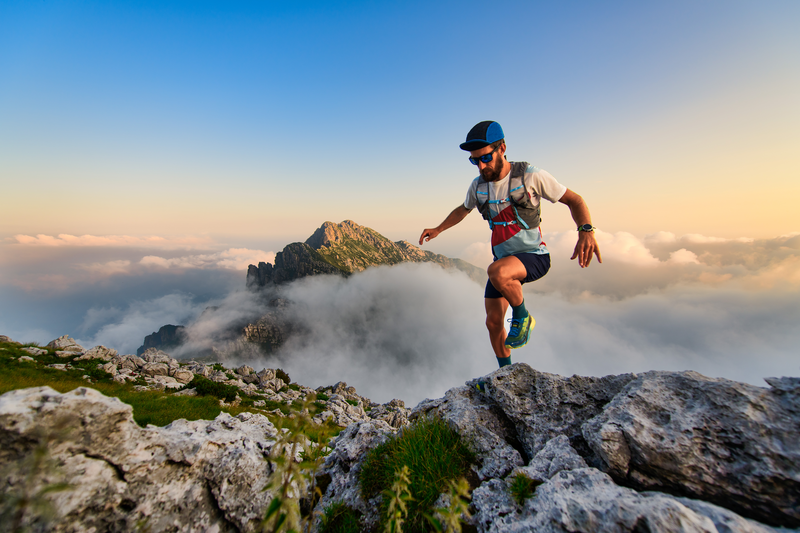
95% of researchers rate our articles as excellent or good
Learn more about the work of our research integrity team to safeguard the quality of each article we publish.
Find out more
ORIGINAL RESEARCH article
Front. Aging Neurosci. , 06 November 2018
Sec. Alzheimer's Disease and Related Dementias
Volume 10 - 2018 | https://doi.org/10.3389/fnagi.2018.00363
Throughout life, stress stimuli act upon the brain leading to morphological and functional changes in advanced age, when it is likely to develop neurodegenerative disorders. There is an increasing need to unveil the molecular mechanisms underlying aging, in a world where populations are getting older. Egr-1 (early growth response 1), a transcriptional factor involved in cell survival, proliferation and differentiation – with a role also in memory, cognition and synaptic plasticity, can be implicated in the molecular mechanism of the aging process. Moreover, Heme Oxygenase-1a (HO), a 32 kDa heat-shock protein that converts heme to iron, carbon monoxide and biliverdin, is a key enzyme with neuroprotective properties. Several in vitro and in vivo studies reported that HO-1 could regulate the metabolism of oxysterols, oxidation products of cholesterol that include markers of oxidative stress. Recently, a link between Egr-1 and HO-1 has been demonstrated in mouse lung cells exposed to cigarette smoke. In view of these data, we wanted to investigate whether Egr-1 can be implicated also in the oxysterol metabolism during brain aging. Our results show that Egr-1 expression is differently expressed in the cortex and hippocampus of old mice, as well as the oxysterol profile between these two brain areas. In particular, we show that the cortex experiences in an age-dependent fashion increasing levels of the Egr-1 protein, and that these correlate with the level of HO-1 expression and oxysterol abundance. Such a situation was not observed in the hippocampus. These results are further strenghtened by our observations made with Egr-1 KO mice, confirming our hypothesis concerning the influence of Egr-1 on oxysterol production and accumulation via regulation of the expression of HO-1 in the cortex, but not the hippocampus, of old mice. It is important to notice that most of the oxysterols involved in this process are those usually stimulated by oxidative stress, which would then represent the triggering factor for this mechanism.
Thanks to the efforts made in the past half century in preventing diseases and improving patient conditions, the world population is becoming older. This has certainly been a conquer for human kind in general, but it also raises the question of whether we have for the future to treat aging as a disease factor. In fact, age is a recognized risk factor for adult-onset neurodegenerative processes, including Alzheimer (AD) and Parkinson diseases (PD) (Reitz et al., 2011; Reeve et al., 2014). How aging affects from the molecular point of view the development of neurodegenerative diseases still remains largely undefined.
Numerous studies have recognized Egr-1 as a gene influenced by the aging process (Yau et al., 1996; Desjardins et al., 1997; Blalock et al., 2003; Marrone et al., 2011). Egr-1 is an immediate early gene coding for a zinc finger transcriptional factor involved in cell differentiation, proliferation and survival. Egr-1 expression, which is almost undetectable in mouse embryo nervous system (McMahon et al., 1990), appears and slowly increases in postnatal rat brains reaching consistent levels by day 17 and from that it mantains baseline expression levels until adulthood (Watson and Milbrandt, 1990; Beckmann and Wilce, 1997). In the nervous system, this gene plays an important role in memory and cognition, which are both affected in advanced aging. Relevant changes in Egr-1 expression are observed in rat brain during cortical and hippocampal synaptic formation (Herms et al., 1994), favoring the hypothesis of an involvement of Egr-1 in the regulation of plastic adaptation. In agreement, mice lacking Egr-1 exhibit defects in durable long term potentiation (LTP) and memory (Jones et al., 2001), and Egr-1 is over-expressed in brains of AD patients and AD mouse models (Bakalash et al., 2011; Hendrickx et al., 2013; Gatta et al., 2014). Moreover, Egr-1 is involved in the phosphorylation of tau protein as shown in experiments of Egr-1 deletion and over-expression (Lu et al., 2011). Finally, Egr-1 is rapidly induced by stress stimuli such as hypoxia, OS, brain injury, ischemic stroke, and neuroinflammation (Beckmann et al., 1997; Yan et al., 2000; Khachigian, 2006). OS plays an etiologic role in brain aging, neurodegenerative and cardiovascular diseases, and cancer (Thanan et al., 2014). In this context, we focused our attention on HO-1, a 32 kDa heat-shock protein considered as a new player in aging and neurodegenerative diseses (Chen et al., 2003; Parfenova et al., 2012). In response to OS in the brain, HO-1 is induced in neuronal and glial cells, the greater HO-1 levels being observed within the astrocyte compartment (Manganaro et al., 1995; Snyder et al., 1998). By converting heme to iron, carbon monoxide and biliverdin (Chen and Regan, 2005), HO-1 protects astrocytes against the heme-mediated oxidative injury. On the other hand, HO-1 can also have neurotoxic effects depending on the balance between the free radical scavenging properties of the produced biliverdin/bilirubin (Doré et al., 1999) and the aggravated intracellular OS resulting from the free iron formation (Zhang and Piantadosi, 1992). HO-1 expression has not been rigourously localized in the human brain. In normal conditions, HO-1 is scanty present in neuroglia and in some neuron sub-populations from various brain areas, including cortex and hippocampus (Vincent et al., 1994; Nakaso et al., 2000).
