- 1Department of Neurosurgery, Nanfang Hospital, Southern Medical University, Guangzhou, China
- 2Department of Neurosurgery, Xuanwu Hospital, Capital Medical University, Beijing, China
- 3China International Neuroscience Institute (China-INI), Beijing, China
Background: Microglia have been identified for a century. In this period, their ontogeny and functions have come to light thanks to the tireless efforts of scientists. However, numerous documents are being produced, making it challenging for scholars, especially those new to the field, to understand them thoroughly. Therefore, having a reliable method for quickly grasping a field is crucial.
Methods: We searched and downloaded articles from the Web of Science Core Collection with “microglia” or “microglial” in the title from 2002 to 2021. Eventually, 12,813 articles were located and, using CiteSpace and VOSviewer, the fundamental data, knowledge domains, hot spots, and emerging trends, as well as the influential literature in the field of microglia research, were analyzed.
Results: Following 2011, microglia publications grew significantly. The two prominent journals are Glia and J Neuroinflamm. The United States and Germany dominated the microglia study. The primary research institutions are Harvard Univ and Univ Freiburg, and the leading authors are Prinz Marco and Kettenmann Helmut. The knowledge domains of microglia include eight directions, namely neuroinflammation, lipopolysaccharide, aging, neuropathic pain, macrophages, Alzheimer’s disease, retina, and apoptosis. Microglial phenotype is the focus of research; while RNA-seq, exosome, and glycolysis are emerging topics, a microglial-specific marker is still a hard stone. We also identified 19 influential articles that contributed to the study of microglial origin (Mildner A 2007; Ginhoux F 2010), identity (Butovsky O 2014), homeostasis (Cardona AE 2006; Elmore MRP 2014); microglial function such as surveillance (Nimmerjahn A 2005), movement (Davalos D 2005; Haynes SE 2006), phagocytosis (Simard AR 2006), and synapse pruning (Wake H 2009; Paolicelli RC 2011; Schafer DP 2012; Parkhurst CN 2013); and microglial state/phenotype associated with disease (Keren-Shaul H 2017), as well as 5 review articles represented by Kettenmann H 2011.
Conclusion: Using bibliometrics, we have investigated the fundamental data, knowledge structure, and dynamic evolution of microglia research over the previous 20 years. We hope this study can provide some inspiration and a reference for researchers studying microglia in neuroscience.
Introduction
Microglia are resident macrophages in the central nervous system (Prinz et al., 2019). Since Río Hortega first discovered and described them in 1919 (Sierra et al., 2019), they have advanced quickly thanks to the advancements in molecular biology, microscope imaging technology, and single-cell sequencing technology (Bohlen et al., 2019). As macrophages derived from the yolk sac (Ginhoux et al., 2010), microglia can not only be transformed by bone marrow-derived macrophages (which can be restricted by the blood–brain barrier; Mildner et al., 2007) but also achieve self-renewal (Ajami et al., 2007) in the brain.
As a critical intracranial immune cell, it has been proven to play an essential role in the central nervous system’s development, maturation, homeostasis, and disease (Bohlen et al., 2019). In neural development and the maintenance of adult brain homeostasis, it plays an essential role in the phagocytosis of dead cells, pruning synapses, monitoring synaptic activity, rebuilding neural circuits, and exhibiting immune activity. Microglia are closely related to many diseases, such as stroke (Ma et al., 2017), Alzheimer’s disease (AD) (Boche and Gordon, 2022), Parkinson’s disease (PD) (Joers et al., 2017), depression (Yirmiya et al., 2015), schizophrenia (Xia et al., 2020), and traumatic brain injury (TBI) (Morganti-Kossmann, 2019). Microglia can change their phenotype in a disease state, exert anti-infection activity, lessen nerve damage, and promote nerve repair. However, they do not always provide protection, particularly when microglia-mediated neuroinflammation is challenging to control (Joers et al., 2017; Bai et al., 2020; Xia et al., 2020). In this condition, neurotoxic substances such as reactive oxygen species (ROS), nitric oxide (NO), interleukin-1β (IL-1β), and tumor necrosis factor-α (TNF-α) might be produced. Therefore, understanding the detailed mechanism of phenotypic transformation of microglia under physiological or pathological conditions is of great significance for diagnosing and treating many central nervous system diseases. Prior to this objective, identifying peripheral monocytes, intracranial boundary macrophages, and microglia is of utmost importance. Fortunately, this wish has gradually come true with the advancement of single-cell sequencing and microscopic imaging technology, and some microglia-specific molecular markers have been gradually revealed (Butovsky et al., 2014; Bennett et al., 2018). At the same time, the microglial phenotypic transformation becomes clear with this technology’s support during the disease’s occurrence, development, and outcome (Keren-Shaul et al., 2017).
Due to the development of neuroscience research, the research literature in the field of microglia is also increasing rapidly (Sierra et al., 2019). There is frequently no way to start with such a large body of literature for new researchers. Bibliometrics is an excellent way to solve this problem (Chen, 2006; Hou et al., 2022). Mathematical statistical methods in bibliometrics are often used to systematically explore and evaluate a field’s intellectual base, hotspots, and directions. By using the method of bibliometrics, we can make quantitative statistics and visual analyses of cooperative networks of disciplines, countries, institutions, authors, and journals in the research field (Agarwal et al., 2016). We can also carry out co-word, co-occurrence, co-citation, and coupling analysis of keywords, terms, and references and construct a visual knowledge graph (Chen et al., 2014, 2018). It is helpful for researchers to quickly grasp the basic situation of a specific research field and the hot spots, key points, and difficulties in a specific direction, which traditional reviews cannot do. Bibliometrics is gradually becoming known to the general public and used in literature analysis due to the rapid growth of scientific research literature data. We construct the intellectual foundation of microglia research in the 20 years from 2002 to 2021 using the two-core bibliometrics software CiteSpace and VOSviewer and aim to identify its development path, research trend, and challenges. First, we make a statistical analysis of disciplines, journals, authors, countries, institutions, co-cited authors, and co-cited journals to understand the basic information in this field; secondly, we carry out clustering and burstness analysis of keywords to explore the research direction, hotspots, and evolution over time in the field. Finally, through the co-citation analysis of the references, we understand the core literature in the field on the one hand and the knowledge structure and dynamic changes in the field on the other.
Materials and methods
Data search and download
The literature data for bibliometric analysis is retrieved from the Web of Science Core Collection. We set the following search restrictions: title “microglia” OR “microglial,” publication date “2002-01-01” to “2021-12-31,” document types “article” or “review article,” language “English.” We found 12,815 relevant documents on microglia using the above search method and 12,813 documents after CiteSpace removed duplicates.
CiteSpace
CiteSpace is an information visualization software developed by Chen (2006), a famous bibliometrics expert, based on citation analysis theory. Numerous functions, including cooperative network analysis, co-word analysis, co-citation analysis, and others, can be realized by it (Chen, 2004; Li and Liu, 2020). At the same time, its unique betweenness centrality and burstness analysis have attracted much attention. Betweenness centrality is when an entity connects the content of different groups, indicating that the entity plays a turning point. “Burstness” denotes an abrupt rise in the frequency of a keyword or numerous citations over time in a body of literature; this definition also applies to the abrupt rise in the number of articles published by countries/regions, organizations, or authors (Chen et al., 2014). Betweenness centrality and burstness analysis are very helpful for us to quickly understand the evolution of knowledge structures, milestone papers, research hotspots and frontiers, and emerging topics. The time slice for our analysis is set to 1 year, the g-index to k = 25, and the citation analysis to k = 20. Each analysis selects a node type, and the clustering analysis uses the LLR algorithm. We use CiteSpace to perform subject category co-occurrence analysis, literature clustering, keyword clustering, and the burstness analysis of countries, institutions, keywords, and references.
VOSviewer
Leiden University in the Netherlands developed this software for creating, visualizing, and exploring literature data (van Eck and Waltman, 2010). Its simple interface and unique overlay view are well received. We use it for visual analysis of country/regional cooperation networks, institutional cooperation networks, author cooperation networks, and keyword co-word network graphs (Ding and Yang, 2020; Chen et al., 2021; Liu and Avello, 2021). The parameters were as follows: “method” was set to “association strength” (country) or “LinLog/modularity” (institution, author, keyword); “attraction,” “repulsion,” and “resolution” were adjusted according to the content.
It should be noted that the averaged normalized number of citations (Avg. norm. Citations, i.e., citation intensity described in this manuscript) of a document equals the number of citations of the document divided by the average number of citations of all documents published in the same year and included in the data that is provided to VOSviewer. The normalization corrects the fact that older documents have had more time to receive citations than more recent documents.
Excel is used to export the data produced by CiteSpace and VOSviewer analysis for further sorting, filtering, and analysis. When conducting keyword analysis, we only choose the author’s keywords, which are sufficient even though they are not exhaustive. We standardized the extracted keywords by (1) combining words with the same meaning so that they are treated as synonyms in the software, (2) removing duplicates, and (3) deleting unintentional words (such as numbers).
Results
Publication trends, subject categories, and journals
When evaluating the advancement of scientific investigation, which partly reflects the growth of knowledge in this field, the annual volume of articles is a significant factor to consider. Figure 1’s depiction of the published literature’s time distribution revealed that the number of articles about microglia had continued to rise, particularly since 2011, when it spiked sharply and grew significantly through 2021. We next explored the discipline attributes in the field of microglia research. As seen in Figure 2A, research on microglia is primarily focused on five fields: neurosciences, immunology, biochemistry and molecular biology, pharmacology and pharmacy, and cell biology. However, a discipline burstness analysis (a total of 16 bursts are detected; Figure 2B) reveals that early studies concentrated on pathology and virology, then emerged in anesthesiology and radiology, nuclear medicine, and medical imaging, and finally appeared in toxicology and surgery. Recently, it has been chiefly seen in physics, material science, and developmental biology (Figure 2B).
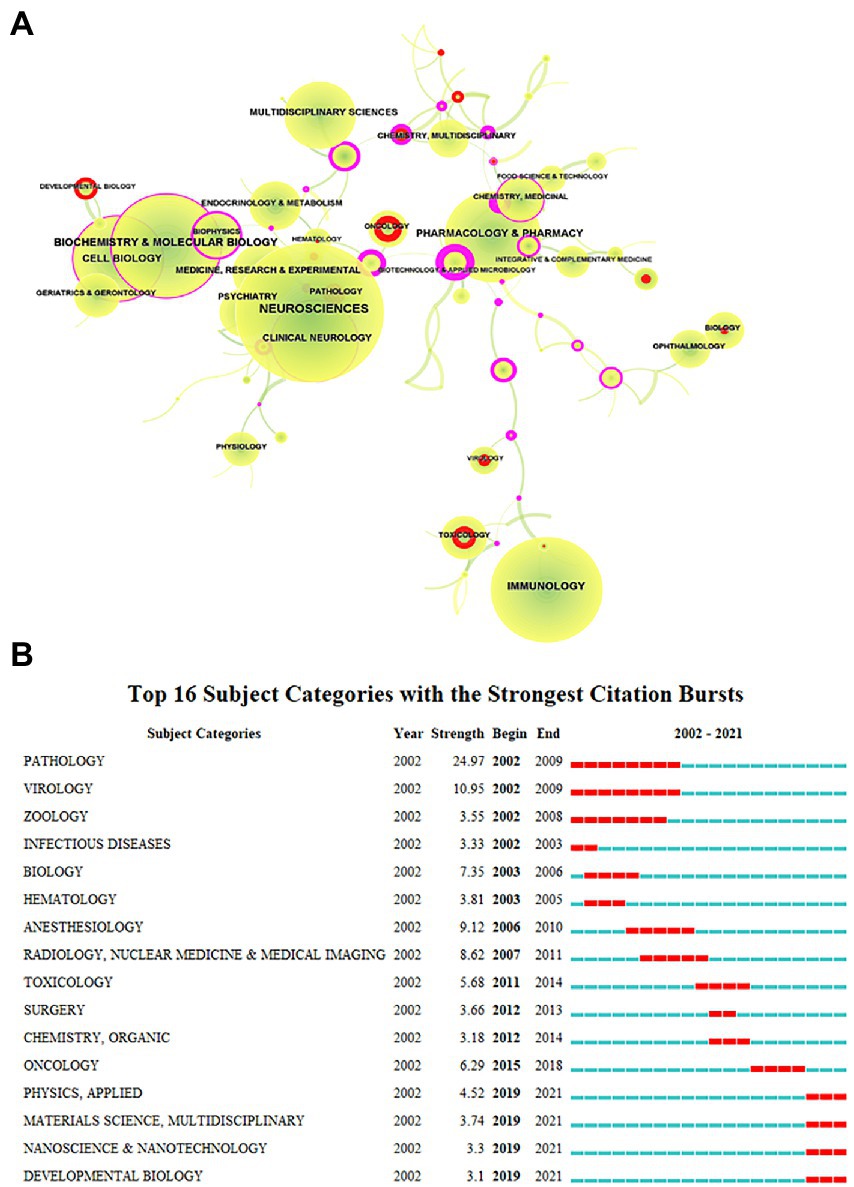
Figure 2. Subject categories of publications analyzed by CiteSpace. (A) The size of the node represents the number of publications, the link represents the degree of connection between disciplines, the color of the node and link represents different years, the color from green to yellow represents 2002 to 2021, red represents the intensity of burst, and purple represents betweenness centrality. (B) The burstness detection of the subject category by CiteSpace, “strength” represents the intensity of the burst, “begin” represents the beginning year of the burst, “end” indicates the ending year of the burst, red dotted line indicates the duration of the burst. The blue line indicates the entire period from 2002 to 2021.
