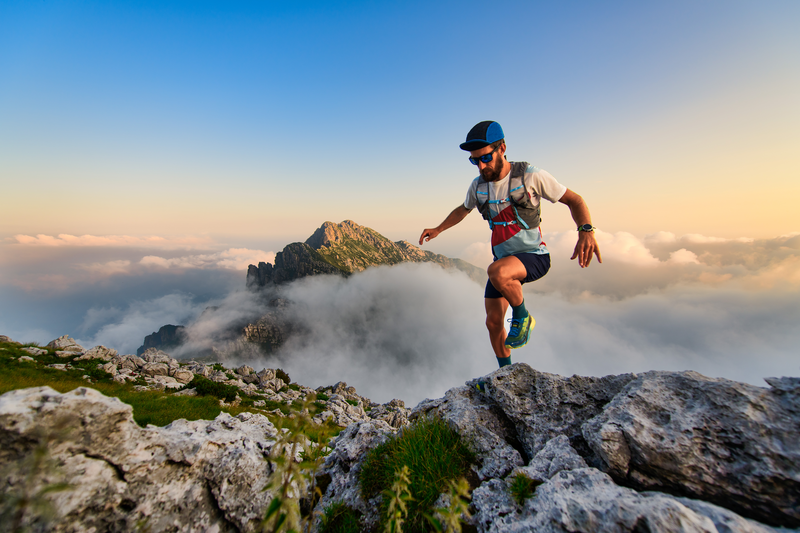
94% of researchers rate our articles as excellent or good
Learn more about the work of our research integrity team to safeguard the quality of each article we publish.
Find out more
ORIGINAL RESEARCH article
Front. Aging Neurosci. , 08 October 2021
Sec. Neuroinflammation and Neuropathy
Volume 13 - 2021 | https://doi.org/10.3389/fnagi.2021.738359
This article is part of the Research Topic New Developments in Understanding Brain and Cerebromicrovascular Aging: Toward Prevention of Vascular Cognitive Impairment and Alzheimer's Disease View all 19 articles
Objectives: This study aimed to investigate the association between plasma von Willebrand factor (VWF) level, ADAMTS13 activity, and neuroimaging features of cerebral small vessel disease (CSVD), including the CSVD neuroimaging markers and the overall CSVD burden.
Methods: CSVD patients admitted to our hospital from 2016 to 2020 were recruited. Plasma VWF level and ADAMTS13 activity were measured. The overall effect of CSVD on the brain was described as a validated CSVD score. We evaluated the association between VWF levels, ADAMTS13 activity, and the increasing severity of CSVD score by the logistic regression model.
Results: We enrolled 296 patients into this study. The mean age of the sample was 69.0 years (SD 7.0). The mean VWF level was 1.31 IU/mL, and the ADAMTS13 activity was 88.01 (SD 10.57). In multivariate regression analysis, lower ADAMTS13 activity and higher VWF level was related to white matter hyperintensity (WMH) [β = −7.31; 95% confidence interval (CI) (−9.40, −4.93); p<0.01; β = 0.17; 95% confidence interval (0.11, 0.23); p<0.01], subcortical infarction (SI) [(β = −9.22; 95% CI (−11.37, −7.06); p<0.01); β = 0.21; 95% confidence interval (0.15, 0.27); p<0.01] independently, but not cerebral microbleed (CMB) [(β = −2.3; 95% CI (−4.95, 0.05); p = 0.22); β = 0.02; 95% confidence interval (−0.05, 0.08); p = 0.63]. Furthermore, ADAMTS13 activity was independently negatively correlated with the overall CSVD burden (odd ratio = 21.33; 95% CI (17.46, 54.60); p < 0.01) after adjustment for age, history of hypertension, and current smoking.
Conclusions: Reducing ADAMTS13 activity change is related to white matter hyperintensity, subcortical infarction, but not with cerebral microhemorrhage. In addition, ADAMTS13 may have played an essential role in the progression of CSVD.