Since the brain is the organ with the highest content of cholesterol (approximately 20% of total body cholesterol content), the role of sterols homeostasis and in particular cholesterol metabolism, is of primary importance in the study of aging related disorders. An interaction of HO-1 induction with sterol regulation was reported both in in vitro and in vivo studies (Vaya et al., 2007; Hascalovici et al., 2009, 2014). The aged and neurodegenerated brain is constantly exposed to OS, which leads to lipid peroxidation and to the generation of oxysterols (Björkhem et al., 2002), oxygenated forms of cholesterol arising also from OS (Iuliano, 2011). Oxysterols are able to induce cytotoxicity and inflammation, worsening the neurodegeneration process (Vaya and Schipper, 2007). Certain oxysterols are used to control brain cholesterol levels, which is synthesized in situ and unable to cross the blood brain barrier. A net flux of 24-HC from the brain to the systemic circulation serves to export cholesterol from the brain (Crick et al., 2015; Iuliano et al., 2015).
To prevent accumulation in the brain, cholesterol is oxidized by the cytochrome p450 enzymes. During aging, it is the balanced production of cholesterol and oxysterols to provide the basis for oxysterol homeostasis in the brain. When this equilibrium is perturbated, the accumulation of oxysterols in the brain can be toxic. In AD patients the level of inflammatory molecules and the expression of HO-1 were related to changes in oxysterols profile and disease progression (Testa et al., 2016). A link between Egr-1 and HO-1 was reported in mouse lung cells exposed to cigarettes smoke (Chen et al., 2010). With this work we have advanced the hypothesis of a contribution of Egr-1 in the regulation of oxysterol production in the aging brain. To test our hypothesis, we studied the expression of Egr-1 and HO-1 in the cortex and hippocampus of young, adult and aged WT and Egr-1 KO mice, and measured the amount of oxysterols at tissue level. Our results are consistent with the following outcome: Egr-1 regulates oxysterols production by controlling the expression of HO-1. This association is clearly present in the brain cortex but not in the hippocampus, and it seems operative in an age-dependent manner.
Male C57BL/6 WT and Egr-1 KO mice aged 3, 12, and 24 months were used (n = 6 animals per strain and age). Animals were housed 3 to 4 per cage, maintained under standard temperature conditions (22°C ± 2°C) and 12-h:12-h light:dark cycles. Throughout the study, they had access to food and water ad libitum. All the experiments were performed in accordance with the EU Directive 2010/63/EU for animal experiments and approved by ethic committee of Department of Medico-Surgical Sciences and Biotechnologies, University of Roma “Sapienza”.
For Western blot analysis and oxysterols determinations, mice were sacrificed by cervical dislocation and the brains were immediately removed and washed in ice cold Phosphate Buffer Saline (PBS). Afterward, brains were divided into two emispheres practicing a sagittal cut and both brain cortices and hippocampi were accurately dissected, frozen on powdered dry ice, and maintained at -80°C until protein extraction and oxysterols analysis.
For immunohistochemistry analysis, the whole brain was extracted, washed in ice cold PBS and fixed for 24h at 4°C with Carnoy’s solution. Afterward, brains were mantained in 70% ethanol untill the paraffin-embending process.
Immunohistochemical analysis was conducted as previously described by our research group (Rosa et al., 2017), with some modifications. In brief, paraffin-embedded tissues were deparaffinized, rehydrated in descending graded alcohols, incubated for 15 min in methanol containing 3% H2O2 to block endogenous peroxidase activity, and then subjected to microwave antigen retrieval for 30 min in sodium citrate buffer (10 mM tri-sodium citrate dihydrate, 0.05% Tween-20, pH 6.0). After preincubation in Super Block (ScyTek Laboratories, Logan, UT, United States) for 10 min, sections were incubated overnight with rabbit polyclonal anti-Egr-1 antibody (sc-110, Santa Cruz Biotechnology, Dallas, TX, United States, dilution 1:100) or rabbit polyclonal anti- HO-1 antibody (HO-1, sc-10789, Santa Cruz Biotechnology, dilution 1:100) at 4°C in humid chamber, washed with PBS, incubated for 10 min at room temperature with UltraTek Anti-Polyvalent (ScyTek Laboratories), washed with PBS and then incubated with the UltraTek HRP (ScyTek Laboratories) according to the manufacturer’s instructions. The sections were then stained with 3-3-diaminobenzidine (ScyTek Laboratories) as chromogen to visualize the reaction product, and were finally counterstained with hematoxylin. Images were acquired with Nikon Eclipse Ni motorized microscope system at 10× and 40× magnification.
Total protein extraction was performed homogenizing cortical and hippocampal samples with a Teflon-glass potter in RIPA buffer (50 mM Tris–HCl pH 8.0, 150 mM NaCl, 1% Nonidet P-40, 1 mM EDTA, 0.5% sodium deoxycholate, 0.1% SDS) with protease inhibitors, 1 mM PMSF, 1mM DTT, and 0.5 mM sodium orthovanadate (Sigma–Aldrich, St. Louis, MO, United States). Protein concentration was determined by the Bradford assay (Bio-Rad, Hercules, CA, United States).
Western blot analysis of brain extracts was carried out as previously described (Ponti et al., 2015), with some modifications. In brief, 40 μg of proteins per sample were resolved on a 10% SDS–PAGE gel and blotted onto a PVDF membrane (Amersham HyBond-P GE Healthcare, Chicago, IL, United States). After blocking at room temperature in 5% dry-milk in PBS containing 0.1% Tween-20 for 1 h, membranes were incubated overnight at 4°C with rabbit polyclonal anti-heme oxygenase 1 (HO-1, sc-10789, Santa Cruz Biotechnology, dilution 1:500), rabbit polyclonal anti-Egr-1 (sc-110, Santa Cruz Biotechnology, dilution 1:500), rabbit polyclonal anti-ERK1/2 (9102, Cell Signaling Technology, Danvers, MA, United States dilution 1:1000), mouse monoclonal anti-phospho-ERK1/2 (p-ERK1/2, 9106, Cell Signaling Technology, dilution 1:1000) and mouse monoclonal anti-β-actin (sc-47778, Santa Cruz Biotechnology, dilution 1:2000) antibody was used for normalization. Membranes were then incubated with anti-rabbit and anti-mouse horseradish peroxidase conjugated secondary antibodies (dilution 1:10000, GE Healthcare Bio-Sciences, Piscataway, NJ, United States). Immunocomplexes were detected by ECL Western Blotting detection system (GE Healthcare Bio-Sciences). Digital images were acquired using a ChemiDoc XRS + System (BioRad). Band intensities were quantified by densitometric analysis using Image Lab software (BioRad), and values were normalized to β-actin.
