- 1Instituto de Investigación en Medicina Molecular y Celular Aplicada (IMMCA) (UNT-CONICET-SIPROSA), Tucumán, Argentina
- 2Department of Experimental Neurodegeneration, Center for Biostructural Imaging of Neurodegeneration, University Medical Center Goettingen, Goettingen, Germany
- 3Laboratory of Neuroanatomy and Experimental Neurology, Department of Morphological Sciences, Center for Research in Molecular Medicine and Chronic Diseases (CIMUS), Instituto de Investigación Sanitaria de Santiago de Compostela (IDIS), University of Santiago de Compostela, Santiago de Compostela, Spain
- 4Networking Research Center on Neurodegenerative Diseases (CIBERNED), Madrid, Spain
- 5Sorbonne Université, Paris Brain Institute -ICM, Institut National de la Santé et de la Recherche Médicale (INSERM), Centre National de la Recherche Scientifique (CNRS), Assistance Publique - Hôpitaux de Paris (APHP), Hôpital de la Pitié Salpêtrière, Paris, France
- 6German Center for Neurodegenerative Diseases Deutsches Zentrum für Neurodegenerative Erkrankungen (DZNE), Göttingen, Germany
- 7Department for NMR-based Structural Biology, Max Planck Institute for Biophysical Chemistry, Göttingen, Germany
- 8Physiology- Dental School of Ribeirão Preto, University of São Paulo, São Paulo, Brazil
- 9Max Planck Institute for Experimental Medicine, Goettingen, Germany
- 10Translational and Clinical Research Institute, Faculty of Medical Sciences, Newcastle University, Newcastle upon Tyne, United Kingdom
Tauopathies are neurodegenerative disorders with increasing incidence and still without cure. The extensive time required for development and approval of novel therapeutics highlights the need for testing and repurposing known safe molecules. Since doxycycline impacts α-synuclein aggregation and toxicity, herein we tested its effect on tau. We found that doxycycline reduces amyloid aggregation of the 2N4R and K18 isoforms of tau protein in a dose-dependent manner. Furthermore, in a cell free system doxycycline also prevents tau seeding and in cell culture reduces toxicity of tau aggregates. Overall, our results expand the spectrum of action of doxycycline against aggregation-prone proteins, opening novel perspectives for its repurposing as a disease-modifying drug for tauopathies.
Introduction
Tauopathies are a group of neurodegenerative diseases characterized by clinical heterogeneity and progressive deposition of amyloid aggregates of abnormally hyper-phosphorylated tau protein within specific brain regions (Lee et al., 2001). In healthy neurons, tau is the major microtubule associated protein (MAP), and plays a crucial role in regulating its dynamics, concomitant axonal transport and neurite outgrowth (Iqbal et al., 2010).
From a biochemical point of view, tau is an intrinsically disordered protein (IDP) with an N-terminal “projection domain” that projects away from microtubules (Hirokawa et al., 1988) and a positively charged C-terminal domain that binds tubulin and promotes self-assembly (Steiner et al., 1990; Dehmelt and Halpain, 2004; Shammas et al., 2015). Six tau isoforms are expressed in the human brain as a consequence of the alternative splicing. This in turn, leads to the expression of the tau proteins 0N3R, 1N3R, 2N3R, 0N4R, 1N4R, and 2N4R, N being the number of 29 amino acid inserts in the N-terminal region of tau and R the microtubule binding repeats (Iqbal et al., 2010).
In a physiological context, tau is regulated by site-specific phosphorylation. However, in pathological conditions, abnormal phosphorylation and aggregation lead to the formation of tau amyloid aggregates called paired helical filaments (PHFs), which ultimately lead to the build-up of cytoplasmic neurofibrillary tangles (NFT) (Kidd, 1963). Although the topographical distribution patterns of the lesions that contain NFTs correlate with the clinical progression of tauopathies such as Alzheimer's disease (AD) (Braak and Braak, 1991), the mechanisms of tau-associated neurodegeneration remain unclear. Likewise, despite the fact that the relationship between tau phosphorylation and aggregation was found to play a central role in the transition from its native state to the pathological form (Grundke-Iqbal et al., 1986; Goedert et al., 1996; von Bergen et al., 2000; Pérez et al., 2002; Mocanu et al., 2008), the relative contribution of each process to disease etiology and progression is poorly understood.
Unfortunately, albeit tremendous efforts and massive investments, therapies capable of preventing, halting, or at least slowing the progression of tau-associated disorders are not available (Panza et al., 2019). In this context, the aging of the human population, the main risk factor for AD and other neurodegenerative diseases, threatens to burden healthcare systems worldwide (Akushevich et al., 2013). Therefore, it is urgent to develop disease-modifying therapies, a process that is proving extremely difficult and takes many years from bench to bedside.
An alternative strategy that would significantly reduce time and costs is “drug repurposing,” which involves the use of pre-existing and approved drugs for new indications (Ashburn and Thor, 2004). Compelling evidence show that many antibiotics, i.e., minocycline and doxycycline (Smith and Leyden, 2005), aside from their antimicrobial action, are capable of halting the noxious amyloid aggregation process of disease-associated proteins (Ward et al., 2011; D'Souza et al., 2017; Socias et al., 2018; Balducci and Forloni, 2019; Gautieri et al., 2019). Therefore, this positions them as promising alternatives for the development of efficient therapies against neurodegenerative disorders. In this regard, we recently demonstrated the ability of doxycycline to reshape early oligomers of α-synuclein, preventing the buildup of pathogenic species and ultimately redirecting the process toward non-toxic off-pathway oligomers (González-Lizárraga et al., 2017).
In the present work, we studied the effect of doxycycline on tau amyloid aggregation pathway and its associated toxicity. By using heparin-induced 2N4R tau fibrillization as well as 4R truncated species that undergo self-aggregation, we assessed the capacity of doxycycline to hinder the tau amyloid pathway. Our results suggest the relevance of tau microtubule binding domain in doxycycline:tau interaction. Additionally, doxycycline also halted the ability of tau seeds to recruit monomers, which is essential for the progression of the pathology. By using cell culture, we also demonstrate the ability of this tetracycline to abate the toxicity related with tau-aggregated species. Present results, with the well-known brain bioavailability, safety, anti-inflammatory and antioxidant abilities of doxycycline, endorse this old drug as an ideal compound to be repurposed for tauopathies.
Materials and Methods
Preparation of Heterologous 2N4R Tau
Expression and purification of recombinant human tau was performed as previously described by Barghorn et al. (2005) using the plasmid tau/pET29b (Addgene, #16316) and E. coli BL21 [DE3]. Briefly, the purity of the protein was assessed by SDS-PAGE. Monomeric tau stock solutions were prepared in 40 mM Tris-HCl, 20 mM MgCl2 pH 7.5 with 0.05% DTT (freshly added). Prior to measurements, protein solutions were centrifuged for 30 min at 12,000 × g and filtered with a 0.22 μm filter (Millex-GV. Millipore). Protein concentration was determined by the measurement of absorbance at 280 nm using extinction coefficient ε = 7,700 cm−1. M−1. Tau/pET29b was a gift from Peter Klein (Addgene plasmid # 16316; http://n2t.net/addgene:16316; RRID:Addgene_16316) (Hedgepeth et al., 1997).
Protein Aggregation
Monomeric tau (22 μM) in 40 mM Tris-HCl, 20 mM MgCl2 pH 7.5 and fresh 0.05% DTT, was incubated in a Thermomixer Comfort® (Eppendorf) at 37°C under orbital agitation at 600 rpm; using 0.2 mg/ml heparin, in the absence or presence of doxycycline.
Thioflavin T Assay
Formation of cross-β structures during tau aggregation was followed by addition of Thioflavin T (ThT) fluorescent probe on aliquots withdrawn from the incubation mixture at different times, according to LeVine (1993, 1999). Changes in the emission fluorescence spectra with the excitation wavelength set at 450 nm were monitored using an ISS (Champaign, IL) PC1 spectrofluorometer. Doxycycline dose-response assay on tau aggregation was fitted to the equation:
where IF is the normalized fluorescence intensity, [Ligand] is doxycycline concentration, and IC50 is the concentration at which aggregation is inhibited at a 50%.
Congo Red Binding Assay
Fresh solution of Congo red (CR) dye with 10 mM in 40 mM Tris-HCl, 20 mM MgCl2 (pH 7.5) was diluted from the stock solution prior to use. The stock solution was prepared as described by Klunk et al. (1989, 1999). Tau was aggregated as previously described in the absence or presence of doxycycline, and aliquots were taken at different time points during incubation. Aliquots of 0.4 μl of fresh CR solution (10 mM) were added to 30 μl of 22 μM tau sample solutions and 170 μl of 40 mM Tris-HCl, 20 mM MgCl2 (pH 7.5) buffer, to reach final concentrations of 20 μM for CR and 3 μM for tau. Samples were vortex-mixed for 15 s and incubated at 23°C at 300 rpm for 30 min. The absorption of each sample was recorded over a range of 400–700 nm on a TECAN Infinite M200 microplate reader. CR absorbance was calculated according to the equation CRAb=(540Ab/25,295)-(475Ab/46,306) as previously described by Klunk et al. (1989).
