- 1Medical Engineering and Technology Research Center, Taishan Medical University, Taian, China
- 2Imaging-X Joint Laboratory, Taian, China
- 3College of Radiology, Taishan Medical University, Taian, China
- 4Institute of Biomedical Engineering, Chinese Academy of Medical Sciences and Peking Union Medical College, Tianjin, China
- 5College of Mechanical and Electronic Engineering, Shandong University of Science and Technology, Qingdao, China
- 6School of Basic Medical Sciences, Taishan Medical University, Taian, China
Background: The hippocampus is an important limbic structure closely related to memory function. However, few studies have focused on the association between hippocampal subfields and age-related memory decline. We investigated the volume alterations of hippocampal subfields at different ages and assessed the correlations with Immediate and Delayed recall abilities.
Materials and Methods: A total of 275 participants aged 20–89 years were classified into 4 groups: Young, 20–35 years; Middle-early, 36–50 years; Middle-late, 51–65 years; Old, 66–89 years. All data were acquired from the Dallas Lifespan Brain Study (DLBS). The volumes of hippocampal subfields were obtained using Freesurfer software. Analysis of covariance (ANCOVA) was performed to analyze alterations of subfield volumes among the 4 groups, and multiple comparisons between groups were performed using the Bonferroni method. Spearman correlation with false discovery rate correction was used to investigate the relationship between memory recall scores and hippocampal subfield volumes.
Results: Apart from no significant difference in the left parasubiculum (P = 0.269) and a slight difference in the right parasubiculum (P = 0.022), the volumes of other hippocampal subfields were significantly different across the adult lifespan (P < 0.001). The hippocampal fissure volume was increased in the Old group, while volumes for other subfields decreased. In addition, Immediate recall scores were associated with volumes of the bilateral molecular layer, granule cell layer of the dentate gyrus (GC-DG), cornus ammonis (CA) 1, CA2/3, CA4, left fimbria and hippocampal amygdala transition area (HATA), and right fissure (P < 0.05). Delayed recall scores were associated with the bilateral molecular layer, GC-DG, CA2/3 and CA4; left tail, presubiculum, CA1, subiculum, fimbria and HATA (P < 0.05).
Conclusion: The parasubiculum volume was not significantly different across the adult lifespan, while atrophy in dementia patients in some studies. Based on these findings, we speculate that volume changes in this region might be considered as a biomarker for dementia disorders. Additionally, several hippocampal subfield volumes were significantly associated with memory scores, further highlighting the key role of the hippocampus in age-related memory decline. These regions could be used to assess the risk of memory decline across the adult lifespan.
Introduction
The hippocampus is an important limbic structure (Richter-Levin, 2004) with a critical role in memory and is particularly vulnerable to aging (Eichenbaum, 2004; Mueller et al., 2011; Malykhin et al., 2017; Zammit et al., 2017). It is regarded as an amalgamated structure (Van, 2004), but few studies have focused on volume changes in hippocampal subfields with normal aging and their relationships with age-related memory decline.
The mammalian hippocampus consists of several subfields with different memory functions (Hunsaker et al., 2008; Yassa and Stark, 2011; Engvig et al., 2012; Reagh et al., 2014). For example, Hunsaker et al. (2008) found that CA1 and CA3 both contribute to episodic memory processing by training memory ability in rats. Another study reported that rats with dentate gyrus (DG) lesions were unable to distinguish between training and testing environments (Yassa and Stark, 2011).
Numerous human studies have reported the functions of hippocampal subfields in various disorders. For example, histological studies suggest that AD variably affects different hippocampal subfields. Especially in the early stage, tangle accumulation and neuron loss are more prominent in the CA1 and subiculum (Rössler et al., 2002; Schönheit et al., 2004). Mueller et al. (2012) suggested that auditory Immediate recall was associated with the CA3 and DG, while auditory Delayed recall and auditory Delayed recognition were related to the CA1 in subjects with temporal lobe epilepsy with hippocampal sclerosis. In those without hippocampal sclerosis, to a lesser degree, auditory Immediate recall was associated with the CA3 and DG, whereas auditory Delayed recall and recognition were more closely related to the fusiform gyrus. In a recent study, the CA1 was implicated in Immediate and Delayed recall of verbal memory in patients with left hippocampal sclerosis, while for patients with right hippocampal sclerosis, the CA1 and epilepsy duration were related to visual memory (Comper et al., 2017). Carlesimo et al. (2015) found no significant correlation between hippocampal subfield volumes and Immediate and Delayed recall scores in healthy participants. They reported that AD patients had significant correlations with both types of memory performance and the CA2-3, CA4-DG, and subiculum. The presubiculum was only associated with Delayed recall. Finally, they found that patients with mild cognitive impairment showed significant correlations between Immediate recall and presubiculum and subiculum volumes. These preliminary studies suggest that a detailed volumetric study of hippocampal subfields may help elucidate the regions involved in specific memory functions.
Extensive studies have reported that the volumes of hippocampal subfields non-linearly decreased with aging. Moreover, hippocampal changes in different subfields are inconsistent (Malykhin et al., 2017; Zheng et al., 2018). Daugherty et al. (2016) observed that the volumes of CA1-2 and CA3-DG significantly decreased with increasing age. However, Malykhin et al. (2017) found no significant difference in CA1-3, but did report marked changes in the subiculum and DG. Others reported volume atrophy in the hippocampus of patients with AD (Wisse et al., 2014; Kälin et al., 2017; Platero et al., 2018). For instance, Wisse et al. (2014) found that AD patients exhibited smaller volumes in the subiculum, CA1, CA3 and DG/CA4. DLB has pathologic overlap with AD (Mckeith et al., 2005). The results of a study of a mouse model expressing mutant β-synuclein (linked to DLB) suggested that the pseudo-immaturity of DG granule cells may be a shared endophenotype in patients with neurodegenerative disorders (Hagihara et al., 2018). Several groups have found that hippocampal atrophy is less severe in DLB than AD (Firbank et al., 2010; Elder et al., 2017; Pang et al., 2018). For instance, Firbank et al. (2010) found that the CA1 and subiculum exhibited less atrophy in DLB patients compared to AD patients. These observations underscore the importance of identifying biomarkers to distinguish between normal and pathological aging.
Researchers have investigated the relationships between hippocampal subfields and memory in case control studies, but few have focused on age-related memory decline (Mueller et al., 2012; Comper et al., 2017). Thus, the association between age-related memory decline and hippocampal subfield volumes in normal humans remains unclear. In this study, an automatic method in Freesurfer software was used to segment the hippocampus into 13 subfields in each hemisphere on 275 normal adults (age 20–89) imaged at the same scanning center. The main purpose was to investigate volume changes in hippocampal subfields across the adult lifespan and examine their associations with memory recall decline based on immediate and delayed recall scores.
Materials and Methods
Participants
T1-weighted MRI data of 315 healthy adults, aged 20–89 years, were selected from the DLBS1. Subjects with incomplete cognitive information were excluded. Finally, total 275 subjects (54.85 ± 20.61 years; 174 females, 101 males) were included in the present study. The population were classified into 4 groups: Young group, 20–35 years; Middle-early, 36–50 years; Middle-late, 51–65 years; Old group, 66–89 years. Participants were recruited through flyers and media advertisements. Participants were right-handed and native English speakers with no history of neurological disease. The participants were well-educated and with high scores on the Mini-Mental State Examination (MMSE > 26). Immediate and Delayed recall abilities were measured with Hopkins Verbal Learning Scoring (Brandt, 1991), and a 20-min delay was used for Delayed recall score measurement. This experiment was approved by the Institutional Review Board of the University of Texas Southwestern Medical Center and the University of Texas at Dallas. All participants provided written informed consent.
sMRI Data Acquisition
All participants underwent T1-weighted imaging on a Philips Achieva 3T scanner (Amsterdam, Netherlands). The parameters were as follows: slice thickness = 1 mm, repetition time = 8.135 ms, echo time = 3.7 ms, matrix = 256 × 256, field of view = 204 × 256. The direction was anterior-posterior.
