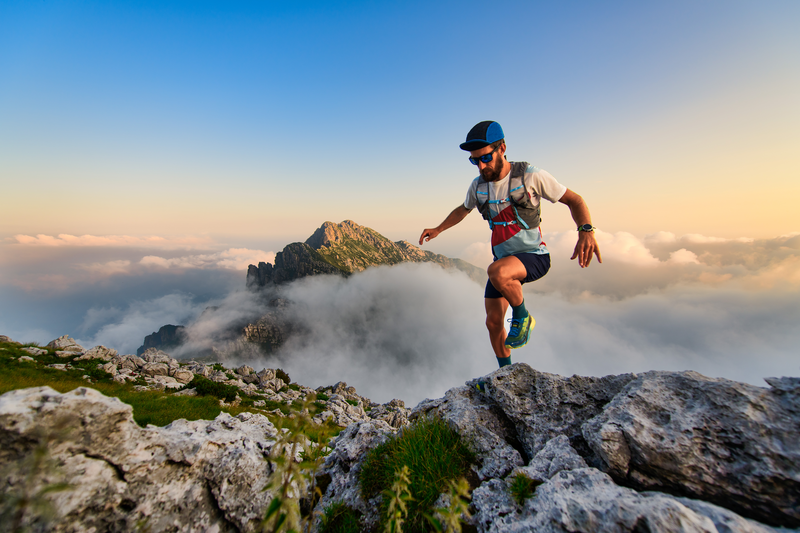
94% of researchers rate our articles as excellent or good
Learn more about the work of our research integrity team to safeguard the quality of each article we publish.
Find out more
MINI REVIEW article
Front. Aging Neurosci. , 13 December 2022
Sec. Alzheimer's Disease and Related Dementias
Volume 14 - 2022 | https://doi.org/10.3389/fnagi.2022.1019942
This article is part of the Research Topic Nutritional Interventions on Age-Related Neurodegenerative Diseases View all 13 articles
Alzheimer′s disease (AD) is an irreversible progressive neurodegenerative disease affecting approximately 50 million people worldwide. It is estimated to reach 152 million by the year 2050. AD is the fifth leading cause of death among Americans age 65 and older. In spite of the significant burden the disease imposes upon patients, their families, our society, and our healthcare system, there is currently no cure for AD. The existing approved therapies only temporarily alleviate some of the disease’s symptoms, but are unable to modulate the onset and/or progression of the disease. Our failure in developing a cure for AD is attributable, in part, to the multifactorial complexity underlying AD pathophysiology. Nonetheless, the lack of successful pharmacological approaches has led to the consideration of alternative strategies that may help delay the onset and progression of AD. There is increasing recognition that certain dietary and nutrition factors may play important roles in protecting against select key AD pathologies. Consistent with this, select nutraceuticals and phytochemical compounds have demonstrated anti-amyloidogenic, antioxidative, anti-inflammatory, and neurotrophic properties and as such, could serve as lead candidates for further novel AD therapeutic developments. Here we summarize some of the more promising dietary phytochemicals, particularly polyphenols that have been shown to positively modulate some of the important AD pathogenesis aspects, such as reducing β-amyloid plaques and neurofibrillary tangles formation, AD-induced oxidative stress, neuroinflammation, and synapse loss. We also discuss the recent development of potential contribution of gut microbiome in dietary polyphenol function.
AD is a complex disease, which makes its pathophysiology difficult to decipher and consequently challenging to treat or cure. The classical pathologic hallmarks of AD include extracellular accumulation of β-amyloid (Aβ) and intracellular Tau protein aggregation which lead to, respectively, neuritic plaques and neurofibrillary tangles’ formation in the brain. The amyloid plaques and tau tangles aggregate trigger successive deleterious events and other chronic aberrant central nervous system (CNS) features such as hyperactive inflammation, oxidative stress, and sub-optimal energy metabolism which eventually lead to synapse loss and neuronal death. These cellular damages manifest in patients as a progressive neurocognitive impairment accompanied by language alterations and a progressive deterioration of a person’s ability to perform everyday activities (Alzheimers Dement, 2020).
Aging is the greatest risk factor for AD. Many genetic risk factors have also been identified, with ApoE4 being the biggest known genetic risk factor. However, there are also lifestyle and environmental risk factors for AD, including lifestyle conditions associated with diabetes and cardiovascular diseases and environmental factors leading to traumatic brain injuries and depression (Armstrong, 2019). Aging and, to a large extent, genetic risk factors, are not amenable to modification (Riedel et al., 2016). In contrast, lifestyle and environmental risks are more readily modifiable. Moreover, there is increasing evidence implicating specific lifestyle factors (e.g., dietary factors such as specific phytochemicals) or environmental factors (e.g., exercise) may protect against AD mechanisms (Xu et al., 2015; Dominguez et al., 2021; Guasch-Ferré and Willett, 2021; Zhang et al., 2021). There is increasing interest in novel AD treatments targeting select relevant lifestyles or environmental factors. In this review, we will focus our discussion on a specific subclass of protective dietary phytochemicals, namely polyphenols, with promise for AD therapeutics.
Dietary components have a direct molecular impact on AD. Over the past decade, a widely distributed subclass of dietary components, polyphenols, have raised great interest in the scientific community for their potential role in protection against AD. Substantial number of clinical trials have been conducted to assess their clinical benefits against AD and associated cognitive impairments using diverse source of polyphenols: either in the form of whole fruit or fruit products such as blueberry, grape juice, pomegranate juice (Krikorian et al., 2010; Krikorian et al., 2012; Bookheimer et al., 2013; Krikorian et al., 2022), or in the form of extracts such as curcumin, grape seed polyphenol extract (GSPE), or pure synthetic material such as resveratrol (Baum et al., 2008; Kennedy et al., 2010; Patel et al., 2011; Mecocci and Polidori, 2012; Ringman et al., 2012; Turner et al., 2015; Moussa et al., 2017). The historical interest in dietary polyphenols is attributed to their high abundance in general food supplies and their antioxidant properties (Scalbert et al., 2005). However, current research of the benefits of dietary polyphenols is largely focused on their interaction and modulation of metabolic pathways regulating inflammation (Maleki et al., 2019; Ansari et al., 2020), endothelial function (Patel et al., 2018; Li et al., 2019; Parsamanesh et al., 2021), fatty acids, amino acids and carbohydrates metabolism (Hanhineva et al., 2010; Wang S et al., 2014; Naveed et al., 2018; Rothenberg et al., 2018; Różańska and Regulska-Ilow, 2018). Collectively, polyphenols’ ability to block free radicals’ activity, repair DNA damage, modulate the gene expression involved in metabolism, and act as signaling molecules to promote antioxidant defense support the development of dietary polyphenols in AD and other diseases (Azqueta and Collins, 2016; Hussain et al., 2016; Jiang, 2019; Maleki et al., 2019; Prasanth et al., 2019; Xing et al., 2019; Ohishi et al., 2021; Shen et al., 2022).
In this review, we summarize the major molecular mechanisms that correlate the health benefits of dietary polyphenols in AD physiopathology, focusing on the potential effects of these polyphenols to protect against Tau- and Aβ-mediated pathogenesis, oxidative stress, inflammation, synapse loss and memory deterioration.
Polyphenols are a class of organic compounds characterized by the presence of more than one phenol structural unit (several hydroxyl groups on aromatic rings). These phytochemicals have a protective role in plants involving in defense against ultraviolet radiation or pathogens invasion. They are mainly found in plant-based food diet (Manach et al., 2004; Maraldi et al., 2014). The number of phenol rings in their molecular structure will define the chemical subclass they belong to. More than 8,000 naturally occurring polyphenols exist and can be grouped in 4 chemical subclasses: flavonoids, phenolic acids, stilbenes, and lignans (Manach et al., 2004; Maraldi et al., 2014).
Flavonoids are the largest and most widespread groups of plant-derived secondary metabolites, with a 15-carbon skeleton, that have been described to exert beneficial effects in the prevention of neurodegenerative diseases (Dai et al., 2006; Kuriyama et al., 2006). Their highly reactive hydroxyl group is largely responsible for their ability to scavenge free radicals and/or chelate metal ions (Kumar et al., 2013; Kumar and Pandey, 2013). Flavonoids can be subdivided into different subgroups: Flavonols, with quercetin and kaempferaol as the representative compounds, are found in all types of food, with higher quantity in onions, broccoli, kale, blueberries and red wine (Herrmann, 1976). In comparison, flavones (luteolin and apigenin) are much less present in fruits and vegetables. Isoflavones are phytoestrogens mainly found in legume such as soya (Coward et al., 1993; Reinli and Block, 1996). Catechin and epicatechin are the basic units of flavanols and they form various oligomers and polymers through C4-C8 or C4-C6 interflavan bonds. Flavanols are found in many types of fruit and in red wine, however green tea and chocolate are the richest sources (Lakenbrink et al., 2000). Anthocyanins (cyanidin, malvidin, etc.) and chalocone (phloretin, arbutin, etc.) are mostly abundant in fruits and vegetables.
Phenolic acids are the most abundant group of bioactive compounds present in almost all plants (Rashmi and Negi, 2020). Phenolic acids are hydroxyl derivatives of benzoic and cinnamic acid. Hydroxybenzoic acid is found in very low quantity in plants. Hydroxycinnamic acids mainly consist of coumaric, sinapic, caffeic and ferulic acids. The richest dietary source of hydroxycinnamic acids are cherries, apples, berries and kiwi (Fleuriet et al., 1990). Caffeic acid is the most abundant hydroxycinnamic acid in many fruits and ferulic acid is mostly found in grains (Krzysztof et al., 1982; Rouau et al., 1997).
Lignans are mostly found in plant seeds and are precursors to phytoestrogens. They are a class of secondary plant metabolites. There is a growing interest in lignans in recent years due to their strong bioactivities in antioxidation, anti-inflammation and neuroprotection (Saleem et al., 2005; Teponno et al., 2016). The richest dietary source of lignans are linseeds (Thompson et al., 1991).
