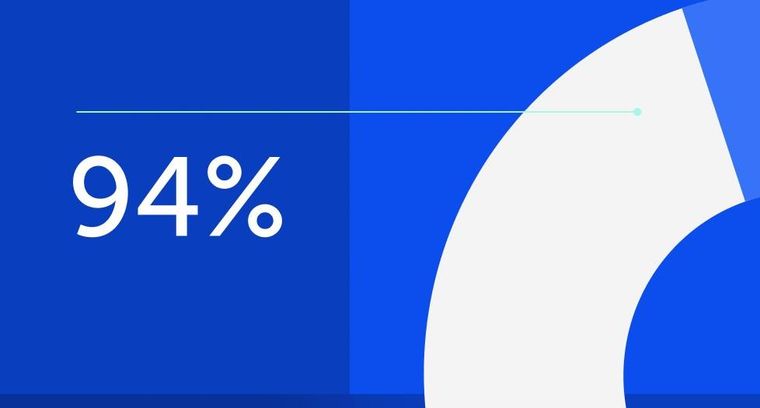
94% of researchers rate our articles as excellent or good
Learn more about the work of our research integrity team to safeguard the quality of each article we publish.
Find out more
ORIGINAL RESEARCH article
Front. Aging Neurosci., 27 October 2017
Sec. Neurocognitive Aging and Behavior
Volume 9 - 2017 | https://doi.org/10.3389/fnagi.2017.00332
This article is part of the Research TopicBrain Networks for Studying Healthy and Pathological Aging Mechanisms and Intervention EfficacyView all 48 articles
There is converging evidence that maintenance of function in the multiple connectivity networks involving the cerebellum is a key requirement for healthy aging. The present study evaluated the effectiveness of a home-based, internet-administered “cerebellar challenge” intervention designed to create progressive challenges to vestibular function, multi-tasking, and dynamic coordination. Participants (n = 98, mean age 68.2, SD 6.6) were randomly allocated to either intervention (the cerebellar challenge training for 10 weeks) or no intervention. All participants undertook an initial series of pre-tests, and then an identical set of post-tests following the intervention period. The test battery comprised five suites of tests designed to evaluate cognitive-sensori-motor-affective functions, including Physical Coordination, Memory, Language Dexterity, Fluid Thinking and Affect. The intervention group showed significant pre- to post improvements in 9 of the 18 tests, whereas the controls improved significantly on one only. Furthermore, the intervention group showed significantly greater improvement than the controls on the “Physical Coordination” suite of tests, with evidence also of differential improvement on the Delayed Picture Recall test. Frequency of intervention use correlated significantly with the improvement in balance and in peg-moving speed. It is concluded that an internet-based cerebellar challenge programme for older adults can lead to benefits in balance, coordination and declarative memory. Limitations and directions for further research are outlined.
The brain and body form a complex, self-regulating system capable of coping with a range of environmental and cognitive challenges, together with the pervasive, age-related progressive impairment in function of many system components. In this article we develop the perspective that the functional networks involving the cerebellum represent a significant part of the degradation in aging. We then briefly review the many interventions that have proved efficacious with older adults, noting the current consensus that multi-component systems designed to maintain a progressive challenge appear to have greater effect than single component systems. On theoretical grounds we argue that interventions designed around “cerebellar challenge”, combining coordinative exercise with cerebellar stimulation, should prove particularly effective. We finish by presenting an evaluation of an internet-based cerebellar challenge system, Zing, in terms of its effectiveness compared with a life-as-usual control group.
Traditional approaches to the causes of cognitive decline with aging considered primarily the frontal lobes (Jackson, 1958; Dempster, 1992; Greenwood, 2000). Over the past three decades there has been an explosion of research on all aspects of aging. Early this century extensive research was undertaken on changes in brain structure with aging (Raz et al., 2005), genetics (Deary et al., 2004; Erraji-Benchekroun et al., 2005), together with risk factors including increased white matter (Bartzokis, 2004; Head et al., 2004; Westlye et al., 2010; Sexton et al., 2014); excess homocysteine (Schafer et al., 2005) and reductions in dopamine and acetylcholamine neurotransmitters (Castner and Goldman-Rakic, 2004; Sarter and Bruno, 2004; Erixon-Lindroth et al., 2005).
Following these discoveries, arguably the greatest recent development has been the change of emphasis from these individual components and processes of the aging brain to consideration of the brain as a whole system. A major recent development in cognitive neuroscience has been the development of techniques for determining functional connectivity (Greicius et al., 2003; Fox et al., 2005; Buckner et al., 2008), and the consequent identification of a range of intrinsic networks (Yeo et al., 2011). The approach has great potential for characterizing the connectivity problems that affect brain function. A recent review (Bamidis et al., 2014) highlights the key role of connectivity changes in brain aging, and its implications for assessment and intervention.
It is notable that the cerebellum is also involved in seven of the major intrinsic networks (Buckner et al., 2011; Bernard et al., 2012; Kipping et al., 2013). It is therefore particularly interesting that circuits involving the cerebellum are strongly affected by age (Seidler et al., 2010; Balsters et al., 2013; Bernard et al., 2013; Humes et al., 2013; Bernard and Seidler, 2014; Koppelmans et al., 2015). Furthermore, it appears that the pattern of cerebellar degeneration with age in healthy adults is analogous to that shown by cerebellar patients (Hulst et al., 2015).
It is long established that there are major declines with age in sensory function (Humes et al., 2013; Wayne and Johnsrude, 2015; Roberts and Allen, 2016), in motor function (Seidler et al., 2010) and proprioceptive function (Goble et al., 2009). The cerebellum is centrally involved in sensorimotor processing (Chadderton et al., 2004, 2014; Ramakrishnan et al., 2016) and the involvement of the cerebellum in cognitive function is now fully established (Balsters et al., 2013; Mariën et al., 2014), as are direct, two-way links between the cerebellum and not only motor cortex but also prefrontal and posterior parietal cortex and the basal ganglia (Strick et al., 2009; Bostan et al., 2013).
Taken together, these results converge on the hypothesis (Bernard and Seidler, 2014) that the cerebellum—given its pervasive connectivity, its involvement in multiple sensory, cognitive and motor circuits; and its central role in adapting to internal changes—may be a critical component in the system degradation with age. This new conceptualization offers the promise that interventions designed to maintain or enhance cerebellar function may alleviate the affects of aging on sensori-motor-cognitive performance.
