- 1Department of Rehabilitation Medicine, Ruijin Hospital, Shanghai Jiao Tong University School of Medicine, Shanghai, China
- 2Department of Rehabilitation Medicine, Shanghai Ruijin Rehabilitation Hospital, Shanghai, China
- 3Department of Neurology, Duke University School of Medicine, Durham, NC, United States
Objectives: Either motor training or repetitive transcranial magnetic stimulation (rTMS) could modulate the neural plasticity after stroke. Therefore, synchronizing the two interventions may optimize the efficiency of recovery. In the present study, we aim to investigate the effect of rTMS along with hand grip training on the neurobehavioral and hand functional recovery in one cohort of subacute stroke patients.
Methods: Thirty-nine stroke patients were enrolled in a single-center, single-blinded, randomized clinical trial. We tested different intervention effects of rTMS and hand grip training (group A), rTMS alone (group B), and hand grip training alone (group C). For the rTMS-treated groups, patients received 10 consecutive sessions of 5-Hz stimulation over the affected hemisphere with 750 pulses. Jebsen–Taylor Hand Function Test (JTHFT), Fugl-Meyer assessment of upper extremity (FMA-UE), grip strength, modified Barthel index (mBI), and ipsilesional motor evoked potential (iMEP) latency were assessed and compared across the groups.
Results: We found that only rTMS along with hand grip training group all improved in JTHFT, FMA-UE, grip strength, and mBI (p ≤ 0.01) compared with the baseline among the three groups. Furthermore, this study demonstrated that rTMS plus hand grip training had much better results in improvement of neurobehavioral outcomes compared to the rTMS alone- and hand grip training alone-treated patients (p < 0.05). However, no significant differences were detected in neurophysiologic outcome between intra-groups and inter-groups (p > 0.05).
Conclusion: These proof-of-concept results suggested that rTMS alone with hand grip training was a unique approach to promote hand functional recovery in stroke patients. It provided important information to design a large-scale multi-center clinical trial to further demonstrate the efficiency of the combination of central and peripheral stimulation.
Clinical Trial Registration: http://www.chictr.org.cn (#ChiCTR1900023443).
Introduction
Despite intensive neurorehabilitation efforts, hand- and finger-related functional abilities remain unsatisfactory following a neurological event. Studies demonstrated that 27% of stroke patients lose integral hand function due to hemiplegia (Fischer et al., 2007). It was no surprise that one of the most commonly expressed goals of individuals who sustained stroke was to engage in neurorehabilitation interventions that could enhance hand function. For stroke survivors, therefore, improving related hand functional abilities and promoting the hand function become crucial for optimal social participation and daily life (Higgins et al., 2013). Previous studies demonstrated that the establishment of neural plasticity after stroke is essential for the motor recovery (Hermann and Chopp, 2012). Furthermore, motor training is capable of promoting neuroplasticity to attenuate the motor dysfunction in stroke patients (Kleim et al., 2003; Adkins et al., 2006). For instance, hand grip training could be beneficial for the finger flexor and extensor tendon to recruit more motor units and improve the innervation and functional neuroplasticity, which played an important role in the motor recovery of stroke survivors (Murphy and Corbett, 2009). From the view of previous trials, hand functional training could regulate the neuronal excitatory input in the nerve reflex circuits and accelerate the process of reorganization of brain connectivity and neuroplasticity (Wolf et al., 2006; Buma et al., 2010).
Repetitive transcranial magnetic stimulation (rTMS) could re-balance inter-hemisphere inhibition (IHI) by either up-regulating or down-regulating cortical excitability (Nowak et al., 2009). The influence of stroke is mainly restricted to the affected hemisphere, and facilitating affected M1 directly might produce more enhancement of motor recovery than suppressing the unaffected M1 excitability (McDonnell and Stinear, 2017). Despite rTMS representing an ideal approach to promote neural plasticity (Fregni et al., 2006; Bolognini et al., 2009), whether the combination of rTMS and motor training could enhance the therapeutic effect and prolong the effective period was largely unknown. The application of motor training combined with rTMS over targeted motor cortex in healthy subjects and chronic stroke patients showed strong support both in the concept (Morris, 1999) and initial experimental evidence (Bütefisch et al., 2004; Izumi et al., 2008; Massie et al., 2013a). However, the brain tissue repair process was more complicated especially in the subacute phase. Providing corresponding proof was necessary and timely.
We aim to investigate the combined effect of high-frequency rTMS (HF-rTMS) and hand grip training on the impaired hand functional recovery in stroke survivors. We hypothesized that HF-rTMS, along with hand grip training, induces significant hand functional recovery in subacute stroke patients as compared to the controls, which was related to the establishment of functional neuroplasticity.
Materials and Methods
General Information
The study protocol was approved by the Institutional Ethics Review Board, Shanghai Ruijin Rehabilitation Hospital, Shanghai, China. The clinical trial was registered in the Chinese Clinical Trial Registry (ChiCTR) with the registration number ChiCTR1900023443. All enrolled patients signed the informed consent prior to the enrollment to this study.
Thirty-nine hemiparetic stroke patients were enrolled at Shanghai Ruijin Rehabilitation Hospital from March 2019 to December 2019. The criteria of enrollment were as follows: (1) confirmed clinical stroke diagnosis, which was based on the Fourth National Conference on Cerebrovascular Diseases in 1995; (2) the first ischemic or hemorrhagic stroke confirmed by CT or MRI scans; (3) 1 to 6 months from stroke onset; (4) age from 40 to 75 years old; (5) Brunnstrom of hemiplegic upper limb and hand staging from 4 to 5; (6) Mini Mental State Examination (MMSE) > 20/30; and (7) informed consent to the study and signed the consent of rTMS. The exclusion criteria were as follows: (1) uncontrolled hypertension; (2) history of seizure or using epileptic drugs either before or after stroke; (3) heart, lung, liver, kidney, or other essential organ functional decline or failure; (4) aphasia, ipsilateral neglect, hemianopia, or affective disorder that affects participant’s ability to comply with study procedure; and (5) known risk factors for TMS such as having a pacemaker, intracranially implanted metal, skull defects, etc.
