- 1College of Sport and Health, Shandong Sport University, Jinan, Shandong, China
- 2School of Kinesiology, Shanghai University of Sport, Shanghai, China
- 3School of Nursing and Rehabilitation, Cheeloo College of Medicine, Shandong University, Jinan, Shandong, China
Background: With aging, the cognitive function of the prefrontal cortex (PFC) declined, postural control weakened, and fall risk increased. As a mind–body exercise, regular Tai Chi practice could improve postural control and effectively prevent falls; however, underlying brain mechanisms remained unclear, which were shed light on by analyzing the effect of Tai Chi on the PFC in older adults by means of functional near-infrared spectroscopy (fNIRS).
Methods: 36 healthy older adults without Tai Chi experience were divided randomly into Tai Chi group and Control group. The experiment was conducted four times per week for 16 weeks; 27 participants remained and completed the experiment. Negotiating obstacle task (NOT) and negotiating obstacle with cognitive task (NOCT) were performed pre- and post-intervention, and Brodmann area 10 (BA10) was detected using fNIRS for hemodynamic response. A three-dimensional motion capture system measured walking speed.
Results: After intervention in the Tai Chi group under NOCT, the HbO2 concentration change value (ΔHbO2) in BA10 was significantly greater (right BA10: p = 0.002, left BA10: p = 0.001), walking speed was significantly faster (p = 0.040), and dual-task cost was significantly lower than pre-intervention (p = 0.047). ΔHbO2 in BA10 under NOCT was negatively correlated with dual-task cost (right BA10: r = −0.443, p = 0.021, left BA10: r = −0.448, p = 0.019). There were strong negative correlations between ΔHbO2 and ΔHbR under NOCT either pre-intervention (left PFC r = −0.841, p < 0.001; right PFC r = −0.795, p < 0.001) or post-intervention (left PFC r = −0.842, p < 0.001; right PFC r = −0.744, p < 0.001).
Conclusion: Tai Chi practice might increase the cognitive resources in older adults through the PFC bilateral activation to prioritize gait performance during negotiating obstacles under a dual-task condition.
Introduction
Cognitive function (Zhang et al., 2018; Chen et al., 2020; Demurtas et al., 2020; Jung et al., 2020; Yue et al., 2020a, b; Cheval et al., 2022) and postural control/stability (Wang et al., 2016; Zou et al., 2019a; Koohsari et al., 2020; Song et al., 2021; Veronese et al., 2021; Zhang et al., 2021; Cunha et al., 2022) are simultaneously declining with aging. In daily life, walking across obstacles and/or walking under dual-task conditions require greater cognitive function (Holtzer et al., 2014; Chen et al., 2017; Zhang et al., 2020; Lu et al., 2021) and fine postural control (Harley et al., 2009). Performing dual task or multi-task like talking over the phone while walking is regularly needed, but is very complex and challenging as it requires cognition-balance integration that are naturally degenerating among older adults (Camicioli et al., 1997; Lundin-Olsson et al., 1997; Hausdorff et al., 2008; Schwenk et al., 2010). The above-mentioned scenario seems to be supported by the capacity-sharing theory (Tombu and Jolicoeur, 2003; Leone et al., 2017; Kunstler et al., 2018), suggesting that single-task and/or dual-task is damaged if cognitive demand exceeds the total capacity of available resources. Failure in negotiating obstacles was reported to associate with the increased risk for age-related falls (Toba et al., 2005), which resulted in negative consequences including bruises, sprains, cerebral trauma, joint dislocation, fractures and even death (Konak et al., 2016).
Cognitive impairment has been identified as an important cause of falls in older adults (Sheridan and Hausdorff, 2007; Verghese et al., 2012), and executive function was closely related to falls, disability and death (Sheridan and Hausdorff, 2007; Kearney et al., 2012; Montero-Odasso et al., 2012; Yu et al., 2021). Executive function referred to the advanced cognitive control process for attaining a specific goal (Moriguchi and Hiraki, 2013). Dual-task and negotiating obstacle tasks (NOTs) were related to different aspects of executive function, i.e., negotiating obstacle related to motor planning and visuospatial ability, whereas dual-task related to attentional allocation and working memory (Galna et al., 2009; Herman et al., 2010; Beurskens and Bock, 2012; Beurskens et al., 2014). Executive function was vulnerable to special population like older adults (West, 1996; Raz et al., 1998; Buckner, 2004; Head et al., 2004; Hedden and Gabrieli, 2004; Gu et al., 2019; Zheng et al., 2021) and other mental or physical disabilities (Zou et al., 2018; Cai et al., 2020; Kong et al., 2022; Sun et al., 2022).
A strong link between walking and cognition, especially the high-order executive function, has been observed (Tinetti et al., 1988; Yogev-Seligmann et al., 2008; Pieruccini-Faria et al., 2019). Several imageology studies have indicated that the prefrontal cortex (PFC) was primarily responsible for executive function during walking and its associated brain network like frontoparietal network was activated (Garavan et al., 2002; Amboni et al., 2013; Holtzer et al., 2014). Of note, the frontal lobe was one of the most sensitive brain structures linked to aging (Hedden and Gabrieli, 2004)and its Brodmann area 10 (BA10) was reported to specifically associate with executive function (Semendeferi et al., 2001), such as memory and recall of information, problem-solving (Szczepanski and Knight, 2014), participation in memory extraction during task execution and complex reasoning tasks (Braver and Bongiolatti, 2002; Roca et al., 2011; Dong et al., 2019). Therefore, BA10 was chosen as the region of interest (ROI) in this study.
The portable functional near-infrared spectroscopy (fNIRS) is feasible in assessing functional brain activities during walking (Atsumori et al., 2010; Nieuwhof et al., 2016; Balardin et al., 2017; Pinti et al., 2018). FNIRS is an imaging technique to measure cortical hemodynamic response (Herold et al., 2018; Yu et al., 2021, 2022) and analyze local cortical activation based on the neurovascular coupling mechanism (Obrig and Villringer, 2003; Di Lorenzo et al., 2019). FNIRS is practically avoids motion artifacts, and is suitable for cortical activity assessment when older adults walk in a real-world environment.
As a mind–body exercise, Tai Chi has gained greatest popularity among aging population due to its associated health benefits (Zou et al., 2021) generating from long-term practice. Taking closer look, Tai Chi has great emphasis on weight shifting during dynamic walking and its eye-body (upper-limb and lower-limb) coordination, such unique features have provided trainees an opportunity to decelerate the progress of cognitive decline and strengthen muscle-related functions (Zou et al., 2019b; Chen et al., 2020). Tai Chi practice could improve postural control and reduce fall risk in older women (Sun et al., 2018, 2019). Song et al.’s finding indicated that Tai Chi practice improved physical stability during the performance of dual tasks in older adults (Song et al., 2018). In comparison with other exercise interventions, Tai Chi might be a superior strategy for fall reduction through benefitting the cognitive function (Nyman, 2021) and neuromuscular function improvements (Hu et al., 2021). Yuan Yang et al. randomly assigned 26 healthy older women to the Tai Chi group and Control group, with Tai Chi group performing Tai Chi exercise for 8 weeks and Control group engaging in daily activities, and after Tai Chi intervention, the Tai Chi group demonstrated elevated HbO2 concentration in the PFC (Yang et al., 2019). Long-term Tai Chi practice improved white matter and delayed the degeneration of brain tissues (Yao et al., 2019).
Regular Tai Chi practice can improve physical postural control ability (Li et al., 2012; Zhou et al., 2015) and cognitive function of older adults to reduce falls (Wu et al., 2013; Zou et al., 2019c). Many previous studies have investigated the effects of Tai Chi on postural control and cognitive function in older adults; however, the researches on the associated brain mechanisms are still scarce. To better understand the role of the PFC in complex walk task and the intervention effect from Tai Chi, we designed a randomized controlled trial with a 16-week Tai Chi intervention, and collected hemodynamic data of the PFC pre- and post-intervention by means of fNIRS. We hypothesized that older adults had higher PFC activation level and better gait performance during negotiating obstacle after Tai Chi practice.
Materials and methods
Participants
Participants were recruited from community-dwellers nearby Shandong Sport University. The inclusion criteria were 65–75 years old; a Mini-Mental State Examination score ≥ 24; without cardiovascular, respiratory, musculoskeletal or neurological diseases; able to live independently and perform daily activities; without any regular exercise in the last 2 years, including Tai Chi; and right-handedness. The exclusion criteria were visual impairment and the use of any medication affecting physical balance and nervous system, such as sleeping pills and tranquillizers, in the last 6 months.
The sample size estimation was performed on G*Power software (Germany) based on a randomized controlled trial (Yang et al., 2019) with a significant increase in HbO2 of the PFC during executive function assessment in older adults after Tai Chi intervention (Tai Chi group: 0.116 ± 0.143 mmol/l, Control group: −0.003 ± 0.071 mmol/l, p < 0.05). By setting significance level to 0.05 and statistical power to 0.85, the effect size and estimated required sample size were calculated to be 0.57 and 24, respectively.
Overall, thirty-six healthy older adults participated in this study and were randomly divided into two groups with 18 people in each group. Thirty-six different random numbers were assigned to all of the participants. Participants with numbers 1–18 were assigned to the Tai Chi group, and the rest were assigned to the Control group. Male participants were assigned to different groups after female participants were assigned to groups to create a similar gender ratio in each group to minimize gender bias. After group assignment, the age and cognitive performance were compared with independent-t tests to ensure no assignment bias. Twenty-seven participants accomplished the intervention, 12 in the Tai Chi group and 15 in the Control group (See details in Table 1). All methods were performed in accordance with the latest guidelines and regulations of the Declaration of Helsinki. This study was approved by the Ethics Review Committee of Shandong Sport University. All participants signed an informed consent form and were told what to do regarding the study.
Study protocol
Participants in the Tai Chi group practiced Tai Chi for 16 weeks (Kim et al., 2016) four times per week, and 60 min once. Each session was composed of 10 min warm-up, 45 min Tai Chi practice and 5 min cool-down. A certified Tai Chi instructor guided the participants to perform the Tai Chi movements. If the participation rate was less than 80%, the participant was excluded. The participants in the Control group received health lectures for 16 weeks, four times per week, and 60 min once. Both groups maintained their daily living habits.
An 8-form simplified version adapted from the 24-form version of Yang style-based Tai Chi was practiced in this study. The movements included Move a Ball, Part Wild Horse’s Mane, Single Whip, Wave Hands like Clouds, Repulse Monkey, Brush Knees, Fair Lady Works at Shuttle, and Grasp Peacock’s Tail (Li, 2014).
The experiment was conducted at the Sports Biomechanics Laboratory of Shandong Sport University. The participants performed the NOT and negotiating obstacle with the cognitive task (NOCT) at their preferred speed along a 6 m walkway. The obstacle consisted of two upright stands with an unfixed crossbar and was positioned in the midpoint of the walkway. If the participant touched the crossbar, it dropped out to prevent tripping over. The obstacle had the length of 60 cm, the width of 3 cm and the height set to 20% of the participant’s leg length (Zhang et al., 2011; Pan et al., 2016) which was determined by the distance between the anterior superior iliac spine and the medial ankle. The start position was adjusted to ensure comfortably negotiating the obstacle with a minimum of 2 steps before the crossing stride. Several practice trials were instructed to avoid contacting the crossbar and to reduce unnecessary movements contributing to optode shift.
Each test was performed at least three times, including a 30-s rest state and a 30-s walk state. During the rest state, the participant was instructed to sit quietly with eyes closed and stay relaxed. The cognitive task was serial subtraction by 3 from a random three-digit number, involving working memory (Maylor and Wing, 1996). Each participant sat and conducted the cognitive task three times (30 s once) to assess the executive function before the experiment. At the beginning, the researcher verbally provided a three-digit number, and the participant reported the calculation results until the task termination. The walk route was back and forth twice with negotiating obstacle four times in a straight line. The task order was random.
