- 1Geriatric Division, Department of Medicine, University of Western Ontario, London, ON, Canada
- 2Falls, Balance and Injury Research Centre, Neuroscience Research Australia, University of New South Wales, Sydney, NSW, Australia
- 3Institute of Medical Physics, Friedrich–Alexander University Erlangen–Nürnberg, Erlangen, Germany
Impairments of balance predispose older people to falls. Some cognitive functions, especially executive functioning have been shown to affect balance and discriminate fallers from non-fallers. Mental flexibility is a component of the executive function and comprises multiple cognitive processes that work together to adjust the course of thoughts or actions according to the changing demands of a situation without the use of explicit instructions. However, the role of mental flexibility in balance in older people remains unclear. The study aim was to examine the relationship between mental flexibility and falls in a cohort of 212 older people (80.6 ± 4.9 years; 62% female). We hypothesized that: (i) participants with impaired balance would have worse mental flexibility compared to those with good balance; and (ii) poor mental flexibility would predict falls in the sub-group with impaired balance. Balance performance was assessed by measuring postural sway while standing on a medium density foam mat with eyes open for 30 s. Mental flexibility was assessed using a computerized short-form of the Wisconsin Card Sorting Test (WCST; 64 cards) with its sub-components comprising general performance, perseveration, failure-to-maintain set and conceptual ability. Falls were measured prospectively for 12-months using monthly calendars. MANCOVA revealed that WCST performance was associated with balance [Wilks’ Lambda = 0.883, F = 2.168; p = 0.013, partial eta squared () = 0.061] due primarily to reduced concept formation ability [F(2,207) = 5.787, p = 0.004, = 0.053]. Negative binomial regression analysis adjusting for age, education, contrast sensitivity, proprioception, inhibition, and inhibitory choice stepping reaction time (iCSRT) revealed that lower concept formation ability was predictive for falls [Incidence Rate Ratio 1.048 (95% confidence interval 1.016–1.081)]. Further, lower concept formation ability partly explained the association between balance and falls: i.e., fallers in the upper balance tertile had reduced concept formation performance whereas non-fallers had similar concept formation performance across the three balance tertiles. These findings suggest that poor mental flexibility affects the ability to maintain steady balance contributing to increased risk of falls in older people.
Introduction
There is growing evidence for interrelationships between balance and cognition in older people and their associations with falls (Schäfer et al., 2006; Yogev-Seligmann et al., 2008). Impaired balance has been consistently identified as a risk factor for falls in older people (Lord et al., 2007), with objective balance measures being good markers of fall risk that can be easily administered in clinical practice (Horak et al., 1989; Piirtola and Era, 2006; Sturnieks et al., 2011). It has been shown that standing balance requires cognitive resources (Teasdale and Simoneau, 2001; Horak, 2006), and older people use cognitive control to regulate sensorimotor processing during balance tasks (Redfern et al., 2001, 2004). Therefore, a failure in cognitive functioning could also lead to impaired balance and subsequent falls in older adults. Especially executive functions have been associated with increased fall risk in older people (Anstey et al., 2009; Hsu et al., 2012; Mirelman et al., 2012).
Executive functions comprise higher-order cognitive processes responsible for planning, controlling and coordinating complex cognitive tasks (Miyake et al., 2000), although no consensus definition has been agreed upon. Impaired executive functions, especially inhibition, have been identified as risk factors for falls in several studies. Mirelman et al. (2012) demonstrated that poor executive function, especially inadequate response inhibition, predicts falls in well-functioning older people, and Anstey et al. (2009) found subtle differences in inhibition are an early marker of increased fall risk, while multiple cognitive domains are impaired in recurrent fallers. We have also shown that slow inhibitory choice stepping reaction time (iCSRT), a test of combined inhibition and rapid step initiation, was predictive of falls in older people and that this association was not mediated by body sway, processing speed or attention (Schoene et al., 2017).
Mental flexibility is a component of executive functioning, but without an agreed definition as well (Ionescu, 2012). Here, it is defined as a construct composed of multiple cognitive processes that work together to adjust the course of thoughts or actions according to the changing demands of a situation without the use of explicit instructions (Berg, 1948). However, depending on definitions, cognitive processes including abstract reasoning, concept formation (understanding implicit task rules), inhibition of irrelevant information, task switching, set shifting and working memory (information updating) comprise the mental flexibility construct (Ionescu, 2012), functions that partially overlap. Mental flexibility is important to shift mental sets to adjust behaviors quickly, e.g., when (unpredictable) environmental demands require proactive and/or reactive responses to avoid a loss of balance. However, to our knowledge no study to date has investigated the effect of mental flexibility on balance and fall risk in older people.
In the above studies on executive functions and falls, relatively easy reaction time tasks for executive functions have been administered for which the task rules were clear to participants. For elucidating the importance of mental flexibility, i.e., abstract reasoning and concept formation for fall risk in older adults, more complex tasks are required for which participants have to implicitly learn the task rules. Previous studies have also not investigated the role of mental flexibility as a confounder or potential effect modifier in the association between balance control and falls in older people. Obtaining insights into these processes would increase our understanding of the complex inter-relationships between cognitive and motor functions and their impact on falls.
The objective of the current study, therefore, was to investigate the importance of more complex mental flexibility components to balance control and falls in older people and their role as potential confounders and moderators in the association between poor balance and falls. We hypothesized that: (i) participants with impaired balance would have worse mental flexibility compared to those with good balance; (ii) poor mental flexibility would predict falls in the sub-group with impaired balance.
Materials and Methods
This is a planned secondary analysis of a cohort study conducted between July and December 2012 on stepping, cognition and falls in 212 independently living older adults in Sydney, Australia (Schoene et al., 2017). The study was conducted according to the Declaration of Helsinki and was approved by the University of New South Wales Human Research Ethics Committee (HREC 11159). All participants gave written informed consent prior to data collection.
Participants
Participants were recruited via a research institute’s volunteer database and from residents of a retirement village in Sydney, Australia. The following inclusion criteria were applied: (1) aged 70 years and older; (2) ambulant with or without walking aids; and (3) able to step without assistance (step size 25–30 cm). People were excluded if they were cognitively impaired [diagnosis of neurodegenerative disease, Rapid Dementia Screening Test ≤4 (Kalbe et al., 2003)], had movement disorders (self-reported) or limiting lower limb pain and visual impairments that could not be corrected [>6/16 on a LogMAR visual acuity chart, color-blind]. Experienced research staff performed the screening, assigned participants to the study, conducted the assessments and informed participants about the follow-up procedures.
Balance Assessment
Sway path in millimeters was measured with a sway-meter that measured displacements of the body at the level of the waist. Testing was performed with participants standing on a medium density foam rubber mat (65 × 65 × 15 cm thick) with eyes open for 30 s (Lord et al., 2003). This device has demonstrated reliability and validity for measuring sway under multiple sensory conditions (Sturnieks et al., 2011). The sample was split into tertiles: good balance (62 to 146 mm, N = 71), fair balance (147 to 209 mm, N = 72), and poor balance (>210 mm, N = 69).