Cerebral small vessel disease (CSVD) is a syndrome with various structural or functional lesions involving perforating vessels that leads to parenchymal injury, which causes clinical, cognitive, neuroimaging, and neuropathological manifestation. Neuroimaging features are diverse so that the total CSVD score was created to capture the overall effect of CSVD on the brain, which incorporates white matter hyperintensity (WMH), cerebral microbleeds (CMB), lacunes, and enlarged perivascular spaces (EPVS; Huijts et al., 2013; Staals et al., 2014). The pathogenesis of the CSVD is not well known, but more evidence suggests that endothelial dysfunction is a crucial link leading to changes in cerebrovascular structure and function in patients with CSVD (Wardlaw et al., 2013; Jackman and Iadecola, 2015). Therefore, searching for endothelial biological markers will provide an essential theoretical basis for in-depth exploration of the pathogenesis of CSVD and the search for new therapeutic targets.
For CSVD, the related markers such as endothelial cell injury, inflammatory response, and coagulation/coagulation markers are well studied, and the connection between homocysteine, asymmetric dimethylarginine (ADMA), von Willebrand factor (VWF), and CSVD has been widely recognized (Wang et al., 2016; Janes et al., 2019; Nam et al., 2019). VWF is considered as a marker of endothelial dysfunction, which was synthesized in endothelial cells and released into the blood circulation via a constitutive pathway (released immediately after completion of molecular synthesis) or a stimulatory regulatory pathway that mediates initial platelet adhesion at sites of vascular injury (Ruggeri, 1999; Lenting et al., 2010). A disintegrin and metalloproteinase with a thrombospondin motif repeat 13 (ADAMTS13) regulate the activity of VWF by cutting ultra-long VWF multimers into smaller, less active molecules and exert its anti-inflammatory and antithrombotic properties (Gerritsen et al., 2001).
Several studies have reported that VWF levels are associated with the degree of WMH and the number of silent subcortical infarctions (Kario et al., 2001; Gottesman et al., 2009), but little is known about the association of VWF and ADAMTS13 activity with subtypes of CSVD and the overall CSVD burden. Therefore, we performed this study to investigate the independence or interactions correlation between multiple CSVD subtypes and the severity of CSVD.
“Investigation on the Status of Cerebrovascular Diseases and Establishing Cohort in Shang Hai Aging Population (ISCDECSHAP)” is a prospective, population-based, and cohort study of stroke incidence and risk factors in an ageing population from Shang Hai City. The ISCDECSHAP study aimed to establish a Chinese CSVD community cohort and was approved by the ethics committee of Hua Shan Hospital and the Fifth People’s Hospital of Shanghai. Written informed consent was obtained from all the patients or their representatives before data collection (Yang et al., 2019). Based on protocol, all the subjects, at least 60 years old, performed cerebral magnetic resonance imaging (MRI), cerebral MRA, carotid artery ultrasound, cognitive function, and hematologic examination. Demographic and clinical data, including gender, age, hypertension, diabetes, smoking history, drinking history, and other health conditions were collected by neurologists through a standardized questionnaire. Three milliliters of fasting venous blood was drawn from the study subjects in the morning, all of which was completed within 1 week. According to the purpose of this study, our inclusion criteria: ≥60 years old CSVD patients; no neurological symptoms and signs; previous experience of non-specific neurological symptoms such as dizziness, vertigo, headache, and tinnitus can be included (the above symptoms should be completely relieved when evaluating the inclusion). Patients who met any one of the following criteria were excluded: history of stroke, cognitive dysfunction, heart disease, malignancies, hepatic or renal diseases, autoimmune diseases, or infection at enrollment. Contents are shown in Figure 1.
