- 1State Key Laboratory of Primate Biomedical Research, Institute of Primate Translational Medicine, Kunming University of Science and Technology, Kunming, China
- 2Yunnan Key Laboratory of Primate Biomedical Research, Kunming, Yunnan, China
Parkinson’s disease (PD) is the second most common neurodegenerative disease. Studies have shown that abnormal accumulation of α-synuclein (α-Syn) in the substantia nigra is a specific pathological characteristic of PD. Abnormal accumulation of α-Syn in PD induces the activation of microglia. Microglia, which are immune cells in the central nervous system, are involved in the function and regulation of inflammation in PD by autophagy. The role of microglial autophagy in the pathophysiology of PD has become a hot-pot issue. This review outlines the pathways of microglial autophagy, and explores the key factor of microglial autophagy in the mechanism of PD and the possibility of microglial autophagy as a potential therapeutic target for PD.
Introduction
Parkinson’s disease (PD) is the second most prevalent neurodegenerative disease and was first reported by James Parkinson in 1817. PD is characterized principally by resting tremor, motor bradykinesia, rigidity, and postural instability, as well as a variety of additional motor and nonmotor symptoms (Jankovic, 2008; Armstrong and Okun, 2020). In 2016, an estimated 6.1 million people were diagnosed with PD worldwide, which is 2.4 times higher than in 1990 (GBD 2016 Parkinson's Disease Collaborators, 2018), as the world population ages and lives longer. Currently, the scientific community is becoming increasingly interested in PD.
In a healthy organism, the homeostasis of the central nervous system (CNS) is dependent on the interactions of various nerve cells. However, in the CNS of PD patients, there is an aberrant build-up of α-synuclein (α-Syn) and a cascade effect of gradual neuronal damage that breaks the appropriate balance, which leads to inflammation in the CNS (Hirsch, 1992). Autophagy is an evolutionarily conserved degradation pathway that is responsible for the digestion and recycling of the majority of intracytoplasmic proteins and organelles. Autophagy maintains homeostasis by delivering cytoplasmic materials to the lysosome for degradation (Bonam et al., 2021). Due to poor autophagy, inappropriately aggregated α-Syn in the CNS of PD patients cannot be removed and accumulated (Aflaki et al., 2017; Karabiyik et al., 2017). Overall, dysregulation of autophagy is thought to play an important role in the abnormal aggregation of α-Syn and the exacerbation of Parkinson’s disease.
Microglia were discovered more than a century ago by using silver carbonate staining (Andres-Barquin, 2002). Microglia are CNS-specific immune cells that play an immunological role in the CNS comparable to that of macrophages, interact with neurons, and conduct a variety of tasks in the CNS (Leng and Edison, 2021). Recent research shows that microglial autophagy is involved in the function and regulation of inflammation in the CNS (Su et al., 2016; Bussi et al., 2017). These findings implied that dysregulation of autophagy in microglia may impact innate immune activities, including phagocytosis and inflammation, which, in turn, contribute to illnesses associated with neuroinflammation (Derecki et al., 2014; Michell-Robinson et al., 2015). To date, many researchers have considered PD to be a neuroinflammatory disease, and the role of microglial autophagy in the pathophysiology of PD has been a hot issue in the field (Cheng et al., 2020; Choi et al., 2020). In this review, we present and highlight the contribution of microglial autophagy to the pathological mechanism of PD and aimed to determine whether microglial autophagy could be a potential target for therapeutic intervention.
Autophagy in microglia
Current research suggests that microglia play a crucial role in neurodegenerative disorders. Microglia, like macrophages, can execute their functions under both normal and pathological conditions, and they can also exert adaptive responses under disease conditions (Liu et al., 2022). Microglia can maintain the normal function of nerve cells through synaptic pruning (Paolicelli et al., 2011), and aberrations in the function of microglia during the phagocytosis of synapses may contribute to synaptic loss and neurodegeneration. In addition, microglia play an important role in clearing away apoptotic or necrotic cells and destroying aberrant protein clumps, such as β-amyloid (Aβ) and aggregated α-Syn (Spangenberg et al., 2016; Yuan et al., 2022). In general, microglia use phagocytosis to participate in autophagy in the CNS, which eliminates the effects of the inflammatory response in the organism and maintains homeostasis (Figure 1). Microglial autophagy is the function of carrying protein aggregates and damaged organelles into lysosomes, which includes autophagosome formation and degradation. Autophagosome biogenesis is initiated upon either autophagy-inducing signals or autophagy receptors binding to their targets. Membrane nucleation occurs at specific locations in phagocytes, which then expand and close to autophagosomes (Hu and Reggiori, 2022). The biogenesis of autophagosomes depends on the function of autophagy-related (ATG) proteins (Nakatogawa, 2020). The autophagosome transfers the encapsulated material into the lysosome through fusion of its outer membrane with the lysosomal limiting membrane, forming an autolysosome. Autolysosomes will degrade autophagic cargo as well as the inner autophagosome membrane (Lőrincz and Juhász, 2020). Autophagic flux is primarily related to the intensity of autophagy being induced and the rate of autophagosome fusion digestion by lysosomes, and it is now believed that dysregulated autophagic flux appears to be a major factor in the neuropathology of PD. It was found that in dopaminergic neurons with α-Syn misfolded, lysosomes appear dysfunctional and the rate of autophagosome clearance is decreased (Chu et al., 2009; Dehay et al., 2010). Activation of transcription factor EB (TFEB) drives lysosomal function to clear α-Syn oligomers thereby exerting neuroprotective effects (Settembre et al., 2011; Decressac et al., 2013).