Stilbenes are poorly present in the human diet. The most known and studied is resveratrol, for which anticarcinogenic effects have been shown in medicinal plants screening. Resveratrol is found in wine at a very low quantity (Bertelli et al., 1998; Bhat and Pezzuto, 2002; Vitrac et al., 2005).
Senile plaques are mainly composed of β-Amyloid protein (Aβ; Masters et al., 1985) that results from proteolysis of the amyloid precursor protein (APP) by the enzymes β-secretase (BACE) and γ-secretase through amyloidogenic pathway. The non-amyloidogenic process is initiated by α-secretase rather than BACE leading to the formation of soluble APPα and C-terminus fragments and preventing Aβ generation. Both enzymes compete in APP proteolysis and their activities strongly affect Aβ production.
Several studies have demonstrated that polyphenols can modulate Aβ production by either increasing α-secretase activity or inhibiting BACE. (−)-Epicatechin, epigallocatechin, epigallocatechin-3-gallate (EGCG) and curcumin are potent inhibitors of amyloidogenic processing (Wang X et al., 2014; Cox et al., 2015; Guo et al., 2017). In vitro experiments conducted in neuronal cell line expressing human APP showed that treatment with EGCG significantly decreased Aβ production (Rezai-Zadeh et al., 2005). These results have been reproduced in vivo, where intraperitoneal injection of epigallocatechin-3-gallate in Tg2576 mouse model of AD decreased Aβ levels and favored the non-amyloidogenic α-secretase mediated pathway (Rezai-Zadeh et al., 2005). Another study demonstrated that curcumin treatment increases α-secretase activity (Narasingappa et al., 2012). Curcuminoids and epigallocatechin-3-gallate treatment inhibit BACE activity in neuronal cells (Wang X et al., 2014). While EGCG alone (Cheng et al., 2012) failed to abolish BACE activity in vivo, in combination with ferulic acid (FA), a BACE modulator (Mori et al., 2013), epigallocatechin-3-gallate could block BACE activity in APP/PS1 AD mice and reduced amyloidosis and improved cognitive function (Mori et al., 2019). The flavones apigenin (Zhao et al., 2013) and nobiletin (Nakajima et al., 2015) were also shown to significantly reduce soluble and insoluble Aβ as well Aβ deposits in the brain in AD mice (Onozuka et al., 2008). Similarly, chronic administration of the flavone baicalein decreases Aβ production (Zhang et al., 2013). Quercetin (3,5,7,3′,4′-pentahydroxyflavone) is a dietary flavonol widely distributed in plants, fruits and vegetables, and it is also effective at modulating contents of soluble and insoluble Aβ in the brain (Sabogal-Guaqueta et al., 2015; Moreno et al., 2017). Altogether, these results show that select polyphenols can modulate α-secretase or BACE activities and reduce Aβ production both in vitro and in vivo, however, there has been very few research on mechanisms of action and how select polyphenols promote non-amyloidogenic or inhibit amyloidogenic processing of APP.
Aβ monomers can assemble into soluble and insoluble Aβ oligomers. Insoluble forms of Aβ mostly deposit into extracellular plaques, while the soluble oligomers are now considered the most toxic species in driving Aβ-mediated synaptic toxicity and neuronal death (Kumar et al., 2013). Polyphenols have been shown to prevent Aβ oligomerization or to remodel Aβ oligomers into nontoxic forms. Ehrnhoefer et al. showed that EGCG inhibits Aβ fibrillogenesis leading to unstructured Aβ oligomers. It promotes the assembly of newly formed oligomers into smaller and amorphous nontoxic protein aggregates (Ehrnhoefer et al., 2008). Another group showed (−)-epigallocatechin-3-gallate could bind to preformed fibrils or large oligomers and remodel them into less toxic assemblies (Bieschke et al., 2010). Curcumin can substantially block Aβ oligomerization in a dose dependent manner (Yang et al., 2005; Reinke and Gestwicki, 2007) and it is able to inhibit fibril formation and destabilize preexisting fibrils (Doytchinova et al., 2020). Resveratrol does not prevent Aβ oligomerization, however it can reduce Aβ cytotoxicity by remodeling the oligomers into nontoxic forms (Feng et al., 2009; Ladiwala et al., 2010; Fu et al., 2014). Wang et al., demonstrated that moderate red wine consumption could reduce Aβ aggregation, and improved cognitive function when administered to AD mice (Wang et al., 2006). The same group investigated the specific compounds responsible for Aβ-lowering activity and demonstrated that dietary supplementation with grape seed polyphenolic extract (GSPE), largely composed of catechin and epicatechin monomer, oligomer and polymer, significantly attenuated the development of AD-type Aβ-related cognitive deterioration (Wang et al., 2008). Further investigation revealed that GSPE is a potent inhibitor for the oligomerization of Aβ peptides. These observations demonstrate that by modulating or remodeling of Aβ oligomers, polyphenols can interfere with the formation of soluble toxic forms of Aβ that are responsible for AD-associated neuronal damages.
Polyphenols can also reduce Aβ pathology by enhancing Aβ clearance. For example, resveratrol was shown to facilitate Aβ clearance in vitro (Marambaud et al., 2005; Vingtdeux et al., 2010) but the mechanism remains poorly understood. Several hypothesis have been proposed, for example resveratrol may promote intracellular degradation of Aβ via mechanism that involves the autophage and lysosome (Marambaud et al., 2005). Resveratrol may also stimulate the brain insulin-degrading enzyme activity (Rege et al., 2015) which in return will degrade Aβ thereby facilitating Aβ clearance.
Aberrant aggregation of microtubule-associated protein Tau is another contributor to AD pathology. Tau phosphorylation regulates its ability to bind microtubules. Hyper phosphorylated Tau forms paired helical filaments (PHF) and neurofibrillary tangle (NTF) inclusions that not only alter the cytoskeletal and associated transport system, but also affect cellular signaling and mitochondrial function (Johnson et al., 2016; Bejanin et al., 2017; Kametani and Hasegawa, 2018).
Tau phosphorylation in neuronal cells is regulated by the balance of the dephosphorylation catalyzed mainly by phosphatase 2A (PP2A; Gong et al., 1993) and phosphorylation catalyzed by cdk5, GSK-3β, PKA and other kinases (Medina et al., 2011; Cavallini et al., 2013). Select polyphenols can modulate Tau hyperphosphorylation and subsequent NFTs formation through inhibiting AD-tau kinases or promoting PP2A. Resveratrol has been shown to inhibit the hyperphosphorylation of Tau (He et al., 2016); additionally, in the senescence accelerated mice P8 (SAMP8), resveratrol inhibits Ser396 Tau phosphorylation by GSK-3β (Porquet et al., 2013). Resveratrol can also modulate Tau hyperphosphorylation by increasing PP2A activity, which leads to Tau dephosphorylation (Schweiger et al., 2017). Similarly, in an okaidic acid-injection model for AD, curcumin treatment inhibited Tau hyperphosphorylation through activation of GSK-3β pathway (Wang et al., 2019). Oral GSPE supplementation is also effective in significantly modulating Tau-mediated pathogenic phenotypes, including Tau hyperphosphorylation, misfolding into fibrillar polymers and subsequently aggregation into AD-type NFT in various tauopathy mouse models (Ho et al., 2009; Wang et al., 2010; Ksiezak-Reding et al., 2012; Santa-Maria et al., 2012). GSPE is largely composed of proanthocyanidin (PAC) catechin and epicatechin in monomeric, oligomeic and polymeric forms. Bioavailability studies conducted in rats by Ferruzzi et al. (2009) demonstrated that methylated and glucuronidated catechin and epicatechin can be found in the plasm following oral administration of GSPE. Moreover, they also reported that following single oral dosing, these polyphenol metabolites were not found in the brain, however, following repeated dosing, these metabolites could be detected in the brain (Ferruzzi et al., 2009). Similar studies were conducted in AD mouse showing that catechin and epicatechin metabolites can only be found in the brain of the mouse fed with monomeric fraction of the GSPE, but not the polymeric fraction of the GSPE. Moreover, they reported that only the monomeric fraction were effective in reducing amyloid neuropathology and improving cognitive function in AD mice (Wang et al., 2012).
Biophysical studies demonstrated that polyphenols may structurally change Tau protein and prevent its self-association. For example, in the presence of arachidonic acid, Tau self-assembles into β-sheet containing filaments, but in the presence of curcumin, arachidonic acid-mediated filament formation is abolished (Rane et al., 2017). Similarly, epicatechin-3-gallate, myricetin(Taniguchi et al., 2005) and rosmarinic acid could also inhibit Tau β-sheet formation (Cornejo et al., 2017).
The brains of AD patients show significant oxidative stress-associated damage including protein oxidation, lipid peroxidation, DNA damage suggesting the imbalance of free radical generation and antioxidant activity in the brain (Christen, 2000). In AD brain, the main sources of oxidative stress are from the free radicals generated from mitochondria and redox-active metals. Lipid peroxidation occurs when these oxidants attack polyunsaturated fatty acids (Ramana et al., 2014). Free radical-induced lipid peroxidation is widespread in AD brain. Reactive oxygen species (ROS) can also attack amino acid side chains or the protein backbones and generate protein carbonyl derivatives (Butterfield and Stadtman, 1997). Protein carbonyl content was found to be significantly increased in the hippocampus and inferior parietal lobule in AD subjects comparing to normal controls (Hensley et al., 1995; Aksenov et al., 2001). ROS-induced oxidation of key enzymes or structural proteins can significantly impair their cellular function leading to neurodegeneration and cell death (Hensley et al., 1995; Butterfield and Stadtman, 1997; Aksenov et al., 2001). ROS can also leading to base alteration, single and double strand breaks or DNA-protein crosslinkings (Sohal and Weindruch, 1996; DNA Oxidation in Alzheimer's Disease, 2006).
