sci. U.S.A. 75, 2429–2433. doi: 10.1073/pnas.75.5.2429
Kam, T.-I., Song, S., Gwon, Y., Park, H., Yan, J.-J., Im, I., et al. (2013). FcγRIIb mediates amyloid-β neurotoxicity and memory impairment in Alzheimer’s disease. J. Clin. Invest. 123, 2791–2802.
Karsten, C. M., Pandey, M. K., Figge, J., Kilchenstein, R., Taylor, P. R., Rosas, M., et al. (2012). Anti-inflammatory activity of IgG1 mediated by Fc galactosylation and association of FcγRIIB and dectin-1. Nat. Med. 18, 1401–1406. doi: 10.1038/nm.2862
Kim, S., Seo, J. H., and Suh, Y. H. (2004). Alpha-synuclein, Parkinson’s disease, and Alzheimer’s disease. Parkinsonism Relat. Disord. 10, (Suppl. 1), S9–S13.
Kim, W. S., Kågedal, K., and Halliday, G. M. (2014). Alpha-synuclein biology in Lewy body diseases. Alzheimers Res. Ther. 6:73. doi: 10.1186/s13195-014-0073-2
Koehler, N. K., Stransky, E., Shing, M., Gaertner, S., Meyer, M., Schreitmüller, B., et al. (2013). Altered serum IgG levels to α-synuclein in dementia with Lewy bodies and Alzheimer’s disease. PLoS One 8:e64649. doi: 10.1371/journal.pone.0064649
Komulainen, P., Pedersen, M., Hänninen, T., Bruunsgaard, H., Lakka, T. A., Kivipelto, M., et al. (2008). BDNF is a novel marker of cognitive function in ageing women: the DR’s EXTRA Study. Neurobiol. Learn. Mem. 90, 596–603. doi: 10.1016/j.nlm.2008.07.014
Landfield, P. W., McEwen, B. S., Sapolsky, R. M., and Meaney, M. J. (1996). Hippocampal Cell Death. Science 272, 1249–1251.
Li, W., Lesuisse, C., Xu, Y., Troncoso, J. C., Price, D. L., and Lee, M. K. (2004). Stabilization of alpha-synuclein protein with aging and familial parkinson’s disease-linked A53T mutation. J. Neurosci. 24, 7400–7409. doi: 10.1523/JNEUROSCI.1370-04.2004
Lima Giacobbo, B., Doorduin, J., Klein, H. C., Dierckx, R., Bromberg, E., and de Vries, E. F. J. (2019). Brain-derived neurotrophic factor in brain disorders: focus on neuroinflammation. Mol. Neurobiol. 56, 3295–3312. doi: 10.1007/s12035-018-1283-6
Loeffler, D. A., Camp, D. M., and Conant, S. B. (2006). Complement activation in the Parkinson’s disease substantia nigra: an immunocytochemical study. J. Neuroinflammation 3:29. doi: 10.1186/1742-2094-3-29
Lunnon, K., Teeling, J. L., Tutt, A. L., Cragg, M. S., Glennie, M. J., and Perry, V. H. (2011). Systemic inflammation modulates Fc receptor expression on microglia during chronic neurodegeneration. J. Immunol. 186, 7215–7224. doi: 10.4049/jimmunol.0903833
Maftei, M., Thurm, F., Schnack, C., Tumani, H., Otto, M., Elbert, T., et al. (2013). Increased levels of antigen-bound β-Amyloid autoantibodies in serum and cerebrospinal fluid of alzheimer’s disease patients. PLoS One 8:e68996. doi: 10.1371/journal.pone.0068996
Maroteaux, L., Campanelli, J. T., and Scheller, R. H. (1988). Synuclein: a neuron-specific protein localized to the nucleus and presynaptic nerve terminal. J. Neurosci. 8, 2804–2815. doi: 10.1523/JNEUROSCI.08-08-02804.1988
Matsubara, S., Koya, T., Takeda, K., Joetham, A., Miyahara, N., Pine, P., et al. (2006). Syk activation in dendritic cells is essential for airway hyperresponsiveness and inflammation. Am. J. Respir. Cell Mol. Biol. 34, 426–433. doi: 10.1165/rcmb.2005-0298OC
Mazzulli, J. R., Xu, Y. H., Sun, Y., Knight, A. L., McLean, P. J., Caldwell, G. A., et al. (2011). Gaucher disease glucocerebrosidase and alpha-synuclein form a bidirectional pathogenic loop in synucleinopathies. Cell 146, 37–52. doi: 10.1016/j.cell.2011.06.001