This study was a randomized, well-designed and controlled, prospective clinical trial conducted at a single center, which included four phases: (1) baseline evaluation, (2) randomization, (3) intervention, and (4) post-intervention evaluation. Thirty-nine stroke patients were randomly assigned by a number generated from a computer randomization table. All of the participants who met the criteria were assigned to three groups (groups A, B, and C) on the basis of the random numbers. Thirty-nine stroke patients with mild motor dysfunction recruited from inpatients and outpatients were randomized into rTMS with hand grip training (group A), rTMS alone (group B), and hand grip training alone (group C). The clinical demographic characteristics of participants are listed in Table 1.
Intervention
Intervention lasted 10 days with two interventions: (1) 5 min of real/sham 5-Hz rTMS. Each session consisted of 5-Hz rTMS for 1 s, which was both preceded and followed by a resting period of 1 s (total time = 150 s); and (2) 5 min of hand grip training. The hand grip training is composed of a repeated 1-Hz rhythmic voluntary grip by holding a ball. Apart from these, all of the participants received the conventional rehabilitation, which involved physical therapy and occupational therapy for 120 min daily for 10 sessions (5 days/week for 2 weeks). The interventions were required to be steadily implemented after conventional rehabilitation. It was worth mentioning that the practice of standardized conventional rehabilitation was requested to avoid involving evaluation projects.
During each intervention, patients seated in a comfortable and adjustable chair with headrest and armrests and took a comfortable supine position, maintaining the head and neck without displacement. Patients were instructed not to move their heads during the treatment period. The upper limb of the unaffected side was naturally placed on the armrest of the seat, and the upper limb of the affected side was placed on the side of the body.
A TMS device code with CCY-IV (Yi Ruide Company, Wuhan, China) with a 90-mm figure-of-eight coil was utilized for rTMS. rTMS intervention and evaluation were conducted in a quiet room and implemented by a trained research staff member. Motor evoked potential (MEP) signals were recorded by an electromyography (EMG) instrument that was connected to the stimulator. Ag-AgCl surface electrodes were firstly placed over the abductor pollicis brevis (APB) muscle of the unaffected hand. rTMS was applied over M1 of the unaffected hemisphere according to electroencephalogram (EEG) 10/20, where the MEP was elicited. The resting motor threshold (RMT) of APB of the unaffected upper extremity was determined by decreasing or increasing the intensity from 50% in a stepwise manner. Plus, the RMT was defined as the minimal stimulus intensity that produced a MEP response of at least 50 μV amplitude with the APB muscle at rest in at least 5 of 10 subsequent stimulations (Rossini et al., 2015). We selected the optimal stimulation site (“hot spot”) in the unaffected hemisphere where the largest MEP could be consistently evoked, with the APB muscle at rest. Following that, the electrodes were placed on APB muscle of the affected hand when detecting the MEP signal of the injured hemisphere. If no MEP could be detected when stimulating over the affected M1, the optimal stimulation site was defined as the symmetric location to the “hot spot” of the unaffected hemisphere according to EEG 10/20. The MEP latency and amplitude of the targeted muscle were measured as the period (ms) between stimulus onset and the start of the largest MEP and their peak-to-peak (mV). The parameters of stimulation were 5 Hz, 100% RMT, and 750 pulses, for a total of 10 sessions (5 days/week for 2 weeks). The sham stimulation was conducted with the coil placed rotated 90°away from the scalp resulting in no current generated in the brain.
Evaluation
Measurements of Jebsen–Taylor Hand Function Test (JTHFT), Fugl-Meyer assessment of upper extremity (FMA-UE), grip strength, modified Barthel Index (mBI), and ipsilesional motor evoked potential (iMEP) latency were assessed at baseline and post-intervention. Primary outcome was administered by a skilled clinician to evaluate the total time of accomplishing the tasks of JTHFT. Secondary outcomes included arm and hand function, force, daily living ability, and cortical excitability that were recorded by the same evaluator.
Jebsen–Taylor Hand Function Test
The JTHFT assesses hand dexterity and consists of seven different subtasks: (1) writing a sentence, (2) turning cards, (3) moving small common objects, (4) simulating feeding, (5) stacking checkers, (6) picking up large light cans, and (7) moving heavy cans (Jebsen et al., 1969). The participants performed each task with the affected limbs and the duration of each task from onset (lifting hand from table) to the completion using a stopwatch to record in seconds (the maximal duration is 120 s per task) and summated as the total score (Radder et al., 2019). The result of JTHFT could explain three factors in relation to basic fine motor skills: motor coordination, speed of movement, and grip force scaling. Motor coordination includes tasks 1, 4, and 5. The speed of movement consists of tasks 2, 3, and 6. Task 7 reflects grip force scaling (Allgower and Hermsdorfer, 2017).
Fugl-Meyer Assessment of Upper Extremity
The FMA-UE included 33 items, and the score range was 0–66. Among them, the hand function part accounted for 24 points. Each item was recorded in an ordinal scale (0 represents severe impairment and 2 represents no impairment) (Gladstone et al., 2002).
Grip Strength
Grip strength was measured by a hand dynamometer (Mathiowetz et al., 1985). The subjects were requested to squeeze the handgrip of the dynamometer maximally for 5 s. Each participant had three attempts, and the interval between the attempts was at least 60 s rest. The highest grip strength was recorded for analysis in kilograms.
