- 1Department of Neurosurgery, Beijing Tiantan Hospital, Capital Medical University, Beijing, China
- 2Department of Neurosurgery, China National Clinical Research Center for Neurological Diseases, Beijing, China
- 3Department of Neurosurgery, Bozhou Hospital of Traditional Chinese Medicine, Bozhou, China
- 4Department of Neurosurgery, PLA General Hospital, Beijing, China
- 5Joint Lab, Chinese Institute for Brain Research, Beijing, China
- 6Center of Stroke, Beijing Institute of Brain Disorders, Beijing, China
Background: Spinal cord stimulation (SCS) is a promising treatment for patients with disorders of consciousness (DoC); however, the laboratory examinations and different electrodes (permanent #39286 vs. temporary percutaneous #3777, Medtronic, USA) that are associated with postoperative outcomes are unclear. The study aims to study the association between the change in postoperative cerebrospinal fluid (CSF) protein level and improvement in consciousness after SCS in DoC patients and to explore whether different electrodes were associated with elevated CSF protein levels.
Materials and methods: A total of 66 DoC patients who received SCS treatment from December 2019 to December 2021 were retrospectively analyzed. Patients were grouped according to their elevated CSF protein level. The clinical characteristics of the patients and SCS stimulation parameters were compared. The preoperative sagittal diameter of the spinal canal is the distance from the midpoint of the posterior border of the vertebral body to the midpoint of the posterior wall of the spinal canal at the level of the superior border of C3. The postoperative sagittal diameter of the spinal canal is the distance from the midpoint of the posterior edge of the vertebral body to the anterior edge of the stimulation electrode. Patients with improved postoperative CRS-R scores greater than 3 or who progressed to the MCS + /eMCS were classified as the improved group and otherwise regarded as poor outcome.
Results: We found that more DoC patients had elevated CSF protein levels among those receiving SCS treatment with permanent electrodes than temporary percutaneous electrodes (P = 0.001), and elevated CSF protein levels were significantly associated with a reduced sagittal diameter (P = 0.044). In DoC patients receiving SCS treatment, we found that elevated CSF protein levels (P = 0.022) and preoperative diagnosis (P = 0.003) were significantly associated with poor outcomes at 3 months. Logistic regression analysis showed that elevated CSF protein levels were significantly associated with poor outcomes (OR 1.008, 95% CI 1.001–1.016, P = 0.032).
Conclusion: The results suggest that reducing the effect of electrode pads on anatomical changes may help improve the outcomes of DoC patients receiving SCS treatment. CSF protein levels are associated with poor postoperative outcomes and whether they are potential biomarkers in DoC patients receiving SCS treatment remain further exploration.
Introduction
Disorders of consciousness (DoC) are among the most frequent complications in patients after severe brain injury and are mainly caused by trauma, stroke, or anoxia (Edlow et al., 2021). DoCs include coma, vegetative state/unresponsive wakefulness syndrome (VS/UWS), and the minimally conscious state (MCS). In recent years, with the development of electrical stimulation technology, neuromodulation technologies represented by deep brain stimulation (DBS) and spinal cord stimulation (SCS) have been increasingly used to treat DoC and have shown encouraging results (Vanhoecke and Hariz, 2017; Xia et al., 2018; Xu et al., 2019; Yang et al., 2022). Originally, SCS was mainly applied to patients with chronic pain, and later in the 1980s, Kanno et al. first reported encouraging results regarding SCS in patients with DoC (Kanno et al., 1987, 1988). The surgical procedure of SCS involves implanting electrodes along the midline of the posterior epidural space of the C2-C4 level and delivering electrical stimulation to the circuitry governing awareness (Guerra et al., 2014). The progress made by researchers has led to the preliminary exploration of the clinical factors that affect the treatment effect of SCS in DoC patients. However, the effects of different electrodes on clinical outcomes and laboratory parameters are still unclear.
Cerebrospinal fluid (CSF) is secreted by the choroid plexus of the ventricle, and its properties are similar to those of plasma and lymph, which act as diagnostic tools for many conditions affecting the central nervous system (Dhiman et al., 2019; Nakada and Kwee, 2019; Gaunitz et al., 2021; Fang et al., 2022). The main proteins in CSF are albumin and macroglobulin, and under pathological conditions such as central nervous system infection, nerve tuberculosis and nerve demyelination, the destruction of the blood–brain barrier will increase the total amount of CSF proteins (Engelhardt and Sorokin, 2009). The increase in CSF protein levels may impair CSF reabsorption, thereby affecting CSF circulation and increasing the risk of hydrocephalus (Miller et al., 1990). Recent studies have shown that DBS can induce proteomic changes in the CSF of patients, and these changes may be related to the underlying mechanism of invasive neuromodulation (Zsigmond et al., 2020). However, there are no studies on the effect of SCS on total CSF protein levels in DoC patients. We proposed that elevated CSF protein levels are associated with poor short-term outcomes, and reduced anatomic structure like sagittal diameter may contribute to it.
Materials and methods
Study participants
We retrospectively reviewed 66 patients with DoC who were treated with SCS at the Neurosurgery Department in Beijing Tiantan Hospital and Peking University International Hospital between December 2019 and December 2021. Considering that the patients could not understand and legally consent to treatment, the SCS procedure and the possible risks were explained to the patients’ legal representatives and/or family members. Once the realistic expectations were explained, their legal representatives and/or family members signed an informed consent document compatible with our institution’s legal and ethical committee regulations, where this trial was approved (2011-0415). When their caregivers provided written informed consent for their participation, the patients were enrolled.