Bis-ANS Fluorescent Assay
Tau was aggregated as described above in the absence or presence of doxycycline. Aliquots were taken at 0 and 24 h incubation, and bis (1-Anilinonaphthalene-8-Sulfonic Acid) also known as bis-ANS was added to a final concentration of 5 μM. Bis-ANS was excited at 395 nm and fluorescence emission was measured from 410 to 610 nm.
Insolubility Assay
Samples of fresh, monomeric tau (22 μM) were incubated with and without heparin (0.2 mg/ml), in the presence and in the absence of doxycycline (100 μM) at 37°C, 600 rpm 72 h. Upon incubation, samples were centrifuged 60 min 41,656 g; the supernatants were recovered and loaded into an Amicon Ultra (Ultracel 10K). Samples were centrifuged and washed 3 times with buffer 40 mM Tris-HCl, 20 mM MgCl2, pH 7.5. Afterwards, samples were treated with guanidine hydrochloride (GnCl) 4 M 24 h at room temperature. Upon guanidine hydrochloride treatment, protein concentration within each sample was measured by optical density at 280 nm. Percentages of recovery in tau, tau:hep and tau:hep:DOX were calculated using each initial total protein content (0 h) as reference.
Infrared Spectroscopy
Samples of tau (100 μM), tau:heparin (100 μM:0.8 mg/ml) and tau:heparin:doxycycline (100 μM:0.8 mg/ml:400 μM) in buffer-D2O 40 mM Tris-HCl, 20 mM MgCl2, pH 7.5, 0.05% DTT, were collected after 24 h incubation at 37°C under orbital agitation (600 rpm) and assembled between two CaF2 windows with a path length of 50 nm in a thermostated cell. The spectra were recorded in a Nicolet 5,700 spectrometer (Thermo Nicolet, Madison, WI) equipped with a DTGS detector as described by Arrondo et al. (1993). The sample chamber was permanently purged with dry air. The buffer spectra were subtracted from that of the solution at the same temperature in order to eliminate the D2O contribution in the Amide I' region to get a flat baseline between 1,900 and 1,700 cm−1. After solvent subtraction, quantitative information on protein structure was obtained through deconvolution and derivation of the Amide I' band into its constituents (Arrondo et al., 1993). To obtain the relative contribution of each component, an iterative process based on minimal square was performed by using curve fit under SpectraCalc software. The mathematical solution of the decomposition may not be unique, but if restrictions are imposed such as the maintenance of the initial band positions in an interval of 1 cm−1, the preservation of the bandwidth within the expected limits, or the agreement with theoretical boundaries or predictions, the result becomes, in practice, unique.
Transmission Electron Microscopy
4 μl of 22 μM tau aggregation samples were adsorbed onto glow-discharged 200 mesh Formvar carbon coated copper grids (Electron Microscopy Sciences) and stained with aqueous UranyLess (Electron Microscopy Sciences). After washing, excess liquid was removed, and grids were allowed to air dry. Transmission electron microscopy micrographs were collected using a Hitachi 7,700 transmission electron microscope.
Protease Resistance Assay
Tau, tau:heparin and tau.heparin:doxycycline samples obtained after 168 h of incubation were used as substrate of proteases in the digestion assay. Reactions were carried out mixing tau samples with proteinase K (1 μg/ml) or trypsin (0.0125 %), for 30 min at 37°C in buffer 40 mM Tris-HCl, 20 mM MgCl2 pH 7.5 with 0.05% DTT. After incubation, the enzymes were inactivated with PMSF 1 mM and subsequently loading buffer was added to each sample for 12% Tris-Glycine SDS-PAGE gel. The gel was stained with colloidal Coomasie Blue incubating with gentle stirring throughout the night. Photographs were taken and the gels were analyzed using Image J 1.47v software (National Institutes of Health, EUA), to obtain the densitometric profiles of the bands of each strip (Ferreira and Rasband, 2012).
Real Time Quaking Induced Conversion (RT-QuIC)
RT-QuIC reactions were performed in black 96-well plates (COSTAR, Corning Incorporated) in which 100 μl of the reaction mixtures were loaded. Mix were prepared to the following final concentrations: 150 mM NaCl, 1 mM EDTA, 10 μM Thioflavin T, 70 μM SDS, and 0.5 μM of the monomeric microtubule binding domain of 2N4R tau (K18 peptide) in PBS buffer (pH= 7.1). Doxycycline was added at the indicated final concentrations. Plates were covered with sealing tape and incubated at 41°C in a plate reader (Infinite M200 fluorescence plate reader, TECAN) with intermittent cycles of 1-min orbital shaking at 432 rpm followed by 2 min incubation and 1 min pause to measure the fluorescence intensity at 480 nm. Three replicates of each sample were measured for 250 amplification cycles. Monomer incorporation rate was determined from RT-QuiC data based on the following equation (Arosio et al., 2015; Ghosh et al., 2018):
where y0 was the signal base line at the lag phase; A was the total increase in fluorescence signal between the lag and stationary phase;k is the growth rate constant and t0.5 was its mid-point of the log phase.
Seeding Assay
Samples of tau:heparin (22 μM:0.2 mg/ml) in buffer 40 mM Tris-HCl, 20 mM MgCl2, pH 7.5, 0.05% DTT, were incubated for 24 h in a Thermomixer Comfort® (Eppendorf) at 37°C under orbital agitation at 600 rpm. These species were diluted 1/10 when harvested and further incubated with 22 μM of fresh monomers in the absence or presence of 100 μM doxycycline. As an internal control heparin at the residual concentration (hepr) of 0.08 mg/ml present in seed aliquots, was added to monomeric tau and the mix incubated in the same experimental conditions. Seeding aggregation was followed using ThT fluorescent probe. Aliquots were taken from the seeding aggregation after 0, 48, and 168 h and mixed with ThT, according to LeVine (LeVine, 1993, 1999), as previously described, using FluoroMax-4 Spectrofluorometer.
Human Neuroblastoma Cell Culture and Cytotoxicity Assay
SH-SY5Y cells were grown in DMEM supplemented with 10% fetal bovine serum (FBS) and 1% penicillin/streptomycin (PS), at 37°C and 5%CO2. For the cell viability assay cells were seeded in 96 wells plates at 15,000 cells/well and maintained in 100 μl of DMEM supplemented with 2% FBS and 1% PS for 24 h at 37°C. Afterwards, cells were treated with a 25 μl aliquot of pre-incubated (37°C, 6,000 rpm 16 h) tau, heparin, tau:heparin, tau:heparin:doxycyline and incubated for 24 h at 37°C 5% CO2. To determine cell viability, the colorimetric MTT metabolic activity assay was used as previously described by Mosmann (1983). All experiments were performed in sextuplicate, and the relative cell viability (%) was expressed as a percentage relative to the untreated cell control.
Statistical Analyses
All data were obtained from at least three independent experiments and expressed as mean ± SD. Multiple-group comparisons were performed with one-way ANOVA and t-test. Differences were considered as statistically significant at p < 0.05. Statistical analyses were carried out with GraphPad Prism 5 (San Diego, California, USA).
Results
Doxycycline Hinders Tau Amyloid Fibril Formation Yielding Novel Species
The ability of doxycycline to interfere with 2N4R tau aggregation was studied in the presence of heparin, a classical model system which induces the formation of tau fibrillary elements similar to NFT (Goedert et al., 1996; Pérez et al., 2002). In agreement with previous reports, we found that heparin efficiently triggered full-length tau amyloid aggregation as monitored by Thioflavin T (ThT) fluorescence emission. Doxycycline inhibited tau aggregation in a dose-dependent manner (Figure 1A), showing an IC50 of 29 μM (Supplementary Figure 1). Since doxycycline exerted optimal inhibition of tau amyloid aggregation at 100 μM, all experiments henceforth were performed at this concentration. In addition, to discard a potential interaction between doxycycline and heparin that could indirectly affect tau aggregation, doxycycline fluorescence emission spectra was studied in the presence of different heparin doses (Supplementary Figure 2). Our results showed none variation in doxycycline emission spectra, suggesting no direct interaction between doxycycline and heparin. Kinetics of heparin–induced tau aggregation showed a classical sigmoidal behavior (Figure 1B), characteristic of the nucleation-polymerization process as previously described (Friedhoff et al., 1998; Barghorn and Mandelkow, 2002; Chirita and Kuret, 2004). The short lag phase was followed by an exponential increase that finally reached a plateau after 24 h of incubation. In the presence of Doxycycline, the lag phase was undetectable under our experimental conditions with a fluorescence steady state significantly reduced regarding that obtained in the absence of the tetracycline. This steady state remained stable up to 168 h (Figure 1B). To validate these findings, we also monitored the extent of tau fibril formation in the absence or presence of doxycycline using Congo Red (CR) binding assay, which served as a complementary measure to detect the presence of amyloid fibril structures in tau samples (Klunk et al., 1989, 1999). In good agreement with ThT analysis, doxycycline inhibited the amyloid aggregation of tau under our experimental conditions (Figure 1C). Interestingly, we also observed that doxycycline had an anti-aggregative effect when added after 2 h of incubation, when early aggregates of tau are already formed (Figure 1C), No aggregation was detected for tau in the absence of heparin and in the presence of doxycycline (Figure 1C). Focus on the initial stages can be found in Supplementary Figure 3.
