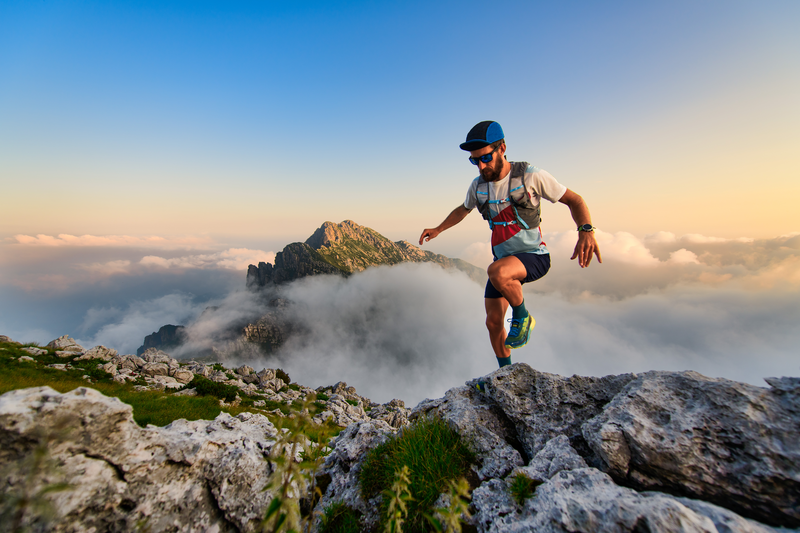
94% of researchers rate our articles as excellent or good
Learn more about the work of our research integrity team to safeguard the quality of each article we publish.
Find out more
ORIGINAL RESEARCH article
Front. Aging Neurosci. , 04 December 2018
Sec. Cellular and Molecular Mechanisms of Brain-aging
Volume 10 - 2018 | https://doi.org/10.3389/fnagi.2018.00389
This article is part of the Research Topic Interphase Between Aging and Neurodegenerative Diseases View all 10 articles
Oxidative stress is one of the main causes of AMD. Hydrogen has anti-oxidative stress and apoptotic effects on retinal injury. However, the effect of hydrogen on AMD is not clear. In this study, fundus radiography, OCT, and FFA demonstrated that HRW reduced the deposition of drusen-like structures in RPE layer, prevented retina from thinning and leakage of ocular fundus vasculature induced by NaIO3. ERG analysis confirmed that HRW effectively reversed the decrease of a-wave and b-wave amplitude in NaIO3-mice. Mechanistically, HRW greatly reduced the oxidative stress reaction through decreased MDA levels, increased SOD production, and decreased ROS content. The OGG1 expression was downregulated which is a marker of oxidative stress. Involvement of oxidative stress was confirmed using oxidative stress inhibitor ALCAR. Moreover, oxidative stress reaction was associated with expression of Sirt1 level and HRW significantly inhibited the downregulation of Sirt1 expression. This result was further confirmed with AICAR which restore Sirt1 expression and activity. In addition, NaIO3-induced retinal damage was related to apoptosis via caspase 8 and caspase 9, but not the caspase 3 pathways, which led to upregulation of Bax and p53, downregulation of Bcl-2, and increase in Jc-1-positive cells in mice. However, HRW effectively reversed these effects that apoptosis induced. These results suggest that HRW protects retinal functions against oxidative stress injury through inhibiting downregulation of Sirt1 and reducing retinal apoptosis. Therefore, we speculated that hydrogen administration is a promising treatment for AMD therapy.
AMD is the leading cause of blindness in the elderly population (Syed et al., 2012). The prevalence of AMD gradually increases with age. About 11 million people in the America suffer from visual impairment due to AMD, with approximately 170 million people in the worldwide (Pennington and DeAngelis, 2016). AMD has also become an important cause of blindness in China due to the aging population. The pathogenesis of AMD is related to many factors, such as metabolic disorders, immunity, inflammation, oxidative stress, and so on. However, oxidative stress is one of the main causes of AMD (Hernández-Zimbrón et al., 2018). During the past few decades, although aggressive and combined treatment regimens, including drugs targeting VEGF receptors, laser coagulation, gene therapy, anti-oxidants, and so on, have been used, the rate of blindness is still increasing (Holz et al., 2014; Rakoczy, 2017; Hernández-Zimbrón et al., 2018). Therefore, developing novel therapeutic agents with less toxicity and understanding their molecular mechanisms are necessary for improving AMD outcomes.
Hydrogen as a reducing agent has therapeutic effects for the reduction of oxidative stress, inflammation, and apoptosis (Xie et al., 2012; Xin et al., 2014; Shao et al., 2016; Lin et al., 2017). For example, hydrogen improves oxidant stress-induced organic injury, including acute kidney injury (Du et al., 2016), acute hepatic failure (Sun et al., 2011), chronic obstructive pulmonary disease (Liu Z. et al., 2017), and spinal cord injury (Ge et al., 2017) in rodents. Moreover, it can delay the progress of some diseases, such as diabetes and hypertension (Takeuchi et al., 2015; Guo et al., 2017). In the eye, several laboratories have shown that hydrogen exerts a neuroprotective effect on retinal ganglion cells and on retinal injury induced by light (Tian et al., 2013) in mice. For AMD studies, NaIO3 is used to induce oxidative stress and results in retinal function injury to mimic the characteristics of clinical AMD (Zieger and Punzo, 2016; Berkowitz et al., 2017). However, it remains unclear whether hydrogen has similar effects on AMD.
Sirt1 is a nicotinamide adenine dinucleotide-dependent protein deacetylase, which has been frequently reported to be involved in neuroprotection, cell apoptosis, cell senescence, oxidative stress, and other processes by deacetylating downstream targets (Balaiya et al., 2017). Sirt1 regulates a variety of transcription factors, including p53, FOXOs, PCG1-α, and so on (Luo et al., 2001; Brunet et al., 2004). Moreover, Sirt1 is also considered a longevity molecule to prevent against age-related diseases (Tissenbaum and Guarente, 2001; Bjørklund et al., 2018). In the eye of the rodent, immunostaining from several laboratories showed Sirt1 expression in the outer nuclear layer, inner nuclear layer, and ganglion cell layer of the retina (Jaliffa et al., 2009). Activation of Sirt1 promotes the resistance of neurons to oxidative stress and blocks damage to retinal neurons (Zeng et al., 2016). Additionally, there is more recent experimental evidence indicating that Sirt1 can directly bind to p53 to promote cell survival under stress by specifically repressing the p53-dependent apoptotic response (Brunet et al., 2004). Thus, the activation of Sirt1 may have a beneficial retinal protective effect by reducing intracellular oxidative stress and apoptosis (Zheng and Lu, 2016; Hou et al., 2017; Li et al., 2017a,b; Yao et al., 2018).