There are many successful interventions for alleviating age-related decline. A recent review (Ballesteros et al., 2015) focused on three modes of intervention: physical activity, computerized cognitive training and social enhancement and concluded that although single domain interventions were effective the simultaneous training of both cognitive and physical domains offers a greater potential on daily life functioning. One of the key problems identified by the authors was the issue of how to combine different interventions and how to evaluate their effectiveness. The systems approach to healthy aging provides a theoretical perspective on this issue, suggesting that if a major cause if impairment is functional loss in the intrinsic connectivity networks, the optimal intervention should target function in the network as a whole, rather than individual components thereof.
Computerized cognitive training (CCT) approaches, using computer programs to boost core cognitive capabilities such as working memory, speed of processing and visual attention have proved highly effective in some studies, but less so in others. Systematic reviews of brain training programmes with older adults (Gross et al., 2012; Kueider et al., 2012) concluded that computerized training is an effective, less labor intensive alternative to cognitive training. In contrast, a recent analysis (Lampit et al., 2014) concluded that the overall effect size of CCT vs. control was small and statistically significant for nonverbal memory, verbal memory, working memory, processing speed, and visuospatial skills but not for executive functions and attention. A meta-analysis for younger groups (Melby-Lervåg and Hulme, 2013) concluded that WM programs produced reliable short-term improvements in WM skills but that the effects were “short-term, specific training effects that do not generalize”.
One of the clear limitations, from a systems view, both of CCT and of direct brain stimulation, is that the intervention is artificial, and isolated from the physical or mental activities involved in normal system functionality. There is strong evidence that natural activities, such as exercise, can improve not only physical fitness but also mental fitness, and even stimulate the growth of new brain neurons and connections (Hillman et al., 2008; Höetting and Röeder, 2013; Kirk-Sanchez and McGough, 2014). An innovative approach, the Long-Lasting Memories intervention, which combines both exercise and CCT approaches (“exergaming”) was shown to have beneficial effects for healthy older adults and for those with Mild Cognitive Impairment (MCI; González-Palau et al., 2014).
A recent discovery has been the differential effects of cardiovascular, high intensity, exercise and “co-ordinative exercise” such as balance training or tai-chi. There is strong evidence that exercise can potentiate the brain for new learning, with coordinative balance exercises leading to neural growth in the hippocampus—a core structure for explicit learning and memory (Niemann et al., 2014)—and also in the cerebellar-cortical loop (Burciu et al., 2013)—a core network for implicit learning and coordination. A further study (Nascimento et al., 2014) concluded that multimodal physical exercise was effective in reducing pro-inflammatory cytokines and in improving brain-derived neurotrophic factor (BDNF) peripheral levels, with positive reflexes on cognition in elderly individuals with MCI.
Recent studies of the effects of exercise on rat brains (Kellermann et al., 2012; Abel and Rissman, 2013) reveal strong effects on epigenetic changes and changes in the cerebellar Purkinje cells following a rat vestibular training exercise (Lee et al., 2015). There is also evidence that BDNF is expressed in the cerebellum following environmental enrichment for rats (Angelucci et al., 2009; Vazquez-Sanroman et al., 2013). Of particular interest, there is evidence (though sparse) that Quadrato exercise (like Tai Chi) led to increased creativity and changes in gray matter and white matter in the cerebellum (Ben-Soussan et al., 2015).
There have also been detailed neuroimaging studies of interventions for special groups. Daily clinic-based balance training for 2 weeks in cerebellar patients and age-matched healthy controls (Burciu et al., 2013) led to enhanced balance performance in the patients, with associated increased gray matter volume in the dorsal premotor cortex and within the cerebellum for both groups. A 6 week balance-training study with Parkinson’s patients and healthy controls (Sehm et al., 2014) led to improved balance which was maintained for the following year, together with increased gray matter in the hippocampus for the controls and in several brain regions for the patients.
Of particular interest regarding functional connectivity, two recent studies with older adults with MCI have established functional connectivity changes following 8 week interventions. Klados et al. (2016) established that the Long Lasting Memories intervention led to increased beta-band EEG activity (reflecting increased bilateral connections in the occipital, parietal, temporal and prefrontal regions) after the intervention. Chirles et al. (2017) undertook a “walking exercise” intervention, and established that following the intervention the MCI group showed increased connectivity in 10 regions spanning frontal, parietal, temporal and insular lobes, together with the cerebellum.
In summary, current neuroimaging and behavioral research appears to be converging to a view that: (i) a systems approach to aging is the most promising framework for understanding the degradation in multiple functions with age; (ii) there is extensive evidence that the cerebellum is one of the key structures affected, and the multiple intrinsic connectivity networks linking the cerebellum with other brain and body structures may well mediate many of the actual deficits shown; (iii) “single system” interventions can be effective, but generally it is better to have multiple domain interventions; (iv) a range of interventions, from coordinative exercise to direct vestibular stimulation are likely to have beneficial effects on cerebellar function.
The above considerations informed the design of the current study. We wished to evaluate the effectiveness of a novel internet-based “vestibular stimulation” intervention, the Zing intervention1. This intervention was originally developed to tune up the coordination abilities of top sporting performers, using a series of graded exercises designed specifically to improve three performance dimensions: sensorimotor coordination, eye movement control and dual tasking. However, extensive feedback had suggested that the programme was valuable for many average performers. Consequently the system was embedded in an internet-based “game” format designed to challenge and stimulate the user to keep improving their performance. Zing Performance offer a number of courses specifically tailored to each individual user, with applications in sporting areas, organizational development, in education.
The Zing system involves a series of graded activities on three dimensions—dynamic activity (patterned movement sequences), focus activity (developing the ability both to concentrate and to “dual task”) and stability activity (coordinative balance). Underpinning the approach is the technique of vestibular stimulation. Rather than cardiovascular exercise, which is designed to have energetic use of highly practised routines, or even coordinative balance such as tai-chi, which does involve learning new actions, vestibular activities are designed to cause abnormal input for the vestibular system, for example by requiring the user to put their head on one side while undertaking tasks. This presents the vestibular system, and the cerebellum, with an immediate challenge, requiring activation of many circuits to cope with the ensuing proprioceptive feedback.
A typical course lasts 6 months and is composed of daily physical activities and digital video games. An example of a low level focus activity (at the time of the study) is given in Figure 1. A video is also available for each activity.
