- 1Department of Pathology, School of Medical Sciences Universiti Sains Malaysia and Hospital Universiti Sains Malaysia, Kubang Kerian, Kelantan, Malaysia
- 2Centre for Drug Research, Universiti Sains Malaysia, Penang, Malaysia
- 3Department of Neuroscience, School of Medical Sciences, Universiti Sains Malaysia, Kubang Kerian, Kelantan, Malaysia
- 4Department of Chemical Pathology, School of Medical Sciences, Universiti Sains Malaysia and Hospital Universiti Sains Malaysia, Kubang Kerian, Kelantan, Malaysia
- 5Department of Anatomy, School of Medical Sciences, Universiti Sains Malaysia, Kubang Kerian, Kelantan, Malaysia
- 6Basic and Medical Sciences Unit, School of Dental Sciences, Universiti Sains Malaysia, Kubang Kerian, Kelantan, Malaysia
Tropical Meliponini bees produce stingless bee honey (SBH). Studies have shown beneficial properties, including antibacterial, bacteriostatic, anti-inflammatory, neurotherapeutic, neuroprotective, wound, and sunburn healing capabilities. High phenolic acid and flavonoid concentrations offer SBH its benefits. SBH can include flavonoids, phenolic acids, ascorbic acid, tocopherol, organic acids, amino acids, and protein, depending on its botanical and geographic origins. Ursolic acid, p-coumaric acid, and gallic acid may diminish apoptotic signals in neuronal cells, such as nuclear morphological alterations and DNA fragmentation. Antioxidant activity minimizes reactive oxygen species (ROS) formation and lowers oxidative stress, inhibiting inflammation by decreasing enzymes generated during inflammation. Flavonoids in honey reduce neuroinflammation by decreasing proinflammatory cytokine and free radical production. Phytochemical components in honey, such as luteolin and phenylalanine, may aid neurological problems. A dietary amino acid, phenylalanine, may improve memory by functioning on brain-derived neurotrophic factor (BDNF) pathways. Neurotrophin BDNF binds to its major receptor, TrkB, and stimulates downstream signaling cascades, which are crucial for neurogenesis and synaptic plasticity. Through BDNF, SBH can stimulate synaptic plasticity and synaptogenesis, promoting learning and memory. Moreover, BDNF contributes to the adult brain’s lasting structural and functional changes during limbic epileptogenesis by acting through the cognate receptor tyrosine receptor kinase B (TrkB). Given the higher antioxidants activity of SBH than the Apis sp. honey, it may be more therapeutically helpful. There is minimal research on SBH’s neuroprotective effects, and the related pathways contribute to it is unclear. More research is needed to elucidate the underlying molecular process of SBH on BDNF/TrkB pathways in producing neuroprotective effects.
Introduction
Several types of honey are well-researched, namely European bee honey (i.e., Manuka honey, jelly bush honey, African jungle honey) and Malaysian bee honey (i.e., Tualang honey and stingless bee honey, SBH; Ahmed and Othman, 2013). Bees are essential in the ecosystem as pollinating and cultivating agents of various plants. It has been used as commercial pollinators, especially stingless bees, due to their non-stinging characteristic (de Oliveira Cruz and de Oliveira Campos, 2009; Bartelli and Nogueira-Ferreira, 2015). Besides, they also play an indispensable role in maintaining genetic variability and fruit quality (Bartelli and Nogueira-Ferreira, 2015). Bee species are divided into Apis sp. (honeybee) and Melipona sp. (stingless bee). In the 1980s, researchers reported that honey could also be collected from the stingless bee. The study has found more than 600 species, including Hymenoptera, Apidae, and Meliponini, the largest stingless bee group of eusocial bees (Lavinas et al., 2019). These species were classified under 60 genera, and Melipona and Trigona were found to be significant in number (Michener, 2013; Lavinas et al., 2019).
Stingless bees are commonly found in various sub-tropical countries, including Africa, America, Australia, Southeast Asia, and Malaysia (Kek et al., 2014; Vijayakumar and Jeyaraaj, 2014). In Malaysia, more than 30 stingless bee species are from the Trigona genus. The most commercialized stingless bee honey is Geniotrigona thoracica Smith, Heterotrigona itama Cockerell, Lepidotrigona Terminata Smith, Tetragonula fuscobalteata Cameron, and Tetraponera Laeviceps (Kelly et al., 2014). Heterotrigona itama is the most popular domesticated stingless bee used in meliponiculture due to its advantages, as it can be easily found, easily kept, and acts as a good pollinating agent for the crop (Kelly et al., 2014; Wong et al., 2019; Shamsudin et al., 2019a). The honey produced by this stingless bee is known as Meliponine honey, SBH, pot-honey, and Kelulut honey (in Malaysia; Souza et al., 2006).
Besides honey, stingless bees also produce other products, including propolis, beebread, cerumen, and bee pollen, which have beneficial medicinal properties (Al-Hatamleh et al., 2020). Stingless bees build their nest in hollows of trunks, branches, and tree roots and in ant or termite nests to store their honey collected from nectars, honeydew, and fruit juices (Ávila et al., 2018; Grüter, 2020). In contrast to Apis mellifera honeybees, stingless bees stores honey in a little resin pot toward the end of the nest (Kek et al., 2014). Stingless bees have well-defined characteristics, such as vestigial sting, travel shorter distances for food searching, high moisture content, and non-sting morphology (Vazhacharickal, 2016; Ávila et al., 2018; Mokaya et al., 2022). Moreover, stingless bees can collect nectar and honeydew from small flowers due to their smaller body size (2–5 mm) compared to Apis mellifera (Vazhacharickal, 2016; Ngaini et al., 2021; Mokaya et al., 2022). Heterotrigona itama species can collect more honey than other SBH families, such as Tetrigona apicalis and Tetragonula laeviceps (Zainin and Hassan, 2021).
SBH has many beneficial effects, including anti-inflammatory, anti-microbial, antibacterial, anti-diabetic, anti-cancer, and wound healing properties (Ávila et al., 2018; Zulkhairi Amin et al., 2018). It was reported that flavonoids and phenolic compounds contribute to the potential beneficial effects via antioxidative and anti-inflammatory activities (Cianciosi et al., 2018; Kwon et al., 2019). For example, luteolin, a flavonoid, can reduce seizure scores, glutamate levels, neuronal cell death, and microglial activation in rat hippocampus of the kainic acid (KA)-induced excitotoxicity model (Lin et al., 2016). Therefore, this led to a postulation that SBH has a high potential to combat oxidative stress, a significant cause of neuroinflammation that can result in neuronal apoptosis and death. For instance, high antioxidant content can prevent lipid peroxidation and protective effects on cell membrane integrity via catabolism suppression (Sawaya and Marcucci, 2011). Moreover, sub-chronic (35 days) supplementation of SBH improves mice’s spatial working and reference memories. These results might be due to the phenylalanine compound, a dietary amino acid that has memory-enhancing effects by acting directly on BDNF, thus improving brain signaling and physiology (Mustafa et al., 2019).
Nutritional value and physicochemical properties of SBH
The stingless bee belongs to the Meliponinae subfamily of bees and produces honey and other bee products, including pollen, beeswax, propolis, and royal jelly (Rasmussen and Cameron, 2009; Mustafa et al., 2018). In contrast, to sting honey bees, stingless bees store their honey in vertical jars formed of cerumen, containing more flavonoids and antioxidant qualities than other kinds of honey (Vit et al., 2004; Abd Jalil et al., 2017; Alvarez-Suarez et al., 2018). The physical properties of honey samples determine the quality, duration of shelf life, and biological activities. Honey properties and composition depend on climatic and environmental factors (Iglesias et al., 2012). The colour of SBH depends on bee colonies, while the taste depends on the plants of collected nectar (Kulkarni et al., 2017). In comparison to honey bees, SBH has more nutrients and mineral composition. Due to their tiny size, bees may fit within a more significant number of florals, increasing the honey’s polyphenol content. Therefore, it increases the variety of bioactive chemicals found in honey (Kek et al., 2014; Biluca et al., 2016; Ranneh et al., 2018).
Studies have reported that SBH is golden, slightly brown, and translucent (Gomes et al., 2010). Colour intensity represents the concentration of mineral content, phenolic content, and pigments. The presence of colour pigments in honey, such as carotenoids and some flavonoids, is indicated by the absorbance (ABS) ABS450 colour intensity (Beretta et al., 2005). A previous study on physicochemical analysis of honey reported higher ABS 450 values of SBH, indicating that this type of honey contains more colour pigments than other Malaysian honey (Chan et al., 2017). A study demonstrated that the ABS450 values of various kind of Malaysian SBH range between 169.89–805 mAU; while a higher ABS450 value in the range of 1029.00–2103.17 mAU were documented in kelulut honey (Khalil et al., 2011a; Moniruzzaman et al., 2013b). The dark brown colour intensity indicates the presence of high phenolic content in honey (Ahmed and Othman, 2013). In this context, kelulut honey has a high ABS450 value and lower ash content, indicating high mineral content.
Moisture content is one of the honey quality factors since it affects the shelf-life length and microbiological activity stability (Lage et al., 2012; Shahnawaz et al., 2013; Sujanto et al., 2021). Honey’s moisture content changes owing to nectar flux strength, maturity level, beekeeper handling method, extraction condition, processing, and storage method, seasonal weather conditions, and regional humidity of the harvest location (Moniruzzaman et al., 2013a,b; Sujanto et al., 2021). Studies have reported that the moisture content in SBH is higher when compared to Apis mellifera honey (Wang and Li, 2011; Silva et al., 2013). Therefore, more outstanding care is needed to handle honey with higher moisture as it can affect the fermentation activity and shelf life (Wang and Li, 2011; da Silva et al., 2013; Silva et al., 2013). To prolong the shelf-life, drying at a low temperature is recommended after harvesting (Chan et al., 2017).
The microbiological stability of honey is determined by its pH since most bacteria prefer neutral or slightly alkaline environments to flourish in (Silva et al., 2009). The organic acid content in honey varies between samples due to the honey’s floral origin, fermentation rate of sugars to alcohol, bee species, and oxidation to carboxylic acids (Mokaya et al., 2022). Honey with a low pH value showed greater microbial stability, thus increasing the shelf-life duration (Lage et al., 2012). Studies have reported that Trigona honey (Heterotrigona and Geniotrigona spp.) has lower pH and higher free acidity than Apis mellifera honey (Shamsudin et al., 2019b). The pH value of Malaysia kelulut honey is comparable to the pH value of SBH from other countries. It was reported that the low pH range (3.22–3.69) of kelulut can inhibit microorganism growth, thus, preventing contamination of honey (Do Nascimento et al., 2015).
Hydroxymethylfurfural (HMF) content in honey acts as an indicator of honey’s freshness. It is influenced by several variables, including storage conditions, pH level, heating time and temperature, and floral origin (de Almeida-Muradian et al., 2013). HMF is formed due to the reaction between sugars—such as fructose—and acids. To maintain the low HMF level in honey, a proper storage environment with low-temperature exposure plays a significant role (Shapla et al., 2018; Mokaya et al., 2022). A low HMF level indicates fresh and high-quality honey. Meanwhile, high HMF level is associated with degradation, heat exposure, poor handling, and storage conditions. HMF concentration could increase in the heating process caused by the acid-catalyzed dehydration reaction of hexose sugars like fructose and glucose (Singh and Bath, 1997; Gomes et al., 2010; Khalil et al., 2010).
Honey is a concentrated carbohydrate solution composed of various reducing sugars, including fructose and glucose. The sugar content level of SBH differs depending on the geographical origin, the flora, and the vegetation in that region (Biluca et al., 2016; Chuttong et al., 2016; Se et al., 2018). The primary sugar, sucrose, are found in small quantity and will be broken down by a digestive enzyme (Grüter, 2020). It also contains non-reducing sugars such as sucrose and maltose (Ávila et al., 2018; Zulkhairi Amin et al., 2018; Sujanto et al., 2021). A study has demonstrated that the sugar content in SBH ranges between 68% and 73%. Several studies have reported that the reduced sugar content of SBH is more than 60% (Biluca et al., 2016; Chuttong et al., 2016; Se et al., 2018). SBH has a high fructose-reducing sugar level (48.1%) but lower than Apis mellifera honey standards (Biluca et al., 2016; Chuttong et al., 2016). A study has demonstrated that the sugar content for Heterotrigona itama, Geniotrigona thoracica, and Lepidotrigona terminate is approximately 35, 34, and 53% of maltose/trehalulose, respectively, (Grüter, 2020). The study has demonstrated that Malaysian Trigona SBH has a low concentration level of fructose and glucose (15.4%–24.7%) when compared to Apis mellifera (51.0%) (Shamsudin et al., 2019b).
Phytochemical compounds found in SBH and their potential neuroprotective effects
Phytochemical compounds are essential in determining honey quality due to their potential properties, such as antimicrobial, anticancer, anti-inflammatory, and antioxidant (Mokaya et al., 2022). The concentration and type of phenolic content and total flavonoid content (TFC) for each kind of honey are affected by floral origins, seasonal factors, and species of bee (Saxena et al., 2010; Tenore et al., 2012; Ismail et al., 2016; Ávila et al., 2019). Flavonoid groups found in honey include flavonols (i.e., myricetin, kaempferol, quercetin, isorhamnetin, pinobanksin, rutin, and galangin), flavones (i.e., genkwanin, luteolin, apigenin, tricetin, and chrysin), and flavanones (i.e., pinocembrin and pinostrobin; Hossen et al., 2017; Zulkhairi Amin et al., 2018). A study has reported that the TFC of Trigona SBH observed were higher when compared to other Malaysia Apis honey (Ismail et al., 2021). Meanwhile, the phenolic group found in honey includes the hydroxybenzoic acids (methyl syringate, gallic acid, syringic acid, benzoic acid, and 4-hydroxybenzoic acid) and hydroxyl-cinnamic acids (chlorogenic, vanillic, caffeic, p-coumaric, and ferulic acids; Hossen et al., 2017; Zulkhairi Amin et al., 2018). A study has reported that the total phenolic content (TPC) of Trigona SBH is higher when compared to Apis Mellifera (Abu Bakar et al., 2017; Ranneh et al., 2018).
Numerous research has examined the health benefits of polyphenols, including their antioxidant and neuroprotective effects on various disorders related to the nervous system (Table 2; Abd Aziz et al., 2019; Hazirah et al., 2019; Arshad et al., 2020). SBH has a high concentration of polyphenol chemicals, which makes it effective in promoting cell growth, preserving the cellular structure, and protective effect against free radicals in the region of injury (Abd Jalil et al., 2017; Meo et al., 2017; Cianciosi et al., 2018; Mohamad et al., 2018). Gallic, coumaric, caffeic acids, catechin, kaempferol, and apigenin are the primary antioxidants in honey (Chew et al., 2018). Studies have suggested that phenolic substances have protective effects on cell membranes by decreasing lipid peroxidation and removing free radicals (Chaudhuri et al., 2007). A study has claimed that both honey bee honey (HBH) and SBH can counteract reactive oxygen species (ROS; Twilley and Lall, 2014), whereby the phenolic acid extracted from SBH can reduce the production of ROS (Borsato et al., 2014). Kaempferol can inhibit the effects of free radicals, including NO synthase (NOS) and cyclooxygenase-2 (COX-2; Crespo et al., 2008).
Moreover, SBH has also been reported to have many beneficial effects, including anti-inflammatory, anti-microbial, antibacterial, anti-diabetic, anti-cancer, and wound healing properties (Table 3; Ávila et al., 2018; Zulkhairi Amin et al., 2018). SBH showed an outstanding ability to reduce infection, antioxidative and anti-inflammatory activity (Kwapong et al., 2013). The high antioxidant content can prevent lipid peroxidation and has protective effects on cell membrane integrity via catabolism suppression (Sawaya and Marcucci, 2011). Studies have demonstrated that flavonoids and phenolic compounds exert potential beneficial effects via antioxidative and anti-inflammatory activities (Table 1; Cianciosi et al., 2018; Kwon et al., 2019). For example, luteolin, a flavonoid, can reduce seizure scores, glutamate levels, neuronal cell death, and microglial activation in rat hippocampus of the KA-induced excitotoxicity model (Lin de Sousa, 2016). Therefore, it led to a postulation that SBH has a high potential to combat oxidative stress, a major cause of neuroinflammation that can result in neuronal apoptosis and death. In conclusion, these beneficial properties of SBH due to the compounds might contribute to neuroprotective potentials on various neurological disorders.
Antioxidant and neuroprotective effects of SBH bioactive compounds
Natural antioxidant compounds in honey, including amino acids, proteins, enzymes, ascorbic acid, carotenoid-like substances, organic acids, Maillard reaction, proteins, and polyphenols, especially flavonoids and phenolic acids, contribute to the various beneficial effects of honey (Zulkhairi Amin et al., 2018). SBH, such as Geniotrigona thoracica and Heterotrigona itama, have been demonstrated to have more antioxidant activity than Manuka honey (Apis mellifera) and higher mineral contents than honey made by Colombian stingless bees (Abu Bakar et al., 2017). Moreover, the higher concentration of polyphenols and phenolic acids contribute to more significant antioxidant effects than other local honey, including Tualang honey (Biluca et al., 2016). Kelulut honey contains higher TPC, and higher antioxidant activity was also demonstrated in the ferric-reducing antioxidant potential (FRAP) assay compared to New Manuka honey. Meanwhile, the study also reported higher TFC when compared to Portuguese heather honey and Spanish rosemary honey (Ferreres et al., 1994; Hazirah et al., 2019).