The purpose of this study was to investigate the protective role of hydrogen in AMD mice. We observed mouse fundus, retinal structure and function, as well as Sirt1 expression following hydrogen administration. We also tested the hypothesis that hydrogen can regulate retinal oxidative stress reactions and apoptosis pathways. These results suggest that hydrogen is promising treatment in AMD therapy.
Briefly, HRW was obtained by placing a metallic magnesium stick into drinking water [Mg + 2H2O→Mg (OH)2 + H2] and hydrogen final concentration was 0.55∼0.65 mM. The magnesium stick contained 99.9% pure metallic magnesium and natural stones in a polypropylene and ceramic container (Nakao et al., 2010).
Healthy 8- to 10-week-old C57BL/6 male mice were purchased from the Beijing Vital River Laboratory Animal Technology Co., Ltd. (Beijing, China). The mice were maintained in a specific pathogen-free grade animal facility under a 12-h light–dark cycle. All procedures were approved by the Committee on Animal Research of Jinzhou Medical University and followed the ARRIVE guidelines pertaining to animal experimentation. NaIO3 (Macklin, Shanghai, China) as an inorganic substance and induced retinal damage is currently recognized as an ideal animal model for AMD research (Kannan and Hinton, 2014). The tail vein injection of NaIO3 to mice can cause oxidative stress reaction in the retina, which induces mice macular degeneration, which is similar to clinical AMD (Wang et al., 2014; Chowers et al., 2017).
Mice were randomly divided into three groups: control group, NaIO3 group, and hydrogen group. Mice in the hydrogen group were given HRW (0.1 ml/g/day, intragastric administration three times daily) by gavage for 7 days prior to a tail vein injection with NaIO3 at a dose of 20 mg/kg, and then HRW treatment continued for 5 days. The vehicle-treated control mice received an equal volume of 0.9% physiological saline.
ALCAR is an inhibitor of oxidative stress (Morigi et al., 2015). To further confirm that hydrogen protects against retinal damage via inhibiting NaIO3-induced oxidative stress, we injected intraperitoneally with ALCAR. In addition, AICAR acts as an agonist of AMPK, which activates Sirt1 after AMPK activation (Tao et al., 2017). To further confirm that hydrogen increases the expression of Sirt1 and protects against NaIO3-induced retinal damage, we injected intraperitoneally with AICAR in NaIO3 mice. So, another group of mice was randomized and pretreated with HRW for 7 days prior to an intraperitoneal injection of ALCAR (0.2 mg/g; Abcam, Cambridge, MA, USA) or AICAR (0.5 mg/g; Abcam), as described in previous studies (Morigi et al., 2015). Mice were then given a tail vein injection of NaIO3 (20 mg/kg) followed by continued HRW administration for 5 days.
After anesthesia with pentobarbital sodium (65 mg/kg) (Yang et al., 2012), fundus photography images were obtained using a retinal imaging system (MicrolV, Phoenix, AZ, United States). OCT images centered on the optic papilla were acquired from anesthetized animals using an OCT system (ISOCT, Optoprobe, Canada).
After anesthesia with pentobarbital sodium (65 mg/kg), sodium fluorescein (6 mg/kg) solution was injected intraperitoneally (Chung et al., 2017), and tropicamide was used. FFA examination was completed using an imaging system (OPTP-RIS, Optoprobe, Canada).
After 12 h of dark adaptation, mice were anesthetized with pentobarbital sodium (65 mg/kg), corneal surface anesthesia was completed with oxybuprocaine hydrochloride eye drops, and tropicamide was used to dilate the pupil. Mice were placed on the operating table. A circular corneal electrode was placed on the surface of the bilateral cornea of the mouse, and a needle-shaped stainless-steel reference electrode was inserted subcutaneously behind the ear of the mouse. A needle-shaped ground electrode punctured the subcutaneous end of the mouse tail (Zhou et al., 2014). The above operation was performed under dark red light. After the baseline stabilized on the display screen, the amplitude changes of the a-wave and b-wave of 3.0 cd.s.m-2 ERG were recorded (ICR, Chongqing, China).
Tissue homogenates were prepared from retinas of each group after adding the tissue cleavage solution and centrifugation for 30 min at 4°C and 12,000 ×g. The protein concentration was measured quantitatively with a BCA protein assay kit (P0010s; Beyotime; Shanghai, China). Equal amounts of protein (2 mg/ml) were separated on a 10% SDS-PAGE with an electrophoresis system. Then, the proteins were transferred to a PVDF membrane after electrophoresis and blocked with 1% fetal bovine serum and incubated in strips at 4°C with the following primary antibodies: anti-Sirt1 (ab12193; 1:1000; Abcam), anti-OGG1 (15125-1-AP; 1:1000; Proteintech; Chicago, IL, United States), anti-caspase 3 (ab44976; 1:1000; Abcam), anti-caspase 8 (ab25901; 1:1000; Abcam), anti-caspase 9 (9509S; 1:1000; CST; Massachusetts, United States), anti-P53 (ab131442; 1:1000; Abcam), anti-Bax (ab32503; 1:1000; Abcam), anti-Bcl-2 (ab32124; 1:1000; Abcam), PTG mouse anti-actin (66009-1-Ig; 1:2000; Proteintech; Chicago, IL, United States), and then with anti-rabbit and anti-mouse secondary antibodies (SA00001-2; SA00001-1; 1: 2000; Proteintech) for 2 h at room temperature. ECL emission (1705060; Bio-rad; Hercules, CA, United States) was used to visualize bands, and images were recorded through a gel imaging system. Images were scanned with ImageJ 4.0 software.
ROS levels were measured using a DHE kit (s0063, Beyotime), according to previous studies (Song et al., 2016). Briefly, mouse eyeballs were fixed and then OCT-embedded. Frozen sections (5 μm) were incubated with DHE dye in a 37°C incubator for 30 min. DHE was incubated with a superoxide anion that results in conversion to the red fluorescent compound ethidium. Fluorescence microscopy under the same exposure conditions was used to observe retinal layer ROS content. In retinal sections, the percentage of the ROS area stained with red fluorescence was normalized to the total area examined and quantified with ImageJ 4.0 analysis software.
The Jc-1 kit (c2006, Beyotime) was used for the detection of the mitochondrial membrane potential, according to a previous study (Mitter et al., 2014). Briefly, frozen tissue sections were incubated with a mixture of Jc-1 stain at 37°C for 20 min in an incubator. The membrane potential of the retina was measured using the same exposure conditions with a fluorescence microscope. The percentage of the apoptosis area stained with green fluorescence was normalized to the total area examined and quantified with ImageJ 4.0 software.
