- 1Department of Neuropsychiatry, Seoul National University Hospital, Seoul, South Korea
- 2Department of Psychiatry, Yonsei University College of Medicine, Seoul, South Korea
- 3Institute of Human Behavioral Medicine, Medical Research Center, Seoul National University, Seoul, South Korea
- 4Department of Psychiatry, Seoul National University College of Medicine, Seoul, South Korea
- 5Institute of Behavioral Science in Medicine, Yonsei University College of Medicine, Seoul, South Korea
Background: Although increased cognitive activity (CA), both current and past, is known to be associated with a decreased occurrence of Alzheimer’s disease (AD) dementia in older adults, the exact neural mechanisms underlying the association between CA during different stages of life and human dementia remain unclear. Therefore, we investigated whether CA during different life stages is associated with cerebral amyloid-beta (Aβ) pathology and AD-related neurodegeneration in non-demented older adults.
Methods: Cross-sectional analyses of data collected between April 2014 and March 2016 from the Korean Brain Aging Study for Early Diagnosis and Prediction of Alzheimer’s Disease (KBASE), an ongoing prospective cohort. In total, 321 community-dwelling, non-demented older adults were involved in this study. Cerebral Aβ deposition and Aβ positivity were measured using 11C-Pittsburgh compound B (PiB)-positron emission tomography (PET). AD-signature region cerebral glucose metabolism (AD-CMglu) and AD-signature region neurodegeneration (AD-ND) positivity were measured using 18F-fluorodeoxyglucose (FDG)-PET. In addition, CA in early, mid, and late life was systematically evaluated using a structured questionnaire.
Results: Of the 321 participants, 254 were cognitively normal (CN) and 67 had mild cognitive impairment (MCI). The mean age of participants was 69.6 years old [standard deviation (SD) = 8.0]. Higher early-life CA (CAearly) was associated with significantly increased AD-CMglu (B = 0.035, SE = 0.013, P = 0.009) and a decreasing trend of AD-ND positivity (OR = 0.65, 95% CI 0.43–0.98, P = 0.04) but was not associated with Aβ deposition or positivity. We observed no association between midlife CA (CAmid) and any AD-related brain changes. Late-life CA (CAlate) showed an association with both global Aβ deposition and AD-CMglu, although it was not statistically significant. Sensitivity analyses controlling for current depression or conducted only for CN individuals revealed similar results.
Conclusion: Our results suggest that CA in early life may be protective against late-life AD-related neurodegeneration, independently of cerebral Aβ pathology.
Introduction
Increased cognitive activity (CA), both current and past, is known to be associated with reduced cognitive decline (Marquine et al., 2012; Wilson et al., 2012, 2013; Hughes et al., 2015; Arfanakis et al., 2016) and the occurrence of Alzheimer’s disease (AD) dementia (Wilson et al., 2002a, 2007; Sattler et al., 2012) in the elderly. However, the exact pathological process underlying this inverse association between CA and AD dementia remains unclear.
To explore the pathological process, several studies investigated the association between the degree of CA and both cerebral amyloid-beta (Aβ) pathology (Landau et al., 2012; Vemuri et al., 2012, 2016, 2017; Wirth et al., 2014; Gidicsin et al., 2015) and neurodegeneration (Valenzuela et al., 2008; Vemuri et al., 2012, 2016, 2017; Gidicsin et al., 2015) using in vivo AD neuroimaging biomarkers. The results from these studies are, however, controversial. One possible explanation for this controversy is that the brain has different physiological or pathological properties during different stages of life. The influence of a certain life experience, such as CA, on the brain may vary at different stages of life. Nevertheless, most previous studies exploring the association between CA and AD biomarkers did not take into account different life stages and simply classified all CA into simple categories, mainly current or past (Landau et al., 2012; Vemuri et al., 2012; Gidicsin et al., 2015), or focused only on either midlife CA (CAmid) (Vemuri et al., 2016, 2017) or late-life CA (CAlate) (Valenzuela et al., 2008).
Early life (i.e., childhood and early adulthood) is a critical period for brain development characterized by neural plasticity (Chugani et al., 1987; Andersen, 2003; Dekhtyar et al., 2016). Previous studies have shown that early-life CA (CAearly) is associated with reduced late-life cognitive decline and progression to cognitive disorders in later life (Wilson et al., 2013, 2015; Dekhtyar et al., 2016), suggesting that CAearly is closely related to increases in cognitive reserve (CR). CR refers to functional rather than structural or quantitative aspects of the brain, and may explain why some people are more resilient to cognitive decline than others who present with the same level of pathology (Stern, 2012). In contrast, CA in mid or late life stages is less beneficial to individuals, given that brain plasticity is limited during mid- and late life (Leuner et al., 2007; Kolb and Gibb, 2011).
The accumulation of cerebral Aβ pathology begins 10–20 years prior to AD dementia (Villemagne et al., 2013) and its prevalence in non-demented persons typically increases from mid- to late life (Jansen et al., 2015). Thus, cerebral Aβ pathology is rarely observed in the early-life period. Therefore, it is more reasonable to assume that CA or other brain affecting activities may influence the occurrence of Aβ pathology when they are applied in mid or late life rather than in early life. Some studies have reported an association between CAmid and Aβ deposition (Wirth et al., 2014; Vemuri et al., 2016). In the case of late life, however, about half of the cognitively healthy elderly already have amyloid or neurodegeneration abnormalities and the estimated frequency of normal AD biomarker status decreases continuously with age (Jack et al., 2014). Therefore, the accumulation of amyloid and/or neurodegeneration itself might reduce participation in CA in late life, although a few studies have reported a beneficial effect of congitive training or exercise in late life on brain function as well as cognive performance (Snowball et al., 2013; Shah et al., 2014; Lampit et al., 2015).
We hypothesized that CA during different stages of life is differentially associated with cerebral Aβ pathology and AD-related neurodegeneration in non-demented older adults. More specifically, we formulated three working hypotheses. First, CAearly is inversely associated with the degree of AD-related neurodegeneration, including neuronal or synaptic dysfunction in late life. Second, CAmid is inversely associated with cerebral Aβ pathology in late life. Third, CAlate is inversely associated with both cerebral Aβ pathology and AD-related neurodegeneration in late life.
To test our hypotheses, we measured cerebral Aβ pathology using 11C-Pittsburgh compound B (PiB)-positron emission tomography (PET) and AD-related neurodegeneration using 18F-fluorodeoxyglucose (FDG)-PET. We selected cerebral glucose metabolism (CMglu) on FDG-PET as a neurodegeneration marker because it is a reliable index of regional neuronal or synaptic function (Sokoloff, 1981; Jueptner and Weiller, 1995), and specific regional hypometabolism in the temporo-parietal cortices is a reliable and sensitive measure of AD-related neurodegeneration, which appears earlier than structural brain changes on magnetic resonance imaging (MRI) (Jack et al., 2014, 2015, 2016). CA in early, mid, and late life was assessed using a structured questionnaire (Wilson et al., 2005, 2007; Barnes et al., 2006). We further investigated the moderating effects of apolipoprotein E ε4 (APOE4) on the relationship between CA and AD-related brain changes, as CA is particularly protective in APOE4 carriers for the risk of dementia onset (Carlson et al., 2008) and Aβ accumulation (Wirth et al., 2014; Vemuri et al., 2016).