We used VOSviewer to make statistics on journal information. Twelve Thousand Eight Hundred Thirteen articles are published in 1,336 journals. The top 10 journals are chosen based on the number of articles, as shown in Table 1. Glia and J Neuroinflamm account for about 1/10 of the articles, followed by J Neurochem, Brain Behav Immun, J Neurosci, J Neuroimmunol account for another 1/10. Among these journals, 5 in Q1, 3 in Q2, 2 in Q3, and 7 have an impact factor of more than 5. Then we analyze the cited journals. The top 10 co-cited journals have all been cited more than 10,000 times. As shown in Table 1, J Neurosci, Glia, P Natl Acad Sci USA, J Biol Chem, Nat Neurosci, J Immunol Science, J Neuroinflamm, Nature, and J Neurochem are the top 10 highly cited journals, of which there are 7 in Q1, 3 in Q2 and 2 with impact factors over 60.
Country and institutional distributions of microglia research
In this study, the cooperative network map of countries/regions in this field was made using VOSviewer software. The results show that 91 countries have participated in this field. The top 10 countries/regions in terms of the number of articles (Table 2) are the United States (3983), People R China (3395), Germany (1233), Japan (1175), South Korea (1175), England (682), Canada (629), Italy (5505), France (430), and Spain (420). Among them, the first four countries accounted for 67.38% of the total articles.
The number of articles published does not fully represent a country’s contribution to a specific field, so on this basis, we explore the average publication time and citation intensity (Figure 3) of documents in each country/region. As can be seen from the figure, the average publication time of most of the top countries is around 2014, while People R China is around 2017 (Figure 3A). According to the citation intensity of articles in various countries, it is found that the citation intensity is higher in the United States, Germany, England, and the Netherlands, slightly lower in Canada, Italy, and France, and poor in People R China, Japan, and South Korea (Figure 3B; Table 2). We continue to analyze the betweenness centrality (which can measure the country’s role in the knowledge dissemination process) of each country. Betweenness centrality greater than 0.1 is considered to play a more critical role, while the United States, Germany, South Korea, People R China, Canada, France, and England are all greater than 0.1 (Table 2). CiteSpace was used to carry out burstness detection. We discovered that 11 countries/regions had reached the stage of a surge in the number of bursts (Figure 3C). We also discovered that the volume of articles from Iran, Mexico, Scotland, and Russia had significantly increased over the past 2 years, indicating that these countries/regions may go on to play a more prominent role in this field (Figure 3C). The clustering view shows much cooperation between countries, with the United States-People R China-Japan-South Korea, Germany-Netherlands-Switzerland, and England-France-Spain-Italy constituting the three largest cooperation groups (Figure 3D).
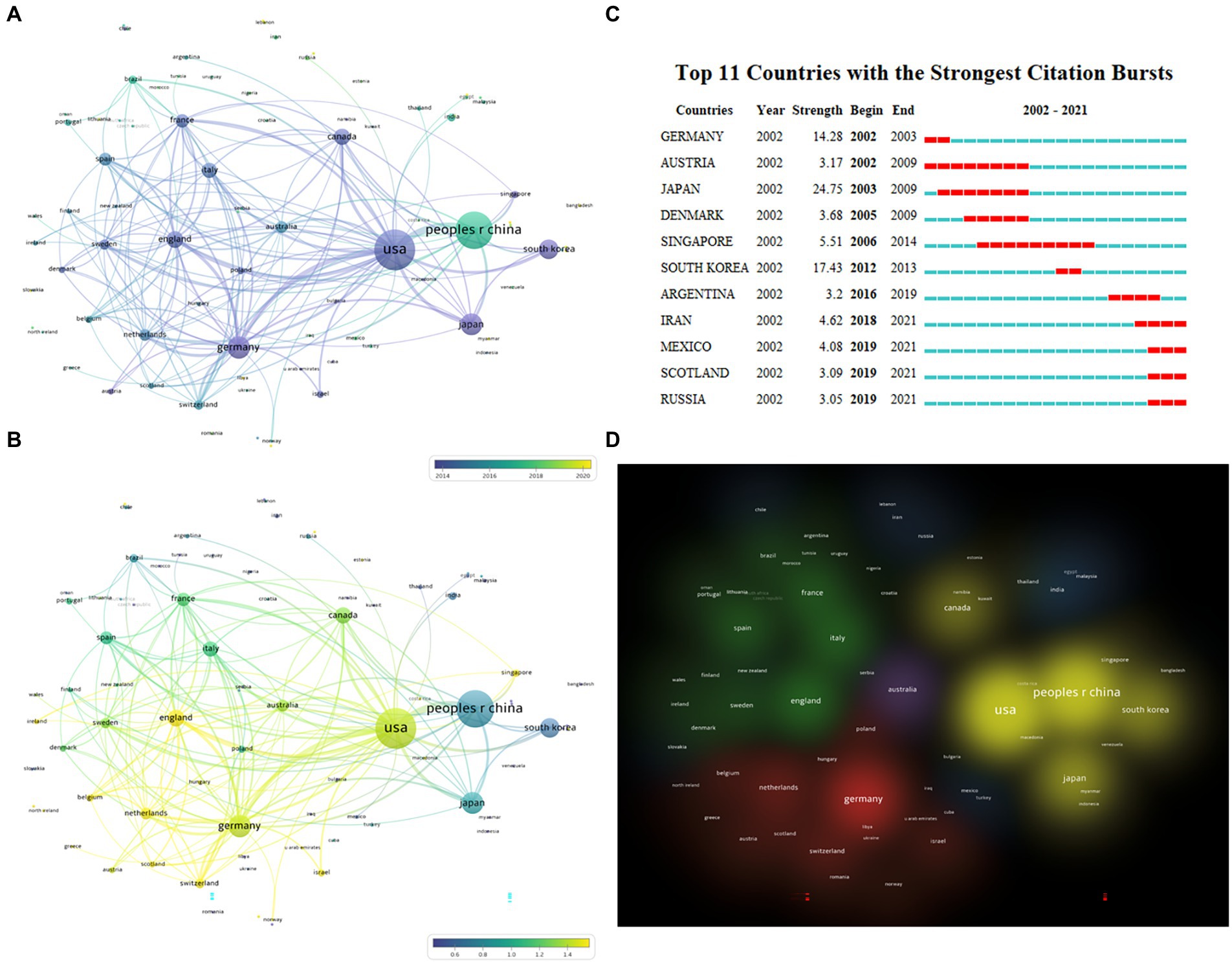
Figure 3. Distribution of publications from different countries/regions. (A) Overlay view of countries/regions cooperation network with average publication year, which is indicated by color from dark blue to bright yellow (VOSviewer). (B) Overlay view of countries/regions cooperation network with normalized average citations. Dark blue means lower citations, while bright yellow means higher citations (VOSviewer). (C) The burstness detection of national publications by CiteSpace, “strength” represents the intensity of the burst, “begin” represents the beginning year of the burst, “end” indicates the ending year of the burst, red dotted line indicates the duration of the burst. The blue line indicates the entire period from 2002 to 2021. (D) Cluster view of cooperative relationships between countries, with the same color representing closely cooperative countries (VOSviewer).
Then we use a similar method to analyze the research institutions; the top 10 institutions are listed in Supplementary Table S1, which are Harvard Univ (267), Kyushu Univ (204), Shanghai Jiao Tong Univ (193), Fudan Univ (153), Univ Freiburg (145), Chinese Acad Sci (140), Kyung Hee Univ (138), Sun Yat Sen Univ (137), Huazhong Univ Sci and Technol (136), and Univ British Columbia (134). Regarding betweenness centrality, Harvard Univ (0.15) ranks first, followed by Kyushu Univ (0.07). In terms of citation intensity, Harvard Univ (2.67) and Univ Freiburg (2.29) are cited most intensively (Supplementary Table S1). According to the cooperative network map (Figure 4A), there are eight large cooperative groups, with Harvard Univ, Univ Freiburg, Shanghai Jiao Tong Univ, Kyushu Univ, Kyung Hee Univ, China Med Univ, Cambridge Univ, and McGill Univ. as the core. We also used CiteSpace for burstness detection, which developed rapidly after 2017. We found that 9 institutions met the criteria (Figure 4B), with the top three being Wuhan Univ, Zhengzhou Univ., and Univ. Edinburgh, respectively, suggesting that these institutions have more potential for development.
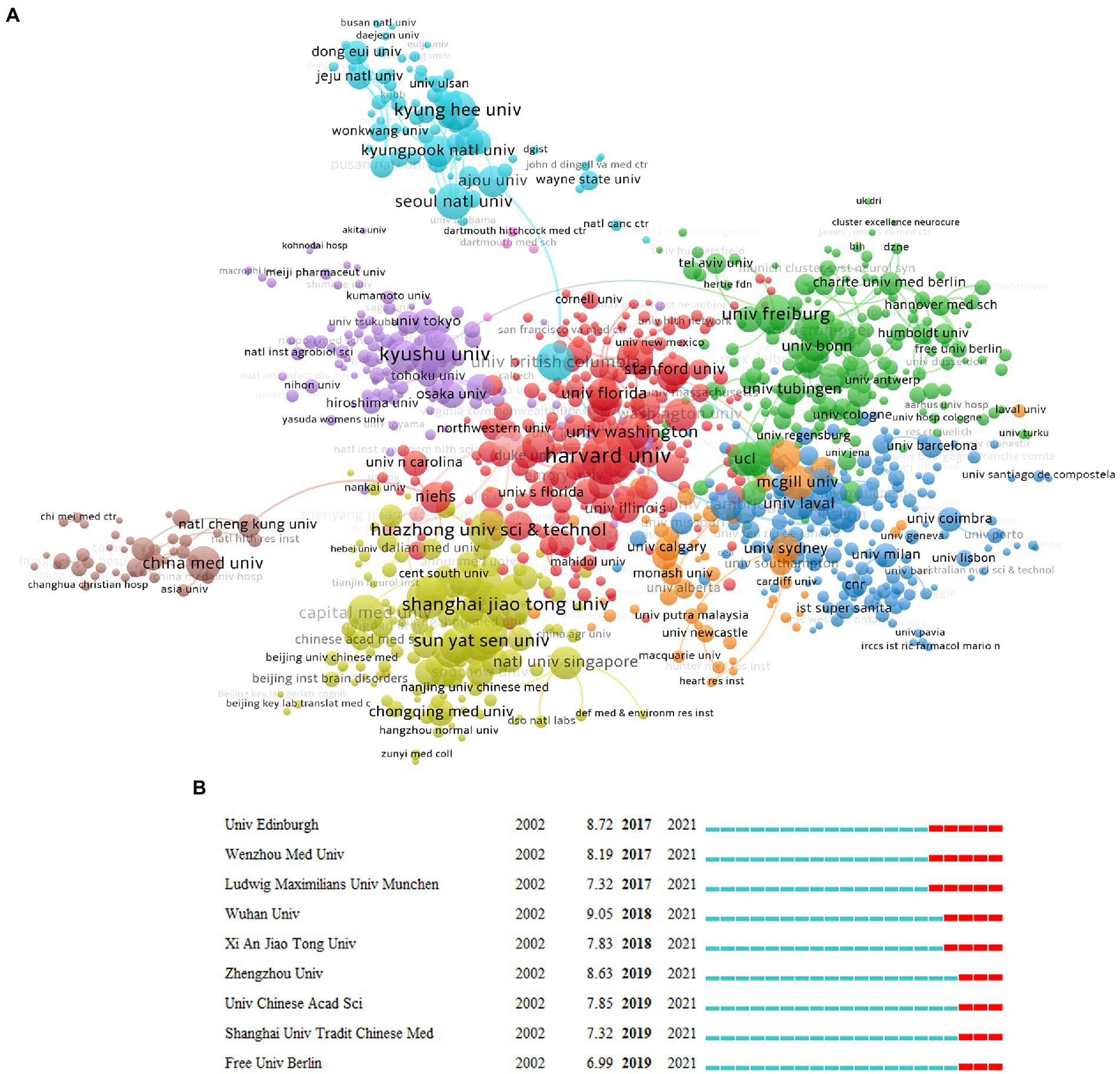
Figure 4. Distribution of publications from different institutions. (A) The network diagram of institutional cooperation, in which the same color represents a closely cooperative organization (VOSviewer). (B) The burstness detection of the number of institutional publications (lasting until 2021) by CiteSpace, “strength” represents the intensity of the burst, “begin” represents the beginning year of the burst, and “end” indicates the ending year of the burst, red dotted line indicates the duration of the burst. The blue line indicates the entire period from 2002 to 2021.