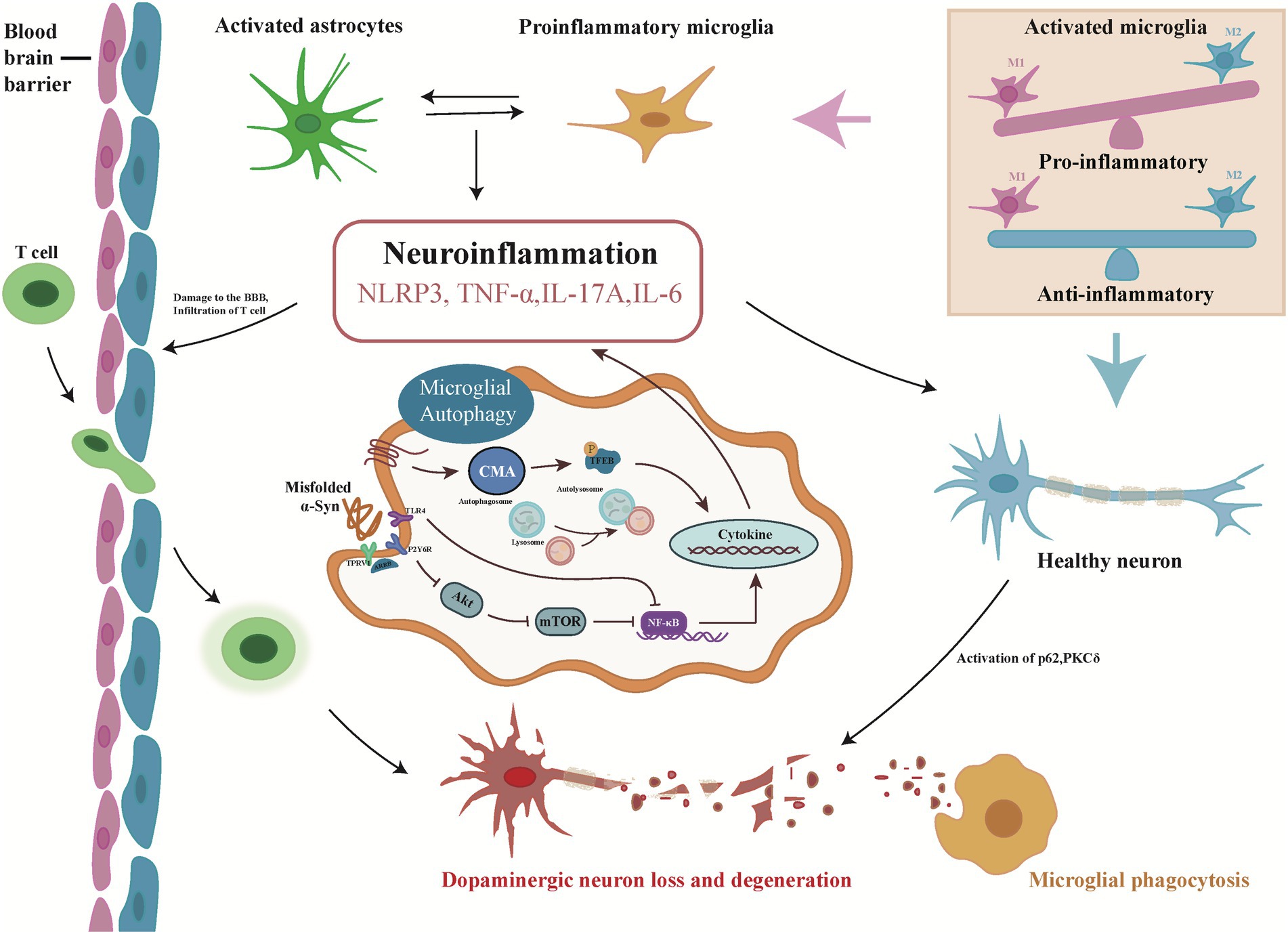
Figure 1. Overview of the microglial autophagy process in Parkinson’s disease. Misfolded α-Syn triggers autophagy in microglia, induces neuroinflammation and releases pro-inflammatory cytokines through canonical (Akt/mTOR), noncanonical (TLR4), and chaperone-mediated (CMA) autophagy pathways. Inflammatory factors accelerate the damage and loss of dopaminergic neurons by disrupting the blood–brain barrier and activating PKCδ, p62, etc., triggering the next autophagy. In the process, pro-inflammatory cytokines, in turn, activate resting microglia, which further activate astrocytes and accelerate neuroinflammation.
Microglial phagocytosis and autophagy
Microglia, which are resident brain macrophages, are commonly believed to orchestrate the brain inflammatory response during infections and disease (Sierra et al., 2013). The most significant functional similarity between microglia and macrophages is their ability to perform phagocytosis, which involves three primary steps: recognition, endocytosis, and digestion (Wolf et al., 2017). Phagocytosis is initiated by the activation of membrane receptors, which directly recognize the target to be phagocytosed. In neurological diseases, it has been shown that autophagy can be affected by interfering with the phagocytic function of microglia. Studies have shown that autophagy can regulate phagocytosis through various steps in the phagocytic cascade (Li G. et al., 2021). Restored microglial phagocytosis after peripheral interferon-beta (IFN-β) injection can consequently increase the initiation of brain autophagy (He et al., 2020). Some neurological medications, such as the antidepressant fluoxetine, can increase autophagy further by boosting microglia phagocytosis, thereby targeting neuroinflammation and exerting neuroprotective effects (Park et al., 2021). Berglund discovered that autophagy-related phagocytosis of microglia was critical for recovery from neuroinflammation. Microglia dominate the destruction and removal of myelin sheaths through atypical forms of autophagy in this way and are involved in recovery from demyelinating diseases (Berglund et al., 2020).
Mechanism of microglial autophagy
Microglia are CNS-specific phagocytes that can perform phagocytosis by rapidly extending and retracting protrusions to recognize aberrant cells or chemicals in the environment (Davalos et al., 2005). Microglia perform autophagy in both canonical and noncanonical manners (Jülg et al., 2020).
Canonical autophagy is the sequential assembly of the autophagic machinery via recognition-phagocytosis-digestion. Microglia phagocytose redundant cellular components and deliver them to lysosomes to complete autophagy (Hickey and Kimura, 1988; Lee et al., 2019), and the Unc-51-like autophagy activating kinase complex, class III phosphatidylinositol 3-kinase (PI3KC3) complex, and ATG9/PI3P effector protein recruitment are involved. Song used lipopolysaccharide (LPS) to induce the overexpression of miR-Let7A in microglia and discovered that miR-Let7A may have a role in microglial autophagy during CNS inflammation (Song et al., 2015). Fabbrizio discovered that P2X7 increased the expression of the autophagy marker LC3II via the mTOR pathway in Amyotrophic lateral sclerosis rats, thereby modulating autophagy in microglia (Fabbrizio et al., 2017). Wang increased Ccl5 and Cxcl10 expression in a non-p53-dependent manner, indicating that microglial autophagy plays an important role in controlling neurogenesis and restricting local immune responses in postnatal neural stem cells in a noncell-autonomous manner (Wang et al., 2017). Jin discovered that tumor necrosis factor-α (TNF-α) inhibited microglial autophagy via the AKT/mTOR signaling pathway; however, enhanced autophagy could promote microglial polarization to the M2 phenotype and promote the resolution of inflammation in PD (Jin et al., 2018). Li showed that a miR-223 inhibitor induced autophagosome and autolysosome production in microglial BV2 cells via the ATG16L1-LC3 pathway in response to LPS stimulation (Li et al., 2019). Sakai found that defective autophagy in microglia of the prefrontal cortex exacerbated repeated social defeat (RSD)-induced social avoidance (Sakai et al., 2022).
