- 1Research Group in Cognitive and Affective Neuroscience (NECEA), Department of Clinical Psychology and Psychobiology, University of Santiago de Compostela, A Coruña, Spain
- 2Economic Analysis and Modeling Group, Instituto de Estudios y Desarrollo de Galicia (IDEGA), Santiago de Compostela, Spain
- 3Pediatric Gastroenterology, Hepatology and Nutrition Unit, Hospital Clínico Universitario de Santiago, Instituto de Investigación Sanitaria de Santiago de Compostela (IDIS), Santiago de Compostela, Spain
- 4Unit of Investigation in Nutrition, Growth and Human Development of Galicia, Department of Forensic Sciences, Pathological Anatomy, Gynecology and Obstetrics, and Pediatrics, University of Santiago de Compostela, Santiago de Compostela, Spain
- 5CIBEROBN (Physiopathology of Obesity and Nutrition), Institute of Health Carlos III (ISCIII), Madrid, Spain
- 6Section of Endocrinology-Nutrition Area, Hospital Clínico Universitario de Santiago de Compostela, Santiago de Compostela, Spain
- 7Department of Social, Basic and Methodology Psychology, University of Santiago de Compostela, Santiago de Compostela, Spain
Background: Since many of the risk factors for cognitive decline can be modified by diet, the study of nutrition and its relationships with cognitive status in aging has increased considerably in recent years. However, there are hardly any studies that have assessed cognitive status using a comprehensive set of neuropsychological tests along with measures of functional capacity and mood and that have related it to nutritional status measured from several nutritional parameters that have shown its relationships with cognitive function.
Objective: To test the differences in depressive symptomatology and in several measures of nutritional status between three groups classified according to their cognitive status (CS hereafter).
Method: One hundred thirteen participants from nursing homes in Galicia, Spain, underwent a comprehensive neuropsychological examination, including a general screening test (MMSE) and tests for different cognitive domains along with measures of activities of daily living (ADL) and assessment of depressive symptomatology (GDS-SF). According to established clinical criteria, participants were divided into three CS groups, Cognitively Intact (CI), Mild Cognitive Impairment (MCI), and All-Cause Dementia (ACD). Nutritional status was also examined using blood-derived measures, body mass index (BMI) and a nutritional screening test (MNA-SF). Differences between CS groups in all nutritional variables were studied by one-way ANOVAs with post-hoc Bonferroni correction or Kruskal-Wallis with Games-Howell post-hoc correction when appropriate. Multinomial logistic regression was also applied to test the association between nutritional variables and CS.
Results: Differences between CS groups were statistically significant for depressive symptomatology, vitamin A and D, albumin, selenium (Se), uric acid (UA), and BMI. The results of multinomial logistic regression found positive associations between groups with better CS and higher concentrations of vitamins A and D, transthyretin (TTR), albumin, Se, and UA, while negative associations were found for BMI.
Conclusion: Higher serum levels of vitamin A, vitamin D, TTR, albumin, Se, and UA could act as protective factors against cognitive decline, whereas higher BMI could act as a risk factor.
Introduction
Demographic trends indicate that population is aging (Klímová and Vališ, 2018). In this context, there is an increase in health problems or conditions whose main risk factor is advanced age, including cognitive impairment (Power et al., 2019). The lack of effective treatments for cognitive impairment makes preventive strategies a public priority to preserve health and quality of life in advanced stages of life (Dye et al., 2017; Ma et al., 2017) and reduce the economic cost in public health resources (Miquel et al., 2018).
The aging process involves changes in cognitive functions associated with advancing age and genetic factors, as well as lifestyle-related variables. Therefore, assessment of the relationships between modifiable lifestyle factors, such as diet, and nutritional status and cognitive status (CS hereafter), is important to characterize nutritional patterns that may have a neuroprotective role, and thus aid in the prevention of cognitive decline.
It has been suggested that individuals at risk for cognitive decline may be identified by combinations of biological markers (Nettiksimmons et al., 2015). Blood-derived measures, compared to other commonly used methods to predict cognitive decline such as magnetic resonance imaging (MRI), fluorodeoxyglucose-Positron emission tomography (PET), cerebrospinal fluid (CSF), or protein levels from lumbar puncture, are less expensive and more familiar to patients and potential research participants (Nettiksimmons et al., 2015).
Taking the previous into account, the aim of this study was to look for nutritional indicators, mostly from blood-derived measures, that differ among groups classified according to their CS. This may help to identify markers that precede cognitive decline or that can identify individuals at risk for cognitive decline and dementia.
The study of nutrition and its relationship to cognitive function and dementia has increased in recent years. Yet, to our knowledge, there is a lack of studies that have assessed cognitive status using a comprehensive set of neuropsychological tests along with measures of activities of daily living (ADL) and assessment of affective state, and that have related it to nutritional status measured from blood-derived measures, anthropometric measures, and a nutritional status screening test combined.
The relationships between blood-derived measures and cognitive function have been widely studied for some biochemical variables and micronutrients, while others remain almost unstudied. The group of vitamins is among the micronutrients that have received most attention and accumulates more evidence on its relationships with cognitive function. For this group, the most studied has been vitamin D because of its neuroprotective, anti-inflammatory, and antioxidant effects (Sultan et al., 2020). Results on vitamin D and cognitive function suggest that its main circulating form, 25-hydroxyvitamin D (25-OH vitamin D), is closely associated to cognitive function and that may play an important role against cognitive decline (Kalueff and Tuohimaa, 2007; Buell and Dawson-Hughes, 2008; Seamans et al., 2010; Schlögl and Holick, 2014; Toffanello et al., 2014; Wilson et al., 2014; Jorde et al., 2015; Kueider et al., 2016; Goodwill et al., 2018; Duchaine et al., 2020; reviews in Dickens et al., 2011; Van der Schaft et al., 2013; Goodwill and Szoeke, 2017; Chai et al., 2019; Sultan et al., 2020). Serum folate and serum vitamin B12 levels have also been related to cognitive decline and dementia, although findings are inconclusive (Elias et al., 2006; Kang et al., 2006; Michelakos et al., 2013; Moore et al., 2014; Rabensteiner et al., 2020; for a review see Rosenberg, 2008; O’Leary et al., 2012). One of the less studied vitamins in this context is vitamin A, and its derived factors such as retinoids. In recent years, there has been an increasing number of studies, mainly in animal models, linking retinoids to the etiology of Alzheimer’s Disease (AD) (Goodman and Pardee, 2003; Goodman, 2006; Malaspina and Michael-Titus, 2008; Carratu et al., 2015). Some authors even propose that retinoic acid may have therapeutic properties for the treatment of neurodegenerative diseases such as AD (Lee et al., 2009) given their role in the modulation of neurogenesis, neuronal survival and synaptic plasticity (Olson and Mello, 2010). Nevertheless, to our knowledge studies testing relationships between this vitamin and cognitive function in aging are lacking.
Aging is also associated with increased levels of circulating cytokines and proinflammatory markers (Lim et al., 2013; Michaud et al., 2013) such as interleukins, Tumor Necrosis Factor-α (TNF-α), and C-Reactive Protein (CRP). This group of biochemical parameters is receiving increasing attention as inflammation may affect the central nervous system modulating cognitive function and mediating in cognitive impairment (Ray et al., 2007; McAfoose and Baune, 2009; Gorelick, 2010; Ownby, 2010; O’Bryant et al., 2010; Valls-Pedret et al., 2012; Koyama et al., 2013; Lim et al., 2013; Michaud et al., 2013; Petersen et al., 2020).
There is also evidence for relationships between cognitive changes in aging and other blood-derived measures, although supported by fewer studies. Thus, serum visceral proteins, such as prealbumin or transthyretin (TTR) and albumin, have been both considered markers of nutritional status (Smith, 2017; Keller, 2019) and cognitive function (Onem et al., 2010; Velayudhan et al., 2012; Nettiksimmons et al., 2015; Araghi et al., 2021). Although selenium (Se) is known to play an antioxidant role as it is the main constituent of antioxidant enzymes that are expressed in different tissues, including the brain (Cardoso et al., 2014), there are few studies on the relationship between Se concentrations and CS or cognitive impairment (Cardoso et al., 2010, 2014; Berr et al., 2012; for a review see Smorgon et al., 2004). Another natural antioxidant compound with beneficial properties is uric acid (UA) (Tuven et al., 2017; for a review see Tana et al., 2018). However, UA may also act as a pro-inflammatory compound and there still exists discussion on its oxidant-antioxidant properties (Sautin and Johnson, 2008). UA levels have been associated with cognitive performance (Annanmaki et al., 2008), MCI (Rinaldi et al., 2003; Pellecchia et al., 2016), different types of dementia (Rinaldi et al., 2003; Kim et al., 2006; Schlesinger and Schlesinger, 2008; Ruggiero et al., 2009; Al-Khateeb et al., 2015; Wen et al., 2017; Xu et al., 2017; Latourte et al., 2018; Serdarevic et al., 2020; Boccardi et al., 2021) and risk of dementia (González-Aramburu et al., 2014; for a review see Schlesinger and Schlesinger, 2008; for a meta-analysis see Khan et al., 2016; Zhou et al., 2021). Other micronutrients or blood biochemistry variables that have been related to CS although studies are more scarce, are serum iron, ferritin, and transferrin (Umur et al., 2011; Yavuz et al., 2012), total cholesterol (TC), High Density Lipoprotein Cholesterol (HDL-C), Low Density Lipoprotein Cholesterol (LDL-C) (Van Exel et al., 2002; Van Vliet, 2012; Crichton et al., 2014; Lv et al., 2016; Ma et al., 2017), zinc (Cuajungco and Fagét, 2003; Lam et al., 2008; Nuttall and Oteiza, 2014), Mean Corpuscular Volume (MCV) (Gamaldo et al., 2013), calcium (Schram et al., 2007), insulin (Stolk et al., 1997), gamma-glutamyl transferase (GGT) (Lee et al., 2020; Tang et al., 2021), alanine aminotransferase (ALT) (Nho et al., 2019), and glucose (Korol and Gold, 1998; Seetharaman et al., 2015).
The study of the relationships between cognitive and nutritional status should also consider anthropometric indexes of obesity, such as Body Mass Index (BMI). Although the relationships between obesity and cognitive function have been widely studied the findings from previous studies are inconclusive (for a meta-analysis see Danat et al., 2019). Obesity has also been linked to depression (Noh et al., 2015). The evidence from epidemiological and clinical trials suggest a bidirectional connection between overweight and psychological health (for a review see Luppino et al., 2010). Given that obesity is a prevalent condition at older ages, and that the study of the relationships between BMI and CS has led to discrepant results, BMI measurement was also included in the present study.
Relationships between cognitive impairment or dementia and depressive symptoms have also been the subject of previous research studies. Depressive symptoms have been related to lower cognitive performance (Ganguli et al., 2006; Godin et al., 2007) and some authors even suggest that depression may be a risk factor for the development of cognitive impairment (Van Den Kommer et al., 2013). However, a meta-analysis by Huang et al. (2011) suggests that dementia may be also a risk factor for depression. Taking the above into account, depressive symptomatology was also assessed in the present study.