Miller, D. B., and O’Callaghan, J. P. (2003). Effects of aging and stress on hippocampal structure and function. Metabolism 52, (Suppl. 2), 17–21. doi: 10.1016/s0026-0495(03)00296-8
Miller, D. W., Hague, S. M., Clarimon, J., Baptista, M., Gwinn-Hardy, K., Cookson, M. R., et al. (2004). Alpha-synuclein in blood and brain from familial Parkinson disease with SNCA locus triplication. Neurology 62, 1835–1838. doi: 10.1212/01.wnl.0000127517.33208.f4
Mitoma, H., Manto, M., and Hampe, C. S. (2017). Pathogenic roles of glutamic acid decarboxylase 65 autoantibodies in cerebellar ataxias. J. Immunol. Res. 2017:2913297. doi: 10.1155/2017/2913297
Mitra, S., Turconi, G., Darreh-Shori, T., Mätlik, K., Aquilino, M., Eriksdotter, M., et al. (2021). Increased Endogenous GDNF in mice protects against age-related decline in neuronal cholinergic markers. Front. Aging Neurosci. 13:714186. doi: 10.3389/fnagi.2021.714186
Miyashita, Y. (2004). Cognitive memory: cellular and network machineries and their top-down control. Science 306, 435–440. doi: 10.1126/science.1101864
Mohamed, H. A., Mosier, D. R., Zou, L. L., Siklós, L., Alexianu, M. E., Engelhardt, J. I., et al. (2002). Immunoglobulin Fcγ receptor promotes immunoglobulin uptake, immunoglobulin-mediated calcium increase, and neurotransmitter release in motor neurons. J. Neurosci. Res. 69, 110–116. doi: 10.1002/jnr.10271
Mori, F., Tanji, K., Yoshimoto, M., Takahashi, H., and Wakabayashi, K. (2002). Demonstration of alpha-synuclein immunoreactivity in neuronal and glial cytoplasm in normal human brain tissue using proteinase K and formic acid pretreatment. Exp. Neurol. 176, 98–104. doi: 10.1006/exnr.2002.7929
Murinello, S., Mullins, R. F., Lotery, A. J., Perry, V. H., and Teeling, J. L. (2014). Fcγ Receptor upregulation is associated with immune complex inflammation in the mouse retina and early age-related macular degeneration. Invest. Ophthalmol. Vis. Sci. 55, 247–258. doi: 10.1167/iovs.13-11821
Murphy, D. D., Rueter, S. M., Trojanowski, J. Q., and Lee, V. M. (2000). Synucleins are developmentally expressed, and alpha-synuclein regulates the size of the presynaptic vesicular pool in primary hippocampal neurons. J. Neurosci. 20, 3214–3220. doi: 10.1523/JNEUROSCI.20-09-03214.2000
Nakashima, K., Kokubo, T., Shichijo, M., Li, Y. F., Yura, T., and Yamamoto, N. (2004). A novel Syk kinase-selective inhibitor blocks antigen presentation of immune complexes in dendritic cells. Eur. J. Pharmacol. 505, 223–228. doi: 10.1016/j.ejphar.2004.10.024
Narayanan, V., Guo, Y., and Scarlata, S. (2005). Fluorescence studies suggest a role for alpha-synuclein in the phosphatidylinositol lipid signaling pathway. Biochemistry 44, 462–470. doi: 10.1021/bi0487140
Nemani, V. M., Lu, W., Berge, V., Nakamura, K., Onoa, B., Lee, M. K., et al. (2010). Increased expression of alpha-synuclein reduces neurotransmitter release by inhibiting synaptic vesicle reclustering after endocytosis. Neuron 65, 66–79. doi: 10.1016/j.neuron.2009.12.023
Nimmerjahn, F., and Ravetch, J. V. (2006). Fcγ receptors: old friends and new family members. Immunity 24, 19–28. doi: 10.1016/j.immuni.2005.11.010
Nimmerjahn, F., and Ravetch, J. V. (2007). Fc-receptors as regulators of immunity. Adv. Immunol. 96, 179–204.