The brain is the most sensitive organ to oxidative stress due to its poor repair capacity, high metabolic rate, and high-level consumption of glucose and oxygen (Halliwell, 1992; Dan Dunn et al., 2015). The brain consumes a ~ 10-fold higher level of oxygen when compared to other organs (Gandhi and Abramov, 2012). Besides, the high polyunsaturated fatty acid in the brain makes it more susceptible to lipid peroxidation. Moreover, the brain is also more vulnerable to hydroxyl radical formation due to the high iron load (Halliwell, 1999; Jellinger, 2003; Mariani et al., 2005). The neurons are more prone to cellular damage caused by excessive free radicals than glial cells (Bolaños et al., 2010). The hippocampus and frontal cortex are the most vulnerable brain regions to damage caused by ROS overproduction (Wang and Michaelis, 2010; Jiang et al., 2017; Roganovic et al., 2019). Moreover, the hippocampus is the most susceptible to neurodegeneration, which is also essential in learning, memory, spatial navigation, and emotional behavior (Rafiee Sardooi et al., 2021).
Oxidative stress plays an essential role in the pathogenesis of various neurological and neurodegenerative diseases, including epilepsy, Alzheimer’s disease, Parkinson’s disease, and amyotrophic lateral sclerosis (ALS; Perry et al., 2002; Migliore et al., 2005; Ashrafi et al., 2007). Overconcentration of oxygen radicals will lead to changes in intracellular calcium ions homeostasis which plays a critical role in neuronal excitability and synaptic transmission. Therefore, it results in the vulnerability of neurons and neuronal loss (Puttachary et al., 2015). The initiating brain lesion and seizure increase ROS production, RNS levels, and oxidative stress (Pearson-Smith and Patel, 2017). The production of free radicals during oxidative stress will cause damage to the structural and functional integrity of neuronal cells in various neurological diseases (Rao et al., 2016; Yaacob et al., 2018). It will cause neuronal insult by protein oxidation, lipid peroxidation and DNA damage (Medda et al., 2020). Neurotoxicity in acute and chronic neurological diseases might be due to oxidative stress due to excessive activation of glutamate receptors (Choi, 1988). Glutamate-induced neurotoxicity led to the overproduction of ROS and RNS, resulting in damage to lipids, protein, DNA, and neuronal cell death (Lucas and Newhouse, 1957; Dugan et al., 1995).
The high oxidative stress will cause a neurotoxic effect which is also a critical factor in cognitive dysfunctionalities, including learning and memory performances (Özbalci et al., 2013; Engwa, 2018). The study has shown a significant correlation between antioxidant activity and polyphenols concentration (Kamaruzzaman et al., 2019; Mohamed et al., 2012). Phenolic compounds in honey can inhibit ROS formation as a defence mechanism against oxidative stress (Mijanur Rahman et al., 2014). In an in vitro model using primary hippocampal neuronal cells, apigenin, a phenolic substance, exerts a protective effect against harm brought on by oxygen–glucose deprivation and reperfusion (Han et al., 2012). Honey contains p-coumaric acid, kaempferol, luteolin, and apigenin, reducing potential oxidative stress (Ferguson et al., 2005). Apigenin prevents KA-induced excitotoxicity in the hippocampus in a dose-dependent manner by quenching reactive oxygen species and preventing glutathione (GSH) depletion (Han et al., 2012). This compound has demonstrated ROS scavenging activity and prevents the GSH level reduction in the KA-induced excitotoxicity model (Han et al., 2012). Other compounds, such as caffeic acid and catechin, were reported to play an essential role in learning and memory (Sutherland et al., 2006; Hu et al., 2008). Antioxidants exert protective effects by attenuating ROS and thus inhibiting unwanted chain reactions (Ha et al., 2019).
SBH extract can reduce the production of ROS, leukocyte migration, and oedema, contributing to anti-inflammatory impact (Borsato et al., 2014). Both in vivo and in vitro studies have shown that certain flavonoids significantly stabilize the lysosomal membrane (Leelaprakash and Dass, 2011). Studies conducted in vitro have demonstrated that phenolic compounds can exert antioxidant activity by acting on lipid bilayers, particularly those found in lipoprotein domains and cell membranes, which are lipid peroxidation targets (Chaudhuri et al., 2007). Lysosomal stabilization is essential for managing the inflammatory response because it prevents the extracellular release of lysosomal components of activated neutrophils, such as bacterial enzymes and proteases, which can worsen tissue inflammation and damage. Inflammation can cause the release of lysosomal enzymes, leading to several disorders (Leelaprakash and Dass, 2011).
Studies have demonstrated that SBH significantly ameliorates oxidative stress through nuclear factor kappa-light-chain-enhancer (NF-κB) p65 and p38 mitogen-activated protein kinase (MAPK), and Nrf2 expression in lipopolysaccharide LPS-induced rats (Ranneh et al., 2019). Moreover, the antioxidant activity of SBH was demonstrated against LPS-induced inflammation by inhibiting nitric oxide production via nitric oxide synthase (Ooi et al., 2021). Studies have proven that consuming honey can decrease oxidative damage, enhance cognitive performance, and suppress memory and learning decline. Antioxidants in honey play a vital role in suppressing anxiety-like behavior in mice (Barros et al., 2006). Kelulut honey, can ameliorate anxiety behavior, significant memory retention and protective effects on pyramidal neuronal cells in the hippocampus brain region (Arshad et al., 2020). Besides, honey also showed positive neuroprotective effects on lead-induced neurotoxicity based on open field test and Morris water maze results as well as significant increase in antioxidant activities (catalase, SOD and GSH) results (Abdulmajeed et al., 2016).
Anti-neuroinflammatory effects and SBH bioactive compounds
Brain inflammation due to brain disruption triggers inflammatory factors secretion from neurons, astrocytes, and microglia, including interleukin-1β (IL-1β) and interleukin-6 (IL-6), interferon-γ (IFNγ), tumor necrosis factor-α (TNF-α) and transforming growth factor-β (TGF-β) (Vezzani et al., 2011, 2013; Kim et al., 2012). Inflammation in the brain causes the disruption of synaptic signaling and plasticity, impairs inhibitory transmission and blood brain barrier (BBB) permeability, lower the seizure threshold and promotes epileptogenesis (Abbott, 2000; Weissberg et al., 2011; Vezzani et al., 2013). Neuroinflammation involves the generation of seizures and directly affects neurovascular and glial functions (Sternberg, 2000; Vezzani et al., 2011, 2012). Meanwhile, systemic inflammation triggers epileptiform neuronal discharge through ionic and neurotransmitter physiology, which are the consequences of impairments in the blood–brain barrier (Sternberg, 2000; David et al., 2009; Heinemann et al., 2012).
SBH antioxidant effect prevents the signaling of NF-ĸB and MAPK also reduces the production of inflammatory factors IL-6, TNF-α and IFN-γ (Ranneh et al., 2019; Biluca et al., 2020). Flavonoid compounds such as quercetin act as anti-inflammatory and antioxidant agents. Several studies have shown that SBH can reduce proinflammatory cytokines IL-6, interleukin-2p70 (IL-2p70), TNF-α and IFN-γ and anti-inflammatory cytokine, IL-10 expression levels. These substances can inhibit the generation of iNOS, COX-2, cytokine production, nicotinamide adenine dinucleotide phosphate (NADPH) oxidase, ROS, and proinflammatory mediators (Vezzani et al., 1999; Wu et al., 2016). Flavonoids can directly affect cellular receptors and proteins (kinases and enzymes), which can cause physiological changes and changes in the way genes are expressed. This could help protect neurons by making inflammatory responses more effective, which can directly change neuroinflammatory cascades or get rid of free radicals (Copmans et al., 2018).
High antioxidant substances in honey are used in studies related to inflammation and the associated diseases, as oxidative stress is the critical factor in determining the inflammatory status (Arulselvan et al., 2016; Al-Hatamleh et al., 2020). Antioxidant activity prevents inflammation from getting worse by blocking enzymes from working. These enzymes are nitric oxide synthases (NOS), cytochrome P450 (CP450), NADPH oxidase (NOX), xanthine oxidase (XO), lipoxygenase (LO), cyclooxygenase (COX), and myeloperoxidase (MP). The latter prevents ROS production, reducing oxidative stress and producing an anti-inflammatory effect (Al-Hatamleh et al., 2020). Flavonoids inhibit enzymes and other inflammatory mediators such as reactive C protein or adhesion molecules. One strategy to alter the sequence of molecular occurrences that leads to the overexpression of those mediators is to suppress transcription factors like NF-ĸB and activator protein-1 (AP-1) by inhibiting protein kinases involved in signal transduction. The study has reported that flavonoids potentially reduce inflammation by boosting antioxidant defences by activating the NF-E2-related factor 2 (Nrf2) (Leelaprakash and Dass, 2011; González-Gallego et al., 2014).
Several studies have demonstrated the ability of SBH to exert various beneficial effects due to the high concentration and diversity of phenolic acid and flavonoid compounds (Sousa de Sousa, 2016; de Oliveira et al., 2017; Alvarez-Suarez et al., 2018; Souza et al., 2021). For example, antioxidant and anti-inflammatory effects due to flavonoids and polyphenols content improve cognitive disabilities and protective effects via neuronal and glial pathways (Mohamed et al., 2012; Kamaruzzaman et al., 2019). Studies have also shown that ursolic acid, p-coumaric acid, and gallic acid might lessen apoptotic symptoms in neuronal cells, such as nuclear morphological alterations, DNA fragmentation, and cell blebbing (Hong et al., 2012). Flavonoids such as quercetin, resveratrol, apigenin, kaempferol, epicatechin, and curcumin can influence mitochondrial processes. These compounds act by inhibiting enzymes, scavenging ROS, or altering the activity of different transcription factors involved in oxidative pathways (Gao et al., 2007; Lagoa et al., 2011; Gibellini et al., 2015).
Cognitive, learning, and memory function and SBH bioactive compounds
Several studies have demonstrated the abilities of honey to promote cognitive functions in the inflammatory-induced model due to the presence of various compounds (Arshad et al., 2020; Yaacob et al., 2020; Adeniyi et al., 2022) Honey can improves anxiety, cognitive behavioral and locomotor activity dysfunctionalities in lipopolysaccharide-induced neuroinflammation model (Adeniyi et al., 2022). SBH and its phenolic content prevent neuroinflammation, act against neuronal damage caused by neurotoxins, and induce memory, learning, and cognitive function in the brain (Rao et al., 2016; Meo et al., 2017; Yaacob et al., 2018). Moreover, the high antioxidant activity of quercetin, a flavonoid compound in SBH, promotes memory performance and synaptic plasticity of hippocampal neuronal cells in the chronic lead exposure experimental model (Mijanur Rahman et al., 2014). Kelulut honey can improve spatial memory impairment in the LPS-induced rats model and anxiety in the metabolic syndrome rat model (Arshad et al., 2020; Yaacob et al., 2020).
Previously, studies have shown that caffeic acid (phenolic acid) and catechin (flavonoids) play an essential role in learning and memory performances (Sutherland et al., 2006; Hu et al., 2008). Other bioactive compounds, including kaempferol, catechin, quercetin, and caffeic acid found, which are also present in SBH, have demonstrated protective effects against memory impairment in various (Dajas et al., 2003; Unno et al., 2007; Park et al., 2010; Cai et al., 2011; Anwar et al., 2012; Zulkhairi Amin et al., 2018). Besides, other flavonoid compounds, quercetin, also play a vital role in memory and synaptic plasticity in memory impairment-induced in vitro models (Hu et al., 2008; Kumar et al., 2008). The study has reported that honey-supplemented rats showed better memory performances when compared to sucrose-only-fed rats (Chepulis et al., 2009). So, it’s clear that sugar content alone does not improve memory. There are also other compounds in honey that do (Kishore et al., 2011; Khalil et al., 2011a, b).
High flavonoid content in SBH may be comparable to other natural products (green tea, blueberry, and Ginkgo biloba) and acts through hippocampus BDNF. Therefore, suggesting that it can contribute to better learning and memory processes (Williams et al., 2008; Li et al., 2009a,b; Hou et al., 2010; Rendeiro et al., 2013). Previously, the flavones compound mimicked neurotrophin’s binding ability by inducing the tyrosine phosphorylation and dimerization of TrkB. Moreover, it can mimic BDNF’s ability to activate the downstream pathways essential in neurogenesis and synaptic plasticity (Jang et al., 2010; Gupta et al., 2013; Wurzelmann et al., 2017; Paul et al., 2021). Furthermore, a study on SBH supplementation showed that it could induce synaptic plasticity and synaptogenesis, thus improving learning and memory performance in the brain (Mustafa et al., 2018). In addition, studies have reported that seizures could stimulate BDNF gene expression levels and upregulate TrkB activation in the epileptic experimental model (McNamara and Scharfman, 2012). Moreover, BDNF contributes to the adult brain’s lasting structural and functional changes during limbic epileptogenesis by acting through the cognate receptor TrkB (Binder et al., 1999; Scharfman et al., 2005).
Studies have demonstrated the amino acid profile and concentration in various honey samples, and the honey’s botanical and geographical origins may affect its phenylalanine content (Shamsudin et al., 2019a). Other study has also highlighted the presence of phenylalanine, a dietary amino acid, in SBH (Uren and Turnley, 2014; Ramli et al., 2019; Slutsky and Etnier, 2019). Previously, studies have suggested a dietary amino acid might have memory-enhancing effects by acting directly on BDNF signaling pathways (Mustafa et al., 2019). This dietary amino acid converts to tyrosine, a vital component of the tyrosine receptor kinase B (TrkB), a BDNF receptor (Lu et al., 2018). Previously, a study has reported that phenylalanine decreases neurite development and promotes neuronal death via lowering BDNF mRNA and protein levels (Li et al., 2010). Moreover, accumulated phenylalanine interferes with brain development, causing microcephaly, intellectual incapacity, and behavioral issues. Neuronal cell loss, white matter abnormalities, dendritic simplicity, and synaptic density decrease may cause these problems. However, future research is needed to understand the phenylalanine-BDNF connection (Huttenlocher, 2000; Li et al., 2010).
SBH as a possible neuroprotective agent and the potential mechanism
SBH consists ten-fold higher concentration of polyphenolic when compared to other honey (Mustafa et al., 2018). Polyphenolic substances including ferulic acid, luteolin, caffeic acid, isoflavones, and flavone can increase the survival, growth, number, and size of neuronal cells (Lin et al., 2010; Yabe et al., 2010; Tangsaengvit et al., 2013; Santos, 2014; Zaidi et al., 2019; Yin et al., 2022). Moreover, other compounds such as genistein, quercetin, liquidity, isorhamnetin, and resveratrol, neurotrophin (nerve growth factor and BDNF) can also help neurites grow (Chen et al., 2009; Nakajima et al., 2011; Xu et al., 2012; Zhang et al., 2012; Karimipour et al., 2019). Other phenolic compounds, like flavonoids (luteolin, daidzein, puerarin, hesperidin) and flavonols (kaempferol, quercetin, and isorhamnetin), can increase the expression of neuron differentiation markers, like neurofilaments light subunit, synaptophysin, and synapsin (Wang et al., 2008; Lin et al., 2010; Xu et al., 2012; Karimipour et al., 2019).
Neurotrophins (NGF, BDNF, NT3, and NT4) and bioactive compounds that activate neurotrophin receptors may be able to treat neurodegenerative and neurological diseases (Xiao and Le, 2016; Machaalani and Chen, 2018). Flavones promote TrkB dimerization and tyrosine phosphorylation to imitate neurotrophin’s binding capacity (Jang et al., 2010; Gupta et al., 2013; Wurzelmann et al., 2017; Paul et al., 2021). In addition, it can stimulate downstream pathways like BDNF. For example, SBH (H.itama) enhances spatial memory by acting on BDNF and inositol tri-phosphate (Mustafa et al., 2019). The possible role of SBH works through BDNF and inositol triphosphate to inhibit NF-ĸB and mitogen-activated protein kinase (MAPK), which supressing inflammatory factors (TNF-α and IL-6) (Arshad et al., 2020).
BDNF/TrkB and the associated signaling pathways
Neurotrophins are a polypeptide growth factor family that bind to specific tyrosine kinases known as Trk receptors. The dimerization of ligand-initiated receptors and phosphorylation of tyrosine residue in the intracellular Trk domains will start the transduction of the BDNF signal (Segal and Greenberg, 1996; Huang and Reichardt, 2001; Yoshii and Constantine-Paton, 2010). BDNF is synthesized and released in an activity-dependent manner, which will bind specifically to TrkB receptors (Klein et al., 1993; Lu, 2003). The neurotrophin binding to the Trk receptor subsequently activates pathways for development and functioning related to the nervous system (Soppet et al., 1991). Neurotrophin brain-derived neurotrophic factor (BDNF) binds to high affinity to its primary receptor, TrkB. Subsequently, trigger intracellular signaling pathway phospholipase C gamma (PLC γ), phosphatidylinositol 3-kinase (PI3K), and mitogen-activated protein kinase/extracellular signal-regulated kinases (MAPK/ERK; Figure 1; Segal and Greenberg, 1996; Park and Poo, 2013).