Author contributions in the field of microglial research
We use the statistical data from VOSviewer as the foundation because Web of Science and CiteSpace base their counts on name abbreviations, which are significantly impacted by duplicate names. The top 10 authors are listed on the left side of the table (Table 3). They are Prinz Marco (70), Kettenmann Helmut (62), Tremblay Marie-Eve (59), followed by Choi Yung Hyun (53), Wu Long-Jun (53), Inoue Kazuhide (51), Wang Wei (47), Hong Jau-Shyong (46), Tsuda Makoto (46), Kim Gi-Young (45). Among the 10 authors, Prinz Marco (3.85) and Kettenmann Helmut (2.40) also have a higher citation intensity indicated by Avg. norm. citations. We also list the top 10 authors in citation intensity (Table 3): Bennett Mariko L. (14.70), Keren-Shaul Hadas (13.81), Barres Ben A. (12.75), followed by Liddelow Shane A. (12.63), Panicker Nikhil (11.93), and Bohlen Christopher J. (11.75). These authors may not have many papers, but their research may have an important impact on the field. We have also analyzed the authors in the references, called the “cited authors”, namely Streit Wj (2797), Hanisch Uk (1869), Ransohoff Rm (1813), Perry Vh (1730), Nimmerjahn A (1722), and Mcgeer Pl (1642). To explore the cooperation between the authors, we have constructed a cooperative network graph of the authors. As shown in Figure 5, different colors represent different clusters. We can see that Prinz Marco (green), Kettenmann Helmut (light red), Tremblay Marie-Eve (pink), Inoue Kazuhide (light pink), Ransohoff Rm-Butovsky O (blue), Hong Jau-shyong-Zhang Wei (red), Wu Longjun (orange), Nakanishi Hiroshi (yellow green), and Kim Hee-sun (brown) has formed a large research group.
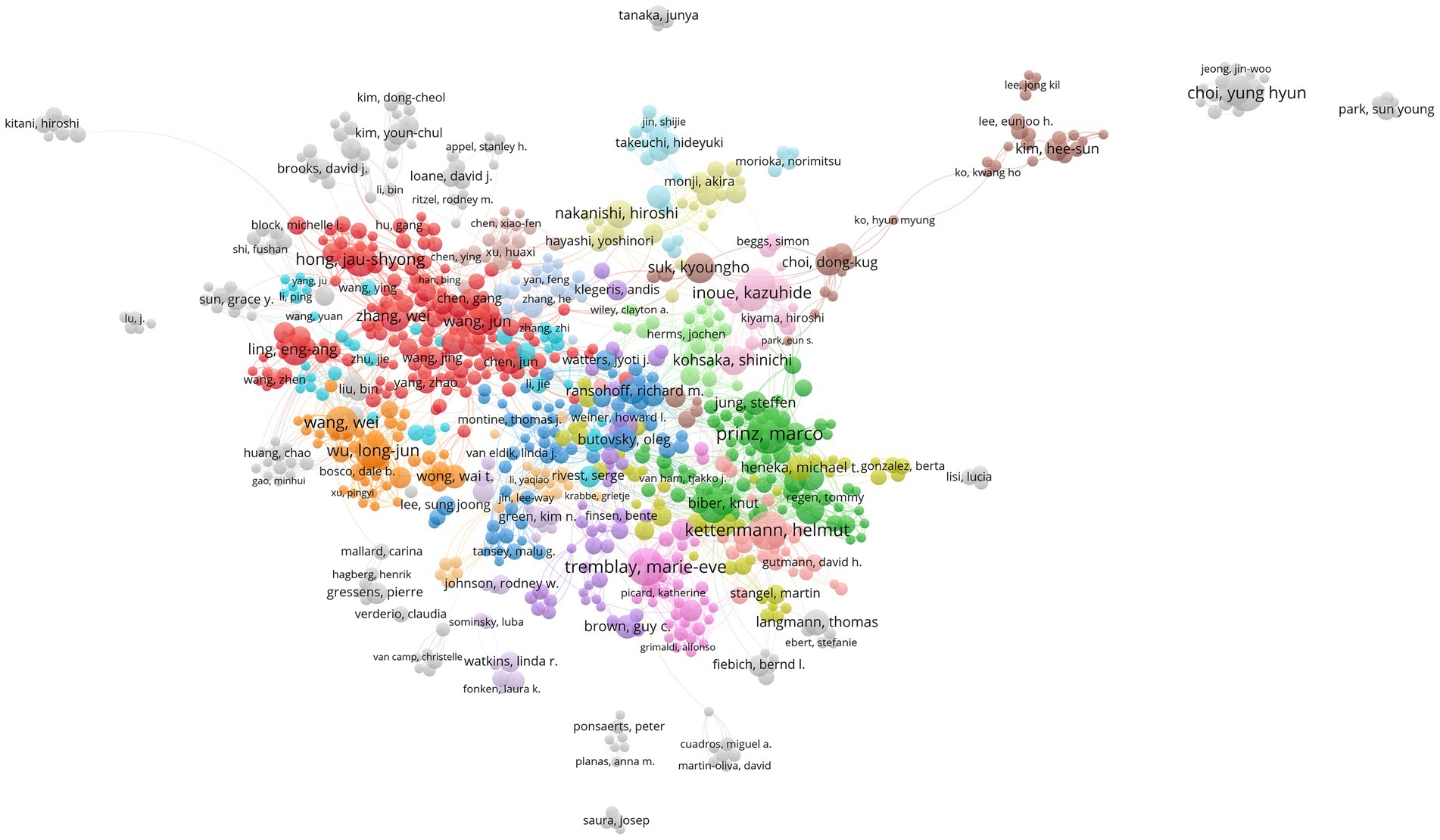
Figure 5. The co-occurrence map of authors in the microglial research field (VOSviewer). The node’s size reflects the co-occurrence frequency of the authors, the link reflects the co-occurrence relationship between the authors, and different colors represent the clustering results of the cooperative network, and the closer the relationship is with the same color.
Knowledge domains, evolutions, and emerging trends
Keywords are the generalization of a field of research. To explore the intellectual structure and dynamic changes in microglia research in the past 20 years, we used VOSviewer and CiteSpace for co-word analysis and clustering. The keywords in the top 20 are shown in Table 4 (keywords A, here, we have removed “microglia”); we can see that the primary research has focused on neuroinflammation. The second is to study the phenotypic changes, cytokine release, phagocytic activity, and cytotoxicity of microglia in different neurodegenerative diseases (AD, PD, etc.).
According to the results of VOSviewer clustering we got 8 clusters (Figure 6) labeled with the top two keywords in each cluster. The first group is neuroinflammation and microglial activation (cluster#1). As the largest group it involves a broad spectrum of diseases such as neurodegenerative diseases TBI and stroke. The main research content involved is the M1/M2 phenotype, microglial polarization and the associated neuroprotection or neurotoxicity functions. The second group is lipopolysaccharide and nf-κb signal pathway (cluster#2); this group was like cluster#1. Other keywords in the group such as NO, TNF-α toll-like receptor, mitogen activated protein kinase (MAPK), and ROS suggest that this group mainly studies the activators and signaling pathways involved in the inflammatory activation of microglia as well as the resulting inflammatory and oxidative stress damage. The third group is hippocampus and aging (cluster#3) which also involves the keywords brain development, nerve regeneration, obesity, depression, gender difference, and hypothalamus. Unlike the first two groups this group explores some functional diseases mainly the functional heterogeneity of microglia and its effects on synaptic pruning and nerve regeneration. The fourth group is astrocytes and neuropathic pain (cluster#4) suggesting that the interaction between microglia and astrocytes greatly influences pain. At the same time combined with other keywords in this group such as minocycline, spinal cord injury, and neurons it can be speculated that this group is involved in the mechanism and treatment of neuropathic pain caused by diseases such as spinal cord injury and implies the potential for using minocycline
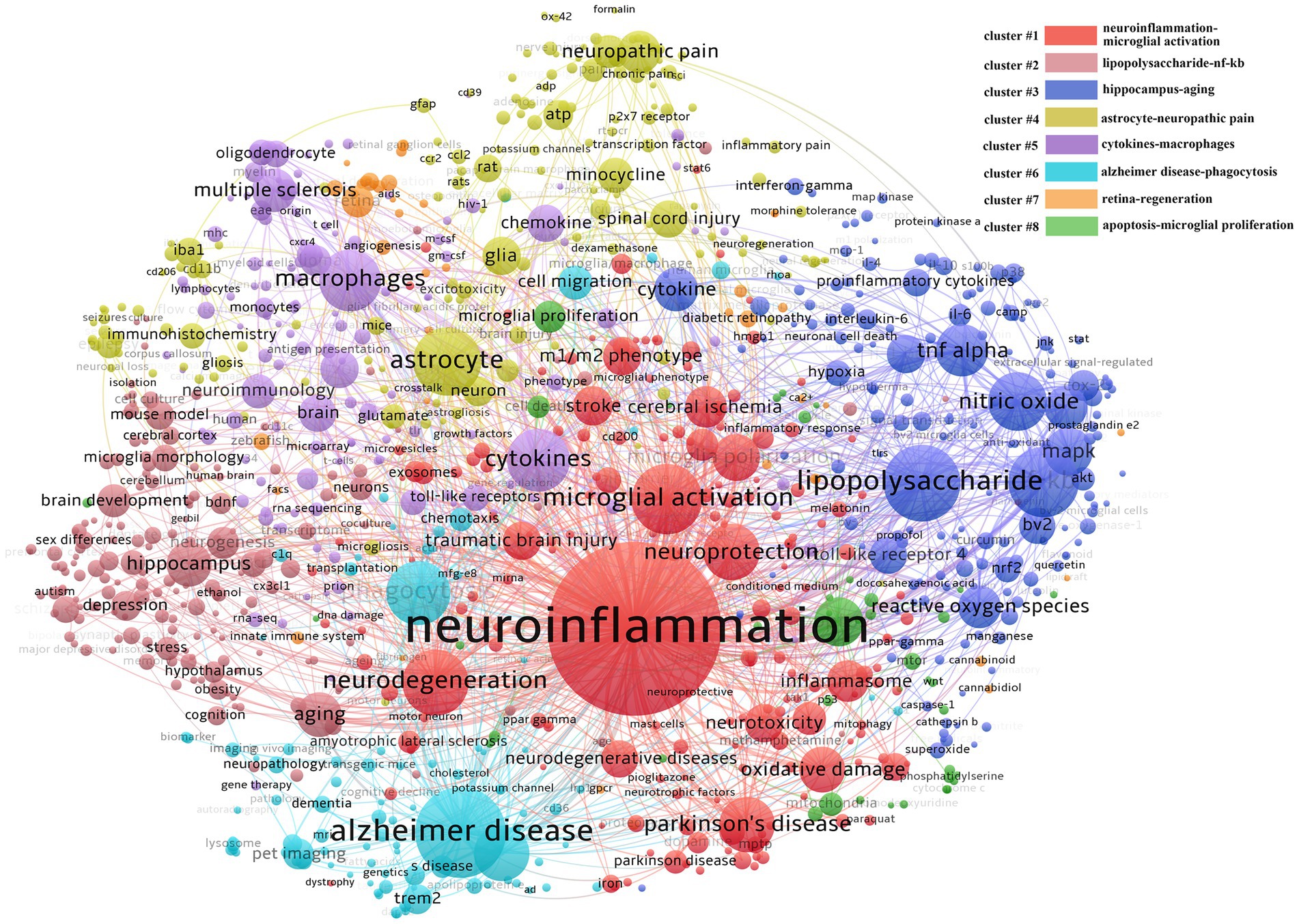
Figure 6. Keywords co-word network and clustering in the field of microglia research. The node size reflects the co-word frequency, the link represents the co-word intensity, different colors represent the clustering results of the co-word network, and the closer the relationship is with the same color.