Brain MRI imaging sequences include T1-weighted images (T1WI), T2-weighted images (T2WI), fluid-attenuated inversion recovery (FLAIR), diffusion-weighted imaging (DWI), and susceptibility-weighted imaging (SWI). The field of view (FOV) is 240 mm × 240 mm, and the matrix = 320 × 256. Coronal and transaxial views of T1WI and T2WI, and transaxial views of T2-FLAIR, DWI, and SWI were collected. The imaging diagnosis of CSVD strictly follows the international CSVD guidelines (Wardlaw et al., 2013). Subcortical lesions (SI): round or oval lesions, 3–15 mm in diameter, with cerebrospinal fluid signal intensity on T1 and T2 sequences, isointensity on DWI, hypointensity on FLAIR, and ring hyperintensity in the periphery. Perivascular space enlargement (EPVS): round or oval, and usually ≤2 mm in diameter, most pronounced in the basal ganglia, with the cerebrospinal fluid signal on T1 and T2 sequences, isointensity on DWI, and hypointensity on FLAIR. White matter hyperintensity (WMH): abnormal signals of varying sizes in periventricular and deep white matter regions, which are hyperintense on T2, FLAIR, and isointense or hypointense on T1. Cerebral microbleed (CMB): it is round on SWI sequence, less than 10 mm in diameter, and hypointense. WMH was graded using a semi-quantitative Fazekas scale (Hainsworth et al., 2015), and high WMH was defined if the scale scored 2–3. According to Staals et al. (2014), the overall burden score of CSVD was rewarded with 1 point whenever one of the following occurred: ≥1 SI (<15 mm); ≥1 CMBs (<5 mm); moderate-severe basal ganglia PVS (semiquantitative scale 2–4 grades; Maclullich et al., 2004); deep WMH (Fazekas score 2/3) or periventricular WMH (Fazekas score 3). The total score of CSVD is 0–4 points, a higher score indicating that the CSVD is more severe. The MRI was independently evaluated by two neurologists, and inter-observer agreement values for the presence of CMB, WMH, EPVS, and lacune were 0.80, 0.83, 0.78, and 0.79, respectively. Any disagreement regarding the presence of CSVD features was resolved by consensus with the third neuroimaging expert.
Human VWF ELISA kit (Abcam) was used to quantitatively measure the VWF level directly in human fasting venous plasma samples obtained on admission. The samples were centrifuged (2,000 g for 5 min) immediately after collection, and plasma samples were stored in a −80 refrigerator. The assay was a sandwich ELISA and measurements were conducted according to the manufacturer’s guidelines. ADAMTS13 enzyme activity was measured by the fluorescent substrate method, AnaSpecUSA provided FRETS-VWF73 (ADAMTS13 fluorescent substrate), and the percentage of enzyme activity indicated that the standard plasma activity was set at 100. The operation steps were performed according to the assay (Kokame et al., 2005). Standard plasma is a mixture of plasma from 20 healthy subjects and provided by the Physical Examination Center of Shanghai Fifth People’s Hospital.
SPSS 21.0 statistical software was applied for data analysis. For each demographic and clinical feature, normal distribution continuous variables were presented as mean ± standard deviation and compared by an independent sample t-test. Abnormal distribution continuous variables were presented as median (interquartile range) and compared by a nonparametric test. The categorical variables are expressed in frequency (percentage) and are expressed in χ2 test or Fisher exact test. Associations between circulating biomarker (ADAMTS13 and VWF) levels and neuroimaging markers of CSVD and overall CSVD burden was performed by regression analysis. Multivariable linear regression was then conducted for each marker of CSVD in separate models adjusting for age, sex, hypertension, diabetes, smoking history, drinking history, and all brain variables. Kendall’s tau-b correlation analysis was used to evaluate the correlation between the ADAMTS13 activity and the total CSVD score. For logistic regression models, age, sex, and variables showing a p < 0.1 on the respective univariate analyses were included in models. A p-value of 0.05 was considered significant.
A total of 296 patients with CSVD were included in this study, of which 125 were male. The mean age of the sample was s 69.0 years (SD 7.0), the median VWF level was 1.28 (1.01–1.50) IU/ml, and the ADAMTS13 activity was 88.04% (SD10.57). SI accounted for 42.3% (n = 125) of the total sample, CMB for 28.0% (n = 83), and high WMH (Fazekas score of 2–3) for 41.6% (n = 123). SI has higher total cholesterol than no SI (p < 0.01). We found that the high WMH and SI group had significantly increased VWF levels (p < 0.01) and decreased ADAMTS13 activity (p < 0.01) compared with the control group, but there was no significant difference in the changes of the above parameters in the CMB group (Table 1).