In summary, although the relationships between cognitive function and nutritional status are increasingly studied, few studies have included a set of neuropsychological tests to assess the main cognitive domains, measures of functional capacity and affective symptomatology. Moreover, to our knowledge, there are hardly any studies that have examined these relationships by establishing a clinical diagnosis of CS in different groups classified according to the severity of their cognitive impairment and limitations in ADLs, based on recognized criteria and that have combined it with blood biochemical variables and anthropometric and nutritional measures. Therefore, in the present study the main objective was to examine the differences between three groups classified according to their CS in the presence of depressive symptomatology, and in several measures of nutritional status: blood markers, BMI, and the Mini Nutritional Assessment-Short Form (MNA-SF).
Materials and Methods
Participants
One hundred ninety-six participants were recruited from 11 public nursery homes from Galicia, Spain. From the original sample, 113 participants from 63 to 97 years were finally included in this study (mean age 83.1 ± 6.2, 62.8% women). The 113 enrolled participants had a complete dataset at the time of data analysis including sociodemographic characteristics, neuropsychological testing, functional capacity, depressive symptoms assessment, blood biomarkers, anthropometric measures, and nutritional assessment. The interval between cognitive and nutritional assessments was less than 6 months for all participants. Exclusion criteria included previous dementia diagnosis, acquired brain damage, current chemotherapy or radiation therapy, and/or an inability to complete the neuropsychological tests due to significant sensory difficulties such as visual and/or hearing impairments not adequately corrected. All the procedures were approved by the Central Ethics and Research Committee of Galicia Autonomous Community (2017/542), and the study was performed in accordance with the ethical standards established in the Declaration of Helsinki. Written consent was obtained from each participant.
Cognitive Status Assessment
Neuropsychological testing was carried out along with ADL assessment and depressive symptomatology examination by the same clinical neuropsychologist. The tests applied were selected to minimize the effects of education and socioeconomic level.
Neuropsychological Examination
A neuropsychological battery composed by tests designed to measure global cognitive function, and specific cognitive domains (attention, learning, memory, visuoconstructive ability, processing speed, and executive function) was applied as follows. The Mini-Mental State Examination (MMSE, Folstein et al., 1975) was used to evaluate global cognitive function. Attention was assessed using Digits subtest (digits forward) of the Wechsler Adult Intelligence Scale (WAIS-IV) (Wechsler, 2008), and Color Trail Test (CTT) (D’Elia et al., 1996) first form. To assess learning and verbal memory processes the administered test was the Rey Auditory Verbal Learning Test (RAVLT, Rey, 1964), and to measure visual immediate memory, the Rey-Osterrieth Complex Figure (ROCF, Rey, 1941) was administered. Working memory was evaluated using digits backward and sequencing of the Digits Subtest (WAIS-IV, Wechsler, 2008). Visuoperceptive and visuoconstructive skills were evaluated with the copy assay of ROCF. Processing speed was evaluated using the Symbol Search and Coding subtest, along with the processing speed index of the WAIS-IV (Wechsler, 2008). Executive function was measured with a phonetic fluency measure using the PMR (Artiola et al., 1999) which was chosen instead of FAS (Borkowski et al., 1967) because these letters are more appropriate for Spanish vocabulary (Artiola et al., 1999), the Five Digits Test (Sedó, 2007) and CTT second form (D’Elia et al., 1996).
Basic and Instrumental Activities of Daily Living
Barthel Index was used to determine participants capacity in basic activities of daily living (BADL, Mahoney and Barthel, 1965). Instrumental activities of daily living (IADL) were assessed using an adaptation of the Lawton and Brody index (Lawton and Brody, 1969), including items for institutionalized participants (i.e., ability to use telephone, shopping, ability to handle finances, public transportation, and nursing home and health services use).
Based on the results obtained from the neuropsychological examination and the performance in ADL, participants were divided in three different CS groups, Cognitively Intact (CI), Mild Cognitive Impairment (MCI), and All Cause Dementia (ACD). This classification was performed by a qualified neuropsychologist following established criteria (see Table 1) drawn from the Spanish Neurology Society (SEN; Robles et al., 2002), the Working Group from the National Institute on Aging and Alzheimer’s Association (NIA-AA; McKhann et al., 2011; Croisile et al., 2012), the Diagnostic and Statistical Manual of Mental Disorders-Fifth Edition (DSM-V; American Psychiatric Association, 2013) and the International Working Group on Mild Cognitive Impairment (Winblad et al., 2004).
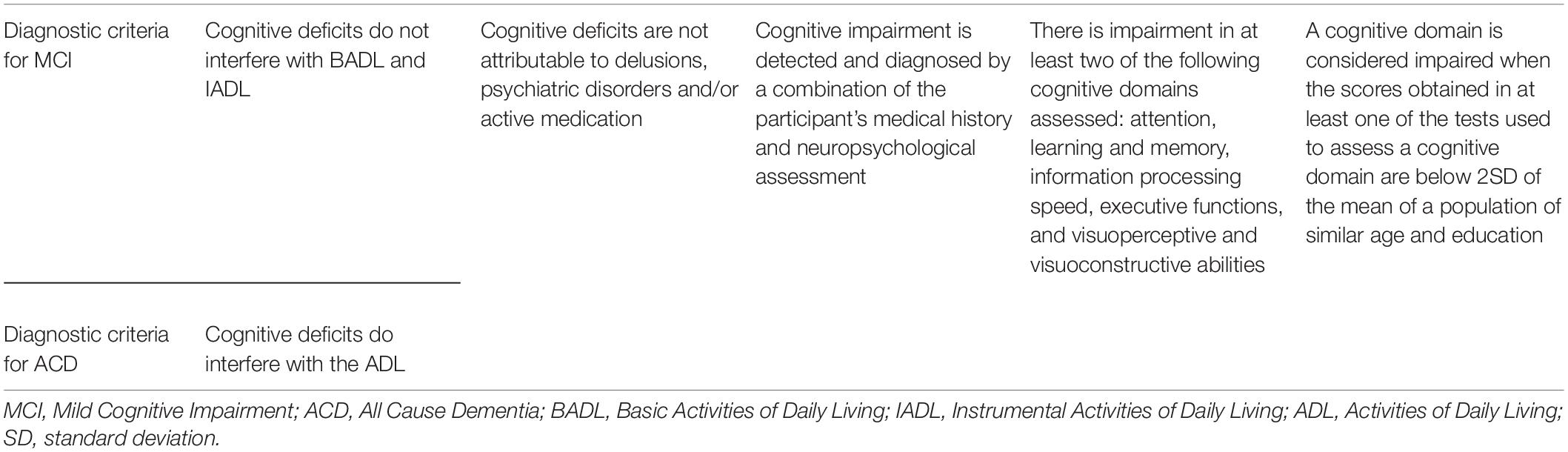
Table 1. Diagnostic criteria (differential: column 1; common: columns 2–5) to classify participants according to their cognitive status.
Depressive Symptomatology Evaluation
To evaluate the existence of depressive symptoms the short form of the Geriatric Depression Scale (GDS-SF, Sheikh and Yesavage, 1986) was administered to each participant (see Table 2).
Nutritional Screening
Blood Collection and Analyses
Blood samples were drawn from the antecubital vein between 08:00 and 09:30 h after an overnight fast and rest. Blood samples were taken from all study participants in the following types of tubes: EDTA K2 for hemogram and blood bank, separator Gel for clinical chemistry determinations, and Silica, for determination of trace elements. The samples were protected from sunlight and refrigerated. The serum and plasma were separated and cryopreserved at –80°C until the analysis was conducted. Routine blood tests were analyzed at the Central Laboratories of the University Clinical Hospital of Santiago de Compostela. Biochemical, hematological basic and advanced determinations of inflammatory parameters, oxidative stress and bone health analytical procedures were performed with instrumentation and reagents from Siemens Healthineers, and selenium was determined by Inductively Coupled Plasma-Mass Spectrometry from Agilent Technologies, according to the manufacturer’s recommendations. Serum 25(OH)D concentrations were quantified using a direct competitive chemiluminescence immunoassay by the LIAISON method. Insulin resistance (IR) was calculated by Homeostatic Model Assessment of IR (HOMA-IR). The normal ranges for the biochemistry variables used are those established by the Galician Public Health Service, reviewed in this case by specialist physicians (see Table 3).
Anthropometric Measures
Body weight and height were measured using properly calibrated scales and a height rod or tape measure, correspondingly. Wheelchair weighing scales were used for measuring the weight for participants unable to stand, as measurement of forearm length or from measurement between knee and heel following the criteria from The “Must” Explanatory Booklet (Malnutrition Advisory Group [MAG], 2003). BMI was calculated for every participant, following the World Health Organization (WHO) criteria (WHO, 2021).
Nutritional Assessment
The Mini Nutritional Assessment-Short Form (MNA-SF; Rubenstein et al., 2001) is a screening tool to help identifying elderly participants who are malnourished or at risk of malnutrition. This instrument has been validated by Kaiser et al. (2009) and was administered to all participants.
Statistical Analyses
To test for possible sex differences between the CS groups (CI, MCI, and ACD) a Chi-Square test was run. Differences in age, years of education, depressive symptoms, and nutritional variables were tested by one-way ANOVAs with Bonferroni post-hoc correction for pairwise multiple comparisons. For ANOVA analyses, the assumption of homogeneity of variances was tested by Levenne’s statistic and normality by the Kolmogorov-Smirnov test for all variables. For variables that did not meet one or both assumptions, the corresponding non-parametric Kruskal-Wallis test was applied to explore differences between groups and the Games-Howell test for pairwise comparisons.
Multinomial logistic regression was used to predict the probability of belonging to the CS group as the dependent variable, on the different variables of nutritional status considered as continuous. The reference group was ACD, and the results are expressed as Odds Ratios (OR) (see Table 4). For these statistical analyses, blood biochemical variables that have been shown to be associated with cognitive functions in previous literature were selected. These included blood count parameters, lipid profile, glycemic profile, protein profile, liver profile, vitamins, minerals, and inflammatory factors (see Table 3). P-values < 0.05 were considered significant. Statistical analyses were performed with SPSS software (version 25, SPSS, Inc., Chicago, IL, United States).
Results
Demographic characteristics, and descriptive statistics for depressive symptoms and nutritional variables are summarized in Tables 2, 3 using means and standard deviations for continuous variables or frequencies for categorical variables. According to the diagnostic criteria (Table 1), 28.3% participants were classified as CI, 43.4% as MCI and 28.3% as ACD. There were no significant differences in age [F(2, 112) = 0.89, p = 0.41] or sex (χ2, p = 0.66) between groups. However, differences in education years were significant [F(2, 112) = 9.74, p < 0.001], with participants in CI group having more years of schooling compared to MCI (p < 0.002) and ACD (p < 0.001). Differences in depressive symptoms were also statistically significant [F(2, 112) = 6.04, p < 0.005], with greater presence of symptomatology in the ACD group compared to CI group (p < 0.005) (see Figure 1). According to the data obtained from the MNA-SF, 81.2% of the total sample was classified as having normal nutritional status (see Table 3). ANOVAs and multinomial logistic regression results (see Table 4) that have reached statistical significance are presented in the following sections.