The fifth group is cytokines and macrophages (cluster#5), which also involves keywords such as multiple sclerosis, oligodendrocytes, neuro-immunity, and chemokines. It suggests macrophage-oligodendrocyte interaction plays a vital role in the pathogenesis and progression of multiple sclerosis. The sixth group is AD and phagocytosis (cluster#6), and the keywords amyloid protein, Trem2, and pet imaging are also involved in the group, indicating that AD has received extensive attention. Currently, the disease is related to the deposition of amyloid protein, so the study of the phagocytosis mechanism of microglia is the mainstream direction. At the same time, the detection and observation of amyloid protein are important for disease diagnosis and therapeutic effect evaluation, so detecting amyloid protein by pet imaging under various molecular markers is very important. The seventh group is the retina and regeneration (cluster#7), with relatively little but professional research. The research object is the retina; combined with zebrafish, diabetic retinopathy, age-related macular degeneration, and muller cells, we can see that the primary research content of this group is the role of retinal microglia in various retinal-related diseases and the exploration of improving nerve regeneration by regulating microglia. The eighth group is the most miniature set, which is related to apoptosis and proliferation (cluster#8), and its group also involves mitochondria, glycolysis, OGD, cell cycle, and cell survival, suggesting that this group is mainly involved in the internal molecular regulation of microglial survival, proliferation, or energy metabolism.
The clustering result of CiteSpace is 9 clusters in timeline view (Supplementary Figure S1), similar to that of VOSviewer but different in that apoptosis and glycolysis clustering are classified into the same group while exosomes belong to the first group in VOSviewer. This difference suggests that the clustering results for these keywords have a high degree of credibility. The timeline shows that each cluster was studied in 2002 and continued to 2021 after continuous development. To further explore the keywords’ evolution, burstness detection was performed. We finally got 228 bursts and made a chart based on the number of burst words each year. According to the graph (Figure 7), there was a spike in the number of keywords from 2002 to 2003, followed by a long period of silence from 2004 to 2017, and then a sharp increase after 2017. The top 30 keywords were then intercepted (Figure 8). We discovered that the 2002 keywords that suddenly increased were primarily related to the inflammatory process; the 2003–2009 keywords are the expansion of the 2002 keywords. Since 2015, there has been a significant shift in focus away from the typical study of microglia pro-inflammatory activation toward the regulation of the M1/M2 phenotype and a greater focus on the gender differences of microglia, phagocytosis (Trem2), and the exosome. We also explored the keywords that lasted until 2021 and found that retinal generation/phenotype remained hot for 5 years, while sex difference/Trem2/zebrafish/RNA-seq/exosome/inflammasome/microglial polarization was the focus of research in the past 5 years (Supplementary Figure S2).
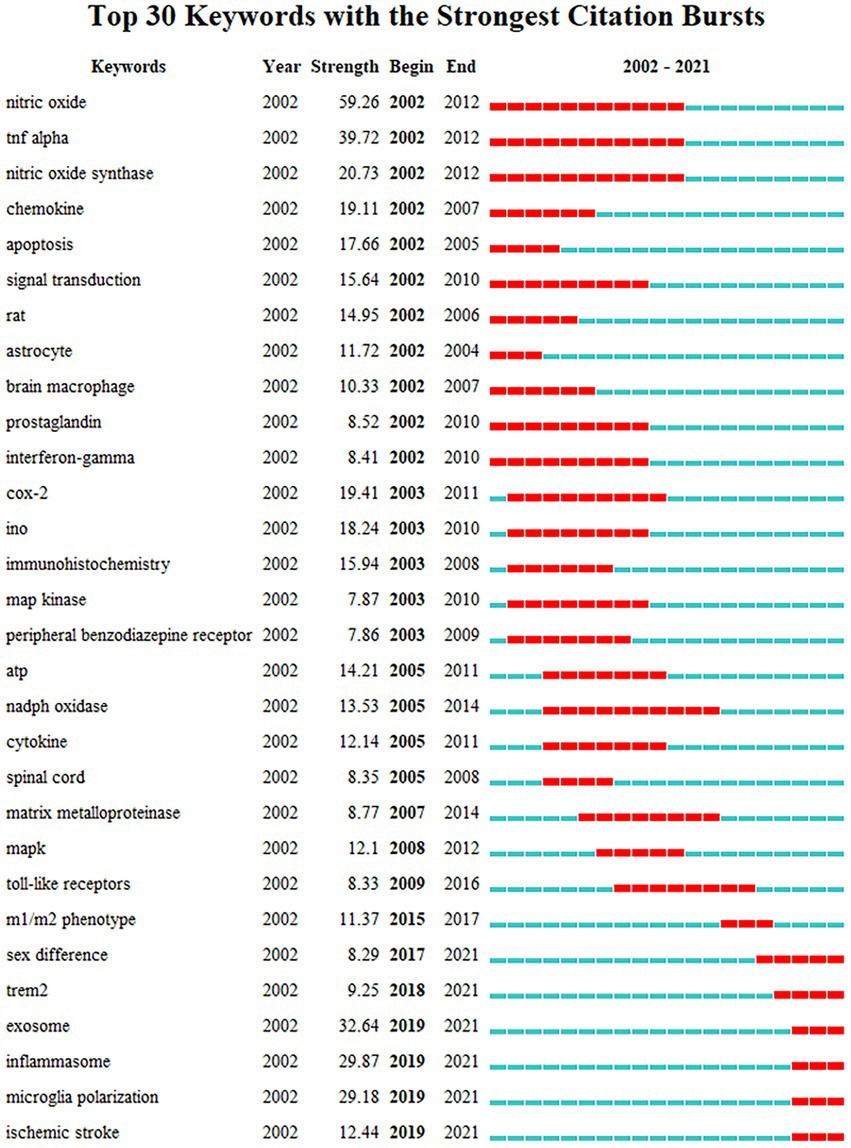
Figure 8. The top 30 burst of keywords analyzed by CiteSpace, “strength” represents the intensity of the burst, “begin” represents the beginning year of the burst, “end” indicates the ending year of the burst, red dotted line indicates the duration of the burst. The blue line indicates the entire period from 2002 to 2021.
We further investigate which keywords have a high citation intensity, which may indicate how significant the literature containing the keyword is. It also suggests that this article’s author is an authority on the subject. These keywords, which included exosome, microglial polarization, pet imaging, CSF1R, synaptic pruning, glycolysis, microglial heterogeneity, and gender differences, were discovered to be primarily concentrated in cluster 1/2/5/6, with a focus on human, chronic stress, aging, neurodegenerative diseases, and nerve regeneration (Table 4, keywords B).
Co-cited analysis of references
Highly cited literature is an essential source of knowledge in a research field, which reflects the research frontier and level in this field and is an important basis for exploring the research context and development direction. According to the analysis of cited literature by CiteSpace, there are 284,695 articles, 1966 nodes, and 12,527 edges. After clustering the cited networks, 17 clusters were obtained (Figure 9; Supplementary Table S2) and labeled with title words or keywords from the citing articles. Silhouette >0.7 in these clusters denotes the plausibility of the clustering findings. From the table, all the clusters are good. These clusters are mainly disease-related (stroke, neuropathic pain, AD, PD, depression, glioma, retina, cardiac arrest, TBI), cell communication-related (synapse, astrocytes), research technology-related (microglial depletion), and mechanism-related (glycolysis, DNA methylation). We further analyzed this cluster’s timeline map (Supplementary Figure S3) and found that stroke, chemokine, neuropathic pain, PD, and AD started earlier. Then came the synaptic pruning of microglia, followed by microglial depletion, depression, glioma, and retina.
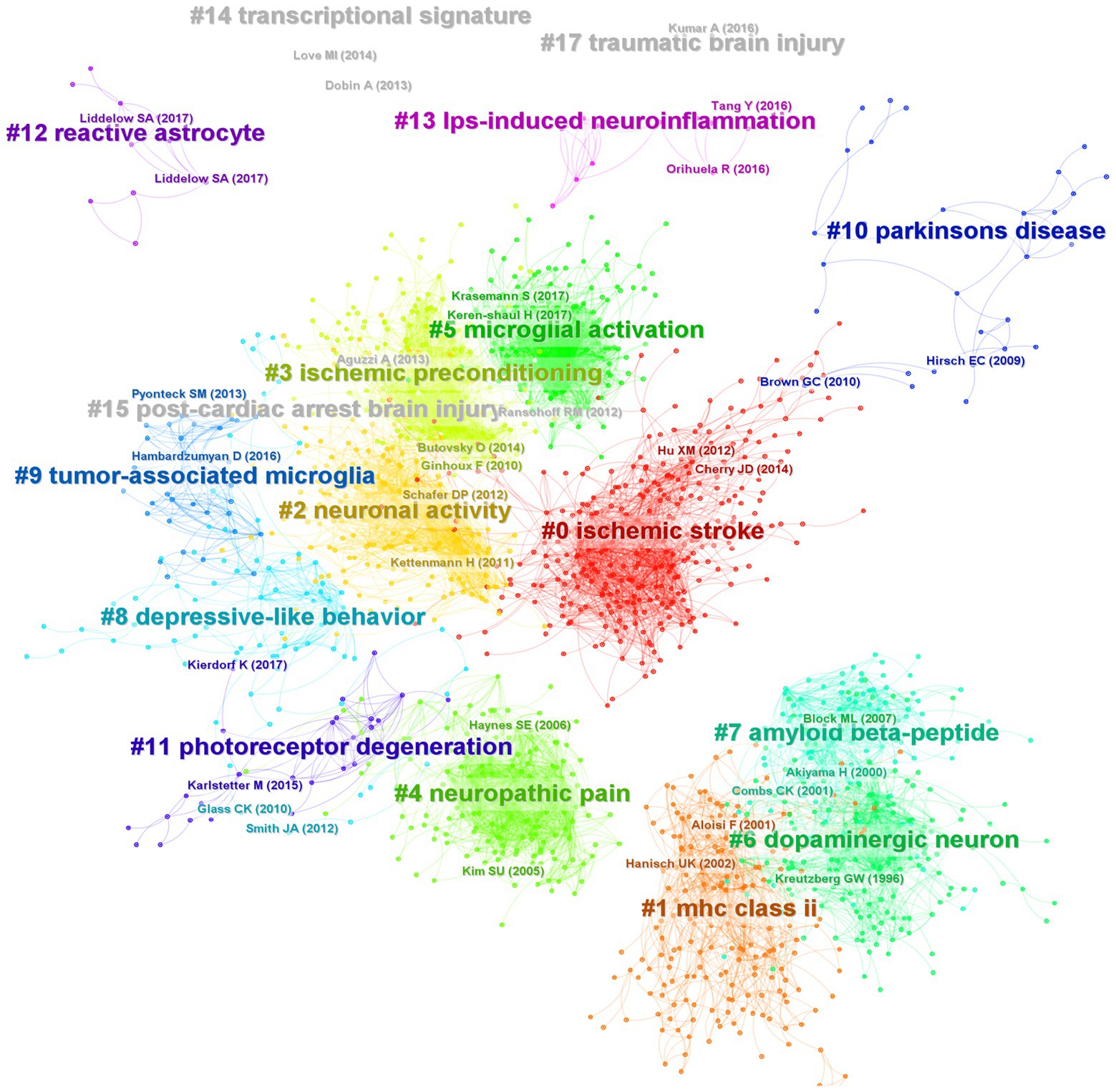
Figure 9. Co-citation network and clustering constructed by references. Each node represents a reference, the link between nodes represents the co-citation intensity, and different colors represent different clusters.