Unlike canonical autophagy, noncanonical pathways do not require the hierarchical contribution of all ATG proteins. A number of noncanonical autophagic pathways have been identified in microglia (Lee et al., 2019). For instance, Choi discovered a neuroprotective function of microglia in the clearance of α-Syn via Toll-like receptors 4 (TLR4)-nuclear factor kappa-B (NF-κB)-p62-mediated synucleinphagy (Choi et al., 2020). Wang identified a role for microglial NF-κB signaling in regulating tau protein diffusion and tau lesion toxicity (Wang et al., 2022). The above studies show that in microglia, both canonical and noncanonical autophagy pathways can be regulated by different methods.
Microglial autophagy and neuroinflammation development
Phagocytic microglia promote autophagy in the CNS mostly due to neuroinflammation. Studies have shown that microglia use protrusions to communicate with neurons and other glial cells during movement according to a variety of internal environments (Davalos et al., 2005; Ladeby et al., 2005; Fourgeaud et al., 2016). Under physiological conditions, this motor communication ability of microglia enables them to act as scavengers, maintain the balance of the internal environment, remove metabolites, and regulate neurogenesis (Colonna and Butovsky, 2017; Stratoulias et al., 2019). Under endogenous or exogenous pathological conditions, microglia, similar to macrophages, will change from a quiescent state to an “activated” state, undergo morphological changes to exert phagocytic function, induce an inflammatory response, and release a variety of pro-inflammatory cytokines. Therefore, activated microglia determine the fate of other surrounding nerve cells to a certain extent, maintain the healthy state of the tissue environment and overcome the development of pathologies such as neuroinflammation (Miyamoto et al., 2016; Hagemeyer et al., 2017; Kam et al., 2020; Tu et al., 2021). Activated microglia can often be categorized as M1 or M2 cells. The activation of M1 microglia is called classical activation. M1 microglia are cytotoxic to neurons and other glial cells by producing a large number of cellular mediators, such as proteases, pro-inflammatory cytokines, and reactive oxygen species (ROS), which lead to pro-inflammatory responses (Daher et al., 2014). Pro-inflammatory cytokines could activate M1 microglia to express pro-inflammatory factors, such as IL-1β, TNF-α, IL-6, nitric oxide (NO) and protease have adverse effects on neurodegenerative diseases (Glass et al., 2010; Tang and Le, 2016). It is now believed that the degree of M1 microglia activation correlates with the loss of dopaminergic neurons in early PD (Ouchi et al., 2005). TNF-α, NO and IL-1β originating from M1 microglia can regulate the neuroinflammatory process in PD (Rojanathammanee et al., 2011). M2 microglia are considered to be another activated state that are capable of participating in the phagocytosis of cellular debris or damaged neurons and release various neurotrophic factors and cytokines, thereby exerting anti-inflammatory effects (Cherry et al., 2014). IL-4, IL-10, IL-13, and transforming growth factor-β could activate M2 microglia to release various cytokines, such as insulin-like growth factor 1 and frizzled receptor 1 (Glass et al., 2010; Tang and Le, 2016), and these cytokines may be involved in neuroprotection and tissue healing. M1 and M2 microglia can transform into each other under certain circumstances, inflammatory mediators released by M1 microglia are involved in mechanisms of neurodegenerative diseases, and M2 microglia are involved in tissue maintenance and repair. Microglia-mediated neuroinflammation is therefore regarded as a double-edged sword in neurodegenerative diseases, with deleterious and beneficial effects on neurons and the surrounding environment (Guo et al., 2022). Aggregated α-Syn can directly activate microglia toward the M1 phenotype (Tang and Le, 2016). Under hypoxic and inflammatory conditions, inhibition of the mTOR pathway was found to prevent microglial activation, reduce neuroinflammation, and protect neurons (Srivastava et al., 2016).
Microglial autophagy in PD patients
The cellular and molecular alterations observed in the brains of PD patients have been further studied in PD animal models. Here, we describe microglial changes in PD patients, the mechanism of microglial autophagy associated with α-Syn disaggregation dopaminergic neuron degeneration in animal models of PD, and microglial autophagy as a potential therapeutic target that may help slow the progression of PD.
In 1988, McGeer detected a large number of HLA-DR-positive reactive microglia (macrophages) in the substantia nigra of PD patients, along with LBs (McGeer et al., 1988). Later, Imamura confirmed that M2 microglia were associated with the CNS under aging and pathological conditions; that is, M2 microglia are sensitive indicators of neuropathological changes and are closely associated with damaged neurons and neurites (Imamura et al., 2003; Table 1). To further understand the differences in the transcriptional levels of microglia in PD, Mastroeni used single-cell laser technology to examine microglia in the brains of PD patients and found that microglia in different brain regions have different transcriptional profiles and functionally regulated genes (Mastroeni et al., 2018). However, in general, the RNA-seq data obtained from the brain tissue of PD patients are relatively small, and it is not possible to draw clear conclusions about the correlation between microglial transcriptional heterogeneity and disease. However, these data strongly demonstrate that microglia play an important role in PD.
Mechanism of microglial autophagy in PD animal models
α-Syn aggregates in substantia nigra dopamine (DA) neurons are important in the pathogenesis of PD (Ghosh et al., 2017). Recent studies have shown that activated microglia accelerate pathological α-Syn accumulation in DA neurons (George et al., 2019; Tan et al., 2020). Microglia exposed to abnormal aggregation of α-Syn can form F-actin-dependent intercellular junctions, thereby establishing cellular networks that can transfer abnormally aggregated α-Syn from overloaded microglia to adjacent inactivated microglia for their rapid and efficient degradation. This degradation process could reduce the inflammatory response of microglia and increase their survival (Scheiblich et al., 2021). The mechanism by which microglia improve the clearance of pathogenic α-Syn aggregates by creating an “on-demand” functional network has been elucidated (Table 1). A study showed that microglia can transmit α-Syn through exosomes and aggravate the accumulation of α-Syn, indicating that the secretion of exosomes can alter the progression of PD (Xia et al., 2019). Guo found that α-Syn oligomers were present in microglia-derived exosomes in the cerebrospinal fluid of patients with Parkinson’s disease, which could induce α-Syn aggregation in neurons, indicating that microglia can accelerate α-Syn aggregation in Parkinson’s disease via exosomes (Guo et al., 2020). Misfolded α-Syn in PD can alter TLRs expression in microglia and modulate persistently activated protein kinase Cδ (PKCδ) dependent nuclear displacement of NF-κB (Li Y. et al., 2021). Choi identified that microglia mediate synaptic phagocytosis through the TLR4-NF-κB-p62 pathway, thereby clearing α-Syn for neuroprotective effects (Choi et al., 2020; Figure 1).
