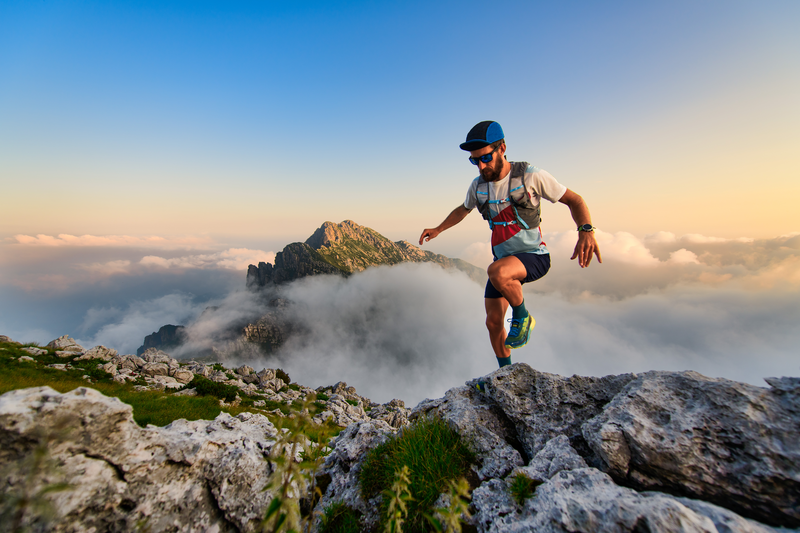
94% of researchers rate our articles as excellent or good
Learn more about the work of our research integrity team to safeguard the quality of each article we publish.
Find out more
ORIGINAL RESEARCH article
Front. Aging Neurosci. , 09 May 2022
Sec. Neuroinflammation and Neuropathy
Volume 14 - 2022 | https://doi.org/10.3389/fnagi.2022.847561
This article is part of the Research Topic Role of Pyroptosis in Neurological Disorders and Its Therapeutic Approaches View all 7 articles
Emerging data indicate that antipsychotic treatment causes brain volume loss and astrocyte death, but the mechanisms remain elusive. Pyroptosis, inflammatory cell death characterized by the formation of inflammatory bodies, increased expression of nod-like receptor proteins (NLRPs) such as NLRP3, and activation of caspases and gasdermin D (GSDMD) are largely associated with innate immunity, inflammation, and cell injury/death. However, the main effect of antipsychotics on astrocyte pyroptotic signaling and the molecular mechanisms remain obscure. In the present study, 72-h treatment with olanzapine, quetiapine, risperidone, or haloperidol significantly decreased the viability of astrocytes. Twenty-four hour treatment with olanzapine, quetiapine, risperidone, or haloperidol dose-dependently increased the protein expression of astrocytic NLRP3, NLRP6, caspase-1, caspase-4, and GSDMD. Co-treatment with a histamine H1 receptor agonist, 2-(3-trifluoromethylphenyl) histamine (FMPH), dose-dependently reduced the increased expression of NLRP3, caspase-1 and GSDMD induced by olanzapine, quetiapine, risperidone, or haloperidol. Moreover, olanzapine, quetiapine, risperidone, or haloperidol treatment induced pore formation in the membranes of astrocytes, and these effects were inhibited by FMPH co-treatment. Taken together, antipsychotic treatment activated astrocyte pyroptotic signaling, and these effects may be related to antipsychotic-induced astrocyte death. H1 receptor activation is an effective treatment strategy to suppress antipsychotic-induced astrocyte pyroptosis and inflammation.
Antipsychotics, in particular olanzapine, risperidone, quetiapine, and haloperidol, are widely used in the treatment of psychotic disorders. However, numerous clinical studies have revealed that patients who receive olanzapine, quetiapine, risperidone, or haloperidol treatment present a significant reduction in brain size, cortical thinning, and a decrease in cortical volume in the frontal and parietal regions (Miller et al., 2001; Molina et al., 2007; Moncrieff and Leo, 2010; Ebdrup et al., 2011; Ho et al., 2011). Consistently with the clinical findings, animal studies have revealed that chronic olanzapine or haloperidol treatment significantly decreases whole-brain volume, particularly in the cortex (Konopaske et al., 2008; Vernon et al., 2011, 2012). Although much effort has been made to determine the mechanisms by which antipsychotics induce cell death (Vucicevic et al., 2014; Boz et al., 2020), the molecular pathways remain elusive.
Astrocytes are the most abundant cell type in the central nervous system (CNS) and have attracted increasing attention in the regulation of neuronal development and function, metabolism, brain injury, and inflammation (Horvath et al., 2010). Chronic olanzapine or haloperidol treatment induced marked astrocyte loss in the monkey parietal cortex (Konopaske et al., 2008). Haloperidol treatment decreased astrocytic markers including glial fibrillary acidic protein (GFAP) and connexin 43 (CX43) in the rat prefrontal cortex (Fatemi et al., 2008). Olanzapine treatment for 3 days induced cell death in cultured astrocytes (He et al., 2020). However, the mechanisms by which antipsychotics induce astrocyte death are unclear.
Pyroptosis has received increased attention due to its involvement in the pathogenesis of various severe diseases such as Alzheimer’s disease, Parkinson’s disease, atherosclerosis, cancer, osteoarthritis, and stroke (An et al., 2020; Cai et al., 2021; Li et al., 2021b; Liu et al., 2021; Wang et al., 2021; Romero et al., 2022). Pyroptosis is a proinflammatory programmed cell death, characterized by the formation of inflammatory bodies, the activation of caspases, the formation of membrane pores, and the release of proinflammatory factors such as interleukin 1β (IL-1β) (Yang et al., 2015; Wu et al., 2019; Ma et al., 2021). Canonical and non-canonical inflammasome activation pathways contribute to pyroptosis (Ma et al., 2021). In the canonical pathway, the inflammasome sensors, nod-like receptor proteins (NLRPs) such as NLRP3 and NLRP6, form inflammasome complexes, leading to activation of caspase-1 (McIlwain et al., 2013). Activated caspase-1 cleaves the inhibitory C-terminal domain of gasdermin D (GSDMD), thus helping to liberate the N-terminal domain of GSDMD. N-GSDMD accumulates inside the cell membrane to form non-selective holes, resulting in cell pyroptosis (Yang et al., 2020). Caspase-1 could also promote the maturation and release of proinflammatory factors including IL-1β, IL-6 and IL-18, inducing an inflammatory response and pyroptosis (Ghimire et al., 2020; Zhang et al., 2020; Yu et al., 2021). In addition to NLRPs, NLR family caspase recruitment domain containing 4 (NLRC4) protein could form the NLRC4 inflammasome to activate caspase-1, resulting in pyroptosis (Franchi et al., 2009; McClellan et al., 2017). In the non-canonical pyroptosis pathway, the upstream sensory complexes of human caspase-4 are absent, and caspase-4 could bind directly to cellular lipopolysaccharide (LPS), which activates caspase-4 (Yu et al., 2021). Activated caspase-4 cleaves GSDMD into N-GSDMD in a manner similar to caspase-1, leading to pore formation and pyroptosis. Moreover, caspase-4 could induce the maturation and secretion of IL-1β via NLRP3/caspase-1 signaling, thereby inducing pyroptosis (Yu et al., 2021).
In pyroptotic signaling, NLRP3 is the most widely studied NLRP and has been reported to be related to various inflammatory diseases (Yang et al., 2021; Yu et al., 2021). Activation of NLRP3 induces the release of IL-1β in the CNS (Cai et al., 2021; Tastan et al., 2021), while inhibition of NLRP3 stops IL-1β production, reduces pyroptosis in the CNS, and improves cognitive function (Cai et al., 2021; Tastan et al., 2021). Moreover, the NLRP3 inflammasome is expressed by astrocytes and is involved in astrocyte dysfunction and the inflammatory response (Johann et al., 2015; Zhu and Tang, 2020). In primary cultured astrocytes, activation of the NLRP3 inflammasome via LPS plus adenosine triphosphate markedly increased the expression of caspase-1 and IL-1β (Zhu et al., 2018). Our previous study has reported that in both cultured astrocytes and the rat prefrontal cortex, olanzapine increased the expression of IL-1β (Li et al., 2021c). Haloperidol increased IL-β in the rat brain (Sonego et al., 2021; Mezzomo et al., 2022). These findings suggest that antipsychotics such as olanzapine and haloperidol might significantly affect the NLRP3/caspase-1 signaling, leading to IL-1β production and astrocyte death. To understand the mechanisms by which antipsychotics cause cell death and brain volume loss, we examined how different antipsychotics, including olanzapine, quetiapine, risperidone and haloperidol affect cell growth; NLRP3/caspase-1 signaling; and NLRP6, NLRC4 and caspase-4 expression in cultured astrocytes.
Moreover, it has been reported that histamine inhibited the secretion of IL-1β, a phenomenon completely abolished by co-treatment with a histamine H1 receptor antagonist, cetirizine, in primary cultured astrocytes (Xu et al., 2018). These findings suggest that astrocyte inflammation may be largely related to H1 receptor antagonism (Xu et al., 2018). However, whether the H1 receptor is related to antipsychotic-induced changes in NLRP3/caspase-1 signaling is unclear. We previously reported that olanzapine treatment induced H1 receptor signaling dysfunction in the rat brain, and this effect was inhibited by using a selective H1 receptor agonist, namely 2-(3-trifluoromethylphenyl) histamine (FMPH) (He et al., 2014; Chen et al., 2020b). Therefore, we investigated whether activation of the H1 receptor via FMPH could inhibit antipsychotic-induced changes in NLRP3/caspase-1 signaling. This study should help to further understand antipsychotic-induced side effects including central inflammation, astrocyte death, and brain volume loss during antipsychotic treatment, and it should provide valuable information to develop effective treatment strategies and novel antipsychotics with fewer side effects.
