- 1Shandong Collaborative Innovation Center for Diagnosis, Treatment & Behavioral Interventions of Mental Disorders, Institute of Mental Health, Jining Medical University, Jining, China
- 2Shandong Key Laboratory of Behavioral Medicine, School of Mental Health, Jining Medical University, Jining, China
- 3Key Laboratory of Alzheimer’s Disease of Zhejiang Province, Institute of Aging, School of Mental Health and The Affiliated Kangning Hospital, Wenzhou Medical University, Wenzhou, China
- 4Oujiang Laboratory, Wenzhou, China
Orexinergic system consisting of orexins and orexin receptors plays an essential role in regulating sleep–wake states, whereas sleep disruption is a common symptom of a number of neurodegenerative diseases. Emerging evidence reveals that the orexinergic system is disturbed in various neurodegenerative diseases, including Alzheimer’s disease (AD), Parkinson’s disease (PD), Huntington’s disease (HD), and multiple sclerosis (MS), whereas the dysregulation of orexins and/or orexin receptors contributes to the pathogenesis of these diseases. In this review, we summarized advanced knowledge of the orexinergic system and its role in sleep, and reviewed the dysregulation of the orexinergic system and its role in the pathogenesis of AD, PD, HD, and MS. Moreover, the therapeutic potential of targeting the orexinergic system for the treatment of these diseases was discussed.
Introduction
Orexinergic system is essential for the maintenance of various physiological processes including sleep–wake states (Gamble et al., 2019; Yukitake et al., 2019; Zhang et al., 2019). Emerging evidence reveals that the orexinergic system is disturbed in various neurodegenerative diseases, such as Alzheimer’s disease (AD), Parkinson’s disease (PD), Huntington’s disease (HD), and multiple sclerosis (MS), whereas the dysregulation of the orexinergic system plays a pivotal role in the pathogenesis of these diseases (Petersen et al., 2005; Asai et al., 2009; Fatemi et al., 2016; Mander et al., 2016; Liguori, 2017). To gain a better understanding of the therapeutic potential of targeting the orexinergic system for the treatment of these diseases, we extensively reviewed the characteristics of the orexinergic system, its function in sleep–wake states, and its role in the pathogenesis of the neurodegenerative diseases and underlying mechanisms.
Overview of Orexinergic System
Orexinergic system consists of orexins and their receptors. Orexin, also named as hypocretin, includes two isoforms, namely, orexin A (OXA) or hypocretin-1 (HCRT-1) and orexin B (OXB) or hypocretin-2 (HCRT-2) (de Lecea et al., 1998; Sakurai et al., 1998; Soya and Sakurai, 2020). OXA and OXB are generated from the same prepro-orexin precursor via differential hydrolysis (Gotter et al., 2012). OXA is a ∼3.5 kDa peptide with 33 amino acids, whereas OXB is a ∼2.9 kDa peptide with 28 amino acids. Both of them are highly conserved in mammalian species (Sakurai et al., 1998; Dyer et al., 1999; Spinazzi et al., 2006). Orexins are mainly expressed in lateral hypothalamic neurons (Wang et al., 2018). Orexin receptors, including orexin 1 receptor (OX1R) and orexin 2 receptor (OX2R), are G-protein-coupled receptors (GPCRs) (de Lecea et al., 1998; Sakurai et al., 1998; Soya and Sakurai, 2020). OX1R is mainly expressed in ventromedial hypothalamic nucleus, dorsal raphe, locus coeruleus, and hippocampus, whereas OX2R is highly expressed in nucleus accumbens, anterior pretectal nucleus, and cerebral cortex (Trivedi et al., 1998; Soya et al., 2013). Both of them are highly conserved in mammals, for example, human OX1R and OX2R share 94 and 95% identity with rat OX1R and OX2R, respectively. Human OX1R and OX2R consist of 425 and 444 amino acids, respectively, and they share approximately 64% sequence identity (Spinazzi et al., 2006). OXA binds to both OX1R and OX2R with similar affinity (Gotter et al., 2012; Wang et al., 2018), whereas OXB prefers to bind to OX2R (Gotter et al., 2012; Wang et al., 2018).
The main function of the orexinergic system is to regulate sleep–wake states (Adamantidis et al., 2007; Gamble et al., 2019; Yukitake et al., 2019; Zhang et al., 2019). Orexin deficiency is the proximal cause of human narcolepsy with cataplexy, which has been comprehensively studied and reviewed (Bassetti et al., 2019; Nepovimova et al., 2019). Consistently, OX2R agonist YNT-185 ameliorates narcolepsy symptoms in a mouse model of narcolepsy-cataplexy (Irukayama-Tomobe et al., 2017). Photostimulation of orexin neurons induces the transition of sleep to wake in mice, indicating that orexin neurons play a key role in promoting sleep-to-wake transition (Adamantidis et al., 2007). Activation of orexin neurons leads to a marked increase of wakefulness time and a reduction of sleep time including both rapid eye movement (REM) sleep and non-REM (NREM) sleep in mice (Sasaki et al., 2011). Consistently, OX2R agonist YNT-185 markedly increases wakefulness time in mice (Irukayama-Tomobe et al., 2017).
Orexinergic System in Neurodegenerative Diseases
Orexinergic System in AD
AD, the most common neurodegenerative disease, is characterized by the clinical manifestations such as progressive memory loss, cognitive deficits, and sleep disruption. Neuritic plaques, neurofibrillary tangles, and neuronal loss are the neuropathological hallmarks of AD. β-Amyloid (Aβ) and hyperphosphorylated Tau are the core components of neuritic plaques and neurofibrillary tangles, respectively (Lane et al., 2018; Qiu et al., 2019).
Although studies indicate that the orexin levels in cerebrospinal fluid (CSF) are associated with circadian alteration in AD and the OXA levels are positively correlated with the cognitive function in AD (Liguori et al., 2020; Shimizu et al., 2020), the dysregulation of the orexinergic system in AD remains inconclusive. For example, a significant reduction of OXA positive neurons and the levels of OXA were observed in patients with AD (Fronczek et al., 2012), whereas both Slats et al. and Liguori et al. reported that there was no difference of OXA in CSF of patients with AD and healthy controls (Slats et al., 2012; Liguori et al., 2014). In addition, Gabelle et al. reported that OXA was increased in AD (Gabelle et al., 2017). The inconsistent results might result from the different methods and sample sizes. For example, studies by Fronczek et al. and Gabelle et al. were performed in postmortem ventricular CSF and the stored CSF samples, respectively (Fronczek et al., 2012; Gabelle et al., 2017), whereas the participants were awaked in studies performed by Slats et al. and Liguori et al., respectively (Slats et al., 2012; Liguori et al., 2014). Moreover, increased orexin along with more fragmented sleep was observed in patients with AD with neuropsychiatric symptoms compared with that in patients with AD without neuropsychiatric symptoms (Liguori et al., 2018).