Apparatus and data acquisition
Walking speed
A three-dimensional motion capture system (Vicon, Oxford Metrics Ltd., Oxford, United Kingdom) with 12 infrared cameras (acquisition frequency 100 Hz) was used in this study. Thirty-nine infrared reflective balls (diameter 14 mm) were stuck on the participant’s key bone marker for gait data collection. These data were named, modelled, and intercepted by the data acquisition software (Vicon Nexus 1.7.1) and imported into the Visual 3D software (C-Motion, United States) for modeling, smoothing, and normalization. The fifth lumbar marker was selected as the reference point to calculate the average walking speed of one gait cycle during negotiating obstacle.
Brain hemodynamics
Using a portable fNIRS (LIGHTNIRS, Shimadzu Corp., Kyoto, Japan), the hemodynamic response was measured (BA10 as ROI). The fNIRS had 16 optodes (8 emitters and 8 detectors, sampling frequency13.3 Hz) with 22 channels, and laser diodes (wavelengths 780, 805, and 830 nm). The distance between the adjacent optods was 3 cm. Using a 3D Digitizer (FASTRAK, Polhemus, Vermont), the Montreal Neurological Institute coordinates of channels were determined (See Figure 1) and provided in the supplementary material. During the task test, participants carried the fNIRS in their backpacks while walking and wore a whole-head fiber holder with the standard head landmarks determined according to the international 10/10 system. The lowest row of optodes touched the eyebrows and the line between the nasion and inion passed through the center of channel 19 and 4. We checked each optode and separated the hair beneath it to ensure the probe fully contacted with the scalp. All the optodes were covered with a black cloth to avoid light contamination. The right BA10 included channels 2, 3, 4, 9, 10, and 11, and the left BA10 included channels 4, 5, 6, 12, 13, 14, and 22.
According to the modified Beer–Lambert law, blood oxygen concentrations were obtained, including oxyhemoglobin (HbO2), deoxyhemoglobin (HbR), and total hemoglobin (HbT). The visual inspection was performed for the raw hemodynamic signals and channels with a prominent bias or technical artifacts were interpolated by adjacent channels. The episodes irrelevant to the tasks were removed. By the MATLAB-based NIRS_SPM toolkit, the data were processed. The wavelet minimum description length algorithm was applied to remove artifacts caused by respiration, heartbeat, vasodilation, contraction, or other errors related to movement, and to eliminate linear drift. High-frequency noise was reduced/removed by a low-pass filter (cut-off frequency 0.15 Hz) based on the canonical hemodynamic response function (Hiura et al., 2018). The average blood oxygen concentrations within the last 5 s of rest state was used for baseline correction to obtain the change values (ΔHbO2, ΔHbR, ΔHbT). The blood oxygen concentration change values in three trials for each task were superimposing averaged to obtain time-series changes of all channels. The channel-wise average data were averaged across all channels in right BA10 and left BA10 respectively, subsequently across all participants. By the Correlation based signal improvement (CBSI) method (Cui et al., 2010), the ΔHbO2 and ΔHbR were corrected for the correlation analysis to assess the cortical activation.
Dual-task score under NOCT
Dual-task score was calculated to evaluate the cognitive performance according to the following equation:
Dual-task score = correct number/total number (correct number + wrong number)/duration (Mirelman et al., 2017).
Dual-task cost
We defined the dual-task cost on walking speed according to the following equation:
Dual-task cost = (single-task walking speed − dual-task walking speed)/single-task walking speed × 100 (Mori et al., 2018).
In general, bigger values indicated a more conservative gait pattern and greater interference by the cognitive tasks.
Statistical analysis
Statistical analysis was performed using the SPSS 19.0 software. We used the Shapiro–Wilk test to analyze the normality of the data and the Leven’s test to measure the homogeneity of variance. If in accordance with normal distribution, a two-way analysis of variance (ANOVA) with repeated measures was used, age as a covariate, group (Tai Chi group and Control group) as the between-subject factor, and time (pre-intervention and post-intervention) as the within-subject factor. To explore the correlation among PFC activity, walking speed, dual-task score and dual-task cost, Pearson’s correlation was performed (strong:|r| ≥ 0.7, moderate: 0.5 ≤ |r| < 0.7, weak: 0.3 ≤ |r| < 0.5). Means and standard errors were calculated. The significant level was set to 0.05. The Bonferroni method was used for multiple comparison corrections. The effect sizes were expressed with partial Eta squared (ηp2), ηp2 < 0.06: small effect size, 0.06 < ηp2 < 0.14: medium effect size, ηp2 > 0.14: strong effect size. Cortical activation pictures were visualized with BrainNet Viewer.
Results
Demographic data
Before the experiment, demographic variables were analyzed for both groups (Table 2). No significant differences in age, height, weight and cognitive performance were observed between the groups (p > 0.05).
Comparison of walking speed pre- and post-intervention
Two-way ANOVA with repeated measures was used to analyze walking speed and an interaction effect of group and time was observed under NOCT, but not under NOT (NOCT: F = 4.582, p = 0.043, ηp2 = 0.160; NOT: F = 0.440, p = 0.513, ηp2 = 0.018). Before the intervention, no significant differences in walking speed were observed between both groups under NOT and NOCT, and participants were at the same baseline level (NOCT: F = 0.038, p = 0.848, ηp2 = 0.002; NOT: F = 0.001, p = 0.969, ηp2 < 0.001). Walking speed post-intervention was significantly faster than pre-intervention in the Tai Chi group under NOCT (F = 4.708, p = 0.040, ηp2 = 0.164) and faster than in the Control group without statistical difference (F = 3.160, p = 0.088, ηp2 = 0.116; Figure 2). Thus, Tai Chi intervention improved walking speed performance of the older adults when negotiating obstacle under a dual-task condition.
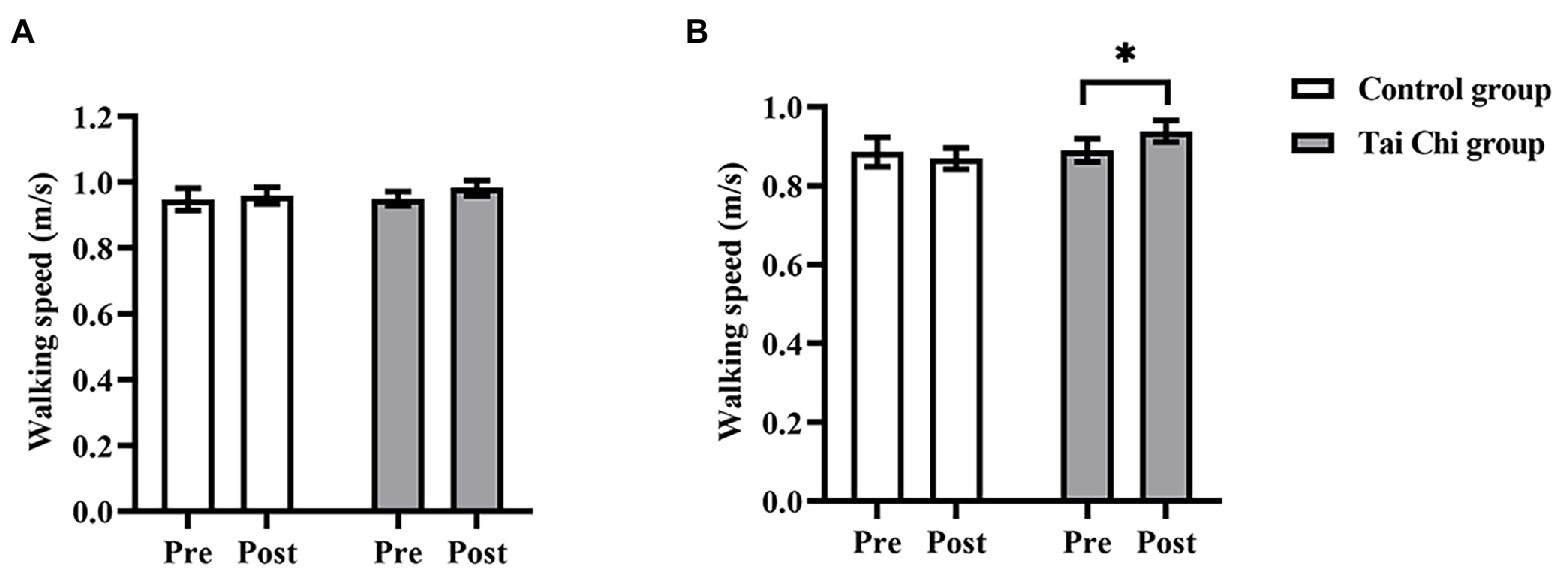
Figure 2. Walking speed in the Tai Chi group and Control group under NOT (A) and NOCT (B) pre- and post- intervention. *Represents significant difference (p < 0.05).
Comparison of dual-task cost pre- and post-intervention
Two-way ANOVA with repeated measures was used to analyze dual-task cost and an interaction effect of group and time was observed (F = 5.067, p = 0.034, ηp2 = 0.174). Post hoc test indicated dual-task cost post-intervention were significantly lower in the Tai Chi group (F = 4.393, p = 0.047, ηp2 = 0.155) and significantly higher in the Control group (F = 4.248, p = 0.050, ηp2 = 0.150), compared to pre-intervention (Figure 3). After the intervention, dual-task cost was significantly lower in the Tai Chi group than in the Control group (F = 5.232, p = 0.037, ηp2 = 0.174). No significant difference between two groups at pre-intervention (F = 0.294, p = 0.593, ηp2 = 0.012), indicating the two groups were at the same baseline level.
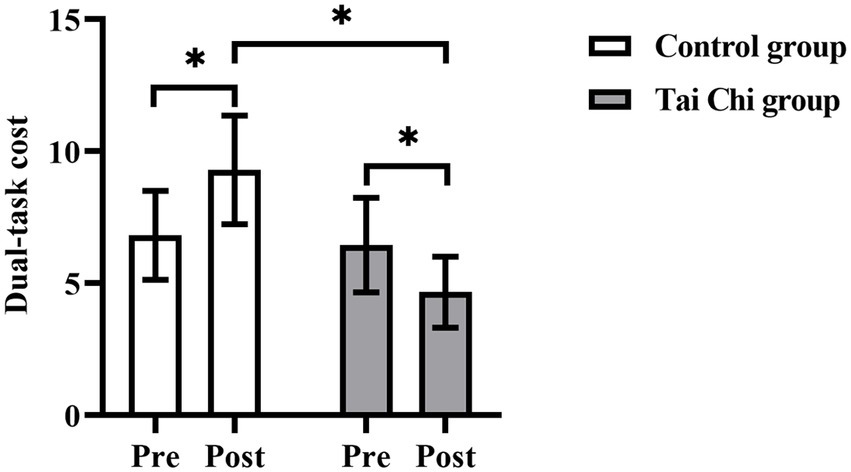
Figure 3. Dual-task cost in the Tai Chi group and Control group pre- and post- intervention. *Represents significant difference (p < 0.05).