In order to understand which articles have a significant influence on this field, we measure them on three dimensions: citation frequency, burstness, and sigma value. Sigma value is the comprehensive evaluation result of betweenness centrality and burstness. Therefore, we use the sigma value to include betweenness centrality attributes. After selecting and summarizing the top 10 articles for each dimension, 19 influential articles were obtained and combined into one table or graph (Table 5; Figure 10). Among them, two articles by Ginhoux F 2010 in Cluster#3 and Keren-Shaul H 2017 in Cluster#5 appeared in three dimensions, indicating their pivotal positions. The long-running controversy was finally resolved in 2010 by Ginhoux F, who, using the lineage tracing method, established that microglia descended from primitive macrophages of the yolk sac in E8. Keren-Shaul H 2017 indicated that microglia in AD changed from resting microglia to the intermediate and disease-related states through two sequential activations (Trem2-independent and Trem2-dependent) by single-cell sequencing, which may play a protective role in AD.
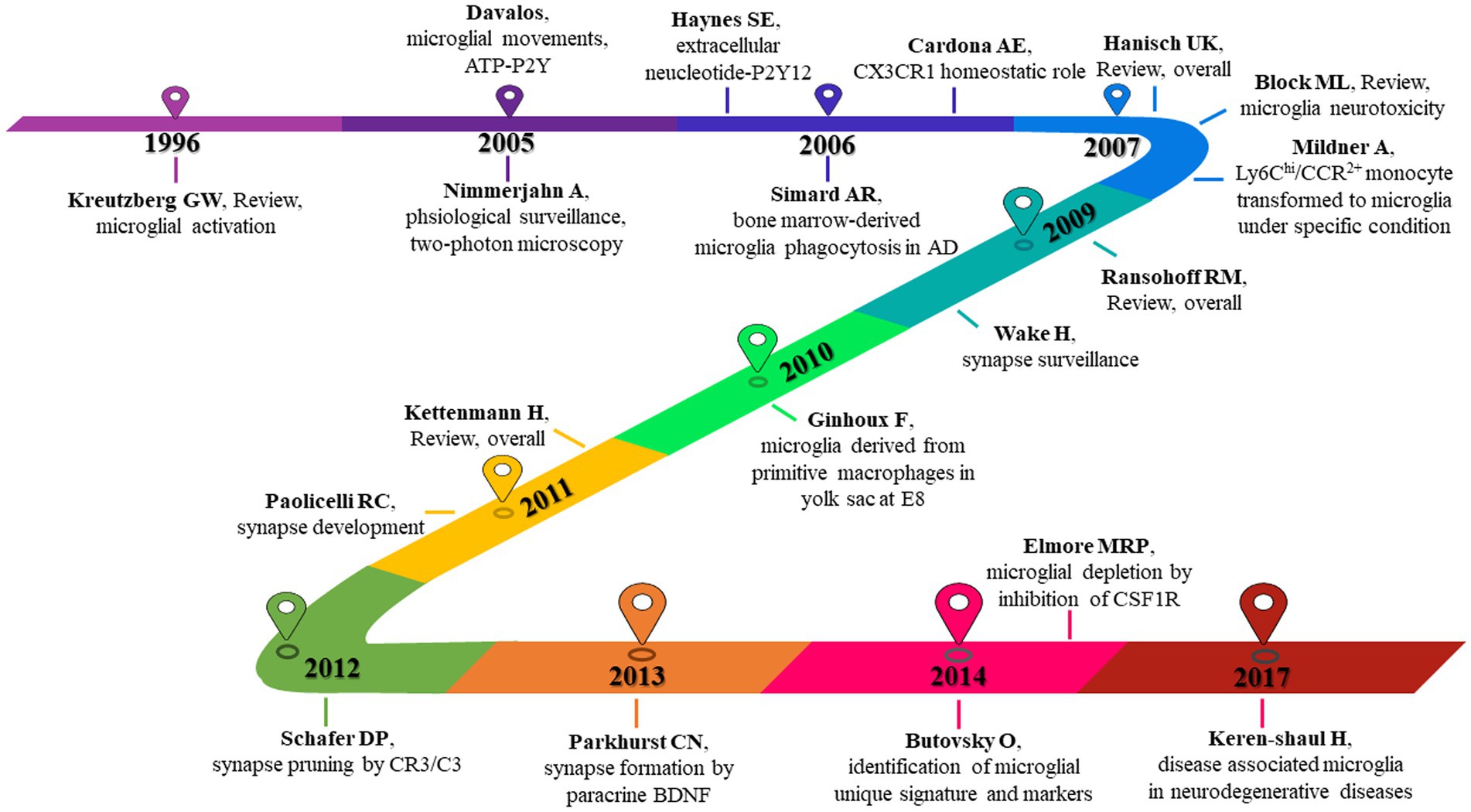
Figure 10. 19 high-impact articles exhibited in the time chart. Nineteen articles were judged comprehensively by the number of publications, burstness, betweenness centrality, and sigma value. The figure lists the author, year, and main findings.
Hanisch UK 2007 in Cluster # 2 and Kettenmann H 2011 in Cluster # 2 provide comprehensive information about microglia’s origin, development, perception, and function. Block ML 2007 in Cluster # 6 reviews the literature on neuronal damage or death caused by the overactivation of microglia before 2007. Paolicelli RC 2011 in Cluster # 2 describes the involvement of microglia in synaptic pruning during nerve development. Using the two-photon imaging technique, Nimmerjahn A 2005 in Cluster # 2 observed microglia dynamically monitoring the surrounding environment. Additionally, microglia can move to tissue after tissue injury, including its processes and cell bodies, and this movement is mediated by ATP-P2Y, as reported by Davalos D. (2005) in Cluster # 2. Wake H 2009 in Cluster # 2 described how microglia could dynamically monitor synapses under physiological circumstances and clear damaged synapses during cerebral ischemia.
By comparing peripheral monocytes and microglia, Butovsky O. (2014) in Cluster # 3 discovered the distinct molecular phenotype of microglia and that TGF-β plays a crucial role in their development and is essential for in vitro culture. Schafer DP 2012 in Cluster # 2 confirmed that microglia could prune the synapses of neurons during development through the CR3/C3 signaling pathway, which depends on neuronal activity. Parkhurst CN 2013 in Cluster # 2 confirmed that microglia could promote learning-related synaptic formation by paracrine BDNF. Elmore MRP 2014 in Cluster # 3 described the importance of CSF1R in microglia survival. It proposed that PLX3397 can be used as an effective way to remove microglia, creating a convenient way for the study of microglia. Ransohoff RM 2009 in Cluster # 2 gave a detailed and complete review of microglia. Kreutzberg GW 1996 in Cluster # 6 reviewed microglial activators, signal pathways and cellular communication. Microglia P2Y12 receptor can sense extracellular nucleotide signals and then undergo morphological changes and migration, which may be the first step in microglial activation, according to Haynes SE 2006 in Cluster # 4. Mildner A 2007 in Cluster # 0 showed that only under specific host conditions can blood-derived Ly-6ChiCCR2+ mononuclear cells enter the brain and transform into microglia. The significance of microglia CX3CR1 for microglia homeostasis was discussed by Cardona AE 2006 in Cluster # 0. The lack of CX3CR1 will make microglia produce obvious neurotoxicity in both physiological and pathological conditions. Microglia derived from bone marrow are crucial for clearing plaque in AD, according to Simard AR 2006 in Cluster # 0.
Discussion
In this paper, we systematically explore the articles related to the study of microglia from 2002 to 2021 by bibliometrics. The number of articles published grew slowly between 2002 and 2011. During these 10 years, 187 articles increased, a growth rate of 86.2%, while it multiplied after 2011, with an increase of 1,213 articles, a growth rate of 300%, and continued its momentum until 2021. People R China has contributed the most, barring the notable rise in the number of articles published by each nation after 2011 (Supplementary Figure S4), when we examine the proportion of articles from different countries/regions each year. In discipline distribution, neurology, immunity, and cell biology-related disciplines account for the most significant proportion. However, in recent years, interdisciplinary phenomena such as physics and material science cannot be ignored, which is expected to open a new direction for studying microglia. Twelve Thousand Eight Hundred thirteen articles published in 1336 journals, and the four journals of Glia, J Neuroinflamm, J Neurochem, J Neurosci ranked among the top 10 in terms of publication volume and total citation frequency analysis, suggesting that these four journals play an important role in microglial research. At the same time, well-known journals such as P Natl Acad Sci United States, Nat Neurosci, Science, Nature have also played an essential role.
The number of articles, citation intensity, burstness, betweenness centrality, and cooperative network can be used to measure the status and contribution of countries, institutions, and authors in a specific research field (Agarwal et al., 2016). According to the analysis of the countries, the United States, Germany, and England hold significant positions in all three major cooperative groups and are the leaders of these groups, suggesting that these three countries/regions are the main foundations for microglial research. Although People R China, Japan, and South Korea produce many papers, the number of citations is low, so they still need to continually raise the caliber and level of their research—which has enormous growth potential. From the analysis of institutions, Harvard Univ, Kyushu Univ and Shanghai Jiao tong Univ have the highest volume of papers. In comparison, only Harvard Univ has a betweenness center greater than 0.1, and Harvard Univ and Univ Freiburg are among the top two in terms of citation intensity. These dimensions suggest Harvard Univ is the core institution leading global microglial research. At the same time, the Univ Freiburg, Kyushu Univ, and Shanghai Jiao tong Univ are also in a critical position, and these institutions are also key nodes in the cooperative network. Regarding the authors, Prinz Marco and Kettenmann Helmut are both from Germany and hold prominent positions in terms of the volume of articles they have published and the frequency of citations, indicating that German researchers are influential in the field of microglia research. In the citation analysis, Streit Wj and Hanisch United Kingdom rank in the top two, which deserves the attention of researchers. It is also worth cautioning against the misunderstanding of the “Citation Burst” (like Figure 3C), which denotes an abrupt rise in the frequency of citations over time in a body of literature. Since Germany is always cited frequently and broadly, it is hard to get a citation burst according to the burstness algorithm. In this dimension, burstness is suitable for detecting the emerging group with a sudden increase in citation.