Retina tissues were weighed and washed in PBS and then homogenized immediately in 10 volumes of PBS at 37°C. After centrifugation, supernatants were collected and stored at -80°C. The levels of activated SOD and oxidative stress product MDA were measured according to the instructions of the kit (A001-3, A003-1; Nanjing Jiancheng Bioengineering Institute, Nanjing, China). The absorbance was read on a microplate reader (Denley Dragon, Wellscan MK3, Thermo, Finland), and the concentrations were calculated based on a standardized curve.
All data are expressed as the mean ± SEM, and one-way ANOVA was used to compare differences between the groups. The LSD test was used to compare multiple pairs of means. Comparison between two groups was done with a t-test. P < 0.05 was considered statistically significant.
To assess the protective effect of HRW treatment on NaIO3-induced retinal damage in mice, we performed eye functional examinations in NaIO3 mice with or without HRW treatment. Fundus photography revealed a large number of yellow-white, drusen-like structures in the fundus of NaIO3-treated mice. We observed a significant reduction in yellow-white deposits on the fundus after HRW treatment (Figure 1A). By OCT, we found that the RPE layer of mice in the NaIO3 group developed a large number of high reflex zones and the retina became thin (Figure 1B). However, HRW prevented retinal thinning and reduced the number of high reflex zones in the RPE layer in mice that received HRW-treatment (Figure 1C, P < 0.01). To further confirm the damage of NaIO3 to the retina, we examined the retinal vascular integrity. The results of the FFA assay showed that retinal blood vessel leakage appeared in NaIO3-treated mice (Figure 1D), but the retinal leakage area in HRW-treated mice was much smaller than that in NaIO3 mice (Figure 1E, P < 0.01). These results confirm the protective effect of HRW on retinal injury.
FIGURE 1. Effects of HRW on NaIO3-induced fundus and retinal impairment in mice. After cotreatment with HRW (0.1 ml/g/day, i.g.) and NaIO3 (20 mg/kg, i.v.) for 12 days, retinas were evaluated with the fundus photography, OCT examination, and FFA examination. (A) Representative images of fundus photography. The black arrow represents yellow-white, drusen-like structures. (B) Representative images of OCT in the mouse retina and (C) quantification of retinal thickness. White arrows indicate areas of hyperreflexia in the RPE area. (D) Representative images of FFA and (E) quantification of the leakage area. White arrowheads represent the leaking area. RPE, retinal pigment epithelium; IS/OS, inner segment/outer segment; ONL, outer nuclear layer; OPL, outer plexiform layer; INL, inner nuclear layer. Values are presented as the mean ± SEM, n = 5, ∗∗P < 0. 01 vs. the NaIO3 group.
Studies have shown that NaIO3 can damage RPE cells and retinal visual function (Zieger and Punzo, 2016). We assessed the effect of HRW on retinal function using an ERG assay in mice. The a-wave reflects the function of the cone cells and the rod cells, and the b-wave represents the function of the bipolar cells. In the dark-adapted 3.0 ERG, a- and b-wave amplitudes were significantly increased in the HRW-treated mice compared to those in the NaIO3 mice, reflecting the recovery of visual function in HRW-treated mice and the resistance to NaIO3-induced functional visual damage by HRW (Figure 2; P < 0.05). This result suggested that HRW can protect the retina from NaIO3-induced damage in mice.
FIGURE 2. Effects of HRW on NaIO3-induced visual function impairment in mice. (A) ERG map of dark adaptation 3.0 in control, NaIO3, and HRW groups. N represents the start of stimulation, a represents the amplitude of the a-wave, and b represents the b-wave amplitude. (B,C) Quantification of the a-wave and b-wave amplitudes, respectively. Values are presented as the mean ± SEM, n = 10, ∗∗P < 0.01 vs. the NaIO3 group.
The mechanism of NaIO3-induced retinal damage is associated with oxidative stress, but whether the protective effect of HRW on the retina is through a reduction in oxidative stress was examined. First, we detected NaIO3-induced retinal damage with H&E staining. It clearly showed that a large amount of melanin deposition appears in the RPE layer retinas from NaIO3 mice compared to retinas from control mice. The amount of the black sediment on the retina in HRW-treated mice is greatly reduced compared to the amount in NaIO3 mice (Figure 3A). Moreover, the retinal layers in NaIO3 mice were thinner than those of the control mice. However, HRW prevents retinal thinning (Figure 3B, P < 0.01). In addition, the thickness of the outer nuclear layer in NaIO3 mice is significantly reduced compared to that in control mice (Figure 3C, P < 0.01), which indicated that NaIO3 caused retinal injury. In addition, we assessed the toxic effect of NaIO3 on the liver using H&E staining. No changes were observed in liver sections between the three groups (Figure 3D), suggesting that NaIO3 selectively damages the eyeball.
FIGURE 3. Effects of HRW on NaIO3-induced retinal morphological impairment in mice. Mouse eyeballs were harvested after cotreatment with HRW and NaIO3 for 12 days. (A) Representative images of H&E-stained eyeball sections from control and NaIO3 mice treated with or without HRW. Yellow arrows indicate drusen-like melanin depositions in the RPE layer. (B) Quantitative analysis of retinal thickness via H&E-stained eyeball sections. The values are expressed as the mean ± SEM, n = 10. (C) Representative images of OCT in the mouse retina in control and NaIO3 groups. Yellow arrows point toward areas of hyperreflexia in the RPE. (D) H&E staining of the liver after 5 days of NaIO3 injection. n = 5, ∗∗P < 0.01 vs. the NaIO3 group.
Next, we observed the oxidative stress reaction in the retina. As Figure 4A shows, HRW significantly increased the activity of the oxidative stress inhibitory enzyme SOD, whereas it decreased MDA content in NaIO3 mice. To further confirm this result, we examined the expression level of OGG1, an oxidative stress marker protein, in the retina. The expression of OGG1 in NaIO3 mice is greatly increased compared to control expression. However, HRW significantly reduced the NaIO3-induced increase in OGG1 expression (Figure 4B; P < 0.05). DHE staining, which reflects the ROS content in the retina, showed a marked decrease in red fluorescence of ROS-stained retinal tissue in HRW-treated mice compared to the retinas of NaIO3 mice (Figure 4C; P < 0.01). These results suggest that HRW can attenuate the oxidative stress reaction by NaIO3-induction in the mouse retina.
FIGURE 4. Effect of HRW on NaIO3-exerted retinal oxidative stress in mice. Retinal tissue collection after cotreatment with HRW and NaIO3 for 12 days. (A) The level of SOD and MDA in the retina was analyzed by SOD and MDA kits. (B) Representative blots and densitometry data of OGG1 expression in the retinas from NaIO3 mice with and without HRW treatment. (C) The activity of ROS was measured by DHE staining, the red staining is positive for ROS content. Data represent the mean ± SEM of three independent experiments, n = 3, ∗P < 0.05, ∗∗P < 0.01 vs. the NaIO3 group.