Comparison of blood oxygen concentration changes pre- and post-intervention
Two-way ANOVA with repeated measures was applied for ΔHbO2 in right BA10 and left BA10. An interaction effect of group and time was identified under NOCT (right: F = 5.332, p = 0.030, ηp2 = 0.182; left: F = 8.901, p = 0.006, ηp2 = 0.271). Post hoc test indicated ΔHbO2 in BA10 were significantly higher after Tai Chi intervention (right: F = 11.404, p = 0.002, ηp2 = 0.322; left: F = 15.851, p = 0.001, ηp2 = 0.398), and significantly higher in the Tai Chi group compared to the Control group (right: F = 4.287, p = 0.049, ηp2 = 0.153; left: F = 6.384, p = 0.019, ηp2 = 0.210; Figures 4, 5). No significant difference between two groups pre-intervention (right: F = 0.947, p = 0.340, ηp2 = 0.038; left: F = 0.574, p = 0.456, ηp2 = 0.023) indicated the two groups were at the same baseline level. Under NOT, there was no interaction effect of group and time (right: F = 0.181, p = 0.674, ηp2 = 0.007; left: F = 0.063, p = 0.803, ηp2 = 0.003). There was the main effect of time but not of group in right BA10 (group: F = 0.628, p = 0.436, ηp2 = 0.026; time F = 13.044, p = 0.001, ηp2 = 0.352). There were not the main effects of group and time in left BA10 (group F = 1.426, p = 0.244, ηp2 = 0.054; time F = 2.879, p = 0.102, ηp2 = 0.103; Figures 4, 6).
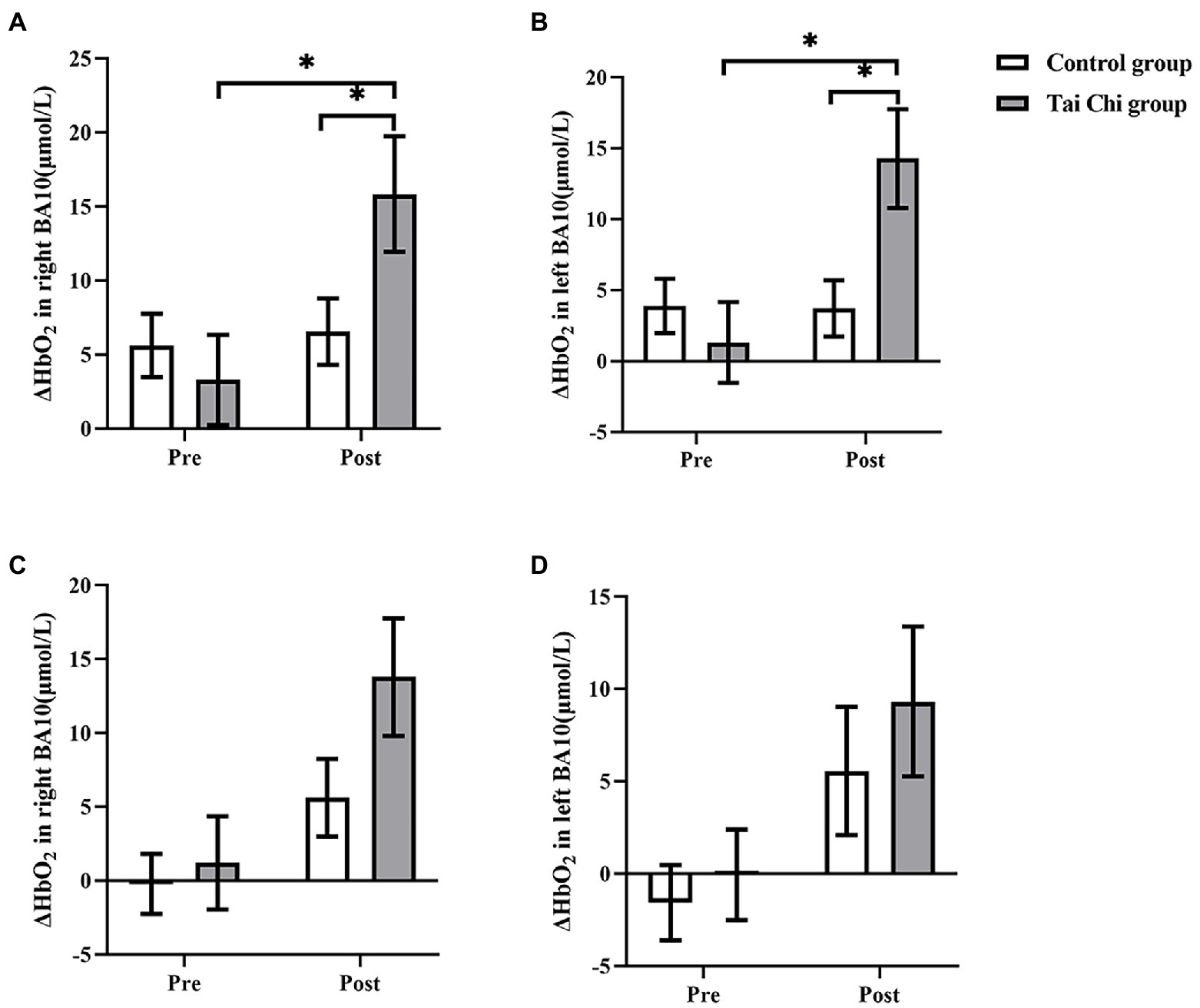
Figure 4. ΔHbO2 in BA10 in the Tai Chi group and Control group under NOCT and NOT pre- and post-intervention. (A–D) represent right BA10 under NOCT, left BA10 under NOCT, right BA10 under NOT, and left BA10 under NOT respectively. *Represents significant difference (p < 0.05).
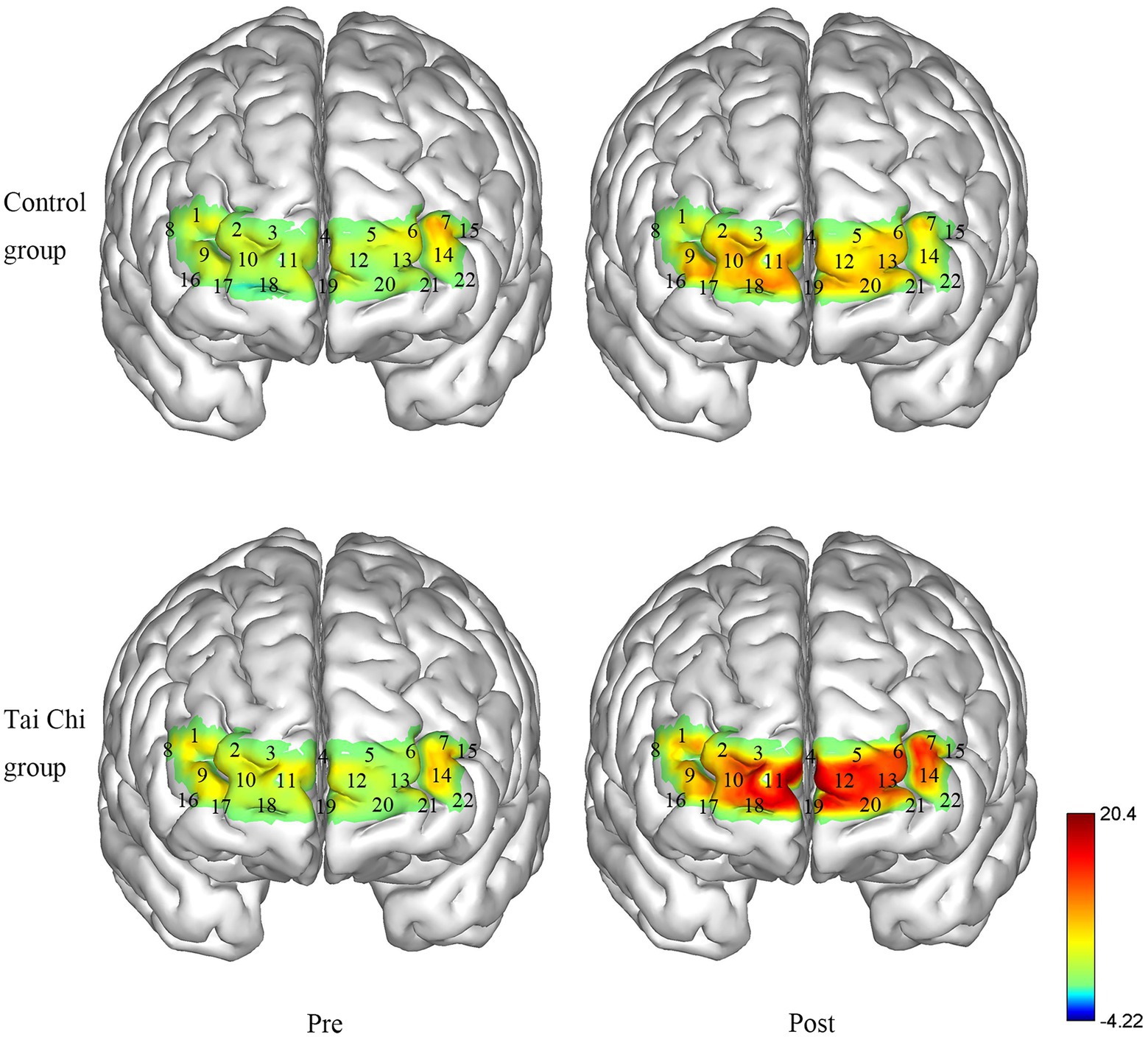
Figure 5. Changes in the ΔHbO2 in the Tai Chi group and Control group under NOCT pre- and post-intervention (μmol/L). The right BA10 includes channels 2, 3, 4, 9, 10, and 11, and the left BA10 includes channels 4, 5, 6, 12, 13, 14, and 22.
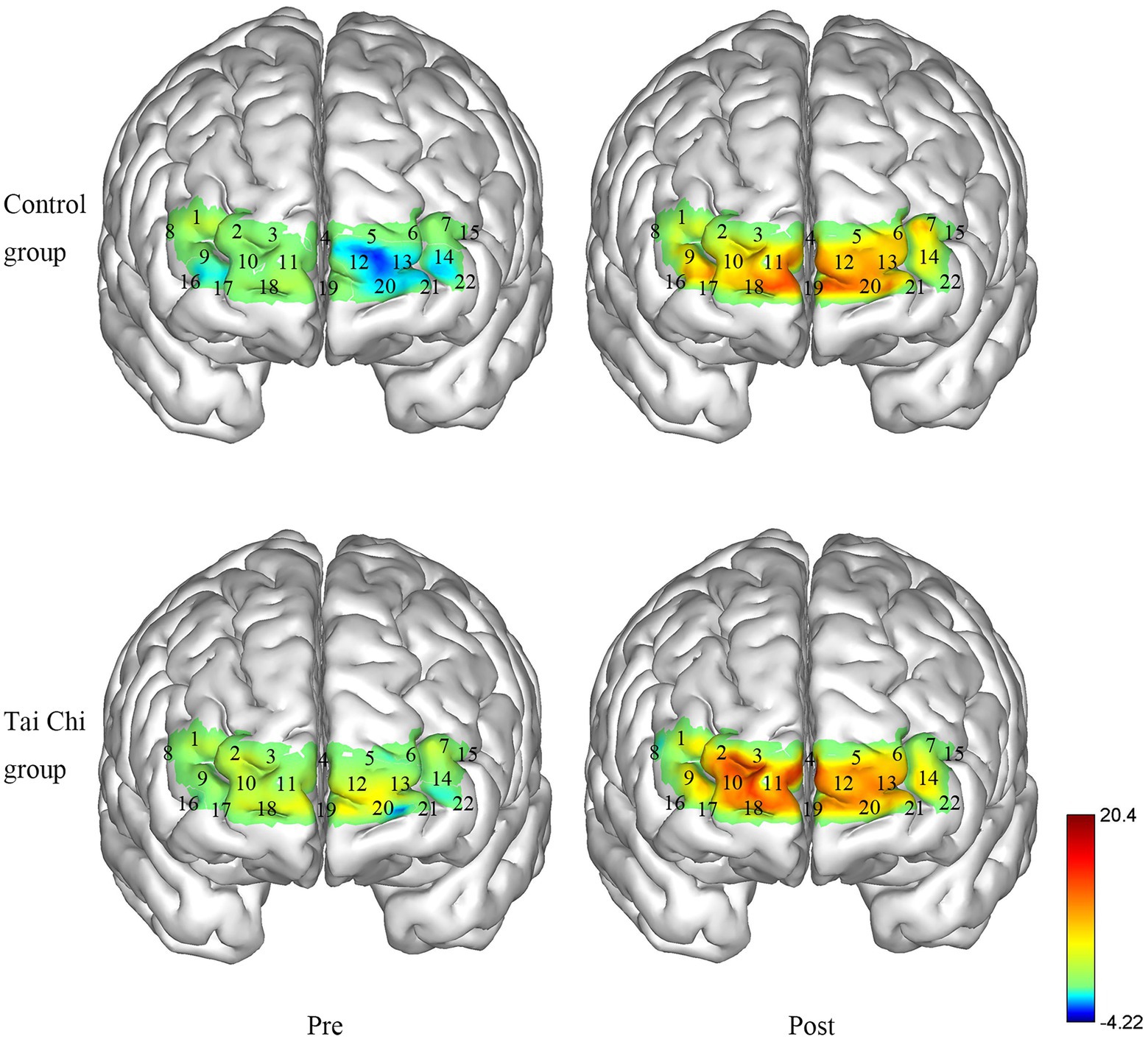
Figure 6. Changes in the ΔHbO2 in the Tai Chi group and Control group under NOT pre- and post-intervention (μmol/L). The right BA10 includes channels 2, 3, 4, 9, 10, and 11, and the left BA10 includes channels 4, 5, 6, 12, 13, 14, and 22.