Modified Barthel Index
The mBI was recorded, which included personal hygiene, self-bathing, feeding, using the toilet, stair climbing, getting dressed, bowel control, bladder control, chair/bed transfer, and ambulation (Shah et al., 1989). The total score is 100 points.
iMEP Latency
The MEP latency reflected the conduction time for neural impulses from the cortex to peripheral muscles and suggested cortical excitability (Bestmann and Krakauer, 2015). The target peripheral muscle in this study was APB. The MEP latency of the targeted muscle was measured as the period (ms) between stimulus onset and the start of the largest MEP. We selected five MEPs per subject with stable waveforms and calculated the mean of the iMEP latency as the measurement of cortical excitability.
Statistical Analysis
According to the previous HF-rTMS studies, they suggested the sample size of n = 8 for each group to be adequate (Gladstone et al., 2002; Guo et al., 2016). Based on our research sample, we calculated the power of test statistic and the value was 0.84 (β = 0.16). Statistical analysis was performed in SPSS version 23.0. Shapiro-Wilk test was used to evaluate whether the assessment scores were normally distributed. Chi-squared test was used for categorical variables while one-way ANOVA or the nonparametric Kruskal–Wallis H test was utilized for continuous variables to compare among the three groups. A paired t test or Wilcoxon rank-sum test was performed to compare the values between baseline and post-intervention within each group. The change of FMA-UE and grip strength were analyzed by one-way ANOVA, while JTHFT, mBI, and iMEP latency changes were compared by nonparametric Kruskal–Wallis H test to determine the between-group differences. Data were presented as means ± standard deviation. p < 0.05 was considered statistically significant.
Results
Forty-four patients were assigned randomly to group A, B, or C. Five patients dropped out of the trial due to personal problems. Finally, thirty-nine patients completed the interventions without incident, severe side effects, or discomfort, indicating that the procedure was well tolerated (Figure 1). No significant differences in clinic–demographic characteristics and FMA-UE score were presented among the groups (Table 1).
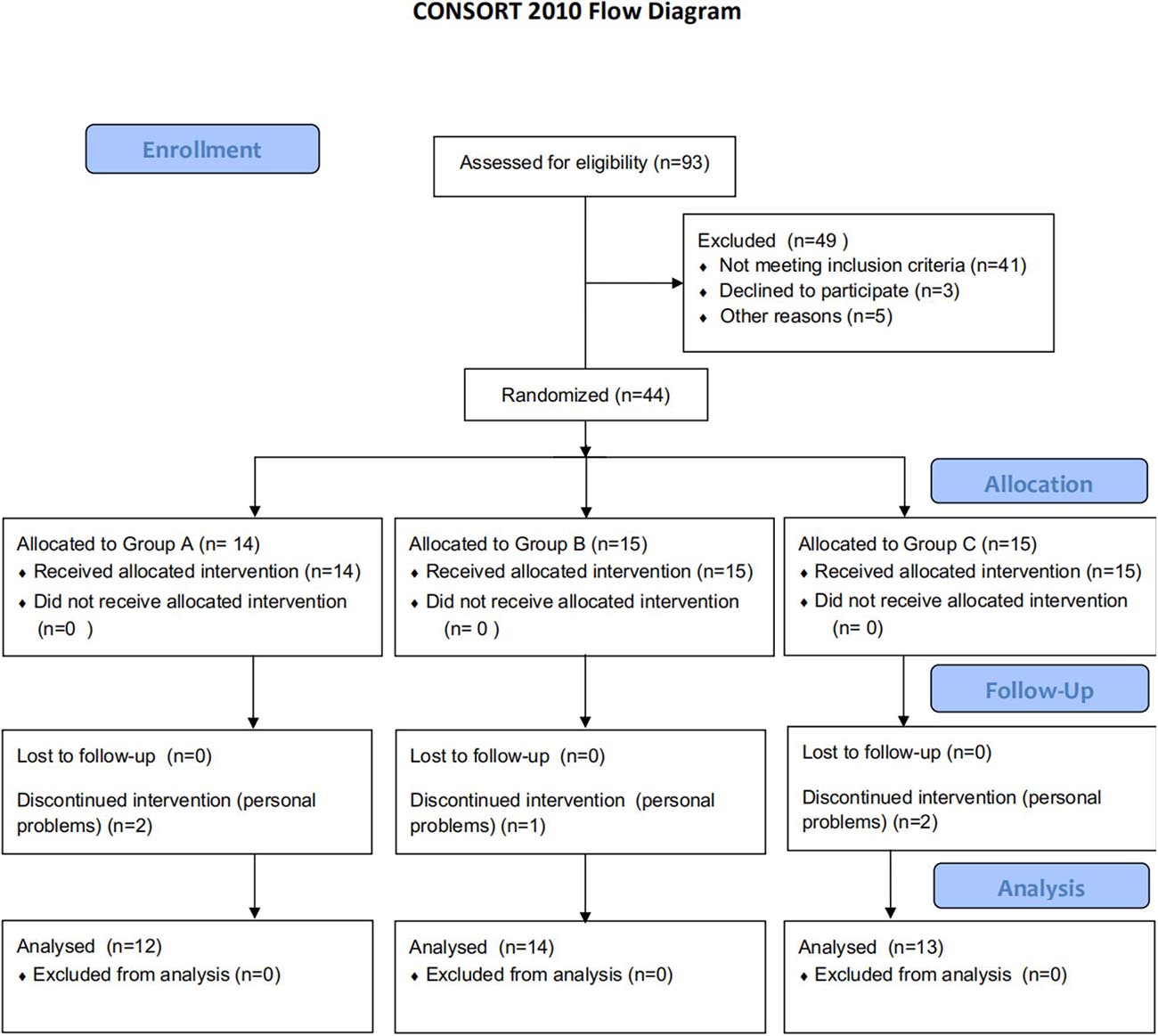
Figure 1. Flowchart of the trial. Group A: high-frequency rTMS during hand grip training; Group B: high-frequency rTMS alone; Group C: hand grip training alone; rTMS: repetitive transcranial magnetic stimulation. CONSORT flow diagram illustrates recruitments, group allocation, follow-up, and analysis.