Imaging Processing
All T1-weighted images were processed using publicly available Freesurfer software2. We conducted the main recon stream (“recon-all”) in Freesurfer 6.0 for volumetric segmentation, including motion correction, skull stripped, intensity normalization, automated Talairach transformation, gray/white matter tessellation, and topology correction (Fischl et al., 2001; Segonne et al., 2007). Subcortical structures were segmented with a non-linear warping atlas (Macdonald, 1997; Fischl et al., 2016), and total hippocampal volumes were obtained. Subsequently, a probabilistic atlas and a modified version of Van Leemput’s algorithm were applied to segment the hippocampus (Van Leemput et al., 2009; Iglesias et al., 2015; Saygin et al., 2017) into 13 subfields in each hemisphere: the CA1, CA2/3, CA4, molecular layer, alveus, GC-DG, HATA, subiculum, presubiculum, parasubiculum, fimbria, hippocampal tail and fissure, as shown in Figure 1. The CA2 and CA3 were combined because of unclear contrast, and the alveus volume was removed due to the thin shape and unreliable segmentation (Iglesias et al., 2015).
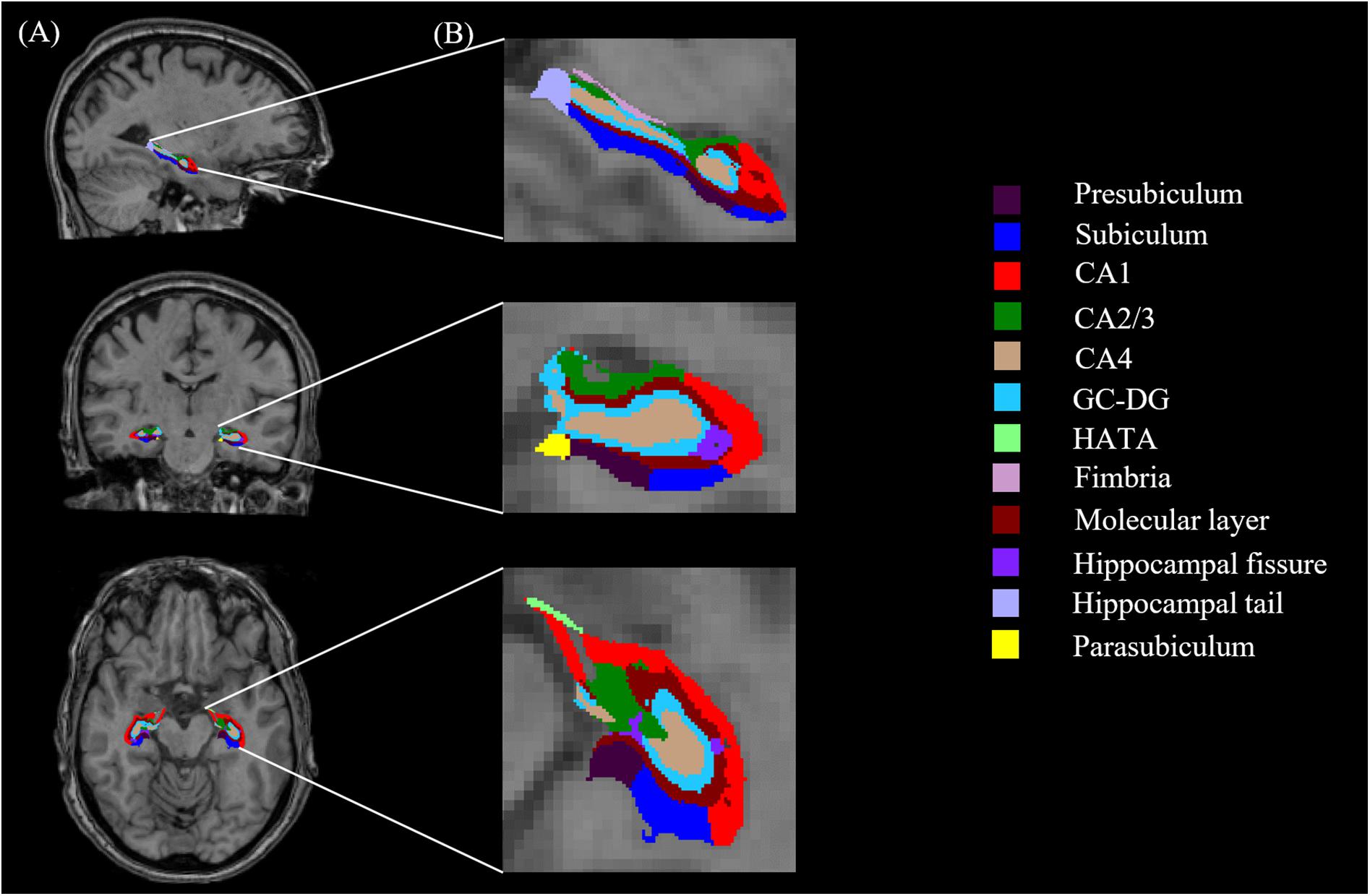
FIGURE 1. Hippocampal segmentation. (A) T1 images of hippocampal subfields in the sagittal, coronal, and axial planes. (B) Magnified views.
Statistical Analysis
Statistical analysis was performed using IBM SPSS software (version 22.0, Armonk, NY, United States) and MATLAB (MathWorks Inc., Natick, MA, United States). Chi-square tests were used to evaluate the differences in gender distribution among groups. One-way ANOVA was performed to assess group differences in education years, while ANCOVA was performed for the differences in MMSE scores, Immediate and Delayed recall scores among the 4 groups, with gender and education years as covariates. For hippocampal subfield volume differences, we considered gender, education years and eTIV as covariates for ANCOVA was performed. Regarding the indexes with significant differences among the 4 groups, multiple comparison between groups was performed using the Bonferroni method. Spearman correlation analyses were applied in MATLAB using a home-written program to verify the correlations between memory recall scores and hippocampal subfields, and correlation coefficients (R) were calculated. Gender, education years, and eTIV were also regarded as covariates for the correlations between memory recall scores and hippocampal subfields. Spearman correlation results were corrected by FDR correction in MATLAB. The significance level of all results was set at P < 0.05.
Results
Differences in Demographics and Recall Scores
The demographic characteristics and Immediate recall and Delayed recall scores for 275 subjects are shown in Table 1 as means and standard deviations. MMSE scores, although still within the normal range, significantly declined with age (P < 0.001). There were significant differences in MMSE scores (P < 0.001), Immediate recall scores (P < 0.001) and Delayed recall scores (P = 0.003). Furthermore, the pairwise comparisons demonstrated apparent declines in MMSE and Immediate recall scores in the Old group (P < 0.05) compared with other groups, as well as lower Delayed recall scores (P < 0.05) compared with the Young and Middle-late groups, as shown in Supplementary Table 1. No significant difference was observed for gender distribution (P = 0.462) or education years (P = 0.338) among the 4 groups.