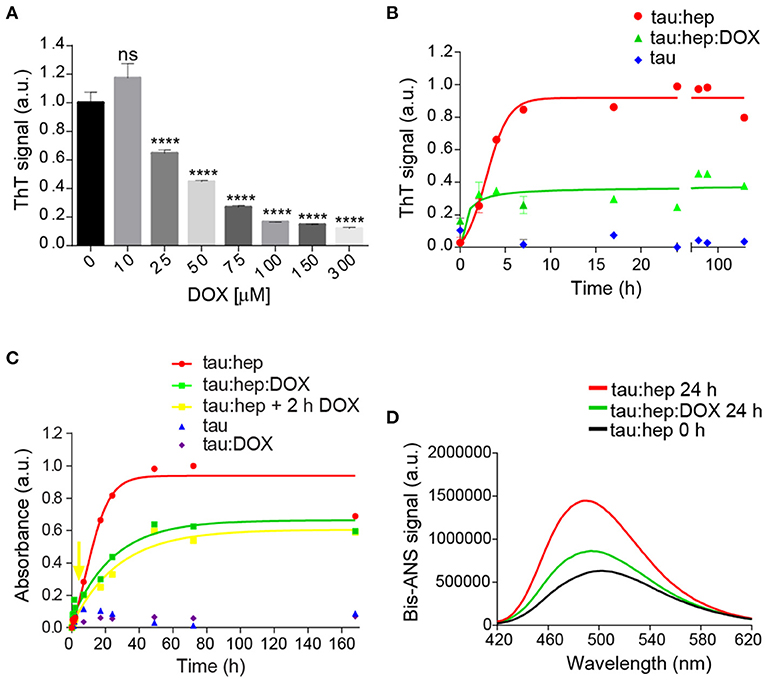
Figure 1. Doxycycline affects tau canonical amyloid aggregation. (A) Dose-response of doxycycline over tau amyloid aggregation monitored by fluorescence emission intensity of 25 μM of thioflavin T in a solution containing samples of 22 μM tau, 0.2 mg/ml heparin and 10, 25, 75, 100, 150, or 300 μM of doxycycline. Samples were incubated at 37°C under orbital agitation and aggregation was assayed after 24 h. n = 3 ns: not significant. ****p ≤ 0.0001. Error bars represent SD. (B) Fluorescence emission intensity of 25 μM thioflavin T in a solution containing samples of tau 22 μM; 0.2 mg/ml heparin; and 100 μM of doxycycline. Samples were incubated at 37°C under orbital agitation and aggregation was assayed by ThT fluorescence emission. (C) Absorbance of 20 μM Congo Red in a solution containing samples of tau 22 μM; 0.2 mg/ml heparin; and 100 μM of doxycycline added at time 0 h (green line and squares) and at 2 h (yellow line and squares). Samples were incubated at 37°C under orbital agitation, and absorbance recorded on a TECAN Infinite M200 microplate reader. (D) Bis-ANS fluorescence signal of tau:heparin solution incubated 0 h (black line) and 24 h at 37°C under orbital agitation in the absence (red line) or presence of doxycycline (green line).
The different kinetics observed in the presence or absence of doxycycline suggests that distinct tau species were formed. To evaluate this hypothesis, we compared the hydrophobic patches exposed to solvent of these species. For this, we studied the interaction of bis-ANS with species prepared with or without doxycycline (Figure 1D). Basal bis-ANS emission spectrum of tau increased when heparin was added to trigger amyloid aggregation, reflecting a gain of hydrophobic surfaces exposure during the process. On the contrary, in the presence of doxycycline, the fluorescence intensity diminished significantly (61%) (Figure 1D). These results indicate that these species were structurally different and the presence of doxycycline led to less hydrophobic residues exposed.
Doxycycline Affects Tau Seeding Ability and Toxicity
Considering that hydrophobicity is one of the primary driving forces behind protein self-assembly processes (Cheon et al., 2007; Marshall et al., 2011), and that brain-derived tau oligomeric species that can spread the pathology have affinity for bis-ANS (Lasagna-Reeves et al., 2012), we assessed the ability of doxycycline at halting the pro-aggregating properties of heparin-induced tau aggregates on monomeric species. For this, tau seeds were produced by incubating monomeric species with heparin at 37°C under orbital agitation for 24 h. Then, aliquots of this solution were added to fresh tau samples (deprived of heparin) with and without 100 μM of doxycycline. Only in the absence of the tetracycline, the seeding effect of pre-aggregated tau on the monomeric protein could be observed (Figure 2A). In the absence of seeds, monomeric tau did not evolve into amyloid species, as indicated by reduced ThT signal. As an internal control, heparin at the residual concentration present in seed aliquots, was added to monomeric tau and the mix incubated in the same experimental conditions. Upon incubation, no amyloid aggregation was observed according to ThT signal (Supplementary Figure 4).
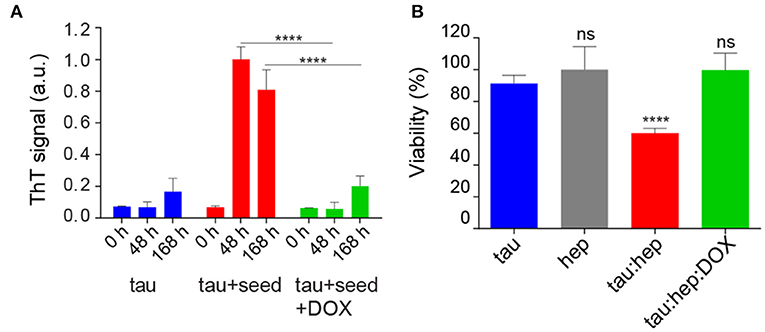
Figure 2. Doxycycline affects tau seeding ability and toxicity. (A) Effect of doxycycline on the seeding capability of pre-aggregated tau species. Pre-incubated tau species were obtained by incubating a tau:heparin solution (tau 22 μM; 0.2 mg/ml heparin) 24 h at 37°C under orbital agitation. 25 μl aliquots of this solution were used as seeds and added to fresh monomeric tau (22 μM) in the absence or presence of doxycycline 100 μM and incubated 0 h, 48 h and 168 h at 37°C under orbital agitation. (B) SH-SY5Y cells were treated with aggregated species of tau incubated in the presence of heparin, with or without doxycycline, and harvested after 16 h of incubation. Viability was measured using an MTT assay and expressed as a percentage relative to the untreated cells. Statistical analyses for both experiments were performed using the ANOVA test. n = 3 ns: not significant. ****p ≤ 0.0001. Error bars represent SD.
As our results showed that doxycycline remodeled tau aggregates affecting the formation of hydrophobic patches, we evaluated the ability of these species to disrupt mitochondrial integrity. For this, we analyzed mitochondrial activity in SH-SY5Y cells using the MTT assay (Mosmann, 1983), which also indirectly reflects the number of viable cells and cytotoxicity. SH-SY5Y cells were incubated with 25 μl aliquots of heparin-induced tau species prepared in the absence or presence of doxycycline and harvested after 16 h of incubation (Flach et al., 2012; Takashima, 2013). Cells were further incubated at 37°C for 24 h and their viability was assessed. Figure 2B shows that, in good agreement with previous reports, heparin-induced tau oligomers harvested after 16 h of incubation in the absence of doxycycline led to a decrease of about 35 % in cell viability. On the contrary, the viability of cells treated with tau species prepared in the presence of doxycycline, showed no significant difference from control on MTT turnover, indicating that the tetracycline counteracts the toxicity resulting from the heparin-induced aggregation process (Figure 2B). As an internal control, a solution of heparin was tested without significant differences from control (Figure 2B).
In summary, Figure 2 shows that the presence of doxycycline interfered with the seeding-ability of tau and rendered tau aggregates less toxic for cultured cells.
Doxycycline Diminished β-Structuration of Tau Aggregates
The impact of doxycycline on the structure of heparin-induced tau aggregates was analyzed by using Fourier-Transform infrared spectroscopy (FTIR). Comparative analysis of the conformationally sensitive band Amide I' (1,700–1,600 cm−1 of the infrared spectrum) of aggregates were obtained in the presence and absence of doxycycline. The tau Amide I' contour is centered at about 1,640 cm−1, which is typical for unfolded proteins (Byler and Susi, 1986; Krimm and Bandekar, 1986). Structural analysis performed by a curve fitting process (see Materials and Methods) allowed for band assignments according to previous reports (Frost et al., 2009), and showed close agreement with elements described by NMR (Frost et al., 2009). Unstructured regions represented more than 40% of Amide I'. β-sheets, loops and turns were also detected (Figure 3A; Table 1) and their relative contributions were in accordance with previous reports (Frost et al., 2009). The band centered at 1,619 cm−1, which has ambiguous assignments (Arrondo et al., 1988, 1994), was attributed to type II polyproline helixes (PPII) since tau has a proline-rich region, with a PPII conformation (Boggon and Eck, 2004; Lau et al., 2016).