A human astrocyte cell line (C1028) was purchased from Shanghai Honsun Biological Technology Co., Ltd. (Shanghai, China). These astrocytes originated from the cortex of the human brain, and therefore should reflect the function of cortical astrocytes (He et al., 2020). Astrocytes were cultured in Dulbecco’s Modified Eagle Medium (DMEM) (Thermo Fisher Scientific, Wuhan, China) with 10% fetal bovine serum and 1% penicillin/streptomycin (Gibco Life Technologies, United States, A3160801 and 15140-122). The cells were cultured normally at 37°C with 5% CO2 and saturated humidity. Antipsychotics including olanzapine (Sigma-Aldrich, St. Louis, MO, United States, PHR1825), quetiapine (Sigma-Aldrich, Q3638), risperidone (Sigma-Aldrich, R3030), and haloperidol (Sigma-Aldrich, H1512) were obtained commercially.
To test the effects of olanzapine, quetiapine, risperidone, and haloperidol on the viability of astrocytes, 5 × 103 cells/well were seeded on 96-well plates. The cells were divided into five groups, which were treated with vehicle or antipsychotic drugs at 0.01, 0.1, 1, or 10 μM. After 24-, 48-, or 72-h treatment, a cell counting kit 8 (cck8) (DOJINDO, Beijing, China, CK04) was used to determine cell viability. Antipsychotics including olanzapine, quetiapine, risperidone, and haloperidol were dissolved in dimethyl sulfoxide (DMSO) (Sigma-Aldrich, St. Louis, MO, United States, D2650) to prepare 10 mM stock solutions. They were subsequently diluted with DMEM to 0.01, 0.1, 1, or 10 μM before use. Control cells were treated with DMEM + DMSO (vehicle).
To determine the dose-dependent effects of olanzapine, risperidone, quetiapine, and haloperidol on the protein expression of NLRP3, NLRP6, caspase-1, caspase-4, GSDMD, and NLRC4 in cultured astrocytes, cells were seeded on a six-well plate at a density of 9 × 105 cells/well. The cells were treated with 0, 0.1, 1, or 10 μM olanzapine, risperidone, quetiapine, or haloperidol. After treatment for 24 h, the cell lysates were collected and stored at –80°C for western blot analysis.
To investigate whether H1 receptor activation could inhibit pyroptosis NLRP3/caspase-1 signaling induced by antipsychotics, cultured astrocytes were co-treated with an antipsychotic and a selective H1 receptor agonist, FMPH (Sigma-Aldrich, T4951). In brief, astrocytes were seeded on six-well plates at a density of 9 × 105 cells/well. The cells were divided into five groups: group 1, control (Con); group 2, antipsychotic drug (10 μM); group 3, antipsychotic drug + FMPH high dose (10 μM) (antipsychotic + FMPH H); group 4, antipsychotic drug + FMPH low dose (5 μM) (antipsychotic + FMPH L); group 5: control + FMPH high dose (10 μM) (FMPH H). The FMPH doses were based on our previous study (Chen et al., 2020b). FMPH was dissolved in water to prepare a 10 mM stock solution, which was then diluted with DMEM to 10 and 5 μM. Cells were treated with FMPH for 2 h, and then were treated with the appropriate combination of FMPH and antipsychotic for 24 h. The control group was treated with DMEM + water. This treatment protocol was based on previous studies (Zhang et al., 2017; Chen et al., 2020a). Pretreatment with FMPH for 2 h allows FMPH to bind to the H1 receptor and exert physiological functions prior to olanzapine exposure. The treatment groups were determined based on previous studies (He et al., 2014) and the results of the pre-tests. Specifically, we examined the dose-dependent effects of FMPH on cell viability and NLRP3 protein expression in astrocytes without olanzapine treatment (Supplementary Figures 1, 2). Treatment with either FMPH high dose or FMPH low dose did not affect cell survival and NLRP3 expression. Therefore, we set up a control + FMPH high-dose group to investigate the effect of FMPH on NLRP3 signaling, but we did not set up a control + FMPH low-dose group. After the treatment, the cell lysates were collected and stored at –80°C.
The effects of antipsychotics and FMPH treatment on cell morphological changes were investigated based on previous studies (Chen et al., 2016; Zheng et al., 2021). In brief, 1 × 105 cells/well were seeded on glass coverslips placed in six-well plates. The cells were treated with vehicle, antipsychotic drug (10 μM), antipsychotic drug + FMPH high dose (10 μM), or FMPH high dose (10 μM) for 24 h. A scanning electron microscope (TESCAN, Czech, VEGA 3 LMU) was used to confirm the morphological changes of cells treated with vehicle, each antipsychotic drug, or each antipsychotic drug + FMPH high dose (Figure 10). Cells were fixed with 2.5% glutaraldehyde and rinsed with phosphate-buffered saline three times. Then, the cells were dehydrated through a graded ethanol series (50, 70, 80, 90, and 100%) and dried by an automated dryer (Leica, German, EM CPD300). The dried specimens were coated with gold-palladium by using an ion sputter coater (QUORUM, United Kingdom, Q150RS). The images were taken by using the scanning electron microscope.
Western blot analysis was performed according to our previous study (He et al., 2020). Radioimmunoprecipitation assay (RIPA) cell lysis buffer was used to homogenize the cells. A BCA protein quantification kit (Absin, Wuhan, China, abs9232) was used to determine the protein concentration of each sample. The protein samples were loaded onto 8% gels (Beyotime, Wuhan, China, P0452S). The electrophoresis conditions were 80 V for 30 min, and then 120 V for 60 min. Then, the proteins were transferred to polyvinylidene difluoride (PVDF) membranes (Millipore, Ireland, IPVH00010). The PVDF membranes were blocked with 5% non-fat milk in tris-buffered saline with tween-20 (TBST) for 1.5 h and then incubated with the primary antibodies at 4°C overnight. The primary antibodies used were: anti-GSDMD (Affinity, AF4012), anti-caspase-4 (Affinity, DF7609), anti-NLRC4 (ABclonal, Wuhan, China, A7382), anti-NLRP6 (ABclonal, A15628), anti-NLRP3 (Cell Signaling Technology, Danvers, MA, United States, D4D8T), anti-caspase-1 (Huabio, Boston, United States, ET1608-69), anti-IL-1β (Affinity, Jiangsu, China, AF5103) and anti-β-actin (Affinity, AF7018). The membranes were washed in TBST and then incubated with goat anti-mouse (1:8,000, Affinity, S0002) or goat anti-rabbit (Proteintech, Wuhan, China, SA00001-2) secondary antibody for 1.5 h at room temperature. The bands were detected with a super-sensitive ECL chemiluminescence kit (Biosharp, Wuhan, China, BL520B).
The data were analyzed with SPSS 22.0 (IBM Corp., Armonk, NY, United States). One-way analysis of variance (ANOVA) followed by Dunnett’s t test was used to analyze the difference in cell viability and the protein expression of caspase-1, caspase-4, NLRC4, NLRP3, NLRP6, GSDMD, and IL-1β. All data are presented as the mean ± standard error of the mean. Statistical significance was defined as p < 0.05.
To evaluate the effects of different antipsychotics—olanzapine, quetiapine, risperidone and haloperidol—on the viability of astrocytes, the cells were incubated with 0, 0.01, 0.1, 1, or 10 μM of each antipsychotic or vehicle for 24, 48, or 72 h. As shown in Figure 1A, after 24-h treatment, olanzapine 1 μM but not 0.01, 0.1, or 10 μM significantly increased cell viability (by 31.5 ± 10.5%, p = 0.014). After 48-h treatment, olanzapine 0.1 and 1 μM slightly increased cell viability (by 17.4 ± 8.0%, p = 0.1, and 11.9 ± 7.8%, p = 0.37, respectively). Olanzapine 0.01 or 10 μM did not affect cell viability. After 72-h treatment, olanzapine 10 μM significantly reduced cell viability (by 17.6 ± 3.7%, p = 0.001).
Figure 1. The effects of 0.01, 0.1, 1, and 10 μM olanzapine (A), quetiapine (B), risperidone (C), and haloperidol (D) treatment for 24, 48, and 72 h on the viability of cultured astrocytes. The data are presented as the mean ± standard error of the mean. *p < 0.05, **p < 0.01 vs. control (one-way analysis of variance and post hoc Dunnett’s multiple comparison test; n = 6–12 cultures/group). Con, control; OLZ, olanzapine; QUE, quetiapine; RIS, risperidone; HAL, haloperidol.