Nimmerjahn, F., and Ravetch, J. V. (2008). Fcγ receptors as regulators of immune responses. Nat. Rev. Immunol. 8, 34–47.
Numakawa, T., Odaka, H., and Adachi, N. (2018). Actions of brain-derived neurotrophin factor in the neurogenesis and neuronal function, and its involvement in the pathophysiology of brain diseases. Int. J. Mol. Sci. 19:3650. doi: 10.3390/ijms19113650
Okun, E., Mattson, M. P., and Arumugam, T. V. (2010). Involvement of Fc receptors in disorders of the central nervous system. Neuromolecular Med. 12, 164–178. doi: 10.1007/s12017-009-8099-5
Orr, C. F., Rowe, D. B., Mizuno, Y., Mori, H., and Halliday, G. M. (2005). A possible role for humoral immunity in the pathogenesis of Parkinson’s disease. Brain 128, 2665–2674. doi: 10.1093/brain/awh625
Pandey, M. K. (2013). Molecular basis for downregulation of C5a-mediated inflammation by IgG1 immune complexes in allergy and asthma. Curr. Allergy Asthma Rep. 13, 596–606. doi: 10.1007/s11882-013-0387-3
Pandey, M. K., and Agrawal, S. (2004). Induction of MLR-Bf and protection of fetal loss: a current double blind randomized trial of paternal lymphocyte immunization for women with recurrent spontaneous abortion. Int. Immunopharmacol. 4, 289–298.
Pandey, M. K., Burrow, T. A., Rani, R., Martin, L. J., Witte, D., Setchell, K. D., et al. (2017). Complement drives glucosylceramide accumulation and tissue inflammation in Gaucher disease. Nature 543, 108–112. doi: 10.1038/nature21368
Pandey, M. K., Caballero, M., Tinch, S. L., Schroeder, L. M., Gross, C., Vorhees, C., et al. (2016). Immune cells attack and neurodegeneration in Gaucher disease. Mol. Genet. Metab. 117:S90.
Pandey, M. K., Saxena, V., and Agrawal, S. (2003). Characterization of mixed lymphocyte reaction blocking antibodies (MLR-Bf) in human pregnancy. BMC Pregnancy Childbirth 3:2. doi: 10.1186/1471-2393-3-2
Panegyres, P. (2004). The contribution of the study of neurodegenerative disorders to the understanding of human memory. QJM 97, 555–567. doi: 10.1093/qjmed/hch096
Papachroni, K. K., Ninkina, N., Papapanagiotou, A., Hadjigeorgiou, G. M., Xiromerisiou, G., Papadimitriou, A., et al. (2007). Autoantibodies to alpha-synuclein in inherited Parkinson’s disease. J. Neurochem. 101, 749–756. doi: 10.1111/j.1471-4159.2006.04365.x
Paris, D., Ait-Ghezala, G., Bachmeier, C., Laco, G., Beaulieu-Abdelahad, D., Lin, Y., et al. (2014). The spleen tyrosine kinase (Syk) regulates Alzheimer amyloid-beta production and Tau hyperphosphorylation. J. Biol. Chem. 289, 33927–33944. doi: 10.1074/jbc.M114.608091
Payton, J. E., Perrin, R. J., Woods, W. S., and George, J. M. (2004). Structural determinants of PLD2 inhibition by alpha-synuclein. J. Mol. Biol. 337, 1001–1009. doi: 10.1016/j.jmb.2004.02.014
Peress, N. S., Fleit, H. B., Perillo, E., Kuljis, R., and Pezzullo, C. (1993). Identification of FcγRI, II and III on normal human brain ramified microglia and on microglia in senile plaques in Alzheimer’s disease. J. Neuroimmunol. 48, 71–79. doi: 10.1016/0165-5728(93)90060-c