Materials and Methods
Participants
This study was part of the Korean Brain Aging Study for Early Diagnosis and Prediction of Alzheimer’s Disease (KBASE), an ongoing prospective cohort study, which began in 2014 and was designed to identify novel biomarkers for AD and explore various lifetime experiences contributing to AD-related brain changes. The current study included 321 community-dwelling elderly individuals without dementia who were at least 55 years old and enrolled between April 2014 and March 2016.
The study participants consisted of 254 cognitively normal (CN) and 67 subjects with mild cognitive impairment (MCI). All individuals with MCI met the current consensus criteria for amnestic MCI: (1) memory complaints confirmed by an informant; (2) objective memory impairment, (3) preserved global cognitive function; (4) independence in functional activities; and (5) no dementia. All MCI individuals had a global clinical dementia rating (CDR) of 0.5. In terms of Criterion 2, the age-, education-, and gender-adjusted z-scores for at least 1 of the 4 episodic memory tests was less than -1.0. The four memory tests included Word List Memory, Word List Recall, Word List Recognition, and Constructional Recall tests, which are included in the Korean version of the Consortium to Establish a Registry for Alzheimer’s Disease (CERAD-K) neuropsychological battery. The CN group consisted of participants with a global CDR of 0 and lack of an MCI or dementia diagnosis. The exclusion criteria were current serious medical, psychiatric, or neurological disorders that may influence mental functioning; the presence of severe communication problems that would hinder the clinical interview or brain imaging process; in vivo devices or a mental status that prevented us from performing the brain MRI; absence of a reliable informant; illiteracy; participation in a different clinical trial; and treatment with an investigational product. The Institutional Review Board of the Seoul National University Hospital and Seoul Metropolitan Government-Seoul National University Boramae Medical Center, South Korea, approved this study, and subjects and their legal representatives provided written consent.
Clinical Assessment
All participants received standardized clinical assessments by trained psychiatrists based on the KBASE clinical assessment protocol, which incorporated the CERAD-K (Lee et al., 2002). KBASE neuropsychological assessments incorporating the CERAD-K neuropsychological battery (Lee et al., 2004) were also administered to all participants by trained neuropsychologists. Genomic DNA was extracted from whole blood and apolipoprotein E (APOE) genotyping was performed as described previously (Wenham et al., 1991). APOE4 carrier status was considered positive if the participant had at least one APOE4 allele.
Assessment of Early-, Mid-, and Late-Life CA
Participant CA was assessed using a 39-item expanded version (Wilson et al., 2005, 2007; Barnes et al., 2006) of a previously reported 25-item autobiographical questionnaire (Wilson et al., 2003; Landau et al., 2012), which was shown to have sufficient internal consistency and temporal stability. Items included relatively common activities with few barriers to participation, such as reading newspapers, magazines, or books; visiting a museum or library; attending a concert, play, or musical; writing letters; and playing games. Individuals completed the questionnaire at a baseline evaluation point. Frequency of participation was rated from 1 (once a year or less) to 5 (daily or approximately daily). There were 9 current (i.e., late life) activities and 30 previous activities including 11 related to childhood (6–12 years of age), 10 related to young adulthood (18 years of age), and 9 related to midlife (40 years of age). Item scores were averaged to yield separate values for each age period. The CAearly score was determined by averaging childhood and young adulthood scores.
PiB-PET Acquisition and Processing
Participants underwent simultaneous three-dimensional (3D) PiB-PET and 3D T1-weighted MRI using a 3.0T Biograph mMR (PET-MR) scanner (Siemens, Washington, DC, United States) according to the manufacturer’s protocols. Details of PiB-PET imaging acquisition and preprocessing are described elsewhere (Supplementary Material).
The automatic anatomic labeling algorithm and a region combining method (Reiman et al., 2009) were conducted to determine regions of interest (ROIs) and to characterize the PiB retention level in the frontal, lateral parietal, posterior cingulate-precuneus, and lateral temporal regions. The standardized uptake value ratios (SUVRs) were calculated by dividing the mean value for all voxels within each ROI by the mean cerebellar uptake value in the same image. Each participant was classified as cerebral Aβ positive if the SUVR value was >1.4. A global cortical ROI consisting of the four ROIs was defined, and a global Aβ deposition value was generated by dividing the mean value for all voxels of the global cortical ROI by the mean cerebellar uptake value in the same image (Choe et al., 2014).
FDG-PET Acquisition and Processing
Participants also underwent FDG-PET imaging using the same PET-MR machine, as described above. Details of FDG-PET image acquisition and preprocessing are described in the Supplementary Material. AD-signature FDG ROIs including the angular gyri, posterior cingulate cortex, and inferior temporal gyri, which are known to be sensitive to changes associated with AD (Jack et al., 2014, 2015) were determined. AD-signature region cerebral glucose metabolism (AD-CMglu) was defined as a voxel-weighted mean SUVR extracted from the AD-signature FDG ROIs, and AD-signature region neurodegeneration (AD-ND) positivity was defined as AD-CMglu <1.386. Detailed methods used to define the threshold for abnormality of each neurodegeneration biomarker are described in the Supplementary Material.
Statistical Analysis
The associations between CA (independent variable) at each life stage and global Aβ deposition or AD-CMglu (dependent variables) were examined using multiple linear regression analyses controlling for age, sex, years of education, and APOE4 carrier status as covariates. Multiple logistic regression analyses were conducted to test the association between CA at each life stage (independent variable) and Aβ or AD-ND positivity (independent variables). In this analysis, we also controlled for age, sex, years of education, and APOE4 carrier status. Sensitivity analyses were conducted using the same analyses, but included only CN subjects to exclude the possibility of recall bias due to MCI. We also performed the same analyses but additionally controlled for geriatric depression using the Geriatric Depression Scale (GDS) (Yesavage et al., 1983), since current depression may influence CA and brain state. We set a P-value less than 0.0167 (=0.05/3) as the threshold for statistical significance, given that CA during the three life stages (i.e., CAearly, CAmid, and CAlate) were explored for AD-related brain changes. In the event that CA significantly influences Aβ-related brain changes, we further explored the moderating effects of APOE4 using a generalized linear model analysis, including a CA × APOE4 interaction term, as well as CA and APOE4 as independent variables, controlling for age, sex, and education as covariates. In this case, a P-value less than 0.05 was indicative of statistical significance. All statistical analyses were conducted using SPSS Statistics version 23.0 (IBM Corp., Armonk, NY, United States).