Figure 1. Sample screen from the Zing intervention. This is an example of a screen from the focus strand, at a low level and aimed at developing dual tasking ability.
The Zing platform therefore provides a user-orientated, motivating framework for delivering a cost-effective cerebellar challenge intervention that satisfies the criteria that have emerged for multimodal, challenging interventions in older adults.
We undertook the study to investigate whether internet-based approaches can indeed be an effective and popular method for older adults, and designed an 8 week intervention. It is important to highlight that although this is a Randomized Control Trial (RCT) study, in that there was a control condition and allocation to condition was random, it is not a full RCT, for which an active intervention condition, matched in time and form to the Zing intervention, would need to be used to counter placebo-type effects. Our view is that this non-equivalent–control RCT (NEC-RCT) design is appropriate for a user-centered trial that has the underlying question “If participants undertake the intervention, will it help them, and, if so, in what ways?” We are not investigating the theoretical issue—is intervention A more effective than intervention B, and if so, why? Each design has its strengths and weaknesses. For the purpose of evaluating whether a low-cost, home-based intervention might be beneficial compared with life-as-usual, the NEC-RCT design is the appropriate one.
A limitation of many previous intervention studies is that the set of tests used from pre-intervention to post-intervention focus on a limited range of performance measures. As noted above, there is reason to expect that a cerebellar challenge intervention might lead to changes in both the sensorimotor domain and in the cognitive domain. There is also longstanding evidence that the cerebellum is involved in emotional processing (Schmahmann and Sherman, 1997), with emerging evidence regarding its involvement in processing emotional salience (Styliadis et al., 2015; Adamaszek et al., 2017). There is also evidence that the resting state networks involving the cerebellum are associated with differences in crystallized intelligence (Pezoulas et al., 2017). Consequently we designed a battery of simple tasks designed to probe sensorimotor, performance, cognitive performance, emotional state and nonverbal reasoning.
The design allows the following hypotheses to be evaluated.
Hypothesis 1. Improvements in balance and sensorimotor coordination. This is the primary applied hypothesis, directly related to attempting to boost balance performance and thus decrease the danger of falling. One in three people of 65 fall at least once per year, with the incidence rising to one half of those over 80 years old (Todd and Skelton, 2004). Falls are a major cost to elderly people and to national health services, estimated to account for 21% of the Dutch health service costs for injuries (Hartholt et al., 2011). Hypothesis 1 states that Zing training will lead to significant improvements in sensori-motor coordination especially balance: (a) for each individual compared with their pre-training; (b) that the intervention group will improve significantly more than a control, no intervention, group.
Hypothesis 2. “Hippocampal” improvements. This hypothesis is derived from the research showing the benefits of coordinative balance training for hippocampal function. Hypothesis 2 states that Zing training will lead to significant improvements in “declarative memory” performance: (a) for each individual compared with their pre-training; (b) that the intervention group will improve significantly more than a control, no intervention, group.
Hypothesis 3. Improvement Specificity. Despite the emerging evidence of cerebellar involvement in affective processing, we would expect any such changes to be of secondary importance in terms of affective state. Consequently, although Zing training may lead to significant “transfer” to other areas, including language, affect and fluid reasoning, any such effects will be minor compared with the specific improvements in hippocampal and sensorimotor skills.
Ninety-eight volunteers (30 male, 68 female) aged 50–85 (mean 68.2, SD 6.6) were recruited through advertisements in local newspapers, churches and social groups. An advert also went out on the University of the Third Age Sheffield website. Participants were all without a known diagnosis of dementia. The ethics committee of the Department of Psychology, University of Sheffield, approved the study. Participants gave fully informed prior consent. They were also informed that their information would be anonymised and kept securely. They were also informed that they could withdraw from the study at any time without needing to give any reason. All participants were healthy older adults.
The aim of this study was to test the effectiveness of vestibular stimulation on physical and mental function. Therefore, a repeated measures design was used. Participants were asked to complete a baseline set of tests at the University of Sheffield Department of Psychology before taking part in the 8 week Zing intervention at home. They were then asked to return to the department for a repeat of the baseline tests.
The same tests were used both pre and post-test. While there may be some practice effects here, it would be expected that this would affect both groups equally, and therefore any relative difference in the intervention group’s performance is likely to be attributable to the exercises.
We wished to evaluate changes in all the core physical, mental and affective domains, using simple but normed tests where possible. We based the battery on the Dyslexia Adult Screening Test (Fawcett and Nicolson, 1998), which covers the majority of the necessary tests in a 30 min package. We constructed a battery of 14 tests, divided into five suites. Suite 1 was for Physical Coordination and comprised the DAST balance test, the two Purdue pegboard (Tiffin and Asher, 1948) tests (Peg Moving and Peg Assembly), and the DAST writing (copying) test. Suite 2 investigated memory. It included two tests of working memory, the DAST backwards digit span test and the South Yorkshire Ageing Study (Tarmey, 2012) Spatial Memory test which determines spatial memory span for non-verbalizable pictures presented in one of eight locations. There was one declarative memory test, the South Yorkshire Ageing Study (Tarmey, 2012) Picture Memory test which assesses recall for a set of 20 pictures of common objects, presented sequentially for 1 s, including both immediate recall and delayed recall after 20 min. The Language Suite comprised the DAST Rapid Naming, Phonological Processing, Reading, Nonsense Passage and Spelling tests. The Fluid Reasoning suite comprised the DAST Nonverbal Reasoning, Semantic Fluency and Verbal Fluency tests. Finally two tests of affect were administered: the Beck Depression Inventory (BDI; Beck et al., 1996) and the Authentic Happiness Inventory (Seligman, 2002).
Participants were required to undertake a minimum of 8 weeks and maximum of 10 weeks balance and sensorimotor coordination training using an online set of activities. These were provided by Zing Performance Ltd., and were designed specifically to stimulate brain regions involved in coordinative balance. Initially, participants had to undergo an assessment to determine their strengths and weaknesses. After this a 30 day programme was set for them, specifically designed to target their biggest needs. Participants were required to do two exercises a day, before rating how difficult they found that particular activity. A screen shot is shown in Figure 1 below. Each week, three exercises were assigned, with two of the three appearing each day. After 30 days, participants were reassessed before continuing onto unit two. It should be noted that a full Zing 360 session programme is designed for 6 months, with two sessions per day. Consequently this study is very much shorter than intended by the Zing designers.