Perez, R. G., Waymire, J. C., Lin, E., Liu, J. J., Guo, F., and Zigmond, M. J. (2002). A role for α-synuclein in the regulation of dopamine biosynthesis. J. Neurosci. 22, 3090–3099. doi: 10.1523/JNEUROSCI.22-08-03090.2002
Polinsky, R. J., McRae, A., Baser, S. M., and Dahlström, A. (1991). Antibody in the CSF of patients with multiple system atrophy reacts specifically with rat locus ceruleus. J. Neurol. Sci. 106, 96–104. doi: 10.1016/0022-510x(91)90201-h
Polman, C. H., and Killestein, J. (2006). Anti-myelin antibodies in multiple sclerosis: Clinically useful? J. Neurol. Neurosurg. Psychiatry 77:712. doi: 10.1136/jnnp.2006.089839
Potter, M. (1983). Structural correlates of immunoglobulin diversity. Surv. Immunol. Res. 2, 27–42. doi: 10.1007/BF02918394
Rafiq, K., Bergtold, A., and Clynes, R. (2002). Immune complex-mediated antigen presentation induces tumor immunity. J. Clin. invest. 110, 71–79. doi: 10.1172/JCI15640
Ravetch, J. V., and Lanier, L. L. (2000). Immune inhibitory receptors. Science 290, 84–89. doi: 10.1126/science.290.5489.84
Richter-Landsberg, C., Gorath, M., Trojanowski, J. Q., and Lee, V. M. (2000). Alpha-synuclein is developmentally expressed in cultured rat brain oligodendrocytes. J. Neurosci. Res. 62, 9–14. doi: 10.1002/1097-4547(20001001)62:1<9::AID-JNR2>3.0.CO;2-U
Ricklin, D., Reis, E. S., and Lambris, J. D. (2012). A sweet spot to control complement-induced inflammation. Nat. Med. 18, 1340–1341. doi: 10.1038/nm.2916
Sardi, S. P., Clarke, J., Viel, C., Chan, M., Tamsett, T. J., Treleaven, C. M., et al. (2013). Augmenting CNS glucocerebrosidase activity as a therapeutic strategy for parkinsonism and other Gaucher-related synucleinopathies. Proc. Natl. Acad. Sci. U.S.A. 110, 3537–3542. doi: 10.1073/pnas.1220464110
Satoh, J.-I., Tabunoki, H., Ishida, T., Yagishita, S., Jinnai, K., Futamura, N., et al. (2012). Phosphorylated Syk expression is enhanced in Nasu-Hakola disease brains. Neuropathology 32, 149–157. doi: 10.1111/j.1440-1789.2011.01256.x
Scherzer, C. R., Grass, J. A., Liao, Z., Pepivani, I., Zheng, B., Eklund, A. C., et al. (2008). GATA transcription factors directly regulate the Parkinson’s disease-linked gene alpha-synuclein. Proc. Natl. Acad. Sci. U.S.A. 105, 10907–10912. doi: 10.1073/pnas.0802437105
Schweig, J. E., Yao, H., Beaulieu-Abdelahad, D., Ait-Ghezala, G., Mouzon, B., Crawford, F., et al. (2017). Alzheimer’s disease pathological lesions activate the spleen tyrosine kinase. Acta Neuropathol. Commun. 5:69. doi: 10.1186/s40478-017-0472-2
Schweig, J. E., Yao, H., Coppola, K., Jin, C., Crawford, F., Mullan, M., et al. (2019). Spleen tyrosine kinase (SYK) blocks autophagic Tau degradation in vitro and in vivo. J. Biol. Chem. 294, 13378–13395. doi: 10.1074/jbc.ra119.008033
Scott, D., and Roy, S. (2012). α-Synuclein inhibits intersynaptic vesicle mobility and maintains recycling-pool homeostasis. J. Neurosci. 32, 10129–10135. doi: 10.1523/JNEUROSCI.0535-12.2012
Scott, K. M., Kouli, A., Yeoh, S. L., Clatworthy, M. R., and Williams-Gray, C. H. (2018). A systematic review and meta-analysis of alpha synuclein auto-antibodies in parkinson’s disease. Front. Neurol. 9:815. doi: 10.3389/fneur.2018.00815