Results
The characteristics of the study participants are shown in Table 1. Both global Aβ deposition and AD-CMglu were weakly correlated with clinical variables. Global Aβ deposition was inversely associated with CERAD total score (Kendall’s tau = -0.19, p < 0.001) and was positively associated with CDR sum of boxes (Kendall’s tau = 0.33, p < 0.001). AD-CMglu showed a similar association with CERAD total score (Kendall’s tau = 0.16, p < 0.001) and was inversely associated with CDR-SOB (Kendall’s tau = -0.26, p < 0.001). Global Aβ deposition and AD-CMglu were weakly correlated with each other (Kendall’s tau = -0.17, P < 0.001). CAearly was moderately correlated with CAmid (Kendall’s tau = 0.52, P < 0.001) and CAlate (Kendall’s tau = 0.43, P < 0.001). CAmid and CAlate were also moderately correlated (Kendall’s tau = 0.51, P < 0.001).
Early-Life CA and AD-Related Brain Changes
We observed no association between CAearly and global Aβ deposition (Figure 1A and Table 2). Similarly, no significant association between CAearly and Aβ positivity was observed (Table 3). In contrast, there was a significant positive association between CAearly and AD-CMglu (Figure 1B and Table 2). We observed a trend for a negative association between CAearly and AD-ND positivity, although this was not statistically significant (Table 3). We explored moderation effects of APOE4 on the association between CAearly and AD-CMglu, which showed a statistically significant result in the main effect analysis. We observed no CAearly × APOE4 interaction on AD-CMglu (Supplementary Table e-1).
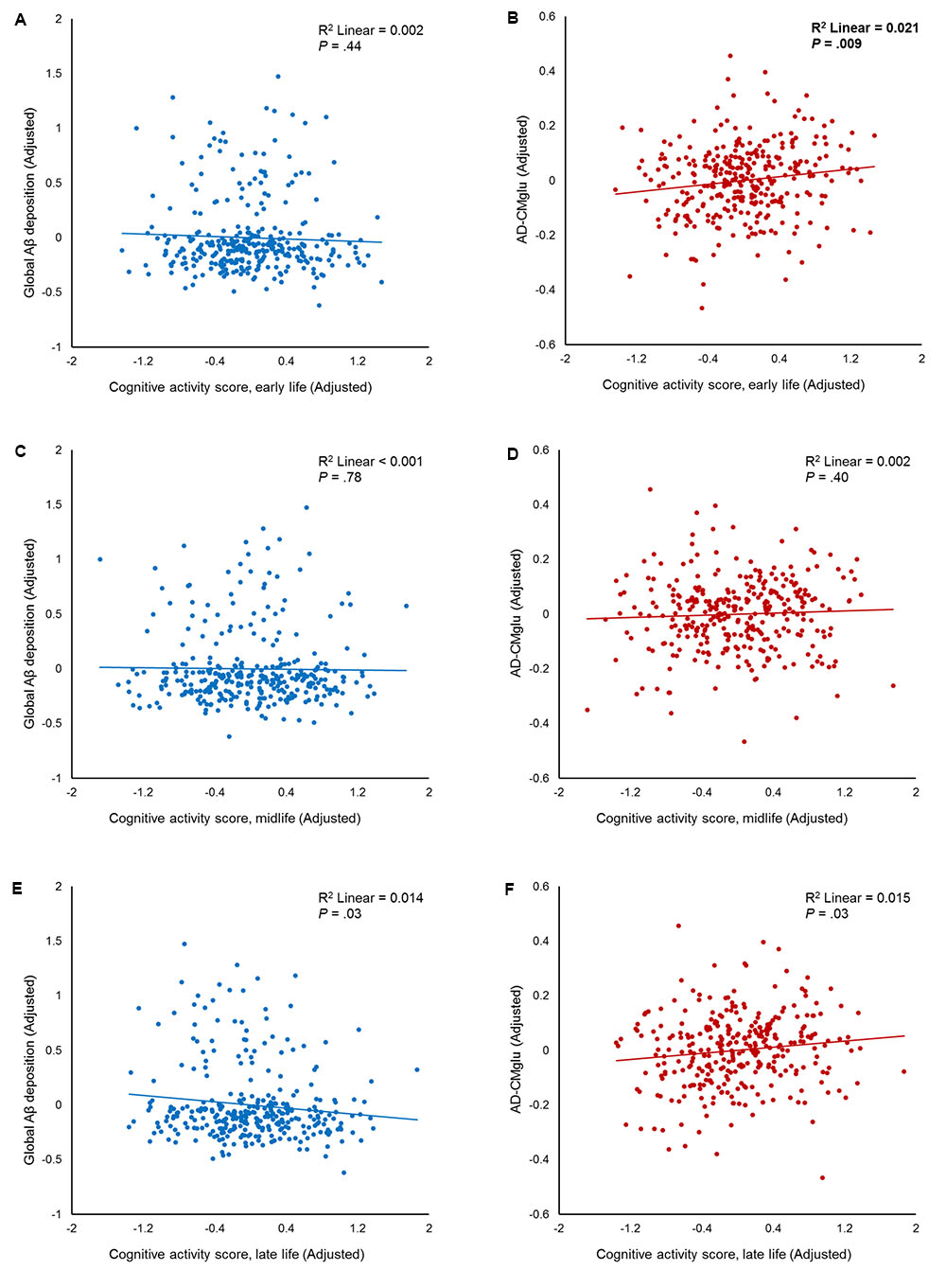
FIGURE 1. Partial regression plots showing the effect of participating in cognitive activity (CA) during each life period. The results of the independent multiple linear regression model with age, gender, education, and apolipoprotein E ε4 as covariates are presented. (A,B) Plots for early-life CA (CAearly) and amyloid-beta (Aβ), as well as for Alzheimer’s disease signature region cerebral glucose metabolism (AD-CMglu). A significant association was observed between CAearly and AD-CMglu, but not Aβ. (C,D) Plots for midlife CA (CAmid) and Aβ, as well as for AD-CMglu. (E,F) Plots for late-life CA (CAlate) and Aβ, as well as for AD-CMglu.