The therapeutic efficacy of flavonoids is historically attributed to their antioxidant potency and natural free radical scavenging properties (Mercer et al., 2005). Chronic administration of nobiletin to AD mice for 2–3 months significantly reduced brain ROS (Nakajima et al., 2015) and other oxidative stress markers (Nakajima et al., 2013). Quercetin has been shown to be strongly effective at scavenging free radicals and preventing oxidant-induced apoptosis (Rice-Evans et al., 1995; Heijnen et al., 2002; Choi et al., 2003). In addition to its high oxygen radical scavenging properties, quercetin also has the ability to inhibit lipid peroxidation (Fiorani et al., 2010) and to chelate iron and other metal ions that could be detrimental to the brain (Rice-Evans et al., 1995; Salganik, 2001). Chronic administration of the flavone apigenin to an AD mouse model induced a significant decrease of oxidative stress accompanied by increased superoxide dismutase and glutathione peroxidase activities (Rice-Evans et al., 1995; Fiorani et al., 2010; Zhao et al., 2013). Curcumin is another strong antioxidant and can effectively stabilize ROS (Basnet and Skalko-Basnet, 2011). It acts on the inner membrane of mitochondria, facilitating their depolarization, thus preventing the formation of ROS (Zhu et al., 2004). It was also shown to stop the free radical proliferation when administered intravenously to rodent (Jiang et al., 2007). Resveratrol can increase the superoxide dismutase enzyme activity hence reduce ROS formation in vivo (Chen et al., 2016). In vivo studies have shown that catechin and epicatechin can prevent ROS formation and lipid peroxidation in AD models. A single oral dose of epicatechin could effectively prevent Aβ-mediated lipid peroxidation and ROS formation in hippocampal formation in rats (Cuevas et al., 2009). Blueberry is rich in anthocyanins and proanthocyanidins. Blueberry extract was shown to be able to increase redox buffer glutathione and protect amyloid toxicity through inhibition of MAP kinase and CREB-mediated ROS signaling (Brewer et al., 2010). In humans, it was shown that blueberry supplementation protects against cognitive decline in people with high risk for developing dementia (Krikorian et al., 2022).
There is increasing consensus that immunological perturbations are major contributors to AD pathogenesis. This is supported by the genome-wide association studies linking myeloid cell-specific genes, such as TYROBP, TREM2 and CD33, with late-onset AD (LOAD). The phenomenon called neuroinflammation is a critical factor in AD pathogenesis. While the exact role of inflammation in AD remains to be investigated, it has been suggested that acute and systemic inflammation, manifested by microgliosis and astrogliosis, can accelerate AD progression and worsen cognitive impairments (Holmes et al., 2009).
Many dietary polyphenols have demonstrated their anti-inflammatory activities both in vitro and in vivo. Among these, curcumin and resveratrol are the most studied molecules for their potential application in AD treatment. Curcumin was shown to be able to block NF-kappa B action and associated inflammation cascade (Singh and Aggarwal, 1995; Hackler et al., 2016). In addition, curcumin also has the ability to inhibit Aβ-induced pro-inflammatory cytokines and chemokines release (Sundaram et al., 2017). Resveratrol attenuates Aβ-mediated microglia inflammation through inhibition of the TLR4/NF-κB and/or NLRP3 and STAT signaling pathway (Capiralla et al., 2012; Feng and Zhang, 2019). Resveratrol can also activate SIRT1 both in vitro and in vivo (Herskovits and Guarente, 2014; Favero et al., 2018). SIRT1 is a histone deacetylase that can epigenetically reprogram inflammation. In animal models, resveratrol treatment improved spatial memory, reduced neuroinflammation and increased neurotrophins in the brain of AD mice (Gong et al., 2010; Sun et al., 2019; Broderick et al., 2020). In humans, resveratrol was shown to modulate neuroinflammation and induce adaptive immunity in patients with AD (Moussa et al., 2017). Subjects with mild–moderate AD treated with synthetic resveratrol showed significant decrease of MMP9 in the cerebral spinal fluid (CSF). MMP9 is a protein that interferes with the blood brain barrier (BBB) function. The decrease of CSF MMP9 in AD suggest that resveratrol may mitigate inflammatory responses in the brain by reducing the permeability of CNS and lower infiltration of leukocytes and other inflammatory agents into the brain. Other polyphenols such as flavonoids fisetin, quercetin and luteolin were also shown to decrease inflammation in different AD mouse models and reduce astrogliosis and microgliosis (Sharma et al., 2007; Currais et al., 2014; Currais et al., 2018). Blueberry and its extract have also been shown to be able to inhibit amyloid-mediated microglia activation through attenuation of p44/42 MAPK signaling both in vitro and in vivo mouse model (Joseph et al., 2003; Zhu et al., 2008).
Selective polyphenols also showed promising effects in rescuing cognitive function in transgenic AD mouse models. For example, old 5xFAD mice chronically treated with 7,8-dihydroxyflavone (7,8-DHF) reduced synapse loss in the brain and performed better at the working memory Y maze test (Devi and Ohno, 2012; Zhang et al., 2014). Oral administration of apigenin led to increased activation of ERK/CREB signaling and learning and memory improvement in 2xFAD mice (Zhao et al., 2013). Learning and memory were improved in both the 1 × FAD and 3xFAD mouse models of AD following treatment with nobiletin (Onozuka et al., 2008; Nakajima et al., 2015). Mice treated with fisetin showed increased activation of ERK/MAPK signaling, increased expression of synaptic proteins and improved cognitive function (Currais et al., 2014; Ahmad et al., 2017; Currais et al., 2018). Flavonoid rutin, a quercetin molecule with the addition of disaccharide rutinose, was also found to be effective in improving cognitive function through increased expression of brain derived neurotrophic factor (BDNF) in rats injected with Aβ (Moghbelinejad et al., 2014). Green tea contains high levels of EGCG can prevent the loss of synaptic proteins and cognitive impairments in a 1xFAD mouse model (Walker et al., 2015). On the same note, anthocyanins were also found to be able to reduce synaptic protein loss and improve memory function (Ali et al., 2017; Kim et al., 2017). Anthocyanin from grape juice was shown to rescue oligomeric Aβ-induced long term potentiation (LTP) deficit in hippocampal slices (Wang et al., 2014a). Wang et al. explored the effect of cocoa flavanols on AD pathogenesis (Wang et al., 2014b). In their study they showed that catechin and epicatechin enriched cocoa extracts interfered with Aβ oligomerization and prevented synaptic deficits. They demonstrated that application of cocoa extracts on mice hippocampal slices could prevent Aβ-induced LTP deficit (Wang et al., 2014b). Cocoa was also shown to prevent Aβ oligomer-induced neurite dystrophy by activating BDNF in neuronal cultures (Cimini et al., 2013). These observations have also been corroborated by clinical studies demonstrating that cocoa flavanols enhance the dentate gyrus function and reduces cognitive decline in humans (Crews et al., 2008; Desideri et al., 2012; Scholey and Owen, 2013; Brickman et al., 2014). Blueberry was shown to improve memory function in APP/PS1 AD mice through increase of ERK signaling and neural sphingomyelin-specific phospholipase C activity (Joseph et al., 2003).
The biological activity of dietary polyphenols largely depends on the bioavailability of the bioactive forms of the parent compounds in the target organs. Once ingested, polyphenols are absorbed and metabolized first in the gastrointestinal tract and then are further modified in the liver through glucuronidation, sulfonation, or methylation, before entering the blood stream. The biological activities of their metabolites can be very different from the parent compounds. For example, Serra et al. explored this relationship between dietary polyphenols and gut metabolism using an anthocyanin-rich extract obtained from Portuguese blueberries and a simulated gastrointestinal digestion process. Both the digested and non-digested extracts displayed different chemical compositions and had different effects on neuroinflammation (Serra et al., 2020). Gut microbiome is receiving increased attention due to their potential role in health and disease (Durack and Lynch, 2019). Recent studies have demonstrated strong links between polyphenol metabolism and the gut microbiome (Hervert-Hernández and Goñi, 2011; Fraga et al., 2019). The gut microbiota can influence the process and metabolism of polyphenols, which may influence the production and diversity of polyphenol metabolites; Polyphenols have the ability to influence the intestinal environment, which allows them to modulate the composition of gut microbiome (De Bruyne et al., 2019). Moreover, there is also bidirectional communications between gut microbiota and the central nervous system, the so-called gut-brain axis and currently, the gut-brain axis is one of the favorable targets for therapeutic treatment of neurodegenerative disorders including AD due to their bidirectional interactions that may affect brain function (Carabotti et al., 2015; Reddy et al., 2020). Curcumin, for example, exhibits beneficial effects against AD despite having limited blood–brain barrier penetration. It is postulated that curcumin becomes a more effective neuroprotective agent after undergoing metabolism by gut microbial and its interaction with the gut-brain axis also allows it to react indirectly with the CNS and exerts its neuroprotective activity (Di Meo et al., 2019; Reddy et al., 2020).
Pathological mechanisms involved in Alzheimer’s pathogenesis include both Aβ and Tau toxicity. Therapeutic strategies aiming at targeting one or the other continue to fail in clinical trials. Polyphenols offer a new approach that can simultaneously target Aβ, Tau, neuroinflammation and oxidative stress, which could lead to better outcomes.
Dietary factors and diet composition can play a critical role in AD prevention. Our review lists few of the numerous polyphenols, considered as confirmed or promising therapeutic candidates due to their potent anti-inflammatory, antioxidant properties and AD-disease modifying activities (Table 1). AD is a multifactorial disease and the current lackluster performance of clinical studies is, in part, due to the prevailing approach targeting individual pathogenic mechanisms. Most of the polyphenolic compounds have exhibited pleiotropic bioactivities (Table 1) which may have advantages over conventional pharmaceutical drugs for the treatment of AD. In spite of their multi-targeting features, clinical development of polyphenols for AD is hampered by their poor absorption and limited brain bioavailability. Moreover, most of the available polyphenol metabolite forms, following digestive and hepatic activity, may not have the same biological activity as the native compound. Therefore, the in vitro biological activities of “parental” polyphenol forms may not relevant to biological activities in vivo, which is the ultimate arbitrator of therapeutic benefit. Future advancement of polyphenols in AD prevention and/or treatment will largely rely on the development of select polyphenols or their derivatives with better brain bioavailability while preserving their multi-targeting bioactivities.