Orexinergic system is implicated in Aβ pathology. First, the levels of OXA were correlated with the level of Aβ42 in patients with AD (Slats et al., 2012; Gabelle et al., 2017; Figure 1A). Importantly, orexin deficiency markedly decreased Aβ pathology in AD model mice, amyloid precursor protein/presenilin 1 (APP/PS1) transgenic mice (Roh et al., 2014). Moreover, sleep disruption, a common symptom of AD, facilitated Aβ accumulation, contributing to neurodegeneration in AD, which might be correlated with the alteration of the orexinergic system (Mander et al., 2016; Liguori, 2017; Figure 1A). Reduced Aβ1–42 was correlated with the decrease of REM sleep and sleep efficiency in AD, whereas the increase of OXA in CSF was correlated with the decrease of REM and sleep efficiency in AD (Liguori et al., 2014). The diurnal variation of Aβ levels in interstitial fluid (ISF) was observed in human. Consistently, the Aβ levels in ISF were positively correlated with the awaked time and negatively correlated with the asleep time in mice (Kang et al., 2009; Figure 1A). In addition, the rate of Aβ1–40 clearance was much faster in sleeping mice than that in awaked mice (Xie et al., 2013). Furthermore, the administration of orexin significantly increased the Aβ levels in ISF during the light phase and orexin receptors antagonist led to the inhibition of the diurnal fluctuation of Aβ in ISF of AD model mice (Kang et al., 2009). On the other hand, increased Aβ contributes to the dysregulation of the orexinergic system and sleep disruption. For example, the administration of Aβ25–35 markedly increased awaked time and reduced NREM sleep in AD mice (Liu et al., 2019; Figure 1A). Aβ25–35 significantly increased the level of OXA in AD mice (Liu et al., 2019; Figure 1A).
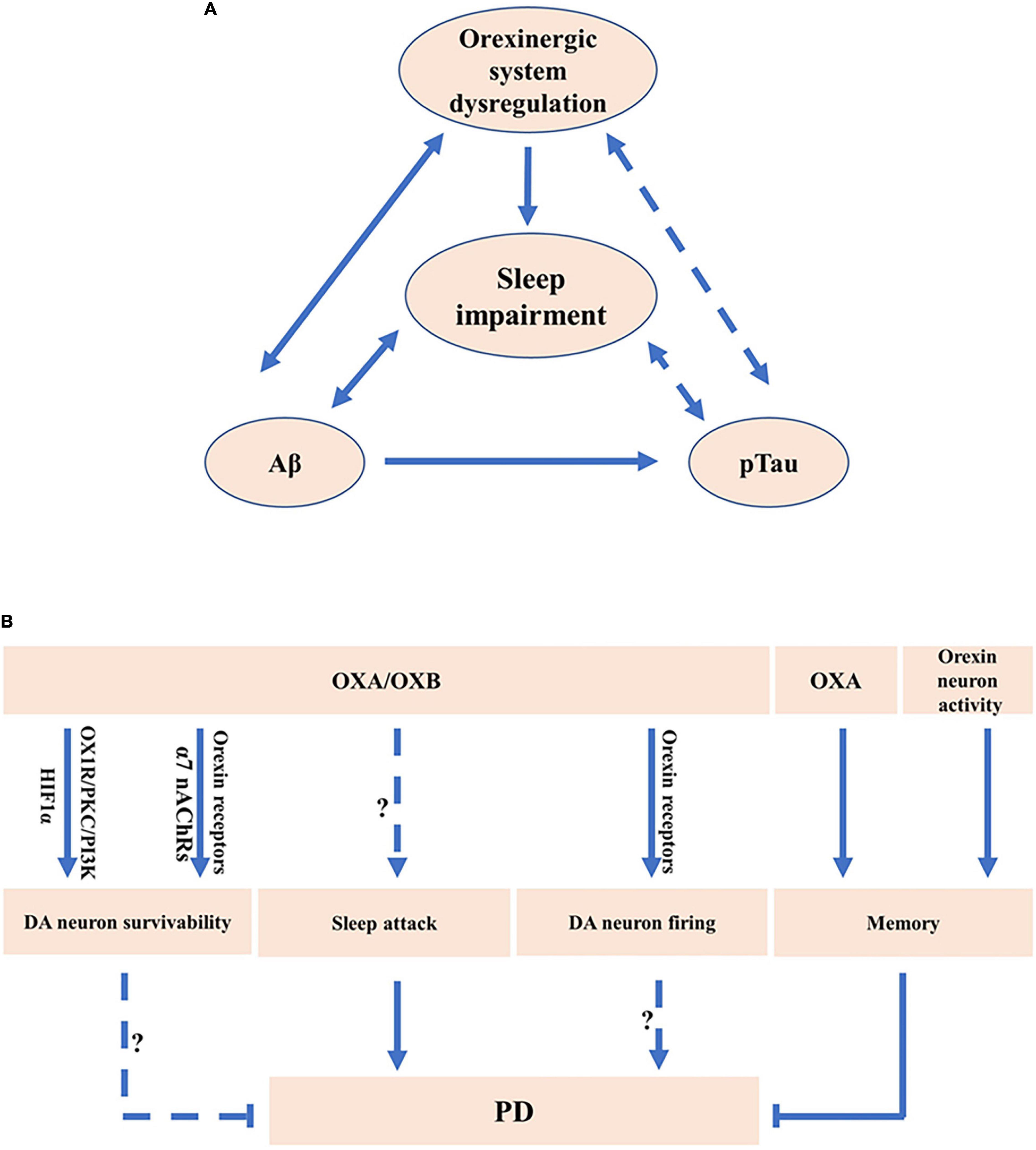
Figure 1. The potential roles of orexinergic system in Alzheimer’s disease (AD) and Parkinson’s disease (PD). (A) The potential roles of orexinergic system in AD. Orexinergic system is involved in the regulation of sleep–wake states. Sleep disruption facilitated Aβ accumulation in AD. Orexin A (OXA) is associated with Aβ. Evidence also suggests a potential relationship between Aβ levels and sleep–wake states. Moreover, OXA in cerebrospinal fluid (CSF) was correlated with pTau. The levels of pTau were correlated with stage 1 of NREM sleep. More experiments are needed to clarify the correlation between pTau and orexinergic system-related sleep dysfunction. (B) The potential roles of orexinergic system in PD. OXA exerted the neuroprotective roles in PD cell model via regulating HIF-1α or orexin 1 receptor (OX1R)/protein kinase C (PKC)/PI3-kinase (PI3K) signaling. Orexin 2 receptor (OX2R) and α7 nAChRs were involved in the promotion of the survivability of DA neurons regulated by orexin B (OXB). OXA treatment or enhancement of orexin neurons activity markedly decreased the memory impairment in PD mice. OXA and OXB significantly increased the rate of spontaneous firing of dopaminergic (DA) neurons via OX1R and OX2R. Sleep attack has been observed in PD. Orexinergic system was involved in sleep disorders, indicating the potential roles of orexinergic system in regulating PD-related sleep disorders.
The orexinergic signaling is associated with Tau pathology in AD (Deuschle et al., 2014; Liguori et al., 2014; Liguori, 2017). The level of OXA in CSF was correlated with Tau and phosphorylated Tau (pTau) in patients with AD (Deuschle et al., 2014; Figure 1A). Consistently, the level of OXA in CSF exhibited an evidently positive correlation with Tau and pTau in patients with moderate to severe AD (Liguori et al., 2014), and even in control subjects (Shimizu et al., 2020). Importantly, the OXA downregulation dramatically decreased the levels of Tau and pTau induced by Aβ25–35 in vitro (Liu et al., 2019; Figure 1A). Moreover, studies show that the levels of pTau were correlated with stage 1 of NREM sleep in AD (Liguori et al., 2014; Figure 1A). It indicates that the role of orexinergic signaling in Tau pathology may be related to sleep dysfunction.