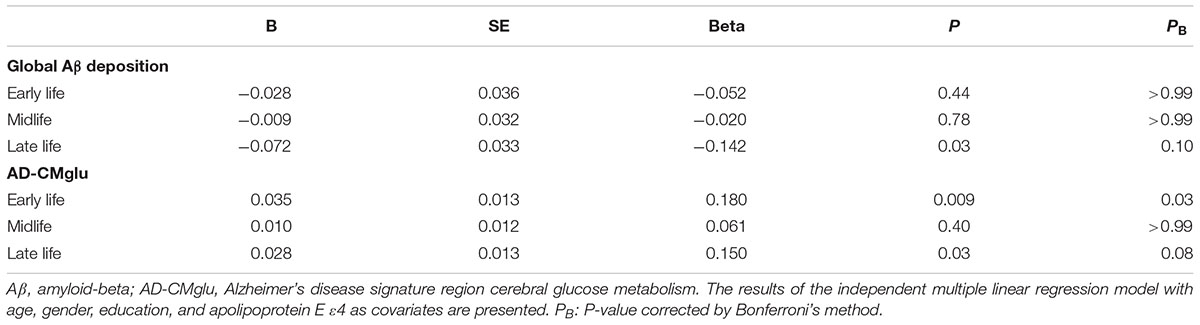
TABLE 2. Association between cognitive activities (CAs) in each life period and global cerebral amyloid-beta (Aβ) deposition and Alzheimer’s disease signature region cerebral glucose metabolism (AD-CMglu).
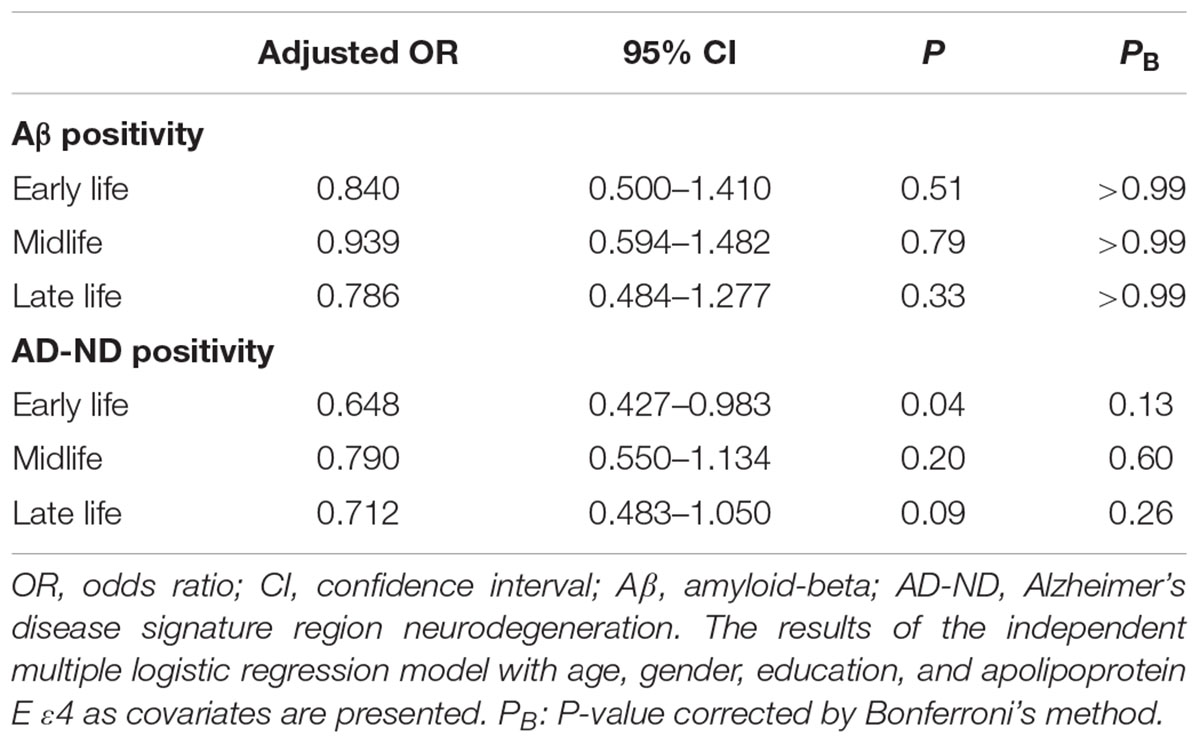
TABLE 3. Association between cognitive activities in each life period and Aβ and AD-signature region neurodegeneration (AD-ND) positivity.
Midlife CA and AD-Related Brain Changes
We observed no association between CAmid and global Aβ deposition or AD-CMglu (Figures 1C,D and Table 2). CAmid was also not associated with Aβ or AD-ND positivity (Table 3).
Late-Life CA and AD-Related Brain Changes
We observed a trend-level association between CAlate and both global Aβ deposition and AD-CMglu, although this association was not significant (Figures 1E,F and Table 2). CAlate was not associated with Aβ or AD-ND positivity (Table 3).
Sensitivity Analysis
Even when the GDS was additionally controlled for age, education, gender, and APOE4, the results from the multiple linear or logistic regression analyses were similar (Supplementary Tables e-2, e-3). When the same analyses were conducted for the CN subgroup only, CAearly showed trend-level associations with AD-CMglu and AD-ND positivity (Supplementary Tables e-4, e-5), although the association was not statistically significant. We observed no association between CAmid or CAlate and any AD-related brain changes. Moreover, because CAearly was correlated with CAmid and CAlate, we controlled for CAearly in addition to age, sex, education, and APOE4 when analyzing the relationship of CAmid or CAlate to AD-related brain changes. As shown in the Supplementary Tables e-6, e-7, the results were almost the same, even after controlling for the effects of CAearly.
Discussion
The results of this study generally support the hypothesis that CA during different life stages is differentially associated with cerebral Aβ pathology and AD-related neurodegeneration in non-demented older adults. With regard to the three working hypotheses, our findings supported the first hypothesis: CAearly was inversely associated with the degree of AD-related neurodegeneration in late life. In contrast, we could not accept the second hypothesis (i.e., an inverse association between CAmid and cerebral Aβ pathology in late life) or the third (i.e., a significant inverse association between CAlate and both cerebral Aβ pathology and AD-related neurodegeneration in late life).
Our study is the first to verify the association between CAearly and AD-CMglu in late life, suggesting the presence of a potential mechanism underlying the inverse association between CAearly and AD dementia or cognitive decline. Previous studies have reported that childhood CA could reduce cognitive decline (Wilson et al., 2013) and music or foreign language training in early life was associated with a lower risk of MCI or AD dementia (Wilson et al., 2015). Another study showed that a complex occupation could not compensate for low school grades at a young age to prevent dementia, suggesting that early life is a critical period for increasing CR against dementia (Dekhtyar et al., 2016). To the best of our knowledge, no previous human studies have focused on the direct relationship between CAearly and brain changes in late life.