Cortical and hippocampal samples from 12 to 18 months old WT and Egr-1 KO mice (n = 6 for each group) were used for oxysterols determinations. In brief, oxysterols were measured by isotope dilution mass spectrometry, essentially as described elsewhere (Iuliano et al., 2003) with the exception of the solid phase extraction (SPE) step, which was repeated twice to eliminate cholesterol. Cholesterol discarded by elution from SPE was measured by isotope dilution mass spectrometry such as oxysterols. Analyses were run on an Agilent 6890N gas chromatograph equipped with a 7683 series automatic liquid sampler, and interfaced with an Agilent 5973 mass spectrometer (Agilent Technologies, Palo Alto, CA, United States). Separation was achieved on a 30 m capillary column (HP-5MS 30 0.25 mm ID, 0.25 mm thickness). The mass spectrometer was set to the selected ion monitoring mode; the molecules were monitored with ions at mass/charge ratio (m/z): 463 m/z for [2H7]-27-HC, [2H7]-24-HC, [2H7]-7α-HC, [2H7]-7β-HC, [2H7]-4α-HC, [2H7]-4β-HC and [2H7]-cholesterol; 456 m/z for [2H0]-27-HC, [2H0]-24-HC, [2H0]- 7α-HC, [2H0]-7β-HC, [2H0]-4α- hydroxycholesterol (4α-HC), [2H0]-4β-HC and [2H0]-cholesterol; 481 m/z for [2H7]-5α,6α-EC, and [2H7]-5β,6β-EC; 474 m/z for [2H0]-5α,6α-EC, and [2H0]-5β,6β-EC; 137 m/z for [2H6]-25-HC, and 131 m/ z for [2H0]-25-HC; 479 m/z for [2H7]-7-KC, and 472 m/z for [2H0]-7-KC; 478 m/z for [2H6]-5α-OH,6-oxocholesterol and 472 m/z for [2H0]-5α-OH,6-oxocholesterol (5α-OH,6-oxo-CH); 546 m/z for [2H7]-CT, and 552 m/ z for [2H0]-CT; 189 m/z for [2H7]- 22-HC and 181 m/z for [2H0]- 22-HC. 5α-OH,6-oxo-CH, CT and theirs deuterated were synthesized and kindly donated by Dr. Marc Poirot (Institut Claudius Régaud, Toulouse, France). 4α-HC, 4β-HC and theirs deuterated were kindly donated by Dr. G. Lizard (Faculté des Sciences Gabriel, Dijon, France). All other oxysterols and deuterated oxysterols were from Avanti Polar Lipids (Alabaster, AL, United States). Quantification of oxysterols was assessed by the internal standard ratio method.
All statistical analyses were performed using GraphPad Prism software (La Jolla, CA, United States). Western blot results are expressed as the mean ± standard deviation (SD) and statistical comparisons were performed by Student’s t-test and one-way analysis of variance (ANOVA). P-values < 0.05 were considered significant.
Among the brain areas involved in memory and cognition, cortex and hippocampus are exposed to morphological and functional changes as age advances. First of all we wanted to compare the pattern of expression of Egr-1 and HO-1 in cortex and hippocampus of adult WT mice. They were stained by immunohistochemistry on paraffin-embedded sections. Figure 1 shows Egr-1 in the brain cortex with its typical nuclear localization, and in the hippocampus within the pyramidal neurons of CA (CA1-CA2-CA3-CA4) but not in the DG. On the other hand, a weak presence of HO-1 was detected in the cortex and hippocampus areas, mainly around vessels.
FIGURE 1. Early growth response-1 and HO-1 expression in adult wild-type mice cortex and hippocampus. Immunohistochemical analysis showing representative brain sections from cortex (left images) and hippocampus (right images) of adult wild-type (WT) mice stained with anti-Egr-1 (upper images) and anti-HO-1 (bottom images) antibodies. Black arrows indicate strongly positive signals. Images: 10× magnification, scale bars: 100 μm; inside squares: 40× magnification, scale bars: 20 μm.
Extensive OS and oxysterol production are emerging components of the neurodegenerative processes involved in brain aging (Sottero et al., 2009). In order to get an oxysterol profile for the cortex and hippocampus of adult WT mice, we investigated the production of 13 different oxysterols. As shown in Figure 2, the oxysterols included in our panel were five of non-enzyimatic origin (7-KC, 7β-HC, 5α-OH,6-oxo-CH, 5α,6α-EC, and 5β,6β-EC), five of enzimatic origin (4β-HC, 4α-HC, 24-HC, 27-HC, and 22-HC), and three of both enzymatic and non-enzymatic origin (25-HC, CT and 7α-HC). The cortex and hippocampus showed distinct oxysterol profiles. The hippocampus was characterized by higher levels of oxysterol 7α-HC, 7β-HC, 7-KC, 5α,6α-EC, 5β,6β-EC, CT, 5α-OH,6-oxo-CH and 22-HC, whereas the cortex had higher levels of 24-HC, 25-HC and 27-HC. 4α-HC and 4β-HC were similarly distributed between cortex and hippocampus. Cholesterol levels (Supplementary Figure S1) measured in the same regions did not result in significant difference in the two brain areas of both WT and KO mice, either at 12 and 18 months of age.
FIGURE 2. Different oxysterols profile between adult wild-type mice cortex and hippocampus. Isotope dilution mass spectrometry analysis showing oxysterols levels in 12 months WT mice cortex (Cx, black bars) and hippocampus (Hp, gray bars) expressed as ng/mg of tissue. 7α-hydroxycholesterol (7α-HC), 7β-hydroxycholesterol (7β-HC), 7-ketocholesterol (7-KC), 5α,6α-epoxycholesterol (5α,6α-EC), 5β,6β-epoxycholesterol (5β,6β-EC), 4α-hydroxycholesterol (4α-HC), 4β-hydroxycholesterol (4β-HC), 5α-cholestane-3β,5,6β-triol (CT), 5α-OH,6-5α-OH,6-oxocholesterol (5α-OH,6-oxo-CH), 24-hydroxycholesterol (24-HC), 25-hydroxycholesterol (25-HC), 27-hydroxycholesterol (27-HC), 22-hydroxycholesterol (22-HC). Results are presented as the mean ± standard deviation (n = 6). ∗p < 0.05; ∗∗p < 0.01; ∗∗∗p < 0.001.