We tried to use CiteSpace and VOSviewer to construct the knowledge domains of microglia. First, we got 14,742 keywords and counted the changes in the number burst keywords over time. Except for the initial period of 2002–2003, when the volume of publications was lower, the burst of keywords was also lower. In contrast, as the number of articles rose sharply in 2012, the burst of keywords showed a transient increase before declining. Until the number of articles reached a steady growth stage after 2017, the number of burst keywords had increased significantly, suggesting that the burst of keywords has a certain lag. Then, from the perspective of specific content, neuroinflammation is not only the most extensive topic of microglial research but also a hot topic (Cherry et al., 2014; Leng and Edison, 2021). Numerous studies have been conducted on a variety of illnesses, including stroke (Bai et al., 2020), neurodegenerative diseases (Krasemann et al., 2017; Kwon and Koh, 2020; Leng and Edison, 2021), and TBI (Jassam et al., 2017), and they have all largely focused on the neuronal damage caused by inflammatory factors, oxidative stress, and lipopolysaccharide as a traditional activator model (Troutman et al., 2021). The concept of microglia polarization, or M1 and M2, has also gradually developed and has become the focus of microglial research (Ajmone-Cat et al., 2013; Byles et al., 2013; Joers et al., 2017; Bai et al., 2020; Mesquida-Veny et al., 2021). However, an increasing number of studies have revealed that the two phenotypes obtained from in vitro experiments are not distinct in vivo and are immutable in different diseases and periods of the same disease (Hu et al., 2015; Ransohoff, 2016; Mesquida-Veny et al., 2021). Microglia are frequently mixed, exhibiting both phenotypes. The idea of physiological state heterogeneity (Li et al., 2019; Cadiz et al., 2022), and gender differences (Fiore et al., 2022; Tansley et al., 2022; Ugidos et al., 2022) has slowly emerged in recent years, creating a new foundation for our complete understanding of microglia. At the same time, single-cell sequencing technology has also opened a new door for this (Butovsky et al., 2014). Disease-related microglia are gradually revealed (Keren-Shaul et al., 2017), which provides new ideas for solving this phenotypic problem. According to the clustering findings, the third group of research topics, which also includes the issue of nerve regeneration (Diaz-Aparicio et al., 2016), focuses on the origin, development, renewal, and death of microglia as well as their relationship with individual development and death (Ginhoux et al., 2010; Elmore et al., 2014; Oosterhof et al., 2018). The primary diseases involved in this problem are brain development (Bohlen et al., 2019), depression (Yirmiya et al., 2015), stress (Frank et al., 2018), autism (Sarn, 2021), and cognitive impairment (Feng et al., 2017). In this process, the effects of microglia on neurite development, synaptic remodeling, and nerve regeneration are the main research topics rather than neuroinflammation. Other than the two major research areas mentioned above, several diseases are being studied, including neuropathic pain (microglial-astrocyte communication) (Yu et al., 2019), multiple sclerosis (macrophage-oligodendrocyte communication) (Chiu et al., 2013), AD (Trem2-phagocytosis) (Boche and Gordon, 2022), and retinopathy (neurogenesis) (Okunuki et al., 2018; Wang et al., 2020), in which the unique role of microglia should be noted. Neuroinflammation still runs through the study of these diseases. Finally, it is worth mentioning that the minor groups, mitochondria, and glycolysis groups, suggest that attention has been paid to the transformation of energy metabolism in the activation process of microglia, which is called metabolic reprogramming (Baik et al., 2019; Bernier et al., 2020; Gu et al., 2021; Luo et al., 2021). It might also be a reference point for microglia with abnormal phenotypic change and function. According to research hotspots in recent years, the extraction of keywords, and protruding words from highly cited articles, microglia pyroptosis, exosomes, mesenchymal stem cells, and microglial imaging (PK11195, TSPO, pet imaging) are also receiving more attention, which can compensate for the dearth of clustering research (Delpech et al., 2019; Voet et al., 2019; Kreisl et al., 2020).
Literature is the basis of knowledge structures. We will break this into five sections to make it easier to understand. Firstly, the review articles, which are (Kreutzberg, 1996; Block et al., 2007; Hanisch and Kettenmann, 2007; Ransohoff and Perry, 2009; Kettenmann et al., 2011), can help us to understand the basic research situation and progress of microglia quickly. The second is the monitoring function of microglia. According to Nimmerjahn et al. (2005) and Davalos et al. (2005), microglia can move in a specific direction when the P2Y receptor (especially P2Y12, Haynes et al., 2006) is active. This function of microglia includes monitoring and detecting the microenvironment under physiological or pathological conditions.
Furthermore, Cardona AE 2006 confirmed the critical function of the CX3CR1 receptor in microglia, maintaining the homeostasis of the microenvironment. The third is the origin of microglia. Simard et al. (2006) found that bone marrow-derived microglia could phagocytize plaques in AD. Mildner et al. (2007) found that peripheral monocytes could colonize intracranial microglia only under specific conditions (such as blood–brain barrier injury). Until 2010, Ginhoux et al. (2010) used lineage tracing to confirm that microglia were transformed from yolk sac primordial macrophages on the eighth day of the embryonic stage, which resolved the dispute for many years. Then comes the synaptic pruning function of microglia. In 2009, Wake et al. (2009) found that microglia have a synaptic monitoring function. Paolicelli et al. (2011) confirmed that microglia play an important role in synaptic development. Schafer et al. (2012) and Parkhurst et al. (2013) found that microglia can achieve synaptic pruning function through CR3/C3 and promote synaptic formation by paracrine BDNF. Finally, a recent study, Butovsky et al. (2014), used single-cell sequencing technology to identify specific microglia genes and molecular markers, but these were not expressed in monocytes or other macrophages. Elmore et al. (2014), who used PLX3397 to target microglial CSF1R and deplete microglia, opened a more direct path for the study of microglia function. Keren-Shaul et al. (2017) defines a group of disease-related microglia in neurodegenerative diseases. According to our analysis of the top 30 burst literature (Supplementary Figure S5), the two recent articles, Krasemann et al. (2017) and Hammond et al. (2019), also fall under the use of single-cell sequencing technology to define the distinctive phenotype of microglia in the course of the disease. This direction is more likely to provide in-depth insights into the mechanism of microglial phenotype and disease association and extend to a broader disease spectrum. We also performed a clustering analysis of the co-cited documents to enhance the creation of the knowledge domains using keywords. Only the transcriptional signature/DNA methylation, post-cardiac arrest brain injury/cardiac arrest, and TBI/TBI formed new clusters in the clustering view, where the cited documents formed 17 clusters, the majority of which are related to keyword clustering. It was proposed that the study of microglia and the development of specific disease research increasingly rely on single-cell sequencing (De Vlaminck et al., 2022; Marsh et al., 2022; Sankowski et al., 2022) and epigenetic regulation (Martins-Ferreira et al., 2021).
This paper also has some limitations. First, because this paper only considers literature published between 2002 and 2021, there may be some omissions in the important literature, especially those published within the last 3 years, because the related analysis of the number of citations may be impacted by the publication time; for instance, Wendeln’s 2018 research on immune memory and microglia priming (Wendeln et al., 2018), and Bottcher’s 2019 research on the sequencing of human microglia (Böttcher et al., 2019). Secondly, for accuracy, only the author’s keywords are used in keyword analysis because some articles may not have author keywords, meaning some information may be omitted.
Finally, according to the knowledge domains and dynamic evolution we analyzed, some possible future research directions can also be put forward. Mainly including: 1. Microglial development (Wurm et al., 2021; Iyer et al., 2022) and phenotypes in physiological or pathological conditions, also involving microglial heterogeneity in spatial and temporal (Masuda et al., 2019), gender (Dockman et al., 2022; Fiore et al., 2022), or other dimensions. 2. The critical role of communication/conversation between microglia-neurons (Cserép et al., 2021) and microglia-astrocytes (Han et al., 2021) under ontogeny, physiology, and pathology. 3. Studies on the functional mechanism of microglia by metabolic reprogramming (Minhas et al., 2021), exosomes (Zhu et al., 2022), and epigenetic modification (Martins-Ferreira et al., 2021). 4. In terms of animal models, zebrafish (Silva et al., 2021) may be given more attention. 5. More extensive applications of pet imaging (Kreisl et al., 2020), two-or multi-photon imaging (Scheiblich et al., 2021), and single-cell sequencing (Sankowski et al., 2022) will continue to contribute to the study of microglia. 6. The disease spectrum of microglial study will be broadened, and the popularity of neurodegeneration (Silvin et al., 2022), stroke (Beuker et al., 2022), psychiatric disorders (Fang et al., 2021), and TBI (Luo et al., 2021) research will continue. 7. It is imperative to define, standardize, and unify the nomenclature of microglia (Paolicelli et al., 2022), which will lay a solid foundation for the study of microglia.
Conclusion
In this study, we explore the study of microglia from 2002 to 2021 using bibliometrics methods in conjunction with CiteSpace and VOSviewer software. The fundamental data, knowledge domains, evolution, and emerging trends were built with the intention of supplying pertinent researchers with concepts to comprehend and delve deeply into the field of microglia research.
Data availability statement
The original contributions presented in the study are included in the article/Supplementary material, further inquiries can be directed to the corresponding authors.
Author contributions
GL contributed to the conception and performed the experiment of the study. TL performed the experiment and contributed significantly to the analysis and manuscript preparation. AY performed the data analyses. XZ helped write the manuscript. SQ and WF helped perform the analysis with constructive discussions. All authors contributed to the article and approved the submitted version.
Funding
This study was supported by grants from The National Nature Science Foundation of China (NSFC): No. 82101398.
Acknowledgments
Thanks for my wife, Lin Huang’s spiritual encouragement and part of the chart for my work.
Conflict of interest
The authors declare that the research was conducted in the absence of any commercial or financial relationships that could be construed as a potential conflict of interest.
Publisher’s note
All claims expressed in this article are solely those of the authors and do not necessarily represent those of their affiliated organizations, or those of the publisher, the editors and the reviewers. Any product that may be evaluated in this article, or claim that may be made by its manufacturer, is not guaranteed or endorsed by the publisher.
Supplementary material
The Supplementary material for this article can be found online at: https://www.frontiersin.org/articles/10.3389/fnagi.2022.1057214/full#supplementary-material
Abbreviations
AD, Alzheimer’s disease; PD, Parkinson’s disease; TBI, traumatic brain injury; ROS, reactive oxygen species; NO, nitric oxide; IL-1β, interleukin-1β; TNF-α, tumor necrosis factor-α; Avg. norm. citations, averaged normalized number of citations; MAPK, mitogen activated protein kinase; C.C., citation counts; Bur., burstness intensity; Sig., sigma value.