The association between different CSVD neuroimaging markers and ADAMTS13 activity was investigated. Adjusted for age, sex, alcohol, smoking, hypertension, and diabetes, lower ADAMTS13 activity and higher VWF level were related with high WMH [β= −6.26; 95% CI (−7.55, −2.82) p<0.01; β = 0.18; 95% CI (0.12, 0.25); p<0.01] and SI [β = −5.19; 95% CI (−7.55, −2.82) p<0.01; β = 0.13; 95% CI (0.07, 0.20); p<0.01]. Model II includes all brain variables, The presence of SI and WMH still remain associated with lower ADAMTS13 activity and higher VWF level (Table 2; Supplementary Table 1). In addition, we calculated the ADAMTS13:VWF ratio; the results showed that compared with ADAMTS13 alone, ADAMTS13:VWF ratio had a more pronounced association with SI [β = −23.24; 95% CI (−29.31, −17.16); p<0.01] and WMH [β = −17.35; 95% CI (−24.35, −10.35); p < 0.01] (Table 3). The final ADAMTS13:VWF model explained 32.3% (adjusted R squared) of the variance, which was higher than the 28.2% (adjusted R squared) of the ADAMTS13-only model in this study.
Patients were classified into four groups by ADAMTS13 activity and VWF level quartiles, respectively. In our study population, approximately half of the CSVD total score of patients was 1 (26.3%) or 2 (32.1%). A number of patients scored 3 (28.7%) and 4 (16.2%). Of the patients with a CSVD score of 4, the majority had ADAMTS13 activity (78.8%) among ADAMTS13Q1<80.3%. In addition, the correlation analysis shows that the ADAMTS13 activity was negatively related to the total CSVD score (Kendall’s tau-b = −0.48, p<0.01; Table 4). In the adjusted statistical model (adjusted for age, sex, alcohol use, current smoking, hypertension, and diabetes mellitus), the increase in total CSVD score was negatively correlated with ADAMTS13Q1<80.3% (OR = 21.33, 95% CI (17.46, 54.60) p < 0.01) and ADAMTS13Q280.3–89.0% (OR = 2.89, 95% CI (1.75, 17.50) p < 0.05), respectively. Moreover, lower VWF levels (VWFQ1<1.14 IU/ml) can be regarded as a protective factor for CSVD (OR = 0.37, 95% CI (0.20, 0.66) p < 0.01; Table 5).
In our study, we investigated the association between plasma VWF level, ADAMTS13 activity, and neuroimaging features of CSVD, including the overall CSVD burden. After adjustment for established CSVD-related risk factors, we confirmed that ADAMTS13 activity was reversely correlated to WMH, SI, and overall CSVD burden independently.
The prevalence of CSVD in the elderly population over 60 years can reach 60%. Studies have shown that about 45% of dementia and 20% of stroke is caused by CSVD (Sudlow and Warlow, 1997; Gorelick et al., 2011). Furthermore, CSVD may double the risk of recurrent stroke (Debette and Markus, 2010). In recent years, large population-based studies report that low ADAMTS13 activity was correlated with a risk of ischemic stroke (Sonneveld et al., 2015) and dementia (Wolters et al., 2018) independent of other known demographic and cerebrovascular risk factors, but studies on the relationship between ADAMTS13 activity and CSVD are little. The association between VWF and neuroimaging markers of CSVD has been demonstrated, including silent lacunar cerebral infarcts, periventricular hyperintensity, and deep white matter hyperintensity (Kario et al., 2001; Gottesman et al., 2009; Nagai et al., 2012). However, it has not reflected changes between VWF with the overall CSVD burden. In our study, we demonstrated that higher VWF level and lower ADAMTS13 activity correlated with WMH and SI, but not with CMB after adjusting for traditional risk factors and brain variables. The complex mechanism involved is unknown, but there are several possible explanations as follows.