In order to advance the hypothesis of whether Egr-1 is involved in oxysterol metabolism changes in brain aging, we checked the levels of Egr-1 protein expression by western blot in the brain cortex (Figure 3A) and hippocampus (Figure 3B) of young (3 months), adult (12 months) and aged (24 months) WT mice. Egr-1 levels were found to increase 1.4 folds from three to 12 months and 1.8 folds up to 24 months. These changes were observed only in the cortex, suggesting a possible role of Egr-1 for cortex aging.
FIGURE 3. Egr-1 protein expression in the cortex and hippocampus of young, adult and aged wild-type mice. Protein levels of Egr-1 in the cortex (A) and hippocampus (B) of 3, 12 and 24 months old WT mice. The image shows representative western blots (upper panels) and bar graphs (bottom panels) obtained from the relative densitometric analysis, as described in Materials and Methods. Results are presented as the mean ± standard deviation (n = 6). ∗∗∗p < 0.001.
It has been reported that HO-1 is involved in oxysterol metabolism during neurodegeneration (Leoni, 2009; Schipper et al., 2009). It has been also shown that Egr-1 regulates HO-1 expression induced by cigarette smoke in mouse lung cells (Chen et al., 2010). Furthermore, the Egr-1 promoter has been described to be activated by the extracellular signal-regulated kinase (ERK) 1/2, which has been documented to be phosphorylated by many stress stimuli, including OS (Park and Koh, 1999; Hartney et al., 2011; Chen et al., 2012). Based on these observations, we compared by western blot the levels of ERK1/2 phosphorylation (Figures 4A,B) and HO-1 (Figures 4C,D) expression in the cortex of adult (12 months) and aged (18 months) WT (Figures 4A–C) and Egr-1 KO (Figures 4B–D) mice. Our results show that in WT mice the phosphorylation of ERK1/2 and HO-1 expression are the highest in the cortex of 18 months old mice, where also the Egr-1 levels are highest. In contrast, HO-1 expression is significantly reduced in the cortex of age-matched Egr-1 KO mice, although the stronger phosphorylation of ERK1/2. Similar experiments to assay in the hippocampus the link between Egr-1 and HO-1 were performed. No statistically significant differences between the levels of ERK1/2 phosphorylation (Figures 5A,B) and the expression of HO-1 (Figures 5C,D) in the hippocampus of adult and aged WT (Figures 5A–C) and Egr-1 KO mice (Figures 5B–D) were observed.
FIGURE 4. ERK1/2 phosphorylation and HO-1 protein expression in the cortex of adult and aged wild-type and Egr-1 KO mice. ERK1/2, p-ERK1/2 and HO-1protein levels in the cortex of 12 and 18 months old WT (A–C) and Egr-1 KO (B–D) mice. The image shows representative western blots (upper panels) and bar graphs (bottom panels) obtained from the relative densitometric analysis, as described in Materials and Methods. Results are presented as the mean ± standard deviation (n = 6). ∗p < 0.05; ∗∗∗p < 0.001.
FIGURE 5. ERK1/2 phosphorylation and HO-1 protein expression in the hippocampus of adult and aged wild-type and Egr-1 KO mice. ERK1/2, p-ERK1/2 and HO-1 protein levels in the hippocampus of 12 and 18 months old WT (A–C) and Egr-1 KO (B–D) mice. The image shows representative western blots (upper panels) and bar graphs (bottom panels) obtained from the relative densitometric analysis, as described in Materials and Methods. Results are presented as the mean ± standard deviation (n = 6).
Thereafter, we were asking whether the correlation found between the Egr-1 and HO-1 expression levels in the cortex of aging mice were reflected also in a change of oxysterol levels. We then compared the oxysterol levels in the old WT brain cortex with those found in old Egr-1 KO mice brain cortex. Figure 6 shows that in the cortex of old KO mice the oxysterols 7α-HC, 7β-HC; 7-KC, 5α,6α-EC, 5β,6β-EC, CT and 5α-OH,6-oxo-CH were significantly reduced.
FIGURE 6. Oxysterols levels in aged wild-type and Egr-1 KO mice brain cortex. Isotope dilution mass spectrometry analysis showing oxysterols levels in 18 months wild-type (WT, black bars) and Egr-1 knock-out (KO, gray bars) mice cortex (Cx) expressed as ng/mg of tissue. 7α-HC, 7β-HC, 7-KC, 5α,6α-EC, 5β,6β-EC, 4α-HC, 4β-HC, CT, 5α-OH,6-oxo-CH, 24-HC, 25-HC, 27-HC, 22-HC. Results are presented as the mean ± standard deviation (n = 6). ∗p < 0.05; ∗∗p < 0.01; ∗∗∗p < 0.001.
Finally, given that HO-1 expression does not seem, according to our data, to be influenced by Egr-1 in the hippocampus, we asked the question of whether also oxysterol metabolism appeared to gain independence from Egr-1 control in old mice hippocampus. As shown in Figure 7, we haven’t registered any significative change in the content of oxysterols in the hippocampus of aged mice, though 7α-HC, 7β-HC, 7-KC, 5α,6α-EC and 5β,6β-EC showed an upward trend.
FIGURE 7. Oxysterols levels in aged wild-type and Egr-1 KO mice hippocampus. Isotope dilution mass spectrometry analysis showing oxysterols levels in 18 months wild-type (WT, black bars) and Egr-1 knock-out (KO, gray bars) mice hippocampus (Hp) expressed as ng/mg of tissue. 7α-HC, 7β-HC, 7-KC, 5α,6α-EC, 5β,6β-EC, 4α-HC, 4β-HC, CT, 5α-OH,6-oxo-CH, 24-HC, 25-HC, 27-HC, 22-hydroxycholesterol (22-HC). Results are presented as the mean ± standard deviation (n = 6).
In this study about brain aging, we brought to light a new regulatory circuit linking the early transcriptional factor Egr-1 to the brain oxysterol production and accumulation in the elderly. Moreover, Egr-1, likely by regulating the expression of HO-1, could have an influence in the accumulation of oxysterols in the aged brain, specifically in the cortex. The results reported here show the increase in Egr-1, the 32 kDa heat shock protein HO-1 and oxysterol levels in an age-related manner in the mouse cortex. These changes do not occur in the hippocampus area, nor are they observed in the cortex of age-matched Egr-1 KO mice, highlighting the likely role of Egr-1 in this process. Egr-1 is a stress-induced early gene and a transcriptional factor able to regulate the expression of several genes involved in cell survival, death and proliferation. Several studies have established an involvement of Egr-1 in synaptic plasticity and cognitive function, but completely unknown is whether Egr-1 is involved in their natural decline with age as well. On the other hand, Egr-1 is implicated in the development of neurodegenerative disorders, thus becoming a hallmark for such diseases (Koldamova et al., 2014; Minatohara et al., 2015; Duclot and Kabbaj, 2017). In AD brains, Egr-1 is found to be upregulated (Gómez Ravetti et al., 2010), while in aged mice lacking Egr-1 LTP is impaired (Jones et al., 2001). The aging brain is constantly exposed to OS, which triggers and exacerbates brain functional deficit (Uttara et al., 2009). Oxysterols as a form of oxidized cholesterol are implicated in OS, in particular the B-ring oxysterols are generated by autoxidation and by free radical-mediated mechanisms (Iuliano, 2011) and are regulated by HO-1, an enzyme with a dual function that can be protective or toxic depending on its levels and context (Chen, 2014).