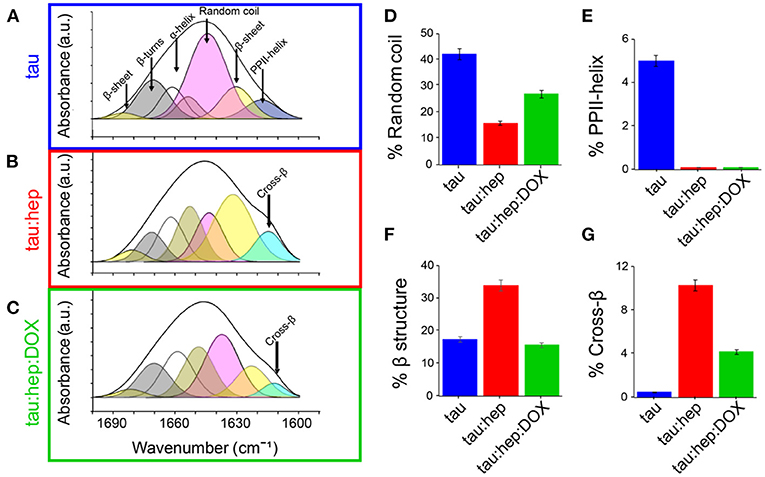
Figure 3. Doxycycline induces a different structural arrangement in tau aggregates. Tau FTIR Amide I' curve fitting of (A) 100 μM tau (B) 100 μM tau in the presence of heparin (0.8 mg/ml). (C) 100 μM tau in the presence of heparin (0.8 mg/ml) and 100 μM doxycycline. Relative contribution of each component: (D) Random coil, (E) PPHII-helix, (F) β structure, (G) Cross-β. Error bars represent SD.
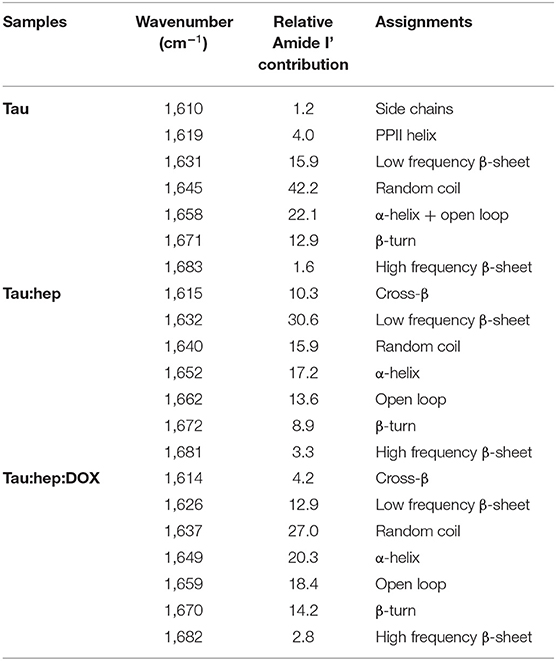
Table 1. FTIR-based evaluation of secondary structure content in different species of human tau incubated in the absence or presence of heparin and doxycycline for 24 h.
Tau Amide I' spectrum remains stable after 24 h of incubation, which demonstrates the structural stability of the protein under our experimental conditions. However, in the presence of heparin, significant changes were detected (Figure 3B). As expected according with ThT spectroscopy studies (Figure 1B), a band located at around 1,614 cm−1 assignable to cross-β structure (Zandomeneghi et al., 2009; Sarroukh et al., 2013) emerges, together with a significant increase in β-sheet structure. This β-structuration occurred at the expense of the random coil (Figures 3B,D; Table 1). The PPII-helix contribution was undetectable (Figure 3E) even using high derivative or deconvolution factors.
The presence of doxycycline significantly diminished the 1,614 cm−1 band contribution, suggesting that this tetracycline hindered the heparin-induced gain of cross-β structures associated with amyloid aggregation of tau (Figures 3C,G). Moreover, the overall β-structuration of the protein was decreased (curves shaded in yellow and light blue) (Figure 3F), while non-structured regions (curve shaded in pink) remained more conserved (Figure 3D).
Doxycycline Interferes With Heparin-Induced Tau Fibril Formation
To evaluate whether doxycycline could affect heparin-induced full length (2N4R) tau aggregation, we used transmission electron microscopy (TEM). Samples of monomeric and aggregated tau species incubated with or without doxycycline and harvested after 168 h were analyzed (Figure 4A). ThT fluorescence and infrared spectroscopy data showed that monomeric tau had no detectable assembly after 168 h incubation. In the presence of heparin, tau aggregated into long fibrils (Figure 4B). On the contrary, in the presence of doxycycline, long fibrils were not detected but instead, a mixture of oligomers and short fibrils abounded in all observed fields (Figure 4C).
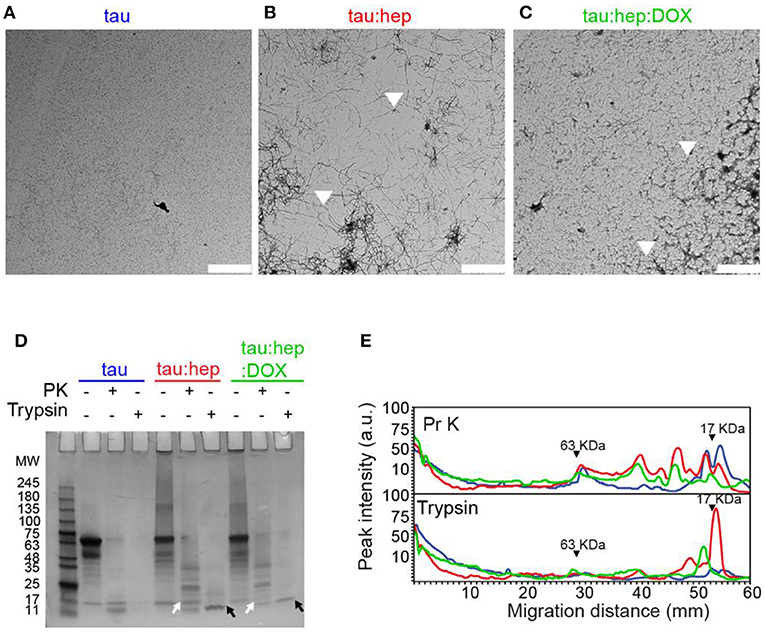
Figure 4. Doxycycline interferes with heparin-induced tau fibril formation. (A–C) Transmission electron microscopy (TEM) of different 2N4R tau samples incubated at 37°C under orbital agitation and harvested after 168 h of incubation. Scale bar corresponds to 2 μm. (D) Partial digestion profile of tau samples incubated in the same conditions as A, treated and not treated with 1 μg/ml proteinase K and with 0.0125% trypsin. Digestion products were resolved in a 12% tris-glycine gel stained with colloidal Coomasie Blue. Molecular weight marker in kDa. Comparison between digestion products of tau: hep and tau:hep:DOX aggregates obtained by PK (white arrows) and Trypsin (black arrows) proteolysis. SDS-PAGE gel image was carefully selected (from at least three experiments) to be representative. (E) Densitometric analysis of the SDS-PAGE gel B was performed by using Image J 1.47v software.
Protease resistance profiles of full-length tau prepared in the absence or presence of doxycycline is depicted in Figures 4D,E. Samples of monomeric full-length tau, as well as heparin-induced tau fibrils prepared in the absence or presence of doxycycline, were harvested after 168 h of incubation and treated with proteinase K (PK) or trypsin. Digestion products were resolved in a 12% Tris-Glycine SDS- PAGE gel (Figure 4D), and the corresponding densitometric analysis is shown in Figure 4E. Our data revealed that monomeric tau was digested into peptides of <11 kDa when treated with PK and trypsin. The trypsin limited digestion of tau fibrillar species resulted in a resistant core that comprises approximately the second half of R1, R2, R3 and the first half of R4 (Wang et al., 2010). The heparin-induced tau fibrils exhibited fragments resistant to both proteases and were retained in the stacking gel as previously reported (Wegmann et al., 2012). However, excised fragments from this fibril core were observed in the gel. In presence of PK we observed several fragments between 35 and 11 KDa, while for trypsin treatment we observed a prominent band between 11 and 17 KDa. In presence of doxycycline, the SDS-PAGE and the densitogram showed a significant change in the digestion profile for both proteases compared to samples formed in presence of solely heparin. These digestion products were enriched with low molecular weight elements. This data suggests that doxycycline might interact with the microtubule-binding region, inducing novel conformational arrangements and exposing previously-inaccessible sites to protease cleavage.
In addition, we also studied the effect of doxycycline on the formation of heparin-induced insoluble tau species through an insolubility assay. In this regards, the amount of soluble species present in samples containing tau, tau:hep and tau:hep:Dox were measured after 72 h of incubation at 37°C, 600 rpm. As shown in Figure 5 the incubation of tau in the presence of heparin led to a decreased amount of soluble species of the protein when compared to control without the glycosaminoglycan. Conversely, the presence of doxycycline favored the permanence of tau in the supernatant fraction as soluble species in the tau:hep:Dox sample (Figure 5; Supplementary Table 1).
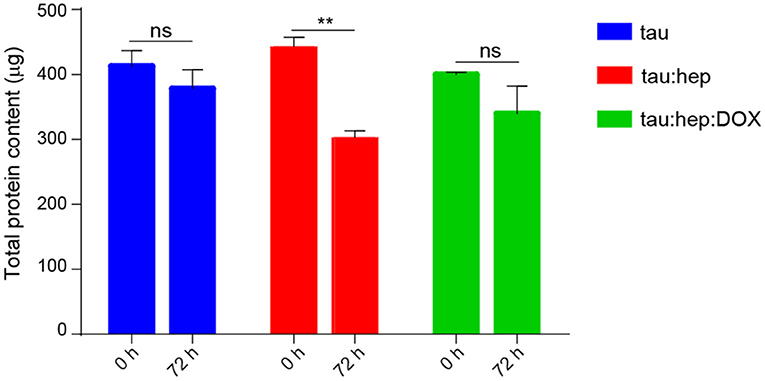
Figure 5. Effect of doxycycline on the formation of heparin-induced insoluble tau species. Samples of fresh, monomeric tau (22 μM) were incubated with and without heparin (0.2 mg/ml), in the presence and in the absence of doxycycline (100 μM) for 72 h. Upon incubation, samples were centrifuged and the supernatants were recovered to be treated with guanidine hydrochloride (GnCl). Protein concentration of each sample was measured by DO at 280 nm. n = 3 ns: not significant. **p ≤ 0.05. Error bars represent SEM.