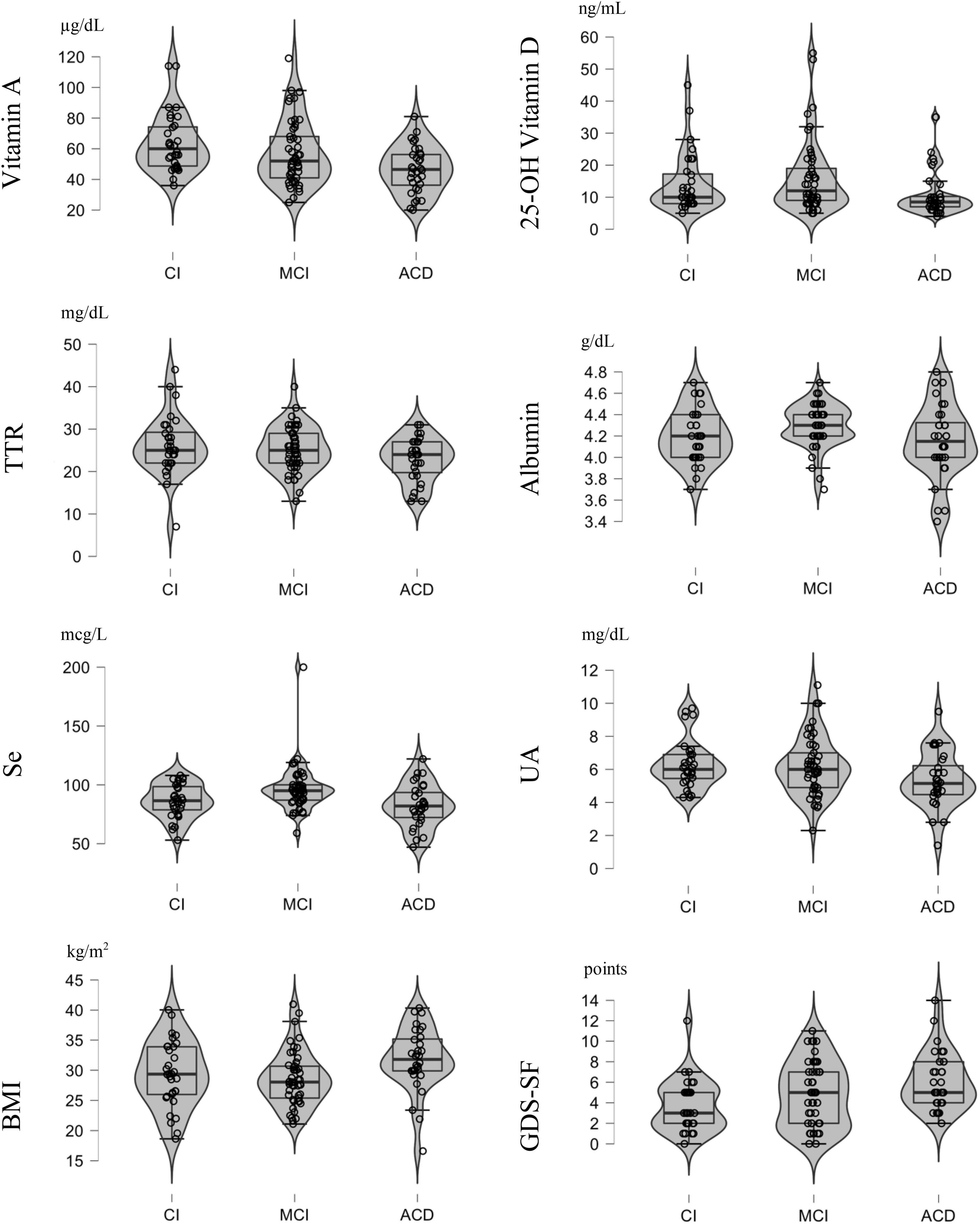
Figure 1. Distribution graphs of the variables which achieved statistically significant differences across cognitive status groups (CI, Cognitively Intact; MCI, Middle Cognitive Impairment; ACD, All Cause Dementia).
Vitamins
Analysis of variance showed significant differences between the groups in serum vitamin A concentrations [F(2, 112) = 6.65, p < 0.005] and post hoc pairwise comparisons confirmed those differences between CI and ACD groups (p < 0.001). The assumption of homogeneity of variances was met, although the variable vitamin A was not normally distributed according to the results of the Kolmogorov-Smirnov test [F(2, 112) = 0.11, p < 0.005]. Kruskal-Wallis test was administered as the corresponding non-parametric technique [H(2, 112) = 11.94, p < 0.005] confirming the results found by the ANOVA analysis. Regression results (Table 4) showed that higher levels of serum vitamin A were positively associated with greater odds of belonging to CI or MCI groups with respect to the reference group (ACD). The serum 25-OH vitamin D concentrations also differed significantly across groups [F(2, 112) = 3.12, p < 0.05]. Post hoc pairwise comparisons supported those differences between the MCI and ACD groups (p < 0.05) and indicated that MCI participants had higher levels of this vitamin than ACD participants, with no differences between CI and MCI groups. The assumption of homogeneity of variances was met, although the variable vitamin D was also not normally distributed according to the results of the Kolmogorov-Smirnov test [F(2, 112) = 0.21, p < 0.001]. The Kruskal-Wallis test [H(2, 112) = 9.52, p < 0.05] confirmed the results found by the ANOVA analysis. Higher blood vitamin D levels were positively related to higher odds of belonging to MCI compared to ACD, according to regression results. The results for the CI group were not statistically significant.
Proteins
There were no significant variations in serum TTR concentrations across groups, according to ANOVA. The hypothesis of homogeneity of variances and the assumption of normality were satisfied. No significant differences were detected in pairwise comparisons either. According to the regression results (Table 4), higher levels of serum TTR were positively associated with a higher likelihood of belonging to the CI group with respect to ACD. The results for the MCI group did not reach statistical significance (Table 4). ANOVA analyses revealed significant differences in serum albumin concentrations across CS groups [F(2, 112) = 3.43, p < 0.05], and post hoc pairwise comparisons between MCI and ACD groups corroborated those differences (p < 0.05). The assumption of homogeneity of variances was not met, so Games-Howell as robust technique of equality of means was also applied to confirm the results, although no significant differences were found between the groups. The variable albumin was not either normally distributed according to the results of the Kolmogorov-Smirnov test [F(2, 112) = 0.13, p < 0.001]. Kruskal-Wallis test was administered as the corresponding non-parametric technique [H(2, 112) = 7.39, p < 0.05] confirming the results found by the ANOVA analysis. Higher serum albumin concentrations were positively associated with greater odds of belonging to the MCI group with respect to ACD group, according to regression results. Statistical significance was not reached in the results for the CI group.
Antioxidants
Significant differences between the groups in serum Se concentrations were found [F(2, 112) = 7.23, p < 0.001] and post hoc pairwise comparisons confirmed those differences between CI and MCI groups (p < 0.05) and between MCI and ACD groups (p < 0.001). The hypothesis of homogeneity of variances and the assumption of normality were satisfied. Regression results showed that higher serum Se concentrations were positively associated with increased odds of belonging to MCI group with respect to ACD group (Table 4). Additionally, ANOVA showed significant differences between CS groups in serum UA concentrations [F(2, 112) = 3.33, p < 0.05]. Post hoc pairwise comparisons did not reach statistical significance. The assumption of homogeneity of variances was met, although UA was not normally distributed according to the results of the Kolmogorov-Smirnov test [F(2, 112) = 0.09, p < 0.05]. Kruskal-Wallis test was administered [H(2, 112) = 6.09, p < 0.05] confirming the results found by the ANOVA analysis. Also, higher levels of serum UA were positively associated with greater odds of belonging to the CI and MCI groups with respect to the ACD group according to regression results.
Body Mass Index
Significant differences in BMI between CS groups were found in ANOVA analyses [F(2, 112) = 4.12, p < 0.05] and post hoc pairwise comparisons confirmed those differences between MCI and ACD groups (p < 0.05). The hypothesis of homogeneity of variances and the assumption of normality were satisfied. Regression results showed that higher levels of BMI were negatively associated with greater odds of belonging to MCI group with respect to the reference group (see Table 4). The results for the CI group did not reach statistical significance. However, it is worth highlighting that the mean BMI for the groups with better CS (CI and MCI) was in the overweight range, while the mean BMI for the most cognitively impaired group (ACD) was in the obese range (see Table 3).
Discussion
Because nutritional status could be considered as a potential marker for cognitive decline and a target in the search for preventive strategies to delay the onset or progression of cognitive impairment, in the current study, we examined the differences in nutritional status, assessed by blood markers, BMI and the MNA-SF nutritional screening test, across three groups of institutionalized older participants classified according to their CS. Additionally, the possible differences in depressive symptomatology between CS groups were also examined.
Significant differences between CS groups were found for concentrations of vitamin A, vitamin D, albumin, Se, UA, and BMI. Moreover, better CS was associated with higher concentrations of vitamins A and D, TTR, albumin, Se and UA, while worse CS was associated with higher BMI. Finally, depressive symptomatology was greater in the ACD group compared to CI group.
In the following sections we will discuss our results in the context of previous literature. For a smoother reading the cognitive assessment and/or the diagnostic criteria applied by the studies included in the discussion are indicated in Table 5.
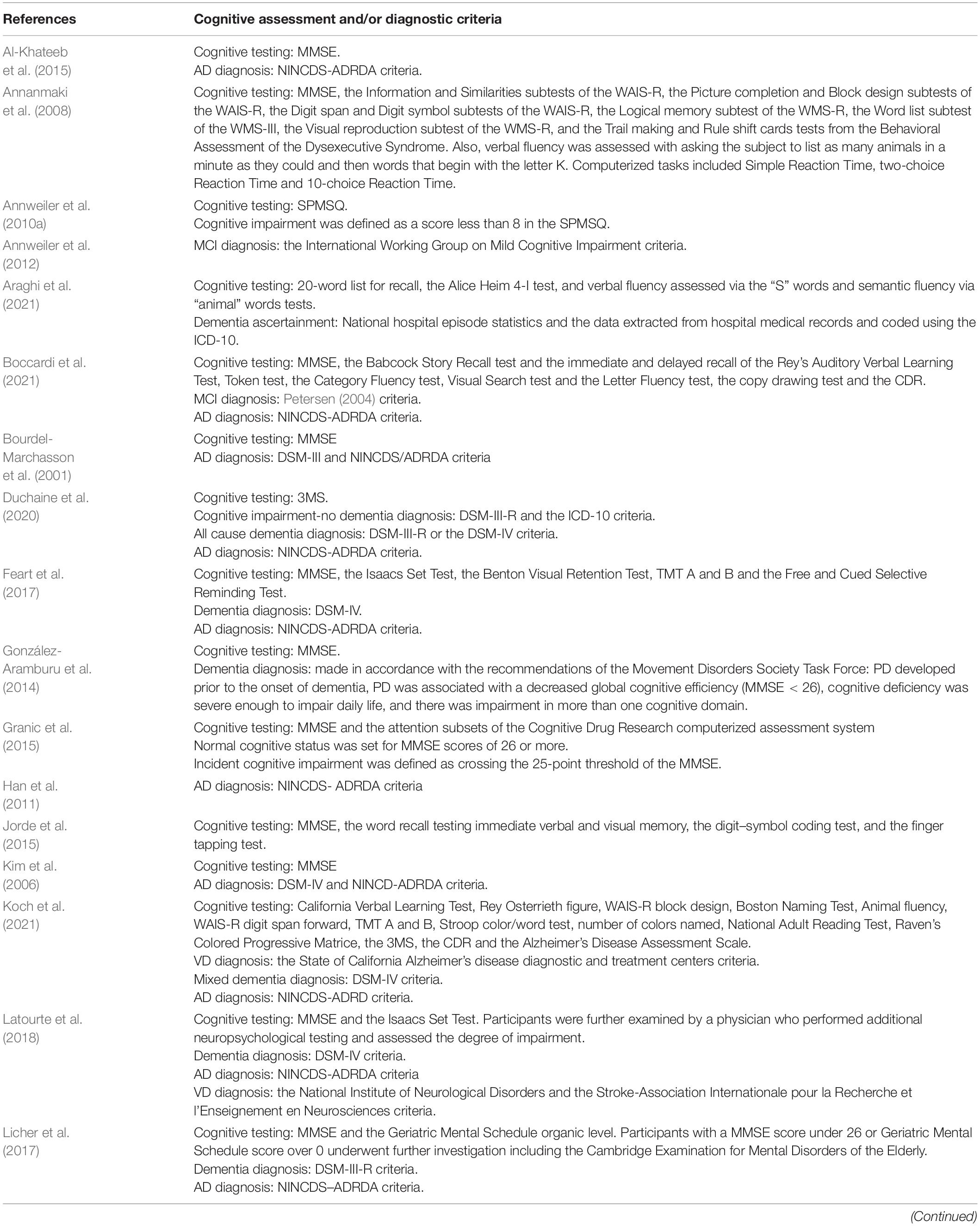
Table 5. Cognitive assessment or diagnostic criteria applied by the studies included in the discussion section.