References
Agarwal, A., Durairajanayagam, D., Tatagari, S., Esteves, S. C., Harlev, A., Henkel, R., et al. (2016). Bibliometrics: tracking research impact by selecting the appropriate metrics. Asian J. Androl. 18, 296–309. doi: 10.4103/1008-682X.171582
Ajami, B., Bennett, J. L., Krieger, C., Tetzlaff, W., and Rossi, F. M. V. (2007). Local self-renewal can sustain CNS microglia maintenance and function throughout adult life. Nat. Neurosci. 10, 1538–1543. doi: 10.1038/nn2014
Ajmone-Cat, M. A., Mancini, M., De Simone, R., Cilli, P., and Minghetti, L. (2013). Microglial polarization and plasticity: evidence from organotypic hippocampal slice cultures. Glia 61, 1698–1711. doi: 10.1002/glia.22550
Bai, Q., Xue, M., and Yong, V. W. (2020). Microglia and macrophage phenotypes in intracerebral haemorrhage injury: therapeutic opportunities. Brain 143, 1297–1314. doi: 10.1093/brain/awz393
Baik, S. H., Kang, S., Lee, W., Choi, H., Chung, S., Kim, J. I., et al. (2019). A breakdown in metabolic reprogramming causes microglia dysfunction in Alzheimer’s disease. Cell Metab. 30, 493–507.e6. doi: 10.1016/j.cmet.2019.06.005
Bennett, F. C., Bennett, M. L., Yaqoob, F., Mulinyawe, S. B., Grant, G. A., Hayden Gephart, M., et al. (2018). A combination of ontogeny and CNS environment establishes microglial identity. Neuron 98, 1170–1183.e8. doi: 10.1016/j.neuron.2018.05.014
Bernier, L. P., York, E. M., and Mac Vicar, B. A. (2020). Immunometabolism in the brain: how metabolism shapes microglial function. Trends Neurosci. 43, 854–869. doi: 10.1016/j.tins.2020.08.008
Beuker, C., Schafflick, D., Strecker, J. K., Heming, M., Li, X., Wolbert, J., et al. (2022). Stroke induces disease-specific myeloid cells in the brain parenchyma and pia. Nat. Commun. 17:945. doi: 10.1038/s41467-022-28593-1
Block, M. L., Zecca, L., and Hong, J. S. (2007). Microglia-mediated Neurotoxicity: Uncovering the Molecular Mechanisms. Nat. Rev. Neurosci. 8, 57–69. doi: 10.1038/nrn2038
Boche, D., and Gordon, M. N. (2022). Diversity of transcriptomic microglial phenotypes in aging and Alzheimer’s disease. Alzheimers Dement. 18, 360–376. doi: 10.1002/alz.12389
Bohlen, C. J., Friedman, B. A., Dejanovic, B., and Sheng, M. (2019). Microglia in brain development, homeostasis, and Neurodegeneration. Annu. Rev. Genet. 53, 263–288. doi: 10.1146/annurev-genet-112618-043515
Böttcher, C., Schlickeiser, S., Sneeboer, M. A. M., Kunkel, D., Knop, A., Paza, E., et al. (2019). Human microglia regional heterogeneity and phenotypes determined by multiplexed single-cell mass cytometry. Nat. Neurosci. 22, 78–90. doi: 10.1038/s41593-018-0290-2
Butovsky, O., Jedrychowski, M. P., Moore, C. S., Cialic, R., Lanser, A. J., Gabriely, G., et al. (2014). Identification of a unique TGF-beta-dependent molecular and functional signature in microglia. Nat. Neurosci. 17, 131–143. doi: 10.1038/nn.3599
Byles, V., Covarrubias, A. J., Ben-Sahra, I., Lamming, D. W., Sabatini, D. M., Manning, B. D., et al. (2013). The TSC-mTOR pathway regulates macrophage polarization. Nat. Commun. 4:2834. doi: 10.1038/ncomms3834
Cadiz, M. P., Jensen, T. D., Sens, J. P., Zhu, K., Song, W. M., Zhang, B., et al. (2022). Culture shock: microglial heterogeneity, activation, and disrupted single-cell microglial networks in vitro. Mol. Neurodegener. 28:26. doi: 10.1186/s13024-022-00531-1
Chen, C. (2004). Searching for intellectual turning points: progressive knowledge domain visualization. Proc. Natl. Acad. Sci. U. S. A. 101, 5303–5310. doi: 10.1073/pnas.0307513100
Chen, C. (2006). Cite space II: detecting and visualizing emerging trends and transient patterns in scientific literature. J. Am. Soc. Inf. Sci. Technol. 57, 359–377. doi: 10.1002/asi.20317
Chen, C., Dubin, R., and Kim, M. C. (2014). Emerging trends and new developments in regenerative medicine: a scientometric update (2000 – 2014). Expert. Opin. Biol. Ther. 14, 1295–1317. doi: 10.1517/14712598.2014.920813
Chen, Y., Li, Y., Guo, L., Hong, J., Zhao, W., Hu, X., et al. (2021). Bibliometric analysis of the Inflammasome and Pyroptosis in brain. Front. Pharmacol. 11:15. doi: 10.3389/fphar.2020.626502
Chen, C., Song, M., and Heo, G. E. (2018). A scalable and adaptive method for finding semantically equivalent cue words of uncertainty. J. Informetr. 12, 158–180. doi: 10.1016/j.joi.2017.12.004
Cherry, J. D., Olschowka, J. A., and O’Banion, M. K. (2014). Neuroinflammation and M2 microglia: the good, the bad, and the inflamed. J. Neuroinflammation 11:98. doi: 10.1186/1742-2094-11-98
Chiu, I. M., Morimoto, E. T., Goodarzi, H., Liao, J. T., O’Keeffe, S., Phatnani, H. P., et al. A neurodegeneration-specific gene-expression signature of acutely isolated microglia from an amyotrophic lateral sclerosis mouse model. Cell Rep.. (2013). 4(2:385–401. doi: 10.1016/j.celrep.2013.06.018
Cserép, C., Pósfai, B., and Dénes, Á. (2021). Shaping neuronal fate: functional heterogeneity of direct microglia-neuron interactions. Neuron 109, 222–240. doi: 10.1016/j.neuron.2020.11.007
Davalos, D., Grutzendler, J., Yang, G., Kim, J. V., Zuo, Y., Jung, S., et al. (2005). ATP mediates rapid microglial response to local brain injury in vivo. Nat. Neurosci. 8, 752–758. doi: 10.1038/nn1472
De Vlaminck, K., Van Hove, H., Kancheva, D., Scheyltjens, I., Pombo Antunes, A. R., Bastos, J., et al. (2022). Differential plasticity and fate of brain-resident and recruited macrophages during the onset and resolution of neuroinflammation. Immunity 55, 2085–2102.e9. doi: 10.1016/j.immuni.2022.09.005
Delpech, J. C., Herron, S., Botros, M. B., and Ikezu, T. (2019). Neuroimmune crosstalk through extracellular vesicles in health and disease. Trends Neurosci. 42, 361–372. doi: 10.1016/j.tins.2019.02.007
Diaz-Aparicio, I., Beccari, S., Abiega, O., and Sierra, A. (2016). Clearing the corpses: regulatory mechanisms, novel tools, and therapeutic potential of harnessing microglial phagocytosis in the diseased brain. Neural Regen. Res. 11, 1533–1539. doi: 10.4103/1673-5374.193220
Ding, X., and Yang, Z. (2020). Knowledge mapping of platform research: a visual analysis using VOSviewer and cite space. Electron. Commer. Res. 22, 787–809. doi: 10.1007/s10660-020-09410-7
Dockman, R. L., Carpenter, J. M., Diaz, A. N., Benbow, R. A., and Filipov, N. M. (2022). Sex differences in behavior, response to LPS, and glucose homeostasis in middle-aged mice. Behav. Brain Res. 418:113628. doi: 10.1016/j.bbr.2021.113628
Elmore, M. R. P., Najafi, A. R., Koike, M. A., Dagher, N. N., Spangenberg, E. E., Rice, R. A., et al. (2014). Colony-stimulating factor 1 receptor signaling is necessary for microglia viability, unmasking a microglia progenitor cell in the adult brain. Neuron 82, 380–397. doi: 10.1016/j.neuron.2014.02.040
Fang, X., Jiang, S., Wang, J., Bai, Y., Kim, C. S., Blake, D., et al. (2021). Chronic unpredictable stress induces depression-related behaviors by suppressing AgRP neuron activity. Mol. Psychiatry 26, 2299–2315. doi: 10.1038/s41380-020-01004-x
Feng, X., Valdearcos, M., Uchida, Y., Lutrin, D., Maze, M., and Koliwad, S. K. (2017). Microglia mediate postoperative hippocampal inflammation and cognitive decline in mice. JCI Insight 7:e91229. doi: 10.1172/jci.insight.91229
Fiore, N. T., Yin, Z., Guneykaya, D., Gauthier, C. D., Hayes, J. P., D’Hary, A., et al. (2022). Sex-specific transcriptome of spinal microglia in neuropathic pain due to peripheral nerve injury. Glia 70, 675–696. doi: 10.1002/glia.24133
Frank, M. G., Fonken, L. K., Annis, J. L., Watkins, L. R., and Maier, S. F. (2018). Stress disinhibits microglia via down-regulation of CD200R: a mechanism of neuroinflammatory priming. Brain Behav. Immun. 69, 62–73. doi: 10.1016/j.bbi.2017.11.001
Ginhoux, F., Greter, M., Leboeuf, M., Nandi, S., See, P., Gokhan, S., et al. (2010). Fate mapping analysis reveals that adult microglia derive from primitive macrophages. Science 330, 841–845. doi: 10.1126/science.1194637
Gu, C., Wang, F., Zhang, Y. T., Wei, S. Z., Liu, J. Y., Sun, H. Y., et al. (2021). Microglial MT1 activation inhibits LPS-induced neuroinflammation via regulation of metabolic reprogramming. Aging Cell 20:e13375. doi: 10.1111/acel.13375
Hammond, T. R., Dufort, C., Dissing-Olesen, L., Giera, S., Young, A., Wysoker, A., et al. (2019). Single-cell RNA sequencing of microglia throughout the mouse lifespan and in the injured brain reveals complex cell-state changes. Immunity 50, 253–271.e6. doi: 10.1016/j.immuni.2018.11.004
Han, R. T., Kim, R. D., Molofsky, A. V., and Liddelow, S. A. (2021). Astrocyte-immune cell interactions in physiology and pathology. Immunity 54, 211–224. doi: 10.1016/j.immuni.2021.01.013
Hanisch, U. K., and Kettenmann, H. (2007). Microglia: active sensor and versatile effector cells in the normal and pathologic brain. Nat. Neurosci. 10, 1387–1394. doi: 10.1038/nn1997
Haynes, S. E., Hollopeter, G., Yang, G., Kurpius, D., Dailey, M. E., Gan, W. B., et al. (2006). The P2Y12 receptor regulates microglial activation by extracellular nucleotides. Nat. Neurosci. 9, 1512–1519. doi: 10.1038/nn1805
Hou, J., Su, H., Kuang, X., Qin, W., Liu, K., Pan, K., et al. (2022). Knowledge domains and emerging trends of osteoblasts-osteoclasts in bone disease from 2002 to 2021: a Bibliometrics analysis and visualization study. Front. Endocrinol. 13:922070. doi: 10.3389/fendo.2022.922070
Hu, X., Leak, R. K., Shi, Y., Suenaga, J., Gao, Y., Zheng, P., et al. (2015). Microglial and macrophage polarization—new prospects for brain repair. Nat. Rev. Neurol. 11, 56–64. doi: 10.1038/nrneurol.2014.207
Iyer, H., Shen, K., Meireles, A. M., and Talbot, W. S. (2022). A lysosomal regulatory circuit essential for the development and function of microglia. Sci. Adv. 8:eabp 8321. doi: 10.1126/sciadv.abp8321
Jassam, Y. N., Izzy, S., Whalen, M., McGavern, D. B., and El Khoury, J. (2017). Neuroimmunology of traumatic brain injury: time for a paradigm shift. Neuron 95, 1246–1265. doi: 10.1016/j.neuron.2017.07.010
Joers, V., Tansey, M. G., Mulas, G., and Carta, A. R. (2017). Microglial phenotypes in Parkinson’s disease and animal models of the disease. Prog. Neurobiol. 155, 57–75. doi: 10.1016/j.pneurobio.2016.04.006
Keren-Shaul, H., Spinrad, A., Weiner, A., Matcovitch-Natan, O., Dvir-Szternfeld, R., Ulland, T. K., et al. (2017). A unique microglia type associated with restricting development of Alzheimer’s disease. Cells 169, 1276–1290.e17. doi: 10.1016/j.cell.2017.05.018
Kettenmann, H., Hanisch, U. K., Noda, M., and Verkhratsky, A. (2011). Physiology of microglia. Physiol. Rev. 91, 461–553. doi: 10.1152/physrev.00011.2010
Krasemann, S., Madore, C., Cialic, R., Baufeld, C., Calcagno, N., El Fatimy, R., et al. (2017). The TREM2-APOE pathway drives the transcriptional phenotype of dysfunctional microglia in neurodegenerative diseases. Immunity 47, 566–581.e9. doi: 10.1016/j.immuni.2017.08.008
Kreisl, W. C., Kim, M. J., Coughlin, J. M., Henter, I. D., Owen, D. R., and Innis, R. B. (2020). PET imaging of neuroinflammation in neurological disorders. Lancet Neurol. 19, 940–950. doi: 10.1016/S1474-4422(20)30346-X
Kreutzberg, G. W. (1996). Microglia: a sensor for pathological events in the CNS. Trends Neurosci. 19, 312–318. doi: 10.1016/0166-2236(96)10049-7
Kwon, H. S., and Koh, S. H. (2020). Neuroinflammation in neurodegenerative disorders: the roles of microglia and astrocytes. Transl. Neurodegener. 9:42. doi: 10.1186/s40035-020-00221-2
Leng, F., and Edison, P. (2021). Neuroinflammation and microglial activation in Alzheimer disease: where do we go from here? Nat. Rev. Neurol. 17, 157–172. doi: 10.1038/s41582-020-00435-y
Li, Q., Cheng, Z., Zhou, L., Darmanis, S., Neff, N. F., Okamoto, J., et al. (2019). Developmental heterogeneity of microglia and brain myeloid cells revealed by deep single-cell RNA sequencing. Neuron 101, 207–223. doi: 10.1016/j.neuron.2018.12.006
Li, J., and Liu, J. (2020). Science mapping of tunnel fires: a scientometric analysis-based study. Fire. Technol 56, 2111–2135. doi: 10.1007/s10694-020-00969-z
Liu, Y., and Avello, M. (2021). Status of the research in fitness apps: a bibliometric analysis. Telemat Inform. 57:101506. doi: 10.1016/j.tele.2020.101506
Luo, G., Wang, X., Cui, Y., Cao, Y., Zhao, Z., and Zhang, J. (2021). Metabolic reprogramming mediates hippocampal microglial M1 polarization in response to surgical trauma causing perioperative neurocognitive disorders. J. Neuroinflammation 18:267. doi: 10.1186/s12974-021-02318-5
Ma, Y., Wang, J., Wang, Y., and Yang, G. Y. (2017). The biphasic function of microglia in ischemic stroke. Prog. Neurobiol. 157, 247–272. doi: 10.1016/j.pneurobio.2016.01.005
Marsh, S. E., Walker, A. J., Kamath, T., Dissing-Olesen, L., Hammond, T. R., de Soysa, T. Y., et al. (2022). Dissection of artifactual and confounding glial signatures by single-cell sequencing of mouse and human brain. Nat. Neurosci. 25, 306–316. doi: 10.1038/s41593-022-01022-8
Martins-Ferreira, R., Leal, B., Costa, P. P., and Ballestar, E. (2021). Microglial innate memory and epigenetic reprogramming in neurological disorders. Prog. Neurobiol. 200:101971. doi: 10.1016/j.pneurobio.2020.101971
Masuda, T., Sankowski, R., Staszewski, O., Böttcher, C., Amann, L., Sagar, N., et al. (2019). Spatial and temporal heterogeneity of mouse and human microglia at single-cell resolution. Nature 566, 388–392. doi: 10.1038/s41586-019-0924-x
Mesquida-Veny, F., Del Río, J. A., and Hervera, A. (2021). Macrophagic and microglial complexity after neuronal injury. Prog. Neurobiol. 200:101970. doi: 10.1016/j.pneurobio.2020.101970
Mildner, A., Schmidt, H., Nitsche, M., Merkler, D., Hanisch, U. K., Mack, M., et al. (2007). Microglia in the adult brain arise from Ly-6ChiCCR2+ monocytes only under defined host conditions. Nat. Neurosci. 10, 1544–1553. doi: 10.1038/nn2015
Minhas, P. S., Latif-Hernandez, A., McReynolds, M. R., Durairaj, A. S., Wang, Q., Rubin, A., et al. (2021). Restoring metabolism of myeloid cells reverses cognitive decline in ageing. Nature 590, 122–128. doi: 10.1038/s41586-020-03160-0
Morganti-Kossmann, M. C. (2019). The complexity of neuroinflammation consequent to traumatic brain injury: from research evidence to potential treatments. Acta Neuropathol. 25, 731–755.