Age Effects on Hippocampal Subfield Volume
Hippocampal subfield volumes change with aging. Table 2 summarizes the statistical analysis for the volume of the hippocampal subfields. Besides no significant difference in the left parasubiculum (P = 0.269) and a slight difference in the right parasubiculum (P = 0.022), other subfields were significantly different among the 4 groups (P < 0.001). Supplementary Table 2 lists the statistical results of pairwise comparisons in subfields with significant differences among the 4 groups. Histograms in Figure 2 demonstrate pairwise comparisons on hippocampal subfield volumes. Most were significantly decreased in the Old group compared with the other 3 groups, including the bilateral hippocampal tail, subiculum, CA1, molecular layer, GC-DG, CA2/3, CA4, fimbria, HATA, and right presubiculum (P < 0.05). The left presubiculum volume was only significantly different between the Old and Young groups (P < 0.001). A significant decline of right fimbria volume was also observed in the Middle-late group relative to the Young group (P = 0.015). Interestingly, the hippocampal fissure volume was increased in the Old group relative to other groups for the left hemisphere (P < 0.05), as well as relative to the Young and Middle-early groups for the right (P < 0.05). In addition, although there were slight differences among the 4 groups in right parasubiculum, no significance was found between groups (P > 0.05).
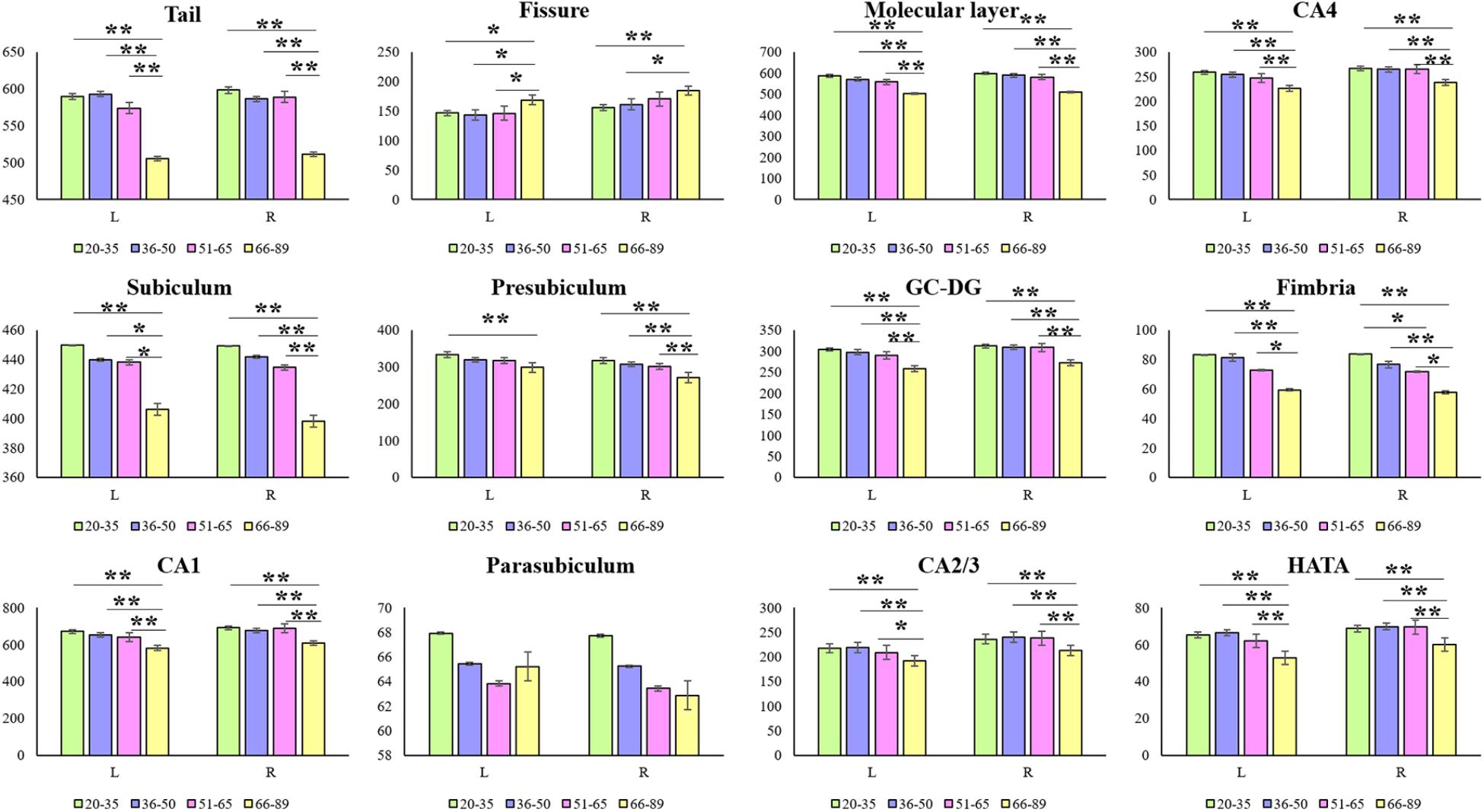
FIGURE 2. Mean volumes of hippocampal subfields in the Young, Middle-early, Middle-late, and Old groups. L, left hemisphere; R, right hemisphere; The Y-axis indicates the mean volume of hippocampal subfields in each group. ∗P < 0.05, ∗∗P < 0.001; 95% confidence intervals.
Associations Between Memory Recall Scores and Hippocampal Subfields
Table 3 lists the Spearman correlation analysis results. There were significant correlations between bilateral whole hippocampal volume and Immediate and Delayed recall scores (P < 0.05). Furthermore, the results showed that regarding Immediate recall scores, there were positive correlations with the bilateral molecular layer, GC-DG, CA1, CA2/3 and CA4, left fimbria and HATA (P < 0.05). In addition, a negative association was observed for the right fissure (R = -0.1411, P = 0.0358). Delayed recall scores were strongly and positively associated with the bilateral GC-DG, molecular layer, CA2/3 and CA4, left tail, presubiculum, subiculum, CA1, fimbria and HATA (P < 0.05). In addition, we found that 10 subfields on the left were related to Delayed recall scores, compared to 4 on the right.