The association between CAearly and AD-CMglu in late life may be explained by the influence of CAearly on brain developmental processes (Chugani et al., 1987; Benes et al., 1994; Paus et al., 1999; Andersen, 2003), such as synaptogenesis and pruning during the early-life period in particular (Tau and Peterson, 2010). As activity-dependent mechanisms could modulate these processes, especially in early life (Bourgeois et al., 1989; Goodman and Shatz, 1993; Hata and Stryker, 1994; Kleim et al., 1996; Baker et al., 2017), it may be that CAearly promotes synaptogenesis and/or pruning in humans offers a plausible explanation. Metabolic changes measured by FDG-PET may reflect energy expenditures of these processes (Chugani et al., 1987). Other animal studies also suggest that early-life cognitive enrichment has various protective effects on the brain by increasing neurotrophic factors (Wolf et al., 2006) or gene/protein expression related to synaptic plasticity (Costa et al., 2007). However, the influence of common genetic predisposition cannot be completely excluded when addressing the association between CAearly and neurodegeneration in late life. A certain genetic factor may be related to both more CA participation in early life and less neurodegeneration in late life (Fox et al., 2010).
Educational level is associated with the level of CA, regardless of life period (Wilson et al., 2002b, 2013; Barnes et al., 2006; Gidicsin et al., 2015). Our data also show a similar association between years of education and CAearly (Kendall’s tau = 0.43, P < 0.001), CAmid (Kendall’s tau = 0.48, P < 0.001), and CAlate (Kendall’s tau = 0.45, P < 0.001). A previous report showed that higher-level education, related to early-life enrichment, was associated with reduced age-related alterations of cerebrospinal fluid (CSF) neurodegeneration biomarkers (e.g., CSF total-tau, phosphorylated-tau), but not with amyloid biomarkers (CSF Aβ) (Almeida et al., 2015), similar to our observation for CAearly, Aβ pathology, and neurodegeneration. Nevertheless, because the aim of this study was to investigate the differential effect of CA during different life stages on in vivo AD pathology, we applied a lifetime CA questionnaire instead of simply using years of education as a measure of CA. In the current study, CAearly had a significant inverse relationship with AD-related neurodegeneration, while CAmid and CAlate did not, after controlling for the level of education. This finding suggests that CAearly itself is potentially protective against late-life neurodegeneration or related cognitive decline, regardless of educational attainment.
An exploratory analysis to investigate the moderating effects of APOE4 revealed no significant interaction between CAearly and APOE4 on AD-CMglu. This finding may be explained by previous reports indicating that APOE4-related cognitive changes generally occur during mid or late life, as opposed to early life (Ruiz et al., 2010; Richter-Schmidinger et al., 2011; Wisdom et al., 2011). A previous meta-analysis of 20 studies also demonstrated that APOE4 was not associated with cognitive function in young adults, adolescents, or children (Ihle et al., 2012).
Midlife CA was not associated with late-life Aβ deposition, which did not support our second hypothesis. Similar to our current finding, Mayo investigators reported no association between CAmid and late-life Aβ deposition, in general, in non-demented elderly (Vemuri et al., 2016, 2017). They also showed that high CAmid was associated with lower Aβ deposition in highly educated APOE4 carriers (Vemuri et al., 2016). They proposed that a reverse causality may explain their finding: among highly educated APOE4 carriers, those with higher Aβ deposition in middle age are most likely to experience subtle cognitive symptoms at that time and, consequently, avoid intellectual activity (Vemuri et al., 2016). We conducted similar analyses for highly educated (>14 years) APOE4 carriers, but did not find any significant associations between CAmid and Aβ deposition. Such discrepancies may be associated with the time frame for CAmid. We defined CAmid as CA at the age of 40 years, while Mayo investigators measured CAmid at 50–65 years of age. Younger individuals are less likely to be influenced by the reverse causality issue. With respect to neurodegeneration, no association between CAmid and AD-CMglu or AD-ND positivity was observed, which is consistent with previous reports (Vemuri et al., 2016, 2017).
Although not statistically significant, CAlate showed a trend association with global Aβ deposition and AD-CMglu. This may be explained by reverse causality: as previously mentioned in the section “Introduction”; elderly individuals with greater AD pathologies may participate in less CA (Jack et al., 2013; Villemagne et al., 2013). This explanation was further supported by the sensitivity analysis conducted for the CN subgroup. In the CN subgroup, no trend level association was observed between CAlate and AD-related brain changes, which is consistent with previous reports (Landau et al., 2012; Wirth et al., 2014; Gidicsin et al., 2015).
In a sensitivity analysis, we controlled for the effect of CAearly as well as education when analyzing the relationship between CAmid or CAlate and global Aβ deposition and AD-CMglu, because CAmid and CAlate were correlated with CAearly. Controlling for CAearly did not change the results, indicating that the negative findings for the relationship of CAmid or CAlate with AD-related brain change were significant, regardless of the influence of CAearly.
There are several limitations to our study. First, although we used well-validated and reliable questionnaires, retrospective measurements of CA may have a recall bias. Current depression and memory impairment have the potential to affect retrospective measurements based on subjective recall. To mitigate the potential risk, we conducted two sensitivity analyses. We controlled for current depression using the GDS score. This did not change the overall results of our study. Furthermore, the same analyses conducted for the CN group revealed potential associations between CAearly and both AD-CMglu and AD-ND positivity, although not statistically significant. Future long-term prospective studies are required to confirm our findings. Second, as for AD-related neurodegeneration, we measured cerebral glucose metabolism by FDG-PET. Although we defined AD-CMglu or AD-ND positivity by applying AD-signature regions showing typical AD-pattern hypometabolism, glucose metabolism may be influenced by non-AD pathologies, such as vascular pathology and non-AD degenerative conditions (Kato et al., 2016). Tau-PET imaging (Saint-Aubert et al., 2016) or CSF phosphorylated tau measurements (Blennow and Hampel, 2003) may provide information to address this issue. Third, we did not consider the influence of potential confounding factors, which may affect the in vivo AD pathologies, such as physical activity (Shah et al., 2014), social interaction (Bennett et al., 2006), diet (Berti et al., 2015), oxidative stress (Markesbery, 1997), and various physical conditions, including hypertension, diabetes, obesity, and other chronic illnesses (Chui et al., 2012), although we excluded individuals with serious medical or neurological disorders that may influence mental functioning.
Conclusion
Our results support that CA in early life is probably protective against late-life AD-related neurodegeneration, independently of cerebral Aβ pathology. In contrast, CA in midlife and late life appears to have no or limited association with AD-related brain changes, including amyloid pathology and neurodegeneration. With respect to prevention of dementia and cognitive impairment in late life, a cognitively active lifestyle in childhood and early adulthood needs to be more emphasized.
Ethics Statement
This study protocol was approved by the Institutional Review Boards of Seoul National University Hospital (C-1401-027-547) and SNU-SMG Boramae Center, Seoul, South Korea (26-2015-60), and was conducted in accordance with the recommendations of the current version of the Declaration of Helsinki. All subjects provided written informed consents.