Inclusion and exclusion criteria
The inclusion criteria were as follows: (1) Clinical diagnosis of DoC (Kondziella et al., 2020; Yang et al., 2022); (2) DoC (including the VS and the MCS) lasting for more than 28 days; (3) Aged 18–70 years; (4) No significant improvement in the level of consciousness within 1 month before enrollment; and (5) Family members of the patients gave informed consent and signed the informed consent form. The exclusion criteria were as follows: (1) Coma caused by neurodegenerative diseases or postoperative coma after craniocerebral tumor operation and (2) Serious untreated complications before enrollment.
Data collection
Data were extracted from the enrolled patients’ medical records between December 2019 and December 2021. The baseline variables included age, sex, pathogenesis, duration of DoC, diagnosis, hydrocephalus, and paroxysmal sympathetic hyperactivity (PSH). After obtaining informed consent, 2–3 ml of middle CSF samples were taken during routine lumbar puncture. The samples were kept on ice and transferred to −20°C refrigerator within 10 min.
We also collected CSF samples from patients preoperatively and on the 7th day postoperatively, and the total CSF protein level was determined by rate nephelometry. The detection process was conducted in accordance with the manufacturer’s instructions.
Surgical procedure and stimulation protocol
39286 electrode is 56.4 mm long, 7.6 mm wide, 1.9 mm thick, 4 mm contact length, 1.5 mm contact spacing, 42.5 mm contact tip to contact tip, and 65cm electrode tip to wire tip. In total, 3,777 electrode contact is 3 mm, the spacing is 6 mm, the length from the electrode contact tip to the contact tip is 66mm, and the total length from the electrode tip to the contact tip is 75 cm. The detailed procedure for the placement of permanent electrodes (39286, Medtronic, USA) can be found in our previous study (Yang et al., 2022; Figure 1). A midline incision at the C5 level was made under general anesthesia. After the C5 spinous process was exposed, a 2-cm-wide opening was made in the C5 spinous process and lamina. A silicone electrode model was inserted at the C2-C4 level and then replaced with a Medtronic 39286 electrode. The stimulation protocol was applied during the daytime, approximately 12 h per day. The cranial electrode was the negative pole, and the caudal electrode was the effective pole. The posterior columns were stimulated at a frequency of 70 Hz and a pulse width of 120 μs. The stimulation parameters were chosen to remain below the motor threshold, as a motor response usually occurs above 2∼2.5 V.
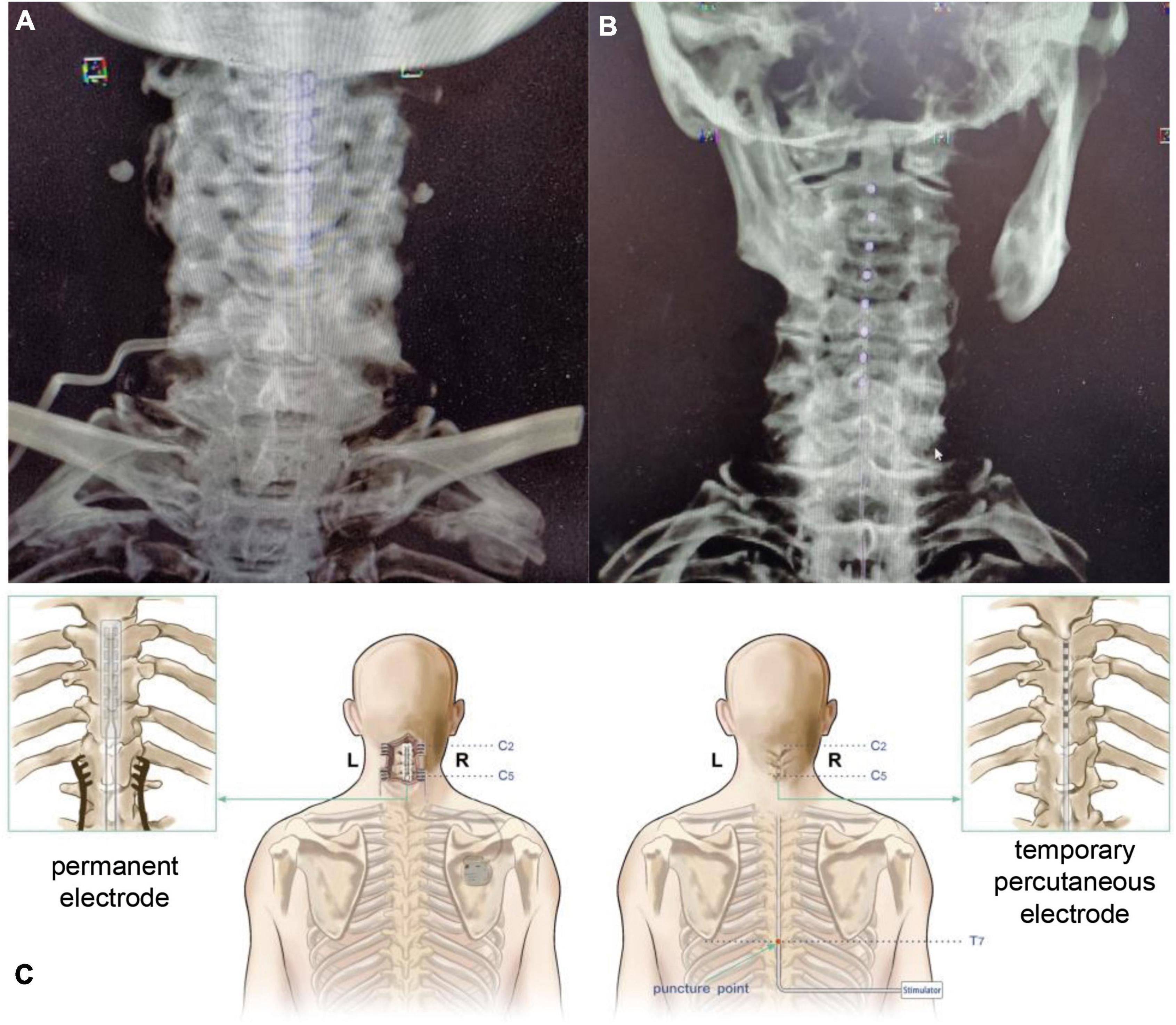
Figure 1. Spinal cord stimulation (SCS) surgical procedure with different electrodes. (A) Postoperative CT VRT reconstruction of patients receiving permanent electrode implantation. (B) Postoperative CT VRT reconstruction of patients receiving temporary percutaneous electrode implantation. (C) Schematic diagram of SCS surgeries with different electrodes. SCS, spinal cord stimulation, CT, computed tomography, VRT, volume rendering technique.