FE, FC, LH, and JuW wrote, edited, and reviewed the manuscript. H-YL, CY, and JeW reviewed and edited the manuscript. All authors contributed to the article and approved the submitted version.
Funding was provided by the P50 AT008661-01 from the National Center for complementary and Integrative Health (NCCIH) and the Office of Dietary Supplements (ODS). In addition, JuW holds a position in the Research and Development unit of the Basic and Biomedical Research and Training Program at the James J. Peters Veterans Affairs Medical Center.
The authors declare that the research was conducted in the absence of any commercial or financial relationships that could be construed as a potential conflict of interest.
All claims expressed in this article are solely those of the authors and do not necessarily represent those of their affiliated organizations, or those of the publisher, the editors and the reviewers. Any product that may be evaluated in this article, or claim that may be made by its manufacturer, is not guaranteed or endorsed by the publisher.
Ahmad, A., Ali, T., Park, H. Y., Badshah, H., Rehman, S. U., and Kim, M. O. (2017). Neuroprotective effect of Fisetin against amyloid-Beta-induced cognitive/synaptic dysfunction, Neuroinflammation, and Neurodegeneration in adult mice. Mol. Neurobiol. 54, 2269–2285. doi: 10.1007/s12035-016-9795-4
Aksenov, M. Y., Aksenova, M. V., Butterfield, D. A., Geddes, J. W., and Markesbery, W. R. (2001). Protein oxidation in the brain in Alzheimer's disease. Neuroscience 103, 373–383. doi: 10.1016/S0306-4522(00)00580-7
Ali, T., Kim, M. J., Rehman, S. U., Ahmad, A., and Kim, M. O. (2017). Anthocyanin-loaded PEG-gold nanoparticles enhanced the Neuroprotection of Anthocyanins in an Abeta 1-42 mouse model of Alzheimer's disease. Mol. Neurobiol. 54, 6490–6506. doi: 10.1007/s12035-016-0136-4
Ansari, M. Y., Ahmad, N., and Haqqi, T. M. (2020). Oxidative stress and inflammation in osteoarthritis pathogenesis: role of polyphenols. Biomed. Pharmacother. 129:110452. doi: 10.1016/j.biopha.2020.110452
Armstrong, A. R. (2019). Risk factors for Alzheimer's disease. Folia Neuropathol. 57, 87–105. doi: 10.5114/fn.2019.85929
Azqueta, A., and Collins, A. (2016). Polyphenols and DNA damage: a mixed blessing. Nutrients 8. doi: 10.3390/nu8120785
Basnet, P., and Skalko-Basnet, N. (2011). Curcumin: an anti-inflammatory molecule from a curry spice on the path to cancer treatment. Molecules 16, 4567–4598. doi: 10.3390/molecules16064567
Baum, L., Lam, C. W. K., Cheung, S. K.-K., Kwok, T., Lui, V., Tsoh, J., et al. (2008). Six-month randomized, placebo-controlled, double-blind, pilot clinical trial of Curcumin in patients with Alzheimer disease. J. Clin. Psychopharmacol. 28, 110–113. doi: 10.1097/jcp.0b013e318160862c
Bejanin, A., Schonhaut, D. R., La Joie, R., Kramer, J. H., Baker, S. L., Sosa, N., et al. (2017). Tau pathology and neurodegeneration contribute to cognitive impairment in Alzheimer's disease. Brain 140, 3286–3300. doi: 10.1093/brain/awx243
Bertelli, A., Bertelli, A. A., Gozzini, A., and Giovannini, L. (1998). Plasma and tissue resveratrol concentrations and pharmacological activity. Drugs Exp. Clin. Res. 24, 133–138.
Bhat, K. P., and Pezzuto, J. M. (2002). Cancer chemopreventive activity of resveratrol. Ann. N. Y. Acad. Sci. 957, 210–229. doi: 10.1111/j.1749-6632.2002.tb02918.x
Bieschke, J., Russ, J., Friedrich, R. P., Ehrnhoefer, D. E., Wobst, H., Neugebauer, K., et al. (2010). EGCG remodels mature alpha-synuclein and amyloid-beta fibrils and reduces cellular toxicity. Proc. Natl. Acad. Sci. U. S. A. 107, 7710–7715. doi: 10.1073/pnas.0910723107
Bookheimer, S. Y., Renner, B. A., Ekstrom, A., Li, Z., Henning, S. M., Brown, J. A., et al. (2013). Pomegranate juice augments memory and FMRI activity in middle-aged and older adults with mild memory complaints. Evid. Based Complement. Alternat. Med. 2013:946298. doi: 10.1155/2013/946298
Brewer, G. J., Torricelli, J. R., Lindsey, A. L., Kunz, E. Z., Neuman, A., Fisher, D. R., et al. (2010). Age-related toxicity of amyloid-beta associated with increased pERK and pCREB in primary hippocampal neurons: reversal by blueberry extract. J. Nutr. Biochem. 21, 991–998. doi: 10.1016/j.jnutbio.2009.08.005
Brickman, A. M., Khan, U. A., Provenzano, F. A., Yeung, L. K., Suzuki, W., Schroeter, H., et al. (2014). Enhancing dentate gyrus function with dietary flavanols improves cognition in older adults. Nat. Neurosci. 17, 1798–1803. doi: 10.1038/nn.3850
Broderick, T. L., Rasool, S., Li, R., Zhang, Y., Anderson, M., Al-Nakkash, L., et al. (2020). Neuroprotective effects of chronic resveratrol treatment and exercise training in the 3xTg-AD mouse model of Alzheimer’s disease. Int. J. Mol. Sci. 21:7337. doi: 10.3390/ijms21197337
Butterfield, D. A., and Stadtman, E. R. (1997). “Chapter 7 protein oxidation processes in aging brain,” in Advances in cell aging and gerontology. eds. P. S. Timiras and E. E. Bittar (Elsevier), 161–191.
Capiralla, H., Vingtdeux, V., Zhao, H., Sankowski, R., Al-Abed, Y., Davies, P., et al. (2012). Resveratrol mitigates lipopolysaccharide- and Aβ-mediated microglial inflammation by inhibiting the TLR4/NF-κB/STAT signaling cascade. J. Neurochem. 120, 461–472. doi: 10.1111/j.1471-4159.2011.07594.x
Carabotti, M., Scirocco, A., Maselli, M. A., and Severi, C. (2015). The gut-brain axis: interactions between enteric microbiota, central and enteric nervous systems. Ann. Gastroenterol. 28, 203–209.
Cavallini, A., Brewerton, S., Bell, A., Sargent, S., Glover, S., Hardy, C., et al. (2013). An unbiased approach to identifying tau kinases that phosphorylate tau at sites associated with Alzheimer disease. J. Biol. Chem. 288, 23331–23347. doi: 10.1074/jbc.M113.463984
Chen, W. M., Shaw, L. H., Chang, P. J., Tung, S. Y., Chang, T. S., Shen, C. H., et al. (2016). Hepatoprotective effect of resveratrol against ethanol-induced oxidative stress through induction of superoxide dismutase in vivo and in vitro. Exp. Ther. Med. 11, 1231–1238. doi: 10.3892/etm.2016.3077
Cheng, X. R., Zhou, J. W., Zhou, Y., Cheng, J. P., Yang, R. F., Zhou, W. X., et al. (2012). The green tea polyphenol (2)-epigallocatechin-3-gallate (EGCG) is not a beta-secretase inhibitor. Bioorg. Med. Chem. Lett. 22, 1408–1414. doi: 10.1016/j.bmcl.2011.12.034
Choi, Y. J., Kang, J. S., Park, J. H., Lee, Y. J., Choi, J. S., and Kang, Y. H. (2003). Polyphenolic flavonoids differ in their antiapoptotic efficacy in hydrogen peroxide-treated human vascular endothelial cells. J. Nutr. 133, 985–991. doi: 10.1093/jn/133.4.985
Christen, Y. (2000). Oxidative stress and Alzheimer disease. Am. J. Clin. Nutr. 71, 621S–629S. doi: 10.1093/ajcn/71.2.621s
Cimini, A., Gentile, R., D'Angelo, B., Benedetti, E., Cristiano, L., Avantaggiati, M. L., et al. (2013). Cocoa powder triggers neuroprotective and preventive effects in a human Alzheimer's disease model by modulating BDNF signaling pathway. J. Cell. Biochem. 114, 2209–2220. doi: 10.1002/jcb.24548
Cornejo, A., Aguilar Sandoval, F., Caballero, L., Machuca, L., Munoz, P., Caballero, J., et al. (2017). Rosmarinic acid prevents fibrillization and diminishes vibrational modes associated to beta sheet in tau protein linked to Alzheimer's disease. J. Enzyme Inhib. Med. Chem. 32, 945–953. doi: 10.1080/14756366.2017.1347783
Coward, L., Barnes, N. C., Setchell, K. D. R., and Barnes, S. (1993). Genistein, daidzein, and their. Beta.-glycoside conjugates: antitumor isoflavones in soybean foods from American and Asian diets. J. Agric. Food Chem. 41, 1961–1967. doi: 10.1021/jf00035a027
Cox, C. J., Choudhry, F., Peacey, E., Perkinton, M. S., Richardson, J. C., Howlett, D. R., et al. (2015). Dietary (−)-epicatechin as a potent inhibitor of βγ-secretase amyloid precursor protein processing. Neurobiol. Aging 36, 178–187. doi: 10.1016/j.neurobiolaging.2014.07.032
Crews, W. D. Jr., Harrison, D. W., and Wright, J. W. (2008). A double-blind, placebo-controlled, randomized trial of the effects of dark chocolate and cocoa on variables associated with neuropsychological functioning and cardiovascular health: clinical findings from a sample of healthy, cognitively intact older adults. Am. J. Clin. Nutr. 87, 872–880.