Behavioral Measures
Jebsen–Taylor Hand Function Test
The change of JTHFT total score between baseline and post-intervention of the three groups (all p ≤ 0.01) were statistically significant (Table 2). Group A and group B also showed significant differences in JTHFT without writing, motor coordination, speed of movement, and grip force scaling (p < 0.05). Unfortunately, group C only showed significant difference in JTHFT without writing and motor coordination (p < 0.05), which, as mentioned above, indicated that both rTMS only and rTMS with hand grip training were effective in improving hand function in stroke patients. In addition, compared to group C, group A showed better enhancement in the JTHFT score (p < 0.05) (Table 3 and Figures 2A,B), suggesting that HF-rTMS combined with hand grip training was significantly greater than hand grip training only in hand function recovery among the stroke survivors.
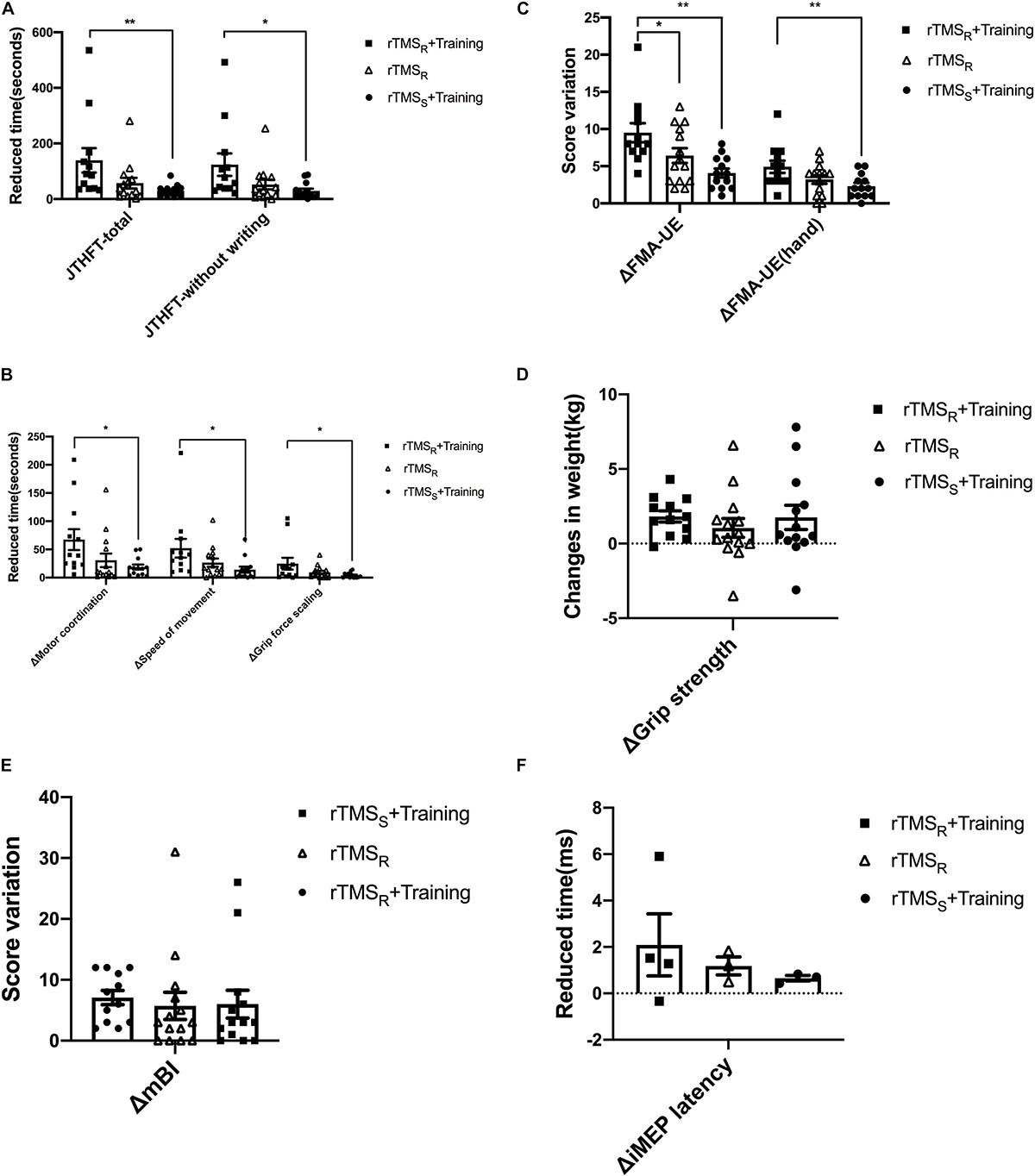
Figure 2. Behavioral and neurophysiological changes among the three groups (mean±SEM). rTMSR+Training: rTMS and hand grip training (Group A); rTMSR: rTMS alone (Group B); rTMSS+Training: hand grip training alone (Group C). rTMS: repetitive transcranial magnetic stimulation. One-way ANOVA or nonparametric Kruskal–Wallis H test was used to compare the behavioral and neurophysiological changes among the three groups, and multiple comparisons with the whole pairwise comparation. *p < 0.05, **p < 0.01.
Fugl-Meyer Assessment of Upper Extremity
Evaluation of FMA-UE and FMA-UE (hand) between baseline and post-intervention among the groups was significantly different (Table 2). The data showed that FMA-UE scores were greatly improved in all groups (p ≤ 0.01). Additionally, compared to group B (p < 0.05) and group C (p < 0.01), group A showed better potential in enhancing the score of FMA-UE and FMA-UE (hand) (Table 3 and Figure 2C), further suggesting that HF-rTMS with hand grip training was particularly effective in promoting hand and upper limb motor function in stroke patients with mild impairment.