Wisconsin Card Sorting Test Short-Form (WCST)
Mental flexibility was assessed using the Wisconsin Card Sorting Test (WCST). This test measures implicit rule learning, abstract reasoning and set-shifting. In this test, participants matched stimulus cards according to a rule (color, shape, or number) without being informed of the rule. After successful understanding of the rule (10 correct matches), the rule is changed to enforce a shift in cognitive sets. After each trial, visual feedback was displayed for 500 ms. Four general executive processes are measured with the WCST: (1) General Performance: total correct responses; (2) Perseveration: Perseverative responses or perseverative errors (inability to use feedback from previous errors to produce correct behaviors – only perseverative responses that are also errors); (3) Failure to maintain set: the number of times the participant fails to make between five to nine correct responses in a row; (4) Conceptual Ability: number of trials needed to complete the first category (concept formation) and proportion (in %) of consecutive correct responses occurring in runs of three or more (conceptual level responses) – this ability measures how many trials a participant takes to understand the implicit task rule to switch to a new mental set (i.e., new rule) (Haaland et al., 1987). A WCST short form (64 trials) was computer-administered using a freely available and validated software tool [The Psychology Experiment Building Language (PEBL), version 0.141] (Piper et al., 2012; Fox et al., 2013).
Attentional Network Test (ANT)
The ANT combines cued reaction time and flanker tasks and requires participants to signal by button press whether a central arrow points left or right (Fan et al., 2002). The arrow appears above or below fixation and may or may not be accompanied by flankers which can emerge in congruent or incongruent form. Scores for three attentional networks are generated by measuring how response times are influenced by alerting cues, spatial cues, and flankers. The latter is used to assess the efficiency of the executive network (inhibitory control) and requires individuals to quickly suppress their prepotent response in order to resolve conflicts correctly by indicating the direction of congruent and incongruent flankers. It is calculated by subtracting the mean RT of all congruent flanking conditions, summed across cue types, from the mean RT of incongruent flanking conditions (Fan et al., 2002). We used this score as a measure of inhibition and to determine if measures of WCST measure a different component of executive functioning.
Inhibitory Choice Stepping Reaction Time (iCSRT)
The iCSRT is a development of the CSRT (Lord and Fitzpatrick, 2001; Schoene et al., 2011, 2017). Participants were asked to stand on the two stance panels. Stance and target panels were displayed on the screen. For “go” trials, participants were instructed to step onto a panel as quickly as possible when the corresponding arrow on the monitor changed color from white to green. For no-go trials (33% of trials), signaled by an arrow on the screen changing from white to purple, participants had to suppress the prepotent stepping response. Stimuli were presented for 100 ms and time between trials was randomized, occurring 0.5 to 1 s after returning both feet to stance panels. Immediate visual feedback of step completion was provided. After six practice trials (one stimulus for each target panel with two stimuli to be withheld), a random sequence of 36 trials was presented. For this secondary analysis we used the time in milliseconds for step RT (iCSRT-RT) as fall-related measure of executive functioning.
Additional Measures
Socio-demographic and medical information was collected by self-report questionnaires. The 12-item World Health Organization Disability Assessment Schedule (WHODAS) 2.0 was used as a generic assessment of health and disability; participants reported their level of impairment for several instrumental activities of daily living on a five-point Likert scale2. To record the number of comorbidities we used the Functional Comorbidity Index for which participants are required to report whether they had one or more of 18 chronic conditions and from which a sum score was calculated (Groll et al., 2005). Depressive symptoms were measured using the nine-item Patient Health Questionnaire (PHQ-9) (Kroenke et al., 2001). Concern about falling was measured by the iconographical falls-efficacy scale (Icon-FES), a questionnaire which depicts line drawings of a person undertaking a range of simple through to more demanding activities of daily living (Delbaere et al., 2011). Sum scores for the PHQ-9 and Icon-FES were calculated with high scores indicating increased depressive symptoms and concern about falling, respectively.
Sensorimotor performance was assessed as part of the Physiological Profile Assessment, comprising four tests in addition to postural sway (Lord et al., 2003):
Contrast sensitivity was assessed with the Melbourne Edge Test (Verbaken and Johnston, 1986), using a chart comprising of 20 circular test patches (25 mm diameter), with a series of edges of reducing contrast and of variable orientation;
Proprioception was measured with a lower limb matching task. Errors in degrees were recorded on a protractor inscribed on a vertical clear acrylic sheet (60 × 60 × 1 cm) placed between the legs;
Maximum isometric knee extension strength was measured in the dominant leg while seated with a knee angle of 90 degrees;
Simple reaction time was recorded using a randomly presented light stimulus and a finger-press as the response.
Falls
Falls were monitored prospectively over 12 months with daily falls calendars that were sent back monthly using pre-paid envelopes. If calendars were not returned, follow-up phone calls were made within 14 days of end of each month to remind participants to send back their calendars and to obtain additional information on falls. A fall was defined as “unintentionally coming to the ground, floor or lower level” (Lamb et al., 2005). People that experienced at least one fall in the follow-up period were classified as fallers.
Statistical Analysis
Missing data (less than 2%) were imputed using the Expectation Maximization method (Little’s missing completely at random test p > 0.05). Sample characteristics were compared using Analyses of Variance (ANOVAs) and chi squared tests. Based on previous study findings, showing that contrast sensitivity, proprioception, hand reaction time, and quadriceps strength were associated with balance control of older people while standing on a medium-density foam mat (Lord and Ward, 1994), balance performance was compared between groups applying Analysis of Covariance (ANCOVA) adjusting for these variables and other covariates we considered necessary to control for following comparison of demographic and sensorimotor data between the groups (Table 1). As sub-scores of the WCST are highly correlated (also observed in the current study – Supplementary Table S1), and are associated with both age and education level (Haaland et al., 1987; Barkley, 1997), multivariate analysis of covariance procedures (MANCOVAS) were performed to compare groups, with significant differences in Wilk’s Lambda used to determine whether mental flexibility was different between groups. Univariate ANOVAs were then applied to determine which variable of the WCST differed between groups using Bonferroni adjustments. (Partial) Eta squared (η2/) was calculated as effect size. Univariate and multiple negative binomial regression analyses (with individual follow-up time in months used as offset variable) were applied to determine the associations between balance as categorical variable (tertiles; good balance as reference category) and WCST and fall rate (number of falls; capped at 10 falls). Incidence rate ratios (IRR) with 95% confidence intervals were calculated. WCST performance was also investigated as a potential confounder in the association of balance and fall risk using a 10% change-in-estimate criterion as an indicator of a confounding effect (Budtz-Jorgensen et al., 2007). Potential effect modification by WCST performance was determined using interaction terms. The level of significance was set to 5%. Analyses were performed with SPSS for Windows (version 25, IBM).