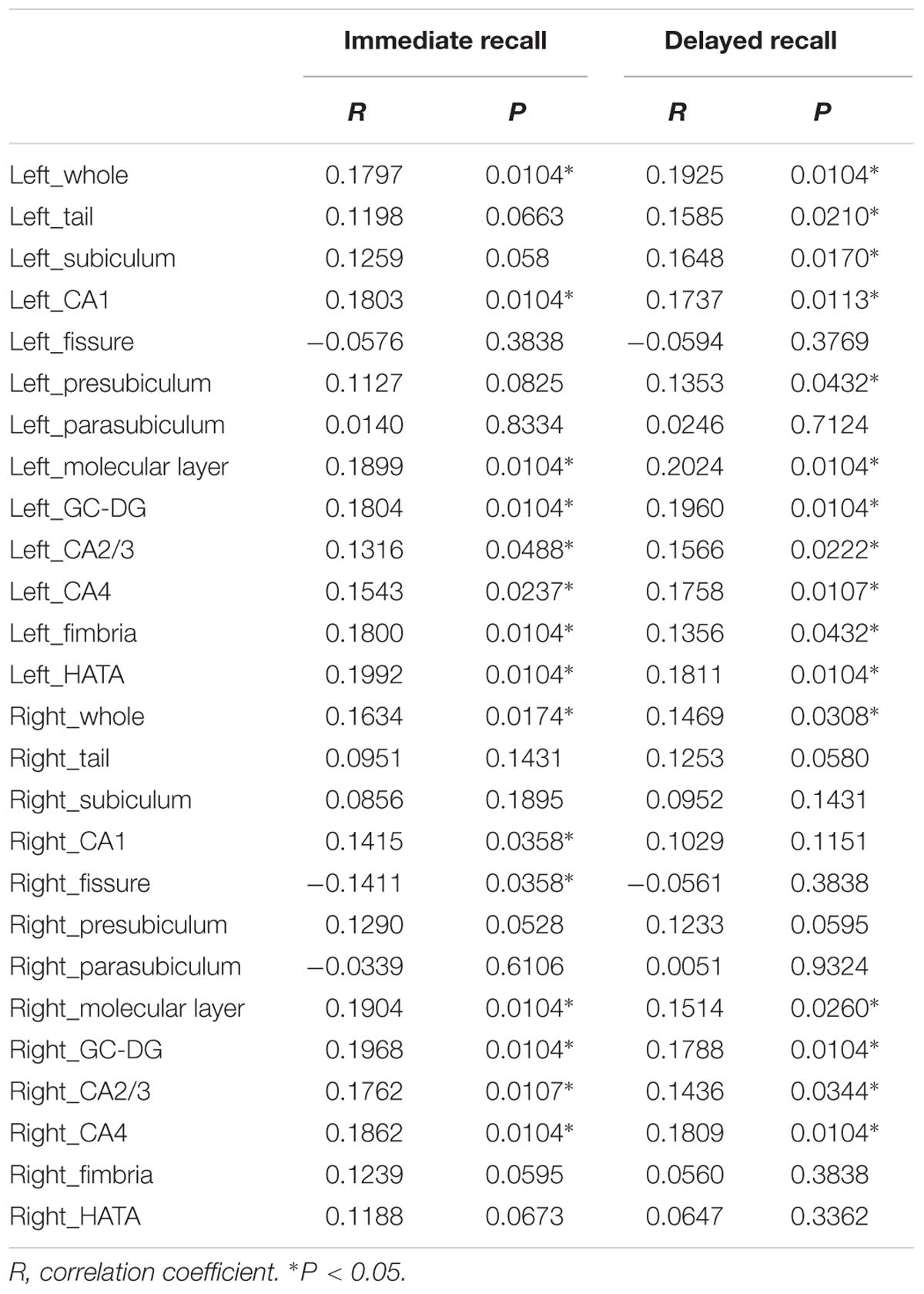
TABLE 3. Correlation analysis between hippocampal subfield volumes and Immediate recall and Delayed recall scores.
Discussion
In this study, we measured hippocampal subfield volume changes and their correlations with Immediate recall and Delayed recall scores across the adult lifespan. Nearly all volumes showed significant declines in the Old group except the left parasubiculum, while there was a significant increase in the fissure volume. Several hippocampal subfields were significantly related to Immediate and Delayed recall scores. Moreover, we also observed a stronger relationship between delayed recall scores and left hippocampus volumes.
Age Effects on Hippocampal Subfield Volumes
Differences in segmentation methods impact the number and volume of hippocampal subfields reported. A popular segmentation method yields the CA1-4, DG, and subiculum (Perrotin et al., 2015; Riggins et al., 2018), as well as the presubiculum in some studies (de Flores et al., 2014; Carlesimo et al., 2015). We performed the more detailed segmentation proposed by Iglesias et al. (2015) that includes the CA1, CA2/3, CA4, molecular layer, alveus, GC-DG, HATA, subiculum, presubiculum, parasubiculum, fimbria, tail, and fissure.
Previous studies reported that the effects of age impact several specific subfields rather than the entire hippocampus (de Flores et al., 2014; Wisse et al., 2014; Malykhin et al., 2017). de Flores et al. (2014) manually segmented the hippocampus into the CA1, subiculum, and other regions. They observed that CA1 volume non-linearly decreased with aging and dropped at about 50 years, and subiculum volume linearly decreased with aging; no other subfields exhibited volume decreases. Malykhin et al. (2017) subsequently described negative correlations between the total subiculum and DG volumes with aging, whereas there was no significance for the total CA1-3. We observed significant differences in volumes of the CA1, CA2/3, CA4, GC-DG, and subiculum. One possible reason for the differences is that de Flores et al. (2014) combined the CA2/3/4 and DG into a single region of interest (ROI) called “others,” while Malykhin et al. (2017) combined CA1, 2 and 3 into a single ROI called “CA1-3.” Different subfield combinations may obscure age-related changes of single subfields. Additionally, animal studies revealed that fimbria lesions may impair object discrimination (Wible et al., 1992; Antoniadis and Mcdonald, 2006). Extrapolating these animal studies to humans, the reduction of fimbria volume in this study might be considered as an early biomarker for visual dysfunction.
Using the same segmentation method, significant non-linear age-related declines in CA2/3, CA4 and GC-DG volumes, and a significant linear increase in fissure volume were recently reported (Zheng et al., 2018). They found no significant difference in parasubiculum volume. These are similar to our results, but Zheng and colleagues found no significant volume differences in the hippocampal tail, presubiculum, subiculum, CA1, molecular layer, fimbria, or HATA. However, according to their raw plots and change trends, a non-linear decline trend was evident. The main reason underlying the discrepant results might be the dataset; they included 54 subjects, while we assessed 275.
Decreased hippocampal subfield volumes have been widely reported in dementia disorders such as AD and DLB (Delli Pizzi et al., 2016; Mak et al., 2016, 2017). One group found that the volumes of the CA1, CA2-3, CA4, DG, and total subiculum (subiculum, presubiculum, and parasubiculum) are decreased in AD (Mak et al., 2017). In the present study, the volumes of all subfields except the parasubiculum were decreased in the Old group, which is similar to observations in subjects with dementia disorders. This suggests that these subfields may not be useful biomarkers to investigate normal aging and dementia. According to our results, only the parasubiculum did not show significant atrophy in the Old group, indicating its use as a dementia biomarker. This is supported by prior studies implicating the parasubiculum involved in dementia, as well as its important role in the medial temporal memory system (Caballerobleda and Witter, 1993; Glasgow and Chapman, 2007; Ding, 2013). In an immunohistochemistry study of an AD mouse model, Weidensteiner and his colleagues identified plaque depositions in the subiculum/parasubiculum (Weidensteiner et al., 2009). Earlier research reports described severe pathology in the parasubiculum of subjects with Creutzfeldt-Jakob disease (Guentchev et al., 1997; Kaneko et al., 1999). In addition, Fukutani et al. (1995) reported that compared to healthy subjects, AD patients had fewer unaffected neurons and more intra and extracellular neurofibrillary tangles in the parasubiculum, entorhinal cortex, prosubiculum and CA1. Other studies reported similar results noting severely affected neurons in the parasubiculum of AD brains (Chanpalay et al., 1986; Yamaguchi et al., 1988; Kalus et al., 1989; Fukutani et al., 2000). Iglesias et al. (2016) described parasubiculum atrophy in AD patients. Regarding AD progression, Dong et al. (2018) reported that parasubiculum volume is significantly correlated with neuropsychological test scores in patients with amnestic mild cognitive impairment. Based on these findings, we hypothesize that the parasubiculum may be a potential biomarker for dementia disorders. Further research is necessary to replicate these findings in other samples and advance our understanding.