Author Contributions
KK and DL designed the study, acquired and interpreted the data, and were major contributors to the writing of the manuscript and critically revising the manuscript for intellectual content. MB, DY, JL, and CK acquired and analyzed the data and helped to draft the manuscript. KK and DY analyzed the imaging data. DL served as the principal investigator and supervised the study. All authors read and approved the final manuscript.
Funding
This study was supported by a grant from Ministry of Science and ICT (Grant No. NRF-2014M3C7A1046042).
Conflict of Interest Statement
The authors declare that the research was conducted in the absence of any commercial or financial relationships that could be construed as a potential conflict of interest.
Supplementary Material
The Supplementary Material for this article can be found online at: https://www.frontiersin.org/articles/10.3389/fnagi.2018.00070/full#supplementary-material
References
Almeida, R. P., Schultz, S. A., Austin, B. P., Boots, E. A., Dowling, N. M., Gleason, C. E., et al. (2015). Effect of cognitive reserve on age-related changes in cerebrospinal fluid biomarkers of Alzheimer disease. JAMA Neurol. 72, 699–706. doi: 10.1001/jamaneurol.2015.0098
Andersen, S. L. (2003). Trajectories of brain development: point of vulnerability or window of opportunity? Neurosci. Biobehav. Rev. 27, 3–18. doi: 10.1016/s0149-7634(03)00005-8
Arfanakis, K., Wilson, R. S., Barth, C. M., Capuano, A. W., Vasireddi, A., Zhang, S., et al. (2016). Cognitive activity, cognitive function, and brain diffusion characteristics in old age. Brain Imaging Behav. 10, 455–463. doi: 10.1007/s11682-015-9405-5
Baker, L. M., Laidlaw, D. H., Cabeen, R., Akbudak, E., Conturo, T. E., Correia, S., et al. (2017). Cognitive reserve moderates the relationship between neuropsychological performance and white matter fiber bundle length in healthy older adults. Brain Imaging Behav. 11, 632–639. doi: 10.1007/s11682-016-9540-7
Barnes, L. L., Wilson, R. S., de Leon, C. F., and Bennett, D. A. (2006). The relation of lifetime cognitive activity and lifetime access to resources to late-life cognitive function in older African Americans. Aging Neuropsychol. Cogn. 13, 516–528. doi: 10.1080/138255890969519
Benes, F. M., Turtle, M., Khan, Y., and Farol, P. (1994). Myelination of a key relay zone in the hippocampal formation occurs in the human brain during childhood, adolescence, and adulthood. Arch. Gen. Psychiatry 51, 477–484.
Bennett, D. A., Schneider, J. A., Tang, Y., Arnold, S. E., and Wilson, R. S. (2006). The effect of social networks on the relation between Alzheimer’s disease pathology and level of cognitive function in old people: a longitudinal cohort study. Lancet Neurol. 5, 406–412.
Berti, V., Murray, J., Davies, M., Spector, N., Tsui, W. H., Li, Y., et al. (2015). Nutrient patterns and brain biomarkers of Alzheimer’s disease in cognitively normal individuals. J. Nutr. Health Aging 19, 413–423. doi: 10.1007/s12603-014-0534-0
Blennow, K., and Hampel, H. (2003). CSF markers for incipient Alzheimer’s disease. Lancet Neurol. 2, 605–613.
Bourgeois, J.-P., Jastreboff, P. J., and Rakic, P. (1989). Synaptogenesis in visual cortex of normal and preterm monkeys: evidence for intrinsic regulation of synaptic overproduction. Proc. Natl. Acad. Sci. U.S.A. 86, 4297–4301.
Carlson, M. C., Helms, M. J., Steffens, D. C., Burke, J. R., Potter, G. G., and Plassman, B. L. (2008). Midlife activity predicts risk of dementia in older male twin pairs. Alzheimers Dement. 4, 324–331. doi: 10.1016/j.jalz.2008.07.002
Choe, Y. M., Sohn, B. K., Choi, H. J., Byun, M. S., Seo, E. H., Han, J. Y., et al. (2014). Association of homocysteine with hippocampal volume independent of cerebral amyloid and vascular burden. Neurobiol. Aging 35, 1519–1525. doi: 10.1016/j.neurobiolaging.2014.01.013
Chugani, H. T., Phelps, M. E., and Mazziotta, J. C. (1987). Positron emission tomography study of human brain functional development. Ann. Neurol. 22, 487–497.
Chui, H. C., Zheng, L., Reed, B. R., Vinters, H. V., and Mack, W. J. (2012). Vascular risk factors and Alzheimer’s disease: are these risk factors for plaques and tangles or for concomitant vascular pathology that increases the likelihood of dementia? An evidence-based review. Alzheimers Res. Ther. 4:1.
Costa, D. A., Cracchiolo, J. R., Bachstetter, A. D., Hughes, T. F., Bales, K. R., Paul, S. M., et al. (2007). Enrichment improves cognition in AD mice by amyloid-related and unrelated mechanisms. Neurobiol. Aging 28, 831–844.
Dekhtyar, S., Wang, H.-X., Fratiglioni, L., and Herlitz, A. (2016). Childhood school performance, education and occupational complexity: a life-course study of dementia in the Kungsholmen Project. Int. J. Epidemiol. 45, 1207–1215.
Fox, S. E., Levitt, P., and Nelson, C. A. III (2010). How the timing and quality of early experiences influence the development of brain architecture. Child Dev. 81, 28–40. doi: 10.1111/j.1467-8624.2009.01380.x
Gidicsin, C. M., Maye, J. E., Locascio, J. J., Pepin, L. C., Philiossaint, M., Becker, J. A., et al. (2015). Cognitive activity relates to cognitive performance but not to Alzheimer disease biomarkers. Neurology 85, 48–55. doi: 10.1212/WNL.0000000000001704
Goodman, C. S., and Shatz, C. J. (1993). Developmental mechanisms that generate precise patterns of neuronal connectivity. Cell 72, 77–98.
Hata, Y., and Stryker, M. (1994). Control of thalamocortical afferent rearrangement by postsynaptic activity in developing visual cortex. Science 265, 1732–1735. doi: 10.1126/science.8085163
Hughes, T. F., Becker, J. T., Lee, C. W., Chang, C. C., and Ganguli, M. (2015). Independent and combined effects of cognitive and physical activity on incident MCI. Alzheimers Dement. 11, 1377–1384. doi: 10.1016/j.jalz.2014.11.007
Ihle, A., Bunce, D., and Kliegel, M. (2012). APOE ε4 and cognitive function in early life: a meta-analysis. Neuropsychology 26, 267–277. doi: 10.1037/a0026769
Jack, C. R., Wiste, H. J., Weigand, S. D., Rocca, W. A., Knopman, D. S., Mielke, M. M., et al. (2014). Age-specific population frequencies of cerebral β-amyloidosis and neurodegeneration among people with normal cognitive function aged 50–89 years: a cross-sectional study. Lancet Neurol. 13, 997–1005.