Nimmerjahn, A., Kirchhoff, F., and Helmchen, F. (2005). Resting microglial cells are highly dynamic surveillants of brain parenchyma in vivo. Science 308, 1314–1318. doi: 10.1126/science.1110647
Okunuki, Y., Mukai, R., Pearsall, E. A., Klokman, G., Husain, D., Park, D. H., et al. (2018). Microglia inhibit photoreceptor cell death and regulate immune cell infiltration in response to retinal detachment. Proc. Natl. Acad. Sci. U. S. A. 115, E6264–E6273. doi: 10.1073/pnas.1719601115
Oosterhof, N., Kuil, L. E., van der Linde, H. C., Burm, S. M., Berdowski, W., van Ijcken, W. F. J., et al. (2018). Colony-stimulating factor 1 receptor (CSF1R) regulates microglia density and distribution, but not microglia differentiation in vivo. Cell Rep. 24, 1203–1217.e6. doi: 10.1016/j.celrep.2018.06.113
Paolicelli, R. C., Bolasco, G., Pagani, F., Maggi, L., Scianni, M., Panzanelli, P., et al. (2011). Synaptic pruning by microglia is necessary for Normal brain development. Science 333, 1456–1458. doi: 10.1126/science.1202529
Paolicelli, R. C., Sierra, A., Stevens, B., Tremblay, M. E., Aguzzi, A., Ajami, B., et al. (2022). Microglia states and nomenclature: a field at its crossroads. Neuron 110, 3458–3483. doi: 10.1016/j.neuron.2022.10.020
Parkhurst, C. N., Yang, G., Ninan, I., Savas, J. N., Yates, J. R., Lafaille, J. J., et al. (2013). Microglia promote learning-dependent synapse formation through brain-derived neurotrophic factor. Cells 155, 1596–1609. doi: 10.1016/j.cell.2013.11.030
Prinz, M., Jung, S., and Priller, J. (2019). Microglia biology: one century of evolving concepts. Cells 179, 292–311. doi: 10.1016/j.cell.2019.08.053
Ransohoff, R. M. (2016). A polarizing question. Do M1 and M2 microglia exist? Nat. Neurosci. 19, 987–991. doi: 10.1038/nn.4338
Ransohoff, R. M., and Perry, V. H. (2009). Microglial physiology: unique stimuli, specialized responses. Annu. Rev. Immunol. 27, 119–145. doi: 10.1146/annurev.immunol.021908.132528
Sankowski, R., Monaco, G., and Prinz, M. (2022). Evaluating microglial phenotypes using single-cell technologies. Trends Neurosci. 45, 133–144. doi: 10.1016/j.tins.2021.11.001
Sarn, N. (2021). Cytoplasmic-predominant Pten increases microglial activation and synaptic pruning in a murine model with autism-like phenotype. Mol Psychiatry 14, 1458–1471.
Schafer, D. P., Lehrman, E. K., Kautzman, A. G., Koyama, R., Mardinly, A. R., Yamasaki, R., et al. (2012). Microglia sculpt postnatal neural circuits in an activity and complement-dependent manner. Neuron 74, 691–705. doi: 10.1016/j.neuron.2012.03.026
Scheiblich, H., Dansokho, C., Mercan, D., Schmidt, S. V., Bousset, L., Wischhof, L., et al. (2021). Microglia jointly degrade fibrillar alpha-synuclein cargo by distribution through tunneling nanotubes. Cells 184, 5089–5106.e21. doi: 10.1016/j.cell.2021.09.007
Sierra, A., Paolicelli, R. C., and Kettenmann, H. (2019). Cien Años de Microglía: milestones in a century of microglial research. Trends Neurosci. 42, 778–792. doi: 10.1016/j.tins.2019.09.004
Silva, N. J., Dorman, L. C., Vainchtein, I. D., Horneck, N. C., and Molofsky, A. V. (2021). In situ and transcriptomic identification of microglia in synapse-rich regions of the developing zebrafish brain. Nat. Commun. 12:5916. doi: 10.1038/s41467-021-26206-x
Silvin, A., Uderhardt, S., Piot, C., Da Mesquita, S., Yang, K., Geirsdottir, L., et al. (2022). Dual ontogeny of disease-associated microglia and disease inflammatory macrophages in aging and neurodegeneration. Immunity 55, 1448–1465.e6. doi: 10.1016/j.immuni.2022.07.004
Simard, A. R., Soulet, D., Gowing, G., Julien, J. P., and Rivest, S. (2006). Bone Marrow-derived Microglia Play a Critical Role in Restricting Senile Plaque Formation in Alzheimer’s Disease, vol. 14.
Tansley, S., Uttam, S., Ureña Guzmán, A., Yaqubi, M., Pacis, A., Parisien, M., et al. (2022). Single-cell RNA sequencing reveals time-and sex-specific responses of mouse spinal cord microglia to peripheral nerve injury and links Apo E to chronic pain. Nat. Commun. 11:843. doi: 10.1038/s41467-022-28473-8
Troutman, T. D., Kofman, E., and Glass, C. K. (2021). Exploiting dynamic enhancer landscapes to decode macrophage and microglia phenotypes in health and disease. Mol. Cell 81, 3888–3903. doi: 10.1016/j.molcel.2021.08.004
Ugidos, I. F., Pistono, C., Korhonen, P., Gómez-Budia, M., Sitnikova, V., Klecki, P., et al. (2022). Sex differences in Poststroke inflammation: a focus on microglia across the lifespan. Stroke 53, 1500–1509. doi: 10.1161/STROKEAHA.122.039138
van Eck, N. J., and Waltman, L. (2010). Software survey: VOSviewer, a computer program for bibliometric mapping. Scientometrics 84, 523–538. doi: 10.1007/s11192-009-0146-3
Voet, S., Srinivasan, S., Lamkanfi, M., and van Loo, G. (2019). Inflammasomes in neuroinflammatory and neurodegenerative diseases. EMBO Mol. Med. 11:e10248. doi: 10.15252/emmm.201810248
Wake, H., Moorhouse, A. J., Jinno, S., Kohsaka, S., and Nabekura, J. (2009). Resting microglia directly monitor the functional state of synapses in vivo and determine the fate of ischemic terminals. J. Neurosci. 29, 3974–3980. doi: 10.1523/JNEUROSCI.4363-08.2009
Wang, S. K., Xue, Y., and Cepko, C. L. (2020). Microglia modulation by TGF-β1 protects cones in mouse models of retinal degeneration. J. Clin. Invest. 130, 4360–4369. doi: 10.1172/JCI136160
Wendeln, A. C., Degenhardt, K., Kaurani, L., Gertig, M., Ulas, T., Jain, G., et al. (2018). Innate immune memory in the brain shapes neurological disease hallmarks. Nature 556, 332–338. doi: 10.1038/s41586-018-0023-4
Wurm, J., Konttinen, H., Andressen, C., Malm, T., and Spittau, B. (2021). Microglia development and maturation and its implications for induction of microglia-like cells from human iPSCs. Int. J. Mol. Sci. 22:3088. doi: 10.3390/ijms22063088
Xia, Y., Zhang, Z., Lin, W., Yan, J., Zhu, C., Yin, D., et al. (2020). Modulating microglia activation prevents maternal immune activation induced schizophrenia-relevant behavior phenotypes via arginase 1 in the dentate gyrus. Neuropsychopharmacology 45, 1896–1908. doi: 10.1038/s41386-020-0743-7
Yirmiya, R., Rimmerman, N., and Reshef, R. (2015). Depression as a microglial disease. Trends Neurosci. 38, 637–658. doi: 10.1016/j.tins.2015.08.001
Yu, T., Zhang, X., Shi, H., Tian, J., Sun, L., Hu, X., et al. (2019). P2Y12 regulates microglia activation and excitatory synaptic transmission in spinal lamina II neurons during neuropathic pain in rodents. Cell Death Dis. 10:165. doi: 10.1038/s41419-019-1425-4
Keywords: bibliometric analysis, CiteSpace, knowledge domain, microglia, VOSviewer
Citation: Liu G, Li T, Yang A, Zhang X, Qi S and Feng W (2023) Knowledge domains and emerging trends of microglia research from 2002 to 2021: A bibliometric analysis and visualization study. Front. Aging Neurosci. 14:1057214. doi: 10.3389/fnagi.2022.1057214
Edited by:
Jagdeep K. Sandhu, National Research Council, CanadaCopyright © 2023 Liu, Li, Yang, Zhang, Qi and Feng. This is an open-access article distributed under the terms of the Creative Commons Attribution License (CC BY). The use, distribution or reproduction in other forums is permitted, provided the original author(s) and the copyright owner(s) are credited and that the original publication in this journal is cited, in accordance with accepted academic practice. No use, distribution or reproduction is permitted which does not comply with these terms.
*Correspondence: Songtao Qi, ✉ cWlzb25ndGFvbmZ5eUAxMjYuY29t; Wenfeng Feng, ✉ ZmVuZ3dmMTk2N0AxNjMuY29t
†These authors have contributed equally to this work