Cuevas, E., Limon, D., Perez-Severiano, F., Diaz, A., Ortega, L., Zenteno, E., et al. (2009). Antioxidant effects of epicatechin on the hippocampal toxicity caused by amyloid-beta 25-35 in rats. Eur. J. Pharmacol. 616, 122–127. doi: 10.1016/j.ejphar.2009.06.013
Currais, A., Farrokhi, C., Dargusch, R., Armando, A., Quehenberger, O., Schubert, D., et al. (2018). Fisetin reduces the impact of aging on behavior and physiology in the rapidly aging SAMP8 mouse. J. Gerontol. A Biol. Sci. Med. Sci. 73, 299–307. doi: 10.1093/gerona/glx104
Currais, A., Prior, M., Dargusch, R., Armando, A., Ehren, J., Schubert, D., et al. (2014). Modulation of p 25 and inflammatory pathways by fisetin maintains cognitive function in Alzheimer's disease transgenic mice. Aging Cell 13, 379–390. doi: 10.1111/acel.12185
Dai, Q., Borenstein, A. R., Wu, Y., Jackson, J. C., and Larson, E. B. (2006). Fruit and vegetable juices and Alzheimer's disease: the kame project. Am. J. Med. 119, 751–759. doi: 10.1016/j.amjmed.2006.03.045
De Bruyne, T., Steenput, B., Roth, L., De Meyer, G. R. Y., Santos, C. N. D., Valentova, K., et al. (2019). Dietary polyphenols targeting arterial stiffness: interplay of contributing mechanisms and gut microbiome-related metabolism. Nutrients 11. doi: 10.3390/nu11030578
Desideri, G., Kwik-Uribe, C., Grassi, D., Necozione, S., Ghiadoni, L., Mastroiacovo, D., et al. (2012). Benefits in cognitive function, blood pressure, and insulin resistance through cocoa flavanol consumption in elderly subjects with mild cognitive impairment: the cocoa, cognition, and aging (CoCoA) study. Hypertension 60, 794–801. doi: 10.1161/HYPERTENSIONAHA.112.193060
Devi, L., and Ohno, M. (2012). 7, 8-dihydroxyflavone, a small-molecule Trk B agonist, reverses memory deficits and BACE1 elevation in a mouse model of Alzheimer's disease. Neuropsychopharmacology 37, 434–444. doi: 10.1038/npp.2011.191
Di Meo, F., Margarucci, S., Galderisi, U., Crispi, S., and Peluso, G. (2019). Curcumin, Gut Microbiota, and Neuroprotection. Nutrients 11. doi: 10.3390/nu11102426
DNA Oxidation in Alzheimer's Disease (2006). Antioxid. Redox Signal. 8, 2039–2045. doi: 10.1089/ars.2006.8.2039
Dominguez, L. J., Veronese, N., Vernuccio, L., Catanese, G., Inzerillo, F., Salemi, G., et al. (2021). Nutrition, physical activity, and other lifestyle factors in the prevention of cognitive decline and dementia. Nutrients 13. doi: 10.3390/nu13114080
Doytchinova, I., Atanasova, M., Salamanova, E., Ivanov, S., and Dimitrov, I. (2020). Curcumin inhibits the primary nucleation of amyloid-Beta peptide: a molecular dynamics study. Biomol. Ther. 10. doi: 10.3390/biom10091323
Durack, J., and Lynch, S. V. (2019). The gut microbiome: relationships with disease and opportunities for therapy. J. Exp. Med. 216, 20–40. doi: 10.1084/jem.20180448
Ehrnhoefer, D. E., Bieschke, J., Boeddrich, A., Herbst, M., Masino, L., Lurz, R., et al. (2008). EGCG redirects amyloidogenic polypeptides into unstructured, off-pathway oligomers. Nat. Struct. Mol. Biol. 15, 558–566. doi: 10.1038/nsmb.1437
Favero, G., Stacchiotti, A., and Rezzani, R. (2018). Resveratrol and SIRT1 activators for the treatment of aging and age-related diseases, resveratrol-adding life to years, not adding years to life. Intech Open.
Feng, Y., Wang, X. P., Yang, S. G., Wang, Y. J., Zhang, X., Du, X. T., et al. (2009). Resveratrol inhibits beta-amyloid oligomeric cytotoxicity but does not prevent oligomer formation. Neurotoxicology 30, 986–995. doi: 10.1016/j.neuro.2009.08.013
Feng, L., and Zhang, L. (2019). Resveratrol suppresses Aβ-induced microglial activation through the TXNIP/TRX/NLRP3 signaling pathway. DNA Cell Biol. 38, 874–879. doi: 10.1089/dna.2018.4308
Ferruzzi, M. G., Lobo, J. K., Janle, E. M., Cooper, B., Simon, J. E., Wu, Q. L., et al. (2009). Bioavailability of gallic acid and catechins from grape seed polyphenol extract is improved by repeated dosing in rats: implications for treatment in Alzheimer's disease. J. Alzheimer's Dis. 18, 113–124. doi: 10.3233/JAD-2009-1135
Fiorani, M., Guidarelli, A., Blasa, M., Azzolini, C., Candiracci, M., Piatti, E., et al. (2010). Mitochondria accumulate large amounts of quercetin: prevention of mitochondrial damage and release upon oxidation of the extramitochondrial fraction of the flavonoid. J. Nutr. Biochem. 21, 397–404. doi: 10.1016/j.jnutbio.2009.01.014
Fraga, C. G., Croft, K. D., Kennedy, D. O., and Tomás-Barberán, F. A. (2019). The effects of polyphenols and other bioactives on human health. Food Funct. 10, 514–528. doi: 10.1039/C8FO01997E
Fu, Z., Aucoin, D., Ahmed, M., Ziliox, M., Van Nostrand, W. E., and Smith, S. O. (2014). Capping of abeta 42 oligomers by small molecule inhibitors. Biochemistry 53, 7893–7903. doi: 10.1021/bi500910b
Gong, Q.-H., Li, F., Jin, F., and Shi, J.-S. (2010). Resveratrol attenuates neuroinflammation-mediated cognitive deficits in rats. J. Health Sci. 56, 655–663. doi: 10.1248/jhs.56.655
Gong, C. X., Singh, T. J., Grundke-Iqbal, I., and Iqbal, K. (1993). Phosphoprotein phosphatase activities in Alzheimer disease brain. J. Neurochem. 61, 921–927. doi: 10.1111/j.1471-4159.1993.tb03603.x
Guasch-Ferré, M., and Willett, W. C. (2021). The Mediterranean diet and health: a comprehensive overview. J. Intern. Med. 290, 549–566. doi: 10.1111/joim.13333
Guo, Y., Zhao, Y., Nan, Y., Wang, X., Chen, Y., and Wang, S. (2017). (−)-Epigallocatechin-3-gallate ameliorates memory impairment and rescues the abnormal synaptic protein levels in the frontal cortex and hippocampus in a mouse model of Alzheimer's disease. Neuroreport 28, 590–597. doi: 10.1097/WNR.0000000000000803
Hackler, L. Jr., Ozsvari, B., Gyuris, M., Sipos, P., Fabian, G., Molnar, E., et al. (2016). The Curcumin analog C-150, influencing NF-kappa B, UPR and Akt/notch pathways has potent anticancer activity in vitro and in vivo. PLoS One 11:e0149832. doi: 10.1371/journal.pone.0149832
Hanhineva, K., Törrönen, R., Bondia-Pons, I., Pekkinen, J., Kolehmainen, M., Mykkänen, H., et al. (2010). Impact of dietary polyphenols on carbohydrate metabolism. Int. J. Mol. Sci. 11, 1365–1402. doi: 10.3390/ijms11041365
He, X., Li, Z., Rizak, J. D., Wu, S., Wang, Z., He, R., et al. (2016). Resveratrol attenuates formaldehyde induced hyperphosphorylation of tau protein and cytotoxicity in N2a cells. Front. Neurosci. 10:598. doi: 10.3389/fnins.2016.00598
Heijnen, C. G., Haenen, G. R., Oostveen, R. M., Stalpers, E. M., and Bast, A. (2002). Protection of flavonoids against lipid peroxidation: the structure activity relationship revisited. Free Radic. Res. 36, 575–581. doi: 10.1080/10715760290025951
Hensley, K., Hall, N., Subramaniam, R., Cole, P., Harris, M., Aksenov, M., et al. (1995). Brain regional correspondence between Alzheimer's disease histopathology and biomarkers of protein oxidation. J. Neurochem. 65, 2146–2156. doi: 10.1046/j.1471-4159.1995.65052146.x
Herrmann, K. (1976). Flavonols and flavones in food plants: a review. Int. J. Food Sci. Technol. 11, 433–448. doi: 10.1111/j.1365-2621.1976.tb00743.x
Herskovits, A. Z., and Guarente, L. (2014). SIRT1 in neurodevelopment and brain senescence. Neuron 81, 471–483. doi: 10.1016/j.neuron.2014.01.028
Hervert-Hernández, D., and Goñi, I. (2011). Dietary polyphenols and human gut microbiota: a review. Food Rev. Int. 27, 154–169.
