- 1Department of Physiology, College of Korean Medicine, Kyung Hee University, Seoul, South Korea
- 2Inist ST Co. Ltd., Seongnam-si, South Korea
Parkinson’s disease (PD) is a chronic progressive neurodegenerative movement disorder characterized by the selective loss of dopaminergic neurons within the substantia nigra (SN). While the precise etiology of dopaminergic neuronal demise is elusive, multiple lines of evidence indicate that neuroinflammation is involved in the pathogenesis of PD. We have previously demonstrated that subcutaneous administration of bee venom (BV) phospholipase A2 (bvPLA2) suppresses dopaminergic neuronal cell death in a PD mouse model. In the present study, we established standardized methods for producing bvPLA2 agent isolated from crude BV at good manufacturing practice (GMP) facility. The therapeutic efficacy of purified bvPLA2 agent was examined in MPTP-induced PD mice. Importantly, administration of purified bvPLA2 in a dose-dependent manner reversed motor deficits in PD mice as well as inhibited loss of dopaminergic neurons within the SN of PD mice. The concentration-dependent action of standardized bvPLA2 appeared to be related to the induction of CD4+CD25+Foxp3+ regulatory T cells (Tregs), which, in part, inhibits T helper 1 (Th1) and Th17 polarization and suppresses microglial activation in PD mice. Taken together, these results suggest that standardized bvPLA2 purified from BV shows a neuroprotective effect against PD and thus has a potential target for treatment of PD.
Introduction
Parkinson’s disease (PD) is known as the second most common neurodegenerative disease to affect about 3% of the population over the age of 65 (Lang and Lozano, 1998; de Lau et al., 2004). It is generally characterized by the selective loss of dopaminergic neurons as significant neuropathological hallmarks (Bertram and Tanzi, 2005). Until recently, several animal models have been developed to discover PD pathogenesis as well as search for neuroprotective therapeutic targets (Wang et al., 2015). Among all of these models, MPTP model has been widely used to initiate PD in animal models by penetrating blood-brain barrier (BBB) and destroying dopaminergic neurons in the substantia nigra (SN; Sakaguchi et al., 2008).
While the etiology of PD is unclear, a considerable body of research suggests that inflammatory responses play an important role in the development and progression of PD (Tufekci et al., 2012). Emerging evidence indicates the enhanced inflammatory responses, infiltration of T cells into the brain and glial cell activation are prominent features of PD (Wang et al., 2015). Recently, significant efforts recently have been focused on developing novel anti-inflammatory agents for PD treatment (Samii et al., 2009).
Bee venom (BV) consists of a complex mixture of peptides, enzymes, lipids and bioactive amines. Accumulating evidence suggests a wide range of pharmaceutical properties of BV. Accordingly, BV therapy has been developed to treat various diseases, including inflammatory diseases (Lee et al., 2014) and neurodegenerative diseases (Hossen et al., 2017).
One of the major components of BV is phospholipase A2 (PLA2), comprising approximately 10%–12% of the dry weight of the venom in the European honeybee, Apis mellifera (Habermann, 1972). The PLA2 derived from BV (bvPLA2) belongs to group III secretory PLA2 (sPLA2) that has been implicated in diverse cellular responses, such as signal transduction, host defense, blood coagulation, and pain relief (Hossen et al., 2016). Interestingly, several lines of evidence have indicated the therapeutic effect of bvPLA2 in neurodegenerative diseases, including prion disease (Jeong et al., 2011). Consistent with this finding, we previously reported the neuroprotective effect of bvPLA2 against neurodegenerative diseases including Alzheimer’s disease (Ye et al., 2016) and PD (Chung et al., 2015). The cellular action of bvPLA2 appeared to mainly suppress immune responses via stimulation of dendritic cells, ultimately enhancing the function of regulatory T cells which play an essential role in maintaining immune tolerance (Sakaguchi et al., 2008).
It appears that generating clinical-grade and sterile pharmaceutical products from BV is challenging, mostly related to identification, isolation, and purification of bioactive components from BV (Ameratunga et al., 1995; Lee et al., 2014). In the present study, we developed an effective strategy for therapeutic components based on bvPLA2 which were isolated and purified from BV at good manufacturing practice (GMP) facility. To evaluate the translational relevance, we tested the neuroprotective effects of the standardized bvPLA2 in MPTP-induced mouse model of PD. Additionally, dose-dependent effect of bvPLA2 isolated from BV on MPTP-induced PD mice was investigated to determine an optimal dose. Thus, the present study may shed new light on developing new therapeutic targets for PD to provide a basis for standardization and GMP of bvPLA2 drug.
Materials and Methods
Animals
All experiments were performed in accordance with the approved animal protocols and guidelines established by Kyung Hee University. Briefly, 7- to 8-week-old male C57BL/6J mice were purchased from The Jackson Laboratory (Bar Harbor, ME, USA). All mice were maintained under pathogen-free conditions on a 12-h light/dark cycle and temperature-controlled conditions, with food and water ad libitum.