Jack, C. R. Jr., Bennett, D. A., Blennow, K., Carrillo, M. C., Feldman, H. H., Frisoni, G. B., et al. (2016). A/T/N: an unbiased descriptive classification scheme for Alzheimer disease biomarkers. Neurology 87, 539–547. doi: 10.1212/WNL.0000000000002923
Jack, C. R. Jr., Wiste, H. J., Lesnick, T. G., Weigand, S. D., Knopman, D. S., Vemuri, P., et al. (2013). Brain β-amyloid load approaches a plateau. Neurology 80, 890–896. doi: 10.1212/WNL.0b013e3182840bbe
Jack, C. R. Jr., Wiste, H. J., Weigand, S. D., Knopman, D. S., Mielke, M. M., Vemuri, P., et al. (2015). Different definitions of neurodegeneration produce similar amyloid/neurodegeneration biomarker group findings. Brain 138(Pt 12), 3747–3759. doi: 10.1093/brain/awv283
Jansen, W. J., Ossenkoppele, R., Knol, D. L., Tijms, B. M., Scheltens, P., Verhey, F. R., et al. (2015). Prevalence of cerebral amyloid pathology in persons without dementia: a meta-analysis. JAMA 313, 1924–1938. doi: 10.1001/jama.2015.4668
Jueptner, M., and Weiller, C. (1995). Does measurement of regional cerebral blood flow reflect synaptic activity?—Implications for PET and fMRI. Neuroimage 2, 148–156.
Kato, T., Inui, Y., Nakamura, A., and Ito, K. (2016). Brain fluorodeoxyglucose (FDG) PET in dementia. Ageing Res. Rev. 30, 73–84. doi: 10.1016/j.arr.2016.02.003
Kleim, J. A., Lussnig, E., Schwarz, E. R., Comery, T. A., and Greenough, W. T. (1996). Synaptogenesis and Fos expression in the motor cortex of the adult rat after motor skill learning. J. Neurosci. 16, 4529–4535.
Kolb, B., and Gibb, R. (2011). Brain plasticity and behaviour in the developing brain. J. Can. Acad. Child Adolesc. Psychiatry 20, 265–276.
Lampit, A., Hallock, H., Suo, C., Naismith, S. L., and Valenzuela, M. (2015). Cognitive training-induced short-term functional and long-term structural plastic change is related to gains in global cognition in healthy older adults: a pilot study. Front. Aging Neurosci. 7:14. doi: 10.3389/fnagi.2015.00014
Landau, S. M., Marks, S. M., Mormino, E. C., Rabinovici, G. D., Oh, H., O’Neil, J. P., et al. (2012). Association of lifetime cognitive engagement and low β-amyloid deposition. Arch. Neurol. 69, 623–629. doi: 10.1001/archneurol.2011.2748
Lee, D. Y., Lee, K. U., Lee, J. H., Kim, K. W., Jhoo, J. H., Kim, S. Y., et al. (2004). A normative study of the CERAD neuropsychological assessment battery in the Korean elderly. J. Int. Neuropsychol. Soc. 10, 72–81. doi: 10.1017/S1355617704101094
Lee, J. H., Lee, K. U., Lee, D. Y., Kim, K. W., Jhoo, J. H., Kim, J. H., et al. (2002). Development of the Korean version of the consortium to establish a registry for Alzheimer’s Disease assessment packet (CERAD-K): clinical and neuropsychological assessment batteries. J. Gerontol. B Psychol. Sci. Soc. Sci. 57, 47–53.
Leuner, B., Kozorovitskiy, Y., Gross, C. G., and Gould, E. (2007). Diminished adult neurogenesis in the marmoset brain precedes old age. Proc. Natl. Acad. Sci. U.S.A. 104, 17169–17173. doi: 10.1073/pnas.0708228104
Markesbery, W. R. (1997). Oxidative stress hypothesis in Alzheimer’s disease. Free Radic. Biol. Med. 23, 134–147.
Marquine, M. J., Segawa, E., Wilson, R. S., Bennett, D. A., and Barnes, L. L. (2012). Association between cognitive activity and cognitive function in older Hispanics. J. Int. Neuropsychol. Soc. 18, 1041–1051. doi: 10.1017/S135561771200080X
Paus, T., Zijdenbos, A., Worsley, K., Collins, D. L., Blumenthal, J., Giedd, J. N., et al. (1999). Structural maturation of neural pathways in children and adolescents: in vivo study. Science 283, 1908–1911.
Reiman, E. M., Chen, K., Liu, X., Bandy, D., Yu, M., Lee, W., et al. (2009). Fibrillar amyloid-β burden in cognitively normal people at 3 levels of genetic risk for Alzheimer’s disease. Proc. Natl. Acad. Sci. U.S.A. 106, 6820–6825. doi: 10.1073/pnas.0900345106
Richter-Schmidinger, T., Alexopoulos, P., Horn, M., Maus, S., Reichel, M., Rhein, C., et al. (2011). Influence of brain-derived neurotrophic-factor and apolipoprotein E genetic variants on hippocampal volume and memory performance in healthy young adults. J. Neural Transm. 118, 249–257. doi: 10.1007/s00702-010-0539-8
Ruiz, J. R., Castillo, R., Labayen, I., Moreno, L. A., Fuentes, M. G., Lamuno, D. G., et al. (2010). Individual and combined effects of ApoE and MTHFR 677C/T polymorphisms on cognitive performance in Spanish adolescents: the AVENA study. J. Pediatr. 156, 978–984, 984.e1. doi: 10.1016/j.jpeds.2009.12.018
Saint-Aubert, L., Almkvist, O., Chiotis, K., Almeida, R., Wall, A., and Nordberg, A. (2016). Regional tau deposition measured by [18F]THK5317 positron emission tomography is associated to cognition via glucose metabolism in Alzheimer’s disease. Alzheimers Res. Ther. 8:38. doi: 10.1186/s13195-016-0204-z
Sattler, C., Toro, P., Schonknecht, P., and Schroder, J. (2012). Cognitive activity, education and socioeconomic status as preventive factors for mild cognitive impairment and Alzheimer’s disease. Psychiatry Res. 196, 90–95. doi: 10.1016/j.psychres.2011.11.012
Shah, T., Verdile, G., Sohrabi, H., Campbell, A., Putland, E., Cheetham, C., et al. (2014). A combination of physical activity and computerized brain training improves verbal memory and increases cerebral glucose metabolism in the elderly. Transl. Psychiatry 4:e487. doi: 10.1038/tp.2014.122
Snowball, A., Tachtsidis, I., Popescu, T., Thompson, J., Delazer, M., Zamarian, L., et al. (2013). Long-term enhancement of brain function and cognition using cognitive training and brain stimulation. Curr. Biol. 23, 987–992. doi: 10.1016/j.cub.2013.04.045
Sokoloff, L. (1981). Relationships among local functional activity, energy metabolism, and blood flow in the central nervous system. Fed. Proc. 40, 2311–2316.
