- 1Department of Pharmacy, University of Malakand, Chakdara, Pakistan
- 2Department of Pharmacy, University of Swabi, Swabi, Pakistan
- 3University of Chinese Academy of Sciences, Beijing, China
- 4Key Laboratory for Biomedical Effects of Nanomaterials and Nanosafety, CAS Center for Excellence in Nanoscience, National Center for Nanoscience and Technology, Beijing, China
- 5Suliman Bin Abdullah Aba-Alkhail Centre for Interdisciplinary Research in Basic Sciences, International Islamic University Islamabad, Islamabad, Pakistan
- 6Institute of Basic Medical Sciences (IBMS), Khyber Medical University, Peshawar, Pakistan
- 7Department of Pharmacy, Sarhad University of Science and Information Technology (SUIT), Peshawar, Pakistan
Modern research has revealed that dietary consumption of flavonoids and flavonoids-rich foods significantly improve cognitive capabilities, inhibit or delay the senescence process and related neurodegenerative disorders including Alzheimer’s disease (AD). The flavonoids rich foods such as green tea, cocoa, blue berry and other foods improve the various states of cognitive dysfunction, AD and dementia-like pathological alterations in different animal models. The mechanisms of flavonoids have been shown to be mediated through the inhibition of cholinesterases including acetylcholinesterase (AChE), and butyrylcholinesterase (BChE), β-secretase (BACE1), free radicals and modulation of signaling pathways, that are implicated in cognitive and neuroprotective functions. Flavonoids interact with various signaling protein pathways like ERK and PI3-kinase/Akt and modulate their actions, thereby leading to beneficial neuroprotective effects. Moreover, they enhance vascular blood flow and instigate neurogenesis particularly in the hippocampus. Flavonoids also hamper the progression of pathological symptoms of neurodegenerative diseases by inhibiting neuronal apoptosis induced by neurotoxic substances including free radicals and β-amyloid proteins (Aβ). All these protective mechanisms contribute to the maintenance of number, quality of neurons and their synaptic connectivity in the brain. Thus flavonoids can thwart the progression of age-related disorders and can be a potential source for the design and development of new drugs effective in cognitive disorders.
Introduction
Flavonoids represent a diverse group of naturally occurring compounds which are biosynthesized from phenylalanine, and are ubiquitous to green pigments in the plant kingdom (Havsteen, 2002). Flavonoids have a long history of medical use for the treatment of various medical ailments (Rice-Evans and Packer, 2003). Their great diversity, distribution and easy isolation make them a dominant class of therapeutic agents. Flavonoids are the major building blocks for the synthesis of various drugs and may itself be used as natural products, thus play a pivotal role in the domain of drug design and discovery (Havsteen, 1983). Until now, more than 7,000 flavonoids have been reported from natural sources including medicinal plants, vegetables, fruits and wines. Flavonoids have the ability to bind with numerous body proteins and modify the transporters, enzymes, hormones, DNA, chelation of heavy metals and scavenge the free radicals; therefore, possess strong antioxidant properties (Havsteen, 1983; Robak and Gryglewski, 1988; Morel et al., 1993; Cushnie and Lamb, 2005). A myriad number of pharmacological studies have been reported that suggest their usefulness in the management of diabetes mellitus (DM), cancer, cardiovascular diseases, neurological disorders, inflammation and microbial diseases (Middleton et al., 2000; Marder and Paladini, 2002; Galati and O’Brien, 2004; Cushnie and Lamb, 2005).
Recent studies have shown that regular use of flavonoid-rich foodstuffs can effectively enhance cognitive capabilities in humans (Macready et al., 2009; Socci et al., 2017; Bakoyiannis et al., 2019). Additionally, several flavonoids have been reported to restrain the progression of pathologies of Alzheimer’s disease (AD) and this has been stem from their ability to quash the cognitive deficits in numerous normal and transgenic preclinical animal models (Macready et al., 2009; Spencer, 2010b; Bakoyiannis et al., 2019). The beneficial effects of flavonoids rich foods like cocoa, green tea and blue berry can be attributed to the interactions of flavonoids and their metabolites with numerous cellular and molecular targets (Yevchak et al., 2008; Mastroiacovo et al., 2014). For instance, the specific interactions of flavonoids with receptors within the ERK and PI3-kinase/Akt signaling pathways have been reported to augment the expression of neuromodulatory and neuroprotective proteins as well as enhance the number and strength of different types of neurons (Schroeter et al., 2002; Vauzour et al., 2007a; Spencer, 2008). Concomitantly, their beneficial effects on the cerebrovascular system can improve the cognitive performance of individuals via an enhancement in blood flow and stimulation of neurogenesis in brain. Several other mechanisms regarding the beneficial use of flavonoids have been recently reported (Spencer, 2009; Spencer et al., 2009). Flavonoids attenuate the initiation and progression of AD-like pathological symptoms and related neurodegenerative disorders (Williams and Spencer, 2012). The possible mechanisms for these effects include the inhibition of neuronal apoptosis induced by neuro-inflammation, oxidative stress, inhibition of key enzymes involved in the fabrication of amyloid plaques and other pathological products (Williams and Spencer, 2012). Flavonoids thus mediate their neuroprotective effects by maintaining the neuronal quality and number in the key brain areas and thus prevent the onset/progression of diseases responsible for the decrease in the cognitive function.
Methods
Recent scientific literature published in high quality journals were collected using various search engines including Google Scholar, SciFinder, Science Direct, PubMed, Web of Science, EBSCO, Scopus, JSTOR and other web sources. The scientific literature preferably on dietary flavonoids in context to their neuroprotective properties and their mechanism of action were selected. Literature with scientific rigor published up to 2017 was included.
Flavonoids Distribution in Nature
Flavonoids represent a major group of secondary metabolites which are extensively distributed in nature especially in green plants. Majority of natural flavonoids are pigments, and are usually allied with some vital pharmacological functions. Flavonoids are differentiated from each other on the basis of differences in the aglycon ring structure and state of oxidation/reduction. Moreover, based on the extent of hydroxylation of aglycon, positions of the hydroxyl groups, saturation of pyran ring and differences in the derivatization of the hydroxyl groups are major differentiating features among the various classes of flavonoids. The major nutritional sources of flavonoids include fruits, juices, vegetables, tea, cereals and wines (Manach et al., 2004). Some common flavonoids include quercetin, kaempferol (flavonols), myricetin, predominantly present in the onions, leeks and broccoli, fruits flavones including luteolin and apigenin are abundant in celery and parsley. Other common types of flavonoids include isoflavones (daidzein, genistein), which are naturally distributed in soy and soy products, flavanones including naringenin and hesperetin, present in the citrus fruits and tomatoes. Flavanols, that are represented by epigallocatechin gallate (EGCG), catechin, epicatechin and epigallocatechin are mainly sequestered in the green tea, red wine, and chocolate, whereas, anthocyanidins including malvidin, pelargonidin and cyanidinare are widely distributed in the berry fruits and red wine (Manach et al., 2005; Figure 1).
Chemistry
Flavonoids are abundantly present as polyphenols in plants that are the products of secondary metabolites. The basic chemical structure of flavonoids contains two benzene rings (A and C) connected by a pyran ring B (Figure 2). One of the benzene ring (A) is fused with the pyran ring while the other benzene ring (C) is attached as substituent to the pyran ring. Depending upon the pattern of substitution of benzene rings, and that of substitution, oxidation and saturation of pyran ring, various derivatives of flavonoids can be synthesized that possess unique physicochemical properties and biological activities acceptable for the efficient management of neurodegenerative diseases.
Classification
Flavonoids are classified into various groups depending on the position at which the benzene ring (C) is attached to the pyran and the degree of unsaturation and oxidation of pyran ring. These different flavonoids have a dominant role in various pharmacological activities. Each sub-type is discussed below.
Isoflavones
The class of flavonoids in which the benzene ring (C) is attached to the position 3 of the pyran ring is shown in Figure 3. Isoflavone are majorly found in various natural products especially soybean (Wang and Murphy, 1994). Several researchers have also synthesized various derivatives of isoflavone by different synthetic approaches. Wang in 2005 has synthesized various derivatives of isoflavones by Suzuki coupling (Ding and Wang, 2005). Various derivatives of this famous group of easily biodegradable antioxidant have also been synthesized with triazin (Jha et al., 1981). Similarly, utilizing the catalytic approaches, including enzymatic or using a heterogeneous catalyst have been reported for efficient synthesis of isoflavone (Kochs and Grisebach, 1986; Hoshino et al., 1988). The structures of some well-known isoflavones are given in Figure 3.
Neoflavonoids
In this class of flavonoids, the benzene ring (C) is attached to the position 4 of pyran ring. The general structure of neoflavonoids is shown in Figure 2. Neoflavonoids, are naturally occurring heterocyclic compounds, mostly famous for their antidiabetic activity (Donnelly and Boland, 1995). The neoflavonoids consist of neoflavones and neoflavenes. The most prominent source of neoflavonoids is natural but several researchers have also synthesized various analogs. Some natural sources, from which the neoflavonoids are reported, are Echinop sniveus (Singh and Pandey, 1990), Dalbergia odorifera (Chan et al., 1997), Nepalese propolis (Awale et al., 2005), Polygonum perfoliatum (Sun and Sneden, 1999) among other important medicinal plants.
Flavones
The flavones contain a double bond on the pyran ring between position 2 and 3, and a carbonyl (ketone) at position 4. Depending upon the taxonomic position of various plants, the flavones contain hydroxyl substituents at both the aromatic rings. Some commonly employed flavones from both the natural and synthetic origin are shown in Figure 4. The history of flavones from natural sources is very common since their synthetic history is also long (Fukui et al., 1968).
Flavonols
Chemically, flavonols are the alcoholic derivatives of flavones. The flavonols differ from the flavones in the hydroxyl group at position 3 of pyran ring. Generally, they can also be called as 3-hydroxyflavones. Mostly, the flavonols are synthesized by synthetic procedures. A very well-known synthesis of flavonols is by oxidation and cyclization of chalcones which ends with 3-hydroxyflavonols. Figure 5 shows the various important flavonols. In some cases, one or more hydrogen of hydroxyl group is replaced by a glucose moiety leading to a flavonol glycoside. As obvious from Figure 5 that pachypodol is not exactly a flavonol but its hydroxyl group is converted into a methoxy group. However, due to its structure resemblance, it can be classified as a derivative of 3-hydroxyflavone, a flavonol.
Flavanones
The flavanones, saturated flavones, are also known as dihydroflavones. The only difference between flavones and flavanones is the absence of double bond between position 2 and 3. These types of compounds are shown in Figure 6.
Flavanonols
The flavanonols are the 3-hydroxy flavanones and are also called dihydroflavonols. These are the flavonoids with saturated pyran ring having a hydroxyl group at position 3 and a carbonyl group at position 4. Some common examples of this class of flavonoids are shown in Figure 7.
Flavanols
The flavanols, also called flavan-3-ol are the types of flavonoids which lack the carbonyl group at position 4. The pyran ring in these types of compounds is saturated and disubstituted at position 2 and 3. This property of the structure leads to four possible diastereomers of a flavanol. In flavanols, the benzene ring (C) is attached to position 2 while the hydroxyl groups at position 3 of pyran ring. The structures of this type of flavonoids are shown in Figure 8. Of these, flavonoids not exactly fit in the definition of flavanol because of a lack of hydroxyl group at position 3. But, still can be categorized under the heading of flavanols as it is structurally similar to other flavanol except the hydroxyl group at position 3.
Anthocyanidins
They are the only flavonoids which impart color. They are available in the cations form (as chloride salts). They are the salt derivatives of 2-phenylchromenylium (flavylium) cation. This group contains aurantinidin, capensinidin, cyaniding, delphinidin, europinidin, hirsutidin, malvidin, pelargonidin, peonidin, petunidin, pulchellidin and rosinidin. All of them are different from each other on the basis of the attached groups (denoted by R) as shown in Figure 2.
Chalcones
Although they do not have the pyran ring but are classified as flavonoids because of having a similar synthetic approach to flavonoids. Moreover, in chalcones, the pyran moiety is available as open structure. The open structure has a carbonyl conjugated to a double bond making an α, β-unsaturated ring system, an ideal Michael acceptor for many organic reactions. The structure of chalcone is shown in Figure 2.
Flavonoids and Alzheimer’S Disease
AD, a neurodegenerative disorder, which is characterized by a gradual memory loss, cognitive dysfunction, imperfection in the routine activities, and a decrease in the intellectual learning process (Sadiq et al., 2015; Ayaz et al., 2017b; Ovais et al., 2018). AD is the most common cause of dementia and affects approximately 5%–8% of individuals over age 65, 15%–20% of individuals over age 75, and 25%–50% of individuals over age 85. It is estimated that 35.6 million people are living with dementia worldwide (Duthey, 2013). Although, the exact etiology of AD is still not known, several mechanistic features including the deficiency of cholinesterases, deposition of β-amyloid plaques, hyperphosphorylation of tau proteins and generation of oxidative stress have been implicated in the development as well as progression of AD (Kamal et al., 2015; Ullah et al., 2016). Due to the diverse nature of these pathological targets, the development of useful anti-AD drugs is still a challenging task for the scientific community. Consequently, multiple targets including the inhibition of key enzyme implicated in AD like acetylcholinesterase (AChE), butyrylcholinesterase (BChE), β-amyloid cleaving enzyme (BACE-1), monoamine oxidase (MAO) and antioxidant agents are currently under investigation as a new therapeutic class of anti-Alzheimer’s agents (Grill and Cummings, 2010; Ahmad et al., 2016; Balducci and Forloni, 2018; Chaudhary et al., 2018).