Dysregulation of orexinergic signaling may be associated with neurodegeneration and neuronal loss in AD. First, AD was primarily associated with the loss of basal forebrain cholinergic neurons (Zajo et al., 2016). Basal forebrain was one of the major projection targets of orexin neurons (Li and de Lecea, 2020). Orexin receptors were expressed in the basal forebrain (Li and de Lecea, 2020), while orexin neurons directly communicated with the cholinergic neurons in the basal forebrain through synapses (Li and de Lecea, 2020). Moreover, the administration of OXA in basal forebrain ameliorated distracter-induced attention deficiency in rats (Zajo et al., 2016). These results indicate that the orexinergic system may be involved in AD pathology by regulating the cholinergic pathway in the basal forebrain.
Orexinergic System in PD
PD is characterized by the selective loss of dopaminergic (DA) neurons in the substantia nigra (SN) and the formation of Lewy body (Kalia and Lang, 2015; Reich and Savitt, 2019). The clinical symptoms include both motor symptoms such as resting tremor and bradykinesia and non-motor symptoms such as pain, sleep disorder, and cognition dysfunction (Kalia and Lang, 2015).
The dysregulation of the orexinergic system in PD is inconclusive as the number of studies is limited. First, it was reported that the level of OXA was significantly decreased in ventricular CSF of patients with advanced PD compared with controls (Drouot et al., 2003). Consistently, the number of orexin neurons was markedly decreased in patients with PD compared with that in controls (Fronczek et al., 2007). However, the level of OXA was within the normal range in CSF obtained from patients with PD by lumbar puncture (Yasui et al., 2006).
Orexinergic system is implicated in the neuroprotective effect on DA neurons (Cui et al., 2010; Feng et al., 2014; Guerreiro et al., 2015; Hadadianpour et al., 2017; Pasban-Aliabadi et al., 2017; Liu M. F. et al., 2018). The number of OXA positive neurons tended to be decreased in hypothalamus of PD model rats (Cui et al., 2010). OXA significantly ameliorated 1-methyl-4-phenylpyridinium (MPP+)-induced injury in SH-SY5Y cells, which was mediated by hypoxia inducible factor-1 alpha (HIF-1α) (Feng et al., 2014; Figure 1B). Moreover, OXA significantly attenuated 6-hydroxydopamine (6-OHDA)-induced cell toxicity in SH-SY5Y cells, which was mediated by OX1R/protein kinase C (PKC)/PI3-kinase (PI3K) signaling (Pasban-Aliabadi et al., 2017; Figure 1B). In addition, OXA significantly attenuated 1-methyl-4-phenyl-1, 2, 3, 6-tetrahydropyridine (MPTP)-induced DA neuron loss in the SN of mice, contributing to the improvement of cognitive and motor ability (Liu M. F. et al., 2018), whereas OX1R antagonist SB334867 could inhibit the protective effect (Liu M. F. et al., 2018; Figure 1B). Consistently, OXA significantly ameliorated 6-OHDA-induced impairments of locomotor function, sensorimotor function, and muscle tone in rats (Hadadianpour et al., 2017). Furthermore, OXB increased the survivability of DA neurons mediated by OX2R but not by OX1R, whereas nicotine markedly improved the neuroprotective effects of OXB on DA neuron via the activation of α7 nicotinic acetylcholine receptors (α7 nAChRs) (Guerreiro et al., 2015; Figure 1B). The above evidence indicates that orexins and orexin receptors are involved in the protective effect on DA neurons in PD models.
Sleep disruption is a common symptom in PD (Asai et al., 2009; Nie et al., 2019). Most of these patients suffer from excessive sleepiness during daytime and some of them present the sleep attack (Tracik and Ebersbach, 2001; Brodsky et al., 2003; Asai et al., 2009). Some characteristics of sleep attack in patients with PD are similar to narcolepsy symptoms (Arnulf et al., 2000; Asai et al., 2009). Dopamine agonists were one of the major factors influencing the emergence of sleep attack, suggesting the close correlation between DA system and sleep attack in PD (Paus et al., 2003; Figure 1B). Electrophysiological analysis revealed that the administration of OXA and OXB observably enhanced the rate of spontaneous firing of DA neurons in the SN of rats and inhibition of OX1R and OX2R significantly decreased the firing rate of DA neurons in the SN, implying the roles of the orexinergic pathway in regulating DA system (Liu C. et al., 2018; Figure 1B). Moreover, OXA application in the hippocampus or chemogenetic activation of orexin neurons significantly ameliorated the memory impairment in A53T transgenic mice, a PD model mice (Stanojlovic et al., 2019; Figure 1B). It indicates that the orexinergic system plays a key role in sleep disruption and memory defects in patients with PD.
Orexinergic System in HD
HD, a kind of polyglutamine disorder described first by George Huntington, presents autosomal dominant inheritance and is characterized by a progressive decline of cognition, motor, and behavioral functions (Wyant et al., 2017; Ghosh and Tabrizi, 2018; Croce and Yamamoto, 2019). Given that the molecular mechanisms underlying HD pathogenesis remain unclear, there is no effective treatment for HD (Ghosh and Tabrizi, 2018). Orexinergic system is involved in the pathogenesis of HD. A significant reduction of orexin neurons in the LH and hypothalamus was observed in patients with HD compared with that in controls (Petersen et al., 2005; Gabery et al., 2010; Figure 2A). Consistently, a significant decrease of OXA and OXB was observed in HD model mice (Petersen et al., 2005; Figure 2A).