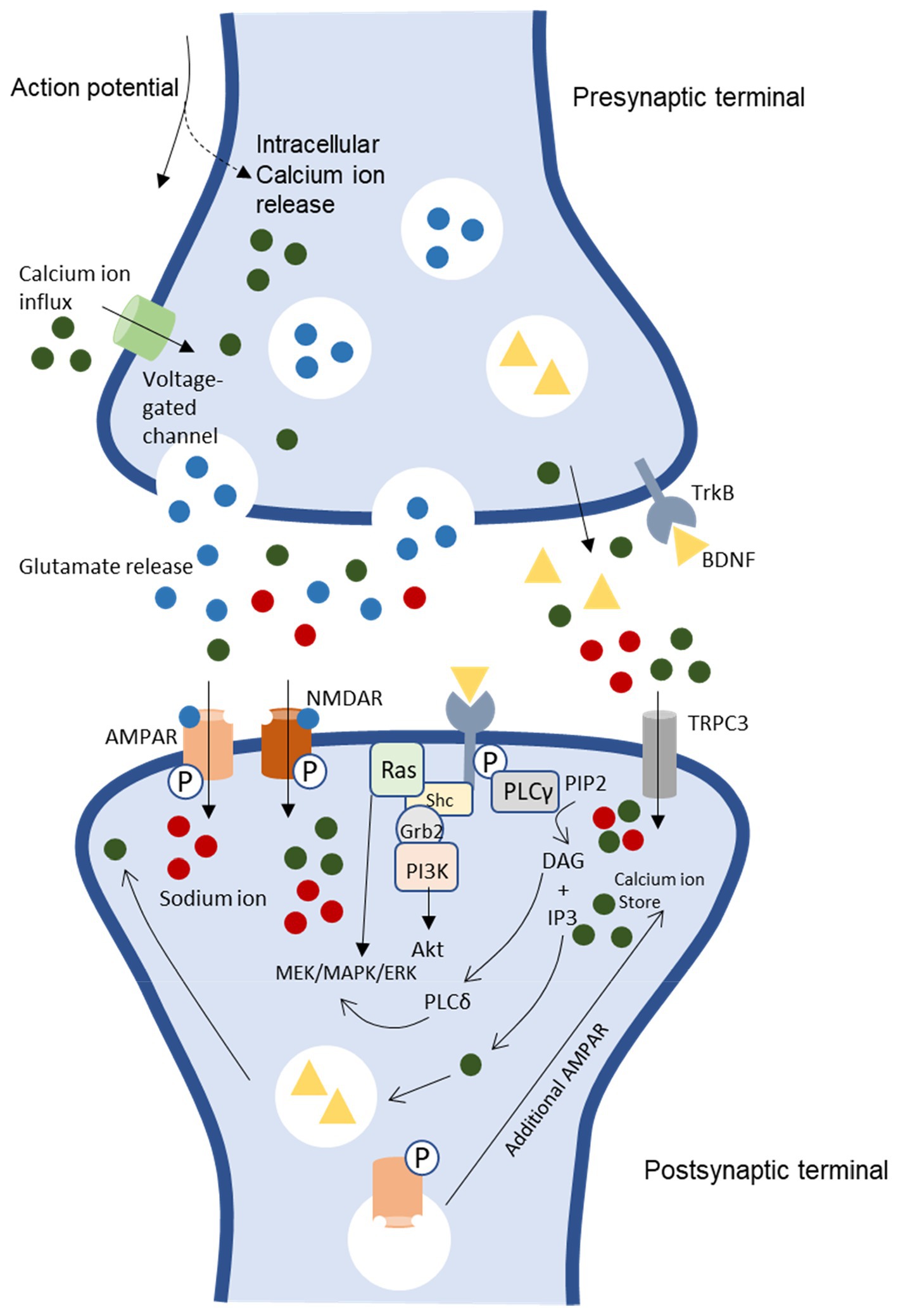
Figure 1. BDNF/TrkB signaling pathways and association to LTP induction and neuronal excitability [CaMKII, Ca2+/calmodulin-dependent protein kinase II, NMDAR, N-methyl-D-aspartate receptor, AMPAR. α-amino-3-hydroxy-5-methyl-4-isoxazolepropionic acid receptor, PKC, protein kinase C, TRPC 3, transient receptor potential-3 channels, IP3, inositol 1,4,5-triphosphate, Shc, src homology 2 domain-containing adapter protein, MAPK/ERK, mitogen-activated protein kinase/ extracellular signal-regulated kinases, PLC γ, phospholipase C gamma, PI3K, phosphatidylinositol 3-kinase, DAG, diacylglycerol, TrkB, tyrosine receptor kinase B (Murray and Holmes, 2011)].
These downstream signaling pathways are essential in neurogenesis and synaptic plasticity (Tanaka et al., 1997; Mathew and Hablitz, 2008; Yoshii and Constantine-Paton, 2014). ERK is essential for neurogenesis and promotes survival by activating genes that promote survival and suppressing proteins that promote apoptosis (Bonni et al., 1999; Barnabé-Heider and Miller, 2003). Furthermore, MAPK/ERK pathway is essential for regulating protein synthesis-dependent plasticity by upregulating the phosphorylation of ribosomal protein and translation factors (Kelleher et al., 2004; Klann and Dever, 2004). Meanwhile, the TrkB-PI3K-Akt pathway is essential in maintaining synaptic plasticity through protein translation and transportation of synaptic proteins (Sarbassov et al., 2005). This pathway, TrkB-PI3K-Akt acting via the mammalian rapamycin target (mTOR), involves protein translation (Sarbassov et al., 2005). Besides, the PI3K-Akt signaling pathway also involves the regulation of synaptic transportation (Yoshii and Constantine-Paton, 2007).
BDNF is essential in trafficking the N-methyl-D-aspartate (NMDA) receptor subunits to the membrane increasing calcium influx and additional BDNF release (Figure 1). The induction of early long-term potentiation, E-LTP, requires both post-synaptic calcium ion (Ca2+) influx and activation of the NMDAR complex (Hartmann et al., 2001; Walz et al., 2006). Backpropagating action potentials also influence calcium influx by opening voltage-dependent calcium channels. Sufficient calcium building up locally will lead to postsynaptic BDNF release, thus cause increased presynaptic vesicle cycling. These pathways support synaptic plasticity and LTP (Sabatini et al., 2001; Waters et al., 2005). Moreover, TrkB receptors interact with glutamate receptors due to postsynaptic activation. Once the membrane has sufficiently depolarized, it causes the removal of the magnesium ion (Mg2+) block on NMDAR channels, and L-glutamate binding allows NMDAR to open, which triggers Ca2+ influx (Bliss and Collingridge, 1993; Lynch, 2004).
Furthermore, BDNF–TrkB triggers cation influx through canonical transient receptor potential-3 channels (TRPC 3). Activating TrkB and PLC depletes IP3-dependent Ca2+ stores, triggering Ca2+ and sodium ion (Na+) influx through TRPC3 and perhaps enhancing synaptic Ca2+ entry via voltage-gated channels or NMDARs (Li et al., 1999; Amaral and Pozzo-Miller, 2007). As a result, transient activation of numerous enzymes is necessary to induce E-LTP due to the high spike in intracellular Ca2+ concentration and encouragement of Ca2+ inflow (Sweatt, 1999). For instance, protein kinase C (PKC) and Ca2+/calmodulin-dependent protein kinase II (CaMKII). E-LTP induction also needs the transient activation of CaMKII and PKC, and continuous activation is required to maintain E-LTP (Li et al., 1999; Amaral and Pozzo-Miller, 2007).
CaMKII and PKC become independently active during E-LTP’s maintenance phase (Sweatt, 1999). This causes the phosphorylation of α-amino-3-hydroxy-5-methyl-4-isoxazole propionic acid (AMPA) receptors, enhancing their activity and trafficking to and from the synaptic plasma membrane and insertion of the GluR1 subunit into the postsynaptic membrane (Malenka, 2003; Malenka and Bear, 2004; Shepherd and Huganir, 2007). TrkB and the release of Ca2+ from internal IP3-sensitive storage are likely implicated in the mechanisms by which BDNF increased the synaptic delivery of glutamate subunits, GluR1 (Reichardt, 2006; Lin et al., 2009). Studies have reported that LTP depends on AMPA receptor trafficking to the synaptic plasma membrane. Late-long-term potentiation (L-LTP) generated during E-LTP persists for an extended period, up to hours or days, and involves gene transcription and protein synthesis. Due to this, L-LTP induction is a good candidate for long-term molecular memory (LTM; Morris et al., 2003; Sutton and Schuman, 2006; Whitlock et al., 2006; Zhou et al., 2006).
BDNF/TrkB signaling association in synaptic plasticity
In the hippocampus, LTP is characterized by an activity-dependent change in synaptic strength and cellular processes that underlie learning and memory (Bliss and Collingridge, 1993). Various studies have suggested that the synaptic changes triggered by LTP are critical for memory formation and consolidation of long-term memories (Bliss and Collingridge, 1993; Selcher et al., 2002). Modifications in the individual excitatory synaptic strength promote the refinement and information storage related to learning and memory. E-LTP will elevate the strength of synapses within a short period, while higher stimulation will last for a longer duration, also known as L-LTP (Huang and Kandel, 1994). Besides LTP, LTD is a type of activity-dependent synaptic weakening that lasts long. LTD is crucial in synaptic connectivity refinement throughout development (Dudek and Bear, 1993). LTD or depotentiation mechanisms might decrease hyperexcitability due to the similarities of low-frequency stimulation patterns with LTD induction (Albensi et al., 2004).
Studies have demonstrated a high correlation between hippocampus neurogenesis and cognitive ability (Zhou et al., 2004). Improvement of learning and memory abilities are associated with increased neurogenesis and new neurons formed in the hippocampus during enrichment, which is critical for improving the ability to store new information in long-term memory (Bruel-Jungerman et al., 2005). BDNF–TrkB signaling action on the postsynaptic neuron is crucial for excitatory synaptic transmission and LTP induction (Yury et al., 2002). An increase in neuronal excitability will induce synaptic plasticity thus, enhance BDNF signaling. A study has suggested that BDNF–TrkB signaling triggers glutamate release and binds to postsynaptic receptors for LTP induction. The reduction or absence of BDNF and TrkB cause LTP inhibition and learning dysfunctionality (Korte et al., 1995; Figurov et al., 1996; Minichiello et al., 1999). Therefore, shows that BDNF is crucial in neurogenesis, axonal and dendritic sprouting and LTP via a TrkB-dependent manner (Patapoutian and Reichardt, 2001). However, this might cause neuronal hyperactivity, resulting in excitotoxicity (Patapoutian and Reichardt, 2001).
Studies have reported that NMDAR subunits, NR2A-NMDA receptor plays an essential role in long-term potential (LTP) production, and the NR2B-NMDA receptor plays a vital role in long-term depression (LTD) induction in the CA1 region of hippocampal brain region (Liu et al., 2004; Evans, 2008). A study has shown that the expression level of NMDA subunit receptor levels changes upon the application of BDNF. The expression level of NR1 and NR2A increase while NR2B decreases (Glazner and Mattson, 2000). Calcium ions released upon activation of NMDA receptors can cause necrosis (cell swelling) and decrease apoptosis (cell shrinkage and chromatin fragmentation; Farmer et al., 2004). These properties might be beneficial and harmful because they induce hyperexcitability in the dentate gyrus neuronal circuit, resulting in widespread excitotoxicity (Scharfman et al., 1999; Feng et al., 2003; Koyama et al., 2004). Dysfunctionality in excitatory neuronal networks might play a pivotal role in various diseases (Bliss and Collingridge, 1993). NMDA and AMPA receptors are involved in the induction of LTP and epileptogenesis (Liu et al., 2004; Evans, 2008).
BDNF release and Trk receptor activity trigger the development of hyperexcitable circuits, and synaptic plasticity and promote NMDA receptors in the exercise KA model (Koyama et al., 2004). The application of BDNF activates the sodium channel and triggers the influx of calcium ions. Therefore, resulting in postsynaptic neuron depolarization of dentate granule cells (Kafitz et al., 1999). High stimulation will trigger calcium ion influx via NMDA receptors activation, and AMPA receptors generate excitatory postsynaptic potentials, EPSC (Nowak et al., 1984; Malenka et al., 1988; Nicoll and Malenka, 1999). Both AMPA/ kainate receptor-mediated EPSCs are involved in moderating fast glutamatergic synaptic activity (Represa et al., 1987; Cossart et al., 2002). Besides, BDNF also facilitates excitatory synaptic transmission in the hippocampal CA1 region (Kang and Schuman, 1995). However, the excessive calcium ion influx can lead to glutamate excitotoxicity via NMDA receptors, which contribute to brain insults such as anoxia, ischemia, and seizure, thus resulting in neuronal damage (Meldrum, 1993; Murray and Holmes, 2011).
BDNF/TrkB signaling association in neurological disorder
Activation of the TrkB receptor also plays a pivotal role in the central nervous system in promoting excitatory glutamate-mediated synaptic transmission and inhibitory synaptic transmission. BDNF and its receptor, TrkB, are highly expressed in neuronal and astrocytes in several brain regions, including the hippocampus and cerebral cortex (Croll et al., 1999; He et al., 2004; Holt et al., 2019). Various studies have shown that BDNF plays a vital role in exerting neuroprotective effects against glutamate toxicity (Almeida et al., 2005; Jiang et al., 2005). The pattern of BDNF expression observed in this study was similar to the pattern shown in various neurodegenerative models. BDNF as a treatment application significantly reduces neuronal cell death in the neurotoxin-induced model via ERK-induced signaling pathways (Ventimiglia et al., 1995; Bogush et al., 2007). Therefore, it shows that BDNF might exert neuroprotective effects against neuronal insults.
Studies on the relationship mechanism between neuronal plasticity and seizures were usually associated with the hippocampus brain region (Chen et al., 2016). Studies have suggested that the elevated neurotrophic factors expression level following seizure induction might be associated with maintaining long-lasting structural and functional changes contributing to epileptogenesis (Merlio et al., 1993; Conner et al., 1997). BDNF is crucial in developing and maintaining neuronal populations within the central nervous system BDNF can be found in neurons, astrocytes, and microglia, which have a pivotal role in neuronal excitability in various conditions, including epilepsy (Parpura and Zorec, 2010). BDNF expression level was upregulated in pyramidal neuronal cells in the hippocampal region, amygdala, entorhinal cortex, and piriform cortex (Conner et al., 1997). The principal neurons in the hippocampus are excitatory neurons that utilize glutamate receptors to generate fast-acting postsynaptic currents (EPSCs; Yehezkel, 2008). These brain regions are the main areas affected during epilepsy (Binder et al., 2001).
The expression level of BDNF changes during neurotoxicity and in neurodegenerative diseases (Ballarín et al., 1991; Tanila, 2017). Neurotoxin-induced neurodegenerative model induces robust upregulation of BDNF expression levels (Ballarín et al., 1991; Sathanoori et al., 2004). The upregulation of BDNF expression level induces glutamatergic transmission, and limbic circuit neural activity cause potentiation induction (Patterson et al., 1992). BDNF also triggers axonal growth, promotes basal dendritic granule cells formation, facilitates dendritic spine morphological changes, and triggers neurogenesis in the adult brain, such as the dentate gyrus (Lowenstein and Arsenault, 1996; Murphy et al., 1998; Wilson Horch et al., 1999; Danzer et al., 2002; Lee et al., 2002; Tyler and Pozzo-Miller, 2003). The study suggested that the growth-associated activity induced by BDNF might contribute to excitability changes, epileptogenesis, and chronic epilepsy (Lähteinen et al., 2004).
Robust upregulation of BDNF expression was observed after seizures regardless of the method applied (Bengzon et al., 1993; Humpel et al., 1993). Activity-dependent BDNF release and Trk receptor activity cause abnormal mossy fiber sprouting resulting in hyperexcitable circuits activity (Koyama et al., 2004). The study has shown that BDNF does not increase severity in the KA-induced model but the severity of injury in pyramidal neurons of the CA3 hippocampal region. Brain insult, hypoxia, and seizure cause the expression level of BDNF mRNA level to increase in dentate granule cells, promoting BDNF release from mossy fiber and anterograde transport. Therefore, it resulted in the activation of TrKB receptors in hilus and CA3 stratum lucidum. The study explains that vital excitatory mossy fiber input into CA3 pyramidal cells might primarily promote epileptogenesis through BDNF (Binder et al., 2001).
Conclusion
The bioactive compounds in SBH contribute to antioxidant and anti-inflammatory effects, which is crucial to combat various neurological disorders. These beneficial effects might contribute to the neuroprotective effects by preventing further damage to the central nervous system. Studies have suggested that flavonoids and phenolic compounds present in honey might potentially reduce or prevent these behavioral and cognitive abnormalities caused by oxidative stress, protein processing, neurotrophic signaling, synaptic dysfunction, inflammation, and cell death. The flavones compound can mimic the ability of BDNF on the downstream pathways that is essential in neurogenesis and synaptic plasticity. Moreover, before considering phenylalanine compounds as treatments for various neurological-related disorders, more studies are required. Studies are needed to learn more about the effects of neurotrophic factors and how they work at the molecular level. These results could help build better treatments for neurological disorders and dysfunctions. More investigation is required to fully understand the bioactive substances, molecular processes, and essential elements of SBH that influence the nootropic activity to build this new potential quality standard. This entails developing new delivery methods, such as nanoparticles or other formulations that promote brain uptake, then assessed in the same model to determine the most effective.
Author contributions
All authors listed have made a substantial, direct, and intellectual contribution to the work and approved it for publication.
Funding
This article is funded by the Ministry of Higher Education Malaysia for Fundamental Research Grant Scheme with Project Code: FRGS/1/2021/SKK06/USM/02/13 and Universiti Sains Malaysia RU Top-down 2020; 1001/PPSP/8070015.