Currently, only five drugs have been marketed for the management of AD, among them four drugs including galantamine, tacrine, rivastigmine and donepezil are cholinesterase inhibitors whereas, the fifth one is the glutamatergic system modifier called memantine (Ayaz et al., 2015). No anti-amyloid drug is currently clinically available, though several agents are in the different phases of clinical trials (Vassar, 2014). Due to the toxicity associated with the use of currently available drugs and their limited therapeutic effectiveness, the search for new anti-AD drugs is still underway (Ayaz et al., 2014; Ahmad et al., 2015). Consequently, the multi-targeting natural products based pure pharmacological moieties having more bio-safety and promising cognitive enhancing capabilities are among the potential therapeutic agents (Baptista et al., 2014; Bakhtiari et al., 2017; Farooqui, 2017; Khan et al., 2018). Flavonoids including epicatechin-3-gallate, gossypetin, quercetin and myricetin are reported to block β-amyloid, and tau aggregation, scavenge free radicals and sequester metal ions at clinically low concentrations (Ono et al., 2003; Weinreb et al., 2004; Reznichenko et al., 2006; Ansari et al., 2009). Furthermore, xanthone flavonoids have also been reported to scavenge the reactive oxygen species (ROS), inhibit MAO and AChE enzymes (Zhang et al., 2006; Khan et al., 2009; Jayasena et al., 2013). Hence, flavonoids are a promising lead class of compounds for the efficient design and development of multipotent anti-AD drugs.
Amyloid Precursor Protein (APP), Amyloid Beta (Aβ) and Alzheimer’s Disease
The amyloid precursor protein (APP) belongs to a group of transmembrane proteins having large extracellular domains (Wasco et al., 1993; Ali et al., 2017; Ayaz et al., 2017a). While members of the APP-like proteins family shares several extracellular domains like E1, E2; however, the amyloid beta (Aβ) domain is unique to the APP protein. APP is produced in the endoplasmic reticulum (ER) and subsequently transported via the Golgi apparatus to the trans-Golgi-network (TGN) where APP is found abundantly (Hartmann et al., 1997). APP is transported from TNG by TNG-derived vesicles to the surface of cells where it is enzymatically cleaved by α-secretase, γ-secretases and resulting in the formation of a soluble molecule called sAPPα. 13. This usual process of APP breakdown is non-amylogenic and does not produce Aβ. However, the processing of APP via successive actions of beta amyloid cleaving enzyme (BACE-1) and γ-secretase lead to the formation of Aβ as shown in Figure 9 (Nordstedt et al., 1993).
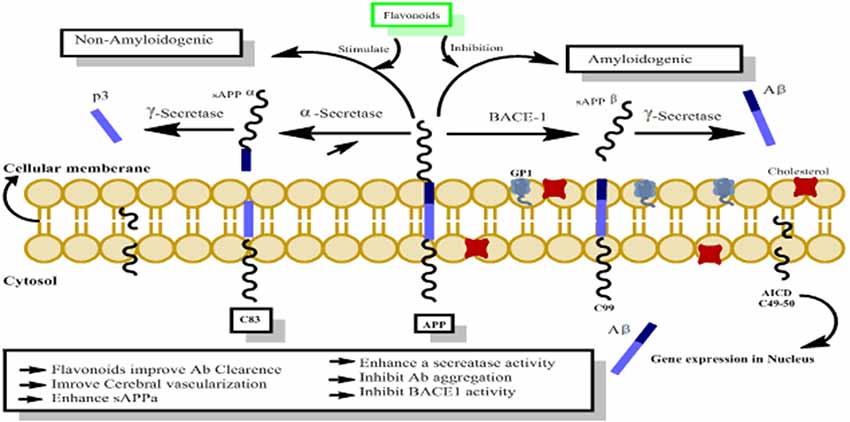
Figure 9. The probable mechanism of flavonoids activating non-amyloidogenic pathway through stimulation of α, γ secretases activities, while inhibiting the neurotoxic amylogenic pathway by inhibition of BACE-1 enzyme.
Pathological Aspects and Drug Targets
Flavonoids as Cholinesterase Inhibitors
Cholinesterases including AChE, and BChE are involved in the breakdown of acetylcholine (ACh), which is responsible for the impulse transmission across various synapses (Voet and Voet, 1995). Due to the scarcity of ACh in AD, the use of cholinesterase inhibitors is among the useful therapeutic options to maintain the accumulation of neurotransmitter for a long time at the synapse (Bachman et al., 1992). The data regarding the currently available drugs indicate that employing this approach is the most useful target in AD symptomatic therapy, thus streamlining the eventual clinical approval of four drugs (Atta-Ur-Rahman et al., 2004). This approach is also successfully employed in the management of Parkinson’s disease, ataxia and dementia (Ahmad et al., 2003). Owing to the unwanted effects and limited efficacy of the currently available drugs, there is a dire need to develop more safe and effective drugs (Schneider, 2001). Several flavonoids including genistein, kaempferol, apigenin, naringin, quercetin, diosmin, silymarin and silibinin were tested against cholinesterases (AChE, BChE). Among these flavonoids, quercetin was found most active and exhibiting a 76.2% inhibition of AChE. Other compounds including genistein, leteolin and silibinin showed a 65.7, 54.9 and 51.4% inhibitions against BChE, respectively (Orhan et al., 2007). In a published report, Uriarte-Pueyo and Calvo (2011) summarized 128 flavonoids with respect to their AChE inhibitory potentials. Based on their potency as cholinesterase inhibitors, they were considered to be promising therapeutic agents in the development of new anti-Alzheimer drugs.
Flavonoids as Free Radicals’ Scavengers
Free radicals are generated during the aerobic respiration and are counteracted by the bodily diverse system of antioxidants. When the free radicals are generated in excess, they lead to oxidative stress and thus disturb the functions of different proteins, lipids and essential body elements (Markesbery and Lovell, 2007). Besides their role in several disease processes, free radicals are implicated in the inflammatory damage to neurons and development of AD. The oxidative stress is a key aspect of AD as indicated from the elevated level of oxidative stress markers (Lovell and Markesbery, 2007). Moreover, low concentrations of antioxidants and antioxidant activity have been detected in the plasma of patients diagnosed with AD (Mecocci et al., 2002; Rinaldi et al., 2003). Additionally, the elevated lipid and protein oxidation byproducts were also observed in the transgenic animal models of AD (Resende et al., 2008). The AD pathogenic markers including Aβ and neurofibrillary tangles (NFTs) were also high in animals having oxidative stress, which may suggest that the free radicals are among the initiators of AD (Dumont and Beal, 2011). Nearly all the ROS are generated in the mitochondria (Kowaltowski et al., 2009). In AD patients a deficiency of cytochrome c oxidase leads to the mitochondrial dysfunction and results in the excessive generation of ROS (Müller et al., 2010). Aβ is also considered as mitochondrial poison and is known to initiate the excessive release of free radicals in the presence of metal ions (Butterfield et al., 2007). In this regard, the use of ions like clioquinol is known to exhibit useful effects in transgenic animal models of AD (Grossi et al., 2009).
Activation of glial cells is another hallmark of AD and neurodegenerative disorders (Craft et al., 2005; Balducci and Forloni, 2018). The activation of microglia not only generates pro-inflammatory cytokines but also increases the formation of superoxide anions using NADPH oxidase (NOX). The presence of elevated levels of NOX subunits in the brains of AD and the subsequent improvement of cognitive and cerebrovascular functions after NOX gene removal from the transgenic animals support its potential involvement in the pathogenesis of AD (Park L. et al., 2008). Moreover, in the activated glial cells, inducible nitric oxide synthase (iNOS) sets free the NO, which subsequently reacts with the superoxide and forms peroxinitrite thereby exerting nitrosative stress. Their involvement has been supported by the genetic removal of iNOS which results in the amelioration of gliosis, reduction in Aβ load and phosphorylation of tau proteins in the transgenic animals (Nathan et al., 2005). Catechins and polyphenols of green tea are strong antioxidants, which chelate metal ions and scavenge free radicals (Singh et al., 2008). EGCG prevents oxidative stress-induced DNA damage by transferring an electron to the ROS-induced radical sites (Singh et al., 2008). The green tea suppresses propagation of chain reaction during the lipid peroxidation initiated by the iron ascorbate in the mitochondrial membranes of brain. Among the catechins, EGCG is observed to be the most efficient scavenger (Mandel et al., 2008). EGCG inhibits fibril formation during Aβ aggregation and attenuates the lipid peroxidation as initiated by the Aβ (Choi et al., 2001; Lee et al., 2009). EGCG also inhibits Aβ-induced apoptosis, caspase activity, thus enhancing the survival of hippocampus neurons (Choi et al., 2001).
Effectiveness in Alzheimer’s Disease and Dementia
The effectiveness of flavonoids in the prevention of AD and cognitive dysfunctions in animal models has been reported, which signify their therapeutic use in the management of neurological disorders. Flavonoids mediate their anti-amyloidogenic effect by targeting key enzymes implicated in the pathological production and accumulation of amyloid plaques (Aβ). Anthocyanin-rich flavonoids found in bilberry and black currant extracts have been recently reported to prevent behavioral abnormalities and alter APP processing in APP/PS1 mouse model of AD (Vepsäläinen et al., 2013). Likewise, chronic therapy with tannic acid using transgenic PSAPP animal model of cerebral amyloidosis has revealed potential amelioration of transgene-mediated deficits in the memory and behavior of animals. A citrus flavonoid nobiletin, has been reported to improve Aβ mediated memory deficits and reduce Aβ load in the hippocampus of transgenic animals (Onozuka et al., 2008). Furthermore, chronic administration of grapes polyphenols leads to improvement in the memory and diminish the level of soluble Aβ oligomers in the brain tissues of Tg2576 animals (Wang et al., 2008). Luteolin, a citrus flavonoid has been shown to decrease the formation of Aβ peptides in APP transgenic neuronal cells and lower the activity of BACE1 (Rezai-Zadeh et al., 2009). Moreover, chronic administration of polyphenol-rich grape seed extracts and curcumin for 9 months inhibit the deposition of Aβ in the brain of AD animals (Rezai-Zadeh et al., 2009).
Numerous studies have demonstrated various beneficial aspects of green tea. Epigallocatechin-3-gallate (EGCG), a green tea polyphenol has been reported to reduce the Aβ load via inhibition of APP modulating enzyme (Rezai-Zadeh et al., 2005, 2008). The naturally occurring flavonoids including curcumin and EGCG are reported to restrain Aβ-mediated BACE1 upregulation in the neuronal cultures (Shimmyo et al., 2008). Isorhamnetin has shown a neuroprotective effect against Aβ-induced memory impairment (Asha and Sumathi, 2016). It enhances cognition and memory by uplifting antioxidant defense system, cholinergic signaling, and synaptic plasticity (Ishola et al., 2019). Kaempferol attenuates cognitive deficit through regulating antioxidants and neuro-inflammation (Kouhestani et al., 2018), promotes memory retention and density of hippocampal CA1 neurons (Darbandi et al., 2016). The flavonoid, quercetin has potential therapeutic benefit in AD. Quercetin produces a reduction in plaque burden and mitochondrial dysfunction through the activation of AMPK and may be one of the mechanisms by which quercetin improves cognitive functioning (Wang et al., 2014).
The EGCG-induced increase of non-amyloidogenic APP processing was observed to be carried out through the estrogen receptor-α/phosphoinositide 3-kinase/Ak-transforming based mechanisms. As the post-menopausal depletion of estrogen has been linked to an increased risk of AD development, thus, selective estrogen receptor modulators can be an alternative therapeutic option in the treatment of AD. The use of EGCG mediated estrogen receptor modulation could be an alternative to estrogen-based therapy in the management of this disease (Fernandez et al., 2010). EGCG also produce beneficial neuroprotective effects via inhibition of amyloid fibrils sheet rich in Aβ and inhibition of fibrillogenesis. The fibrillogenesis reticence is mediated by direct binding with unfolded polypeptides and inhibition of their conversion to neurotoxic intermediates (Ehrnhoefer et al., 2008). Moreover, EGCG is capable of splitting large size Aβ fibrils to small proteins and thus are not able to aggregate and thereby devoid of any toxic effects (Bieschke et al., 2010). The flavonoid, myricetin has shown potential in vitro anti-amyloid activity and thus possesses prospective beneficial effect for neurodegeneration related cognitive disorders (Ono et al., 2003; Hirohata et al., 2007). In general, these reports advocate that some flavonoids have the capability to interrupt fibrillization process of Aβ formation, inhibit a vital enzyme BACE1 implicated in the formation of Aβ, which lead to inhibition of Aβ production. Nevertheless, further studies are required to uncover the neuro-modulating potentials and underlying mechanisms of flavonoids for clinical use.