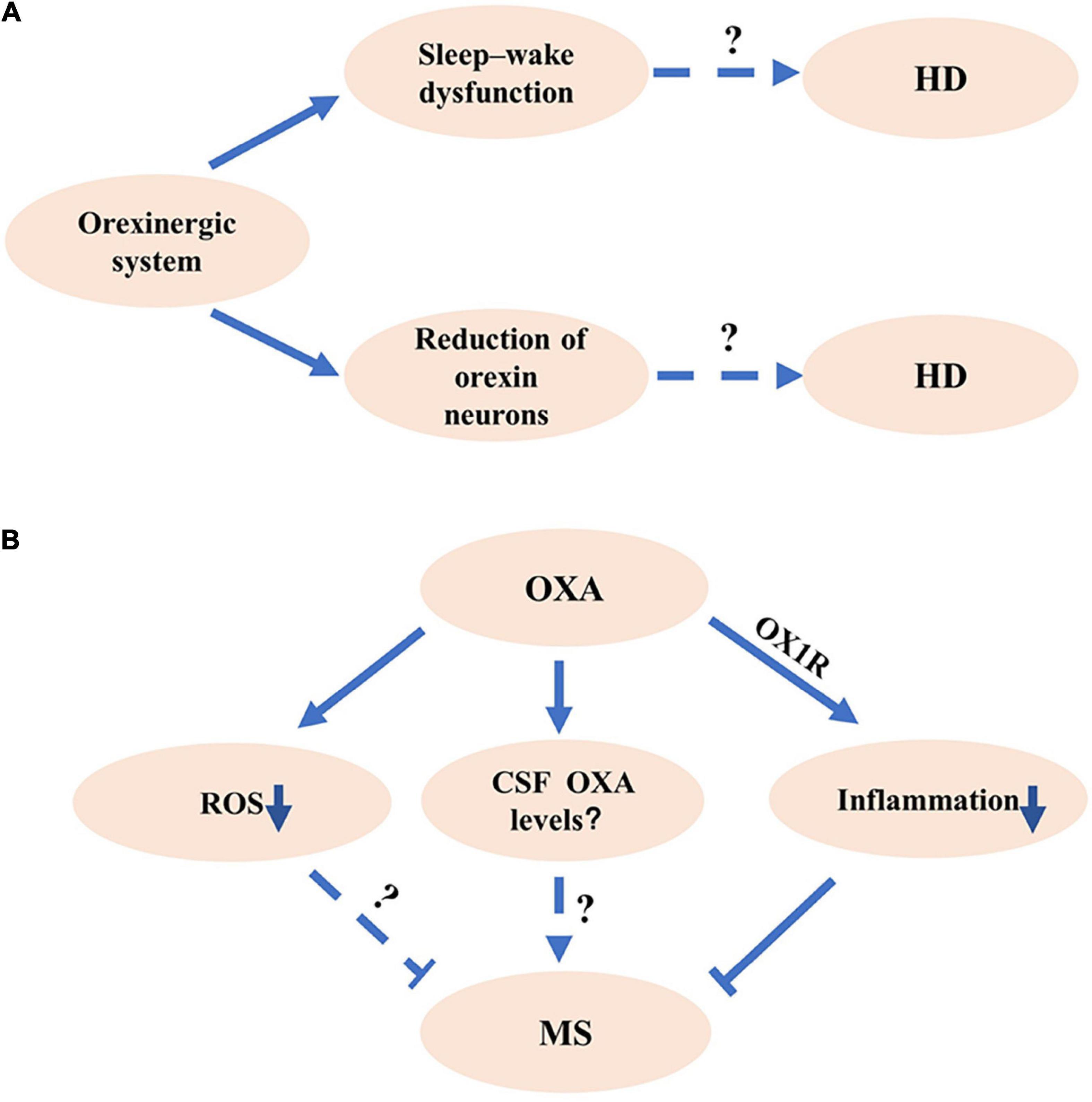
Figure 2. The potential roles of orexinergic system in Huntington’s disease (HD) and multiple sclerosis (MS). (A) The potential roles of orexinergic system in HD. Studies show that the number of OX neurons decreased in HD. Abnormal sleep existed in HD. The details of orexinergic signaling in HD need to be further investigated. (B) The potential roles of orexinergic system in MS. The OXA/OX1R signaling significantly decreased the inflammation and pathological processes in EAE mice. Moreover, OXA could markedly reduce the reactive oxygen species (ROS) and microglial activation, indicating that the orexinergic system might involve in MS pathology by regulating ROS and microglial activation. More experiments are needed to determine the relationship between orexin levels and MS pathology at different courses.
The abnormal sleep characteristics were observed in HD model mice, including a shorter slow-wave sleep (SWS) duration, more episodes of every vigilance state during the periods of light and dark cycle, and a particular β rhythm with the frequency of 20–35 Hz (Jeantet et al., 2013; Lebreton et al., 2015). Promoting sleep with the pharmacological method effectively slowed the decline of cognition performance in HD mice (Pallier et al., 2007). Imposing a drug-induced relative regular rhythm of wake–sleep contributed to the enhancement of cognition ability in HD mice (Pallier and Morton, 2009). In addition, the administration of OX1R and OX2R antagonist significantly attenuated the β activity during the sleep stage of SWS and REM, reduced sleep–wake rhythm deficiency, and efficiently improved the behavioral performance in HD mice (Cabanas et al., 2019; Figure 2A). These results indicate that modulating orexinergic system may have a therapeutic potential for the improvement of sleep and cognitive performance in HD.
Dorsal striatum is the major pathological region in HD (Page et al., 2000). OXA increased the surface levels of α-amino-3-hydroxy-5-methyl-4-isoxazolepropioinc acid (AMPA) receptor in the striatum, leading to the functional changes of striatum circuits (Shin et al., 2009). Both OX1R and OX2R were expressed in dorsal striatum (Zhang et al., 2007). It suggests that orexinergic system may involve in HD pathology by regulating the activity of striatum circuits. Thus, modulating orexinergic system in dorsal striatum-related circuits may be a potential target for the HD treatment.
Orexinergic System in MS
MS is a kind of long-term inflammation-related autoimmune disease with the major characteristic of demyelinating in the central nervous system, ultimately leading to the neurodegeneration (Correale et al., 2017; Gencer et al., 2019). As the progress of MS is variable, MS is classified into relapsing remitting MS (RRMS), secondary progressive MS (SPMS), primary progressive form of MS (PPMS), and progressive relapsing form of MS (PRMS) (Lublin and Reingold, 1996; Correale et al., 2017; Hughes et al., 2018). The major characteristic of patients with RRMS is experiencing partial or complete recovery from the emergence of recurring symptoms of the disease (Antel et al., 2012; Correale et al., 2017). After periods of years, some of the patients slowly turn into gradual deterioration, the stage called SPMS (Antel et al., 2012; Correale et al., 2017). About 15% of the patients present a progressive decline of neurological function without obvious relapses, which is called PPMS (Antel et al., 2012; Correale et al., 2017). A group of patients with MS who undergo episodes of distinct clinical relapse are taken as PRMS (Hughes et al., 2018). Although environmental element, inflammation, and genetic factors are implicated in MS pathology, the precise mechanisms of MS remain unclear (Correale et al., 2017).
Sleep disturbance is a trigger of relapse in MS, indicating the potential roles of the orexinergic pathway in MS (Sahraian et al., 2017). A significant decrease of OXA in CSF was observed in a female MS patient with hypersomnia (Oka et al., 2004). However, Constantinescu et al. showed that there was no significant deficiency of OXA in patients with MS compared with controls with other inflammatory-related brain disorders or non-inflammatory brain diseases (Constantinescu et al., 2011; Burfeind et al., 2016). A recent study showed that serum OXA was remarkably decreased in patients with MS compared with that in healthy cases (Gencer et al., 2019). Moreover, serum OXA was robustly lower in patients with SPMS than that in patients with RRMS, indicating the serum OXA may correlate with the progression of MS (Gencer et al., 2019). Given the complexity and variety of the pathological courses of MS, further investigation is necessary to determine the orexin levels in CSF of patients with MS at different courses (Figure 2B).
Evidence indicates that neuroinflammation and glial activation are the important pathological features of MS (Datta et al., 2017; Grajchen et al., 2018). Experimental autoimmune encephalomyelitis (EAE) was a commonly used animal model of MS (Becquet et al., 2019). OXA significantly reduced the formation of reactive oxygen species (ROS) in primary cultured microglia (Tunisi et al., 2019; Figure 2B). Moreover, the intraperitoneal injection of OXA decreased lipopolysaccharide (LPS)-induced activation of microglia in the prefrontal cortex, which was blocked by OX1R antagonist SB334867 (Tunisi et al., 2019), indicating the regulation role of the orexinergic system in neuroinflammation (Figure 2B). In addition, the OXA treatment significantly reduced the inflammation and decreased the pathological scores in EAE mice, which was inhibited by SB334867 (Fatemi et al., 2016; Figure 2B). Consistently, the administration of OXA dramatically reduced clinical symptoms, decreased neuroinflammation, and alleviated demyelinating and glial activation in EAE mice (Becquet et al., 2019; Figure 2B). All the above studies indicate that the orexinergic system exerts the neuroprotection roles by inhibiting the neuroinflammation in MS (Becquet et al., 2019).