Sirt1 plays an important role in various retinal diseases, including anti-oxidant and anti-apoptotic effects in mice (Han et al., 2017). In this study, we assessed whether HRW reduced the oxidative stress reaction through the regulation of Sirt1 expression. Western blotting indicated that Sirt1 expression was significantly downregulated in NaIO3 mice, and HRW treatment reversed the NaIO3-induced downregulation of Sirt1 expression (Figure 5A; P < 0.05).
We next tested whether HRW inhibits the downregulation of Sirt1 through a reduction in oxidative stress. Mice were injected with ALCAR, an inhibitor of oxidative stress, before NaIO3 treatment, and then we measured Sirt1 and OGG1 expression. We found that ALCAR significantly reduced the NaIO3-induced downregulation of Sirt1 and increased OGG1 expression in the mouse retina (Figure 5B; P < 0.01). These results confirm that HRW indeed inhibits the downregulation of Sirt1 expression by decreasing oxidative stress.
FIGURE 5. HRW upregulates Sirt1 expression in the retina by inhibiting oxidative stress. (A) Representative blots and quantitative analysis of Sirt1 protein expression at 5 days after NaIO3 injection. Mice were pretreated with ALCAR (0.2 mg/g, i.p.) (B) or AICAR (0.5 mg/g, i.p.) (C) before NaIO3 injection. The expression of Sirt1 and OGG1 were measured by Western blotting. Data represent the mean ± SEM of three independent experiments, n = 3–6, ∗P < 0.05, ∗∗P < 0.01 vs. the NaIO3 group.
In addition, we further analyzed whether HRW protects NaIO3-induced retinal damage through the regulation of Sirt1 expression. Mice were treated with the Sirt1 indirect activator AICAR prior to NaIO3 administration. We found that AICAR treatment significantly increased the expression of Sirt1 in NaIO3 mice (Figure 5C; P < 0.01). At the same time, we measured the expression of OGG1. AICAR could also inhibit the NaIO3-induced OGG1 upregulation (Figure 5C; P < 0.01), suggesting that HRW could inhibit the downregulation of oxidative stress-induced Sirt1 expression.
Studies have shown that NaIO3 induces retinal damage by activating the pathway of apoptosis (Jaliffa et al., 2009; Balmer et al., 2015; Zeng et al., 2016). We first examined apoptosis in the retina of NaIO3 mice. TUNEL staining showed that TUNEL-positive cells (green) were mainly concentrated in the outer nuclear layer of the retina in the NaIO3 mice. HRW greatly decreased the number of TUNEL-positive cells in the retina (Figure 6B; P < 0.01). Further, we analyzed the expression of apoptosis pathway-related proteins in the mouse retina. However, no change was observed in the expression of caspase 3 between mice in all groups (P > 0.05). HRW significantly reduced the expression of caspase 8 (P < 0.01), caspase 9 (Figure 6A; P < 0.01), p53, and Bax, whereas it increased Bcl-2 expression (Figure 6C; P < 0.01) in NaIO3 mice. Additionally, Jc-1 can be used to detect early apoptosis by indicating changes in the mitochondrial membrane potential (Wang et al., 2015). As shown in Figure 6D, early apoptotic cells (green) are abundantly apparent in the retina of NaIO3 mice, and the green fluorescence intensity of Jc-1-staining in HRW-treated mice is significantly decreased (P < 0.01). These results suggest that HRW could inhibit NaIO3-induced retinal apoptosis via caspase 8 and caspase 9 apoptotic pathways.
FIGURE 6. HRW reduces apoptosis in the retina by upregulating Sirt1. (A) Representative blot and quantitative analysis of caspase 3, caspase 8, and caspase 9 protein expression levels at 5 days after NaIO3 injection with or without HRW administration. (B) Representative images of TUNEL-stained retinal sections from control and NaIO3 mice with or without HRW administration. TUNEL-positive cells are shown by asterisks. Data represent the mean ± SEM of three independent experiments. n = 10. (C) Representative blot and the quantitative analysis of protein expression for p53, Bax, and Bcl-2 expression in the retina. n = 4. (D) Representative images of Jc-1-stained retinal sections and the quantification of green fluorescence intensity in retinal sections. n = 5. Representative Western blot and the quantitative analysis of caspase 8, caspase 9, p53, Bax, and Bcl-2 protein expression after ALCAR (E) or AICAR (F) injection in retinas from NaIO3 mice. Values were presented as the mean ± SEM. n = 3–6, ∗∗P < 0.01 vs. the NaIO3 group.
Sirt1 has anti-apoptotic and anti-oxidative stress effects in the rat (Yang et al., 2013; Qi et al., 2015). To demonstrate the anti-apoptotic effect of Sirt1, we examined the expression of apoptosis-related regulators after administration of the Sirt1 indirect agonist AICAR in mice. AICAR significantly inhibited the expression of caspase 8, caspase 9, p53, and Bax, but increased the expression of Bcl-2 in NaIO3 mice (Figure 6E; P < 0.01). These results confirm that HRW has an anti-apoptotic effect through the increase of Sirt1 expression in NaIO3-induced retinal damage.
In addition, we further determined the relationship between oxidative stress and apoptosis using an inhibitor of oxidative stress in NaIO3 mice. After mice were given ALCAR, we examined the expression of proapoptotic proteins (caspase 8, caspase 9, p53, and Bax) and the anti-apoptotic protein Bcl-2. ALCAR significantly inhibited the upregulation of proapoptotic protein expression and downregulation of Bcl-2 protein in NaIO3 mice (Figure 6F; P < 0.01). These results suggest that HRW could effectively inhibit the downregulation of Sirt1 expression through antioxidative stress and has a further antiapoptotic effect on NaIO3-induced retinal injury.
Previous studies have indicated that hydrogen has ideal therapeutic effects for oxidant stress and inflammation (Xin et al., 2014; Lin et al., 2017). Although some studies have demonstrated the anti-apoptosis and anti-oxidative stress effects of hydrogen on light-induced retinal injury in the rodent (Takeuchi et al., 2015), the effect of hydrogen on AMD is still unknown. In the present study, we investigated the retinal protective effects and mechanisms of action of hydrogen in NaIO3-induced mice. Our data demonstrated that HRW could reduce retinal damage through a decreased oxidative stress reaction and inhibit the NaIO3-induced downregulation of Sirt1 expression (Figures 4, 5A). Involvement of oxidative stress and the Sirt1 protein was confirmed using the oxidative stress inhibitor ALCAR and the Sirt1 activator AICAR (Figures 5B,C). Moreover, HRW inhibits apoptosis via the caspase 8 and 9 pathways, but not the caspase 3 pathway, in NaIO3 mice retinas (Figure 6). Interestingly, we found that the injury of NaIO3 is tissue selective and NaIO3 mouse liver sections did not display a significant toxic effect with H&E staining (Figure 3).