Grip Strength
Significant improvement of grip strength was only found in group A after intervention (p ≤ 0.001), which indicated that HF-rTMS with hand grip training was capable of increasing power-grip force in stroke patients (Table 2). However, there were no significant differences found between groups in grip strength improvements (p > 0.05) (Table 3 and Figure 2D).
Modified Barthel Index
In the three groups, assessment of mBI revealed a significant increase at post- relative to pre-intervention (p < 0.05) in Table 2. However, no significant difference was detected between groups (p > 0.05) (Table 3 and Figure 2E).
Neurophysiological Measures
iMEP Latency
The number of iMEP latency detected in these three groups is displayed in the Supplementary Material 1. Both group A and group C increased one patient in the detected number of iMEP. Notably, the iMEP latency shortened in all groups with no significant difference (p > 0.05) (Supplementary Material 2). In addition, there was similarly no statistical difference presented between groups (p > 0.05) (Figure 2F and Supplementary Table 3).
Discussion
In this trial, we conducted a randomized and sham-controlled clinical research to detect the differential effects of HF-rTMS with hand grip training, HF-rTMS alone over the affected hemisphere, and hand grip training alone on the recovery of hand function in adult stroke patients suffering from mild impairment. From a clinical perspective, we found that all the three methods were beneficial to improve hand function and daily living ability in subacute adult stroke patients. Besides, we also detected that HF-rTMS with hand grip training method was superior to HF-rTMS alone and hand grip training alone. The results suggested that both central and peripheral stimulation were capable of enhancing hand functional recovery. Furthermore, our study provides evidence that the combination of central and peripheral stimulation could be the optimal approach for post-stroke rehabilitation and could increase the efficacy of rTMS.
Over the past decade, peripheral stimulation such as motor training was found favorable to promote injured cortical repair and remodeling via stimulating angiogenesis, neurogenesis, synaptogenesis, and dendritic plasticity (Zhang and Chopp, 2009). In recent years, numerous researches have been conducted to develop methods of improving the neurorehabilitation efficacy in stroke patients via non-invasive brain stimulation (Hummel and Cohen, 2006). rTMS, based on the principle of electromagnetic induction, induced the current generated by the coil placed over the surface of the skull to activate neurons in the cortical and subcortical regions, yielding neuronal depolarization (Kobayashi and Pascual-Leone, 2003). A single TMS stimulus could act on inhibitory or excitatory axons to depolarize them and deliver them backward. TMS induced changes in cell excitability and permeability (Ridding and Rothwell, 2007), which could influence cortical plasticity (Muller-Dahlhaus and Ziemann, 2015). Either rTMS or motor training could modulate neural plasticity and played a role in remodeling brain networks. In consequence, the hypothesis that the bond of rTMS and motor training might maximize their respective therapeutic effect had been proposed. Previous studies aiming to investigate the effect of combined rTMS and motor training were mainly time-locked, which referred to rTMS generally preceded or followed by motor training (Higgins et al., 2013; Kakuda et al., 2016). In recent several years, TMS was implemented during the procedure of motor training to further improve the efficacy of stroke motor function rehabilitation. These studies showed that coupling rTMS with motor training could have a synergic impact on motor recovery after stroke (Buetefisch et al., 2011; Massie et al., 2013a). The synergic effect could be explained by the rTMS-induced modulation of neural plasticity and the consolidation with motor training-induced (Buetefisch et al., 2011). Previous researches demonstrated that TMS plus motor training significantly improved the longevity of motor memory (Bütefisch et al., 2004) and hand function after stroke (Izumi et al., 2008). Another study revealed that functional rTMS (EMG-triggered rTMS) promoted greater excitatory changes and selectively modulated agonistic muscle activity (Massie et al., 2013a). Furthermore, consecutive multi-session functional rTMS could equally enhance cortical excitability and improve stabilities of motor skills (Massie et al., 2013b). However, the participants mainly included healthy subjects and chronic stroke patients, and the therapeutic frequency and intensity of rTMS were variable. Considering that the effect of rTMS on the cortical excitability is subject to intra-individual and inter-individual variability (Bestmann et al., 2010; Cohen et al., 2010), and that the optimal rTMS protocol is undetermined, developing more efficient clinical protocol that fits stroke patients with mild motor dysfunction is desirable and necessary. Our results provided a highly efficient approach to subacute stroke patients with mild dysfunction to promote hand function and useful experimental data for further larger-scale clinical trial.
Neuroplasticity presumably occurred in the connection between motor cortex neurons, which was naturally triggered during the period of muscle contraction, and simultaneously motivated over the motor cortex by rTMS (Edwardson et al., 2013). Considering our results from this standpoint, the rTMS plus hand grip training group was supposed to gain stronger excitability in the affected hemisphere. Unfortunately, the changes in the cortical excitability after HF-rTMS during hand grip training were not found. The following reasons may account for this result: the factors contributing to functional impairment included loss of white matter projection, diaschisis, and interhemispheric imbalance (Auriat et al., 2015). The detection method of recording iMEP latency was not able to find structural changes; other methods such as functional magnetic resonance imaging should be evaluated in future studies. According to the IHI model, which is based on interhemispheric imbalance, suppressing the unaffected hemisphere excitability or facilitating the affected hemisphere excitability could promote motor function recovery in stroke patients (Nowak et al., 2009). However, a novel model for neurorehabilitation named bimodal balance-recovery model suggested that the IHI model was oversimplified or even incorrect. The new model links interhemispheric balancing and functional recovery to the structural reserve spared by the lesion; it could be utilized to tailor treatment for individual patients (di Pino et al., 2014). Similarly, the small sample size could be unfavorable and has limited our study.