Flavonoids as Tau Modifying Agents
Several reported studies describe the effects of flavonoids in the formation of highly phoshorylated tau proteins, a pathological hallmark of AD (Calcul et al., 2012; Baptista et al., 2014). For instance, myrecetin and epicatechin-5-gallate have been reported to avert heparin-mediated tau formation (Taniguchi et al., 2005). Epicatechin-5-gallate administration in the transgenic animal models of AD has been shown to modulate tau profiles by suppressing the formation of sarkosyl-soluble phosphorylated tau isoforms (Rezai-Zadeh et al., 2008). In other studies using grape seed proanthocyanidin extract (GSPE), tau neuropathology was significantly reduced in animals model of AD via inhibition of tau peptide aggregations, its destabilization and its eventual clearance (Pasinetti et al., 2010; Wang et al., 2010). Hyperphosphorylation of tau proteins with subsequent accumulation as NFTs is a major contributor in the cognitive dysfunctions. Several kinases like GSK-3β are known to contribute to the phosphorylation of tau protein and are implicated in the pathogenesis of AD. Flavonoids inhibit the activities of several kinases and thus aid in the prevention of AD. For instance, indirubins restrain the activities of protein kinases including CDK5/p25 and GSK-3β, both of which are implicated in the abnormal phosphorylation of tau proteins observed in the AD patients (Figure 10; Leclerc et al., 2001). Another flavonoid, morin is reported to inhibit the activity of GSK-3β and obstruct GSK-3β-mediated phosphorylation of tau proteins. Morin also diminishes Aβ-mediated phosphorylation of tau proteins and provides protection against Aβ induced cytotoxicity in human neuroblastoma cells. Furthermore, morin therapy has been shown to reduce tau hyperphosphorylation in the hippocampal neurons of transgenic animals (3xTg-AD mice; Gong et al., 2011). Cyanidin 3-O-glucoside (Cy3G) has also afforded a significant protection against cognitive dysfunctions induced by administration of Aβ in animal models which is mediated by modulation of GSK-3β/tau (Qin et al., 2013).
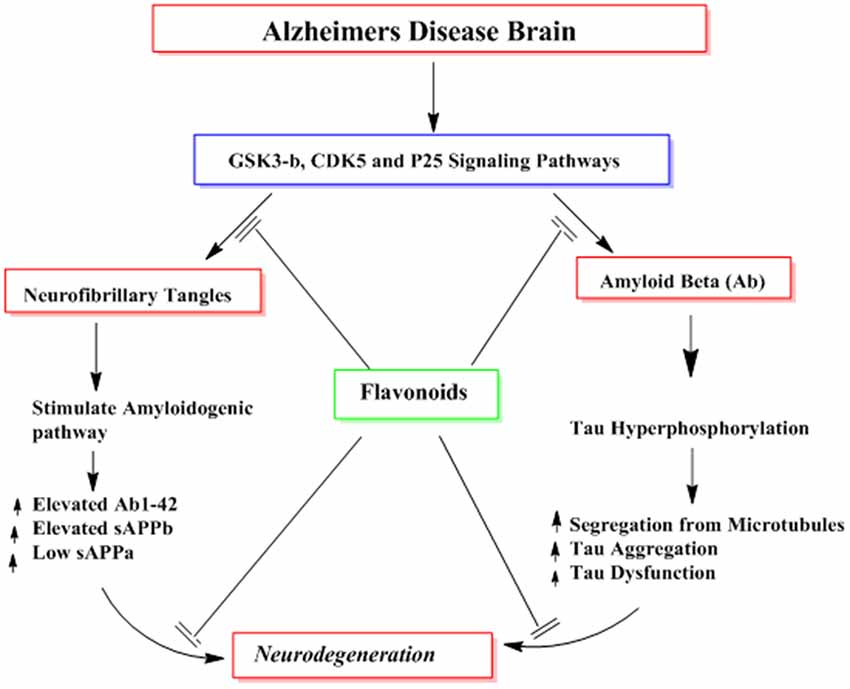
Figure 10. The probable mechanisms of flavonoids in inhibiting different signaling pathways implicated in the formation of neurofibrillary tangles (NFTs) and amyloid plaques (Aβ).
Neuro-Inflammation and Neurotoxins Modulating Effects
The neurodegenerative outcomes observed in various neurological disorders appear to be elicited by several events like neuro-inflammation, depletion of endogenous antioxidants, glutamatergic excitotoxicity and neurotoxicity mediated by various metabolic products (Jellinger, 2001). Scientific evidence suggest that flavonoids might counteract the underlying mechanisms of neuronal injuries and can hamper the progression of different neurodegenerative disorders (Mandel and Youdim, 2004; Spencer, 2008). Consumption of green tea has been reported to reduce the risk of Parkinson’s disease, attenuate neurodegeneration and ischemic hippocampal injury, which can be attributed to the presence of EGCG (Lee et al., 2000; Weinreb et al., 2004). EGCG is also known to modulate various signaling pathways particularly protein kinase C and PI3-kinase which are implicated in the neuroprotection and reduce the nigral damage by chelating free radicals (Mandel et al., 2005; Weinreb et al., 2009).
Various in vitro studies also corroborated the idea that flavonoids prevent the pathological aspects of Parkinson’s disease by inhibiting the formation of endogenous neurotoxin 5-S-cysteinyldopamine (Vauzour et al., 2007b). Moreover, the neuroprotective effects of flavonoids have also been reported in other diseases like Huntington disease, mediated via ERK pathway (Maher et al., 2006, 2011). Naringenin, a citrus flavanone has been reported to reduce the neuronal injury via inhibition of lipopolysaccharide/interferon-γ-induced glial cells activation and inhibition of p38/STAT-1 pathway (Vafeiadou et al., 2009). Naringenin also inhibits the production of nitric oxide in the activated microglia cells. Blueberry flavonoids also have been shown to attenuate the production of TNF-α, nitric oxide and IL-1β in activated microglia cells (Lau et al., 2007). Other flavonoids including quercetin, wogonin, bacalein and EGCG have been shown to modulate neuro-inflammation and microglial/astrocyte-mediated nitric oxide production (Lee et al., 2003; Chen et al., 2005). All these actions are mediated by transformation of protein, lipid kinase signaling pathways, nitric oxide production, pro-inflammatory transcription factors, downstream regulation of iNOS and cyclooxygenase (COX-2) expression, free radicals scavenging, NOX activation and liberation of cytokine (Jang et al., 2008; Zheng et al., 2008). EGCG and genistein are reported to enhance the production of glutathione via PI3-kinase-reliant regulation of nuclear factor erythroid 2–related factor 2 (Nrf2)-induced antioxidant pathway (Hernandez-Montes et al., 2006).
Flavonoids for Better Cognition
Several studies highlight the beneficial effects of flavonoid-rich foodstuffs’ consumption on cognition (Commenges et al., 2000; Letenneur et al., 2007; Spencer, 2010a). Isoflavones from soy and soy-derived foods have been reported to improve learning and memory possibly by their potential to mimic the activity of estrogens in brain (File et al., 2001). These isoflavones also modulate the neuronal concentrations of ACh and neurotrophic factors including the brain derived neurotrophic factor (BDNF) and nerve growth factor (NGF) in the hippocampus and frontal cortex regions of brain (Pan et al., 1999a,b).
The use of flavonoids rich foods including grapes juice, cocoa and blueberry have shown to possess potential cognition-enhancing effects (Krikorian et al., 2010; Scholey et al., 2010; Shukitt-Hale, 2012). Behavioral evidences suggest that periodic consumption of flavonoids rich fruits like pomegranate, blueberry, grapes, strawberry, as well as pure compounds including quercetin and EGCG are able to improve cognitive performance as indicated from the improvement in the overall scores of memory acquisition, short and long term memory, memory retention and retrieval (Joseph et al., 1999; Hartman et al., 2006). The above mentioned fruits are rich in flavanols and anthocyanins which improve cognitive and spatial working memory deficits in animal models (Joseph et al., 1998; Shukitt-Hale et al., 2009). Additionally, pure EGCG can improve the retention of spatial memory (van Praag et al., 2007). Flavonoids from blueberry also improve the processing of spatial memory via its action on the dentate gyrus (DG), which is highly sensitive to the effects of aging (Small et al., 2004; Burke and Barnes, 2006). Blueberry flavonoids have been reported to boost up precursor cells proliferation in the DG of animal models, thus increasing DG neurogenesis and improve cognitive capabilities (Casadesus et al., 2004). However, further characterization of these food supplements, isolation of pure natural compounds and their comparison to the already established flavonoids may provide more useful insights into the memory enhancing properties of dietary flavonoids.
Flavonoids Interactions With Useful Signaling Pathways
Flavonoids are able to preferentially bind with the neuronal receptors including GABAA, tyrosine receptor kinase B (TrkB), δ-opioid, estrogen, testosterone, nicotinic and adenosine receptors and mediate the various neuropharmacological actions (Ji et al., 1996; Katavic et al., 2007; Fernandez et al., 2008; Lee et al., 2010). Several reports regarding the beneficial neuroprotective effects of flavonoids and their metabolites via interactions with neuronal signaling pathways have been published (Spencer, 2007; Incani et al., 2010). They interact with several protein kinase and lipid kinase signaling pathways like tyrosine kinase, mitogen-activated kinase (MAPK), PI3K/Akt, protein kinase C and nuclear factor κB pathway (Gamet-Payrastre et al., 1999; Schroeter et al., 2001; Incani et al., 2010). When flavonoids bound to these receptors, they may stimulate or inhibit the receptors and thus mediate their actions via modulation of gene expression or phosphorylation. Subsequently, they modulate the synaptic protein synthesis, neuronal plasticity and other morphological changes responsible for neurodegenerative disorders and impairment in cognition. For instance, flavonoids and their metabolites have been reported to interact with MAPKs signaling pathways (MEK1 and MEK2 receptors) which result in downstream activation of cAMP response element binding protein (CREB), thus leading to significant changes in synaptic plasticity and memory (Finkbeiner et al., 1997; Impey et al., 1998). Supplementation of flavanols and anthocyanins rich blueberry have been reported to enhance cognitive performance in animals via activation of CREB and elevation of BDNF levels in hippocampus (Williams et al., 2008). Furthermore, chronic administration of green tea catechins can reduce the levels of Aβ1–42 oligomers, elevate the activities of kinase A/cAMP-response element binding protein (PKA/CREB) pathway and up-regulated the action of synaptic plasticity related proteins in the hippocampus (Li et al., 2009). Moreover, flavonoids stabilize hypoxia-inducible factor-1 (HIF-1) and Nrf2 transcription factors (Park S. S. et al., 2008), activate peroxisome proliferator-activated receptor-γ coactivator-1 (PGC-1α) pathway (Zhang et al., 2010), and act as modulators of peroxisome proliferator-activated receptor gamma (PPAR-γ; Feng et al., 2016). These molecular changes produced by flavonoids may improve AD pathophysiology by protecting neurons against oxidative stress, improve mitochondrial dysfunction, reduce insulin resistance, and thus ameliorate cognitive impairment (Figure 11).
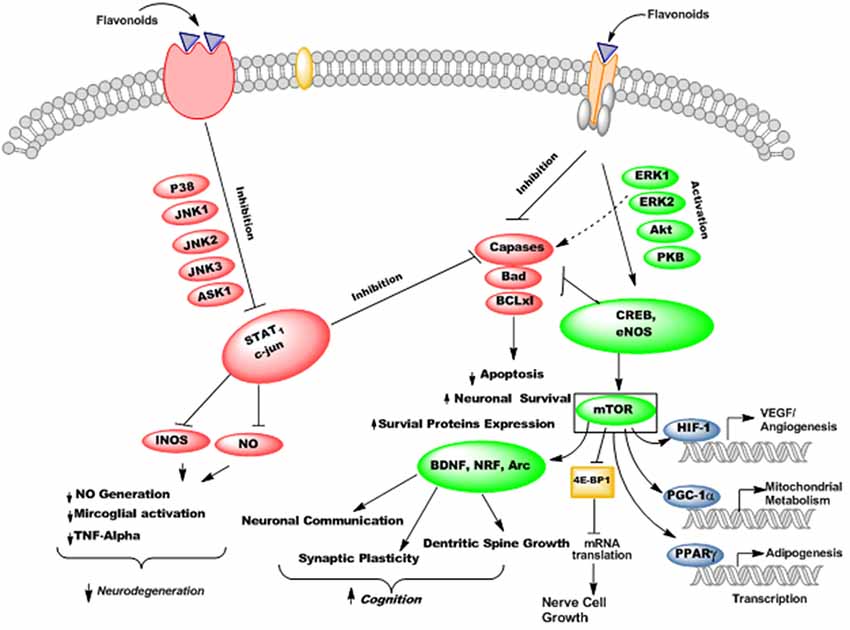
Figure 11. The probable mechanisms of flavonoids stimulating/inhibiting signaling pathways implicated in cognitive performance and neurodegeneration.