BvPLA2 Isolation, Preparation, Manufacturing, and Quality Management
A standardized BV PLA2 was prepared by Inist St Co. Limited (Eumseong-gun, South Korea). For isolation and purification, raw BV was purchased from Bee Venom Lab LLC (Tbilisi, GA, USA) and dissolved in high performance liquid chromatography (HPLC) grade water at a concentration of 1 mg/ml. Then the diluted samples were applied to PTFE membrane filter (pore size 0.45 μm; Sigma-Aldrich, St Louis, MO, USA). To reduce the volume for the subsequent steps, the filtered mixtures were further concentrated by a tangential flow filtration (TFF) system, fitted with Pellicon 3 devices with Ultracel-10kDa membrane (Merck Millipore, Billerica, MA, USA). For manufacturing, the purified bvPLA2 was dried with freeze-drying and was collected as a white powder. The bvPLA2 content was determined using HPLC system and then diluted to a concentration of 0.1 mg/ml. Undesired substances including allergen were removed by membrane filters (pore size 0.22 μm PVDF sterile membrane filter; Jet Bio-Filtration Co., Ltd, Guangzhou, China). The separation and detection were carried out on reversed-phase (RP)-HPLC system on a C18 column (pore size: 180 Å; Sigma-Aldrich, St Louis, MO, USA) using a Waters 2695 liquid chromatograph and a Waters 2489 UV-visible detector (Waters Corporation, Milford, MA, USA). The sample was chromatographed at 25°C at a flow rate of 2 ml/min. The elution was performed with a linear gradient of 0%–80% acetonitrile in 0.1% trifluoroacetic acid (TFA; Sigma-Aldrich, St Louis, MO, USA) and the elution profile was monitored at 220 nm. The area of the peak detected was used to measure the recovery of bvPLA2 and the separation profiles of purified bvPLA2 were compared with those of commercial standard bvPLA2 (Sigma-Aldrich, St Louis, MO, USA). The bioactivity of purified bvPLA2 was compared with inactive mutated recombinant bvPLA2-H34A (Lee et al., Manuscript submitted for publication). In order to measure bioactivity of purified bvPLA2, PLA2 activity was measured with EnzCheck PLA2 Assay Kit (Invitrogen, Carlsbad, CA, USA) according to the supplier’s instructions. All these procedures were carried out at an aseptic GMP facility. For quality management, the purity test was performed to ensure that there was no detectable heavy metals, insoluble particulate matter, endotoxins, or microbes. A quality control using endotoxin assays was performed by Charles River Laboratories Korea (Incheon, South Korea). Certificate of Analysis, which specifies the pyrogenicity of the endotoxin assessed by kinetic turbidimetric assay, was supplied. The commercial standard endotoxin was dissolved and diluted with Limulus amebocyte lysate (LAL) reagent water (LRW) and tris aminomethane buffer in order to determine the endotoxin level of purified standard bvPLA2. Changes in the bvPLA2 content were examined for 3 months in stability test. Hence, based on these observations, the purified bvPLA2 appeared to be appropriate as standardized bvPLA2.
MPTP-Induced PD Mouse Model
1-Methyl-4-phenyl-1,2,3,6-tetrahydropyridine (MPTP; 20 mg/kg; Sigma-Aldrich, St Louis, MO, USA) was intraperitoneally (i.p.) administered to mice four times a day at 2 h intervals, inducing severe and persistent depletions of dopamine as previously described (Jackson-Lewis and Przedborski, 2007). During the experiment, mice were monitored for their physical condition and weight loss. The mortality rate of mice after MPTP injection was 0%–30% in each group and the animals surviving with <20% weight loss were included in the analysis.
BvPLA2 Treatment
One day after the last MPTP injection, MPTP-injected mice were received with either bvPLA2 or phosphate buffered saline (PBS). For administration, bvPLA2 was dissolved in PBS and administered by once daily subcutaneous (s.c.) injections for six consecutive days in the concentration range of 0.01–0.5 mg/kg. Mice treated with commercial standard bvPLA2 (Sigma-Aldrich, St Louis, MO, USA) by s.c. injection at a dose of 0.5 mg/kg for 6 days was used to compare the effect of purified bvPLA2.
Immunohistochemistry
Immunohistochemical analysis was carried out, as previously described with minor modifications (Chung et al., 2015). Briefly, the tissues were incubated with the relevant primary antibody [tyrosine hydroxylase (TH; Mukhopadhyay and Stahl, 1995), Iba1 (1:2,000, Wako Pure Chemic Industries, Osaka, Japan), and ED1 (1:500, Serotec, Oxford, UK)] at 4°C overnight. Then, the tissues were incubated with biotinylated goat anti-secondary antibody (1:200, Vector Laboratories, Burlingame, CA, USA) and horseradish peroxidase (HRP)-conjugated streptavidin-biotin complex (Vectastain Elite ABC kit; Vector Laboratories) and visualized with diaminobenzidine (DAB). The stained cells were analyzed under a bright field microscope (Nikon, Tokyo, Japan).
Unbiased Stereological Estimation
Unbiased stereological estimation of the total number of TH-, ED1-, or H&E-positive cells was made using an optical fractionator as described previously, with minor modifications (West, 1993). In brief, the sections used for counting covered the entire SN from the rostral tip of the SN pars compacta (SNpc) to the caudal end of the SN pars reticulate (SNr). The counting was carried out using the Olympus CAST-Grid system (Olympus, Ballerup, Denmark). The counting frame was placed randomly on the first counting area and moved systematically over all counting areas until the entire delineated area was sampled. The total number of cells was calculated according to the optical fractionator equation (West et al., 1991).
Flow Cytometry
Flow cytometric detection of regulatory T cells was performed using fluorescein isothiocyanate (FITC)-conjugated anti-mouse CD4 (clone GK1.5; eBioscience, San Diego, CA, USA), phycoerythrin (PE)-conjugated anti-mouse CD25 (clone PC61.5; eBioscience, San Diego, CA, USA), and Alexa Fluor 647 anti-mouse Foxp3 (clone MF23; BD Pharmingen™, San Jose, CA, USA). The single-cell splenocytes were washed with PBS and stained with FITC-conjugated anti-CD4 and PE-labeled anti-CD25 antibodies in staining buffer. The cells were subsequently fixed and stained with Alexa Fluor 647 anti-Foxp3 antibody overnight at 4°C in the dark. After washing, the cells were stored at 4°C in the dark for subsequent detection.
Enzyme-Linked Immunosorbent Assay (ELISA)
To assess CD4+ T helper (Th) cell subsets from splenocytes, we measured cytokine production by enzyme-linked immunosorbent assay (ELISA). First, the splenocytes at a concentration of 1 × 107 cells/ml were prepared from mice. Then, splenic CD4+ T cells were isolated by positive selection using anti-CD4 (L3T4) MicroBeads (Miltenyi Biotec, Bergisch Gladbach, Germany) according to the manufacturer’s instructions. The purified splenic CD4+ T cells were stimulated for 12 h with 50 ng/ml of phorbol myristate acetate (Sigma-Aldrich, St Louis, MO, USA) and 1,000 ng/ml of ionomycin (Sigma-Aldrich, St Louis, MO, USA). The supernatants were taken and the levels of IFN-γ, IL-4, and IL-17A were measured from these supernatants by ELISA kits (BD Biosciences, San Jose, CA, USA) according to the supplier’s instructions.