For temporary percutaneous electrode placement (3777, Medtronic, USA), the patient was placed in a prone position, and the T7-8 intervertebral space was positioned under the C-arm as the puncture point. The electrode (Medtronic, 3777) was inserted at the C2 level and fixed. The day after the temporary SCS operation, electric stimulation was applied to the patient’s dorsal column at a voltage of 2.5 V and a frequency of 70 Hz with a 120 μs wave width, which is consistent with the SCS treatment using permanent electrodes. The overall stimulation lasted for 21 days, and then the electrode was removed.
Imaging data
Before and after surgery, 64-slice spiral computed tomography (CT) (Philips, Netherlands) was used to obtain plain cervical CT scans, coronal scans, and sagittal scans of the enrolled patients. The axial images of the upper edge of the C3 vertebral body were obtained from the imaging system. The data were measured and processed independently by two senior clinicians using ImageJ (1.42) software, and the measurement results were averaged. Finally, the sagittal diameter of the spinal canal was obtained. The preoperative sagittal diameter of the spinal canal is the distance from the midpoint of the posterior border of the vertebral body to the midpoint of the posterior wall of the spinal canal at the level of the superior border of C3. The postoperative sagittal diameter of the spinal canal is the distance from the midpoint of the posterior edge of the vertebral body to the anterior edge of the stimulation electrode. Patients with a sagittal diameter reduction of more than 1.5 #39286 electrode pads (3 units) were classified as the reduced sagittal diameter group (Figure 2).
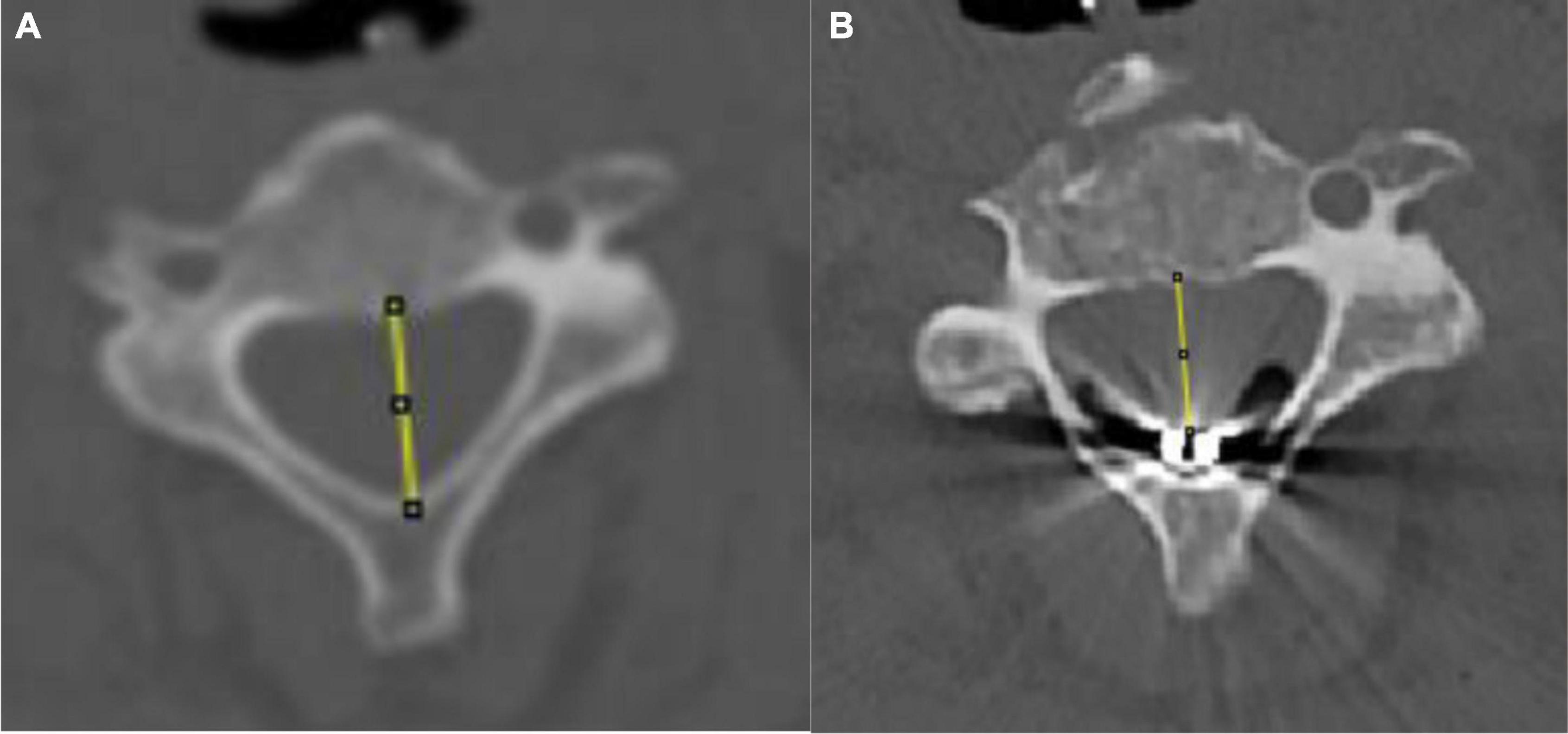
Figure 2. Schematic of pre- and postoperative sagittal diameter. (A) Preoperative sagittal diameter evaluation. (B) Postoperative sagittal diameter evaluation.