Flavonoids possess PI3-kinase modulating potentials (Figure 12), by directly interacting with its ATP binding site (Vlahos et al., 1994). Moreover, quercetin and its metabolites inhibit prosurvival Akt/PKB signaling pathways through inhibition of PI3-kinase activity (Spencer et al., 2003). On the contrary, some flavanones like hesperetin activate Akt/PKB signaling pathway and impart prosurvival characteristics in the cortical neurons (Vauzour et al., 2007a). Moreover, epicatechin-5-gallate has been reported to modulate neurotransmission, synaptogenesis and plasticity mediated through stimulation of extracellular signal regulated kinase (ERK), PI3K reliant raise in CREB phosphorylation and upregulation of GluR2 levels in cortical neurons (Schroeter et al., 2007).
In a study, the chronic ingestion of blueberry is reported to increase Akt phosphorylation, activation of downstream mammalian target of rapamycin (mTOR) receptor and increase the content of Arc/Arg3.1 (activity-regulated cytoskeletal-associated protein) in the hippocampus (Williams et al., 2008). As Arc is regulated by BDNF and is important in the long term potentiation (LTP), therefore these changes may be related to the improvement of spatial memory and cognition (Waltereit et al., 2001; Yin et al., 2002). This has been supported by various studies regarding the effects of flavonoids on changes in the neuronal morphologies (van Praag et al., 2007).
Overview of Mechanisms Underpinning the Therapeutic Effects of Flavonoids in Neurodegeneration
Flavonoids by virtue of their low molecular weight, impact multiple cellular targets simultaneously and thus mediate their beneficial neuropharmacological effects in neurodegeneration. Flavonoids interact with several neuronal and glial signaling pathways implicated in neuronal functions and survival (Williams et al., 2004; Spencer, 2010a). They also up-regulate the body antioxidant system and expression of proteins related to neuronal repair and synaptic plasticity (Kong et al., 2000; Eggler et al., 2008). They modulate cerebral blood flow and inhibit neuropathological processing in different regions of brain (Dinges, 2006). The probable mechanism underlying these neuromodulatory properties of flavonoids is shown in Figure 13.
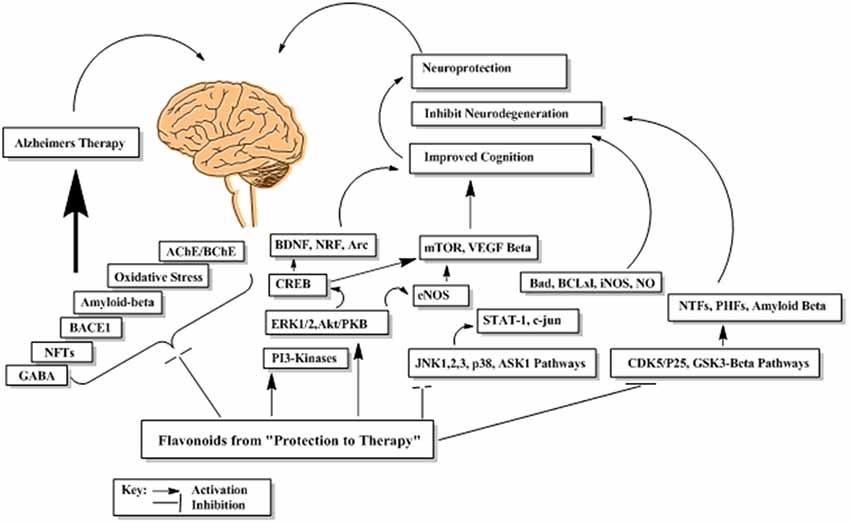
Figure 13. The probable abridged mechanism of flavonoids in enhancing cognition and suppression of neurodegeneration.
Toxicological Propensity of Flavonoids
The wide availability of flavonoids and their recent increase consumption by humans has raised important questions regarding the potential toxicity of these dietary components. Although majority of natural products are well tolerated; however, flavonoids and related phytochemicals have been shown to induce neurobehavioral and endocrine disrupting effects (Bugel et al., 2016; Patisaul, 2017). The toxicity of flavonoids is very low in animals. For rats, the LD50 has been reported as 2–10 g per animal for most flavonoids. Similar doses in humans are quite unrealistic. As a precaution, doses less than 1 mg per adult per day have been recommended for humans (Galati and O’Brien, 2004). High doses of quercetin over several years has shown to result in the formation of tumors in mice. However, in other long-term studies, no carcinogenicity was found (Dunnick and Halley, 1992). Flavonoids can either inhibit or induce human cytochrome P450 (CYPs) depending upon their structures, concentrations. The interactions of flavonoids with CYP3A4, the predominant human hepatic and intestinal CYP responsible for metabolizing 50% of therapeutic agents is of particular interest. The simultaneous administration of flavonoids and clinically used drugs may cause flavonoid–drug interactions by modulating the pharmacokinetics of certain drugs (Hodek et al., 2002; Galati and O’Brien, 2004).
Conclusion and Future Directions
The dietary use of flavonoid-rich foodstuffs has the propensity to lessen age-related decline in cognition and may restore memory functions as well as attenuate the development of conditions associated with dementia. The therapeutic importance of natural products in neurodegeneration has been attributed from their various modulatory neuropharmacological properties (Table 1). Further studies are required especially well-designed clinical trials to endorse the clinical effectiveness of flavonoids in neurodegeneration associated clinical signs and symptoms. Moreover, various in vivo studies should be designed to obtain a better insight of flavonoids efficacy with regard to their bioavailability, potential toxicities and accumulation at the target sites in the aging brain. For instance, providing a direct link between behavioral responses in test animals/humans to changes in the cortical, and hippocampal areas, the underlying molecular events linked to synaptic plasticity, effects on neuronal stem cells proliferation and changes in the cerebral blood flow will provide guidelines for flavonoids-based dietary applications and subsequent clinical recommendations in neurological disorders. The use of imaging and spectroscopic techniques like MRI and NMR can provide a better understanding of flavonoids-based changes in cerebral blood flow, quantitative changes in neuronal stem cells, progenitor cells and gray matter density along with electrophysiological changes. All these efforts will provide mechanism based links between flavonoids therapy and brain functions and information related to their effective doses. In relation to AD and dementia, it is most important to explore the anti-amyloid and tau modifying effects of flavonoids both in in vitro and in vivo models. In this regard, tau modifying potentials of flavonoids have been investigated at preliminary level, yet detail studies on destabilization effects of β-amyloid, tau proteins and effects on microglial activation need to be explored. Furthermore, a recommendation regarding the dose/daily intake and duration of therapy must be provided for safe and efficacious results. Molecules which improve the function of CREB are reported to consolidate memory by promoting the gene expression responsible for the synaptic morphology and long term memory. Compounds which activate the function of upstream regulators of CREB, like Akt and ERK are considered to be highly potential memory enhancer drugs. Flavonoids are reported to concentrate in the brain and activate ERK–CREB and Akt–CREB mediated memory and are thus are promising candidates for the development of memory enhancing drugs. Regardless of significant progress in the understanding of flavonoids biology, majority of clinicians mistakenly considered them only as simple antioxidants, which is a major barrier in the development of bioactive flavonoids at the preclinical level. Now it is well known that flavonoids are much more likely to prevent both normal and disease-mediated decline in cognitive functions by modulating cellular and molecular functions of brain. Thus, flavonoids represent a group of vital precursor molecules in the quest to discover new generation of memory-enhancing agents that may be able to counteract and perhaps even quash age-related decline in cognitive functions.
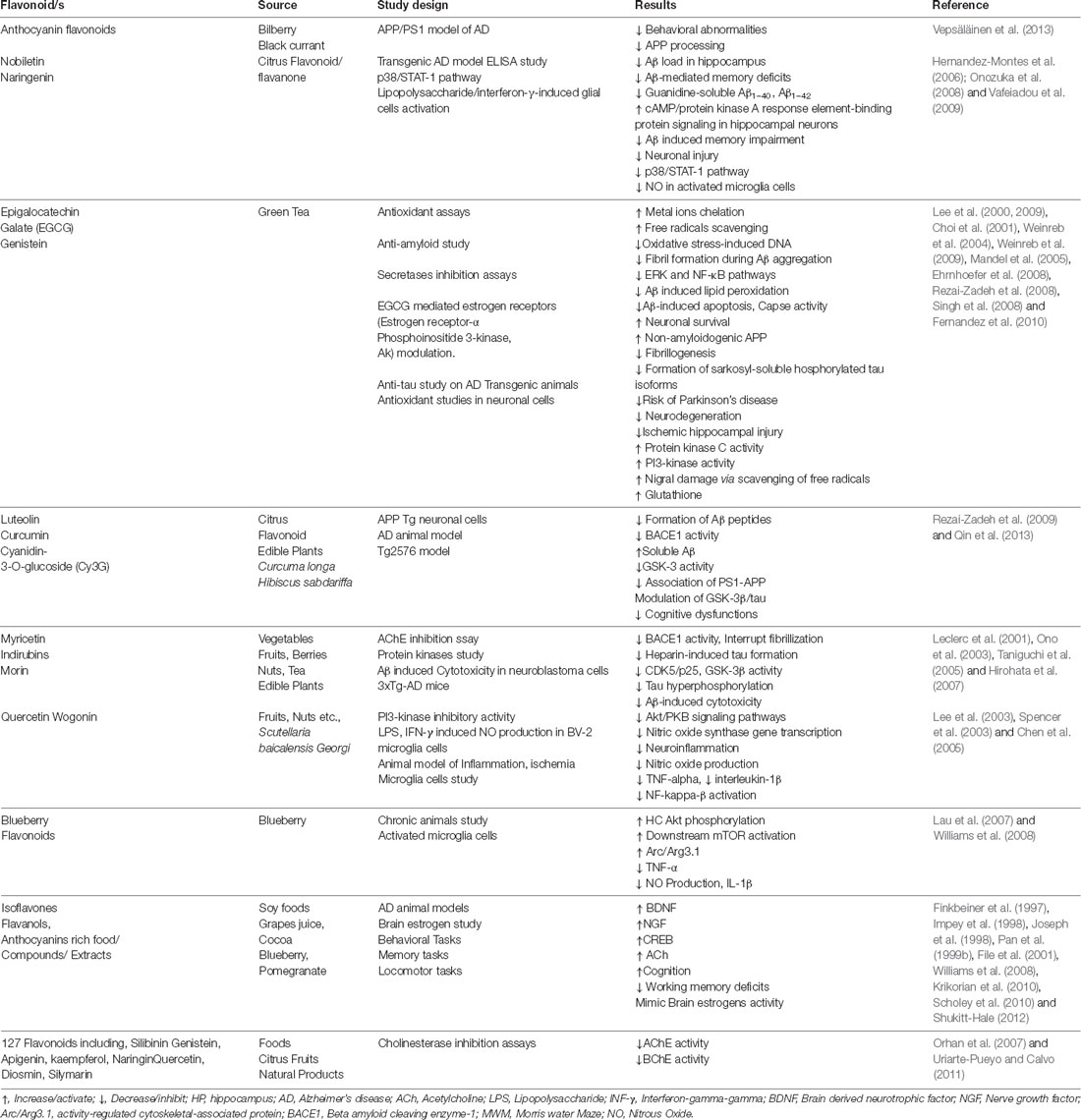
Table 1. Summary of the prospective neuropharmacological activities of essential oils and bioactive compounds isolated from medicinal plants.
Author Contributions
MA conceived the idea, carried out literature survey and drafted the manuscript. AS helped in chemistry of flavonoids and corrected the final version of the manuscript. MJ, FU, MO and IU provided useful guidelines, technical support at every step of the manuscript drafting and technical editing. MS and JA performed language and improved technical/scientific aspects of the manuscript, drafted and refined the final version of manuscript. All authors have read and approved the final version of manuscript for publication.
Funding
This work has received no specific grant from any funding agency in the public, commercial, or not for-profit sectors.
Abbreviations
AD, Alzheimer disease; NFTs, Neurofibrillary tangles; Aβ, Amyloid beta; iNOS, Inducible nitric oxide synthase; BACE1, Beta amyloid cleaving enzyme; APP, Amyloid precursor protein; ACh, Acetylcholine; AChE, Acetylcholinesterase; BChE, Butyrylcholinesterase; TGN, trans-Golgi-network; EGCG, Epigallocatechin-3-gallate; Cy3G, Cyanidin 3-O-glucoside; ROS, Reactive oxygen species; RNS, Reactive nitrogen species; CAT, catalase; CREB, cAMP response element binding protein; JNK, c-jun N-terminal kinase; p38, protein kinases; BDNF, Brain derived neurotrophic factor; SOD, Superoxide dismutase; COX-2, Cyclooxygenase 2; DG, dentate gyrus; HIF-1, Hypoxia-inducible factor 1; TrkB, Tyrosine receptor kinase beta; MAPKs, Mitogen-activated kinases; mTOR, Mammalian target of rapamycin; Nrf2, Nuclear factor erythroid 2–related factor 2; PGC-1α, Peroxisome proliferator-activated receptor-γ coactivator-1; PPAR-γ, Peroxisome proliferator-activated receptor gamma.