Pole Test
The degree of bradykinesia of the mouse was measured by the pole test with a slight modification of a previous protocol (Ogawa et al., 1985). Briefly, a tube of ~50 cm in length and ~1 cm in diameter was wrapped in gauze and a wooden pole was attached to the top. The time at which the mice turned completely downward and the total time to climb down the pole were measured with a cut-off limit of 30 s. Each mouse was given five trials, and the average of the best three measurements was used as the result. Trials, where the mouse jumped or slid down the pole, were excluded.
Statistical Analysis
Statistical analysis was performed using GraphPad Prism (v5.0; GraphPad) software. Each data was compared between conditions using unpaired T-test or one-way analysis of variance (ANOVA), or Kruskal-Wallis test followed by post hoc group comparisons. Data are expressed as the means ± standard error of the mean (SEM); P < 0.05 was considered significant.
Results
Standardization of the Manufacturing Process of Bee Venom PLA2
In the present study, we developed standardized methods for preparing bvPLA2 from active components of European honeybee, Apis mellifera (Figure 1A). To purify the crude BV, the extract was ultra-filtrated and subsequently concentrated to increase the yield of bvPLA2, as well as to remove undesirable products. Purified standard bvPLA2 were identified and separated using RP-HPLC system with a C18 column (Figure 1B). Commercial standard bvPLA2 was also used to identify the separation profiles of purified bvPLA2 and determine the content of purified of bvPLA2. Additionally, the bioactivity test was carried out to test specific PLA2 activity (Figures 1C,D). To ensure that purified bvPLA2 was safe, we performed the quality control tests, resulting in that it had an allowable endotoxin level approved by US Food and Drug Administration (FDA; Table 1). Therefore, based on these observations, we considered this purified bvPLA2 as properly manufactured and standardized agent.
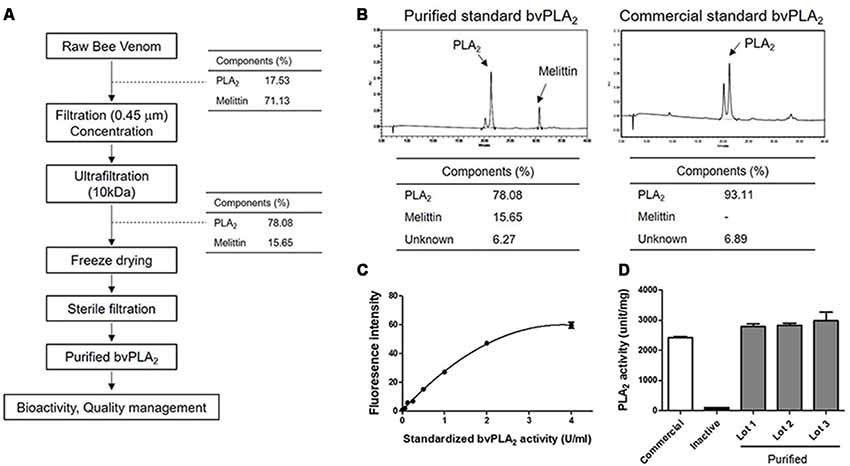
Figure 1. Developing methods for preparing bee venom phospholipase A2 (bvPLA2) standard reagent from BV. (A) Purification and manufacturing process of PLA2 from raw BV at a good manufacturing practice (GMP) facility. (B) Identification and determination of the purity of purified and commercial standard bvPLA2. (C) Standard curve of purified standard bvPLA2. (D) The activity of PLA2 from purified bvPLA2 isolated from BV (Lot 1–3), inactive recombinant mutant bvPLA2 (bvPLA2-H34A), and commercial bvPLA2. Combined results were plotted from at least two independent experiments. The data are expressed as the means ± standard error of the mean (SEM).
BvPLA2 Improves Motor Activity in a Dose-Dependent Manner in MPTP-Induced PD Mice
We first examined whether purified standard bvPLA2 protected behavioral deficits in PD mice. As shown in Figure 2A, purified bvPLA2 was given to MPTP-treated mice at a dose of 0.01, 0.1, or 0.5 mg/kg for six consecutive days, beginning 1 day after the last MPTP injection. On day 6 after MPTP treatment, MPTP-challenged mice took much longer than the control mice to turn downward (Figure 2B) and to descend the pole (Figure 2C), indicating basal ganglia-related movement disorders in MPTP-treated mice. Treatment of purified bvPLA2 significantly shortened the time to turn and to down in MPTP-treated mice. However, inactive mutant bvPLA2 (H34A) treatment induced no significant difference compared with MPTP-treated mice. No significant differences were detected between purified standard bvPLA2 group and commercial standard bvPLA2 group treated with the same dose (0.5 mg/kg). As observed, administration of purified standard bvPLA2 improved motor deficits induced by MPTP in a concentration-dependent manner.
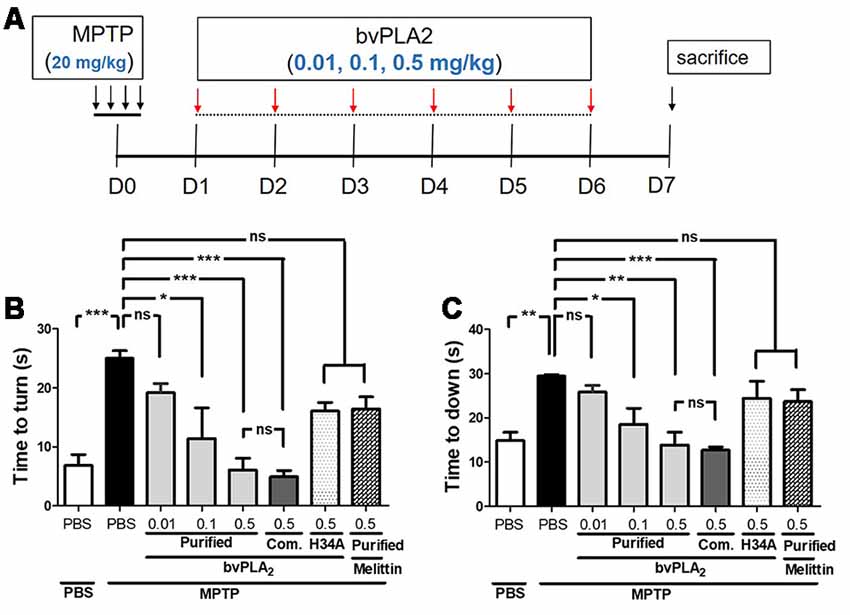
Figure 2. Dose-dependent neuroprotection of purified standard bvPLA2 on motor deficits in MPTP-injected mice. (A) Standard bvPLA2 purified from BV (0.01, 0.1, or 0.5 mg/kg), commercial standard bvPLA2 (Com. bvPLA2; 0.5 mg/kg), inactive recombinant mutant bvPLA2 (bvPLA2-H34A; 0.5 mg/kg), or standard melittin purified from BV (0.5 mg/kg) was administered to the mice for 6 days, beginning 1 day after MPTP injection. (B,C) The motor ability of mice was evaluated on a pole test on day 6 post-MPTP. Time to orient downward (B) and total time to descend (C) were measured. The data are expressed as the means ± SEM, n = 5–8 per group; *p < 0.05, **p < 0.01, ***p < 0.001, ns, not significant.