JFK coma recovery scale–revised
The consciousness level of each patient was assessed using the CRS-R16. On the day before surgery and the 30th day after surgery, the CRS-R score was independently evaluated by 2 clinicians. For patients with an auditory score of ≤2 points, a visual score of ≤1 point, a motor score of ≤2 points, a language score of ≤2 points, a communication score of 0 points and an arousal score of ≤2 points, the diagnosis was VS. For patients with an auditory score of 3 to 4, a visual score of 2 to 5, a motor score of 3 to 5, a language score of 3, a communication score of 1 and an arousal score of ≤2, the diagnosis was MCS. Patients with improved postoperative CRS-R scores greater than 3 or who progressed to the MCS + /eMCS were classified as the improved group and otherwise regarded as poor outcome.
Statistical analysis
SPSS 22.0 software was used to process the data. The Kolmogorov–Smirnov test was used to determine normality. Normally distributed measurement data are expressed as the mean ± standard deviation. Age was compared using an independent samples t-test. Chi-square tests were used for categorical variables. P for trend was tested using one-way analysis of variance (ANOVA) for continuous variables and linear-by-linear association for categorical variables. Logistic regression analysis was performed to determine the factors related to outcomes with default parameters. The significance threshold was a two-sided P-value less than 0.05.
Results
Baseline characteristics of patients with disorders of consciousness after spinal cord stimulation
Between December 2019 and December 2021, SCS was performed on 66 patients, 47 males (71.2%) and 19 females (28.8%), with an average age of 46.38 ± 13.33 years. The characteristics are summarized in Table 1. Twelve patients were allocated to the improved group, while 54 were allocated to the non-improved group. There was no significant difference in age (P = 0.087) or sex (0.732) between the groups. In this cohort, 27 (40.9%) cases were caused by traumatic brain injury, 11 (16.7%) cases were caused by anoxia and 28 (42.4%) cases were caused by stroke, and there was no significant difference between the groups (P = 0.998). We also found no significant difference in the duration of DoC (P = 0.839). There were significantly more patients in a MCS in the improved group (P = 0.003). There were significantly more patients with elevated CSF protein levels in the non-improved group (P = 0.022). Regarding complications, there was no significant difference in the occurrence of hydrocephalus (P = 0.752) or PSH (P = 0.752) between the groups.
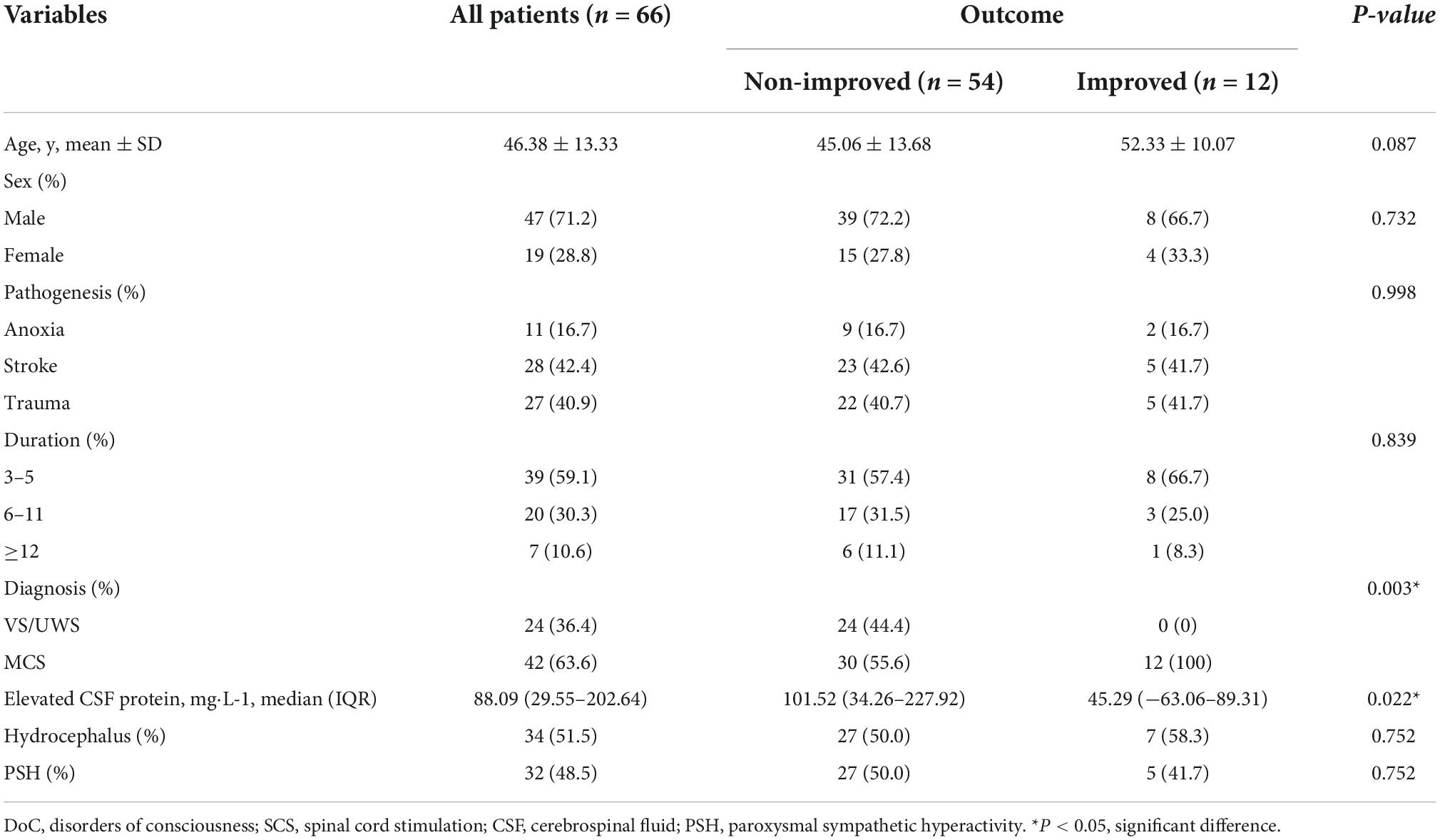
Table 1. Baseline characteristics of patients with disorders of consciousness (DoC) after spinal cord stimulation (SCS).