Data were converted to standard scores (mean 100, SD 15) to allow direct comparison across tasks. Where possible, population norms and standard deviations were used to normalize test scores. The population norm for age 55+ was used for all participants, irrespective of age, to represent absolute performance rather than age-adjusted performance.
In order to facilitate comparison of the improvements (or otherwise) in performance from pre-test to post-test, effect sizes were calculated using the formula ES = (post-test − pre-test) / SD (all groups on pre-test), which is a form of Cohen, 1988 applied to change analysis. No change would result in an effect size of 0, whereas a score of +1.0 indicates a change of one standard deviation unit. Cohen (1988) suggests that effect sizes of 0.8, 0.5 and 0.2 be labeled large, medium and small, respectively.
Effect sizes for the two groups are shown in Figure 2. Tests have been grouped in order of the hypotheses. The Physical Coordination suite—postural stability, peg moving, peg assembly and handwriting speed are on the left, then the Memory Suite—immediate picture recall, delayed picture recall, immediate spatial memory and immediate verbal memory. Group 3 include the affect measures—the BDI (reverse scored such that higher means less depressed) and the Authentic Happiness Index. The remaining tests are the Language Suite and the Fluid Thinking Suite but were not predicted to be affected by the intervention.
Next correlational analyses were undertaken utilizing data collected automatically on “compliance” for the Zing group. Of the 53 participants allocated to the Zing group, 38 completed at least 40 sessions, as requested, but the differential uptake allowed us to investigate the effects both of frequency of Zing use (sessions per week) and the duration (number of weeks). For the frequency of Zing use significant correlations were found for peg movement (r = 0.31, p < 0.05), and for postural stability (r = 0.303, p < 0.05). A significant correlation with number of weeks of the intervention occurred only for nonverbal reasoning (r = 0.288, p < 0.05).
Inferential statistical tests were then undertaken for the 16 tests within the five suites of tests. First repeated measures multivariate analyses of variance were undertaken for each suite separately on the data for pre-test and post-test for each test within the suite.
For the control group, none of the set of MANOVAs approached significance. In fact the only individual comparison to reach the uncorrected 0.05 significance level was for peg moving (F(1,43) = 6.26, p = 0.016).
For the Zing group, the MANOVA analyses of the change from pre-test to post-test were highly significant for the suites for Physical Coordination, for Declarative Memory, for Language, and for Fluid Thinking (F(1,47) = 22.95, p < 0.001; F(1,52) = 10.71, p = 0.002; F(1,52) = 15.99, p < 0.001; F(1,52) = 5.72, p = 0.020 respectively), whereas there was no difference for the Affect suite. It is not sensible to undertake a Bonferroni correction for multiple comparison when all comparisons are significant in the same direction (Moran, 2003), and consequently uncorrected probabilities are reported. The changes for Balance, Peg Assembly and Peg Movement were significant (F(1,50) = 14.07, p < 0.001; F(1,51) = 5.53, p = 0.023; F(1,50) = 4.10, p = 0.048 respectively). The improvements for Delayed Picture Recall, Immediate Picture Recall and Memory Span were also significant (F(1,52) = 14.44, p < 0.001; F(1,52) = 15.41, p < 0.001; F(1,52) = 4.20, p = 0.046 respectively). Two of the improvements for nonsense passage reading, 1 min reading, rapid naming and spelling were significant [F(1,52) = 3.72, p = 0.059; F(1,52) = 6.28, p = 0.015; F(1,52) = 4.73, p = 0.034; F(1,52) = 3.28, p = 0.076 respectively). The improvement for verbal fluency was also significant (F(1,52) = 5.13, p = 0.028).
Finally, in the most stringent test of the changes, a series of multivariate 2-factor analyses of variance was undertaken, with the independent groups factor being the group (Zing vs. Control) and the repeated measure being time-of-test (pre-test vs. post-test. Manovas were undertaken separately for each of the five suites (see Table 1). For the MANOVA entry, only the key statistic, the interaction term between time of test (pre vs. post) and Group is reported. A significant interaction would typically indicate that the Intervention led to a significant difference between groups at post-test whereas performance at pre-test was equivalent.
It may be seen that the only suite returning a significant MANOVA result was the Physical Coordination suite. For each of the four tests a univariate two factor mixed measures analysis of variance was undertaken, with the within-group variable being time-of-test (pre-intervention vs. post-intervention) and the between-group variable being group (intervention vs. control). Significant (uncorrected) interactions—all reflecting greater improvement for the intervention group than the control group—were obtained for postural stability and for peg assembly. By contrast, there were no differences for peg moving speed or writing speed.
The MANOVA results for the other four suites of tests were not close to significance. Uncorrected significant differences were obtained for Delayed Picture Memory and for Nonsense Passage Reading.
Finally, correlations with age were calculated. Significant correlations were found for performance on the majority of tests, with correlations between age and each dependent variable in descending order being −0.47 (Nonverbal reasoning), −0.40 (peg assembly), −0.38 (immediate picture memory), −0.35 (immediate picture memory), −0.34 (writing), −0.28 (spatial memory), −0.27 (semantic fluency), −0.26 (postural stability) and −0.26 (spelling). Correlations between age and the amount of improvement for the Zing group were also calculated. Few correlations were significant, with only peg assembly (−0.37) being more extreme than −0.25.
The primary issue addressed by this study was whether a home-based cerebellar challenge internet-administered intervention was feasible for use with older adults and, if used, whether it would result in better balance, and hence reduce danger of falling (Hypothesis 1). A secondary, theoretical issue, was whether the intervention might also improve cognitive functions previously found to be improved by coordinative balance training (Hypothesis 2).
A set of five “suites” of tests was applied before and after the intervention, allowing comparison with a “life as usual” control group. Comparing individual performances across the intervention period, the control group performance remained roughly constant, with no significant change for 17 of the 18 tests. By contrast, the intervention participants showed significant improvement in their scores for 9 of the 18 tests administered, with only the tests of the Affect suite showing no significant multivariate improvement.
Furthermore, a series of two factor multivariate analyses of variance revealed that the intervention group improved significantly more than the control group on the Physical Coordination suite, but not on the memory suite, the affect suite, the language suite or the fluency suite.