Conflict of interest
The reviewer SLT declared a past co-authorship with the author SNHH to the handling editor. The authors declare that the research was conducted in the absence of any commercial or financial relationships that could be construed as a potential conflict of interest.
Publisher’s note
All claims expressed in this article are solely those of the authors and do not necessarily represent those of their affiliated organizations, or those of the publisher, the editors and the reviewers. Any product that may be evaluated in this article, or claim that may be made by its manufacturer, is not guaranteed or endorsed by the publisher.
References
Abbott, N. J. (2000). Inflammatory mediators and modulation of blood-brain barrier permeability. Cell. Mol. Neurobiol. 20, 131–147. doi: 10.1023/A:1007074420772
Abd Al Haleem, E. N., Ahmed, H. I., and El-Naga, R. N. (2021). Lycopene and Chrysin through mitigation of Neuroinflammation and oxidative stress exerted antidepressant effects in clonidine-induced depression-like behavior in rats. J. Diet. Suppl. 11, 1–20. doi: 10.1080/19390211.2021.1988797
Abd Aziz, C. B., Ahmad Suhaimi, S. Q., Hasim, H., Ahmad, A. H., Long, I., and Zakaria, R. (2019). Effects of Tualang honey in modulating nociceptive responses at the spinal cord in offspring of prenatally stressed rats. J. Integr. Med. 17, 66–70. doi: 10.1016/j.joim.2018.12.002
Abd Jalil, M. A., Kasmuri, A. R., and Hadi, H. (2017). Stingless bee honey, the natural wound healer: a review. Skin Pharmacol. Physiol. 30, 66–75. doi: 10.1159/000458416
Abdul Malik, N., Mohamed, M., Mustafa, M. Z., and Zainuddin, A. (2020). In vitro modulation of extracellular matrix genes by stingless bee honey in cellular aging of human dermal fibroblast cells. J. Food Biochem. 44:e13098. doi: 10.1111/jfbc.13098
Abdulmajeed, W. I., Sulieman, H. B., Zubayr, M. O., Imam, A., Amin, A., Biliaminu, S. A., et al. (2016). Honey prevents neurobehavioural deficit and oxidative stress induced by lead acetate exposure in male wistar rats-a preliminary study. Metab. Brain Dis. 31, 37–44. doi: 10.1007/s11011-015-9733-6
Abu Bakar, M. F., Sanusi, S. B., Abu Bakar, F. I., Cong, O. J., and Mian, Z. (2017). Physicochemical and antioxidant potential of raw unprocessed honey from Malaysian stingless bees. Pak. J. Nutr. 16, 888–894. doi: 10.3923/pjn.2017.888.894
Adeniyi, I. A., Babalola, K. T., Adekoya, V. A., Oyebanjo, O., Ajayi, A. M., and Onasanwo, S. A. (2022). Neuropharmacological effects of honey in lipopolysaccharide-induced neuroinflammation, cognitive impairment, anxiety and motor impairment. Nutr. Neurosci. 26, 1–14. doi: 10.1080/1028415X.2022.2063578
Ahmad, F., Seerangan, P., Mustafa, M. Z., Osman, Z. F., Abdullah, J. M., and Idris, Z. (2019). Anti-Cancer properties of Heterotrigona itama sp. honey via induction of apoptosis in malignant glioma cells. Malays. J Med. Sci. 26, 30–39. doi: 10.21315/mjms2019.26.2.4
Ahmed, H., Khan, M. A., Ali Zaidi, S. A., and Muhammad, S. (2021). In Silico and in vivo: Evaluating the therapeutic potential of Kaempferol, quercetin, and Catechin to treat chronic epilepsy in a rat model. Front. Bioeng. Biotechnol. 9:754952. doi: 10.3389/fbioe.2021.754952
Ahmed, S., and Othman, N. H. (2013). Review of the medicinal effects of tualang honey and a comparison with manuka honey. Malays. J. Med. Sci. 20, 6–13. doi: 10.5539/jas.v9n13p3
Al Kafaween, M. A., Hilmi, A. B. M., Khan, R. S., Bouacha, M., and Amonov, M. (2019). Effect of Trigona honey on Escherichia coli cell culture growth: in vitro study. J. Apither. 5, 10–17. doi: 10.5455/ja.20190407083601
Albensi, B. C., Ata, G., Schmidt, E., Waterman, J. D., and Janigro, D. (2004). Activation of long-term synaptic plasticity causes suppression of epileptiform activity in rat hippocampal slices. Brain Res. 998, 56–64. doi: 10.1016/j.brainres.2003.11.010
Al-Hatamleh, M. A. I., Boer, J. C., Wilson, K. L., Plebanski, M., Mohamud, R., and Mustafa, M. Z. (2020). Antioxidant-based medicinal properties of stingless bee products: recent Progress and future directions. Biomol. Ther. 10. doi: 10.3390/biom10060923
Almeida, R. D., Manadas, B. J., Melo, C. V., Gomes, J. R., Mendes, C. S., Grãos, M. M., et al. (2005). Neuroprotection by BDNF against glutamate-induced apoptotic cell death is mediated by ERK and PI3-kinase pathways. Cell Death Differ. 12, 1329–1343. doi: 10.1038/sj.cdd.4401662
Alvarez-Suarez, J. M., Giampieri, F., Brenciani, A., Mazzoni, L., Gasparrini, M., González-Paramás, A. M., et al. (2018). Apis mellifera vs Melipona beecheii Cuban polifloral honeys: a comparison based on their physicochemical parameters, chemical composition and biological properties. LWT 87, 272–279. doi: 10.1016/j.lwt.2017.08.079
Amaral, M. D., and Pozzo-Miller, L. (2007). TRPC3 channels are necessary for brain-derived neurotrophic factor to activate a nonselective cationic current and to induce dendritic spine formation. J. Neurosci. 27, 5179–5189. doi: 10.1523/JNEUROSCI.5499-06.2007
Anwar, J., Spanevello, R. M., Thomé, G., Stefanello, N., Schmatz, R., Gutierres, J., et al. (2012). Effects of caffeic acid on behavioral parameters and on the activity of acetylcholinesterase in different tissues from adult rats. Pharmacol. Biochem. Behav. 103, 386–394. doi: 10.1016/j.pbb.2012.09.006
Arshad, N., Lin, T. S., and Yahaya, M. F. (2020). Stingless bee honey reduces anxiety and improves memory of the metabolic disease-induced rats. CNS Neurol. Disord. Drug Targets 19, 115–126. doi: 10.2174/1871527319666200117105133
Arulselvan, P., Fard, M. T., Tan, W. S., Gothai, S., Fakurazi, S., Norhaizan, M. E., et al. (2016). Role of antioxidants and natural products in inflammation. Oxid. Med. Cell. Longev. 2016:5276130. doi: 10.1155/2016/5276130
Ashrafi, M. R., Shams, S., Nouri, M., Mohseni, M., Shabanian, R., Yekaninejad, M. S., et al. (2007). A probable causative factor for an old problem: selenium and glutathione peroxidase appear to play important roles in epilepsy pathogenesis. Epilepsia 48, 1750–1755. doi: 10.1111/j.1528-1167.2007.01143.x
Ávila, S., Beux, M. R., Ribani, R. H., and Zambiazi, R. C. (2018). Stingless bee honey: quality parameters, bioactive compounds, health-promotion properties and modification detection strategies. Trends Food Sci. Technol. 81, 37–50. doi: 10.1016/j.tifs.2018.09.002
Ávila, S., Hornung, P. S., Teixeira, G. L., Malunga, L. N., Apea-Bah, F. B., Beux, M. R., et al. (2019). Bioactive compounds and biological properties of Brazilian stingless bee honey have a strong relationship with the pollen floral origin. Food Res. Int. 123, 1–10. doi: 10.1016/j.foodres.2019.01.068
Aziz, M. S. A., Giribabu, N., Rao, P. V., and Salleh, N. (2017). Pancreatoprotective effects of Geniotrigona thoracica stingless bee honey in streptozotocin-nicotinamide-induced male diabetic rats. Biomed. Pharmacother. 89, 135–145. doi: 10.1016/j.biopha.2017.02.026
Ballarín, M., Ernfors, P., Lindefors, N., and Persson, H. (1991). Hippocampal damage and kainic acid injection induce a rapid increase in mRNA for BDNF and NGF in the rat brain. Exp. Neurol. 114, 35–43. doi: 10.1016/0014-4886(91)90082-N
Barnabé-Heider, F., and Miller, F. D. (2003). Endogenously produced neurotrophins regulate survival and differentiation of cortical progenitors via distinct signaling pathways. J. Neurosci. 23, 5149–5160. doi: 10.1523/JNEUROSCI.23-12-05149.2003
Barros, D., Amaral, O. B., Izquierdo, I., Geracitano, L., do Carmo Bassols Raseira, M., Henriques, A. T., et al. (2006). Behavioral and genoprotective effects of Vaccinium berries intake in mice. Pharmacol. Biochem. Behav. 84, 229–234. doi: 10.1016/j.pbb.2006.05.001
Bartelli, B. F., and Nogueira-Ferreira, F. H. (2015). Pollination services provided by Melipona quadrifasciata Lepeletier (Hymenoptera: Meliponini) in greenhouses with Solanum lycopersicum L.(Solanaceae). Sociobiology 61, 510–516. doi: 10.13102/sociobiology.v61i4.510-516
Bengzon, J., Kokaia, Z., Ernfors, P., Kokaia, M., Leanza, G., Nilsson, O. G., et al. (1993). Regulation of neurotrophin and traka, trkb and trkc tyrosine kinase receptor messenger RNA expression in kindling. Neuroscience 53, 433–446. doi: 10.1016/0306-4522(93)90207-V
Beretta, G., Granata, P., Ferrero, M., Orioli, M., and Maffei Facino, R. (2005). Standardization of antioxidant properties of honey by a combination of spectrophotometric/fluorimetric assays and chemometrics. Anal. Chim. Acta 533, 185–191. doi: 10.1016/j.aca.2004.11.010
Biluca, F. C., Braghini, F., Gonzaga, L. V., Costa, A. C. O., and Fett, R. (2016). Physicochemical profiles, minerals and bioactive compounds of stingless bee honey (Meliponinae). J. Food Compos. Anal. 50, 61–69. doi: 10.1016/j.jfca.2016.05.007
Biluca, F. C., da Silva, B., Caon, T., Mohr, E. T. B., Vieira, G. N., Gonzaga, L. V., et al. (2020). Investigation of phenolic compounds, antioxidant and anti-inflammatory activities in stingless bee honey (Meliponinae). Food Res. Int. 129:108756. doi: 10.1016/j.foodres.2019.108756
Binder, D. K., Croll, S. D., Gall, C. M., and Scharfman, H. E. (2001). BDNF and epilepsy: too much of a good thing? Trends Neurosci. 24, 47–53. doi: 10.1016/s0166-2236(00)01682-9
Binder, D. K., Routbort, M. J., and McNamara, J. O. (1999). Immunohistochemical evidence of seizure-induced activation of trk receptors in the mossy fiber pathway of adult rat hippocampus. J. Neurosci. 19, 4616–4626. doi: 10.1523/jneurosci.19-11-04616.1999
Bliss, T. V. P., and Collingridge, G. L. (1993). A synaptic model of memory: long-term potentiation in the hippocampus. Nature 361, 31–39. doi: 10.1038/361031a0
Bogush, A., Pedrini, S., Pelta-Heller, J., Chan, T., Yang, Q., Mao, Z., et al. (2007). AKT and CDK5/p35 mediate brain-derived neurotrophic factor induction of DARPP-32 in medium size spiny neurons in vitro. J. Biol. Chem. 282, 7352–7359. doi: 10.1074/jbc.M606508200
Bolaños, J. P., Almeida, A., and Moncada, S. (2010). Glycolysis: a bioenergetic or a survival pathway? Trends Biochem. Sci. 35, 145–149. doi: 10.1016/j.tibs.2009.10.006
Bonni, A., Brunet, A., West, A. E., Datta, S. R., Takasu, M. A., and Greenberg, M. E. (1999). Cell survival promoted by the Ras-MAPK signaling pathway by transcription-dependent and-independent mechanisms. Science 286, 1358–1362. doi: 10.1126/science.286.5443.1358
Borsato, D. M., Prudente, A. S., Döll-Boscardin, P. M., Borsato, A. V., Luz, C. F. P., Maia, B. H. L. N. S., et al. (2014). Topical anti-inflammatory activity of a Monofloral honey of Mimosa scabrella provided by Melipona marginata during winter in southern Brazil. J. Med. Food 17, 817–825. doi: 10.1089/jmf.2013.0024
Bruel-Jungerman, E., Laroche, S., and Rampon, C. (2005). New neurons in the dentate gyrus are involved in the expression of enhanced long-term memory following environmental enrichment. Eur. J. Neurosci. 21, 513–521. doi: 10.1111/j.1460-9568.2005.03875.x
Budin, S. B., Jubaidi, F. F., Mohd Noor Azam, S. N. F., Mohamed Yusof, N. L., Taib, I. S., and Mohamed, J. (2017). Kelulut honey supplementation prevents sperm and testicular oxidative damage in streptozotocin-induced diabetic rats. Jurnal Teknologi 79, 89–95. doi: 10.11113/jt.v79.9674
Cai, M., Shin, B. Y., Kim, D. H., Kim, J. M., Park, S. J., Park, C. S., et al. (2011). Neuroprotective effects of a traditional herbal prescription on transient cerebral global ischemia in gerbils. J. Ethnopharmacol. 138, 723–730. doi: 10.1016/j.jep.2011.10.016
Carvalho, C. A. L., Sodré, G. S., Fonseca, A. A. O., Alves, R. M. O., Souza, B. A., and Clarton, L. (2009). Physicochemical characteristics and sensory profile of honey samples from stingless bees (Apidae: Meliponinae) submitted to a dehumidification process. An. Acad. Bras. Cienc. 81, 143–149. doi: 10.1590/S0001-37652009000100015
Chan, B. K., Haron, H., Talib, R. A., and Subramaniam, P. (2017). Physical properties, antioxidant content and anti-oxidative activities of Malaysian stingless kelulut (Trigona spp.) honey. J. Agric. Sci. 9, 32–40. doi: 10.5539/JAS.V9N13P32
Chang, S., Li, X., Zheng, Y., Shi, H., Zhang, D., Jing, B., et al. (2022). Kaempferol exerts a neuroprotective effect to reduce neuropathic pain through TLR4/NF-ĸB signaling pathway. Phytother. Res. 36, 1678–1691. doi: 10.1002/ptr.7396
Chaudhuri, S., Banerjee, A., Basu, K., Sengupta, B., and Sengupta, P. K. (2007). Interaction of flavonoids with red blood cell membrane lipids and proteins: antioxidant and antihemolytic effects. Int. J. Biol. Macromol. 41, 42–48. doi: 10.1016/j.ijbiomac.2006.12.003
Chen, N.-C., Chuang, Y. C., Huang, C. W., Lui, C. C., Lee, C. C., Hsu, S. W., et al. (2016). Interictal serum brain-derived neurotrophic factor level reflects white matter integrity, epilepsy severity, and cognitive dysfunction in chronic temporal lobe epilepsy. Epilepsy Behav. 59, 147–154. doi: 10.1016/j.yebeh.2016.02.029
Chen, Z., Wang, J. L., Liu, R. T., Ren, J. P., Wen, L. Q., Chen, X. J., et al. (2009). Liquiritin potentiate neurite outgrowth induced by nerve growth factor in PC12 cells. Cytotechnology 60, 125–132. doi: 10.1007/s10616-009-9226-8
Chepulis, L. M., Starkey, N. J., Waas, J. R., and Molan, P. C. (2009). The effects of long-term honey, sucrose or sugar-free diets on memory and anxiety in rats. Physiol. Behav. 97, 359–368. doi: 10.1016/j.physbeh.2009.03.001