Seehafer, S. S., Ramirez-Montealegre, D., Wong, A. M., Chan, C.-H., Castaneda, J., Horak, M., et al. (2011). Immunosuppression alters disease severity in juvenile Batten disease mice. J. Neuroimmunol. 230, 169–172. doi: 10.1016/j.jneuroim.2010.08.024
Sharon, R., Bar-Joseph, I., Frosch, M. P., Walsh, D. M., Hamilton, J. A., and Selkoe, D. J. (2003). The formation of highly soluble oligomers of alpha-synuclein is regulated by fatty acids and enhanced in Parkinson’s disease. Neuron 37, 583–595. doi: 10.1016/s0896-6273(03)00024-2
Shushakova, N., Skokowa, J., Schulman, J., Baumann, U., Zwirner, J., Schmidt, R. E., et al. (2002). C5a anaphylatoxin is a major regulator of activating versus inhibitory FcgammaRs in immune complex-induced lung disease. J. Clin. Invest. 110, 1823–1830. doi: 10.1172/JCI16577
Skaper, S. D. (2012). The neurotrophin family of neurotrophic factors: an overview. Methods Mol. Biol. 846, 1–12. doi: 10.1007/978-1-61779-536-7_1
Skoff, A. M., Resta, C., Swamydas, M., and Adler, J. E. (2003). Nerve growth factor (NGF) and glial cell line-derived neurotrophic factor (GDNF) regulate substance P release in adult spinal sensory neurons. Neurochem. Res. 28, 847–854. doi: 10.1023/a:1023211107073
Smith, L. M., Schiess, M. C., Coffey, M. P., Klaver, A. C., and Loeffler, D. A. (2012). α-Synuclein and Anti-α-Synuclein Antibodies in Parkinson’s Disease, Atypical Parkinson Syndromes, REM Sleep Behavior Disorder, and Healthy Controls. PLoS One 7:e52285. doi: 10.1371/journal.pone.0052285
Spillantini, M. G., Divane, A., and Goedert, M. (1995). Assignment of human alpha-synuclein (SNCA) and beta-synuclein (SNCB) genes to chromosomes 4q21 and 5q35. Genomics 27, 379–381.
Spillantini, M. G., Schmidt, M. L., Lee, V. M. Y., Trojanowski, J. Q., Jakes, R., and Goedert, M. (1997). α-Synuclein in Lewy bodies. Nature 388, 839–840.
Stephenson, J., Nutma, E., van der Valk, P., and Amor, S. (2018). Inflammation in CNS neurodegenerative diseases. Immunology 154, 204–219. doi: 10.1111/imm.12922
Terryberry, J. W., Thor, G., and Peter, J. B. (1998). Autoantibodies in neurodegenerative diseases: antigen-specific frequencies and intrathecal analysis. Neurobiol. Aging 19, 205–216. doi: 10.1016/s0197-4580(98)00049-9
Ulvestad, E., Williams, K., Vedeler, C., Antel, J., Nyland, H., Mørk, S., et al. (1994b). Reactive microglia in multiple sclerosis lesions have an increased expression of receptors for the Fc part of IgG. J. Neurol. Sci. 121, 125–131. doi: 10.1016/0022-510x(94)90340-9
Ulvestad, E., Williams, K., Matre, R., Nyland, H., Olivier, A., and Antel, J. (1994a). Fc receptors for IgG on cultured human microglia mediate cytotoxicity and phagocytosis of antibody-coated targets. J. Neuropathol. Exp. Neurol. 53, 27–36. doi: 10.1097/00005072-199401000-00004
Vacirca, D., Delunardo, F., Matarrese, P., Colasanti, T., Margutti, P., Siracusano, A., et al. (2012). Autoantibodies to the adenosine triphosphate synthase play a pathogenetic role in Alzheimer’s disease. Neurobiol. Aging 33, 753–766.