Two-way ANOVA with repeated measures was applied for ΔHbR in right BA10 and left BA10. No group and time interactions were found under NOT and NOCT (NOT: right: F = 0.029, p = 0.867, ηp2 = 0.001; left: F = 0.028, p = 0.868, ηp2 = 0.001; NOCT: right: F = 0.322, p = 0.576, ηp2 = 0.013; Left: F = 0.447, p = 0.510, ηp2 = 0.018; Figure 7). The main effects of group and time in right BA10 were not statistically significant under NOT and NOCT (NOT: group: F < 0.001, p = 0.990, ηp2 < 0.001; time: F = 3.146, p = 0.089, ηp2 = 0.116; NOCT: group: F = 0.826, p = 0.372, ηp2 = 0.033; time: F = 0.308, p = 0.584, ηp2 = 0.013). The main effect of group in left BA10 was not statistically significant, while the main effect of time was statistically significant under NOT (group: F = 0.010, p = 0.920, ηp2 < 0.001; time: F = 5.159, p = 0.032, ηp2 = 0.177). The main effect of group in left BA10 was statistically significant, while the main effect of time was not statistically significant under NOCT (group: F = 4.279, p = 0. 050, ηp2 = 0.151; time: F = 0.921, p = 0.347, ηp2 = 0.037).
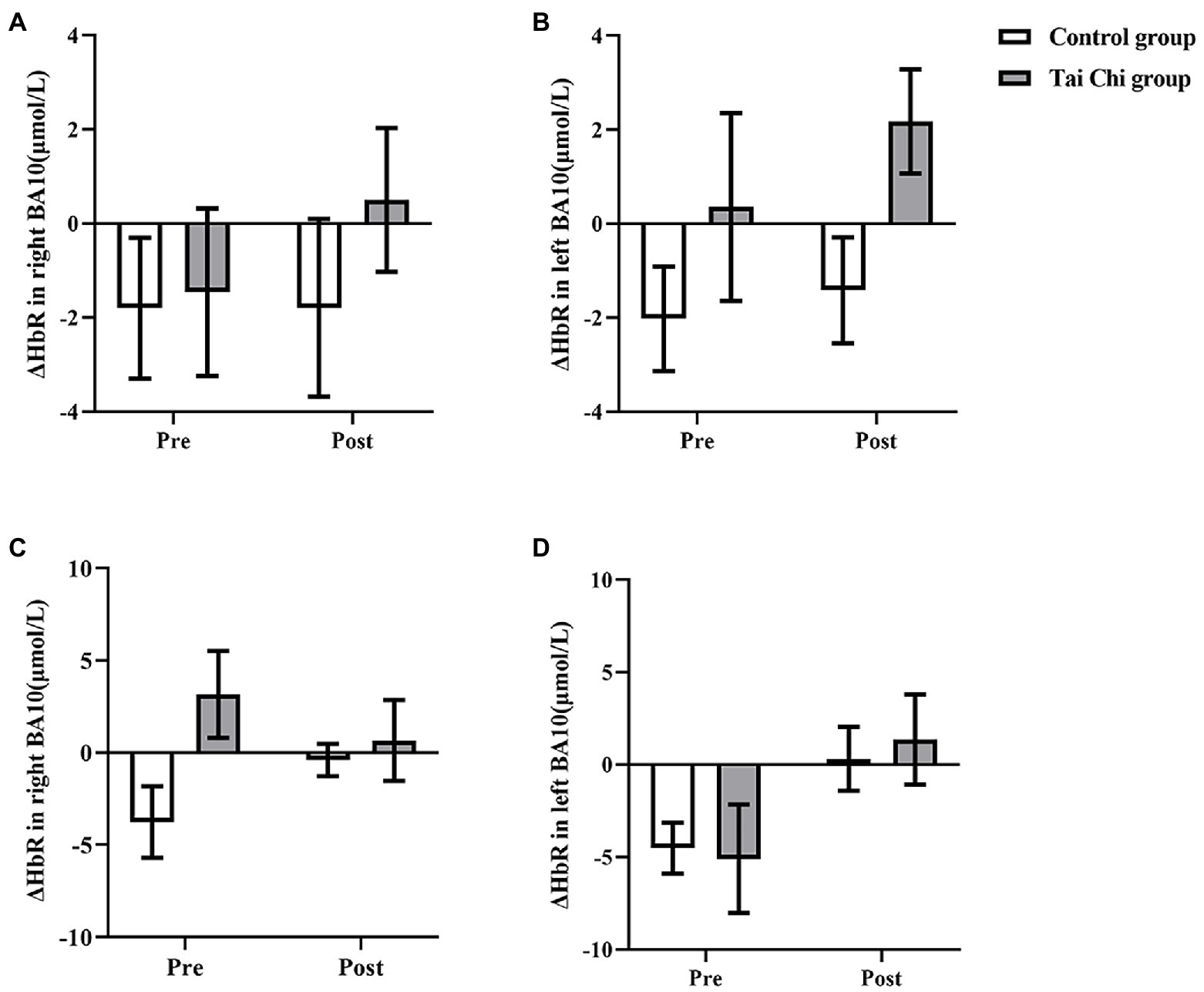
Figure 7. ΔHbR in BA10 in the Tai Chi group and Control group under NOCT and NOT pre- and post-intervention. (A–D) represent right BA10 under NOCT, left BA10 under NOCT, right BA10 under NOT, and left BA10 under NOT respectively. *Represents significant difference (p < 0.05).
Two-way ANOVA with repeated measures was applied for ΔHbT in right BA10 and left BA10. An interaction effect of group and time was identified under NOCT (right: F = 6.117, p = 0.021, ηp2 = 0.203; left: F = 7.274, p = 0.013, ηp2 = 0.233). Post hoc test indicated ΔHbT in BA10 was significantly higher after Tai Chi intervention (right: F = 9.090, p = 0.006, ηp2 = 0.275; left: F = 11.213, p = 0.003, ηp2 = 0.318). ΔHbT in left BA10 was significantly higher whereas ΔHbT in right BA10 was higher without statistical difference in the Tai Chi group compared to the Control group (right: F = 4.081, p = 0.055, ηp2 = 0.145; left: F = 7.796, p = 0.010, ηp2 = 0.245; Figure 8). No significant differences between two groups at pre-intervention (right: F = 0.668, p = 0.422, ηp2 = 0.027; left: F = 0.028, p = 0.869, ηp2 = 0.001) indicated two groups were at the same baseline level. Under NOT, there was no interaction effect of group and time (right: F = 0.090, p = 0.767, ηp2 = 0.004; left: F = 0.017, p = 0.899, ηp2 = 0.001). The main effect of group was not statistically significant (right: F = 0.289, p = 0.596, ηp2 = 0.0126; left: F = 0.161, p = 0.692, ηp2 = 0.007). The main effect of time was statistically significant (right: F = 10.200, p = 0.004, ηp2 = 0.298; left: F = 15.647, p = 0.001, ηp2 = 0.395).
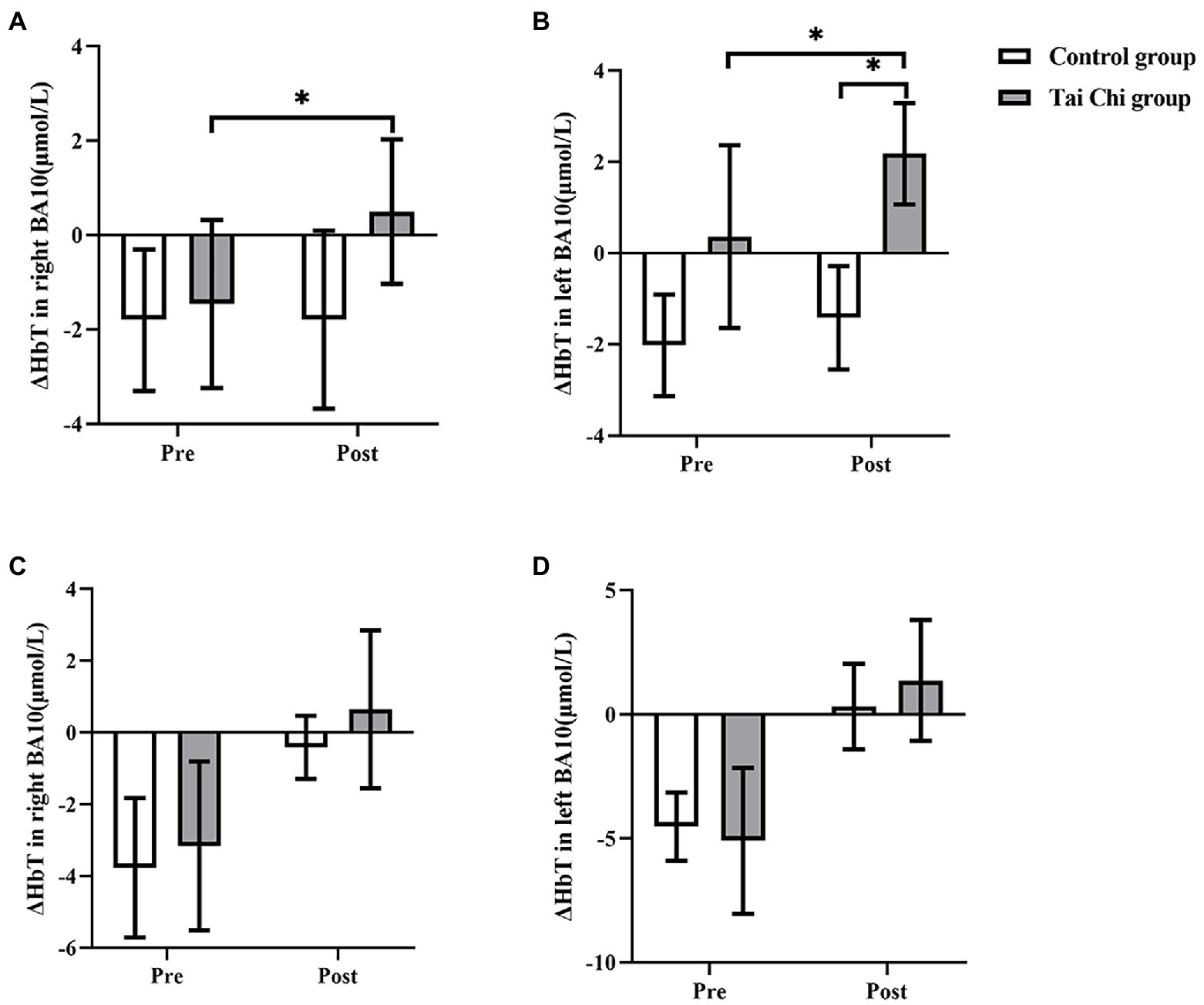
Figure 8. ΔHbT in BA10 in the Tai Chi group and Control group under NOCT and NOT pre- and post-intervention. (A–D) represent right BA10 under NOCT, left BA10 under NOCT, right BA10 under NOT, and left BA10 under NOT respectively. *Represents significant difference (p < 0.05).