Doxycycline Interacts With Tau Microtubule Binding Domains Inhibiting Its Self-Aggregation
To confirm the hypothesis that doxycycline interacts with the microtubule-binding region of tau, we evaluated the effect of this tetracycline on the aggregation of a 4R truncated tau (K18 peptide), which can reach the fibrillary state without an inducer (Von Bergen et al., 2006). In order to evaluate the effect of different doxycycline concentrations on K18 peptide aggregation we used the real time quaking induced complementation (RT-QuIC) assay (Wilham et al., 2010). The results obtained showed that ThT fluorescence intensity values decreased at higher concentrations of doxycycline (Figure 6A), abolishing the fluorescent signal at 70 μM. Furthermore, we observed a significantly lower K18 monomer incorporation rate per cycle in samples treated with 1, 10, and 70 μM doxycycline (Figure 6B). Our results showed that doxycycline inhibited in a dose dependent manner K18 peptide amyloid aggregation, by blocking monomer incorporation to the growing fibrils. We suggest that doxycycline binds to the microtubule-binding region, considered the core of tau fibrils (Wischik et al., 1988), disrupting the intermolecular interactions of tau at the level of these key repetitions in the process of amyloid aggregation.
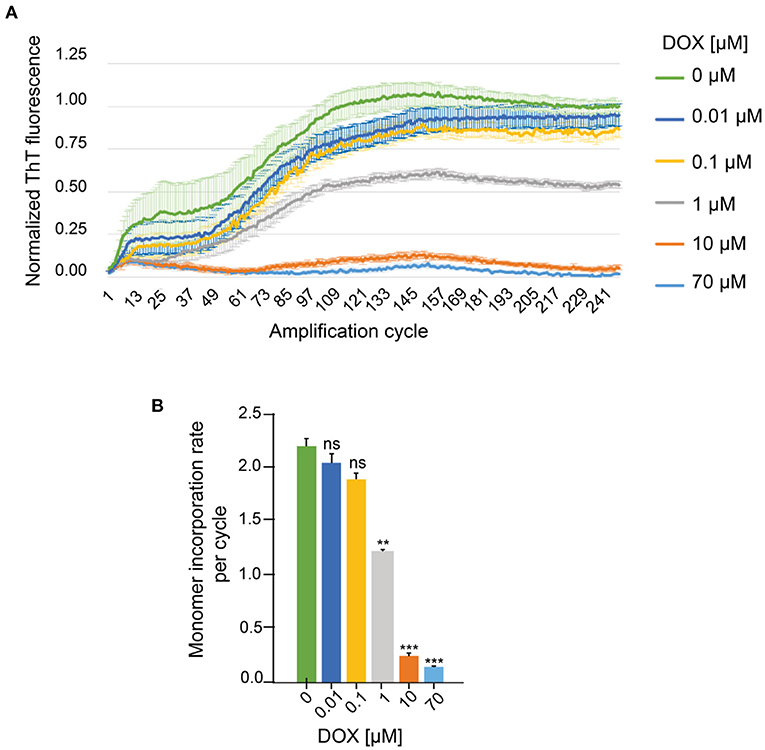
Figure 6. Effect of different doxycycline concentrations on in vitro amplification of K18 peptides by RT-QuIC. (A) ThT fluorescence of 0.5 μM monomeric K18 incubated in the absence or presence of doxycycline. Three replicates of each sample were measured for 250 amplification cycles. (B) Monomer incorporation rate per RT-QuIC cycle. n = 5 ns: not significant. **p < 0.005. ***p < 0.0005. Error bars represent SD.
Discussion
Recent results from our group and others (Smith and Leyden, 2005; González-Lizárraga et al., 2017; Reglodi et al., 2017; Bortolanza et al., 2018; Napp et al., 2018) have shed light regarding the ability of tetracyclines in interfering with the toxicity and seeding of aggregate-prone proteins. In the present work, we extend these studies to tau. Our results demonstrate that doxycycline can disrupt tau aggregation in both heparin-induced and K18 self-assembling heparin-free systems (Figures 1, 6). It is important to note that tau amyloid formation in the presence of doxycycline produces, in addition to oligomers, short fibrillary species (Figure 4C). This effect is distinct from what has been previously reported for doxycycline on α-synuclein aggregation, where only oligomers are observed (González-Lizárraga et al., 2017).
The surface hydrophobicity of tau aggregates has been associated with both cellular toxicity and their ability to serve as nucleation seeds for monomers (Penke et al., 2020). Interaction of hydrophobic aggregated species with cell membranes, which induces permeability and ultimately dysfunction, is thought to be the mechanism responsible for toxicity (Cheon et al., 2007; Bolognesi et al., 2010; Campioni et al., 2010). Even inter-neuronal propagation of aggregated tau, often referred to as “spreading,” is governed by the hydrophobicity of pro-aggregant species (Penke et al., 2020). Our results show that tau aggregates grown in the presence of doxycycline present less hydrophobic surfaces (Figure 2A), which could explain why tau species produced in the presence of this tetracycline are incapable of seeding (Figure 2A) and ultimately trigger decreased toxicity in cell cultures (Figure 2B).
Structural studies revealed that tau species produced in the presence of doxycycline have less β-structuration (Figures 3C,F,G). This difference could ultimately affect the clearance of these species, since aggregates enriched in β-structures can directly or indirectly damage cellular proteostatic mechanisms, such as ubiquitin-proteasome (UPS), chaperone-mediated autophagy (CMA), and macroautophagy (Bence et al., 2001; Keck et al., 2003; Hetz and Mollereau, 2014; Yu et al., 2014; Ciechanover and Kwon, 2015). Therefore, the formation of tau species of smaller size (Figure 4C) and lower β-structure content (Figures 3C,F,G) suggests that doxycycline could also indirectly preserve the function of these proteostatic control systems.
The pattern of proteolytic digestion of species obtained in the absence or presence of doxycycline also revealed differences in the packing of the resultant fibrils (long fibrils and short filaments). Densitometric profiles revealed that digestion products of species formed in the presence of doxycycline were enriched in low molecular weight elements (Figures 4D,E). Trypsin limited digestion of PHFs in vitro results in a resistant fragment comprising the amyloid core, including approximately the second half of R1, R2, R3 and the first half of R4 (Wang et al., 2010). Therefore, the absence of this 17 KDa trypsin resistant fragment in the mixture containing doxycycline suggests that the tetracycline might interact with the tau microtubule binding region which forms the amyloid core. The fact that doxycycline also inhibits K18 self-aggregation supports the hypothesis that the 4R pro-aggregant domain of tau is involved in doxycycline interaction. Moreover, these mechanisms observed in vitro could have impact in neuroprotection, since the presence of smaller aggregated species with higher susceptibility to proteases would also favor their “clearance” by the intracellular degradation systems. Likewise, taking into account that amyloid fibrils trigger microglia activation and chronic inflammatory response, which in turn lead to neurodegeneration (Glass et al., 2010; Gustot et al., 2015; Laurent et al., 2018), the doxycycline-increased susceptibility to proteases could be beneficial for neuron survival.
From a clinical perspective, doxycycline has been used for decades in human health and has proven to be a safe and well-tolerated drug (Sloan and Scheinfeld, 2008; Holmes and Charles, 2009). Moreover, due to its anti-inflammatory actions, doxycycline therapy is not restricted to the treatment of microbial infections, but has also been demonstrated to be useful for the management of periodontal and skin inflammatory pathologies (Golub et al., 1991; Humbert et al., 1991). When used as an antimicrobial compound, doxycycline (200 mg twice a day) achieves a concentration of about 3 μM in the cerebral spinal fluid (CSF) (Yim et al., 1985). Therefore, considering its brain penetration, the tau/doxycycline ratio from our experiments, and the concentration of tau in CSF (~244 pg/ml) (Sunderland et al., 2003), sub-antibiotic doses would be high enough to exert neuroprotection.
Interestingly, Umeda et al. (2016) found, in a mouse model of AD, that older mice required higher concentrations of the antibiotic compound rifampicin to achieve a degree of protection comparable to that observed in younger mice. Therefore, antibiotic therapy could be especially valuable at an early stage of the disease. Likewise, considering the feasibility of doxycycline therapy for chronic treatments, as those required for these neurodegeneration, it is important to note that since sub-antibiotic doses would be high enough to reach neuroprotective concentrations in the brain, a selective pressure over native microbiota should not be imposed. Likewise, several reports found no evidence of microbiota disturbance during long-term doxycycline therapy even after 2 years of treatment with sub-antibiotic doses (20 mg/day) (Walker et al., 2005; Gu et al., 2012). Interestingly, two sub-antibiotic doxycycline-formulations (Periostat and Oracea) have already been approved by de US FDA for long-term treatment of periodontal pathologies and the chronic inflammatory skin disease rosacea (Gu et al., 2012).