Clinical features of disorders of consciousness patients receiving spinal cord stimulation according to elevated cerebrospinal fluid protein levels
The clinical features of DoC patients receiving SCS according to elevated CSF protein levels are summarized in Table 2. The mean age of patients in the elevated CSF protein level group was slightly lower than that in the stable CSF protein level group, albeit not significant (P = 0.201). There were also no significant differences in sex (P = 0.099), pathogenesis (P = 0.532), duration of DoC (P = 0.379) or diagnosis (P = 0.062) in the elevated CSF protein level group. Complications such as hydrocephalus (P = 0.369) and PSH (P = 0.369) were also not different.
Logistic regression analysis associated with outcomes
We analyzed the potential factors associated with outcomes in DoC patients receiving SCS treatment, and the results are shown in Table 3. Age, sex, pathogenesis and duration of DoC were all considered in the logistic regression analysis. However, after adjusting for all potential covariables, the results showed that an elevated CSF protein level was the only significant factor associated with poor outcomes (OR 1.008, 95% CI 1.001–1.016, P = 0.032).
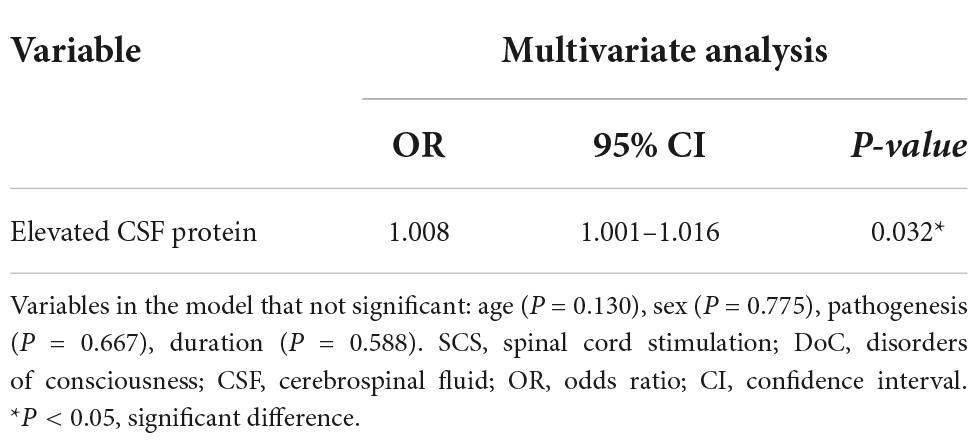
Table 3. Logistic regression analysis of the risk of poor short-term outcomes after SCS in DoC patients.
The association between cerebrospinal fluid protein level and surgery-related factors
To further examine whether surgery-related factors could affect CSF protein levels, we analyzed the relationship between SCS electrodes and the sagittal diameter or CSF protein level. The results showed that in the permanent electrode group, there were significantly more patients with a reduced sagittal diameter (P < 0.001) and elevated CSF protein level (P = 0.001) than in the temporary puncture electrode group (Supplementary Table 1). We also found more DoC patients with elevated CSF protein levels in the reduced sagittal diameter group (P = 0.044, Supplementary Table 2).
Discussion
In this cohort study, we found that in DoC patients receiving SCS treatment, an elevated CSF protein level was significantly associated with poor outcomes at 3 months. This result suggests that CSF protein levels may play a role in the process of rehabilitation and may be a potential biomarker in DoC patients receiving SCS treatment. Logistic regression analysis showed that elevated CSF protein levels were significantly associated with poor outcomes. We also found more DoC patients with elevated CSF protein levels among those receiving SCS treatment with permanent electrodes rather than temporary percutaneous electrodes and more DoC patients with a reduced sagittal diameter in the permanent electrodes group. Furthermore, we found that elevated CSF protein levels were significantly associated with a reduced sagittal diameter. The reason an elevated CSF protein level was more significant in the permanent electrode group was because of the larger size of the electrode pads.
In recent decades, because the pathogenesis of DoC has not been clearly stated, developments in the treatment of DoC has stagnated and different treatments have minimal effects. In recent years, with the development of neuromodulation technology, many emerging tools have been applied to the evaluation, diagnosis, and treatment of DoC (Della Pepa et al., 2013). SCS, as an invasive neuromodulation technique, is being increasingly used in the treatment of DoC (Mattogno et al., 2017; Xu et al., 2019; Wang et al., 2020; Yang et al., 2022). The underlying mechanisms of SCS for the treatment of DoC mainly include increasing cerebral blood perfusion, increasing the local glucose metabolic rate, releasing catecholamines and regulating oxidative stress (decreasing superoxide radical content) (Clavo et al., 2008; Liu et al., 2008; Wu et al., 2008; Visocchi et al., 2011; Si et al., 2018). Previous studies have shown that SCS can cause changes in CSF proteomics, and researchers have explored the key proteins that may be involved in the mechanism of SCS for treating chronic pain (Royds et al., 2020). However, the changes in total CSF protein levels after SCS treatment have rarely been studied, especially in patients with DoC.