HRW can improve the quality of human life and prevent the occurrence of diseases (Mizuno et al., 2018). HRW also can protect the retina from injury in eye diseases in rats (Qi et al., 2015; Chen et al., 2016). We investigated the protective effect of HRW in AMD mice. After mice were given HRW and NaIO3, through fundus photography and OCT we found that HRW reduced the deposition of yellow-white, drusen-like structures induced by NaIO3 and prevented the thinning of the retina (Figures 1A,B). Consistently, it reduced the degree of retinal degeneration. We further found that HRW reduced the area of fundus leakage induced by NaIO3 and maintained the integrity of fundus vessels (Figure 1D). Further, when we observed the retinal function by ERG, we found that HRW increased the survival of rod and cone cells and reversed the degree of visual function impairment by NaIO3 (Figure 2).
Oxidative stress is one of the many factors in the pathogenesis of AMD (Hernández-Zimbrón et al., 2018). NaIO3 induces retinal injury through oxidative stress (Kannan and Hinton, 2014; Berkowitz et al., 2017). As hydroxyl radicals, hydrogen can combine with excess oxygen free radicals, causing inhibition of the oxidative stress reaction by decreasing MDA content and upregulating SOD (Wu et al., 2017). There is growing evidence that hydrogen plays important roles in ROS reduction (Liu et al., 2014). After administration of NaIO3, the RPE layer of the retina became thinner or even ruptured. There was a large amount of black deposits, similar to drusen, between the RPE layer and Bruch’s membrane (Figure 3A). The ONL membrane and the overall thickness of the retina were decreased (Figure 3C). HRW can reduce retinal morphological damage induced by NaIO3 (Figure 3B). The index of oxidative stress showed that HRW decreased the content of MDA in oxidative stress products and prevented the decrease of SOD activity with the oxidative stress inhibitor after NaIO3 treatment (Figure 4A). In addition, we observed the amount of ROS induced by NaIO3 was reduced by HRW because there was a decreased number of ROS-positive cell as shown by the fluorescent intensity of DHE staining in the retina (Figure 4C). Further, to determine that HRW can inhibit oxidative stress, we measured the expression of the oxidative stress marker protein OGG1. The results of our study showed that hydrogen significantly decreased oxidant levels induced by NaIO3 (Figure 4B).
As a deacetylase, Sirt1 is closely related to apoptosis and oxidative stress (Chen et al., 2016). Sirt1 plays a protective role in regulating apoptosis in the retina. With increasing age, the expression of Sirt1 increases in the retina, thus increasing the resistance of the retina to the outside damage (Zeng and Yang, 2015). Hydrogen can activate Sirt1 and protect against damage from diseases, and hydrogen can inhibit oxidative stress and regulate the expression of Sirt1. In our studies, the expression of Sirt1 was downregulated by NaIO3 administration (Figure 5A). However, HRW could elevate the expression of Sirt1 by inhibiting oxidative stress (Figure 5B). Furthermore, oxidative stress inhibitors and Sirt1 activators caused an increase in Sirt1 levels and decrease in OGG1 levels, suggesting that the antioxidant stress of HRW can prevent the downregulation of Sirt1 induced by NaIO3 (Figure 5C). Our study indicated that hydrogen directly mediates the expression of Sirt1 by anti-oxidative stress.
Studies have shown that hydrogen inhibits apoptosis by activating Bcl-2 and inhibiting Bax and caspase 3 (He et al., 2016; Chen et al., 2017). Sirt1 inhibits apoptosis by upregulating the expression of Bax (Liu S. et al., 2017). Sirt1 can inhibit apoptosis by regulating the Bcl-2 family and caspase 3 (Gu et al., 2018). Some studies suggest that oxidative stress inhibits the activity of Sirt1 and then regulates p53-dependent apoptosis (He et al., 2017). In this study, we used TUNEL staining to demonstrate that HRW has an anti-apoptotic effect (Figure 6B). HRW also reduced the expression of caspase 8 and caspase 9 but did not affect caspase 3 expression (Figure 6A). Moreover, our studies further found that HRW decreased the expression of p53 and Bax, but increased the expression of Bcl-2 after NaIO3 administration (Figure 6C), suggesting that the anti-apoptotic effect of hydrogen was through the caspase 8 and caspase 9 pathways. The measurement of the mitochondrial membrane potential also confirmed the role of the anti-apoptotic effect of HRW (Figure 6D). The HRW inhibition of apoptosis was mimicked by oxidative stress inhibitors and Sirt1 activators (Figures 6E,F). These results suggest that hydrogen could promote cell survival in the retina after oxidative stress, and hydrogen inhibits apoptosis by regulating the expression of Sirt1. Our results suggest that hydrogen inhibits oxidative stress and the downregulation of Sirt1 induced by NaIO3 and plays a role in preventing apoptosis.
Molecular hydrogen as a medical gas could be used in antioxidant therapy in numerous human diseases (Kurokawa et al., 2015). The primary advantage of HRW is that safe means of delivering hydrogen (Chen et al., 2016). Hydrogen is a colorless, transparent, odorless, and tasteless gas. It is insoluble in water as the lightest gas. In vitro experiments were carried out under normal pressure. Because of the characteristics of hydrogen and the limitations of our current laboratory conditions, in our studies, AMD animal model was used to confirm the therapeutic effect of hydrogen on AMD. At the same time, we used oxidative stress inhibitors and Sirt1 activators to confirm the role of hydrogen in anti-oxidative stress and anti-apoptosis of AMD. We will conduct in vitro experiments if conditions permit to further support our point of view.
In summary, we found that hydrogen can reverse the production and progress of drusen, improve the function of the optic nerve and the integrity of fundus vessels. Hydrogen regulates the expression of Sirt1 in the retina by inhibiting oxidative stress. Hydrogen inhibits the downregulation of Sirt1 induced by NaIO3 and inhibits apoptosis induced by NaIO3 via regulation of Sirt1 expression. Our findings suggest that hydrogen is an effective therapeutic strategy for the pathogenesis of oxidative stress in AMD.
LL and YL conceived and designed the experiments. YL, RL, JH, JX, XH, and FR performed the experiments. LL, YL, RL, and FR analyzed the data. LL contributed reagents, materials, and analysis tools. LL and YL wrote the paper. XH contributed the process of revised article.