Neuroinflammation and oxidative stress play important roles in the pathogenesis of PD. During these processes, microglia are activated and involved in the progressive degeneration and loss of nigrostriatal DA neurons (Table 1). PKCδ drives a persistent inflammatory response in microglia, leading to dopaminergic neuronal neurotoxicity, which, in turn, causes neurobehavioral deficits (Gordon et al., 2016). Mutations in the oxidative stress sensor DJ-1 cause family PD. Knockdown of DJ-1 in microglia increased neurotoxicity in DA neurons and significantly increased α-Syn-induced secretion of pro-inflammatory cytokines (Nash et al., 2017). The study showed that in the microglia of PD mice, the expression level of PGC-1α (peroxisome proliferator-activated receptor-γ coactivator-1α), which is closely related to the inflammatory response, was increased, and PGC-1α/Iba1 colocalization was increased. The downregulation of PGC-1α expression can inhibit the activity of microglia and reduce the activation of microglia, thereby protecting DA neurons (Guan et al., 2021). Further study showed that when the blood–brain barrier was disrupted, effector T cells transferred into the brain and aggravated the degeneration of nigrostriatal DA neurons, the activation of microglia and motor impairment (Liu et al., 2017), and inhibiting IL-17A secretion by Th17 cells in the substantia nigra ameliorated the above symptoms. IL-17A exacerbates DA neuron loss only in the presence of microglia, suggesting a crucial role for microglia in DA neuron degeneration (Liu et al., 2019). Subbarayan examined T-cell-deficient and T-cell-normal rats and showed that T cells may be directly involved in α-Syn-mediated loss of DA neurons in PD and activation of microglia (Subbarayan et al., 2020). The expression of β-repressor protein 1 (ARRB1) and β-repressor protein 2 (ARRB2) in microglia is reciprocally regulated, and Fang found that ARRB1 ablation ameliorated the pathological features of PD, whereas ARRB2 knockout aggravated the pathological features of PD (Fang et al., 2021). Nucleotide-binding oligomerization domain-, leucine-rich repeat-, and pyrin domain-containing 3 (NLRP3), the most well-studied inflammasome, is abundantly expressed in the activated microglia and has gained considerable attention (Wu A. G. et al., 2021). The excessive activation of NLRP3 inflammasome could impair microglial autophagy and further aggravates the pathogenesis of neurodegenerative diseases. α-Syn aggregates activate NLRP3 inflammatory bodies in microglia through interaction with TLRs and activation of NF-κB (Béraud et al., 2011; Panicker et al., 2019). Chen investigated whether the p38-TFEB pathway promoted microglial activation by inhibiting CMA-mediated NLRP3 degradation in Parkinson’s disease, which could be a potential therapeutic strategy for PD (Chen et al., 2021; Figure 1).
Autophagy of microglia and PD therapeutic advances and future directions
Numerous studies have been conducted on the role of microglia-induced neuroinflammation and microglial autophagy in the pathogenesis of PD to identify new strategies for the treatment of PD through pathways related to microglial activation and neuroinflammation. It was found that the stimulation of P2Y6R accelerated the activation of microglial cells and the release of pro-inflammatory cytokines; that is, blocking P2Y6R may inhibit microglial activation and phagocytosis (Yang et al., 2017). Activation of NLRP3-mediated inflammatory responses impairs microglial autophagy and exacerbates 1-methyl-4-phenyl-1,2,3,6-tetrahydropyridine (MPTP)-induced pro-inflammatory responses to PD-like symptoms (Cheng et al., 2020; Qin et al., 2021). Ahmed uses andrographolide to enhance microglial activation by attenuating NLRP3 inflammasome activation in microglia, enabling the rescue of dopaminergic neurons and ameliorating behavioral deficits in animals (Ahmed et al., 2021). Yuan used photothermal nanomaterials to control the opening of transient receptor potential cation channel subfamily V member 1 (TRPV1) channels on the surface of microglia to enhance phagocytosis by microglia and accelerate the phagocytosis and degradation of α-Syn, thereby ameliorating PD (Yuan et al., 2022; Figure 1).
Based on related research, the efficacy of some drugs was further explored. NLY01 is a glucagon-like peptide-1 receptor (GLP-1R) inhibitor that can exert neuroprotective effects by directly regulating the activated microglia-mediated transformation of astrocytes to the A1 neurotoxic phenotype in PD (Yun et al., 2018). Nilotinib was found to inhibit microglial activation and the production of pro-inflammatory cytokines and mediators by inhibiting the NF-κB signaling pathway. The anti-inflammatory properties of nilotinib may further reduce inflammatory neurotoxicity to protect against DA neuron loss, indicating its potential use in PD (Wu et al., 2021b). Cannabidiol (CBD) can inhibit IL-6 release and improve microglial activation, and there is a correlation between CBD-induced signal transducer and activator of transcription 3 (STAT3) and NF-κB phosphorylation, indicating that CBD may have therapeutic potential for treating neurological diseases involving neuroinflammation (Wu et al., 2021a; Table 1).
Conclusion
In this review, we described the role of microglial autophagy in PD pathogenesis and explored potential therapeutic strategies for PD. Microglia participate in the process of autophagy through phagocytosis and regulate the occurrence and development of neuroinflammation (Tang and Le, 2016; Table 1). In PD, α-Syn and neuroinflammation trigger signaling pathways such as NLRP3, which activate microglia (Chen et al., 2021). Activated microglia alter the aggregation and propagation of misfolded α-Syn through autophagy to further influence neurons and neurobehavior. In this process, only properly activated microglia contribute to the removal of harmful substances, and excessive autophagy and/or dysregulated autophagic flux can lead to neurotoxicity (Qin et al., 2021). It is well known that autophagy in microglia exerts neuroprotective effect by regulating phagocytosis and inflammation (Tang and Le, 2016; He et al., 2020); however, the mechanism of autophagy in microglia is complex. Therefore, further examination of the mechanism of microglial autophagy in PD can help us identify better strategies regarding microglial autophagy in the field of PD therapy.
Author contributions
All authors listed have made a substantial, direct, and intellectual contribution to the work and approved it for publication.
Funding
The authors are grateful to be supported by the National Natural Science Foundation of China (31960120) and the Applied Basic Research Programs of Science and Technology Commission Foundation of Yunnan Province (202105AC160041 and 2018FB052).
Conflict of interest
The authors declare that the research was conducted in the absence of any commercial or financial relationships that could be construed as a potential conflict of interest.