Vitamins
In the present study higher levels of vitamin A were associated with better CS and with greater odds of belonging to the CI and MCI groups compared with ACD group. Very little has been found in the previous literature on vitamin A concentrations and cognitive function in aging. Significant reductions in serum vitamin A levels were found in participants with dementia (Raszewski et al., 2016) and in AD patients (Bourdel-Marchasson et al., 2001; Rinaldi et al., 2003). Rinaldi et al. (2003) also examined the associations with MCI, and found peripheral levels of antioxidants, such as UA, vitamin C, vitamin E, and vitamin A, were similarly lower in patients with MCI and AD compared to controls. The above presented findings from this study go a step further, given that the CI group presented the highest vitamin A levels, followed by the MCI group and finally the ACD group, so it appears that CS decreases as vitamin A concentrations does (see Table 3). Bourdel-Marchasson et al. (2001) suggested that lower plasma retinol in well-nourished elderly AD patients compared to control subjects may mean that this antioxidant vitamin has been consumed by excessive free radical production and Rinaldi et al. (2003) propose that given that MCI may represent a prodromal stage for AD, and oxidative damage is one of the earliest pathophysiological events in AD, increasing antioxidant intake in patients with MCI could be beneficial to reduce the risk of progression to dementia.
Due to its anticholinesterase, antioxidant and anti-inflammatory potential, vitamin A has been shown to have a memory-restoring function in mice (Sodhi and Singh, 2013) and to inhibit the formation and extension of beta-amyloid fibrins (Ono and Yamada, 2012). Likewise, it has been reported that brain fluid or plasma concentrations of vitamin A and beta-carotene are lower in AD and that an increase in their concentrations has been shown to slow the progression of dementia, due to their antioxidant, cell-protective, and antiaggregatory effects in in vitro and in vivo models (Ono and Yamada, 2012). Conversely, in a recent study Koch et al. (2021) found no relationship between plasma concentrations of retinol, tocopherol and carotenoids among other antioxidants and the risk of mixed dementia, vascular dementia (VD) or AD. Further studies are needed to understand the relationships between vitamin A and cognition, especially in aging.
In the present study, higher serum 25-OH vitamin D concentrations were found in the groups with better CS and were associated with higher odds of belonging to the MCI group compared to the ACD group. This vitamin has received the most attention in the study of the association between its serum levels and cognitive functioning both in the elderly without impairment and in those with cognitive impairment or dementia, although a significant number of studies have only used measures of global cognitive screening or in combination with some test of attention or memory functions. Moreover, the results obtained are not free of divergences. Specifically, in non-impaired participants associations between higher 25-OH vitamin D levels and better cognitive function examined through global screening tests have been previously reported (Przybelski and Binkley, 2007; Annweiler et al., 2010a; Wilson et al., 2014) whereas studies having included global cognitive performance screening measures along with tests for specific cognitive domains have reported divergent results (Table 5). Thus, Jorde et al. (2015) found that performance in the MMSE and specific measures of memory, psychomotor speed, attention, and logical reasoning test improved with increasing serum 25-OH vitamin D, while Granic et al. (2015) found low and high season-specific 25-OH vitamin D quartiles to be associated with poorer performance compared with those participants in the middle quartiles in attention tasks. Concerning the association between 25-OH vitamin D and cognitive decline, MCI, or dementia the results indicate that lower levels are related to higher risk of cognitive decline (Toffanello et al., 2014; Wilson et al., 2014; Feart et al., 2017) and dementia (Licher et al., 2017), that MCI patients present lower mean serum 25OHD concentrations (Annweiler et al., 2012), and that older participants with vitamin D deficiency and insufficiency compared to those with vitamin D sufficiency, had double risk of all cause dementia (Feart et al., 2017). Conversely, Duchaine et al. (2020) did not find significant associations between 25-OH vitamin D and cognitive decline or dementia. Even found that higher 25-OH vitamin D levels were associated to increased risk of dementia and AD for women. Overall, the evidence collected through multiple systematic reviews and meta-analyses confirms the relationships between higher vitamin D levels and better cognitive performance or lower risk of cognitive impairment or dementia (Annweiler et al., 2009; Dickens et al., 2011; Van der Schaft et al., 2013; Goodwill and Szoeke, 2017; Sommer et al., 2017; Chai et al., 2019; Sultan et al., 2020). Vitamin D has been associated with many neurological functions, and its deficiency with neurological dysfunction (for a review see Annweiler et al., 2010b). Although there are a growing number of studies examining the relationships between vitamin D concentrations and different types of dementia, there are hardly any studies examining the differences between different cognitive statuses that have been classified according to the severity of cognitive impairment and the impact it causes in the activities of daily living, following established criteria after a comprehensive neuropsychological examination. The present results add further support to the well-established knowledge of the neuroprotective attributes of vitamin D (Kalueff and Tuohimaa, 2007; for a review see Buell and Dawson-Hughes, 2008).
Proteins
In our sample, higher levels of TTR were positively associated with better CS and with a higher likelihood of belonging to the CI group with respect to ACD. In agreement with the present results, previous studies have found that higher TTR levels were associated with better cognitive function. Araghi et al. (2021) found in participants at mean age 55 that higher global and specific-domain cognitive scores in memory, reasoning, and verbal fluency were linked with higher serum TTR. Also, in AD participants, lower plasma TTR levels were found when compared to non-demented controls (Han et al., 2011; Velayudhan et al., 2012). More specifically, Velayudhan et al. (2012) found that TTR levels were significantly lower in AD cases with rapid cognitive decline and with severe cognitive impairment and that TTR levels may additionally be predictive of subsequent cognitive decline. However, Araghi et al. (2021) failed to found such predictive properties. Conversely, a more recent study by Tien et al. (2019) found higher plasma TTR levels in MCI patients when compared to healthy participants, and even that higher plasma TTR was associated with the conversion from MCI to AD dementia.
Regarding albumin, higher concentrations were found in the groups with better CS (CI and MCI) and its levels were positively associated with a greater likelihood of belonging to the MCI group with respect to ACD. These findings are in the line of previous studies like Wang et al. (2018) who found that low serum albumin levels were associated with an increased risk of MCI. Ng et al. (2008) also found that poor cognitive performance increased with decreasing quintiles of albumin, and multivariate analyses showed that the lowest quintile of albumin was associated with a twofold increased risk of poorer cognitive performance, independent of other nutritional or risk factors. In the existent research on albumin concentrations and dementia, Kim et al. (2006) found significant reductions in albumin concentrations in AD patients compared to controls.
Finally, it should be emphasized that the majority of participants in the present study were within normal ranges for serum prealbumin and serum albumin levels. Since both proteins are considered markers of nutritional status (Smith, 2017; Keller, 2019), this is congruent with the results of the MNA-SF, which also indicated that most participants had normal nutritional status (see Table 3).
Antioxidants
In this study, higher Se levels were found for the groups with better CS and were also associated with increased odds of belonging to MCI group with respect to ACD group. Although there are few studies on the relationship between Se concentrations and CS or cognitive impairment, these results are consistent with previous literature which indicates that serum Se levels are significantly lower in patients with dementia and in patients with cognitive impairment without dementia (Smorgon et al., 2004; Cardoso et al., 2014). In fact, it has been proposed that deficiency in Se may act as a risk factor for cognitive decline (Berr et al., 2012; Cardoso et al., 2014). Moreover, a systematic review conducted by Loef et al. (2011) found that there is still insufficient evidence for a role of Se in the treatment for AD, but that it may have a potential preventive relevance. The findings on this mineral may be explained by the antioxidant role that it plays for the central nervous system and other body tissues (Rahman, 2007; Mehdi et al., 2013; Cardoso et al., 2014).
Higher serum UA concentrations were found for the group with better CS and with greater odds of belonging to the CI and MCI groups with respect to the ACD group. These findings are in agreement with previous studies that have found lower serum UA levels in patients with MCI (Rinaldi et al., 2003; Xue et al., 2017), and that low UA concentrations can even act as a risk factor for MCI (Xue et al., 2017). Moreover, lower serum UA levels have been also related to VD (Xu et al., 2017) and AD (Rinaldi et al., 2003; Kim et al., 2006; Al-Khateeb et al., 2015; Boccardi et al., 2021). Low UA concentrations have been also found in patients with Parkinson’s Disease (PD) (Wen et al., 2017; for a review see Schlesinger and Schlesinger, 2008) and are associated to worse cognitive performance (Annanmaki et al., 2008) and later occurrence of MCI in early PD stages (Pellecchia et al., 2016). However, other authors did not find serum UA levels to have a significant impact on the risk of dementia in PD (González-Aramburu et al., 2014). Some of these findings have been reported in a systematic review and meta-analysis by Khan et al. (2016) who concluded that serum UA was lower in patients with dementia, specifically, the associations were stronger with AD and Parkinson’s Disease Dementia (PDD) than with VD. There is also evidence on the relationship between low serum concentrations of UA and increased risk of dementia. A recent systematic review and meta-analysis by Zhou et al. (2021) found low concentrations of UA to act as a potential risk factor for AD and PDD but not for VD. However, it has also been found evidence in the opposite direction and higher concentrations of UA have been associated with an increased likelihood of dementia. Latourte et al. (2018) found increased risk of dementia with higher serum UA levels, analyses by dementia subtypes showed stronger associations with mixed or VD than with AD dementia. Ruggiero et al. (2009) also found higher UA concentrations in persons affected by dementia. The present results would give support to the proposed antioxidant role of UA, which has been implied in reducing plasma oxidative stress, eliminating free radicals, and playing a preventive role against neurodegenerative processes (Tuven et al., 2017; for a review see Tana et al., 2018).