Associations Between Memory and Hippocampal Subfields
Hippocampal subfield volumes are closely correlated with memory ability (Daugherty et al., 2017). The strong association between the CA1 and memory performance was previously reported (Adamowicz et al., 2017). Furthermore, neuronal density in the CA1 was significantly associated with preoperative Immediate and Delayed recall scores in patients undergoing temporal lobectomy (Baxendale et al., 1998). Another study reported that the CA1 is involved in t Immediate and Delayed recall in patients with left hippocampal sclerosis (Comper et al., 2017). Notably, we obtained similar results in healthy subjects. Other studies reached different conclusions. Animal literature supports a role of the CA1 in intermediate and long-term memory but not short-term memory (Remondes and Schuman, 2004; Vago et al., 2007). One group compared healthy and cognitively impaired elderly subjects and concluded that the CA1 is associated with Delayed recall Discriminability but not Immediate or Short Free Recall Discriminability, while, the CA3 and DG are associated with Immediate and Short Free Recall Discriminability (Mueller et al., 2011). In our study, the CA1, CA2/3 and GC-DG were related to both Immediate and Delayed recall scores. There are two possible explanations for this discrepancy. Firstly, their study only included 50 subjects. Secondly, some of their subjects with cognitive impairment might have been affected by AD, which could affect structure-memory associations. In accordance with our results, Finke et al. (2016) found that the decreased Delayed recall scores were accompanied by atrophy of the CA2/3 and CA4/DG in encephalitis patients with leucine-rich, glioma-inactivated 1 antibodies.
Another study suggested that conversion of patients with PD from no cognitive impairment to mild cognitive impairment was related to the baseline volumes of the GC-DG, left parasubiculum, left HATA, and right CA4. The authors postulated that parasubiculum and HATA atrophy might affect the integrity of hippocampal-amygdala network that underlies information processed (Foo et al., 2017). Their results indicate that these subfields might be critical to cognition. Additionally, preliminary animal studies reported that the parasubiculum may be more involved in online spatial information processing rather than long-term information storage (Kesner and Giles, 1998; Liu et al., 2004; Tang et al., 2016). However, we did not find significant correlations between recall scores and parasubiculum volume. This discrepancy may be due to variable ways of measuring cognition or subject differences.
Lim et al. (2013) found that lower volumes of the subiculum and presubiculum predicted poorer Delayed verbal recall ability in PD patients. Subsequently, Stav and colleagues reached a similar conclusion in AD patients (Stav et al., 2016). These studies indicate that the subiculum and presubiculum might be related to Delayed verbal recall ability, which is consistent with our results, although we only observed a slight association for the presubiculum.
The molecular layer including neurons of the subiculum and CA fields lies above the subiculum and underneath the fissure. Few studies have reported correlations between molecular layer volume and Immediate and Delayed recall abilities. Cantero et al. (2016) found that lower volumes of the DG and molecular layer were associated with Delayed memory. A recent study concluded that molecular layer volume was positively associated with general cognition from childhood to adulthood (Tamnes et al., 2018). Therefore, we considered that the current study might reach more accurate conclusions about the impacts of molecular layer volume on memory function. One group found that the number of synapses in molecular layer was highly correlated with Delayed recall and Delayed recognition abilities, but there was no association with Immediate recall ability (Scheff et al., 2006). In our study, we observed that molecular layer volume was correlated with both Immediate and Delayed recall abilities. We plan to perform molecular biology experiments to explore the relationship between this brain region and memory.
We found that Immediate and Delayed recall scores were associated with the volume of left fimbria, a white matter structure that extends from the alveus and eventually forms the fornix. Consistent with our results, rats with fimbria-fornix lesions exhibited short-term memory impairment (Winters and Dunnett, 2004; Addy et al., 2005). Moreover, Tomaiuolo et al. (2004) observed that Immediate and Delayed recall scores were both associated with fornix volume.
Our results should be considered in the context of several limitations. First, there is a gender distribution imbalance, with data from 101 males and 174 females. In addition, our subjects were aged 20–89 years and lack of development children; and this should be addressed in further studies. Second, vascular risk increases with aging, and the effect of blood pressure on hippocampus volume is not negligible (Shing et al., 2011). All data included in this study were acquired from DLBS dataset, so we were unable to obtain more clinical information, which may have influenced our results. Third, this was a cross-sectional study; longitudinal assessments will be necessary to confirm our findings. Moreover, there are some limitations regarding the segmentation method. Since the atlas was developing using data from elderly subjects, there may be slight hippocampal atrophy. Finally, although the MRI scans were ultra-high resolution MRI, there were some unclear boundaries between subfields, such as CA fields or the interface between CA4/GC-DG.
Conclusion
We explored hippocampal subfield volumes alterations in subjects of different ages and correlated these changes with Immediate recall and Delayed recall scores. Multiple hippocampal subfields were smaller in the Old group, in addition to parasubiculum, which is reportedly atrophied in some dementia disorders. We therefore speculate that a significant decline in parasubiculum volume maybe a potential biomarker for dementia disorders, but further investigation is needed to support this hypothesis. Taken together, our results indicate that subfield volume changes are related to age-related memory decline and might be regarded as biomarkers in postponing memory decline across the adult lifespan.
Data Availability Statement
The datasets for this study can be found in the Dallas Lifespan Brain Study (DLBS) [http://fcon_1000.projects.nitrc.org/indi/retro/dlbs.html].
Ethics Statement
The data included in this study was obtained from the Dallas Lifespan Brain Study (DLBS), which is available on the International Neuroimaging Data-sharing Initiative (INDI). This study was carried out in accordance with the recommendations of ’UT Southwestern Institutional Review Board’ with written informed consent from all subjects. All subjects gave written informed consent in accordance with the Declaration of Helsinki. The protocol was approved by the UT Southwestern Institutional Review Board.
Author Contributions
DC and TY designed the study. FZ performed the imaging processing and wrote the manuscript. ZmL and DZ organized and filtered the data. LZ, SZ, and YZ analyzed the data. XL and DC contributed to the statistical analysis. DC, CL, LS, ZpL, KH, WL, and JQ reviewed and edited the manuscript. All the authors read and approved the submitted manuscript.
Funding
We are grateful for the support from the China National Key Research and Development Program (2016YFC0103400), and the Medical and Health Science and Technology Development Project of Shandong Province, China (2016WS0603 and 2015WS0107). JQ was supported by the Taishan Scholars Program of Shandong Province (ts201712065).
Conflict of Interest Statement
The authors declare that the research was conducted in the absence of any commercial or financial relationships that could be construed as a potential conflict of interest.
Acknowledgments
The authors are very grateful to the owner of the DLBS dataset and International Neuroimaging Data-Sharing Initiative Group for sharing this data publicly, so that we can do more research.
Supplementary Material
The Supplementary Material for this article can be found online at: https://www.frontiersin.org/articles/10.3389/fnagi.2018.00320/full#supplementary-material
Abbreviations
AD, Alzheimer’s disease; ANCOVA, analysis of covariance; ANOVA, analysis of variance; CA, cornus ammonis; DG, dentate gyrus; DLB, dementia with Lewy bodies; DLBS, Dallas Lifespan Brain Study; eTIV, estimated total intracranial volume; FDR, false discovery rate; GC-DG, granule cell layer of the dentate gyrus; HATA, hippocampal amygdala transition area; MMSE, Mini-Mental State Examination; MRI, magnetic resonance imaging; PD, Parkinson’s disease.