This trial was conducted safely with no seizures and other specific discomfort, and no incident happened. Thus, our results also provided additional evidence of the safety of implementing HF-rTMS during hand grip training and HF-rTMS alone in the clinical setting. Meanwhile, we concluded that HF-rTMS was an effective way to promote hand function in subacute adult stroke patients with mild impairment. In recent years, HF-rTMS has been reported to have a more effective impact when compared to low-frequency rTMS (LF-rTMS) in animal and human studies (Sasaki et al., 2013; Caglayan et al., 2019). An animal study detected that intervening 20-Hz HF-rTMS on acute and subacute ischemic injury models in mice induced excellent outcomes compared to the 1-Hz LF-rTMS. They simultaneously demonstrated that HF-rTMS decreased apoptosis and infarct volume; activated neurogenesis, neuronal survival, and neuronal plasticity; and increased regional cerebral blood flow. It has a strong support for the rationale of using HF-rTMS in post-stroke patients aiming at improving motor functional recovery (Caglayan et al., 2019). Besides, a clinical study targeted at stroke patients detected that 10-Hz HF-rTMS applied over the affected hemisphere induced significant improvement on motor functional recovery compared to the 1-Hz LF-rTMS (Sasaki et al., 2013). A meta-analysis revealed that facilitating affected M1 excitability could be directly beneficial compared to the suppressing unaffected M1 excitability in improving post-stroke recovery (McDonnell and Stinear, 2017). Future studies can focus on investigating the differential effect of LF-rTMS or HF-TMS plus hand grip training on hand functional recovery.
Though there was no significant difference detected between rTMS alone and hand grip training alone groups in FMA-UE score, the rTMS alone group showed enhancement in FMA-UE and the change had a minimal clinically important difference (Page et al., 2012). The short intervention period (10 sessions lasting 2 weeks) could explain the reason of no significant difference in grip strength among the groups (Stock et al., 2019). However, focusing on post-stroke patients with mild motor impairments, having no significant difference among these groups in mBI might be due to the sensitivity of the measurement.
The limitations of our study are as follows: (1) this study was a single-center trial and lacked sufficient subjects to administer subgroup analysis according to the lesion, stroke volume, the course of disease, and the severity of stroke; (2) we collected data to evaluate the short-term benefit from the proposed interventions, but we did not collect long-term data to assess the sustained benefits; and (3) the proportion of patients with positive MEP was lower than prior reported studies, and it limited our understanding of the mechanism of motor improvement by rTMS. Further larger-scale clinical trials are necessary to confirm our data and to promote this novel rehabilitation therapy.
Conclusion
Our study demonstrated the short-term benefit of combined 10 sessions of 5-Hz rTMS over the affected hemisphere with concurrent hand grip training protocol. It provided important preliminary data to plan a large-sample, multi-center study to systematically evaluate the benefit of rTMS in a stroke population.
Data Availability Statement
The original contributions presented in the study are included in the article/Supplementary Material, further inquiries can be directed to the corresponding author/s.
Ethics Statement
The studies involving human participants were reviewed and approved by Institutional Ethics Review Board, Shanghai Ruijin Rehabilitation Hospital, Shanghai, China. The patients/participants provided their written informed consent to participate in this study.
Author Contributions
JW and QX: conception and design of the study and data confirmation. YY: data collection, analysis of data, statistical analysis, and drafting the manuscript. HP: data segmentation and analysis of data. WP and YL: evaluating the patients and statistical analysis. XS and CN: acquisition of data. WF: analysis and interpretation of data. All authors contributed to the study and article and approved the submitted version. All authors contributed to the article and approved the submitted version.
Funding
This work was supported by Shanghai Municipal Key Clinical Specialty (Grant No. SHSLCZDZK02701, QX), Shanghai Jiao Tong University School of Medicine—Institute of Neuroscience, Chinese Academy of Sciences, Leading Startup Project of Brain Diseases Clinical Research Center (Grant No. 2017NKX002, QX), Shanghai Science and Technology Commission (Grant No. 18511108203, QX), Shanghai Municipal Health Commission (Grant No. 2019SY004, QX), and the National Natural Science Foundation of China (Grant No. 81802232, JW).
Conflict of Interest
The authors declare that the research was conducted in the absence of any commercial or financial relationships that could be construed as a potential conflict of interest.
Acknowledgments
We thank our colleagues at the Department of Rehabilitation Medicine of Ruijin Hospital and Shanghai Ruijin Rehabilitation Hospital.