Perspective of Targeting Orexinergic System
Given the dysfunction of orexinergic pathway is implicated in the pathogenesis of AD, PD, HD, and MS, the orexinergic system may be a potential therapeutic target for the treatment of these diseases (Petersen et al., 2005; Asai et al., 2009; Fatemi et al., 2016; Mander et al., 2016; Liguori, 2017). Dysfunction of sleep–wake, abnormal protein aggregation, and neuroinflammation are the common features of these diseases (Glass et al., 2010; Correale et al., 2017; Illarioshkin et al., 2018). First, dysregulation of the orexinergic system is implicated in the disruption of the sleep–wake process in AD, PD, HD, and MS (Asai et al., 2009; Mander et al., 2016; Liguori, 2017; Sahraian et al., 2017; Cabanas et al., 2019; Turkoglu et al., 2020). On the one hand, sleep disruption contributes to neurodegeneration by facilitating disease-associated protein accumulation such as Aβ (Mander et al., 2016; Liguori, 2017). On the other hand, sleep disruption also attenuates the clearance of Aβ, whereas sleeping facilitates Aβ clearance in AD model mice (Xie et al., 2013). Moreover, abnormal protein aggregation-induced neuroinflammation aggravates the neurodegeneration and neuronal loss in AD and PD (Glass et al., 2010). Thus, targeting the orexinergic system might ameliorate sleep impairment attenuating abnormal protein aggregation and subsequent neuroinflammation and neurodegeneration.
Conclusion
Mounting evidence shows that the orexinergic system is involved in the sleep–wake process, whereas sleep disruption is a common symptom of many neurodegenerative diseases including AD, PD, HD, and MS. Moreover, dysfunction of the orexinergic pathway is implicated in the pathogenesis of AD, PD, HD, and MS, which is associated with sleep impairment, pathogenic protein aggregation, neuronal loss, and activation of neuroinflammation (Figures 1, 2). However, the exact roles of the orexinergic system in the above diseases and underlying mechanisms are still not fully understood. Thus, it is essential to further investigate the dysregulation of the orexinergic system in neurodegenerative diseases and its role in the pathogenesis of these diseases.
Author Contributions
QW and FC wrote the manuscript. YW conceived and revised the manuscript. All the authors approved the final manuscript.
Funding
This work was funded by the Research Fund for Lin He’s Academician Workstation of New Medicine and Clinical Translation in Jining Medical University (No. JYHL2018MS03), Technology Development Project of Shandong Province Medicine and Health Science (No. 202002081082), NSFC cultivation project of Jining Medical University (No. JYP2019KJ20), A Project of Shandong Province Higher Educational Science and Technology Program (No. J18KA124), and Research Fund for the Doctoral Program of Jining Medical University, the National Natural Science Foundation of China (NSFC) (Grant Nos. 81771147 and 81971019) to YW.
Conflict of Interest
The authors declare that the research was conducted in the absence of any commercial or financial relationships that could be construed as a potential conflict of interest.
Publisher’s Note
All claims expressed in this article are solely those of the authors and do not necessarily represent those of their affiliated organizations, or those of the publisher, the editors and the reviewers. Any product that may be evaluated in this article, or claim that may be made by its manufacturer, is not guaranteed or endorsed by the publisher.
References
Adamantidis, A. R., Zhang, F., Aravanis, A. M., Deisseroth, K., and de Lecea, L. (2007). Neural substrates of awakening probed with optogenetic control of hypocretin neurons. Nature 450, 420–424. doi: 10.1038/nature06310
Antel, J., Antel, S., Caramanos, Z., Arnold, D. L., and Kuhlmann, T. (2012). Primary progressive multiple sclerosis: part of the MS disease spectrum or separate disease entity? Acta Neuropathol. 123, 627–638. doi: 10.1007/s00401-012-0953-0
Arnulf, I., Bonnet, A. M., Damier, P., Bejjani, B. P., Seilhean, D., Derenne, J. P., et al. (2000). Hallucinations, REM sleep, and Parkinson’s disease: a medical hypothesis. Neurology 55, 281–288. doi: 10.1212/wnl.55.2.281
Asai, H., Hirano, M., Furiya, Y., Udaka, F., Morikawa, M., Kanbayashi, T., et al. (2009). Cerebrospinal fluid-orexin levels and sleep attacks in four patients with Parkinson’s disease. Clin. Neurol. Neurosurg. 111, 341–344. doi: 10.1016/j.clineuro.2008.11.007
Bassetti, C. L. A., Adamantidis, A., Burdakov, D., Han, F., Gay, S., Kallweit, U., et al. (2019). Narcolepsy–clinical spectrum, aetiopathophysiology, diagnosis and treatment. Nat. Rev. Neurol. 15, 519–539. doi: 10.1038/s41582-019-0226-9
Becquet, L., Abad, C., Leclercq, M., Miel, C., Jean, L., Riou, G., et al. (2019). Systemic administration of orexin a ameliorates established experimental autoimmune encephalomyelitis by diminishing neuroinflammation. J. Neuroinflammation 16:64. doi: 10.1186/s12974-019-1447-y
Brodsky, M. A., Godbold, J., Roth, T., and Olanow, C. W. (2003). Sleepiness in Parkinson’s disease: a controlled study. Mov. Disord. 18, 668–672. doi: 10.1002/mds.10429
Burfeind, K. G., Yadav, V., and Marks, D. L. (2016). Hypothalamic dysfunction and multiple sclerosis: implications for fatigue and weight dysregulation. Curr. Neurol. Neurosci. Rep. 16:98. doi: 10.1007/s11910-016-0700-3
Cabanas, M., Pistono, C., Puygrenier, L., Rakesh, D., Jeantet, Y., Garret, M., et al. (2019). Neurophysiological and behavioral effects of anti-orexinergic treatments in a mouse model of Huntington’s disease. Neurotherapeutics 16, 784–796. doi: 10.1007/s13311-019-00726-3
Constantinescu, C. S., Niepel, G., Patterson, M., Judd, A., Braitch, M., Fahey, A. J., et al. (2011). Orexin A (hypocretin-1) levels are not reduced while cocaine/amphetamine regulated transcript levels are increased in the cerebrospinal fluid of patients with multiple sclerosis: no correlation with fatigue and sleepiness. J. Neurol. Sci. 307, 127–131. doi: 10.1016/j.jns.2011.04.024