The possible mechanisms underlying the change in total CSF protein level after SCS treatment may be due to the disruption of the blood–brain barrier by intraoperative local procedures or the disturbance of regional CSF circulation. Traditionally, CSF protects the brain and spinal cord from mechanical injury, but the understanding of the dynamic metabolism and function of CSF has rapidly improved in recent decades (Louveau et al., 2015). It has been reported that CSF is responsible for transporting hormones and waste protein via dynamic circulation, and increased CSF protein levels have been reported to play a role in neurodegenerative diseases such as Alzheimer’s disease, aging and other pathological processes, including subarachnoid hemorrhage and trauma (May et al., 1990; Iliff et al., 2012; Kress et al., 2014; Puvenna et al., 2016; Fesharaki-Zadeh, 2019; Coulibaly and Provencio, 2020). Disruption of the blood–brain barrier by intraoperative procedures may lead to the leakage of plasma proteins into CSF (Johnston and Teo, 2000). Previous studies have shown that nerve root compression caused by various factors can cause damage to the blood–brain barrier, therefore increasing the total CSF protein level, and the degree of increase in the total CSF protein level is positively correlated with the degree of nerve root compression (Skouen et al., 1994). SCS electrode implantation involves blunt traction on the surrounding tissue and requires removing the spinous process and part of the laminae in permanent electrode implantation procedures, which inevitably causes slight physical damage to the nerve root, as confirmed by intraoperative electrophysiological monitoring. The disruption of the blood–brain barrier caused by this physical injury may be one of the reasons for the increase in CSF protein levels in certain patients after SCS. Another reason for the increase in CSF protein levels may be due to the certain impact of SCS electrode implantation on the structure of the local CSF circulation pathway. This may be because the SCS electrode affects the normal circulation of CSF, causing a decrease in the turnover rate of CSF, which in turn leads to an increase in CSF protein levels (Chen et al., 2010). Thus, the circulation of CSF could be disrupted and cause worse outcome in those patients receiving SCS treatment. Our study also showed that after the implantation of SCS electrodes, the anatomical morphology of the implantation site is changed to a certain extent. The dura is partially compressed, the sagittal diameter of the spinal canal is reduced, and there is a significant correlation with elevated CSF protein levels (Son et al., 2018).
Furthermore, we found that elevated CSF protein levels after SCS were associated with lower patient emergence rate at 3 months. Previous studies have shown similar results: at the proteomic level, DBS, an invasive neuroregulatory procedure, can up- or downregulate the expression level of various proteins in CSF and peripheral blood and may further affect prognosis (Zsigmond et al., 2020). Additionally, Royds et al. studied the characteristics of CSF in patients with chronic pain who received SCS and found significant alterations in protein-like growth hormones. However, the sample size of that study was too small (limited to 4 patients), so the authors found no correlation between changes in CSF protein level and outcomes. Proteomic-level alterations in CSF proteins could affect the prognosis of DoC patients undergoing SCS treatment, but this requires further validation. Since elevated CSF protein levels are significantly associated with postoperative anatomical changes and affect patient outcomes, we believe that reducing the size of the electrode pads may have a potential role in improving patient outcomes. There is currently no dedicated commercial electrode developed for the treatment of DoC. Most of the current electrodes are designed for chronic pain, and larger electrode pads are selected to cover a larger area. Therefore, improving the electrodes used in surgery can further reduce anatomical changes and may further improve outcomes in patients with DoC treated with SCS.
Our study has several limitations. First, a predictive model was not generated due to the limited number of patients enrolled in this study. Second, in the current study, we tracked the change in the total CSF protein level for a short period of time and did not explore the time-course trend of the total CSF protein level, which may further clarify the effects of such factors on patient outcomes. Finally, we did not perform further proteomic analysis of CSF in this study. Future research on CSF composition may help to further elucidate this issue and explore treatment methods. Large-scale, long-term follow-up studies are still needed to validate the preliminary results obtained in this study.
Conclusion
In this study, we found that elevated CSF protein levels were significantly associated with poor outcomes in DoC patients after SCS treatment and significantly related to a reduced sagittal diameter, which suggests that CSF protein levels may play a role in rehabilitation. The level of CSF protein may also serve as a biomarker in DoC patients receiving SCS treatment and reducing the effect of electrode pads on anatomical changes may help improve the outcomes of DoC patients receiving SCS treatment.
Data availability statement
The raw data supporting the conclusions of this article will be made available by the authors, without undue reservation.
Ethics statement
The studies involving human participants were reviewed and approved by the Ethics Committee of Beijing Tiantan Hospital. The patients/participants provided their written informed consent to participate in this study.
Author contributions
QH and YY designed the study and wrote the manuscript. TL, YX, XX, YD, XC, and XG collected the data and samples. JH and JZ supervised the study. All authors contributed to the article and approved the submitted version.
Funding
This research was supported by the following funding sources: National Natural Science Foundation of China (No. 81600919), Beijing Municipal Science and Technology Commission (Nos. Z161100000516165 and Z171100001017162), and Beijing Nova Program (Z181100006218050).
Acknowledgments
We thank Xingyue Zhang for reviewing our statistical analysis, which was essential for the successful completion of this study.
Conflict of interest
The authors declare that the research was conducted in the absence of any commercial or financial relationships that could be construed as a potential conflict of interest.
Publisher’s note
All claims expressed in this article are solely those of the authors and do not necessarily represent those of their affiliated organizations, or those of the publisher, the editors and the reviewers. Any product that may be evaluated in this article, or claim that may be made by its manufacturer, is not guaranteed or endorsed by the publisher.