This study was supported by the Natural Science Foundation of Liaoning Province (No. 201602292), Liaoning BaiQianWan Talents Program (No. 2017101), and Liaoning Distinguished Professor Project (No. LNTP20183501).
The authors declare that the research was conducted in the absence of any commercial or financial relationships that could be construed as a potential conflict of interest.
AICAR, 5-aminoimidazole-4-carboxamide1-β-D-ribofuranoside; ALCAR, acetyl-L-carnitine; AMD, age-related macular degeneration; AMPK, AMP-activated protein kinase; ANOVA, analysis of variance; Bax, BCL2-associated X protein; Bcl-2, B-cell lymphoma-2; DHE, dihydroethidium; ECL, enhanced chemiluminescence; ERG, electroretinography; FFA, fundus fluorescein angiography; FOXOs, Forkhead box protein Os; H&E, hematoxylin and eosin; HRW, hydrogen-rich water; Jc-1, mitochondrial membrane potential assay kit with JC-1; LSD, least significant difference; MDA, malondialdehyde; NaIO3, sodium iodate; OCT, optical correlation tomography; OGG1, 8-oxoguanine DNA glycosylase; PBS, phosphate buffer saline; PCG1-α, receptor γ coactivator-1 α; PVDF, polyvinylidene difluoride membrane; ROS, reactive oxygen species; RPE, retinal pigment epithelium; SDS-PAGE, sodium dodecyl sulfate polyacrylamide gel electrophoresis; SEM, standard error of the mean; Sirt1, silent information regulator 2 homolog 1; SOD, superoxide dismutase; TUNEL, TdT-mediated dUTP Nick-end labeling; VEGF, vascular endothelial growth factor.
Balaiya, S., Abu-Amero, K. K., Kondkar, A. A., and Chalam, K. V. (2017). Sirtuins expression and their role in retinal diseases. Oxid. Med. Cell. Longev. 2017:3187594. doi: 10.1155/2017/3187594
Balmer, J., Zulliger, R., Roberti, S., and Enzmann, V. (2015). Retinal cell death caused by sodium iodate involves multiple caspase-dependent and caspase-independent cell-death pathways. Int. J. Mol. Sci. 16, 15086–15103. doi: 10.3390/ijms160715086
Berkowitz, B. A., Podolsky, R. H., Lenning, J., Khetarpal, N., Tran, C., Wu, J. Y., et al. (2017). Sodium iodate produces a strain-dependent retinal oxidative stress response measured in vivo using quest mri. Invest. Ophthalmol. Vis. Sci. 58, 3286–3293. doi: 10.1167/iovs.17-21850
Bjørklund, G., Dadar, M., Martins, N., Chirumbolo, S., Goh, B. H., Smetania, K., et al. (2018). Brief challenges on medicinal plants: an eye-opening look at ageing-related disorders. Basic Clin. Pharmacol. Toxicol. 122, 539–558. doi: 10.1111/bcpt.12972
Brunet, A., Sweeney, L. B., Sturgill, J. F., Chua, K. F., Greer, P. L., Lin, Y., et al. (2004). Stress-dependent regulation of FOXO transcription factors by the SIRT1 deacetylase. Science 303, 2011–2015. doi: 10.1126/science.1094637
Chen, K., Wang, N., Diao, Y., Dong, W., Sun, Y., Liu, L., et al. (2017). Hydrogen-rich saline attenuates brain injury induced by cardiopulmonary bypass and inhibits microvascular endothelial cell apoptosis via the PI3K/Akt/GSK3β signaling pathway in rats. Cell. Physiol. Biochem. 43, 1634–1647. doi: 10.1159/000484024
Chen, T., Tao, Y., Yan, W., Yang, G., Chen, X., Cao, R., et al. (2016). Protective effects of hydrogen-rich saline against N-methyl-N-nitrosourea-induced photoreceptor degeneration. Exp. Eye Res. 148, 65–73. doi: 10.1016/j.exer.2016.05.017
Chowers, G., Cohen, M., Marks-Ohana, D., Stika, S., Eijzenberg, A., Banin, E., et al. (2017). Course of sodium iodate-induced retinal degeneration in albino and pigmented mice. Invest. Ophthalmol. Vis. Sci. 58, 2239–2249. doi: 10.1167/iovs.16-21255
Chung, Y. R., Choi, J. A., Koh, J. Y., and Yoon, Y. H. (2017). Ursodeoxycholic acid attenuates endoplasmic reticulum stress-related retinal pericyte loss in streptozotocin-induced diabetic mice. J. Diadetes Res. 2017:1763292. doi: 10.1155/2017/1763292
Du, H., Sheng, M., Wu, L., Zhang, Y., Shi, D., Weng, Y., et al. (2016). Hydrogen-rich saline attenuates acute kidney injury after liver transplantation via activating p53-mediated autophagy. Transplantation 100, 563–570. doi: 10.1097/TP.0000000000001052
Ge, L., Wei, L. H., Du, C. Q., Song, G. H., Xue, Y. Z., Shi, H. S., et al. (2017). Hydrogen-rich saline attenuates spinal cord hemisection-induced testicular injury in rats. Oncotarget 8, 42314–42331. doi: 10.18632/oncotarget.15876
Gu, X., Cai, Z., Cai, M., Liu, K., Liu, D., Zhang, Q., et al. (2018). AMPK/SIRT1/p38 MAPK signaling pathway regulates alcohol-induced neurodegeneration by resveratrol. Mol. Med. Rep. 17, 5402–5408. doi: 10.3892/mmr.2018.8482
Guo, J., Dong, W., Jin, L., Wang, P., Hou, Z., and Zhang, Y. (2017). Hydrogen rich saline prevents bone loss in diabetic rats induced by streptozotocin. Int. Orthop. 41, 2119–2128. doi: 10.1007/s00264-017-3581-4
Han, C., Gu, Y., Shan, H., Mi, W., Sun, J., Shi, M., et al. (2017). O-GlcNAcylation of SIRT1 enhances its deacetylase activity and promotes cytoprotection under stress. Nat. Commun. 8:1491. doi: 10.1038/s41467-017-01654-6
He, X., Wang, S. Y., Yin, C. H., Wang, T., Jia, C. W., and Ma, Y. M. (2016). Hydrogen-rich water exerting a protective effect on ovarian reserve function in a mouse model of immune premature ovarian failure induced by zona pellucida 3. Chin. Med. J. 129, 2331–2337. doi: 10.4103/0366-6999.190668
He, X., Wu, C., Cui, Y., Zhu, H., Gao, Z., Li, B., et al. (2017). The aldehyde group of gossypol induces mitochondrial apoptosis via ROS-SIRT1-p53-PUMA pathway in male germline stem cell. Oncotarget 8, 100128–100140. doi: 10.18632/oncotarget.22044
Hernández-Zimbrón, L. F., Zamora-Alvarado, R., Ochoa-De la Paz, L., Velez-Montoya, R., Zenteno, E., Gulias-Cañizo, R., et al. (2018). Age-related macular degeneration; new paradigms for treatment and management of AMD. Oxid. Med. Cell. Longev. 2018:8374647. doi: 10.1155/2018/8374647
Holz, F. G., Schmitz-Valckenberg, S., and Fleckenstein, M. (2014). Recent developments in the treatment of age-related macular degeneration. J. Clin. Invest. 124, 1430–1438. doi: 10.1172/JCI71029
Hou, M., Zuo, X., Li, C., Zhang, Y., and Teng, Y. (2017). Mir-29b regulates oxidative stress by targeting SIRT1 in ovarian cancer cells. Cell. Physiol. Biochem. 43, 1767–1776. doi: 10.1159/000484063
Jaliffa, C., Ameqrane, I., Dansault, A., Leemput, J., Vieira, V., Lacassagne, E., et al. (2009). Sirt1 involvement in rd10 mouse retinal degeneration. Invest. Ophthalmol. Vis. Sci. 50, 3562–3572. doi: 10.1167/iovs.08-2817
Kannan, R., and Hinton, D. R. (2014). Sodium iodate induced retinal degeneration: new insights from an old model. Neural Regen. Res. 9, 2044–2045. doi: 10.4103/1673-5374.147927
Kurokawa, R., Seo, T., Sato, B., Hirano, S., and Sato, F. (2015). Convenient methods for ingestion of molecular hydrogen: drinking, injection, and inhalation. Med. Gas Res. 5:13. doi: 10.1186/s13618-015-0034-2
Li, J., Zhao, L., Urabe, G., Fu, Y., and Guo, L. W. (2017a). Epigenetic intervention with a BET inhibitor ameliorates acute retinal ganglion cell death in mice. Mol. Vis. 23, 149–159.