Body Mass Index
In our sample, the two groups with better CS (CI and MCI) had lower BMI compared with ACD group, whose mean values were within obesity range. Additionally, a lower BMI was also associated to increased odds of belonging to MCI group with respect to ACD group. These findings are in agreement with the results of a systematic review conducted by Gorospe and Dave (2007) where increased BMI was associated with an increased risk of dementia. Furthermore, a recent study conducted by Ma et al. (2020) found that having a higher body weight or abdominal obesity are associated with a higher incidence of dementia. There is also evidence related to specific cognitive functions. Poorer language and executive functions performance was associated with higher BMI in a sample of moderately old men, while relatively better performance was associated with higher BMI in the oldest-old (Schmeidler et al., 2019). Zhang et al. (2018) found that participants in the highest BMI quartile had highest mean MMSE scores, and the lowest mean MMSE scores were found in those classified in the lowest BMI quartile. Nevertheless, the findings on BMI have been generally inconsistent, as on a meta-analysis conducted by Danat et al. (2019) thirteen studies found an inverse association between dementia and BMI, while three studies showed a positive association.
The inconsistency of these findings could be due to comorbidity with other chronic conditions such as diabetes, differences between the populations studied (e.g., European, or Asian population), differences in sex and age, as well as body fat distribution. Several mechanisms have been proposed to explain the associations between obesity and impaired cognitive function, including inflammation, elevated leptin, insulin resistance, neuronal degradation, and impaired brain metabolism or blood flow (Nguyen et al., 2014; Anjum et al., 2018).
Inflammatory markers, such as high levels of CRP have been found in the obese, where increased brain atrophy, increased white matter hyperintensities, and decreased total gray matter brain volume have also been observed (Prickett et al., 2015). In the present study, inflammatory markers such as IL, TNF, and CRP were analyzed in all participants and no significant differences were found among CS groups. Other suggested mechanisms underlying the relation between CS and obesity are related to comorbidities, which are common among obese adults, such as insulin resistance and type 2 diabetes, hypertension, impaired glucose tolerance, and dyslipidemia (Miquel et al., 2018). Again, the present results did not show significant differences among CS groups in glucose, insulin, or parameters of lipid profile. Thereafter, the above referred possible relationship between obesity and inflammation or other comorbidities cannot be established in this study.
Limitations
The present study has some limitations. First, no significant differences were found between the CS groups in biochemical variables that have been related to cognitive function in previous literature, such as vitamin B12, folate, glucose, cholesterol, or inflammatory markers. Second, associations between CS and nutritional status were examined in a cross-sectional design, which does not allow us to analyze the progression in CS of the participants. Third, given the cross-sectional design of the study, the main conclusion should be taken with caution. Finally, having included only institutionalized participants may make the present results less generalizable to the general population, but the study design emphasized the need to have more control of access to the population to obtain both CS and nutritional data in a more homogeneous setting.
Conclusion
To our knowledge, this is the first study to examine the relationships between cognitive and nutritional status by establishing the CS of the participants and classifying them into three groups based on the results obtained from a comprehensive neuropsychological examination comprising the main cognitive domains and assessment of ADLs following established clinical criteria, assessment of depressive symptomatology and including, to explore nutritional status, a wide variety of blood biomarkers, anthropometric measures, and a nutritional screening test.
From the present results it can be concluded that increased serum concentrations of vitamin A, vitamin D, TTR, albumin, Se and UA could act as a protective factor against cognitive impairment, whereas higher BMI could act as a risk factor. Ideally, future work should include large samples of institutionalized and independently living elderly, comprehensive neurocognitive screening and adequate assessment of nutritional status including multiple measures, as well as intervention and longitudinal designs to test the effects on cognitive function resulting from nutritional interventions and change over time.
Data Availability Statement
The raw data supporting the conclusions of this article will be made available by the authors, without undue reservation.
Ethics Statement
The studies involving human participants were reviewed and approved by the Central Ethics and Research Committee of Galicia Autonomous Community (2017/542). The patients/participants provided their written informed consent to participate in this study.
Rest Of The Members Of Nutriage Study Researchers
Melchor Fernández, Carlos Dieguez, Lucía Gayoso, Lourdes Vázquez Oderiz, Ángeles Romero, and Nicolás Piedrafita.
Author Contributions
ML performed the cognitive status assessment in all participants. ML and EA analyzed, interpreted the data, and wrote the manuscript. ML, MR, EA, and PP-Á designed the study, and collected and interpreted the data. LF, CA, ML, EA, and PP-Á designed the data analyses. RL and M-ÁM-O supervised the collection and analysis of nutritional data, and critically reviewed the manuscript. Melchor Fernández, Carlos Dieguez, Lucía Gayoso, Lourdes Vázquez Oderiz, Ángeles Romero, and Nicolás Piedrafita involved in the design, data collection and funding management of the NUTRIAGE study. All authors contributed to the article and approved the submitted version.
Funding
NUTRIAGE (0359_NUTRIAGE_1_E) was a research project 75% co-financed by the Interreg V-A Spain-Portugal Program (POCTEP) 2014–2020 through the European Regional Development Fund (ERDF) of the European Union.
Conflict of Interest
The authors declare that the research was conducted in the absence of any commercial or financial relationships that could be construed as a potential conflict of interest.
Publisher’s Note
All claims expressed in this article are solely those of the authors and do not necessarily represent those of their affiliated organizations, or those of the publisher, the editors and the reviewers. Any product that may be evaluated in this article, or claim that may be made by its manufacturer, is not guaranteed or endorsed by the publisher.
Acknowledgments
We thank the participants for their collaboration, as well as the management of the nursing homes and their workers who facilitated the organization of the participants and the collection of data.
References
Al-Khateeb, E., Althaher, A., Al-Khateeb, M., Al-Musawi, H., Azzouqah, O., Al-Shweiki, S., et al. (2015). Relation between uric acid and Alzheimer’s disease in elderly Jordanians. J. Alzheimers Dis. 44, 859–865. doi: 10.3233/JAD-142037
American Psychiatric Association (2013). Diagnostic and Statistical Manual of Mental Disorders, 5th Edn. Washington, DC: American Psychiatric Association.
Anjum, I., Fayyaz, M., Wajid, A., Sohail, W., and Ali, A. (2018). Does obesity increase the risk of dementia: a literature review. Cureus 10:e2660. doi: 10.7759/cureus.2660
Annanmaki, T., Pessala-Driver, A., Hokkanen, L., and Murros, K. (2008). Uric acid associates with cognition in Parkinson’s disease. Parkinsonism Relat. Disord. 14, 576–578. doi: 10.1016/j.parkreldis.2007.11.001
Annweiler, C., Allali, G., Allain, P., Bridenbaugh, S., Schott, A. M., Kressig, R. W., et al. (2009). Vitamin D and cognitive performance in adults: a systematic review. Eur. J. Neurol. 16, 1083–1089. doi: 10.1111/j.1468-1331.2009.02755.x
Annweiler, C., Fantino, B., Schott, A. M., Krolak-Salmon, P., Allali, G., and Beauchet, O. (2012). Vitamin D insufficiency and mild cognitive impairment: cross-sectional association. Eur. J. Neurol. 19, 1023–1029. doi: 10.1111/j.1468-1331.2012.03675.x
Annweiler, C., Schott, A. M., Allali, G., Bridenbaugh, S. A., Kressig, R. W., Allain, P., et al. (2010a). Association of vitamin D deficiency with cognitive impairment in older women: cross-sectional study. Neurology 74, 27–32. doi: 10.1212/WNL.0b013e3181beecd3
Annweiler, C., Schott, A. M., Berrut, G., Chauviré, V., Le, Gall D, Inzitari, M., et al. (2010b). Vitamin D and ageing: neurological issues. Neuropsychobiology 62, 139–150. doi: 10.1159/000318570
Araghi, M., Shipley, M. J., Anand, A., Mills, N. L., Kivimaki, M., Singh-Manoux, A., et al. (2021). Serum transthyretin and risk of cognitive decline and dementia: 22-year longitudinal study. Neurol. Sci. 42, 5093–5100. doi: 10.1007/s10072-021-05191-5
Artiola, L., Hermosillo, D., Heaton, R., and Pardee, R. E. (1999). Manual de Normas y Procedimientos Para la Batería Neuropsicológica en Español. Tucson, AZ: m Press.
Berr, C., Arnaud, J., and Akbaraly, T. N. (2012). Selenium and cognitive impairment: a brief-review based on results from the EVA study. BioFactors 38, 139–144. doi: 10.1002/biof.1003
Boccardi, V., Carino, S., Marinelli, E., Lapenna, M., Caironi, G., Bianco, A. R., et al. (2021). Uric acid and late-onset Alzheimer’s disease: results from the ReGAl 2.0 project. Aging Clin. Exp. Res. 33, 361–366. doi: 10.1007/s40520-020-01541-z
Borkowski, J. G., Benton, A. L., and Spreen, O. (1967). Word fluency and brain damage. Neuropsychologia 5, 135–140. doi: 10.1016/0028-3932(67)90015-2
Bourdel-Marchasson, I., Delmas-Beauviex, M. C., Peuchant, E., Richard-Harston, S., Decamps, A., Reigneir, B., et al. (2001). Antioxidant defences and oxidative stress markers in erythrocytes and plasma from normally nourished elderly Alzheimer patients. Age Ageing 30, 235–241. doi: 10.1093/ageing/30.3.235
Buell, J. S., and Dawson-Hughes, B. (2008). Vitamin D and neurocognitive dysfunction: preventing “D”ecline? Mol. Aspects Med. 29, 415–422. doi: 10.1016/j.mam.2008.05.001
Cardoso, B., Silva Bandeira, V., Jacob-Filho, W., and Franciscato Cozzolino, S. M. (2014). Selenium status in elderly: relation to cognitive decline. J. Trace Elements Med. Biol. 28, 422–426. doi: 10.1016/j.jtemb.2014.08.009
Cardoso, B. R., Ong, T. P., Jacob-Filho, W., Jaluul, O., Freitas, M. I. D. Á, and Cozzolino, S. M. F. (2010). Nutritional status of selenium in Alzheimer’s disease patients. Br. J. Nutr. 103, 803–806. doi: 10.1017/S0007114509992832
Carratu, M., Marasco, C., Signorile, A., Scuderi, C., and Steardo, L. (2015). Are retinoids a promise for Alzheimer’s disease management? Curr. Med. Chem. 19, 6119–6125. doi: 10.2174/0929867311209066119
Chai, B., Gao, F., Wu, R., Dong, T., Gu, C., Lin, Q., et al. (2019). Vitamin D deficiency as a risk factor for dementia and Alzheimer’s disease: an updated meta-analysis. BMC Neurol. 19:284. doi: 10.1186/s12883-019-1500-6
Crichton, G. E., Elias, M. F., Davey, A., Sullivan, K. J., and Robbins, M. A. (2014). Higher HDL cholesterol is associated with better cognitive function: the maine-syracuse study. J. Int. Neuropsychol. Soc. 29, 961–970. doi: 10.1017/S1355617714000885
Croisile, B., Auriacombe, S., Etcharry-Bouyx, F., and Vercelletto, M. (2012). The new 2011 recommendations of the National Institute on Aging and the Alzheimer’s Association on diagnostic guidelines for Alzheimer’s disease: preclinal stages, mild cognitive impairment, and dementia. Rev. Neurol. 168, 471–482. doi: 10.1016/j.neurol.2011.11.007
Cuajungco, M. P., and Fagét, K. Y. (2003). Zinc takes the center stage: its paradoxical role in Alzheimer’s disease. Brain Res. Rev. 41, 44–56. doi: 10.1016/S0165-0173(02)00219-9
Danat, I. M., Clifford, A., Partridge, M., Zhou, W., Bakre, A. T., Chen, A., et al. (2019). Impacts of overweight and obesity in older age on the risk of dementia: a systematic literature review and a meta-analysis. J. Alzheimers Dis. 70, 87–99. doi: 10.3233/JAD-180763
D’Elia, L., Satz, P., Uchiyama, C. L., and White, T. (1996). PAR | CTT | Color Trails Test. Color Trails Test. Available online at: https://www.parinc.com/Products/Pkey/77 (accessed 2022).