Dual-task score under NOCT
The statistical analysis results revealed no interaction effects in group and time (F = 0.587, p = 0.451, ηp2 = 0.024). No significant differences were observed in dual-task score pre-intervention between two groups (F = 0.587, p = 0.451, ηp2 = 0.024). The main effects of group and time were not statistically significant (group: F = 0.738, p = 0. 399, ηp2 = 0.030; time: F = 0.770, p = 0.389, ηp2 = 0.031).
Correlation among PFC ΔHbO2, dual-task score, dual-task cost and walking speed under NOCT
Before the intervention under NOCT, ΔHbO2 in left BA10 was negatively correlated with dual-task cost (p = 0.001). Whereas ΔHbO2 in right BA10 was not correlated with dual-task cost (p = 0.199) and walking speed (p = 0.287). After the intervention under NOCT, ΔHbO2 in left BA10 was negatively correlated with dual-task cost (p = 0.019) and positively correlated with walking speed (p = 0.016). ΔHbO2 in right BA10 was negatively correlated with dual-task cost (p = 0.021) and positively correlated with walking speed (p = 0.015; see the details in Table 3).
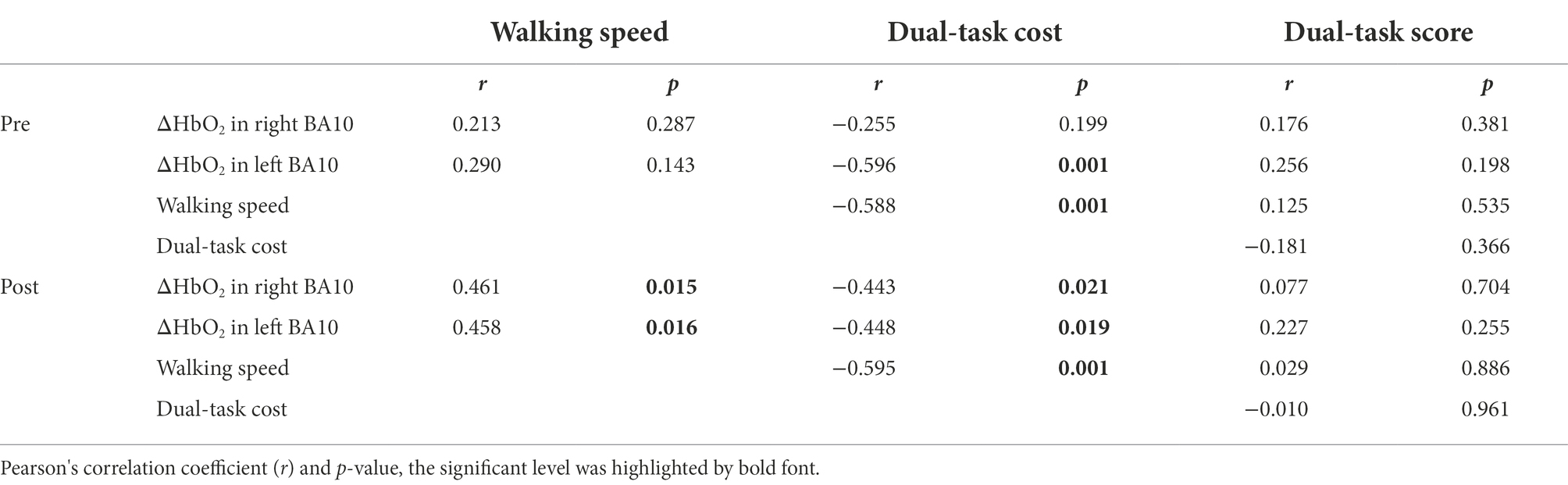
Table 3. Pearson’s correlation analysis among ΔHbO2, walking speed, dual-task cost and dual-task score under NOCT.
Dual-task cost was negatively correlated with walking speed under NOCT (pre-intervention: p = 0.001; post-intervention: p = 0.001), whereas dual-task scores were not correlated with ΔHbO2, dual-task cost and walking speed (p > 0.05), either pre- or post-intervention (see the details in Table 3).
After the CBSI correction, there were strong negative correlations between ΔHbO2 and ΔHbR under NOCT, either pre-intervention (left PFC r = −0.841, p < 0.001; right PFC r = −0.795, p < 0.001) or post-intervention (left PFC r = −0.842, p < 0.001; right PFC r = −0.744, p < 0.001). There were strong/moderate negative correlations between ΔHbO2 and ΔHbR under NOT, either pre-intervention (left PFC r = −0.739, p < 0.001; right PFC r = −0.690, p < 0.001) or post-intervention (left PFC r = −0.782, p < 0.001; right PFC r = −0.540, p = 0.004).
Discussion
In this study, we investigated the effects of a 16-week Tai Chi intervention on cortical activation and gait performance of older adults during negotiating obstacle in single-task and dual-task conditions. After Tai Chi intervention under NOCT, ΔHbO2 with a significant increase and ΔHbR with no significant change or slight increase, which was probably induced by head motion in the more naturalistic experiment (Cui et al., 2010) i.e., walking and negotiating obstacles. After the CBSI correction, there were strong negative correlations between ΔHbO2 and ΔHbR under NOCT either pre-intervention or post-intervention. The research results revealed: (1) after Tai Chi intervention, PFC activation was bilateral with lower dual-task cost under NOCT, meanwhile these were not observed under NOT; (2) PFC ΔHbO2 under NOCT was negatively correlated with dual-task cost and not correlated with dual-task score.
With aging, walking was less automated and required more attentional resources. When walking became more difficult under a dual-task condition, PFC showed higher activation (Mirelman et al., 2017). In the Tai Chi group, when older adults performed cognitive task and negotiating obstacle simultaneously, the PFC was bilaterally activated and compensated for the cognitive resource deficit. Our findings were in line with the revised scaffolding theory of aging and cognition, suggesting that the older adults may adjust to age-related neurodegeneration through recruiting additional neural networks (Reuter-Lorenz and Park, 2014). Increased PFC activation was an indicator of brain adaption with compensation to maintain the performance as a result of declining neural functions and structures (Park and Reuter-Lorenz, 2009). After Tai Chi intervention, lower dual-task cost exhibited a more active gait pattern and less interference from cognitive task. PFC activation under NOCT was negatively correlated with dual-task cost. The high-level prefrontal activity was linked to optimizing gait performance during a complex walking task, such as negotiating obstacle. When encountering an obstacle (Ohsugi et al., 2013; Clark et al., 2014), successful gait adaptability was crucial and associated with processing speed for precise foot placements and cognitive capacity for step length adjustments (Caetano et al., 2017). Impaired gait adaptability contributed to high risk of falls in older adults and reduced executive function significantly enhanced the link (Caetano et al., 2016, 2018). Healthy older adults required additional PFC resources for task demands, and this overactivation might lead to better external performance (Albinet et al., 2014; Dupuy et al., 2015; Hyodo et al., 2016). For older adults, Tai Chi practice possibly enabled higher PFC activation and better gait performance to cope with challenges during walking.
PFC activation under NOCT was negatively correlated with dual-task cost, and not correlated with dual-task score. PFC activation might allocate more attention to motor ability but not calculation task, which was not consistent with that of Takayuki Mori et al. (2018). Their findings indicated that healthy participants prioritized the cognitive task during dual-task walking. One possible explanation for the difference was a greater age range (range 30–74 years) in contrast with this study (range 65–75 years). Some studies have suggested that young and older adults spontaneously prioritized gait stability during walking if no specific prioritization instruction or allocation of attention was provided, this strategy by which they tended to prioritize motor ability was known as ‘posture first’(Shumway-Cook et al., 1997). Moreover, when given the choice between a cognitive or motor performance aid, older adults selected the option to supplement gait performance (Li et al., 2001), and this postural prioritization strategy helped to prevent the loss of balance (Shumway-Cook et al., 1997; Li et al., 2001; Yogev-Seligmann et al., 2010; Chen et al., 2022). Interestingly, after Tai Chi intervention, walking speed was not faster, and PFC activation was not greater under NOT. This finding might indicate the available cognitive resources were sufficient for maintaining gait performance during negotiating obstacle, and kept resource-saving in older adults, although Tai Chi practice increased the capacity of available resources.
Originating from martial arts, Tai Chi was a mind–body exercise that integrated flexibility and coordination, with performance driven by an inner to outer process (i.e., mind-initiated and directed inside, movement synergies outside; Li, 2014; Yang et al., 2019). Tai Chi practice required not only simple to complex, balance-challenging, multi-joint directed postural control movements, but also memory training for complex movement sequences and associated rhythms, as well as various higher cognitive functions (e.g., visuospatial ability, attention, multitask planning, and processing) to maintain postural stability and orientation. During negotiating obstacle, the visuospatial ability was required to load all information about the obstacle (such as the distance to the obstacle, and the size of the obstacle), and Tai Chi focused on visuospatial training. When physical-spatial orientations and action directions were frequently inconsistent during Tai Chi practice, older adults needed to deal with this conflicting information and select a proper action, which required specific cognitive activities, including motion recall and task switching (Yang et al., 2019). Long-term Tai Chi practice could lead to changes in the local brain structures (Wei et al., 2014) and enhance functional connectivity (Tao et al., 2016, 2017). In a study analyzing the brain structures of older adults with long-term Tai Chi practice, the frontal middle sulcus was significantly thicker, suggesting that Tai Chi practice could cause structural changes in the PFC (Wei et al., 2013). The frontal middle sulcus was located in the dorsolateral PFC and partially overlapped with BA10 (the ROI in this study), which provided the evidence that Tai Chi practice changed the local structures of the PFC and improved executive function. The HAROLD model (hemispheric asymmetry reduction in older adults) stated the prefrontal activity was inclined to manifest less lateralization in older adults than in younger adults, attributing to a compensatory function or a dedifferentiation process (Cabeza, 2002). From a computational benefit hypothesis, the functional lateralization could be known as a special case of the functional specialization of the brain (Kosslyn, 1987) to process the information more efficiently. The age-related hemispheric asymmetry reduction reflected the decrease in neural processing capacity by performing simultaneous processing (Rogers et al., 2004). After Tai Chi intervention, the bilateral activation mode could help offset age-related neurocognitive deficits, which could be achieved by alternative neurocognitive networks (Reuter-Lorenz and Lustig, 2005).