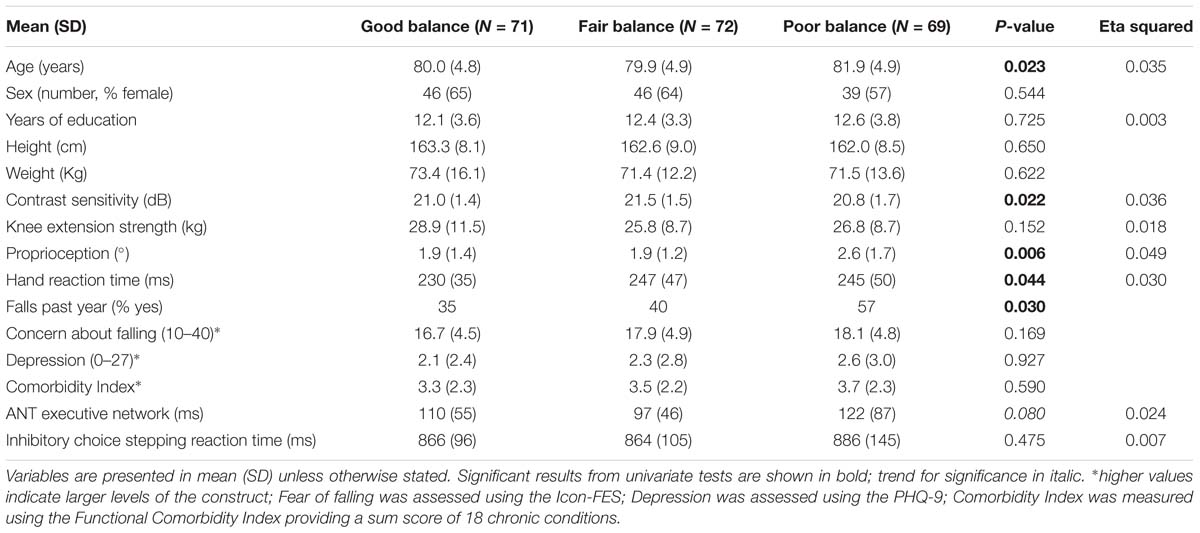
Table 1. Demographics, health, psychological, sensory, and fall risk profile of participants stratified by balance performance.
Results
Participant characteristics are displayed in Table 1. Consistent with previous findings, those with poor balance were older and performed worse in tests of contrast sensitivity, proprioception and hand reaction time than those with good balance (Lord and Ward, 1994). In contrast, knee extension strength did not differ among the balance groups in this study. A trend for significance was observed for the ability to resolve conflict (inhibition-ANT executive network), with individuals in the fair balance group being better than those in the poor balance group (p = 0.025).
Balance
As expected, sway paths differed among the three balance groups in univariate analysis [F(2,209) = 100.695, p < 0.001, η2 = 0.491]. After controlling for age, contrast sensitivity, proprioception, knee extension strength, simple reaction time and ANT performance, significant differences remained [F(2,203) = 80.861, p < 0.001, = 0.446] (Table 2), with Bonferroni post hoc tests showing linear differences between each tertile.
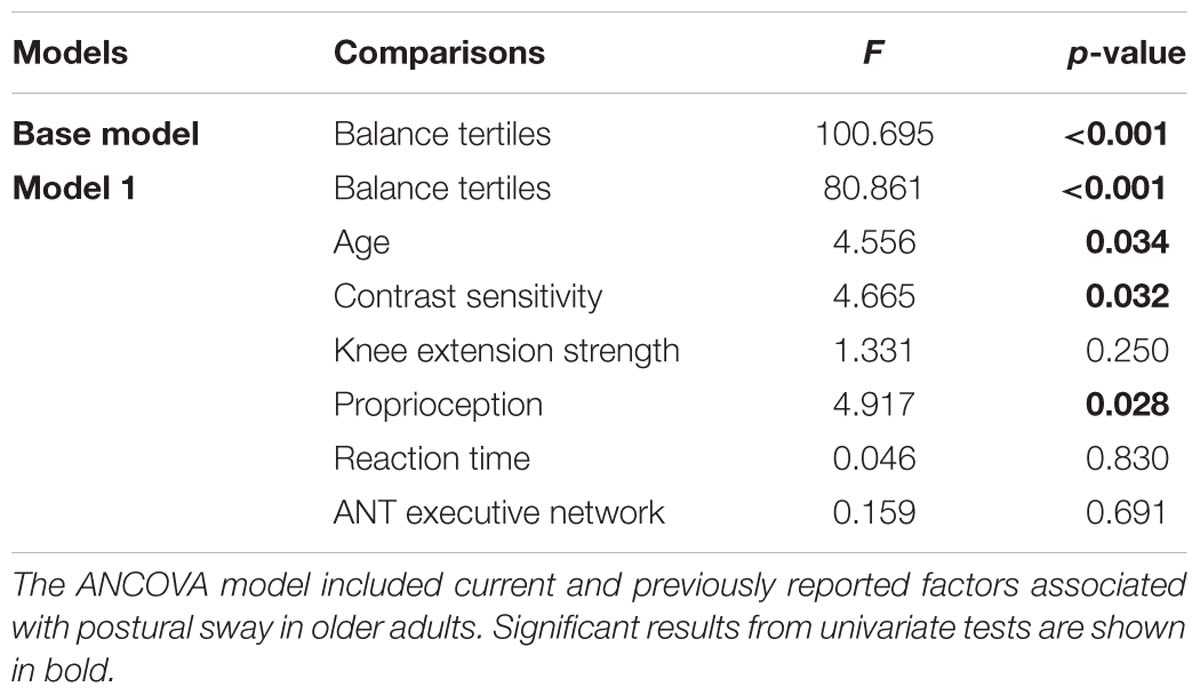
Table 2. Unadjusted (ANOVA) and adjusted (ANCOVA) analyses for the association between postural sway and balance tertiles.
Mental Flexibility
The MANCOVA, controlling for age and education, revealed the balance groups had different cognitive flexibility profiles [Wilks’ Lambda = 0.883, F = 2.168; p = 0.013, = 0.061]. Post hoc univariate ANOVAs revealed the difference lied in the number of trials required to complete the first category [concept formation, F(2,207) = 5.787, p = 0.004, = 0.053]. Bonferroni-adjusted pairwise comparisons showed the poor balance group required more trials than the good balance group (p = 0.003) (Table 3). Comparing upper and lower balance group tertiles, there was also a trend indicating the poor balance group gave fewer correct conceptual responses compared to the good balance group [44.7 vs. 47.3%, respectively, F(1,136) = 3.581, p = 0.05].
Falls
Two-hundred-seven participants (97.6%) completed the 12-month follow-up for falls. During the 2507 person-months of follow-up (mean 11.8 months), 93 participants (44%) experienced at least one fall. The proportion of fallers differed between groups, with 35% of the good balance group, 40% of the fair balance group, and 57% of the poor balance group reporting at least one fall. The mean number of falls for the good balance group was 0.65 (SD 1.17), for the fair balance group 1.01 (1.63) and for the poor balance group 1.74 (SD 3.92). Negative binomial regression analysis revealed that balance status (sway tertiles) was associated with an increased rate of falls. Using the good balance group as the reference, the fall rate for both, the fair and poor balance groups increased [IRR 1.91 (1.14–3.22), p = 0.015 and IRR 2.80 (1.68–4.65), p < 0.001, respectively].
Concept formation was also associated with an increased fall rate after adjusting for age and education [IRR 1.06 (1.03–1.09), p < 0.001]. With respect to the balance tertiles, concept formation predicted falls in the poor balance but not in the fair or good balance groups with the incidence rate in the opposite direction for the good balance group [poor balance: IRR 1.06 (1.02–1.09), p = 0.002; fair balance: IRR 1.06 (0.98–1.14), p = 0.170; good balance: IRR 0.89 (0.75–1.05), p = 0.172]. When entering both variables along with age, education in a multiple negative binomial regression model (enter method), balance status remained significant but was reduced by 13% for the fair balance group and by 24% for the poor balance group (Table 4). Adding contrast sensitivity and proprioception further reduced the effect of balance status with no change in the predictive value of concept formation (model 3, Table 4). This confounding was driven by notably poorer concept formation performance in the fallers and no corresponding reduction in the non-fallers within the poor balance group (Figure 1). Finally, when ANT executive network performance and iCSRT_RT were entered into the model, balance status (tertile 3), concept formation, contrast sensitivity and iCSRT_RT remained significant predictors of falls status (model 4, Table 4), with no evidence of an effect modification by balance status × concept formation (p = 0.250). ANT executive network performance was not associated with falls in this sample.