Footnotes
References
Adamowicz, D. H., Roy, S., Salmon, D. P., Galasko, D. R., Hansen, L. A., Masliah, E., et al. (2017). Hippocampal α-synuclein in dementia with lewy bodies contributes to memory impairment and is consistent with spread of pathology. J. Neurosci. 37, 1675–1684. doi: 10.1523/JNEUROSCI.3047-16.2016
Addy, N. A., Pocivavsek, A., and Levin, E. D. (2005). Reversal of clozapine effects on working memory in rats with fimbria-fornix lesions. Neuropsychopharmacology 30, 1121–1127. doi: 10.1038/sj.npp.1300669
Antoniadis, E. A., and Mcdonald, R. J. (2006). Fornix, medial prefrontal cortex, nucleus accumbens, and mediodorsal thalamic nucleus: roles in a fear-based context discrimination task. Neurobiol. Learn. Mem. 85, 71–85. doi: 10.1016/j.nlm.2005.08.011
Baxendale, S. A., Van, P. W., Thompson, P. J., Duncan, J. S., Harkness, W. F., and Shorvon, S. D. (1998). Hippocampal cell loss and gliosis: relationship to preoperative and postoperative memory function. Neuropsychiatry Neuropsychol. Behav. Neurol. 11, 12–21.
Brandt, J. (1991). The hopkins verbal learning test: development of a new memory test with six equivalent forms. Clin. Neuropsychol. 5, 125–142. doi: 10.1080/13854049108403297
Caballerobleda, M., and Witter, M. P. (1993). Regional and laminar organization of projections from the presubiculum and parasubiculum to the entorhinal cortex: an anterograde tracing study in the rat. J. Comp. Neurol. 328:115.
Cantero, J. L., Iglesias, J. E., Leemput, K. V., and Atienza, M. (2016). Regional hippocampal atrophy and higher levels of plasma amyloid-beta are associated with subjective memory complaints in nondemented elderly subjects. J. Gerontol. 71, 1210–1215. doi: 10.1093/gerona/glw022
Carlesimo, G. A., Piras, F., Orfei, M. D., Iorio, M., Caltagirone, C., and Spalletta, G. (2015). Atrophy of presubiculum and subiculum is the earliest hippocampal anatomical marker of Alzheimer’s disease. Alzheimers Dement. 1:24. doi: 10.1016/j.dadm.2014.12.001
Chanpalay, V., Köhler, C., Haesler, U., Lang, W., and Yasargil, G. (1986). Distribution of neurons and axons immunoreactive with antisera against neuropeptide Y in the normal human hippocampus. J. Comp. Neurol. 248, 376–394. doi: 10.1002/cne.902480307
Comper, S. M., Jardim, A. P., Corso, J. T., Gaça, L. B., Noffs, M., Lancellotti, C., et al. (2017). Impact of hippocampal subfield histopathology in episodic memory impairment in mesial temporal lobe epilepsy and hippocampal sclerosis. Epilepsy Behav. E B 75:183. doi: 10.1016/j.yebeh.2017.08.013
Daugherty, A. M., Bender, A. R., Raz, N., and Ofen, N. (2016). Age differences in hippocampal subfield volumes from childhood to late adulthood. Hippocampus 26, 220–228. doi: 10.1002/hipo.22517
Daugherty, A. M., Flinn, R., and Ofen, N. (2017). Hippocampal CA3-dentate gyrus volume uniquely linked to improvement in associative memory from childhood to adulthood. Neuroimage 153:75. doi: 10.1016/j.neuroimage.2017.03.047
de Flores, R., La Joie, R., Landeau, B., Perrotin, A., Mézenge, F., de La Sayette. V., et al. (2014). Effects of age and Alzheimer’s disease on hippocampal subfields: comparison between manual and freesurfer volumetry. Hum. Brain Mapp. 10, 463–474. doi: 10.1002/hbm.22640
Delli Pizzi, S., Franciotti, R., Bubbico, G., Thomas, A., Onofrj, M., and Bonanni, L. (2016). Atrophy of hippocampal subfields and adjacent extrahippocampal structures in dementia with Lewy bodies and Alzheimer’s disease. Neurobiol. Aging 40, 103–109. doi: 10.1016/j.neurobiolaging.2016.01.010
Ding, S. L. (2013). Comparative anatomy of the prosubiculum, subiculum, presubiculum, postsubiculum, and parasubiculum in human, monkey, and rodent. J. Comp. Neurol. 521, 4145–4162. doi: 10.1002/cne.23416
Dong, W. K., Lim, H. K., Joo, S., Na, R. L., and Chang, U. L. (2018). The association between hippocampal subfield volumes and education in cognitively normal older adults and amnestic mild cognitive impairment patients. Neuropsychiatr. Dis. Treat. 14:143. doi: 10.2147/NDT.S151659
Eichenbaum, H. (2004). Hippocampus: cognitive processes and neural representations that underlie declarative memory. Neuron 44, 109–120. doi: 10.1016/j.neuron.2004.08.028
Elder, G. J., Mactier, K., Colloby, S. J., Watson, R., Blamire, A. M., O’Brien, J. T., et al. (2017). The influence of hippocampal atrophy on the cognitive phenotype of dementia with Lewy bodies. Int. J. Geriatr. Psychiatry 32, 1182–1189. doi: 10.1002/gps.4719
Engvig, A., Fjell, A. M., Westlye, L. T., Skaane, N. V., Sundseth,Ø., and Walhovd, K. B. (2012). Hippocampal subfield volumes correlate with memory training benefit in subjective memory impairment. Neuroimage 61, 188–194. doi: 10.1016/j.neuroimage.2012.02.072
Finke, C., Prüss, H., Heine, J., Reuter, S., Kopp, U. A., Wegner, F., et al. (2016). Evaluation of cognitive deficits and structural hippocampal damage in encephalitis with leucine-rich, glioma-inactivated 1 antibodies. JAMA Neurol. 74, 50–59. doi: 10.1001/jamaneurol.2016.4226
Firbank, M. J., Blamire, A. M., Teodorczuk, A., Teper, E., Burton, E. J., Mitra, D., et al. (2010). High resolution imaging of the medial temporal lobe in Alzheimer’s disease and dementia with Lewy bodies. J. Alzheimers Dis. 21, 1129–1140. doi: 10.3233/JAD-2010-100138
Fischl, B., Liu, A., and Dale, A. M. (2001). Automated manifold surgery: constructing geometrically accurate and topologically correct models of the human cerebral cortex. IEEE Trans. Med. Imaging 20, 70–80. doi: 10.1109/42.906426
Fischl, B., Salat, D. H., Busa, E., Albert, M., Dieterich, M., Haselgrove, C., et al. (2016). Whole brain segmentation: automated labeling of neuroanatomical structures in the human brain. Neuron 33, 341–355. doi: 10.1016/S0896-6273(02)00569-X
Foo, H., Mak, E., Chander, R. J., Ng, A., Au, W. L., Sitoh, Y. Y., et al. (2017). Associations of hippocampal subfields in the progression of cognitive decline related to Parkinson’s disease. Neuroimage Clin. 14, 37–42. doi: 10.1016/j.nicl.2016.12.008