Regarding the benefits of doxycycline in neurology, daily doses of doxycycline and rifampicin during 3 month were found to significantly ameliorate dysfunctional behavior and cognitive decline in a clinical trial with more than one hundred patients with probable AD and mild dementia (Loeb et al., 2004). However, a later trial suggested that neither rifampicin nor doxycycline were capable of hindering the progression of neurodegeneration in diagnosed AD patients (Molloy et al., 2013). Since neurodegeneration involves the death of neurons leading to irreversible brain injury and, considering that amyloid aggregation is one of the first events of the deleterious cascade that takes place in tauopathies, it is plausible to think that drugs such as doxycycline that inhibit tau aggregation would be more suitable candidates for preventive rather than for palliative therapy. Therefore, the controversial results from clinical trials may be explained considering the administration time of the drug since once the appropriate starting points for treatment have passed its therapeutic action could decrease.
In summary, our results extend the spectrum of the anti-aggregation action of doxycycline over tau, a key player during the development and progression of tauopathies such as AD. Altogether, the anti-aggregant ability on tau reported herein, in addition to its long safety record, as well as its brain penetration and low concentration required, prompt us to propose doxycycline as a valuable candidate for the development of a therapy against Alzheimer's disease and related tauopathies.
Data Availability Statement
The raw data supporting the conclusions of this article will be made available by the authors, without undue reservation.
Author Contributions
RC, RR-V, and SSo conceived and supervised the study. LM designed and performed protein purification and aggregation assays. LM, FG-L, and SSe designed and performed fluorescence techniques. LM and SSo designed and performed seeding experiments. LM and SSo designed and performed cellular culture assays. LM and RC performed FTIR experiments. LM designed and performed TEM and digestion experiments. AD-M, MC-O, MZ, and TF designed and performed RT-QuIC studies. ED provided new tools and reagents. LM and SSo devised the overall format of manuscript and together with RC and FG-L wrote the manuscript. VP, DP, PPM, and TF contributed to the experimental design and revised the manuscript. All authors contributed to the discussion and were involved in editing the final manuscript.
Funding
This work benefited from equipment and services from ICMQuant (electron microscopy) and Celis (cell culture) core facilities, at the Paris Brain Institute (ICM), two platforms supported by Investissements d'Avenir (ANR-10-IAIHU-06) and the Translational Research Infrastructure for Biotherapies in Neurosciences (ANR-11-INBS-0011-NeurATRIS). We also thank Mr. Claude Burgio and SkyBio for invaluable support. This work was supported by grants from PIP-CONICET 0183, PICT3379, PICT2018-02989 PIP-CONICET 11220130100619CO, PICT-MINCyT 2012–2882, PIUNT-UNT D542/1. LM, FG-L and SSe were supported by fellowships from the Argentinean Government (Becas Internas Doctorales y Posdoctoral from Consejo Nacional de Investigaciones Científicas y Técnicas). AD-M is supported by a postdoctoral fellowship from the Galician Government (Programa de axuda á etapa posdoutoral, XUGA, GAIN, ED481B 2017/053). TF is supported by the Deutsche Forschungsgemeinschaft (DFG, German Research Foundation) under Germany's Excellence Strategy - EXC 2067/1- 390729940. VP is the recipient of a Thesis scholarship from Association France Parkinson.
Conflict of Interest
The authors declare that the research was conducted in the absence of any commercial or financial relationships that could be construed as a potential conflict of interest.
Supplementary Material
The Supplementary Material for this article can be found online at: https://www.frontiersin.org/articles/10.3389/fnagi.2021.635760/full#supplementary-material
Abbreviations
MAP, microtubule associated protein; IDP, intrinsically disordered protein; PHF, paired helical filaments; NFT, neurofibrillary tangle; AD, Alzheimer's disease; DOX, doxycycline; PPII, type II polyproline helix.
References
Akushevich, I., Kravchenko, J., Ukraintseva, S., Arbeev, K., and Yashin, A. I. (2013). Time trends of incidence of age-associated diseases in the US elderly population: medicare-based analysis. Age Ageing 42, 494–500. doi: 10.1093/ageing/aft032
Arosio, P., Knowles, T. P. J., and Linse, S. (2015). On the lag phase in amyloid fibril formation. Phys. Chem. Chem. Phys. 17, 7606–7618. doi: 10.1039/C4CP05563B
Arrondo, J. L. R., Goñi, F. M., Castresana, J., and Valpuesta, J. M. (1994). Structure and thermal denaturation of crystalline and noncrystalline cytochrome oxidase as studied by infrared spectroscopy. Biochemistry 33, 11650–11655. doi: 10.1021/bi00204a029
Arrondo, J. L. R., Muga, A., Castresana, J., and Goñi, F. M. (1993). Quantitative studies of the structure of proteins in solution by fourier-transform infrared spectroscopy. Prog. Biophys. Mol. Biol. 59, 23–56. doi: 10.1016/0079-6107(93)90006-6
Arrondo, J. L. R., Young, N. M., and Mantsch, H. H. (1988). The solution structure of concanavalin A probed by FT-IR spectroscopy. Biochim. Biophys. Acta (BBA)/Protein Struct. Mol. 952, 261–268. doi: 10.1016/0167-4838(88)90125-2
Ashburn, T. T., and Thor, K. B. (2004). Drug repositioning: identifying and developing new uses for existing drugs. Nat. Rev. Drug Discov. 3, 673–683. doi: 10.1038/nrd1468
Balducci, C., and Forloni, G. (2019). Doxycycline for Alzheimer's disease: fighting β-amyloid oligomers and neuroinflammation. Front. Pharmacol. 10:738. doi: 10.3389/fphar.2019.00738
Barghorn, S., and Mandelkow, E. (2002). Toward a unified scheme for the aggregation of tau into Alzheimer paired helical filaments. Biochemistry 41, 14885–14896. doi: 10.1021/bi026469j
Barghorn, S., Biernat, J., and Mandelkow, E. (2005). Purification of recombinant tau protein and preparation of Alzheimer-paired helical filaments in vitro. Methods Mol. Biol. 299, 35–51. doi: 10.1385/1-59259-874-9:035
Bence, N. F., Sampat, R. M., and Kopito, R. R. (2001). Impairment of the ubiquitin-proteasome system by protein aggregation. Production 292, 1552–1555. doi: 10.1126/science.292.5521.1552
Boggon, T. J., and Eck, M. J. (2004). Structure and regulation of Src family kinases. Oncogene 23, 7918–7927. doi: 10.1038/sj.onc.1208081
Bolognesi, B., Kumita, J. R., Barros, T. P., Esbjorner, E. K., Luheshi, L. M., Crowther, D. C., et al. (2010). ANS binding reveals common features of cytotoxic amyloid species. ACS Chem. Biol. 5, 735–740. doi: 10.1021/cb1001203
Bortolanza, M., Nascimento, G. C., Socias, S. B., Ploper, D., Chehín, R. N., Raisman-Vozari, R., et al. (2018). Tetracycline repurposing in neurodegeneration: focus on Parkinson's disease. J. Neural Transm. 125, 1403–1415. doi: 10.1007/s00702-018-1913-1
Braak, H., and Braak, E. (1991). Neuropathological stageing of Alzheimer-related changes. Acta Neuropathol. 82, 239–259. doi: 10.1007/BF00308809
Byler, D. M., and Susi, H. (1986). Examination of the secondary structure of proteins by deconvolved FTIR spectra. Biopolymers 25, 469–487. doi: 10.1002/bip.360250307
Campioni, S., Mannini, B., Zampagni, M., Pensalfini, A., Parrini, C., Evangelisti, E., et al. (2010). A causative link between the structure of aberrant protein oligomers and their toxicity. Nat. Chem. Biol. 6, 140–147. doi: 10.1038/nchembio.283
Cheon, M., Chang, I., Mohanty, S., Luheshi, L. M., Dobson, C. M., Vendruscolo, M., et al. (2007). Structural reorganisation and potential toxicity of oligomeric species formed during the assembly of amyloid fibrils. PLoS Comput. Biol. 3, 1727–1738. doi: 10.1371/journal.pcbi.0030173
Chirita, C. N., and Kuret, J. (2004). Evidence for an intermediate in tau filament formation. Biochemistry 43, 1704–1714. doi: 10.1021/bi036034b
Ciechanover, A., and Kwon, Y. T. a. (2015). Degradation of misfolded proteins in neurodegenerative diseases: therapeutic targets and strategies. Exp. Mol. Med. 47:e147. doi: 10.1038/emm.2014.117
Dehmelt, L., and Halpain, S. (2004). The MAP2/Tau family of microtubule-associated proteins. Genome Biol. 6, 1–10. doi: 10.1186/gb-2004-6-1-204
D'Souza, A., Flynn, K., Chhabra, S., Dhakal, B., Hamadani, M., Jacobsen, K., et al. (2017). Rationale and design of DUAL study: doxycycline to Upgrade response in light chain (AL) amyloidosis (DUAL): a phase 2 pilot study of a two-pronged approach of prolonged doxycycline with plasma cell-directed therapy in the treatment of AL amyloidosis. Contemp. Clin. Trials Commun. 8, 33–38. doi: 10.1016/j.conctc.2017.08.012
Ferreira, T., and Rasband, W. (2012). ImageJ User Guide User Guide ImageJ. Image J user Guid. 1.46r.