BvPLA2 Is a Regulator of Peripheral Regulatory T Cell Differentiation
Growing body of evidence supported the role of regulatory T cells in the disease progression of PD both in human PD patients (Chen et al., 2015) and animal models of PD (Reynolds et al., 2010). Here, we speculated that the action of purified standard bvPLA2 on improving sensorimotor function in PD mice may be related to the induction of Tregs in the periphery.
To address our hypothesis, we first measured the cellular proportions of Tregs in MPTP mice after treatment with different doses of bvPLA2 (Figure 3). In fact, no significant difference in CD4+CD25+Foxp3+ Treg populations was detected between control and MPTP mice on 7 days after MPTP challenge (Figure 3B). Importantly, administration of bvPLA2 at a concentration of 0.1 and 0.5 mg/kg induced an increase in the number of splenic Tregs on MPTP-treated mice, when compared with mice treated with MPTP only. Whereas lower doses of bvPLA2 (0.01 mg/kg) induced no significant differences in the population of Treg cells. Similarly, commercial bvPLA2 (0.5 mg/kg) stimulated Treg induction in the periphery with no significant difference compared with purified standard bvPLA2 (0.5 mg/kg). All these findings support the notion that bvPLA2 induces the expansion of CD4+CD25+Foxp3+ Treg in the periphery in PD, which can suppress the inflammatory response.
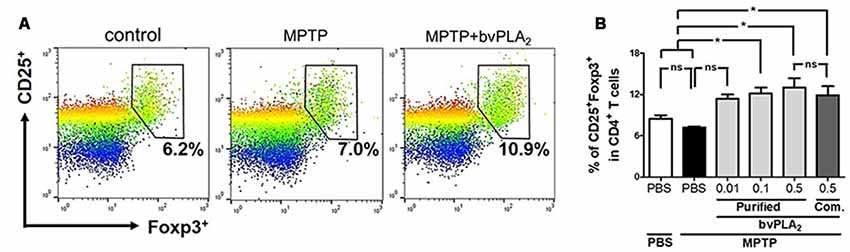
Figure 3. Dose-dependent effect of purified standard bvPLA2 on inducing differentiation of Treg cells in MPTP-treated mice. (A,B) On day 7 after MPTP treatment, CD4+CD25+Foxp3+ regulatory T cells in splenocytes were analyzed by flow cytometry. The percentage of CD25+Foxp3+ cells in CD4+ T cells were assessed in MPTP-treated mice with bvPLA2 treatment (purified bvPLA2: 0.01, 0.1, 0.5 mg/kg; commercial (Com.) bvPLA2: 0.5 mg/kg) for consecutive 6 days after 1 day of MPTP challenge. Combined results from two independent experiments with at least three mice per group were plotted. MPTP+bvPLA2 group: standard bvPLA2, purified from crude BV, was injected from Day 1 to Day 6 post-MPTP at a concentration of 0.5 mg/kg. The data are expressed as the means ± SEM; *p < 0.05, **p < 0.01, ***p < 0.001, ns, not significant.
BvPLA2 Inhibits the Expansion of Th1 and Th17 Effector Cells That Are Associated With PD
Since the balance of CD4+ T cell subsets is highly correlated with disease activity in PD (Chen et al., 2015), we further explored whether T helper subset balance was altered in PD. As shown in Figure 4, we assessed the differentiation of T helper (Th) cells based on their cytokine signature: IFN-γ-secreting Th1, IL-4-secreting Th2 and IL-17A-producing Th17 cells (Zhu et al., 2010). Obviously, MPTP treatment increased IFN-γ-secreting Th1 cells, but not IL-4-secreting Th2, indicating a shifted Th1/Th2 balance towards Th1 in PD mice (Figure 4). Additionally, we asked whether purified standard bvPLA2 could reverse the altered balance of Th subsets in PD mice. Impressively, bvPLA2 treatment significantly reduced the secretion of the two pro-inflammatory cytokines IFN-γ and IL-17A in a dose-dependent fashion (Figure 4). Commercial bvPLA2 injection (0.5 mg/kg) also exhibited a similar suppressive effect on Th1- and Th17-polarizing cytokines associated with PD. These results showed that specific CD4+ T subsets, including Th1 and Th17 cells, were markedly differentiated in PD induced by MPTP. Impressively, purified standard bvPLA2 in a dose-dependent manner suppressed MPTP-mediated imbalance of CD4+ T cell subsets.