Chew, C. Y., Chua, L. S., Soontorngun, N., and Lee, C. T. (2018). Discovering potential bioactive compounds from Tualang honey. Agric. Nat. Resour. 52, 361–365. doi: 10.1016/j.anres.2018.10.011
Chuttong, B., Chanbang, Y., Sringarm, K., and Burgett, M. (2016). Physicochemical profiles of stingless bee (Apidae: Meliponini) honey from south East Asia (Thailand). Food Chem. 192, 149–155. doi: 10.1016/j.foodchem.2015.06.089
Cianciosi, D., Forbes-Hernández, T., Afrin, S., Gasparrini, M., Reboredo-Rodriguez, P., Manna, P., et al. (2018). Phenolic compounds in honey and their associated health benefits: a review. Molecules 23. doi: 10.3390/molecules23092322
Conner, J. M., Lauterborn, J. C., Yan, Q., Gall, C. M., and Varon, S. (1997). Distribution of brain-derived neurotrophic factor (BDNF) protein and mRNA in the Normal adult rat CNS: evidence for anterograde axonal transport. J. Neurosci. 17, 2295–2313. doi: 10.1523/JNEUROSCI.17-07-02295.1997
Copmans, D., Orellana-Paucar, A. M., Steurs, G., Zhang, Y., Ny, A., Foubert, K., et al. (2018). Methylated flavonoids as anti-seizure agents: naringenin 4′, 7-dimethyl ether attenuates epileptic seizures in zebrafish and mouse models. Neurochem. Int. 112, 124–133. doi: 10.1016/j.neuint.2017.11.011
Cossart, R., Epsztein, J., Tyzio, R., Becq, H., Hirsch, J., Ben-Ari, Y., et al. (2002). Quantal release of glutamate generates pure Kainate and mixed AMPA/Kainate EPSCs in hippocampal neurons. Neuron 35, 147–159. doi: 10.1016/S0896-6273(02)00753-5
Crespo, I., García-Mediavilla, M. V., Almar, M., González, P., Tuñón, M. J., Sánchez-Campos, S., et al. (2008). Differential effects of dietary flavonoids on reactive oxygen and nitrogen species generation and changes in antioxidant enzyme expression induced by proinflammatory cytokines in Chang liver cells. Food Chem. Toxicol. 46, 1555–1569. doi: 10.1016/j.fct.2007.12.014
Croll, S. D., Suri, C., Compton, D. L., Simmons, M. V., Yancopoulos, G. D., Lindsay, R. M., et al. (1999). Brain-derived neurotrophic factor transgenic mice exhibit passive avoidance deficits, increased seizure severity and in vitro hyperexcitability in the hippocampus and entorhinal cortex. Neuroscience 93, 1491–1506. doi: 10.1016/S0306-4522(99)00296-1
da Silva, I. A. A., da Silva, T. M. S., Camara, C. A., Queiroz, N., Magnani, M., de Novais, J. S., et al. (2013). Phenolic profile, antioxidant activity and palynological analysis of stingless bee honey from Amazonas, northern Brazil. Food Chem. 141, 3552–3558. doi: 10.1016/j.foodchem.2013.06.072
Dajas, F., Rivera-Megret, F., Blasina, F., Arredondo, F., Abin-Carriquiry, J. A., Costa, G., et al. (2003). Neuroprotection by flavonoids. Braz. J. Med. Biol. Res. 36, 1613–1620. doi: 10.1590/S0100-879X2003001200002
Dan Dunn, J., Alvarez, L. A. J., Zhang, X., and Soldati, T. (2015). Reactive oxygen species and mitochondria: a nexus of cellular homeostasis. Redox Biol. 6, 472–485. doi: 10.1016/j.redox.2015.09.005
Danzer, S. C., Crooks, K. R. C., Lo, D. C., and McNamara, J. O. (2002). Increased expression of brain-derived neurotrophic factor induces formation of basal dendrites and axonal branching in dentate granule cells in hippocampal explant cultures. J. Neurosci. 22, 9754–9763. doi: 10.1523/JNEUROSCI.22-22-09754.2002
David, Y., Cacheaux, L. P., Ivens, S., Lapilover, E., Heinemann, U., Kaufer, D., et al. (2009). Astrocytic potassium and glutamate buffering controls synaptic responses in a frequency-dependent manner. Epilepsia 50, 10588–10599. doi: 10.1523/JNEUROSCI.2323-09.2009
de Almeida-Muradian, L. B., Stramm, K. M., Horita, A., Barth, O. M., da Silva de Freitas, A., and Estevinho, L. M. (2013). Comparative study of the physicochemical and palynological characteristics of honey from M elipona subnitida and a pis mellifera. Int. J. Food Sci. Technol. 48, 1698–1706. doi: 10.1111/ijfs.12140
de Oliveira Cruz, D., and de Oliveira Campos, A. (2009). Polinização por abelhas em cultivos protegidos. Curr. Agric. Sci. Technol. 15, 5–10. Available at: https://periodicos.ufpel.edu.br/ojs2/index.php/CAST/article/view/1979
de Oliveira, R. G., Luna, A. C., Freitas, L. S., and Araújo, E. D. (2017). Screening for quality indicators and phenolic compounds of biotechnological interest in honey samples from six species of stingless bees (Hymenoptera: Apidae). Food Sci. Technol. 37, 552–557. doi: 10.1590/1678-457X.25716
de Oliveira Neto, E. A., and Santos, D. D. C. (2011). Physical-chemical analysis of honeys from Africanized and native bees. Rev. Inst. Adolfo Lutz 70, 132–138. doi: 10.53393/rial.2011.v70.32562
de Sousa, J. M. B. (2016). Sugar profile, physicochemical and sensory aspects of monofloral honeys produced by different stingless bee species in Brazilian semi-arid region. LWT Food Sci. Technol. 65, 645–651. doi: 10.1016/j.lwt.2015.08.058
Do Nascimento, A. S., Marchini, L., Carvalho, C., Araújo, D., Olinda, R., and Silveira, T. (2015). Physical-chemical parameters of honey of stingless bee (Hymenoptera: Apidae). Am. Chem. Sci. J. 7, 139–149. doi: 10.9734/ACSJ/2015/17547
Dudek, S. M., and Bear, M. F. (1993). Bidirectional long-term modification of synaptic effectiveness in the adult and immature hippocampus. J. Neurosci. 13, 2910–2918. doi: 10.1523/JNEUROSCI.13-07-02910.1993
Dugan, L. L., Sensi, S. L., Canzoniero, L. M., Handran, S. D., Rothman, S. M., Lin, T. S., et al. (1995). Mitochondrial production of reactive oxygen species in cortical neurons following exposure to N-methyl-D-aspartate. J. Neurosci. 15, 6377–6388. doi: 10.1523/JNEUROSCI.15-10-06377.1995
Engwa, G. A. (2018). Free radicals and the role of plant phytochemicals as antioxidants against oxidative stress-related diseases. Phytochem. Source Antioxidants Role Dis. Prev. 7, 49–74. doi: 10.5772/intechopen.76719
Evans, G. L. (2008). The Induction of Long-Term Potentiation Attenuates Kainic Acid-Induced Excitotoxicity. Vancouver, BC: University of British Columbia.
Farmer, J., Zhao, X., van Praag, H., Wodtke, K., Gage, F. H., and Christie, B. R. (2004). Effects of voluntary exercise on synaptic plasticity and gene expression in the dentate gyrus of adult male Sprague–dawley rats in vivo. Neuroscience 124, 71–79. doi: 10.1016/j.neuroscience.2003.09.029
Fatima, I. J., Mohd Hilmi, A. B., Salwani, I., and Lavaniya, M. (2018). Physicochemical characteristics of Malaysian stingless bee honey from trigona species, IIUM Med. J. Malays. 17, 187–191. doi: 10.31436/imjm.v17i1.1030
Feng, L., Molnár, P., and Nadler, J. V. (2003). Short-term frequency-dependent plasticity at recurrent mossy Fiber synapses of the epileptic brain. J. Neurosci. 23, 5381–5390. doi: 10.1523/JNEUROSCI.23-12-05381.2003
Ferguson, L. R., Zhu, S., and Harris, P. J. (2005). Antioxidant and antigenotoxic effects of plant cell wall hydroxycinnamic acids in cultured HT-29 cells. Mol. Nutr. Food Res. 49, 585–593. doi: 10.1002/mnfr.200500014
Ferreres, F., Andrade, P., and Tomás-Barberán, F. A. (1994). Flavonoids from Portuguese heather honey. Z. Lebensm. Unters. Forsch. 199, 32–37. doi: 10.1007/BF01192949
Figurov, A., Pozzo-Miller, L. D., Olafsson, P., Wang, T., and Lu, B. (1996). Regulation of synaptic responses to high-frequency stimulation and LTP by neurotrophins in the hippocampus. Nature 381, 706–709. doi: 10.1038/381706a0
Gandhi, S., and Abramov, A. Y. (2012). Mechanism of oxidative stress in neurodegeneration. Oxid. Med. Cell. Longev. 2012:428010. doi: 10.1155/2012/428010
Gao, J., Chi, Z. F., Liu, X. W., Shan, P. Y., and Wang, R. (2007). Mitochondrial dysfunction and ultrastructural damage in the hippocampus of pilocarpine-induced epileptic rat. Neurosci. Lett. 411, 152–157. doi: 10.1016/j.neulet.2006.10.022
Gibellini, L., Bianchini, E., de Biasi, S., Nasi, M., Cossarizza, A., and Pinti, M. (2015). (2015) ‘natural compounds modulating mitochondrial functions’. Evid. Based Complement. Alternat. Med. 2015, 1–13. doi: 10.1155/2015/527209
Glazner, G. W., and Mattson, M. P. (2000). Differential effects of BDNF, ADNF9, and TNFα on levels of NMDA receptor subunits, calcium homeostasis, and neuronal vulnerability to Excitotoxicity. Exp. Neurol. 161, 442–452. doi: 10.1006/exnr.1999.7242
Gomes, S., Dias, L. G., Moreira, L. L., Rodrigues, P., and Estevinho, L. (2010). Physicochemical, microbiological and antimicrobial properties of commercial honeys from Portugal. Food Chem. Toxicol. 48, 544–548. doi: 10.1016/j.fct.2009.11.029
González-Gallego, J., García-Mediavilla, M. V., Sánchez-Campos, S., and Tuñón, M. J. (2014). “Chapter 32—anti-inflammatory and immunomodulatory properties of dietary flavonoids” in Polyphenols in Human Health and Disease. eds. R. R. Watson, V. R. Preedy, and S. Zibadi (San Diego, CA: Academic Press), 435–452.
Gupta, V. K., You, Y., Li, J. C., Klistorner, A., and Graham, S. L. (2013). Protective effects of 7, 8-dihydroxyflavone on retinal ganglion and RGC-5 cells against excitotoxic and oxidative stress. J. Mol. Neurosci. 49, 96–104. doi: 10.1007/s12031-012-9899-x
Ha, M. C., Tadi, P., and Ciofoaia, G. A. (2019). Anterior Cerebral Artery Stroke. Treasure Island (FL): StatPearls Publishing. Available at: https://www.ncbi.nlm.nih.gov/books/NBK537333/
Halliwell, B. (1992). Reactive oxygen species and the central nervous system. J. Neurochem. 59, 1609–1623. doi: 10.1111/j.1471-4159.1992.tb10990.x
Halliwell, B. (1999). Antioxidant defence mechanisms: from the beginning to the end (of the beginning). Free Radic. Res. 31, 261–272. doi: 10.1080/10715769900300841
Han, J.-Y., Ahn, S. Y., Kim, C. S., Yoo, S. K., Kim, S. K., Kim, H. C., et al. (2012). Protection of apigenin against kainate-induced excitotoxicity by anti-oxidative effects. Biol. Pharm. Bull. 35, 1440–1446. doi: 10.1248/bpb.b110686
Hartmann, M., Heumann, R., and Lessmann, V. (2001). Synaptic secretion of BDNF after high-frequency stimulation of glutamatergic synapses. EMBO J. 20, 5887–5897. doi: 10.1093/emboj/20.21.5887
Hazirah, H., Yasmin, A. M., and Norwahidah, A. (2019). Antioxidant properties of stingless bee honey and its effect on the viability of lymphoblastoid cell line. Med. Health 14, 91–105. doi: 10.17576/MH.2019.1401.08
He, X.-P., Kotloski, R., Nef, S., Luikart, B. W., Parada, L. F., and McNamara, J. O. (2004). Conditional deletion of TrkB but not BDNF prevents Epileptogenesis in the kindling model. Neuron 43, 31–42. doi: 10.1016/j.neuron.2004.06.019
Heinemann, U. W. E., Kaufer, D., and Friedman, A. (2012). Blood-brain barrier dysfunction, TGFβ signaling, and astrocyte dysfunction in epilepsy. Glia 60, 1251–1257. doi: 10.1002/glia.22311
Holt, L. M., Hernandez, R. D., Pacheco, N. L., Torres Ceja, B., Hossain, M., and Olsen, M. L. (2019). Astrocyte morphogenesis is dependent on BDNF signaling via astrocytic TrkB.T1. eLife 8:e44667. doi: 10.7554/eLife.44667
Hong, S.-Y., Jeong, W.-S., and Jun, M. (2012). Protective effects of the key compounds isolated from Corni fructus against β-amyloid-induced neurotoxicity in PC12 cells. Molecules 17, 10831–10845. doi: 10.3390/molecules170910831
Hossen, M. S., Ali, M. Y., Jahurul, M. H. A., Abdel-Daim, M. M., Gan, S. H., and Khalil, M. I. (2017). Beneficial roles of honey polyphenols against some human degenerative diseases: a review. Pharmacol. Rep. 69, 1194–1205. doi: 10.1016/j.pharep.2017.07.002
Hou, Y., Aboukhatwa, M. A., Lei, D. L., Manaye, K., Khan, I., and Luo, Y. (2010). Anti-depressant natural flavonols modulate BDNF and beta amyloid in neurons and hippocampus of double TgAD mice. Neuropharmacology 58, 911–920. doi: 10.1016/j.neuropharm.2009.11.002
Hu, P., Wang, M., Chen, W. H., Liu, J., Chen, L., Yin, S. T., et al. (2008). Quercetin relieves chronic lead exposure-induced impairment of synaptic plasticity in rat dentate gyrus in vivo. Naunyn Schmiedeberg's Arch. Pharmacol. 378, 43–51. doi: 10.1007/s00210-008-0301-z
Huang, Y. Y., and Kandel, E. R. (1994). Recruitment of long-lasting and protein kinase A-dependent long-term potentiation in the CA1 region of hippocampus requires repeated tetanization. Learn. Memory 1, 74–82. doi: 10.1101/lm.1.1.74
Huang, E. J., and Reichardt, L. F. (2001). Neurotrophins: roles in neuronal development and function. Annu. Rev. Neurosci. 24, 677–736. doi: 10.1146/annurev.neuro.24.1.677
Humpel, C., Wetmore, C., and Olson, L. (1993). Regulation of brain-derived neurotrophic factor messenger RNA and protein at the cellular level in pentylenetetrazol-induced epileptic seizures. Neuroscience 53, 909–918. doi: 10.1016/0306-4522(93)90476-V
Huttenlocher, P. R. (2000). The neuropathology of phenylketonuria: human and animal studies. Eur. J. Pediatr. 159, S102–S106. doi: 10.1007/PL00014371
Iglesias, A., Feás, X., Rodrigues, S., Seijas, J. A., Vázquez-Tato, M. P., Dias, L. G., et al. (2012). Comprehensive study of honey with protected denomination of origin and contribution to the enhancement of legal specifications. Molecules 17, 8561–8577. doi: 10.3390/molecules17078561
Ismail, N. I., Abdul Kadir, M. R., Mahmood, N. H., Singh, O. P., Iqbal, N., and Zulkifli, R. M. (2016). Apini and Meliponini foraging activities influence the phenolic content of different types of Malaysian honey. J. Apic. Res. 55, 137–150. doi: 10.1080/00218839.2016.1207388
Ismail, N. I., Kadir, M. R. A., Zulkifli, R. M., and Mohamed, M. (2021). Comparison of physicochemical, total protein and antioxidant profiles between Malaysian apis and trigona honeys. Malaysian J. Anal. Sci. 25, 243–256.