The effects of quetiapine on cell viability are shown in Figure 1B. Similarly to olanzapine, 24-h treatment with quetiapine 0.1 μM increased cell viability (by 21.7 ± 5.4%, p = 0.001). As treatment was prolonged, quetiapine 0.01 μM significantly increased cell viability (by 21.3 ± 5.2%, p = 0.001). However, quetiapine 0.1–10 μM did not alter cell viability. After 72-h treatment, quetiapine 0.01–10 μM treatment slightly but significantly decreased cell viability (0.01 μM, by 10.4 ± 7.9%, p = 0.01; 0.1 μM, by 7.1 ± 1.7%, p = 0.035; 1 μM, by 8.1 ± 1.6%, p = 0.035; 10 μM, by 8.2 ± 2.5%, p = 0.049, respectively).
The effects of risperidone on the viability of astrocytes are shown in Figure 1C. After 24-h treatment, risperidone 0.01–10 μM did not affect cell viability. After 48 h, risperidone 0.01–1 μM evidentially increased cell viability (0.01 μM, by 19.1 ± 4.9%, p = 0.000; 0.1 μM, by 17.7 ± 5.0%, p = 0.001; 1 μM, by 27.4 ± 5.0%, p = 0.001). After 72 h, risperidone 0.1–10 μM slightly decreased cell viability (0.1 μM, by 9.5 ± 2.7%, p = 0.004; 1 μM, by 7.1 ± 2.6%, p = 0.031; 10 μM, by 7.5 ± 2.6%, p = 0.019).
The effects of haloperidol on the viability of astrocytes are shown in Figure 1D. In contrast to olanzapine, quetiapine, risperidone, and haloperidol treatment for 24 did not alter cell viability. After 48 h, haloperidol 10 μM decreased cell viability (by 20.3 ± 4.7%, p = 0.004). Moreover, after 72-h treatment, haloperidol 0.01–10 μM reduced cell viability (0.01 μM, by 7.2 ± 3.5%, p = 0.015; 0.1 μM, by 8.8 ± 3.6%, p = 0.002; 1 μM, by 14.0 ± 3.5%, p = 0.002; 10 μM, by 34.0 ± 3.5%, p = 0.001). Overall, during short-term treatment, olanzapine, quetiapine, and risperidone, but not haloperidol, slightly increased astrocyte viability. However, as treatment was prolonged, all four antipsychotics induce cell death. Haloperidol had the largest effect of inducing astrocyte death.
Astrocytes were treated with olanzapine 0, 0.1, 1, or 10 μM for 24 h, and western blot analysis was used to examine the protein expression of NLRP3, caspase-1, GSDMD, NLRC4, NLRP6, caspase-4, and IL-1β. As shown in Figures 2A–C, olanzapine 0.1 μM significantly increased NLRP3 (by 67.7 ± 19.6%, p = 0.015), but it did not affect the expression of caspase-1, GSDMD, NLRC4, NLRP6, or caspase-4. Olanzapine 1 μM upregulated NLRP3 (by 150.9 ± 9.0%, p = 0.000), NLRC4 (by 194.5 ± 8.8%, p = 0.000), NLRP6 (by 74.5 ± 6.7%, p = 0.007), and caspase-4 (by 111.2 ± 14.4%, p = 0.005). Olanzapine 1 μM did not affect the expression of caspase-1 or GSDMD. Olanzapine 10 μM markedly increased NLRP3 (by 230.2 ± 11.8%, p = 0.000), caspase-1 (by 267.0 ± 47.2%, p = 0.001), and GSDMD 35 kDa (by 186.2 ± 52.4%, p = 0.006), but not GSDMD 53 kDa. Olanzapine 10 μM also increased NLRC4 (by 324.0 ± 19.9%, p = 0.000), NLRP6 (by 160.4 ± 20.6%, p = 0.000), and caspase-4 (by 239.1 ± 19.3%, p = 0.000). Moreover, olanzapine 0.1–10 μM markedly upregulated IL-1β (0.1 μM, by 116.0 ± 11.9%, p = 0.003; 1 μM, by 321.4 ± 20.0%, p = 0.000; 10 μM, 514.1 ± 19.2%, p = 0.000, respectively) (Figures 2D,E). Overall, olanzapine dose-dependently increased NLRP3, NLRP6, NLRC4, caspase-1, caspase-4, and GSDMD 35 kDa, changes that could activate the relevant signaling and be associated with olanzapine-induced inflammation.
Figure 2. Olanzapine treatment activated NLRP3/caspase-1 signaling, and increased NLRC4, NLRP6, and IL-1β protein expression in cultured astrocytes. Cultured cells were treated with 0.1, 1, or 10 μM olanzapine for 24 h. (A–E) Western blot was used to evaluate the expression of NLRP3, caspase-1 (20 kDa), GSDMD (53 and 35 kDa), NLRC4, NLRP6, and IL-1β. The data are presented as the mean ± standard error of the mean. *p < 0.05, **p < 0.01, ***p < 0.001 vs. control (one-way analysis of variance and post hoc Dunnett’s multiple comparison test; n = 3–4 cultures/western blot group). Con, control; OLZ, olanzapine.
As shown in Figures 3A–C, quetiapine 0.1 μM did not affect the protein expression of NLRP3, caspase-1, GSDMD, or NLRP6, but this treatment significantly increased the expression of NLRC4 (by 77.4 ± 21.9%, p = 0.029) and caspase-4 (by 109.5 ± 10.8%, p = 0.005). These data suggest that a relatively low dose of quetiapine could activate NLRC4 and caspase-4 signaling. When the concentration of quetiapine increased to 1 μM, besides increasing the expression of NLRC4 (by 155.1 ± 11.9%, p = 0.000) and caspase-4 (by 173.9 ± 12.9%, p = 0.000), quetiapine also increased the expression of GSDMD 35 kDa (by 93.2 ± 25.2%, p = 0.04) and NLRP6 (by 107.4 ± 14.5%, p = 0.002). After 24-h treatment with quetiapine 10 μM, there was significant upregulation of NLRP3, caspase-1, GSDMD 35 kDa, NLRC4, NLRP6, and caspase-4 (NLRP3, by 42.3 ± 3.0%, p = 0.02; caspase-1, by 228.3 ± 45.0%, p = 0.034; GSDMD 35 kDa, by 82.9 ± 24.7%, p = 0.000; NLRC4, by 230.3 ± 12.1%, p = 0.000; NLRP6, by 201.5 ± 17.6%, p = 0.000; caspase-4, by 313.7 ± 23.9%, p = 0.000). Furthermore, quetiapine 0.1–10 μM markedly upregulated IL-1β expression (0.1 μM, by 30.7 ± 1.5%, p = 0.05; 1 μM, by 154.6 ± 16.7%, p = 0.034; 10 μM, 222.2 ± 8.5%, p = 0.034, respectively) (Figures 3D,E). Taken together, a relatively low dose of quetiapine upregulates NLRC4 and caspase-4 but not NLRP3. As the concentration increases, both NLRP3 and NLRP6 signaling are also activated.
Figure 3. Quetiapine treatment activated NLRP3/caspase-1 signaling and upregulated NLRC4, NLRP6, and IL-1β protein expression in cultured astrocytes. Cells were treated with 0.1, 1, or 10 μM quetiapine for 24 h. (A–E) Western blot was used to examine the expression of NLRP3, caspase-1 (20 kDa), GSDMD (53 and 35 kDa), NLRC4, NLRP6, and IL-1β. The data are presented as the mean ± standard error of the mean. *p < 0.05, **p < 0.01, ***p < 0.001 vs. control (one-way analysis of variance and post hoc Dunnett’s multiple comparison test; n = 3–4 cultures/western blot group). Con, control; QUE, quetiapine.
The effects of 24-h risperidone treatment on NLRP3, capase-1, GSDMD, NLRC4, NLRP6, and caspase-4 protein expression are shown in Figures 4A–C. Low-dose risperidone (0.1 μM) upregulated NLRC4 (by 92.1 ± 16.9%, p = 0.05) and GSDMD 35 kDa (by 54.1 ± 9.9%, p = 0.05), but not NLRP3, caspase-1, NLRP6, or caspase-4 (p > 0.05). Treatment with risperidone 1 μM elevated the expression of NLRP3 (by 50.3 ± 9.8%, p = 0.024), caspase-1 (by 174.4 ± 13.2%, p = 0.011), NLRC4 (by 185.7 ± 9.5%, p = 0.000), NLRP6 (by 54.0 ± 2.1%, p = 0.001), caspase-4 (by 257.0 ± 18.3%, p = 0.000), and GSDMD 35 kDa (by 222.7 ± 76.2%, p = 0.034), but not GSDMD 53 kDa. Risperidone at a relatively higher dose (10 μM) notably increased NLRP3 (by 62.1 ± 15.4%, p = 0.007), caspase-1 (by 352.7 ± 53.8%, p = 0.000), GSDMD 35 kDa (by 493.1 ± 41.3%, p = 0.034), NLRC4 (by 357.4 ± 66.5%, p = 0.000), NLRP6 (by 95.3 ± 9.6%, p = 0.000), and caspase-4 (by 401.7 ± 24.5%, p = 0.000). Risperidone 0.1–10 μM upregulated IL-1β (0.1 μM, by 161.1 ± 16.2%, p = 0.05; 1 μM, by 401.7 ± 16.6%, p = 0.034; 10 μM, 438.4 ± 9.1%, p = 0.034, respectively) (Figures 4D,E).