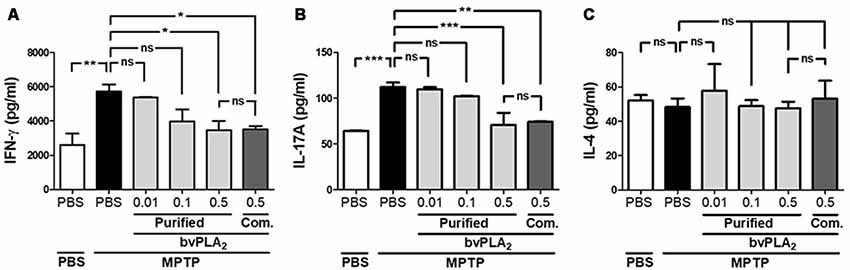
Figure 4. Dose-dependent inhibitory effect of purified standard bvPLA2 on Parkinson’s disease (PD)-related Th1 and Th17 polarization. Th1, Th2, and Th17-polarizing conditions were analyzed by enzyme-linked immunosorbent assay (ELISA) of Th1 cytokine (IFN-γ; A), Th2 cytokine (IL-4; C), and Th17 cytokine (IL-17A; B) from the supernatants of splenic CD4+ T cells in MPTP-injected mice treated with purified standard bvPLA2 (0.01, 0.1, or 0.5 mg/kg) or commercial standard bvPLA2 (Com. bvPLA2; 0.5 mg/kg). The data are expressed as the means ± SEM, n = 3–5 per group; *p < 0.05, **p < 0.01, ***p < 0.001, ns, not significant.
BvPLA2 Protects Dopaminergic Neurons Against MPTP Neurotoxicity in a Concentration-Dependent Manner
We further evaluated whether purified standard bvPLA2 exhibited a dose-dependent neuroprotection against neuronal degeneration of PD. Expectedly, the immunohistochemistry data revealed apoptotic cell death in H&E stained and loss of dopaminergic neurons in TH stained sections of MPTP-challenged mice (Figure 5). In contrast, there was a dramatic neuronal protection in SN of MPTP mice following bvPLA2 treatment in a dose-dependent manner. When administered at a comparative lower dose (0.01 mg/kg), bvPLA2 administration did not significantly rescue MPTP-induced neuronal loss. Similar results were observed in MPTP mice given with commercial bvPLA2 at a dose of 0.5 mg/kg. These data indicate that purified bvPLA2 effectively attenuated the loss of dopaminergic neurons associated with PD in a concentration-dependent way.
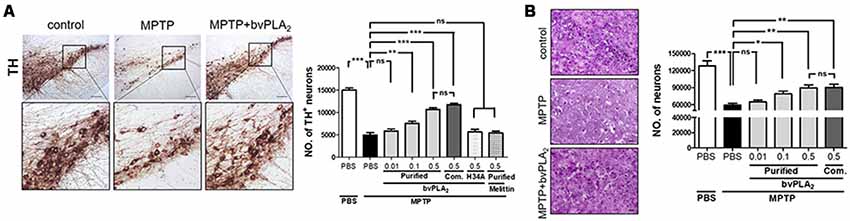
Figure 5. Dose-dependent suppressive effect of purified standard bvPLA2 on neuronal death of MPTP-challenged mice. Immunohistochemical staining for tyrosine hydroxylase (TH; A) or H&E (B) was carried out on MPTP mice with the treatment of standard bvPLA2 purified from crude BV (0.01, 0.1, or 0.5 mg/kg) or commercial standard bvPLA2 (Com. bvPLA2; 0.5 mg/kg) on day 7 post-MPTP. Representative images of immunohistochemical staining for TH-positive or H&E stained cells in substantia nigra (SN) are shown. The number of stained cells was quantified by using an unbiased stereology. High-magnification images of boxed regions on TH-stained sections are also shown. MPTP+bvPLA2 group: standard bvPLA2, purified from crude BV, was injected from Day 1 to Day 6 post-MPTP at a concentration of 0.5 mg/kg. The data are expressed as the means ± SEM. n = 4–8 per group; *p < 0.05, **p < 0.01, ***p < 0.001, ns, not significant. Scale bar: 100 μm (A); 50 μm (B).
BvPLA2 Suppresses Microglial Activation Caused by MPTP in Mice in a Dose-Dependent Manner
Mounting evidence has demonstrated that microglial activation may play a key role in amplifying the neuroinflammatory response, which can exacerbate dopaminergic neuronal death (Kim and Joh, 2006). Hence, we examined the expression levels of microglia markers, such as Iba1 and ED1, to explore whether purified standard bvPLA2 could directly suppress the activation of microglia. As shown in Figure 6A, the level of Iba1-positive microglia was markedly increased in the SN of MPTP mice at 7 days after MPTP post-injection, whereas bvPLA2 administration clearly reduced the expression of Iba1 in PD mice a concentration-dependent manner. Similarly, MPTP caused a significant increase in ED1-positive neuronal cells compared with the control group, while administration of purified bvPLA2 markedly reduced the number of ED1+ microglia in the brains of MPTP-challenged mice (Figures 6B,C). The effect of commercial standard bvPLA2 (0.5 mg/kg) appeared to be similar to that of 0.5 mg/kg of purified bvPLA2, showing no difference between the two groups. All these findings support the notion that purified bvPLA2 inhibits microglial activation in MPTP-treated mice in a dose-dependent manner.
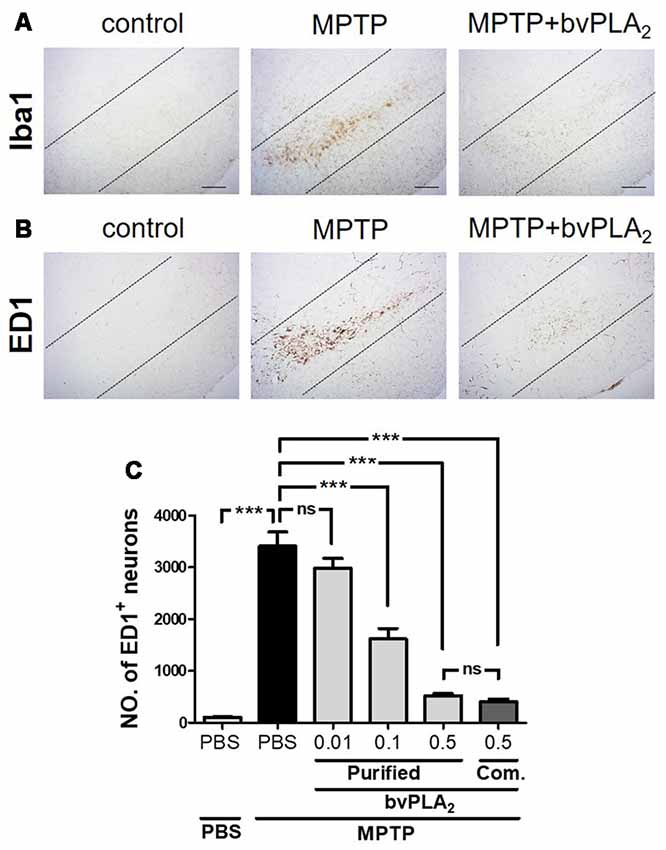
Figure 6. Dose-dependent inhibitory effect of purified standard bvPLA2 on microglial activation in the MPTP mouse model of PD. Immunohistochemical analysis was performed on MPTP-challenged mice treated with standard bvPLA2 purified from crude BV (0.01, 0.1, or 0.5 mg/kg), or commercial standard bvPLA2 (Com. bvPLA2; 0.5 mg/kg) for consecutive 6 days. Representative images of sections containing Iba1- (A) or ED1-positive (B) neurons are displayed and dotted lines indicate SN pars compacta (SNpc). MPTP+bvPLA2 group: standard bvPLA2, purified from crude BV, was injected from Day 1 to Day 6 post-MPTP at a concentration of 0.5 mg/kg. (C) Unbiased stereological estimation for ED1-postive neurons was performed in SN region. The data are expressed as the means ± SEM. n = 3–5 per group; ***p < 0.001, ns, not significant. Scale bar: 100 μm.