Publisher’s note
All claims expressed in this article are solely those of the authors and do not necessarily represent those of their affiliated organizations, or those of the publisher, the editors and the reviewers. Any product that may be evaluated in this article, or claim that may be made by its manufacturer, is not guaranteed or endorsed by the publisher.
References
Aflaki, E., Westbroek, W., and Sidransky, E. (2017). The complicated relationship between Gaucher disease and parkinsonism: insights from a rare disease. Neuron 93, 737–746. doi: 10.1016/j.neuron.2017.01.018
Ahmed, S., Kwatra, M., Ranjan Panda, S., Murty, U. S. N., and Naidu, V. G. M. (2021). Andrographolide suppresses NLRP3 inflammasome activation in microglia through induction of parkin-mediated mitophagy in in-vitro and in-vivo models of Parkinson disease. Brain Behav. Immun. 91, 142–158. doi: 10.1016/j.bbi.2020.09.017
Andres-Barquin, P. J. (2002). Santiago Ramón y Cajal and the Spanish school of neurology. Lancet Neurol. 1, 445–452. doi: 10.1016/S1474-4422(02)00192-8
Armstrong, M. J., and Okun, M. S. (2020). Diagnosis and treatment of Parkinson disease: a review. JAMA 323, 548–560. doi: 10.1001/jama.2019.22360
Béraud, D., Twomey, M., Bloom, B., Mittereder, A., Ton, V., Neitzke, K., et al. (2011). α-Synuclein alters toll-like receptor expression. Front. Neurosci. 5:80. doi: 10.3389/fnins.2011.00080
Berglund, R., Guerreiro-Cacais, A. O., Adzemovic, M. Z., Zeitelhofer, M., Lund, H., Ewing, E., et al. (2020). Microglial autophagy-associated phagocytosis is essential for recovery from neuroinflammation. Sci. Immunol. 5:eabb5077. doi: 10.1126/sciimmunol.abb5077
Bonam, S. R., Tranchant, C., and Muller, S. (2021). Autophagy-lysosomal pathway as potential therapeutic target in Parkinson's disease. Cells 10:3547. doi: 10.3390/cells10123547
Bussi, C., Peralta Ramos, J. M., Arroyo, D. S., Gaviglio, E. A., Gallea, J. I., Wang, J. M., et al. (2017). Autophagy down regulates pro-inflammatory mediators in BV2 microglial cells and rescues both LPS and alpha-synuclein induced neuronal cell death. Sci. Rep. 7:43153. doi: 10.1038/srep43153
Chen, J., Mao, K., Yu, H., Wen, Y., She, H., Zhang, H., et al. (2021). p38-TFEB pathways promote microglia activation through inhibiting CMA-mediated NLRP3 degradation in Parkinson's disease. J. Neuroinflammation 18:295. doi: 10.1186/s12974-021-02349-y
Cheng, J., Liao, Y., Dong, Y., Hu, H., Yang, N., Kong, X., et al. (2020). Microglial autophagy defect causes parkinson disease-like symptoms by accelerating inflammasome activation in mice. Autophagy 16, 2193–2205. doi: 10.1080/15548627.2020.1719723
Cherry, J. D., Olschowka, J. A., and O'Banion, M. K. (2014). Neuroinflammation and M2 microglia: the good, the bad, and the inflamed. J. Neuroinflammation 11:98. doi: 10.1186/1742-2094-11-98
Choi, I., Zhang, Y., Seegobin, S. P., Pruvost, M., Wang, Q., Purtell, K., et al. (2020). Microglia clear neuron-released α-synuclein via selective autophagy and prevent neurodegeneration. Nat. Commun. 11:1386. doi: 10.1038/s41467-020-15119-w
Chu, Y., Dodiya, H., Aebischer, P., Olanow, C. W., and Kordower, J. H. (2009). Alterations in lysosomal and proteasomal markers in Parkinson's disease: relationship to alpha-synuclein inclusions. Neurobiol. Dis. 35, 385–398. doi: 10.1016/j.nbd.2009.05.023
Colonna, M., and Butovsky, O. (2017). Microglia function in the central nervous system during health and neurodegeneration. Annu. Rev. Immunol. 35, 441–468. doi: 10.1146/annurev-immunol-051116-052358
Daher, J. P. L., Volpicelli-Daley, L. A., Blackburn, J. P., Moehle, M. S., and West, A. B. (2014). Abrogation of α-synuclein-mediated dopaminergic neurodegeneration in LRRK2-deficient rats. Proc. Natl. Acad. Sci. U. S. A. 111, 9289–9294. doi: 10.1073/pnas.1403215111
Davalos, D., Grutzendler, J., Yang, G., Kim, J. V., Zuo, Y., Jung, S., et al. (2005). ATP mediates rapid microglial response to local brain injury in vivo. Nat. Neurosci. 8, 752–758. doi: 10.1038/nn1472
Decressac, M., Mattsson, B., Weikop, P., Lundblad, M., Jakobsson, J., and Björklund, A. (2013). TFEB-mediated autophagy rescues midbrain dopamine neurons from α-synuclein toxicity. Proc. Natl. Acad. Sci. U. S. A. 110, E1817–E1826. doi: 10.1073/pnas.1305623110
Dehay, B., Bové, J., Rodríguez-Muela, N., Perier, C., Recasens, A., Boya, P., et al. (2010). Pathogenic lysosomal depletion in Parkinson's disease. J. Neurosci. 30, 12535–12544. doi: 10.1523/JNEUROSCI.1920-10.2010
Derecki, N. C., Katzmarski, N., Kipnis, J., and Meyer-Luehmann, M. (2014). Microglia as a critical player in both developmental and late-life CNS pathologies. Acta Neuropathol. 128, 333–345. doi: 10.1007/s00401-014-1321-z
Fabbrizio, P., Amadio, S., Apolloni, S., and Volonté, C. (2017). P2X7 receptor activation modulates autophagy in SOD1-G93A mouse microglia. Front. Cell. Neurosci. 11:249. doi: 10.3389/fncel.2017.00249
Fang, Y., Jiang, Q., Li, S., Zhu, H., Xu, R., Song, N., et al. (2021). Opposing functions of β-arrestin 1 and 2 in Parkinson's disease via microglia inflammation and Nprl3. Cell Death Differ. 28, 1822–1836. doi: 10.1038/s41418-020-00704-9
Fourgeaud, L., Través, P. G., Tufail, Y., Leal-Bailey, H., Lew, E. D., Burrola, P. G., et al. (2016). TAM receptors regulate multiple features of microglial physiology. Nature 532, 240–244. doi: 10.1038/nature17630