Dickens, A. P., Lang, I. A., Langa, K. M., Kos, K., and Llewellyn, D. J. (2011). Vitamin D, cognitive dysfunction and dementia in older adults. CNS Drugs 25, 629–639. doi: 10.2165/11593080-000000000-00000
Duchaine, C. S., Talbot, D., Nafti, M., Giguère, Y., Dodin, S., Tourigny, A., et al. (2020). Vitamin D status, cognitive decline and incident dementia: the Canadian Study of Health and Aging. Can. J. Public Health 111, 312–321. doi: 10.17269/s41997-019-00290-5
Dye, L., Boyle, N. B., Champ, C., and Lawton, C. (2017). The relationship between obesity and cognitive health and decline. Proc. Nutr. Soc. 76, 443–454. doi: 10.1017/S0029665117002014
Elias, M. F., Robbins, M. A., Budge, M. M., Elias, P. K., Brennan, S. L., Johnston, C., et al. (2006). Homocysteine, folate, and vitamins B6 and B12 blood levels in relation to cognitive performance: the Maine-Syracuse study. Psychosom. Med. 68, 547–554. doi: 10.1097/01.psy.0000221380.92521.51
Feart, C., Helmer, C., Merle, B., Herrmann, F. R., Annweiler, C., Dartigues, J. F., et al. (2017). Associations of lower vitamin D concentrations with cognitive decline and long-term risk of dementia and Alzheimer’s disease in older adults. Alzheimers Dement. 13, 1207–1216. doi: 10.1016/j.jalz.2017.03.003
Folstein, M. F., Folstein, S. E., and McHugh, P. R. (1975). Mini-mental state. A practical method for grading the cognitive state of patients for the clinician. J. Psychiatr. Res. 12, 189–198. doi: 10.1016/0022-3956(75)90026-6
Gamaldo, A. A., Ferrucci, L., Rifkind, J., Longo, D. L., and Zonderman, A. B. (2013). Relationship between mean corpuscular volume and cognitive performance in older adults. J. Am. Geriatr. Soc. 61, 84–89. doi: 10.1111/jgs.12066
Ganguli, M., Du, Y., Dodge, H. H., Ratcliff, G. G., and Chang, C. C. H. (2006). Depressive symptoms and cognitive decline in late life: a prospective epidemiological study. Arch. Gen. Psychiatry 63, 153–160. doi: 10.1001/archpsyc.63.2.153
Godin, O., Dufouil, C., Ritchie, K., Dartigues, J. F., Tzourio, C., Pérès, K., et al. (2007). Depressive symptoms, major depressive episode and cognition in the elderly: the three-city study. Neuroepidemiology 28, 101–108. doi: 10.1159/000101508
González-Aramburu, I., Sánchez-Juan, P., Sierra, M., Fernández-Juan, E., Sánchez-Quintana, C., Berciano, J., et al. (2014). Serum uric acid and risk of dementia in Parkinson’s disease. Parkinsonism Relat. Disord. 20, 637–639. doi: 10.1016/j.parkreldis.2014.02.023
Goodman, A. B. (2006). Retinoid receptors, transporters, and metabolizers as therapeutic targets in late onset Alzheimer disease. J. Cell. Physiol. 209, 598–603. doi: 10.1002/jcp.20784
Goodman, A. B., and Pardee, A. B. (2003). Evidence for defective retinoid transport and function in late onset Alzheimer’s disease. Proc. Natl. Acad. Sci. U.S.A. 100, 2901–2905. doi: 10.1073/pnas.0437937100
Goodwill, A. M., Campbell, S., Simpson, S., Bisignano, M., Chiang, C., Dennerstein, L., et al. (2018). Vitamin D status is associated with executive function a decade later: data from the Women’s Healthy Ageing Project. Maturitas 107, 56–62. doi: 10.1016/j.maturitas.2017.10.005
Goodwill, A. M., and Szoeke, C. (2017). A Systematic review and meta-analysis of the effect of low Vitamin D on cognition. J. Am. Geriatr. Soc. 65, 2161–2168. doi: 10.1111/jgs.15012
Gorelick, P. B. (2010). Role of inflammation in cognitive impairment: results of observational epidemiological studies and clinical trials. Ann. N. Y. Acad. Sci. 1207, 155–162. doi: 10.1111/j.1749-6632.2010.05726.x
Gorospe, E. C., and Dave, J. K. (2007). The risk of dementia with increased body mass index. Age Ageing 36, 23–29. doi: 10.1093/ageing/afl123
Granic, A., Hill, T. R., Kirkwood, T. B. L., Davies, K., Collerton, J., Martin-Ruiz, C., et al. (2015). Serum 25-hydroxyvitamin D and cognitive decline in the very old: the Newcastle 85+ study. Eur. J. Neurol. 22, 106–115. doi: 10.1111/ene.12539
Han, S. H., Jung, E. S., Sohn, J. H., Hong, H. J., Hong, H. S., Kim, J. W., et al. (2011). Human serum transthyretin levels correlate inversely with Alzheimer’s disease. J. Alzheimers Dis. 25, 77–84. doi: 10.3233/JAD-2011-102145
Huang, C. Q., Wang, Z. R., Li, Y. H., Xie, Y. Z., and Liu, Q. X. (2011). Cognitive function and risk for depression in old age: a meta-analysis of published literature. Int. Psychogeriatr. 23, 516–525. doi: 10.1017/S1041610210000049
Jorde, R., Mathiesen, E. B., Rogne, S., Wilsgaard, T., Kjærgaard, M., Grimnes, G., et al. (2015). Vitamin D and cognitive function: the tromsø study. J. Neurol. Sci. 355, 155–161. doi: 10.1016/j.jns.2015.06.009
Kaiser, M. J., Bauer, J. M., Ramsch, C., Uter, W., Guigoz, Y., Cederholm, T., et al. (2009). Validation of the Mini Nutritional Assessment short-form (MNA§-SF): a practical tool for identification of nutritional status. J. Nutr. Health Aging 13, 782–788. doi: 10.1007/s12603-009-0214-7
Kalueff, A. V., and Tuohimaa, P. (2007). Neurosteroid hormone Vitamin D and its utility in clinical nutrition. Curr. Opin. Clin. Nutr. Metab. Care 10, 12–19. doi: 10.1097/MCO.0b013e328010ca18
Kang, J. H., Irizarry, M. C., and Grodstein, F. (2006). Prospective study of plasma folate, Vitamin B12, and cognitive function and decline. Epidemiology 17, 650–657. doi: 10.1097/01.ede.0000239727.59575.da
Keller, U. (2019). Nutritional laboratory markers in malnutrition. J. Clin. Med. 8:775. doi: 10.3390/jcm8060775
Khan, A. A., Quinn, T. J., Hewitt, J., Fan, Y., and Dawson, J. (2016). Serum uric acid level and association with cognitive impairment and dementia: systematic review and meta-analysis. Age 38:16. doi: 10.1007/s11357-016-9871-8
Kim, T. S., Pae, C. U., Yoon, S. J., Jang, W. Y., Lee, N. J., Kim, J. J., et al. (2006). Decreased plasma antioxidants in patients with Alzheimer’s disease. Int. J. Geriatr. Psychiatry 21, 344–348. doi: 10.1002/gps.1469
Klímová, B., and Vališ, M. (2018). Nutritional interventions as beneficial strategies to delay cognitive decline in healthy older individuals. Nutrients 10:905. doi: 10.3390/nu10070905
Koch, M., Furtado, J. D., Cronjé, H. T., DeKosky, S. T., Fitzpatrick, A. L., Lopez, O. L., et al. (2021). Plasma antioxidants and risk of dementia in older adults. Alzheimers Dement. 7:e12208. doi: 10.1002/trc2.12208
Korol, D. L., and Gold, P. E. (1998). Glucose, memory, and aging. Am. J. Clin. Nutr. 67, 764S–771S. doi: 10.1093/ajcn/67.4.764S
Koyama, A., O’Brien, J., Weuve, J., Blacker, D., Metti, A. L., and Yaffe, K. (2013). The role of peripheral inflammatory markers in dementia and Alzheimer’s disease: a meta-analysis. J. Gerontol. Ser. A Biol. Sci. Med. Sci. 68, 433–440. doi: 10.1093/gerona/gls187
Kueider, A. M., Tanaka, T., An, Y., Kitner-Triolo, M. H., Palchamy, E., Ferrucci, L., et al. (2016). State and trait-dependent associations of Vitamin-D with brain function during aging. Neurobiol. Aging 39, 38–45. doi: 10.1016/j.neurobiolaging.2015.11.002.State
Lam, P. K., Kritz-Silverstein, D., Barrett-Connor, E., Milne, D., Nielsen, F., Gamst, A., et al. (2008). Plasma trace elements and cognitive function in older men and women: the Rancho Bernardo study. J. Nutr. Health Aging 12, 22–27. doi: 10.1007/BF02982160
Latourte, A., Soumaré, A., Bardin, T., Perez-Ruiz, F., Debette, S., and Richette, P. (2018). Uric acid and incident dementia over 12 years of follow-up: a population-based cohort study. Ann. Rheum. Dis. 77, 328–335. doi: 10.1136/annrheumdis-2016-210767
Lawton, M. P., and Brody, E. M. (1969). Assessment of older people: self-maintaining and instrumental activities of daily living. Gerontologist 9, 179–186. doi: 10.1093/geront/9.3_part_1.179
Lee, H. P., Casadesus, G., Zhu, X., Lee, H. G., Perry, G., Smith, M. A., et al. (2009). All-trans retinoic acid as a novel therapeutic strategy for Alzheimer’s disease. Expert Rev. Neurotherap. 9, 1615–1621. doi: 10.1586/ern.09.86
Lee, Y. B., Han, K., Park, S., Kim, S. M., Kim, N. H., Choi, K. M., et al. (2020). Gamma-glutamyl transferase variability and risk of dementia: a nationwide study. Int. J. Geriatr. Psychiatry 35, 1105–1114. doi: 10.1002/gps.5332
Licher, S., De Bruijn, R. F. A. G., Wolters, F. J., Zillikens, M. C., Ikram, M. A., and Ikram, M. K. (2017). Vitamin D and the risk of dementia: the rotterdam study. J. Alzheimers Dis. 60, 989–997. doi: 10.3233/JAD-170407
Lim, A., Krajina, K., and Marsland, A. L. (2013). Peripheral inflammation and cognitive aging. Inflamm. Psychiatry 28, 175–187. doi: 10.1159/000346362
Litvan, I., Goldman, J. G., Tröster, A. I., Schmand, B. A., Weintraub, D., Petersen, R. C., et al. (2012). Diagnostic criteria for mild cognitive impairment in Parkinson’s disease: movement disorder society task force guidelines. Mov. Disord. 27, 349–356. doi: 10.1002/mds.24893
Loef, M., Schrauzer, G. N., and Walach, H. (2011). Selenium and Alzheimer’s disease: a systematic review. J. Alzheimers Dis. 26, 81–104. doi: 10.3233/JAD-2011-110414
Luppino, F. S., De Wit, L. M., Bouvy, P. F., Stijnen, T., Cuijpers, P., Penninx, B. W. J. H., et al. (2010). Overweight, obesity, and depression: a systematic review and meta-analysis of longitudinal studies. Arch. Gen. Psychiatry 67, 220–229. doi: 10.1001/archgenpsychiatry.2010.2
Lv, Y. B., Yin, Z. X., Chei, C. L., Brasher, M. S., Zhang, J., Kraus, V. B., et al. (2016). Serum cholesterol levels within the high normal range are associated with better cognitive performance among Chinese elderly. J. Nutr. Health Aging 20, 280–287. doi: 10.1007/s12603-016-0701-6
Ma, C., Yin, Z., Zhu, P., Luo, J., Shi, X., and Gao, X. (2017). Blood cholesterol in late-life and cognitive decline: a longitudinal study of the Chinese elderly. Mol. Neurodegener. 12:24. doi: 10.1186/s13024-017-0167-y
Ma, Y., Ajnakina, O., Steptoe, A., and Cadar, D. (2020). Higher risk of dementia in English older individuals who are overweight or obese. Int. J. Epidemiol. 49, 1353–1365. doi: 10.1093/ije/dyaa099
Mahoney, F. I., and Barthel, D. (1965). Functional evaluation: the Barthel Index. Maryland State Med. J. 14, 56–61.