Hypothesis 1 is therefore clearly supported. Not surprisingly—but crucial for applied purposes—the intervention group did improve significantly on balance compared both with their own pre-intervention performance and with the control group’s change in balance over the period of the study. There was also transfer of this training effect to manual dexterity (as indicated by the “peg assembly” task).
Clear support is also provided for Hypothesis 2, though the effect is masked by the inclusion of tests of working memory and declarative memory within the memory suite. It is clear from the effect sizes and the between-groups anova data that there was a significant benefit for the Zing group for the tests of declarative memory (especially the key task of delayed picture recall, which is more akin to a realistic memory use task) but not for the tests of working memory. It is therefore legitimate to infer that, consistent with the literature on the benefits of balance training on hippocampal function, there was transfer of this benefit to declarative memory in the delayed picture recall condition (Hypothesis 2).
The specificity of the differential benefit findings to the hypotheses suggests strongly that the changes are not a practice, placebo or Hawthorne effect (Hypothesis 3). Given the null effect on affect, we examined the individual scores on the BDI in order to investigate whether participants more at risk of depression showed differential effects. Of the four participants in the intervention group classifiable as at least mildly depressed, one showed marked improvement. However, the 2 participants in the non-intervention group initially showing mild depression also showed marked improvement. We conclude that, at least in this group of heathy older adults, the results do not suggest that there is a direct effect of the cerebellar challenge intervention on affective state.
Of the 52 participants selected for the intervention condition, 38 (73%) completed the requested 40 sessions over 8 weeks. Analyses of the “dose effect” (that is, correlations of performance improvement with number of intervention sessions for the intervention group) revealed significant correlations with intervention frequency for peg movement, reading and balance, with a significant correlation with intervention duration for nonverbal reasoning.
In terms of the participants’ response to the intervention, it should be noted that the Zing platform was a prototype version, not yet publicly available and in the process of substantial development and improvement. A sizeable minority of the Zing participants reported difficulties in accessing the system initially, though subsequently problems were relatively small.
It is important to acknowledge the limitations of this study. First, the population sampled was by no means random, involving respondents to a circular. They should therefore be seen as relatively high functioning and with good self efficacy. Furthermore, they represented a spread of ages, with 2 under 55, 27 aged 55–64, 55 aged 65–74 and 15 aged 75 and over. There was a considerable imbalance between the sexes, with 30 male and 68 female. All were living at home in reasonable health. All of these factors reduce the strength of inferences that can be made regarding generalization to the full population of community based older adults, and highlight the need for further research.
Prior research has established that home-based balance exercises are among the most cost-effective methods of improving balance ability and hence reducing falls in older adults. Recent developments in cognitive neuroscience have revealed that “coordinative” balance training is likely to have beneficial effects not only on physical coordination but also on hippocampal function. Our theoretical analyses suggested that a multi-component, cerebellar challenge intervention should prove highly effective, combining the effectiveness of coordinative exercise with that of direct cerebellar stimulation, and therefore improving function in the intrinsic connectivity networks involving the cerebellum. The study design did not include brain imaging, and therefore it is not possible to assess directly any underlying neural changes. Furthermore the study design does not permit comparison of the Zing approach with a suitable active control. Nonetheless, the results were encouraging.
The present study is unique in two ways: first we investigated a highly cost-effective internet-based “cerebellar challenge” intervention, “Zing”. Second we investigated physical coordination, mental coordination, language, fluid thinking and affect using a specially developed battery of tests. Significant benefits (comparing initial performance with post-intervention performance) were found for the intervention group on the majority of the tests, excluding only those for affect. Furthermore, significantly greater improvements were found for the intervention group (compared with the control group) for balance, for physical coordination and for declarative memory retrieval.
Further research, including research using an active control intervention, would be needed to pinpoint the theoretical causes of the improvements obtained. Nonetheless, given the minimal cost and considerable ease of access of the intervention, it provides a promising approach to improving the overall cerebellar-related function, protecting against subsequent balance problems, and may also benefit declarative memory in older adults.
Both authors contributed to all aspects of the empirical work, the data analysis and the article writing. ZG had a stronger focus on the empirical work, and RIN had a stronger focus on design and theoretical interpretation.
The research was undertaken as part of ZG’s doctoral research at the University of Sheffield. This was supported by a fees-only award from the University of Sheffield. The research was undertaken in 2014/15. In 2016 Nicolson was appointed to the scientific advisory board of Zing Performance Outreach, a non-profit charitable organization.
Zing Performance Inc. provided free registration on the Zing Programme for the intervention participants and £30 in acknowledgment for those completing the intervention. Neither author received financial support from Zing Performance Ltd.
We gratefully acknowledge the support of Samantha Critchley, Mark Manser and Gareth Dore of Zing Performance in helping to resolve any difficulties encountered by the participants. We acknowledge with thanks the contributions made in the pre-test and post-test assessments by Caroline Carta, Penny Jackson, Phil Roughsedge and Laura Scott.
Abel, J. L., and Rissman, E. F. (2013). Running-induced epigenetic and gene expression changes in the adolescent brain. Int. J. Dev. Neurosci. 31, 382–390. doi: 10.1016/j.ijdevneu.2012.11.002
Adamaszek, M., D’Agata, F., Ferrucci, R., Habas, C., Keulen, S., Kirkby, K. C., et al. (2017). Consensus paper: cerebellum and emotion. Cerebellum 16, 552–576. doi: 10.1007/s12311-016-0815-8
Angelucci, F., De Bartolo, P., Gelfo, F., Foti, F., Cutuli, D., Bossù, P., et al. (2009). Increased concentrations of nerve growth factor and brain-derived neurotrophic factor in the rat cerebellum after exposure to environmental enrichment. Cerebellum 8, 499–506. doi: 10.1007/s12311-009-0129-1
Ballesteros, S., Kraft, E., Santana, S., and Tziraki, C. (2015). Maintaining older brain functionality: a targeted review. Neurosci. Biobehav. Rev. 55, 453–477. doi: 10.1016/j.neubiorev.2015.06.008
Balsters, J. H., Whelan, C. D., Robertson, I. H., and Ramnani, N. (2013). Cerebellum and cognition: evidence for the encoding of higher order rules. Cereb. Cortex 23, 1433–1443. doi: 10.1093/cercor/bhs127
Bamidis, P. D., Vivas, A. B., Styliadis, C., Frantzidis, C., Klados, M., Schlee, W., et al. (2014). A review of physical and cognitive interventions in aging. Neurosci. Biobehav. Rev. 44, 206–220. doi: 10.1016/j.neubiorev.2014.03.019
Bartzokis, G. (2004). Age-related myelin breakdown: a developmental model of cognitive decline and Alzheimer’s disease. Neurobiol. Aging 25, 5–18. doi: 10.1016/j.neurobiolaging.2003.03.001
Beck, A., Steer, R., and Brown, G. (1996). Beck Depression Inventory. San Antonio, TX: The Psychological Corporation.