Conflict of Interest Statement
The authors declare that the research was conducted in the absence of any commercial or financial relationships that could be construed as a potential conflict of interest.
References
Ahmad, W., Ahmad, B., Ahmad, M., Iqbal, Z., Nisar, M., and Ahmad, M. (2003). In vitro inhibition of acetylcholinesterase, buty-rylcholinesterase and lipoxygenase by crude extract of Myricaria elegans Royle. J. Biol. Sci. 11, 1046–1049. doi: 10.3923/jbs.2003.1046.1049
Ahmad, S., Ullah, F., Ayaz, M., Sadiq, A., and Imran, M. (2015). Antioxidant and anticholinesterase investigations of Rumex hastatus D. Don: potential effectiveness in oxidative stress and neurological disorders. Biol. Res. 48:20. doi: 10.1186/s40659-015-0010-2
Ahmad, S., Ullah, F., Sadiq, A., Ayaz, M., Imran, M., Ali, I., et al. (2016). Chemical composition, antioxidant and anticholinesterase potentials of essential oil of Rumex hastatus D. Don collected from the North West of Pakistan. BMC Complement. Altern. Med. 16:29. doi: 10.1186/s12906-016-0998-z
Ali, M., Muhammad, S., Shah, M. R., Khan, A., Rashid, U., Farooq, U., et al. (2017). Neurologically potent molecules from Crataegus oxyacantha; Isolation, anticholinesterase inhibition, and molecular docking. Front. Pharmacol. 8:327. doi: 10.3389/fphar.2017.00327
Ansari, M. A., Abdul, H. M., Joshi, G., Opii, W. O., and Butterfield, D. A. (2009). Protective effect of quercetin in primary neurons against Aβ (1–42): relevance to Alzheimer’s disease. J. Nutr. Biochem. 20, 269–275. doi: 10.1016/j.jnutbio.2008.03.002
Asha, D., and Sumathi, T. (2016). Nootropic activity of isorhamnetin in amyloid β 25–35 induced cognitive dysfunction and its related mRNA expressions in Alzheimer’s disease. Int. J. Pharm. Sci. Res. 7, 3233–3242. doi: 10.13040/IJPSR.0975-8232.7(8).3233-42
Atta-Ur-Rahman, Atia-Tul-Wahab, Nawas, S. A., and Choudhary, I. M. (2004). New cholinesterase inhibiting bisbenzylisoquinoline alkaloids from Cocculus pendulus. Chem. Pharm. Bull. 52, 802–806. doi: 10.1248/cpb.52.802
Awale, S., Shrestha, S. P., Tezuka, Y., Ueda, J.-Y., Matsushige, K., and Kadota, S. (2005). Neoflavonoids and related constituents from Nepalese propolis and their nitric oxide production inhibitory activity. J. Nat. Prod. 68, 858–864. doi: 10.1021/np050009k
Ayaz, M., Junaid, M., Ahmed, J., Ullah, F., Sadiq, A., Ahmad, S., et al. (2014). Phenolic contents, antioxidant and anticholinesterase potentials of crude extract, subsequent fractions and crude saponins from Polygonum hydropiper L. BMC Complement. Altern. Med. 14:145. doi: 10.1186/1472-6882-14-145
Ayaz, M., Junaid, M., Ullah, F., Sadiq, A., Khan, M. A., Ahmad, W., et al. (2015). Comparative chemical profiling, cholinesterase inhibitions and anti-radicals properties of essential oils from Polygonum hydropiper L: a preliminary anti-Alzheimer’s study. Lipids Health Dis. 14:141. doi: 10.1186/s12944-015-0145-8
Ayaz, M., Sadiq, A., Junaid, M., Ullah, F., Subhan, F., and Ahmed, J. (2017a). Neuroprotective and anti-aging potentials of essential oils from aromatic and medicinal plants. Front. Aging Neurosci. 9:168. doi: 10.3389/fnagi.2017.00168
Ayaz, M., Junaid, M., Ullah, F., Subhan, F., Sadiq, A., Ali, G., et al. (2017b). Anti-Alzheimer’s studies on β-sitosterol isolated from Polygonum hydropiper L. Front Pharmacol. 8:697. doi: 10.3389/fphar.2017.00697
Bachman, D. L., Wolf, P. A., Linn, R. T., Knoefel, J. E., Cobb, J., Belanger, A., et al. (1992). Prevalence of dementia and probable senile dementia of the Alzheimer type in the Framingham study. Neurology 42, 115–119. doi: 10.1212/wnl.42.1.115
Bakhtiari, M., Panahi, Y., Ameli, J., and Darvishi, B. (2017). Protective effects of flavonoids against Alzheimer’s disease-related neural dysfunctions. Biomed. Pharmacother. 93, 218–229. doi: 10.1016/j.biopha.2017.06.010
Bakoyiannis, I., Daskalopoulou, A., Pergialiotis, V., and Perrea, D. (2019). Phytochemicals and cognitive health: are flavonoids doing the trick? Biomed. Pharmacother. 109, 1488–1497. doi: 10.1016/j.biopha.2018.10.086
Balducci, C., and Forloni, G. (2018). Novel targets in Alzheimer’s disease: a special focus on microglia. Pharmacol. Res. 130, 402–413. doi: 10.1016/j.phrs.2018.01.017
Baptista, F. I., Henriques, A. G., Silva, A. M., Wiltfang, J., and da Cruz e Silva, O. A. (2014). Flavonoids as therapeutic compounds targeting key proteins involved in Alzheimer’s disease. ACS Chem. Neurosci. 5, 83–92. doi: 10.1021/cn400213r
Bieschke, J., Russ, J., Friedrich, R. P., Ehrnhoefer, D. E., Wobst, H., Neugebauer, K., et al. (2010). EGCG remodels mature α-synuclein and amyloid-β fibrils and reduces cellular toxicity. Proc. Natl. Acad. Sci. U S A 107, 7710–7715. doi: 10.1073/pnas.0910723107
Bugel, S. M., Bonventre, J. A., and Tanguay, R. L. (2016). Comparative developmental toxicity of flavonoids using an integrative zebrafish system. Toxicol. Sci. 154, 55–68. doi: 10.1093/toxsci/kfw139
Burke, S. N., and Barnes, C. A. (2006). Neural plasticity in the ageing brain. Nat. Rev. Neurosci. 7, 30–40. doi: 10.1038/nrn1809
Butterfield, D. A., Reed, T., Newman, S. F., and Sultana, R. (2007). Roles of amyloid β-peptide-associated oxidative stress and brain protein modifications in the pathogenesis of Alzheimer’s disease and mild cognitive impairment. Free Radic. Biol. Med. 43, 658–677. doi: 10.1016/j.freeradbiomed.2007.05.037
Calcul, L., Zhang, B., Jinwal, U. K., Dickey, C. A., and Baker, B. J. (2012). Natural products as a rich source of tau-targeting drugs for Alzheimer’s disease. Future Med. Chem. 4, 1751–1761. doi: 10.4155/fmc.12.124
Casadesus, G., Shukitt-Hale, B., Stellwagen, H. M., Zhu, X., Lee, H.-G., Smith, M. A., et al. (2004). Modulation of hippocampal plasticity and cognitive behavior by short-term blueberry supplementation in aged rats. Nutr. Neurosci. 7, 309–316. doi: 10.1080/10284150400020482
Chan, S.-C., Chang, Y.-S., and Kuo, S.-C. (1997). Neoflavonoids from Dalbergia odorifera. Phytochemistry 46, 947–949. doi: 10.1016/s0031-9422(97)00365-8
Chaudhary, A., Maurya, P. K., Yadav, B. S., Singh, S., and Mani, A. (2018). Current therapeutic targets for Alzheimer’s disease. J. Biomed. 3, 74–84. doi: 10.7150/jbm.26783
Chen, J.-C., Ho, F.-M., Chao, P.-D. L., Chen, C.-P., Jeng, K.-C. G., Hsu, H.-B., et al. (2005). Inhibition of iNOS gene expression by quercetin is mediated by the inhibition of IκB kinase, nuclear factor-kappa B and STAT1, and depends on heme oxygenase-1 induction in mouse BV-2 microglia. Eur. J. Pharmacol. 521, 9–20. doi: 10.1016/j.ejphar.2005.08.005
Choi, Y.-T., Jung, C.-H., Lee, S.-R., Bae, J.-H., Baek, W.-K., Suh, M.-H., et al. (2001). The green tea polyphenol (−)-epigallocatechin gallate attenuates β-amyloid-induced neurotoxicity in cultured hippocampal neurons. Life Sci. 70, 603–614. doi: 10.1016/s0024-3205(01)01438-2
Commenges, D., Scotet, V., Renaud, S., Jacqmin-Gadda, H., Barberger-Gateau, P., and Dartigues, J.-F. (2000). Intake of flavonoids and risk of dementia. Eur. J. Epidemiol. 16, 357–363. doi: 10.1023/A:1007614613771
Craft, J. M., Watterson, D. M., and Van Eldik, L. J. (2005). Neuroinflammation: a potential therapeutic target. Expert Opin. Ther. Targets 9, 887–900. doi: 10.1517/14728222.9.5.887
Cushnie, T. P. T., and Lamb, A. J. (2005). Antimicrobial activity of flavonoids. Int. J. Antimicrob. Agents 26, 343–356. doi: 10.1016/j.ijantimicag.2005.09.002
Darbandi, N., Ramezani, M., Khodagholi, F., and Noori, M. (2016). Kaempferol promotes memory retention and density of hippocampal CA1 neurons in intra-cerebroventricular STZ-induced experimental AD model in Wistar rats. Biologija 62, 157–168. doi: 10.6001/biologija.v62i3.3368
Ding, K., and Wang, S. (2005). Efficient synthesis of isoflavone analogues via a Suzuki coupling reaction. Tetrahedron Lett. 46, 3707–3709. doi: 10.1016/j.tetlet.2005.03.143
Dinges, D. F. (2006). Cocoa flavanols, cerebral blood flow, cognition and health: going forward. J. Cardiovasc. Pharmacol. 47, S223–S225. doi: 10.1097/00005344-200606001-00019
Donnelly, D., and Boland, G. (1995). Isoflavonoids and neoflavonoids: naturally occurring O-heterocycles. Nat. Prod. Rep. 12, 321–338. doi: 10.1039/np9951200321
Dumont, M., and Beal, M. F. (2011). Neuroprotective strategies involving ROS in Alzheimer disease. Free Radic. Biol. Med. 51, 1014–1026. doi: 10.1016/j.freeradbiomed.2010.11.026
Dunnick, J. K., and Halley, J. R. (1992). Toxicity and carcinogenicity studies of quercetin, a natural component of foods. Fundam. Appl. Toxicol. 19, 423–431. doi: 10.1016/0272-0590(92)90181-g
Duthey, B. (2013). Background paper 6.11: Alzheimer disease and other dementias. A Public Health Approach to Innovation 1–74. Available online: http://www.who.int/medicines/areas/priority_medicines/BP611Alzheimer.pdf. Accessed June 8, 2014.
Eggler, A. L., Gay, K. A., and Mesecar, A. D. (2008). Molecular mechanisms of natural products in chemoprevention: induction of cytoprotective enzymes by Nrf2. Mol. Nutr. Food Res. 52, S84–S94. doi: 10.1002/mnfr.200700249
Ehrnhoefer, D. E., Bieschke, J., Boeddrich, A., Herbst, M., Masino, L., Lurz, R., et al. (2008). EGCG redirects amyloidogenic polypeptides into unstructured, off-pathway oligomers. Nat. Struct. Mol. Biol. 15, 558–566. doi: 10.1038/nsmb.1437
Farooqui, A. A. (2017). Neuroprotective Effects of Phytochemicals in Neurological Disorders. New York, NY: John Wiley and Sons.