First, low ADAMTS13 activity may be associated with endothelial cell dysfunction. Endothelial dysfunction may lead to increased permeability of the blood-brain barrier (BBB), which not only interrupts the oxygen and nutrient supply to the brain but also leads to extravasation of blood components that damage the surrounding white matter regions (Zlokovic, 2008; Gorelick et al., 2011; Xu et al., 2015). Cao et al. (2019) observed that ADAMTS13-deficient mice promote BBB disruption and reduce microvascular and capillary perfusion, and cerebral blood flow by changing endothelial junctions. However, there is a tight link between WMH and BBB impairment that plays a crucial role in early white matter degeneration (Kerkhofs et al., 2021). For SI, its underlying mechanism may have partially overlapping pathophysiology with WMH, but somewhat different (Wardlaw et al., 2015; Lin et al., 2017). VWF, an indicator of endothelial dysfunction, is the first step in mediated adhesion of platelets to damaged endothelial cells in thrombus formation. When endothelial cells are dysfunctional, VWF will be released in the form of large-molecular-weight multimers, which aggravate vascular damage (Lip and Blann, 1997). ADAMTS13 alleviates thrombogenesis and inflammation by adjusting VWF size and regulates microvascular thrombosis by altering the interaction of VWF with platelets (Pillai et al., 2016; Gogia et al., 2017). A study has reported that increased levels of functional VWF accelerate the formation of more microthrombi in multiple SI elderly patients (Kario et al., 2001). In this study, we obtained similar results in VWF and found that low ADAMTS13 activity was an independent risk factor for multiple SIs, and there was no association between ADAMTS13 activity and VWF levels, similar to previous studies (Andersson et al., 2012; Sonneveld et al., 2015; Denorme et al., 2017).The possible reason is that low local ADAMTS13 activity is not sufficient to cleave the supramaximal VWF multimers, eventually leading to the formation of microthrombiin microvascular injury. A second possible reason is that ADAMTS13 activity accelerates atherosclerosis. In animal models, lack of ADAMTS13 was found to promote the formation of plaques and vascular inflammation by generating signals for recruitment and extravasation of monocytes in the early stages of atherosclerosis, which are the pathological changes associated with CSVD (Gandhi et al., 2012; Jin et al., 2012). Ultimately, this may trigger or worsen the development of CSVD.
Furthermore, we confirmed that the severity of the overall CSVD burden was negatively correlated with ADAMTS13 activity. In another study, Nezu et al. (2015) observed that endothelial dysfunction was related to the severity of CSVD by using flow-mediated dilation (FMD) to measure endothelium-dependent vasodilation. However, ADAMTS13 was shown to be able to augment microvascular endothelial endothelium-dependent dilation (EDR) dependent on PI3K/Akt pathway in vivo (Zhou et al., 2019). Therefore, this implies ADAMTS13 may have played an essential role in the development of CSVD.
The innovation of this study is that we first reported the association between plasma VWF level, ADAMTS13 activity, and neuroimaging features of CSVD, including the overall CSVD burden in the community cohort. However, there are some limitations as follows. First, this study is a single-center cross-sectional study, with a relatively small number of study subjects and the included biological marker indicators are not comprehensive enough. Because we could not integrate multiple CSVD biomarkers into a panel, it will help us understand the pathogenesis of CSVD better. Second, the activity of ADAMTS13 was also demographically statistically different among different races and regions, and the subjects we included were all from Shanghai, China, so our results need to be validated in a more extensive prospective study.
In conclusion, reducing ADAMTS13 activity is related to white matter hyperintensity and subcortical infarction, but not with cerebral microhemorrhage. In addition, this implied that ADAMTS13 may have played an essential role in the development of CSVD. Exploring the differences of various biomarkers between various neuroimaging features of CSVD, and the association of these markers with clinical manifestations of CSVD will help to deeply elucidate the pathogenesis of CSVD and may provide a new theoretical basis and new targets for the early prevention and treatment of CSVD.
The raw data supporting the conclusions of this article will be made available by the authors, without undue reservation.
The studies involving human participants were reviewed and approved by Shanghai Fifth People’s Hospital, Fudan University. The patients/participants provided their written informed consent to participate in this study.
WS: conceptualization, data collection, experiment, formal analysis, and writing original draft. SZ and YL: data collection. WL: validation, supervision, and experiment. XY and DW: review and editing, provide guidance. All authors contributed to the article and approved the submitted version.
This study was supported by grants from correlation between retinal vascular changes and cerebral small vessel disease of Shanghai Fifth People’s Hospital (2018WYZD01), Shanghai Committee of Science and Technology (201409004900 and 20S31904400), Cooperation Programme of Fudan University—Minhang District Joint Health Center (2021FM22), Elite training of Shanghai Fifth People’s Hospital (2020WYRCJY04), Beijing Bethune Charitable Foundation (AX083CS), and Minhang District High Level Specialist Backbone Physician Training Plan (2020MZYS09).
The authors declare that the research was conducted in the absence of any commercial or financial relationships that could be construed as a potential conflict of interest.
All claims expressed in this article are solely those of the authors and do not necessarily represent those of their affiliated organizations, or those of the publisher, the editors and the reviewers. Any product that may be evaluated in this article, or claim that may be made by its manufacturer, is not guaranteed or endorsed by the publisher.