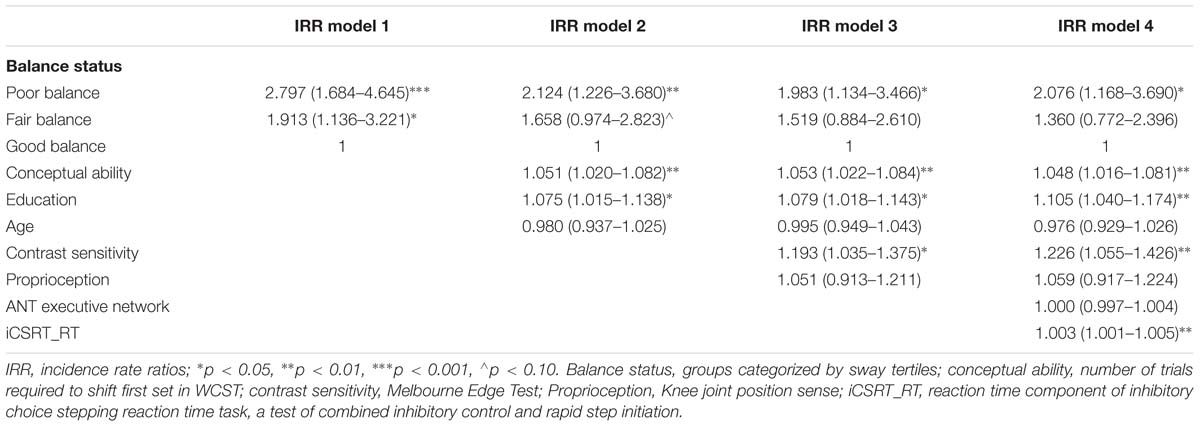
Table 4. Unadjusted and adjusted models for the association between balance status and fall rates with 95% confidence intervals.
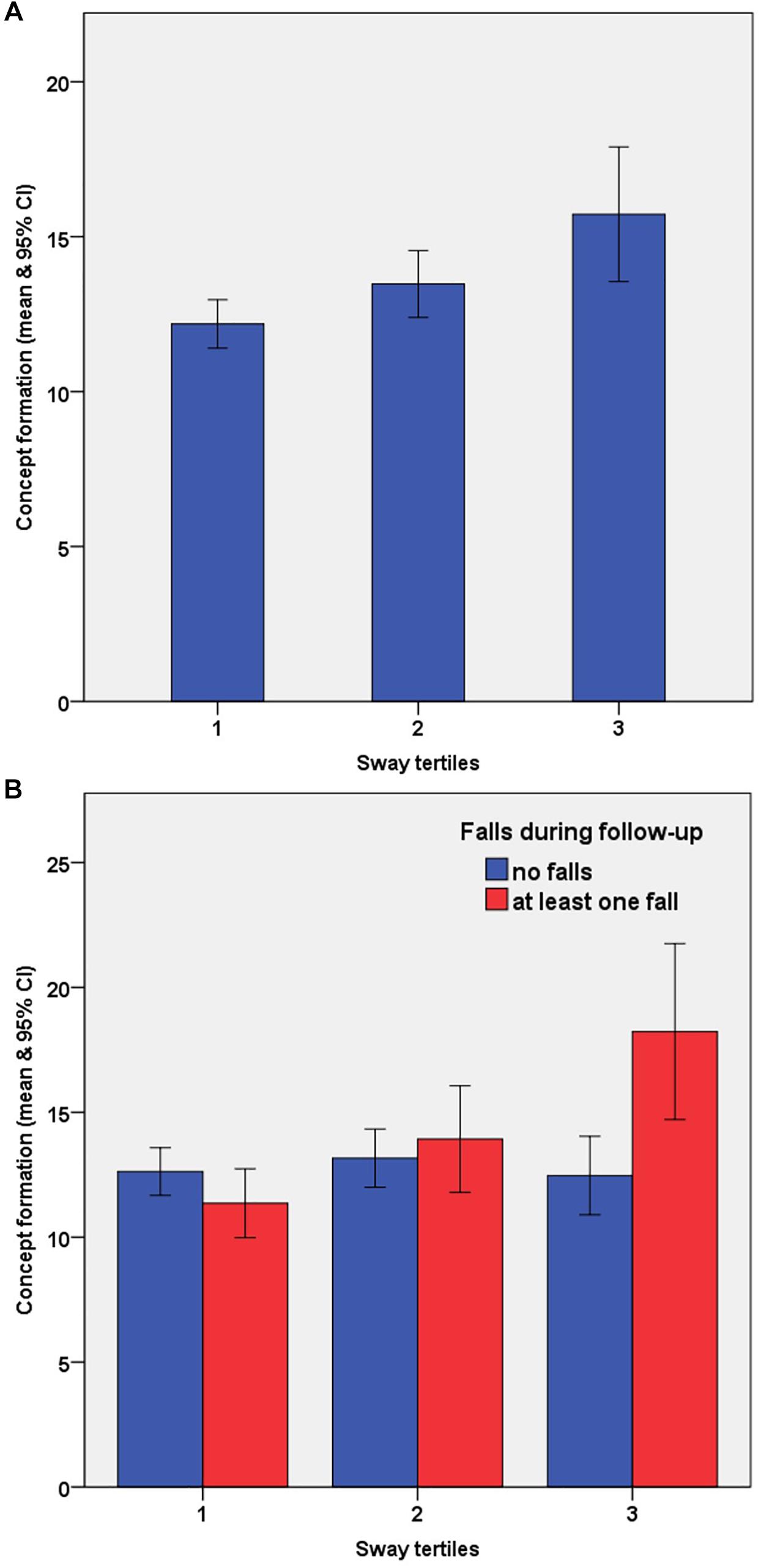
Figure 1. Concept formation ability as measured by the number of trials needed to shift first set during the short version of the Wisconsin Card Sorting Test (WCST) according to balance (sway tertiles) and faller status (one or more falls). Panel (A) shows a decline in concept formation performance across sway tertiles; Panel (B) shows the additional impact of faller status. Across sway tertiles the concept formation ability did not differ in non-fallers (blue). However, with decreasing balance status, fallers had greater difficulties to implicitly understand the first rule (red).
Discussion
This study found significant associations between cognitive functioning, balance and falls in older people without major cognitive impairments. Consistent with previous findings, poor balance was associated with an increased risk of falling (Piirtola and Era, 2006; Deandrea et al., 2010). Individuals with reduced balance control also performed worse in the computerized short version of the WCST, a test of mental flexibility, mainly due to poor concept formation. Specifically, the poor balance group required more than three additional trials to shift the first mental set demonstrating an impaired ability to implicitly learn a rule. Further analyses demonstrated that this conceptual ability impairment statistically confounded the association between balance status and falls. Compared to people with good balance, those with impaired balance were at increased risk of falling when their concept formation was also poor.