Figure 4. Risperidone treatment activated NLRP3/caspase-1 signaling and increased NLRC4, NLRP6, and IL-1β protein expression in cultured astrocytes. Cells were treated with 0.1, 1, or 10 μM risperidone for 24 h. (A–E) Western blot was used to examine the expression of NLRP3, caspase-1 (20 kDa), GSDMD (53 and 35 kDa), NLRC4, NLRP6, and IL-1β. The data are presented as the mean ± standard error of the mean. *p < 0.05, **p < 0.01, ***p < 0.001 vs. control (one-way analysis of variance and post hoc Dunnett’s multiple comparison test; n = 3–4 cultures/western blot group). Con, control; RIS, risperidone.
Haloperidol 0.1 μM did not affect the expression of the investigated proteins (all p > 0.05) (Figures 5A–C). At 1 μM, there was significant upregulation of NLRP3 (by 93.3 ± 11.9%, p = 0.014), but not caspase-1 or GSDMD. Haloperidol 1 μM increased the expression of NLRC4 (by 148.8 ± 28.5%, p = 0.001), NLRP6 (by 142.8 ± 31.9%, p = 0.008), and caspase-4 (by 387.3 ± 155.3%, p = 0.012). Moreover, haloperidol 10 μM increased NLRP3 (by 182.3 ± 26.5%, p = 0.000), caspase-1 (by 254.3 ± 78.4%, p = 0.012), GSDMD 35 kDa (by 475.3 ± 102.8%, p = 0.001), NLRC4 (by 257.1 ± 15.7%, p = 0.000), NLRP6 (by 179.2 ± 27.0%, p = 0.002), and caspase-4 (by 617.2 ± 116.4%, p = 0.011). Haloperidol 0.1–10 μM markedly upregulated IL-1β (0.1 μM, by 265.7 ± 29.2%, p = 0.000; 1 μM, by 549.3 ± 10.8%, p = 0.000; 10 μM, 704.1 ± 13.3%, p = 0.000, respectively) (Figures 5D,E). Based on the results presented in sections 3.2–3.5, each of the tested antipsychotics—olanzapine, quetiapine, risperidone, and haloperidol—significantly increased the expression of proteins involved in pyroptotic signaling. These effects may be largely responsible for the astrocyte death induced by 72-h antipsychotic treatment.
Figure 5. Haloperidol treatment activated NLRP3/caspase-1 signaling and increased NLRC4, NLRP6, and IL-1β protein expression in cultured astrocytes. Cells were treated with 0.1, 1, or 10 μM haloperidol for 24 h. (A–E) Western blot was used to examine the expression of NLRP3, caspase-1 (20 kDa), GSDMD (53 and 35 kDa), NLRC4, NLRP6, and IL-1β. The data are presented as the mean ± standard error of the mean. *p < 0.05, **p < 0.01, ***p < 0.001 vs. control (one-way analysis of variance and post hoc Dunnett’s multiple comparison test; n = 3–4 cultures/western blot group). Con, control; HAL, haloperidol.
To determine whether the effects of antipsychotics on astrocytic NLRP3/caspase-1 signaling are related to the histamine H1 receptor, we examined whether the selective H1 receptor agonist FMPH could suppress antipsychotic-induced increased protein expression of NLRP3, caspase-1, GSDMD and IL-1β. Compared with vehicle, 24-h olanzapine treatment significantly upregulated NLRP3 (by 116.2 ± 12.4%, p = 0.000), caspase-1 (by 512.5 ± 3.6%, p = 0.000), GSDMD 35 kDa (by 419.9 ± 24.8%, p = 0.000), and IL-1β (by 157.1 ± 21.9%, p = 0.021) (Figures 6A–F). Co-treatment with FMPH high or low dose inhibited olanzapine-induced expression of NLRP3 (high dose, by 75.7 ± 1.6%, p = 0.000; low dose, by 55.0 ± 2.1%, p = 0.000), caspase-1 (high dose, by 269.8 ± 14.6%, p = 0.000; low dose, by 98.5 ± 12.6%, p = 0.026), GSDMD 35 kDa (high dose, by 239.3 ± 24.6%, p = 0.000; low dose, by 123.7 ± 37.8%, p = 0.026), and IL-1β (high dose, by122.9 ± 13.0%, p = 0.021; low dose, by 41.9 ± 14.5%, p = 0.083).
Figure 6. 2-(3-Trifluoromethylphenyl) histamine (FMPH) co-treatment inhibited olanzapine-induced NLRP3/caspase-1 signaling activation and reduced IL-1β protein expression in cultured astrocytes. (A–F) Cultured cells were treated with vehicle [dimethyl sulfoxide (DMSO) + water], olanzapine 10 μM, olanzapine 10 μM + FMPH 10 μM, olanzapine 10 μM + FMPH 5 μM, or FMPH 10 μM for 24 h. Western blot was used to examine the expression of NLRP3, caspase-1 (20 kDa), GSDMD (53 and 35 kDa), and IL-1β. The data are presented as the mean ± standard error of the mean. *p < 0.05, ***p < 0.001 vs. Con; $p < 0.05, p < 0.001 vs. OLZ (one-way analysis of variance and post hoc Dunnett’s multiple comparison test; n = 4 cultures/western blot group). Con, control; OLZ, olanzapine; OLZ + FMPH H, olanzapine + FMPH high dose; OLZ + FMPH L, olanzapine + FMPH low dose; FMPH H, FMPH high dose.
Compared with vehicle, 24-h quetiapine treatment significantly upregulated NLRP3 (by 103.6 ± 5.5%, p = 0.000), caspase-1 (by 402.4 ± 17.4%, p = 0.021), GSDMD 35 kDa (by 325.5 ± 60.7%, p = 0.000), and IL-1β (by 177.2 ± 30.8%, p = 0.000) (Figures 7A–F). Co-treatment with either FMPH high or low dose counteracted the quetiapine-induced increased expression of NLRP3 (high dose, by 58.7 ± 8.2%, p = 0.000; low dose, by 27.6 ± 11.0%, p = 0.064), caspase-1 (high dose, by 200.8 ± 11.1%, p = 0.021; low dose, by 85.3 ± 13.4%, p = 0.021), GSDMD 35 kDa (high dose, by 205.9 ± 33.3%, p = 0.004; low dose, by 98.8 ± 39.2%, p = 0.207), and IL-1β (high dose, by 123.6 ± 6.5%, p = 0.000; low dose, by 70.1 ± 11.3%, p = 0.015).
Figure 7. 2-(3-Trifluoromethylphenyl) histamine (FMPH) co-treatment reversed quetiapine induced activation of NLRP3/caspase-1 signaling and inhibited IL-1β protein expression in cultured astrocytes. (A–F) Cultured cells were treated with vehicle [dimethyl sulfoxide (DMSO) + water], quetiapine 10 μM, quetiapine10 μM + FMPH 10 μM, quetiapine 10 μM + FMPH 5 μM, or FMPH 10 μM for 24 h. Western blot was used to examine the expression of NLRP3, caspase-1 (20 kDa), GSDMD (53 and 35 kDa), and IL-1β. The data are presented as the mean ± standard error of the mean. *p < 0.05, ***p < 0.001 vs. Con; $p < 0.05, p < 0.01, p < 0.001 vs. QUE (one-way analysis of variance and post hoc Dunnett’s multiple comparison test; n = 4 cultures/western blot group). Con, control; QUE, quetiapine; QUE + FMPH H, quetiapine + FMPH high dose; QUE + FMPH L, quetiapine + FMPH low dose; FMPH H, FMPH high dose.
Compared with vehicle, 24-h risperidone treatment significantly upregulated NLRP3 (by 215.1 ± 10.6%, p = 0.000), caspase-1 (by 402.4 ± 17.4%, p = 0.021), GSDMD 35 kDa (by 281.6 ± 61.7%, p = 0.002), and IL-1β (by 259.3 ± 18.4%, p = 0.000) (Figures 8A–F). Co-treatment with either FMPH high or low dose ameliorated the risperidone-induced increased expression of NLRP3 (high dose, by 136.2 ± 4.6%, p = 0.000; low dose, by 63.3 ± 8.1%, p = 0.006), caspase-1 (high dose, by 200.8 ± 11.1%, p = 0.021; low dose, by 85.3 ± 13.4%, p = 0.021), GSDMD 35 kDa (high dose, by 198.0 ± 38.7%, p = 0.028; low dose, by 85.5 ± 47.2%, p = 0.513), and IL-1β (high dose, by 157.2 ± 6.0%, p = 0.000; low dose, by 57.1 ± 10.3%, p = 0.000).
Figure 8. 2-(3-Trifluoromethylphenyl) histamine (FMPH) inhibited risperidone-induced activation of NLRP3/caspase-1 signaling and inhibited IL-1β protein expression in cultured astrocytes. (A–F) Cultured cells were treated with vehicle [dimethyl sulfoxide (DMSO) + water], risperidone 10 μM, risperidone 10 μM + FMPH 10 μM, risperidone 10 μM + FMPH 5 μM, or FMPH 10 μM for 24 h. Western blot was used to investigate the expression of NLRP3, caspase-1 (20 kDa), GSDMD (53 and 35 kDa), and IL-1β. The data are presented as mean ± standard error of the mean. *p < 0.05, **p < 0.01, ***p < 0.001 vs. Con; $p < 0.05, p < 0.01, p < 0.001 vs. RIS (one-way analysis of variance and post hoc Dunnett’s multiple comparison test; n = 4 cultures/western blot group). Con, control; RIS, risperidone; RIS + FMPH H, risperidone + FMPH high dose; risperidone + FMPH L, risperidone + FMPH low dose; FMPH H, FMPH high dose.