We gratefully thank all the patients, general practitioners, and hospital colleagues who participated in our study.
The Supplementary Material for this article can be found online at: https://www.frontiersin.org/articles/10.3389/fnagi.2021.738359/full#supplementary-material.
Andersson, H. M., Siegerink, B., Luken, B. M., Crawley, J. T., Algra, A., Lane, D. A., et al. (2012). High VWF, low ADAMTS13 and oral contraceptives increase the risk of ischemic stroke and myocardial infarction in young women. Blood 119, 1555–1560. doi: 10.1182/blood-2011-09-380618
Cao, Y., Xu, H., Zhu, Y., Shi, M. J., Wei, L., Zhang, J., et al. (2019). ADAMTS13 maintains cerebrovascular integrity to ameliorate Alzheimer-like pathology. PLoS Biol. 17:e3000313. doi: 10.1371/journal.pbio.3000313
Debette, S., and Markus, H. S. (2010). The clinical importance of white matter hyperintensities on brain magnetic resonance imaging: systematic review and meta-analysis. BMJ 341:c3666. doi: 10.1136/bmj.c3666
Denorme, F., Kraft, P., Pareyn, I., Drechsler, C., Deckmyn, H., Vanhoorelbeke, K., et al. (2017). Reduced ADAMTS13 levels in patients with acute and chronic cerebrovascular disease. PLoS One 12:e0179258. doi: 10.1371/journal.pone.0179258
Gandhi, C., Khan, M. M., Lentz, S. R., and Chauhan, A. K. (2012). ADAMTS13 reduces vascular inflammation and the development of early atherosclerosis in mice. Blood 119, 2385–2391. doi: 10.1182/blood-2011-09-376202
Gerritsen, H. E., Robles, R., Lämmle, B., and Furlan, M. (2001). Partial amino acid sequence of purified von Willebrand factor-cleaving protease. Blood 98, 1654–1661. doi: 10.1182/blood.v98.6.1654
Gogia, S., Kelkar, A., Zhang, C., Dayananda, K. M., and Neelamegham, S. (2017). Role of calcium in regulating the intra- and extracellular cleavage of von Willebrand factor by the protease ADAMTS13. Blood Adv. 1, 2063–2074. doi: 10.1182/bloodadvances.2017009027
Gorelick, P. B., Scuteri, A., Black, S. E., Decarli, C., Greenberg, S. M., Iadecola, C., et al. (2011). Vascular contributions to cognitive impairment and dementia:a statement for healthcare professionals from the american heart association/american stroke association. Stroke 42, 2672–2713. doi: 10.1161/STR.0b013e3182299496
Gottesman, R. F., Cummiskey, C., Chambless, L., Wu, K. K., Aleksic, N., Folsom, A. R., et al. (2009). Hemostatic factors and subclinical brain infarction in a community-based sample:the ARIC study. Cerebrovasc Dis. 28, 589–594. doi: 10.1159/000247603
Hainsworth, A. H., Oommen, A. T., and Bridges, L. R. (2015). Endothelial cells and human cerebral small vessel disease. Brain Pathol. 25, 44–50. doi: 10.1111/bpa.12224
Huijts, M., Duits, A., van Oostenbrugge, R. J., Kroon, A. A., de Leeuw, P. W., and Staals, J. (2013). Accumulation of MRI markers of cerebral small vessel disease is associated with decreased cognitive function. A study in first-ever lacunar stroke and hypertensive patients. Front Aging Neurosci 5:72. doi: 10.3389/fnagi.2013.00072
Jackman, K., and Iadecola, C. (2015). Neurovascular regulation in the ischemic brain. Antioxid. Redox Signal. 22, 149–160. doi: 10.1089/ars.2013.5669