Li, X. N., Chen, L., Luo, B., Li, X., Wang, C. Y., Zou, W., et al. (2017b). Hydrogen sulfide attenuates chronic restrain stress-induced cognitive impairment by upreglulation of Sirt1 in hippocampus. Oncotarget 8, 100396–100410. doi: 10.18632/oncotarget.22237
Lin, C. P., Chuang, W. C., Lu, F. J., and Chen, C. Y. (2017). Anti-oxidant and anti-inflammatory effects of hydrogen-rich water alleviate ethanol-induced fatty liver in mice. World J. Gastroenterol. 23, 4920–4934. doi: 10.3748/wjg.v23.i27.4920
Liu, F. T., Xu, S. M., Xiang, Z. H., Li, X. N., Li, J., Yuan, H. B., et al. (2014). Molecular hydrogen suppresses reactive astrogliosis related to oxidative injury during spinal cord injury in rats. CNS Neurosci. Ther. 20, 778–786. doi: 10.1111/cns.12258
Liu, S., Yang, H., Hu, B., and Zhang, M. (2017). Sirt1 regulates apoptosis and extracellular matrix degradation in resveratrol-treated osteoarthritis chondrocytes via the Wnt/β-catenin signaling pathways. Exp. Ther. Med. 14, 5057–5062. doi: 10.3892/etm.2017.5165
Liu, Z., Geng, W., Jiang, C., Zhao, S., Liu, Y., Zhang, Y., et al. (2017). Hydrogen-rich saline inhibits tobacco smoke-induced chronic obstructive pulmonary disease by alleviating airway inflammation and mucus hypersecretion in rats. Exp. Biol. Med. 242, 1534–1541. doi: 10.1177/1535370217725249
Luo, J., Nikolaev, A. Y., Imai, S., Chen, D., Su, F., Shiloh, A., et al. (2001). Negative control of p53 by Sir2alpha promotes cell survival under stress. Cell 107, 137–148. doi: 10.1016/S0092-8674(01)00524-4
Mitter, S. K., Song, C., Qi, X., Mao, H., Rao, H., Akin, D., et al. (2014). Dysregulated autophagy in the RPE is associated with increased susceptibility to oxidative stress and AMD. Autophagy 10, 1989–2005. doi: 10.4161/auto.36184
Mizuno, K., Sasaki, A. T., Ebisu, K., Tajima, K., Kajimoto, O., Nojima, J., et al. (2018). Hydrogen-rich water for improvements of mood, anxiety, and autonomic nerve function in daily life. Med. Gas Res. 7, 247–255. doi: 10.4103/2045-9912.222448
Morigi, M., Perico, L., Rota, C., Longaretti, L., Conti, S., Rottoli, D., et al. (2015). Sirtuin 3-dependent mitochondrial dynamic improvements protect against acute kidney injury. J. Clin. Invest. 125, 715–726. doi: 10.1172/JCI77632
Nakao, A., Toyoda, Y., Sharma, P., Evans, M., and Guthrie, N. (2010). Effectiveness of hydrogen rich water on antioxidant status of subjects with potential metabolic syndrome: an open label pilot study. J. Clin. Biochem. Nutr. 46, 140–149. doi: 10.3164/jcbn.09-100
Pennington, K. L., and DeAngelis, M. M. (2016). Epidemiology of age-related macular degeneration (AMD): associations with cardiovascular disease phenotypes and lipid factors. Eye Vis. 3:34. doi: 10.1186/s40662-016-0063-5
Qi, L. S., Yao, L., Liu, W., Duan, W. X., Wang, B., Zhang, L., et al. (2015). Sirtuin type 1 mediates the retinal protective effect of hydrogen-rich saline against light-induced damage in rats. Invest. Ophthalmol. Vis. Sci. 56, 8268–8279. doi: 10.1167/iovs.15-17034
Rakoczy, E. P. (2017). Gene therapy for the long terms treatment of wet AMD. Lancet 390, 6–7. doi: 10.1016/S0140-6736(17)31262-X
Shao, A., Wu, H., Hong, Y., Tu, S., Sun, X., Hu, Q., et al. (2016). Hydrogen-rich saline attenuated subarachnoid hemorrhage-induced early brain injury in rats by suppressing inflammatory response: possible involvement of NF-κB pathway and NLRP3 inflammasome. Mol. Neurobiol. 53, 3462–3476. doi: 10.1007/s12035-015-9242-y
Song, H., Vijayasarathy, C., Zeng, Y., Marangoni, D., Bush, R. A., Wu, Z., et al. (2016). NADPH Oxidase contributes to photoreceptor degeneration in constitutively active rake mice. Invest. Ophthalmol. Vis. Sci. 57, 2864–2875. doi: 10.1167/iovs.15-18974
Sun, H., Chen, L., Zhou, W., Hu, L., Li, L., Tu, Q., et al. (2011). The protective role of hydrogen-rich saline in experimental liver injury in mice. J. Hepatol. 54, 471–480. doi: 10.1016/j.jhep.2010.08.011
Syed, B. A., Evans, J. B., and Bielory, L. (2012). Wet AMD market. Nat. Rev. Drug Discov. 11:827. doi: 10.1038/nrd3790
Takeuchi, S., Nagatani, K., Otani, N., Nawashiro, H., Sugawara, T., Wada, K., et al. (2015). Hydrogen improves neurological function through attenuation of blood–brain barrier disruption in spontaneously hypertensive stroke-prone rats. BMC Neurosci. 16:22. doi: 10.1186/s12868-015-0165-3
Tao, X., Chen, L., Cai, L., Ge, S., and Deng, X. (2017). Regulatory effects of the AMPKα-SIRT1 molecular pathway on insulin resistance in PCOS mice: an in vitro and in vivo study. Biochem. Biophys. Res. Commun. 494, 615–620. doi: 10.1016/j.bbrc.2017.09.154
Tian, L., Zhang, L., Xia, F., An, J., Sugita, Y., and Zhang, Z. (2013). Hydrogen-rich saline ameliorates the retina against light-induced damage in rats. Med. Gas Res. 3:19. doi: 10.1186/2045-9912-3-19