Correale, J., Gaitan, M. I., Ysrraelit, M. C., and Fiol, M. P. (2017). Progressive multiple sclerosis: from pathogenic mechanisms to treatment. Brain 140, 527–546. doi: 10.1093/brain/aww258
Croce, K. R., and Yamamoto, A. (2019). A role for autophagy in Huntington’s disease. Neurobiol. Dis. 122, 16–22. doi: 10.1016/j.nbd.2018.08.010
Cui, L. B., Li, B. W., Jin, X. H., Zhao, L., and Shi, J. (2010). Progressive changes of orexin system in a rat model of 6-hydroxydopamine-induced Parkinson’s disease. Neurosci. Bull. 26, 381–387. doi: 10.1007/s12264-010-0410-9
Datta, G., Colasanti, A., Rabiner, E. A., Gunn, R. N., Malik, O., Ciccarelli, O., et al. (2017). Neuroinflammation and its relationship to changes in brain volume and white matter lesions in multiple sclerosis. Brain 140, 2927–2938. doi: 10.1093/brain/awx228
de Lecea, L., Kilduff, T. S., Peyron, C., Gao, X., Foye, P. E., Danielson, P. E., et al. (1998). The hypocretins: hypothalamus-specific peptides with neuroexcitatory activity. Proc. Natl. Acad. Sci. U.S.A. 95, 322–327. doi: 10.1073/pnas.95.1.322
Deuschle, M., Schilling, C., Leweke, F. M., Enning, F., Pollmacher, T., Esselmann, H., et al. (2014). Hypocretin in cerebrospinal fluid is positively correlated with Tau and pTau. Neurosci. Lett. 561, 41–45. doi: 10.1016/j.neulet.2013.12.036
Drouot, X., Moutereau, S., Nguyen, J. P., Lefaucheur, J. P., Creange, A., Remy, P., et al. (2003). Low levels of ventricular CSF orexin/hypocretin in advanced PD. Neurology 61, 540–543. doi: 10.1212/01.wnl.0000078194.53210.48
Dyer, C. J., Touchette, K. J., Carroll, J. A., Allee, G. L., and Matteri, R. L. (1999). Cloning of porcine prepro-orexin cDNA and effects of an intramuscular injection of synthetic porcine orexin-B on feed intake in young pigs. Domest. Anim. Endocrinol. 16, 145–148. doi: 10.1016/s0739-7240(99)00011-9
Fatemi, I., Shamsizadeh, A., Ayoobi, F., Taghipour, Z., Sanati, M. H., Roohbakhsh, A., et al. (2016). Role of orexin-A in experimental autoimmune encephalomyelitis. J. Neuroimmunol. 291, 101–109. doi: 10.1016/j.jneuroim.2016.01.001
Feng, Y., Liu, T., Li, X. Q., Liu, Y., Zhu, X. Y., Jankovic, J., et al. (2014). Neuroprotection by orexin-A via HIF-1alpha induction in a cellular model of Parkinson’s disease. Neurosci. Lett. 579, 35–40. doi: 10.1016/j.neulet.2014.07.014
Fronczek, R., Overeem, S., Lee, S. Y., Hegeman, I. M., van Pelt, J., van Duinen, S. G., et al. (2007). Hypocretin (orexin) loss in Parkinson’s disease. Brain 130(Pt 6), 1577–1585. doi: 10.1093/brain/awm090
Fronczek, R., van Geest, S., Frolich, M., Overeem, S., Roelandse, F. W., Lammers, G. J., et al. (2012). Hypocretin (orexin) loss in Alzheimer’s disease. Neurobiol. Aging 33, 1642–1650. doi: 10.1016/j.neurobiolaging.2011.03.014
Gabelle, A., Jaussent, I., Hirtz, C., Vialaret, J., Navucet, S., Grasselli, C., et al. (2017). Cerebrospinal fluid levels of orexin-A and histamine, and sleep profile within the Alzheimer process. Neurobiol. Aging 53, 59–66. doi: 10.1016/j.neurobiolaging.2017.01.011
Gabery, S., Murphy, K., Schultz, K., Loy, C. T., McCusker, E., Kirik, D., et al. (2010). Changes in key hypothalamic neuropeptide populations in Huntington disease revealed by neuropathological analyses. Acta Neuropathol. 120, 777–788. doi: 10.1007/s00401-010-0742-6
Gamble, M. C., Katsuki, F., McCoy, J. G., Strecker, R. E., and McKenna, J. T. (2019). The dual orexinergic receptor antagonist DORA-22 improves the sleep disruption and memory impairment produced by a rodent insomnia model. Sleep 43:zsz241. doi: 10.1093/sleep/zsz241
Gencer, M., Akbayir, E., Sen, M., Arsoy, E., Yilmaz, V., Bulut, N., et al. (2019). Serum orexin-a levels are associated with disease progression and motor impairment in multiple sclerosis. Neurol. Sci. 40, 1067–1070. doi: 10.1007/s10072-019-3708-z
Ghosh, R., and Tabrizi, S. J. (2018). Huntington disease. Handb. Clin. Neurol. 147, 255–278. doi: 10.1016/B978-0-444-63233-3.00017-8
Glass, C. K., Saijo, K., Winner, B., Marchetto, M. C., and Gage, F. H. (2010). Mechanisms underlying inflammation in neurodegeneration. Cell 140, 918–934. doi: 10.1016/j.cell.2010.02.016
Gotter, A. L., Webber, A. L., Coleman, P. J., Renger, J. J., and Winrow, C. J. (2012). International union of basic and clinical pharmacology. LXXXVI. OREXIN receptor function, nomenclature and pharmacology. Pharmacol. Rev. 64, 389–420. doi: 10.1124/pr.111.005546
Grajchen, E., Hendriks, J. J. A., and Bogie, J. F. J. (2018). The physiology of foamy phagocytes in multiple sclerosis. Acta Neuropathol. Commun. 6:124. doi: 10.1186/s40478-018-0628-8
Guerreiro, S., Florence, C., Rousseau, E., Hamadat, S., Hirsch, E. C., and Michel, P. P. (2015). The sleep-modulating peptide orexin-B protects midbrain dopamine neurons from degeneration, alone or in cooperation with nicotine. Mol. Pharmacol. 87, 525–532. doi: 10.1124/mol.114.095703
Hadadianpour, Z., Fatehi, F., Ayoobi, F., Kaeidi, A., Shamsizadeh, A., and Fatemi, I. (2017). The effect of orexin-A on motor and cognitive functions in a rat model of Parkinson’s disease. Neurol. Res. 39, 845–851. doi: 10.1080/01616412.2017.1352185
Hughes, J., Jokubaitis, V., Lugaresi, A., Hupperts, R., Izquierdo, G., Prat, A., et al. (2018). Association of inflammation and disability accrual in patients with progressive-onset multiple sclerosis. JAMA Neurol. 75, 1407–1415. doi: 10.1001/jamaneurol.2018.2109
Illarioshkin, S. N., Klyushnikov, S. A., Vigont, V. A., Seliverstov, Y. A., and Kaznacheyeva, E. V. (2018). Molecular pathogenesis in Huntington’s disease. Biochemistry 83, 1030–1039. doi: 10.1134/S0006297918090043
Irukayama-Tomobe, Y., Ogawa, Y., Tominaga, H., Ishikawa, Y., Hosokawa, N., Ambai, S., et al. (2017). Nonpeptide orexin type-2 receptor agonist ameliorates narcolepsy-cataplexy symptoms in mouse models. Proc. Natl. Acad. Sci. U.S.A. 114, 5731–5736. doi: 10.1073/pnas.1700499114
Jeantet, Y., Cayzac, S., and Cho, Y. H. (2013). Beta oscillation during slow wave sleep and rapid eye movement sleep in the electroencephalogram of a transgenic mouse model of Huntington’s disease. PLoS One 8:e79509. doi: 10.1371/journal.pone.0079509