Feng, X., Weng, D., Zhou, F., Owen, Y. D., Qin, H., Zhao, J., et al. (2016). Activation of PPARγ by a natural flavonoid modulator, apigenin ameliorates obesity-related inflammation via regulation of macrophage polarization. EBioMedicine 9, 61–76. doi: 10.1016/j.ebiom.2016.06.017
Fernandez, S. P., Mewett, K. N., Hanrahan, J. R., Chebib, M., and Johnston, G. A. (2008). Flavan-3-ol derivatives are positive modulators of GABAA receptors with higher efficacy for the α2 subtype and anxiolytic action in mice. Neuropharmacology 55, 900–907. doi: 10.1016/j.neuropharm.2008.06.069
Fernandez, J. W., Rezai-Zadeh, K., Obregon, D., and Tan, J. (2010). EGCG functions through estrogen receptor-mediated activation of ADAM10 in the promotion of non-amyloidogenic processing of APP. FEBS Lett. 584, 4259–4267. doi: 10.1016/j.febslet.2010.09.022
File, S. E., Jarrett, N., Fluck, E., Duffy, R., Casey, K., and Wiseman, H. (2001). Eating soya improves human memory. Psychopharmacology 157, 430–436. doi: 10.1007/s002130100845
Finkbeiner, S., Tavazoie, S. F., Maloratsky, A., Jacobs, K. M., Harris, K. M., and Greenberg, M. E. (1997). CREB: a major mediator of neuronal neurotrophin responses. Neuron 19, 1031–1047. doi: 10.1016/s0896-6273(00)80395-5
Fukui, K., Matsumoto, T., Nakamura, S., Nakayama, M., and Horie, T. (1968). Synthetic studies of the flavone derivatives: VII. The synthesis of jaceidin. Bull. Chem. Soc. Jpn. 41, 1413–1417. doi: 10.1246/bcsj.41.1413
Galati, G., and O’Brien, P. J. (2004). Potential toxicity of flavonoids and other dietary phenolics: significance for their chemopreventive and anticancer properties. Free Radic. Biol. Med. 37, 287–303. doi: 10.1016/j.freeradbiomed.2004.04.034
Gamet-Payrastre, L., Manenti, S., Gratacap, M.-P., Tulliez, J., Chap, H., and Payrastre, B. (1999). Flavonoids and the inhibition of PKC and PI 3-kinase. Gen. Pharmacol. 32, 279–286. doi: 10.1016/s0306-3623(98)00220-1
Gong, E. J., Park, H. R., Kim, M. E., Piao, S., Lee, E., Jo, D.-G., et al. (2011). Morin attenuates tau hyperphosphorylation by inhibiting GSK3β. Neurobiol. Dis. 44, 223–230. doi: 10.1016/j.nbd.2011.07.005
Grill, J. D., and Cummings, J. L. (2010). Novel targets for Alzheimer’s disease treatment. Expert Rev. Neurother. 10, 711–728. doi: 10.1586/ern.10.29
Grossi, C., Francese, S., Casini, A., Rosi, M. C., Luccarini, I., Fiorentini, A., et al. (2009). Clioquinol decreases amyloid-β burden and reduces working memory impairment in a transgenic mouse model of Alzheimer’s disease. J. Alzheimers Dis. 17, 423–440. doi: 10.3233/jad-2009-1063
Hartmann, T., Bieger, S. C., Brühl, B., Tienari, P. J., Ida, N., Allsop, D., et al. (1997). Distinct sites of intracellular production for Alzheimer’s disease Aβ40/42 amyloid peptides. Nat. Med. 3, 1016–1020. doi: 10.1038/nm0997-1016
Hartman, R. E., Shah, A., Fagan, A. M., Schwetye, K. E., Parsadanian, M., Schulman, R. N., et al. (2006). Pomegranate juice decreases amyloid load and improves behavior in a mouse model of Alzheimer’s disease. Neurobiol. Dis. 24, 506–515. doi: 10.1016/j.nbd.2006.08.006
Havsteen, B. (1983). Flavonoids, a class of natural products of high pharmacological potency. Biochem. Pharmacol. 32, 1141–1148. doi: 10.1016/0006-2952(83)90262-9
Havsteen, B. H. (2002). The biochemistry and medical significance of the flavonoids. Pharmacol. Ther. 96, 67–202. doi: 10.1016/s0163-7258(02)00298-x
Hernandez-Montes, E., Pollard, S. E., Vauzour, D., Jofre-Montseny, L., Rota, C., Rimbach, G., et al. (2006). Activation of glutathione peroxidase via Nrf1 mediates genistein’s protection against oxidative endothelial cell injury. Biochem. Biophys. Res. Commun. 346, 851–859. doi: 10.1016/j.bbrc.2006.05.197
Hirohata, M., Hasegawa, K., Tsutsumi-Yasuhara, S., Ohhashi, Y., Ookoshi, T., Ono, K., et al. (2007). The anti-amyloidogenic effect is exerted against Alzheimer’s β-amyloid fibrils in vitro by preferential and reversible binding of flavonoids to the amyloid fibril structure. Biochemistry 46, 1888–1899. doi: 10.1021/bi061540x
Hodek, P., Trefil, P., and Stiborová, M. (2002). Flavonoids-potent and versatile biologically active compounds interacting with cytochromes P450. Chem. Biol. Interact. 139, 1–21. doi: 10.1016/s0009-2797(01)00285-x
Hoshino, Y., Miyaura, N., and Suzuki, A. (1988). Novel synthesis of isoflavones by the palladium-catalyzed cross-coupling reaction of 3-bromochromones with arylboronic acids or its esters. Org. Lett. 61, 3008–3010. doi: 10.1246/bcsj.61.3008
Impey, S., Smith, D. M., Obrietan, K., Donahue, R., Wade, C., and Storm, D. R. (1998). Stimulation of cAMP response element (CRE)-mediated transcription during contextual learning. Nat. Neurosci. 1, 595–601. doi: 10.1038/2830
Incani, A., Deiana, M., Corona, G., Vafeiadou, K., Vauzour, D., Dessì, M. A., et al. (2010). Involvement of ERK, Akt and JNK signalling in H2O2-induced cell injury and protection by hydroxytyrosol and its metabolite homovanillic alcohol. Mol. Nutr. Food Res. 54, 788–796. doi: 10.1002/mnfr.200900098
Ishola, I. O., Osele, M. O., Chijioke, M. C., and Adeyemi, O. O. (2019). Isorhamnetin enhanced cortico-hippocampal learning and memory capability in mice with scopolamine-induced amnesia: role of antioxidant defense, cholinergic and BDNF signaling. Brain Res. 1712, 188–196. doi: 10.1016/j.brainres.2019.02.017
Jang, S., Kelley, K. W., and Johnson, R. W. (2008). Luteolin reduces IL-6 production in microglia by inhibiting JNK phosphorylation and activation of AP-1. Proc. Natl. Acad. Sci. U S A 105, 7534–7539. doi: 10.1073/pnas.0802865105
Jayasena, T., Poljak, A., Smythe, G., Braidy, N., Münch, G., and Sachdev, P. (2013). The role of polyphenols in the modulation of sirtuins and other pathways involved in Alzheimer’s disease. Ageing Res. Rev. 12, 867–883. doi: 10.1016/j.arr.2013.06.003
Jellinger, K. (2001). Cell death mechanisms in neurodegeneration. J. Cell. Mol. Med. 5, 1–17. doi: 10.1111/j.1582-4934.2001.tb00134.x
Jha, H. C., Zilliken, F., and Breitmaier, E. (1981). Isoflavone Synthesis with 1, 3, 5-Triazine. Angew. Chem. Int. Ed. Engl. 20, 102–103. doi: 10.1002/anie.198101021
Ji, X.-D., Melman, N., and Jacobson, K. A. (1996). Interactions of flavonoids and other phytochemicals with adenosine receptors. J. Med. Chem. 39, 781–788. doi: 10.1021/jm950661k
Joseph, J. A., Shukitt-Hale, B., Denisova, N. A., Bielinski, D., Martin, A., McEwen, J. J., et al. (1999). Reversals of age-related declines in neuronal signal transduction, cognitive and motor behavioral deficits with blueberry, spinach, or strawberry dietary supplementation. J. Neurosci. 19, 8114–8121. doi: 10.1523/JNEUROSCI.19-18-08114.1999
Joseph, J., Shukitt-Hale, B., Denisova, N., Prior, R., Cao, G., Martin, A., et al. (1998). Long-term dietary strawberry, spinach, or vitamin E supplementation retards the onset of age-related neuronal signal-transduction and cognitive behavioral deficits. J. Neurosci. 18, 8047–8055. doi: 10.1523/JNEUROSCI.18-19-08047.1998
Kamal, Z., Ullah, F., Ayaz, M., Sadiq, A., Ahmad, S., Zeb, A., et al. (2015). Anticholinesterse and antioxidant investigations of crude extracts, subsequent fractions, saponins and flavonoids of atriplex laciniata L.: potential effectiveness in Alzheimer’s and other neurological disorders. Biol. Res. 48:21. doi: 10.1186/s40659-015-0011-1
Katavic, P. L., Lamb, K., Navarro, H., and Prisinzano, T. E. (2007). Flavonoids as opioid receptor ligands: identification and preliminary Structure-activity relationships. J. Nat. Prod. 70, 1278–1282. doi: 10.1021/np070194x
Khan, H., Marya, Amin, S., Kamal, M. A., and Patel, S. (2018). Flavonoids as acetylcholinesterase inhibitors: current therapeutic standing and future prospects. Biomed. Pharmacother. 101, 860–870. doi: 10.1016/j.biopha.2018.03.007
Khan, M. T. H., Orhan, I., Şenol, F. S., Kartal, M., Şener, B., Dvorská, M., et al. (2009). Cholinesterase inhibitory activities of some flavonoid derivatives and chosen xanthone and their molecular docking studies. Chem. Biol. Interact. 181, 383–389. doi: 10.1016/j.cbi.2009.06.024
Kochs, G., and Grisebach, H. (1986). Enzymic synthesis of isoflavones. Eur. J. Biochem. 155, 311–318. doi: 10.1111/j.1432-1033.1986.tb09492.x
Kong, A.-N. T., Yu, R., Chen, C., Mandlekar, S., and Primiano, T. (2000). Signal transduction events elicited by natural products: role of MAPK and caspase pathways in homeostatic response and induction of apoptosis. Arch. Pharm. Res. 23, 1–16. doi: 10.1007/bf02976458
Kouhestani, S., Jafari, A., and Babaei, P. (2018). Kaempferol attenuates cognitive deficit via regulating oxidative stress and neuroinflammation in an ovariectomized rat model of sporadic dementia. Neural Regen. Res. 13, 1827–1832. doi: 10.4103/1673-5374.238714
Kowaltowski, A. J., de Souza-Pinto, N. C., Castilho, R. F., and Vercesi, A. E. (2009). Mitochondria and reactive oxygen species. Free Radic. Biol. Med. 47, 333–343. doi: 10.1016/j.freeradbiomed.2009.05.004
Krikorian, R., Nash, T. A., Shidler, M. D., Shukitt-Hale, B., and Joseph, J. A. (2010). Concord grape juice supplementation improves memory function in older adults with mild cognitive impairment. Br. J. Nutr. 103, 730–734. doi: 10.1017/s0007114509992364
Lau, F. C., Bielinski, D. F., and Joseph, J. A. (2007). Inhibitory effects of blueberry extract on the production of inflammatory mediators in lipopolysaccharide-activated BV2 microglia. J. Neurosci. Res. 85, 1010–1017. doi: 10.1002/jnr.21205
Leclerc, S., Garnier, M., Hoessel, R., Marko, D., Bibb, J. A., Snyder, G. L., et al. (2001). Indirubins inhibit glycogen synthase kinase-3β and CDK5/P25, two protein kinases involved in abnormal tau phosphorylation in Alzheimer’s disease. A property common to most cyclin-dependent kinase inhibitors? J. Biol. Chem. 276, 251–260. doi: 10.1074/jbc.M002466200
Lee, B.-H., Choi, S.-H., Shin, T.-J., Pyo, M. K., Hwang, S.-H., Kim, B.-R., et al. (2010). Quercetin enhances human α7 nicotinic acetylcholine receptor-mediated ion current through interactions with Ca2+ binding sites. Mol. Cells 30, 245–253. doi: 10.1007/s10059-010-0117-9