Discussion
In the present study, we developed an effective method of bvPLA2 purification to create a valid and safe standardized medicine. Using MPTP animal models, we further demonstrated the therapeutic potential of purified standard bvPLA2 against PD. Additionally, we identified the dose-dependent neuroprotective effect of purified standard bvPLA2 on PD mice.
Growing evidence suggests that neuroinflammation is involved in the development and progression of PD (Wang et al., 2015). It is generally suggested that nerve damage in the pathogenesis of PD can be aggravated by the activated microglia producing an excess of pro-inflammatory cytokines. In contrast, some recent studies have proposed that activated microglia, especially M2 microglia, can be beneficial, at least in the early phase of the neurodegeneration process (Hu et al., 2012). Additionally, a growing body of evidence suggests that neuroinflammatory processes can act as a double-edged sword in diverse pathologic conditions linked to neurodegenerative diseases (Wang et al., 2015). Hence, when identifying a novel anti-inflammatory drug for treating neurodegenerative disease, though evaluation to designate a therapeutic window at a minimum effective dose is necessary.
To our knowledge, the present study was the first to present manufacturing process of bvPLA2 from crude BV, as well as to assess its therapeutic efficacy for treatment of PD. We developed effective strategies to isolate and purified bvPLA2 from active components of crude BV at GMP facilities. The separated bvPLA2 displayed bioactivity with showing a specific activity of about 2,000 unit/mg. In order to evaluate translational relevance and therapeutic potential of purified bvPLA2, we examined the therapeutic effect of purified bvPLA2 by injecting three different doses (0.01, 0.1, 0.5 mg/kg) on MPTP-challenged PD mice. Indeed, it is known that high doses of bvPLA2 (2.5 mg/kg) can cause a variety of allergic reactions, while relatively low doses of bvPLA2 (0.25 mg/kg) induce protective effects against inflammatory conditions (Palm et al., 2013). Previously, we also observed that relatively low doses of bvPLA2 (0.2–0.5 mg/kg) could induce anti-inflammatory effects in murine models of atopic dermatitis (Jung et al., 2017) and asthma (Park et al., 2015). Hence, we assumed the biological function of bvPLA2 may differ in a dose-dependent manner, reflecting that at low doses bvPLA2 act more generally to reduce neuroinflammation. Further studies are required to clarify the paradoxical effect and dose-dependent activity of bvPLA2.
In this study, we found a dose-dependent neuroprotective effect of purified standard bvPLA2 containing ~78% of PLA2 and ~15% of melittin. In line with the experiments with purified bvPLA2, we also examined whether melittin caused a neuroprotective effect against PD by isolated from crude BV with high purity (98.5% of melittin). Administration of purified melittin did not induce any neuroprotective effect on PD mice. Additionally, the inactive mutant of bvPLA2 (bvPLA2-H34A) caused an inhibitory effect on the neuronal death and motor deficits in MPTP-challenged mice. Melittin is a major component of BV, comprising around 50% of dry venom. Recently, many studies have reported the therapeutic effects of melittin against cancers, neurodegenerative diseases and chronic inflammatory diseases including rheumatism (Park et al., 2004; Yang et al., 2011; Rady et al., 2017). It has been suggested that the biological activity of bvPLA2 can be enhanced by melittin (Mingarro et al., 1995; Shen et al., 2010). Certainly, more detailed sets of experimental investigations are needed to verify its potential usefulness in the future applications.
The initial study regarding the involvement of inflammation in PD progression over 30 years ago clearly reported activated microglia in the SN of a PD patient (McGeer et al., 1988). Since then accumulated studies support the role of activated microglia and increased inflammatory mediators in the pathology of PD (Collins et al., 2012). In particular, accumulating evidence suggests the important role of adaptive immune system in the development and progression of PD (Ferrari and Tarelli, 2011). Importantly, the cytotoxic CD4+ T cells have been discovered in the SN of PD patients and animal PD models (Brochard et al., 2009; Stone et al., 2009). Indeed, the adaptive immune system would modulate neuroinflammatory response via regulation of microglia activation (Tansey and Goldberg, 2010). In this study, we observed the inhibitory effect of bvPLA2 isolated and purified from BV on dopaminergic cell loss of MPTP mice. Impressively, induction of immunosuppressive regulatory T cells was observed in MPTP mice treated with purified standard bvPLA2. These results suggest that bvPLA2 may exert anti-inflammatory action via activation of Treg cells, which can suppress the inflammatory process by targeting cytotoxic CD4+ T cells.
In summary, we standardized the purification process to provide standard bvPLA2 from active component of crude BV and observed dose-dependent therapeutic effect of standard bvPLA2 on MPTP-induced PD mice. Based on our results, it appears that bvPLA2 is a potent drug that can promote the survival of dopaminergic neurons, suggesting a novel therapeutic candidate as an add-on to conventional dopaminergic substitution treatments.
Ethics Statement
All experiments were performed in accordance with the approved animal protocols and guidelines established by Kyung Hee University.