Supplementary Material
The Supplementary Material for this article can be found online at: https://www.frontiersin.org/articles/10.3389/fnagi.2021.636184/full#supplementary-material
References
Adkins, D. L., Boychuk, J., Remple, M. S., and Kleim, J. A. (2006). Motor training induces experience-specific patterns of plasticity across motor cortex and spinal cord. J. Appl. Physiol. 101, 1776–1782. doi: 10.1152/japplphysiol.00515.2006
Allgower, K., and Hermsdorfer, J. (2017). Fine motor skills predict performance in the Jebsen Taylor Hand Function Test after stroke. Clin. Neurophysiol. 128, 1858–1871. doi: 10.1016/j.clinph.2017.07.408
Auriat, A. M., Neva, J. L., Peters, S., Ferris, J. K., and Boyd, L. A. (2015). A review of transcranial magnetic stimulation and multimodal neuroimaging to characterize post-stroke neuroplasticity. Front. Neurol. 6:226. doi: 10.3389/fneur.2015.00226
Bestmann, S., and Krakauer, J. W. (2015). The uses and interpretations of the motor-evoked potential for understanding behaviour. Exp. Brain Res. 233, 679–689. doi: 10.1007/s00221-014-4183-7
Bestmann, S., Swayne, O., Blankenburg, F., Ruff, C. C., Teo, J., Weiskopf, N., et al. (2010). The role of contralesional dorsal premotor cortex after stroke as studied with concurrent TMS-fMRI. J. Neurosci. 30, 11926–11937. doi: 10.1523/JNEUROSCI.5642-09.2010
Bolognini, N., Pascual-Leone, A., and Fregni, F. (2009). Using non-invasive brain stimulation to augment motor training-induced plasticity. J. Neuroeng. Rehabil. 6:8. doi: 10.1186/1743-0003-6-8
Buetefisch, C., Heger, R., Schicks, W., Seitz, R., and Netz, J. (2011). Hebbian-type stimulation during robot-assisted training in patients with stroke. Neurorehabil. Neural Repair 25, 645–655. doi: 10.1177/1545968311402507
Buma, F. E., Lindeman, E., Ramsey, N. F., and Kwakkel, G. (2010). Review: functional neuroimaging studies of early upper limb recovery after stroke: a systematic review of the literature. Neurorehabil. Neural Repair 24, 589–608. doi: 10.1177/1545968310364058
Bütefisch, C. M., Khurana, V., Kopylev, L., and Cohen, L. G. (2004). Enhancing encoding of a motor memory in the primary motor cortex by cortical stimulation. J. Neurophysiol. 91, 2110–2116. doi: 10.1152/jn.01038.2003
Caglayan, A. B., Beker, M. C., Caglayan, B., Yalcin, E., Caglayan, A., Yulug, B., et al. (2019). Acute and post-acute neuromodulation induces stroke recovery by promoting survival signaling, neurogenesis, and pyramidal tract plasticity. Front. Cell. Neurosci. 13:144. doi: 10.3389/fncel.2019.00144
Cohen, D. A., Freitas, C., Tormos, J. M., Oberman, L., Eldaief, M., and Pascual-Leone, A. (2010). Enhancing plasticity through repeated rTMS sessions: the benefits of a night of sleep. Clin. Neurophysiol. 121, 2159–2164. doi: 10.1016/j.clinph.2010.05.019
di Pino, G., Pellegrino, G., Assenza, G., Capone, F., Ferreri, F., Formica, D., et al. (2014). Modulation of brain plasticity in stroke: a novel model for neurorehabilitation. Nat. Rev. Neurol. 10, 597–608. doi: 10.1038/nrneurol.2014.162
Edwardson, M. A., Lucas, T. H., Carey, J. R., and Fetz, E. E. (2013). New modalities of brain stimulation for stroke rehabilitation. Exp. Brain Res. 224, 335–358. doi: 10.1007/s00221-012-3315-1
Fischer, H. C., Stubblefield, K., Kline, T., Luo, X., Kenyon, R. V., and Kamper, D. G. (2007). Hand rehabilitation following stroke: a pilot study of assisted finger extension training in a virtual environment. Top. Stroke Rehabil. 14, 1–12. doi: 10.1310/tsr1401-1
Fregni, F., Boggio, P. S., Valle, A. C., Rocha, R. R., Duarte, J., Ferreira, M. J. L., et al. (2006). A sham-controlled trial of a 5-day course of repetitive transcranial magnetic stimulation of the unaffected hemisphere in stroke patients. Stroke 37, 2115–2122. doi: 10.1161/01.STR.0000231390.58967.6b
Gladstone, D. J., Danells, C. J., and Black, S. E. (2002). The fugl-meyer assessment of motor recovery after stroke: a critical review of its measurement properties. Neurorehabil. Neural Repair 16, 232–240. doi: 10.1177/154596802401105171
Guo, Z., Jin, Y., Peng, H., Xing, G., Liao, X., Wang, Y., et al. (2016). Ipsilesional high frequency repetitive transcranial magnetic stimulation add-on therapy improved diffusion parameters of stroke patients with motor dysfunction: a preliminary DTI study. Neural Plast. 2016:6238575. doi: 10.1155/2016/6238575
Hermann, D. M., and Chopp, M. (2012). Promoting brain remodelling and plasticity for stroke recovery: therapeutic promise and potential pitfalls of clinical translation. Lancet Neurol. 11, 369–380. doi: 10.1016/S1474-4422(12)70039-X
Higgins, J., Koski, L., and Xie, H. (2013). Combining rTMS and task-oriented training in the rehabilitation of the arm after stroke: a pilot randomized controlled trial. Stroke Res. Treat. 2013:539146. doi: 10.1155/2013/539146
Hummel, F. C., and Cohen, L. G. (2006). Non-invasive brain stimulation: a new strategy to improve neurorehabilitation after stroke? Lancet Neurol. 5, 708–712. doi: 10.1016/S1474-4422(06)70525-7
Izumi, S.-I., Kondo, T., and Shindo, K. (2008). Transcranial magnetic stimulation synchronized with maximal movement effort of the hemiplegic hand after stroke: a double-blinded controlled pilot study. J. Rehabil. Med. 40, 49–54. doi: 10.2340/16501977-0133
Jebsen, R. H., Taylor, N., Trieschmann, R. B., Trotter, M. J., and Howard, L. A. (1969). An objective and standardized test of hand function. Arch. Phys. Med. Rehabil. 50, 311–319.