Tissenbaum, H. A., and Guarente, L. (2001). Increased dosage of a sir-2 gene extends lifespan in caenorhabditis elegans. Nature 410, 227–230. doi: 10.1038/35065638
Wang, J., Iacovelli, J., Spencer, C., and Saint-Geniez, M. (2014). Direct effect of sodium iodate on neurosensory retina. Invest. Ophthalmol. Vis. Sci. 55, 1941–1953. doi: 10.1167/iovs.13-13075
Wang, Y., Liu, Y., Liu, X., Jiang, L., Yang, G., Sun, X., et al. (2015). Citreoviridin induces autophagy-dependent apoptosis through lysosomal -mitochondrial axis in human liver HepG2 cells. Toxins 7, 3030–3044. doi: 10.3390/toxins7083030
Wu, M. J., Chen, M., Sang, S., Hou, L. L., Tian, M. L., Li, K., et al. (2017). Protective effects of hydrogen rich water on the intestinal ischemia/reperfusion injury due to intestinal intussusception in a rat model. Med. Gas Res. 7, 101–106. doi: 10.4103/2045-9912.208515
Xie, K., Yu, Y., Huang, Y., Zheng, L., Li, J., Chen, H., et al. (2012). Molecular hydrogen ameliorates lipopolysaccharide-induced acute lung injury in mice through reducing inflammation and apoptosis. Shock 37, 548–555. doi: 10.1097/SHK.0b013e31824ddc81
Xin, H. G., Zhang, B. B., Wu, Z. Q., Hang, X. F., Hu, X. S., Ni, W., et al. (2014). Consumption of hydrogen-rich water alleviates renal injury in spontaneous hypertensive rats. Mol. Cell. Biochem. 392, 117–124. doi: 10.1007/s11010-014-2024-4
Yang, X. H., Ren, L. S., Wang, G. P., Zhao, L. L., Zhang, H., Mi, Z. G., et al. (2012). A new method of establishing orthotopic bladder transplantable tumor in mice. Cancer Biol. Med. 9, 261–265. doi: 10.7497/j.issn.2095-3941.2012.04.007
Yang, Y., Duan, W., Liu, Y., Yi, W., Liang, Z., Yan, J., et al. (2013). SIRT1 activation by curcumin pretreatment attenuates mitochondrial oxidative damage induced by myocardial ischemia reperfusion injury. Free Radic. Biol. Med. 65, 667–679. doi: 10.1016/j.freeradbiomed.2013.07.007
Yao, H., Yao, Z., Zhang, S., Zhang, W., and Zhou, W. (2018). Upregulation of SIRT1 inhibits H2O2-induced osteoblast apoptosis via FoxO1/β-catenin pathway. Mol. Med. Rep. 17, 6681–6690. doi: 10.3892/mmr.2018.8657
Zeng, Y., and Yang, K. (2015). Sirtuin 1 participates in the process of age-related retinal degeneration. Biochem. Biophys. Res. Commun. 468, 167–172. doi: 10.1016/j.bbrc.2015.10.139
Zeng, Y., Yang, K., Wang, F., Zhou, L., Hu, Y., Tang, M., et al. (2016). The glucagon like peptide 1 analogue, Exendin-4, attenuates oxidative stress-induced retinal cell death in early diabetic rats through promoting Sirt1 and Sirt3 expression. Exp. Eye Res. 151, 203–211. doi: 10.1016/j.exer.2016.05.002
Zheng, T., and Lu, Y. (2016). SIRT1 protects human lens epithelial cells against oxidative stress by inhibiting p53-dependent apoptosis. Curr. Eye Res. 41, 1068–1075. doi: 10.3109/02713683.2015.1093641
Zhou, P., Kannan, R., Spee, C., Sreekumar, P. G., Dou, G., and Hinton, D. R. (2014). Protection of retina by αB crystallin in sodium iodate induced retinal degeneration. PLoS One 9:e98275. doi: 10.1371/journal.pone.0098275
Keywords: hydrogen, sirt1, oxidative stress, apoptosis, AMD
Citation: Liu Y, Li R, Xie J, Hu J, Huang X, Ren F and Li L (2018) Protective Effect of Hydrogen on Sodium Iodate-Induced Age-Related Macular Degeneration in Mice. Front. Aging Neurosci. 10:389. doi: 10.3389/fnagi.2018.00389
Received: 01 August 2018; Accepted: 06 November 2018;
Published: 04 December 2018.
Edited by:
Walter E. Müller, Goethe-Universität Frankfurt am Main, GermanyReviewed by:
Xue-Jun Sun, Second Military Medical University, ChinaCopyright © 2018 Liu, Li, Xie, Hu, Huang, Ren and Li. This is an open-access article distributed under the terms of the Creative Commons Attribution License (CC BY). The use, distribution or reproduction in other forums is permitted, provided the original author(s) and the copyright owner(s) are credited and that the original publication in this journal is cited, in accordance with accepted academic practice. No use, distribution or reproduction is permitted which does not comply with these terms.
*Correspondence: Lihua Li, bGlsaWh1YTEwMThAc2luYS5jb20=
Disclaimer: All claims expressed in this article are solely those of the authors and do not necessarily represent those of their affiliated organizations, or those of the publisher, the editors and the reviewers. Any product that may be evaluated in this article or claim that may be made by its manufacturer is not guaranteed or endorsed by the publisher.
Research integrity at Frontiers
Learn more about the work of our research integrity team to safeguard the quality of each article we publish.