Kalia, L. V., and Lang, A. E. (2015). Parkinson’s disease. Lancet 386, 896–912. doi: 10.1016/S0140-6736(14)61393-3
Kang, J. E., Lim, M. M., Bateman, R. J., Lee, J. J., Smyth, L. P., Cirrito, J. R., et al. (2009). Amyloid-beta dynamics are regulated by orexin and the sleep-wake cycle. Science 326, 1005–1007. doi: 10.1126/science.1180962
Lane, C. A., Hardy, J., and Schott, J. M. (2018). Alzheimer’s disease. Eur. J. Neurol. 25, 59–70. doi: 10.1111/ene.13439
Lebreton, F., Cayzac, S., Pietropaolo, S., Jeantet, Y., and Cho, Y. H. (2015). Sleep physiology alterations precede plethoric phenotypic changes in R6/1 Huntington’s disease mice. PLoS One 10:e0126972. doi: 10.1371/journal.pone.0126972
Li, S. B., and de Lecea, L. (2020). The hypocretin (orexin) system: from a neural circuitry perspective. Neuropharmacology 167:107993. doi: 10.1016/j.neuropharm.2020.107993
Liguori, C. (2017). Orexin and Alzheimer’s disease. Curr. Top. Behav. Neurosci. 33, 305–322. doi: 10.1007/7854_2016_50
Liguori, C., Mercuri, N. B., Nuccetelli, M., Izzi, F., Bernardini, S., and Placidi, F. (2018). Cerebrospinal fluid orexin levels and nocturnal sleep disruption in Alzheimer’s disease patients showing neuropsychiatric symptoms. J. Alzheimers Dis. 66, 993–999. doi: 10.3233/JAD-180769
Liguori, C., Romigi, A., Nuccetelli, M., Zannino, S., Sancesario, G., Martorana, A., et al. (2014). Orexinergic system dysregulation, sleep impairment, and cognitive decline in Alzheimer disease. JAMA Neurol. 71, 1498–1505. doi: 10.1001/jamaneurol.2014.2510
Liguori, C., Spanetta, M., Izzi, F., Franchini, F., Nuccetelli, M., Sancesario, G. M., et al. (2020). Sleep-wake cycle in Alzheimer’s disease is associated with tau pathology and orexin dysregulation. J. Alzheimers Dis. 74, 501–508. doi: 10.3233/JAD-191124
Liu, C., Xue, Y., Liu, M. F., Wang, Y., Liu, Z. R., Diao, H. L., et al. (2018). Orexins increase the firing activity of nigral dopaminergic neurons and participate in motor control in rats. J. Neurochem. 147, 380–394. doi: 10.1111/jnc.14568
Liu, M. F., Xue, Y., Liu, C., Liu, Y. H., Diao, H. L., Wang, Y., et al. (2018). Orexin-A exerts neuroprotective effects via OX1R in Parkinson’s disease. Front. Neurosci. 12:835. doi: 10.3389/fnins.2018.00835
Liu, Z., Wang, F., Tang, M., Zhao, Y., and Wang, X. (2019). Amyloid beta and tau are involved in sleep disorder in Alzheimer’s disease by orexin A and adenosine A(1) receptor. Int. J. Mol. Med. 43, 435–442. doi: 10.3892/ijmm.2018.3935
Lublin, F. D., and Reingold, S. C. (1996). Defining the clinical course of multiple sclerosis: results of an international survey. national multiple sclerosis society (USA) advisory committee on clinical trials of new agents in multiple sclerosis. Neurology 46, 907–911. doi: 10.1212/wnl.46.4.907
Mander, B. A., Winer, J. R., Jagust, W. J., and Walker, M. P. (2016). Sleep: a novel mechanistic pathway, biomarker, and treatment target in the pathology of Alzheimer’s disease? Trends Neurosci 39, 552–566. doi: 10.1016/j.tins.2016.05.002
Nepovimova, E., Janockova, J., Misik, J., Kubik, S., Stuchlik, A., Vales, K., et al. (2019). Orexin supplementation in narcolepsy treatment: a review. Med. Res. Rev. 39, 961–975. doi: 10.1002/med.21550
Nie, K., Gao, Y., Mei, M., Guo, M., Huang, Z., Wang, L., et al. (2019). The clinical characteristics and cognitive features of mild cognitive impairment in Parkinson’s disease and the analysis of relevant factors. J. Clin. Neurosci. 63, 142–148. doi: 10.1016/j.jocn.2019.01.021
Oka, Y., Kanbayashi, T., Mezaki, T., Iseki, K., Matsubayashi, J., Murakami, G., et al. (2004). Low CSF hypocretin-1/orexin-A associated with hypersomnia secondary to hypothalamic lesion in a case of multiple sclerosis. J. Neurol. 251, 885–886. doi: 10.1007/s00415-004-0442-z
Page, K. J., Besret, L., Jain, M., Monaghan, E. M., Dunnett, S. B., and Everitt, B. J. (2000). Effects of systemic 3-nitropropionic acid-induced lesions of the dorsal striatum on cannabinoid and mu-opioid receptor binding in the basal ganglia. Exp. Brain Res. 130, 142–150. doi: 10.1007/s002210050016
Pallier, P. N., Maywood, E. S., Zheng, Z., Chesham, J. E., Inyushkin, A. N., Dyball, R., et al. (2007). Pharmacological imposition of sleep slows cognitive decline and reverses dysregulation of circadian gene expression in a transgenic mouse model of Huntington’s disease. J. Neurosci. 27, 7869–7878. doi: 10.1523/JNEUROSCI.0649-07.2007
Pallier, P. N., and Morton, A. J. (2009). Management of sleep/wake cycles improves cognitive function in a transgenic mouse model of Huntington’s disease. Brain Res. 1279, 90–98. doi: 10.1016/j.brainres.2009.03.072
Pasban-Aliabadi, H., Esmaeili-Mahani, S., and Abbasnejad, M. (2017). Orexin-A protects human neuroblastoma SH-SY5Y cells against 6-hydroxydopamine-induced neurotoxicity: involvement of PKC and PI3K signaling pathways. Rejuvenation Res. 20, 125–133. doi: 10.1089/rej.2016.1836
Paus, S., Brecht, H. M., Koster, J., Seeger, G., Klockgether, T., and Wullner, U. (2003). Sleep attacks, daytime sleepiness, and dopamine agonists in Parkinson’s disease. Mov. Disord. 18, 659–667. doi: 10.1002/mds.10417
Petersen, A., Gil, J., Maat-Schieman, M. L., Bjorkqvist, M., Tanila, H., Araujo, I. M., et al. (2005). Orexin loss in Huntington’s disease. Hum. Mol. Genet. 14, 39–47. doi: 10.1093/hmg/ddi004
Qiu, K., Zhang, X., Wang, S., Li, C., Wang, X., Li, X., et al. (2019). TMP21 in Alzheimer’s disease: molecular mechanisms and a potential target. Front. Cell. Neurosci. 13:328. doi: 10.3389/fncel.2019.00328
Reich, S. G., and Savitt, J. M. (2019). Parkinson’s disease. Med. Clin. North Am. 103, 337–350. doi: 10.1016/j.mcna.2018.10.014
Roh, J. H., Jiang, H., Finn, M. B., Stewart, F. R., Mahan, T. E., Cirrito, J. R., et al. (2014). Potential role of orexin and sleep modulation in the pathogenesis of Alzheimer’s disease. J. Exp. Med. 211, 2487–2496. doi: 10.1084/jem.20141788