GBD 2016 Parkinson's Disease Collaborators (2018). Global, regional, and national burden of Parkinson's disease, 1990-2016: a systematic analysis for the global burden of disease study 2016. Lancet Neurol. 17, 939–953. doi: 10.1016/S1474-4422(18)30295-3
George, S., Rey, N. L., Tyson, T., Esquibel, C., Meyerdirk, L., Schulz, E., et al. (2019). Microglia affect α-synuclein cell-to-cell transfer in a mouse model of Parkinson's disease. Mol. Neurodegener. 14:34. doi: 10.1186/s13024-019-0335-3
Gerhard, A., Pavese, N., Hotton, G., Turkheimer, F., Es, M., Hammers, A., et al. (2006). In vivo imaging of microglial activation with [11C](R)-PK11195 PET in idiopathic Parkinson's disease. Neurobiol. Dis. 21, 404–412. doi: 10.1016/j.nbd.2005.08.002
Ghosh, D., Mehra, S., Sahay, S., Singh, P. K., and Maji, S. K. (2017). α-Synuclein aggregation and its modulation. Int. J. Biol. Macromol. 100, 37–54. doi: 10.1016/j.ijbiomac.2016.10.021
Ghosh, A., Roy, A., Liu, X., Kordower, J. H., Mufson, E. J., Hartley, D. M., et al. (2007). Selective inhibition of NF-kappaB activation prevents dopaminergic neuronal loss in a mouse model of Parkinson's disease. Proc. Natl. Acad. Sci. U. S. A. 104, 18754–18759. doi: 10.1073/pnas.0704908104
Glass, C. K., Saijo, K., Winner, B., Marchetto, M. C., and Gage, F. H. (2010). Mechanisms underlying inflammation in neurodegeneration. Cells 140, 918–934. doi: 10.1016/j.cell.2010.02.016
Gordon, R., Singh, N., Lawana, V., Ghosh, A., Harischandra, D. S., Jin, H., et al. (2016). Protein kinase Cδ upregulation in microglia drives neuroinflammatory responses and dopaminergic neurodegeneration in experimental models of Parkinson's disease. Neurobiol. Dis. 93, 96–114. doi: 10.1016/j.nbd.2016.04.008
Guan, X., Wu, P., Cao, B., Liu, X., Chen, X., Zhang, W., et al. (2021). PGC-1α-siRNA suppresses inflammation in substantia nigra of PD mice by inhibiting microglia. Int. J. Neurosci. 2021, 1–9. doi: 10.1080/00207454.2021.1910257
Guo, S., Wang, H., and Yin, Y. (2022). Microglia polarization from M1 to M2 in neurodegenerative diseases. Front. Aging Neurosci. 14:815347. doi: 10.3389/fnagi.2022.815347
Guo, M., Wang, J., Zhao, Y., Feng, Y., Han, S., Dong, Q., et al. (2020). Microglial exosomes facilitate α-synuclein transmission in Parkinson's disease. Brain 143, 1476–1497. doi: 10.1093/brain/awaa090
Hagemeyer, N., Hanft, K.-M., Akriditou, M.-A., Unger, N., Park, E. S., Stanley, E. R., et al. (2017). Microglia contribute to normal myelinogenesis and to oligodendrocyte progenitor maintenance during adulthood. Acta Neuropathol. 134, 441–458. doi: 10.1007/s00401-017-1747-1
He, Z., Yang, Y., Xing, Z., Zuo, Z., Wang, R., Gu, H., et al. (2020). Intraperitoneal injection of IFN-γ restores microglial autophagy, promotes amyloid-β clearance and improves cognition in APP/PS1 mice. Cell Death Dis. 11:440. doi: 10.1038/s41419-020-2644-4
Hickey, W. F., and Kimura, H. (1988). Perivascular microglial cells of the CNS are bone marrow-derived and present antigen in vivo. Science 239, 290–292. doi: 10.1126/science.3276004
Hirsch, E. C. (1992). Why are nigral catecholaminergic neurons more vulnerable than other cells in Parkinson's disease? Ann. Neurol. 32, S88–S93. doi: 10.1002/ana.410320715
Hu, Y., and Reggiori, F. (2022). Molecular regulation of autophagosome formation. Biochem. Soc. Trans. 50, 55–69. doi: 10.1042/BST20210819
Imamura, K., Hishikawa, N., Sawada, M., Nagatsu, T., Yoshida, M., and Hashizume, Y. (2003). Distribution of major histocompatibility complex class II-positive microglia and cytokine profile of Parkinson's disease brains. Acta Neuropathol. 106, 518–526. doi: 10.1007/s00401-003-0766-2
Jankovic, J. (2008). Parkinson's disease: clinical features and diagnosis. J. Neurol. Neurosurg. Psychiatry 79, 368–376. doi: 10.1136/jnnp.2007.131045
Jin, M.-M., Wang, F., Qi, D., Liu, W.-W., Gu, C., Mao, C.-J., et al. (2018). A critical role of autophagy in regulating microglia polarization in neurodegeneration. Front. Aging Neurosci. 10:378. doi: 10.3389/fnagi.2018.00378
Jülg, J., Strohm, L., and Behrends, C. (2020). Canonical and non-canonical autophagy pathways in microglia. Mol. Cell. Biol. 41:e00389-20. doi: 10.1128/MCB.00389-20
Kam, T.-I., Hinkle, J. T., Dawson, T. M., and Dawson, V. L. (2020). Microglia and astrocyte dysfunction in parkinson's disease. Neurobiol. Dis. 144:105028. doi: 10.1016/j.nbd.2020.105028
Karabiyik, C., Lee, M. J., and Rubinsztein, D. C. (2017). Autophagy impairment in Parkinson's disease. Essays Biochem. 61, 711–720. doi: 10.1042/EBC20170023
Ladeby, R., Wirenfeldt, M., Dalmau, I., Gregersen, R., García-Ovejero, D., Babcock, A., et al. (2005). Proliferating resident microglia express the stem cell antigen CD34 in response to acute neural injury. Glia 50, 121–131. doi: 10.1002/glia.20159
Lee, J.-W., Nam, H., Kim, L. E., Jeon, Y., Min, H., Ha, S., et al. (2019). TLR4 (toll-like receptor 4) activation suppresses autophagy through inhibition of FOXO3 and impairs phagocytic capacity of microglia. Autophagy 15, 753–770. doi: 10.1080/15548627.2018.1556946
Leng, F., and Edison, P. (2021). Neuroinflammation and microglial activation in Alzheimer disease: where do we go from here? Nat. Rev. Neurol. 17, 157–172. doi: 10.1038/s41582-020-00435-y