This study had some limitations. First, we did not monitor skin blood flow, which might interfere fNIRS signal (Kirilina et al., 2012; Erdogan et al., 2014). Second, no physiological parameters, such as heart rate, and rate of perceived exertion, were measured during Tai Chi intervention, although Tai Chi was considered to be a low to moderate-intensity exercise. Third, the fNIRS equipment had 16 optodes, so that only the PFC was detected, failing to involve other brain regions related to motor control or visual processing, such as the premotor, sensorimotor, and occipital cortices. Fourth, this study recruited participants in a narrow age range from 65 to 75 to avoid the influences of heterogeneity among older adults, which may affect the applicability of our outcomes to other age populations. Fifth, consequence of interindividual response variability should be considered for future studies as these variables are linked to exercise-induced benefits (Herold et al., 2021). Lastly, eye-based measures are recently recommended by a group of experts exercise-cognition field as they can be used in combination with fNIRS, leading to more reliable findings.
Conclusion
Tai Chi practice might increase cognitive resources of older adults through the PFC bilateral activation to prioritize gait performance during negotiating obstacle under a dual-task condition.
Data availability statement
The raw data supporting the conclusions of this article will be made available by the authors, without undue reservation.
Ethics statement
The studies involving human participants were reviewed and approved by the Ethics Review Committee of Shandong Sport University. The patients/participants provided their written informed consent to participate in this study.
Author contributions
DM: conceptualization and project design. YC: methodology, experiment implementation and drafting manuscript. AW: data acquisition, visualization and software. MM: investigation and experiment implement. WS: data curation and methodology. QS: supervision and funding acquisition. All authors contributed to the article and approved the submitted version.
Funding
This work was supported by China Shandong Province Youth Innovative Talent Induction Program (grant number 2019-183).
Acknowledgments
The authors would like to thank Kewang Xie, Zhenjing Cao, Cong Yu, Huifen Zheng, Hengshuo Zhang, Xiao Gao, Xiu Hu, Fang Liu, Teng Zhang, graduate students at Shandong Sport University, for participating in the experiment or data acquisition for this work.
Conflict of interest
The authors declare that the research was conducted in the absence of any commercial or financial relationships that could be construed as a potential conflict of interest.
Publisher’s note
All claims expressed in this article are solely those of the authors and do not necessarily represent those of their affiliated organizations, or those of the publisher, the editors and the reviewers. Any product that may be evaluated in this article, or claim that may be made by its manufacturer, is not guaranteed or endorsed by the publisher.
Supplementary material
The Supplementary material for this article can be found online at: https://www.frontiersin.org/articles/10.3389/fnagi.2022.1000427/full#supplementary-material
References
Albinet, C. T., Mandrick, K., Bernard, P. L., Perrey, S., and Blain, H. (2014). Improved cerebral oxygenation response and executive performance as a function of cardiorespiratory fitness in older women: a fNIRS study. Front. Aging Neurosci. 6:272. doi: 10.3389/fnagi.2014.00272
Amboni, M., Barone, P., and Hausdorff, J. M. (2013). Cognitive contributions to gait and falls: evidence and implications. Mov. Disord. 28, 1520–1533. doi: 10.1002/mds.25674
Atsumori, H., Kiguchi, M., Katura, T., Funane, T., Obata, A., Sato, H., et al. (2010). Noninvasive imaging of prefrontal activation during attention-demanding tasks performed while walking using a wearable optical topography system. J. Biomed. Opt. 15:046002. doi: 10.1117/1.3462996
Balardin, J. B., Zimeo Morais, G. A., Furucho, R. A., Trambaiolli, L., Vanzella, P., Biazoli, C., et al. (2017). Imaging brain function with functional near-infrared spectroscopy in unconstrained environments. Front. Hum. Neurosci. 11:258. doi: 10.3389/fnhum.2017.00258
Beurskens, R., and Bock, O. (2012). Age-related decline of peripheral visual processing: the role of eye movements. Exp. Brain Res. 217, 117–124. doi: 10.1007/s00221-011-2978-3
Beurskens, R., Helmich, I., Rein, R., and Bock, O. (2014). Age-related changes in prefrontal activity during walking in dual-task situations: a fNIRS study. Int. J. Psychophysiol. 92, 122–128. doi: 10.1016/j.ijpsycho.2014.03.005
Braver, T. S., and Bongiolatti, S. R. (2002). The role of frontopolar cortex in subgoal processing during working memory. NeuroImage 15, 523–536. doi: 10.1006/nimg.2001.1019
Buckner, R. L. (2004). Memory and executive function in aging and AD: multiple factors that cause decline and reserve factors that compensate. Neuron 44, 195–208. doi: 10.1016/j.neuron.2004.09.006
Cabeza, R. (2002). Hemispheric asymmetry reduction in older adults: the HAROLD model. Psychol. Aging 17, 85–100. doi: 10.1037//0882-7974.17.1.85
Caetano, M. J. D., Lord, S. R., Brodie, M. A., Schoene, D., Pelicioni, P. H. S., Sturnieks, D. L., et al. (2018). Executive functioning, concern about falling and quadriceps strength mediate the relationship between impaired gait adaptability and fall risk in older people. Gait Posture 59, 188–192. doi: 10.1016/j.gaitpost.2017.10.017
Caetano, M. J., Lord, S. R., Schoene, D., Pelicioni, P. H., Sturnieks, D. L., and Menant, J. C. (2016). Age-related changes in gait adaptability in response to unpredictable obstacles and stepping targets. Gait Posture 46, 35–41. doi: 10.1016/j.gaitpost.2016.02.003
Caetano, M. J. D., Menant, J. C., Schoene, D., Pelicioni, P. H. S., Sturnieks, D. L., and Lord, S. R. (2017). Sensorimotor and cognitive predictors of impaired gait adaptability in older people. J. Gerontol. A Biol. Sci. Med. Sci. 72, glw171–glw1263. doi: 10.1093/gerona/glw171
Cai, K., Yu, Q., Herold, F., Liu, Z., Wang, J., Zhu, L., et al. (2020). Mini-basketball training program improves social communication and white matter integrity in children with autism. Brain Sci. 10:803. doi: 10.3390/brainsci10110803
Camicioli, R., Howieson, D., Lehman, S., and Kaye, J. (1997). Talking while walking: the effect of a dual task in aging and Alzheimer's disease. Neurology 48, 955–958. doi: 10.1212/wnl.48.4.955
Chen, Y., Cao, Z., Mao, M., Sun, W., Song, Q., and Mao, D. (2022). Increased cortical activation and enhanced functional connectivity in the prefrontal cortex ensure dynamic postural balance during dual-task obstacle negotiation in the older adults: a fNIRS study. Brain Cogn. 163:105904. doi: 10.1016/j.bandc.2022.105904
Chen, M., Pillemer, S., England, S., Izzetoglu, M., Mahoney, J. R., and Holtzer, R. (2017). Neural correlates of obstacle negotiation in older adults: an fNIRS study. Gait Posture 58, 130–135. doi: 10.1016/j.gaitpost.2017.07.043
Chen, L. Z., Yuan, X., Zhang, Y., Zhang, S., Zou, L., Yang, L., et al. (2020). Brain functional specialization is enhanced among Tai Chi Chuan practitioners. Arch. Phys. Med. Rehabil. 101, 1176–1182. doi: 10.1016/j.apmr.2020.02.005
Cheval, B., Maltagliati, S., Sieber, S., Cullati, S., Zou, L., Ihle, A., et al. (2022). Better subjective sleep quality partly explains the association between self-reported physical activity and better cognitive function. J. Alzheimers Dis. 87, 919–931. doi: 10.3233/JAD-215484
Clark, D. J., Rose, D. K., Ring, S. A., and Porges, E. C. (2014). Utilization of central nervous system resources for preparation and performance of complex walking tasks in older adults. Front. Aging Neurosci. 6:217. doi: 10.3389/fnagi.2014.00217
Cui, X., Bray, S., and Reiss, A. L. (2010). Functional near infrared spectroscopy (NIRS) signal improvement based on negative correlation between oxygenated and deoxygenated hemoglobin dynamics. NeuroImage 49, 3039–3046. doi: 10.1016/j.neuroimage.2009.11.050
Cunha, P. M., Nunes, J. P., Werneck, A. O., Ribeiro, A. S., da Silva Machado, D. G., Kassiano, W., et al. (2022). Effect of resistance exercise orders on health parameters in trained older women: a randomized crossover trial. Med. Sci. Sports Exerc. doi: 10.1249/mss.0000000000003030 [Epub ahead of print].
Demurtas, J., Schoene, D., Torbahn, G., Marengoni, A., Grande, G., Zou, L., et al. (2020). Physical activity and exercise in mild cognitive impairment and Dementia: an umbrella review of intervention and observational studies. J. Am. Med. Dir. Assoc. 21, 1415–1422. doi: 10.1016/j.jamda.2020.08.031
Di Lorenzo, R., Pirazzoli, L., Blasi, A., Bulgarelli, C., Hakuno, Y., Minagawa, Y., et al. (2019). Recommendations for motion correction of infant fNIRS data applicable to multiple data sets and acquisition systems. NeuroImage 200, 511–527. doi: 10.1016/j.neuroimage.2019.06.056
Dong, D., Wong, L. K. F., and Luo, Z. (2019). Assess BA10 activity in slide-based and immersive virtual reality prospective memory task using functional near-infrared spectroscopy (fNIRS). Appl. Neuropsychol. Adult 26, 465–471. doi: 10.1080/23279095.2018.1443104
Dupuy, O., Gauthier, C. J., Fraser, S. A., Desjardins-Crepeau, L., Desjardins, M., Mekary, S., et al. (2015). Higher levels of cardiovascular fitness are associated with better executive function and prefrontal oxygenation in younger and older women. Front. Hum. Neurosci. 9:66. doi: 10.3389/fnhum.2015.00066
Erdogan, S. B., Yucel, M. A., and Akin, A. (2014). Analysis of task-evoked systemic interference in fNIRS measurements: insights from fMRI. NeuroImage 87, 490–504. doi: 10.1016/j.neuroimage.2013.10.024
Galna, B., Peters, A., Murphy, A. T., and Morris, M. E. (2009). Obstacle crossing deficits in older adults: a systematic review. Gait Posture 30, 270–275. doi: 10.1016/j.gaitpost.2009.05.022
Garavan, H., Ross, T. J., Murphy, K., Roche, R. A., and Stein, E. A. (2002). Dissociable executive functions in the dynamic control of behavior: inhibition, error detection, and correction. NeuroImage 17, 1820–1829. doi: 10.1006/nimg.2002.1326
Gu, Q., Zou, L., Loprinzi, P. D., Quan, M., and Huang, T. (2019). Effects of open versus closed skill exercise on cognitive function: a systematic review. Front. Psychol. 10:1707. doi: 10.3389/fpsyg.2019.01707