Ben-Soussan, T. D., Berkovich-Ohana, A., Piervincenzi, C., Glicksohn, J., and Carducci, F. (2015). Embodied cognitive flexibility and neuroplasticity following Quadrato Motor Training. Front. Psychol. 6:1021. doi: 10.3389/fpsyg.2015.01021
Bernard, J. A., Peltier, S. J., Wiggins, J. L., Jaeggi, S. M., Buschkuehl, M., Fling, B. W., et al. (2013). Disrupted cortico-cerebellar connectivity in older adults. Neuroimage 83, 103–119. doi: 10.1016/j.neuroimage.2013.06.042
Bernard, J. A., and Seidler, R. D. (2014). Moving forward: age effects on the cerebellum underlie cognitive and motor declines. Neurosci. Biobehav. Rev. 42, 193–207. doi: 10.1016/j.neubiorev.2014.02.011
Bernard, J. A., Seidler, R. D., Hassevoort, K. M., Benson, B. L., Welsh, R. C., Wiggins, J. L., et al. (2012). Resting state cortico-cerebellar functional connectivity networks: a comparison of anatomical and self-organizing map approaches. Front. Neuroanat. 6:31. doi: 10.3389/fnana.2012.00031
Bostan, A. C., Dum, R. P., and Strick, P. L. (2013). Cerebellar networks with the cerebral cortex and basal ganglia. Trends Cogn. Sci. 17, 241–254. doi: 10.1016/j.tics.2013.03.003
Buckner, R. L., Andrews-Hanna, J. R., and Schacter, D. L. (2008). The brain’s default network—Anatomy, function, and relevance to disease. Ann. N Y Acad. Sci. 1124, 1–38. doi: 10.1196/annals.1440.011
Buckner, R. L., Krienen, F. M., Castellanos, A., Diaz, J. C., and Yeo, B. T. T. (2011). The organization of the human cerebellum estimated by intrinsic functional connectivity. J. Neurophysiol. 106, 2322–2345. doi: 10.1152/jn.00339.2011
Burciu, R. G., Fritsche, N., Granert, O., Schmitz, L., Spöenemann, N., Konczak, J., et al. (2013). Brain changes associated with postural training in patients with cerebellar degeneration: a voxel-based morphometry study. J. Neurosci. 33, 4594–4604. doi: 10.1523/jneurosci.3381-12.2013
Castner, S. A., and Goldman-Rakic, P. S. (2004). Enhancement of working memory in aged monkeys by a sensitizing regimen of dopamine D1 receptor stimulation. J. Neurosci. 24, 1446–1450. doi: 10.1523/jneurosci.3987-03.2004
Chadderton, P., Margrie, T. W., and Häusser, M. (2004). Integration of quanta in cerebellar granule cells during sensory processing. Nature 428, 856–860. doi: 10.1038/nature02442
Chadderton, P., Schaefer, A. T., Williams, S. R., and Margrie, T. W. (2014). Sensory-evoked synaptic integration in cerebellar and cerebral cortical neurons. Nat. Rev. Neurosci. 15, 71–83. doi: 10.1038/nrn3648
Chirles, T. J., Reiter, K., Weiss, L. R., Alfini, A. J., Nielson, K. A., and Smith, J. C. (2017). Exercise training and functional connectivity changes in mild cognitive impairment and healthy elders. J. Alzheimers Dis. 57, 845–856. doi: 10.3233/jad-161151
Cohen, J. (1988). Statistical Power Analysis for the Behavioral Sciences. 2nd Edn. New York, NY: Academic Press.
Deary, I. J., Wright, A. F., Harris, S. E., Whalley, L. J., and Starr, J. M. (2004). Searching for genetic influences on normal cognitive ageing. Trends Cogn. Sci. 8, 178–184. doi: 10.1016/j.tics.2004.02.008
Dempster, F. N. (1992). The rise and fall of the inhibitory mechanism: toward a unified theory of cognitive development and aging. Dev. Rev. 12, 45–75. doi: 10.1016/0273-2297(92)90003-k
Erixon-Lindroth, N., Farde, L., Wahlin, T. B. R., Sovago, J., Halldin, C., and Bäckman, L. (2005). The role of the striatal dopamine transporter in cognitive aging. Psychiatry Res. 138, 1–12. doi: 10.1016/j.pscychresns.2004.09.005
Erraji-Benchekroun, L., Underwood, M. D., Arango, V., Galfalvy, H., Pavlidis, P., Smyrniotopoulos, P., et al. (2005). Molecular aging in human prefrontal cortex is selective and continuous throughout adult life. Biol. Psychiatry 57, 549–558. doi: 10.1016/j.biopsych.2004.10.034
Fawcett, A. J., and Nicolson, R. I. (1998). The Dyslexia Adult Screening Test. London: The Psychological Corporation.