Li, G., Sherchan, P., Tang, Z., and Tang, J. (2021). Autophagy & Phagocytosis in neurological disorders and their possible cross-talk. Curr. Neuropharmacol. 19, 1912–1924. doi: 10.2174/1570159X19666210407150632
Li, Y., Xia, Y., Yin, S., Wan, F., Hu, J., Kou, L., et al. (2021). Targeting microglial α-Synuclein/TLRs/NF-kappaB/NLRP3 Inflammasome Axis in Parkinson's disease. Front. Immunol. 12:719807. doi: 10.3389/fimmu.2021.719807
Li, Y., Zhou, D., Ren, Y., Zhang, Z., Guo, X., Ma, M., et al. (2019). Mir223 restrains autophagy and promotes CNS inflammation by targeting ATG16L1. Autophagy 15, 478–492. doi: 10.1080/15548627.2018.1522467
Liu, Z., Huang, Y., Cao, B.-B., Qiu, Y.-H., and Peng, Y.-P. (2017). Th17 cells induce dopaminergic neuronal death via LFA-1/ICAM-1 interaction in a mouse model of Parkinson's disease. Mol. Neurobiol. 54, 7762–7776. doi: 10.1007/s12035-016-0249-9
Liu, S.-Y., Qiao, H.-W., Song, T.-B., Liu, X.-L., Yao, Y.-X., Zhao, C.-S., et al. (2022). Brain microglia activation and peripheral adaptive immunity in Parkinson's disease: a multimodal PET study. J. Neuroinflammation 19:209. doi: 10.1186/s12974-022-02574-z
Liu, Z., Qiu, A.-W., Huang, Y., Yang, Y., Chen, J.-N., Gu, T.-T., et al. (2019). IL-17A exacerbates neuroinflammation and neurodegeneration by activating microglia in rodent models of Parkinson's disease. Brain Behav. Immun. 81, 630–645. doi: 10.1016/j.bbi.2019.07.026
Lőrincz, P., and Juhász, G. (2020). Autophagosome-Lysosome Fusion. J. Mol. Biol. 432, 2462–2482. doi: 10.1016/j.jmb.2019.10.028
Mastroeni, D., Nolz, J., Sekar, S., Delvaux, E., Serrano, G., Cuyugan, L., et al. (2018). Laser-captured microglia in the Alzheimer's and Parkinson's brain reveal unique regional expression profiles and suggest a potential role for hepatitis B in the Alzheimer's brain. Neurobiol. Aging 63, 12–21. doi: 10.1016/j.neurobiolaging.2017.10.019
McGeer, P. L., Itagaki, S., Boyes, B. E., and McGeer, E. G. (1988). Reactive microglia are positive for HLA-DR in the substantia nigra of Parkinson's and Alzheimer's disease brains. Neurology 38, 1285–1291. doi: 10.1212/WNL.38.8.1285
Michell-Robinson, M. A., Touil, H., Healy, L. M., Owen, D. R., Durafourt, B. A., Bar-Or, A., et al. (2015). Roles of microglia in brain development, tissue maintenance and repair. Brain 138, 1138–1159. doi: 10.1093/brain/awv066
Miyamoto, A., Wake, H., Ishikawa, A. W., Eto, K., Shibata, K., Murakoshi, H., et al. (2016). Microglia contact induces synapse formation in developing somatosensory cortex. Nat. Commun. 7:12540. doi: 10.1038/ncomms12540
Nakatogawa, H. (2020). Mechanisms governing autophagosome biogenesis. Nat. Rev. Mol. Cell Biol. 21, 439–458. doi: 10.1038/s41580-020-0241-0
Nash, Y., Schmukler, E., Trudler, D., Pinkas-Kramarski, R., and Frenkel, D. (2017). DJ-1 deficiency impairs autophagy and reduces alpha-synuclein phagocytosis by microglia. J. Neurochem. 143, 584–594. doi: 10.1111/jnc.14222
Ouchi, Y., Yoshikawa, E., Sekine, Y., Futatsubashi, M., Kanno, T., Ogusu, T., et al. (2005). Microglial activation and dopamine terminal loss in early Parkinson's disease. Ann. Neurol. 57, 168–175. doi: 10.1002/ana.20338
Panicker, N., Sarkar, S., Harischandra, D. S., Neal, M., Kam, T.-I., Jin, H., et al. (2019). Fyn kinase regulates misfolded α-synuclein uptake and NLRP3 inflammasome activation in microglia. J. Exp. Med. 216, 1411–1430. doi: 10.1084/jem.20182191
Paolicelli, R. C., Bolasco, G., Pagani, F., Maggi, L., Scianni, M., Panzanelli, P., et al. (2011). Synaptic pruning by microglia is necessary for normal brain development. Science 333, 1456–1458. doi: 10.1126/science.1202529
Park, S. H., Lee, Y.-S., Yang, H.-J., and Song, G. J. (2021). Fluoxetine potentiates phagocytosis and autophagy in microglia. Front. Pharmacol. 12:770610. doi: 10.3389/fphar.2021.770610
Qin, Y., Qiu, J., Wang, P., Liu, J., Zhao, Y., Jiang, F., et al. (2021). Impaired autophagy in microglia aggravates dopaminergic neurodegeneration by regulating NLRP3 inflammasome activation in experimental models of Parkinson's disease. Brain Behav. Immun. 91, 324–338. doi: 10.1016/j.bbi.2020.10.010
Rojanathammanee, L., Murphy, E. J., and Combs, C. K. (2011). Expression of mutant alpha-synuclein modulates microglial phenotype in vitro. J. Neuroinflammation 8:44. doi: 10.1186/1742-2094-8-44
Sakai, M., Yu, Z., Hirayama, R., Nakasato, M., Kikuchi, Y., Ono, C., et al. (2022). Deficient autophagy in microglia aggravates repeated social defeat stress-induced social avoidance. Neural Plast. 2022:7503553. doi: 10.1155/2022/7503553
Scheiblich, H., Dansokho, C., Mercan, D., Schmidt, S. V., Bousset, L., Wischhof, L., et al. (2021). Microglia jointly degrade fibrillar alpha-synuclein cargo by distribution through tunneling nanotubes. Cells 184, 5089.e21–5106.e21. doi: 10.1016/j.cell.2021.09.007
Settembre, C., Di Malta, C., Polito, V. A., Garcia Arencibia, M., Vetrini, F., Erdin, S., et al. (2011). TFEB links autophagy to lysosomal biogenesis. Science 332, 1429–1433. doi: 10.1126/science.1204592
Sierra, A., Abiega, O., Shahraz, A., and Neumann, H. (2013). Janus-faced microglia: beneficial and detrimental consequences of microglial phagocytosis. Front. Cell. Neurosci. 7:6. doi: 10.3389/fncel.2013.00006
Song, J., Oh, Y., and Lee, J. E. (2015). miR-Let7A modulates autophagy induction in LPS-activated microglia. Exp. Neurobiol. 24, 117–125. doi: 10.5607/en.2015.24.2.117