Flach, K., Hilbrich, I., Schiffmann, A., Gärtner, U., Krüger, M., Leonhardt, M., et al. (2012). Tau oligomers impair artificial membrane integrity and cellular viability. J. Biol. Chem. 287, 43223–43233. doi: 10.1074/jbc.M112.396176
Friedhoff, P., Von Bergen, M., Mandelkow, E. M., Davies, P., and Mandelkow, E. (1998). A nucleated assembly mechanism of Alzheimer paired helical filaments. Proc. Natl. Acad. Sci. U.S.A. 95, 15712–15717. doi: 10.1073/pnas.95.26.15712
Frost, B., Ollesch, J., Wille, H., and Diamond, M. I. (2009). Conformational diversity of wild-type tau fibrils specified by templated conformation change. J. Biol. Chem. 284, 3546–3551. doi: 10.1074/jbc.M805627200
Gautieri, A., Beeg, M., Gobbi, M., Rigoldi, F., Colombo, L., and Salmona, M. (2019). The anti-amyloidogenic action of doxycycline: a molecular dynamics study on the interaction with Aβ42. Int. J. Mol. Sci. 20. doi: 10.3390/ijms20184641
Ghosh, S., Kundu, A., and Chattopadhyay, K. (2018). Small molecules attenuate the interplay between conformational fluctuations, early oligomerization and amyloidosis of alpha synuclein. Sci. Rep. 8, 1–16. doi: 10.1038/s41598-018-23718-3
Glass, C. K., Saijo, K., Winner, B., Marchetto, M. C., and Gage, F. H. (2010). Mechanisms underlying inflammation in neurodegeneration. Cell 140, 918–934. doi: 10.1016/j.cell.2010.02.016
Goedert, M., Jakes, R., Spillantini, M. G., Hasegawa, M., Smith, M., and Crowther, R. A. (1996). Assembly of microtubule-associated protein tau into Alzheimer-like filaments induced by sulphated glycosaminoglycans. Nature 383, 550–553. doi: 10.1038/383550a0
Golub, L. M., Ramamurthy, N. S., McNamara, T. F., Greenwald, R. A., and Rifkin, B. R. (1991). Tetracyclines inhibit connective tissue breakdown: new therapeutic implications for an old family of drugs. Crit. Rev. Oral Biol. Med. 2, 297–321. doi: 10.1177/10454411910020030201
González-Lizárraga, F., Socías, S. B., Ávila, C. L., Torres-Bugeau, C. M., Barbosa, L. R. S., Binolfi, A., et al. (2017). Repurposing doxycycline for synucleinopathies: remodelling of α-synuclein oligomers towards non-toxic parallel beta-sheet structured species. Sci. Rep. 7:41755. doi: 10.1038/srep41755
Grundke-Iqbal, I., Iqbal, K., and Tung, Y. C. (1986). Abnormal phosphorylation of the microtubule-associated protein τ (tau) in Alzheimer cytoskeletal pathology. Proc. Natl. Acad. Sci. U.S.A. 83, 44913–44917. doi: 10.1073/pnas.83.13.4913
Gu, Y., Walker, C., Ryan, M. E., Payne, J. B., and Golub, L. M. (2012). Non-antibacterial tetracycline formulations: clinical applications in dentistry and medicine. J. Oral Microbiol. 4, 1–14. doi: 10.3402/jom.v4i0.19227
Gustot, A., Gallea, J. I., Sarroukh, R., Celej, M. S., Ruysschaert, J. M., and Raussens, V. (2015). Amyloid fibrils are the molecular trigger of inflammation in Parkinson's disease. Biochem. J. 471, 323–333. doi: 10.1042/BJ20150617
Hedgepeth, C. M., Conrad, L. J., Zhang, J., Huang, H. C., Lee, V. M. Y., and Klein, P. S. (1997). Activation of the Wnt signaling pathway: a molecular mechanism for lithium action. Dev. Biol. 185, 82–91. doi: 10.1006/dbio.1997.8552
Hetz, C., and Mollereau, B. (2014). Disturbance of endoplasmic reticulum proteostasis in neurodegenerative diseases. Nat. Rev. Neurosci. 15, 233–249. doi: 10.1038/nrn3689
Hirokawa, N., Shiomura, Y., and Okabe, S. (1988). Tau proteins : the molecular structure and mode of binding on microtubules. J. Cell Biol. 107, 1449–1459. doi: 10.1083/jcb.107.4.1449
Holmes, N. E., and Charles, P. G. P. (2009). Safety and efficacy review of doxycycline. Clin. Med. Ther. 1, 471–482. doi: 10.4137/CMT.S2035
Humbert, P., Treffel, P., Chapuis, J. F., Buchet, S., Derancourt, C., and Agache, P. (1991). The tetracyclines in dermatology. J. Am. Acad. Dermatol. 25, 691–697. doi: 10.1016/0190-9622(91)70255-Z
Iqbal, K., Liu, F., Gong, C.-X., and Grundke-Iqbal, I. (2010). Tau in Alzheimer disease and related tauopathies. Curr. Alzheimer Res. 7, 656–664. doi: 10.2174/156720510793611592
Keck, S., Nitsch, R., Grune, T., and Ullrich, O. (2003). Proteasome inhibition by paired helical filament-tau in brains of patients with Alzheimer's disease. J. Neurochem. 85, 115–122. doi: 10.1046/j.1471-4159.2003.01642.x
Kidd, M. (1963). Paired helical filaments in electron microscopy in Alzheimer's disease. Nature 197, 192–193. doi: 10.1038/197192b0
Klunk, W. E., Jacob, R. F., and Mason, R. P. (1999). Quantifying amyloid by congo red spectral shift assay. Methods Enzymol. 309, 285–305. doi: 10.1016/S0076-6879(99)09021-7
Klunk, W. E., Pettegrew, J. W., and Abraham, D. J. (1989). Quantitative evaluation of Congo red binding to amyloid-like proteins with a beta-pleated sheet conformation. J. Histochem. Cytochem. 37, 1273–1281. doi: 10.1177/37.8.2666510
Krimm, S., and Bandekar, J. (1986). Vibrational spectroscopy and conformation of peptides, polypeptides, and proteins. J. Am. Chem. Soc. 77, 181–364. doi: 10.1016/S0065-3233(08)60528-8
Lasagna-Reeves, C., Castillo-Carranza, D., Sengupta, U., Guerrero-Munoz, M. J., Kiritoshi, T., Neugebauer, V., et al. (2012). Alzheimer brain-derived tau oligomers propagate pathology from endogenous tau. Sci. Rep. 2:700. doi: 10.1038/srep00700
Lau, D. H. W., Hogseth, M., Phillips, E. C., O'Neill, M. J., Pooler, A. M., Noble, W., et al. (2016). Critical residues involved in tau binding to fyn: implications for tau phosphorylation in Alzheimer's disease. Acta Neuropathol. Commun. 4, 1–13. doi: 10.1186/s40478-016-0317-4
Laurent, C., Buée, L., and Blum, D. (2018). Tau and neuroinflammation: what impact for Alzheimer's Disease and tauopathies? Biomed. J. 41, 21–33. doi: 10.1016/j.bj.2018.01.003
Lee, V. M., Goedert, M., and Trojanowski, J. Q. (2001). Neurodegenerative tauopathies. Annu. Rev. Neurosci. 24, 1121–1159. doi: 10.1146/annurev.neuro.24.1.1121
LeVine, H. I. (1993). Thioflavine T interaction with synthetic Alzheimer's disease β-amyloid peptides: detection of amyloid aggregation in solution. Protein Sci. 2, 404–410. doi: 10.1002/pro.5560020312
LeVine, H. I. (1999). Quantification of beta-Sheet amyloid fibril structures with thioflavin T. Methods Enzymol. 309, 274–284. doi: 10.1016/S0076-6879(99)09020-5
Loeb, M. B., Molloy, D. W., Smieja, M., Standish, T., Goldsmith, C. H., Mahony, J., et al. (2004). A randomized, controlled trial of doxycycline and rifampin for patients with Alzheimer's disease. J. Am. Geriatr. Soc. 52, 381–387. doi: 10.1111/j.1532-5415.2004.52109.x
Marshall, K. E., Morris, K. L., Charlton, D., O'Reilly, N., Lewis, L., Walden, H., et al. (2011). Hydrophobic, aromatic, and electrostatic interactions play a central role in amyloid fibril formation and stability. Biochemistry 50, 2061–2071. doi: 10.1021/bi101936c
Mocanu, M. M., Nissen, A., Eckermann, K., Khlistunova, I., Biernat, J., Drexler, D., et al. (2008). The potential for β-structure in the repeat domain of tau protein determines aggregation, synaptic decay, neuronal loss, and coassembly with endogenous Tau in inducible mouse models of tauopathy. J. Neurosci. 28, 737–748. doi: 10.1523/JNEUROSCI.2824-07.2008
Molloy, D. W., Standish, T. I., Zhou, Q., and Guyatt, G. (2013). A multicenter, blinded, randomized, factorial controlled trial of doxycycline and rifampin for treatment of Alzheimer's disease: the DARAD trial. Int. J. Geriatr. Psychiatry 28, 463–470. doi: 10.1002/gps.3846
Mosmann, T. (1983). Rapid colorimetric assay for cellular growth and survival: application to proliferation and cytotoxicity assays. J. Immunol. Methods 65, 55–63. doi: 10.1016/0022-1759(83)90303-4