Ho, L., Yemul, S., Wang, J., and Pasinetti, G. M. (2009). Grape seed polyphenolic extract as a potential novel therapeutic agent in tauopathies. J. Alzheimers Dis. 16, 433–439. doi: 10.3233/JAD-2009-0969
Holmes, C., Cunningham, C., Zotova, E., Woolford, J., Dean, C., Kerr, S., et al. (2009). Systemic inflammation and disease progression in Alzheimer disease. Neurology 73, 768–774. doi: 10.1212/WNL.0b013e3181b6bb95
Hussain, T., Tan, B., Yin, Y., Blachier, F., Tossou, M. C., and Rahu, N. (2016). Oxidative stress and inflammation: what polyphenols can do for us? Oxidative Med. Cell. Longev. 2016:7432797. doi: 10.1155/2016/7432797
Jiang, T. A. (2019). Health benefits of culinary herbs and spices. J. AOAC Int. 102, 395–411. doi: 10.5740/jaoacint.18-0418
Jiang, J., Wang, W., Sun, Y. J., Hu, M., Li, F., and Zhu, D. Y. (2007). Neuroprotective effect of curcumin on focal cerebral ischemic rats by preventing blood-brain barrier damage. Eur. J. Pharmacol. 561, 54–62. doi: 10.1016/j.ejphar.2006.12.028
Johnson, K. A., Schultz, A., Betensky, R. A., Becker, J. A., Sepulcre, J., Rentz, D., et al. (2016). Tau positron emission tomographic imaging in aging and early Alzheimer disease. Ann. Neurol. 79, 110–119. doi: 10.1002/ana.24546
Joseph, J. A., Arendash, G., Gordon, M., Diamond, D., Shukitt-Hale, B., Morgan, D., et al. (2003). Blueberry supplementation enhances signaling and prevents behavioral deficits in an Alzheimer disease model. Nutr. Neurosci. 6, 153–162. doi: 10.1080/1028415031000111282
Kametani, F., and Hasegawa, M. (2018). Reconsideration of amyloid hypothesis and tau hypothesis in Alzheimer's disease. Front. Neurosci. 12:25. doi: 10.3389/fnins.2018.00025
Kennedy, D. O., Wightman, E. L., Reay, J. L., Lietz, G., Okello, E. J., Wilde, A., et al. (2010). Effects of resveratrol on cerebral blood flow variables and cognitive performance in humans: a double-blind, placebo-controlled, crossover investigation. Am. J. Clin. Nutr. 91, 1590–1597. doi: 10.3945/ajcn.2009.28641
Kim, M. J., Rehman, S. U., Amin, F. U., and Kim, M. O. (2017). Enhanced neuroprotection of anthocyanin-loaded PEG-gold nanoparticles against Abeta 1-42-induced neuroinflammation and neurodegeneration via the NF-KB/JNK/GSK3beta signaling pathway. Nanomedicine 13, 2533–2544. doi: 10.1016/j.nano.2017.06.022
Krikorian, R., Boespflug, E. L., Fleck, D. E., Stein, A. L., Wightman, J. D., Shidler, M. D., et al. (2012). Concord grape juice supplementation and neurocognitive function in human aging. J. Agric. Food Chem. 60, 5736–5742. doi: 10.1021/jf300277g
Krikorian, R., Nash, T. A., Shidler, M. D., Shukitt-Hale, B., and Joseph, J. A. (2010). Concord grape juice supplementation improves memory function in older adults with mild cognitive impairment. Br. J. Nutr. 103, 730–734. doi: 10.1017/S0007114509992364
Krikorian, R., Skelton, M. R., Summer, S. S., Shidler, M. D., and Sullivan, P. G. (2022). Blueberry supplementation in midlife for dementia risk reduction. Nutrients 14:1619. doi: 10.3390/nu14081619
Krzysztof, K., Sosulski, F., and Hogge, L. (1982). Free, esterified, and insoluble-bound phenolic acids. 3. Composition of phenolic acids in cereal and potato flours. J. Agric. Food Chem. 30, 337–340. doi: 10.1021/jf00110a030
Ksiezak-Reding, H., Ho, L., Santa-Maria, I., Diaz-Ruiz, C., Wang, J., and Pasinetti, G. M. (2012). Ultrastructural alterations of Alzheimer's disease paired helical filaments by grape seed-derived polyphenols. Neurobiol. Aging 33, 1427–1439. doi: 10.1016/j.neurobiolaging.2010.11.006
Kumar, S., Mishra, A., and Pandey, A. K. (2013). Antioxidant mediated protective effect of Parthenium hysterophorus against oxidative damage using in vitro models. BMC Complement. Altern. Med. 13:120. doi: 10.1186/1472-6882-13-120
Kumar, S., and Pandey, A. K. (2013). Phenolic content, reducing power and membrane protective activities of Solanum xanthocarpum root extracts. Vegetos 26, 301–307. doi: 10.5958/j.2229-4473.26.1.043
Kuriyama, S., Hozawa, A., Ohmori, K., Shimazu, T., Matsui, T., Ebihara, S., et al. (2006). Green tea consumption and cognitive function: a cross-sectional study from the Tsurugaya project 1. Am. J. Clin. Nutr. 83, 355–361. doi: 10.1093/ajcn/83.2.355
Ladiwala, A. R., Lin, J. C., Bale, S. S., Marcelino-Cruz, A. M., Bhattacharya, M., Dordick, J. S., et al. (2010). Resveratrol selectively remodels soluble oligomers and fibrils of amyloid Abeta into off-pathway conformers. J. Biol. Chem. 285, 24228–24237. doi: 10.1074/jbc.M110.133108
Lakenbrink, C., Lapczynski, S., Maiwald, B., and Engelhardt, U. H. (2000). Flavonoids and other polyphenols in consumer brews of tea and other caffeinated beverages. J. Agric. Food Chem. 48, 2848–2852. doi: 10.1021/jf9908042
Li, H., Xia, N., Hasselwander, S., and Daiber, A. (2019). Resveratrol and vascular function. Int. J. Mol. Sci. 20. doi: 10.3390/ijms20246252
Maleki, S. J., Crespo, J. F., and Cabanillas, B. (2019). Anti-inflammatory effects of flavonoids. Food Chem. 299:125124. doi: 10.1016/j.foodchem.2019.125124
Manach, C., Scalbert, A., Morand, C., Remesy, C., and Jimenez, L. (2004). Polyphenols: food sources and bioavailability. Am. J. Clin. Nutr. 79, 727–747. doi: 10.1093/ajcn/79.5.727
Maraldi, T., Vauzour, D., and Angeloni, C. (2014). Dietary polyphenols and their effects on cell biochemistry and pathophysiology 2013. Oxidative Med. Cell. Longev. 2014:576363. doi: 10.1155/2014/576363
Marambaud, P., Zhao, H., and Davies, P. (2005). Resveratrol promotes clearance of Alzheimer's disease amyloid-beta peptides. J. Biol. Chem. 280, 37377–37382. doi: 10.1074/jbc.M508246200
Masters, C. L., Multhaup, G., Simms, G., Pottgiesser, J., Martins, R. N., and Beyreuther, K. (1985). Neuronal origin of a cerebral amyloid: neurofibrillary tangles of Alzheimer's disease contain the same protein as the amyloid of plaque cores and blood vessels. EMBO J. 4, 2757–2763. doi: 10.1002/j.1460-2075.1985.tb04000.x
Mecocci, P., and Polidori, M. C. (2012). Antioxidant clinical trials in mild cognitive impairment and Alzheimer's disease. Biochim. Biophys. Acta - Mol. Basis Dis. 1822, 631–638. doi: 10.1016/j.bbadis.2011.10.006
Medina, M., Garrido, J. J., and Wandosell, F. G. (2011). Modulation of GSK-3 as a therapeutic strategy on tau pathologies. Front. Mol. Neurosci. 4:24. doi: 10.3389/fnmol.2011.00024
Mercer, L. D., Kelly, B. L., Horne, M. K., and Beart, P. M. (2005). Dietary polyphenols protect dopamine neurons from oxidative insults and apoptosis: investigations in primary rat mesencephalic cultures. Biochem. Pharmacol. 69, 339–345. doi: 10.1016/j.bcp.2004.09.018
Moghbelinejad, S., Nassiri-Asl, M., Farivar, T. N., Abbasi, E., Sheikhi, M., Taghiloo, M., et al. (2014). Rutin activates the MAPK pathway and BDNF gene expression on beta-amyloid induced neurotoxicity in rats. Toxicol. Lett. 224, 108–113. doi: 10.1016/j.toxlet.2013.10.010
Moreno, L., Puerta, E., Suarez-Santiago, J. E., Santos-Magalhaes, N. S., Ramirez, M. J., and Irache, J. M. (2017). Effect of the oral administration of nanoencapsulated quercetin on a mouse model of Alzheimer's disease. Int. J. Pharm. 517, 50–57. doi: 10.1016/j.ijpharm.2016.11.061
Mori, T., Koyama, N., Guillot-Sestier, M. V., Tan, J., and Town, T. (2013). Ferulic acid is a nutraceutical beta-secretase modulator that improves behavioral impairment and alzheimer-like pathology in transgenic mice. PLoS One 8:e55774. doi: 10.1371/journal.pone.0055774
Mori, T., Koyama, N., Tan, J., Segawa, T., Maeda, M., and Town, T. (2019). Combined treatment with the phenolics (−)-epigallocatechin-3-gallate and ferulic acid improves cognition and reduces Alzheimer-like pathology in mice. J. Biol. Chem. 294, 2714–2731. doi: 10.1074/jbc.RA118.004280
Moussa, C., Hebron, M., Huang, X., Ahn, J., Rissman, R. A., Aisen, P. S., et al. (2017). Resveratrol regulates neuro-inflammation and induces adaptive immunity in Alzheimer’s disease. J. Neuroinflammation 14:1. doi: 10.1186/s12974-016-0779-0
Nakajima, A., Aoyama, Y., Nguyen, T. T., Shin, E. J., Kim, H. C., Yamada, S., et al. (2013). Nobiletin, a citrus flavonoid, ameliorates cognitive impairment, oxidative burden, and hyperphosphorylation of tau in senescence-accelerated mouse. Behav. Brain Res. 250, 351–360. doi: 10.1016/j.bbr.2013.05.025