Spangenberg, E. E., Lee, R. J., Najafi, A. R., Rice, R. A., Elmore, M. R. P., Blurton-Jones, M., et al. (2016). Eliminating microglia in Alzheimer's mice prevents neuronal loss without modulating amyloid-β pathology. Brain 139, 1265–1281. doi: 10.1093/brain/aww016
Srivastava, I. N., Shperdheja, J., Baybis, M., Ferguson, T., and Crino, P. B. (2016). mTOR pathway inhibition prevents neuroinflammation and neuronal death in a mouse model of cerebral palsy. Neurobiol. Dis. 85, 144–154. doi: 10.1016/j.nbd.2015.10.001
Stratoulias, V., Venero, J. L., Tremblay, M.-È., and Joseph, B. (2019). Microglial subtypes: diversity within the microglial community. EMBO J. 38:e101997. doi: 10.15252/embj.2019101997
Su, P., Zhang, J., Wang, D., Zhao, F., Cao, Z., Aschner, M., et al. (2016). The role of autophagy in modulation of neuroinflammation in microglia. Neuroscience 319, 155–167. doi: 10.1016/j.neuroscience.2016.01.035
Subbarayan, M. S., Hudson, C., Moss, L. D., Nash, K. R., and Bickford, P. C. (2020). T cell infiltration and upregulation of MHCII in microglia leads to accelerated neuronal loss in an α-synuclein rat model of Parkinson's disease. J. Neuroinflammation 17:242. doi: 10.1186/s12974-020-01911-4
Tan, E.-K., Chao, Y.-X., West, A., Chan, L.-L., Poewe, W., and Jankovic, J. (2020). Parkinson disease and the immune system - associations, mechanisms and therapeutics. Nat. Rev. Neurol. 16, 303–318. doi: 10.1038/s41582-020-0344-4
Tang, Y., and Le, W. (2016). Differential roles of M1 and M2 microglia in neurodegenerative diseases. Mol. Neurobiol. 53, 1181–1194. doi: 10.1007/s12035-014-9070-5
Tu, H.-Y., Yuan, B.-S., Hou, X.-O., Zhang, X.-J., Pei, C.-S., Ma, Y.-T., et al. (2021). α-Synuclein suppresses microglial autophagy and promotes neurodegeneration in a mouse model of Parkinson's disease. Aging Cell 20:e13522. doi: 10.1111/acel.13522
Wang, C., Fan, L., Khawaja, R. R., Liu, B., Zhan, L., Kodama, L., et al. (2022). Microglial NF-κB drives tau spreading and toxicity in a mouse model of tauopathy. Nat. Commun. 13:1969. doi: 10.1038/s41467-022-29552-6
Wang, C., Yeo, S., Haas, M. A., and Guan, J.-L. (2017). Autophagy gene FIP200 in neural progenitors non-cell autonomously controls differentiation by regulating microglia. J. Cell Biol. 216, 2581–2596. doi: 10.1083/jcb.201609093
Wolf, S. A., Boddeke, H. W. G. M., and Kettenmann, H. (2017). Microglia in physiology and disease. Annu. Rev. Physiol. 79, 619–643. doi: 10.1146/annurev-physiol-022516-034406
Wu, J., Chen, N., Liu, Y., Godlewski, G., Kaplan, H. J., Shrader, S. H., et al. (2021a). Studies of involvement of G-protein coupled receptor-3 in cannabidiol effects on inflammatory responses of mouse primary astrocytes and microglia. PLoS One 16:e0251677. doi: 10.1371/journal.pone.0251677
Wu, J., Xu, X., Zheng, L., Mo, J., Jin, X., and Bao, Y. (2021b). Nilotinib inhibits microglia-mediated neuroinflammation to protect against dopaminergic neuronal death in Parkinson's disease models. Int. Immunopharmacol. 99:108025. doi: 10.1016/j.intimp.2021.108025
Wu, A.-G., Zhou, X.-G., Qiao, G., Yu, L., Tang, Y., Yan, L., et al. (2021). Targeting microglial autophagic degradation in NLRP3 inflammasome-mediated neurodegenerative diseases. Ageing Res. Rev. 65:101202. doi: 10.1016/j.arr.2020.101202
Xia, Y., Zhang, G., Han, C., Ma, K., Guo, X., Wan, F., et al. (2019). Microglia as modulators of exosomal alpha-synuclein transmission. Cell Death Dis. 10:174. doi: 10.1038/s41419-019-1404-9
Yang, T.-T., Lin, C., Hsu, C.-T., Wang, T.-F., Ke, F.-Y., and Kuo, Y.-M. (2013). Differential distribution and activation of microglia in the brain of male C57BL/6J mice. Brain Struct. Funct. 218, 1051–1060. doi: 10.1007/s00429-012-0446-x
Yang, X., Lou, Y., Liu, G., Wang, X., Qian, Y., Ding, J., et al. (2017). Microglia P2Y6 receptor is related to Parkinson's disease through neuroinflammatory process. J. Neuroinflammation 14:38. doi: 10.1186/s12974-017-0795-8
Yuan, J., Liu, H., Zhang, H., Wang, T., Zheng, Q., and Li, Z. (2022). Controlled activation of TRPV1 channels on microglia to boost their autophagy for clearance of alpha-Synuclein and enhance therapy of Parkinson's disease. Adv. Mater. 34:e2108435. doi: 10.1002/adma.202108435
Keywords: Parkinson’s disease, microglia, microglial autophagy, microglial phagocytosis, neuroinflammation
Citation: Zhu R, Luo Y, Li S and Wang Z (2022) The role of microglial autophagy in Parkinson’s disease. Front. Aging Neurosci. 14:1039780. doi: 10.3389/fnagi.2022.1039780
Edited by:
Zihua Wang, Fujian Medical University, ChinaReviewed by:
Tz-Chuen Ju, Tunghai University, TaiwanFeng Li, Fudan University, China
Zhengwen Ma, Shanghai Jiao Tong University, China
Copyright © 2022 Zhu, Luo, Li and Wang. This is an open-access article distributed under the terms of the Creative Commons Attribution License (CC BY). The use, distribution or reproduction in other forums is permitted, provided the original author(s) and the copyright owner(s) are credited and that the original publication in this journal is cited, in accordance with accepted academic practice. No use, distribution or reproduction is permitted which does not comply with these terms.
*Correspondence: Zhengbo Wang, d2FuZ3piQGxwYnIuY24=
†These authors have contributed equally to this work