van Rooijen, B. D., van Leijenhorst-Groener, K. A., Claessens, M. M., and Subramaniam, V. (2009). Tryptophan fluorescence reveals structural features of alpha-synuclein oligomers. J. Mol. Biol. 394, 826–833. doi: 10.1016/j.jmb.2009.10.021
Vidarsson, G., Dekkers, G., and Rispens, T. (2014). IgG Subclasses and Allotypes: From Structure to Effector Functions. Front. Immunol. 5:520. doi: 10.3389/fimmu.2014.00520
Vyshkina, T., and Kalman, B. (2008). Autoantibodies and neurodegeneration in multiple sclerosis. Lab. Invest. 88, 796–807. doi: 10.1038/labinvest.2008.53
Wang, A. V., Scholl, P. R., and Geha, R. S. (1994). Physical and functional association of the high affinity immunoglobulin G receptor (Fc gamma RI) with the kinases Hck and Lyn. J. Exp. Med. 180, 1165–1170. doi: 10.1084/jem.180.3.1165
Wang, T. T. (2019). IgG Fc Glycosylation in Human Immunity. Curr. Top. Microbiol. Immunol. 423, 63–75. doi: 10.1007/82_2019_152
Wyss-Coray, T. (2016). Ageing, neurodegeneration and brain rejuvenation. Nature 539, 180–186. doi: 10.1038/nature20411
Yang, C., Hou, X., Feng, Q., Li, Y., Wang, X., Qin, L., et al. (2019). Lupus serum IgG induces microglia activation through Fc fragment dependent way and modulated by B-cell activating factor. J. Transl. Med. 17:426. doi: 10.1186/s12967-019-02175-0
Yuasa, T., Kubo, S., Yoshino, T., Ujike, A., Matsumura, K., Ono, M., et al. (1999). Deletion of Fcγ Receptor IIB Renders H-2b Mice Susceptible to Collagen-induced Arthritis. J. Exp. Med. 189, 187–194. doi: 10.1084/jem.189.1.187
Yue, Q., Meng, Z., Wang, L., Sun, Q., Wang, S., Li, J., et al. (2019). The activation of immunoglobulin G Fc receptors (FcγRs) with immunoreceptor tyrosine-based activation motifs (ITAMs) promotes cognitive impairment in aged rats with diabetes. Exp. Gerontol. 125:110660. doi: 10.1016/j.exger.2019.110660
Zhang, W., Wang, T., Pei, Z., Miller, D. S., Wu, X., Block, M. L., et al. (2005). Aggregated alpha-synuclein activates microglia: a process leading to disease progression in Parkinson’s disease. FASEB J. 19, 533–542. doi: 10.1096/fj.04-2751com
Keywords: aging, neuro-immune crosstalk, memory deficits, autoimmunity, alpha-synuclein
Citation: Pandey MK (2022) The Role of Alpha-Synuclein Autoantibodies in the Induction of Brain Inflammation and Neurodegeneration in Aged Humans. Front. Aging Neurosci. 14:902191. doi: 10.3389/fnagi.2022.902191
Received: 22 March 2022; Accepted: 19 April 2022;
Published: 26 May 2022.
Edited by:
Federico Rodriguez-Porcel, Medical University of South Carolina, United StatesCopyright © 2022 Pandey. This is an open-access article distributed under the terms of the Creative Commons Attribution License (CC BY). The use, distribution or reproduction in other forums is permitted, provided the original author(s) and the copyright owner(s) are credited and that the original publication in this journal is cited, in accordance with accepted academic practice. No use, distribution or reproduction is permitted which does not comply with these terms.
*Correspondence: Manoj Kumar Pandey, bWFub2oucGFuZGV5QGNjaG1jLm9yZw==
Disclaimer: All claims expressed in this article are solely those of the authors and do not necessarily represent those of their affiliated organizations, or those of the publisher, the editors and the reviewers. Any product that may be evaluated in this article or claim that may be made by its manufacturer is not guaranteed or endorsed by the publisher.
Research integrity at Frontiers
Learn more about the work of our research integrity team to safeguard the quality of each article we publish.