Harley, C., Wilkie, R. M., and Wann, J. P. (2009). Stepping over obstacles: attention demands and aging. Gait Posture 29, 428–432. doi: 10.1016/j.gaitpost.2008.10.063
Hausdorff, J. M., Schweiger, A., Herman, T., Yogev-Seligmann, G., and Giladi, N. (2008). Dual-task decrements in gait: contributing factors among healthy older adults. J. Gerontol. A Biol. Sci. Med. Sci. 63, 1335–1343. doi: 10.1093/gerona/63.12.1335
Head, D., Buckner, R. L., Shimony, J. S., Williams, L. E., Akbudak, E., Conturo, T. E., et al. (2004). Differential vulnerability of anterior white matter in nondemented aging with minimal acceleration in dementia of the Alzheimer type: evidence from diffusion tensor imaging. Cereb. Cortex 14, 410–423. doi: 10.1093/cercor/bhh003
Hedden, T., and Gabrieli, J. D. (2004). Insights into the ageing mind: a view from cognitive neuroscience. Nat. Rev. Neurosci. 5, 87–96. doi: 10.1038/nrn1323
Herman, T., Mirelman, A., Giladi, N., Schweiger, A., and Hausdorff, J. M. (2010). Executive control deficits as a prodrome to falls in healthy older adults: a prospective study linking thinking, walking, and falling. J. Gerontol. A Biol. Sci. Med. Sci. 65, 1086–1092. doi: 10.1093/gerona/glq077
Herold, F., Törpel, A., Hamacher, D., Budde, H., Zou, L., Strobach, T., et al. (2021). Causes and consequences of Interindividual response variability: a call to apply a more rigorous research Design in Acute Exercise-Cognition Studies. Front. Physiol. 12:682891. doi: 10.3389/fphys.2021.682891
Herold, F., Wiegel, P., Scholkmann, F., and Muller, N. G. (2018). Applications of functional near-infrared spectroscopy (fNIRS) neuroimaging in exercise(−)cognition science: a systematic, methodology-focused review. J. Clin. Med. 7. doi: 10.3390/jcm7120466
Hiura, M., Nariai, T., Takahashi, K., Muta, A., Sakata, M., Ishibashi, K., et al. (2018). Dynamic exercise elicits dissociated changes between tissue oxygenation and cerebral blood flow in the prefrontal cortex: a study using NIRS and PET. Adv. Exp. Med. Biol. 1072, 269–274. doi: 10.1007/978-3-319-91287-5_43
Holtzer, R., Epstein, N., Mahoney, J. R., Izzetoglu, M., and Blumen, H. M. (2014). Neuroimaging of mobility in aging: a targeted review. J. Gerontol. A Biol. Sci. Med. Sci. 69, 1375–1388. doi: 10.1093/gerona/glu052
Hu, Y., Kattan, C., Kontos, D., Zhu, W., and Hernandez, M. E. (2021). Benefits of tai ji quan practice on neuromuscular functions in older adults: a systematic review and meta-analysis. Complement. Ther. Clin. Pract. 42:101295. doi: 10.1016/j.ctcp.2020.101295
Hyodo, K., Dan, I., Kyutoku, Y., Suwabe, K., Byun, K., Ochi, G., et al. (2016). The association between aerobic fitness and cognitive function in older men mediated by frontal lateralization. NeuroImage 125, 291–300. doi: 10.1016/j.neuroimage.2015.09.062
Jung, M., Zou, L., Yu, J. J., Ryu, S., Kong, Z., Yang, L., et al. (2020). Does exercise have a protective effect on cognitive function under hypoxia? A systematic review with meta-analysis. J. Sport Health Sci. 9, 562–577. doi: 10.1016/j.jshs.2020.04.004
Kearney, F., Harwood, R., Gladman, J., Lincoln, N., and Masud, T. (2012). The relationship between executive function and falls and gait abnormalities in older adults: a systematic review. Eur. Geriatr. Med. 3, S50–S51. doi: 10.1016/j.eurger.2012.07.060
Kim, T. H., Pascual-Leone, J., Johnson, J., and Tamim, H. (2016). The mental-attention Tai Chi effect with older adults. BMC Psychol 4:29. doi: 10.1186/s40359-016-0137-0
Kirilina, E., Jelzow, A., Heine, A., Niessing, M., Wabnitz, H., Bruhl, R., et al. (2012). The physiological origin of task-evoked systemic artefacts in functional near infrared spectroscopy. NeuroImage 61, 70–81. doi: 10.1016/j.neuroimage.2012.02.074
Konak, H. E., Kibar, S., and Ergin, E. S. (2016). The effect of single-task and dual-task balance exercise programs on balance performance in adults with osteoporosis: a randomized controlled preliminary trial. Osteoporos. Int. 27, 3271–3278. doi: 10.1007/s00198-016-3644-1
Kong, C., Chen, A., Ludyga, S., Herold, F., Healy, S., Zhao, M., et al. (2022). Associations between meeting 24-hour movement guidelines and quality of life among children and adolescents with autism spectrum disorder. J. Sport Health Sci. doi: 10.1016/j.jshs.2022.08.003 [Epub ahead of print].
Koohsari, M. J., McCormack, G. R., Nakaya, T., Shibata, A., Ishii, K., Yasunaga, A., et al. (2020). Walking-friendly built environments and objectively measured physical function in older adults. J. Sport Health Sci. 9, 651–656. doi: 10.1016/j.jshs.2020.02.002
Kosslyn, S. M. (1987). Seeing and imagining in the cerebral hemispheres: a computational approach. Psychol. Rev. 94, 148–175. doi: 10.1037/0033-295X.94.2.148
Kunstler, E. C. S., Finke, K., Gunther, A., Klingner, C., Witte, O., and Bublak, P. (2018). Motor-cognitive dual-task performance: effects of a concurrent motor task on distinct components of visual processing capacity. Psychol. Res. 82, 177–185. doi: 10.1007/s00426-017-0951-x
Leone, C., Feys, P., Moumdjian, L., D'Amico, E., Zappia, M., and Patti, F. (2017). Cognitive-motor dual-task interference: a systematic review of neural correlates. Neurosci. Biobehav. Rev. 75, 348–360. doi: 10.1016/j.neubiorev.2017.01.010
Li, F. (2014). Transforming traditional tai Ji Quan techniques into integrative movement therapy-tai Ji Quan: moving for better balance. J. Sport Health Sci. 3, 9–15. doi: 10.1016/j.jshs.2013.11.002
Li, F., Harmer, P., Fitzgerald, K., Eckstrom, E., Stock, R., Galver, J., et al. (2012). Tai Chi and postural stability in patients with Parkinson's disease. N. Engl. J. Med. 366, 511–519. doi: 10.1056/NEJMoa1107911
Li, K. Z., Lindenberger, U., Freund, A. M., and Baltes, P. B. (2001). Walking while memorizing: age-related differences in compensatory behavior. Psychol. Sci. 12, 230–237. doi: 10.1111/1467-9280.00341
Lu, S., Herold, F., Zhang, Y., Lei, Y., Kramer, A. F., Jiao, C., et al. (2021). Higher handgrip strength is linked to better cognitive performance in Chinese adults with hypertension. Brain Sci. 11:985. doi: 10.3390/brainsci11080985
Lundin-Olsson, L., Nyberg, L., and Gustafson, Y. (1997). “Stops walking when talking” as a predictor of falls in elderly people. Lancet 349:617. doi: 10.1016/s0140-6736(97)24009-2
Maylor, E. A., and Wing, A. M. (1996). Age differences in postural stability are increased by additional cognitive demands. J. Gerontol. B Psychol. Sci. Soc. Sci. 51, P143–P154. doi: 10.1093/geronb/51b.3.p143
Mirelman, A., Maidan, I., Bernad-Elazari, H., Shustack, S., Giladi, N., and Hausdorff, J. M. (2017). Effects of aging on prefrontal brain activation during challenging walking conditions. Brain Cogn. 115, 41–46. doi: 10.1016/j.bandc.2017.04.002
Montero-Odasso, M., Verghese, J., Beauchet, O., and Hausdorff, J. M. (2012). Gait and cognition: a complementary approach to understanding brain function and the risk of falling. J. Am. Geriatr. Soc. 60, 2127–2136. doi: 10.1111/j.1532-5415.2012.04209.x
Mori, T., Takeuchi, N., and Izumi, S. I. (2018). Prefrontal cortex activation during a dual task in patients with stroke. Gait Posture 59, 193–198. doi: 10.1016/j.gaitpost.2017.09.032
Moriguchi, Y., and Hiraki, K. (2013). Prefrontal cortex and executive function in young children: a review of NIRS studies. Front. Hum. Neurosci. 7:867. doi: 10.3389/fnhum.2013.00867
Nieuwhof, F., Reelick, M. F., Maidan, I., Mirelman, A., Hausdorff, J. M., Olde Rikkert, M. G., et al. (2016). Measuring prefrontal cortical activity during dual task walking in patients with Parkinson's disease: feasibility of using a new portable fNIRS device. Pilot Feasibility Stud. 2:59. doi: 10.1186/s40814-016-0099-2
Nyman, S. R. (2021). Tai Chi for the prevention of falls among older adults: a critical analysis of the evidence. J. Aging Phys. Act. 29, 343–352. doi: 10.1123/japa.2020-0155
Obrig, H., and Villringer, A. (2003). Beyond the visible-imaging the human brain with light. J. Cereb. Blood Flow Metab. 23, 1–18. doi: 10.1097/01.WCB.0000043472.45775.29
Ohsugi, H., Ohgi, S., Shigemori, K., and Schneider, E. B. (2013). Differences in dual-task performance and prefrontal cortex activation between younger and older adults. BMC Neurosci. 14:10. doi: 10.1186/1471-2202-14-10
Pan, H. F., Hsu, H. C., Chang, W. N., Renn, J. H., and Wu, H. W. (2016). Strategies for obstacle crossing in older adults with high and low risk of falling. J. Phys. Ther. Sci. 28, 1614–1620. doi: 10.1589/jpts.28.1614
Park, D. C., and Reuter-Lorenz, P. (2009). The adaptive brain: aging and neurocognitive scaffolding. Annu. Rev. Psychol. 60, 173–196. doi: 10.1146/annurev.psych.59.103006.093656
Pieruccini-Faria, F., Sarquis-Adamson, Y., and Montero-Odasso, M. (2019). Mild cognitive impairment affects obstacle negotiation in older adults: results from "gait and brain study". Gerontology 65, 164–173. doi: 10.1159/000492931
Pinti, P., Aichelburg, C., Gilbert, S., Hamilton, A., Hirsch, J., Burgess, P., et al. (2018). A review on the use of wearable functional near-infrared spectroscopy in naturalistic environments(). Jpn. Psychol. Res. 60, 347–373. doi: 10.1111/jpr.12206
Raz, N., Gunning-Dixon, F. M., Head, D., Dupuis, J. H., and Acker, J. D. (1998). Neuroanatomical correlates of cognitive aging: evidence from structural magnetic resonance imaging. Neuropsychology 12, 95–114. doi: 10.1037//0894-4105.12.1.95
Reuter-Lorenz, P. A., and Lustig, C. (2005). Brain aging: reorganizing discoveries about the aging mind. Curr. Opin. Neurobiol. 15, 245–251. doi: 10.1016/j.conb.2005.03.016
Reuter-Lorenz, P. A., and Park, D. C. (2014). How does it STAC up? Revisiting the scaffolding theory of aging and cognition. Neuropsychol. Rev. 24, 355–370. doi: 10.1007/s11065-014-9270-9
Roca, M., Torralva, T., Gleichgerrcht, E., Woolgar, A., Thompson, R., Duncan, J., et al. (2011). The role of area 10 (BA10) in human multitasking and in social cognition: a lesion study. Neuropsychologia 49, 3525–3531. doi: 10.1016/j.neuropsychologia.2011.09.003
Rogers, L. J., Zucca, P., and Vallortigara, G. (2004). Advantages of having a lateralized brain. Proc. Biol. Sci. 271, S420–S422. doi: 10.1098/rsbl.2004.0200
Schwenk, M., Zieschang, T., Oster, P., and Hauer, K. (2010). Dual-task performances can be improved in patients with dementia: a randomized controlled trial. Neurology 74, 1961–1968. doi: 10.1212/WNL.0b013e3181e39696