Fukutani, Y., Cairns, N. J., Shiozawa, M., Sasaki, K., Sudo, S., Isaki, K., et al. (2000). Neuronal loss and neurofibrillary degeneration in the hippocampal cortex in late-onset sporadic Alzheimer’s disease. Psychiatry Clin. Neurosci. 54, 523–529. doi: 10.1046/j.1440-1819.2000.00747.x
Fukutani, Y., Kobayashi, K., Nakamura, I., Watanabe, K., Isaki, K., and Cairns, N. J. (1995). Neurons, intracellular and extracellular neurofibrillary tangles in subdivisions of the hippocampal cortex in normal ageing and Alzheimer’s disease. Neurosci. Lett. 200, 57–60. doi: 10.1016/0304-3940(95)12083-G
Glasgow, S. D., and Chapman, C. A. (2007). Local generation of theta-frequency EEG activity in the parasubiculum. J. Neurophysiol. 97(6), 3868–3879. doi: 10.1152/jn.01306.2006
Guentchev, M., Hainfellner, J. A., Trabattoni, G. R., and Budka, H. (1997). Distribution of parvalbumin-immunoreactive neurons in brain correlates with hippocampal and temporal cortical pathology in creutzfeldt-jakob disease. J. Neuropathol. Exp. Neurol. 56, 1119–1124. doi: 10.1097/00005072-199710000-00005
Hagihara, H., Fujita, M., Umemori, J., Hashimoto, M., and Miyakawa, T. (2018). Immature-like molecular expression patterns in the hippocampus of a mouse model of dementia with Lewy body-linked mutant beta-synuclein. Mol. Brain 11:38. doi: 10.1186/s13041-018-0378-3
Hunsaker, M. R., Lee, B., and Kesner, R. P. (2008). Evaluating the temporal context of episodic memory: the role of CA3 and CA1. Behav. Brain Res. 188, 310–315. doi: 10.1016/j.bbr.2007.11.015
Iglesias, J. E., Augustinack, J. C., Nguyen, K., Player, C. M., Player, A., Wright, M., et al. (2015). A computational atlas of the hippocampal formation using ex vivo, ultra-high resolution MRI: application to adaptive segmentation of in vivo MRI. Neuroimage 115, 117–137. doi: 10.1016/j.neuroimage.2015.04.042
Iglesias, J. E., Van, L. K., Augustinack, J., Insausti, R., Fischl, B., and Reuter, M. (2016). Bayesian longitudinal segmentation of hippocampal substructures in brain MRI using subject-specific atlases. Neuroimage 141, 542–555. doi: 10.1016/j.neuroimage.2016.07.020
Kälin, A. M., Park, M. T., Chakravarty, M. M., Lerch, J. P., Michels, L., Schroeder, C., et al. (2017). Subcortical shape changes, hippocampal atrophy and cortical thinning in future Alzheimer’s disease patients. Front. Aging Neurosci. 9:38. doi: 10.3389/fnagi.2017.00038
Kalus, P., Braak, H., Braak, E., and Bohl, J. R. (1989). The presubicular region in Alzheimer’s disease: topography of amyloid deposits and neurofibrillary changes. Brain Res. 494, 198–203. doi: 10.1016/0006-8993(89)90164-9
Kaneko, M., Arai, K., Hattori, T., and Imai, T. (1999). Parahippocampal pathology in creutzfeldt-jakob disease. Clin. Neuropathol. 18, 9–16.
Kesner, R. P., and Giles, R. (1998). Neural circuit analysis of spatial working memory: role of pre- and parasubiculum, medial and lateral entorhinal cortex. Hippocampus 8, 416–423. doi: 10.1002/(SICI)1098-1063(1998)8:4<416::AID-HIPO9>3.0.CO;2-E
Lim, H. K., Hong, S. C., Jung, W. S., Ahn, K. J., Won, W. Y., Hahn, C., et al. (2013). Automated segmentation of hippocampal subfields in drug-naïve patients with Alzheimer disease. AJNR Am. J. Neuroradiol. 34, 747–751. doi: 10.3174/ajnr.A3293
Liu, P., Jarrard, L. E., and Bilkey, D. K. (2004). Excitotoxic lesions of the pre- and parasubiculum disrupt the place fields of hippocampal pyramidal cells. Hippocampus 14, 107–116. doi: 10.1002/hipo.10161
Macdonald, J. D. (1997). A Method for Identifying Geometrically Simple Surfaces From Three Dimensional Images. ıMontreal, QC: Mcgill University.
Mak, E., Gabel, S., Su, L., Williams, G. B., Arnold, R., Passamonti, L., et al. (2017). Multi-modal MRI investigation of volumetric and microstructural changes in the hippocampus and its subfields in mild cognitive impairment, Alzheimer’s disease, and dementia with Lewy bodies. Int. Psychogeriatr. 29, 545–555. doi: 10.1017/S1041610216002143
Mak, E., Li, S., Williams, G. B., Watson, R., Firbank, M., Blamire, A., et al. (2016). Differential atrophy of hippocampal subfields: a comparative study of dementia with Lewy bodies and Alzheimer disease. Am. J. Geriatr. Psychiatry 24, 136–143. doi: 10.1016/j.jagp.2015.06.006
Malykhin, N. V., Huang, Y., Hrybouski, S., and Olsen, F. (2017). Differential vulnerability of hippocampal subfields and anteroposterior hippocampal subregions in healthy cognitive aging. Neurobiol. Aging 59, 121–134. doi: 10.1016/j.neurobiolaging.2017.08.001
Mckeith, I. G., Dickson, D. W., Lowe, J., Emre, M., O’Brien, J. T., Feldman, H., et al. (2005). Diagnosis and management of dementia with Lewy bodies: third report of the DLB consortium. Neurology 65, 1863–1872. doi: 10.1212/01.wnl.0000187889.17253.b1
Mueller, S. G., Chao, L. L., Berman, B., and Weiner, M. W. (2011). Evidence for functional specialization of hippocampal subfields detected by MR subfield volumetry on high resolution images at 4T. Neuroimage 56, 851–857. doi: 10.1016/j.neuroimage.2011.03.028
Mueller, S. G., Laxer, K. D., Scanlon, C., Garcia, P., Mcmullen, W. J., Loring, D. W., et al. (2012). Different structural correlates for verbal memory impairment in temporal lobe epilepsy with and without mesial temporal lobe sclerosis. Hum. Brain Mapp. 33, 489–499. doi: 10.1002/hbm.21226
Pang, C. C., Kiecker, C., O’Brien, J. T., Noble, W., and Chang, R. C. (2018). Ammon’s Horn 2 (CA2) of the hippocampus: a long-known region with a new potential role in neurodegeneration. Neuroscientist doi: 10.1177/1073858418778747 [Epub ahead of print].
Perrotin, A., De, F. R., Lamberton, F., Poisnel, G., La, J. R., de la Sayette, V., et al. (2015). Hippocampal subfield volumetry and 3D surface mapping in subjective cognitive decline. J. Alzheimers Dis. Jad 48 (Suppl 1), S141–S150. doi: 10.3233/JAD-150087
Platero, C., Lin, L., and Tobar, M. C. (2018). Longitudinal neuroimaging hippocampal markers for diagnosing Alzheimer’s disease. Neuroinformatics doi: 10.1007/s12021-018-9380-2 [Epub ahead of print],.