Learning rules of abstract concepts is not obviously related to causes of falling in everyday life. Confirming our second hypothesis, we found older people with impaired balance and poor mental flexibility were more likely to fall. Our findings may indicate fallers have poorer executive function in general, rather than specific mental flexibility deficits confirming results from cognitive and brain-imaging studies that demonstrate executive functions, including mental flexibility reflect some common, and distinct components (Miyake et al., 2000; Wager et al., 2005). It has been previously reported that older people are susceptible to falls if they perform poorly in inhibition tasks (Anstey et al., 2009; Mirelman et al., 2012; Schoene et al., 2017). It has also been demonstrated that poor concept formation is due to lack of inhibition, rather than reduced ability for abstraction (Hartman and Stratton-Salib, 2007). In the current study inhibition was assessed with the executive network of the Attentional Network Test, which requires participants to suppress their prepotent response in order to correctly indicate the direction of congruent in incongruent (conflict resolution) Flanker tasks (Fan et al., 2002). We found a trend that the performance in this task was associated with balance, indicating the relevance of inhibitory processes on balance control. This is in accordance to recent findings that demonstrate the importance of perceptual inhibition for sensory integration during standing balance tasks in older people (Redfern et al., 2018). However, we did not identify a meaningful contribution of this task to falls in older people. This may indicate compensatory processes in our relatively healthy sample without major cognitive impairments and sufficient physical function to do the tests involved in this study. Similarly, we have previously found that a stepping conflict resolution task could not discriminate faller groups whereas the iCSRT (response selection) could (Schoene et al., 2017). The rate of commission errors during the iCSRT task would have been a more genuine reflection of inhibition. However, response selection and response inhibition should not be separated as they are linked with each other in that first the automatic response is inhibited to enable a switch to the controlled response selection (Mostofsky and Simmonds, 2008). The WCST is a demonstrated test of executive function (Greve et al., 2005) and provides distinct information in neuropsychological assessments (Greve et al., 1998). Our finding that concept formation and iCSRT are significant and independent risk factors for falls, implies that more than one executive network influences fall risk. Importantly, this may indicate that both speed dependent (based on reaction time tasks) and non-speed dependent (based on implicit rule learning, without a reaction time component) executive functions are important in this regard.
The interrelationships between sensory, motor, and cognitive functions become stronger with increasing age (Schäfer et al., 2006), and balance and executive functions are inter-connected during standing tasks (Redfern et al., 2009; Taylor et al., 2017). Confirming our first hypothesis, we found that people in different balance tertiles also differed in their concept formation ability. This suggests that understanding an implicit rule may be a sensitive marker of impaired body sway. It is possible that individuals with better concept formation are more efficient in gathering sensory information that signal when corrections in the center of mass displacement are necessary. However, according to the benchmark values reported by Cohen (1988) this can be considered a small effect. This is not surprising as balance depends on multiple sensory, motor, and cognitive functions. Particularly, in individuals with poor balance, worse mental flexibility was associated with more falls, further confirming our second hypothesis. Relative to the good balance reference group, the confounding effect was larger in the poor balance compared to the fair balance group, demonstrating increased cognitive-motor interrelationships in older people with reduced levels of functioning.
In related research conducted with nursing home residents, more than 50% of falls were due to incorrect weight shifting and poor obstacle negotiation (Robinovitch et al., 2013). These postural challenges require the integration of feedback for correct modulation of motor responses and it has been reported age-related declines in WCST are associated with inefficient feedback utilization (Fristoe et al., 1997; Ashendorf and McCaffrey, 2008). This in turn, is mostly due to poor working memory and slow processing speed (Fristoe et al., 1997), which are cognitive domains associated with falls in older people (Anstey et al., 2009). Moreover, anticipation, sensory perception, and cognitive processing of potential balance threats require cognitive resources and this is particularly the case for older adults (Rankin et al., 2000; Bernard-Demanze et al., 2009). Situational awareness, the understanding of factors that contribute to optimal task performance in expected and unexpected conditions, also affects these factors (Caserta and Abrams, 2007), i.e., a quick appraisal of the individual-environment interaction facilitates protective postural responses (Melzer et al., 2001). Indeed, findings from the current study demonstrate that non-fallers react faster in a complex stepping task (Schoene et al., 2017). Implicit rule learning may be important for navigating through complex environments and older fallers may be too slow and/or too inaccurate to process the required information. Reduced mental flexibility may compromise the ability to use feedback from the body and environment to modulate center of mass displacement required to respond appropriately to internal or external changes.
Our findings may in part explain the poorer performance of balance-impaired people in motor-cognitive dual-task paradigms (Brauer et al., 2001; Plummer-D’Amato et al., 2011). Older people with poorer postural control need to prioritize their balance to avoid falling and thus cannot shift their attention to a secondary task as well as younger people or older adults with good balance (Shumway-Cook and Woollacott, 2000), a phenomenon that has been termed the “posture-first strategy.” While the number of trials required to shift the first set was a predictor for falls in the poor balance group, this was not so in the good balance group. In fact, the effect tended in the opposite direction, further supporting the idea of a protective effect of higher levels of mental flexibility on the risk of falling. Hence, it may be that preserved mental flexibility can compensate partially for reduced balance ability, but if both are impaired fall risk increases substantially. This finding is consistent with the study by Holtzer et al. (2012), who found executive function provided a protective effect against a decline in gait speed in older people with a higher cognitive reserve. Further studies could include real life simulations to examine how poor concept formation is related to the process of falling. Such work could incorporate stressors, such as complex individual-environment interactions and anxiety as these have been shown to impair mental flexibility (Hillier et al., 2006; Han et al., 2011) as well as balance-related protective factors.
Consistent with previous reports (Lord et al., 1991; Ivers et al., 1998; de Boer et al., 2004), we found contrast sensitivity was associated with falling. Impaired contrast sensitivity is also associated with reduced stride length in older people (Duggan et al., 2017) and increased body sway, especially under conditions of reduced proprioceptive feedback, such as when standing on compliant surfaces (Lord et al., 1991). Limited evidence indicates a relationship between contrast sensitivity and cognition. Risacher et al. (2013) found contrast sensitivity became progressively worse in older people classified into healthy control, subjective cognitive complaints, mild cognitive impairment, and Alzheimer’s disease groups. Further, Anstey et al. (2006) noted contrast sensitivity was significantly associated with cognitive performance in tasks of low attentional demand (processing speed) but not in tasks requiring executive control (e.g., working memory) after controlling for processing speed, supporting the (partially) independent contributions of executive function and sensory function on fall risk in older people.
Executive functions are amenable to improvements by cognitive training in older people without cognitive impairments (Kueider et al., 2012). Furthermore, exercise can improve cognitive functioning (Gregory et al., 2013) in addition to reducing fall risk (Sherrington et al., 2017). While most research has examined aerobic training (Ludyga et al., 2016), findings from one study suggest a positive effect of resistance training on cognitive outcomes in older adults (Liu-Ambrose et al., 2012). Seated cognitive training can also improve motor functions (Verghese et al., 2010; Milman et al., 2014; Smith-Ray et al., 2015), and videogames and virtual reality interventions with high attentional switching demands may contribute to improved mental flexibility (Canty et al., 2014; Cardoso-Leite and Bavelier, 2014), as well as balance control in older adults (Schoene et al., 2014). Our findings suggest the incorporation of implicit learning tasks in interventions for older people may be beneficial to improve balance and reduce fall risk. For instance, in a cognitive-motor intervention using gamified virtual reality environments, individuals could be exposed to changing and complex, unforeseen (daily life) scenarios, during which they have to extract implicit information to anticipate and avoid postural threats in order to accrue points.