Janes, F., Cifù, A., Pessa, M. E., Domenis, R., Gigli, G. L., Sanvilli, N., et al. (2019). ADMA as a possible marker of endothelial damage. a study in young asymptomatic patients with cerebral small vessel disease. Sci. Rep. 9:14207. doi: 10.1038/s41598-019-50778-w
Jin, S. Y., Tohyama, J., Bauer, R. C., Cao, N. N., Rader, D. J., and Zheng, X. L. (2012). Genetic ablation of Adamts13 gene dramatically accelerates the formation of early atherosclerosis in a murine model. Arterioscler. Thromb. Vasc. Biol. 32, 1817–1823. doi: 10.1161/ATVBAHA.112.247262
Kario, K., Matsuo, T., Kobayashi, H., Hoshide, S., and Shimada, K. (2001). Hyperinsulinemia and hemostatic abnormalities are associated with silent lacunar cerebral infarcts in elderly hypertensive subjects. J. Am Coll. Cardiol. 37, 871–877. doi: 10.1016/s0735-1097(00)01172-4
Kerkhofs, D., Wong, S. M., Zhang, E., Staals, J., Jansen, J. F. A., van Oostenbrugge, R. J., et al. (2021). Baseline blood-brain barrier leakage and longitudinal microstructural tissue damage in the periphery of white matter hyperintensities. Neurology 96, e2192–e2200. doi: 10.1212/WNL.0000000000011783
Kokame, K., Nobe, Y., Kokubo, Y., Okayama, A., and Miyata, T. (2005). FRETS-VWF73, a first fluorogenic substrate for ADAMTS13 assay. Br. J. Haematol. 129, 93–100. doi: 10.1111/j.1365-2141.2005.05420.x
Lenting, P. J., Pegon, J. N., Groot, E., and de Groot, P. G. (2010). Regulation of von Willebrand factor-platelet interactions. Thromb. Haemost. 104, 449–455. doi: 10.1160/TH09-11-0777
Lin, J., Wang, D., Lan, L., and Fan, Y. (2017). Multiple factors involved in the pathogenesis of white matter lesions. Biomed. Res. Int. 2017:9372050. doi: 10.1155/2017/9372050
Lip, G. Y., and Blann, A. (1997). von Willebrand factor:a marker of endothelial dysfunction in vascular disorders. Cardiovasc. Res. 34, 255–265. doi: 10.1016/s0008-6363(97)00039-4
Maclullich, A. M., Wardlaw, J. M., Ferguson, K. J., Starr, J. M., Seckl, J. R., and Deary, I. J. (2004). Enlarged perivascular spaces are associated with cognitive function in healthy elderly men. J. Neurol. Neurosurg. Psychiatry 75, 1519–1523. doi: 10.1136/jnnp.2003.030858
Nagai, M., Hoshide, S., and Kario, K. (2012). Association of prothrombotic status with markers of cerebral small vessel disease in elderly hypertensive patients. Am. J. Hypertens. 25, 1088–1094. doi: 10.1038/ajh.2012.85
Nam, K. W., Kwon, H. M., Jeong, H. Y., Park, J. H., Kwon, H., and Jeong, S. M. (2019). Serum homocysteine level is related to cerebral small vessel disease in a healthy population. Neurology 92, e317–e325. doi: 10.1212/WNL.0000000000006816
Nezu, T., Hosomi, N., Aoki, S., Kubo, S., Araki, M., Mukai, T., et al. (2015). Endothelial dysfunction is associated with the severity of cerebral small vessel disease. Hypertens. Res. 38, 291–297. doi: 10.1038/hr.2015.4
Pillai, V. G., Bao, J., Zander, C. B., McDaniel, J. K., Chetty, P. S., Seeholzer, S. H., et al. (2016). Human neutrophil peptides inhibit cleavage of von Willebrand factor by ADAMTS13:a potential link of inflammation to TTP. Blood 128, 110–119. doi: 10.1182/blood-2015-12-688747
Ruggeri, Z. M. (1999). Structure and function of von Willebrand factor. Thromb. Haemost 82, 576–584.