Nakajima, A., Aoyama, Y., Shin, E. J., Nam, Y., Kim, H. C., Nagai, T., et al. (2015). Nobiletin, a citrus flavonoid, improves cognitive impairment and reduces soluble Abeta levels in a triple transgenic mouse model of Alzheimer's disease (3XTg-AD). Behav. Brain Res. 289, 69–77. doi: 10.1016/j.bbr.2015.04.028
Narasingappa, R. B., Javagal, M. R., Pullabhatla, S., Htoo, H. H., Rao, J. K., Hernandez, J. F., et al. (2012). Activation of alpha-secretase by curcumin-aminoacid conjugates. Biochem. Biophys. Res. Commun. 424, 691–696. doi: 10.1016/j.bbrc.2012.07.010
Naveed, M., Hejazi, V., Abbas, M., Kamboh, A. A., Khan, G. J., Shumzaid, M., et al. (2018). Chlorogenic acid (CGA): a pharmacological review and call for further research. Biomed. Pharmacother. 97, 67–74. doi: 10.1016/j.biopha.2017.10.064
Ohishi, T., Fukutomi, R., Shoji, Y., Goto, S., and Isemura, M. (2021). The beneficial effects of principal polyphenols from green tea, coffee, wine, and curry on obesity. Molecules 26. doi: 10.3390/molecules26020453
Onozuka, H., Nakajima, A., Matsuzaki, K., Shin, R. W., Ogino, K., Saigusa, D., et al. (2008). Nobiletin, a citrus flavonoid, improves memory impairment and Abeta pathology in a transgenic mouse model of Alzheimer's disease. J. Pharmacol. Exp. Ther. 326, 739–744. doi: 10.1124/jpet.108.140293
Parsamanesh, N., Asghari, A., Sardari, S., Tasbandi, A., Jamialahmadi, T., Xu, S., et al. (2021). Resveratrol and endothelial function: a literature review. Pharmacol. Res. 170:105725. doi: 10.1016/j.phrs.2021.105725
Patel, R. V., Mistry, B. M., Shinde, S. K., Syed, R., Singh, V., and Shin, H. S. (2018). Therapeutic potential of quercetin as a cardiovascular agent. Eur. J. Med. Chem. 155, 889–904. doi: 10.1016/j.ejmech.2018.06.053
Patel, K. R., Scott, E., Brown, V. A., Gescher, A. J., Steward, W. P., and Brown, K. (2011). Clinical trials of resveratrol. Ann. N. Y. Acad. Sci. 1215, 161–169. doi: 10.1111/j.1749-6632.2010.05853.x
Porquet, D., Casadesus, G., Bayod, S., Vicente, A., Canudas, A. M., Vilaplana, J., et al. (2013). Dietary resveratrol prevents Alzheimer's markers and increases life span in SAMP8. Age (Dordr.) 35, 1851–1865. doi: 10.1007/s11357-012-9489-4
Prasanth, M. I., Sivamaruthi, B. S., Chaiyasut, C., and Tencomnao, T. (2019). A review of the role of green tea (Camellia sinensis) in Antiphotoaging, stress resistance, Neuroprotection, and autophagy. Nutrients 11. doi: 10.3390/nu11020474
Ramana, K. V., Srivastava, S., and Singhal, S. S. (2014). Lipid peroxidation products in human health and disease 2014. Oxidative Med. Cell. Longev. 2014:162414. doi: 10.1155/2014/162414
Rane, J. S., Bhaumik, P., and Panda, D. (2017). Curcumin inhibits tau aggregation and disintegrates preformed tau filaments in vitro. J. Alzheimers Dis. 60, 999–1014. doi: 10.3233/JAD-170351
Rashmi, H. B., and Negi, P. S. (2020). Phenolic acids from vegetables: a review on processing stability and health benefits. Food Res. Int. 136:109298. doi: 10.1016/j.foodres.2020.109298
Reddy, V. P., Aryal, P., Robinson, S., Rafiu, R., Obrenovich, M., and Perry, G. (2020). Polyphenols in Alzheimer's disease and in the gut-brain Axis. Microorganisms 8. doi: 10.3390/microorganisms8020199
Rege, S. D., Geetha, T., Broderick, T. L., and Babu, J. R. (2015). Resveratrol protects beta amyloid-induced oxidative damage and memory associated proteins in H19-7 hippocampal neuronal cells. Curr. Alzheimer Res. 12, 147–156. doi: 10.2174/1567205012666150204130009
Reinke, A. A., and Gestwicki, J. E. (2007). Structure-activity relationships of amyloid beta-aggregation inhibitors based on curcumin: influence of linker length and flexibility. Chem. Biol. Drug Des. 70, 206–215. doi: 10.1111/j.1747-0285.2007.00557.x
Reinli, K., and Block, G. (1996). Phytoestrogen content of foods--a compendium of literature values. Nutr. Cancer 26, 123–148. doi: 10.1080/01635589609514470
Rezai-Zadeh, K., Shytle, D., Sun, N., Mori, T., Hou, H., Jeanniton, D., et al. (2005). Green tea epigallocatechin-3-gallate (EGCG) modulates amyloid precursor protein cleavage and reduces cerebral amyloidosis in Alzheimer transgenic mice. J. Neurosci. 25, 8807–8814. doi: 10.1523/JNEUROSCI.1521-05.2005
Rice-Evans, C. A., Miller, N. J., Bolwell, P. G., Bramley, P. M., and Pridham, J. B. (1995). The relative antioxidant activities of plant-derived polyphenolic flavonoids. Free Radic. Res. 22, 375–383.
Riedel, B. C., Thompson, P. M., and Brinton, R. D. (2016). Age, APOE and sex: triad of risk of Alzheimer's disease. J. Steroid Biochem. Mol. Biol. 160, 134–147. doi: 10.1016/j.jsbmb.2016.03.012
Ringman, J. M., Frautschy, S. A., Teng, E., Begum, A. N., Bardens, J., Beigi, M., et al. (2012). Oral curcumin for Alzheimer's disease: tolerability and efficacy in a 24-week randomized, double blind, placebo-controlled study. Alzheimers Res. Ther. 4:43. doi: 10.1186/alzrt146
Rothenberg, D. O., Zhou, C., and Zhang, L. (2018). A review on the weight-loss effects of oxidized tea polyphenols. Molecules 23. doi: 10.3390/molecules23051176
Rouau, X., Lempereur, I., and Abecassis, J. (1997). Genetic and agronomic variation in arabinoxylan and ferulic acid contents of durum wheat (Triticum durum L.) grain and its milling fractions. J. Cereal Sci., 103–110.
Różańska, D., and Regulska-Ilow, B. (2018). The significance of anthocyanins in the prevention and treatment of type 2 diabetes. Adv. Clin. Exp. Med. 27, 135–142. doi: 10.17219/acem/64983
Sabogal-Guaqueta, A. M., Munoz-Manco, J. I., Ramirez-Pineda, J. R., Lamprea-Rodriguez, M., Osorio, E., and Cardona-Gomez, G. P. (2015). The flavonoid quercetin ameliorates Alzheimer's disease pathology and protects cognitive and emotional function in aged triple transgenic Alzheimer's disease model mice. Neuropharmacology 93, 134–145. doi: 10.1016/j.neuropharm.2015.01.027
Saleem, M., Kim, H. J., Ali, M. S., and Lee, Y. S. (2005). An update on bioactive plant lignans. Nat. Prod. Rep. 22, 696–716. doi: 10.1039/b514045p
Salganik, R. I. (2001). The benefits and hazards of antioxidants: controlling apoptosis and other protective mechanisms in cancer patients and the human population. J. Am. Coll. Nutr. 20:464S-472S; discussion 473S-475S. doi: 10.1080/07315724.2001.10719185
Santa-Maria, I., Diaz-Ruiz, C., Ksiezak-Reding, H., Chen, A., Ho, L., Wang, J., et al. (2012). GSPE interferes with tau aggregation in vivo: implication for treating tauopathy. Neurobiol. Aging 33, 2072–2081. doi: 10.1016/j.neurobiolaging.2011.09.027
Scalbert, A., Manach, C., Morand, C., Remesy, C., and Jimenez, L. (2005). Dietary polyphenols and the prevention of diseases. Crit. Rev. Food Sci. Nutr. 45, 287–306. doi: 10.1080/1040869059096
Scholey, A., and Owen, L. (2013). Effects of chocolate on cognitive function and mood: a systematic review. Nutr. Rev. 71, 665–681. doi: 10.1111/nure.12065
Schweiger, S., Matthes, F., Posey, K., Kickstein, E., Weber, S., Hettich, M. M., et al. (2017). Resveratrol induces dephosphorylation of tau by interfering with the MID1-PP2A complex. Sci. Rep. 7:13753. doi: 10.1038/s41598-017-12974-4
Serra, D., Henriques, J. F., Serra, T., Bento Silva, A., Bronze, M. R., Dinis, T. C. P., et al. (2020). An anthocyanin-rich extract obtained from Portuguese blueberries maintains its efficacy in reducing microglia-driven Neuroinflammation after simulated digestion. Nutrients 12. doi: 10.3390/nu12123670
Sharma, V., Mishra, M., Ghosh, S., Tewari, R., Basu, A., Seth, P., et al. (2007). Modulation of interleukin-1beta mediated inflammatory response in human astrocytes by flavonoids: implications in neuroprotection. Brain Res. Bull. 73, 55–63. doi: 10.1016/j.brainresbull.2007.01.016
Shen, N., Wang, T., Gan, Q., Liu, S., Wang, L., and Jin, B. (2022). Plant flavonoids: classification, distribution, biosynthesis, and antioxidant activity. Food Chem. 383:132531. doi: 10.1016/j.foodchem.2022.132531
Singh, S., and Aggarwal, B. B. (1995). Activation of transcription factor NF-kappa B is suppressed by curcumin (diferuloylmethane) [corrected]. J. Biol. Chem. 270, 24995–25000. doi: 10.1074/jbc.270.42.24995
Sohal, R. S., and Weindruch, R. (1996). Oxidative stress, caloric restriction, and aging. Science 273, 59–63. doi: 10.1126/science.273.5271.59