Fox, M. D., Snyder, A. Z., Vincent, J. L., Corbetta, M., Van Essen, D. C., and Raichle, M. E. (2005). The human brain is intrinsically organized into dynamic, anticorrelated functional networks. Proc. Natl. Acad. Sci. U S A 102, 9673–9678. doi: 10.1073/pnas.0504136102
Goble, D. J., Coxon, J. P., Wenderoth, N., Van Impe, A., and Swinnen, S. P. (2009). Proprioceptive sensibility in the elderly: degeneration, functional consequences and plastic-adaptive processes. Neurosci. Biobehav. Rev. 33, 271–278. doi: 10.1016/j.neubiorev.2008.08.012
González-Palau, F., Franco, M., Bamidis, P., Losada, R., Parra, E., Papageorgiou, S. G., et al. (2014). The effects of a computer-based cognitive and physical training program in a healthy and mildly cognitive impaired aging sample. Aging Ment. Health 18, 838–846. doi: 10.1080/13607863.2014.899972
Greenwood, P. M. (2000). The frontal aging hypothesis evaluated. J. Int. Neuropsycholog. Soc. 6, 705–726. doi: 10.1017/s1355617700666092
Greicius, M. D., Krasnow, B., Reiss, A. L., and Menon, V. (2003). Functional connectivity in the resting brain: a network analysis of the default mode hypothesis. Proc. Natl. Acad. Sci. U S A 100, 253–258. doi: 10.1073/pnas.0135058100
Gross, A. L., Parisi, J. M., Spira, A. P., Kueider, A. M., Ko, J. Y., Saczynski, J. S., et al. (2012). Memory training interventions for older adults: a meta-analysis. Aging Ment. Health 16, 722–734. doi: 10.1080/13607863.2012.667783
Hartholt, K. A., van Beeck, E. F., Polinder, S., van der Velde, N., van Lieshout, E. M. M., Panneman, M. J. M., et al. (2011). Societal consequences of falls in the older population: injuries, healthcare costs, and long-term reduced quality of life. J. Trauma 71, 748–753. doi: 10.1097/ta.0b013e3181f6f5e5
Head, D., Buckner, R. L., Shimony, J. S., Williams, L. E., Akbudak, E., Conturo, T. E., et al. (2004). Differential vulnerability of anterior white matter in nondemented aging with minimal acceleration in dementia of the Alzheimer type: evidence from diffusion tensor imaging. Cereb. Cortex 14, 410–423. doi: 10.1093/cercor/bhh003
Hillman, C. H., Erickson, K. I., and Kramer, A. F. (2008). Be smart, exercise your heart: exercise effects on brain and cognition. Nat. Rev. Neurosci. 9, 58–65. doi: 10.1038/nrn2298
Höetting, K., and Röeder, B. (2013). Beneficial effects of physical exercise on neuroplasticity and cognition. Neurosci. Biobehav. Rev. 37, 2243–2257. doi: 10.1016/j.neubiorev.2013.04.005
Hulst, T., van der Geest, J. N., Thürling, M., Goericke, S., Frens, M. A., Timmann, D., et al. (2015). Ageing shows a pattern of cerebellar degeneration analogous, but not equal, to that in patients suffering from cerebellar degenerative disease. Neuroimage 116, 196–206. doi: 10.1016/j.neuroimage.2015.03.084
Humes, L. E., Busey, T. A., Craig, J., and Kewley-Port, D. (2013). Are age-related changes in cognitive function driven by age-related changes in sensory processing? Atten. Percept. Psychophys. 75, 508–524. doi: 10.3758/s13414-012-0406-9
Kellermann, T., Regenbogen, C., De Vos, M., Möessnang, C., Finkelmeyer, A., and Habel, U. (2012). Effective connectivity of the human cerebellum during visual attention. J. Neurosci. 32, 11453–11460. doi: 10.1523/jneurosci.0678-12.2012
Kipping, J. A., Grodd, W., Kumar, V., Taubert, M., Villringer, A., and Margulies, D. S. (2013). Overlapping and parallel cerebello-cerebral networks contributing to sensorimotor control: an intrinsic functional connectivity study. Neuroimage 83, 837–848. doi: 10.1016/j.neuroimage.2013.07.027
Kirk-Sanchez, N. J., and McGough, E. L. (2014). Physical exercise and cognitive performance in the elderly: current perspectives. Clin. Interv. Aging 9, 51–62. doi: 10.2147/cia.s39506
Klados, M. A., Styliadis, C., Frantzidis, C. A., Paraskevopoulos, E., and Bamidis, P. D. (2016). Beta-band functional connectivity is reorganized in mild cognitive impairment after combined computerized physical and cognitive training. Front. Neurosci. 10:55. doi: 10.3389/fnins.2016.00055
Koppelmans, V., Hirsiger, S., Mérillat, S., Jäencke, L., and Seidler, R. D. (2015). Cerebellar gray and white matter volume and their relation with age and manual motor performance in healthy older adults. Hum. Brain Mapp. 36, 2352–2363. doi: 10.1002/hbm.22775
Kueider, A. M., Parisi, J. M., Gross, A. L., and Rebok, G. W. (2012). Computerized cognitive training with older adults: a systematic review. PLoS One 7:e40588. doi: 10.1371/journal.pone.0040588
Lampit, A., Hallock, H., and Valenzuela, M. (2014). Computerized cognitive training in cognitively healthy older adults: a systematic review and meta-analysis of effect modifiers. PLoS Med. 11:e1001756. doi: 10.1371/journal.pmed.1001756
Lee, R. X., Huang, J.-J., Huang, C., Tsai, M.-L., and Yen, C.-T. (2015). Plasticity of cerebellar Purkinje cells in behavioral training of body balance control. Front. Syst. Neurosci. 9:113. doi: 10.3389/fnsys.2015.00113
Mariën, P., Ackermann, H., Adamaszek, M., Barwood, C. H. S., Beaton, A., Desmond, J., et al. (2014). Consensus paper: language and the cerebellum: an ongoing enigma. Cerebellum 13, 386–410. doi: 10.1007/s12311-013-0540-5
Melby-Lervåg, M., and Hulme, C. (2013). Is working memory training effective? A meta-analytic review. Dev. Psychol. 49, 270–291. doi: 10.1037/a0028228
Moran, D. (2003). Arguments for rejecting the sequential Bonferroni in ecological studies. Oikos 100, 403–405. doi: 10.1034/j.1600-0706.2003.12010.x
Nascimento, C. M. C., Pereira, J. R., de Andrade, L. P., Garuffi, M., Talib, L. L., Forlenza, O. V., et al. (2014). Physical exercise in MCI elderly promotes reduction of pro-inflammatory cytokines and improvements on cognition and BDNF peripheral levels. Curr. Alzheimer Res. 11, 799–805. doi: 10.2174/156720501108140910122849
Niemann, C., Godde, B., and Voelcker-Rehage, C. (2014). Not only cardiovascular, but also coordinative exercise increases hippocampal volume in older adults. Front. Aging Neurosci. 6:170. doi: 10.3389/fnagi.2014.00170