Author Contributions
KK and HB conceived and designed the project. KK, SL, JS, and HJ performed the experiments and analyzed the data. J-TH was in charge of standardization of the manufacturing process of bvPLA2 from crude BV. The manuscript was written by KK and HB.
Funding
This work was supported by the Basic Science Research Program through the National Research Foundation (NRF) of South Korea funded by the Ministry of Science, ICT and Future Planning (NRF-2017R1A2B3009574), the Korea Health Technology R&D Project through the Korea Health Industry Development Institute (KHIDI), funded by the Ministry of Health and Welfare, South Korea (HI17C0978).
Conflict of Interest Statement
J-TH was employed by company Inist ST Co. Ltd.
The remaining authors declare that the research was conducted in the absence of any commercial or financial relationships that could be construed as a potential conflict of interest.
References
Ameratunga, R. V., Hawkins, R., Prestidge, R., and Marbrook, J. (1995). A high efficiency method for purification and assay of bee venom phospholipase A2. Pathology 27, 157–160. doi: 10.1080/00313029500169782
Bertram, L., and Tanzi, R. E. (2005). The genetic epidemiology of neurodegenerative disease. J. Clin. Invest. 115, 1449–1457. doi: 10.1172/jci24761
Brochard, V., Combadiere, B., Prigent, A., Laouar, Y., Perrin, A., Beray-Berthat, V., et al. (2009). Infiltration of CD4+ lymphocytes into the brain contributes to neurodegeneration in a mouse model of Parkinson disease. J. Clin. Invest. 119, 182–192. doi: 10.1172/JCI36470
Chen, Y., Qi, B., Xu, W., Ma, B., Li, L., Chen, Q., et al. (2015). Clinical correlation of peripheral CD4+cell subsets, their imbalance and Parkinson’s disease. Mol. Med. Rep. 12, 6105–6111. doi: 10.3892/mmr.2015.4136
Chung, E. S., Lee, G., Lee, C., Ye, M., Chung, H. S., Kim, H., et al. (2015). Bee venom phospholipase A2, a novel Foxp3+ regulatory T cell inducer, protects dopaminergic neurons by modulating neuroinflammatory responses in a mouse model of Parkinson’s disease. J. Immunol. 195, 4853–4860. doi: 10.4049/jimmunol.1500386
Collins, L. M., Toulouse, A., Connor, T. J., and Nolan, Y. M. (2012). Contributions of central and systemic inflammation to the pathophysiology of Parkinson’s disease. Neuropharmacology 62, 2154–2168. doi: 10.1016/j.neuropharm.2012.01.028
de Lau, L. M., Giesbergen, P. C., de Rijk, M. C., Hofman, A., Koudstaal, P. J., and Breteler, M. M. (2004). Incidence of parkinsonism and Parkinson disease in a general population: the Rotterdam study. Neurology 63, 1240–1244. doi: 10.1212/01.wnl.0000140706.52798.be
Ferrari, C. C., and Tarelli, R. (2011). Parkinson’s disease and systemic inflammation. Parkinsons. Dis. 2011:436813. doi: 10.4061/2011/436813
Hossen, M. S., Ali, M. Y., Jahurul, M. H. A., Abdel-Daim, M. M., Gan, S. H., and Khalil, M. I. (2017). Beneficial roles of honey polyphenols against some human degenerative diseases: a review. Pharmacol. Rep. 69, 1194–1205. doi: 10.1016/j.pharep.2017.07.002
Hossen, M. S., Shapla, U. M., Gan, S. H., and Khalil, M. I. (2016). Impact of bee venom enzymes on diseases and immune responses. Molecules 22:E25. doi: 10.3390/molecules22010025
Hu, X., Li, P., Guo, Y., Wang, H., Leak, R. K., Chen, S., et al. (2012). Microglia/macrophage polarization dynamics reveal novel mechanism of injury expansion after focal cerebral ischemia. Stroke 43, 3063–3070. doi: 10.1161/strokeaha.112.659656
Jackson-Lewis, V., and Przedborski, S. (2007). Protocol for the MPTP mouse model of Parkinson’s disease. Nat. Protoc. 2, 141–151. doi: 10.1038/nprot.2006.342
Jeong, J. K., Moon, M. H., Bae, B. C., Lee, Y. J., Seol, J. W., and Park, S. Y. (2011). Bee venom phospholipase A2 prevents prion peptide induced-cell death in neuronal cells. Int. J. Mol. Med. 28, 867–873. doi: 10.3892/ijmm.2011.730
Jung, K. H., Baek, H., Kang, M., Kim, N., Lee, S. Y., and Bae, H. (2017). Bee venom phospholipase A2 ameliorates house dust mite extract induced atopic dermatitis like skin lesions in mice. Toxins 9:E68. doi: 10.3390/toxins9020068
Kim, Y. S., and Joh, T. H. (2006). Microglia, major player in the brain inflammation: their roles in the pathogenesis of Parkinson’s disease. Exp. Mol. Med. 38, 333–347. doi: 10.1038/emm.2006.40
Lang, A. E., and Lozano, A. M. (1998). Parkinson’s disease. First of two parts. N. Engl. J. Med. 339, 1044–1053. doi: 10.1056/NEJM199810083391506
Lee, J. A., Son, M. J., Choi, J., Jun, J. H., Kim, J. I., and Lee, M. S. (2014). Bee venom acupuncture for rheumatoid arthritis: a systematic review of randomised clinical trials. BMJ Open 4:e006140. doi: 10.1136/bmjopen-2014-006140
McGeer, P. L., Itagaki, S., Boyes, B. E., and McGeer, E. G. (1988). Reactive microglia are positive for HLA-DR in the substantia nigra of Parkinson’s and Alzheimer’s disease brains. Neurology 38, 1285–1291. doi: 10.1212/wnl.38.8.1285
Mingarro, I., Pérez-Payá, E., Pinilla, C., Appel, J. R., Houghten, R. A., and Blondelle, S. E. (1995). Activation of bee venom phospholipase A2 through a peptide-enzyme complex. FEBS Lett. 372, 131–134. doi: 10.1016/0014-5793(95)00964-b
Mukhopadhyay, A., and Stahl, P. (1995). Bee venom phospholipase A2 is recognized by the macrophage mannose receptor. Arch. Biochem. Biophys. 324, 78–84. doi: 10.1006/abbi.1995.9926
Ogawa, N., Hirose, Y., Ohara, S., Ono, T., and Watanabe, Y. (1985). A simple quantitative bradykinesia test in MPTP-treated mice. Res. Commun. Chem. Pathol. Pharmacol. 50, 435–441.