Sun, X.-Y., Dong, Q.-X., Zhu, J., Sun, X., Zhang, L.-F., Qiu, M., et al. (2019). Resveratrol rescues tau-induced cognitive deficits and neuropathology in a mouse model of tauopathy. Curr. Alzheimer Res. 16, 710–722. doi: 10.2174/1567205016666190801153751
Sundaram, J. R., Poore, C. P., Sulaimee, N. H. B., Pareek, T., Cheong, W. F., Wenk, M. R., et al. (2017). Curcumin ameliorates Neuroinflammation, Neurodegeneration, and memory deficits in p 25 transgenic mouse model that bears hallmarks of Alzheimer's disease. J/ Alzheimer's Dis. 60, 1429–1442. doi: 10.3233/JAD-170093
Taniguchi, S., Suzuki, N., Masuda, M., Hisanaga, S., Iwatsubo, T., Goedert, M., et al. (2005). Inhibition of heparin-induced tau filament formation by phenothiazines, polyphenols, and porphyrins. J. Biol. Chem. 280, 7614–7623. doi: 10.1074/jbc.M408714200
Teponno, R. B., Kusari, S., and Spiteller, M. (2016). Recent advances in research on lignans and neolignans. Nat. Prod. Rep. 33, 1044–1092. doi: 10.1039/C6NP00021E
Thompson, L. U., Robb, P., Serraino, M., and Cheung, F. (1991). Mammalian lignan production from various foods. Nutr. Cancer 16, 43–52. doi: 10.1080/01635589109514139
Turner, R. S., Thomas, R. G., Craft, S., van Dyck, C. H., Mintzer, J., Reynolds, B. A., et al. (2015). A randomized, double-blind, placebo-controlled trial of resveratrol for Alzheimer disease. Neurology 85, 1383–1391. doi: 10.1212/WNL.0000000000002035
Vingtdeux, V., Giliberto, L., Zhao, H., Chandakkar, P., Wu, Q., Simon, J. E., et al. (2010). AMP-activated protein kinase signaling activation by resveratrol modulates amyloid-beta peptide metabolism. J. Biol. Chem. 285, 9100–9113. doi: 10.1074/jbc.M109.060061
Vitrac, X., Bornet, A., Vanderlinde, R., Valls, J., Richard, T., Delaunay, J. C., et al. (2005). Determination of stilbenes (delta-viniferin, trans-astringin, trans-piceid, cis- and trans-resveratrol, epsilon-viniferin) in Brazilian wines. J. Agric. Food Chem. 53, 5664–5669. doi: 10.1021/jf050122g
Walker, J. M., Klakotskaia, D., Ajit, D., Weisman, G. A., Wood, W. G., Sun, G. Y., et al. (2015). Beneficial effects of dietary EGCG and voluntary exercise on behavior in an Alzheimer's disease mouse model. J. Alzheimers Dis. 44, 561–572. doi: 10.3233/JAD-140981
Wang, J., Bi, W., Cheng, A., Freire, D., Vempati, P., Zhao, W., et al. (2014a). Targeting multiple pathogenic mechanisms with polyphenols for the treatment of Alzheimer's disease-experimental approach and therapeutic implications. Front. Aging Neurosci. 6:42. doi: 10.3389/fnagi.2014.00042
Wang, J., Ferruzzi, M. G., Ho, L., Blount, J., Janle, E. M., Gong, B., et al. (2012). Brain-targeted proanthocyanidin metabolites for Alzheimer's disease treatment. J. Neurosci. 32, 5144–5150. doi: 10.1523/JNEUROSCI.6437-11.2012
Wang, J., Ho, L., Zhao, W., Ono, K., Rosensweig, C., Chen, L., et al. (2008). Grape-derived polyphenolics prevent Abeta oligomerization and attenuate cognitive deterioration in a mouse model of Alzheimer's disease. J. Neurosci. 28, 6388–6392. doi: 10.1523/JNEUROSCI.0364-08.2008
Wang, J., Ho, L., Zhao, Z., Seror, I., Humala, N., Dickstein, D. L., et al. (2006). Moderate consumption of cabernet sauvignon attenuates Abeta neuropathology in a mouse model of Alzheimer's disease. FASEB J. 20, 2313–2320. doi: 10.1096/fj.06-6281com
Wang, X., Kim, J. R., Lee, S. B., Kim, Y. J., Jung, M. Y., Kwon, H. W., et al. (2014). Effects of curcuminoids identified in rhizomes of Curcuma longa on BACE-1 inhibitory and behavioral activity and lifespan of Alzheimer's disease drosophila models. BMC Complement. Altern. Med. 14:88. doi: 10.1186/1472-6882-14-88
Wang, S., Moustaid-Moussa, N., Chen, L., Mo, H., Shastri, A., Su, R., et al. (2014). Novel insights of dietary polyphenols and obesity. J. Nutr. Biochem. 25, 1–18. doi: 10.1016/j.jnutbio.2013.09.001
Wang, J., Santa-Maria, I., Ho, L., Ksiezak-Reding, H., Ono, K., Teplow, D. B., et al. (2010). Grape derived polyphenols attenuate tau neuropathology in a mouse model of Alzheimer's disease. J. Alzheimers Dis. 22, 653–661. doi: 10.3233/JAD-2010-101074
Wang, H., Sui, H., Zheng, Y., Jiang, Y., Shi, Y., Liang, J., et al. (2019). Curcumin-primed exosomes potently ameliorate cognitive function in AD mice by inhibiting hyperphosphorylation of the tau protein through the AKT/GSK-3beta pathway. Nanoscale 11, 7481–7496. doi: 10.1039/C9NR01255A
Wang, J., Varghese, M., Ono, K., Yamada, M., Levine, S., Tzavaras, N., et al. (2014b). Cocoa extracts reduce oligomerization of amyloid-beta: implications for cognitive improvement in Alzheimer's disease. J. Alzheimers Dis. 41, 643–650. doi: 10.3233/JAD-132231
Xing, L., Zhang, H., Qi, R., Tsao, R., and Mine, Y. (2019). Recent advances in the understanding of the health benefits and molecular mechanisms associated with green tea polyphenols. J. Agric. Food Chem. 67, 1029–1043. doi: 10.1021/acs.jafc.8b06146
Xu, W., Tan, L., Wang, H. F., Jiang, T., Tan, M. S., Tan, L., et al. (2015). Meta-analysis of modifiable risk factors for Alzheimer's disease. J. Neurol. Neurosurg. Psychiatry 86, 1299–1306. doi: 10.1136/jnnp-2015-310548
Yang, F., Lim, G. P., Begum, A. N., Ubeda, O. J., Simmons, M. R., Ambegaokar, S. S., et al. (2005). Curcumin inhibits formation of amyloid beta oligomers and fibrils, binds plaques, and reduces amyloid in vivo. J. Biol. Chem. 280, 5892–5901. doi: 10.1074/jbc.M404751200
Zhang, Z., Liu, X., Schroeder, J. P., Chan, C. B., Song, M., Yu, S. P., et al. (2014). 7, 8-dihydroxyflavone prevents synaptic loss and memory deficits in a mouse model of Alzheimer's disease. Neuropsychopharmacology 39, 638–650. doi: 10.1038/npp.2013.243
Zhang, S. Q., Obregon, D., Ehrhart, J., Deng, J., Tian, J., Hou, H., et al. (2013). Baicalein reduces beta-amyloid and promotes nonamyloidogenic amyloid precursor protein processing in an Alzheimer's disease transgenic mouse model. J. Neurosci. Res. 91, 1239–1246. doi: 10.1002/jnr.23244
Zhang, X. X., Tian, Y., Wang, Z. T., Ma, Y. H., Tan, L., and Yu, J. T. (2021). The epidemiology of Alzheimer's disease modifiable risk factors and prevention. J. Prev Alzheimers Dis. 8, 313–321. doi: 10.14283/jpad.2021.15
Zhao, L., Wang, J. L., Liu, R., Li, X. X., Li, J. F., and Zhang, L. (2013). Neuroprotective, anti-amyloidogenic and neurotrophic effects of apigenin in an Alzheimer's disease mouse model. Molecules 18, 9949–9965. doi: 10.3390/molecules18089949
Zhu, Y., Bickford, P. C., Sanberg, P., Giunta, B., and Tan, J. (2008). Blueberry opposes beta-amyloid peptide-induced microglial activation via inhibition of p 44/42 mitogen-activation protein kinase. Rejuvenation Res. 11, 891–901. doi: 10.1089/rej.2008.0757
Keywords: polyphenol, Alzheimer, oxidative stress, neuroinflammation, synaptic plasticity
Citation: El Gaamouch F, Chen F, Ho L, Lin H-Y, Yuan C, Wong J and Wang J (2022) Benefits of dietary polyphenols in Alzheimer’s disease. Front. Aging Neurosci. 14:1019942. doi: 10.3389/fnagi.2022.1019942
Received: 15 August 2022; Accepted: 21 November 2022;
Published: 13 December 2022.
Edited by:
Yashi Mi, University of Arizona, United StatesReviewed by:
Shaowei Wang, University of Southern California, United StatesCopyright © 2022 El Gaamouch, Chen, Ho, Lin, Yuan, Wong and Wang. This is an open-access article distributed under the terms of the Creative Commons Attribution License (CC BY). The use, distribution or reproduction in other forums is permitted, provided the original author(s) and the copyright owner(s) are credited and that the original publication in this journal is cited, in accordance with accepted academic practice. No use, distribution or reproduction is permitted which does not comply with these terms.
*Correspondence: Jun Wang, anVuLndhbmdAbXNzbS5lZHU=
Disclaimer: All claims expressed in this article are solely those of the authors and do not necessarily represent those of their affiliated organizations, or those of the publisher, the editors and the reviewers. Any product that may be evaluated in this article or claim that may be made by its manufacturer is not guaranteed or endorsed by the publisher.
Research integrity at Frontiers
Learn more about the work of our research integrity team to safeguard the quality of each article we publish.