Based on the above assumptions about the role of Egr-1 in brain, we wanted to investigate the expression of Egr-1, HO-1 and the levels of oxysterols in the cortex and hippocampus of adult and old mice, i.e., in an age-dependent fashion, to establish whether a link could exist between the levels of Egr-1 and OH-1, and between the level of Egr-1 and the type and amount of oxysterol accumulated, so to infer about and what consequences could a change in these levels of Egr-1 and of HO-1 may have on the accumulation of oxysterols in the same areas. To the purpose of challenging the effect of Egr-1 on the expression of HO-1 and oxysterol accumulation, we gathered data from WT and Egr-1 KO mice and compared the results according to the area of interest (cortex or hippocampus), and the mouse age (12 or 18 months).
Our data highlight some differences in the regulation of Egr-1 protein expression in the mouse brain cortex and hippocampus in the aging brain from WT mice. We found that Egr-1 expression increases in the cortex in an age-dependent fashion. We can only speculate about the cause behind our evidences. They can be likely due to a response to the many stress stimuli, including OS, hypoxia and inflammation that occur in the aged brain. In the hippocampus, however, Egr-1 does not seem to be under the same kind of regulation. In fact, we did not observe any significant change of expression. These data are in agreement with a previous study showing that Egr-1 levels in the brain do not change with age in resting mice, but only when they are exposed to a learning task (Desjardins et al., 1997).
Brain oxysterols are widely accepted markers of OS (Leoni and Caccia, 2011). Several studies have focused on 24-HC and 27-HC, which are produced by enzymatic routes and play an important role in neurodegenerative diseases, Alzheimer’s included (Bjorkhem et al., 2006; Hughes et al., 2013). For the first time to our knowledge, we provided a comprehensive oxysterol profile for the cortex and hippocampus of adult mice. We showed that distribution and abundance of oxysterols was different between cortex and hippocampus, and highlighted that two distinct brain areas, with different functions, have their own oxysterol composition.
Heme oxygenase-1 is considered a key enzyme for oxysterol metabolism (Vaya et al., 2007; Hascalovici et al., 2009). In this context, old mice cortex has increased levels of HO-1 compared to younger mice. This result is in agreement with other studies reporting that HO-1 increases with age, is induced by stress and the high levels correlate with cognitive deficits (Schipper, 1999, 2004; Hirose et al., 2003). Surprisingly, in absence of Egr-1 the expression of HO-1 in the cortex was strongly inhibited in old mice compared to younger KO mice. This result is in line with previous observations, although in mouse lung cells exposed to cigarette smoke, where Egr-1 was able to regulate HO-1 (Chen et al., 2010). Finally, our data showed that this signaling may be sustained by the phosphorylation of ERK1/2 in the old brain cortex, which has already been described to be more activated during aging and to be associated to synaptic dysfunction (Ogundele et al., 2018). Furthermore, in smooth muscle cells Egr-1 was demonstrated to be activated via ERK1/2 under OS conditions (Pagel and Deindl, 2012).
Based on our hypothesis, stimulation of HO-1 in the brain cortex of old mice should result in enhanced oxysterol levels, whereas deletion of Egr-1 should interfere with this process. In fact, old WT mice cortex showed higher levels of oxysterols than the cortex of younger mice but not in the old Egr-1 KO mice which presented much lower oxysterol levels. It is important to notice that the changes concern mainly those oxysterols which have been demonstrated to be induced by OS, i.e., 7α-HC, 7β-HC, 7-KC, 5α,6α-EC and 5β,6β-EC (Iuliano, 2011; Mutemberezi et al., 2016). We suggest that oxysterol production in the aging brain cortex is based on the oxidative induction of Egr-1 via ERK1/2 phosphorylation, which, in turn, activates HO-1 (Figure 8). It would be interesting to explore whether a single or a group of oxysterols may act themselves as a source of OS able to maintain ERK1/2 in a phosphorylated state and Egr-1 levels high.
FIGURE 8. Proposed mechanism for oxysterols production in the old mice brain cortex. High levels of oxidative stress (OS) experienced by the aged mouse brain cause the phosphorylation of ERK1/2, which induce EGR-1 in the cortex. EGR-1, in turn, activates HO-1, which regulates oxysterols metabolism. Specific oxysterols, or a group of them, may maintain and exacerbate the OS within the brain cortex.
Since we have reported the absence of stimulation of Egr-1 and no difference in ERK1/2 phosphorylation in the hippocampus of old mice, we finally wanted to examine whether HO-1 and oxysterols expression was in this case not anymore driven by an Egr-1-dependent regulation in this brain area. Our results confirmed that the HO-1 and oxysterol levels were not significantly different between the hippocampus of adult and of old WT and Egr-1 KO mice, confirming our hypothesis.
In conclusion, our results show that Egr-1 can be stimulated in the cortex of old mice suggesting a role in the oxysterol accumulation by regulating the HO-1 expression. This new function covered by Egr-1 seems to be brain region-specific because it is not present in the hippocampus.
PR conceived and designed the experiments. PR, CZ, and ACr performed the experiments. PR, CZ, A-MC, LI, and GR analyzed the data. ACa, ACo, and LI contributed reagents, materials, and analysis tools. PR, ACo, A-MC, and ACa wrote the paper. LI and GR revised the paper.
The authors declare that the research was conducted in the absence of any commercial or financial relationships that could be construed as a potential conflict of interest.
The Supplementary Material for this article can be found online at: https://www.frontiersin.org/articles/10.3389/fnagi.2018.00363/full#supplementary-material
FIGURE S1 | Cholesterol levels in adult and aged wild-type and Egr-1 KO mice brain cortex and hippocampus. Isotope dilution mass spectrometry analysis showing cholesterol levels in 12 (white bars) and 18 months (black bars) WT and Egr-1 knock-out (KO) mice cortex (Cx) and hippocampus (Hp) expressed as μg/mg of tissue. Results are presented as the mean ± standard deviation (n = 6).