Reagh, Z. M., Watabe, J., Ly, M., Murray, E., and Yassa, M. A. (2014). Dissociated signals in human dentate gyrus and CA3 predict different facets of recognition memory. J. Neurosci. Official J. Soc. Neurosci. 34, 13301–13313. doi: 10.1523/JNEUROSCI.2779-14.2014
Remondes, M., and Schuman, E. M. (2004). Role for a cortical input to hippocampal area CA1 in the consolidation of a long-term memory. Nature 431, 699–703. doi: 10.1038/nature02965
Richter-Levin, G. (2004). The amygdala, the hippocampus, and emotional modulation of memory. Neuroscientist 10, 31–39. doi: 10.1177/1073858403259955
Riggins, T., Geng, F., Botdorf, M., Canada, K., Cox, L., and Hancock, G. R. (2018). Protracted hippocampal development is associated with age-related improvements in memory during early childhood. Neuroimage 174, 127–137. doi: 10.1016/j.neuroimage.2018.03.009
Rössler, M., Zarski, R., Bohl, J., and Ohm, T. G. (2002). Stage-dependent and sector-specific neuronal loss in hippocampus during Alzheimer’s disease. Acta Neuropathol. 103, 363–369. doi: 10.1007/s00401-001-0475-7
Saygin, Z. M., Kliemann, D., Iglesias, J. E., van der Kouwe, A. J. W., Boyd, E., Reuter, M., et al. (2017). High-resolution magnetic resonance imaging reveals nuclei of the human amygdala: manual segmentation to automatic atlas. Neuroimage 155, 370–380. doi: 10.1016/j.neuroimage.2017.04.046
Scheff, S. W., Price, D. A., Schmitt, F. A., and Mufson, E. J. (2006). Hippocampal synaptic loss in early Alzheimer’s disease and mild cognitive impairment. Neurobiol. Aging 27, 1372–1384. doi: 10.1016/j.neurobiolaging.2005.09.012
Schönheit, B., Zarski, R., and Ohm, T. G. (2004). Spatial and temporal relationships between plaques and tangles in Alzheimer-pathology. Neurobiol. Aging 25, 697–711. doi: 10.1016/j.neurobiolaging.2003.09.009
Segonne, F., Pacheco, J., and Fischl, B. (2007). Geometrically accurate topology-correction of cortical surfaces using nonseparating loops. IEEE Trans. Med. Imaging 26, 518–529. doi: 10.1109/TMI.2006.887364
Shing, Y. L., Rodrigue, K. M., Kennedy, K. M., Fandakova, Y., Bodammer, N., Werkle-Bergner, M., et al. (2011). Hippocampal subfield volumes: age, vascular risk, and correlation with associative memory. Front. Aging Neurosci. 3:2. doi: 10.3389/fnagi.2011.00002
Stav, A. L., Johansen, K. K., Auning, E., Kalheim, L. F., Selnes, P., Bjørnerud, A., et al. (2016). Hippocampal subfield atrophy in relation to cerebrospinal fluid biomarkers and cognition in early Parkinsonâ€s disease: a cross-sectional study. NPJ Parkinsons Dis. 2:15030. doi: 10.1038/npjparkd.2015.30
Tamnes, C. K., Bos, M., Van, F. D. K., Peters, S., and Crone, E. A. (2018). Longitudinal development of hippocampal subregions from childhood to adulthood. Dev. Cogn. Neurosci. 30, 212–222. doi: 10.1016/j.dcn.2018.03.009
Tang, Q., Burgalossi, A., Ebbesen, C. L., Sanguinetti-Scheck, J. I., Schmidt, H., Tukker, J. J., et al. (2016). Functional architecture of the rat parasubiculum. J. Neurosci. Official J. Soc. Neurosci. 36, 2289–2301. doi: 10.1523/JNEUROSCI.3749-15.2016
Tomaiuolo, F., Carlesimo, G. A., Di, P. M., Petrides, M., Fera, F., Bonanni, R., et al. (2004). Gross morphology and morphometric sequelae in the hippocampus, fornix, and corpus callosum of patients with severe non-missile traumatic brain injury without macroscopically detectable lesions: a T1 weighted MRI study. J. Neurol. Neurosurg. Psychiatry 75, 1314–1322. doi: 10.1136/jnnp.2003.017046
Vago, D. R., Bevan, A., and Kesner, R. P. (2007). The role of the direct perforant path input to the CA1 subregion of the dorsal hippocampus in memory retention and retrieval. Hippocampus 17, 977–987. doi: 10.1002/hipo.20329
Van, P. C. (2004). Relationship between hippocampal volume and memory ability in healthy individuals across the lifespan: review and meta-analysis. Neuropsychologia 42, 1394–1413. doi: 10.1016/j.neuropsychologia.2004.04.006
Van Leemput, K., Bakkour, A., Benner, T., Wiggins, G., Wald, L. L., Augustinack, J., et al. (2009). Automated segmentation of hippocampal subfields from ultra-high resolution in vivo MRI. Hippocampus 19, 549–557. doi: 10.1002/hipo.20615
Weidensteiner, C., Metzger, F., Bruns, A., Bohrmann, B., Kuennecke, B., and Von, K. M. (2009). Cortical hypoperfusion in the B6.PS2APP mouse model for Alzheimer’s disease: comprehensive phenotyping of vascular and tissular parameters by MRI. Magn. Reson. Med. 62, 35–45. doi: 10.1002/mrm.21985
Wible, C. G., Shiber, J. R., and Olton, D. S. (1992). Hippocampus, fimbria-fornix, amygdala, and memory: object discriminations in rats. Behav. Neurosci. 106, 751–761. doi: 10.1037/0735-7044.106.5.751
Winters, B. D., and Dunnett, S. B. (2004). Selective lesioning of the cholinergic septo-hippocampal pathway does not disrupt spatial short-term memory: a comparison with the effects of fimbria-fornix lesions. Behav. Neurosci. 118, 546–562. doi: 10.1037/0735-7044.118.3.546
Wisse, L. E. M., Geert Jan, B., Heringa, S. M., Kuijf, H. J., Koek, D. H. L., Luijten, P. R., et al. (2014). Hippocampal subfield volumes at 7T in early Alzheimer’s disease and normal aging. Neurobiol. Aging 35, 2039–2045. doi: 10.1016/j.neurobiolaging.2014.02.021
Yamaguchi, H., Hirai, S., Morimatsu, M., Shoji, M., and Ihara, Y. (1988). A variety of cerebral amyloid deposits in the brains of the Alzheimer-type dementia demonstrated by β protein immunostaining. Acta Neuropathol. 76, 541–549. doi: 10.1007/BF00689591
Yassa, M. A., and Stark, C. E. L. (2011). Pattern separation in the hippocampus. Trends Neurosci. 34, 515–525. doi: 10.1016/j.tins.2011.06.006
Zammit, A. R., Ezzati, A., Zimmerman, M. E., Lipton, R. B., Lipton, M. L., and Katz, M. J. (2017). Roles of hippocampal subfields in verbal and visual episodic memory. Behav. Brain Res. 317, 157–162. doi: 10.1016/j.bbr.2016.09.038
Keywords: hippocampal subfields, lifespan, immediate recall, delayed recall, memory decline
Citation: Zheng F, Cui D, Zhang L, Zhang S, Zhao Y, Liu X, Liu C, Li Z, Zhang D, Shi L, Liu Z, Hou K, Lu W, Yin T and Qiu J (2018) The Volume of Hippocampal Subfields in Relation to Decline of Memory Recall Across the Adult Lifespan. Front. Aging Neurosci. 10:320. doi: 10.3389/fnagi.2018.00320
Received: 28 June 2018; Accepted: 24 September 2018;
Published: 10 October 2018.
Edited by:
Panteleimon Giannakopoulos, Université de Genève, SwitzerlandReviewed by:
Lu Zhang, Georgia Institute of Technology, United StatesStefano Delli Pizzi, Università degli Studi “G. d’Annunzio" Chieti - Pescara, Italy
Copyright © 2018 Zheng, Cui, Zhang, Zhang, Zhao, Liu, Liu, Li, Zhang, Shi, Liu, Hou, Lu, Yin and Qiu. This is an open-access article distributed under the terms of the Creative Commons Attribution License (CC BY). The use, distribution or reproduction in other forums is permitted, provided the original author(s) and the copyright owner(s) are credited and that the original publication in this journal is cited, in accordance with accepted academic practice. No use, distribution or reproduction is permitted which does not comply with these terms.
*Correspondence: Tao Yin, Ym1lNTAwQDE2My5jb20= Jianfeng Qiu, amZxaXUxMDBAZ21haWwuY29t
†These authors have contributed equally to this work