We need to acknowledge some limitations. First, participants were able to step without assistance, had no major cognitive impairments and good visual acuity. Therefore, results may not be generalizable to frailer populations. Second, all tests were done in one single session and it cannot be ruled out that fatigue played a role. However, whenever required or wished individualized breaks were taken in between tests.
Conclusion
We found older people with impaired balance had poorer mental flexibility, particularly concept formation, compared to those with good balance. Further, impaired concept formation confounded the association between balance status and falls. Our study confirms previous findings that balance is associated with higher order cognitive functions and shows older people with both impaired balance and reduced mental flexibility are at a substantially increased risk of falling. Training interventions targeting the preservation or improvement of mental flexibility may help prevent falls in this population by improving postural stability as well as cognition.
Ethics Statement
The study was conducted according to the Declaration of Helsinki and was approved by the University of New South Wales Human Research Ethics Committee. All participants gave written informed consent prior to data collection.
Author Contributions
DS and SL designed the study and acquired the data. BT, DS, FP-F, SL, and WK analyzed the data. All authors interpreted the results and drafted the manuscript.
Funding
This work was supported by an International Postgraduate Research Scholarship (DS) and a NHMRC Senior Principal Research Fellowship (SL). The funders had no role in study design, data collection and analysis, decision to publish, or preparation of the manuscript.
Conflict of Interest Statement
SL acknowledges the physiological profile assessment (NeuRA FallScreen) is commercially available through Neuroscience Research Australia.
The remaining authors declare that the research was conducted in the absence of any commercial or financial relationships that could be construed as a potential conflict of interest.
Supplementary Material
The Supplementary Material for this article can be found online at: https://www.frontiersin.org/articles/10.3389/fnagi.2019.00133/full#supplementary-material
Footnotes
References
Anstey, K. J., Butterworth, P., Borzycki, M., and Andrews, S. (2006). Between- and within-individual effects of visual contrast sensitivity on perceptual matching, processing speed, and associative memory in older adults. Gerontology 52, 124–130. doi: 10.1159/000090958
Anstey, K. J., Wood, J., Kerr, G., Caldwell, H., and Lord, S. R. (2009). Different cognitive profiles for single compared with recurrent fallers without dementia. Neuropsychology 23, 500–508. doi: 10.1037/a0015389
Ashendorf, L., and McCaffrey, R. J. (2008). Exploring age-related decline on the Wisconsin Card Sorting Test. Clin. Neuropsychol. 22, 262–272. doi: 10.1080/13854040701218436
Barkley, R. A. (1997). Behavioral inhibition, sustained attention, and executive functions: constructing a unifying theory of ADHD. Psychol. Bull. 121, 65–94. doi: 10.1037/0033-2909.121.1.65
Berg, E. A. (1948). A simple objective technique for measuring flexibility in thinking. J. Gen. Psychol. 39, 15–22. doi: 10.1080/00221309.1948.9918159
Bernard-Demanze, L., Dumitrescu, M., Jimeno, P., Borel, L., and Lacour, M. (2009). Age-related changes in posture control are differentially affected by postural and cognitive task complexity. Curr. Aging Sci. 2, 139–149.
Brauer, S. G., Woollacott, M., and Shumway-Cook, A. (2001). The interacting effects of cognitive demand and recovery of postural stability in balance-impaired elderly persons. J. Gerontol. A Biol. Sci. Med. Sci. 56, M489–M496.
Budtz-Jorgensen, E., Keiding, N., Grandjean, P., and Weihe, P. (2007). Confounder selection in environmental epidemiology: assessment of health effects of prenatal mercury exposure. Ann. Epidemiol. 17, 27–35. doi: 10.1016/j.annepidem.2006.05.007
Canty, A. L., Fleming, J., Patterson, F., Green, H. J., Man, D., and Shum, D. H. (2014). Evaluation of a virtual reality prospective memory task for use with individuals with severe traumatic brain injury. Neuropsychol. Rehabil. 24,238–265. doi: 10.1080/09602011.2014.881746
Cardoso-Leite, P., and Bavelier, D. (2014). Video game play, attention, and learning: how to shape the development of attention and influence learning? Curr. Opin. Neurol. 27, 185–191. doi: 10.1097/WCO.0000000000000077
Caserta, R. J., and Abrams, L. (2007). The relevance of situation awareness in older adults’ cognitive functioning: a review. Eur. Rev. Aging Phys. Act. 4, 3–13. doi: 10.1007/s11556-007-0018-x
de Boer, M. R., Pluijm, S. M., Lips, P., Moll, A. C., Volker-Dieben, H. J., Deeg, D. J., et al. (2004). Different aspects of visual impairment as risk factors for falls and fractures in older men and women. J. Bone Miner. Res. 19, 1539–1547. doi: 10.1359/jbmr.040504
Deandrea, S., Lucenteforte, E., Bravi, F., Foschi, R., La Vecchia, C., and Negri, E. (2010). Risk factors for falls in community-dwelling older people: a systematic review and meta-analysis. Epidemiology 21, 658–668. doi: 10.1097/EDE.0b013e3181e89905
Delbaere, K., Smith, S. T., and Lord, S. R. (2011). Development and initial validation of the Iconographical Falls Efficacy Scale. J. Gerontol. A Biol. Sci. Med. Sci. 66, 674–680. doi: 10.1093/gerona/glr019
Duggan, E., Donoghue, O., Kenny, R. A., Cronin, H., Loughman, J., and Finucane, C. (2017). Time to refocus assessment of vision in older adults? Contrast sensitivity but not visual acuity is associated with gait in older adults. J. Gerontol. A Biol. Sci. Med. Sci.72, 1663–1668. doi: 10.1093/gerona/glx021
Fan, J., Mccandliss, B. D., Sommer, T., Raz, A., and Posner, M. I. (2002). Testing the efficiency and independence of attentional networks. J. Cogn. Neurosci. 14, 340–347. doi: 10.1162/089892902317361886
Fox, C. J., Mueller, S. T., Gray, H. M., Raber, J., and Piper, B. J. (2013). Evaluation of a short-form of the Berg Card Sorting Test. PLoS One 8:e63885. doi: 10.1371/journal.pone.0063885
Fristoe, N. M., Salthouse, T. A., and Woodard, J. L. (1997). Examination of age-related deficits on the Wisconsin Card Sorting Test. Neuropsychology 11, 428–436. doi: 10.1037//0894-4105.11.3.428
Gregory, M. A., Gill, D. P., and Petrella, R. J. (2013). Brain health and exercise in older adults. Curr. Sports Med. Rep. 12, 256–271.