22-HC, 22-hydroxycholesterol; 24-HC, 24-hydroxycholesterol; 25-HC, 25-hydroxycholesterol; 27-HC, 27-hydroxycholesterol; 4α-HC, 4α-hydroxycholesterol; 4β-HC, 4β-hydroxycholesterol; 5α,6α-EC, 5α,6α-epoxycholesterol; 5β,6β-EC, 5β,6β-epoxycholesterol; 5α-OH,6-oxo-CH, 5α-OH,6-5α-OH,6-oxocholesterol; 7α-HC, 7α-hydroxycholesterol; 7β-HC, 7β-hydroxycholesterol; 7-KC, 7-ketocholesterol; CA, Cornu Ammonis; CT, 5α-cholestane-3β,5,6β-triol; Cx, cortex; DG, Dentate Girus; Egr-1, Early growth response-1; HO-1, heme oxygenase-1; Hp, hippocampus; KO, knock-out; OS, oxidative stress; WT, wild-type.
Bakalash, S., Pham, M., Koronyo, Y., Salumbides, B. C., Kramerov, A., Seidenberg, H., et al. (2011). Egr1 expression is induced following glatiramer acetate immunotherapy in rodent models of glaucoma and Alzheimer’s Disease. Investig. Opthalmol. Vis. Sci. 52, 9033–9046. doi: 10.1167/iovs.11-7498
Beckmann, A. M., Davidson, M. S., Goodenough, S., and Wilce, P. A. (1997). Differential expression of Egr-1-like DNA-binding activities in the naive rat brain and after excitatory stimulation. J. Neurochem. 69, 2227–2237. doi: 10.1046/j.1471-4159.1997.69062227.x
Beckmann, A. M., and Wilce, P. A. (1997). Egr transcription factors in the nervous system. Neurochem. Int. 31, 477–510. doi: 10.1016/S0197-0186(96)00136-2
Bjorkhem, I., Heverin, M., Leoni, V., Meaney, S., and Diczfalusy, U. (2006). Oxysterols and Alzheimer’s disease. Acta Neurol. Scand. 114, 43–49. doi: 10.1111/j.1600-0404.2006.00684.x
Björkhem, I., Meaney, S., and Diczfalusy, U. (2002). Oxysterols in human circulation: which role do they have? Curr. Opin. Lipidol. 13, 247–253. doi: 10.1097/00041433-200206000-00003
Blalock, E. M., Chen, K.-C., Sharrow, K., Herman, J. P., Porter, N. M., Foster, T. C., et al. (2003). Gene microarrays in hippocampal aging: statistical profiling identifies novel processes correlated with cognitive impairment. J. Neurosci. 23, 3807–3819. doi: 10.1523/JNEUROSCI.23-09-03807.2003
Chen, C.-A., Chen, T.-S., and Chen, H.-C. (2012). Extracellular signal-regulated kinase plays a proapoptotic role in podocytes after reactive oxygen species treatment and inhibition of integrin–extracellular matrix interaction. Exp. Biol. Med. 237, 777–783. doi: 10.1258/ebm.2012.011157
Chen, H., Wang, L., Gong, T., Yu, Y., Zhu, C., Li, F., et al. (2010). EGR-1 regulates Ho-1 expression induced by cigarette smoke. Biochem. Biophys. Res. Commun. 396, 388–393. doi: 10.1016/j.bbrc.2010.04.102
Chen, J. (2014). Heme oxygenase in neuroprotection: from mechanisms to therapeutic implications. Rev. Neurosci. 25, 269–280. doi: 10.1515/revneuro-2013-0046
Chen, J., and Regan, R. F. (2005). Increasing expression of heme oxygenase-1 by proteasome inhibition protects astrocytes from heme-mediated oxidative injury. Curr. Neurovasc. Res. 2, 189–196. doi: 10.2174/1567202054368344
Chen, J., Tu, Y., Moon, C., Nagata, E., and Ronnett, G. V. (2003). Heme oxygenase-1 and heme oxygenase-2 have distinct roles in the proliferation and survival of olfactory receptor neurons mediated by cGMP and bilirubin, respectively. J. Neurochem. 85, 1247–1261. doi: 10.1046/j.1471-4159.2003.01776.x
Crick, P. J., William Bentley, T., Abdel-Khalik, J., Matthews, I., Clayton, P. T., Morris, A. A., et al. (2015). Quantitative charge-tags for sterol and oxysterol analysis. Clin. Chem. 61, 400–411. doi: 10.1373/clinchem.2014.231332
Desjardins, S., Mayo, W., Vallée, M., Hancock, D., Le Moal, M., Simon, H., et al. (1997). Effect of aging on the basal expression of c-Fos, c-Jun, and Egr-1 proteins in the hippocampus. Neurobiol. Aging 18, 37–44. doi: 10.1016/S0197-4580(96)00206-0
Doré, S., Takahashi, M., Ferris, C. D., Zakhary, R., Hester, L. D., Guastella, D., et al. (1999). Bilirubin, formed by activation of heme oxygenase-2, protects neurons against oxidative stress injury. Proc. Natl. Acad. Sci. U. S. A 96, 2445–2450. doi: 10.1073/pnas.96.5.2445
Duclot, F., and Kabbaj, M. (2017). The role of early growth response 1 (EGR1) in brain plasticity and neuropsychiatric disorders. Front. Behav. Neurosci. 11:35. doi: 10.3389/fnbeh.2017.00035
Gatta, V., D’Aurora, M., Granzotto, A., Stuppia, L., and Sensi, S. L. (2014). Early and sustained altered expression of aging-related genes in young 3xTg-AD mice. Cell Death Dis. 5, e1054. doi: 10.1038/cddis.2014.11
Gómez Ravetti, M., Rosso, O. A., Berretta, R., Moscato, P., and He, W. (2010). Uncovering molecular biomarkers that correlate cognitive decline with the changes of hippocampus’ gene expression profiles in Alzheimer’s Disease. PLoS One 5:e10153. doi: 10.1371/journal.pone.0010153
Hartney, T., Birari, R., Venkataraman, S., Villegas, L., Martinez, M., Black, S. M., et al. (2011). Xanthine oxidase-derived ros upregulate Egr-1 via ERK1/2 in PA smooth muscle cells; model to test impact of extracellular ROS in chronic hypoxia. PLoS One 6:e27531. doi: 10.1371/journal.pone.0027531
Hascalovici, J. R., Song, W., Liberman, A., Vaya, J., Khatib, S., Holcroft, C., et al. (2014). Neural HO-1/sterol interactions in vivo: implications for Alzheimer’s disease. Neuroscience 280, 40–49. doi: 10.1016/j.neuroscience.2014.09.001
Hascalovici, J. R., Song, W., Vaya, J., Khatib, S., Fuhrman, B., Aviram, M., et al. (2009). Impact of heme oxygenase-1 on cholesterol synthesis, cholesterol efflux and oxysterol formation in cultured astroglia. J. Neurochem. 108, 72–81. doi: 10.1111/j.1471-4159.2008.05741.x