Jafari, A., Babaei, P., Rohampour, K., and Rashtiani, S. (2022). The effect of Kaempferol on autophagy and Nrf-2 Signaling in a rat model of Aβ1-42-induced Alzheimer’s disease TT. Casp. J. Neurol. Sci. 8, 7–16. doi: 10.32598/CJNS.8.28.179.2
Jang, S.-W., Liu, X., Yepes, M., Shepherd, K. R., Miller, G. W., Liu, Y., et al. (2010). A selective TrkB agonist with potent neurotrophic activities by 7,8-dihydroxyflavone. Proc. Natl. Acad. Sci. U. S. A. 107, 2687–2692. doi: 10.1073/pnas.0913572107
Jellinger, K. A. (2003). General aspects of neurodegeneration. J. Neural Trans. Suppl. 65, 101–144. doi: 10.1007/978-3-7091-0643-3_7
Jiang, W., Li, B., Chen, Y., and Gao, S. (2017). The toxic influence of dibromoacetic acid on the hippocampus and pre-frontal cortex of rat: involvement of neuroinflammation response and oxidative stress. Metab. Brain Dis. 32, 2009–2019. doi: 10.1007/s11011-017-0095-0
Jiang, X., Tian, F., Mearow, K., Okagaki, P., Lipsky, R. H., and Marini, A. M. (2005). The excitoprotective effect of N-methyl-d-aspartate receptors is mediated by a brain-derived neurotrophic factor autocrine loop in cultured hippocampal neurons. J. Neurochem. 94, 713–722. doi: 10.1111/j.1471-4159.2005.03200.x
Kafitz, K. W., Rose, C. R., Thoenen, H., and Konnerth, A. (1999). Neurotrophin-evoked rapid excitation through TrkB receptors. Nature 401, 918–921. doi: 10.1038/44847
Kamaruzzaman, M. A., Chin, K.-Y., and Mohd Ramli, E. S. (2019). A review of potential beneficial effects of honey on bone health. Evid. Based Complement. Alternat. Med. 2019, 1–10. doi: 10.1155/2019/8543618
Kang, H., and Schuman, E. M. (1995). Long-lasting Neurotrophin-induced enhancement of synaptic transmission in the adult Hippocampus. Science 267, 1658–1662. doi: 10.1126/science.7886457
Karimipour, M., Rahbarghazi, R., Tayefi, H., Shimia, M., Ghanadian, M., Mahmoudi, J., et al. (2019). Quercetin promotes learning and memory performance concomitantly with neural stem/progenitor cell proliferation and neurogenesis in the adult rat dentate gyrus. Int. J. Dev. Neurosci. 74, 18–26. doi: 10.1016/j.ijdevneu.2019.02.005
Kek, S. P., Chin, N. L., Yusof, Y. A., Tan, S. W., and Chua, L. S. (2014). Total phenolic contents and colour intensity of Malaysian honeys from the Apis spp. and Trigona spp. bees. Agric. Agric. Sci. Procedia 2, 150–155. doi: 10.1016/j.aaspro.2014.11.022
Kelleher, R. J., Govindarajan, A., Jung, H. Y., Kang, H., and Tonegawa, S. (2004). Translational control by MAPK Signaling in long-term synaptic plasticity and memory. Cells 116, 467–479. doi: 10.1016/S0092-8674(04)00115-1
Kelly, N., Farisya, M. S. N., Kumara, T. K., and Marcela, P. (2014). Species diversity and external Nest characteristics of stingless bees in Meliponiculture. Pertanika J. Trop. Agric. Sci. 37, 293–298. Available at: http://www.pertanika.upm.edu.my/
Khalil, M. I., Alam, N., Moniruzzaman, M., Sulaiman, S. A., and Gan, S. H. (2011b). Phenolic acid composition and antioxidant properties of Malaysian honeys. J. Food Sci. 76, C921–C928. doi: 10.1111/j.1750-3841.2011.02282.x
Khalil, M. I., Mahaneem, M., Jamalullail, S. M. S., Alam, N., and Sulaiman, S. A. (2011a). Evaluation of radical scavenging activity and colour intensity of nine Malaysian honeys of different origin. J. ApiProduct and ApiMed. Sci. 3, 4–11. doi: 10.3896/IBRA.4.03.1.02
Khalil, M. I., Sulaiman, S. A., and Gan, S. H. (2010). High 5-hydroxymethylfurfural concentrations are found in Malaysian honey samples stored for more than one year. Food Chem. Toxicol. 48, 2388–2392. doi: 10.1016/j.fct.2010.05.076
Kim, S. Y., Buckwalter, M., Soreq, H., Vezzani, A., and Kaufer, D. (2012). Blood–brain barrier dysfunction–induced inflammatory signaling in brain pathology and epileptogenesis. Epilepsia 53, 37–44. doi: 10.1111/j.1528-1167.2012.03701.x
Kishore, R. K., Halim, A. S., Syazana, M. S. N., and Sirajudeen, K. N. S. (2011). Tualang honey has higher phenolic content and greater radical scavenging activity compared with other honey sources. Nutr. Res. 31(4, 322–325. doi: 10.1016/j.nutres.2011.03.001
Klann, E., and Dever, T. E. (2004). Biochemical mechanisms for translational regulation in synaptic plasticity. Nat. Rev. Neurosci. 5, 931–942. doi: 10.1038/nrn1557
Klein, R., Smeyne, R. J., Wurst, W., Long, L. K., Auerbach, B. A., Joyner, A. L., et al. (1993). Targeted disruption of the trkB neurotrophin receptor gene results in nervous system lesions and neonatal death. Cells 75, 113–122. doi: 10.1016/S0092-8674(05)80088-1
Korte, M., Carroll, P., Wolf, E., Brem, G., Thoenen, H., and Bonhoeffer, T. (1995). Hippocampal long-term potentiation is impaired in mice lacking brain-derived neurotrophic factor. Proc. Natl. Acad. Sci. 92, 8856–8860. doi: 10.1073/pnas.92.19.8856
Koyama, R., Yamada, M. K., Fujisawa, S., Katoh-Semba, R., Matsuki, N., and Ikegaya, Y. (2004). Brain-derived neurotrophic factor induces Hyperexcitable Reentrant circuits in the dentate gyrus. J. Neurosci. 24, 7215–7224. doi: 10.1523/JNEUROSCI.2045-04.2004
Kulkarni, S. R., Gurve, S. S., and Chormule, A. J. (2017). Effect of different indigenous bee attractants in onion (Allium cepa L.) crop. Ann. Plant Prot. Sci. 25, 78–82. Available at: https://www.researchgate.net/publication/330442639_Effect_of_different_indigenous_bee_attractants_in_Onion_Allium_cepa_L_crop
Kumar, A., Sehgal, N., Kumar, P., Padi, S. S. V., and Naidu, P. S. (2008). Protective effect of quercetin against ICV colchicine-induced cognitive dysfunctions and oxidative damage in rats. Phytother. Res. 22, 1563–1569. doi: 10.1002/ptr.2454
Kwapong, P. K., Ilechie, A. A., and Kusi, R. (2013). Comparative antibacterial activity of stingless bee honey and standard antibiotics against common eye pathogens. J. Microbiol. Biotechnol. Res 3, 162–168. Available at: http://hdl.handle.net/123456789/8290
Kwon, J. Y., Jeon, M. T., Jung, U. J., Kim, D. W., Moon, G. J., and Kim, S. R. (2019). Perspective: therapeutic potential of flavonoids as alternative medicines in epilepsy. Adv. Nutr. 10, 778–790. doi: 10.1093/advances/nmz047
Lage, L. G. A., Coelho, L. L., Resende, H. C., Tavares, M. G., Campos, L. A. O., and Fernandes-Salomão, T. M. (2012). Honey physicochemical properties of three species of the brazilian Melipona. An. Acad. Bras. Cienc. 84, 605–608. doi: 10.1590/S0001-37652012005000051
Lagoa, R., Graziani, I., Lopez-Sanchez, C., Garcia-Martinez, V., and Gutierrez-Merino, C. (2011). Complex I and cytochrome c are molecular targets of flavonoids that inhibit hydrogen peroxide production by mitochondria. Biochim. Biophys. Acta (BBA)-Bioenerg. 1807, 1562–1572. doi: 10.1016/j.bbabio.2011.09.022
Lähteinen, S., Pitkänen, A., Knuuttila, J., Törönen, P., and Castrén, E. (2004). Brain-derived neurotrophic factor signaling modifies hippocampal gene expression during epileptogenesis in transgenic mice. Eur. J. Neurosci. 19, 3245–3254. doi: 10.1111/j.0953-816X.2004.03440.x
Lavinas, F. C., Macedo, E. H. B. C., Sá, G. B. L., Amaral, A. C. F., Silva, J. R. A., Azevedo, M. M. B., et al. (2019). Brazilian stingless bee propolis and geopropolis: promising sources of biologically active compounds. Rev. Bras 29, 389–399. doi: 10.1016/j.bjp.2018.11.007
Lee, J., Duan, W., and Mattson, M. P. (2002). Evidence that brain-derived neurotrophic factor is required for basal neurogenesis and mediates, in part, the enhancement of neurogenesis by dietary restriction in the hippocampus of adult mice. J. Neurochem. 82, 1367–1375. doi: 10.1046/j.1471-4159.2002.01085.x
Leelaprakash, G., and Dass, S. M. (2011). Invitro anti-inflammatory activity of methanol extract of Enicostemma axillare. Int. J. Drug Dev. Res. 3, 189–196. doi: 10.25004/ijpsdr.2017.090301
Li, D., Gu, X., Lu, L., and Liang, L. (2010). Effects of phenylalanine on the survival and neurite outgrowth of rat cortical neurons in primary cultures: possible involvement of brain-derived neurotrophic factor. Mol. Cell. Biochem. 339, 1–7. doi: 10.1007/s11010-009-0364-2
Li, H.-S., Xu, X.-Z. S., and Montell, C. (1999). Activation of a TRPC3-dependent cation current through the neurotrophin BDNF. Neuron 24, 261–273. doi: 10.1016/S0896-6273(00)80838-7
Li, Q., Zhao, H. F., Zhang, Z. F., Liu, Z. G., Pei, X. R., Wang, J. B., et al. (2009a). Long-term administration of green tea catechins prevents age-related spatial learning and memory decline in C57BL/6 J mice by regulating hippocampal cyclic amp-response element binding protein signaling cascade. Neuroscience 159, 1208–1215. doi: 10.1016/j.neuroscience.2009.02.008
Li, Q., Zhao, H. F., Zhang, Z. F., Liu, Z. G., Pei, X. R., Wang, J. B., et al. (2009b). Long-term green tea catechin administration prevents spatial learning and memory impairment in senescence-accelerated mouse prone-8 mice by decreasing Aβ1-42 oligomers and upregulating synaptic plasticity–related proteins in the hippocampus. Neuroscience 163, 741–749. doi: 10.1016/j.neuroscience.2009.07.014
Lin, T. Y., Lu, C. W., and Wang, S. J. (2016). Luteolin protects the hippocampus against neuron impairments induced by kainic acid in rats. Neurotoxicology 55, 48–57. doi: 10.1016/j.neuro.2016.05.008
Lin, D.-T., Makino, Y., Sharma, K., Hayashi, T., Neve, R., Takamiya, K., et al. (2009). Regulation of AMPA receptor extrasynaptic insertion by 4.1 N, phosphorylation and palmitoylation. Nat. Neurosci. 12, 879–887. doi: 10.1038/nn.2351
Lin, C.-W., Wu, M. J., Liu, I. Y. C., Su, J. D., and Yen, J. H. (2010). Neurotrophic and Cytoprotective action of Luteolin in PC12 cells through ERK-dependent induction of Nrf2-driven HO-1 expression. J. Agric. Food Chem. 58, 4477–4486. doi: 10.1021/jf904061x
Liu, L., Wong, T. P., Pozza, M. F., Lingenhoehl, K., Wang, Y., Sheng, M., et al. (2004). Role of NMDA receptor subtypes in governing the direction of hippocampal synaptic plasticity. Science 304, 1021–1024. doi: 10.1126/science.1096615
Liu, Z., Yao, X., Sun, B., Jiang, W., Liao, C., Dai, X., et al. (2021). Pretreatment with kaempferol attenuates microglia-mediate neuroinflammation by inhibiting MAPKs–NF–κB signaling pathway and pyroptosis after secondary spinal cord injury. Free Radic. Biol. Med. 168, 142–154. doi: 10.1016/j.freeradbiomed.2021.03.037
Lopez-Sanchez, C., Poejo, J., Garcia-Lopez, V., Salazar, J., Garcia-Martinez, V., and Gutierrez-Merino, C. (2022). Kaempferol prevents the activation of complement C3 protein and the generation of reactive A1 astrocytes that mediate rat brain degeneration induced by 3-nitropropionic acid. Food Chem. Toxicol. 164:113017. doi: 10.1016/j.fct.2022.113017
Lowenstein, D. H., and Arsenault, L. (1996). Dentate granule cell layer collagen explant cultures: spontaneous axonal growth and induction by brain-derived neurotrophic factor or basic fibroblast growth factor. Neuroscience 74, 1197–1208. doi: 10.1016/0306-4522(96)00226-6
Lu, B. (2003). BDNF and activity-dependent synaptic modulation. Learn. Memory 10, 86–98. doi: 10.1101/lm.54603
Lu, L., Ben, X., Xiao, L., Peng, M., and Zhang, Y. (2018). AMP-activated protein kinase activation in mediating phenylalanine-induced neurotoxicity in experimental models of phenylketonuria. J. Inherit. Metab. Dis. 41, 679–687. doi: 10.1007/s10545-017-0115-6
Lucas, D. R., and Newhouse, J. P. (1957). The toxic effect of sodium L-glutamate on the inner layers of the retina. A.M.A. Arch. Ophthalmol. 58, 193–201. doi: 10.1001/archopht.1957.00940010205006
Lynch, M. A. (2004). Long-term potentiation and memory. Physiol. Rev. 84, 87–136. doi: 10.1152/physrev.00014.2003
Machaalani, R., and Chen, H. (2018). Brain derived neurotrophic factor (BDNF), its tyrosine kinase receptor B (TrkB) and nicotine. Neurotoxicology 65, 186–195. doi: 10.1016/j.neuro.2018.02.014
Malenka, R. C. (2003). Synaptic plasticity and AMPA receptor trafficking. Ann. N. Y. Acad. Sci. 1003, 1–11. doi: 10.1196/annals.1300.001
Malenka, R. C., and Bear, M. F. (2004). LTP and LTD: an embarrassment of riches. Neuron 44, 5–21. doi: 10.1016/j.neuron.2004.09.012
Malenka, R. C., Kauer, J. A., Zucker, R. S., and Nicoll, R. A. (1988). Postsynaptic calcium is sufficient for potentiation of hippocampal synaptic transmission. Science 242, 81–84. doi: 10.1126/science.2845577
Mariani, E., Polidori, M. C., Cherubini, A., and Mecocci, P. (2005). Oxidative stress in brain aging, neurodegenerative and vascular diseases: an overview. J. Chromatogr. B 827, 65–75. doi: 10.1016/j.jchromb.2005.04.023
Mathew, S. S., and Hablitz, J. J. (2008). Calcium release via activation of presynaptic IP3 receptors contributes to kainate-induced IPSC facilitation in rat neocortex. Neuropharmacology. 2008/05/14 55, 106–116. doi: 10.1016/j.neuropharm.2008.05.005
McNamara, J. O., and Scharfman, H. E. (2012). “Temporal lobe epilepsy and the BDNF receptor, TrkB” in Jasper's Basic Mechanisms of the Epilepsies. eds. J. L. Noebels, M. Avoli, and M. A. Rogawski, et al. (Bethesda (MD): National Center for Biotechnology Information)
Medda, N., Patra, R., Ghosh, T. K., and Maiti, S. (2020). Neurotoxic mechanism of arsenic: synergistic effect of mitochondrial instability, oxidative stress, and hormonal-neurotransmitter impairment. Biol. Trace Elem. Res. 198, 8–15. doi: 10.1007/s12011-020-02044-8
Meldrum, B. S. (1993). Excitotoxicity and selective neuronal loss in epilepsy. Brain Pathol. 3, 405–412. doi: 10.1111/j.1750-3639.1993.tb00768.x
Meo, S. A., Al-Asiri, S. A., Mahesar, A. L., and Ansari, M. J. (2017). Role of honey in modern medicine. Saudi J. Biol. Sci. 24, 975–978. doi: 10.1016/j.sjbs.2016.12.010
Merlio, J.-P., Ernfors, P., Kokaia, Z., Middlemas, D. S., Bengzon, J., Kokaia, M., et al. (1993). Increased production of the TrkB protein tyrosine kinase receptor after brain insults. Neuron 10, 151–164. doi: 10.1016/0896-6273(93)90307-D
Michener, C. D. (2013). “The Meliponini. Vit., SRM Pedro & DW Roubik” in PotHoney: A Legacy of Stingless Bees (New York: Springer), 3–17.