Pezoulas, V. C., Zervakis, M., Michelogiannis, S., and Klados, M. A. (2017). Resting-state functional connectivity and network analysis of cerebellum with respect to crystallized IQ and gender. Front. Hum. Neurosci. 11:189. doi: 10.3389/fnhum.2017.00189
Ramakrishnan, K. B., Voges, K., De Proprisl, L., De Zeeuw, C. I., and D’Angelo, E. (2016). Tactile stimulation evokes long-lasting potentiation of purkinje cell discharge in vivo. Front. Cell. Neurosci. 10:36. doi: 10.3389/fncel.2016.00036
Raz, N., Lindenberger, U., Rodrigue, K. M., Kennedy, K. M., Head, D., Williamson, A., et al. (2005). Regional brain changes in aging healthy adults: general trends, individual differences and modifiers. Cereb. Cortex 15, 1676–1689. doi: 10.1093/cercor/bhi044
Roberts, K. L., and Allen, H. A. (2016). Perception and cognition in the ageing brain: a brief review of the short- and long-term links between perceptual and cognitive decline. Front. Aging Neurosci. 8:39. doi: 10.3389/fnagi.2016.00039
Sarter, M., and Bruno, J. P. (2004). Developmental origins of the age-related decline in cortical cholinergic function and associated cognitive abilities. Neurobiol. Aging 25, 1127–1139. doi: 10.1016/j.neurobiolaging.2003.11.011
Schafer, J. H., Glass, T. A., Bolla, K. I., Mintz, M., Jedlicka, A. E., and Schwartz, B. S. (2005). Homocysteine and cognitive function in a population-based study of older adults. J. Am. Geriatr. Soc. 53, 381–388. doi: 10.1111/j.1532-5415.2005.53153.x
Schmahmann, J. D., and Sherman, J. C. (1997). Cerebellar cognitive affective syndrome. Cereb. Cogn. 41, 433–440. doi: 10.1016/s0074-7742(08)60363-3
Sehm, B., Taubert, M., Conde, V., Weise, D., Classen, J., Dukart, J., et al. (2014). Structural brain plasticity in Parkinson’s disease induced by balance training. Neurobiol. Aging 35, 232–239. doi: 10.1016/j.neurobiolaging.2013.06.021
Seidler, R. D., Bernard, J. A., Burutolu, T. B., Fling, B. W., Gordon, M. T., Gwin, J. T., et al. (2010). Motor control and aging: links to age-related brain structural, functional, and biochemical effects. Neurosci. Biobehav. Rev. 34, 721–733. doi: 10.1016/j.neubiorev.2009.10.005
Seligman, M. E. P. (2002). Authentic happiness inventory. Available online at: http://www.authentichappiness.sas.upenn.edu/testcenter
Sexton, C. E., Walhovd, K. B., Storsve, A. B., Tamnes, C. K., Westlye, L. T., Johansen-Berg, H., et al. (2014). Accelerated changes in white matter microstructure during aging: a longitudinal diffusion tensor imaging study. J. Neurosci. 34, 15425–15436. doi: 10.1523/JNEUROSCI.0203-14.2014
Strick, P. L., Dum, R. P., and Fiez, J. A. (2009). Cerebellum and nonmotor function. Annu. Rev. Neurosci. 32, 413–434. doi: 10.1146/annurev.neuro.31.060407.125606
Styliadis, C., Ioannides, A. A., Bamidis, P. D., and Papadelis, C. (2015). Distinct cerebellar lobules process arousal, valence and their interaction in parallel following a temporal hierarchy. Neuroimage 110, 149–161. doi: 10.1016/j.neuroimage.2015.02.006
Tarmey, D. (2012). Ageing, Cognition, and Sensorimotor Processing: Difficulties in Co-Ordinating Distributed Systems? Sheffield: University of Sheffield.
Tiffin, J., and Asher, E. J. (1948). The purdue pegboard: norms and studies of reliability and validity. J. Appl. Psychol. 32, 243–247. doi: 10.1037/h0061266
Todd, C., and Skelton, D. (2004). “What are the main risk factors for falls among older people and what are the most effective interventions to prevent these falls?,” Copenhagen: WHO Regional Office for Europe (Health Evidence Network Report), Available online at: http://www.euro.who.int/document/E82552.pdf. [Accessed May 6, 2010]
Vazquez-Sanroman, D., Sanchis-Segura, C., Toledo, R., Hernandez, M. E., Manzo, J., and Miquel, M. (2013). The effects of enriched environment on BDNF expression in the mouse cerebellum depending on the length of exposure. Behav. Brain Res. 243, 118–128. doi: 10.1016/j.bbr.2012.12.047
Wayne, R. V., and Johnsrude, I. S. (2015). A review of causal mechanisms underlying the link between age-related hearing loss and cognitive decline. Ageing Res. Rev. 23, 154–166. doi: 10.1016/j.arr.2015.06.002
Westlye, L. T., Walhovd, K. B., Dale, A. M., Bjørnerud, A., Due-Tønnessen, P., Engvig, A., et al. (2010). Life-span changes of the human brain white matter: diffusion tensor imaging (DTI) and volumetry. Cereb. Cortex 20, 2055–2068. doi: 10.1093/cercor/bhp280
Keywords: declarative memory, cerebellum, hippocampus, sensorimotor, balance, vestibular stimulation, functional networks
Citation: Gallant Z and Nicolson RI (2017) “Cerebellar Challenge” for Older Adults: Evaluation of a Home-Based Internet Intervention. Front. Aging Neurosci. 9:332. doi: 10.3389/fnagi.2017.00332
Received: 02 August 2016; Accepted: 27 September 2017;
Published: 27 October 2017.
Edited by:
Panagiotis D. Bamidis, Aristotle University of Thessaloniki, GreeceReviewed by:
Richard Camicioli, University of Alberta, CanadaCopyright © 2017 Gallant and Nicolson. This is an open-access article distributed under the terms of the Creative Commons Attribution License (CC BY). The use, distribution or reproduction in other forums is permitted, provided the original author(s) or licensor are credited and that the original publication in this journal is cited, in accordance with accepted academic practice. No use, distribution or reproduction is permitted which does not comply with these terms.
*Correspondence: Roderick I. Nicolson, cm9kLm5pY29sc29uQGVkZ2VoaWxsLmFjLnVr
†Present address: Roderick I. Nicolson, Department of Psychology, Edge Hill University, Ormskirk, United Kingdom
Disclaimer: All claims expressed in this article are solely those of the authors and do not necessarily represent those of their affiliated organizations, or those of the publisher, the editors and the reviewers. Any product that may be evaluated in this article or claim that may be made by its manufacturer is not guaranteed or endorsed by the publisher.
Research integrity at Frontiers
Learn more about the work of our research integrity team to safeguard the quality of each article we publish.