Lee, H., Kim, Y. O., Kim, H., Kim, S. Y., Noh, H. S., Kang, S. S., et al. (2003). Flavonoid wogonin from medicinal herb is neuroprotective by inhibiting inflammatory activation of microglia. FASEB J. 17, 1943–1944. doi: 10.1096/fj.03-0057fje
Lee, J. W., Lee, Y. K., Ban, J. O., Ha, T. Y., Yun, Y. P., Han, S. B., et al. (2009). Green tea (−)-epigallocatechin-3-gallate inhibits β-amyloid-induced cognitive dysfunction through modification of secretase activity via inhibition of ERK and NF-κB pathways in mice. J. Nutr. 139, 1987–1993. doi: 10.3945/jn.109.109785
Lee, S.-R., Suh, S.-I., and Kim, S.-P. (2000). Protective effects of the green tea polyphenol (−)-epigallocatechin gallate against hippocampal neuronal damage after transient global ischemia in gerbils. Neurosci. Lett. 287, 191–194. doi: 10.1016/s0304-3940(00)01159-9
Letenneur, L., Proust-Lima, C., Le Gouge, A., Dartigues, J.-F., and Barberger-Gateau, P. (2007). Flavonoid intake and cognitive decline over a 10-year period. Am. J. Epidemiol. 165, 1364–1371. doi: 10.1093/aje/kwm036
Li, Q., Zhao, H., Zhang, Z., Liu, Z., Pei, X., Wang, J., et al. (2009). Long-term green tea catechin administration prevents spatial learning and memory impairment in senescence-accelerated mouse prone-8 mice by decreasing Aβ 1–42 oligomers and upregulating synaptic plasticity-related proteins in the hippocampus. Neuroscience 163, 741–749. doi: 10.1016/j.neuroscience.2009.07.014
Lovell, M. A., and Markesbery, W. R. (2007). Oxidative damage in mild cognitive impairment and early Alzheimer’s disease. J. Neurosci. Res. 85, 3036–3040. doi: 10.1002/jnr.21346
Macready, A. L., Kennedy, O. B., Ellis, J. A., Williams, C. M., Spencer, J. P., and Butler, L. T. (2009). Flavonoids and cognitive function: a review of human randomized controlled trial studies and recommendations for future studies. Genes Nutr. 4, 227–242. doi: 10.1007/s12263-009-0135-4
Maher, P., Akaishi, T., and Abe, K. (2006). Flavonoid fisetin promotes ERK-dependent long-term potentiation and enhances memory. Proc. Natl. Acad. Sci. U S A 103, 16568–16573. doi: 10.1073/pnas.0607822103
Maher, P., Dargusch, R., Bodai, L., Gerard, P., Purcell, J., and Marsh, J. L. (2011). ERK activation by the polyphenols fisetin and resveratrol provides neuroprotection in multiple models of Huntington’s disease. Hum. Mol. Genet. 20, 261–270. doi: 10.1093/hmg/ddq460
Manach, C., Scalbert, A., Morand, C., Rémésy, C., and Jiménez, L. (2004). Polyphenols: food sources and bioavailability. Am. J. Clin. Nutr. 79, 727–747. doi: 10.1093/ajcn/79.5.727
Manach, C., Williamson, G., Morand, C., Scalbert, A., and Rémésy, C. (2005). Bioavailability and bioefficacy of polyphenols in humans. I. Review of 97 bioavailability studies. Am. J. Clin. Nutr. 81, 230S–242S. doi: 10.1093/ajcn/81.1.230s
Mandel, S. A., Amit, T., Weinreb, O., Reznichenko, L., and Youdim, M. B. (2008). Simultaneous manipulation of multiple brain targets by green tea catechins: a potential neuroprotective strategy for Alzheimer and Parkinson diseases. CNS Neurosci. Ther. 14, 352–365. doi: 10.1111/j.1755-5949.2008.00060.x
Mandel, S. A., Avramovich-Tirosh, Y., Reznichenko, L., Zheng, H., Weinreb, O., Amit, T., et al. (2005). Multifunctional activities of green tea catechins in neuroprotection. Neurosignals 14, 46–60. doi: 10.1159/000085385
Marder, M., and Paladini, A. C. (2002). GABAA-receptor ligands of flavonoid structure. Curr. Top. Med. Chem. 2, 853–867. doi: 10.2174/1568026023393462
Mandel, S., and Youdim, M. B. (2004). Catechin polyphenols: neurodegeneration and neuroprotection in neurodegenerative diseases. Free Radic. Biol. Med. 37, 304–317. doi: 10.1016/j.freeradbiomed.2004.04.012
Markesbery, W. R., and Lovell, M. A. (2007). Damage to lipids, proteins, DNA, and RNA in mild cognitive impairment. Arch. Neurol. 64, 954–956. doi: 10.1001/archneur.64.7.954
Mastroiacovo, D., Kwik-Uribe, C., Grassi, D., Necozione, S., Raffaele, A., Pistacchio, L., et al. (2014). Cocoa flavanol consumption improves cognitive function, blood pressure control and metabolic profile in elderly subjects: the Cocoa, Cognition and Aging (CoCoA) Study—a randomized controlled trial. Am. J. Clin. Nutr. 101, 538–548. doi: 10.3945/ajcn.114.092189
Mecocci, P., Polidori, M. C., Cherubini, A., Ingegni, T., Mattioli, P., Catani, M., et al. (2002). Lymphocyte oxidative DNA damage and plasma antioxidants in Alzheimer disease. Arch. Neurol. 59, 794–798. doi: 10.1001/archneur.59.5.794
Middleton, E., Kandaswami, C., and Theoharides, T. C. (2000). The effects of plant flavonoids on mammalian cells: implications for inflammation, heart disease and cancer. Pharmacol. Rev. 52, 673–751.
Morel, I., Lescoat, G. R., Cogrel, P., Sergent, O., Pasdeloup, N., Brissot, P., et al. (1993). Antioxidant and iron-chelating activities of the flavonoids catechin, quercetin and diosmetin on iron-loaded rat hepatocyte cultures. Biochem. Pharmacol. 45, 13–19. doi: 10.1016/0006-2952(93)90371-3
Müller, W. E., Eckert, A., Kurz, C., Eckert, G. P., and Leuner, K. (2010). Mitochondrial dysfunction: common final pathway in brain aging and Alzheimer’s disease—therapeutic aspects. Mol. Neurobiol. 41, 159–171. doi: 10.1007/s12035-010-8141-5
Nathan, C., Calingasan, N., Nezezon, J., Ding, A., Lucia, M. S., La Perle, K., et al. (2005). Protection from Alzheimer’s-like disease in the mouse by genetic ablation of inducible nitric oxide synthase. J. Exp. Med. 202, 1163–1169. doi: 10.1084/jem.20051529
Nordstedt, C., Caporaso, G., Thyberg, J., Gandy, S., and Greengard, P. (1993). Identification of the Alzheimer β/A4 amyloid precursor protein in clathrin-coated vesicles purified from PC12 cells. J. Biol. Chem. 268, 608–612.
Ono, K., Yoshiike, Y., Takashima, A., Hasegawa, K., Naiki, H., and Yamada, M. (2003). Potent anti-amyloidogenic and fibril-destabilizing effects of polyphenols in vitro: implications for the prevention and therapeutics of Alzheimer’s disease. J. Neurochem. 87, 172–181. doi: 10.1046/j.1471-4159.2003.01976.x
Onozuka, H., Nakajima, A., Matsuzaki, K., Shin, R.-W., Ogino, K., Saigusa, D., et al. (2008). Nobiletin, a citrus flavonoid, improves memory impairment and Aβ pathology in a transgenic mouse model of Alzheimer’s disease. J. Pharmacol. Exp. Ther. 326, 739–744. doi: 10.1124/jpet.108.140293
Orhan, I., Kartal, M., Tosun, F., and Şener, B. (2007). Screening of various phenolic acids and flavonoid derivatives for their anticholinesterase potential. Z. Naturforsch. C 62, 829–832. doi: 10.1515/znc-2007-11-1210
Ovais, M., Zia, N., Ahmad, I., Khalil, A. T., Raza, A., Ayaz, M., et al. (2018). Phyto-Therapeutic and Nanomedicinal Approach to Cure Alzheimer Disease: Present Status and Future Opportunities. Front. Aging Neurosci. 10:284. doi: 10.3389/fnagi.2018.00284
Pan, Y., Anthony, M., and Clarkson, T. B. (1999a). Effect of estradiol and soy phytoestrogens on choline acetyltransferase and nerve growth factor mRNAs in the frontal cortex and hippocampus of female rats. Proc. Soc. Exp. Biol. Med. 221, 118–125. doi: 10.3181/00379727-221-44393
Pan, Y., Anthony, M., and Clarkson, T. B. (1999b). Evidence for up-regulation of brain-derived neurotrophic factor mRNA by soy phytoestrogens in the frontal cortex of retired breeder female rats. Neurosci. Lett. 261, 17–20. doi: 10.1016/s0304-3940(98)00994-x
Park, S. S., Bae, I., and Lee, Y. J. (2008). Flavonoids-induced accumulation of hypoxia-inducible factor (HIF)-1α/2α is mediated through chelation of iron. J. Cell. Biochem. 103, 1989–1998. doi: 10.1002/jcb.21588
Park, L., Zhou, P., Pitstick, R., Capone, C., Anrather, J., Norris, E. H., et al. (2008). Nox2-derived radicals contribute to neurovascular and behavioral dysfunction in mice overexpressing the amyloid precursor protein. Proc. Natl. Acad. Sci. U S A 105, 1347–1352. doi: 10.1073/pnas.0711568105
Pasinetti, G. M., Ksiezak-Reding, H., Santa-Maria, I., Wang, J., and Ho, L. (2010). Development of a grape seed polyphenolic extract with anti-oligomeric activity as a novel treatment in progressive supranuclear palsy and other tauopathies. J. Neurochem. 114, 1557–1568. doi: 10.1111/j.1471-4159.2010.06875.x
Patisaul, H. B. (2017). Endocrine disruption by dietary phyto-oestrogens: impact on dimorphic sexual systems and behaviours. Proc. Nutr. Soc. 76, 130–144. doi: 10.1017/s0029665116000677
Qin, L., Zhang, J., and Qin, M. (2013). Protective effect of cyanidin 3-O-glucoside on β-amyloid peptide-induced cognitive impairment in rats. Neurosci. Lett. 534, 285–288. doi: 10.1016/j.neulet.2012.12.023
Resende, R., Moreira, P. I., Proença, T., Deshpande, A., Busciglio, J., Pereira, C., et al. (2008). Brain oxidative stress in a triple-transgenic mouse model of Alzheimer disease. Free Radic. Biol. Med. 44, 2051–2057. doi: 10.1016/j.freeradbiomed.2008.03.012
Rezai-Zadeh, K., Arendash, G. W., Hou, H., Fernandez, F., Jensen, M., Runfeldt, M., et al. (2008). Green tea epigallocatechin-3-gallate (EGCG) reduces β-amyloid mediated cognitive impairment and modulates tau pathology in Alzheimer transgenic mice. Brain Res. 1214, 177–187. doi: 10.1016/j.brainres.2008.02.107
Rezai-Zadeh, K., Douglas Shytle, R., Bai, Y., Tian, J., Hou, H., Mori, T., et al. (2009). Flavonoid-mediated presenilin-1 phosphorylation reduces Alzheimer’s disease β-amyloid production. J. Cell. Mol. Med. 13, 574–588. doi: 10.1111/j.1582-4934.2008.00344.x
Rezai-Zadeh, K., Shytle, D., Sun, N., Mori, T., Hou, H., Jeanniton, D., et al. (2005). Green tea epigallocatechin-3-gallate (EGCG) modulates amyloid precursor protein cleavage and reduces cerebral amyloidosis in Alzheimer transgenic mice. J. Neurosci. 25, 8807–8814. doi: 10.1523/JNEUROSCI.1521-05.2005
Reznichenko, L., Amit, T., Zheng, H., Avramovich-Tirosh, Y., Youdim, M. B. H., and Mandel, S. (2006). Reduction of iron regulated amyloid precursor protein and β-amyloid peptide by (−)-epigallocatechin 3 gallate in cell cultures: implications for iron chelation in Alzheimer’s disease. J. Neurochem. 97, 527–536. doi: 10.1111/j.1471-4159.2006.03770.x
Rice-Evans, C. A., and Packer, L. (2003). Flavonoids in Health and Disease. Boca Raton, FL: CRC Press.