Greve, K. W., Ingram, F., and Bianchini, K. J. (1998). Latent structure of the Wisconsin Card Sorting Test in a clinical sample. Arch. Clin. Neuropsychol. 13, 597–609. doi: 10.1016/s0887-6177(97)00075-9
Greve, K. W., Stickle, T. R., Love, J. M., Bianchini, K. J., and Stanford, M. S. (2005). Latent structure of the Wisconsin Card Sorting Test: a confirmatory factor analytic study. Arch. Clin. Neuropsychol. 20, 355–364. doi: 10.1016/j.acn.2004.09.004
Groll, D. L., To, T., Bombardier, C., and Wright, J. G. (2005). The development of a comorbidity index with physical function as the outcome. J. Clin. Epidemiol. 58, 595–602. doi: 10.1016/j.jclinepi.2004.10.018
Haaland, K. Y., Vranes, L. F., Goodwin, J. S., and Garry, P. J. (1987). Wisconsin Card Sort Test performance in a healthy elderly population. J. Gerontol. 42, 345–346. doi: 10.1093/geronj/42.3.345
Han, D. H., Park, H. W., Kee, B. S., Na, C., Na, D. H., and Zaichkowsky, L. (2011). Performance enhancement with low stress and anxiety modulated by cognitive flexibility. Psychiatry Investig. 8, 221–226. doi: 10.4306/pi.2011.8.3.221
Hartman, M., and Stratton-Salib, B. C. (2007). Age differences in concept formation. J. Clin. Exp. Neuropsychol. 29, 198–214. doi: 10.1080/13803390600630294
Hillier, A., Alexander, J. K., and Beversdorf, D. Q. (2006). The effect of auditory stressors on cognitive flexibility. Neurocase 12, 228–231. doi: 10.1080/13554790600878887
Holtzer, R., Wang, C., Lipton, R., and Verghese, J. (2012). The protective effects of executive functions and episodic memory on gait speed decline in aging defined in the context of cognitive reserve. J. Am. Geriatr. Soc. 60, 2093–2098. doi: 10.1111/j.1532-5415.2012.04193.x
Horak, F. B. (2006). Postural orientation and equilibrium: what do we need to know about neural control of balance to prevent falls? Age Ageing 35(Suppl. 2), ii7–ii11. doi: 10.1093/ageing/afl077
Horak, F. B., Shupert, C. L., and Mirka, A. (1989). Components of postural dyscontrol in the elderly: a review. Neurobiol. Aging 10, 727–738. doi: 10.1016/0197-4580(89)90010-9
Hsu, C. L., Nagamatsu, L. S., Davis, J. C., and Liu-Ambrose, T. (2012). Examining the relationship between specific cognitive processes and falls risk in older adults: a systematic review. Osteoporos. Int. 23, 2409–2424. doi: 10.1007/s00198-012-1992-z
Ionescu, T. (2012). Exploring the nature of cognitive flexibility. New Ideas Psychol. 30, 190–200. doi: 10.1016/j.newideapsych.2011.11.001
Ivers, R. Q., Cumming, R. G., Mitchell, P., and Attebo, K. (1998). Visual impairment and falls in older adults: the Blue Mountains Eye Study. J. Am. Geriatr. Soc. 46, 58–64. doi: 10.1111/j.1532-5415.1998.tb01014.x
Kalbe, E., Calabrese, P., Schwalen, S., and Kessler, J. (2003). The Rapid Dementia Screening Test (RDST): a new economical tool for detecting possible patients with dementia. Dement. Geriatr. Cogn. Disord. 16, 193–199. doi: 10.1159/000072802
Kroenke, K., Spitzer, R. L., and Williams, J. B. (2001). The PHQ-9: validity of a brief depression severity measure. J. Gen. Intern. Med. 16, 606–613. doi: 10.1046/j.1525-1497.2001.016009606.x
Kueider, A. M., Parisi, J. M., Gross, A. L., and Rebok, G. W. (2012). Computerized cognitive training with older adults: a systematic review. PLoS One 7:e40588. doi: 10.1371/journal.pone.0040588
Lamb, S. E., Jorstad-Stein, E. C., Hauer, K., and Becker, C. (2005). Development of a common outcome data set for fall injury prevention trials: the Prevention of Falls Network Europe consensus. J. Am. Geriatr. Soc. 53, 1618–1622. doi: 10.1111/j.1532-5415.2005.53455.x
Liu-Ambrose, T., Nagamatsu, L. S., Voss, M. W., Khan, K. M., and Handy, T. C. (2012). Resistance training and functional plasticity of the aging brain: a 12-month randomized controlled trial. Neurobiol. Aging 33, 1690–1698. doi: 10.1016/j.neurobiolaging.2011.05.010
Lord, S. R., Clark, R. D., and Webster, I. W. (1991). Visual acuity and contrast sensitivity in relation to falls in an elderly population. Age Ageing 20, 175–181. doi: 10.1093/ageing/20.3.175
Lord, S. R., and Fitzpatrick, R. C. (2001). Choice stepping reaction time: a composite measure of falls risk in older people. J. Gerontol. A Biol. Sci. Med. Sci. 56, M627–M632.
Lord, S. R., Menz, H. B., and Tiedemann, A. (2003). A physiological profile approach to falls risk assessment and prevention. Phys. Ther. 83, 237–252.
Lord, S. R., Sherrington, C., Menz, H. B., and Close, J. C. T. (2007). Falls in Older People: Risk Factors and Strategies for Prevention. New York, NY: Cambridge University Press.
Lord, S. R., and Ward, J. A. (1994). Age-associated differences in sensori-motor function and balance in community dwelling women. Age Ageing 23, 452–460. doi: 10.1093/ageing/23.6.452
Ludyga, S., Gerber, M., Brand, S., Holsboer-Trachsler, E., and Puhse, U. (2016). Acute effects of moderate aerobic exercise on specific aspects of executive function in different age and fitness groups: a meta-analysis. Psychophysiology 53, 1611–1626. doi: 10.1111/psyp.12736
Melzer, I., Benjuya, N., and Kaplanski, J. (2001). Age-related changes of postural control: effect of cognitive tasks. Gerontology 47, 189–194. doi: 10.1159/000052797
Milman, U., Atias, H., Weiss, A., Mirelman, A., and Hausdorff, J. M. (2014). Can cognitive remediation improve mobility in patients with Parkinson’s disease? Findings from a 12 week pilot study. J. Parkinsons Dis. 4, 37–44. doi: 10.3233/JPD-130321
Mirelman, A., Herman, T., Brozgol, M., Dorfman, M., Sprecher, E., Schweiger, A., et al. (2012). Executive function and falls in older adults: new findings from a five-year prospective study link fall risk to cognition. PLoS One 7:e40297. doi: 10.1371/journal.pone.0040297
Miyake, A., Friedman, N. P., Emerson, M. J., Witzki, A. H., Howerter, A., and Wager, T. D. (2000). The unity and diversity of executive functions and their contributions to complex “Frontal Lobe” tasks: a latent variable analysis. Cogn. Psychol. 41, 49–100. doi: 10.1006/cogp.1999.0734
Mostofsky, S. H., and Simmonds, D. J. (2008). Response inhibition and response selection: two sides of the same coin. J. Cogn. Neurosci. 20, 751–761. doi: 10.1162/jocn.2008.20500
Piirtola, M., and Era, P. (2006). Force platform measurements as predictors of falls among older people - a review. Gerontology 52, 1–16. doi: 10.1159/000089820
Piper, B. J., Li, V., Eiwaz, M. A., Kobel, Y. V., Benice, T. S., Chu, A. M., et al. (2012). Executive function on the psychology experiment building language tests. Behav. Res. Methods 44, 110–123. doi: 10.3758/s13428-011-0096-6
Plummer-D’Amato, P., Altmann, L. J., and Reilly, K. (2011). Dual-task effects of spontaneous speech and executive function on gait in aging: exaggerated effects in slow walkers. Gait Posture 33, 233–237. doi: 10.1016/j.gaitpost.2010.11.011
Rankin, J. K., Woollacott, M. H., Shumway-Cook, A., and Brown, L. A. (2000). Cognitive influence on postural stability: a neuromuscular analysis in young and older adults. J. Gerontol. A Biol. Sci. Med. Sci. 55, M112–M119.