Semendeferi, K., Armstrong, E., Schleicher, A., Zilles, K., and Van Hoesen, G. W. (2001). Prefrontal cortex in humans and apes: a comparative study of area 10. Am. J. Phys. Anthropol. 114, 224–241. doi: 10.1002/1096-8644(200103)114:3<224::Aid-ajpa1022>3.0.Co;2-i
Sheridan, P. L., and Hausdorff, J. M. (2007). The role of higher-level cognitive function in gait: executive dysfunction contributes to fall risk in Alzheimer's disease. Dement. Geriatr. Cogn. Disord. 24, 125–137. doi: 10.1159/000105126
Shumway-Cook, A., Woollacott, M., Kerns, K. A., and Baldwin, M. (1997). The effects of two types of cognitive tasks on postural stability in older adults with and without a history of falls. J. Gerontol. A Biol. Sci. Med. Sci. 52, M232–M240. doi: 10.1093/gerona/52a.4.m232
Song, Q., Li, L., Zhang, C., Sun, W., and Mao, D. (2018). Long-term Tai Chi practitioners have superior body stability under dual task condition during stair ascent. Gait Posture 66, 124–129. doi: 10.1016/j.gaitpost.2018.08.008
Song, Q., Zhang, X., Mao, M., Sun, W., Zhang, C., Chen, Y., et al. (2021). Relationship of proprioception, cutaneous sensitivity, and muscle strength with the balance control among older adults. J. Sport Health Sci. 10, 585–593. doi: 10.1016/j.jshs.2021.07.005
Sun, Z., Herold, F., Cai, K., Yu, Q., Dong, X., Liu, Z., et al. (2022). Prediction of outcomes in mini-basketball training program for preschool children with autism using machine learning models. Int. J. Ment. Health Promot. 24, 143–158. doi: 10.32604/ijmhp.2022.020075
Sun, W., Ma, X., Wang, L., Zhang, C., Song, Q., Gu, H., et al. (2019). Effects of Tai Chi Chuan and brisk walking exercise on balance ability in elderly women: a randomized controlled trial. Mot. Control. 23, 100–114. doi: 10.1123/mc.2017-0055
Sun, W., Wang, L., Zhang, C., Song, Q., Gu, H., and Mao, D. (2018). Detraining effects of regular Tai Chi exercise on postural control ability in older women: a randomized controlled trial. J. Exerc. Sci. Fit. 16, 55–61. doi: 10.1016/j.jesf.2018.06.003
Szczepanski, S. M., and Knight, R. T. (2014). Insights into human behavior from lesions to the prefrontal cortex. Neuron 83, 1002–1018. doi: 10.1016/j.neuron.2014.08.011
Tao, J., Chen, X., Egorova, N., Liu, J., Xue, X., Wang, Q., et al. (2017). Tai Chi Chuan and Baduanjin practice modulates functional connectivity of the cognitive control network in older adults. Sci. Rep. 7:41581. doi: 10.1038/srep41581
Tao, J., Liu, J., Egorova, N., Chen, X., Sun, S., Xue, X., et al. (2016). Increased hippocampus-medial prefrontal cortex resting-state functional connectivity and memory function after Tai Chi Chuan practice in elder adults. Front. Aging Neurosci. 8:25. doi: 10.3389/fnagi.2016.00025
Tinetti, M. E., Speechley, M., and Ginter, S. F. (1988). Risk factors for falls among elderly persons living in the community. N. Engl. J. Med. 319, 1701–1707. doi: 10.1056/nejm198812293192604
Toba, K., Okochi, J., Takahashi, T., Matsubayashi, K., Nishinaga, M., Yamada, S., et al. (2005). Development of a portable fall risk index for elderly people living in the community. Nihon Ronen Igakkai Zasshi 42, 346–352. doi: 10.3143/geriatrics.42.346
Tombu, M., and Jolicoeur, P. (2003). A central capacity sharing model of dual-task performance. J. Exp. Psychol. Hum. Percept. Perform. 29, 3–18. doi: 10.1037//0096-1523.29.1.3
Verghese, J., Holtzer, R., Lipton, R. B., and Wang, C. (2012). Mobility stress test approach to predicting frailty, disability, and mortality in high-functioning older adults. J. Am. Geriatr. Soc. 60, 1901–1905. doi: 10.1111/j.1532-5415.2012.04145.x
Veronese, N., Smith, L., Barbagallo, M., Yang, L., Zou, L., Haro, J. M., et al. (2021). Sarcopenia and fall-related injury among older adults in five low- and middle-income countries. Exp. Gerontol. 147:111262. doi: 10.1016/j.exger.2021.111262
Wang, H., Wei, A., Lu, Y., Yu, B., Chen, W., Lu, Y., et al. (2016). Simplified Tai Chi program training versus traditional Tai Chi on the functional movement screening in older adults. Evid. Based Complement. Alternat. Med. 2016, 5867810–5867816. doi: 10.1155/2016/5867810
Wei, G. X., Dong, H. M., Yang, Z., Luo, J., and Zuo, X. N. (2014). Tai Chi Chuan optimizes the functional organization of the intrinsic human brain architecture in older adults. Front. Aging Neurosci. 6:74. doi: 10.3389/fnagi.2014.00074
Wei, G. X., Xu, T., Fan, F. M., Dong, H. M., Jiang, L. L., Li, H. J., et al. (2013). Can Taichi reshape the brain? A brain morphometry study. PLoS One 8:e61038. doi: 10.1371/journal.pone.0061038
West, R. L. (1996). An application of prefrontal cortex function theory to cognitive aging. Psychol. Bull. 120, 272–292. doi: 10.1037/0033-2909.120.2.272
Wu, Y., Wang, Y., Burgess, E. O., and Wu, J. (2013). The effects of tai chi exercise on cognitive function in older adults: a meta-analysis. J. Sport Health Sci. 2, 193–203. doi: 10.1016/j.jshs.2013.09.001
Yang, Y., Chen, T., Shao, M., Yan, S., Yue, G. H., and Jiang, C. (2019). Effects of tai chi Chuan on inhibitory control in elderly women: an fNIRS study. Front. Hum. Neurosci. 13:476. doi: 10.3389/fnhum.2019.00476
Yao, J., Song, Q., Zhang, K., Hong, Y., Li, W., Mao, D., et al. (2019). The effect of tai chi practice on brain white matter structure: a diffusion tensor magnetic resonance imaging study. Res. Sports Med. 27, 121–130. doi: 10.1080/15438627.2018.1502184
Yogev-Seligmann, G., Hausdorff, J. M., and Giladi, N. (2008). The role of executive function and attention in gait. Mov. Disord. 23:329-342; quiz 472. doi: 10.1002/mds.21720
Yogev-Seligmann, G., Rotem-Galili, Y., Mirelman, A., Dickstein, R., Giladi, N., and Hausdorff, J. M. (2010). How does explicit prioritization alter walking during dual-task performance? Effects of age and sex on gait speed and variability. Phys. Ther. 90, 177–186. doi: 10.2522/ptj.20090043
Yu, Q., Herold, F., Becker, B., Klugah-Brown, B., Zhang, Y., Perrey, S., et al. (2021). Cognitive benefits of exercise interventions: an fMRI activation likelihood estimation meta-analysis. Brain Struct. Funct. 226, 601–619. doi: 10.1007/s00429-021-02247-2
Yu, Q., Herold, F., Ludyga, S., Cheval, B., Zhang, Z., Mücke, M., et al. (2022). Neurobehavioral mechanisms underlying the effects of physical exercise break on episodic memory during prolonged sitting. Complement. Ther. Clin. Pract. 48:101553. doi: 10.1016/j.ctcp.2022.101553
Yue, C., Yu, Q., Zhang, Y., Herold, F., Mei, J., Kong, Z., et al. (2020a). Regular tai chi practice is associated with improved memory as well as structural and functional alterations of the hippocampus in the elderly. Front. Aging Neurosci. 12:586770. doi: 10.3389/fnagi.2020.586770
Yue, C., Zhang, Y., Jian, M., Herold, F., Yu, Q., Mueller, P., et al. (2020b). Differential effects of tai chi Chuan (motor-cognitive training) and walking on brain networks: a resting-state fMRI study in Chinese women aged 60. Healthcare (Basel) 8:67. doi: 10.3390/healthcare8010067
Zhang, Y., Li, C., Zou, L., Liu, X., and Song, W. (2018). The effects of mind-body exercise on cognitive performance in elderly: a systematic review and meta-analysis. Int. J. Environ. Res. Public Health 15:2791. doi: 10.3390/ijerph15122791
Zhang, C., Mao, D., Riskowski, J. L., and Song, Q. (2011). Strategies of stepping over obstacles: the effects of long-term exercise in older adults. Gait Posture 34, 191–196. doi: 10.1016/j.gaitpost.2011.04.008
Zhang, Y., Zou, L., Chen, S. T., Bae, J. H., Kim, D. Y., Liu, X., et al. (2021). Effects and moderators of exercise on Sarcopenic components in Sarcopenic elderly: a systematic review and meta-analysis. Front Med (Lausanne) 8:649748. doi: 10.3389/fmed.2021.649748
Zhang, J., Zou, L., Jiao, C., Zhang, M., Wang, L., Song, W., et al. (2020). Cognitive benefits of activity engagement among 12, 093 adults aged over 65 years. Brain Sci. 10:967. doi: 10.3390/brainsci10120967
Zheng, K., Zou, L., Wei, G., and Huang, T. (2021). Concurrent performance of executive function during acute bouts of exercise in adults: a systematic review. Brain Sci. 11. doi: 10.3390/brainsci11101364
Zhou, J., Chang, S., Cong, Y., Qin, M., Sun, W., Lian, J., et al. (2015). Effects of 24 weeks of tai chi exercise on postural control among elderly women. Res. Sports Med. 23, 302–314. doi: 10.1080/15438627.2015.1040918
Zou, L., Han, J., Li, C., Yeung, A. S., Hui, S. S., Tsang, W. W. N., et al. (2019a). Effects of tai chi on lower limb proprioception in adults aged over 55: a systematic review and meta-analysis. Arch. Phys. Med. Rehabil. 100, 1102–1113. doi: 10.1016/j.apmr.2018.07.425
Zou, L., Huang, T., Tsang, T., Pan, Z., Wang, C., Liu, Y., et al. (2018). Hard martial arts for cognitive function across the lifespan: a systematic review. ARCH BUDO 14, 41–58.
Zou, L., Loprinzi, P. D., Yeung, A. S., Zeng, N., and Huang, T. (2019b). The beneficial effects of mind-body exercises for people with mild cognitive impairment: a systematic review with meta-analysis. Arch. Phys. Med. Rehabil. 100, 1556–1573. doi: 10.1016/j.apmr.2019.03.009
Zou, L., Loprinzi, P. D., Yu, J. J., Yang, L., Li, C., Yeung, A. S., et al. (2019c). Superior effects of modified Chen-style tai chi versus 24-style tai chi on cognitive function, fitness, and balance performance in adults over 55. Brain Sci. 9:102. doi: 10.3390/brainsci9050102
Keywords: Tai Chi, functional near-infrared spectroscopy, dual-task, negotiating obstacle, older adult, prefrontal cortex
Citation: Chen Y, Wan A, Mao M, Sun W, Song Q and Mao D (2022) Tai Chi practice enables prefrontal cortex bilateral activation and gait performance prioritization during dual-task negotiating obstacle in older adults. Front. Aging Neurosci. 14:1000427. doi: 10.3389/fnagi.2022.1000427
Edited by:
Liye Zou, Shenzhen University, ChinaReviewed by:
Yu Qian, University of Macau, ChinaManuel Enrique Hernandez, University of Illinois at Urbana-Champaign, United States
Copyright © 2022 Chen, Wan, Mao, Sun, Song and Mao. This is an open-access article distributed under the terms of the Creative Commons Attribution License (CC BY). The use, distribution or reproduction in other forums is permitted, provided the original author(s) and the copyright owner(s) are credited and that the original publication in this journal is cited, in accordance with accepted academic practice. No use, distribution or reproduction is permitted which does not comply with these terms.
*Correspondence: Yan Chen, MjE1NzU1NTgzQHFxLmNvbQ==; Dewei Mao, bV9kX3dlaUBzaW5hLmNvbQ==
†These authors have contributed equally to this work