Kakuda, W., Abo, M., Sasanuma, J., Shimizu, M., Okamoto, T., Kimura, C., et al. (2016). Combination protocol of low-frequency rTMS and intensive occupational therapy for post-stroke upper limb hemiparesis: a 6-year experience of more than 1700 Japanese patients. Transl. Stroke Res. 7, 172–179. doi: 10.1007/s12975-016-0456-8
Kleim, J. A., Jones, T. A., and Schallert, T. (2003). Motor enrichment and the induction of plasticity before or after brain injury. Neurochem. Res. 28, 1757–1769. doi: 10.1023/a:1026025408742
Kobayashi, M., and Pascual-Leone, A. (2003). Transcranial magnetic stimulation in neurology. Lancet Neurol. 2, 145–156. doi: 10.1016/S1474-4422(03)00321-1
Massie, C. L., Tracy, B. L., and Malcolm, M. P. (2013a). Functional repetitive transcranial magnetic stimulation increases motor cortex excitability in survivors of stroke. Clin. Neurophysiol. 124, 371–378. doi: 10.1016/j.clinph.2012.07.026
Massie, C. L., Tracy, B. L., Paxton, R. J., and Malcolm, M. P. (2013b). Repeated sessions of functional repetitive transcranial magnetic stimulation increases motor cortex excitability and motor control in survivors of stroke. NeuroRehabilitation 33, 185–193. doi: 10.3233/NRE-130944
Mathiowetz, V., Kashman, N., Volland, G., Weber, K., Dowe, M., and Rogers, S. (1985). Grip and pinch strength: normative data for adults. Arch. Phys. Med. Rehabil. 66, 69–74.
McDonnell, M. N., and Stinear, C. M. (2017). TMS measures of motor cortex function after stroke: a meta-analysis. Brain Stimul. 10, 721–734. doi: 10.1016/j.brs.2017.03.008
Morris, R. G. M. (1999). D.O. Hebb: the organization of behavior, Wiley: New York; 1949. Brain Res. Bull. 50:437. doi: 10.1016/S0361-9230(99)00182-3
Muller-Dahlhaus, F., and Ziemann, U. (2015). Metaplasticity in human cortex. Neuroscientist 21, 185–202. doi: 10.1177/1073858414526645
Murphy, T. H., and Corbett, D. (2009). Plasticity during stroke recovery: from synapse to behaviour. Nat. Rev. Neurosci. 10, 861–872. doi: 10.1038/nrn2735
Nowak, D. A., Grefkes, C., Ameli, M., and Fink, G. R. (2009). Interhemispheric competition after stroke: brain stimulation to enhance recovery of function of the affected hand. Neurorehabil. Neural Repair 23, 641–656. doi: 10.1177/1545968309336661
Page, S. J., Fulk, G. D., and Boyne, P. (2012). Clinically important differences for the upper-extremity Fugl-Meyer Scale in people with minimal to moderate impairment due to chronic stroke. Phys. Ther. 92, 791–798. doi: 10.2522/ptj.20110009
Radder, B., Prange-Lasonder, G. B., Kottink, A. I. R., Holmberg, J., Sletta, K., van Dijk, M., et al. (2019). Home rehabilitation supported by a wearable soft-robotic device for improving hand function in older adults: a pilot randomized controlled trial. PLoS One 14:e0220544. doi: 10.1371/journal.pone.0220544
Ridding, M. C., and Rothwell, J. C. (2007). Is there a future for therapeutic use of transcranial magnetic stimulation? Nat. Rev. Neurosci. 8, 559–567. doi: 10.1038/nrn2169
Rossini, P. M., Burke, D., Chen, R., Cohen, L. G., Daskalakis, Z., Di Iorio, R., et al. (2015). Non-invasive electrical and magnetic stimulation of the brain, spinal cord, roots and peripheral nerves: basic principles and procedures for routine clinical and research application. An updated report from an I.F.C.N. Committee. Clin. Neurophysiol. 126, 1071–1107. doi: 10.1016/j.clinph.2015.02.001
Sasaki, N., Mizutani, S., Kakuda, W., and Abo, M. (2013). Comparison of the effects of high- and low-frequency repetitive transcranial magnetic stimulation on upper limb hemiparesis in the early phase of stroke. J. Stroke Cerebrovasc. Dis. 22, 413–418. doi: 10.1016/j.jstrokecerebrovasdis.2011.10.004
Shah, S., Vanclay, F., and Cooper, B. (1989). Improving the sensitivity of the Barthel Index for stroke rehabilitation. J. Clin. Epidemiol. 42, 703–709. doi: 10.1016/0895-4356(89)90065-6
Stock, R., Thrane, G., Askim, T., Anke, A., and Mork, P. J. (2019). Development of grip strength during the first year after stroke. J. Rehabil. Med. 51, 248–256. doi: 10.2340/16501977-2530
Wolf, S. L., Winstein, C. J., Miller, J. P., Taub, E., Uswatte, G., Morris, D., et al. (2006). Effect of constraint-induced movement therapy on upper extremity function 3 to 9 months after strokethe EXCITE randomized clinical trial. JAMA 296, 2095–2104. doi: 10.1001/jama.296.17.2095
Keywords: hand function, neuro-modulation, stroke, transcranial magnetic stimulation, rehabilitation
Citation: Yang Y, Pan H, Pan W, Liu Y, Song X, Niu CM, Feng W, Wang J and Xie Q (2021) Repetitive Transcranial Magnetic Stimulation on the Affected Hemisphere Enhances Hand Functional Recovery in Subacute Adult Stroke Patients: A Randomized Trial. Front. Aging Neurosci. 13:636184. doi: 10.3389/fnagi.2021.636184
Received: 01 December 2020; Accepted: 09 March 2021;
Published: 19 May 2021.
Edited by:
Yuanli Zhao, Capital Medical University, ChinaReviewed by:
Laura Mordillo-Mateos, National Paraplegic Hospital, SpainHenri Leinonen, University of California, Irvine, United States
Copyright © 2021 Yang, Pan, Pan, Liu, Song, Niu, Feng, Wang and Xie. This is an open-access article distributed under the terms of the Creative Commons Attribution License (CC BY). The use, distribution or reproduction in other forums is permitted, provided the original author(s) and the copyright owner(s) are credited and that the original publication in this journal is cited, in accordance with accepted academic practice. No use, distribution or reproduction is permitted which does not comply with these terms.
*Correspondence: Jixian Wang, d2FuZ2ppeGlhbjZAMTYzLmNvbQ==; Qing Xie, cnVpamluX3hxQDE2My5jb20=
†These authors have contributed equally to this work and share first authorship