Rinaldi, P., Polidori, M. C., Metastasio, A., Mariani, E., Mattioli, P., Cherubini, A., et al. (2003). Plasma antioxidants are similarly depleted in mild cognitive impairment and in Alzheimer’s disease. Neurobiol. Aging 24, 915–919. doi: 10.1016/s0197-4580(03)00031-9
Robak, J., and Gryglewski, R. J. (1988). Flavonoids are scavengers of superoxide anions. Biochem. Pharmacol. 37, 837–841. doi: 10.1016/0006-2952(88)90169-4
Sadiq, A., Mahmood, F., Ullah, F., Ayaz, M., Ahmad, S., Haq, F. U., et al. (2015). Synthesis, anticholinesterase and antioxidant potentials of ketoesters derivatives of succinimides: a possible role in the management of Alzheimer’s. Chem. Cent. J. 9:31. doi: 10.1186/s13065-015-0107-2
Schneider, L. J. (2001). Treatment of Alzheimer’s disease with cholinesterase inhibitors. Clin. Geriatr. Med. 17, 337–358. doi: 10.1016/S0749-0690(05)70072-0
Scholey, A. B., French, S. J., Morris, P. J., Kennedy, D. O., Milne, A. L., and Haskell, C. F. (2010). Consumption of cocoa flavanols results in acute improvements in mood and cognitive performance during sustained mental effort. J. Psychopharmacol. 24, 1505–1514. doi: 10.1177/0269881109106923
Schroeter, H., Bahia, P., Spencer, J. P., Sheppard, O., Rattray, M., Cadenas, E., et al. (2007). (−) Epicatechin stimulates ERK-dependent cyclic AMP response element activity and up-regulates GluR2 in cortical neurons. J. Neurochem. 101, 1596–1606. doi: 10.1111/j.1471-4159.2006.04434.x
Schroeter, H., Boyd, C., Spencer, J. P. E., Williams, R. J., Cadenas, E., and Rice-Evans, C. (2002). MAPK signaling in neurodegeneration: influences of flavonoids and of nitric oxide. Neurobiol. Aging 23, 861–880. doi: 10.1016/s0197-4580(02)00075-1
Schroeter, H., Spencer, J. P., Catherine, R.-E., and Williams, R. J. (2001). Flavonoids protect neurons from oxidized low-density-lipoprotein-induced apoptosis involving c-Jun N-terminal kinase (JNK), c-Jun and caspase-3. Biochem. J. 358, 547–557. doi: 10.1042/bj3580547
Shimmyo, Y., Kihara, T., Akaike, A., Niidome, T., and Sugimoto, H. (2008). Epigallocatechin-3-gallate and curcumin suppress amyloid β-induced β-site APP cleaving enzyme-1 upregulation. Neuroreport 19, 1329–1333. doi: 10.1097/wnr.0b013e32830b8ae1
Shukitt-Hale, B. (2012). Blueberries and neuronal aging. Gerontology 58, 518–523. doi: 10.1159/000341101
Shukitt-Hale, B., Cheng, V., and Joseph, J. A. (2009). Effects of blackberries on motor and cognitive function in aged rats. Nutr. Neurosci. 12, 135–140. doi: 10.1179/147683009x423292
Singh, M., Arseneault, M., Sanderson, T., Murthy, V., and Ramassamy, C. (2008). Challenges for research on polyphenols from foods in Alzheimer’s disease: bioavailability, metabolism, and cellular and molecular mechanisms. J. Agric. Food Chem. 56, 4855–4873. doi: 10.1021/jf0735073
Singh, R., and Pandey, V. (1990). Nivetin, a neoflavonoid from Echinops niveus. Phytochemistry 29, 680–681. doi: 10.1016/0031-9422(90)85148-9
Small, S. A., Chawla, M. K., Buonocore, M., Rapp, P. R., and Barnes, C. A. (2004). Imaging correlates of brain function in monkeys and rats isolates a hippocampal subregion differentially vulnerable to aging. Proc. Natl. Acad. Sci. U S A 101, 7181–7186. doi: 10.1073/pnas.0400285101
Socci, V., Tempesta, D., Desideri, G., De Gennaro, L., and Ferrara, M. (2017). Enhancing human cognition with cocoa flavonoids. Front. Nutr. 4:19. doi: 10.3389/fnut.2017.00019
Spencer, J. P. (2007). The interactions of flavonoids within neuronal signalling pathways. Genes Nutr. 2, 257–273. doi: 10.1007/s12263-007-0056-z
Spencer, J. P. (2008). Flavonoids: modulators of brain function? Br. J. Nutr. 99, ES60–ES77. doi: 10.1017/s0007114508965776
Spencer, J. P. E. (2009). Flavonoids and brain health: multiple effects underpinned by common mechanisms. Genes Nutr. 4, 243–250. doi: 10.1007/s12263-009-0136-3
Spencer, J. P. (2010a). Beyond antioxidants: the cellular and molecular interactions of flavonoids and how these underpin their actions on the brain. Proc. Nutr. Soc. 69, 244–260. doi: 10.1017/s0029665110000054
Spencer, J. P. (2010b). The impact of fruit flavonoids on memory and cognition. Br. J. Nutr. 104, S40–S47. doi: 10.1017/s0007114510003934
Spencer, J. P., Rice-Evans, C., and Williams, R. J. (2003). Modulation of pro-survival Akt/protein kinase B and ERK1/2 signaling cascades by quercetin and its in vivo metabolites underlie their action on neuronal viability. J. Biol. Chem. 278, 34783–34793. doi: 10.1074/jbc.m305063200
Spencer, J. P. E., Vauzour, D., and Rendeiro, C. (2009). Flavonoids and cognition: the molecular mechanisms underlying their behavioural effects. Arch. Biochem. Biophys. 492, 1–9. doi: 10.1016/j.abb.2009.10.003
Sun, X., and Sneden, A. T. (1999). Neoflavonoids from Polygonum perfoliatum. Planta Med. 65, 671–673. doi: 10.1055/s-2006-960846
Taniguchi, S., Suzuki, N., Masuda, M., Hisanaga, S.-I., Iwatsubo, T., Goedert, M., et al. (2005). Inhibition of heparin-induced tau filament formation by phenothiazines, polyphenols, and porphyrins. J. Biol. Chem. 280, 7614–7623. doi: 10.1074/jbc.m408714200
Ullah, F., Ayaz, M., Sadiq, A., Hussain, A., Ahmad, S., Imran, M., et al. (2016). Phenolic, flavonoid contents, anticholinesterase and antioxidant evaluation of Iris germanica var; florentina. Nat. Prod. Res. 30, 1440–1444. doi: 10.1080/14786419.2015.1057585
Uriarte-Pueyo, I., and Calvo, M. I. (2011). Flavonoids as acetylcholinesterase inhibitors. Curr. Med. Chem. 18, 5289–5302. doi: 10.2174/092986711798184325
Vafeiadou, K., Vauzour, D., Lee, H. Y., Rodriguez-Mateos, A., Williams, R. J., and Spencer, J. P. (2009). The citrus flavanone naringenin inhibits inflammatory signalling in glial cells and protects against neuroinflammatory injury. Arch. Biochem. Biophys. 484, 100–109. doi: 10.1016/j.abb.2009.01.016
van Praag, H., Lucero, M. J., Yeo, G. W., Stecker, K., Heivand, N., Zhao, C., et al. (2007). Plant-derived flavanol (−) epicatechin enhances angiogenesis and retention of spatial memory in mice. J. Neurosci. 27, 5869–5878. doi: 10.1523/JNEUROSCI.0914-07.2007
Vassar, R. (2014). BACE1 inhibitor drugs in clinical trials for Alzheimer’s disease. Alzheimers Res. Ther. 6:89. doi: 10.1186/s13195-014-0089-7
Vauzour, D., Vafeiadou, K., Rice-Evans, C., Williams, R. J., and Spencer, J. P. (2007a). Activation of pro-survival Akt and ERK1/2 signalling pathways underlie the anti-apoptotic effects of flavanones in cortical neurons. J. Neurochem. 103, 1355–1367. doi: 10.1111/j.1471-4159.2007.04841.x
Vauzour, D., Vafeiadou, K., and Spencer, J. P. (2007b). Inhibition of the formation of the neurotoxin 5-S-cysteinyl-dopamine by polyphenols. Biochem. Biophys. Res. Commun. 362, 340–346. doi: 10.1016/j.bbrc.2007.07.153
Vepsäläinen, S., Koivisto, H., Pekkarinen, E., Mäkinen, P., Dobson, G., McDougall, G. J., et al. (2013). Anthocyanin-enriched bilberry and blackcurrant extracts modulate amyloid precursor protein processing and alleviate behavioral abnormalities in the APP/PS1 mouse model of Alzheimer’s disease. J. Nutr. Biochem. 24, 360–370. doi: 10.1016/j.jnutbio.2012.07.006
Vlahos, C. J., Matter, W. F., Hui, K. Y., and Brown, R. F. (1994). A specific inhibitor of phosphatidylinositol 3-kinase, 2-(4-morpholinyl)-8-phenyl-4H-1-benzopyran-4-one (LY294002). J. Biol. Chem. 269, 5241–5248.
Voet, D., and Voet, J. G. (1995). Serine Proteases Biochemistry (p. 390). 2nd Edn. New York, NY: John Wiley & Sons.
Waltereit, R., Dammermann, B., Wulff, P., Scafidi, J., Staubli, U., Kauselmann, G., et al. (2001). Arg3. 1/Arc mRNA induction by Ca2+ and cAMP requires protein kinase A and mitogen-activated protein kinase/extracellular regulated kinase activation. J. Neurosci. 21, 5484–5493. doi: 10.1523/JNEUROSCI.21-15-05484.2001
Wang, J., Ho, L., Zhao, W., Ono, K., Rosensweig, C., Chen, L., et al. (2008). Grape-derived polyphenolics prevent Aβ oligomerization and attenuate cognitive deterioration in a mouse model of Alzheimer’s disease. J. Neurosci. 28, 6388–6392. doi: 10.1523/JNEUROSCI.0364-08.2008
Wang, D.-M., Li, S.-Q., Wu, W.-L., Zhu, X.-Y., Wang, Y., and Yuan, H.-Y. (2014). Effects of long-term treatment with quercetin on cognition and mitochondrial function in a mouse model of Alzheimer’s disease. Neurochem. Res. 39, 1533–1543. doi: 10.1007/s11064-014-1343-x
Wang, H.-J., and Murphy, P. A. (1994). Isoflavone content in commercial soybean foods. J. Agric. Food Chem. 42, 1666–1673. doi: 10.1021/jf00044a016
Wang, J., Santa-Maria, I., Ho, L., Ksiezak-Reding, H., Ono, K., Teplow, D. B., et al. (2010). Grape derived polyphenols attenuate tau neuropathology in a mouse model of Alzheimer’s disease. J. Alzheimers Dis. 22, 653–661. doi: 10.3233/jad-2010-101074
Wasco, W., Gurubhagavatula, S., Paradis, M. D., Romano, D. M., Sisodia, S. S., Hyman, B. T., et al. (1993). Isolation and characterization of APLP2 encoding a homologue of the Alzheimer’s associated amyloid β protein precursor. Nat. Genet. 5, 95–100. doi: 10.1038/ng0993-95
Weinreb, O., Amit, T., Mandel, S., and Youdim, M. B. (2009). Neuroprotective molecular mechanisms of (−)-epigallocatechin-3-gallate: a reflective outcome of its antioxidant, iron chelating and neuritogenic properties. Genes Nutr. 4, 283–296. doi: 10.1007/s12263-009-0143-4
Weinreb, O., Mandel, S., Amit, T., and Youdim, M. B. (2004). Neurological mechanisms of green tea polyphenols in Alzheimer’s and Parkinson’s diseases. J. Nutr. Biochem. 15, 506–516. doi: 10.1016/j.jnutbio.2004.05.002
Williams, C. M., El Mohsen, M. A., Vauzour, D., Rendeiro, C., Butler, L. T., Ellis, J. A., et al. (2008). Blueberry-induced changes in spatial working memory correlate with changes in hippocampal CREB phosphorylation and brain-derived neurotrophic factor (BDNF) levels. Free Radic. Biol. Med. 45, 295–305. doi: 10.1016/j.freeradbiomed.2008.04.008
Williams, R. J., and Spencer, J. P. (2012). Flavonoids, cognition, and dementia: actions, mechanisms and potential therapeutic utility for Alzheimer disease. Free Radic. Biol. Med. 52, 35–45. doi: 10.1016/j.freeradbiomed.2011.09.010
Williams, R. J., Spencer, J. P., and Rice-Evans, C. (2004). Flavonoids: antioxidants or signalling molecules? Adv. Exp. Med. Biol. 36, 838–849. doi: 10.1016/j.freeradbiomed.2004.01.001
Yevchak, A. M., Loeb, S. J., and Fick, D. M. (2008). Promoting cognitive health and vitality: a review of clinical implications. Geriatr. Nurs. 29, 302–310. doi: 10.1016/j.gerinurse.2007.10.017
Yin, Y., Edelman, G. M., and Vanderklish, P. W. (2002). The brain-derived neurotrophic factor enhances synthesis of Arc in synaptoneurosomes. Proc. Natl. Acad. Sci. U S A 99, 2368–2373. doi: 10.1073/pnas.042693699
Zhang, K., Lu, J., Mori, T., Smith-Powell, L., Synold, T. W., Chen, S., et al. (2010). Baicalin increases VEGF expression and angiogenesis by activating the ERRα/PGC-1α pathway. Cardiovasc. Res. 89, 426–435. doi: 10.1093/cvr/cvq296
Zhang, H.-Y., Yang, D.-P., and Tang, G.-Y. (2006). Multipotent antioxidants: from screening to design. Drug Discov Today 11, 749–754. doi: 10.1016/j.drudis.2006.06.007
Keywords: Alzheimer’s disease, polyphenols, amyloid beta, cholinesterases, antioxidant, signaling pathways and cognition
Citation: Ayaz M, Sadiq A, Junaid M, Ullah F, Ovais M, Ullah I, Ahmed J and Shahid M (2019) Flavonoids as Prospective Neuroprotectants and Their Therapeutic Propensity in Aging Associated Neurological Disorders. Front. Aging Neurosci. 11:155. doi: 10.3389/fnagi.2019.00155
Received: 05 June 2018; Accepted: 11 June 2019;
Published: 26 June 2019.
Edited by:
Nibaldo C. Inestrosa, Pontifical Catholic University of Chile, ChileReviewed by:
Denis Gris, Université de Sherbrooke, CanadaDong-Gyu Jo, Sungkyunkwan University, South Korea
Copyright © 2019 Ayaz, Sadiq, Junaid, Ullah, Ovais, Ullah, Ahmed and Shahid. This is an open-access article distributed under the terms of the Creative Commons Attribution License (CC BY). The use, distribution or reproduction in other forums is permitted, provided the original author(s) and the copyright owner(s) are credited and that the original publication in this journal is cited, in accordance with accepted academic practice. No use, distribution or reproduction is permitted which does not comply with these terms.
*Correspondence: Muhammad Ayaz, ayazuop@gmail.com