Migliore, L., Fontana, I., Colognato, R., Coppede, F., Siciliano, G., and Murri, L. (2005). Searching for the role and the most suitable biomarkers of oxidative stress in Alzheimer’s disease and in other neurodegenerative diseases. Neurobiol. Aging 26, 587–595. doi: 10.1016/j.neurobiolaging.2004.10.002
Mijanur Rahman, M., Gan, S. H., and Khalil, M. I. (2014). “Neurological effects of honey: current and future prospects” in Evid Based Complement. Altern. Med., vol. 2014, 958721. doi: 10.1155/2014/958721
Minichiello, L., Korte, M., Wolfer, D., Kühn, R., Unsicker, K., Cestari, V., et al. (1999). Essential role for TrkB receptors in Hippocampus-mediated learning. Neuron 24, 401–414. doi: 10.1016/S0896-6273(00)80853-3
Mohamad, M. A. M., Mazlan, M. A., Ibrahim, M., Mat Yusof, A., Shamsuddin, S. A. A., Nik Hassan, N. F., et al. (2018). The effect of Malaysian stingless bee, Trigona spp. honey in promoting proliferation of the undifferentiated stem cell. Asia Pac. J. Mol. Biol. Biotechnol. 27, 10–19. doi: 10.35118/apjmbb.2019.027.1.02
Mohamed, M., Sulaiman, S. A., Jaafar, H., and Sirajudeen, K. N. S. (2012). Effect of different doses of Malaysian honey on reproductive parameters in adult male rats. Andrologia 44, 182–186. doi: 10.1111/j.1439-0272.2010.01159.x
Mohd Rafie, A. Z., Syahir, A., Wan Ahmad, W. A. N., Mustafa, M. Z., and Mariatulqabtiah, A. R. (2018). Supplementation of stingless bee honey from Heterotrigona itama improves antiobesity parameters in high-fat diet induced obese rat model. Evid. Based Complement Alternat Med 2018:6371582. doi: 10.1155/2018/6371582
Mokarrami, S., Jahanshahi, M., Elyasi, L., Badelisarkala, H., and Khalili, M. (2022). Naringin prevents the reduction of the number of neurons and the volume of CA1 in a scopolamine-induced animal model of Alzheimer’s disease (AD): a stereological study. Int. J. Neurosci. 18, 1–8. doi: 10.1080/00207454.2022.2102981
Mokaya, H. O., Nkoba, K., Ndunda, R. M., and Vereecken, N. J. (2022). Characterization of honeys produced by sympatric species of Afrotropical stingless bees (Hymenoptera, Meliponini). Food Chem. 366:130597. doi: 10.1016/j.foodchem.2021.130597
Moniruzzaman, M., Khalil, M. I., Sulaiman, S. A., and Gan, S. H. (2013a). Physicochemical and antioxidant properties of Malaysian honeys produced by Apis cerana, Apis dorsata and Apis mellifera. BMC Complement. Altern. Med. 13:43. doi: 10.1186/1472-6882-13-43
Moniruzzaman, M., Sulaiman, S. A., Khalil, M. I., and Gan, S. H. (2013b). Evaluation of physicochemical and antioxidant properties of sourwood and other Malaysian honeys: a comparison with manuka honey. Chem. Cent. J. 7:138. doi: 10.1186/1752-153X-7-138
Morris, R. G. M., Moser, E. I., Riedel, G., Martin, S. J., Sandin, J., Day, M., et al. (2003). Elements of a neurobiological theory of the hippocampus: the role of activity-dependent synaptic plasticity in memory. Philos. Trans. R. Soc. Lond. 358, 773–786. doi: 10.1098/rstb.2002.1264
Muhammad Abdul Kadar, N. N., Ahmad, F., Teoh, S. L., and Yahaya, M. F. (2022). Comparable benefits of stingless bee honey and Caffeic acid in mitigating the negative effects of metabolic syndrome on the brain. Antioxidants 11:2154. doi: 10.3390/antiox11112154
Murphy, D. D., Cole, N. B., and Segal, M. (1998). Brain-derived neurotrophic factor mediates estradiol-induced dendritic spine formation in hippocampal neurons. Proc. Natl. Acad. Sci. 95, 11412–11417. doi: 10.1073/pnas.95.19.11412
Murray, P. S., and Holmes, P. V. (2011). An overview of brain-derived neurotrophic factor and implications for excitotoxic vulnerability in the hippocampus. Int. J. Pept. 2011:654085. doi: 10.1155/2011/654085
Mustafa, M., Yaacob, N., and Sulaiman, S. (2018). Reinventing the honey industry: opportunities of the stingless bee. Malays. J. Med. Sci. 25, 1–5. doi: 10.21315/mjms2018.25.4.1
Mustafa, M. Z., Zulkifli, F. N., Fernandez, I., Mariatulqabtiah, A. R., Sangu, M., Nor Azfa, J., et al. (2019). Stingless bee honey improves spatial memory in mice, probably associated with brain-derived neurotrophic factor (BDNF) and inositol 1,4,5-triphosphate receptor type 1 (Itpr1) genes. Evid. Based Complement. Altern. Med. 2019:8258307. doi: 10.1155/2019/8258307
Nakajima, K., Niisato, N., and Marunaka, Y. (2011). Genistein enhances the NGF-induced neurite outgrowth. Biomed. Res. 32, 351–356. doi: 10.2220/biomedres.32.351
Nayan, N. S., Yazid, M. A. M., Nallappan, K., Amran, A. A., Zaidi, N. S., Zakaria, F., et al. (2020). In vitro modulation of endogenous antioxidant enzyme activities and oxidative stress in autism Lymphoblastoid cell line (ALCL) by stingless bee honey treatment. Oxid. Med. Cell. Longev. 2020:4539891. doi: 10.1155/2020/4539891
Ng, W.-J., Lye, P.-Y., Chan, Y.-J., Lau, Z.-K., and Ee, K.-Y. (2017). Synergistic effect of trigona honey and ampicillin on Staphylococcus aureus isolated from infected wound. Int. J. Pharmacol. 13, 403–407. doi: 10.3923/ijp.2017.403.407
Ngaini, Z., Hussain, H., Kelabo, E. S., Wahi, R., and Farooq, S. (2021). Chemical profiling, biological properties and environmental contaminants of stingless bee honey and propolis. J. Apic. Res. 60, 1–17. doi: 10.1080/00218839.2021.1948745
Nicoll, R. A., and Malenka, R. C. (1999). Expression mechanisms underlying NMDA receptor-dependent long-term potentiation. Ann. N. Y. Acad. Sci. 868, 515–525. doi: 10.1111/j.1749-6632.1999.tb11320.x
Nordin, A., Omar, N., Sainik, N. Q. A. V., Chowdhury, S. R., Omar, E. A., Saim, A. B., et al. (2018). Low dose stingless bee honey increases viability of human dermal fibroblasts that could potentially promote wound healing. Wound Med. 23, 22–27. doi: 10.1016/j.wndm.2018.09.005
Nowak, L., Bregestovski, P., Ascher, P., Herbet, A., and Prochiantz, A. (1984). Magnesium gates glutamate-activated channels in mouse central neurones. Nature 307, 462–465. doi: 10.1038/307462a0
Ooi, T. C., Yaacob, M. H., Rajab, N. F., Shahar, S., and Sharif, R. (2021). The stingless bee honey protects against hydrogen peroxide-induced oxidative damage and lipopolysaccharide-induced inflammation in vitro. Saudi J. Biol. Sci. 28, 2987–2994. doi: 10.1016/j.sjbs.2021.02.039
Özbalci, B., Boyaci, İ. H., Topcu, A., Kadılar, C., and Tamer, U. (2013). Rapid analysis of sugars in honey by processing Raman spectrum using chemometric methods and artificial neural networks. Food Chem. 136, 1444–1452. doi: 10.1016/j.foodchem.2012.09.064
Pan, X., Liu, X., Zhao, H., Wu, B., and Liu, G. (2020). Antioxidant, anti-inflammatory and neuroprotective effect of kaempferol on rotenone-induced Parkinson’s disease model of rats and SH-S5Y5 cells by preventing loss of tyrosine hydroxylase. J. Funct. Foods 74:104140. doi: 10.1016/j.jff.2020.104140
Park, S., Moon, N. R., Kang, S., and Kim, D. S. (2022). Ferulic acid and vinpocetine intake improves memory function by enhancing insulin sensitivity and reducing neuroinflammation and oxidative stress in type 2 diabetic animals with induced Alzheimer’s disease. J. Funct. Foods 95:105180. doi: 10.1016/j.jff.2022.105180
Park, H., and Poo, M. (2013). Neurotrophin regulation of neural circuit development and function. Nat. Rev. Neurosci. 14, 7–23. doi: 10.1038/nrn3379
Park, S. H., Sim, Y. B., Han, P. L., Lee, J. K., and Suh, H. W. (2010). Antidepressant-like effect of kaempferol and quercitirin, isolated from Opuntia ficus-indica var. saboten. Exp. Neurobiol. 19, 30–38. doi: 10.5607/en.2010.19.1.30
Parpura, V., and Zorec, R. (2010). Gliotransmission: Exocytotic release from astrocytes. Brain Res. Rev. 63, 83–92. doi: 10.1016/j.brainresrev.2009.11.008
Patapoutian, A., and Reichardt, L. F. (2001). Trk receptors: mediators of neurotrophin action. Curr. Opin. Neurobiol. 11, 272–280. doi: 10.1016/S0959-4388(00)00208-7
Patterson, S. L., Grover, L. M., Schwartzkroin, P. A., and Bothwell, M. (1992). Neurotrophin expression in rat hippocampal slices: a stimulus paradigm inducing LTP in CA1 evokes increases in BDNF and NT-3 mRNAs. Neuron 9, 1081–1088. doi: 10.1016/0896-6273(92)90067-N
Paul, R., Nath, J., Paul, S., Mazumder, M. K., Phukan, B. C., Roy, R., et al. (2021). Suggesting 7,8-dihydroxyflavone as a promising nutraceutical against CNS disorders. Neurochem. Int. 148:105068. doi: 10.1016/j.neuint.2021.105068
Pearson-Smith, J. N., and Patel, M. (2017). Metabolic dysfunction and oxidative stress in epilepsy. Int. J. Mol. Sci. doi: 10.3390/ijms18112365
Perry, G., Nunomura, A., Hirai, K., Zhu, X., Pérez, M., Avila, J., et al. (2002). Is oxidative damage the fundamental pathogenic mechanism of Alzheimer’s and other neurodegenerative diseases? Free Radic. Biol. Med. 33, 1475–1479. doi: 10.1016/s0891-5849(02)01113-9
Puttachary, S., Sharma, S., Stark, S., and Thippeswamy, T. (2015). Seizure-induced oxidative stress in temporal lobe epilepsy. Biomed. Res. Int. 2015:745613. doi: 10.1155/2015/745613
Rafiee Sardooi, A., Reisi, P., and Yazdi, A. (2021). Protective effect of honey on learning and memory impairment, depression and neurodegeneration induced by chronic unpredictable mild stress TT. Physiol-Pharmacol 25, 21–35. doi: 10.32598/ppj.25.1.90
Ramli, A. S., Basrawi, F., Yusof, M. H. B., Oumer, A. N., Johari, N. A., Muhamadb, A., et al. (2019). Experimental analysis on a novel low-temperature vacuum drying with induced nucleation technique for dewatering stingless bees honey. Dry. Technol. 37, 149–155. doi: 10.1080/07373937.2018.1444634
Ranneh, Y., Ali, F., Zarei, M., Akim, A. M., Hamid, H. A., and Khazaai, H. (2018). Malaysian stingless bee and Tualang honeys: a comparative characterization of total antioxidant capacity and phenolic profile using liquid chromatography-mass spectrometry. LWT 89, 1–9. doi: 10.1016/j.lwt.2017.10.020
Ranneh, Y., et al. (2019). Stingless bee honey protects against lipopolysaccharide induced-chronic subclinical systemic inflammation and oxidative stress by modulating Nrf2, NF-κB and p38 MAPK. Nutr. Metab. 16:15. doi: 10.1186/s12986-019-0341-z
Rao, P. V., Akim, A. M., Hamid, H. A., Khazaai, H., Fadel, A., and Mahmoud, A. M. (2016). Biological and therapeutic effects of honey produced by honey bees and stingless bees: a comparative review. Rev. Bras 26, 657–664. doi: 10.1016/j.bjp.2016.01.012
Rasmussen, C., and Cameron, S. (2009). Global stingless bee phylogeny supports ancient divergence, vicariance, and long distance dispersal. Biol. J. Linn. Soc. 99, 206–232. doi: 10.1111/j.1095-8312.2009.01341.x
Reichardt, L. F. (2006). Neurotrophin-regulated signalling pathways. Philos. Transact. R. Soc. B Biol. Sci. 361, 1545–1564. doi: 10.1098/rstb.2006.1894
Rendeiro, C., Vauzour, D., Rattray, M., Waffo-Téguo, P., Mérillon, J. M., Butler, L. T., et al. (2013). Dietary levels of pure flavonoids improve spatial memory performance and increase hippocampal brain-derived neurotrophic factor. PLoS One 8:e63535. doi: 10.1371/journal.pone.0063535
Represa, A., Tremblay, E., and Ben-Ari, Y. (1987). Kainate binding sites in the hippocampal mossy fibers: localization and plasticity. Neuroscience 20, 739–748. doi: 10.1016/0306-4522(87)90237-5
Roganovic, M., Pantovic, S., and Dizdarevic, S. (2019). Role of the oxidative stress in the pathogenesis of epilepsy. Neurol. Sci. Neurophysiol. 36, 1–8. doi: 10.5152/NSN.2019.11632
Sabatini, B. L., Maravall, M., and Svoboda, K. (2001). Ca2+ signaling in dendritic spines. Curr. Opin. Neurobiol. 11, 349–356. doi: 10.1016/S0959-4388(00)00218-X
Sahlan, M., Rahmawati, O., Pratami, D., Raffiudin, R., Mukti, R., and Hermasyah, H. (2020). The effects of stingless bee (Tetragonula biroi) honey on streptozotocin-induced diabetes mellitus in rats. Saudi J. Biol. Sci. 27, 2025–2030. doi: 10.1016/j.sjbs.2019.11.039
Saiful Yazan, L., Muhamad Zali, M. F., Mohd Ali, R., Zainal, N. A., Esa, N., Sapuan, S., et al. (2016). Chemopreventive properties and toxicity of Kelulut honey in Sprague Dawley rats induced with Azoxymethane. BioMed Res. Int. 2016:4036926. doi: 10.1155/2016/4036926
Santos, N. A. G. D. (2014). Caffeic acid phenethyl ester (CAPE) protects PC12 cells from MPP+ toxicity by inducing the expression of neuron-typical proteins. Neurotoxicology 45, 131–138. doi: 10.1016/j.neuro.2014.09.007
Sarbassov, D. D., Ali, S. M., and Sabatini, D. M. (2005). Growing roles for the mTOR pathway. Curr. Opin. Cell Biol. 17, 596–603. doi: 10.1016/j.ceb.2005.09.009
Sathanoori, M., Dias, B. G., Nair, A. R., Banerjee, S. B., Tole, S., and Vaidya, V. A. (2004). Differential regulation of multiple brain-derived neurotrophic factor transcripts in the postnatal and adult rat hippocampus during development, and in response to kainate administration. Mol. Brain Res. 130, 170–177. doi: 10.1016/j.molbrainres.2004.08.002
Sawaya, A. I. B. S. C., and Marcucci, M. (2011). Analytical methods applied to diverse types of Brazilian propolis. Chem. Cent. J. 5:27. doi: 10.1186/1752-153X-5-27
Saxena, S., Gautam, S., and Sharma, A. (2010). Physical, biochemical and antioxidant properties of some Indian honeys. Food Chem. 118, 391–397. doi: 10.1016/j.foodchem.2009.05.001
Scharfman, H., Goodman, J., Macleod, A., Phani, S., Antonelli, C., and Croll, S. (2005). Increased neurogenesis and the ectopic granule cells after intrahippocampal BDNF infusion in adult rats. Exp. Neurol. 192, 348–356. doi: 10.1016/j.expneurol.2004.11.016
Scharfman, H. E., Goodman, J. H., and Sollas, A. L. (1999). Actions of brain-derived neurotrophic factor in slices from rats with spontaneous seizures and mossy fiber sprouting in the dentate gyrus. J. Neurosci. 19, 5619–5631. doi: 10.1523/JNEUROSCI.19-13-05619.1999
Se, K. W., Ghoshal, S. K., Wahab, R. A., Ibrahim, R. K. R., and Lani, M. N. (2018). A simple approach for rapid detection and quantification of adulterants in stingless bees (Heterotrigona itama) honey. Food Res. Int. 105, 453–460. doi: 10.1016/j.foodres.2017.11.012
Segal, R. A., and Greenberg, M. E. (1996). Intracellular Signaling pathways activated by neuropathic factors. Annu. Rev. Neurosci. 19, 463–489. doi: 10.1146/annurev.ne.19.030196.002335
Selcher, J. C., Weeber, E. J., Varga, A. W., Sweatt, J. D., and Swank, M. (2002). Protein kinase signal transduction cascades in mammalian associative conditioning. Neuroscientist 8, 122–131. doi: 10.1177/107385840200800208
Selvaraju, K., Vikram, P., Soon, J. M., Krishnan, K. T., and Mohammed, A. (2019). Melissopalynological, physicochemical and antioxidant properties of honey from west coast of Malaysia. J. Food Sci. Technol. 56, 2508–2521. doi: 10.1007/s13197-019-03728-3
Shahnawaz, M., Sheikh, S. A., Hussain, M., Razaq, A., and Khan, S. S. (2013). A study on the determination of physicochemical properties of honey from different valleys of Gilgit-Baltistan. Int. J. Agric. Sci. Res. 2, 49–53. Available at: https://www.semanticscholar.org/paper/A-study-on-the-determination-of-physicochemical-of-Shahnawaz-Sheikh/0fcf8bb9693d2f00094827ae5da107f96f59f609
Shamsudin, S., Selamat, J., Sanny, M., Abd Razak, S. B., Jambari, N. N., Mian, Z., et al. (2019b). Influence of origins and bee species on physicochemical, antioxidant properties and botanical discrimination of stingless bee honey. Int. J. Food Prop. 22, 239–264. doi: 10.1080/10942912.2019.1576730
Shamsudin, S., Selamat, J., Sanny, M., Shamsul Bahari, A. R., Jambari, N. N., and Khatib, A. (2019a). A comparative characterization of physicochemical and antioxidants properties of processed Heterotrigona itama honey from different origins and classification by Chemometrics analysis. Molecules 24:3898. doi: 10.3390/molecules24213898
Shapla, U. M., Solayman, M., Alam, N., Khalil, M. I., and Gan, S. H. (2018). 5-Hydroxymethylfurfural (HMF) levels in honey and other food products: effects on bees and human health. Chem. Cent. J. 12:35. doi: 10.1186/s13065-018-0408-3
Shepherd, J. D., and Huganir, R. L. (2007). The cell biology of synaptic plasticity: AMPA receptor trafficking. Annu. Rev. Cell Dev. Biol. 23, 613–643. doi: 10.1146/annurev.cellbio.23.090506.123516
Shooshtari, M. K., Sarkaki, A., Mansouri, S. M. T., Badavi, M., Khorsandi, L., and Ghasemi Dehcheshmeh, M. (2020). Protective effects of Chrysin against memory impairment, cerebral hyperemia and oxidative stress after cerebral hypoperfusion and reperfusion in rats. Metab. Brain Dis. 35, 401–412. doi: 10.1007/s11011-019-00527-9
Silva, T. M. S., dos Santos, F. P., Evangelista-Rodrigues, A., da Silva, E. M. S., da Silva, G. S., de Novais, J. S., et al. (2013). Phenolic compounds, melissopalynological, physicochemical analysis and antioxidant activity of jandaíra (Melipona subnitida) honey. J. Food Compos. Anal. 29, 10–18. doi: 10.1016/j.jfca.2012.08.010
Silva, L. R., Videira, R., Monteiro, A. P., Valentão, P., and Andrade, P. B. (2009). Honey from Luso region (Portugal): physicochemical characteristics and mineral contents. Microchem. J. 93, 73–77. doi: 10.1016/j.microc.2009.05.005
Singh, N., and Bath, P. K. (1997). Quality evaluation of different types of Indian honey. Food Chem. 58, 129–133. doi: 10.1016/S0308-8146(96)00231-2
Singh, A., Upadhayay, S., and Mehan, S. (2021). Inhibition of c-JNK/p38MAPK signaling pathway by Apigenin prevents neurobehavioral and neurochemical defects in ethidium bromide-induced experimental model of multiple sclerosis in rats: Evidence from CSF, blood plasma and brain samples. Phytomed. Plus 1:100139. doi: 10.1016/j.phyplu.2021.100139
Slutsky, A. B., and Etnier, J. L. (2019). Caloric restriction, physical activity, and cognitive performance: a review of evidence and a discussion of the potential mediators of BDNF and TrkB. Int. J. Sport Exercise Psychol. 17, 89–105. doi: 10.1080/1612197X.2016.1223422
Soppet, D., Escandon, E., Maragos, J., Middlemas, D. S., Reid, S. W., and Blair, J. (1991). The neurotrophic factors brain-derived neurotrophic factor and neurotrophin-3 are ligands for the trkB tyrosine kinase receptor. Cells 65, 895–903. doi: 10.1016/0092-8674(91)90396-G
Sousa, J. M. B., Aquino, I. S., Magnani, M., de Albuquerque, J. R., Dos Santos, G. G., De Souza, E. L., et al. (2013). Physicochemical aspects and sensory profile of stingless bee honeys from Seridó region. State of Rio Grande do Norte, Brazil’, Semina: Ciências Agrárias 34, 1765–1774. doi: 10.5433/1679-0359.2013v34n4p1765
Souza, E. C. A., Menezes, C., and Flach, A. (2021). Stingless bee honey (Hymenoptera, Apidae, Meliponini): a review of quality control, chemical profile, and biological potential. Apidologie 52, 113–132. doi: 10.1007/s13592-020-00802-0
Souza, B. A., Roubik, D., Barth, O. M., and Heard, T. (2006). Composition of stingless bee honey: setting quality standards. Interciencia 31, 867–875. Avaiable at http://ve.scielo.org/scielo.php?pid=S0378-18442006001200008&script=sci_arttext
Sternberg, E. M. (2000). Interactions between the immune and neuroendocrine systems. Prog. Brain Res. 122, 35–42. doi: 10.1016/s0079-6123(08)62129-9
Sujanto, I. S. R., Ramly, N. S., Ghani, A. A., Huat, J. T. Y., Alias, N., and Ngah, N. (2021). The composition and functional properties of stingless bee honey: a review. Malays. J. Appl. Sci. 6, 111–127. doi: 10.37231/myjas.2021.6.1.281
Sun, Z., Meng, F. H., Tu, L. X., and Sun, L. (2019). Myricetin attenuates the severity of seizures and neuroapoptosis in pentylenetetrazole kindled mice by regulating the of BDNF-TrkB signaling pathway and modulating matrix metalloproteinase-9 and GABAA. Exp. Ther. Med. 17, 3083–3091. doi: 10.3892/etm.2019.7282
Sutherland, B. A., Rahman, R. M. A., and Appleton, I. (2006). Mechanisms of action of green tea catechins, with a focus on ischemia-induced neurodegeneration. J. Nutr. Biochem. 17, 291–306. doi: 10.1016/j.jnutbio.2005.10.005
Sutton, M. A., and Schuman, E. M. (2006). Dendritic protein synthesis, synaptic plasticity, and memory. Cells 127, 49–58. doi: 10.1016/j.cell.2006.09.014
Sweatt, J. D. (1999). Toward a molecular explanation for long-term potentiation. Learn. Mem. 6, 399–416. doi: 10.1101/lm.6.5.399
Tanaka, T., Saito, H., and Matsuki, N. (1997). Inhibition of GABAA synaptic responses by brain-derived neurotrophic factor (BDNF) in rat Hippocampus. J. Neurosci. 17, 2959–2966. doi: 10.1523/JNEUROSCI.17-09-02959.1997
Tangsaengvit, N., Kitphati, W., Tadtong, S., Bunyapraphatsara, N., and Nukoolkarn, V. (2013). Neurite outgrowth and neuroprotective effects of quercetin from Caesalpinia mimosoides Lamk. On cultured P19-derived neurons. Evid. Based Complement. Alternat. Med. 2013:838051. doi: 10.1155/2013/838051
Tanila, H. (2017). The role of BDNF in Alzheimer’s disease. Neurobiol. Dis. 97, 114–118. doi: 10.1016/j.nbd.2016.05.008
Tenore, G. C., Ritieni, A., Campiglia, P., and Novellino, E. (2012). Nutraceutical potential of monofloral honeys produced by the Sicilian black honeybees (Apis mellifera ssp. sicula). Food Chem. Toxicol. 50, 1955–1961. doi: 10.1016/j.fct.2012.03.067
Twilley, D., and Lall, N. (2014). “African plants with dermatological and ocular relevance,” in Toxicological Survey of African Medicinal Plants. Elsevier, 493–512.