Palm, N. W., Rosenstein, R. K., Yu, S., Schenten, D. D., Florsheim, E., and Medzhitov, R. (2013). Bee venom phospholipase A2 induces a primary type 2 response that is dependent on the receptor ST2 and confers protective immunity. Immunity 39, 976–985. doi: 10.1016/j.immuni.2013.10.006
Park, S., Baek, H., Jung, K. H., Lee, G., Lee, H., Kang, G. H., et al. (2015). Bee venom phospholipase A2 suppresses allergic airway inflammation in an ovalbumin-induced asthma model through the induction of regulatory T cells. Immun. Inflamm. Dis. 3, 386–397. doi: 10.1002/iid3.76
Park, H. J., Lee, S. H., Son, D. J., Oh, K. W., Kim, K. H., Song, H. S., et al. (2004). Antiarthritic effect of bee venom: inhibition of inflammation mediator generation by suppression of NF-kappaB through interaction with the p50 subunit. Arthritis Rheum. 50, 3504–3515. doi: 10.1002/art.20626
Rady, I., Siddiqui, I. A., Rady, M., and Mukhtar, H. (2017). Melittin, a major peptide component of bee venom and its conjugates in cancer therapy. Cancer Lett. 402, 16–31. doi: 10.1016/j.canlet.2017.05.010
Reynolds, A. D., Stone, D. K., Hutter, J. A., Benner, E. J., Mosley, R. L., and Gendelman, H. E. (2010). Regulatory T cells attenuate Th17 cell-mediated nigrostriatal dopaminergic neurodegeneration in a model of Parkinson’s disease. J. Immunol. 184, 2261–2271. doi: 10.4049/jimmunol.0901852
Sakaguchi, S., Yamaguchi, T., Nomura, T., and Ono, M. (2008). Regulatory T cells and immune tolerance. Cell 133, 775–787. doi: 10.1016/j.cell.2008.05.009
Samii, A., Etminan, M., Wiens, M. O., and Jafari, S. (2009). NSAID use and the risk of Parkinson’s disease: systematic review and meta-analysis of observational studies. Drugs Aging 26, 769–779. doi: 10.2165/11316780-000000000-00000
Shen, L. R., Ding, M. H., Zhang, L. W., Zhang, W. G., Liu, L., and Li, D. (2010). Expression of a bee venom phospholipase A2 from Apis cerana cerana in the baculovirus-insect cell. J. Zhejiang Univ. Sci. B 11, 342–349. doi: 10.1631/jzus.b0900254
Stone, D. K., Reynolds, A. D., Mosley, R. L., and Gendelman, H. E. (2009). Innate and adaptive immunity for the pathobiology of Parkinson’s disease. Antioxid. Redox Signal. 11, 2151–2166. doi: 10.1089/ARS.2009.2460
Tansey, M. G., and Goldberg, M. S. (2010). Neuroinflammation in Parkinson’s disease: its role in neuronal death and implications for therapeutic intervention. Neurobiol. Dis. 37, 510–518. doi: 10.1016/j.nbd.2009.11.004
Tufekci, K. U., Meuwissen, R., Genc, S., and Genc, K. (2012). Inflammation in Parkinson’s disease. Adv. Protein Chem. Struct. Biol. 88, 69–132. doi: 10.1016/B978-0-12-398314-5.00004-0
Wang, Q., Liu, Y., and Zhou, J. (2015). Neuroinflammation in Parkinson’s disease and its potential as therapeutic target. Transl. Neurodegener. 4:19. doi: 10.1186/s40035-015-0042-0
West, M. J. (1993). New stereological methods for counting neurons. Neurobiol. Aging 14, 275–285. doi: 10.1016/0197-4580(93)90112-o
West, M. J., Slomianka, L., and Gundersen, H. J. (1991). Unbiased stereological estimation of the total number of neurons in thesubdivisions of the rat hippocampus using the optical fractionator. Anat. Rec. 231, 482–497. doi: 10.1002/ar.1092310411
Yang, E. J., Kim, S. H., Yang, S. C., Lee, S. M., and Choi, S. M. (2011). Melittin restores proteasome function in an animal model of ALS. J. Neuroinflammation 8:69. doi: 10.1186/1742-2094-8-69
Ye, M., Chung, H. S., Lee, C., Yoon, M. S., Yu, A. R., Kim, J. S., et al. (2016). Neuroprotective effects of bee venom phospholipase A2 in the 3xTg AD mouse model of Alzheimer’s disease. J. Neuroinflammation 13:10. doi: 10.1186/s12974-016-0476-z
Keywords: Parkinson’s disease, regulatory T cells, bee venom phospholipase A2, neuroinflammation, dose-dependent response
Citation: Kim KH, Lee SY, Shin J, Hwang J-T, Jeon HN and Bae H (2019) Dose-Dependent Neuroprotective Effect of Standardized Bee Venom Phospholipase A2 Against MPTP-Induced Parkinson’s Disease in Mice. Front. Aging Neurosci. 11:80. doi: 10.3389/fnagi.2019.00080
Received: 31 October 2018; Accepted: 19 March 2019;
Published: 05 April 2019.
Edited by:
Seung-Nam Kim, Dongguk University Seoul, South KoreaReviewed by:
Craig Doupnik, USF Health, United StatesMohamed M. Abdel-Daim, Suez Canal University, Egypt
Roberta Marongiu, Cornell University, United States
Copyright © 2019 Kim, Lee, Shin, Hwang, Jeon and Bae. This is an open-access article distributed under the terms of the Creative Commons Attribution License (CC BY). The use, distribution or reproduction in other forums is permitted, provided the original author(s) and the copyright owner(s) are credited and that the original publication in this journal is cited, in accordance with accepted academic practice. No use, distribution or reproduction is permitted which does not comply with these terms.
*Correspondence: Hyunsu Bae, aGJhZUBraHUuYWMua3I=