Supplementary material
The Supplementary Material for this article can be found online at: https://www.frontiersin.org/articles/10.3389/fnagi.2022.1032740/full#supplementary-material
References
Chen, C. P., Chen, R. L., and Preston, J. E. (2010). The influence of cerebrospinal fluid turnover on age-related changes in cerebrospinal fluid protein concentrations. Neurosci. Lett. 476, 138–141. doi: 10.1016/j.neulet.2010.04.015
Clavo, B., Robaina, F., Montz, R., Carames, M. A., Otermin, E., and Carreras, J. L. (2008). Effect of cervical spinal cord stimulation on cerebral glucose metabolism. Neurol. Res. 30, 652–654. doi: 10.1179/174313208X305373
Coulibaly, A. P., and Provencio, J. J. (2020). Aneurysmal subarachnoid hemorrhage: An overview of inflammation-induced cellular changes. Neurotherapeutics 17, 436–445. doi: 10.1007/s13311-019-00829-x
Della Pepa, G. M., Fukaya, C., La Rocca, G., Zhong, J., and Visocchi, M. (2013). Neuromodulation of vegetative state through spinal cord stimulation: Where are we now and where are we going? Stereotact. Funct. Neurosurg. 91, 275–287. doi: 10.1159/000348271
Dhiman, K., Blennow, K., Zetterberg, H., Martins, R. N., and Gupta, V. B. (2019). Cerebrospinal fluid biomarkers for understanding multiple aspects of Alzheimer’s disease pathogenesis. Cell. Mol. Life Sci. 76, 1833–1863. doi: 10.1007/s00018-019-03040-5
Edlow, B. L., Claassen, J., Schiff, N. D., and Greer, D. M. (2021). Recovery from disorders of consciousness: Mechanisms, prognosis and emerging therapies. Nat. Rev. Neurol. 17, 135–156. doi: 10.1038/s41582-020-00428-x
Engelhardt, B., and Sorokin, L. (2009). The blood-brain and the blood-cerebrospinal fluid barriers: Function and dysfunction. Semin. Immunopathol. 31, 497–511. doi: 10.1007/s00281-009-0177-0
Fang, Y., Huang, L., Wang, X., Si, X., Lenahan, C., Shi, H., et al. (2022). A new perspective on cerebrospinal fluid dynamics after subarachnoid hemorrhage: From normal physiology to pathophysiological changes. J. Cereb. Blood Flow Metab. 42, 543–558. doi: 10.1177/0271678X211045748
Fesharaki-Zadeh, A. (2019). Chronic traumatic encephalopathy: A brief overview. Front. Neurol. 10:713. doi: 10.3389/fneur.2019.00713
Gaunitz, S., Tjernberg, L. O., and Schedin-Weiss, S. (2021). What can N-glycomics and N-glycoproteomics of cerebrospinal fluid tell us about Alzheimer disease? Biomolecules 11:858. doi: 10.3390/biom11060858
Guerra, A., Costantini, E. M., Maatta, S., Ponzo, D., and Ferreri, F. (2014). Disorders of consciousness and electrophysiological treatment strategies: A review of the literature and new perspectives. Curr. Pharm. Des. 20, 4248–4267. doi: 10.2174/13816128113196660648
Iliff, J. J., Wang, M., Liao, Y., Plogg, B. A., Peng, W., Gundersen, G. A., et al. (2012). A paravascular pathway facilitates Csf flow through the brain parenchyma and the clearance of interstitial solutes, including amyloid beta. Sci. Transl. Med. 4:147ra11. doi: 10.1126/scitranslmed.3003748
Johnston, I., and Teo, C. (2000). Disorders of Csf hydrodynamics. Childs Nerv. Syst. 16, 776–799. doi: 10.1007/s003810000383
Kanno, T., Kamei, Y., Yokoyama, T., and Jain, V. K. (1987). Neurostimulation for patients in vegetative status. Pacing Clin. Electrophysiol. 10, 207–208. doi: 10.1111/j.1540-8159.1987.tb05949.x
Kanno, T., Kamei, Y., Yokoyama, T., Shoda, M., Tanji, H., and Nomura, M. (1988). [Effects of neurostimulation on the reversibility of neuronal function: Experience of treatment for vegetative status]. No Shinkei Geka 16, 157–163.
Kondziella, D., Bender, A., Diserens, K., van Erp, W., Estraneo, A., Formisano, R., et al. (2020). European academy of neurology guideline on the diagnosis of coma and other disorders of consciousness. Eur. J. Neurol. 27, 741–756. doi: 10.1111/ene.14151
Kress, B. T., Iliff, J. J., Xia, M., Wang, M., Wei, H. S., Zeppenfeld, D., et al. (2014). Impairment of paravascular clearance pathways in the aging brain. Ann. Neurol. 76, 845–861. doi: 10.1002/ana.24271
Liu, J. T., Tan, W. C., and Liao, W. J. (2008). Effects of electrical cervical spinal cord stimulation on cerebral blood perfusion, cerebrospinal fluid catecholamine levels, and oxidative stress in comatose patients. Acta Neurochir. Suppl. 101, 71–76. doi: 10.1007/978-3-211-78205-7_12
Louveau, A., Smirnov, I., Keyes, T. J., Eccles, J. D., Rouhani, S. J., Peske, J. D., et al. (2015). Structural and functional features of central nervous system lymphatic vessels. Nature 523, 337–341. doi: 10.1038/nature14432
Mattogno, P. P., Barbagallo, G., Iacopino, G., Pappalardo, G., Lar, G., Signorelli, F., et al. (2017). Recovery from chronic diseases of consciousness: State of the art in neuromodulation for persistent vegetative state and minimally conscious state. Acta Neurochir. Suppl. 124, 19–25. doi: 10.1007/978-3-319-39546-3_4
May, C., Kaye, J. A., Atack, J. R., Schapiro, M. B., Friedland, R. P., and Rapoport, S. I. (1990). Cerebrospinal fluid production is reduced in healthy aging. Neurology 40, 500–503. doi: 10.1212/wnl.40.3_part_1.500