Stern, Y. (2012). Cognitive reserve in ageing and Alzheimer’s disease. Lancet Neurol. 11, 1006–1012. doi: 10.1016/s1474-4422(12)70191-6
Tau, G. Z., and Peterson, B. S. (2010). Normal development of brain circuits. Neuropsychopharmacology 35, 147–168. doi: 10.1038/npp.2009.115
Valenzuela, M. J., Sachdev, P., Wen, W., Chen, X., and Brodaty, H. (2008). Lifespan mental activity predicts diminished rate of hippocampal atrophy. PLoS One 3:e2598. doi: 10.1371/journal.pone.0002598
Vemuri, P., Knopman, D. S., Lesnick, T. G., Przybelski, S. A., Mielke, M. M., Graff-Radford, J., et al. (2017). Evaluation of amyloid protective factors and Alzheimer Disease neurodegeneration protective factors in elderly individuals. JAMA Neurol. 74, 718–726. doi: 10.1001/jamaneurol.2017.0244
Vemuri, P., Lesnick, T. G., Przybelski, S. A., Knopman, D. S., Machulda, M., Lowe, V. J., et al. (2016). Effect of intellectual enrichment on AD biomarker trajectories: longitudinal imaging study. Neurology 86, 1128–1135. doi: 10.1212/WNL.0000000000002490
Vemuri, P., Lesnick, T. G., Przybelski, S. A., Knopman, D. S., Roberts, R. O., Lowe, V. J., et al. (2012). Effect of lifestyle activities on Alzheimer disease biomarkers and cognition. Ann. Neurol. 72, 730–738. doi: 10.1002/ana.23665
Villemagne, V. L., Burnham, S., Bourgeat, P., Brown, B., Ellis, K. A., Salvado, O., et al. (2013). Amyloid beta deposition, neurodegeneration, and cognitive decline in sporadic Alzheimer’s disease: a prospective cohort study. Lancet Neurol. 12, 357–367. doi: 10.1016/S1474-4422(13)70044-9
Wenham, P. R., Newton, C. R., and Price, W. H. (1991). Analysis of apolipoprotein E genotypes by the amplification refractory mutation system. Clin. Chem. 37, 241–244.
Wilson, R., Bennett, D., Bienias, J., Aggarwal, N., De Leon, C. M., Morris, M., et al. (2002a). Cognitive activity and incident AD in a population-based sample of older persons. Neurology 59, 1910–1914.
Wilson, R. S., Barnes, L. L., Krueger, K. R., Hoganson, G., Bienias, J. L., and Bennett, D. A. (2005). Early and late life cognitive activity and cognitive systems in old age. J. Int. Neuropsychol. Soc. 11, 400–407.
Wilson, R. S., Bennett, D. A., Bienias, J. L., Mendes de Leon, C. F., Morris, M. C., and Evans, D. A. (2003). Cognitive activity and cognitive decline in a biracial community population. Neurology 61, 812–816.
Wilson, R. S., Boyle, P. A., Yang, J., James, B. D., and Bennett, D. A. (2015). Early life instruction in foreign language and music and incidence of mild cognitive impairment. Neuropsychology 29, 292–302. doi: 10.1037/neu0000129
Wilson, R. S., Boyle, P. A., Yu, L., Barnes, L. L., Schneider, J. A., and Bennett, D. A. (2013). Life-span cognitive activity, neuropathologic burden, and cognitive aging. Neurology 81, 314–321. doi: 10.1212/WNL.0b013e31829c5e8a
Wilson, R. S., De Leon, C. F. M., Barnes, L. L., Schneider, J. A., Bienias, J. L., Evans, D. A., et al. (2002b). Participation in cognitively stimulating activities and risk of incident Alzheimer disease. JAMA 287, 742–748.
Wilson, R. S., Scherr, P. A., Schneider, J. A., Tang, Y., and Bennett, D. A. (2007). Relation of cognitive activity to risk of developing Alzheimer disease. Neurology 69, 1911–1920. doi: 10.1212/01.wnl.0000271087.67782.cb
Wilson, R. S., Segawa, E., Boyle, P. A., and Bennett, D. A. (2012). Influence of late-life cognitive activity on cognitive health. Neurology 78, 1123–1129. doi: 10.1212/WNL.0b013e31824f8c03
Wirth, M., Villeneuve, S., La Joie, R., Marks, S. M., and Jagust, W. J. (2014). Gene-environment interactions: lifetime cognitive activity, APOE genotype, and β-amyloid burden. J. Neurosci. 34, 8612–8617. doi: 10.1523/JNEUROSCI.4612-13.2014
Wisdom, N. M., Callahan, J. L., and Hawkins, K. A. (2011). The effects of apolipoprotein E on non-impaired cognitive functioning: a meta-analysis. Neurobiol. Aging 32, 63–74. doi: 10.1016/j.neurobiolaging.2009.02.003
Wolf, S. A., Kronenberg, G., Lehmann, K., Blankenship, A., Overall, R., Staufenbiel, M., et al. (2006). Cognitive and physical activity differently modulate disease progression in the amyloid precursor protein (APP)-23 model of Alzheimer’s disease. Biol. Psychiatry 60, 1314–1323.
Keywords: cognitive activity, early life, midlife, late life, Alzheimer’s disease, neurodegeneration, amyloid beta deposition, the KBASE study
Citation: Ko K, Byun MS, Yi D, Lee JH, Kim CH and Lee DY (2018) Early-Life Cognitive Activity Is Related to Reduced Neurodegeneration in Alzheimer Signature Regions in Late Life. Front. Aging Neurosci. 10:70. doi: 10.3389/fnagi.2018.00070
Received: 16 October 2017; Accepted: 01 March 2018;
Published: 22 March 2018.
Edited by:
Ai-Ling Lin, University of Kentucky, United StatesReviewed by:
Jorge Valero, Achucarro Basque Center for Neuroscience, SpainFanny Elahi, University of California, San Francisco, United States
Copyright © 2018 Ko, Byun, Yi, Lee, Kim and Lee. This is an open-access article distributed under the terms of the Creative Commons Attribution License (CC BY). The use, distribution or reproduction in other forums is permitted, provided the original author(s) and the copyright owner are credited and that the original publication in this journal is cited, in accordance with accepted academic practice. No use, distribution or reproduction is permitted which does not comply with these terms.
*Correspondence: Dong Young Lee, c2VsZnBzeUBzbnUuYWMua3I=
†Information of the KBASE Research Group is provided in the online Supplemental Material.