Malaspina, A., and Michael-Titus, A. T. (2008). Is the modulation of retinoid and retinoid-associated signaling a future therapeutic strategy in neurological trauma and neurodegeneration? J. Neurochem. 104, 584–595. doi: 10.1111/j.1471-4159.2007.05071.x
McAfoose, J., and Baune, B. T. (2009). Evidence for a cytokine model of cognitive function. Neurosci. Biobehav. Rev. 33, 355–366. doi: 10.1016/j.neubiorev.2008.10.005
McKhann, G. M., Knopman, D. S., Chertkow, H., Hyman, B. T., Jack, C. R., Kawas, C. H., et al. (2011). The diagnosis of dementia due to Alzheimer’s disease: recommendations from the National Institute on Aging-Alzheimer’s Association workgroups on diagnostic guidelines for Alzheimer’s disease. Alzheimers Dement. 7, 263–269. doi: 10.1016/j.jalz.2011.03.005
Mehdi, Y., Hornick, J. L., Istasse, L., and Dufrasne, I. (2013). Selenium in the environment, metabolism and involvement in body functions. Molecules 18, 3292–3311. doi: 10.3390/molecules18033292
Michaud, M., Balardy, L., Moulis, G., Gaudin, C., Peyrot, C., Vellas, B., et al. (2013). Proinflammatory cytokines, aging, and age-related diseases. J. Am. Med. Direct. Assoc. 14, 877–882. doi: 10.1016/j.jamda.2013.05.009
Michelakos, T., Kousoulis, A. A., Katsiardanis, K., Dessypris, N., Anastasiou, A., Katsiardani, K. P., et al. (2013). Serum folate and B12 levels in association with cognitive impairment among seniors: results from the VELESTINO study in greece and meta-analysis. J. Aging Health 25, 589–616. doi: 10.1177/0898264313482488
Miquel, S., Champ, C., Day, J., Aarts, E., Bahr, B. A., Bakker, M., et al. (2018). Poor cognitive ageing: vulnerabilities, mechanisms and the impact of nutritional interventions. Ageing Res. Rev. 42, 40–55. doi: 10.1016/j.arr.2017.12.004
Moore, E. M., Ames, D., Mander, A. G., Carne, R. P., Brodaty, H., Woodward, M. C., et al. (2014). Among vitamin B12 deficient older people, high folate levels are associated with worse cognitive function: combined data from three cohorts. J. Alzheimers Dis. 39, 661–668. doi: 10.3233/JAD-131265
Nettiksimmons, J., Ayonayon, H., Harris, T., Phillips, C., Rosano, C., Satterfield, S., et al. (2015). Development and validation of risk index for cognitive decline using blood-derived markers. Neurology 84, 696–702. doi: 10.1212/WNL.0000000000001263
Ng, T. P., Feng, L., Niti, M., and Yap, K. B. (2008). Albumin, haemoglobin, BMI and cognitive performance in older adults. Age Ageing 37, 423–429. doi: 10.1093/ageing/afn102
Nguyen, J. C. D., Killcross, A. S., and Jenkins, T. A. (2014). Obesity and cognitive decline: role of inflammation and vascular changes. Front. Neurosci. 8:375. doi: 10.3389/fnins.2014.00375
Nho, K., Kueider-Paisley, A., Ahmad, S., Mahmoudiandehkordi, S., Arnold, M., Risacher, S. L., et al. (2019). Association of altered liver enzymes with Alzheimer disease diagnosis, cognition, neuroimaging measures, and cerebrospinal fluid biomarkers. JAMA Netw. Open 2, 1–20. doi: 10.1001/jamanetworkopen.2019.7978
Noh, J. W., Kwon, Y. D., Park, J., and Kim, J. (2015). Body mass index and depressive symptoms in middle aged and older adults. BMC Public Health 15:310. doi: 10.1186/s12889-015-1663-z
Nuttall, J. R., and Oteiza, P. I. (2014). Zinc and the aging brain. Genes Nutr. 9:379. doi: 10.1007/s12263-013-0379-x
O’Bryant, S. E., Xiao, G., Barber, R., Reisch, J., Doody, R., Fairchild, T., et al. (2010). A serum protein-based algorithm for the detection of Alzheimer disease. Arch. Neurol. 67, 1077–1081. doi: 10.1001/archneurol.2010.215
O’Leary, F., Allman-Farinelli, M., and Samman, S. (2012). Vitamin B12 status, cognitive decline and dementia: a systematic review of prospective cohort studies. Br. J. Nutr. 108, 1948–1961. doi: 10.1017/S0007114512004175
Olson, C. R., and Mello, C. V. (2010). Significance of Vitamin A to brain function, behavior and learning. Mol. Nutr. Food Res. 54, 489–495. doi: 10.1002/mnfr.200900246
Onem, Y., Terekeci, H., Kucukardali, Y., Sahan, B., Solmazgül, E., Şenol, M. G., et al. (2010). Albumin, hemoglobin, body mass index, cognitive and functional performance in elderly persons living in nursing homes. Arch. Gerontol. Geriatr. 50, 56–59. doi: 10.1016/j.archger.2009.01.010
Ono, K., and Yamada, M. (2012). Vitamin A and Alzheimer’s disease. Geriatr. Gerontol. Int. 12, 180–188. doi: 10.1111/j.1447-0594.2011.00786.x
Ownby, R. L. (2010). Neuroinflammation and cognitive aging. Curr. Psychiatry Rep. 12, 39–45. doi: 10.1007/s11920-009-0082-1
Pellecchia, M. T., Savastano, R., Moccia, M., Picillo, M., Siano, P., Erro, R., et al. (2016). Lower serum uric acid is associated with mild cognitive impairment in early Parkinson’s disease: a 4-year follow-up study. J. Neural Trans. 123, 1399–1402. doi: 10.1007/s00702-016-1622-6
Petersen, M., Hall, J., Parsons, T., Johnson, L., and O’Bryant, S. (2020). Combining select blood-based biomarkers with neuropsychological assessment to detect mild cognitive impairment among Mexican Americans. J. Alzheimers Dis. 75, 739–750. doi: 10.3233/JAD-191264
Petersen, R. C. (2004). Mild cognitive impairment as a diagnostic entity. J. Intern. Med. 256, 183–194. doi: 10.1111/j.1365-2796.2004.01388.x
Power, R., Prado-Cabrero, A., Mulcahy, R., Howard, A., and Nolan, J. M. (2019). The role of nutrition for the aging population: implications for cognition and Alzheimer’s disease. Annu. Rev. Food Sci. Technol. 10, 619–639. doi: 10.1146/annurev-food-030216-030125
Prickett, C., Brennan, L., and Stolwyk, R. (2015). Examining the relationship between obesity and cognitive function: a systematic literature review. Obes. Res. Clin. Pract. 9, 93–113. doi: 10.1016/j.orcp.2014.05.001
Przybelski, R. J., and Binkley, N. C. (2007). Is vitamin D important for preserving cognition? A positive correlation of serum 25-hydroxyvitamin D concentration with cognitive function. Arch. Biochem. Biophys. 460, 202–205. doi: 10.1016/j.abb.2006.12.018
Rabensteiner, J., Hofer, E., Fauler, G., Fritz-Petrin, E., Benke, T., Dal-Bianco, P., et al. (2020). The impact of folate and vitamin B12 status on cognitive function and brain atrophy in healthy elderly and demented Austrians, a retrospective cohort study. Aging 12, 15478–15491. doi: 10.18632/aging.103714
Rahman, K. (2007). Studies on free radicals, antioxidants, and co-factors. Clin. Interv. Aging 2, 219–236.
Raszewski, G., Chwedorowicz, R., Chwedorowicz, A., and Rothenberg, K. G. (2016). Homocysteine, antioxidant vitamins and lipids as biomarkers of neurodegeneration in Alzheimer’s disease versus non-Alzheimer’s dementia. Ann. Agric. Environ. Med. 23, 193–196. doi: 10.5604/12321966.1196878
Ray, S., Britschgi, M., Herbert, C., Takeda-Uchimura, Y., Boxer, A., Blennow, K., et al. (2007). Classification and prediction of clinical Alzheimer’s diagnosis based on plasma signaling proteins. Nat. Med. 13, 1359–1362. doi: 10.1038/nm1653
Rey, A. (1941). L’examen psychologique dans les cas d’encéphalopathie traumatique. Arch. Psychol. 28, 286–340.
Rinaldi, P., Polidori, M. C., Metastasio, A., Mariani, E., Mattioli, P., Cherubini, A., et al. (2003). Plasma antioxidants are similarly depleted in mild cognitive impairment and in Alzheimer’s disease. Neurobiol. Aging 24, 915–919. doi: 10.1016/S0197-4580(03)00031-9
Robles, A., Del Ser, T., Alom, J., Peña-Casanova, J., Barquero, M. S., Bermejo, F., et al. (2002). Propuesta de criterios para el diagnóstico clínico del deterioro cognitivo ligero, la demencia y la enfermedad de Alzheimer. Neurologia 17, 17–32.