Similarly to the other three antipsychotics, compared with vehicle, 24-h haloperidol treatment significantly upregulated NLRP3 (by 160.1 ± 11.5%, p = 0.000), caspase-1 (by 376.8 ± 8.5%, p = 0.000), GSDMD 35 kDa (by 417.1 ± 60.4%, p = 0.000) and IL-1β (by 135.8 ± 6.8%, p = 0.000) (Figures 9A–F). Co-treatment with either FMPH high or low dose counteracted the haloperidol-induced increased expression of NLRP3 (high dose, by 106.8 ± 7.1%, p = 0.001; low dose, by 59.6 ± 4.7%, p = 0.046), caspase-1 (high dose, by 252.6 ± 16.8%, p = 0.000; low dose, by 118.3 ± 16.4%, p = 0.000), GSDMD 35 kDa (high dose, by 260.0 ± 31.5%, p = 0.001; low dose, by 150.9 ± 52.4%, p = 0.061) and IL-1β (high dose, by 107.7 ± 8.3%, p = 0.001; low dose, by 92.3 ± 12.3%, p = 0.004).
Figure 9. Co-treatment with 2-(3-trifluoromethylphenyl) histamine (FMPH) suppressed haloperidol-induced activation of NLRP3/caspase-1 and inhibited IL-1β protein expression in cultured astrocytes. (A–F) Cultured cells were treated with vehicle [dimethyl sulfoxide (DMSO) + water], haloperidol 10 μM, haloperidol 10 μM + FMPH 10 μM, haloperidol 10 μM + FMPH 5 μM, or FMPH 10 μM for 24 h. Western blot was used to investigate the expression of NLRP3, caspase-1 (20 kDa), GSDMD (53 and 35 kDa), and IL-1β. The data are presented as mean ± standard error of the mean. ***p < 0.001 vs. Con; $p < 0.05, p < 0.01, p < 0.001 vs. HAL (one-way analysis of variance and post hoc Dunnett’s multiple comparison test; n = 4 cultures/western blot group). Con, control; HAL, haloperidol; HAL + FMPH H, haloperidol + FMPH high dose; haloperidol + FMPH L, haloperidol + FMPH low dose; FMPH H, FMPH high dose.
Astrocyte pyroptosis involves cell swelling, pore formation in the cell membrane and increased membrane permeabilization (Chen et al., 2016; Zheng et al., 2021). The present study found that FMPH high dose co-treatment significantly inhibited olanzapine-, quetiapine-, risperidone-, or haloperidol-induced increased expression of NLRP3, caspase-1, GSDMD and IL-1β. Therefore, the effects of antipsychotics and FMPH high dose treatment on astrocyte cell morphological changes were examined. As shown in Figure 10, compared with vehicle, FMPH treatment did not affect the cell morphology. Compared with vehicle, certain cells treated with 10 μM of olanzapine, quetiapine, risperidone, or haloperidol had numerous pits or pores of different sizes across the cell membranes. FMPH 10 μM significantly inhibited the pore formation in the membrane induced by treatment with olanzapine, quetiapine, risperidone, or haloperidol. These findings showed that astrocytes treated with olanzapine, quetiapine, risperidone, or haloperidol exhibited pyroptosis-like morphological changes, and these effects could be partly inhibited by FMPH treatment.
Figure 10. 2-(3-Trifluoromethylphenyl) histamine (FMPH) inhibited antipsychotic-induced pore formation in the cell membrane. SEM Images of astrocytes treated with vehicle, antipsychotic drug (olanzapine/quetiapine/risperidone/haloperidol), or antipsychotic drug + FMPH 10 μM were taken by a Tescan Vega-3 LMU emission scanning electron microscope operating at 20 kV. Red arrow indicates pores in the cell membrane. Con, control; OLZ, olanzapine; QUE, quetiapine; RIS, risperidone; HAL, haloperidol; FMPH,2-(3-Trifluoromethylphenyl) histamine.
Emerging data have shown that astrocytes are the primary homeostatic cells in the CNS and that they affect neuronal development and function, metabolism, neuronal injury, and inflammation. In the present study, we have found that second-generation antipsychotics, including olanzapine, quetiapine, and risperidone, increased the viability of astrocytes during short-term treatment (24–48 h), but decreased their viability during long-term treatment (72 h). Consistently with this study, we had previously found that short-term (24 h) olanzapine treatment increased the protein expression of astrocyte markers, including calcium binding protein B (S100B) and GFAP, in cultured astrocytes, while long-term (72 h) olanzapine treatment led to astrocyte death (He et al., 2020). These findings are consistent with animal studies, which have shown that short-term olanzapine treatment activated astrocytes in the rat brain (He et al., 2020) while chronic exposure to olanzapine reduced the number of astrocytes in the parietal cortex of monkeys (Konopaske et al., 2008). Furthermore, previous studies have reported that risperidone increased S100B secretion from C6 glioma cells after 1-h treatment (de Souza et al., 2013) and increased S100B expression of primary cultured astrocytes after 24-h treatment (Nardin et al., 2011). In mice, chronic quetiapine treatment mitigated astrocyte activation induced by cuprizone (Shao et al., 2015). In contrast to the second-generation antipsychotics, the first-generation antipsychotic drug haloperidol did not increase the viability of astrocytes during short-term (24 h) treatment, but decreased the viability as treatment was prolonged to 48 and 72 h. Consistently with this study, researchers have reported that in primary astrocytes, haloperidol (10 μM) did not change S100B secretion after 24-h treatment (Nardin et al., 2011). Chronic haloperidol treatment induced astrocyte loss in the monkey parietal cortex (Konopaske et al., 2008). Our findings suggest that chronic treatment with the antipsychotics olanzapine, quetiapine, risperidone, or haloperidol induces astrocyte death, and these effects may contribute at least partly to antipsychotic-induced brain volume loss and cortical thinning.
Currently, how antipsychotics induce astrocyte death remains elusive. Numerous studies have reported that antipsychotic treatment, in particular olanzapine and risperidone, is associated with increased expression of proinflammatory cytokines such as IL-1β and IL-6, suggesting the possible involvement of proinflammatory programmed cell death (Zhang et al., 2014; Cruz Jung et al., 2016; He et al., 2019; May et al., 2020). As has been reported, activation of NLRP3/caspase-1 and NLRC4 signaling facilitates IL-1β and IL-6 secretion and induces pyroptosis in astrocytes (Liu and Chan, 2014; Mao et al., 2018; Voet et al., 2019). An increase in IL-1β also promotes astrocyte death (van Kralingen et al., 2013). In the present study, treatment with olanzapine, quetiapine, risperidone, or haloperidol significantly increased the expression of NLRP3, NLRP6, NLRC4, caspase-1, caspase-4, GSDMD, and IL-1β in cultured astrocytes. Therefore, NLRP/caspase-1 and NLRC4 signaling in astrocytes may be partly responsible for the increased IL-1β expression and astrocyte death induced by antipsychotics. Moreover, each of the tested antipsychotics increased the protein expression of GSDMD 35 kDa but not GSDMD 53 kDa, which represent the cleaved and full-length forms of GSDMD, respectively. Activated GSDMD (35 kDa) could accumulate inside the cell membrane and facilitate the formation of membrane pores, inducing cell blebbing and pyroptosis. In our work, cell imaging revealed that antipsychotic treatment induced pore formation in the cell membrane. These findings suggest that antipsychotics may induce astrocyte pyroptosis, and this effect may be largely related to NLRP/caspase/GSDMD signaling. However, the exact mechanism by which antipsychotics induce pore formation is unknown. A recent study in astrocytes reported that activated GSDMD increased osmotic pressure (OP) and intracellular calcium, and these effects contributed to astrocyte swelling and membrane blebbing (Zheng et al., 2021). Additional studies that investigate the effects of antipsychotics on OP and calcium flux and whether these effects are related to GSDMD are warranted.
Antipsychotics could bind directly to a variety of neurotransmitter receptors to regulate physiological functions. Elucidating the receptors involved in antipsychotic-induced activation of pyroptotic signaling has important implications for developing strategies to inhibit antipsychotic-induced astrocyte death. The H1 receptor is expressed on astrocytes (Fukui et al., 1991; Karpati et al., 2019), and olanzapine, quetiapine, and risperidone have affinity for the H1 receptor. In this study, the H1 receptor agonist FMPH markedly reduced olanzapine-, quetiapine-, and risperidone-induced increased expression of NLRP3, caspase-1, GSDMD and IL-1β. FMPH also inhibited olanzapine-, quetiapine-, and risperidone-induced cell membrane pore formation. Hence, activation of NLRP3/caspase-1 signaling and cell pyroptosis induced by second-generation antipsychotics may be partly related to their antagonistic effects on the H1 receptor. However, the mechanism by which the H1 receptor regulates NLRP3/caspase-1 signaling is unclear. A recent study reported that NLRP3 signaling and pyroptosis was inhibited by rapamycin (which binds to mammalian target of rapamycin [mTOR]) in macrophages (Yang et al., 2021). Previous studies have also suggested that the NLRP3 inflammasome is activated by potassium efflux and calcium influx (Li et al., 2021a). Therefore, future studies should investigate the role of the H1 receptor in regulating mTOR and calcium influx. Furthermore, it has been reported that activation of the hypothalamic H1 receptor, such as by using betahistine (an H1 receptor agonist/H3 receptor antagonist), reduced olanzapine-induced food intake and weight gain in rodents and patients (Deng et al., 2012; Poyurovsky et al., 2013; Barak et al., 2016; Smith et al., 2018). Besides counteracting obesity, perhaps activating the H1 receptor by using betahistine, or another H1 receptor agonist, could also inhibit NLRP3/caspase-1 signaling and thus pyroptosis and brain volume loss induced by chronic antipsychotic treatment. It is worth performing preclinical studies in rodents to investigate whether central activation of the H1 receptor using betahistine or FMPH could reverse antipsychotic-induced central inflammation and astrocyte loss.