Miller, G., Towfighi, J., and Page, R. B. (1990). Spinal cord ganglioglioma presenting as hydrocephalus. J. Neurooncol. 9, 147–152. doi: 10.1007/BF02427835
Nakada, T., and Kwee, I. L. (2019). Fluid dynamics inside the brain barrier: Current concept of interstitial flow, glymphatic flow, and cerebrospinal fluid circulation in the brain. Neuroscientist 25, 155–166. doi: 10.1177/1073858418775027
Puvenna, V., Engeler, M., Banjara, M., Brennan, C., Schreiber, P., Dadas, A., et al. (2016). Is phosphorylated tau unique to chronic traumatic encephalopathy? phosphorylated tau in epileptic brain and chronic traumatic encephalopathy. Brain Res. 1630, 225–240. doi: 10.1016/j.brainres.2015.11.007
Royds, J., Conroy, M. J., Dunne, M. R., Cassidy, H., Matallanas, D., Lysaght, J., et al. (2020). Examination and characterisation of burst spinal cord stimulation on cerebrospinal fluid cellular and protein constituents in patient responders with chronic neuropathic pain - a pilot study. J. Neuroimmunol. 344:577249. doi: 10.1016/j.jneuroim.2020.577249
Si, J., Dang, Y., Zhang, Y., Li, Y., Zhang, W., Yang, Y., et al. (2018). Spinal cord stimulation frequency influences the hemodynamic response in patients with disorders of consciousness. Neurosci. Bull. 34, 659–667. doi: 10.1007/s12264-018-0252-4
Skouen, J. S., Larsen, J. L., and Vollset, S. E. (1994). Cerebrospinal fluid protein concentrations related to clinical findings in patients with sciatica caused by disk herniation. J. Spinal Disord. 7, 12–18. doi: 10.1097/00002517-199407010-00002
Son, B. C., Ko, H. C., and Choi, J. G. (2018). Compression of thoracic spinal cord with decreased cerebrospinal fluid space after implantation of paddle lead spinal cord stimulation at T9: A three-dimensional myelographic computed tomography study. World Neurosurg. 118:e323–e334. doi: 10.1016/j.wneu.2018.06.186
Vanhoecke, J., and Hariz, M. (2017). Deep brain stimulation for disorders of consciousness: Systematic review of cases and ethics. Brain Stimul. 10, 1013–1023. doi: 10.1016/j.brs.2017.08.006
Visocchi, M., Della Pepa, G. M., Esposito, G., Tufo, T., Zhang, W., Li, S., et al. (2011). Spinal cord stimulation and cerebral hemodynamics: Updated mechanism and therapeutic implications. Stereotact. Funct. Neurosurg. 89, 263–274. doi: 10.1159/000329357
Wang, Y., Bai, Y., Xia, X., Yang, Y., He, J., and Li, X. (2020). Spinal cord stimulation modulates complexity of neural activities in patients with disorders of consciousness. Int. J. Neurosci. 130, 662–670. doi: 10.1080/00207454.2019.1702543
Wu, M., Komori, N., Qin, C., Farber, J. P., Linderoth, B., and Foreman, R. D. (2008). Extracellular signal-regulated kinase (Erk) and protein kinase B (Akt) pathways involved in spinal cord stimulation (Scs)-induced vasodilation. Brain Res. 1207, 73–83. doi: 10.1016/j.brainres.2007.12.072
Xia, X., Yang, Y., Guo, Y., Bai, Y., Dang, Y., Xu, R., et al. (2018). Current status of neuromodulatory therapies for disorders of consciousness. Neurosci. Bull. 34, 615–625. doi: 10.1007/s12264-018-0244-4
Xu, Y., Li, P., Zhang, S., Wang, Y., Zhao, X., Wang, X., et al. (2019). Cervical spinal cord stimulation for the vegetative state: A preliminary result of 12 cases. Neuromodulation 22, 347–354. doi: 10.1111/ner.12903
Yang, Y., He, Q., Xia, X., Dang, Y., Chen, X., He, J., et al. (2022). Long-term functional prognosis and related factors of spinal cord stimulation in patients with disorders of consciousness. CNS Neurosci. Ther. 28, 1249–1258. doi: 10.1111/cns.13870
Keywords: disorders of consciousness, spinal cord stimulation, cerebrospinal fluid protein, sagittal diameter, outcome
Citation: He Q, Li T, Xiong Y, Xia X, Dang Y, Chen X, Geng X, He J, Yang Y and Zhao J (2022) Elevated cerebrospinal fluid protein levels associated with poor short-term outcomes after spinal cord stimulation in patients with disorders of consciousness. Front. Aging Neurosci. 14:1032740. doi: 10.3389/fnagi.2022.1032740
Received: 31 August 2022; Accepted: 17 October 2022;
Published: 03 November 2022.
Edited by:
Yi Guo, Jinan University, ChinaReviewed by:
Juanning Si, Beijing Information Science and Technology University, ChinaLinling Li, Shenzhen University, China
Shun Yao, Center for Pituitary Tumor Surgery, Department of Neurosurgery, The First Affiliated Hospital, Sun Yat-sen University, China
Gan Huang, UCLouvain, Belgium
Copyright © 2022 He, Li, Xiong, Xia, Dang, Chen, Geng, He, Yang and Zhao. This is an open-access article distributed under the terms of the Creative Commons Attribution License (CC BY). The use, distribution or reproduction in other forums is permitted, provided the original author(s) and the copyright owner(s) are credited and that the original publication in this journal is cited, in accordance with accepted academic practice. No use, distribution or reproduction is permitted which does not comply with these terms.
*Correspondence: Jianghong He, aGVfamlhbmdob25nQHNpbmEuY24=; Yi Yang, eWFuZ3lpXzgxbmtAMTYzLmNvbQ==
†These authors have contributed equally to this work