Rosenberg, I. H. (2008). Effects of folate and vitamin B12 on cognitive function in adults and the elderly. Food Nutr. Bull. 29(2 Suppl), S132–S142. doi: 10.1177/15648265080292s118
Rubenstein, L. Z., Harker, J. O., Salvà, A., Guigoz, Y., and Vellas, B. (2001). Screening for undernutrition in geriatric practice: developing the Short-Form Mini-Nutritional Assessment (MNA-SF). J. Gerontol. Ser. A Biol. Sci. Med. Sci. 56, 366–372. doi: 10.1093/gerona/56.6.M366
Ruggiero, C., Cherubini, A., Lauretani, F., Bandinelli, S., Maggio, M., Di Iorio, A., et al. (2009). Uric acid and dementia in community-dwelling older persons. Dement. Geriatr. Cogn. Disord. 27, 382–389. doi: 10.1159/000210040
Sautin, Y. Y., and Johnson, R. J. (2008). Uric acid: the oxidant-antioxidant paradox. Nucl. Nucl. Nucleic Acids 27, 608–619. doi: 10.1080/15257770802138558
Schlesinger, I., and Schlesinger, N. (2008). Uric acid in Parkinson’s disease. Mov. Disord. 23, 1653–1657. doi: 10.1002/mds.22139
Schlögl, M., and Holick, M. F. (2014). Vitamin D and neurocognitive function. Clin. Interv. Aging 9, 559–568. doi: 10.2147/CIA.S51785
Schmeidler, J., Mastrogiacomo, C. N., Beeri, M. S., Rosendorff, C., and Silverman, J. M. (2019). Distinct age-related associations for body mass index and cognition in cognitively healthy very old veterans. Int. Psychogeriatr. 31, 895–899. doi: 10.1017/S1041610218001412
Schram, M. T., Trompet, S., Kamper, A. M., De Craen, A. J. M., Hofman, A., Euser, S. M., et al. (2007). Serum calcium and cognitive function in old age. J. Am. Geriatr. Soc. 55, 1786–1792. doi: 10.1111/j.1532-5415.2007.01418.x
Seamans, K. M., Hill, T. R., Scully, L., Meunier, N., Andrillo-Sanchez, M., Polito, A., et al. (2010). Vitamin D status and measures of cognitive function in healthy older European adults. Eur. J. Clin. Nutr. 64, 1172–1178. doi: 10.1038/ejcn.2010.117
Sedó, M. (2007). Test de los Cinco Dígitos. Available online at: http://web.teaediciones.com (accessed 2022).
Seetharaman, S., Andel, R., McEvoy, C., Aslan, A. K. D., Finkel, D., and Pedersen, N. L. (2015). Blood glucose, diet-based glycemic load and cognitive aging among dementia-free older adults. J. Gerontol. Ser. A Biol. Sci. Med. Sci. 70, 471–479. doi: 10.1093/gerona/glu135
Serdarevic, N., Stanciu, A. E., Begic, L., and Uncanin, S. (2020). Serum Uric Acid Concentration in Patients with Cerebrovascular Disease (Ischemic Stroke and Vascular Dementia). Med. Arch. (Sarajevo, Bosnia and Herzegovina) 74, 95–99. doi: 10.5455/medarh.2020.74.95-99
Sheikh, J. I., and Yesavage, J. A. (1986). Geriatric Depression Scale (GDS): recent evidence and development of a shorter version. Clin. Gerontol. 5, 165–173. doi: 10.1300/J018v05n01_09
Smith, S. H. (2017). Using albumin and prealbumin to assess nutritional status. Nursing 47, 65–66. doi: 10.1097/01.NURSE.0000511805.83334.df
Smorgon, C., Mari, E., Atti, A. R., Dalla Nora, E., Zamboni, P. F., Calzoni, F., et al. (2004). Trace elements and cognitive impairment: an elderly cohort study. Arch. Gerontol. Geriatr. 38, 393–402. doi: 10.1016/j.archger.2004.04.050
Sodhi, R. K., and Singh, N. (2013). All-trans retinoic acid rescues memory deficits and neuropathological changes in mouse model of streptozotocin-induced dementia of Alzheimer’s type. Prog. NeuroPsychopharmacol. Biol. Psychiatry 40, 38–46. doi: 10.1016/j.pnpbp.2012.09.012
Sommer, I., Griebler, U., Kien, C., Auer, S., Klerings, I., Hammer, R., et al. (2017). Vitamin D deficiency as a risk factor for dementia: a systematic review and meta-analysis. BMC Geriatr. 17:16. doi: 10.1186/s12877-016-0405-0
Stolk, R. P., Breteler, M., Ott, A., Pols, H., Lamberts, S., Grobbee, D. E., et al. (1997). Insulin and cognitive function elderly population. Diabetes Care 20, 792–795. doi: 10.2337/diacare.20.5.792
Sultan, S., Taimuri, U., Basnan, S. A., Ai-Orabi, W. K., Awadallah, A., Almowald, F., et al. (2020). Low Vitamin D and its association with cognitive impairment and dementia. J. Aging Res. 2020, 6097820. doi: 10.1155/2020/6097820
Tana, C., Ticinesi, A., Prati, B., Nouvenne, A., and Meschi, T. (2018). Uric acid and cognitive function in older individuals. Nutrients 10:975. doi: 10.3390/nu10080975
Tang, Z., Chen, X., Zhang, W., Sun, X., Hou, Q., Li, Y., et al. (2021). Association Between gamma-glutamyl transferase and mild cognitive impairment in Chinese women. Front. Aging Neurosci. 13:630409. doi: 10.3389/fnagi.2021.630409
Tien, Y. T., Lee, W. J., Liao, Y. C., Wang, W. F., Jhang, K. M., Wang, S. J., et al. (2019). Plasma transthyretin as a predictor of amnestic mild cognitive impairment conversion to dementia. Sci. Rep. 9:18691. doi: 10.1038/s41598-019-55318-0
Toffanello, E. D., Coin, A., Perissinotto, E., Zambon, S., Sarti, S., Veronese, N., et al. (2014). Vitamin D deficiency predicts cognitive decline in older men and women: the Pro.V.A. Study. Neurology 83, 2292–2298. doi: 10.1212/WNL.0000000000001080
Tuven, B., Soysal, P., Unutmaz, G., Kaya, D., and Isik, A. T. (2017). Uric acid may be protective against cognitive impairment in older adults, but only in those without cardiovascular risk factors. Exp. Gerontol. 89, 15–19. doi: 10.1016/j.exger.2017.01.002
Umur, E. E., Oktenli, C., Celik, S., Tangi, F., Sayan, O., Sanisoglu, Y. S., et al. (2011). Increased iron and oxidative stress are separately related to cognitive decline in elderly. Geriatr. Gerontol. Int. 11, 504–509. doi: 10.1111/j.1447-0594.2011.00694.x
Valls-Pedret, C., Lamuela-Raventós, R. M., Medina-Remón, A., Quintana, M., Corella, D., Pintó, X., et al. (2012). Polyphenol-rich foods in the mediterranean diet are associated with better cognitive function in elderly subjects at high cardiovascular risk. J. Alzheimers Dis. 29, 773–782. doi: 10.3233/JAD-2012-111799
Van Den Kommer, T. N., Comijs, H. C., Aartsen, M. J., Huisman, M., Deeg, D. J. H., and Beekman, A. T. F. (2013). Depression and cognition: how do they interrelate in old age? Am. J. Geriatr. Psychiatry 21, 398–410. doi: 10.1016/j.jagp.2012.12.015
Van der Schaft, J., Koek, H. L., Dijkstra, E., Verhaar, H. J. J., van der Schouw, Y. T., and Emmelot-Vonk, M. H. (2013). The association between vitamin D and cognition: a systematic review. Ageing Res. Rev. 12, 1013–1023. doi: 10.1016/j.arr.2013.05.004
Van Exel, E., De Craen, A. J. M., Gussekloo, J., Houx, P., Bootsma-Van Der Wiel, A., Macfarlane, P. W., et al. (2002). Association between high-density lipoprotein and cognitive impairment in the oldest old. Ann. Neurol. 51, 716–721. doi: 10.1002/ana.10220
Van Vliet, P. (2012). Cholesterol and late-life cognitive decline. J. Alzheimers Dis. 30(Suppl 2), S147–S162. doi: 10.3233/JAD-2011-111028
Velayudhan, L., Killick, R., Hye, A., Kinsey, A., Güntert, A., Lynham, S., et al. (2012). Plasma transthyretin as a candidate marker for Alzheimer’s disease. J. Alzheimers Dis. 28, 369–375. doi: 10.3233/JAD-2011-110611
Wang, L., Wang, F., Liu, J., Zhang, Q., and Lei, P. (2018). Inverse relationship between baseline serum albumin levels and risk of mild cognitive impairment in elderly: a seven-year retrospective cohort study. Tohoku J. Exp. Med. 246, 51–57. doi: 10.1620/tjem.246.51
Wen, M., Zhou, B., Chen, Y.-H., Ma, Z.-L., Gou, Y., Zhang, C.-L., et al. (2017). Serum uric acid levels in patients with Parkinson’s disease: a meta-analysis. PLoS One 12:e0173731. doi: 10.1371/journal.pone.0173731
Wilson, V. K., Houston, D. K., Kilpatrick, L., Lovato, J., Yaffe, K., Cauley, J. A., et al. (2014). Relationship between 25-hydroxyvitamin D and cognitive function in older adults: the health, aging and body composition study. J. Am. Geriatr. Soc. 62, 636–641. doi: 10.1111/jgs.12765
Winblad, B., Palmer, K., Kivipelto, M., Jelic, V., Fratiglioni, L., Wahlund, L. O., et al. (2004). Mild cognitive impairment - Beyond controversies, towards a consensus: report of the International Working Group on Mild Cognitive Impairment. J. Intern. Med. 256, 240–246. doi: 10.1111/j.1365-2796.2004.01380.x
Xu, Y., Wang, Q., Cui, R., Lu, K., Liu, Y., and Zhao, Y. (2017). Uric acid is associated with vascular dementia in Chinese population. Brain Behav. 7:e00617. doi: 10.1002/brb3.617
Xue, L. L., Liu, Y. B., Xue, H. P., Xue, J., Sun, K. X., Wu, L. F., et al. (2017). Low uric acid is a risk factor in mild cognitive impairment. Neuropsychiatr. Dis. Treat. 13, 2363–2367. doi: 10.2147/NDT.S145812
Yavuz, B. B., Cankurtaran, M., Haznedaroglu, I. C., Halil, M., Ulger, Z., Altun, B., et al. (2012). Iron deficiency can cause cognitive impairment in geriatric patients. J. Nutr. Health Aging 16, 220–224. doi: 10.1007/s12603-011-0351-7
Zhang, T., Yan, R., Chen, Q., Ying, X., Zhai, Y., Li, F., et al. (2018). Body mass index, waist-to-hip ratio and cognitive function among Chinese elderly: a cross-sectional study. BMJ Open 8:22055. doi: 10.1136/bmjopen-2018-022055
Keywords: cognitive status, mild cognitive impairment (MCI), dementia, nutritional status, nutritional markers, blood biomarkers, nursing homes, aging
Citation: Leirós M, Amenedo E, Rodríguez M, Pazo-Álvare P, Franco L, Leis R, Martínez-Olmos M-Á, Arce C and the Rest of NUTRIAGE Study Researchers (2022) Cognitive Status and Nutritional Markers in a Sample of Institutionalized Elderly People. Front. Aging Neurosci. 14:880405. doi: 10.3389/fnagi.2022.880405
Received: 21 February 2022; Accepted: 19 April 2022;
Published: 24 May 2022.
Edited by:
Parnetti Lucilla, University of Perugia, ItalyReviewed by:
Hudson Sousa Buck, University of São Paulo, BrazilHeather M. Wilkins, University of Kansas Medical Center Research Institute, United States
Copyright © 2022 Leirós, Amenedo, Rodríguez, Pazo-Álvare, Franco, Leis, Martínez-Olmos, Arce and the Rest of NUTRIAGE Study Researchers. This is an open-access article distributed under the terms of the Creative Commons Attribution License (CC BY). The use, distribution or reproduction in other forums is permitted, provided the original author(s) and the copyright owner(s) are credited and that the original publication in this journal is cited, in accordance with accepted academic practice. No use, distribution or reproduction is permitted which does not comply with these terms.
*Correspondence: María Leirós, maria.leiros.lorenzo@usc.es