It should be noted that we could not exclude that other receptors may be associated with antipsychotic-induced NLRP3/caspase-1 signaling activation. Haloperidol has low affinity for the H1 receptor, but it did activate NLRP3/caspase-1 signaling. In astrocytes, D2 receptor antagonism induced astrocyte inflammation (Shao et al., 2013), while D2 receptor activation suppressed astrocyte inflammation and restricted NLRP3 inflammasome and caspase-1 activation (Zhu et al., 2018). Olanzapine, quetiapine, risperidone, or haloperidol all have high affinity for the D2 receptor. These findings suggest that antipsychotic-induced activation of NLRP3/caspase-1 signaling in astrocytes may also be related to the D2 receptor. However, it is well known that the D2 receptor is associated with the therapeutic effects of antipsychotics (Seeman, 2002). Therefore, the D2 receptor may not be an ideal target to treat antipsychotic-induced astrocyte death. In the present study, although haloperidol may work on the D2 receptor rather than the H1 receptor to activate NLRP3/caspase-1 signaling, activation of the H1 receptor by FMPH might offset part of the effect of haloperidol on NLRP3/caspase-1 signaling. It is also possible that FMPH-induced inhibition of NLRP3 signaling is an indirect effect during haloperidol treatment.
Furthermore, we have demonstrated the involvement of NLRP3 signaling in antipsychotic-induced astrocyte death. Recent findings have revealed that pharmacological blockade of NLRP3 signaling by using salidroside, Dl-3-n-butylphthalide (NBP), gliquidone, or dimethyl fumarate significantly inhibited astrocyte-mediated neuroinflammation, reduced mitochondrial impairment, improved neuronal survival, and slowed the progression of various disease (Cai et al., 2021; Kim et al., 2021; Que et al., 2021; Tastan et al., 2021). Therefore, we suggest that inhibition of NLRP3 signaling may help suppress antipsychotic-induced inflammation and astroglia death. Future studies could investigate whether NLRP3 inhibitors such as MCC950, NBP, and gliquidone could inhibit antipsychotic-induced astrocyte death, providing potential therapeutic agents to counteract antipsychotic-induced brain cell death.
The limitation of this study was that we did not examine the effects of antipsychotics on NLRP3/caspase-1 signaling in vivo. In the future, we will investigate the chronic effects of olanzapine, quetiapine, risperidone and haloperidol on the NLRP3/caspase-1 signaling, inflammatory factors such as IL-1β, and astrocyte survival in the cortex as well as other brain regions of rodents.
Treatment with olanzapine, quetiapine, risperidone, or haloperidol time-dependently affected the viability of cultured astrocytes. During chronic treatment, antipsychotics induced astrocyte death and dose-dependently activated NLRP3/caspase-1 signaling. These effects may be partly responsible for antipsychotic induced brain volume loss. Co-treatment with a histamine H1 receptor agonist reduced the antipsychotic-induced activation of NLRP3/caspase-1 signaling, suggesting that H1 receptor activation may be an effective strategy to ameliorate antipsychotic-induced inflammation, astrocyte death, and brain volume loss. This study may help develop strategies to inhibit antipsychotic-induced astrocyte death and to develop novel antipsychotic drugs with fewer inflammatory and toxic side effects.
The original contributions presented in the study are included in the article/Supplementary Material, further inquiries can be directed to the corresponding authors.
MH, TS, and YL designed the study. MH, GG, RZ, JF, YZ, RL, and BL performed the experiments and managed the project. MH, JF, and RZ undertook the statistical analysis and wrote the first draft of the manuscript. MH and TS contributed to the interpretation of the results and revised the manuscript. All authors have contributed to and have approved the final manuscript.
This work was supported by the National Natural Science Foundation of China (81803515 and 21975191), the Natural Science Foundation of Hubei Province (2021CFB301 and 2021CFB299), and the Hubei Provincial Key Laboratory for Applied Toxicology (AT 2021-02).
The authors declare that the research was conducted in the absence of any commercial or financial relationships that could be construed as a potential conflict of interest.
All claims expressed in this article are solely those of the authors and do not necessarily represent those of their affiliated organizations, or those of the publisher, the editors and the reviewers. Any product that may be evaluated in this article, or claim that may be made by its manufacturer, is not guaranteed or endorsed by the publisher.
We thank Chao Deng and XuFeng Huang for assistance with data explanation.
The Supplementary Material for this article can be found online at: https://www.frontiersin.org/articles/10.3389/fnagi.2022.847561/full#supplementary-material
Supplementary Figure 1 | Treatment with 1, 5, 10, or 20 μM 2-(3-trifluoromethylphenyl) histamine (FMPH) did not affect astrocyte viability.
Supplementary Figure 2 | Treatment with 5 or 10 μM 2-(3-trifluoromethylphenyl) histamine (FMPH) did not affect NLRP3 protein expression in cultured astrocytes. Cultured cells were treated with vehicle (water), FMPH 5 μM, or FMPH 10 μM for 24 h. Western blot was used to investigate NLRP3 protein expression. The data are presented as the mean ± standard error of the mean. p < 0.05 (one-way analysis of variance and post hoc Dunnett’s multiple comparison test; n = 4 samples/group).
An, S., Hu, H., Li, Y., and Hu, Y. (2020). Pyroptosis plays a role in osteoarthritis. Aging Dis. 11, 1146–1157. doi: 10.14336/AD.2019.1127
Barak, N., Beck, Y., and Albeck, J. H. (2016). Betahistine decreases olanzapine-induced weight gain and somnolence in humans. J. Psychopharmacol. 30, 237–241. doi: 10.1177/0269881115626349
Boz, Z., Hu, M., Yu, Y., and Huang, X. F. (2020). N-acetylcysteine prevents olanzapine-induced oxidative stress in mHypoA-59 hypothalamic neurons. Sci. Rep. 10:19185. doi: 10.1038/s41598-020-75356-3
Cai, Y., Chai, Y., Fu, Y., Wang, Y., Zhang, Y., Zhang, X., et al. (2021). Salidroside ameliorates Alzheimer’s disease by targeting nlrp3 inflammasome-mediated pyroptosis. Front. Aging Neurosci. 13:809433. doi: 10.3389/fnagi.2021.809433
Chen, X., Yu, Y., Zheng, P., Jin, T., He, M., Zheng, M., et al. (2020b). Olanzapine increases AMPK-NPY orexigenic signaling by disrupting H1R-GHSR1a interaction in the hypothalamic neurons of mice. Psychoneuroendocrinology 114:104594. doi: 10.1016/j.psyneuen.2020.104594
Chen, Q., Men, Y., Wang, D., Xu, D., Liu, S., Xiao, S., et al. (2020a). Porcine reproductive and respiratory syndrome virus infection induces endoplasmic reticulum stress, facilitates virus replication, and contributes to autophagy and apoptosis. Sci. Rep. 10:13131. doi: 10.1038/s41598-020-69959-z
Chen, X., He, W. T., Hu, L. C., Li, J. X., Fang, Y., Wang, X., et al. (2016). Pyroptosis is driven by non-selective gasdermin-D pore and its morphology is different from MLKL channel-mediated necroptosis. Cell Res. 26, 1007–1020. doi: 10.1038/cr.2016.100
Cruz Jung, I., Machado, A. K., da Cruz, I. B. M., Barbisan, F., Azzolin, V. F., Duarte, T., et al. (2016). Haloperidol and Risperidone at high concentrations activate an in vitro inflammatory response of RAW 264.7 macrophage cells by induction of apoptosis and modification of cytokine levels. Psychopharmacology 233, 1715–1723. doi: 10.1007/s00213-015-4079-7
de Souza, D. F., Wartchow, K., Hansen, F., Lunardi, P., Guerra, M. C., Nardin, P., et al. (2013). Interleukin-6-induced S100B secretion is inhibited by haloperidol and risperidone. Prog. Neuro. Psychopharmacol. Biol. Psychiatry 43, 14–22. doi: 10.1016/j.pnpbp.2012.12.001