Hendrickx, A., Pierrot, N., Tasiaux, B., Schakman, O., Brion, J.-P., Kienlen-Campard, P., et al. (2013). Epigenetic induction of EGR-1 expression by the amyloid precursor protein during exposure to novelty. PLoS One 8:e74305. doi: 10.1371/journal.pone.0074305
Herms, J., Zurmöhle, U., Schlingensiepen, R., Brysch, W., and Schlingensiepen, K. H. (1994). Developmental expression of the transcription factor zif268 in rat brain. Neurosci. Lett. 165, 171–174. doi: 10.1016/0304-3940(94)90737-4
Hirose, W., Ikematsu, K., and Tsuda, R. (2003). Age-associated increases in heme oxygenase-1 and ferritin immunoreactivity in the autopsied brain. Leg. Med. 5(Suppl. 1), S360–S366. doi: 10.1016/S1344-6223(02)00133-5
Hughes, T. M., Rosano, C., Evans, R. W., and Kuller, L. H. (2013). Brain cholesterol metabolism, oxysterols, and dementia. J. Alzheimers Dis. 33, 891–911. doi: 10.3233/JAD-2012-121585
Iuliano, L. (2011). Pathways of cholesterol oxidation via non-enzymatic mechanisms. Chem. Phys. Lipids 164, 457–468. doi: 10.1016/j.chemphyslip.2011.06.006
Iuliano, L., Crick, P. J., Zerbinati, C., Tritapepe, L., Abdel-Khalik, J., Poirot, M., et al. (2015). Cholesterol metabolites exported from human brain. Steroids 99, 189–193. doi: 10.1016/j.steroids.2015.01.026
Iuliano, L., Micheletta, F., Natoli, S., Ginanni Corradini, S., Iappelli, M., Elisei, W., et al. (2003). Measurement of oxysterols and alpha-tocopherol in plasma and tissue samples as indices of oxidant stress status. Anal. Biochem. 312, 217–223. doi: 10.1016/s0003-2697(02)00467-0
Jones, M. W., Errington, M. L., French, P. J., Fine, A., Bliss, T. V. P., Garel, S., et al. (2001). A requirement for the immediate early gene Zif268 in the expression of late LTP and long-term memories. Nat. Neurosci. 4, 289–296. doi: 10.1038/85138
Khachigian, L. M. (2006). Early growth response-1 in cardiovascular pathobiology. Circ. Res. 98, 186–191. doi: 10.1161/01.RES.0000200177.53882.c3
Koldamova, R., Schug, J., Lefterova, M., Cronican, A. A., Fitz, N. F., Davenport, F. A., et al. (2014). Genome-wide approaches reveal EGR1-controlled regulatory networks associated with neurodegeneration. Neurobiol. Dis. 63, 107–114. doi: 10.1016/j.nbd.2013.11.005
Leoni, V. (2009). Oxysterols as markers of neurological disease – a review. Scand. J. Clin. Lab. Invest. 69, 22–25. doi: 10.1080/00365510802651858
Leoni, V., and Caccia, C. (2011). Oxysterols as biomarkers in neurodegenerative diseases. Chem. Phys. Lipids 164, 515–524. doi: 10.1016/j.chemphyslip.2011.04.002
Lu, Y., Li, T., Qureshi, H. Y., Han, D., and Paudel, H. K. (2011). Early growth response 1 (Egr-1) regulates phosphorylation of microtubule-associated protein tau in mammalian brain. J. Biol. Chem. 286, 20569–20581. doi: 10.1074/jbc.M111.220962
Manganaro, F., Chopra, V. S., Mydlarski, M. B., Bernatchez, G., and Schipper, H. M. (1995). Redox perturbations in cysteamine-stressed astroglia: implications for inclusion formation and gliosis in the aging brain. Free Radic. Biol. Med 19, 823–835. doi: 10.1016/0891-5849(95)02008-X
Marrone, D. F., Adams, A. A., and Satvat, E. (2011). Increased pattern separation in the aged fascia dentata. Neurobiol. Aging 32, 2317.e23–2332.e23. doi: 10.1016/j.neurobiolaging.2010.03.021
McMahon, A. P., Champion, J. E., McMahon, J. A., and Sukhatme, V. P. (1990). Developmental expression of the putative transcription factor Egr-1 suggests that Egr-1 and c-fos are coregulated in some tissues. Development 108, 281–287.
Minatohara, K., Akiyoshi, M., and Okuno, H. (2015). Role of Immediate-Early Genes in Synaptic Plasticity and Neuronal Ensembles Underlying the Memory Trace. Front. Mol. Neurosci. 8:78. doi: 10.3389/fnmol.2015.00078
Mutemberezi, V., Guillemot-Legris, O., and Muccioli, G. G. (2016). Oxysterols: from cholesterol metabolites to key mediators. Prog. Lipid Res. 64, 152–169. doi: 10.1016/j.plipres.2016.09.002
Nakaso, K., Kitayama, M., Mizuta, E., Fukuda, H., Ishii, T., Nakashima, K., et al. (2000). Co-induction of heme oxygenase-1 and peroxiredoxin I in astrocytes and microglia around hemorrhagic region in the rat brain. Neurosci. Lett. 293, 49–52. doi: 10.1016/S0304-3940(00)01491-9
Ogundele, O. M., Pardo, J., Francis, J., Goya, R. G., and Lee, C. C. (2018). A Putative mechanism of age-related synaptic dysfunction based on the impact of IGF-1 receptor signaling on synaptic CaMKIIα phosphorylation. Front. Neuroanat. 12:35. doi: 10.3389/fnana.2018.00035
Pagel, J.-I., and Deindl, E. (2012). Disease progression mediated by Egr-1 associated signaling in response to oxidative stress. Int. J. Mol. Sci. 13, 13104–13117. doi: 10.3390/ijms131013104
Parfenova, H., Leffler, C. W., Basuroy, S., Liu, J., and Fedinec, A. L. (2012). Antioxidant roles of heme oxygenase, carbon monoxide, and bilirubin in cerebral circulation during seizures. J. Cereb. Blood Flow Metab. 32, 1024–1034. doi: 10.1038/jcbfm.2012.13
Park, J. A., and Koh, J. Y. (1999). Induction of an immediate early gene egr-1 by zinc through extracellular signal-regulated kinase activation in cortical culture: its role in zinc-induced neuronal death. J. Neurochem. 73, 450–456. doi: 10.1046/j.1471-4159.1999.0730450.x
Ponti, D., Bastianelli, D., Rosa, P., Pacini, L., Ibrahim, M., Rendina, E. A., et al. (2015). The expression of B23 and EGR1 proteins is functionally linked in tumor cells under stress conditions. BMC Cell Biol. 16:27. doi: 10.1186/s12860-015-0073-5