Redfern, M. S., Chambers, A. J., Sparto, P. J., Furman, J. M., and Jennings, J. R. (2018). Perceptual inhibition associated with sensory integration for balance in older adults. Dement. Geriatr. Cogn. Disord. 46, 266–274. doi: 10.1159/000493748
Redfern, M. S., Jennings, J. R., Martin, C., and Furman, J. M. (2001). Attention influences sensory integration for postural control in older adults. Gait Posture 14, 211–216. doi: 10.1016/s0966-6362(01)00144-8
Redfern, M. S., Jennings, J. R., Mendelson, D., and Nebes, R. D. (2009). Perceptual inhibition is associated with sensory integration in standing postural control among older adults. J. Gerontol. B Psychol. Sci. Soc. Sci. 64, 569–576. doi: 10.1093/geronb/gbp060
Redfern, M. S., Talkowski, M. E., Jennings, J. R., and Furman, J. M. (2004). Cognitive influences in postural control of patients with unilateral vestibular loss. Gait Posture 19, 105–114. doi: 10.1016/s0966-6362(03)00032-8
Risacher, S. L., Wudunn, D., Pepin, S. M., Magee, T. R., Mcdonald, B. C., Flashman, L. A., et al. (2013). Visual contrast sensitivity in Alzheimer’s disease, mild cognitive impairment, and older adults with cognitive complaints. Neurobiol. Aging 34, 1133–1144. doi: 10.1016/j.neurobiolaging.2012.08.007
Robinovitch, S. N., Feldman, F., Yang, Y., Schonnop, R., Leung, P. M., Sarraf, T., et al. (2013). Video capture of the circumstances of falls in elderly people residing in long-term care: an observational study. Lancet 381, 47–54. doi: 10.1016/S0140-6736(12)61263-X
Schäfer, S., Huxhold, O., and Lindenberger, U. (2006). Healthy mind in healthy body? A review of sensorimotor–cognitive interdependencies in old age. Eur. Rev. Aging Phys. Act. 3, 45–54. doi: 10.1007/s11556-006-0007-5
Schoene, D., Delbaere, K., and Lord, S. R. (2017). Impaired response selection during stepping predicts falls in older people-a cohort study. J. Am. Med. Dir. Assoc. 18, 719–725. doi: 10.1016/j.jamda.2017.03.010
Schoene, D., Lord, S. R., Verhoef, P., and Smith, S. T. (2011). A novel video game–based device for measuring stepping performance and fall risk in older people. Arch. Phys. Med. Rehabil. 92, 947–953. doi: 10.1016/j.apmr.2011.01.012
Schoene, D., Valenzuela, T., Lord, S. R., and De Bruin, E. D. (2014). The effect of interactive cognitive-motor training in reducing fall risk in older people: a systematic review. BMC Geriatr. 14:107. doi: 10.1186/1471-2318-14-107
Sherrington, C., Michaleff, Z. A., Fairhall, N., Paul, S. S., Tiedemann, A., Whitney, J., et al. (2017). Exercise to prevent falls in older adults: an updated systematic review and meta-analysis. Br. J. Sports Med. 51, 1750–1758. doi: 10.1136/bjsports-2016-096547
Shumway-Cook, A., and Woollacott, M. (2000). Attentional demands and postural control: the effect of sensory context. J Gerontol. A Biol. Sci. Med. Sci. 55, M10–M16.
Smith-Ray, R. L., Hughes, S. L., Prohaska, T. R., Little, D. M., Jurivich, D. A., and Hedeker, D. (2015). Impact of cognitive training on balance and gait in older adults. J. Gerontol. B Psychol. Sci. Soc. Sci. 70, 357–366. doi: 10.1093/geronb/gbt097
Sturnieks, D. L., Arnold, R., and Lord, S. R. (2011). Validity and reliability of the Swaymeter device for measuring postural sway. BMC Geriatr. 11:63. doi: 10.1186/1471-2318-11-63
Taylor, M. E., Lord, S. R., Delbaere, K., Kurrle, S. E., Mikolaizak, A. S., and Close, J. C. T. (2017). Reaction time and postural sway modify the effect of executive function on risk of falls in older people with mild to moderate cognitive impairment. Am. J. Geriatr. Psychiatry 25, 397–406. doi: 10.1016/j.jagp.2016.10.010
Teasdale, N., and Simoneau, M. (2001). Attentional demands for postural control: the effects of aging and sensory reintegration. Gait Posture 14, 203–210. doi: 10.1016/s0966-6362(01)00134-5
Verbaken, J. H., and Johnston, A. W. (1986). Population norms for edge contrast sensitivity. Am. J. Optom. Physiol. Opt. 63, 724–732. doi: 10.1097/00006324-198609000-00005
Verghese, J., Mahoney, J., Ambrose, A. F., Wang, C., and Holtzer, R. (2010). Effect of cognitive remediation on gait in sedentary seniors. J. Gerontol. A Biol. Sci. Med. Sci. 65, 1338–1343. doi: 10.1093/gerona/glq127
Wager, T. D., Sylvester, C. Y., Lacey, S. C., Nee, D. E., Franklin, M., and Jonides, J. (2005). Common and unique components of response inhibition revealed by fMRI. Neuroimage 27, 323–340. doi: 10.1016/j.neuroimage.2005.01.054
Keywords: accidental falls, aged, balance, cognition, executive functions, mental flexibility
Citation: Pieruccini-Faria F, Lord SR, Toson B, Kemmler W and Schoene D (2019) Mental Flexibility Influences the Association Between Poor Balance and Falls in Older People – A Secondary Analysis. Front. Aging Neurosci. 11:133. doi: 10.3389/fnagi.2019.00133
Received: 09 January 2019; Accepted: 20 May 2019;
Published: 12 June 2019.
Edited by:
Eling D. de Bruin, Karolinska Institute (KI), SwedenReviewed by:
Patrick Eggenberger, Swiss Federal Laboratories for Materials Science and Technology, SwitzerlandTulika Nandi, University of Southern California, United States
Oron Levin, KU Leuven, Belgium
Copyright © 2019 Pieruccini-Faria, Lord, Toson, Kemmler and Schoene. This is an open-access article distributed under the terms of the Creative Commons Attribution License (CC BY). The use, distribution or reproduction in other forums is permitted, provided the original author(s) and the copyright owner(s) are credited and that the original publication in this journal is cited, in accordance with accepted academic practice. No use, distribution or reproduction is permitted which does not comply with these terms.
*Correspondence: Daniel Schoene, ZGFuaWVsLnNjaG9lbmVAZmF1LmRl