Sonneveld, M. A., de Maat, M. P., Portegies, M. L., Kavousi, M., Hofman, A., Turecek, P. L., et al. (2015). Low ADAMTS13 activity is associated with an increased risk of ischemic stroke. Blood 126, 2739–2746. doi: 10.1182/blood-2015-05-643338
Staals, J., Makin, S. D., Doubal, F. N., Dennis, M. S., and Wardlaw, J. M. (2014). Stroke subtype, vascular risk factors and total MRI brain small-vessel disease burden. Neurology 83, 1228–1234. doi: 10.1212/WNL.0000000000000837
Sudlow, C. L., and Warlow, C. P. (1997). Comparable studies of the incidence of stroke and its pathological types. Results from an international collaboration. Stroke 28, 491–499. doi: 10.1161/01.str.28.3.491
Wang, X., Chappell, F. M., Valdes Hernandez, M., Lowe, G., Rumley, A., Shuler, K., et al. (2016). Endothelial function, inflammation, thrombosis and basal ganglia perivascular spaces in patients with stroke. J. Stroke Cerebrovasc. Dis. 25, 2925–2931. doi: 10.1016/j.jstrokecerebrovasdis.2016.08.007
Wardlaw, J. M., Smith, E. E., Biessels, G. J., Cordonnier, C., Fazekas, F., Frayne, R., et al. (2013). Neuroimaging standards for research into small vessel disease and its contribution to ageing and neurodegeneration. Lancet. Neurol. 12, 822–838. doi: 10.1016/S1474-4422(13)70124-8
Wardlaw, J. M., Valdés Hernández, M. C., and Muñoz-Maniega, S. (2015). What are white matter hyperintensities made of? Relevance to vascular cognitive impairment. J. Am. Heart Assoc. 4:001140. doi: 10.1161/JAHA.114.001140
Wolters, F. J., Boender, J., de Vries, P. S., Sonneveld, M. A., Koudstaal, P. J., de Maat, M. P., et al. (2018). Von Willebrand factor and ADAMTS13 activity in relation to risk of dementia:a population-based study. Sci. Rep. 8:5474. doi: 10.1038/s41598-018-23865-7
Xu, X., Chan, K. W., Knutsson, L., Artemov, D., Xu, J., Liu, G., et al. (2015). Dynamic glucose enhanced (DGE) MRI for combined imaging of blood-brain barrier break down and increased blood volume in brain cancer. Magn. Reson. Med. 74, 1556–1563. doi: 10.1002/mrm.25995
Yang, X., Zhang, S., Dong, Z., Zi, Y., Luo, Y., Jin, Z., et al. (2019). Insulin resistance is a risk factor for overall cerebral small vessel disease burden in old nondiabetic healthy adult population. Front. Aging Neurosci. 11:127. doi: 10.3389/fnagi.2019.00127
Zhou, S., Jiang, S., Guo, J., Xu, N., Wang, Q., Zhang, G., et al. (2019). ADAMTS13 protects mice against renal ischemia-reperfusion injury by reducing inflammation and improving endothelial function. Am. J. Physiol. Renal Physiol. 316, F134–F145. doi: 10.1152/ajprenal.00405.2018
Keywords: cerebral small vessel disease, ADAMTS13, von Willebrand factor, overall cerebral small vessel disease burden, white matter hyperintensity (WMH), subcortical infarction
Citation: Sun W, Luo Y, Zhang S, Lu W, Liu L, Yang X and Wu D (2021) The Relationship Between ADAMTS13 Activity and Overall Cerebral Small Vessel Disease Burden: A Cross-Sectional Study Based on CSVD. Front. Aging Neurosci. 13:738359. doi: 10.3389/fnagi.2021.738359
Received: 08 July 2021; Accepted: 23 August 2021;
Published: 08 October 2021.
Edited by:
Stefano Tarantini, University of Oklahoma Health Sciences Center, United StatesReviewed by:
Peter J. Toth, University of Pécs, HungaryCopyright © 2021 Sun, Luo, Zhang, Lu, Liu, Yang and Wu. This is an open-access article distributed under the terms of the Creative Commons Attribution License (CC BY). The use, distribution or reproduction in other forums is permitted, provided the original author(s) and the copyright owner(s) are credited and that the original publication in this journal is cited, in accordance with accepted academic practice. No use, distribution or reproduction is permitted which does not comply with these terms.
*Correspondence: Danhong Wu, ZGFuaG9uZ3d1QGZ1ZGFuLmVkdS5jbg==; Xiaoli Yang, eGlhb2xpeWFuZ3Nsc0BzaW5hLmNvbQ==
Disclaimer: All claims expressed in this article are solely those of the authors and do not necessarily represent those of their affiliated organizations, or those of the publisher, the editors and the reviewers. Any product that may be evaluated in this article or claim that may be made by its manufacturer is not guaranteed or endorsed by the publisher.
Research integrity at Frontiers
Learn more about the work of our research integrity team to safeguard the quality of each article we publish.