Deng, C., Lian, J. M., Pai, N., and Huang, X. F. (2012). Reducing olanzapine-induced weight gain side effect by using betahistine: a study in the rat model. J. Psychopharmacol. 26, 1271–1279. doi: 10.1177/0269881112449396
Ebdrup, B. H., Skimminge, A., Rasmussen, H., Aggernaes, B., Oranje, B., Lublin, H., et al. (2011). Progressive striatal and hippocampal volume loss in initially antipsychotic-naive, first-episode schizophrenia patients treated with quetiapine: relationship to dose and symptoms. Int. J. Neuropsychopharmacol. 14, 69–82. doi: 10.1017/S1461145710000817
Fatemi, S. H., Folsom, T. D., Reutiman, T. J., Pandian, T., Braun, N. N., and Haug, K. (2008). Chronic psychotropic drug treatment causes differential expression of connexin 43 and GFAP in frontal cortex of rats. Schizophr. Res. 104, 127–134. doi: 10.1016/j.schres.2008.05.016
Franchi, L., Eigenbrod, T., Munoz-Planillo, R., and Nunez, G. (2009). The inflammasome: a caspase-1-activation platform that regulates immune responses and disease pathogenesis. Nat. Immunol. 10, 241–247. doi: 10.1038/ni.1703
Fukui, H., Inagaki, N., Ito, S., Kubo, A., Kondoh, H., Yamatodani, A., et al. (1991). Histamine H1-receptors on astrocytes in primary cultures: a possible target for histaminergic neurones. Agents Actions Suppl. 33, 161–180. doi: 10.1007/978-3-0348-7309-3_12
Ghimire, L., Paudel, S., Jin, L., and Jeyaseelan, S. (2020). The NLRP6 inflammasome in health and disease. Mucosal Immunol. 13, 388–398. doi: 10.1038/s41385-020-0256-z
He, M., Huang, X. F., Gao, G., Zhou, T., Li, W., Hu, J., et al. (2019). Olanzapine-induced endoplasmic reticulum stress and inflammation in the hypothalamus were inhibited by an ER stress inhibitor 4-phenylbutyrate. Psychoneuroendocrinology 104, 286–299. doi: 10.1016/j.psyneuen.2019.03.017
He, M., Qian, K., Zhang, Y., Huang, X. F., Deng, C., Zhang, B., et al. (2020). Olanzapine-induced activation of hypothalamic astrocytes and Toll-like receptor-4 signaling via endoplasmic reticulum stress were related to olanzapine-induced weight gain. Front. Neurosci. 14:589650. doi: 10.3389/fnins.2020.589650
He, M., Zhang, Q., Deng, C., Wang, H., Lian, J., and Huang, X. F. (2014). Hypothalamic histamine H1 receptor-AMPK signaling time-dependently mediates olanzapine-induced hyperphagia and weight gain in female rats. Psychoneuroendocrinology 42, 153–164. doi: 10.1016/j.psyneuen.2014.01.018
Ho, B. C., Andreasen, N. C., Ziebell, S., Pierson, R., and Magnotta, V. (2011). Long-term antipsychotic treatment and brain volumes: a longitudinal study of first-episode schizophrenia. Arch. Gen. Psychiatry 68, 128–137. doi: 10.1001/archgenpsychiatry.2010.199
Horvath, T. L., Sarman, B., Garcia-Caceres, C., Enriori, P. J., Sotonyi, P., Shanabrough, M., et al. (2010). Synaptic input organization of the melanocortin system predicts diet-induced hypothalamic reactive gliosis and obesity. Proc. Natl. Acad. Sci. U.S.A. 107, 14875–14880. doi: 10.1073/pnas.1004282107
Johann, S., Heitzer, M., Kanagaratnam, M., Goswami, A., Rizo, T., Weis, J., et al. (2015). NLRP3 Inflammasome is expressed by astrocytes in the SOD1 mouse model of ALS and in human sporadic ALS patients. Glia 63, 2260–2273. doi: 10.1002/glia.22891
Karpati, A., Yoshikawa, T., Naganuma, F., Matsuzawa, T., Kitano, H., Yamada, Y., et al. (2019). Histamine H1 receptor on astrocytes and neurons controls distinct aspects of mouse behaviour. Sci. Rep. 9:16451. doi: 10.1038/s41598-019-52623-6
Kim, J., Park, J. H., Shah, K., Mitchell, S. J., Cho, K., and Hoe, H. S. (2021). The anti-diabetic drug gliquidone modulates lipopolysaccharide-mediated microglial neuroinflammatory responses by inhibiting the NLRP3 inflammasome. Front. Aging Neurosci. 13:754123. doi: 10.3389/fnagi.2021.754123
Konopaske, G. T., Dorph-Petersen, K. A., Sweet, R. A., Pierri, J. N., Zhang, W., Sampson, A. R., et al. (2008). Effect of chronic antipsychotic exposure on astrocyte and oligodendrocyte numbers in macaque monkeys. Biol. Psychiatry 63, 759–765. doi: 10.1016/j.biopsych.2007.08.018
Li, D. Y., Liu, M. H., Li, Z., Zheng, G., Chen, A. M., Zhao, L., et al. (2021b). Sterol-resistant SCAP overexpression in vascular smooth muscle cells accelerates atherosclerosis by increasing local vascular inflammation through activation of the NLRP3 inflammasome in mice. Aging Dis. 12, 747–763. doi: 10.14336/Ad.2020.1120
Li, W. T., Huang, X. F., Deng, C., Zhang, B. H., Qian, K., He, M., et al. (2021c). Olanzapine induces inflammation and immune response via activating ER stress in the rat prefrontal cortex. Curr. Med. Sci. 41, 788–802. doi: 10.1007/s11596-021-2401-7
Li, C. G., Chen, M. Y., He, X. H., and Ouyang, D. Y. (2021a). A mini-review on ion fluxes that regulate NLRP3 inflammasome activation. Acta Biochim. Biophys. Sin. 53, 131–139. doi: 10.1093/abbs/gmaa155
Liu, J., Zheng, J., Xu, Y., Cao, W., Wang, J., Wang, B., et al. (2021). Enriched environment attenuates pyroptosis to improve functional recovery after cerebral ischemia/reperfusion injury. Front. Aging Neurosci. 13:717644. doi: 10.3389/fnagi.2021.717644
Liu, L., and Chan, C. (2014). IPAF inflammasome is involved in interleukin-1β production from astrocytes, induced by palmitate; implications for Alzheimer’s disease. Neurobiol. Aging 35, 309–321. doi: 10.1016/j.neurobiolaging.2013.08.016
Ma, D., Yang, B., Guan, B., Song, L., Liu, Q., Fan, Y., et al. (2021). A bibliometric analysis of pyroptosis from 2001 to 2021. Front. Immunol. 12:731933. doi: 10.3389/fimmu.2021.731933
Mao, L., Kitani, A., Strober, W., and Fuss, I. J. (2018). The role of NLRP3 and IL-1beta in the pathogenesis of inflammatory bowel disease. Front. Immunol. 9:2566. doi: 10.3389/fimmu.2018.02566
May, M., Slitzky, M., Rostama, B., Barlow, D., and Houseknecht, K. L. (2020). Antipsychotic-induced immune dysfunction: a consideration for COVID-19 risk. Brain Behav. Immun. Health 6:100097. doi: 10.1016/j.bbih.2020.100097
McClellan, S. A., Jerome, A., Suvas, S., and Hazlett, L. D. (2017). NLRC4 regulates caspase-1 and IL-1beta production in a CD11b(low) Ly6G(low) population of cells required for resistance to Pseudomonas aeruginosa keratitis. PLoS One 12:e0185718. doi: 10.1371/journal.pone.0185718
McIlwain, D. R., Berger, T., and Mak, T. W. (2013). Caspase functions in cell death and disease. Cold Spring Harb. Perspect. Biol. 5:a008656. doi: 10.1101/cshperspect.a008656
Mezzomo, N. F., da Silva Schmitz, I., de Lima, V. B., Dorneles, G. P., Schaffer, L. F., Boeck, C. R., et al. (2022). Reversal of haloperidol-induced orofacial dyskinesia and neuroinflammation by isoflavones. Mol. Biol. Rep. 49, 1917–1923. doi: 10.1007/s11033-021-07003-7
Miller, D. D., Andreasen, N. C., O’Leary, D. S., Watkins, G. L., Boles Ponto, L. L., and Hichwa, R. D. (2001). Comparison of the effects of risperidone and haloperidol on regional cerebral blood flow in schizophrenia. Biol. Psychiatry 49, 704–715. doi: 10.1016/s0006-3223(00)01001-5
Molina, V., Reig, S., Sanz, J., Palomo, T., Benito, C., Sanchez, J., et al. (2007). Changes in cortical volume with olanzapine in chronic schizophrenia. Pharmacopsychiatry 40, 135–139. doi: 10.1055/s-2007-981479
Moncrieff, J., and Leo, J. (2010). A systematic review of the effects of antipsychotic drugs on brain volume. Psychol. Med. 40, 1409–1422. doi: 10.1017/S0033291709992297
Nardin, P., Tramontina, A. C., Quincozes-Santos, A., Tortorelli, L. S., Lunardi, P., Klein, P. R., et al. (2011). In vitro S100B secretion is reduced by apomorphine: effects of antipsychotics and antioxidants. Prog. Neuropsychopharmacol. Biol. Psychiatry 35, 1291–1296. doi: 10.1016/j.pnpbp.2011.04.004
Poyurovsky, M., Fuchs, C., Pashinian, A., Levi, A., Weizman, R., and Weizman, A. (2013). Reducing antipsychotic-induced weight gain in schizophrenia: a double-blind placebo-controlled study of reboxetine-betahistine combination. Psychopharmacology 226, 615–622. doi: 10.1007/s00213-012-2935-2