Napp, A., Houbart, V., Demelenne, A., Merville, M. P., Crommen, J., Dumoulin, M., et al. (2018). Separation and determination of alpha-synuclein monomeric and oligomeric species using two electrophoretic approaches. Electrophoresis 39, 3022–3031. doi: 10.1002/elps.201800224
Panza, F., Imbimbo, B. P., Lozupone, M., Greco, A., Seripa, D., Logroscino, G., et al. (2019). Disease-modifying therapies for tauopathies: agents in the pipeline. Expert Rev. Neurother. 19, 397–408. doi: 10.1080/14737175.2019.1606715
Penke, B., Szucs, M., and Bogár, F. (2020). Oligomerization and conformational change turn monomeric β-amyloid and tau proteins toxic: their role in Alzheimer's pathogenesis. Molecules 25, 1–29. doi: 10.3390/molecules25071659
Pérez, M., Valpuesta, J. M., Medina, M., Montejo de Garcini, E., and Avila, J. (2002). Polymerization of τ into filaments in the presence of heparin: the minimal sequence required for τ - τ interaction. J. Neurochem. 67, 1183–1190. doi: 10.1046/j.1471-4159.1996.67031183.x
Reglodi, D., Renaud, J., Tamas, A., Tizabi, Y., Socías, S. B., Del-Bel, E., et al. (2017). Novel tactics for neuroprotection in Parkinson's disease: role of antibiotics, polyphenols and neuropeptides. Prog. Neurobiol. 155, 120–148. doi: 10.1016/j.pneurobio.2015.10.004
Sarroukh, R., Goormaghtigh, E., Ruysschaert, J. M., and Raussens, V. (2013). ATR-FTIR: A “rejuvenated” tool to investigate amyloid proteins. Biochim. Biophys. Acta Biomembr. 1828, 2328–2338. doi: 10.1016/j.bbamem.2013.04.012
Shammas, S. L., Garcia, G. A., Kumar, S., Kjaergaard, M., Horrocks, M. H., Shivji, N., et al. (2015). A mechanistic model of tau amyloid aggregation based on direct observation of oligomers. Nat. Commun. 6, 1–10. doi: 10.1038/ncomms8025
Sloan, B., and Scheinfeld, N. (2008). The use and safety of doxycycline hyclate and other second-generation tetracyclines. Expert Opin. Drug Saf. 7, 571–577. doi: 10.1517/14740338.7.5.571
Smith, K., and Leyden, J. J. (2005). Safety of doxycycline and minocycline: a systematic review. Clin. Ther. 27, 1329–1342. doi: 10.1016/j.clinthera.2005.09.005
Socias, S. B., González-Lizárraga, F., Avila, C. L., Vera, C., Acuña, L., Sepulveda-Diaz, J. E., et al. (2018). Exploiting the therapeutic potential of ready-to-use drugs: repurposing antibiotics against amyloid aggregation in neurodegenerative diseases. Prog. Neurobiol. 162, 17–36. doi: 10.1016/j.pneurobio.2017.12.002
Steiner, B., Mandelkow, E. M., Biernat, J., Gustke, N., Meyer, H. E., Schmidt, B., et al. (1990). Phosphorylation of microtubule-associated protein tau: identification of the site for Ca2(+)-calmodulin dependent kinase and relationship with tau phosphorylation in Alzheimer tangles. EMBO J. 9, 3539–3544. doi: 10.1002/j.1460-2075.1990.tb07563.x
Sunderland, T., Linker, G., Mirza, N., Putnam, K. T., Friedman, D. L., Kimmel, L. H., et al. (2003). Decreased β-Amyloid 1-42 and Increased Tau Levels in Cerebrospinal Fluid of Patients With Alzheimer Disease. JAMA J. Am. Med. Assoc. 289, 2094–2103. doi: 10.1001/jama.289.16.2094
Takashima, A. (2013). Tauopathies and tau oligomers. J. Alzheimer's Dis. 37, 565–568. doi: 10.3233/JAD-130653
Umeda, T., Ono, K., Sakai, A., Yamashita, M., Mizuguchi, M., Klein, W. L., et al. (2016). Rifampicin is a candidate preventive medicine against amyloid b and tau oligomers. doi: 10.1093/brain/aww042
Von Bergen, M., Barghorn, S., Müller, S. A., Pickhardt, M., Biernat, J., Mandelkow, E. M., et al. (2006). The core of tau-paired helical filaments studied by scanning transmission electron microscopy and limited proteolysis. Biochemistry 45, 6446–6457. doi: 10.1021/bi052530j
von Bergen, M., Friedhoff, P., Biernat, J., Heberle, J., Mandelkow, E. M., and Mandelkow, E. (2000). Assembly of τ protein into Alzheimer paired helical filaments depends on a local sequence motif (306VQIVYK311) forming β structure. Proc. Natl. Acad. Sci. U.S.A. 97, 5129–5134. doi: 10.1073/pnas.97.10.5129
Walker, C., Preshaw, P. M., Novak, J., Hefti, A. F., Bradshaw, M., and Powala, C. (2005). Long-term treatment with sub-antimicrobial dose doxycycline has no antibacterial effect on intestinal flora. J. Clin. Periodontol. 32, 1163–1169. doi: 10.1111/j.1600-051X.2005.00840.x
Wang, Y., Garg, S., Mandelkow, E.-M., and Mandelkow, E. (2010). Proteolytic processing of tau. Biochem. Soc. Trans. 38, 955–961. doi: 10.1042/BST0380955
Ward, J. E., Ren, R., Toraldo, G., SooHoo, P., Guan, J., O'Hara, C., et al. (2011). Doxycycline reduces fibril formation in a transgenic mouse model of AL amyloidosis. Blood 118, 6610–6617. doi: 10.1182/blood-2011-04-351643
Wegmann, S., Medalsy, I. D., Mandelkow, E., and Müller, D. J. (2012). The fuzzy coat of pathological human Tau fibrils is a two-layered polyelectrolyte brush. Proc. Natl. Acad. Sci. U.S.A. 110, E313–E321. doi: 10.1073/pnas.1212100110
Wilham, J. M., Orrú, C. D., Bessen, R. A., Atarashi, R., Sano, K., Race, B., et al. (2010). Rapid end-point quantitation of prion seeding activity with sensitivity comparable to bioassays. PLoS Pathogens 6:e1001217. doi: 10.1371/journal.ppat.1001217
Wischik, C. M., Novak, M., Thogersen, H. C., Edwards, P. C., Runswick, M. J., Jakes, R., et al. (1988). Isolation of a fragment of tau derived from the core of the paired helical filament of Alzheimer disease. Proc. Natl. Acad. Sci. U.S.A. 85, 4506–4510. doi: 10.1073/pnas.85.12.4506
Yim, C. W., Flynn, N. M., and Fitzgerald, F. T. (1985). Penetration of oral doxycycline into the cerebrospinal fluid of patients with latent or neurosyphilis. Antimicrob. Agents Chemother. 28, 347–348. doi: 10.1128/AAC.28.2.347
Yu, A., Shibata, Y., Shah, B., Calamini, B., Lo, D. C., and Morimoto, R. I. (2014). Protein aggregation can inhibit clathrin-mediated endocytosis by chaperone competition. Proc. Natl. Acad. Sci. U.S.A. 111, E1481–E1490. doi: 10.1073/pnas.1321811111
Keywords: tauopathies, Alzheimer's disease, doxycycline, protein aggregation, tau
Citation: Medina L, González-Lizárraga F, Dominguez-Meijide A, Ploper D, Parrales V, Sequeira S, Cima-Omori M-S, Zweckstetter M, Del Bel E, Michel PP, Outeiro TF, Raisman-Vozari R, Chehín R and Socias SB (2021) Doxycycline Interferes With Tau Aggregation and Reduces Its Neuronal Toxicity. Front. Aging Neurosci. 13:635760. doi: 10.3389/fnagi.2021.635760
Received: 30 November 2020; Accepted: 22 February 2021;
Published: 22 March 2021.
Edited by:
Christian Neri, INSERM UMR 8256, Institut National de la Santé et de la Recherche Médicale, FranceReviewed by:
Tuane Cristine Ramos Gonçalves Vieira, Federal University of Rio de Janeiro, BrazilAbhisek Mukherjee, University of Texas Health Science Center at Houston, United States
Copyright © 2021 Medina, González-Lizárraga, Dominguez-Meijide, Ploper, Parrales, Sequeira, Cima-Omori, Zweckstetter, Del Bel, Michel, Outeiro, Raisman-Vozari, Chehín and Socias. This is an open-access article distributed under the terms of the Creative Commons Attribution License (CC BY). The use, distribution or reproduction in other forums is permitted, provided the original author(s) and the copyright owner(s) are credited and that the original publication in this journal is cited, in accordance with accepted academic practice. No use, distribution or reproduction is permitted which does not comply with these terms.
*Correspondence: Sergio B. Socias, sbsocias@yahoo.com.ar; Rosana Chehín, rosanachehin@gmail.com
†These authors share senior authorship