Reeve, A., Simcox, E., and Turnbull, D. (2014). Ageing and Parkinson’s disease: why is advancing age the biggest risk factor? Ageing Res. Rev. 14, 19–30. doi: 10.1016/j.arr.2014.01.004
Reitz, C., Brayne, C., and Mayeux, R. (2011). Epidemiology of Alzheimer disease. Nat. Rev. Neurol. 7, 137–152. doi: 10.1038/nrneurol.2011.2
Rosa, P., Sforna, L., Carlomagno, S., Mangino, G., Miscusi, M., Pessia, M., et al. (2017). Overexpression of large-conductance calcium-activated potassium channels in human glioblastoma stem-like cells and their role in cell migration. J. Cell. Physiol. 232, 2478–2488. doi: 10.1002/jcp.25592
Schipper, H. M. (1999). Glial HO-1 expression, iron deposition and oxidative stress in neurodegenerative diseases. Neurotox. Res. 1, 57–70. doi: 10.1007/BF03033339
Schipper, H. M. (2004). Heme oxygenase expression in human central nervous system disorders. Free Radic. Biol. Med. 37, 1995–2011. doi: 10.1016/j.freeradbiomed.2004.09.015
Schipper, H. M., Song, W., Zukor, H., Hascalovici, J. R., and Zeligman, D. (2009). Heme oxygenase-1 and neurodegeneration: expanding frontiers of engagement. J. Neurochem. 110, 469–485. doi: 10.1111/j.1471-4159.2009.06160.x
Snyder, S. H., Jaffrey, S. R., and Zakhary, R. (1998). Nitric oxide and carbon monoxide: parallel roles as neural messengers. Brain Res. Brain Res. Rev. 26, 167–175. doi: 10.1016/S0165-0173(97)00032-5
Sottero, B., Gamba, P., Gargiulo, S., Leonarduzzi, G., and Poli, G. (2009). Cholesterol oxidation products and disease: an emerging topic of interest in medicinal chemistry. Curr. Med. Chem. 16, 685–705. doi: 10.2174/092986709787458353
Testa, G., Staurenghi, E., Zerbinati, C., Gargiulo, S., Iuliano, L., Giaccone, G., et al. (2016). Changes in brain oxysterols at different stages of Alzheimer’s disease: their involvement in neuroinflammation. Redox Biol. 10, 24–33. doi: 10.1016/j.redox.2016.09.001
Thanan, R., Oikawa, S., Hiraku, Y., Ohnishi, S., Ma, N., Pinlaor, S., et al. (2014). Oxidative stress and its significant roles in neurodegenerative diseases and cancer. Int. J. Mol. Sci. 16, 193–217. doi: 10.3390/ijms16010193
Uttara, B., Singh, A., Zamboni, P., and Mahajan, R. (2009). Oxidative stress and neurodegenerative diseases: a review of upstream and downstream antioxidant therapeutic options. Curr. Neuropharmacol. 7, 65–74. doi: 10.2174/157015909787602823
Vaya, J., and Schipper, H. M. (2007). Oxysterols, cholesterol homeostasis, and Alzheimer disease. J. Neurochem. 102, 1727–1737. doi: 10.1111/j.1471-4159.2007.04689.x
Vaya, J., Song, W., Khatib, S., Geng, G., and Schipper, H. M. (2007). Effects of heme oxygenase-1 expression on sterol homeostasis in rat astroglia. Free Radic. Biol. Med. 42, 864–871. doi: 10.1016/j.freeradbiomed.2006.12.022
Vincent, S. R., Das, S., and Maines, M. D. (1994). Brain heme oxygenase isoenzymes and nitric oxide synthase are co-localized in select neurons. Neuroscience 63, 223–231. doi: 10.1016/0306-4522(94)90018-3
Watson, M. A., and Milbrandt, J. (1990). Expression of the nerve growth factor-regulated NGFI-A and NGFI-B genes in the developing rat. Development 110, 173–183.
Yan, S.-F., Fujita, T., Lu, J., Okada, K., Shan Zou, Y., Mackman, N., et al. (2000). Egr-1, a master switch coordinating upregulation of divergent gene families underlying ischemic stress. Nat. Med. 6, 1355–1361. doi: 10.1038/82168
Yau, J. L., Olsson, T., Morris, R. G., Noble, J., and Seckl, J. R. (1996). Decreased NGFI-A gene expression in the hippocampus of cognitively impaired aged rats. Brain Res. Mol. Brain Res. 42, 354–357. doi: 10.1016/S0169-328X(96)00220-3
Keywords: Egr-1, aging brain, oxysterols, HO-1, oxidative stress, cortex, hippocampus
Citation: Rosa P, Zerbinati C, Crestini A, Canudas A-M, Ragona G, Confaloni A, Iuliano L and Calogero A (2018) Heme Oxygenase-1 and Brain Oxysterols Metabolism Are Linked to Egr-1 Expression in Aged Mice Cortex, but Not in Hippocampus. Front. Aging Neurosci. 10:363. doi: 10.3389/fnagi.2018.00363
Received: 26 June 2018; Accepted: 23 October 2018;
Published: 06 November 2018.
Edited by:
Ashok Kumar, University of Florida, United StatesReviewed by:
Manoj Kumar Jaiswal, Icahn School of Medicine at Mount Sinai, United StatesCopyright © 2018 Rosa, Zerbinati, Crestini, Canudas, Ragona, Confaloni, Iuliano and Calogero. This is an open-access article distributed under the terms of the Creative Commons Attribution License (CC BY). The use, distribution or reproduction in other forums is permitted, provided the original author(s) and the copyright owner(s) are credited and that the original publication in this journal is cited, in accordance with accepted academic practice. No use, distribution or reproduction is permitted which does not comply with these terms.
*Correspondence: Antonella Calogero, YW50b25lbGxhLmNhbG9nZXJvQHVuaXJvbWExLml0
Disclaimer: All claims expressed in this article are solely those of the authors and do not necessarily represent those of their affiliated organizations, or those of the publisher, the editors and the reviewers. Any product that may be evaluated in this article or claim that may be made by its manufacturer is not guaranteed or endorsed by the publisher.
Research integrity at Frontiers
Learn more about the work of our research integrity team to safeguard the quality of each article we publish.