Que, R., Zheng, J., Chang, Z., Zhang, W., Li, H., Xie, Z., et al. (2021). Dl-3-n-Butylphthalide rescues dopaminergic neurons in Parkinson’s disease models by inhibiting the NLRP3 inflammasome and ameliorating mitochondrial impairment. Front. Immunol. 12:794770. doi: 10.3389/fimmu.2021.794770
Romero, A., Dongil, P., Valencia, I., Vallejo, S., Hipolito-Luengo, A. S., Diaz-Araya, G., et al. (2022). Pharmacological blockade of NLRP3 inflammasome/IL-1beta-positive loop mitigates endothelial cell senescence and dysfunction. Aging Dis. 13, 284–297. doi: 10.14336/AD.2021.0617
Shao, W., Zhang, S. Z., Tang, M., Zhang, X. H., Zhou, Z., Yin, Y. Q., et al. (2013). Suppression of neuroinflammation by astrocytic dopamine D2 receptors via alphaB-crystallin. Nature 494, 90–94. doi: 10.1038/nature11748
Shao, Y., Peng, H., Huang, Q., Kong, J., and Xu, H. (2015). Quetiapine mitigates the neuroinflammation and oligodendrocyte loss in the brain of C57BL/6 mouse following cuprizone exposure for one week. Eur. J. Pharmacol. 765, 249–257. doi: 10.1016/j.ejphar.2015.08.046
Smith, R. C., Maayan, L., Wu, R., Youssef, M., Jing, Z., Sershen, H., et al. (2018). Betahistine effects on weight-related measures in patients treated with antipsychotic medications: a double-blind placebo-controlled study. Psychopharmacology 235, 3545–3558. doi: 10.1007/s00213-018-5079-1
Sonego, A. B., Prado, D. D. S., and Guimaraes, F. S. (2021). PPARgamma receptors are involved in the effects of cannabidiol on orofacial dyskinesia and cognitive dysfunction induced by typical antipsychotic in mice. Prog. Neuropsychopharmacol. Biol. Psychiatry 111:110367. doi: 10.1016/j.pnpbp.2021.110367
Tastan, B., Arioz, B. I., Tufekci, K. U., Tarakcioglu, E., Gonul, C. P., Genc, K., et al. (2021). Dimethyl fumarate alleviates NLRP3 inflammasome activation in microglia and sickness behavior in LPS-challenged mice. Front. Immunol. 12:737065. doi: 10.3389/fimmu.2021.737065
van Kralingen, C., Kho, D. T., Costa, J., Angel, C. E., and Graham, E. S. (2013). Exposure to inflammatory cytokines IL-1beta and TNFalpha induces compromise and death of astrocytes; implications for chronic neuroinflammation. PLoS One 8:e84269. doi: 10.1371/journal.pone.0084269
Vernon, A. C., Natesan, S., Crum, W. R., Cooper, J. D., Modo, M., Williams, S. C., et al. (2012). Contrasting effects of haloperidol and lithium on rodent brain structure: a magnetic resonance imaging study with postmortem confirmation. Biol. Psychiatry 71, 855–863. doi: 10.1016/j.biopsych.2011.12.004
Vernon, A. C., Natesan, S., Modo, M., and Kapur, S. (2011). Effect of chronic antipsychotic treatment on brain structure: a serial magnetic resonance imaging study with ex vivo and postmortem confirmation. Biol. Psychiatry 69, 936–944. doi: 10.1016/j.biopsych.2010.11.010
Voet, S., Srinivasan, S., Lamkanfi, M., and van Loo, G. (2019). Inflammasomes in neuroinflammatory and neurodegenerative diseases. EMBO Mol. Med. 11:e10248. doi: 10.15252/emmm.201810248
Vucicevic, L., Misirkic-Marjanovic, M., Paunovic, V., Kravic-Stevovic, T., Martinovic, T., Ciric, D., et al. (2014). Autophagy inhibition uncovers the neurotoxic action of the antipsychotic drug olanzapine. Autophagy 10, 2362–2378. doi: 10.4161/15548627.2014.984270
Wang, Q., Luo, Y. Q., Chaudhuri, K. R., Reynolds, R., Tan, E. K., and Pettersson, S. (2021). The role of gut dysbiosis in Parkinson’s disease: mechanistic insights and therapeutic options. Brain 144, 2571–2593. doi: 10.1093/brain/awab156
Wu, J., Lin, S., Wan, B., Velani, B., and Zhu, Y. (2019). Pyroptosis in liver disease: new insights into disease mechanisms. Aging Dis. 10, 1094–1108. doi: 10.14336/AD.2019.0116
Xu, J., Zhang, X., Qian, Q., Wang, Y., Dong, H., Li, N., et al. (2018). Histamine upregulates the expression of histamine receptors and increases the neuroprotective effect of astrocytes. J. Neuroinflammation 15:41. doi: 10.1186/s12974-018-1068-x
Yang, D., He, Y., Munoz-Planillo, R., Liu, Q., and Nunez, G. (2015). Caspase-11 requires the pannexin-1 channel and the purinergic P2X7 pore to mediate pyroptosis and endotoxic shock. Immunity 43, 923–932. doi: 10.1016/j.immuni.2015.10.009
Yang, F., Ye, X. J., Chen, M. Y., Li, H. C., Wang, Y. F., Zhong, M. Y., et al. (2021). Inhibition of NLRP3 inflammasome activation and pyroptosis in macrophages by taraxasterol is associated with its regulation on mTOR signaling. Front. Immunol. 12:632606. doi: 10.3389/fimmu.2021.632606
Yang, Y., Liu, P. Y., Bao, W., Chen, S. J., Wu, F. S., and Zhu, P. Y. (2020). Hydrogen inhibits endometrial cancer growth via a ROS/NLRP3/caspase-1/GSDMD-mediated pyroptotic pathway. BMC Cancer 20:28. doi: 10.1186/s12885-019-6491-6
Yu, P., Zhang, X., Liu, N., Tang, L., Peng, C., and Chen, X. (2021). Pyroptosis: mechanisms and diseases. Signal Transduct. Target Ther. 6:128. doi: 10.1038/s41392-021-00507-5
Zhang, J., Jiang, N., Zhang, L., Meng, C., Zhao, J., and Wu, J. (2020). NLRP6 expressed in astrocytes aggravates neurons injury after OGD/R through activating the inflammasome and inducing pyroptosis. Int. Immunopharmacol. 80:106183. doi: 10.1016/j.intimp.2019.106183
Zhang, Q., He, M., Deng, C., Wang, H., and Huang, X. F. (2014). Effects of olanzapine on the elevation of macrophage infiltration and pro-inflammatory cytokine expression in female rats. J. Psychopharmacol. 28, 1161–1169. doi: 10.1177/0269881114555250
Zhang, Z., Zhao, L., Zhou, Y., Lu, X., Wang, Z., Wang, J., et al. (2017). Taurine ameliorated homocysteine-induced H9C2 cardiomyocyte apoptosis by modulating endoplasmic reticulum stress. Apoptosis 22, 647–661. doi: 10.1007/s10495-017-1351-9
Zheng, Z., Wang, T., Chen, J., Qiu, H., Zhang, C., Liu, W., et al. (2021). Inflammasome-Induced osmotic pressure and the mechanical mechanisms underlying astrocytic swelling and membrane blebbing in pyroptosis. Front. Immunol. 12:688674. doi: 10.3389/fimmu.2021.688674
Zhu, J., Hu, Z., Han, X., Wang, D., Jiang, Q., Ding, J., et al. (2018). Dopamine D2 receptor restricts astrocytic NLRP3 inflammasome activation via enhancing the interaction of beta-arrestin2 and NLRP3. Cell Death Differ. 25, 2037–2049. doi: 10.1038/s41418-018-0127-2
Keywords: antipsychotics, astrocytes, brain volume loss, pyroptosis, NLRP3, caspase-1, GSDMD
Citation: He M, Fan J, Zhou R, Gao G, Li R, Zuo Y, Li B, Li Y and Sun T (2022) NLRP3/Caspase-1-Mediated Pyroptosis of Astrocytes Induced by Antipsychotics Is Inhibited by a Histamine H1 Receptor-Selective Agonist. Front. Aging Neurosci. 14:847561. doi: 10.3389/fnagi.2022.847561
Received: 02 January 2022; Accepted: 15 April 2022;
Published: 09 May 2022.
Edited by:
Xiang Cai, Capital Medical University, ChinaReviewed by:
Yueqing Su, Fujian Medical University, ChinaCopyright © 2022 He, Fan, Zhou, Gao, Li, Zuo, Li, Li and Sun. This is an open-access article distributed under the terms of the Creative Commons Attribution License (CC BY). The use, distribution or reproduction in other forums is permitted, provided the original author(s) and the copyright owner(s) are credited and that the original publication in this journal is cited, in accordance with accepted academic practice. No use, distribution or reproduction is permitted which does not comply with these terms.
*Correspondence: Taolei Sun, c3VudGxAd2h1dC5lZHUuY24=; Yanmei Li, NDE2NDk0MTk3QHFxLmNvbQ==
†These authors have contributed equally to this work
Disclaimer: All claims expressed in this article are solely those of the authors and do not necessarily represent those of their affiliated organizations, or those of the publisher, the editors and the reviewers. Any product that may be evaluated in this article or claim that may be made by its manufacturer is not guaranteed or endorsed by the publisher.
Research integrity at Frontiers
Learn more about the work of our research integrity team to safeguard the quality of each article we publish.