Tyler, W. J., and Pozzo-Miller, L. (2003). Miniature synaptic transmission and BDNF modulate dendritic spine growth and form in rat CA1 neurones. J. Physiol. 553, 497–509. doi: 10.1113/jphysiol.2003.052639
Unno, K., Takabayashi, F., Yoshida, H., Choba, D., Fukutomi, R., Kikunaga, N., et al. (2007). Daily consumption of green tea catechin delays memory regression in aged mice. Biogerontology 8, 89–95. doi: 10.1007/s10522-006-9036-8
Uren, R. T., and Turnley, A. M. (2014). Regulation of neurotrophin receptor (Trk) signaling: suppressor of cytokine signaling 2 (SOCS2) is a new player. Front. Mol. Neurosci. 7:39. doi: 10.3389/fnmol.2014.00039
Vazhacharickal, P. J. (2016). Nesting Behavior and Habitats of the Stingless Bee Trigona iridipennis Smith in Kerala: The Current Status of Knowledge. Grin Verlag Publisher. Available at: https://www.researchgate.net/publication/311970073
Ventimiglia, R., Mather, P. E., Jones, B. E., and Lindsay, R. M. (1995). The NeurotroDhins BDNF, NT-3 and NT-4/5 promote survival and morphological and biochemical differentiation of striatal neurons in vitro. Eur. J. Neurosci. 7, 213–222. doi: 10.1111/j.1460-9568.1995.tb01057.x
Vezzani, A., Aronica, E., Mazarati, A., and Pittman, Q. J. (2013). Epilepsy and brain inflammation. Exp. Neurol. 244, 11–21. doi: 10.1016/j.expneurol.2011.09.033
Vezzani, A., Balosso, S., and Ravizza, T. (2012). Inflammation and epilepsy. Handb. Clin. Neurol. 107, 163–175. doi: 10.1016/B978-0-444-52898-8.00010-0
Vezzani, A., French, J., Bartfai, T., and Baram, T. Z. (2011). The role of inflammation in epilepsy. Nat. Rev. Neurol. 7, 31–40. doi: 10.1038/nrneurol.2010.178
Vezzani, A., Sperk, G., and Colmers, W. F. (1999). Neuropeptide Y: emerging evidence for a functional role in seizure modulation. Trends Neurosci. 22, 25–30. doi: 10.1016/S0166-2236(98)01284-3
Vijayakumar, K., and Jeyaraaj, R. (2014). Taxonomic notes on stingless bee Trigona (Tetragonula) iridipennis Smith (Hymenoptera: Apidae) from India. J. Threatened Taxa 6, 6480–6484. doi: 10.11609/JoTT.o3773.6480-4
Vit, P., Medina, M., and Enríquez, E. (2004). Quality standards for medicinal uses of Meliponinae honey in Guatemala, Mexico and Venezuela. Bee World 85, 2–5. doi: 10.1080/0005772X.2004.11099603
Walz, C., Jüngling, K., Lessmann, V., and Gottmann, K. (2006). Presynaptic plasticity in an immature neocortical network requires NMDA receptor activation and BDNF release. J. Neurophysiol. 96, 3512–3516. doi: 10.1152/jn.00018.2006
Wang, P., Jeng, C. J., Chien, C. L., and Wang, S. M. (2008). Signaling mechanisms of daidzein-induced axonal outgrowth in hippocampal neurons. Biochem. Biophys. Res. Commun. 366, 393–400. doi: 10.1016/j.bbrc.2007.11.147
Wang, J., and Li, Q. X. (2011). Chemical composition, characterization, and differentiation of honey botanical and geographical origins. Adv. Food Nutr. Res. 62, 89–137. doi: 10.1016/B978-0-12-385989-1.00003-X
Wang, X., and Michaelis, E. K. (2010). Selective neuronal vulnerability to oxidative stress in the brain. Front. Aging Neurosci. 2:12. doi: 10.3389/fnagi.2010.00012
Waters, J., Schaefer, A., and Sakmann, B. (2005). Backpropagating action potentials in neurones: measurement, mechanisms and potential functions. Prog. Biophys. Mol. Biol. 87, 145–170. doi: 10.1016/j.pbiomolbio.2004.06.009
Weissberg, I., Reichert, A. S., Heinemann, U., and Friedman, A. (2011). Blood-brain barrier dysfunction in epileptogenesis of the temporal lobe. Epilepsy Res. Treat. 2011:143908. doi: 10.1155/2011/143908
Whitlock, J. R., Heynen, A. J., Shuler, M. G., and Bear, M. F. (2006). Learning induces long-term potentiation in the hippocampus. Science 313, 1093–1097. doi: 10.1126/science.1128134
Williams, C. M., El Mohsen, M. A., Vauzour, D., Rendeiro, C., Butler, L. T., Ellis, J. A., et al. (2008). Blueberry-induced changes in spatial working memory correlate with changes in hippocampal CREB phosphorylation and brain-derived neurotrophic factor (BDNF) levels. Free Radic. Biol. Med. 45, 295–305. doi: 10.1016/j.freeradbiomed.2008.04.008
Wilson Horch, H., Krüttgen, A., Portbury, S. D., and Katz, L. C. (1999). Destabilization of cortical dendrites and spines by BDNF. Neuron 23, 353–364. doi: 10.1016/S0896-6273(00)80785-0
Wong, P., Hii, S., Koh, C., Moh, T., and Gindi, S. R. A. (2019). Chemical analysis on the honey of Heterotrigona itama and Tetrigona binghami from Sarawak, Malaysia. Sains Malaysiana 48, 1635–1642. doi: 10.17576/jsm-2019-4808-09
Wu, L.-H., Lin, C., Lin, H. Y., Liu, Y. S., Wu, C. Y., Tsai, C. F., et al. (2016). Naringenin suppresses neuroinflammatory responses through inducing suppressor of cytokine signaling 3 expression. Mol. Neurobiol. 53, 1080–1091. doi: 10.1007/s12035-014-9042-9
Wurzelmann, M., Romeika, J., and Sun, D. (2017). Therapeutic potential of brain-derived neurotrophic factor (BDNF) and a small molecular mimics of BDNF for traumatic brain injury. Neural Regen. Res. 12, 7–12. doi: 10.4103/1673-5374.198964
Xiao, N., and Le, Q.-T. (2016). Neurotrophic factors and their potential applications in tissue regeneration. Arch. Immunol. Ther. Exp. 64, 89–99. doi: 10.1007/s00005-015-0376-4
Xu, S. L., Choi, R. C., Zhu, K. Y., Leung, K. W., Guo, A. J., Bi, D., et al. (2012). Isorhamnetin, a flavonol aglycone from Ginkgo biloba L., induces neuronal differentiation of cultured PC12 cells: potentiating the effect of nerve growth factor. Evid. Based Complement. Alternat. Med. 2012:278273. doi: 10.1155/2012/278273
Yaacob, W. M. H. W., Long, I., Zakaria, R., and Othman, Z. (2020). Tualang honey and its Methanolic fraction improve LPS-induced learning and memory impairment in male rats: comparison with Memantine. Curr. Nutr. Food Sci. 16, 333–342. doi: 10.2174/1573401315666181130103456
Yaacob, M., Rajab, N. F., Shahar, S., and Sharif, R. (2018). Stingless bee honey and its potential value: a systematic review. Food Res. 2, 124–133. doi: 10.26656/fr.2017.2(2).212
Yabe, T., Hirahara, H., Harada, N., Ito, N., Nagai, T., Sanagi, T., et al. (2010). Ferulic acid induces neural progenitor cell proliferation in vitro and in vivo. Neuroscience 165, 515–524. doi: 10.1016/j.neuroscience.2009.10.023
Yadav, R. K., Mehan, S., Sahu, R., Kumar, S., Khan, A., Makeen, H. A., et al. (2022). Protective effects of apigenin on methylmercury-induced behavioral/neurochemical abnormalities and neurotoxicity in rats. Hum. Exp. Toxicol. 41:9603271221084276. doi: 10.1177/09603271221084276
Yehezkel, B.-A. (2008). Epilepsies and neuronal plasticity: for better or for worse? Dialogues Clin. Neurosci. 10, 17–27. doi: 10.31887/DCNS.2008.10.1/ybenari
Yin, L., Zhang, Y., Wang, L., Wu, H., Azi, F., Tekliye, M., et al. (2022). Neuroprotective potency of a soy whey fermented by Cordyceps militaris SN-18 against hydrogen peroxide-induced oxidative injury in PC12 cells. Eur. J. Nutr. 61, 779–792. doi: 10.1007/s00394-021-02679-w
Yoshii, A., and Constantine-Paton, M. (2007). BDNF induces transport of PSD-95 to dendrites through PI3K-AKT signaling after NMDA receptor activation. Nat. Neurosci. 10, 702–711. doi: 10.1038/nn1903
Yoshii, A., and Constantine-Paton, M. (2010). Postsynaptic BDNF-TrkB signaling in synapse maturation, plasticity, and disease. Dev. Neurobiol. 70, 304–322. doi: 10.1002/dneu.20765
Yoshii, A., and Constantine-Paton, M. (2014). Postsynaptic localization of PSD-95 is regulated by all three pathways downstream of TrkB signaling. Front. Synap. Neurosci. 6:6. doi: 10.3389/fnsyn.2014.00006
Yury, K., Hanse, E., Kafitz, K. W., and Konnerth, A. (2002). Postsynaptic induction of BDNF-mediated long-term potentiation. Science 295, 1729–1734. doi: 10.1126/science.1067766
Zaidi, H., Ouchemoukh, S., Amessis-Ouchemoukh, N., Debbache, N., Pacheco, R., Serralheiro, M. L., et al. (2019). Biological properties of phenolic compound extracts in selected Algerian honeys—the inhibition of acetylcholinesterase and α-glucosidase activities. Eur. J. Integr. Med. 25, 77–84. doi: 10.1016/j.eujim.2018.11.008
Zainin, M. L., and Hassan, N. A. (2021). Heterotrigona Itama (Kelulut) honey and its potential value: a review. Asean J. Life Sci. 1, 42–45. Available at: https://ajls.journals.unisel.edu.my/index.php/ajls/article/view/24
Zhang, X., Bu, X., Jiang, Y., Sun, G., Jiang, R., Huang, X., et al. (2019). The antidepressant effects of apigenin are associated with the promotion of autophagy via the mTOR/AMPK/ULK1 pathway. Mol. Med. Rep. 20, 2867–2874. doi: 10.3892/mmr.2019.10491
Zhang, S.-H., Liu, D., Hu, Q., Zhu, J., Wang, S., Zhou, S., et al. (2019). Ferulic acid ameliorates pentylenetetrazol-induced seizures by reducing neuron cell death. Epilepsy Res. 156:106183. doi: 10.1016/j.eplepsyres.2019.106183
Zhang, F., Wang, Y. Y., Liu, H., Lu, Y. F., Wu, Q., Liu, J., et al. (2012, 2012). Resveratrol produces neurotrophic effects on cultured dopaminergic neurons through prompting astroglial BDNF and GDNF release. Evid. Based Complement. Alternat. Med. doi: 10.1155/2012/937605
Zhang, Y., Zhao, J., Afzal, O., Kazmi, I., Al-Abbasi, F. A., and Altamimi, A. S. A. (2021). Neuroprotective role of chrysin-loaded poly(lactic-co-glycolic acid) nanoparticle against kindling-induced epilepsy through Nrf2/ARE/HO-1 pathway. J. Biochem. Mol. Toxicol. 35:e22634. doi: 10.1002/jbt.22634
Zhou, Q., Homma, K. J., and Poo, M. (2004). Shrinkage of dendritic spines associated with long-term depression of hippocampal synapses. Neuron 44, 749–757. doi: 10.1016/j.neuron.2004.11.011
Zhou, Y., Wu, H., Li, S., Chen, Q., Cheng, X. W., Zheng, J., et al. (2006). Requirement of TORC1 for late-phase long-term potentiation in the hippocampus. PLoS One 1:e16. doi: 10.1371/journal.pone.0000016
Keywords: stingless bee honey, brain-derived neurotrophic, epileptogenesis, antioxidant, anti-inflammatory, tyrosine receptor kinase B, neuroprotective
Citation: Zulkifli NA, Hassan Z, Mustafa MZ, Azman WNW, Hadie SNH, Ghani N and Mat Zin AA (2023) The potential neuroprotective effects of stingless bee honey. Front. Aging Neurosci. 14:1048028. doi: 10.3389/fnagi.2022.1048028
Edited by:
Raymond Thomas, Memorial University of Newfoundland, CanadaReviewed by:
Brahm Kumar Tiwari, SGT University, IndiaSeong Lin Teoh, National University of Malaysia, Malaysia
Copyright © 2023 Zulkifli, Hassan, Mustafa, Azman, Hadie, Ghani and Mat Zin. This is an open-access article distributed under the terms of the Creative Commons Attribution License (CC BY). The use, distribution or reproduction in other forums is permitted, provided the original author(s) and the copyright owner(s) are credited and that the original publication in this journal is cited, in accordance with accepted academic practice. No use, distribution or reproduction is permitted which does not comply with these terms.
*Correspondence: Anani Aila Mat Zin, ✉ YWlsYWtiQHVzbS5teQ==