Sahraian, M. A., Rezaali, S., Hosseiny, M., Doosti, R., Tajik, A., and Naser Moghadasi, A. (2017). Sleep disorder as a triggering factor for relapse in multiple sclerosis. Eur. Neurol. 77, 258–261. doi: 10.1159/000470904
Sakurai, T., Amemiya, A., Ishii, M., Matsuzaki, I., Chemelli, R. M., Tanaka, H., et al. (1998). Orexins and orexin receptors: a family of hypothalamic neuropeptides and G protein-coupled receptors that regulate feeding behavior. Cell 92, 573–585. doi: 10.1016/s0092-8674(02)09256-5
Sasaki, K., Suzuki, M., Mieda, M., Tsujino, N., Roth, B., and Sakurai, T. (2011). Pharmacogenetic modulation of orexin neurons alters sleep/wakefulness states in mice. PLoS One 6:e20360. doi: 10.1371/journal.pone.0020360
Shimizu, S., Takenoshita, N., Inagawa, Y., Tsugawa, A., Hirose, D., Kaneko, Y., et al. (2020). Positive association between cognitive function and cerebrospinal fluid orexin a levels in Alzheimer’s disease. J. Alzheimers Dis. 73, 117–123. doi: 10.3233/JAD-190958
Shin, H. S., Cho, H. S., Sung, K. W., and Yoon, B. J. (2009). Orexin-A increases cell surface expression of AMPA receptors in the striatum. Biochem. Biophys. Res. Commun. 378, 409–413. doi: 10.1016/j.bbrc.2008.11.051
Slats, D., Claassen, J. A., Lammers, G. J., Melis, R. J., Verbeek, M. M., and Overeem, S. (2012). Association between hypocretin-1 and amyloid-beta42 cerebrospinal fluid levels in Alzheimer’s disease and healthy controls. Curr. Alzheimer Res. 9, 1119–1125. doi: 10.2174/156720512804142840
Soya, S., and Sakurai, T. (2020). Evolution of orexin neuropeptide system: structure and function. Front. Neurosci. 14:691. doi: 10.3389/fnins.2020.00691
Soya, S., Shoji, H., Hasegawa, E., Hondo, M., Miyakawa, T., Yanagisawa, M., et al. (2013). Orexin receptor-1 in the locus coeruleus plays an important role in cue-dependent fear memory consolidation. J. Neurosci. 33, 14549–14557. doi: 10.1523/JNEUROSCI.1130-13.2013
Spinazzi, R., Andreis, P. G., Rossi, G. P., and Nussdorfer, G. G. (2006). Orexins in the regulation of the hypothalamic-pituitary-adrenal axis. Pharmacol. Rev. 58, 46–57. doi: 10.1124/pr.58.1.4
Stanojlovic, M., Pallais, J. P., Lee, M. K., and Kotz, C. M. (2019). Pharmacological and chemogenetic orexin/hypocretin intervention ameliorates Hipp-dependent memory impairment in the A53T mice model of Parkinson’s disease. Mol. Brain 12:87. doi: 10.1186/s13041-019-0514-8
Tracik, F., and Ebersbach, G. (2001). Sudden daytime sleep onset in Parkinson’s disease: polysomnographic recordings. Mov. Disord. 16, 500–506. doi: 10.1002/mds.1083
Trivedi, P., Yu, H., MacNeil, D. J., Van der Ploeg, L. H., and Guan, X. M. (1998). Distribution of orexin receptor mRNA in the rat brain. FEBS Lett. 438, 71–75. doi: 10.1016/s0014-5793(98)01266-6
Tunisi, L., Forte, N., Fernandez-Rilo, A. C., Mavaro, I., Capasso, R., D’Angelo, L., et al. (2019). Orexin-A prevents lipopolysaccharide-induced neuroinflammation at the level of the intestinal barrier. Front. Endocrinol. 10:219. doi: 10.3389/fendo.2019.00219
Turkoglu, R., Benbir, G., Ozyurt, S., Arsoy, E., Akbayir, E., Turan, S., et al. (2020). Sleep disturbance and cognitive decline in multiple sclerosis patients with isolated optic neuritis as the first demyelinating event. Int. Ophthalmol. 40, 151–158. doi: 10.1007/s10792-019-01157-x
Wang, C., Wang, Q., Ji, B., Pan, Y., Xu, C., Cheng, B., et al. (2018). The orexin/receptor system: molecular mechanism and therapeutic potential for neurological diseases. Front. Mol. Neurosci. 11:220. doi: 10.3389/fnmol.2018.00220
Wyant, K. J., Ridder, A. J., and Dayalu, P. (2017). Huntington’s disease-update on treatments. Curr. Neurol. Neurosci. Rep. 17:33. doi: 10.1007/s11910-017-0739-9
Xie, L., Kang, H., Xu, Q., Chen, M. J., Liao, Y., Thiyagarajan, M., et al. (2013). Sleep drives metabolite clearance from the adult brain. Science 342, 373–377. doi: 10.1126/science.1241224
Yasui, K., Inoue, Y., Kanbayashi, T., Nomura, T., Kusumi, M., and Nakashima, K. (2006). CSF orexin levels of Parkinson’s disease, dementia with Lewy bodies, progressive supranuclear palsy and corticobasal degeneration. J. Neurol. Sci. 250, 120–123. doi: 10.1016/j.jns.2006.08.004
Yukitake, H., Fujimoto, T., Ishikawa, T., Suzuki, A., Shimizu, Y., Rikimaru, K., et al. (2019). TAK-925, an orexin 2 receptor-selective agonist, shows robust wake-promoting effects in mice. Pharmacol. Biochem. Behav. 187:172794. doi: 10.1016/j.pbb.2019.172794
Zajo, K. N., Fadel, J. R., and Burk, J. A. (2016). Orexin A-induced enhancement of attentional processing in rats: role of basal forebrain neurons. Psychopharmacology 233, 639–647. doi: 10.1007/s00213-015-4139-z
Zhang, B., Guo, D., Han, L., Rensing, N., Satoh, A., and Wong, M. (2019). Hypothalamic orexin and mechanistic target of rapamycin activation mediate sleep dysfunction in a mouse model of tuberous sclerosis complex. Neurobiol. Dis. 134:104615. doi: 10.1016/j.nbd.2019.104615
Keywords: orexinergic system, Alzheimer’s disease, Parkinson’s disease, Huntington’s disease, multiple sclerosis
Citation: Wang Q, Cao F and Wu Y (2021) Orexinergic System in Neurodegenerative Diseases. Front. Aging Neurosci. 13:713201. doi: 10.3389/fnagi.2021.713201
Received: 22 May 2021; Accepted: 20 July 2021;
Published: 17 August 2021.
Edited by:
Yasmina Manso, Center for Biomedical Research on Neurodegenerative Diseases (CIBERNED), SpainReviewed by:
Marian Henryk Lewandowski, Jagiellonian University, PolandJim R. Fadel, University of South Carolina, United States
Copyright © 2021 Wang, Cao and Wu. This is an open-access article distributed under the terms of the Creative Commons Attribution License (CC BY). The use, distribution or reproduction in other forums is permitted, provided the original author(s) and the copyright owner(s) are credited and that the original publication in this journal is cited, in accordance with accepted academic practice. No use, distribution or reproduction is permitted which does not comply with these terms.
*Correspondence: Yili Wu, eWlsaV93dTIwMDRAeWFob28uY2E=; d3V5aWxpQHdtdS5lZHUuY2E=